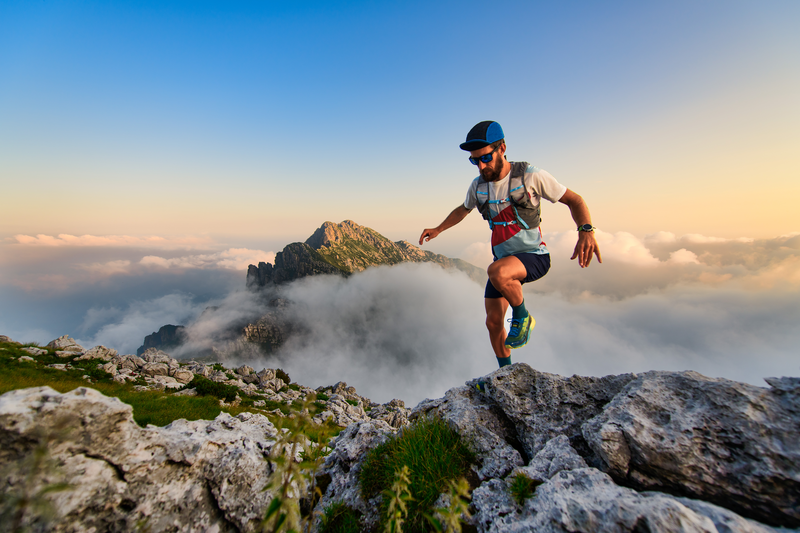
94% of researchers rate our articles as excellent or good
Learn more about the work of our research integrity team to safeguard the quality of each article we publish.
Find out more
REVIEW article
Front. Microbiol. , 12 November 2021
Sec. Antimicrobials, Resistance and Chemotherapy
Volume 12 - 2021 | https://doi.org/10.3389/fmicb.2021.747019
This article is part of the Research Topic Nanotechnology for Antimicrobials, Volume 2. View all 9 articles
Injudicious use of antibiotics has been the main driver of severe bacterial non-susceptibility to commonly available antibiotics (known as drug resistance or antimicrobial resistance), a global threat to human health and healthcare. There is an increase in the incidence and levels of resistance to antibacterial drugs not only in nosocomial settings but also in community ones. The drying pipeline of new and effective antibiotics has further worsened the situation and is leading to a potentially “post-antibiotic era.” This requires novel and effective therapies and therapeutic agents for combating drug-resistant pathogenic microbes. Nanomaterials are emerging as potent antimicrobial agents with both bactericidal and potentiating effects reported against drug-resistant microbes. Among them, the photothermally active nanomaterials (PANs) are gaining attention for their broad-spectrum antibacterial potencies driven mainly by the photothermal effect, which is characterized by the conversion of absorbed photon energy into heat energy by the PANs. The current review capitalizes on the importance of using PANs as an effective approach for overcoming bacterial resistance to drugs. Various PANs leveraging broad-spectrum therapeutic antibacterial (both bactericidal and synergistic) potentials against drug-resistant pathogens have been discussed. The review also provides deeper mechanistic insights into the mechanisms of the action of PANs against a variety of drug-resistant pathogens with a critical evaluation of efflux pumps, cell membrane permeability, biofilm, and quorum sensing inhibition. We also discuss the use of PANs as drug carriers. This review also discusses possible cytotoxicities related to the therapeutic use of PANs and effective strategies to overcome this. Recent developments, success stories, challenges, and prospects are also presented.
Recent years have witnessed a sharp increase in the incidence and magnitude of bacterial drug resistance [also known as antibiotic resistance (ABR), or antimicrobial resistance (AMR)] (Khare et al., 2021a, b; Tiwari et al., 2021). Initially considered largely a nosocomial problem, bacterial drug resistance has now reached community environments (Shriram et al., 2018; Anand et al., 2019; Friedrich, 2019; Taneja and Sharma, 2019; Khare et al., 2021a, b; Tiwari et al., 2021). The global rise of bacterial drug resistance poses serious threats to human health and the environment. Drug resistance is the ability of bacterial pathogens to survive the drugs/antibiotics to which they are exposed (Anand et al., 2020). The World Health Organization (WHO) has declared bacterial drug resistance a global menace that undermines the practice of medicines (Morrison and Zembower, 2020). The statistics on drug-resistant infections project around 10 million deaths per year by 2050, with Asia and Africa accounting for 4.7 million and 4.1 million deaths, respectively (O’Neill, 2014).
Inappropriate and/or injudicious use of conventional antibiotics is reported to be the main driving force behind the increasing resistance to drugs (Tiwari et al., 2021) and the condition is worsening further due to the drying pipeline of new and effective antibiotics (Khare et al., 2021b). There are reports of increasing levels of bacterial drug resistance, ranging from multidrug resistance (MDR) (acquisition of non-susceptibility to at least one agent in 3 or more antibiotic classes) to extensive drug resistance (XDR) (non-susceptibility to at least one agent in all but 2 or fewer antibiotic classes) and pandrug resistance (PDR) (non-susceptibility to all antibiotic classes) (Exner et al., 2017; Spengler et al., 2017; Shriram et al., 2018; Koch et al., 2021). Since the first reports of drug resistance on Enterobacteria in the 1950s, numerous bacterial strains with drug-resistant phenotypes have been reported, and this number of strains as well as their abilities to resist antibiotics are ever increasing. The increase in frequency, magnitude, spread, and persistence of drug-resistant bacterial phenotypes has contributed significantly to the challenge. The emergence of new resistance mechanisms (along with known drug exclusion/resistance mechanisms) in these bacterial strains contribute to the inefficacy of existing drugs, prolonged illness, and increased expenditures (WHO, 2020). This situation demands newer strategies for the eradication of drug-resistant bacteria, including new and effective antibacterial agents with potential clinical applications. Although there are some successful events to identify potent antibacterial agents, for example metal/metal oxide nanomaterials, their translational success is poor (Mapara et al., 2015; Khare et al., 2021a). Main challenges for limited translation to clinic include poor data availability on interactions with human cells, tissues and organs, optimal dose, recognition of appropriate routes and possible toxicities and side effects following acute and long-term exposure (Lee et al., 2019).
Over the past few decades, several reports have highlighted the effective use of nanomaterials as potent antibacterial agents, including inorganic and organic nanomaterials. Among them, metal nanoparticles, in particular silver (Ag-NPs) and gold nanoparticles (Au-NPs) are studied more extensively to assess their bactericidal activity (Mapara et al., 2015), as well as drug resistance reversal activities (Hemeg, 2017; Lee et al., 2019; Khare et al., 2021b). However, the less stable (Levard et al., 2012) and toxic nature (Sahu and Hayes, 2017) of these NPs have limited their clinical application. Overall, multifactorial resistance against such agents offered by bacterial pathogens, in addition to the toxicity issues and potential side effects along with their stability and drug-releasing issues in dynamic gut environment, are limiting the clinical applications of potent, conventional antibacterial nanomaterials. Liposomes were seen as attractive additional or alternative antibacterial drugs and/or drug carriers. However, issues related to the use of liposomes have limited their clinical uses, including physical and chemical instability issues, the thermolabile nature of liposomes, in addition to other issues such as low loading capacity (Eleraky et al., 2020).
In recent years, photothermal therapy (PTT) has attracted attention from the scientific community and is emerging as a potent (Guo et al., 2020), non-invasive approach for combating pathogens, including drug resistant ones (Yang et al., 2021). PTT involves the exploration of photothermally active nanomaterials (PANs) including gold, carbon, iron oxide (FeO), etc. that exhibit high absorption in the near-infrared (NIR) region of the electromagnetic spectrum (Bao et al., 2016; Li X. et al., 2020). The mechanism of action lies in the ability of PANs to absorb NIR light and its rapid conversion into heat, thereby increasing the surrounding temperature of the irradiated organism (Borzenkov et al., 2020). However, there are some limitations, which need attention before full-potential exploration of PTTs and PANs, in particular, as therapeutic antibacterial agents effective against drug-resistant pathogens.
In this review, we present an account of recent developments in the identification of potent PANs and the exploration of PAN-based PTTs to combat drug-resistant pathogens by targeting their main drug-resistant determinants. In addition, the use of PANs as drug carriers has also been discussed. This review also discusses the possible cytotoxicities related to the therapeutic use of PANs and effective strategies to overcome them. Contemporary developments, major success stories, challenges, and prospects are also presented.
Photothermally active nanomaterials are known to exhibit broad-spectrum antibacterial effects driven mainly by the photothermal effect characterized by the conversion of absorbed photon energy into heat energy (Borzenkov et al., 2020). PANs exhibit these photothermal properties because of the d-d band energy transitions and surface plasmons (surface electron resonance) referred to as excitation of electrons by incident light photons. They are highly dependent on the concentration of nanoparticles, wavelength, conversion efficiency, irradiation intensity, morphology, and the type of nanomaterial used (Borzenkov et al., 2020). As the properties of plasmonic nanoparticles that exhibit surface electron resonance strongly depend on the synthesis process and the surrounding environment of the nanoparticles, the properties of non-plasmonic nanoparticles depend less on the dimensions and shape (Borzenkov et al., 2019). Applications of PANs have been reported in the field of chemotherapy, cancer treatment, and photodynamic therapeutics (Hu et al., 2018; Yang et al., 2019).
There are reports on organic and inorganic PANs constituted with various metals, carbon, polymers, noble gases, metal oxides, and nanoscale metals targeting bacterial pathogens, including drug-resistant phenotypes (Feng et al., 2017). Approaches including PTT and photodynamic therapy (PDT) exploring the PANs are gaining momentum for the treatment of bacterial infections. PDT is the non-invasive therapeutic approach for bacterial infection treatments that uses low-power laser radiations, singlet oxygen, and photosensitizer for bacterial ablation (Rajesh et al., 2011). However, PDTs are finding their limited applications mainly due to their low delivery efficiency, while PTTs are gaining momentum as potent agents for containing bacterial infections (Chen et al., 2020). De Aberasturi et al. (2015) advocated various applications of these plasmonic nanomaterials in PTT and PDT. In PTT, photothermal agents (PTAs) transform light energy into thermal energy, and the generated heat triggers the rupturing of cell membranes, denaturation of proteins, and ablation of bacteria. Researchers have identified different types of PTAs with organic/inorganic compounds, metals, and carbon-based nanomaterials (Bao et al., 2016). Their easy synthesis, rapid body clearance after use, photostability, and high photothermal conversion efficiency make PTAs effective antibacterial agents with potential for therapeutic applications (Chen et al., 2020). Furthermore, an exhibition of extraordinary light-matter interactions, plasmonic properties, free electron pairs, energy bandgap, optical conductivity, optical wavelength, conductance, and morphological characteristics of nanomaterials are also considered essential factors of PTAs in the PTT (Liu S. et al., 2020). PANs are used as antibacterial agents by irradiation of light ranging from invisible NIR spectra with 650–900 nm wavelength to the ultraviolet (UV) spectrum, resulting in thermal relaxation and increased temperature (Borzenkov et al., 2020). Due to the easy operational, safe profiling, and deep tissue penetration capabilities, NIR light is preferred in PTTs.
Various PANs such as zinc-based nanomaterials (Li et al., 2019a), silver-based nanomaterials (Zhu et al., 2020), gold-based nanomaterials (Li X. et al., 2020), metal organic frameworks (MOFs) (Luo et al., 2021), copper-based nanomaterials (Han et al., 2021), and graphene-based nanomaterials (Lv et al., 2021) exhibiting photothermal activity have been investigated to combat drug resistant bacteria (Ren et al., 2020). Due to their structural compositions and chemical nature, MOFs are considered the front-runner antibacterial PANs (Zheng et al., 2021). Han et al. (2020a) developed copper-doped MOFs possessing enhanced photocatalytic activity and photothermal effects to treat Staphylococcus aureus-infected wounds under 660-nm light irradiation, which resulted in striking antibacterial efficiency (99.7%). Silver phosphate (Ag3PO4) nanoparticles, decorated with defective titanium oxide in black urchin shape (TiO2) (BU-TiO2-X/Ag3PO4) in the nanospike shape, showed high antibacterial efficiency against Escherichia coli (99.76%) and S. aureus (99.85%) under light irradiation (Xu et al., 2021). Similarly, zinc oxide carbon dots modified graphite carbon nitride nanocomposites (ZnO/CDots/g-C3N4) showed high antibacterial efficiency against S. aureus (99.97%) and E. coli (99.99%) under visible light irradiation (Xiang et al., 2020). Furthermore, in vivo clinical studies in mice wounds infected with S. aureus revealed the wound healing abilities of nanocomposites.
Bacterial pathogens, especially MDR or XDR strains, have a highly active self-defense armory that helps them survive against antimicrobial drugs, antibiotics, and pesticides (Khare et al., 2021a). These mechanisms often work in tandem, ensuring bacterial protection against wide-spectrum drugs, and thus driving them into “superbugs.” The main determinants of drug resistance include cell permeability and drug efflux pumps, the ability of these strains to modify or destroy applied antibiotics, and the modification of antibiotic targets (Figure 1). In particular, PANs have been characterized as striking antibacterial agents with the potential to combat drug-resistant strains, as they have been reported to target the major drug-resistant determinants of these bacterial pathogens (Table 1).
Figure 1. Major bacterial drug resistance mechanisms. Adapted from Khare et al. (2021a). Copyright (2021) Frontiers Media.
Table 1. Photothermally active nanomaterials against bacteria with targeted drug-resistant determinants.
Several recent reports are coming on organic and inorganic nanomaterials for their photothermal ablation/destruction of drug-resistant bacteria (Zhao et al., 2021). Various metal oxides, non-metal oxides, and semiconductor-based nanomaterials have been investigated for their potential photothermal and photocatalytic activity against drug-resistant bacteria that possess different inhibition mechanisms such as radical formation, efflux, and biofilm inhibition for the killing of bacteria and are discussed below.
Photothermally active nanomaterials are known to target and destroy/inactivate bacterial cell membranes, the first and most powerful line of bacterial defense, by decreasing cell permeability and the generation of reactive oxygen species (ROS) (Agnihotri et al., 2020). Singlet oxygen (1O2), electron (e–), hydroxyl radicals (•OH), and superoxide radicals (O2•–) have been reported to be directly involved in the loosening of permeability of the microbial membrane and the ultimate death of the target microorganism (Mapara et al., 2015; Feng et al., 2017). These radicals formed can cause damage to the cell membrane through lipid peroxidation, leading to the destruction of organelles and the ultimate death of microbes. Under oxygen deprivation conditions such as anaerobic environments, bacterial cells behave as electron acceptors, where direct entry of electrons leads to cell death. Several nanomaterials such as graphene oxide (GO) (Linklater et al., 2018), copper oxide (CuO) (Kung et al., 2017), zinc oxide (ZnO) (Patra et al., 2015), and titanium dioxide (TiO2) (Ranjan and Ramalingam, 2016) have been used for the inactivation of bacteria as cell permeabilizers. GO nanomaterials with sharp edges as unique property are known for the ablation of drug-resistant bacteria by causing piercing in membranes along with oxidative stress induction (Menazea and Ahmed, 2020). The interaction between GO and the bacterial membrane increases the microbial acquisition and can be used as PTAs attributable to greater heat transfer ability, where the photothermal effect of GO can be activated using the NIR and visible spectrum (Vangara et al., 2018). Europium-vancomycin-functionalized reduced-GO (Eu-Van-rGO), responsive to NIR light, were reported for their bactericidal properties against MDR strains through a heat generation mechanism (Yang et al., 2014). Furthermore, Li X. et al. (2020) reported highly effective photoactive gold nanoclusters decorated with amine-functionalized GO (Au-GO-NH2) nanosheets that photothermally inactivated bacteria by capturing them, generating heat, and producing ROS.
In addition to the bactericidal activities, there are also reports on the synergetic effects of nanomaterials and antibiotics against drug-resistant pathogens. The synergetic effect of reduced GO and gold nanostar, known as rGO/AuNS nanocomposites under 808 nm NIR irradiation, resulted in increased photothermal and antibacterial activity through membrane disruption and hyperthermal effect, leading to complete death of methicillin-resistant S. aureus (MRSA) (Feng et al., 2019). Similarly, the combination of Ag-NPs with GO the rGO/Ag nanocomposites under NIR irradiation showed enhanced photothermal activity leading to effective bactericidal potencies by disrupting the microbial membrane and generating ROS in Klebsiella pneumoniae and E. coli (Tan et al., 2020). Recent reports on oxygen-deficient Ni/reduced graphene oxide (Ni/rGO) nanocomposites demonstrated enhanced photothermal and photocatalytic activity under NIR irradiation by increasing GSH loss and destroying the microbial cell membrane through the formation of O2•– and •OH radicals (Zhang Z. et al., 2021). The synergistic effects of GO with azithromycin antibiotics such as modified polyethyleneimine-citraconic anhydride (PEI-CA) and azithromycin-loaded GO nanosheets (AZI@GO-PEI-CA) as antimicrobial agents have been studied where PEI-CA improved membrane anchoring and AZI-GO resulted in inhibition of membrane piercing, protein and ribosome synthesis in S. aureus and E. coli strains, presenting them an intriguing tool for microbial infection abatement (Huo et al., 2021). The use of human serum albumin (HSA)/rGO/Cladophora glomerata (Cladophoraceae) bionanocomposites as a PTA was also identified with antimicrobial activity under sunlight/NIR irradiation mediated by ROS generation (Amina et al., 2021). Similar results were observed using graphitic carbon nitride-based nanomaterials (g-C3N4) with photocatalytic antibacterial activity (Kong et al., 2021). Because of environmental friendliness and inexpensive production costs, ZnO-based nanomaterials have been shown to be exceptionally appealing for microbial photoinactivation via NIR and UV radiation. ZnO nanoparticles are considered to be primarily involved in bacterial inactivation by generating ROS and inducing apoptosis (Mishra et al., 2017).
In addition to ROS generation, other mechanisms such as controlled release of Zn2+ ions from ZnO nanoparticles also play an important role in bacterial killing. Liu Z. et al. (2020) investigated the photothermal effects of nanocomposites of zeolite imidazole framework-8 encapsulated with humic acid (HA) (ZIF-8) (HuA@ZIF-8) under NIR light for controlled release of Zn2+ ions, resulting in a synergistic effect and the ablation of S. aureus and E. coli. In addition to ZnO and GO-based nanoparticles, the development of semiconductors with a low level of toxicity and highly active nature has emerged as a novel strategy for photothermal antimicrobial activity against Pseudomonas aeruginosa, E. coli and S. aureus (Yougbaré et al., 2020). Semiconductors such as molybdenum disulfide (MoS2) have attracted attention for their high band gap and photothermal properties (Gupta et al., 2020). MoS2 nanomaterials could result in ROS generation and ablation of bacteria with antioxidant enzyme-like activity (Yu et al., 2021). Studies on chitosan modified MoS2 coating loaded with Ag-NPs designed on titanium plates (CS/Ag/MoS2-Ti) exhibited both photodynamic and photothermal efficiency under 660 nm visible light, resulting in ROS production and bacteria killing (Zhu et al., 2020). Furthermore, in vivo investigations showed the antibacterial efficiency of CS/Ag/MoS2-Ti nanomaterials against S. aureus and E. coli with 98.66% and 99.77% antibacterial activity (Zhu et al., 2020).
Besides, the broad-spectrum antimicrobial properties of nanozymes are gaining attention and are seen as a novel class of therapeutics. Wang et al. (2021) reported the use of Cu single atom sites/N doped porous carbon (Cu SASs/NPC) nanozyme with enhanced photothermal and peroxidase-like activity in the presence of H2O2 resulting in the generation of •OH radicals to remove the bacterial cell membrane under NIR irradiation. Similarly, the coupling of copper sulfide and lignin as lignin-copper sulfide nanocomposites against E. coli and S. aureus under NIR light inactivation resulted in synergistic photothermal effects, peroxidase-like activity, and permeabilization of the cell membrane in the presence of H2O2 (Xie et al., 2021). However, the catalytic effects of nanozymes are reduced in wound infection microenvironments because of the overexpression of GSH levels (as a result of anaerobic glycolysis). However, there have been some recent efforts to overcome these problems and improve the chances of clinical applications of nanozymes. For example, Li Y. et al. (2021) developed an ionic covalent organic framework-based nanozyme that has self-promoting antibacterial potencies with good biocompatibility as a glucose-triggered cascade atalyst against bacterial wound infection. Along with the effective conversion of glucose to H2O2, gluconic acid produced by providing a compatible catalytic environment improved peroxidase activity to generate more hydroxyl radicals, toxic to bacterial pathogens. Zhang Y. et al. (2021) reported in vivo antibacterial activity of oxygen-vacancy molybdenum trioxide nanodots (MoO3–XNDs) that possess photodynamic, photothermal, and nanozyme activity against ESBL E. coli and MRSA during NIR irradiation that increased while treating wound healing.
Furthermore, due to their high biocompatibility and low toxicity levels, quaternary ammonium compounds (QACs) are also being used to keep bacteria heat sensitive and thus cause damage to cell membranes (Jiao et al., 2017). Studies on the synergistic effect of QACs with carbon-based dots such as Cu-RCDs-C35 under 808 nm NIR irradiation resulted in effective killing of E. coli and S. aureus bacteria through ROS generation (Chu et al., 2021). The in vivo anti-infective properties of Cu-RCD-C35 were evaluated using the S. aureus infected mice model by quantitatively evaluating the efficacy of Cu-RCD-C35 bacteria around wounds using lasers that result in bactericidal activities. Figure 2 shows how PANs induce bactericidal effects on pathogens through ROS generation and consequent cell membrane damage.
Figure 2. Antibacterial potency of photothermally active nanomaterials via inducing the generation of reactive oxygen species for targeting cell membranes and their permeability.
The exploration of PANs as bacterial drug efflux pump inhibitors (EPIs) has attracted attention as an effective way to combat drug-resistant bacterial pathogens (Chopra and Dhingra, 2020). EPs facilitate and regulate the efflux of toxic substances, metabolites, and antibiotics from bacterial cells into the outer environment (Shriram et al., 2018; Yu et al., 2020). Recent publications have confirmed the overexpression of multidrug EPs as one of the major resistance mechanisms in bacteria to throw out most antibiotics, including the last line of defense such as colistin (Kim et al., 2020). Reports on the biocompatibility of PANs along with strong EP inhibitory potentials have provided a worthwhile platform for research aimed at addressing the challenges of bacterial drug resistance (Yang et al., 2021). Similarly, EPs have been found to play an important role in biofilm formation that leads to bacterial drug resistance (Alav et al., 2018). Studies have reported that various EPs such as NorA in MRSA (El-Baky et al., 2019), MexAN-OprM in P. aeruginosa (Alav et al., 2018), AdeFGH in Acinetobacter baumannii (He et al., 2015) are directly involved in biofilm formation. Nano-strategies are also being explored for their antibiofilm potentials by inhibiting these EPs (Dey et al., 2022).
Because of the ferromagnetic properties at ambient temperature, ZnO is considered the choice of nanomaterials by researchers. In addition, the photothermal effectiveness of these oxides can be increased by doping them with metals, resulting in increased magnetic and optical properties (Naveed Ul Haq et al., 2017). Thiolated chitosan-coated cobalt zinc oxide (Co-ZnO) nanomaterials were observed with higher photothermal activity upon sunlight activation, resulting in inhibition of the efflux pump and ROS generation in MRSA (Iqbal et al., 2019). Similarly, studies on methylene blue (MB) photosensitizer and Concanavalin A (ConA) attached dextran capped gold (GNPDEX) nanoparticles (MB@GNPDEX-ConA) showed EP inhibition and other multi-target modality by singlet oxygen generation and cytotoxicity, resulting in enhanced killing of MDR Klebsiella (Khan et al., 2020).
In addition to ZnO and Au NPs, nanoceria, also known as cerium oxide, have the unusual property of flipping their oxidation states due to a high energy band gap with a wide excitation energy and show high catalytic activity (Thakur et al., 2019). Therefore, doping cerium oxide nanomaterials with metal/transition metals led to the generation of surface defects with high-electron-trapping systems that ultimately enhanced the photothermal/photocatalytic activity and further lead to cytotoxicity and ROS production. Furthermore, investigations on thiolated iron-doped nanoceria in visible light photoactivation resulted in ROS production (singlet oxygen and inhibition of hydroxyl radicals) and efflux pump inhibition in pathogens originated from hospital effluents, which revealed its photocatalytic activity against bacterial pathogens with MDR (Khan et al., 2019).
In therapeutic practice, PTTs have been commonly used to treat biofilm-related infections and develop new technologies to combat drug-resistant bacteria (Tan et al., 2018). Biofilms are primarily defined as complex frameworks, frequently linked with the onset of different infectious diseases that have raised various issues of AMR and HGT. They have emerged as one of the main resistant mechanisms by creating different communities that can adhere to the surface using extra polymeric material such as exopolysaccharide and glycocalyx for their survival in the environment (Sadekuzzaman et al., 2015). The biofilm formation of microorganisms ultimately protects them from harsh environmental and physiological conditions/factors such as dehydration, biocides, etc. (Dunne, 2002). Besides, biofilm formation is influenced by different environmental stress factors. Further, quorum sensing (QS), overexpression of multidrug EPs, and persistence cell formation are the characteristics of the biofilm microenvironment and nanomaterials are finding their use in targeting them for curing bacterial infections (Rao et al., 2021).
Gold nanocomposites are intensively investigated PTAs for photothermal lysis against several bacterial species. Gold (core) and copper (I, II) sulfide (shell) nanocomposites (Au@Cu2–XS) under NIR irradiation have been investigated with the effect of the decomposition of exopolysaccharides and proteins, primary components of the biofilm that lead to the effective killing of Enterococcus faecalis (95%) and Fusobacterium nucleus (98%) in biofilm (Cao et al., 2021). Evaluation of further in vivo root canal infection models from Beagle dog premolars demonstrated excellent antibacterial activities of Au@Cu2–XS nanomaterial as novel agents with potential clinical applications in root canal therapy (Cao et al., 2021). A photo-illuminated nanostructured TiO2 based thin film (NsARC) was used to grow E. coli, S. aureus, Saccharomyces cerevisiae, and P. aeruginosa showed antimicrobial activity and biofilm disruption resulting in efficient photocatalytic activity (Wasa et al., 2021). Similar results were observed in cerium oxide nanoparticles doped with tin (Sn-doped CeO2) synthesized from Allophylus cobbe exhibiting concentration-dependent antibiofilm activity under visible light in Listeria monocytogenes and S. aureus at 512 mg/mL with a 2 log CFU reduction (Naidi et al., 2021). In addition to Au nanocomposites, plasmonic silver nanocomposite films under NIR irradiation have been studied to photothermally eradicate biofilms in clinically relevant E. coli and S. aureus (Merkl et al., 2021). Dai et al. (2021) studied nanocomposites based on MSN (FITC Cu2–XSNPs MSNs) as combined NIR-activated photodynamic/PTT for the ablation of biofilms against P. aeruginosa and S. aureus (90% inhibition rate at a concentration of 160 μg/mL concentration). Furthermore, Zhu et al. (2021) studied biofilm breakdown using a cationic chitosan-coated ruthenium dioxide nanozyme (QCS-RuO2@RBT, SRT NS) that exhibited NIR radiation enhanced peroxidase-like activity, resulting in cleavage of extracellular DNA through •OH formation and almost 100% disruption in the biofilm of MDR P. aeruginosa. The authors further confirmed the excellent in vivo antibacterial activities against chronic P. aeruginosa lung infections in the mouse model (Zhu et al., 2021). In another interesting study, Hu et al. (2017) developed surface-adaptive gold nanoparticles (Au-NPs) that exhibited a self-adaptive target to the acidic microenvironment of the MRSA biofilm, which was ablated by nanoparticles under NIR light irradiations; in particular, no damage was observed to the healthy tissues around the biofilm. Further in vivo studies on bactericidal effects in rabbit models infected with MRSA resulted in photothermal killing of bacteria (Hu et al., 2017). MRSA biofilms are the main bacterial infections that are involved in bone implant failure (Khatoon et al., 2018; Li Y. et al., 2020). Recent studies on the elimination of MRSA biofilm infection in bone implant using polyvinyl alcohol modified with chitosan, polydopamine and NO release donor in the red phosphorous nanofilm deposited in titanium implant (Ti-RP/PCP/RSNO) under NIR light irradiation resulted in the formation of peroxynitrite (•ONOO–) that affected the gene regulation of biofilm formation factors, i.e., staphylococcal accessory regulator (SarA), intercellular adhesion gene A (icaA), and intercellular adhesion gene D (icaD); and virulence factors, i.e., staphylococcal enterotoxin A (SEA) and α-hemolysis resulting in inhibition of biofilm formation and damaging MRSA (Li Y. et al., 2020). In vivo studies further confirmed excellent osteogenesis and biofilm eradication by NO released from the RP/PCP/RSNO system under NIR irradiation, indicating non-invasive tissue reconstruction of MRSA-infected tissues through phototherapy and immunotherapy (Li Y. et al., 2020). Similar in vivo studies for the elimination of MRSA biofilms and excess inflammation from severely infected wound models of abscess using deoxyribonuclease (DNase)–carbon monoxide (CO)@mesoporous polydopamine nanoparticles (MPDA NPs) under NIR irradiation and resulted in biofilm extracellular DNA degradation by DNase I and their destruction by released CO gas molecules leading to synergistic PTT (Yuan et al., 2021). The anti-inflammatory properties of the released CO resulted in alleviation of the inflammatory response, therefore accelerating the wound healing process and showing their potential therapeutic applications in the MRSA biofilm caused clinical problems (Yuan et al., 2021). Figure 3 illustrates the eradication of pathogens from the ESKAPE group that form biofilms using polydopamine nanoparticles (PDA-NPs) under NIR irradiation. In conclusion, though there is considerable progress in laboratory-based in vitro and in vivo studies for establishing the antibacterial potencies in general and antibiofilm activities, in particular of PAN-based antibiofilm agents, there is not much translational success, and there is much scope for improvement in their journey from the laboratory to the bedside.
Figure 3. Eradication of ESKAPE panel pathogens biofilms using photothermal polydopamine nanoparticles (PDA-NPs). (1) characterization of PDA-NPs, (2) photothermal properties of PDA-NPs at NIR radiation (880 nm); (3) CLSM image for photothermal killing of PDA-NPs against S. aureus biofilms (48 h old) in suspension; (4) killing of ESKAPE-panel pathogens using PDA-NPs (48 h); (5) photothermal killing after NIR-irradiation of biofilms exposed to PDA-NPs; (6) CLSM image of S. aureus biofilms after photothermal treatment (48 h). Adapted from Gao et al. (2021). Copyright (2021) Elsevier.
Spurred by the successful photothermal bactericidal applications of Au, Ti, and Ce-based nanocomposites, nanoswimmers are being explored for in vivo deep-layer biofilm elimination considering their excellent self-propelling and penetrating abilities (Cui et al., 2020). The NIR light-driven nanoswimmer was found to be efficient with self-propulsion, penetrating, and thermal-triggered release of the vancomycin (Van) antibiotic as chemo/PTT, resulting in in vivo biofilm elimination and the treatment of biofilm-associated infections (Cui et al., 2020). Figure 4 shows the mechanisms for the photothermal and photocatalytic eradication of MRSA biofilms.
Figure 4. Mechanism for photothermal and Photocatalytic Eradication of MRSA Biofilms and Osseointegration Using Oxide Perovskite-Based P–N Heterojunction under Enhanced Near–Infrared (p-type CTO, perovskite-type calcium titanate; p-type RP, perovskite-type red phosphorous; ALP, alkaline phosphatase; OCN, Osteocalcin; OPN, Osteopontin; OSN, Osterix; RunX-2, runt-related transcription factor 2; COL I, Collagen type I; MSCs, mesenchymal stem cells; CB, conduction band; VB, valence band). Adapted from Mao et al. (2021). Copyright (2021) Wiley-VCH.
Quorum sensing is characterized as microbial communication systems driven by various compounds or auto-inducers mediated through bacterial metabolic pathways. Various molecular mechanisms of QS systems such as acyl-homoserine lactones, peptide auto-inducers, and auto-inducer 2 are adopted by microbes for intercellular signaling (Irie and Parsek, 2008). As a result, there is a growing desire for commercially feasible, innocuous/anti-QS agents that have parallel action modes that address both antibiofilm formation and anti-QS. QS inhibitors (QSI), including nanomaterials and photothermal therapeutics, have been reported with potential to disrupt the QS system and inhibit biofilm formation (Geske et al., 2005). In recent years, PANs have been reported as effective agents for eliminating microbial QS systems. Although this area is far from its full potential exploration and requires more and comprehensive investigation, however, the recent trends are encouraging.
Sun et al. (2020) developed a synergistic antibiofilm system combining QSI, antibiotic, and PDT in multidrug-delivering hollow carbon nitride spheres (HCNSs), which successfully inhibited the QS system by influencing the production of virulence factors and surface hydrophobicity leading to a reduction in biofilm formation to 10.3%, even lower than the projected additive value (37.4%, ∗∗p = 0.002). In addition, in vivo studies were carried out to evaluate the cytotoxicity and efficacy of the nanosystem in MRSA biofilm implanted periprosthetic infection (PPI) mice model, which resulted in the killing of bacteria and regeneration of the epidermis and wound healing (Sun et al., 2020). Similarly, investigations in NIR light-enhanced protease-conjugated gold nanorods (200 μg/mL) revealed their anti-QS ability by eliminating exotoxins (86%) and biofilm in E. coli (70.5%) and S. aureus (93.3%) (Li et al., 2019c). Figure 5 illustrates the antibiofilm and anti-QS potencies of PANs.
Figure 5. Antibiofilm and quorum-sensing inhibitory potencies of photothermally active nanomaterials.
Inadequate drug concentration at the target infection site, drug degradation before reaching the target, increase in frequency of drug administration, low bioavailability, limited penetration at the infection site, increased side effects associated with higher drug dosage are some of the common problems known to compromise the efficacy of antibiotics and ultimately can lead to the increase in drug resistance of pathogens (Kalhapure et al., 2015; Canaparo et al., 2019; Li Z. et al., 2021). To overcome some of these problems, effective and safer drug delivery systems comprising PANs that encapsulate or conjugate antibacterial drug(s) have been explored in recent years (Table 2). Au-NPs, Ag-NPs, GO nanomaterials, FeO nanomaterials, and black phosphorus are some of the nanomaterials with photothermal conversion efficiencies under NIR irradiation that have been significantly utilized for rapid antibacterial drug delivery at the target infection site. The synergistic combination of antibiotics and PANs as drug carriers enhances antibacterial activity and also reduces toxic side effects; for example, effective eradication of drug resistant bacteria through combined photothermal chemotherapy has been studied using a biocompatible thermo-responsive inspired drug delivery nanotransporter (TRIDENT) (Qing et al., 2019). The TRIDENT nanosphere encapsulating imipenem and the photosensitizer molecule IR780 (IMP/IR780@TRN) releases imipenem at the target site via the phase transition generated by the temperature induced by NIR and damages the bacterial cell membrane, facilitating imipenem permeation and eventual bacterial death with the log10 (CFU mL–1) value reduced to 4.23 ± 0.01 for E. coli and 4.14 ± 0.02 for S. aureus (Qing et al., 2019). With a further increase in body weight, a reduction in the size of the skin lesion (by approximately 70% for MDREC and 60% for MRSA) was observed in a mouse model of skin infected with MDR E. coli and MRSA. Another study developed an NIR light-driven nano swimmer (HSMV) that effectively combines PTT and chemotherapy in one system that performs efficient self-propulsion and penetrates deep into the bacterial biofilm, increasing the photothermal removal of S. aureus (>90%) and the killing of bacterial cells plus a 5-fold log reduction (∼99.99%) in biofilm colonies to eradicate bacterial biofilms (Cui et al., 2020). Furthermore, in vivo studies showed antibiofilm efficacy in mouse implant-related PPI model with complete wound healing and negligible damage to major organs such as heart, spleen, liver, kidney, and lungs, indicating good therapeutic biosafety of HSMV (Cui et al., 2020).
In recent years, reduced accumulation of antibiotics in healthy tissues and prevention of exposure of the commensal microflora to a sublethal dose of antibiotics are the main challenges faced in the development of effective drug delivery systems. In an interesting attempt, vancomycin and thiol-poly(ethylene glycol) (mPEG-SH) molecules modified polydopamine nanoparticles (PDA-PEG-Van) exhibiting photothermal activity with long-term stability and specific MRSA target ability resulted in a multivalent hydrogen bond interaction between vancomycin and the MRSA cell wall to treat MRSA bacterial infections and further prevention of damage to healthy tissue (Hu et al., 2019). Similarly, Zhao et al. (2019) developed a nanotherapeutic decorated with a bioinspired heteromultivalent ligand that specifically recognized P. aeruginosa by a glycomimetic shell that protects native cells from infection in addition to bacterial biofilm prevention (rate of up to 85%) and biofilm dispersal by more than 80% with severely damaged ruptured cell surface. Finally, the in vivo study evaluated the efficacy of nanotherapeutic against acute pneumonia reporting bacterial clearance from the lungs (p < 0.05), downregulated amount of inflammatory cells (neutrophils, lymphocytes, and macrophages) and decreased cytokine levels (p < 0.05), effectively reducing the immune response and eliminating acute bacterial pneumonia (Zhao et al., 2019). Reports on an injectable system of hollow microspheres that is effective in treating subcutaneous abscesses under NIR irradiation demonstrated an increase in the cytotoxicity of bacteria in the abscesses and spatial stabilization of encapsulated vancomycin at the injection site (Chiang et al., 2015).
Other antimicrobial drugs, such as antimicrobial peptides (AMPs), enzymes, and plant products, have also been identified to be administered via PANs, expanding the number of therapy options. The development of amino-modified mesoporous silica-coated gold nanorods encapsulating cinnamaldehyde (CA@AuMN-HA) under NIR irradiation showed strong antibacterial activity with controlled CA released property and up to 90% inhibition when MRSA was incubated with CA@AuMN-HA (80 μg/mL) and exposed to an 808 nm laser (Sun et al., 2018). A further study on cytotoxicity and histological analysis demonstrated a slight influence on cell viability at a high concentration of 320 μg/mL with no histological abnormalities or organ damage, concluding excellent biocompatibility and non-toxicity of the synergistic system for clinical application. Furthermore, Moorcroft et al. (2020) designed a nanoparticle-loaded hydrogel consisting of AMP IK8-loaded liposome incorporated into polyethylene glycol (PEG) along with gold nanorods under laser irradiation that led to photothermally triggered release of IK8 and enhanced AMP efficacy in P. aeruginosa and S. aureus. Despite the potential of AMPs as an antibiotic alternative, clinical translation is still greatly hindered by proteolytic instability in vivo. Therefore, the encapsulation of IK8 within the lipid bilayer restricts the access of protease by providing a protective barrier and, therefore, can facilitate the treatment of an infectious environment rich in proteolytic enzymes, such as open wounds. Henceforth, considering the advantages of PANs, including high drug bioavailability, no or lesser toxicity, and efficient delivery of antibiotics in healthy host tissue, the PANs present tremendous therapeutic potential as antimicrobial drug carriers.
One of the important factors in developing nanoparticle-based drug delivery is the high drug loading since most of the nanocarriers have low drug loading which led to the use of a large amount of nanomaterial causing adverse side effects and increased in the cost of nanotherapeutics (Della Rocca et al., 2012). Therefore, to alleviate these limitations, PANs with high loading capacity are being successfully developed, for example, a hydrogel/liposome system containing IK8 loaded liposome and lipid-coated nanorods that allowed the encapsulation of IK8 (770 ± 21 μg mL–1) against S. aureus (32 μg/mL) (Moorcroft et al., 2020). Another study reported that polydopamine (PDA) coated gold nanorods (GNRs) grafted with glycol chitosan (GCS), i.e., GCS-PDA@GNRs showed a high loading capacity of daptomycin (DAP) in GCS-PDA@GNRs (552.32 μg of DAP at a 0.2 mg/mL of DAP concentration) (He et al., 2018). In addition to high drug loading capacity, PANs can also load two drugs simultaneously, thus enhancing the efficacy of treatment, for example, Sun Y. et al. (2019) developed HCNS/A&L@HA nanomaterial where luteolin (L) as a QSI and ampicillin (A) antibiotic were encapsulated in HCNSs capped by hyaluronic acid (HA) showed sequenced release of luteolin (first release) and ampicillin (second released) with biofilm inhibition efficiency of 64.2%. Similarly, PANs were also reported to be capable of loading plant essential oils, known for their striking antimicrobial properties (Sun et al., 2018). These case studies highlight the high loading capacity and drug loading diversity as advantages of PANs over other nanomaterials.
However, despite these advantages, some limitations still exist with the PAN application that need to be further addressed. These include drug loading efficiency; accurate antibacterial mechanism identification; difficulty in killing heat resistant bacteria when treated at high temperatures such as 45°C, and poor tissue penetration by a light source limiting the clinical application of PANs. Therefore, the development of photosensitizers based on the second NIR window (NIR-II, 1000–1700 nm) is seen to be the focus of future research in this area (Wei et al., 2020).
Foreign matter, especially at the nanoscale, needs to be thoroughly and carefully examined for potential cytotoxicities prior to its clinical applications. Furthermore, they must be investigated to minimize their impacts on normal (or non-target) cells. One of the greatest concerns associated with the therapeutic/clinical use of PANs is that they can easily pass through cells and tissues and could enter the systemic circulation. Chen et al. (2019) highlighted some of the issues related to the surface effect of nanomaterials that can easily be changed by ROS to become toxic and damaging to bacterial cells. However, for better antibacterial applications of PTT, an extensive understanding of PTA and potential toxicities/side effects is required (Chen et al., 2020). In one such attempt, studies on the cytotoxicity of photothermally active copper sulfide (CuS) nanoplates demonstrated that cell viability decreased in human umbilical vein endothelial cells (HUVECs) and mouse leukemic monocyte macrophage cells (RAW 264.7) when treated with higher concentrations (>100 μg/mL) (Feng et al., 2015). During the photothermal effect of nanomaterials, heat dissipation in the energy loss pathway occurs after the light is absorbed by the nanoparticles; therefore, the maintenance of uniform heating of nanoparticles is highly recommended for the uniformity of the reaction. To obtain controlled photothermal responses, it is crucial to acquire nanoparticles with low heterogenicity in size and shape, narrow absorbance line width, and high extinction. Nanoparticles with established pharmacokinetic aspects (absorption, distribution, metabolism, and excretion) are needed to minimize dosage to avoid unwanted side effects or toxicity (Kim et al., 2019). Some approaches to minimize the side effects of PANs are discussed below.
The use of high temperature in PTT can be painful to the patient and can cause injuries to nearby tissues. Hence, to minimize this side effect and maximize antibacterial efficiency, lowering the temperature can significantly help. Therefore, the combination of PTT with PDT (PTDT) is an emerging synergistic therapeutic approach (Sun J. et al., 2019). PDT is dependent on the photosensitizer (PS) that produces ROS and causes the death of bacteria by destroying cellular DNA, lipids, and proteins. An increase in temperature results in an enhancement of the bacterial cell membrane, facilitating the uptake of PS and PDT agents (Sahu et al., 2016). The development of a single NIR laser-excited PTDT system resulted in the generation of low temperature, resulting in the killing of bacteria and ROS production with a 95% antibacterial rate in S. aureus and E. coli (Sahu et al., 2016). As PDT is limited by oxygen concentration, to combat the biofilm microenvironment, non-catalytic antibacterial therapy is used as an alternative to PDT with synergistic therapy (de Melo et al., 2021). A combination of a PDA@Au-hydroxyapatite hybrid system with a low concentration of H2O2 showed enhanced antibacterial activity for E. coli (96%) and S. aureus (95.2%) resulting in a significant increase in wound healing (Xu et al., 2018). Li et al. (2019d) developed a biocompatible phototherapeutic system composed of MoS2, IR780 photosensitizer, and arginine–glycine–aspartic acid–cysteine (RGDC), where magnetron-sputtered MoS2 showed photothermal property plus IR780 that produced ROS with NIR irradiation (λ = 700–1100 nm) photodynamic property together with the assistance of glutathione oxidation that provided synergistic and rapid killing of bacteria 98.99 ± 0.42% eradication ratio against a biofilm of S. aureus. Synergistic killing of bacteria by combining the photodynamic activity of GO and the photocatalytic activity of Ag-NPs (GO/Ag/collagen coating) under laser irradiation resulted in high bacterial efficiency against E. coli (96.3%) and S. aureus (99.4%) reported by Xie et al. (2017). Li J. et al. (2021) Designed nanoparticles embedded at the semiconductor metal interface called the interfacial Schottky junction of bismuth sulfide (Bi2S3) and titanium carbide (Ti3C2Tx) nanosheets that exhibited photocatalytic activity resulting in the eradication of S. aureus (99.86%) and E. coli (99.92%) under NIR irradiation.
Direct bacterial targets are one of the effective approaches to combat bacterial infections that help reduce side effects and enhance therapeutic efficiency (Huang et al., 2019). Alhmoud et al. (2017) used S. aureus targeting antibodies to increase efficiency and reduce side effects, resulting in reduced cell viability (99.99%) and increased antibacterial efficiency (up to 10 times). However, because of drawbacks such as instability, rapid decay, complex reaction process, and high price, the use of other molecules, such as small-size DNA/RNA aptamers, took over because of their negligible immunogenicity, high loading capacity, easy synthesis, and mainly great stability. Ocsoy et al. (2017) synthesized gold nanorods decorated with DNA aptamer showed great photothermal anti-MRSA activity with 95% bacterial inactivation. However, these strategies are effective only when the pathogen is known; therefore, to target unknown bacteria, the use of cationic molecules emerged as another potential targeting strategy. Fan et al. (2019) used cationic Magainin I (Mag I) as magainin-modified polydopamine nanoparticles to improve PDA affinity. The cell-derived membrane has many advantages in its use in vitro as a coating material for targeted therapy. Wang C. et al. (2018) used the extrusion method to coat Au-Ag nanocages with S. aureus pretreated macrophage membranes. The American national standards stated that the laser range for in vitro use, maximal penetration depth, and maximum permissible exposure for skin is 1000–1100 nm and 1 W/cm2. Compared with the continuous mode of laser, the effective strategy to be found was the pulsed laser with a short duration of time with less heat diffusion and specific killing of bacteria. Kirui et al. (2019) and Yuan et al. (2015) used a nanosecond pulsed laser and a femtosecond pulsed laser, respectively, and showed that antibacterial PTT did not cause obvious cytotoxicity in vivo. The new trend of using non-chemical antibacterial means for the disinfection of environments is grabbing the attention. Wu et al. (2021) developed safe and biodegradable photosensitive red phosphorus nanoparticles (RNPs) that exhibit strong photothermal and photocatalytic ability that resulted in the death of S. aureus (99.98%). Investigations on silver sulfide (Ag2S) nanoparticles decorated nanocubes (Ag2S/NCs) exhibiting excellent photothermal properties and biocompatibility resulted in increased antibacterial efficiency against S. aureus (97.3%) under light irradiation (Xiong et al., 2019). Li et al. (2019b) studied the synergistic effect of lysozyme-assisted anti-infection and photothermal eradication of in vivo MRSA using naturally derived biodegradable human hair melanosome nanostructures resulting in highly efficient antibacterial activity (97.19%) and tissue reconstruction, thus accelerating tissue repair. Mao et al. (2018) reported repeatable killing of bacteria (E. coli 98.90% and S. aureus 99.51%) through fabricated hybrid hydrogen gel embedded with 2D few-layer black phosphorus nanosheets (BP) via electrostatic interaction.
The microenvironment surrounding the bacteria is often pH dependent, due to the release of various enzymes and products into the surroundings. Zhang et al. (2019) synthesized Rhenium trioxide (ReO3) nanocubes were effective in the acidic infectious environment, however, rapidly degraded in healthy tissues. In addition, toxins and enzymes also showed activity to trigger and release PTAs. Similarly, Yang et al. (2017) developed a supramolecular complex that reduces radicle anions on the bacterial surface such as E. coli. Specific PTT was achieved by the photothermal effects of radicle anion under NIR irradiation. Xie et al. (2018) designed a hybrid polydopamine (PDA)/Ag3PO4/GO coating and observed that controlled release and photocatalytic Ag3PO4 produce synergistic antimicrobial efficiency up to 99.53 and 99.66% against E. coli and S. aureus, respectively. Other approaches such as bacterial capture and killing are also used as alternative strategies. Studies have reported that prussian blue nanoparticles synthesized as photosensitive hydrogels exhibiting strong photothermal properties under NIR irradiation resulted in the capture of bacteria in hydrogels and its further killing by perturbation of bacterial cell membranes in S. aureus (99.97%) and E. coli (99.93%) (Han et al., 2020b). Similar results were observed in MRSA-infected osteomyelitis to treat tissue infections using magnetically iron oxide-targeted carbon nanotubes (Fe2O3/CNT), resulting in microwave-assisted bacterial killing (Qiao et al., 2020). However, more studies are needed to further reduce the cytotoxicities of PANs.
In the current review, we have meticulously reviewed the literature and summarized the ongoing advances in the photothermal inactivation of drug-resistant bacterial pathogens using nanoparticles under broad-spectrum irradiation, i.e., ultraviolet, visible, and infrared light, with a potential approach for addressing the AMR problems. Several nanomaterials, especially metal and metal oxide bases, are known for their antibacterial potencies; however, their associated toxicities, potential side effects to non-target cells, cost, and lesser biocompatibility have limited their therapeutic applications. To overcome these limitations, PANs are emerging as potential alternative approaches due to their striking antibacterial potencies with less or no toxicities for the host and biocompatibilities. These nanomaterials have many advantages for generating fast and efficient localized temperatures to eradicate drug-resistant bacterial strains. These nanoagents absorb NIR and UV light for the lysis of bacteria and their ultimate killing via targeting the drug resistance determinants. They target the cell membranes of bacteria by hyperthermia and ROS production in the presence of H2O2 and O2, leading to DNA damage and other protein inactivation. Efflux pumps are also among the main targets that are directly targeted by PANs. PANs have also found their effective use for inhibition of biofilms and QS by influencing bacterial virulence factors and autoinducing factors (AIPs). In addition, the combination of PANs with antibiotics and other materials such as nanocomposites is also being explored for enhanced photothermal effect for bacterial ablation. Interestingly, PANs have also been shown to be potent antibacterial drug carriers with controlled release and target efficiencies. Thus, PANs are of great significance in turning into wide-spectrum antibacterial therapeutics that can combat threatening AMR and contain superbugs. However, more comprehensive investigations are needed to identify new PANs with striking antibacterial potency (both bactericidal and synergistic) with less or no toxic impact on hosts, and such investigations should be undertaken. Studies are needed to decipher the underlying mechanism of action targeted by PANs for effective bactericidal and drug resistance reversal activities. One more issue to be taken into account is the need for higher cell/tissue penetration by the PANs, and thus should be the focus of future research. All these and other pharmacokinetic and pharmacodynamic studies are needed prior to clinical applications and translational success of PANs as effective antibacterial agents against drug-resistant pathogens.
VK and JP conceived the idea, wrote and revised the manuscript, and supervised the manuscript. KK, SR, PB, VS, and UA contributed to the literature survey, wrote the manuscript, and drew the figures and tables. JP performed the project administration and funded acquisition. All authors made a substantial contribution to the manuscript revision and approved it for publication.
The authors declare that the research was conducted in the absence of any commercial or financial relationships that could be construed as a potential conflict of interest.
All claims expressed in this article are solely those of the authors and do not necessarily represent those of their affiliated organizations, or those of the publisher, the editors and the reviewers. Any product that may be evaluated in this article, or claim that may be made by its manufacturer, is not guaranteed or endorsed by the publisher.
VK acknowledges the use of facilities created under the DST-FIST, DBT-Star, and DBT-BUILDER schemes implemented at the Modern College of Arts, Science and Commerce, Pune, India.
Agnihotri, S., Mohan, T., Jha, D., Gautam, H. K., and Roy, I. (2020). Dual Modality FeS Nanoparticles with Reactive Oxygen Species-Induced and Photothermal Toxicity toward Pathogenic Bacteria. ACS Omega 5, 597–602. doi: 10.1021/acsomega.9b03177
Alav, I., Sutton, J. M., and Rahman, K. M. (2018). Role of bacterial efflux pumps in biofilm formation. J. Antimicrob. Chemother. 73, 2003–2020. doi: 10.1093/jac/dky042
Al-Bakri, A. G., and Mahmoud, N. N. (2019). Photothermal-induced antibacterial activity of gold nanorods loaded into polymeric hydrogel against Pseudomonas aeruginosa biofilm. Molecules 24:2661. doi: 10.3390/molecules24142661
Alhmoud, H., Cifuentes-Rius, A., Delalat, B., Lancaster, D. G., and Voelcker, N. H. (2017). Gold-decorated porous silicon nanopillars for targeted hyperthermal treatment of bacterial infections. ACS Appl. Mater. Interfaces. 9, 33707–33716. doi: 10.1021/acsami.7b13278
Amina, M., Al Musayeib, N. M., Alarfaj, N. A., El-Tohamy, M. F., and Al-Hamoud, G. A. (2021). Facile multifunctional-mode of fabricated biocompatible human serum albumin/reduced graphene oxide/Cladophora glomerata nanoparticles for bacteriostatic phototherapy, bacterial tracking and antioxidant potential. Nanotechnology 32:31. doi: 10.1088/1361-6528/abf457
Anand, U., Jacobo-Herrera, N., Altemimi, A., and Lakhssassi, N. (2019). A comprehensive review on medicinal plants as antimicrobial therapeutics: Potential avenues of biocompatible drug discovery. Metabolites 9:258. doi: 10.3390/metabo9110258
Anand, U., Nandy, S., Mundhra, A., Das, N., Pandey, D. K., and Dey, A. (2020). A review on antimicrobial botanicals, phytochemicals and natural resistance modifying agents from Apocynaceae family: Possible therapeutic approaches against multidrug resistance in pathogenic microorganisms. Drug Resist. Updat. 51:100695. doi: 10.1016/j.drup.2020.100695
Bao, Z., Liu, X., Liu, Y., Liu, H., and Zhao, K. (2016). Near-infrared light-responsive inorganic nanomaterials for photothermal therapy. Asian J. Pharm. Sci. 11, 349–364. doi: 10.1016/j.ajps.2015.11.123
Borzenkov, M., Pallavicini, P., and Chirico, G. (2019). Photothermally Active Inorganic Nanoparticles: from Colloidal Solutions to Photothermally Active Printed Surfaces and Polymeric Nanocomposite Materials. Eur. J. Inorg. Chem. 41, 4397–4404. doi: 10.1002/ejic.201900836
Borzenkov, M., Pallavicini, P., Taglietti, A., D’Alfonso, L., Collini, M., and Chirico, G. (2020). Photothermally active nanoparticles as a promising tool for eliminating bacteria and biofilms. Beilstein J. Nanotechnol. 11, 1134–1146. doi: 10.3762/BJNANO.11.98
Canaparo, R., Foglietta, F., Giuntini, F., Pepa, C., Della, Dosio, F., et al. (2019). Recent developments in antibacterial therapy: Focus on stimuli-responsive drug-delivery systems and therapeutic nanoparticles. Molecules 24:1991. doi: 10.3390/molecules24101991
Cao, J., Sun, Q., Shen, A. G., Fan, B., and Hu, J. M. (2021). Nano Au@Cu2-xS with near-infrared photothermal and peroxidase catalytic activities redefines efficient antibiofilm-oriented root canal therapy. Chem. Eng. J. 422:130090. doi: 10.1016/j.cej.2021.130090
Chen, J., Ning, C., Zhou, Z., Yu, P., Zhu, Y., Tan, G., et al. (2019). Nanomaterials as photothermal therapeutic agents. Prog. Mater. Sci. 99, 1–26. doi: 10.1016/j.pmatsci.2018.07.005
Chen, Y., Gao, Y., Chen, Y., Liu, L., Mo, A., and Peng, Q. (2020). Nanomaterials-based photothermal therapy and its potentials in antibacterial treatment. J. Control. Release. 328, 251–262. doi: 10.1016/j.jconrel.2020.08.055
Chiang, W. L., Lin, T. T., Sureshbabu, R., Chia, W. T., Hsiao, H. C., Liu, H. Y., et al. (2015). A rapid drug release system with a NIR light-activated molecular switch for dual-modality photothermal/antibiotic treatments of subcutaneous abscesses. J. Control. Release. 199, 53–62. doi: 10.1016/j.jconrel.2014.12.011
Chopra, B., and Dhingra, A. K. (2020). Efflux Pump and its Inhibitors: Novel Targets to Combat Drug Resistance. Anti-Infective Agents 19:3. doi: 10.2174/2211352518999201123191915
Chu, X., Zhang, P., Wang, Y., Sun, B., Liu, Y., Zhang, Q., et al. (2021). Near-infrared carbon dot-based platform for bioimaging and photothermal/photodynamic/quaternary ammonium triple synergistic sterilization triggered by single NIR light source. Carbon N. Y. 176, 126–138. doi: 10.1016/j.carbon.2021.01.119
Cui, T., Wu, S., Sun, Y., Ren, J., and Qu, X. (2020). Self-Propelled Active Photothermal Nanoswimmer for Deep-Layered Elimination of Biofilm in Vivo. Nano Lett. 20, 7350–7358. doi: 10.1021/acs.nanolett.0c02767
Dai, X., Ma, J., Chen, N., Cai, Y., He, Y., Li, X., et al. (2021). MSNs-Based Nanocomposite for Biofilm Imaging and NIR-Activated Chem/Photothermal/Photodynamic Combination Therapy. ACS Appl. Bio Mater. 4, 2810–2820. doi: 10.1021/acsabm.1c00034
De Aberasturi, D. J., Serrano-Montes, A. B., and Liz-Marzán, L. M. (2015). Modern Applications of Plasmonic Nanoparticles: From Energy to Health. Adv. Opt. Mater. 3, 602–617. doi: 10.1002/adom.201500053
de Melo, M. A., de, C., Celiešiūtė-Germanienė, R., Šimonis, P., and Stirkė, A. (2021). Antimicrobial photodynamic therapy (aPDT) for biofilm treatments. Possible synergy between a PDT and pulsed electric fields. Virulence 12, 2247–2272. doi: 10.1080/21505594.2021.1960105
Della Rocca, J., Liu, D., and Lin, W. (2012). Are high drug loading nanoparticles the next step forward for chemotherapy? Nanomedicine 23, 1–7. doi: 10.2217/nnm.11.191.Are
Dey, N., Kamatchi, C., Vickram, A. S., Anbarasu, K., Thanigaivel, S., Palanivelu, J., et al. (2022). Role of nanomaterials in deactivating multiple drug resistance efflux pumps – A review. Environ. Res. 204:111968. doi: 10.1016/j.envres.2021.111968
Dunne, W. M. (2002). Bacterial adhesion: Seen any good biofilms lately? Clin. Microbiol. Rev. 15, 155–166. doi: 10.1128/CMR.15.2.155-166.2002
El-Baky, R. M. A., Sandle, T., John, J., Abuo-Rahma, G. E. D. A., and Hetta, H. F. (2019). A novel mechanism of action of ketoconazole: Inhibition of the nora efflux pump system and biofilm formation in multidrug-resistant staphylococcus aureus. Infect. Drug Resist. 12, 1703–1718. doi: 10.2147/IDR.S201124
Eleraky, N. E., Allam, A., Hassan, S. B., and Omar, M. M. (2020). Nanomedicine fight against antibacterial resistance: an overview of the recent pharmaceutical innovations. Pharmaceutics. 12:142. doi: 10.3390/pharmaceutics12020142
Exner, M., Bhattacharya, S., Christiansen, B., Gebel, J., Goroncy-Bermes, P., Hartemann, P., et al. (2017). Antibiotic resistance: What is so special about multidrug-resistant Gram-negative bacteria? GMS Hyg. Infect. Control. 2017:290. doi: 10.3205/dgkh000290
Fan, X. L., Li, H. Y., Ye, W. Y., Zhao, M. Q., Huang, D., Fang, Y., et al. (2019). Magainin-modified polydopamine nanoparticles for photothermal killing of bacteria at low temperature. Colloids Surf. B Biointerf. 183:110423. doi: 10.1016/j.colsurfb.2019.110423
Feng, W., Nie, W., Cheng, Y., Zhou, X., Chen, L., Qiu, K., et al. (2015). In vitro and in vivo toxicity studies of copper sulfide nanoplates for potential photothermal applications. Nanomed. Nanotechnol. Biol. Med. 11, 901–912. doi: 10.1016/j.nano.2014.12.015
Feng, Y., Chen, Q., Yin, Q., Pan, G., Tu, Z., and Liu, L. (2019). Reduced Graphene Oxide Functionalized with Gold Nanostar Nanocomposites for Synergistically Killing Bacteria through Intrinsic Antimicrobial Activity and Photothermal Ablation. ACS Appl. Bio Mater. 2, 747–756. doi: 10.1021/acsabm.8b00608
Feng, Y., Liu, L., Zhang, J., Aslan, H., and Dong, M. (2017). Photoactive antimicrobial nanomaterials. J. Mater. Chem. B. 44, 8631–8652. doi: 10.1039/c7tb01860f
Friedrich, A. W. (2019). Control of hospital acquired infections and antimicrobial resistance in Europe: the way to go. Wiener Medizinische Wochenschrift. 169, 25–30. doi: 10.1007/s10354-018-0676-5
Gao, G., Jiang, Y. W., Jia, H. R., and Wu, F. G. (2019). Near-infrared light-controllable on-demand antibiotics release using thermo-sensitive hydrogel-based drug reservoir for combating bacterial infection. Biomaterials 188, 83–95. doi: 10.1016/j.biomaterials.2018.09.045
Gao, R., van der Mei, H. C., Ren, Y., Chen, H., Chen, G., Busscher, H. J., et al. (2021). Thermo-resistance of ESKAPE-panel pathogens, eradication and growth prevention of an infectious biofilm by photothermal, polydopamine-nanoparticles in vitro. Nanomed. Nanotechnol. Biol. Med. 2021:102324. doi: 10.1016/j.nano.2020.102324
Geske, G. D., Wezeman, R. J., Siegel, A. P., and Blackwell, H. E. (2005). Small molecule inhibitors of bacterial quorum sensing and biofilm formation. J. Am. Chem. Soc. 127, 12762–12763. doi: 10.1021/ja0530321
Guo, X., Cao, B., Wang, C., Lu, S., and Hu, X. (2020). In vivo photothermal inhibition of methicillin-resistant Staphylococcus aureus infection by in situ templated formulation of pathogen-targeting phototheranostics. Nanoscale 12, 7651–7659. doi: 10.1039/d0nr00181c
Gupta, D., Chauhan, V., and Kumar, R. (2020). A comprehensive review on synthesis and applications of molybdenum disulfide (MoS2) material: Past and recent developments. Inorg. Chem. Commun. 121:108200. doi: 10.1016/j.inoche.2020.108200
Han, D., Han, Y., Li, J., Liu, X., Yeung, K. W. K., Zheng, Y., et al. (2020a). Enhanced photocatalytic activity and photothermal effects of cu-doped metal-organic frameworks for rapid treatment of bacteria-infected wounds. Appl. Catal. B Environ. 261:118248. doi: 10.1016/j.apcatb.2019.118248
Han, D., Li, Y., Liu, X., Li, B., Han, Y., Zheng, Y., et al. (2020b). Rapid bacteria trapping and killing of metal-organic frameworks strengthened photo-responsive hydrogel for rapid tissue repair of bacterial infected wounds. Chem. Eng. J. 396:125194. doi: 10.1016/j.cej.2020.125194
Han, D. L., Yu, P. L., Liu, X. M., Xu, Y., De, and Wu, S. L. (2021). Polydopamine modified CuS@HKUST for rapid sterilization through enhanced photothermal property and photocatalytic ability. Rare Met. 2021:1. doi: 10.1007/s12598-021-01786-1
He, D., Yang, T., Qian, W., Qi, C., Mao, L., Yu, X., et al. (2018). Combined photothermal and antibiotic therapy for bacterial infection via acidity-sensitive nanocarriers with enhanced antimicrobial performance. Appl. Mater. Today 12, 415–429. doi: 10.1016/j.apmt.2018.07.006
He, X., Lu, F., Yuan, F., Jiang, D., Zhao, P., Zhu, J., et al. (2015). Biofilm formation caused by clinical acinetobacter baumannii isolates is associated with overexpression of the AdeFGH efflux pump. Antimicrob. Agents Chemother. 59, 4817–4825. doi: 10.1128/AAC.00877-15
Hemeg, H. A. (2017). Nanomaterials for alternative antibacterial therapy. Int. J. Nanomedicine. 12, 8211–8225. doi: 10.2147/IJN.S132163
Hu, B., Zhang, L. P., Chen, X. W., and Wang, J. H. (2013). Gold nanorod-covered kanamycin-loaded hollow SiO2 (HSKAu rod) nanocapsules for drug delivery and photothermal therapy on bacteria. Nanoscale 5, 246–252. doi: 10.1039/c2nr32457a
Hu, D., Li, H., Wang, B., Ye, Z., Lei, W., Jia, F., et al. (2017). Surface-Adaptive Gold Nanoparticles with Effective Adherence and Enhanced Photothermal Ablation of Methicillin-Resistant Staphylococcus aureus Biofilm. ACS Nano 11, 9330–9339. doi: 10.1021/acsnano.7b04731
Hu, D., Zou, L., Li, B., Hu, M., Ye, W., and Ji, J. (2019). Photothermal Killing of Methicillin-Resistant Staphylococcus aureus by Bacteria-Targeted Polydopamine Nanoparticles with Nano-Localized Hyperpyrexia. ACS Biomater. Sci. Eng. 5, 5169–5179. doi: 10.1021/acsbiomaterials.9b01173
Hu, J. J., Cheng, Y. J., and Zhang, X. Z. (2018). Recent advances in nanomaterials for enhanced photothermal therapy of tumors. Nanoscale 48, 22657–22672. doi: 10.1039/c8nr07627h
Huang, B., Tan, L., Liu, X., Li, J., and Wu, S. (2019). A facile fabrication of novel stuff with antibacterial property and osteogenic promotion utilizing red phosphorus and near-infrared light. Bioact. Mater. 4, 17–21. doi: 10.1016/j.bioactmat.2018.11.002
Huang, W. C., Tsai, P. J., and Chen, Y. C. (2007). Functional gold nanoparticles as photothermal agents for selective-killing of pathogenic bacteria. Nanomedicine 2, 777–787. doi: 10.2217/17435889.2.6.777
Huo, S., Gao, Y., Fang, L., Jiang, Z., Xie, Q., Meng, Q., et al. (2021). Graphene oxide with acid-activated bacterial membrane anchoring for improving synergistic antibacterial performances. Appl. Surf. Sci. 551:149444. doi: 10.1016/j.apsusc.2021.149444
Iqbal, G., Faisal, S., Khan, S., Shams, D. F., and Nadhman, A. (2019). Photo-inactivation and efflux pump inhibition of methicillin resistant Staphylococcus aureus using thiolated cobalt doped ZnO nanoparticles. J. Photochem. Photobiol. B Biol. 192, 141–146. doi: 10.1016/j.jphotobiol.2019.01.021
Irie, Y., and Parsek, M. R. (2008). Quorum sensing and microbial biofilms. Curr. Top. Microbiol. Immunol. 322, 67–84. doi: 10.1007/978-3-540-75418-3_4
Jiao, Y., Niu, L., Ma, S., Li, J., Tay, F. R., and Chen, J. (2017). Quaternary ammonium-based biomedical materials: State-of-the-art, toxicological aspects and antimicrobial resistance. Prog. Polym. Sci. 71, 53–90. doi: 10.1016/j.progpolymsci.2017.03.001
Kalhapure, R. S., Suleman, N., Mocktar, C., Seedat, N., and Govender, T. (2015). Nanoengineered drug delivery systems for enhancing antibiotic therapy. J. Pharm. Sci. 104, 872–905. doi: 10.1002/jps.24298
Khan, S., Faisal, S., Shams, D. F., Zia, M., and Nadhman, A. (2019). Photo-inactivation of bacteria in hospital effluent via thiolated iron-doped nanoceria. IET Nanobiotech. 13, 875–879. doi: 10.1049/iet-nbt.2019.0149
Khan, S., Khan, S. N., Akhtar, F., Misba, L., Meena, R., and Khan, A. U. (2020). Inhibition of multi-drug resistant Klebsiella pneumoniae: Nanoparticles induced photoinactivation in presence of efflux pump inhibitor. Eur. J. Pharm. Biopharm. 157, 165–174. doi: 10.1016/j.ejpb.2020.10.007
Khare, T., Anand, U., Dey, A., Assaraf, Y. G., Chen, Z. S., Liu, Z., et al. (2021a). Exploring Phytochemicals for Combating Antibiotic Resistance in Microbial Pathogens. Front. Pharmacol. 12:720726. doi: 10.3389/fphar.2021.720726
Khare, T., Mahalunkar, S., Shriram, V., Gosavi, S., and Kumar, V. (2021b). Embelin-loaded chitosan gold nanoparticles interact synergistically with ciprofloxacin by inhibiting efflux pumps in multidrug-resistant Pseudomonas aeruginosa and Escherichia coli. Environ. Res. 199:111321. doi: 10.1016/j.envres.2021.111321
Khatoon, Z., McTiernan, C. D., Suuronen, E. J., Mah, T. F., and Alarcon, E. I. (2018). Bacterial biofilm formation on implantable devices and approaches to its treatment and prevention. Heliyon. 4:e01067. doi: 10.1016/j.heliyon.2018.e01067
Kim, M., Lee, J. H., and Nam, J. M. (2019). Plasmonic Photothermal Nanoparticles for Biomedical Applications. Adv. Sci. 6:1900471. doi: 10.1002/advs.201900471
Kim, T. H., Raiz, A., Unni, A. D., Murhekar, S., Donose, B. C., Floetenmeyer, M., et al. (2020). Combating Antibiotic-Resistant Gram-Negative Bacteria Strains with Tetracycline-Conjugated Carbon Nanoparticles. Adv. Biosyst. 4:2000074. doi: 10.1002/adbi.202000074
Kirui, D. K., Weber, G., Talackine, J., and Millenbaugh, N. J. (2019). Targeted laser therapy synergistically enhances efficacy of antibiotics against multi-drug resistant Staphylococcus aureus and Pseudomonas aeruginosa biofilms. Nanomed. Nanotechnol. Biol. Med. 20:102018. doi: 10.1016/j.nano.2019.102018
Koch, N., Islam, N. F., Sonowal, S., Prasad, R., and Sarma, H. (2021). Environmental antibiotics and resistance genes as emerging contaminants: Methods of detection and bioremediation. Curr. Res. Microb. Sci. 2:100027. doi: 10.1016/j.crmicr.2021.100027
Kong, X., Liu, X., Zheng, Y., Chu, P. K., Zhang, Y., and Wu, S. (2021). Graphitic carbon nitride-based materials for photocatalytic antibacterial application. Mater. Sci. Eng. R Reports. 145:100610. doi: 10.1016/j.mser.2021.100610
Kung, M. L., Tai, M. H., Lin, P. Y., Wu, D. C., Wu, W. J., Yeh, B. W., et al. (2017). Silver decorated copper oxide (Ag@CuO) nanocomposite enhances ROS-mediated bacterial architecture collapse. Coll. Surf. B Biointerf. 155, 399–407. doi: 10.1016/j.colsurfb.2017.04.041
Lai, B. H., and Chen, D. H. (2013). Vancomycin-modified LaB6@SiO2/Fe3O 4 composite nanoparticles for near-infrared photothermal ablation of bacteria. Acta Biomater. 9, 7573–7579. doi: 10.1016/j.actbio.2013.03.023
Lee, N. Y., Ko, W. C., and Hsueh, P. R. (2019). Nanoparticles in the treatment of infections caused by multidrug-resistant organisms. Front. Pharmacol. 10:1153. doi: 10.3389/fphar.2019.01153
Levard, C., Hotze, E. M., Lowry, G. V., and Brown, G. E. (2012). Environmental transformations of silver nanoparticles: Impact on stability and toxicity. Environ. Sci. Technol. 2012:2037405. doi: 10.1021/es2037405
Li, J., Li, Z., Liu, X., Li, C., Zheng, Y., Yeung, K. W. K., et al. (2021). Interfacial engineering of Bi2S3/Ti3C2Tx MXene based on work function for rapid photo-excited bacteria-killing. Nat. Commun. 12:1224. doi: 10.1038/s41467-021-21435-6
Li, J., Liu, X., Tan, L., Cui, Z., Yang, X., Liang, Y., et al. (2019a). Zinc-doped Prussian blue enhances photothermal clearance of Staphylococcus aureus and promotes tissue repair in infected wounds. Nat. Commun. 10:4490. doi: 10.1038/s41467-019-12429-6
Li, J., Liu, X., Zhou, Z., Tan, L., Wang, X., Zheng, Y., et al. (2019b). Lysozyme-assisted photothermal eradication of methicillin-resistant Staphylococcus aureus infection and accelerated tissue repair with natural melanosome nanostructures. ACS Nano. 13, 11153–11167. doi: 10.1021/acsnano.9b03982
Li, M., Li, L., Su, K., Liu, X., Zhang, T., Liang, Y., et al. (2019c). Highly Effective and Noninvasive Near-Infrared Eradication of a Staphylococcus aureus Biofilm on Implants by a Photoresponsive Coating within 20 Min. Adv. Sci. 6:201900599. doi: 10.1002/advs.201900599
Li, W., Geng, X., Liu, D., and Li, Z. (2019d). Near-infrared light-enhanced protease-conjugated gold nanorods as a photothermal antimicrobial agent for elimination of exotoxin and biofilms. Int. J. Nanomed. 14, 8047–8058. doi: 10.2147/IJN.S212750
Li, X., Li, S., Bai, Q., Sui, N., and Zhu, Z. (2020). Gold nanoclusters decorated amine-functionalized graphene oxide nanosheets for capture, oxidative stress, and photothermal destruction of bacteria. Coll. Surf. B Biointerf. 196:111313. doi: 10.1016/j.colsurfb.2020.111313
Li, Y., Liu, X., Li, B., Zheng, Y., Han, Y., Chen, D. F., et al. (2020). Near-Infrared Light Triggered Phototherapy and Immunotherapy for Elimination of Methicillin-Resistant Staphylococcus aureus Biofilm Infection on Bone Implant. ACS Nano. 14, 8157–8170. doi: 10.1021/acsnano.0c01486
Li, Y., Wang, L., Liu, H., Pan, Y., Li, C., Xie, Z., et al. (2021). Ionic covalent-organic framework nanozyme as effective cascade catalyst against bacterial wound infection. Small 17:2100756. doi: 10.1002/smll.202100756
Li, Z., Bai, H., Jia, S., Yuan, H., Gao, L. H., and Liang, H. (2021). Design of functional polymer nanomaterials for antimicrobial therapy and combatting resistance. Mater. Chem. Front. 5, 1236–1252. doi: 10.1039/d0qm00837k
Lin, J., He, Z., Liu, F., Feng, J., Huang, C., Sun, X., et al. (2020). Hybrid hydrogels for synergistic periodontal antibacterial treatment with sustained drug release and NIR-responsive photothermal effect. Int. J. Nanomed. 15, 5377–5387. doi: 10.2147/IJN.S248538
Lin, Y., Liu, X., Liu, Z., and Xu, Y. (2021). Visible-Light-Driven Photocatalysis-Enhanced Nanozyme of TiO2 Nanotubes@MoS2 Nanoflowers for Efficient Wound Healing Infected with Multidrug-Resistant Bacteria. Small 2021:202103348. doi: 10.1002/smll.202103348
Linklater, D. P., Baulin, V. A., Juodkazis, S., and Ivanova, E. P. (2018). Mechano-bactericidal mechanism of graphene nanomaterials. Interface Focus. 8:20170060. doi: 10.1098/rsfs.2017.0060
Liu, S., Pan, X., and Liu, H. (2020). Two-Dimensional Nanomaterials for Photothermal Therapy. Angew. Chemie - Int. Ed. 59, 5890–2900. doi: 10.1002/anie.201911477
Liu, Y., Guo, Z., Li, F., Xiao, Y., Zhang, Y., Bu, T., et al. (2019). Multifunctional Magnetic Copper Ferrite Nanoparticles as Fenton-like Reaction and Near-Infrared Photothermal Agents for Synergetic Antibacterial Therapy. ACS Appl. Mater. Interfaces. 11, 31649–31660. doi: 10.1021/acsami.9b10096
Liu, Z., Tan, L., Liu, X., Liang, Y., Zheng, Y., Yeung, K. W. K., et al. (2020). Zn2+-assisted photothermal therapy for rapid bacteria-killing using biodegradable humic acid encapsulated MOFs. Colloids Surfaces B Biointerfaces. 188:110781. doi: 10.1016/j.colsurfb.2020.110781
Luo, Y., Liu, X., Tan, L., Li, Z., Yeung, K. W. K., Zheng, Y., et al. (2021). Enhanced photocatalytic and photothermal properties of ecofriendly metal-organic framework heterojunction for rapid sterilization. Chem. Eng. J. 405:126730. doi: 10.1016/j.cej.2020.126730
Lv, R., Liang, Y. Q., Li, Z. Y., Zhu, S. L., Cui, Z. D., and Wu, S. L. (2021). Flower-like CuS/graphene oxide with photothermal and enhanced photocatalytic effect for rapid bacteria-killing using visible light. Rare Met. 2021:4. doi: 10.1007/s12598-021-01759-4
Mao, C., Xiang, Y., Liu, X., Cui, Z., Yang, X., Li, Z., et al. (2018). Repeatable Photodynamic Therapy with Triggered Signaling Pathways of Fibroblast Cell Proliferation and Differentiation to Promote Bacteria-Accompanied Wound Healing. ACS Nano 12, 1747–1759. doi: 10.1021/acsnano.7b08500
Mao, C., Zhu, W., Xiang, Y., Zhu, Y., Shen, J., Liu, X., et al. (2021). Enhanced Near-Infrared Photocatalytic Eradication of MRSA Biofilms and Osseointegration Using Oxide Perovskite-Based P–N Heterojunction. Adv. Sci. 8:2002211. doi: 10.1002/advs.202002211
Mapara, N., Sharma, M., Shriram, V., Bharadwaj, R., Mohite, K. C., and Kumar, V. (2015). Antimicrobial potentials of Helicteres isora silver nanoparticles against extensively drug-resistant (XDR) clinical isolates of Pseudomonas aeruginosa. Appl. Microbiol. Biotechnol. 99, 10655–10667. doi: 10.1007/s00253-015-6938-x
Meeker, D. G., Jenkins, S. V., Miller, E. K., Beenken, K. E., Loughran, A. J., Powless, A., et al. (2016). Synergistic Photothermal and Antibiotic Killing of Biofilm-Associated Staphylococcus aureus Using Targeted Antibiotic-Loaded Gold Nanoconstructs. ACS Infect. Dis. 2, 241–250. doi: 10.1021/acsinfecdis.5b00117
Menazea, A. A., and Ahmed, M. K. (2020). Synthesis and antibacterial activity of graphene oxide decorated by silver and copper oxide nanoparticles. J. Mol. Struct. 1218:128536. doi: 10.1016/j.molstruc.2020.128536
Merkl, P., Zhou, S., Zaganiaris, A., Shahata, M., Eleftheraki, A., Thersleff, T., et al. (2021). Plasmonic Coupling in Silver Nanoparticle Aggregates and Their Polymer Composite Films for near -Infrared Photothermal Biofilm Eradication. ACS Appl. Nano Mater. 4, 5330–5339. doi: 10.1021/acsanm.1c00668
Mishra, P. K., Mishra, H., Ekielski, A., Talegaonkar, S., and Vaidya, B. (2017). Zinc oxide nanoparticles: a promising nanomaterial for biomedical applications. Drug Discov. Today. 22, 1825–1834. doi: 10.1016/j.drudis.2017.08.006
Moorcroft, S. C. T., Roach, L., Jayne, D. G., Ong, Z. Y., Ong, Z. Y., and Evans, S. D. (2020). Nanoparticle-Loaded Hydrogel for the Light-Activated Release and Photothermal Enhancement of Antimicrobial Peptides. ACS Appl. Mater. Interfaces. 12, 24544–24554. doi: 10.1021/acsami.9b22587
Morrison, L., and Zembower, T. R. (2020). Antimicrobial Resistance. Gastrointest. Endosc. Clin. N. Am. 30, 619–635. doi: 10.1016/j.giec.2020.06.004
Naidi, S. N., Khan, F., Tan, A. L., Harunsani, M. H., Kim, Y. M., and Khan, M. M. (2021). Photoantioxidant and antibiofilm studies of green synthesized Sn-doped CeO2nanoparticles using aqueous leaf extracts of: Pometia pinnata. New J. Chem. 17, 7816–7829. doi: 10.1039/d1nj00416f
Naveed Ul Haq, A., Nadhman, A., Ullah, I., Mustafa, G., Yasinzai, M., and Khan, I. (2017). Synthesis Approaches of Zinc Oxide Nanoparticles: The Dilemma of Ecotoxicity. J. Nanomater. 2017:8510342. doi: 10.1155/2017/8510342
Ocsoy, I., Yusufbeyoglu, S., Yılmaz, V., McLamore, E. S., Ildız, N., and Ülgen, A. (2017). DNA aptamer functionalized gold nanostructures for molecular recognition and photothermal inactivation of methicillin-Resistant Staphylococcus aureus. Colloids Surfaces B Biointerfaces. 159, 16–22. doi: 10.1016/j.colsurfb.2017.07.056
O’Neill, J. (2014). Antimicrobial Resistance: Tackling a Crisis for the Health and Wealth of Nations. Rev. Antimicrob. Resist. 2014:2014.
Patra, P., Roy, S., Sarkar, S., Mitra, S., Pradhan, S., Debnath, N., et al. (2015). Damage of lipopolysaccharides in outer cell membrane and production of ROS-mediated stress within bacteria makes nano zinc oxide a bactericidal agent. Appl. Nanosci. 5, 857–866. doi: 10.1007/s13204-014-0389-z
Qiao, Y., Liu, X., Li, B., Han, Y., Zheng, Y., Yeung, K. W. K., et al. (2020). Treatment of MRSA-infected osteomyelitis using bacterial capturing, magnetically targeted composites with microwave-assisted bacterial killing. Nat. Commun. 11:4446. doi: 10.1038/s41467-020-18268-0
Qiao, Y., Ma, F., Liu, C., Zhou, B., Wei, Q., Li, W., et al. (2018). Near-Infrared Laser-Excited Nanoparticles to Eradicate Multidrug-Resistant Bacteria and Promote Wound Healing. ACS Appl. Mater. Interfaces. 10, 193–206. doi: 10.1021/acsami.7b15251
Qin, Z., Zheng, Y., Du, T., Wang, Y., Gao, H., Quan, J., et al. (2021). Cysteamine: A key to trigger aggregation-induced NIR-II photothermal effect and silver release booming of gold-silver nanocages for synergetic treatment of multidrug-resistant bacteria infection. Chem. Eng. J. 414:128779. doi: 10.1016/j.cej.2021.128779
Qing, G., Zhao, X., Gong, N., Chen, J., Li, X., Gan, Y., et al. (2019). Thermo-responsive triple-function nanotransporter for efficient chemo-photothermal therapy of multidrug-resistant bacterial infection. Nat. Commun. 10:4336. doi: 10.1038/s41467-019-12313-3
Rajesh, S., Koshi, E., Philip, K., and Mohan, A. (2011). Antimicrobial photodynamic therapy: An overview. J. Indian Soc. Periodontol. 15, 323–327. doi: 10.4103/0972-124X.92563
Ranjan, S., and Ramalingam, C. (2016). Titanium dioxide nanoparticles induce bacterial membrane rupture by reactive oxygen species generation. Environ. Chem. Lett. 14, 487–494. doi: 10.1007/s10311-016-0586-y
Rao, H., Choo, S., Mahalingam, S. R. R., Adisuri, D. S., Madhavan, P., Akim, A. M., et al. (2021). Approaches for mitigating microbial biofilm-related drug resistance: a focus on micro- and nanotechnologies. Molecules 26:1870. doi: 10.3390/molecules26071870
Ren, Y., Liu, H., Liu, X., Zheng, Y., Li, Z., Li, C., et al. (2020). Photoresponsive Materials for Antibacterial Applications. Cell Reports Phys. Sci. 1:100245. doi: 10.1016/j.xcrp.2020.100245
Sadekuzzaman, M., Yang, S., Mizan, M. F. R., and Ha, S. D. (2015). Current and Recent Advanced Strategies for Combating Biofilms. Compr. Rev. Food Sci. Food Saf. 14, 491–509. doi: 10.1111/1541-4337.12144
Sahu, A., Lee, J. H., Lee, H. G., Jeong, Y. Y., and Tae, G. (2016). Prussian blue/serum albumin/indocyanine green as a multifunctional nanotheranostic agent for bimodal imaging guided laser mediated combinatorial phototherapy. J. Control. Release. 236, 90–99. doi: 10.1016/j.jconrel.2016.06.031
Sahu, S. C., and Hayes, A. W. (2017). Toxicity of nanomaterials found in human environment. Toxicol. Res. Appl. 2017:2397847317726352. doi: 10.1177/2397847317726352
Shriram, V., Khare, T., Bhagwat, R., Shukla, R., and Kumar, V. (2018). Inhibiting bacterial drug efflux pumps via phyto-therapeutics to combat threatening antimicrobial resistance. Front. Microbiol. 9:2990. doi: 10.3389/fmicb.2018.02990
Song, J., Li, J., Bai, X., Kang, L., Ma, L., Zhao, N., et al. (2021). Cu nanoparticle-decorated two-dimensional carbon nanosheets with superior photothermal conversion efficiency of 65 % for highly efficient disinfection under near-infrared light. J. Mater. Sci. Technol. 87, 83–94. doi: 10.1016/j.jmst.2021.01.057
Spengler, G., Kincses, A., Gajdács, M., and Amaral, L. (2017). New roads leading to old destinations: Efflux pumps as targets to reverse multidrug resistance in bacteria. Molecules. 22:468. doi: 10.3390/molecules22030468
Sun, J., Song, L., Fan, Y., Tian, L., Luan, S., Niu, S., et al. (2019). Synergistic Photodynamic and Photothermal Antibacterial Nanocomposite Membrane Triggered by Single NIR Light Source. ACS Appl. Mater. Interfaces. 11, 26581–26589. doi: 10.1021/acsami.9b07037
Sun, P., Zhang, Y., Ran, X., Liu, C., Wang, Z., Ren, J., et al. (2018). Phytochemical-encapsulated nanoplatform for “on-demand” synergistic treatment of multidrug-resistant bacteria. Nano Res. 11, 3762–3770. doi: 10.1007/s12274-017-1947-y
Sun, Y., Qin, H., Yan, Z., Zhao, C., Ren, J., and Qu, X. (2019). Combating Biofilm Associated Infection In Vivo: Integration of Quorum Sensing Inhibition and Photodynamic Treatment based on Multidrug Delivered Hollow Carbon Nitride Sphere. Adv. Funct. Mater. 29, 1–12. doi: 10.1002/adfm.201808222
Sun, Y., Qin, H., Yan, Z., Zhao, C., Ren, J., and Qu, X. (2020). Combating Biofilm Associated Infection In Vivo: Integration of Quorum Sensing Inhibition and Photodynamic Treatment based on Multidrug Delivered Hollow Carbon Nitride Sphere. Adv. Funct. Mater. 29:1808222. doi: 10.1002/adfm.201909740
Tan, L., Li, J., Liu, X., Cui, Z., Yang, X., Zhu, S., et al. (2018). Rapid Biofilm Eradication on Bone Implants Using Red Phosphorus and Near-Infrared Light. Adv. Mater. 30, 1801808. doi: 10.1002/adma.201801808
Tan, S., Wu, X., Xing, Y., Lilak, S., Wu, M., and Zhao, J. X. (2020). Enhanced synergetic antibacterial activity by a reduce graphene oxide/Ag nanocomposite through the photothermal effect. Colloids Surfaces B Biointerfaces. 185:110616. doi: 10.1016/j.colsurfb.2019.110616
Taneja, N., and Sharma, M. (2019). Antimicrobial resistance in the environment: The Indian scenario. Indian J. Med. Res. 149, 119–128. doi: 10.4103/ijmr.IJMR_331_18
Thakur, N., Manna, P., and Das, J. (2019). Synthesis and biomedical applications of nanoceria, a redox active nanoparticle. J. Nanobiotech. 17:84. doi: 10.1186/s12951-019-0516-9
Tiwari, P., Khare, T., Shriram, V., Bae, H., and Kumar, V. (2021). Plant synthetic biology for producing potent phyto-antimicrobials to combat antimicrobial resistance. Biotechnol. Adv. 48:107729. doi: 10.1016/j.biotechadv.2021.107729
Vangara, A., Pramanik, A., Gao, Y., Gates, K., Begum, S., and Ray, P. C. (2018). Fluorescence resonance energy transfer based highly efficient theranostic nanoplatform for two-photon bioimaging and two-photon excited photodynamic therapy of multiple drug resistance bacteria. ACS Appl. Bio Mater. 1, 298–309. doi: 10.1021/acsabm.8b00071
Wang, C., Wang, Y., Zhang, L., Miron, R. J., Liang, J., Shi, M., et al. (2018). Pretreated Macrophage-Membrane-Coated Gold Nanocages for Precise Drug Delivery for Treatment of Bacterial Infections. Adv. Mater. 30:1804023. doi: 10.1002/adma.201804023
Wang, S. G., Chen, Y. C., and Chen, Y. C. (2018). Antibacterial gold nanoparticle-based photothermal killing of vancomycin-resistant bacteria. Nanomedicine 13, 1405–1416. doi: 10.2217/nnm-2017-0380
Wang, X., Shi, Q., Zha, Z., Zhu, D., Zheng, L., Shi, L., et al. (2021). Copper single-atom catalysts with photothermal performance and enhanced nanozyme activity for bacteria-infected wound therapy. Bioact. Mater. 6, 4389–4401. doi: 10.1016/j.bioactmat.2021.04.024
Wasa, A., Land, J. G., Gorthy, R., Krumdieck, S., Bishop, C., Godsoe, W., et al. (2021). Antimicrobial and biofilm-disrupting nanostructured TiO2 coating demonstrating photoactivity and dark activity. FEMS Microbiol. Lett. 368:fnab039. doi: 10.1093/femsle/fnab039
Wei, G., Yang, G., Wang, Y., Jiang, H., Fu, Y., Yue, G., et al. (2020). Phototherapy-based combination strategies for bacterial infection treatment. Theranostics 10, 12241–12262. doi: 10.7150/thno.52729
WHO (2020). World Health Organization. Available online at: https://www.who.int/news-room/fact-sheets/detail/antimicrobial-resistance (accessed October 03, 2021).
Wu, Q., Liu, X., Li, B., Tan, L., Han, Y., Li, Z., et al. (2021). Eco-friendly and degradable red phosphorus nanoparticles for rapid microbial sterilization under visible light. J. Mater. Sci. Technol. 67, 70–79. doi: 10.1016/j.jmst.2020.04.084
Xiang, Y., Zhou, Q., Li, Z., Cui, Z., Liu, X., Liang, Y., et al. (2020). A Z-scheme heterojunction of ZnO/CDots/C3N4 for strengthened photoresponsive bacteria-killing and acceleration of wound healing. J. Mater. Sci. Technol. 57, 1–11. doi: 10.1016/j.jmst.2020.05.016
Xie, X., Mao, C., Liu, X., Tan, L., Cui, Z., Yang, X., et al. (2018). Tuning the Bandgap of Photo-Sensitive Polydopamine/Ag3PO4/Graphene Oxide Coating for Rapid, Noninvasive Disinfection of Implants. ACS Cent. Sci. 4, 724–738. doi: 10.1021/acscentsci.8b00177
Xie, X., Mao, C., Liu, X., Zhang, Y., Cui, Z., Yang, X., et al. (2017). Synergistic Bacteria Killing through Photodynamic and Physical Actions of Graphene Oxide/Ag/Collagen Coating. ACS Appl. Mater. Interfaces. 9, 26417–26428. doi: 10.1021/acsami.7b06702
Xie, Y., Qian, Y., Li, Z., Liang, Z., Liu, W., Yang, D., et al. (2021). Near-infrared-activated efficient bacteria-killing by lignin-based copper sulfide nanocomposites with an enhanced photothermal effect and peroxidase-like activity. ACS Sustain. Chem. Eng. 9, 6479–6488. doi: 10.1021/acssuschemeng.1c01589
Xiong, K., Li, J., Tan, L., Cui, Z., Li, Z., Wu, S., et al. (2019). Ag2S decorated nanocubes with enhanced near-infrared photothermal and photodynamic properties for rapid sterilization. Colloids Interface Sci. Commun. 33:100201. doi: 10.1016/j.colcom.2019.100201
Xu, X., Liu, X., Tan, L., Cui, Z., Yang, X., Zhu, S., et al. (2018). Controlled-temperature photothermal and oxidative bacteria killing and acceleration of wound healing by polydopamine-assisted Au-hydroxyapatite nanorods. Acta Biomater. 77, 352–364. doi: 10.1016/j.actbio.2018.07.030
Xu, Y., Liu, X., Zheng, Y., Li, C., Kwok Yeung, K. W., Cui, Z., et al. (2021). Ag3PO4 decorated black urchin-like defective TiO2 for rapid and long-term bacteria-killing under visible light. Bioact. Mater. 6, 1575–1587. doi: 10.1016/j.bioactmat.2020.11.013
Yang, D., Tu, Y., Wang, X., Cao, C., Hu, Y., Shao, J., et al. (2021). A photo-triggered antifungal nanoplatform with efflux pump and heat shock protein reversal activity for enhanced chemo-photothermal synergistic therapy. Biomater. Sci. 9, 3293–3299. doi: 10.1039/d1bm00457c
Yang, X., Li, Z., Ju, E., Ren, J., and Qu, X. (2014). Reduced graphene oxide functionalized with a luminescent rare-earth complex for the tracking and photothermal killing of drug-resistant bacteria. Chem. - A Eur. J. 20, 394–398. doi: 10.1002/chem.201303964
Yang, Y., He, P., Wang, Y., Bai, H., Wang, S., Xu, J. F., et al. (2017). Supramolecular Radical Anions Triggered by Bacteria In Situ for Selective Photothermal Therapy. Angew. Chemie - Int. Ed. 56, 16239–16242. doi: 10.1002/anie.201708971
Yang, Z., Sun, Z., Ren, Y., Chen, X., Zhang, W., Zhu, X., et al. (2019). Advances in nanomaterials for use in photothermal and photodynamic therapeutics (Review). Mol. Med. Rep. 20, 5–15. doi: 10.3892/mmr.2019.10218
Yin, M., Qiao, Z., Yan, D., Yang, M., Yang, L., Wan, X., et al. (2021). Ciprofloxacin conjugated gold nanorods with pH induced surface charge transformable activities to combat drug resistant bacteria and their biofilms. Mater. Sci. Eng. C. 128:112292. doi: 10.1016/j.msec.2021.112292
Yin, W., Yu, J., Lv, F., Yan, L., Zheng, L. R., Gu, Z., et al. (2016). Functionalized Nano-MoS2 with Peroxidase Catalytic and Near-Infrared Photothermal Activities for Safe and Synergetic Wound Antibacterial Applications. ACS Nano. 10, 11000–11011. doi: 10.1021/acsnano.6b05810
Yougbaré, S., Mutalik, C., Krisnawati, D. I., Kristanto, H., Jazidie, A., Nuh, M., et al. (2020). Nanomaterials for the photothermal killing of bacteria. Nanomaterials 10:1123. doi: 10.3390/nano10061123
Yu, Y., Lu, L., Yang, Q., Zupanic, A., Xu, Q., and Jiang, L. (2021). Using MoS2 Nanomaterials to Generate or Remove Reactive Oxygen Species: A Review. ACS Appl. Nano Mater. 4, 7523–7537. doi: 10.1021/acsanm.1c00751
Yu, Z., Tang, J., Khare, T., and Kumar, V. (2020). The alarming antimicrobial resistance in ESKAPEE pathogens: Can essential oils come to the rescue? Fitoterapia 140:104433. doi: 10.1016/j.fitote.2019.104433
Yuan, P., Ding, X., Guan, Z., Gao, N., Ma, R., Jiang, X. F., et al. (2015). Plasmon-Coupled Gold Nanospheres for Two-Photon Imaging and Photoantibacterial Activity. Adv. Healthc. Mater. 4, 674–678. doi: 10.1002/adhm.201400524
Yuan, Z., Lin, C., Dai, L., He, Y., Hu, J., Xu, K., et al. (2021). Near-Infrared Light-Activatable Dual-Action Nanoparticle Combats the Established Biofilms of Methicillin-Resistant Staphylococcus aureus and Its Accompanying Inflammation. Small 17:e2007522. doi: 10.1002/smll.202007522
Zhang, W., Yang, C., Lei, Z., Guan, G., He, S. A., Zhang, Z., et al. (2019). New strategy for specific eradication of implant-related infections based on special and selective degradability of rhenium trioxide nanocubes. ACS Appl. Mater. Interfaces. 11, 25691–25701. doi: 10.1021/acsami.9b07359
Zhang, Y., Li, D., Tan, J., Chang, Z., Liu, X., Ma, W., et al. (2021). Near-Infrared Regulated Nanozymatic/Photothermal/Photodynamic Triple-Therapy for Combating Multidrug-Resistant Bacterial Infections via Oxygen-Vacancy Molybdenum Trioxide Nanodots. Small 17:e2005739. doi: 10.1002/smll.202005739
Zhang, Y., Ma, J., Wang, D., Xu, C., Sheng, S., Cheng, J., et al. (2020). Fe-TCPP@CS nanoparticles as photodynamic and photothermal agents for efficient antimicrobial therapy. Biomater. Sci. 8, 6526–6532. doi: 10.1039/d0bm01427c
Zhang, Z., Sun, J., Chen, X., Wu, G., Jin, Z., Guo, D., et al. (2021). The synergistic effect of enhanced photocatalytic activity and photothermal effect of oxygen-deficient Ni/reduced graphene oxide nanocomposite for rapid disinfection under near-infrared irradiation. J. Hazard. Mater. 419:126462. doi: 10.1016/j.jhazmat.2021.126462
Zhao, Y., Chen, L., Wang, Y., Song, X., Li, K., Yan, X., et al. (2021). Nanomaterial-based strategies in antimicrobial applications: Progress and perspectives. Nano Res. 2021:4. doi: 10.1007/s12274-021-3417-4
Zhao, Y., Yu, C., Yu, Y., Wei, X., Duan, X., Dai, X., et al. (2019). Bioinspired Heteromultivalent Ligand-Decorated Nanotherapeutic for Enhanced Photothermal and Photodynamic Therapy of Antibiotic-Resistant Bacterial Pneumonia. ACS Appl. Mater. Interfaces. 11, 39648–39661. doi: 10.1021/acsami.9b15118
Zheng, Q., Liu, X., Zheng, Y., Yeung, K. W. K., Cui, Z., Liang, Y., et al. (2021). The recent progress on metal-organic frameworks for phototherapy. Chem. Soc. Rev. 2021:56. doi: 10.1039/d1cs00056j
Zhu, M., Liu, X., Tan, L., Cui, Z., Liang, Y., Li, Z., et al. (2020). Photo-responsive chitosan/Ag/MoS2 for rapid bacteria-killing. J. Hazard. Mater. 2020:121122. doi: 10.1016/j.jhazmat.2019.121122
Keywords: antibiotics, multidrug resistance, nanoparticles, drug efflux pumps, ROS, antimicrobial resistance, photothermal agents, photothermal therapy 3
Citation: Kaur K, Reddy S, Barathe P, Shriram V, Anand U, Proćków J and Kumar V (2021) Combating Drug-Resistant Bacteria Using Photothermally Active Nanomaterials: A Perspective Review. Front. Microbiol. 12:747019. doi: 10.3389/fmicb.2021.747019
Received: 25 July 2021; Accepted: 15 October 2021;
Published: 12 November 2021.
Edited by:
Renata Katsuko Takayama Kobayashi, State University of Londrina, BrazilCopyright © 2021 Kaur, Reddy, Barathe, Shriram, Anand, Proćków and Kumar. This is an open-access article distributed under the terms of the Creative Commons Attribution License (CC BY). The use, distribution or reproduction in other forums is permitted, provided the original author(s) and the copyright owner(s) are credited and that the original publication in this journal is cited, in accordance with accepted academic practice. No use, distribution or reproduction is permitted which does not comply with these terms.
*Correspondence: Jarosław Proćków, amFyb3NsYXcucHJvY2tvd0B1cHdyLmVkdS5wbA==; Vinay Kumar, dmluYXltYWxpazEyM0BnbWFpbC5jb20=; orcid.org/0000-0002-9569-2411
Disclaimer: All claims expressed in this article are solely those of the authors and do not necessarily represent those of their affiliated organizations, or those of the publisher, the editors and the reviewers. Any product that may be evaluated in this article or claim that may be made by its manufacturer is not guaranteed or endorsed by the publisher.
Research integrity at Frontiers
Learn more about the work of our research integrity team to safeguard the quality of each article we publish.