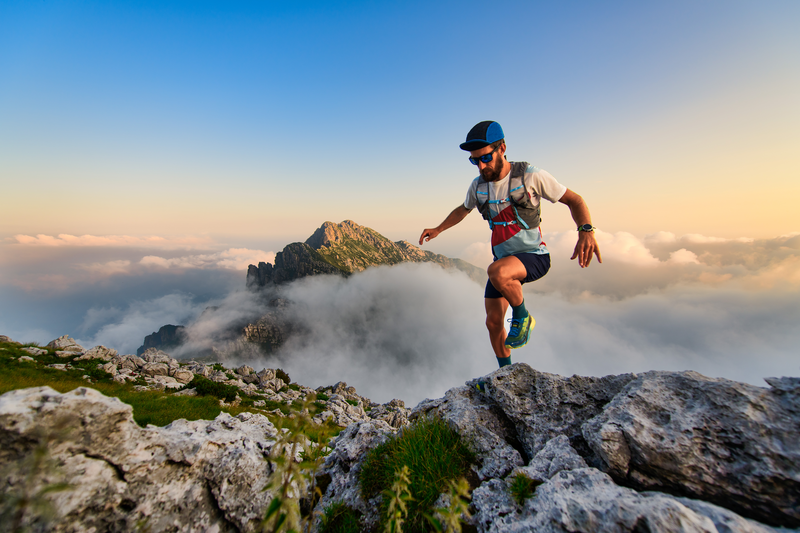
94% of researchers rate our articles as excellent or good
Learn more about the work of our research integrity team to safeguard the quality of each article we publish.
Find out more
REVIEW article
Front. Microbiol. , 07 October 2021
Sec. Infectious Agents and Disease
Volume 12 - 2021 | https://doi.org/10.3389/fmicb.2021.737299
This article is part of the Research Topic Molecular Adaptations of Vibrionaceae to Changing Environments, Volume II View all 5 articles
Vibrio parahaemolyticus (Vp) is an aquatic Gram-negative bacterium that may infect humans and cause gastroenteritis and wound infections. The first pandemic of Vp associated infection was caused by the serovar O3:K6 and epidemics caused by the other serovars are increasingly reported. The two major virulence factors, thermostable direct hemolysin (TDH) and/or TDH-related hemolysin (TRH), are associated with hemolysis and cytotoxicity. Vp strains lacking tdh and/or trh are avirulent and able to colonize in the human gut and cause infection using other unknown factors. This pathogen is well adapted to survive in the environment and human host using several genetic mechanisms. The presence of prophages in Vp contributes to the emergence of pathogenic strains from the marine environment. Vp has two putative type-III and type-VI secretion systems (T3SS and T6SS, respectively) located on both the chromosomes. T3SS play a crucial role during the infection process by causing cytotoxicity and enterotoxicity. T6SS contribute to adhesion, virulence associated with interbacterial competition in the gut milieu. Due to differential expression, type III secretion system 2 (encoded on chromosome-2, T3SS2) and other genes are activated and transcribed by interaction with bile salts within the host. Chromosome-1 encoded T6SS1 has been predominantly identified in clinical isolates. Acquisition of genomic islands by horizontal gene transfer provides enhanced tolerance of Vp toward several antibiotics and heavy metals. Vp consists of evolutionarily conserved targets of GTPases and kinases. Expression of these genes is responsible for the survival of Vp in the host and biochemical changes during its survival. Advanced genomic analysis has revealed that various genes are encoded in Vp pathogenicity island that control and expression of virulence in the host. In the environment, the biofilm gene expression has been positively correlated to tolerance toward aerobic, anaerobic, and micro-aerobic conditions. The genetic similarity analysis of toxin/antitoxin systems of Escherichia coli with VP genome has shown a function that could induce a viable non-culturable state by preventing cell division. A better interpretation of the Vp virulence and other mechanisms that support its environmental fitness are important for diagnosis, treatment, prevention and spread of infections. This review identifies some of the common regulatory pathways of Vp in response to different stresses that influence its survival, gut colonization and virulence.
Vibrio parahaemolyticus (Vp) is a facultative anaerobic Gram-negative bacterium, belonging to the family Vibrionaceae. Serotyping scheme of Vp is based on the antigenic properties of the somatic (O) and capsule (K) antigens, which includes a combination of more than 10 different O and 70 K antigens (Iguchi et al., 1995). This organism is ubiquitous in marine, estuarine ecosystems and a leading cause of seafood-borne diarrheal disease in humans (Li et al., 2019). Vp infected patients typically present with gastroenteritis symptoms such as abdominal cramping, nausea, vomiting, and fever. Patients with comorbidities such as diabetes, liver disease and alcoholism are more likely to develop septicemia. Since its discovery in 1950, this bacterium has been isolated in widespread outbreaks and in sporadic cases of gastroenteritis worldwide (Fujino et al., 1953; Nair et al., 2007). The first pandemic of Vp-associated diarrheal infection emerged in 1996 with a new serotype O3:K6 from Calcutta (now Kolkata), India, which has spread to Asia, Europe, and Americas in the following years (Nair et al., 2007). The number of serovariants all over the world has increased to 49 by 2016 (Han et al., 2016). During 1990–2019, Vp was responsible for more than 40 global outbreaks (Igere and Ekundayo, 2020).
The pathogenicity of Vp depends on a variety of virulence factors. Details of known virulence factors of this pathogen are presented in Table 1. Typically, expression of virulence in Vp is strongly associated with thermostable direct hemolysin (TDH) and/or the TDH-related hemolysin (TRH; Fattel et al., 2019). Stains that lacked tdh, trh, and T3SS2 caused several diarrheal outbreaks and expressed virulence in animal models (Bhoopong et al., 2007; Chao et al., 2010; Ottaviani et al., 2012; Banerjee et al., 2014; Martinez-Urtaza et al., 2016). However, the exact mechanism is yet to be established.
Whole genome sequencing of Vp revealed the presence of two sets of type III secretion systems (T3SS; T3SS1, and T3SS2; Matsuda et al., 2020). Of these, T3SS1 gene cluster is ubiquitous in both pathogenic and nonpathogenic strains. Whereas, the T3SS2 gene cluster located in an 80-kb Vp pathogenicity island on the chromosome-2 and has been linked with virulence strains that cause acute gastroenteritis (Okada et al., 2009).
To survive in the environments and in the human host, Vp has several adaptive mechanisms for temperatures, salinity, oxidative stress, and bile salts. Conversion of viable but non-culturable (VBNC) from biofilm formation, quorum sensing regulators, and toxin–antitoxin systems are activated in response to various environmental conditions (Letchumanan et al., 2017; Banerjee et al., 2018). In addition, the intake of prophages and horizontal gene transfer into bacterial chromosomes enhance fitness of the pathogen to encounter several adverse changes in the host (Yang et al., 2020). This review highlights the gaps in our knowledge on how the inherent and acquired factors enhance adaptive mechanisms in Vp that mark the pathogen to survive and enhance the pathogenesis under several stressful conditions.
Adaptation to changing environmental conditions is an important approach for the survival of bacterial pathogens. The combination of climatic and aquatic environmental changes has shaped an ideal condition for the emergence, spread, and resurgence of several infectious diseases, affecting millions of people annually (Deb et al., 2012; Hales, 2019). Many anthropogenic factors, like industrial development, human behavior, and intensive animal farming, have contributed to upsurge in the incidence of infectious diseases (Gage et al., 2008). Of the several factors, the ecosystem variability is most important, as it enhances genomic alternation of some of the pathogenic microorganisms through horizontal gene transfer or mutation, giving rise to new transmission links (Siliakus et al., 2017). Bacteria frequently encounter numerous environmental abiotic stresses (heat, cold, osmotic, salt, oxidation, pH, and radiation) and biotic stresses (antimicrobial compounds and microbial toxins) in their natural life cycle (Beales, 2004). These environmental stresses cause damage to cellular components and inhibit the function of macromolecules that causes bacteria to adapt to varying environmental changes for its survival and proliferation (Brooks et al., 2011; Collin and Hernroth, 2020). Several other defense mechanisms like different growth phases, catalases, and genetic changes can also help them adapt in such stressful environments (Liu et al., 2016; Lovell, 2017). Most of the pathogens equivocate between the mammalian host and natural habitats to utilize the environmental signals to coordinate virulence related gene expression (Thomas and Wigneshweraraj, 2014).
Gastrointestinal pathogens, namely, Vibrio spp., Shigella spp., and Yersinia spp., and pathogenic Escherichia coli explore different signals within the human host by expressing several virulence genes. Among these, temperature is one of important activators (Freestone, 2013; Fang et al., 2016). Most of the environmental strains may have conserved virulence-associated mechanisms, but the clinical strains showed a notable difference in response to the human temperature (37°C) such as biofilm formation, motility, and protease production (Mahoney et al., 2010; Hu and Zhang, 2020; Qian et al., 2020). The ecology of Vp is largely influenced by temperature and salinity (Urmersbach et al., 2015). Vp can grow in a wide range of temperature from 16 to 42°C, but the optimum temperature for growth is 37°C (Beuchat, 1982). Rise in the temperature could lead to increase in the abundance of Vp in the environment and therefore could lead to an escalation in the incidence of infections, especially among shellfish consumers during summer months (Yeung and Boor, 2004; Duan and Su, 2005; Chiang and Chou, 2009).
Low temperatures alter gene expression in Vp, for, e.g., the cold-shock proteins (CspA and CspD) are known to protect the Vp at low temperatures (Yang et al., 2009; Zhu et al., 2017). Several stress proteins are formed under the high temperature condition, e.g., heat-shock protein families such as Hsp60 (GroEL and GroES) and Hsp70 (DnaJ, DnaK, and GrpE; Segal and Ron, 1998). These changing temperatures can affect the pathogenicity or the virulence factor expression in Vp (Wong et al., 2002; Chiang et al., 2008). The heat shock causes higher expression of tdh (Wong et al., 2002), whereas the T3SS-1 gets downregulated at 15°C and a putative chaperone, hemolytic activity, and the T6SS are upregulated at higher temperatures (Salomon et al., 2013; Urmersbach et al., 2015; Li et al., 2019). The higher temperature also favors an increase in the expression of urease that helps in survival in the gastric acids (Park et al., 2009). The environmental strains that lack the putative virulence genes (tdh/trh) have the ability to regulate or control differently conserved “virulence-related traits” in response to human body temperature (Mahoney et al., 2010).
The bacterial cells enter into the VBNC state under extreme conditions, namely, starvation condition and temperature stress (Ramamurthy et al., 2014). Viable but non-culturable state of Vp is likely to cause a food safety threat to public health, as the organism has been identified by the culture-based methods (Fakruddin et al., 2013; Yoon and Lee, 2020). Vp enters into the VBNC state in vitro under starvation conditions after 12 days at 4°C or after 30 days in artificial seawater (Mizunoe et al., 2000; Yoon et al., 2017). Under such conditions, Vp shows reduced metabolic activities, losses of colony-forming ability, decreased in ATP synthesis as well as transcription of RNA (Trevors et al., 2012; Jia et al., 2014).
Accumulation of reactive oxygen species (ROS) has been identified as one of the key factors related to the formation of VBNC cells (Cabiscol et al., 2000). Hydrogen peroxide, hydroxyl-free radical, and superoxide anion are the major ROS compounds that enter across the membrane and cell wall under aerobic conditions and degrade the polyunsaturated proteins and fatty acids. This condition causes a reduction in membrane fluidity, which enhances the formation of VBNC cells (McDougald et al., 2002). Vp can develop specific survival mechanisms using several antioxidant defense systems against ROS, including alkyl hydroperoxide reductase (AhpC), catalase, and peroxidase (KatG; Lai and Wong, 2013). Using suitable stimuli in the medium supplemented with catalase or sodium pyruvate (H2O2-degrading compounds), the VBNC cells have been resuscitated to culturable cells (Mizunoe et al., 2000).
Interestingly, the VBNC state of Vp has shown the ability of adhesion and virulence expression under in vitro conditions (Chiang et al., 2005; Tang et al., 2018). In addition, the VBNC state of Vp exhibited the strong cytotoxic activity to HEp-2 cell lines and able to colonize in vivo (Baffone et al., 2003; Wong et al., 2004). Vp can develop specific survival mechanisms using several antioxidant defense systems against ROS, namely, alkyl hydroperoxide reductase (AhpC), catalase, and KatG (Lai and Wong, 2013).
Vp can survive varying concentrations of NaCl (0.5%–10.5%) for their growth and maintain an osmotic balance with their external environment. The optimal concentration of 3% NaCl has been used in the different media for the growth and isolation of this Vibrio (Wong and Wang, 2004). To support the cell stability in the presence of high salinity concentrations, Vp has different halophilic proteins, characterized by a large number of acidic amino acids, negatively charged with hydrated carboxyl groups, and less in hydrophobic amino acids (Ongagna-Yhombi and Boyd, 2013; Gregory and Boyd, 2021). Lysine decarboxylase (encoded by cadA) is another acid-resistance transcriptional system that has been well-characterized in enteric pathogens (Neely and Olson, 1996; Merrell and Camilli, 1999). Under acidic conditions, cadA has induced the adaptation of the amino acid lysine to the basic product cadaverine, which increases the hydroxide ions (Soksawatmaekhin et al., 2004). In Vp, cadA is responsible for decarboxylation of lysine and transcriptional expression of the lysine decarboxylase in the presence of external lysine (Tanaka et al., 2008). In Vp, the cadA expression was significantly higher in a minimal medium with 3% NaCl than with 1% NaCl (Whitaker et al., 2010). The importance of cadA was further demonstrated in Vp mutant strain, which had a shorter survival in high saline condition (Kalburge et al., 2014).
RNA polymerase, which is a sigma S (RpoS) factor, generally regulates various genes and enhances cross-protection against several environmental stresses (Stokes et al., 2003). RpoS is important for cell survival under oxidative and acidic stress conditions and also controls the expression of mechanosensitive channels in different enteric bacteria including Vp (Vasudevan and Venkitanarayanan, 2006; Tan et al., 2010). Expression of rpoS in Vp is generally higher to support the survival in the high saline conditions. rposS mutant stain exhibited significantly decreased resistance when grown in 3% NaCl, indicating the importance of this gene’s function (Huang and Wong, 2012). rpoS also plays a crucial role in the survival of Vp under the stressed conditions of cold and hyperosmolarity (Whitaker et al., 2010). Vp uses catalases, alkyl hydroperoxide reductases, antioxidative enzymes, and antioxidative encoding genes, which protect against different oxidizing agents such as hydrogen peroxide (H2O2), peracetic acid (C2H4O3), and chlorine dioxide (ClO2; Chen et al., 2016; Yu et al., 2016). These oxidizing agents are commonly used in food and health care industries to inactivate vegetative cells and spores of food-borne pathogenic bacteria and for the treatment of fresh produce, seafood, and ready-to-eat food (Leggett et al., 2015; Tso et al., 2019). Several genes encoding key antioxidant enzymes, namely, katE, oxyR, rpoS, and ahpC, protect Vp from oxidizing agents (Chen et al., 2016; Yu et al., 2016).
Ethanol is commonly used as a food preservative or to disinfect microorganism from the surface of utensils and equipment to maintain a hygienic environment in food processing industries. However, microorganisms significantly increased their resistance to ethanol after adaptation to a sublethal dose of ethanol (Chiang et al., 2006). Vp resistance up to a concentration of 8% ethanol at 47°C. Under this condition, several changes have been observed in Vp including cell morphology, expression of a different protein, catalase, higher expression of tdh, changes in the fatty acid profile, and increased susceptibility to high salt, crystal violet, and organic acid stresses (Chiang and Chou, 2008; Chiang et al., 2008).
Cholic and chenodeoxycholic acid are the primary bile acids synthesized in the liver, which are conjugated to glycine or taurine before secretion. The colonic bacteria in the intestine covert the primary bile acids to the secondary bile acids (deoxycholic acid and lithocholic acid). Bile salts are needed in the human gastrointestinal tract to support break down of fats, aid digestion, absorb vitamins, and inactivate bacterial toxins (Begley et al., 2005; Sistrunk et al., 2016). In addition, bile salts also prevent colonization of pathogenic bacteria in the gastrointestinal tract (Urdaneta and Casadesús, 2017). Enteric pathogens sense bile as an environmental cue to control or regulate their virulence factors by decreasing membrane permeability, inducing biofilm formation and efflux pumps, and upregulating redox and DNA damage repair genes (Gunn, 2000; Merritt and Donaldson, 2009).
Vp uses bile salts as an environmental signal to upregulate virulence genes during infection (Rivera-Cancel and Orth, 2017). In the gut, the presence of conjugated bile acids enhances the expression of tdh and activation of T3SS2 systems, which consequently increases the enterotoxicity with acute gastroenteritis (Osawa and Yamai, 1996; Letchumanan et al., 2017). In the presence of bile salts, Vp uses inner-membrane proteins, VtrA, VtrB, and VtrC, which activate the T3SS2 (Li et al., 2016). The highly conserved VtrA/VtrC form a 1:1 protein complex through their periplasmic domains to form a membrane-bound receptor and activates VtrB on the surface of the membrane to induce the expression of T3SS2-related genes through the Vp pathogenicity island (PAI) promoters (Letchumanan et al., 2017). The protein complex creates a barrel-like structure that can bind to bile salts and trigger the cell to produce toxins.
Expressions of virulence-associated genes in Vp depend on the bacterial cell density. This phenomenal change has been recognized as Quorum Sensing (QS), which is very well-documented in most of the vibrios. Quorum Sensing regulates the gene expression with respect to changes in the bacterial cell density by autoinduction of various genes. At a low-cell density level of vibrios, Sigma 54-dependent factor regulates genes such as aphA and opaR (Kalburge et al., 2017). The role of aphA and opaR is important in the gut colonization of Vp. LuxR family transcriptional regulator aphA is important for the expression of opaR and the regulation of lateral flagella (Lu et al., 2019). Double mutant of aphA and opaR genes affects gut colonization of Vp in vivo (Kalburge et al., 2017). OpaR controls metalloprotease, serine, and protease genes that regulate environmental survival and bacterial virulence (Chang and Lee, 2020). An extracellular serine protease encoding gene prtA involved in nutrient uptake as well as hemolytic and cytotoxic activities is required for establishing infection (Chang and Lee, 2018).
The quorum sensing synthase gene cqsA, which corresponds to VPA0711 in Vp strain RIMD2210633 genome, has been reported to give the signal through 3-hydroxyundecan-4 one molecule that regulates colony morphology and upregulation of another QS-associated gene opaR (Wu et al., 2019). At a low cell density of Vp in the environment and host, nitric oxide (NO) activates the master QS regulatory gene opaR. Nitric oxide is associated with the bacterial life cycle by involving an active role in the regulation of biofilm production, metabolism, and singling pathways of cyclic di-GMP (Ueno et al., 2019). This cyclic di-GMP is programmed by a capsular polysaccharide (CpsQ), which is part of the membrane fusion protein (MFP). The protein-encoding MFP locus is required for biofilm production in Vp (Zhang et al., 2021).
The lux operon encodes various genes, which are self-regulated and produce luminescent proteins. This was originally discovered in Vibrio fishceri. luxM and luxS are implicated in the adaptation of Vp in various niches (Guo et al., 2018). At high concentrations, due to the presence of lux operon, Vp produces a biomolecule called autoinducer (AI, 4-hydroxy-5-methyl-3(2h)-furanone and naphthalene derivatives), which supports QS-mediated regulation of biofilm formation and virulence (Vendeville et al., 2005; Mizan et al., 2017). Swarming is important for Vp to grow on different surfaces. A three-gene operon (scrABC) has been identified for swarming effect, which encodes a pyridoxal-phosphate-dependent enzyme, an extracellular solute-binding protein, and a membrane-bound GGDEF- and EAL-motif sensory protein. These genes may control up- and downregulation of lateral flagellar gene expression and capsular polysaccharide (CPS) production in Vp. In addition, a gene encoding a diguanidylate cyclase/phosphodiesterase GGDEF-EAL domain protein inversely regulates the swarming effect and CPS production in bacteria by modulating small signaling nucleotide cyclic di-GMP (Boles and McCarter, 2002; Trimble and McCarter, 2011). An omics-based study identified a hypothetical protein, VP0610, which acts on bacterial phosphotransferase and QS systems and that yield several phenotypical changes in Vp such as biofilm formation, swimming motility, and swarming effect (Jiang et al., 2021).
Vp causes diarrhea in humans and several infections in fish/shrimps. Horizontal Gene Transfer (HGT) in Vp make changes in serovars and provide several functional benefits including antimicrobial resistance. HGT make alterations in the genome of Vp by making modifications in the total G+C content, location of superintegrons and prophages and thereby facilitating the emergence of pandemic strains of Vp (Espejo et al., 2017). The presence of acquired genes helps this bacterium to adapt to various conditions in humans and the marine environment (Makino et al., 2003). Some of the genes that acquired thorough HGT support the regulation of ToxR and many of them are involved in the up- or downregulation either in the human host or environment (Figure 1). In the aquatic environment, chitin supports natural transformation of genetic materials in Vp. Overexpression of the master regulator TfoX occurs in the presence of chitin, which allows the natural transformation of Vp (Chimalapati et al., 2018). The serovar O3:K6 has been recognized as the first pandemic strain and from which other serovars, like O4:K68, O1:K25, O1:KUT, and O6:K1, are emerged and spread in several countries. The whole-genome analysis of these serovariants has shown several recombination events like insertion and duplication genetic traits in the lipopolysaccharide and capsular polysaccharide loci via HGT (Espejo et al., 2017). Capsular modification is beneficial for bacterial persistence, adaptation to diverse environmental conditions, and preventing bacteria from physical and chemical stress in various niches (Klein et al., 2018; Rendueles et al., 2018).
Figure 1. Up- and downregulated in genes in V. parahaemolyticus for adaptation and fitness in human and environmental niches. HGT, horizontal gene transfer; VBNC, viable but non-culturable; T3SS, type III secretion system; T6SS, type VI secretion system.
Pathogenicity island (PAI) and most of the AMR encoding genes in Vp are acquired mostly by HGT. AMR encoding genes that correspond to resistance to ampicillin, streptomycin, amikacin, kanamycin, tetracycline, chloramphenicol, and colistin have been reported in Vp (Li et al., 2014; Elmahdi et al., 2018; Jiang et al., 2019). Resistance to third-generation cephalosporins have also been reported in Vp isolated from shrimps (Liu et al., 2013; Letchumanan et al., 2015).
Transferable conjugative plasmids are responsible for the transmission of most of these AMR determinants (Liu et al., 2013; Han et al., 2015). In addition, Vp develops mutations in the chromosome to confer resistance to fluoroquinolones (Lei et al., 2020). Some of the genes transferred into the PAI and transposons help in the integration of foreign DNA into the genome of Vp. In the genomic island of Vp, a Na+/H+ antiporter encoding gene nhaA has shown to transport ions across the membrane to balance the pH (Klein et al., 2018). Zinc is an important micronutrient required for bacterial metalloenzyme activation, intracellular invasion, survival, and replication within the host. In Vp, horizontally acquired gene zunA has been identified, which helps in zinc uptake through ZnuACB transportation and provides better adaptability of Vp in the new environment (Liu et al., 2013). The genes encoded on the chromosome-1 and 2 of Vp express several essential functional proteins (Figure 2). The horizontally acquired gene vpaH expresses a histone-like nucleoid structure (H-NS), which regulates the biosynthesis of lateral flagella and supports motility and QS (Park et al., 2005). Genomic analysis of Vp has also shown a set of horizontally acquired gene encoding the T3SS and toxin–antitoxin functions (Ramisetty and Santhosh, 2016).
Figure 2. Major virulence and essential functional proteins of V. parahaemolyticus. Expressed gene functions are shown separately for chromosome-1 and 2.
A comparative genomic analysis of environmental and clinical strains identified several types of prophages and the majority of them were found in environmental strains. Bacteriophages are known to infect bacteria and hijack various cellular machinery of the host. Phages are self-replicating, self-limiting, and resist secondary metabolites produced by the bacteria. Many of these products are beneficial to the host by enhancing the fitness to survive in the different environmental conditions (Wendling et al., 2021). Most of the marine viruses identified to have bacteriophages, which play a crucial role in controlling bacterial mortality, gene expression, and also promote the horizontal gene transfer (Zabala et al., 2009; Simmons et al., 2018). The prophages such as Vp58.5, Vp882, and VP06 in Vp enhance the function of ultraviolet sensitivity, DNA methylase, and quorum sense and resist environmental stress, respectively (Lan et al., 2009; Zabala et al., 2009; Wong et al., 2019). Strain-specific Martha12B12 phage that encode a hypothetical protein-encoding gene VpaChn25_0724 was found to not only influence bacterial growth, motility, biofilm formation, production of secretomes, and also protects bacteria against host phagocytosis (Yang et al., 2020).
Prophages not only determine the evolutionary tread of Vp but also help the bacterium in its normal function and expression of virulence. The presence of the phage VfO3K6 in Vp was found to be associated with acute hepatopancreatic necrosis disease in shrimps (Yu et al., 2020). The epidemic strength of Vp has been correlated with the acquisition of specific open reading the frame (ORF)-8 by the infective phage f237. The ORF-8 protein has been reported for adhesion of Vp in the host intestine and also the surface of marine plankton (Nasu et al., 2000). A clinical non-toxigenic Vp strain isolated from Chile was found to have a prophage-like element that was similar to f237 phage that encoded gene for the Zonula occludens toxin (Pérez-Reytor et al., 2020). This toxin has been recognized as an important factor for Vibrio cholerae for intestinal permeation in the mammalian host cell and destabilization of the cytoskeleton of the host cell (Fasano et al., 1995). As a defense mechanism, bacteria have the clustered regularly interspaced short palindromic repeats (CRISPR) along with Cas proteins, which damage the DNA from similar bacteriophages during subsequent infections (Deveau et al., 2010).
Toxin–Antitoxin (TA) is a set of closely linked genes that collectively encode both a “toxin” protein and a corresponding “antitoxin.” Toxin–antitoxin systems are classified into several types based on how the antitoxin neutralizes the toxin. Toxin–Antitoxin system may be located in the plasmid or chromosome of bacteria and contributes to virulence and bacterial fitness in different environmental conditions. Some of the chromosomal TA systems are associated with the cell functions such as responding to stress and causing cell cycle arrest (Van Melderen and Saavedra De Bast, 2009). When the bacterial cells are under stress, the TA system supports induction of VBNC state (Hayes and Low, 2009).
Genomic analysis of Vp strain RIMD2210633 has shown two gene clusters, vp1829/vp1830 and vp1842/vp1843, which had homology with E. coli TA system that encoded for DinJ/YafQ, comprising the DinJ antitoxin protein/ribosome-dependent RNase YafQ toxin (Yamaguchi and Inouye, 2011). These gene clusters are responsible for bacterial cell life at natural and different environmental conditions. The gene cluster of vp1842/vp1843 in Vp was reported to locate within a superintegron of chromosome-1, which is involved in cell growth and regulating the morphology of bacteria (Zhang J. et al., 2017). The gene vp1843 was expressed in E. coli and identified to inhibit cell growth by halting the cell division by the induction of chromosomal DNA degradation (Zhang J. et al., 2017) and to cause the induction of VBNC state in Vp (Hino et al., 2014; Zhang J. et al., 2017). The gene cluster of vp1842/vp1843 in Vp was found highly conserved in most of Vibrio species and that was identified to induce the VBNC state (Hino et al., 2014). Further, Vp1843 has been identified in the part of RelE/ParE toxin superfamily to regulate multiple functions in the bacteria like protein synthesis inhibitory activity and ribonuclease activity. Through TA mechanism, environmental Vp could acquire virulence plasmid genes encoding PirAvp/PirBvp and translate into Cry insecticidal toxin-like proteins with pore-forming activity (Lee et al., 2015). Death on curing (Doc) and prevent host death (Phd) encoding genes were identified in the TA systems in many vibrios. Multiple copies of these genes provide fitness to the PAI in Vp (Klein et al., 2018).
The TDH and TRH encoding genes are regulated by ToxR, which is a membrane-localized regulatory protein that plays an essential role in the expression of virulence and modulating bacterial persistence. ToxR is commonly present in most of the Vibrio species, but sequence similarities of this gene in Vp and other vibrios remain less than 60% (Matz et al., 2011). Based on the nucleotide constitute, five alleles of TDH and two alleles of TRH have been reported in clinical Vp strains. These toxins are important for the establishment of disease in the host and to cause gastroenteritis and diarrhea. The gene tlh encode thermolabile hemolysin, which is a phospholipase A2. However, the contribution TLH to Vp pathogenicity is unknown (Zhang and Austin, 2005). Production of hemolysin has been correlated with bacterial cell density. Decreased hemolysin production was reported with an increased cell density of Vp (Mahoney et al., 2010). In other bacterial pathogens, hemolysin secretion and functions are different and also depended on the bacterial cell density. At higher concentrations, it can lyse erythrocytes, kill epithelial cells, and damage leukocytes by inducing pores. At low concentration, hemolysin is able to alter its own cellular functions and induce host proteases, especially mesotrypsin that cause detachment of cell, cell membrane damage, and cell death (Hongwei et al., 2021).
T3SS and T6SS are encoded on the genomic islands of both clinical and environmental strains of Vp. T3SS is acquired by HGT and present in both the chromosomes of Vp as T3SS1 and T3SS2. The genes encoded in the T3SS1 express several proteins responsible for bacterial survival in the different environmental conditions by regulating biofilm production, motility, and cytotoxicity. T3SS1’s VopQ effector protein control host metabolic processes like a glycolytic, tricarboxylic acid cycle, and amino acid and also alter the host cell redox homeostasis (Nguyen et al., 2020). Genes encoded in the T3SS2 involve in the negative regulation of cellular inflammatory response, which supports bacterial survival in the host through evasion of phagocytosis (Li et al., 2019). The gene VPA0226 encoded on T3SS2 secretes lipase in the host cell cytoplasm, which is indirectly used to esterify cholesterol that allows the bacteria to escape from the cell by damaging the plasma membrane (Chimalapati et al., 2020). Collectively, T3SS effector proteins support Vp to reside, propagate within a vacuole, and able to adopt a customized intracellular lifestyle within the cytosol of a broad range of host (De Souza Santos and Orth, 2019). The function of T3SS seems niche specific. In oysters, the persistence of Vp is due to the expression of type 1 pili, type IV pili, and flagellar system, which are not associated with T3SS (Aagesen et al., 2013). Similar to T3SS, T6SS are tightly regulated by external factors and deliver several proteins that help the pathogen to compete with other bacteria populations and provide better fitness to survive in the environment (Ben-Yaakov and Salomon, 2019; Fridman et al., 2020). In addition, the effector proteins of T6SS induce the pathogenesis in the host cell by enhancing the mammalian cell adhesion, provoke an autophagy response in the macrophages, and regulate the QS (Yu et al., 2015; Zhang Y. et al., 2017).
Vp is a marine pathogen that causes gastroenteritis due to consumption of contaminated raw or undercooked seafood. Wound infections/septicemia produced by this pathogen is mainly due to exposure to coastal waters and a weak immune system. Vp is influenced by several factors in the marine environment and in the human host. Some of the important environmental factors such as inorganic pollutants, heavy metals, salt concentrations, temperature, changes in the pH, and nutritional availability play a crucial role in the life history and ecology of Vp. This pathogen has several genetic mechanisms to adapt into various such challenges that threat its survival. Quorum sensing and biofilm formation are the two important adaptive features commonly used by the vibrios. Exposer of Vp under stressed conditions lead to activation of various gene regulation systems for conversion of normal cells into VBNC state. In the environment, Vp uses several aquatic animals like shrimp, crab, and oysters to access growth factors such as amino acid, lipids, vitamins, and minerals to keep the cells metabolically active until it enters into the host. In addition, HGT of catabolic system encoding genes support the bacteria for its enhanced survival and fitness in different niches. In the host, ToxR regulation stimulates secretion systems and other virulence genes. Though the basic process of Vp associated infection is known, there is a paucity of information on how the pathogen initiates the infection in the host using different gene machinery and host cell response. Hence, there is a need for more detailed molecular/functional studies coupled with genomic analysis to understand pathogenesis of Vp.
GP and GC were equally contributed in writing the manuscript. TR corrected and edited the manuscript. All authors contributed to the article and approved the submitted version.
The authors declare that the research was conducted in the absence of any commercial or financial relationships that could be construed as a potential conflict of interest.
All claims expressed in this article are solely those of the authors and do not necessarily represent those of their affiliated organizations, or those of the publisher, the editors and the reviewers. Any product that may be evaluated in this article, or claim that may be made by its manufacturer, is not guaranteed or endorsed by the publisher.
Aagesen, A. M., Phuvasate, S., Su, Y. C., and Häse, C. C. (2013). Persistence of Vibrio parahaemolyticus in the Pacific oyster, Crassostrea gigas, is a multifactorial process involving pili and flagella but not type III secretion systems or phase variation. Appl. Environ. Microbiol. 79, 3303–3305. doi: 10.1128/AEM.00314-13
Baffone, W., Citterio, B., Vittoria, E., Casaroli, A., Campana, R., Falzano, L., et al. (2003). Retention of virulence in viable but non-culturable halophilic Vibrio spp. Int. J. Food. Microbiol. 89, 31–39. doi: 10.1016/s0168-1605(03)00102-8
Banerjee, S. K., Kearney, A. K., Nadon, C. A., Peterson, C.-Y., Tyler, K., Bakouche, L., et al. (2014). Phenotypic and genotypic characterization of Canadian clinical isolates of Vibrio parahaemolyticus collected from 2000 to 2009. J. Clin. Microbiol. 52, 1081–1088. doi: 10.1128/JCM.03047-13
Banerjee, S. K., Rutley, R., and Bussey, J. (2018). Diversity and dynamics of the Canadian coastal Vibrio community: an emerging trend detected in the temperate regions. J. Bacteriol. 200:e00787-17. doi: 10.1128/JB.00787-17
Beales, N. (2004). Adaptation of microorganisms to cold temperatures, weak acid preservatives, low pH, and osmotic stress: a Review. Compr. Rev. Food Sci. Food Saf. 3, 1–20. doi: 10.1111/j.1541-4337.2004.tb00057.x
Begley, M., Gahan, C. G. M., and Hill, C. (2005). The interaction between bacteria and bile. FEMS Microbiol. Rev. 29, 625–651. doi: 10.1016/j.femsre.2004.09.003
Bej, A. K., Patterson, D. P., Brasher, C. W., Vickery, M. C. L., Jones, D. D., and Kaysner, C. A. (1999). Detection of total and hemolysin-producing Vibrio parahaemolyticus in shellfish using multiplex PCR amplification of tl, tdh and trh. J. Microbiol. Methods 36, 215–225. doi: 10.1016/S0167-7012(99)00037-8
Ben-Yaakov, R., and Salomon, D. (2019). The regulatory network of Vibrio parahaemolyticus type VI secretion system 1. Environ. Microbiol. 21, 2248–2260. doi: 10.1111/1462-2920.14594
Bhoopong, P., Palittapongarnpim, P., Pomwised, R., Kiatkittipong, A., Kamruzzaman, M., Nakaguchi, Y., et al. (2007). Variability of properties of Vibrio parahaemolyticus strains isolated from individual patients. J. Clin. Microbiol. 45, 1544–1550. doi: 10.1128/JCM.02371-06
Boles, B. R., and McCarter, L. L. (2002). Vibrio parahaemolyticus scrABC, a novel operon affecting swarming and capsular polysaccharide regulation. J. Bacteriol. 184, 5946–5954. doi: 10.1128/jb.184.21.5946-5954.2002
Broberg, C. A., Calder, T. J., and Orth, K. (2011). Vibrio parahaemolyticus cell biology and pathogenicity determinants. Microbes Infect. 13, 992–1001. doi: 10.1016/j.micinf.2011.06.013
Broberg, C. A., Zhang, L., Gonzalez, H., Laskowski-Arce, M. A., and Orth, K. (2010). A Vibrio effector protein is an inositol phosphatase and disrupts host cell membrane integrity. Science 329, 1660–1662. doi: 10.1126/science.1192850
Brooks, A. N., Turkarslan, S., Beer, K. D., Lo, F. Y., and Baliga, N. S. (2011). Adaptation of cells to new environments. Wiley Interdiscip. Rev. Syst. Biol. Med. 3, 544–561. doi: 10.1002/wsbm.136
Burdette, D. L., Seemann, J., and Orth, K. (2009). Vibrio VopQ induces PI3- kinase- independent autophagy and antagonizes phagocytosis. Mol. Microbiol. 73, 639–649. doi: 10.1111/j.1365-2958.2009.06798.x
Cabiscol, E., Tamarit, J., and Ros, J. (2000). Oxidative stress in bacteria and protein damage by reactive oxygen species. Int. Microbiol. 3, 3–8.
Cai, Q., and Zhang, Y. (2018). Structure, function and regulation of the thermostable direct hemolysin (TDH) in pandemic Vibrio parahaemolyticus. Microb. Pathog. 123, 242–245. doi: 10.1016/j.micpath.2018.07.021
Calder, T., Kinch, L. N., Fernandez, J., Salomon, D., Grishin, N. V., and Orth, K. (2014). Vibrio type III effector VPA1380 is related to the cysteine protease domain of large bacterial toxins. PLoS One 9:e104387. doi: 10.1371/journal.pone.0104387
Chang, S. C., and Lee, C. Y. (2018). OpaR and RpoS are positive regulators of a virulence factor PrtA in Vibrio parahaemolyticus. Microbiology (Reading) 164, 221–231. doi: 10.1099/mic.0.000591
Chang, S. C., and Lee, C. Y. (2020). Quorum-Sensing regulator OpaR directly represses seven protease genes in Vibrio parahaemolyticus. Front. Microbiol. 11:534692. doi: 10.3389/fmicb.2020.534692
Chao, G., Jiao, X., Zhou, X., Wang, F., Yang, Z., Huang, J., et al. (2010). Distribution of genes encoding four pathogenicity islands (VPaIs), T6SS, biofilm, and type I pilus in food and clinical strains of Vibrio parahaemolyticus in China. Foodborne Pathog. Dis. 6, 649–658. doi: 10.1089/fpd.2009.0441
Chen, C. L., Fen, S. Y., Chung, C. H., Yu, S. C., Chien, C. L., and Wong, H. C. (2016). Functions of VPA1418 and VPA0305 catalase genes in growth of Vibrio parahaemolyticus under oxidative stress. Appl. Environ. Microbiol. 82, 1859–1867. doi: 10.1128/AEM.02547-15
Chiang, M. L., and Chou, C. C. (2008). Expression of superoxide dismutase, catalase and thermostable-direct hemolysin by, and growth in the presence of various nitrogen and carbon sources of heat-shocked and ethanol-shocked Vibrio parahaemolyticus. Int. J. Food Microbiol. 121, 268–274. doi: 10.1016/j.ijfoodmicro.2007.11.001
Chiang, M. L., and Chou, C. C. (2009). Survival of Vibrio parahaemolyticus under environmental stresses as influenced by growth phase and pre-adaptation treatment. Food Microbiol. 26, 391–395. doi: 10.1016/j.fm.2009.01.005
Chiang, M. L., Ho, W. L., and Chou, C. C. (2006). Response of Vibrio parahaemolyticus to ethanol shock. Food Microbiol. 23, 461–467. doi: 10.1016/j.fm.2005.07.001
Chiang, M. L., Ho, W. L., and Chou, C. C. (2008). Ethanol shock changes the fatty acid profile and survival behavior of Vibrio parahaemolyticus in various stress conditions. Food Microbiol. 25, 359–365. doi: 10.1016/j.fm.2007.10.002
Chiang, M. L., Yu, R. C., and Chou, C. C. (2005). Fatty acid composition, cell morphology and responses to challenge by organic acid and sodium chloride of heat-shocked Vibrio parahaemolyticus. Int. J. Food Microbiol. 104, 179–187. doi: 10.1016/j.ijfoodmicro.2005.02.007
Chimalapati, S., de Souza Santos, M., Lafrance, A. E., Ray, A., Lee, W. R., and Rivera- Cancel, G. (2020). Vibrio deploys type 2 secreted lipase to esterify cholesterol with host fatty acids and mediate cell egress. ELife 9:e58057. doi: 10.7554/eLife.58057
Chimalapati, S., de Souza Santos, M., Servage, K., De Nisco, N. J., Dalia, A. B., and Orth, K. (2018). Natural transformation in Vibrio parahaemolyticus: a rapid method to create genetic deletions. J. Bacteriol. 200:e00032-18. doi: 10.1128/JB.00032-18
Collin, B., and Hernroth, B. (2020). Experimental evaluation of survival of Vibrio parahaemolyticus in fertilized cold-water sediment. J. Appl. Microbiol. 129, 75–84. doi: 10.1111/jam.14618
De Souza Santos, M., and Orth, K. (2019). The role of the type III secretion system in the intracellular lifestyle of enteric pathogens. Microbiol. Spectr. 7:3. doi: 10.1128/microbiolspec.BAI-0008-2019
Deb, A. K., Kanungo, S., Deb, M., and Nair, G. B. (2012). Impact of climate change on health and strategies for mitigation and adaptation. WHO South East Asia J. Public Health 1, 8–19. doi: 10.4103/2224-3151.206918
Deveau, H., Garneau, J. E., and Moineau, S. (2010). CRISPR/Cas system and its role in phage-bacteria interactions. Annu. Rev. Microbiol. 64, 475–493. doi: 10.1146/annurev.micro.112408.134123
Duan, I., and Su, Y. C. (2005). Occurrence of Vibrio parahaemolyticus in two Oregon oyster growing bays. J. Food. Sci. 70, M58–M63.
Elmahdi, S., Parveen, S., Ossai, S., DaSilva, L. V., Jahncke, M., Bowers, J., et al. (2018). Vibrio parahaemolyticus and Vibrio vulnificus recovered from oysters during an oyster relay study. Appl. Environ. Microbiol. 84:e01790-17. doi: 10.1128/AEM.01790-17
Espejo, R. T., García, K., and Plaza, N. (2017). Insight into the origin and evolution of the Vibrio parahaemolyticus pandemic strain. Front. Microbiol. 8:1397. doi: 10.3389/fmicb.2017.01397
Fakruddin, M., Mannan, K. S. B., and Andrews, S. (2013). Viable but nonculturable bacteria: food safety and public health perspective. ISRN Microbiol. 2013:703813.
Fang, F. C., Frawley, E. R., Tapscott, T., and Vázquez-Torres, A. (2016). Bacterial stress responses during host infection. Cell Host Microbe 20, 133–143. doi: 10.1016/j.chom.2016.07.009
Fasano, A., Fiorentini, C., Donelli, G., Uzzau, S., Kaper, J. B., Margaretten, K., et al. (1995). Zonula occludens toxin modulates tight junctions through protein kinase C-dependent actin reorganization, in vitro. J. Clin. Invest. 96, 710–720. doi: 10.1172/JCI118114
Fattel, L., Panossian, B., Salloum, T., Abboud, E., and Tokajian, S. (2019). Genomic Features of Vibrio parahaemolyticus from Lebanon and comparison to globally diverse strains by whole-genome sequencing. Foodborne Pathog. Dis. 11, 778–787. doi: 10.1089/fpd.2018.2618
Freestone, P. (2013). Communication between bacteria and their hosts. Scientifica (Cairo) 2013:361073. doi: 10.1155/2013/361073
Fridman, C. M., Keppel, K., Gerlic, M., Bosis, E., and Salomon, D. A. (2020). Comparative genomics methodology reveals a widespread family of membrane- disrupting T6SS effectors. Nat. Commun. 11:1085. doi: 10.1038/s41467-020-14951-4
Fujino, T., Okuno, Y., Nakada, D., Aoyama, A., Fukai, K., Mukai, T., et al. (1953). On the bacteriological examination of Shirasu food poisoning. Med. J. Osaka Univ. 4, 299–304.
Gage, K. L., Burkot, T. R., Eisen, R. J., and Hayes, E. B. (2008). Climate and vectorborne diseases. Am. J. Prev. Med. 35, 436–450. doi: 10.1016/j.amepre.2008.08.030
Gregory, G. J., and Boyd, E. F. (2021). Stressed out: bacterial response to high salinity using compatible solute biosynthesis and uptake systems, lessons from Vibrionaceae. Comput. Struct. Biotechnol. J. 19, 1014–1027. doi: 10.1016/j.csbj.2021.01.030
Gunn, J. S. (2000). Mechanisms of bacterial resistance and response to bile. Microbes Infect. 2, 907–913. doi: 10.1016/s1286-4579(00)00392-0
Guo, M., Fang, Z., Sun, L., Sun, D., Wang, Y., Li, C., et al. (2018). Regulation of thermostable direct hemolysin and biofilm formation of Vibrio parahaemolyticus by quorum-sensing genes luxM and luxS. Curr. Microbiol. 75, 1190–1197. doi: 10.1007/s00284-018-1508-y
Hales, S. (2019). Climate change, extreme rainfall events, drinking water and enteric disease. Rev. Environ. Health 34, 1–3. doi: 10.1515/reveh-2019-2001
Han, C., Tang, H., Ren, C., Zhu, X., and Han, D. (2016). Sero-prevalence and genetic diversity of pandemic V. parahaemolyticus strains occurring at a global scale. Front. Microbiol. 7:567. doi: 10.3389/fmicb.2016.00567
Han, J. E., Mohney, L. L., Tang, K. F. J., Pantoja, C. R., and Lightner, D. V. (2015). Plasmid mediated tetracycline resistance of Vibrio parahaemolyticus associated with acute hepatopancreatic necrosis disease (AHPND) in shrimps. Aquac. Rep. 2, 17–21. doi: 10.1016/j.aqrep.2015.04.003
Hayes, C. S., and Low, D. A. (2009). Signals of growth regulation in bacteria. Curr. Opin. Microbiol. 12, 667–673. doi: 10.1016/j.mib.2009.09.006
Hino, M., Zhang, J., Takagi, H., Miyoshi, T., Uchiumi, T., Nakashima, T., et al. (2014). Characterization of putative toxin/antitoxin systems in Vibrio parahaemolyticus. J. Appl. Microbiol. 117, 185–195. doi: 10.1111/jam.12513
Hiyoshi, H., Kodama, T., Saito, K., Gotoh, K., Matsuda, S., Akeda, Y., et al. (2011). VopV, an F-actin-binding type III secretion effector, is required for Vibrio parahaemolyticus-induced enterotoxicity. Cell Host Microbe 10, 401–409. doi: 10.1016/j.chom.2011.08.014
Hiyoshi, H., Okada, R., Matsuda, S., Gotoh, K., Akeda, Y., Iida, T., et al. (2015). Interaction between the type III effector VopO and GEF-H1 activates the RhoA-ROCK pathway. PLoS Pathog. 11:e1004694. doi: 10.1371/journal.ppat.1004694
Hongwei, G., Cai, X., Zhang, X., Luo, J., Zhang, X., Cai, W., et al. (2021). A bacterial “shield and sword”: a previously uncharacterized two-component system protects uropathogenic Escherichia coli from host-derived oxidative insults and promotes hemolysin-mediated host cell pyroptosis. bioRxiv [preprint] 19, 440293. doi: 10.1101/2021.04.19.440293
Hu, Z., and Zhang, W. (2020). Signalling natural products from human pathogenic bacteria. ACS Infect. Dis. 6, 25–33. doi: 10.1021/acsinfecdis.9b00286
Huang, W. S., and Wong, H. C. (2012). Characterization of low salinity stress in Vibrio parahaemolyticus. J. Food Prot. 75, 231–237. doi: 10.4315/0362-028X.JFP-11-321
Igere, B. E., and Ekundayo, T. C. (2020). Global mapping of cholera Vibrio and outbreaks in the pre-millennium development goals (MDG)/sustainable development goals (SDG) and MDGs/SDGs era of 1990-2019. Microb. Pathog. 149:104319. doi: 10.1016/j.micpath.2020.104319
Iguchi, T., Kondo, S., and Hisatsune, K. (1995). Vibrio parahaemolyticus O serotypes from O1 to O13 all produce R-type lipopolysaccharide: SDS-PAGE and compositional sugar analysis. FEMS Microbiol. Lett. 130, 287–292. doi: 10.1111/j.1574-6968.1995.tb07733.x
Jia, J., Chen, Y., Jiang, Y., Tang, J., Yang, L., Liang, C., et al. (2014). Visualized analysis of cellular fatty acid profiles of Vibrio parahaemolyticus strains under cold stress. FEMS Microbiol. Lett. 357, 92–98. doi: 10.1111/1574-6968.12498
Jiang, F., Lei, T., Wang, Z., He, M., Zhang, J., and Wang, J. (2021). A novel gene vp0610 negatively regulates biofilm formation in Vibrio parahaemolyticus. Front. Microbiol. 12:656380. doi: 10.3389/fmicb.2021.656380
Jiang, Y., Chu, Y., Xie, G., Li, F., Wang, L., Huang, J., et al. (2019). Antimicrobial resistance, virulence and genetic relationship of Vibrio parahaemolyticus in seafood from coasts of Bohai Sea and Yellow Sea, China. Int. J. Food Microbiol. 290, 116–124. doi: 10.1016/j.ijfoodmicro.2018.10.005
Kalburge, S. S., Carpenter, M. R., Rozovsky, S., and Boyd, E. F. (2017). Quorum sensing regulators are required for metabolic fitness in Vibrio parahaemolyticus. Infect. Immun. 85:e00930-16. doi: 10.1128/IAI.00930-16
Kalburge, S. S., Whitaker, W. B., and Boyd, E. F. (2014). High-salt preadaptation of Vibrio parahaemolyticus enhances survival in response to lethal environmental stresses. J. Food Prot. 77, 246–253. doi: 10.4315/0362-028X.JFP-13-241
Klein, S., Pipes, S., and Lovell, C. R. (2018). Occurrence and significance of pathogenicity and fitness islands in environmental vibrios. AMB Express 8:177. doi: 10.1186/s13568-018-0704-2
Kodama, T., Rokuda, M., Park, K. S., Cantarelli, V. V., Matsuda, S., Iida, T., et al. (2007). Identification and characterization of VopT, a novel ADP-ribosyltransferase effector protein secreted via the Vibrio parahaemolyticus type III secretion system 2. Cell Microbiol. 9, 2598–2609. doi: 10.1111/j.1462-5822.2007.00980.x
Krachler, A. M., Ham, H., and Orth, K. (2011). Outer membrane adhesion factor multivalent adhesion molecule 7 initiates host cell binding during infection by Gram-negative pathogens. Proc. Natl. Acad. Sci. U.S.A. 108, 11614–11619. doi: 10.1073/pnas.1102360108
Lai, W. B., and Wong, H. C. (2013). Influence of combinations of sublethal stresses on the control of Vibrio parahaemolyticus and its cellular oxidative response. Food Control 33, 186–192. doi: 10.1016/j.foodcont.2013.02.036
Lan, S. F., Huang, C. H., Chang, C. H., Liao, W. C., Lin, I. H., and Jian, W. N. (2009). Characterization of a new plasmid-like prophage in a pandemic Vibrio parahaemolyticus O3:K6 strain. Appl. Environ. Microbiol. 75, 2659–2667. doi: 10.1128/AEM.02483-08
Lee, C. T., Chen, I. T., Yang, Y. T., Ko, T. P., Huang, Y. T., Huang, J. Y., et al. (2015). The opportunistic marine pathogen Vibrio parahaemolyticus becomes virulent by acquiring a plasmid that expresses a deadly toxin. Proc. Natl. Acad. Sci. U.S.A. 112, 10798–10803. doi: 10.1073/pnas.1503129112
Leggett, M. J., Schwarz, J. S., Burke, P. A., McDonnell, G., Denyer, S. P., and Maillard, J. Y. (2015). Mechanism of sporicidal activity for the synergistic combination of peracetic acid and hydrogen peroxide. Appl. Environ. Microbiol. 82, 1035–1039. doi: 10.1128/AEM.03010-15
Lei, T., Jiang, F., He, M., Zhang, J., Zeng, H., and Chen, M. (2020). Prevalence, virulence, antimicrobial resistance, and molecular characterization of fluoroquinolone resistance of Vibrio parahaemolyticus from different types of food samples in China. Int. J. Food Microbiol. 317:108461. doi: 10.1016/j.ijfoodmicro.2019.108461
Letchumanan, V., Chan, K. G., Khan, T. M., Bukhari, S. I., Ab Mutalib, N. S., Goh, B. H., et al. (2017). Bile Sensing: the activation of Vibrio parahaemolyticus virulence. Front. Microbiol. 8:728. doi: 10.3389/fmicb.2017.00728
Letchumanan, V., Yin, W. F., Lee, L. H., and Chan, K. G. (2015). Prevalence and antimicrobial susceptibility of Vibrio parahaemolyticus isolated from retail shrimps in Malaysia. Front. Microbiol. 6:33. doi: 10.3389/fmicb.2015.00033
Li, L., Meng, H., Gu, D., Li, Y., and Jia, M. (2019). Molecular mechanisms of Vibrio parahaemolyticus pathogenesis. Microbiol. Res. 222, 43–51. doi: 10.1016/j.micres.2019.03.003
Li, L., Wong, H. C., Nong, W., Cheung, M. K., Law, P. T., and Kam, K. M. (2014). Comparative genomic analysis of clinical and environmental strains provides insight into the pathogenicity and evolution of Vibrio parahaemolyticus. BMC Genomics 15:1135. doi: 10.1186/1471-2164-15-1135
Li, P., Rivera-Cancel, G., Kinch, L. N., Salomon, D., Tomchick, D. R., Grishin, N. V., et al. (2016). Bile salt receptor complex activates a pathogenic type III secretion system. ELife 5:e15718. doi: 10.7554/eLife.15718
Liu, B., Liu, H., Pan, Y., Xie, J., and Zhao, Y. (2016). Comparison of the effects of environmental parameters on the growth variability of Vibrio parahaemolyticus coupled with strain sources and genotypes analysis. Front. Microbiol. 7:994. doi: 10.3389/fmicb.2016.00994
Liu, M., Yan, M., Liu, L., and Chen, S. (2013). Characterization of a novel zinc transporter znuA acquired by Vibrio parahaemolyticus through horizontal gene transfer. Front. Cell Infect. Microbiol. 3:61. doi: 10.3389/fcimb.2013.00061
Lovell, C. R. (2017). Ecological fitness and virulence features of Vibrio parahaemolyticus in estuarine environments. Appl. Microbiol. Biotechnol. 101, 1781–1794. doi: 10.1007/s00253-017-8096-8099
Lu, R., Tang, H., Qiu, Y., Yang, W., Yang, H., and Zhou, D. (2019). Quorum sensing regulates the transcription of lateral flagellar genes in Vibrio parahaemolyticus. Future Microbiol. 14, 1043–1053. doi: 10.2217/fmb-2019-0048
Luong, P., Kinch, L. N., Brautigam, C. A., Grishin, N. V., Tomchick, D. R., and Orth, K. (2010). Kinetic and structural insights into the mechanism of AMPylation by VopS Fic domain. J. Biol. Chem. 285, 20155–20163.
Mahoney, J. C., Gerding, M. J., Jones, S. H., and Whistler, C. A. (2010). Comparison of the pathogenic potentials of environmental and clinical Vibrio parahaemolyticus strains indicates a role for temperature regulation in virulence. Appl. Environ. Microbiol. 76, 7459–7465. doi: 10.1128/AEM.01450-10
Makino, K., Oshima, K., Kurokawa, K., Yokoyama, K., Uda, T., and Tagomori, K. (2003). Genome sequence of Vibrio parahaemolyticus: a pathogenic mechanism distinct from that of V. cholerae. Lancet 361, 743–749. doi: 10.1016/S0140-6736(03)12659-1
Martinez-Urtaza, J., Powell, A., Jansa, J., Rey, J. L., Montero, O. P., Campello, M. G., et al. (2016). Epidemiological investigation of a foodborne outbreak in Spain associated with U.S. west coast genotypes of Vibrio parahaemolyticus. Springer Plus 5:87. doi: 10.1186/s40064-016-1728-1
Matsuda, S., Hiyoshi, H., Tandhavanant, S., and Kodama, T. (2020). Advances on Vibrio parahaemolyticus research in the postgenomic era. Microbiol. Immunol. 6, 167–181. doi: 10.1111/1348-0421.12767
Matz, C., Nouri, B., McCarter, L., and Martinez-Urtaza, J. (2011). Acquired type III secretion system determines environmental fitness of epidemic Vibrio parahaemolyticus in the interaction with bacterivorous protists. PLoS One 6:e20275. doi: 10.1371/journal.pone.0020275
McDougald, D., Gong, L., Srinivasan, S., Hild, E., Thompson, L., Takayama, K., et al. (2002). Defenses against oxidative stress during starvation in bacteria. Antonie Van Leeuwenhoek 81, 3–13. doi: 10.1023/a:1020540503200
Merrell, D. S., and Camilli, A. (1999). The cadA gene of Vibrio cholerae is induced during infection and plays a role in acid tolerance. Mol. Microbiol. 34, 836–849. doi: 10.1046/j.1365-2958.1999.01650.x
Merritt, M. E., and Donaldson, J. R. (2009). Effect of bile salts on the DNA and membrane integrity of enteric bacteria. J. Med. Microb. 58, 1533–1541. doi: 10.1099/jmm.0.014092-0
Mizan, M. F. R., Bang, H. J., Sadekuzzaman, M., Lee, N., Kim, T. J., and Ha, S. D. (2017). Molecular characteristics, biofilm-forming abilities, and quorum sensing molecules in Vibrio parahaemolyticus strains isolated from marine and clinical environments in Korea. Biofouling 33, 369–378. doi: 10.1080/08927014.2017.1316840
Mizunoe, Y., Wai, S. N., Ishikawa, T., Takade, A., and Yoshida, S. (2000). Resuscitation of viable but nonculturable cells of Vibrio parahaemolyticus induced at low temperature under starvation. FEMS Microbiol. Lett. 186, 115–120.
Nair, G. B., Ramamurthy, T., Bhattacharya, S. K., Dutta, B., Takeda, Y., and Sack, D. A. (2007). Global dissemination of Vibrio parahaemolyticus serotype O3:K6 and its serovariants. Clin. Microbiol. Rev. 20, 39–48. doi: 10.1128/CMR.00025-06
Namgoong, S., Boczkowska, M., Glista, M. J., Winkelman, J. D., Rebowski, G., Kovar, D. R., et al. (2011). Mechanism of actin filament nucleation by Vibrio VopL and implications for tandem W domain nucleation. Nat. Struct. Mol. Biol. 18, 1060–1067.
Nasu, H., Iida, T., Sugahara, T., Yamaichi, Y., Park, K. S., and Yokoyama, K. (2000). A filamentous phage associated with recent pandemic Vibrio parahaemolyticus O3:K6 strains. J. Clin. Microbiol. 38, 2156–2161.
Neely, M. N., and Olson, E. R. (1996). Kinetics of expression of the Escherichia coli cad operon as a function of pH and lysine. J. Bacteriol. 178, 5522–5528. doi: 10.1128/jb.178.18.5522-5528.1996
Nguyen, A. Q., Shimohata, T., Hatayama, S., Tentaku, A., Kido, J., and Bui, T. M. H. (2020). Type III secretion effector VopQ of Vibrio parahaemolyticus modulates central carbon metabolism in epithelial cells. mSphere 5:e00960-19. doi: 10.1128/mSphere.00960-19
Nilsson, W. B., and Turner, J. W. (2016). The thermostable direct hemolysin-related hemolysin (trh) gene of Vibrio parahaemolyticus: sequence variation and implications for detection and function. J. Microbiol. Methods 126, 1–7. doi: 10.1016/j.mimet.2016.04.007
Okada, N., Iida, T., Park, K. S., Goto, N., Yasunaga, T., Hiyoshi, H., et al. (2009). Identification and characterization of a novel type III secretion system in trh-positive Vibrio parahaemolyticus strain TH3996 reveal genetic lineage and diversity of pathogenic machinery beyond the species level. Infect. Immun. 77, 904–913. doi: 10.1128/IAI.01184-08
Okada, R., Zhou, X., Hiyoshi, H., Matsuda, S., Chen, X., Akeda, Y., et al. (2014). The Vibrio parahaemolyticus effector VopC mediates Cdc42-dependent invasion of cultured cells but is not required for pathogenicity in an animal model of infection. Cell Microbiol. 6, 938–947. doi: 10.1111/cmi.12252
Ongagna-Yhombi, S. Y., and Boyd, E. F. (2013). Biosynthesis of the osmoprotectant ectoine, but not glycine betaine, is critical for survival of osmotically stressed Vibrio parahaemolyticus cells. Appl. Environ. Microbiol. 79, 5038–5049. doi: 10.1128/AEM.01008-13
Osawa, R., and Yamai, S. (1996). Production of thermostable direct hemolysin by Vibrio parahaemolyticus enhanced by conjugated bile acids. Appl. Environ. Microbiol. 62, 3023–3025. doi: 10.1128/AEM.62.8.3023-3025.1996
Ottaviani, D., Leoni, F., Serra, R., Serracca, L., Decastelli, L., Rocchegiani, E., et al. (2012). Nontoxigenic Vibrio parahaemolyticus strains causing acute gastroenteritis. J. Clin. Microbiol. 50, 4141–4143. doi: 10.1128/JCM.01993-12
Park, K. S., Arita, M., Iida, T., and Honda, T. (2005). vpaH, a gene encoding a novel histone-like nucleoid structure-like protein that was possibly horizontally acquired, regulates the biogenesis of lateral flagella in trh-positive Vibrio parahaemolyticus TH3996. Infect. Immun. 73, 5754–5761. doi: 10.1128/IAI.73.9.5754-5761.2005
Park, K. S., Lee, S. J., Chung, Y. H., Iida, T., and Honda, T. (2009). Temperature-dependency urease activity in Vibrio parahaemolyticus is related to transcriptional activator UreR. J. Microbiol. Biotechnol. 19, 1456–1463. doi: 10.4014/jmb.0902.0100
Pérez-Reytor, D., Pavón, A., Lopez-Joven, C., Ramírez-Araya, S., Peña-Varas, C., and Plaza, N. (2020). Analysis of the Zonula occludens toxin Found in the genome of the Chilean non-toxigenic Vibrio parahaemolyticus strain PMC53.7. Front. Cell Infect. Microbiol. 10:482. doi: 10.3389/fcimb.2020.00482
Qian, H., Li, W., Guo, L., Tan, L., Liu, H., Wang, J., et al. (2020). Stress response of Vibrio parahaemolyticus and Listeria monocytogenes biofilms to different modified atmospheres. Front. Microbiol. 11:23. doi: 10.3389/fmicb.2020.00023
Raghunath, P. (2015). Roles of thermostable direct hemolysin (TDH) and TDH-related hemolysin (TRH) in Vibrio parahaemolyticus. Front. Microbiol. 5:805. doi: 10.3389/fmicb.2014.00805
Ramamurthy, T., Ghosh, A., Pazhani, G. P., and Shinoda, S. (2014). Current perspectives on viable but non-culturable (VBNC) pathogenic bacteria. Front. Public Health 2:103. doi: 10.3389/fpubh.2014.00103
Ramisetty, B. C., and Santhosh, R. S. (2016). Horizontal gene transfer of chromosomal Type II toxin-antitoxin systems of Escherichia coli. FEMS Microbiol. Lett. 363:fnv238. doi: 10.1093/femsle/fnv238
Rendueles, O., de Sousa, J. A. M., Bernheim, A., Touchon, M., and Rocha, E. P. (2018). Genetic exchanges are more frequent in bacteria encoding capsules. PLoS Genet. 14:e1007862. doi: 10.1371/journal.pgen.1007862
Rivera-Cancel, G., and Orth, K. (2017). Biochemical basis for activation of virulence genes by bile salts in Vibrio parahaemolyticus. Gut Microbes 4, 366–373. doi: 10.1080/19490976.2017.1287655
Salomon, D., Gonzalez, H., Updegraff, B. L., and Orth, K. (2013). Vibrio parahaemolyticus type VI secretion system 1 is activated in marine conditions to target bacteria, and is differentially regulated from system 2. PLoS One 16:e61086. doi: 10.1371/journal.pone.0061086
Segal, G., and Ron, E. Z. (1998). Regulation of heat-shock response in bacteria. Ann. N. Y. Acad. Sci. 851, 147–151. doi: 10.1111/j.1749-6632.1998.tb08988.x
Siliakus, M. F., van der Oost, J., and Kengen, S. W. M. (2017). Adaptations of archaeal and bacterial membranes to variations in temperature, pH and pressure. Extremophiles 21, 651–670. doi: 10.1007/s00792-017-0939-x
Simmons, M., Drescher, K., Nadell, C. D., and Bucci, V. (2018). Phage mobility is a core determinant of phageŰbacteria coexistence in biofilms. ISME J. 12, 531–543. doi: 10.1038/ismej.2017.190
Sistrunk, J. R., Nickerson, K. P., Chanin, R. B., Rasko, D. A., and Faherty, C. S. (2016). Survival of the fittest: how bacterial pathogens utilize bile to enhance infection. Clin. Microbiol. Rev. 29, 819–836. doi: 10.1128/CMR.00031-16
Soksawatmaekhin, W., Kuraishi, A., Sakata, K., Kashiwagi, K., and Igarashi, K. (2004). Excretion and uptake of cadaverine by CadB and its physiological functions in Escherichia coli. Mol. Microbiol. 51, 1401–1412. doi: 10.1046/j.1365-2958.2003.03913.x
Sreelatha, A., Bennett, T. L., Zheng, H., Jiang, Q. X., Orth, K., and Starai, V. J. (2013). Vibrio effector protein, Vop Q, forms a lysosomal gated channel that disrupts host ion homeostasis and autophagic flux. Proc. Natl. Acad. Sci. U.S.A. 110, 11559–11564. doi: 10.1073/pnas.1307032110
Stokes, N. R., Murray, H. D., Subramaniam, C., Gourse, R. L., Louis, P., Bartlett, W., et al. (2003). A role for mechanosensitive channels in survival of stationary phase: regulation of channel expression by RpoS. Proc. Natl. Acad. Sci. U.S.A. 100, 15959–15964. doi: 10.1073/pnas.2536607100
Tan, H. J., Liu, S. H., Oliver, J. D., and Wong, H. C. (2010). Role of RpoS in the susceptibility of low salinity-adapted Vibrio vulnificus to environmental stresses. Int. J. Food Microbiol. 137, 137–142. doi: 10.1016/j.ijfoodmicro.2009.12.006
Tanaka, Y., Kimura, B., Takahashi, H., Watanabe, T., Obata, H., Kai, A., et al. (2008). Lysine decarboxylase of Vibrio parahaemolyticus: kinetics of transcription and role in acid resistance. J. Appl. Microbiol. 104, 1283–1293. doi: 10.1111/j.1365-2672.2007.03652.x
Tandhavanant, S., Matsuda, S., Hiyoshi, H., Iida, T., and Kodama, T. (2018). Vibrio parahaemolyticus senses intracellular K+ to translocate type III secretion system 2 effectors effectively. mBio 9:e01366-18. doi: 10.1128/mBio.01366-18
Tang, J., Jia, J., Chen, Y., Huang, X., Zhang, X., Zhao, L., et al. (2018). Proteomic analysis of Vibrio parahaemolyticus under cold stress. Curr. Microbiol. 75, 20–26. doi: 10.1007/s00284-017-1345-4
Thomas, M. S., and Wigneshweraraj, S. (2014). Regulation of virulence gene expression. Virulence 5, 832–834. doi: 10.1080/21505594.2014.995573
Trevors, J. T., Elsas, J. D., and Bej, A. K. (2012). The molecularly crowded cytoplasm of bacterial cells: dividing cells contrasted with viable but non-culturable (VBNC) bacterial cells. Curr. Issues Mol. Biol. 15, 1–6.
Trimble, M. J., and McCarter, L. L. (2011). Bis-(3’-5’)-cyclic dimeric GMP-linked quorum sensing controls swarming in Vibrio parahaemolyticus. Proc. Natl. Acad. Sci. U.S.A. 108, 18079–18084. doi: 10.1073/pnas.1113790108
Trosky, J. E., Li, Y., Mukherjee, S., Keitany, G., Ball, H., and Orth, K. (2007). VopA inhibits ATP binding by acetylating the catalytic loop of MAPK kinases. J. Biol. Chem. 282, 34299–34305.
Tso, K. M., Ni, B., and Wong, H. C. (2019). Oxidative disinfectants activate different responses in Vibrio parahaemolyticus. J. Food Prot. 82, 1890–1895.
Ueno, T., Fischer, J. T., and Boon, E. M. (2019). Nitric oxide enters quorum sensing via the H-NOX signaling pathway in Vibrio parahaemolyticus. Front. Microbiol. 10:2108. doi: 10.3389/fmicb.2019.02108
Urdaneta, V., and Casadesús, J. (2017). Interactions between bacteria and bile salts in the gastrointestinal and hepatobiliary tracts. Front. Med. 4:163. doi: 10.3389/fmed.2017.00163
Urmersbach, S., Aho, T., Alter, T., Hassan, S. S., Autio, R., and Huehn, S. (2015). Changes in global gene expression of Vibrio parahaemolyticus induced by cold- and heat-stress. BMC Microbiol. 23:229. doi: 10.1186/s12866-015-0565-7
Van Melderen, L., and Saavedra De Bast, M. (2009). Bacterial toxin-antitoxin systems: more than selfish entities? PLoS Genet. 5:e1000437. doi: 10.1371/journal.pgen.1000437
Vasudevan, P., and Venkitanarayanan, K. (2006). Role of the rpoS gene in the survival of Vibrio parahaemolyticus in artificial seawater and fish homogenate. J. Food Prot. 69, 1438–1442. doi: 10.4315/0362-028x-69.6.1438
Vendeville, A., Winzer, K., Heurlier, K., Tang, C. M., and Hardie, K. R. (2005). Making ‘sense’ of metabolism: autoinducer-2, LuxS and pathogenic bacteria. Nat. Rev. Microbiol. 3, 383–396. doi: 10.1038/nrmicro1146
Wang, R. Z., Fang, S., Wu, D. L., Wu, D., Lian, J., Fan, J., et al. (2012). Screening of a ScFv antibody that can neutralize effectively the cytotoxicity of Vibrio parahaemolyticus TLH. Appl. Environ. Microbiol. 78, 4967–4975. doi: 10.1128/AEM.00435-12
Wang, R., Zhong, Y., Gu, X., Yuan, J., Saeed, A. F., and Wang, S. (2015). Corrigendum: the pathogenesis, detection, and prevention of Vibrio parahaemolyticus. Front. Microbiol. 6:437. doi: 10.3389/fmicb.2015.00437
Wendling, C. C., Refardt, D., and Hall, A. R. (2021). Fitness benefits to bacteria of carrying prophages and prophage-encoded antibiotic-resistance genes peak in different environments. Evolution 75, 515–528. doi: 10.1111/evo.14153
Whitaker, W. B., Parent, M. A., Naughton, L. M., Richards, G. P., Blumerman, S. L., and Boyd, E. F. (2010). Modulation of responses of Vibrio parahaemolyticus O3:K6 to pH and temperature stresses by growth at different salt concentrations. Appl. Environ. Microbiol. 76, 4720–4729. doi: 10.1128/AEM.00474-10
Wong, H. C., and Wang, P. (2004). Induction of viable but nonculturable state in Vibrio parahaemolyticus and its susceptibility to environmental stresses. J. Appl. Microbiol. 96, 359–366. doi: 10.1046/j.1365-2672.2004.02166.x
Wong, H. C., Peng, P. Y., Lan, S. L., Chen, Y. C., Lu, K. H., Shen, C. T., et al. (2002). Effects of heat shock on the thermotolerance, protein composition, and toxin production of Vibrio parahaemolyticus. J. Food Prot. 65, 499–507. doi: 10.4315/0362-028x-65.3.499
Wong, H. C., Shen, C. T., Chang, C. N., Lee, Y. S., and Oliver, J. D. (2004). Biochemical and virulence characterization of viable but nonculturable cells of Vibrio parahaemolyticus. J. Food Prot. 67, 2430–2435.
Wong, H. C., Wang, T. Y., Yang, C. W., Tang, C. T., Ying, C., and Wang, C. H. (2019). Characterization of a lytic vibriophage VP06 of Vibrio parahaemolyticus. Res. Microbiol. 170, 13–23. doi: 10.1016/j.resmic.2018.07.003
Wu, K., Zheng, Y., Wu, Q., Chen, H., Fu, S., Kan, B., et al. (2019). Vibrio parahaemolyticus cqsA controls production of quorum sensing signal molecule 3-hydroxyundecan-4-one and regulates colony morphology. J. Microbiol. 57, 1105–1114. doi: 10.1007/s12275-019-9379-x
Yamaguchi, Y., and Inouye, M. (2011). Regulation of growth and death in Escherichia coli by toxin-antitoxin systems. Nat. Rev. Microbiol. 11, 779–790. doi: 10.1038/nrmicro2651
Yang, L., Wang, Y., Yu, P., Ren, S., Zhu, Z., and Jin, Y. (2020). Prophage-related gene VpaChn25_0724 contributes to cell membrane integrity and growth of Vibrio parahaemolyticus CHN25. Front. Cell Infect. Microbiol. 10:595709. doi: 10.3389/fcimb.2020.595709
Yang, L., Zhou, D., Liu, X., Han, H., Zhan, L., Guo, Z., et al. (2009). Cold-induced gene expression profiles of Vibrio parahaemolyticus: a time-course analysis. FEMS Microbiol. Lett. 291, 50–58. doi: 10.1111/j.1574-6968.2008.01434.x
Yarbrough, M. L., Li, Y., Kinch, L. N., Grishin, N. V., Ball, H. L., and Orth, K. (2009). AMPylation of Rho GTPases by Vibrio VopS disrupts effector binding and downstream signaling. Science 323, 269–272.
Yeung, M. P. S., and Boor, K. J. (2004). Epidemiology, pathogenesis, and prevention of foodborne Vibrio parahaemolyticus infections. Foodborne Pathog. Dis. 1, 74–88. doi: 10.1089/153531404323143594
Yoon, J. H., and Lee, S. Y. (2020). Characteristics of viable-but-nonculturable Vibrio parahaemolyticus induced by nutrient-deficiency at cold temperature. Crit. Rev. Food Sci. Nutr. 60, 1302–1320. doi: 10.1080/10408398.2019.1570076
Yoon, J. H., Bae, Y. M., and Lee, S. Y. (2017). Effects of varying concentrations of sodium chloride and acidic conditions on the behavior of Vibrio parahaemolyticus and Vibrio vulnificus cold-starved in artificial sea water microcosms. Food Sci. Biotechnol. 26, 829–839. doi: 10.1007/s10068-017-0105-3
Yu, B., Cheng, H. C., Brautigam, C. A., Tomchick, D. R., and Rosen, M. K. (2011). Mechanism of actin filament nucleation by the bacterial effector VopL. Nat. Struct. Mol. Biol. 18, 1068–1074.
Yu, L. H., The, C. S. J., Yap, K. P., Ung, E. H., and Thong, K. L. (2020). Comparative genomic provides insight into the virulence and genetic diversity of Vibrio parahaemolyticus associated with shrimp acute hepatopancreatic necrosis disease. Infect. Genet. Evol. 83:104347. doi: 10.1016/j.meegid.2020.104347
Yu, S. C., Fen, S. Y., Chien, C. L., and Wong, H. C. (2016). Protective roles of katG-homologous genes against extrinsic peroxides in Vibrio parahaemolyticus. FEMS Microbiol. Lett. 363:fnw038. doi: 10.1093/femsle/fnw038
Yu, Y., Fang, L., Zhang, Y., Sheng, H., and Fang, W. (2015). VgrG2 of type VI secretion system 2 of Vibrio parahaemolyticus induces autophagy in macrophages. Front. Microbiol. 6:168. doi: 10.3389/fmicb.2015.00168
Zabala, B., Hammerl, J. A., Espejo, R. T., and Hertwig, S. (2009). The linear plasmid prophage Vp58.5 of Vibrio parahaemolyticus is closely related to the integrating phage VHML and constitutes a new incompatibility group of telomere phages. J. Virol. 83, 9313–9320. doi: 10.1128/JVI.00672-09
Zhang, J., Ito, H., Hino, M., and Kimura, M. A. (2017). RelE/ParE superfamily toxin in Vibrio parahaemolyticus has DNA nicking endonuclease activity. Biochem. Biophys. Res. Commun. 489, 29–34. doi: 10.1016/j.bbrc.2017.05.105
Zhang, L., Krachler, A. M., Broberg, C. A., Li, Y., Mirzaei, H., Gilpin, C. J., et al. (2012). Type III effector VopC mediates invasion for Vibrio species. Cell Rep. 1, 453–460.
Zhang, X. H., and Austin, B. (2005). Haemolysins in Vibrio species. J. Appl. Microbiol. 98, 1011–1019. doi: 10.1111/j.1365-2672.2005.02583.x
Zhang, Y., Gao, H., Osei-Adjei, G., Zhang, Y., Yang, W., and Yang, H. (2017). Transcriptional regulation of the Type VI secretion system 1 genes by quorum sensing and ToxR in Vibrio parahaemolyticus. Front. Microbiol. 8:2005. doi: 10.3389/fmicb.2017.02005
Zhang, Y., Qiu, Y., Xue, X., Zhang, M., Sun, J., Li, X., et al. (2021). Transcriptional regulation of the virulence genes and the biofilm formation associated operons in Vibrio parahaemolyticus. Gut Pathog. 13:15. doi: 10.1186/s13099-021-00410-y
Zhou, X., Gewurz, B. E., Ritchie, J. M., Takasaki, K., Greenfeld, H., Kieff, E., et al. (2013). A Vibrio parahaemolyticus T3SS effector mediates pathogenesis by independently enabling intestinal colonization and inhibiting TAK1 activation. Cell Rep. 3, 1690–1702.
Zhou, X., Ritchie, J. M., Hiyoshi, H., Iida, T., Davis, B. M., and Waldor, M. K. (2012). The hydrophilic translocator for Vibrio parahaemolyticus, T3SS2, is also translocated. Infect. Immun. 80, 2940–2947. doi: 10.1128/IAI.00402-12
Zhu, C., Sun, B., Liu, T., Zheng, H., Gu, W., He, W., et al. (2017). Genomic and transcriptomic analyses reveal distinct biological functions for cold shock proteins (VpaCspA and VpaCspD) in Vibrio parahaemolyticus CHN25 during low-temperature survival. BMC genomics 18:436. doi: 10.1186/s12864-017-3784-5
Keywords: biofilm, chemotaxis, hemolysin, pathogenicity island, secretion systems, toxin-antitoxin system, Vibrio parahaemoluticus, virulence
Citation: Pazhani GP, Chowdhury G and Ramamurthy T (2021) Adaptations of Vibrio parahaemolyticus to Stress During Environmental Survival, Host Colonization, and Infection. Front. Microbiol. 12:737299. doi: 10.3389/fmicb.2021.737299
Received: 06 July 2021; Accepted: 08 September 2021;
Published: 07 October 2021.
Edited by:
Eckhard Strauch, Bundesanstalt für Risikobewertung (BfR), GermanyReviewed by:
Jessica L. Jones, United States Food and Drug Administration, United StatesCopyright © 2021 Pazhani, Chowdhury and Ramamurthy. This is an open-access article distributed under the terms of the Creative Commons Attribution License (CC BY). The use, distribution or reproduction in other forums is permitted, provided the original author(s) and the copyright owner(s) are credited and that the original publication in this journal is cited, in accordance with accepted academic practice. No use, distribution or reproduction is permitted which does not comply with these terms.
*Correspondence: Gururaja Perumal Pazhani, cGF6aGFuaWdwQGdtYWlsLmNvbQ==
Disclaimer: All claims expressed in this article are solely those of the authors and do not necessarily represent those of their affiliated organizations, or those of the publisher, the editors and the reviewers. Any product that may be evaluated in this article or claim that may be made by its manufacturer is not guaranteed or endorsed by the publisher.
Research integrity at Frontiers
Learn more about the work of our research integrity team to safeguard the quality of each article we publish.