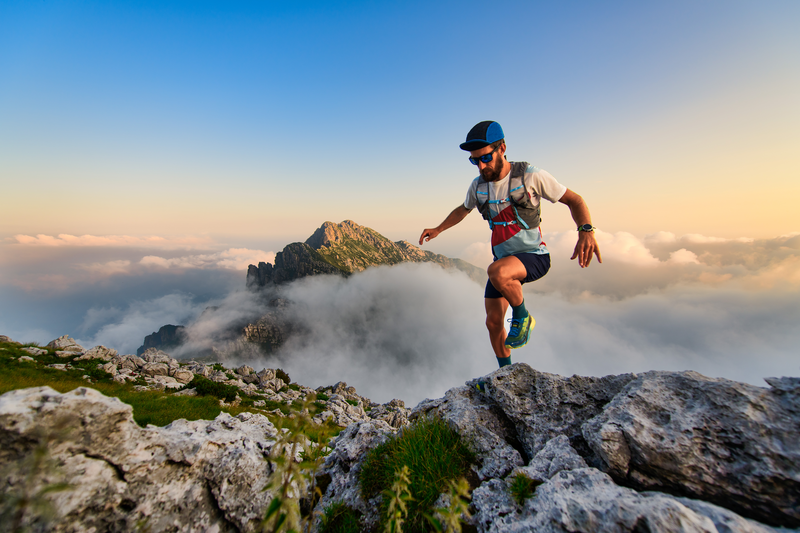
94% of researchers rate our articles as excellent or good
Learn more about the work of our research integrity team to safeguard the quality of each article we publish.
Find out more
ORIGINAL RESEARCH article
Front. Microbiol. , 24 September 2021
Sec. Microbiotechnology
Volume 12 - 2021 | https://doi.org/10.3389/fmicb.2021.735859
With the development of large-scale and intensive poultry farming, environmental disinfection has become particularly important, and the effectiveness of disinfection depends upon the performance of the disinfectants. Quaternate ammonium salt is a group of positively charged polyatomic ions with both antibacterial and antiviral activities. In order to prepare an ideal disinfectant for poultry farms, we combined a quaternate ammonium salt N-dodecyl-2-(piridin-1-ium)acetamide chloride with two other disinfectants (chlorhexidine acetate and glutaraldehyde), respectively. The antimicrobial activity, mutagenicity, and safety of the compound disinfectants were assessed by the European Standard methods using ATCC strains and clinical isolates. The results showed that both compound disinfectants meet the requirements of microbial reduction, and their effectiveness was not affected by organic matter. Quaternary ammonium disinfectant resistance genes were not detected in the strains tested indicating that bacteria are less likely to develop resistance to these compound disinfectants. Ames test showed that there was no detectable mutagenicity in the strains treated with the compound disinfectants. In vivo experiment showed that both compound disinfectants did not have significant pathological effect in mice. The bactericidal effect of the compound disinfectants was not significantly different among strains of different sources (p>0.05). Clinical tests showed that compound disinfectant had a good bactericidal effect on the air and ground of poultry farms. These results show that quaternary ammonium salts in combination with other compounds can enhance the bactericidal effect and can be used safely in poultry feedlots. This study provides a technical reference for the development of a new quaternate ammonium compound disinfectant with strong disinfection effect and low irritation.
Microbial infections are one of the most harmful diseases to the poultry industry (Ho et al., 2010; Medzhitov et al., 2012). They not only cause an increase in mortality and the economic loss of poultry products, but also pose a serious threat to human health. Disinfectants play an important role in reducing the occurrence of infectious diseases that affect the development of the poultry industry. At present, disinfectants commonly used in poultry farms include peroxyacetic acid, sodium hydroxide, povidone-iodine, and quick limes. However, these disinfectants are prone to produce resistance and drug residues after long-term use, and some of them also have the problems of causing irritation and corrosion. Therefore, it is important to develop new broad-spectrum disinfectants.
Quaternary ammonium salt disinfectant plays a vital role in the prevention of animal diseases (Ioannou and Hanlon, 2007; Murguía et al., 2008; Giardino et al., 2016; Zhang et al., 2016; Song et al., 2018). Previous studies have shown that the combination of a quaternary ammonium salt with chlorhexidine gluconate produces a synergistic effect. Chlorhexidine gluconate is one of the most widely used antimicrobial agents because it has broad-spectrum antibacterial properties and is compatible with many kinds of materials (Sandle, 2019). It is often used as a compound or monomer disinfectant (Hidalgo and Dominguez, 2001; Bhende and Spangler, 2004; Chapman et al., 2012). Cationic surfactants also produce synergistic fungicidal effects by combining with nitrogen moiety, pyridine, or quacking (Brandes et al., 1993). Studies have shown that quaternate ammonium salts are vulnerable to organic matter and usually fail when they are used alone. Therefore, the combination of 25% glutaraldehyde, 25% double-chain quaternate ammonium salt, and ethanol not only enhanced the stability but also expanded the bactericidal spectrum (Martin, 1994). Combination of 0.1% dimethyl ammonium chloride, 0.1% methylammonium bromide, and 0.2% isopropanol can kill Salmonella ATCC 10708 and Staphylococcus aureus ATCC 6538 within 20min (Wachman and Karlan, 1990). These studies demonstrated that compound of quaternate ammonium surfactant had broad development and application prospects. In this study, we combined a quaternate ammonium surfactant with other disinfectants and determined their bactericidal activity and clinical applications. Our results indicated that this new compound disinfectant has high bactericidal effect and could be used safely in poultry feedlots.
Experimental animals include 40 SPF Kunming mice (18–22g). Animals were purchased from the Harbin Medical University. A total of 30 chicks of Hy-line variety brown at 1day of age were provided by the Harbin Yinong Poultry Industry Co. The clinical trial was completed in mid-January at a poultry farm in Longjiang County, Qiqihar, where sampling was completed. Quaternary ammonium salt cationic surfactant was synthesized by Professor Lihua Jia of Qiqihar University (Table 1).
ATCC strains and isolates from poultry farm were stored at the Heilongjiang Provincial Engineering Technology Research Center for Prevention and Control of Cattle Diseases (Table 2).
Salmonella typhimurium histidine nutrient-deficient (his−) strains TA97, TA98, TA100, TA1535, and TA1537 were obtained from the Japanese (strain) Bioscience Center (JBSINC). The strains were isolated and screened for the presence of amino acid and biotin synthesis defects, cell wall lipopolysaccharide deficiency (rfa), UVR (uvrA or ΔuvrB) repair defects, and resistance to ampicillin and tetracycline (containing pKM101 or pAQ1 plasmid). The strains meeting the requirement were used in this study. The positive control reagents (2-2-Furyl-3-5-Nitro-2-Furylacrylamide, AF-2 and 2-Aminoanthracene, 2-AA) were purchased from Sigma. Sodium azide (NaN3) was purchased from Merck. 9-aminoacridine and 9-AA were purchased from Acros Organics. CM0067 nutrient broth No.2 was purchased from OXOID. Agar powder, ampicillin, glucose, histidine, tryptophan, biotin, MgSO4·(7H2O), sodium citrate·(2H2O), K2HPO4·(3H2O), KH2PO4, (NH4)2SO4, NADP, G-6-P, KCl, and MgCl2 were purchased from Sigma. Rat liver S9 mixture (made from the combination of sodium phenobarbital and β-naphthoflavone-induced rat liver) was purchased from the Beijing Kangruijie Technology Co. Ethanol (Cat. No. 200802, Quanrui Reagent Co.), chlorhexidine acetate (Cat. NO. 190104, Jiu Tai Reagent Company), Glutaraldehyde (Cat. NO. 190417 Tianjin Hongyan Reagent Factory), and Bovine serum albumin (BSA; Cat. NO. EZ2921B398, BIOFROX) were used in this study.
The pure 1-(alkylcarbamoylmethyl) pyridinium chloride was described as CncmpCl, where n (= 12, 14, 16, respectively) represents the carbon number of alkyl. In this study, C12cmpCl, C14cmpCl, and C16cmpCl were used (Table 1). The FT-IR (BKr), 1HNMR, and ESI–MS (m/z) data of the C12cmpCl were shown below. The compound was obtained in 91% yield with M.p of 118.8–119.5°C. FT-IR spectrum (KBr) νmax: 3399.32, 3207.26, 3056.07, 2953.92, 2917.14, 2851.76, 1666.74, 1638.14, 1568.67, 1491.03, 1466.52, 788.20, 726.90, 706.47, and 677.87cm−1. 1H–NMR (CDCl3, 400MHz): δ 0.88 (t, J =6.8Hz, 3 H, CH3), 1.28 (m, 18 H, CH3–(CH2)9), 1.58 (m, 2 H, CH2–CH2–NH), 3.22 (m, 2 H, CH2–NH), 5.98 (s, 2 H, CO–CH2), 8.04 (t, J =7.4Hz, 2 H), 8.46 (t, J =7.8Hz, 1 H,), 9.44 (d, J =5.2Hz, 2 H), and 9.50 (t, J =2.4Hz, 1 H, NH–CO) ppm. MS (ESI) m/z was [M–Cl−]+ Calcd: 305.3, Found 305.3 (Feng et al., 2015).
Chlorhexidine acetate was dissolved in alcohol and mixed with C12cmpCl at a ratio of 2:1 (ethanol 10%). This quaternary ammonium salt composition was designated as compound disinfectant-1 (hereinafter referred to as C1). The second compound was composed of glutaraldehyde and C12cmpCl at a ratio of 2:1 (hereinafter referred to as C2). The final concentration of C12cmpCl is 0.7 (g/l) in C1 and C2. The final concentration of chlorhexidine and glutaraldehyde is 1.4 (g/l) in C1 and C2, respectively. Disinfectant dilutions were prepared in water of standardized hardness (WSH) immediately before testing (VAH, 2015).
Neutralization test was conducted according to phase 1 tests (EN 1040 and EN 1275) and then according to phase 2, step 1 tests (Draft EN 13727 and EN 13624; EN 13624, 2003; EN 1040, 2005; EN 1275, 2005; CEN, 2015; EN 13727, 2015). PBS containing 0.5% w/v glycine, 0.5% w/v lecithin, and 1% v/v Tween 80 was used as neutralizer in the tests.
Minimum inhibition concentrations of disinfectants were determined by the microdilution method as described by NCCLS, VAH method 7 and EN 14885 (Cockerill, 2000; EN 14885, 2015; VAH, 2015). C12cmpCl were used as the monomer active substances for bactericidal activity tests. Tests were performed using 96-well cell culture plates. Dilute the compound disinfectant stock solution to different concentrations. The concentrations of the compound disinfectants ranged from 0.1 to 1,000mg/l. In the experimental group, 80μl nutrient broth medium, 10μl compound disinfectant, and 10μl bacterial suspension were added to each well. For the blank control, only nutrient broth medium was added. For the positive control, microorganisms and nutrient broth medium were added. The monomer compound disinfectant was added and used as the monomer control. Subsequently, the culture was incubated at 37°C for 24h.
The minimum bactericidal concentration (MBC) test was measured according to the Deutsche Gesellschaft for Hygiene, Microbiologic (DGHH) method and EN 14885 (Begec et al., 2013; EN 14885, 2015; VAH, 2015), and 100μl was added to nutrient agar solid medium and cultured in 37°C incubator for 24h. MBC was judged by the presence of colonies. This measurement was performed twice. Bacterial test in suspensions contained 1.0–5.0×108 colony-forming units (CFU)/ml, except for the practical tests on steel carriers which contained 1.0–5.0×109CFU/ml.
With reference to VAH method 8 and EN 14885 (EN 14885, 2015; VAH, 2015), the compound disinfectants were dissolved in hard water at different dilutions. The test disinfectant solution and the test bacteria were mixed together in suspension and incubated in a 20°C water bath at a constant temperature. Under this condition, four time points (1, 5, 15, and 30min) were selected to determine the killing of the indicator bacteria. Test bacteria suspension (0.1ml) and 10ml of disinfectants were added into a sterile test tube, and mix immediately for four time points. Subsequently, 0.1ml of sample solution was added to a test tube containing 10ml of neutralizer and mixed for 10min. Then, 0.1ml was added to a 10ml nutrient broth tube. After incubating at 37°C for 24h, bacterial growth was evaluated visually. Quantitative test in suspension was performed as described above. After 10min of neutralization, culture was incubated on nutrient medium at 37°C for 24h to enumerate the viable bacteria. Each experiment was repeated for three times.
In order to further evaluate the bactericidal activity of the disinfectants, we used Vah Method 9, EN13727, and EN 14885 (EN 13727, 2005; EN 14885, 2015; VAH, 2015). The organic substance protection test was carried out at 20°C. Bovine serum albumin was selected as an organic substance (3g/l BSA). Among the recommended action time, 1, 5, 15, and 30min were selected. Briefly, the disinfectant solution (8ml) was mixed with BSA (1ml), and 1ml of bacterial suspension was then added. After mixing, it was allowed to grow for four different time points, and then, 0.5ml was transferred to 4.5ml of neutralizer and mixed for 10min. Subsequently, 100μl was spread on nutrient agar medium and incubated at 37°C for 48h for colony enumeration.
Biocide efficacy under practical conditions with organic load (i.e., surface disinfection without mechanical action; VAH method 14.1 and BS EN 13697, 2019) was evaluated using stainless steel carriers (VAH, 2015). A 50-μl drop of bacterial suspension was dried (60min at 37°C) on stainless steel carriers (1cm diameter) and was covered with 100μl of disinfectant for the exposure times suggested by VAH guidelines (i.e., 1, 5, 15, or 30min at 20°C). Subsequently, carriers were transferred to 10ml of neutralizer. Finally, biocide efficacy was determined by colony enumeration.
In order to determine the potential resistance of bacteria to disinfectants, five pairs of primers specific for disinfectant resistance-related genes were designed. Qac E∆1, qac A/B, qac C, qac G, and qac J are all quaternary ammonium disinfectant resistance genes (Rajamohan and Srinivasan, 2010; Table 3). PCR was performed to determine the presence of these genes in bacteria. PCR was performed with the follow conditions: initial denaturation at 93°C for 2min, followed by 35cycles of 93°C for 30s, 56°C for 30s, extension at 72°C for 1min, and a final extension at 72°C for 5min (for amplification of qacEΔ1); initial denaturation at 93°C for 2min, followed by 35cycles of 93°C for 30s, 56°C for 30s, extension at 72°C for 1min, and a final extension at 72°C for 5min (for amplification of qacA/B and qacC:); initial denaturation at 93°C for 2min, followed by 35cycles of 93°C for 30s, 48°C for 30s, extension at 72°C for 1min, and a final extension was performed at 72°C for 5min (for amplification of qacG and qacJ).
The Ames test was conducted in accordance with OECD Guideline 471 (2020) and Díez-Quijada et al. (2019). S. typhimurium histidine nutrient-deficient (his−) strains TA97, TA98, TA100, TA1535, and TA1537 were used as the indicator bacteria in the test. The compound disinfectant was first determined not to inhibit S. typhimurium at the highest concentration before subsequent experiments were performed. Briefly, the bacterial freeze–thaw solution was mixed with nutrient broth and then incubated in a water bath shaker at 37°C and 120r/min for 10h. After amplification, the optical density was measured and the concentration of viable bacteria was estimated to be 1×109/ml for subsequence test. Positive controls included 2-(2-furyl)-3-(5-nitro-2-furyl)acrylamide (AF-2), 2-Aminoanthracene (2-AA), sodium azide, NaN3, 9-aminoacridine (9-AA). Negative control with addition of solvent only was also included. Five concentrations were established according to subject inhibition, and Ames tests were carried out using 6-well plates in the presence/absence of S9 metabolic activation. All samples were incubated at 37°C for approximately 48h, and then, the colonies were enumerated. The experiment was repeated twice. The number of responding colonies in each well was enumerated, and the mean value was obtained separately for each concentration group and expressed as mean standard deviation (±). The average number of revertant colonies per dish/well in the TA1535 and TA1537 test groups was three times higher than the control group, while the average number of revertant colonies per dish/well in the other strains was 2 times higher than the solvent control group and a quantitative relationship was found to be positive; otherwise, the result was considered negative.
The acute oral toxicity test was conducted according to the OECD (OECD, 2008; Alluri et al., 2019). Food was given for 3.5h after administration using the one-time maximum limit method. The dose was 5,000mg/kg body weight, and the dose was administered by oral gavage at 20ml/kg/BW. Poisoning performance was recorded. Weight of test animals, and autopsy of dead and expired animals were performed. Tissue or organ abnormalities were analyzed by histopathological examination.
Immunohistochemistry test was performed as described previously (Xue et al., 2018). Animals were divided into high-dose group, middle-dose group, low-dose group, and control group. Gastric perfusion was performed once a day; the duration of the test was 7, 14, 30 days, and 24h after the last exposure. Euthanasia was performed with cervical dislocation under deep anesthesia. Tissue specimens were fixed in 10% formalin buffer, dehydrated, removed, and paraffin-embedded. Routine histological staining of 5-μm sections was performed with hematoxylin and eosin (H and E).
The test started with determining the LD50 in the chicks. Acute oral toxicity test for chicks was conducted according to OECD method (OECD 205, 1984; OECD 423, 2001; OECD 223, 2016). The compounds were administered by oral gavage at the dose of 2000mg (in 20ml)/kg body weight. Clinical signs and mortality of animals were recorded after oral administration, and LD50 was calculated. Based on LD50, DNA damage test was performed in chicks. One-day-old chicks were fed regularly for 1week. Chicks were randomly divided into five groups (six chicks in each group according to body weight, with a 50/50 split between males and females): group of combined disinfectant (C1/C2), highest-dose group (2000mg/kg), monomeric compound groups (C12cmpCl), negative control group (saline), and positive control group (Ethyl methanesulfonate). The animals were maintained at a constant temperature of 22°C (±3°C) and humidity of approximately 70% throughout the study. After 1week of acclimatization, animals were inoculated by a single gavage at the dose of 2000mg (in 20ml)/kg body weight after 2h of fasting. Animals were monitored for clinical signs and mortality every 2h after administration. If no signs of poisoning or mortality occur after 7days, the experiment was extended to 14days before dissection. Cells were extracted from liver and spleen and thymus tissues, respectively, and lymphocyte DNA damage was determined by single-cell gel electrophoresis (SCGE). The SCGE assay was performed according to the OECD method (OECD TG489, 2014) and Kassie et al., 2002. DNA damage was determined by measuring the length of the comet tail of the cells with a fluorescent microscope.
In order to evaluate the antibacterial stability of the compound disinfectant, we selected Escherichia coli, Salmonella enteritidis, S. aureus, Streptococcus pneumoniae, Pasteurella multocida, and Bacillus subtilise from different sources for evaluation. Based on the results of the carrier quantification test, the logarithmic values of the pathogenic bacteria killed by the compound disinfectants were recorded in different time points (5, 10, 15, and 20min) by selecting the appropriate concentrations. Each group selects 10 strains from diverse sources for MBC test. Multiple comparative analyses were performed using SPSS 19.0 software.
Air disinfection test was performed as described previously (VAH, 2015). Windows and ventilation holes were cleaned and closed before disinfection. The two kinds of compound disinfectants were selected from the range of 3.90–500mg/l according to the results from previous experiments. Disinfectants were sprayed into the air in the house, and the flat-plate landing method was used to collect samples before and 10, 20, 40, and 60min after disinfection. Samples were placed into an incubator for 24–48h, and colonies were enumerated.
First, the disinfectant was diluted with WSH at different concentrations. In the poultry house, three areas of l×lm2 on the ground were selected, and the dirt on the ground was removed. Subsequently, diluted disinfectants with different concentrations were used for disinfection. During disinfection, we selected four areas along a diagonal line (each with an area of 10×10cm2) and collected samples before disinfection and 10, 20, 40, and 60min after disinfection. Sterilized cotton swabs were dipped in the collected samples. The neutralizer was taken and rubbed it on the collected area before being placed into a sterile test tube (5ml/tube) containing the neutralizer. The collected samples were brought back to the laboratory, the bacteria on the cotton swab were fully eluted, and then, the bacterial suspension was diluted 10 times with sterile normal saline. Finally, the samples were incubated in a 37°C incubator for 12–24h for colony enumeration.
PBS containing 0.5% w/v glycine, 0.5% w/v lecithin, and 1% v/v Tween 80 was used as neutralizer in the tests. Contact time was 10min at 20°C for the disinfectant-neutralizer mixture. A control group containing sterile distilled water was used to verify that the neutralizing agent had no bactericidal effect. The control group showed that the neutralizing agent had no antibacterial activity and the neutralizing agent and neutralization product had no effect on the growth of the indicator bacteria.
A series of surfactants were then screened, and the surfactant with the best bacterial inhibition effect was selected for compounding studies based on the minimum inhibition concentration (MIC) test results. Bactericidal activity of the compound disinfectant was determined with the monastic compound as a control. Among the series of cationic surfactants, C12cmpCl exhibited the best antibacterial and antifungal effect (p <0.001). C12cmpCl showed the same inhibitory activity against S. aureus, E. hirae, E. coli, and B. subtilis, with a minimum inhibitory concentration of 31.25mg/l for all of these organisms. The inhibition criteria for Candida albicans and Aspergillus brasiliensis were met when the concentration was increased to 62.50mg/l. The inhibitory concentration against Pseudomonas aeruginosa and Proteus vulgaris was 125mg/l (Table 4). Both C14cmpCl and C16cmpCl did not exhibit as good inhibitory activity as C12cmpCl. Based on these results, we selected C12cmpCl for the subsequent studies.
In the time kill assay, the antibacterial and antifungal effect of the compound disinfectant was significantly higher than that of the monomer component (p <0.001). The bactericidal effect of the compound disinfectant C1 was superior to that of C2, with a MBC of only 3.90mg/l against S. aureus within a 1min action time. The MBC of C2 against S. aureus was approximately four times higher than that of C1. The compound disinfectant C1 showed the same bactericidal and fungicidal activity against E. hirae, E. coli, C. albicans, A. brasiliensis, and B. subtilis. Its bactericidal activity against P. vulgaris and P. aeruginosa was slightly lower with the bactericidal concentration of 15.62mg/l and 31.25mg/l, respectively. The MBC of C2 against P. vulgaris and P. aeruginosa was 62.50mg/l (Table 5). These results showed that MIC and MBC of C1 were lower than those of C2, indicating that antibacterial (bacteriostatic and bactericidal) effect of C1 is better than that of C2. Furthermore, C1 had a more significant bacteriostatic and bactericidal effect on Gram-positive bacteria than that on Gram-negative bacteria.
Table 5. Bactericidal concentrations resulting in a ≥5 logarithmic reduction in the quantitative suspension test.
In order to demonstrate that the bactericidal activity of the compound disinfectant is not affected by organic matter, an organic matter protection test was carried out. The compound disinfectant C1 increased the concentration of C. albicans and B. subtilis by 2-fold within 1min of action time. In the monomeric compound groups, the concentration of S. aureus, E. hirae, E. coli, C. albicans, and B. subtilis was also increased by 2-fold within 1min of action time, while there was no change in the other groups. These results indicated that the compound disinfectant was not affected by organic matter (Table 6).
Before conducting clinical trials in the field, we first established that the germicidal effect of the compound disinfectant on the carrier is the same or similar to the time-based germicidal test (time kill assay) before conducting clinical tests. As the compound is mainly used for air and ground disinfection in poultry farms, carrier tests were carried out on stainless steel sheets in accordance with the relevant standards. The results showed that the bactericidal activity of the compound disinfectants to B. subtilis and A. brasiliensis at 1min was slightly reduced compared to the time kill assay. This may be due to the fact that molds and bacillus are difficult to be killed, and the various environmental factors during the carrier test affect their bactericidal effect. However, the bactericidal effect increased significantly with time, indicating that contact time has an effect on the bactericidal activity. The monomer disinfectant control group was not as sensitive as the compound disinfectants group (Table 7). These results indicated that the carrier test was similar to the results of the time kill assay. Therefore, subsequent animal experiment was performed to determine its clinical bactericidal effect.
Table 7. Bactericidal concentrations resulting in a≥5 logarithmic reduction in the carrier quantitative suspension test.
Disinfectants have been used to kill harmful microorganisms in the environment since their discovery, but misuse of disinfectants can cause microbial tolerance to disinfectants and reduction in the disinfectant killing effectiveness. We designed five pairs of quaternary-specific primers for disinfectant resistance-related genes and analyzed the presence of these genes in pathogenic bacteria to understand their resistance status. The results showed that none of the five quaternary ammonium resistance-associated genes (qac E∆1, qac A/B, qac C, qac G, and qac J) were present in the test strains, indicating that the test strains were less likely to develop resistance to the quaternary ammonium.
S. typhimurium histidine nutrient-deficient (his−) strains TA97, TA98, TA100, TA1535, and TA1537 were selected for Ames test using 6-well plates in the presence/absence of S9 metabolic activation, respectively. The number of responding colonies in each well was counted, and the mean value was obtained for each concentration group. The results showed that under non-metabolic activation condition, the number of colonies in the C1 (25ng/l and 50ng/l) group was 2-fold higher than that in the solvent control group for TA98 strain, but not for TA1535, TA97, TA1535, and TA1537. Similar results were also observed for C2 at the concentrations of 6.25 and 25ng/l, but not at the concentration of 50ng/l. Under metabolic activation conditions, the number of colonies in the C1 group at the concentration of 3.125–25ng/l (but not 50ng/l) was 2-fold higher than that in the solvent control group for TA98 strain. Similar results were also observed for C2 at the concentrations of 3.125–12.5ng/l, but not at the concentration of 25ng/l. Although the number of colonies for the combined disinfectants at all concentrations was 2-fold higher than that in the solvent control group for TA98 and TA97 strains, they did not reach 3-fold higher for TA1537 and TA1535 strains. Importantly, the number of responding colonies was not dose-dependent, indicating a negative result for Ames test for the disinfectants used in this study (Tables 8 and 9).
Table 8. Results of 48h bacterial reversion mutation under non-metabolic activation conditions (mean±SD, n =3).
Table 9. Results of 48h bacterial reversion mutagenesis under metabolic activation conditions (+ rat S9; mean±SD, n =3).
Acute oral toxicity tests were carried out with reference to European standards. Two compound disinfectants had LD50 >5,000mg/kg in mice and LD50 >2000mg/kg in chicks. Pathological tissue findings showed that there were no abnormal changes within the tissues of brain, liver, heart, lung, and kidney in the experimental group comparing to the control group, indicating that the compound disinfectants belong to the actual non-toxic grade (Figure 1).
Figure 1. Results of pathological tissue section: Hematoxylin staining. C1, Compound disinfectant 1; C2, Compound disinfectant 2. Bar=20μm.
After the oral toxicity test, the chicks were dissected and the liver, spleen, and thymus were collected to determine the DNA damage by comet electrophoresis test. The stained DNA samples were observed under a fluorescence microscope, and the undamaged cells showed a round fluorescent core, i.e., a comet head, without a tail. Damaged cells, on the other hand, have comet tails reaching toward the anode and forming a bright head and tail. Photographs were taken with a fluorescent inverted microscope, and at least 100 cells in each sample were randomly selected for determination. The cells were characterized according to the ratio of the amount of DNA in the trailing tail to the total DNA in the cells. The degree of DNA damage was classified into five levels: level 0 (no damage, normal cells, <5%); level 1 (mild damage, 5–20%); level 2 (moderate damage, 20–40%); level 3 (high damage, 40–95%); and level 4 (severe damage, >95%). The results showed that most of the cells in the positive control group were lysed and had typical trailing phenomenon. In contrast, in the negative control group and disinfectant groups, most of the cells had clear nuclei without trailing phenomenon (Figure 2).
Figure 2. Results of in vivo single-cell gel electrophoresis assay. C1, Compound disinfectant 1; C2, Compound disinfectant 2; M, N-dodecyl-2-(piridin-1-ium)acetamide chloride (C12cmpCl); NC, Negative control; and PC, Positive control. The arrow indicates the comet’s trailing tail.
To demonstrate the bactericidal ability, we further tested the combined disinfectants on 10 clinical isolates. The results show that there was no significant difference of bacteriostasis effect among groups of strains from different sources (p>0.05). Two compound disinfectants also had strong effects on Gram-positive bacteria, and they immediately killed most pathogenic bacteria within 10min of action. These results indicate that the compound disinfectants have a stable antibacterial effect on strains from different sources and provide the basis for further clinical application (not shown in the text).
We examined the disinfection of the air and floor of the chicken farm after 10, 20, 40, and 60min of treatment. The results showed that when the action time of the compound disinfectants reached 10min, a good disinfection effect can be achieved. As the action time was extended, the disinfection effect was gradually increased. When the action time was 40min, C1 had a better disinfection effect on air and ground than C2 (p <0.05). When the action time was extended to 60min, the difference between C1 and C2 increased (p <0.01). This indicates that with the increase of time, C1 has a better disinfection effect on the air and can be used for the disinfection of air in chicken farms, while C2 can be used for disinfection of ground in chicken farms. The difference between C1 and C2 was increased with time (Figure 3).
Figure 3. The disinfection effect of Compound disinfectant. Clinical trials were divided into disinfection group (C1, C2) and control group. Four different action times were selected to determine the best action time and the clinical bactericidal effect of the disinfectant according to the logarithmic value of the microbial reduction between each group. (A): Air disinfection; (B): Ground disinfection; ***p< 0.001; **p< 0.01; *p< 0.05, NS: Insignificant difference, n=3. Data were presented as mean±SD; C1, Compound disinfectant 1; and C2, Compound disinfectant 2.
Furthermore, an increase in temperature promotes the activity of the compound disinfectant. During the clinical trials in the field, the results were greatly influenced by the cold weather and the incomplete removal of dirt from the chicken coop. Under laboratory conditions, the bactericidal effect was stable at a fixed temperature, and the bactericidal effect increased as the temperature increased.
With our increasing demand for poultry products, the poultry industry has expanded rapidly. However, due to environmental factors, e.g., excessive feeding density, excessive humidity, and unreasonable light, it is easy to cause serious respiratory diseases, affect weight gain and feed utilization, reduced egg production rate, carcass quality degradation, and even resulted in a considerable number of deaths, which decreases the economic benefits of poultry farming. The environmental conditions of the poultry house have a significant impact on the respiratory mucosa of poultry. A good environment condition can facilitate the healthy growth of chickens and reduce the occurrence of respiratory diseases. Disinfectant can kill pathogenic microorganisms and provide poultry a good growing environment. Therefore, choosing the right disinfectant is the key factor for the success of disinfection. Quaternate ammonium salt is a cationic surfactant that has good bactericidal properties with mild and non-irritating advantages (Faria et al., 2018). If the quaternate ammonium disinfectant is prepared with 70% alcohol, the penetration effect can be significantly increased. For this reason, this study synthesized a quaternate ammonium salt and formulated a new type of broad-spectrum disinfectant based on the combination of this quaternate ammonium salt with other disinfectants in order to overcome the disadvantages, e.g., drug resistance, drug residues, and irritation. Respiratory diseases in poultry are mainly caused by Streptococcus, Staphylococcus, E. coli, and Pasteurella. We evaluated the antibacterial and antifungal activity of two kinds of quaternary ammonium compound disinfectants against these pathogens. The results showed that the compound disinfectants had stronger bactericidal effect compared to previous studies (Kuda et al., 2008). The two compound disinfectants have good antibacterial and bactericidal effects on Streptococcus and Staphylococcus. In addition, the compound disinfectant has a bactericidal effect not only on common microorganisms, but also on P. aeruginosa, B. subtilis, C. albicans, and fungi. The shortest bactericidal effect time of the two compound disinfectants was 1min, and the bactericidal efficacy was more than 99.90% against the indicator bacteria. It should also be noted that the same farm should not use the same disinfectant for a long time. The incidence of drug resistance can be greatly reduced when a different type of disinfectant was used. The quaternary ammonium salt surfactant used in this study has a new structure and has not been described so far. Thus, this compound belongs to a new type of disinfectant, which could potentially reduce the risk of resistance compared to the disinfectants that are commercially available. To further demonstrate that the compound does not induce resistance and mutagenicity, we analyzed the resistance genes and performed bacterial reversion mutation tests using standard strains TA97, TA98, TA100, TA1535, and TA1537. The results showed that the two compound disinfectants were not mutagenic in the experimental dose range. Moreover, five genes associated with quaternary ammonium salt resistance were not observed in the test strains, indicating that resistance is less likely to be induced by the compound disinfectants.
In this study, an acute oral toxicity test was conducted on mice to determine its safety under laboratory conditions. The results showed the compound disinfectants had no observable signs of toxicity in mice. At the same time, we conducted acute oral toxicity tests on chicks and DNA damage tests on chicks, and the results showed that both compound disinfectants were not toxic to chicks, and there was no DNA damage. Moreover, the compound disinfectants were effective against strains of different sources, indicating that they can be potentially applied for the disinfection of poultry farms. In clinical application, Begec et al. (2013) studied the antibacterial effect of ketamine combined with propofol in vitro. Kuda et al. (2008) tested the bactericidal effect of benzalkonium chloride on the surface of an object. In the carrier assay, Kuda et al. chose stainless steel as the carrier to measure the number of colonies falling on the stainless-steel carrier attacked by E. coli and Staphylococcus. In this study, the maximum concentrations of the two compound disinfectants to achieve bactericidal effect on E. coli, P. aeruginosa, S. aureus, B. subtilis, C. albicans, P. vulgaris, E. hirae, and A. brasiliensis on the surface of stainless-steel carriers (1cm) were lower than the concentration of other disinfectants as reported previously (Kuda et al., 2008), indicating that the compound disinfectants were more effective. In clinical applications, we applied these two compound disinfectants to the air and ground of the poultry farm. The bactericidal effect increases with the increase of the action time, indicating a promising prospect of its future application in poultry farms.
The ideal disinfectant should have a good bactericidal effect, good stability and low irritation, and are cheap, convenient, and safe for humans and animals (Burgess et al., 2017; West et al., 2018). However, disinfectant products that are currently available cannot meet this standard. Therefore, in this study, we synthesized a new type of disinfectant. The disinfectant is composed of a new surfactant C12cmpCl that were used as the monomer active substances for bactericidal activity tests and chlorhexidine acetate or glutaraldehyde at a certain ratio. This compounding method can not only significantly enhance the bactericidal capacity of chlorhexidine acetate and glutaraldehyde but also reduce the concentration of cationic surfactant C12cmpCl. In addition, the new disinfectant is less likely to develop resistance and is not mutagenic. The new disinfectant is non-toxic and non-irritating, and has a good bactericidal effect on different types of bacteria from different sources. Although glutaraldehyde itself has certain irritation or toxic effect, such effect is greatly reduced after it is combined with quaternary ammonium salt. The compound disinfectant (C2) can be applied for the floor cleaning and disinfection of poultry houses.
In conclusion, the new compound disinfectant developed in this study is suitable for air and ground disinfection in poultry farms. It has the characteristics of a novel structure, low irritation, and good safety, and most pathogenic bacteria can be killed within 10min. The new compound disinfectant can significantly reduce costs, improve safety, and provide a good prospect for clinical application. Therefore, it is broadly applicable in controlling poultry diseases and promoting the healthy development of animal husbandry.
The original contributions presented in the study are included in the article/supplementary material; further inquiries can be directed to the corresponding author.
ZZ and LY contributed to the conception and design of this work. NC, PQ, and LJ participated in sample collection, laboratory experiments, and data analysis. YY and HW completed Ames trial. LJ synthesized the compound in the pre-experimental stage. NC and ZZ drafted the manuscript. All authors read and approved the final manuscript.
This study was supported by the National Science and Technology Ministry (2013BAD21B01–1), Open Fund Project of Heilongjiang Provincial Key Laboratory of Fine Chemicals (2012–2014), and Heilongjiang Bayi Agricultural University Support Program for San Heng San Zong (TDJH202002).
The authors declared no potential conflicts of interest with respect to the research, authorship, and/or publication of this article.
All claims expressed in this article are solely those of the authors and do not necessarily represent those of their affiliated organizations, or those of the publisher, the editors and the reviewers. Any product that may be evaluated in this article, or claim that may be made by its manufacturer, is not guaranteed or endorsed by the publisher.
Alluri, V. K., Dodda, S., Kilari, E. K., Golakoti, T., and Sengupta, K. (2019). Toxicological assessment of a standardized boswellia serrata gum resin extract. Int. J. Toxicol. 38:6. doi: 10.1177/1091581819858069
Association for Applied Hygiene (VAH) Disinfectants Commission (2015). Requirements and Methods for VAH Certifification of Chemical Disinfection Procedures. Wiesbaden, Germany: mhp Verlag GmbH.
Begec, Z., Yucel, A., Yakupogullari, Y., Erdogan, M. A., Duman, Y., Durmus, M., et al. (2013). The antimicrobial effects of ketamine combined with propofol: An in vitro study. Braz. J. Anes. 63, 461–465. doi: 10.1016/j.bjane.2012.09.004
Bhende, S., and Spangler, D. (2004). In vitro assessment of chlorhexidine gluconate-impregnated polyurethane foam antimicrobial dressing using zone of inhibition assays. Infect. Control. Hosp. Epidemiol. 25, 664–667. doi: 10.1086/502458
Brandes, W., Hanssler, G., Reinecke, P., Scheinpflug, H., and Holmwood, G. (1993). Fungicidal agents: US, US5250560 (A). 10–29.
BS EN 13697 (2019). BS EN 13697:2015+A1:2019. Chemical disinfectants and antiseptics, Quantitative non-porous surface test for the evaluation of bactericidal and/or fungicidal activity of chemical disinfectants used in food, industrial, domestic and institutional areas. Test method and requirements without mechanical action (phase 2, step 2).
Burgess, W., Margolis, A., Gibbs, S., Duarte, R. S., and Jackson, M. (2017). Disinfectant susceptibility profiling of glutaraldehyde-resistant nontuberculous mycobacteria. Infect. Control Hosp. Epidemiol. 38, 1–8. doi: 10.1017/ice.2017.75
CEN (2015). BS EN 13727:2012+A2:2015-Chemical disinfectants and antiseptics. Quantitative suspension test for the evaluation of bactericidal activity in the medical area. Test method and requirements (phase 2, step 1).
Chapman, A. K., Aucott, S. W., and Milstone, A. M. (2012). Safety of chlorhexidine gluconate used for skin antisepsis in the preterm infant. J. Perinatol. 32, 4–9. doi: 10.1038/jp.2011.148
Cockerill, F. R. (2000). Methods for Dilution Antimicrobial Susceptibility Tests for Bacteria that Grow Aerobically: Approved Standard-Fifth Edition, NCCLS Document. Vol. 25, Clinical and Laboratory Standards Institute M7-A5.
Díez-Quijada, L., Prieto, A. I., Puerto, M., Jos, N., and Cameán, A. M. (2019). In vitro mutagenic and genotoxic assessment of a mixture of the cyanotoxins microcystin-lr and cylindrospermopsin. Toxins. 11, 317–334. doi: 10.3390/toxins11060318
EN 1040 (2005). Chemical disinfectants and antiseptics-quantitative suspension test for the evaluation of basic bactericidal activity of chemical disinfectants and antiseptics (phase 1).
EN 1275 (2005). Chemical disinfectants and antiseptics-quantitative suspension test for the evaluation of basic fungicidal or basic yeasticidal activity of chemical disinfectants and antiseptics (phase 1).4.
EN 13624 (2003). Chemical disinfectants and antiseptics-quantitative suspension test for the evaluation of fungicidal activity of chemical disinfectants for instruments used in the medical area (phase 2, step 1).
EN 14885 (2015). Chemical disinfectants and antiseptics-application of European standards for chemical disinfectants and antiseptics.
Faria, E. D., Gomes, M. V., Cláudio, A. F. M., Freire, C., Silvestre, A., and Freire, M. G. (2018). Extraction and recovery processes for cynaropicrin from Cynara cardunculus L. using aqueous solutions of surface-active ionic liquids. Biophys. Rev. 10, 915–925. doi: 10.1007/s12551-017-0387-y
Feng, Z., Guo, X., Jia, L., and Yu, Z. (2015). Surface activity and thermodynamic parameters of Pyridinium surfactants with an Aminoacylmethyl group. Res. Chem. Intermed. 41, 3825–3835. doi: 10.1007/s11164-013-1492-6
Giardino, L., Andrade, F. B., and Beltrami, R. (2016). Antimicrobial effect and surface tension of some chelating solutions with added surfactants. Braz. Dent. J. 27, 584–588.
Hidalgo, E., and Dominguez, C. (2001). Mechanisms underlying chlorhexidine-induced cytotoxicity. Toxicol. In Vitro 15, 271–276. doi: 10.1016/s0887-2333(01)00020-0
Ho, J., Tambyah, P. A., and Paterson, D. L. (2010). Multiresistant gram-negative infections: a global perspective. Curr. Opin. Infect. Dis. 23, 546–553. doi: 10.1097/qco.0b013e32833f0d3e
Ioannou, C., and Hanlon, G. S. (2007). Action of disinfectant quaternary ammonium compounds against staphylococcus aureus. Antimicrob. Agents Chemother 51:296. doi: 10.1128/aac.00375-06
Kassie, F., Rabot, S., Uhl, M., Huber, W., Qin, H. M., Helma, C., et al. (2002). Chemoprotective effect of garden cress (Lepidium sativum) and its constituents towards 2-amino-3-methyl-imidazo[4,5-f]quinoline (IQ)-induced genotoxic effects and colonic preneoplastic lesions. Carcinogenesis 23, 1155–1161.
Kuda, T., Yano, T., and Kuda, M. T. (2008). Resistances to benzalkonium chloride of bacteria dried with food elements on stainless steel surface. Food Sci. Technol. 41, 988–993. doi: 10.1016/j.lwt.2007.06.016
Medzhitov, R., Schneider, D. S., and Soares, M. P. (2012). Disease tolerance as a defense strategy. Science. 335, 936–941. doi: 10.1126/science.1214935
Murguía, M. C., Cristaldi, M. D., Porto, A., Conza, J. D., and Grau, R. J. (2008). Synthesis, surface-active properties, and antimicrobial activities of new neutral and cationic trimeric surfactants. J. Oleo Sci. 57, 301–308.
OECD (1984). Avian Dietary Toxicity Test. OECD Guidelines for the Testing of Chemicals, Section 2: Effects on Biotic Systems (Test No. 205). doi: 10.1787/20745761
OECD (2001). Acute Oral Toxicity-Acute Toxic Class Method. OECD Guidelines for the Testing of Chemicals, Section 4: Health Effects (Test No. 423). doi: 10.1787/20745788
OECD (2008). Acute Oral Toxicity: Up-and-Down Procedure. OECD Guidelines for the Testing of Chemicals, Section 4: Health Effects (Test No. 425).
OECD (2014). Organization for Economic Co-operation and Development. OECD guidance for the Testing of Chemicals in vivo mammalian alkaline comet assay. OECD Environment, Health and Safety Publications Series on Testing and Assessment No. 195.
OECD (2016). Avian Acute Oral Toxicity Test. OECD Guidelines for the Testing of Chemicals, Section 2: Effects on Biotic Systems (Test No. 223).
OECD (2020). Bacterial Reverse Mutation Test. OECD Guidelines for the Testing of Chemicals, Section 4: Health Effects (Test No. 471). doi: 10.1787/20745788
Rajamohan, G., and Srinivasan, V. B. (2010). Molecular and functional characterization of a novel efflux pump, Amv A, mediating antimicrobial and disinfectant resistance in Acinetobacter baumannii. J. Antimicrob. Chemother. 65, 1919–1925.
Sandle, T. (2019). Assessment of the recovery of different bacteria from two cleanroom surface materials. Chimica Oggi-Chem. Today. 37, 31–33.
Song, X., Zuo, G., and Chen, F. (2018). Effect of essential oil and surfactant on the physical and antimicrobial properties of corn and wheat starch films. Int. J. Biol. Macromol. 107, 1302–1309. doi: 10.1016/j.ijbiomac.2017.09.114
West, A. M., Teska, P. J., Lineback, C. B., and Oliver, H. F. (2018). Strain, disinfectant, concentration, and contact time quantitatively impact disinfectant efficacy. Antimicrob. Resist. Infect. Control 7, 49. doi: 10.1186/s13756-018-0340-2
Xue, Y. F., Guo, C. Z., Hu, F., Sun, D. M., Liu, J. H., and Mao, S. Y. (2018). Molecular mechanisms of lipid metabolism disorder in livers of ewes with pregnancy toxemia. Animal 13, 1–8. doi: 10.1017/S1751731118002136
Keywords: quaternary ammonium salt, antibacterial activity, synergistic effect, safety, microorganism
Citation: Chen N, Qin P, Liu Y, Yang Y, Wen H, Jia L, Li J and Zhu Z (2021) Influence of New Compound Disinfectant From N-Dodecyl-2-(Piridin-1-Ium)Acetamide Chloride on Pathogenic Microorganisms in Poultry Houses. Front. Microbiol. 12:735859. doi: 10.3389/fmicb.2021.735859
Received: 03 July 2021; Accepted: 16 August 2021;
Published: 24 September 2021.
Edited by:
Pier-Luc Tremblay, Wuhan University of Technology, ChinaReviewed by:
Agata Piecuch, University of Wrocław, PolandCopyright © 2021 Chen, Qin, Liu, Yang, Wen, Jia, Li and Zhu. This is an open-access article distributed under the terms of the Creative Commons Attribution License (CC BY). The use, distribution or reproduction in other forums is permitted, provided the original author(s) and the copyright owner(s) are credited and that the original publication in this journal is cited, in accordance with accepted academic practice. No use, distribution or reproduction is permitted which does not comply with these terms.
*Correspondence: Zhanbo Zhu, emhhbmJvemh1QDE2My5jb20=
†These authors have contributed equally to this work
Disclaimer: All claims expressed in this article are solely those of the authors and do not necessarily represent those of their affiliated organizations, or those of the publisher, the editors and the reviewers. Any product that may be evaluated in this article or claim that may be made by its manufacturer is not guaranteed or endorsed by the publisher.
Research integrity at Frontiers
Learn more about the work of our research integrity team to safeguard the quality of each article we publish.