- 1Zhuhai Precision Medical Center, Zhuhai People’s Hospital (Zhuhai Hospital Affiliated With Jinan University), Zhuhai, China
- 2Department of Radiation Oncology, Zhuhai People’s Hospital (Zhuhai Hospital Affiliated With Jinan University), Zhuhai, China
- 3Department of Spine and Osteology, Zhuhai People’s Hospital (Zhuhai Hospital Affiliated With Jinan University), Zhuhai, China
- 4Department of Urology, Zhuhai People’s Hospital (Zhuhai Hospital Affiliated With Jinan University), Zhuhai, China
- 5Zhuhai Interventional Medical Center, Zhuhai People’s Hospital (Zhuhai Hospital Affiliated With Jinan University), Zhuhai, China
- 6Department of Clinical Laboratory, Zhuhai People’s Hospital (Zhuhai Hospital Affiliated With Jinan University), Zhuhai, China
- 7Department of Pharmacy, Zhuhai People’s Hospital (Zhuhai Hospital Affiliated With Jinan University), Zhuhai, China
New Delhi metallo-β-lactamases (NDMs), including at least 28 variants, are a rapidly emerging family of β-lactamases worldwide, with a variety of infections caused by NDM-positive strains usually associated with very poor prognosis and high mortality. NDMs are the most prevalent carbapenemases in Escherichia coli (E. coli) worldwide, especially in China. The vast majority of blaNDM cases occur on plasmids, which play a vital role in the dissemination of blaNDM. To systematically explore the relationships between plasmids and blaNDM genes in E. coli and obtain an overall picture of the conjugative and mobilizable blaNDM-positive plasmids, we analyzed the variants of blaNDM, replicon types, phylogenetic patterns, conjugative transfer modules, host STs, and geographical distributions of 114 blaNDM-positive plasmids, which were selected from 3786 plasmids from 1346 complete whole genomes of E. coli from the GenBank database. We also established links among the characteristics of blaNDM-positive plasmids in E. coli. Eight variants of blaNDM were found among the 114 blaNDM-positive plasmids, with blaNDM–5 (74 blaNDM–5 genes in 73 plasmids), and blaNDM–1 (31 blaNDM–1 genes in 28 plasmids) being the most dominant. The variant blaNDM–5 was mainly carried by the IncX3 plasmids and IncF plasmids in E. coli, the former were mainly geographically distributed in East Asia (especially in China) and the United States, and the latter were widely distributed worldwide. IncC plasmids were observed to be the predominant carriers of blaNDM–1 genes in E. coli, which were mainly geographically distributed in the United States and China. Other blaNDM–1-carrying plasmids also included IncM2, IncN2, and IncHI1. Moreover, the overall picture of the conjugative and mobilizable blaNDM-positive plasmids in E. coli was described in our study. Our findings enhance our understanding of the genetic diversity and characteristics of blaNDM-positive plasmids in in E. coli.
Introduction
New Delhi metallo-β-lactamase (NDM) is a metallo-β-lactamase that can hydrolyze almost all β-lactam antibiotics, including carbapenems (Nordmann et al., 2011). NDM-1 was first identified in a Klebsiella pneumoniae strain isolated from a Swedish patient who had been hospitalized in New Delhi, India in 2008 (Yong et al., 2009). So far, 28 variants of NDM have been reported (Farhat and Khan, 2020). A variety of infections caused by NDM-positive strains are reportedly associated with very poor prognosis and high mortality, especially in neonates and high-risk immunocompromised patients (Guducuoglu et al., 2018). NDM-positive strains have been found worldwide, with the highest prevalence in the Indian subcontinent, the Middle East, and the Balkans (Albiger et al., 2015; Wu and Feng, 2019). According to the Study for Monitoring Antimicrobial Resistance Trends (SMART) global surveillance program, blaNDM is the third most common carbapenemase-encoding gene and accounts for 19.42% of carbapenemase positivity after blaKPC (53.18%) and the blaOXA–48-like gene (20.09%) (Karlowsky et al., 2017). In China, the presence of blaKPC (51.6%) and blaNDM (35.7%) is responsible for phenotypic resistance in most carbapenem-resistant Enterobacteriaceae (CRE) strains (Han et al., 2020), according to data from the China Antimicrobial Surveillance Network (CHINET) in 2018. Furthermore, data from SMART and CHINET2018 demonstrated that NDM was the most prevalent carbapenemase in E. coli, especially in China; blaNDM accounted for 93.0 and 97.2% of carbapenem-resistant E. coli isolates from adults and children, respectively (Han et al., 2020).
Antimicrobial resistance (AMR) in CRE isolates is frequently mediated by plasmid-borne genes, in addition to chromosomal determinants (Rozwandowicz et al., 2018). Plasmids remain important microbial components that mediate horizontal gene transfer (HGT) and play a vital role in the dissemination of AMR (Jiang et al., 2020). blaNDM has been reported to be carried on plasmids with a variety of replicon types, most of which belong to limited replicon types (IncX3, IncFII, or IncC) (Wu and Feng, 2019). For CRE isolates, conjugative plasmids have been highlighted as important vehicles for the dissemination of AMR (Smillie et al., 2010; Ravi et al., 2018). The conjugative transfer regions of the conjugative plasmids typically consist of four modules: an origin of transfer (oriT) region, relaxase gene, type IV coupling protein (T4CP) gene, and gene cluster for the bacterial type IV secretion system (T4SS) apparatus (de la Cruz et al., 2010). In addition, mobilizable plasmids are also contributors to AMR, typically carrying the indispensable oriT sites and a limited number of mob genes for their own DNA processing in conjugation, which can be mobilized by conjugative elements (Ramsay and Firth, 2017). Currently, studies on the distribution of conjugative and mobilizable blaNDM-positive carbapenem-resistant plasmids in E. coli are rare. With the increase in the amount of whole-genome/plasmid sequencing data, there is a need for large-scale plasmid analysis of blaNDM-positive plasmids of E. coli.
In this study, we performed in silico typing and comparative analysis of blaNDM-positive plasmids of E. coli using the bacterial genome and plasmid sequences available in the NCBI database. We analyzed the geographical distribution of blaNDM-positive plasmids and compared the replicon types, conjugative transfer modules, and profiles of resistance determinants among blaNDM-positive plasmids of E. coli. This study provides important insights into the phylogeny and evolution of blaNDM-positive E. coli plasmids and further addresses their role in the acquisition and spread of resistance genes.
Materials and Methods
For this study, the data collection and analysis are shown in Supplementary Figure 1.
Plasmid Genomic Sequences
A total of 1346 complete whole genomes of E. coli, including the genomes marked by “Chromosome” and “Complete” in assembly level, were downloaded from the GenBank (Benson et al., 2018) Genome database.1 The download date was April 15, 2021. We extracted 3786 plasmid genomic sequences without duplicates (Supplementary Table 1) from the 1346 complete whole genomes of E. coli. In addition, a total of 35150 bacterial plasmid genomic sequences were downloaded from the NCBI RefSeq database (O’Leary et al., 2016),2 including 6054 plasmids from E. coli (Supplementary Table 2), with the download date as July 14, 2021. The genome data (FASTA DNA format) were downloaded in bulk into a DELL PowerEdge R930 server with a Linux-CentOS7 operating system, using two Bioperl modules (Bio:DB:GenBank and Bio:SeqIO). Perl v5.16.3 was installed in the Linux platform.
Determination of blaNDM-Positive Plasmids of E. coli
The potential β-lactamase genes of plasmids in FASTA DNA format were determined using the ResFinder software version 4.13 (Bortolaia et al., 2020) installed in our server, with a minimum coverage of 60%, minimum identity of 90%, and species of “Escherichia coli.” The term “blaNDM” was used to search in the “Resistance gene” list within the ResFinder results to determine the blaNDM-positive plasmids of E. coli.
Replicon Sequence Analysis of the blaNDM-Positive Plasmids of E. coli
Plasmid replicon typing was performed using the PlasmidFinder software4 (Carattoli and Hasman, 2020). Selecting the database “Enterobacteriaceae,” the DNA files in FASTA format were analyzed in bulk using the PlasmidFinder software version 2.0.1 installed in the Linux platform, with the minimum coverage of 60% and minimum identity of 95%.
Phylogenetic Analyses of the blaNDM-Positive Plasmids of E. coli
The files in GenBank format of the blaNDM-positive plasmids of E. coli were downloaded in bulk using the Bio:DB:GenBank and Bio:SeqIO modules. Files containing protein sequences were extracted from the files in GenBank format using the Bioperl/Bio:SeqIO module. Phylogenetic patterns based on the presence/absence of orthologous gene families of all blaNDM-positive plasmids of E. coli were analyzed in this study. A binary protein presence/absence matrix was created using OrthoFinder5 (Emms and Kelly, 2019) with DIAMOND for sequence similarity searches, and then a hierarchical cluster result was shown by iTOL6 (Letunic and Bork, 2016).
Characterization of the Conjugative Modules of blaNDM-Positive Plasmids
The files in GenBank format of the blaNDM-positive plasmids of E. coli were analyzed in bulk using the software oriTfinder7 (Li et al., 2018) (local version) to determine the presence/absence of oriTs, relaxase genes, T4CP genes, and gene cluster for T4SS. Furthermore, the types of oriTs, relaxase genes, T4CP genes, and gene cluster for T4SS toward the plasmids were identified based on the exhibition of oriTDB database8 (Li et al., 2018).
Multilocus Sequence Typing of E. coli Strains Bearing blaNDM-Positive Plasmids
The blaNDM-positive plasmid-matched host E. coli strains were collected, and their DNA fasta sequences were downloaded in bulk using the Bio:DB:GenBank and Bio:SeqIO modules. The MLST software (Larsen et al., 2012) version 2.0.4 was downloaded from the website9 and installed on the Linux platform. The genomes of E. coli strains were analyzed in bulk using MLST software. The “Escherichia_coli#1” dataset containing the seven housekeeping genes (adk, fumC, gyrB, icd, mdh, purA, and recA) (Wirth et al., 2006) was selected.
Results
General Characteristics of blaNDM-Positive Plasmids of E. coli
Using ResFinder, 1001 (26.4%) plasmids bearing β-lactamase genes were identified from the 3786 plasmids, which were included in the 1346 complete whole genomes of E. coli. Among the 1001 plasmids containing β-lactamase genes, 114 (11.6%) were further identified as blaNDM-positive plasmids, which were distributed in 113 strains of E. coli.
We analyzed and compared the genome sizes of the blaNDM-positive plasmids, plasmids containing β-lactamase genes, and all 3786 plasmids of E. coli. Among the 113 fully sequenced E. coli strains, the genome sizes of 114 blaNDM-positive plasmids varied from 10.49 to 248.8 kb, with the 25% percentile, median, and 75% percentile were 46.16, 75.6, and 128.8 kb, respectively (Figure 1A). For the β-lactamase gene-positive plasmids and all 3786 plasmids of E. coli, their genome sizes varied greatly. Genome sizes of the former ranged from 4.49 to 369.3 kb and those of the latter ranged from 0.3 to 404.2 kb (Figure 1A).
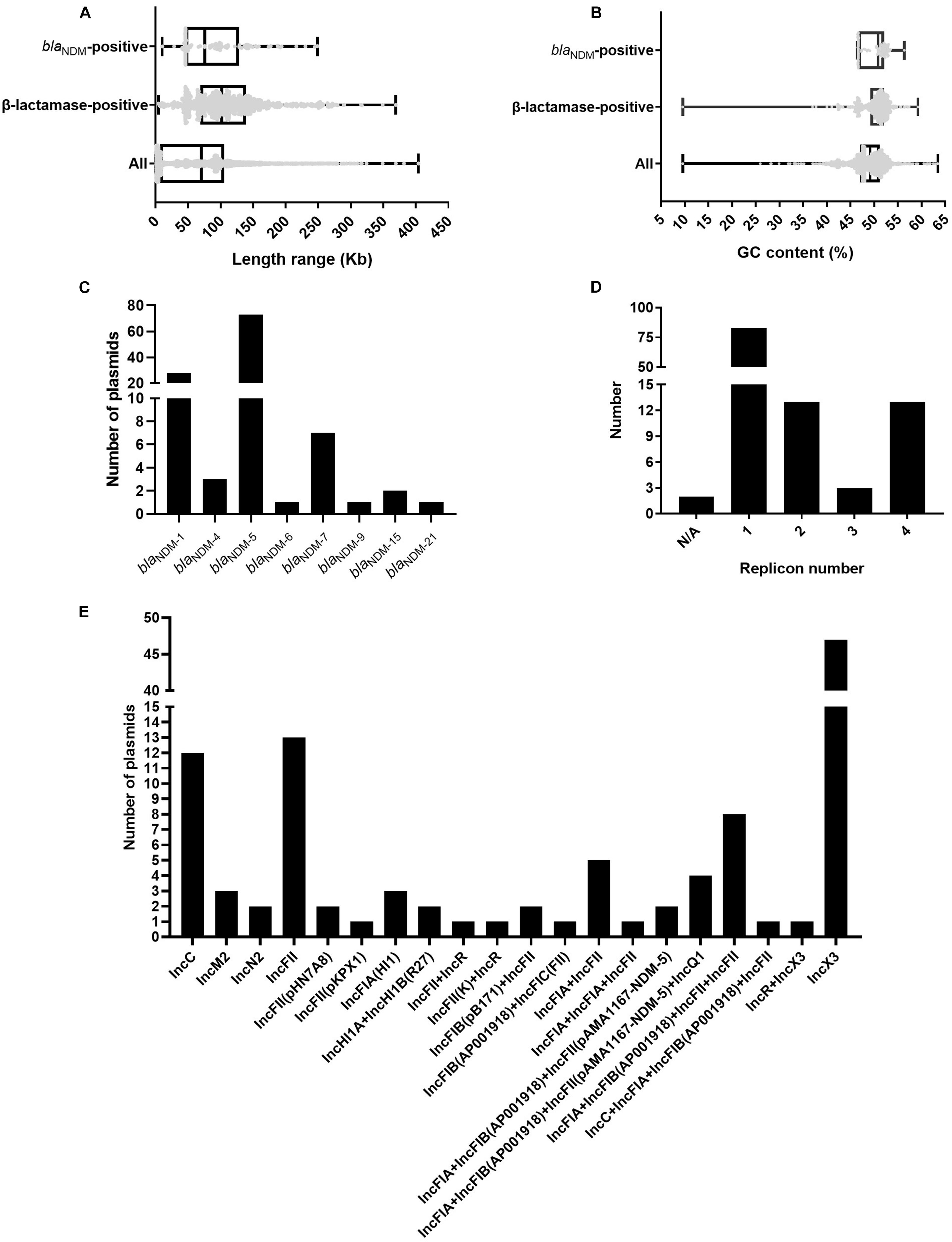
Figure 1. Characteristics of the 114 blaNDM-positive plasmids from 113 completely sequenced E. coli genomes. (A) Box plot of the length distribution of the 114 blaNDM-positive plasmids, the 1001 plasmids carrying β-lactamase genes, and all 3786 plasmids of E. coli. (B) Box plot of the GC content distribution of the 114 blaNDM-positive plasmids, the 1001 plasmids carrying β-lactamase genes, and all 3786 plasmids of E. coli. (C) Histogram of number of variants of blaNDM genes among the 114 blaNDM-positive plasmids. (D) Histogram of number of replicons per plasmid for the 114 blaNDM-positive plasmids. (E) Histogram of number of combination modes of different replicons among the 114 blaNDM-positive plasmids.
We calculated the GC contents of the blaNDM-positive plasmids, plasmids containing β-lactamase genes, and all 3786 plasmids of E. coli. The GC content of the 114 blaNDM-positive plasmids ranged from 46.5 to 56.4%, with a median GC content of 50.8% (25% percentile = 46.7%; 75% percentile = 52.2%) (Figure 1B). For the plasmids containing β-lactamase genes and all 3786 plasmids of E. coli, the range of GC contents varied greatly. The GC content of the former ranged from 9.6 to 59.3%, and those of the latter ranged from 9.6 to 63.5% (Figure 1B).
Variants of blaNDM Genes in the blaNDM-Positive Plasmids of E. coli
Among the 114 blaNDM-positive plasmids, 124 blaNDM genes belonging to eight kinds of variants of blaNDM, including blaNDM–1, blaNDM–4, blaNDM–5, blaNDM–6, blaNDM–7, blaNDM–9, blaNDM–15, and blaNDM–21, were identified. Among the eight variants of blaNDM, blaNDM–5 was found to be the most dominant variant (74 blaNDM–5 genes in 73 plasmids), followed by blaNDM–1 (31 blaNDM–1 genes in 28 plasmids), and blaNDM–7 (7 blaNDM–7 genes in seven plasmids) (Figure 1C).
Replicon Types of Plasmids Carrying blaNDM of E. coli
Replicon typing of the 114 blaNDM-positive plasmids was performed using PlasmidFinder. Among the 114 plasmids, 112 successfully identified their replicon types, including 83 single-replicon plasmids and 29 multi-replicon plasmids (13 plasmids with two replicons, 3 plasmids with three replicons, and 13 plasmids with four replicons) (Figure 1D). For the 83 single-replicon plasmids, plasmids with an IncX3 replicon were found to be the most dominant single-replicon plasmids (47 plasmids), followed by plasmids with an IncFII replicon (13 plasmids) and those with an IncC replicon (12 plasmids) (Figure 1E). Interestingly, the multi-replicon plasmids were mainly classified into IncF plasmids (Figure 1E). In summary, all 114 blaNDM-positive plasmids were mainly classified into IncX3, IncF, and IncC plasmids (Supplementary Figure 2).
Genetic Diversity of the blaNDM-Positive Plasmids of E. coli
To obtain a comprehensive overview of blaNDM-positive plasmids, we constructed phylogenetic trees of all 114 blaNDM-positive plasmids (Figure 2). Based on the phylogenetic patterns of plasmids, combined with the plasmid types and conjugative transfer modules, 109 of the 114 blaNDM-positive plasmids were classified into eight main clades (Clade I—Clade VIII), representing eight representative plasmid patterns carrying blaNDM genes in E. coli. We also investigated the geographical distribution of the eight clades from blaNDM-positive E. coli plasmids.
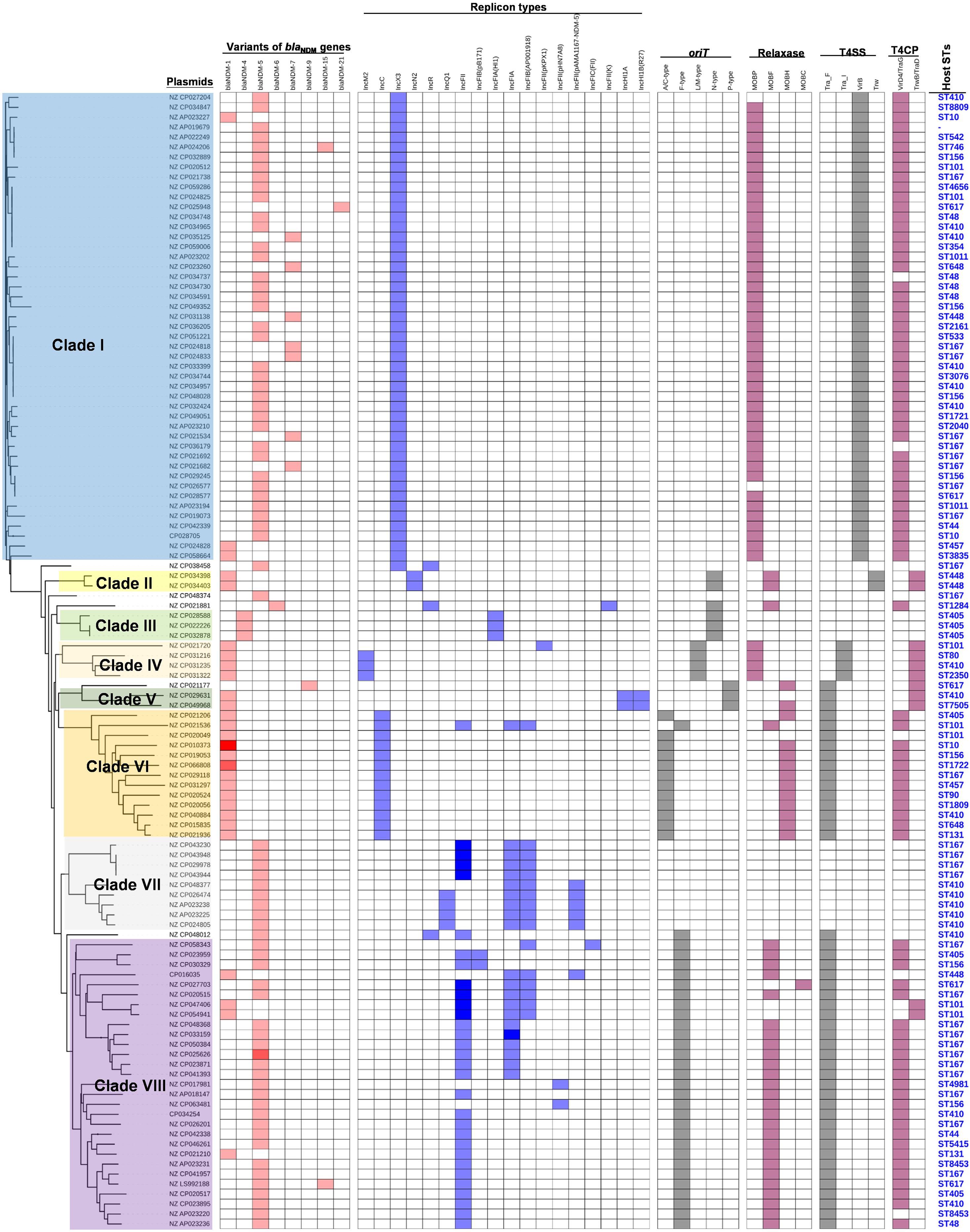
Figure 2. Conjugative transfer modules including oriT, relaxase, T4CP, and T4SS of the representative plasmids from Clade I (A) and Clade VIII (B), respectively.
Clade I: A total of 47 plasmids were identified in the Clade I cluster, accounting for approximately 41.2% of all 114 blaNDM-positive plasmids, which is the clade with the largest number among all the eights clades (Figure 2). Most (76.6%) of the plasmids classified into Clade I carried blaNDM–5 gene. All plasmids below Clade I were single-replicon plasmids with an IncX3 replicon, and most were 46-kb plasmids. For the conjugative transfer modules, almost all the plasmids belonging to Clade I carried relaxases of the MOBP family and T4CPs of the VirD4/TraG subfamily. All 47 Clade I plasmids carrying blaNDM were found to contain VirB-type T4SS gene clusters (Figure 3A). The current version of oriTfinder could not identify the oriT sites of the Clade I plasmids, while 354-bp intergenic sequences flanking the relaxase genes were oriT–like regions, with the inverted repeat (IR) sequence (TAACTA.TAGTTA) (Figures 2, 3A). The STs of E. coli host strains containing all Clade I plasmids were distributed in ST167, ST410, ST48, etc. For the clade with the largest number, Clade I, its plasmids were mainly distributed in East Asia (especially in China) and the United States (Figure 4). Most of the Clade I plasmids were the human origin, some were animal origin (mainly in China) and environment origin (both in Japan and China) (Supplementary Table 3).
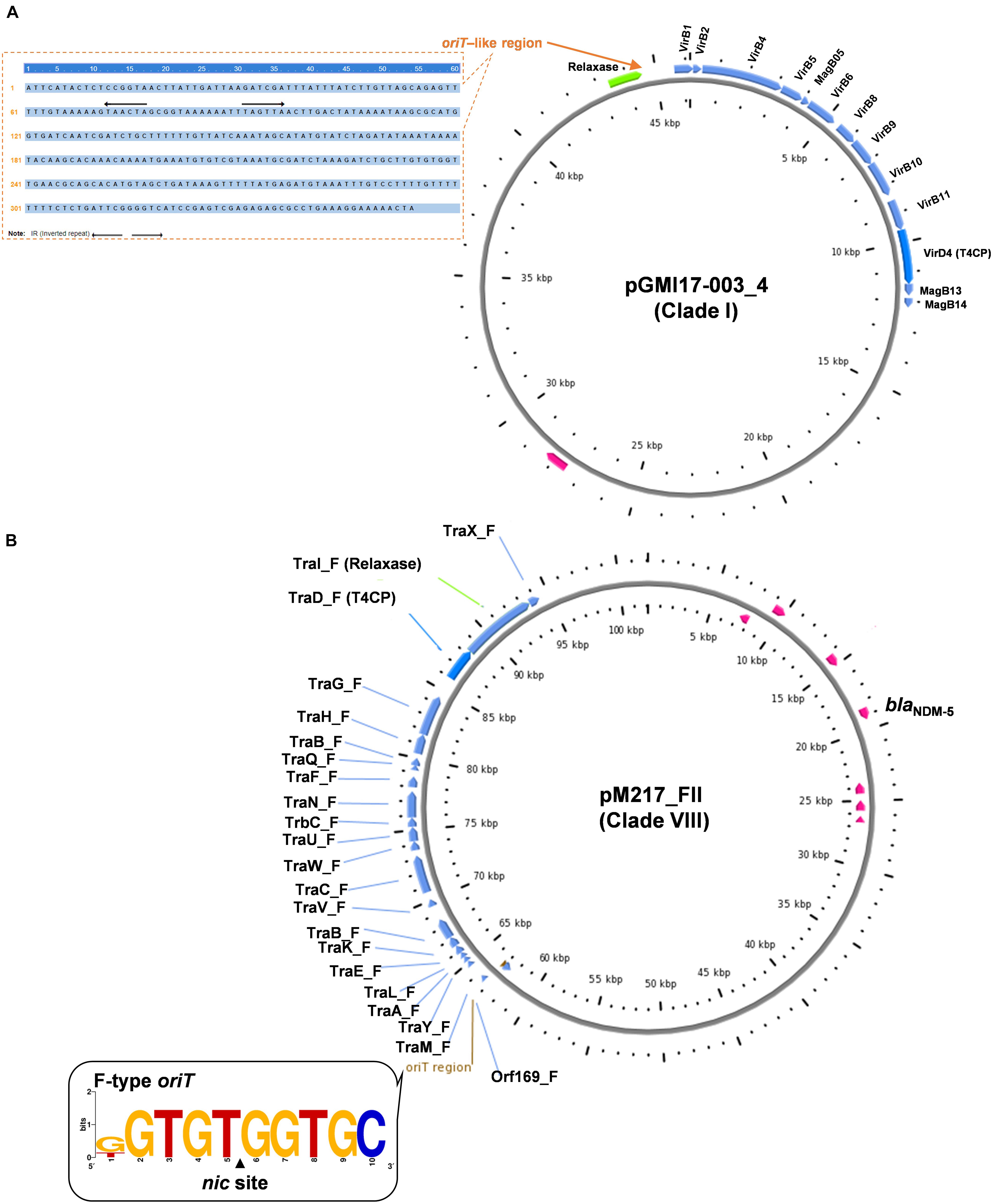
Figure 3. Details of variants of blaNDM genes, replicon types of plasmids, and the conjugative transfer modules of the 114 blaNDM-positive plasmids in E. coli. The five categories of information present in this figure include the phylogenetic tree of 114 blaNDM-positive plasmids, variants of blaNDM genes, replicon types, conjugative transfer modules, and STs of host strains. The gradient of color of each heatmap (variants of blaNDM genes, replicon types, phylogenetic patterns, conjugative transfer modules including oriT, relaxase, T4CP, and T4SS) represents the variable numbers of genes or gene clusters.
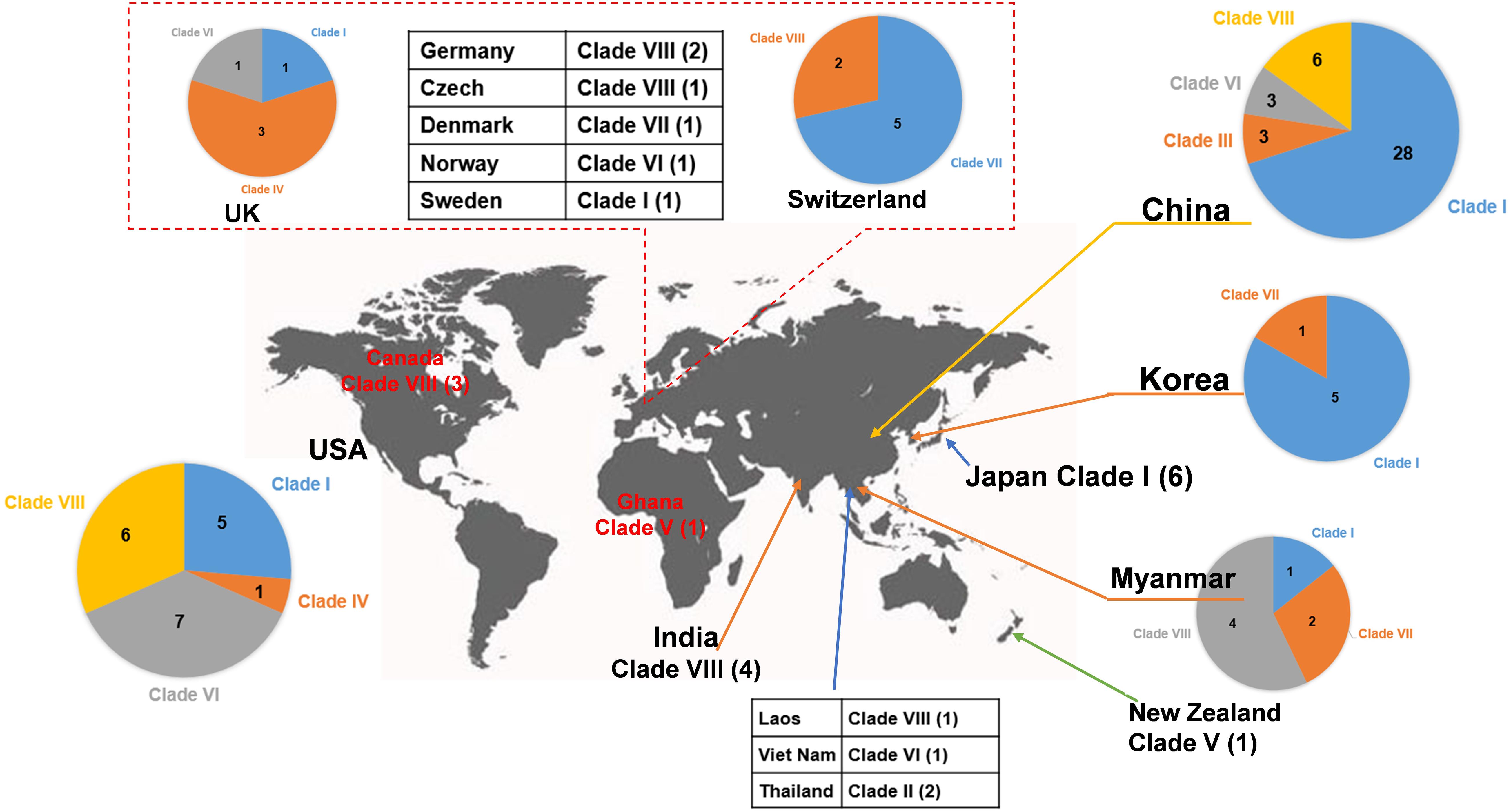
Figure 4. Worldwide distribution of blaNDM-positive plasmids of E. coli. The geographical distribution of the eight clades (Clade I–Clade VIII) from the blaNDM-positive plasmids of E. coli was calculated and displayed by pie chart.
Clade II: Two blaNDM–1-positive IncN2 plasmids of E. coli ST448 were clustered into Clade II (Figure 2). They both carried the N-type oriTs, relaxases of MOBF family, T4CPs of TrwB/TraD subfamily, and Trw type of T4SS gene clusters (Figure 2 and Supplementary Figure 3). Clade II, containing two blaNDM–1-positive IncN2 plasmids, was found to be distributed only in Thailand (human origin) (Figure 4 and Supplementary Table 3).
Clade III: Three blaNDM–4-positive IncF plasmids with IncFIA(HI1) replicon of E. coli ST405 were clustered into Clade III (Figure 2). They were all found to carry only one conjugative transfer module: N-type oriTs, but no relaxases, T4CPs, or T4SS gene clusters were found, indicating that they should be mobilizable plasmids (Supplementary Figure 4). All three members of Clade III, inferred as mobilizable plasmids, were only found to be geographically distributed in China (all human origin) (Figure 4 and Supplementary Table 3).
Clade IV: Four blaNDM–1-positive single-replicon plasmids were classified into Clade IV, including three IncM2 plasmids and one IncFII(pKPX1) plasmid (Figure 2). For the conjugative transfer modules of the four Clade IV plasmids, they all carry the L/M-type oriTs, relaxases of MOBP family, T4CPs of TrwB/TraD subfamily, and Tra_I type of T4SS gene clusters (Figure 2 and Supplementary Figure 5). E. coli host strains containing all four Clade IV plasmids were distributed into four different STs. Members of Clade IV were mainly geographically distributed in the United Kingdom (three plasmids, all human origin) (Figure 4 and Supplementary Table 3).
Clade V: Two blaNDM–1-positive multi-replicon plasmids, both containing IncHI1A and IncHI1B(R27) replicons, were grouped into the clade V cluster (Figure 2). They both carried the P-type oriTs, T4CPs of the TrwB/TraD subfamily, and Tra_F type of T4SS gene clusters (Figure 2 and Supplementary Figure 6). One plasmid of E. coli ST7505 was found to carry a relaxase of the MOBH family; the other plasmid of E. coli ST410 was not able to identify the relaxase gene in the genome of the plasmid. The two members of Clade V were geographically distributed in Ghana and New Zealand, respectively (both human origin) (Figure 4 and Supplementary Table 3).
Clade VI: All 13 plasmids grouped into the clade VI cluster of the phylogenetic tree were found to carry blaNDM–1 gene (Figure 2). All plasmids belonging to Clade VI were identified as IncC plasmids, including 12 single-replicon plasmids with IncC replicon and one multi-replicon plasmid with four replicons (IncC, IncFIA, IncFIB(AP001918), and IncFII). For the conjugative transfer modules, most of the plasmids belonging to Clade VI carry the A/C-type oriTs, relaxases of MOBH family and T4CPs of VirD4/TraG subfamily. All 13 clade VI plasmids carrying blaNDM–1 were found to have Tra_F type T4SS gene clusters (Figures 2, 5). No prevalent STs of E. coli host strains containing all Clade VI plasmids were found. The members of Clade VI were mainly geographically distributed in the United States and China (human origin) (Figure 4 and Supplementary Table 3).
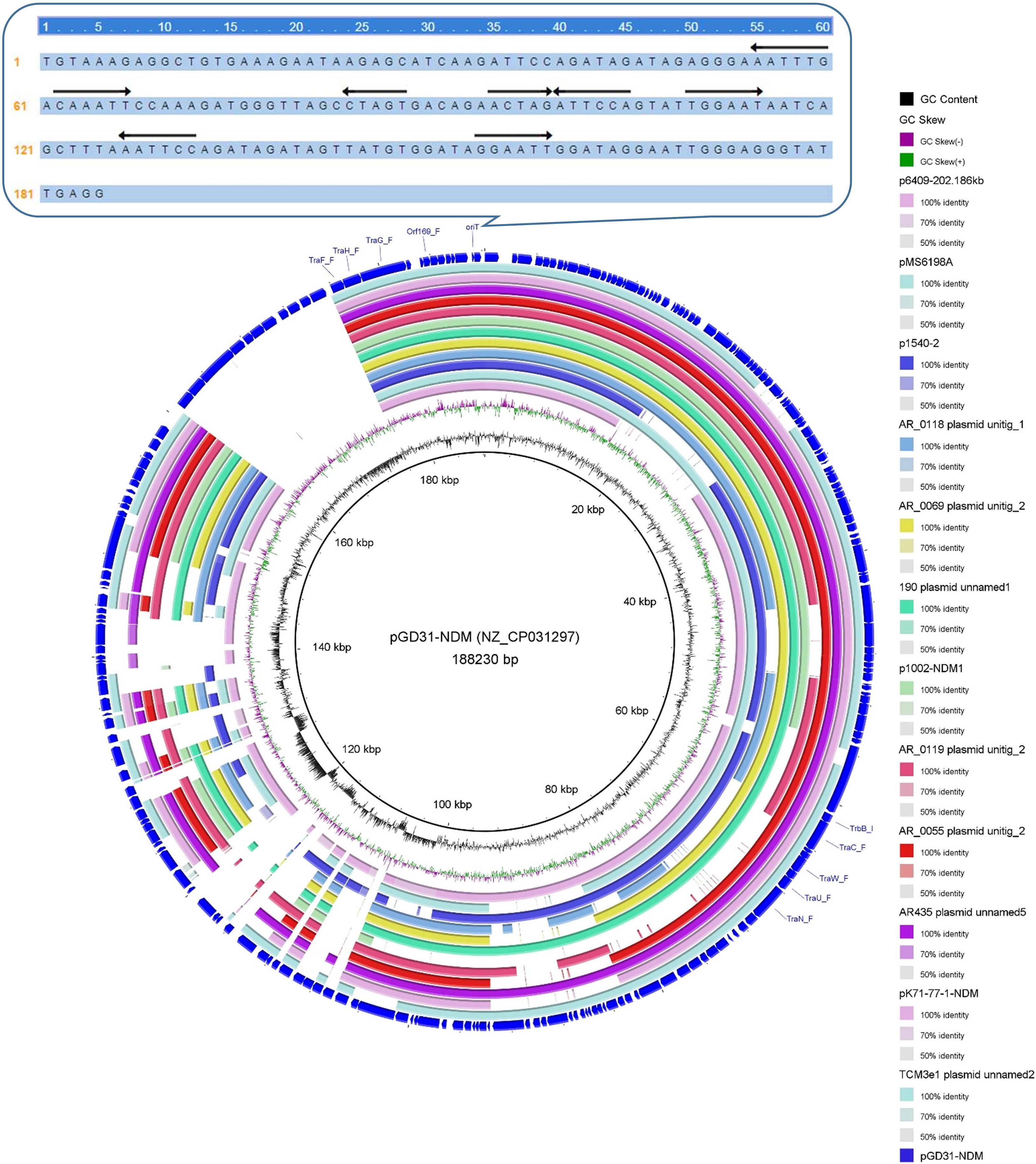
Figure 5. Conjugative transfer modules of 13 blaNDM–1-positive IncC plasmids grouped into the clade VI and the sequence logos of the flanking conserved regions of the nic sites of A/C-type oriT regions.
Clade VII: Nine blaNDM–5-positive IncF plasmids were classified into clade VII (Figure 2). All the plasmids belonging to Clade VII were found to contain both replicon IncFIA and replicon IncFIB(AP001918), including two main kinds of replication combination modes: four plasmids with replicons IncFIA + IncFIB(AP001918) + IncFII + IncFII and four plasmids with replicons IncFIA + IncFIB(AP001918) + IncFII(pAMA1167-NDM-5) + IncQ1. Moreover, no conjugative transfer modules were found in the nine plasmids belonging to Clade VII, indicating that the nine plasmids should be non-transferable plasmids. The STs of E. coli host strains containing Clade VII plasmids were distributed into ST167 and ST410. The members of clade VII were mainly geographically distributed in Switzerland (Figure 4). In addition, clade VII members were also sporadically discovered in Myanmar, Korea, and Denmark. Clade VII plasmids included the human and animal origin (Supplementary Table 3).
Clade VIII: A total of 29 plasmids were identified in the Clade VIII cluster, accounting for approximately 25.4% of all 114 blaNDM-positive plasmids, which is the clade with the second largest number among all eights clades (Figure 2). Most (86.2%) of the plasmids grouped into clade VIII were found to carry blaNDM–5 gene. All plasmids below Clade VIII were IncF plasmids, with replicon IncFII as the most common replicons. For the conjugative transfer modules, all the plasmids belonging to Clade VIII carry the F-type oriTs and Tra_F type of T4SS gene clusters (Figures 2, 3B). Almost all the plasmids of Clade VIII had relaxases of the MOBF family and T4CPs of the VirD4/TraG subfamily. The STs of E. coli host strains containing all Clade VIII plasmids were distributed in ST167, ST101, ST44, ST410 etc. For the clade with the second largest number, Clade VIII, its members were widely distributed all over the world, including East Asia, India, the United States, and some European countries (e.g., Germany, Switzerland, and the Czech Republic) (Figure 4). Most of the Clade VIII plasmids were the human origin, few were animal and environment origin (Supplementary Table 3).
We also analyzed the 6054 plasmids of E. coli downloaded from the NCBI RefSeq database, both with and without host strains. The results indicated that 301 blaNDM-positive plasmids were identified from the 6054 plasmids of E. coli (Supplementary Figure 7). We explored the distribution of the above eight representative plasmid patterns carrying blaNDM genes of E. coli in the 301 blaNDM-positive plasmids. For the blaNDM–5 gene, it was mainly carried by the IncX3 plasmids (Clade I pattern) and IncF plasmids (Clade VII and Clade VIII patterns), consistent with the results based on the 114 blaNDM-positive plasmids with hosts. The IncN plasmids (including IncN2 and IncN replicons) were found to mainly carry the blaNDM–1 gene (Clade II pattern) in the 301 blaNDM-positive plasmids, consistent with the results from the 114 blaNDM-positive plasmids with hosts. The blaNDM–4 gene was carried sporadically by IncF, IncX, and IncR plasmids, not limited to the Clade III pattern from the 114 blaNDM-positive plasmids with hosts. The IncM2 plasmids were found to carry the blaNDM–1 gene (Clade IV pattern) in the 301 blaNDM-positive plasmids, consistent with the results of blaNDM-positive plasmids with hosts. For the IncHI1 and IncHI2 from the 301 blaNDM-positive plasmids, they were found to carry not only the blaNDM–1 (Clade V pattern) but also the blaNDM–5 gene. The IncC plasmids were found to carry the blaNDM–1 gene (Clade VI pattern) in the 301 blaNDM-positive plasmids, consistent with the conclusion from blaNDM-positive plasmids with hosts.
Discussion
NDM carbapenemases are a rapidly emerging and troublesome family of β-lactamases (Pérez-Vázquez et al., 2019; Sugawara et al., 2019; Han et al., 2020). To explore the relationships among plasmids, blaNDM genes, and hosts in E. coli, we systematically analyzed the profiles of resistance determinants, replicon typing, and comparative analysis of 3786 plasmids from 1346 complete whole genomes of E. coli from the GenBank database. Overall, 114 blaNDM-positive plasmids from 113 E. coli strains were identified.
Variants of blaNDM included in the 114 blaNDM-positive plasmids in our study were classified into eight types. The blaNDM–5-carrying plasmids were the most common blaNDM-positive plasmids and accounted for 64.0% of the 114 blaNDM-positive plasmids, followed by blaNDM–1-positive plasmids (24.6%) and blaNDM–7-positive plasmids (6.1%). blaNDM–1 was first identified on a 180-kb plasmid of K. pneumoniae strain 05-506 and on a 140-kb plasmid carried by E. coli strain NF-NDM-1, both isolated from a Swedish patient who had been hospitalized in New Delhi, India, in 2008 (Yong et al., 2009). The variant NDM-5 was first detected in a strain of E. coli EC405 belonging to ST648, isolated from a 41-year-old patient in the United Kingdom with a history of travel to the Indian subcontinent, and blaNDM–5 was localized to an IncF plasmid with a length > 100 kb (Hornsey et al., 2011). The variant NDM-7 was first detected in a strain of E. coli ST599, isolated from the rectum, throat, and infected wounds of a Yemeni patient admitted to the Frankfurt University Hospital of Germany, and blaNDM–7 was localized on a self-transferable IncX3 plasmid of 60 kb (Göttig et al., 2013).
Among the 114 blaNDM-positive plasmids in E. coli, 112 were successfully identified by their replicon types, and mainly classified into IncX3, IncF, and IncC plasmids. Our results also indicated that the 112 blaNDM-positive plasmids contained 83 single-replicon plasmids and 29 multi-replicon plasmids.
IncX3 plasmids have been reported to carry various carbapenemase genes in CRE worldwide (Mouftah et al., 2019). Herein, our work indicated that the IncX3 plasmids were the most prevalent single-replicon plasmids among the 114 blaNDM-positive plasmids in E. coli, which were observed to be the predominant carriers of blaNDM–5 genes, distributed in Clade I of the phylogenetic profiles constructed by the 114 blaNDM-positive plasmids of E. coli. Common types of E. coli strains containing blaNDM–5-positive IncX3 plasmids were ST167, ST410, and ST48, located in East Asia (especially China) and the United States.
In our study, multi-replicon IncF plasmids, especially the plasmids with IncFII replicon, were another common carrier of blaNDM–5 genes, which were distributed in Clade VII and Clade VIII of the phylogenetic profiles of the 114 blaNDM-positive plasmids of E. coli, members of the former were identified as the non-transferable plasmids and those of the latter were identified as the conjugative plasmids. The IncF plasmids, widely distributed in Enterobacteriaceae, are known as conjugative plasmids that contribute to the carriage and spread of AMR genes (Carattoli, 2011), similar to Clade VIII of the phylogenetic profiles of the 114 blaNDM-positive plasmids of E. coli in our own study. However, in our study, we also found nine blaNDM–5-positive IncF plasmids without any classical conjugative transfer modules, classified into Clade VII of the phylogenetic patterns of the 114 blaNDM-positive plasmids of E. coli, which were identified as non-transferable plasmids. The nine non-transferable IncF plasmids of Clade VII were distributed in the E. coli strains of ST167 (four plasmids) and ST410 (five plasmids), mainly located in Switzerland. The blaNDM-positive IncF plasmids in E. coli grouped into Clade VIII, which were the classical conjugative plasmids, mainly distributed in the E. coli strains of ST167 and geographically distributed worldwide (East Asia, India, the United States, and some European countries).
IncC plasmids, almost all single-replicon plasmids, were observed to be the predominant carriers of blaNDM–1 genes in E. coli, which were grouped into Clade VI of the phylogenetic profiles constructed by the 114 blaNDM-positive plasmids of E. coli in this study. E. coli strains containing blaNDM–1-positive IncC plasmids belonged to a variety of STs, and no predominant STs were found, which were mainly geographically distributed in the United States and China. Other types of blaNDM–1-carrying plasmids included IncM2, IncN2, IncHI1, IncX3, and IncF. The large, broad host range IncC plasmids are important contributors to the spread of key antibiotic resistance genes, and over 200 complete sequences of IncC plasmids have been reported (Ambrose et al., 2018).
Bacterial mobile genetic elements, such as conjugative plasmids and transposons, have been highlighted as important vehicles for the dissemination of AMR, which play a central role in facilitating horizontal genetic exchange and therefore promoting the acquisition and spread of resistance genes (Partridge et al., 2018; Jiang et al., 2020). Currently, the genetic context of blaNDM is mainly focused on the insertion sequences and transposons, for example, ISAba125, IS26, and the composite transposon Tn125 (Yong et al., 2009; Göttig et al., 2013; Partridge et al., 2018; Wu and Feng, 2019). In fact, conjugation is a dominant mechanism of HGT, and bacterial genome comparisons highlight conjugative and mobilizable elements as vehicles for dissemination of pathogenesis and AMR determinants (Ramsay and Firth, 2017). Reports on the distribution of various conjugative and mobilizable blaNDM-positive carbapenem-resistant plasmids in E. coli and their conjugative transfer modules are currently scarce. Herein, we performed a comprehensive analysis and comparison of the conjugative transfer modules located on the 114 blaNDM-positive plasmids using the software oriTfinder (Li et al., 2018), the database oriTDB (Li et al., 2018) and the database SecReT4 (Bi et al., 2013). The oriTDB database recorded nine types of plasmid-borne oriT10 (Li et al., 2018). In our study, five types of oriT regions were identified, including A/C-type oriTs in conjugative IncC plasmids carrying blaNDM–1 genes, F-type oriTs in conjugative IncF plasmids bearing blaNDM–5 genes, L/M-type oriTs in mostly blaNDM–1-positive conjugative IncM2 plasmids, N-type oriTs in both blaNDM–1-positive conjugative IncN2 plasmids and blaNDM–4-positive mobilizable plasmids with IncFIA(HI1) replicon, as well as P-type oriTs in blaNDM–1-positive conjugative IncHI1 plasmids. The oriTDB database recorded eight main relaxase families11 (Li et al., 2018), and four relaxase families were found in our study. Relaxases belonging to the MOBP family were found in conjugative IncX3 plasmids and most of the conjugative IncM2 plasmids; Relaxases of MOBF family were found in conjugative IncF plasmids and IncN2 conjugative plasmids; MOBF relaxases were mostly found in conjugative IncC plasmids; the only one relaxase belonging to MOBC was found in one conjugative IncF plasmid. The oriTDB database recorded two main subfamilies of T4CPs12 (Li et al., 2018). In our study, most T4CPs of conjugative IncX3, IncC, and IncF plasmids belong to the VirD4/TraG subfamily, while the T4CPs of conjugative IncN2, IncM2, and IncHI1 plasmids belong to the TrwB/TraD subfamily. The database SecReT4 collected the five main types of T4SS gene clusters, including 18 kinds of systems13 (Bi et al., 2013). Our study demonstrated that four kinds of T4SS gene clusters were found in the blaNDM-positive plasmids of E. coli, including the Tra_F-type of T4SS distributed in the conjugative IncF, IncC, and IncHI1 plasmids, VirB-type T4SS distributed in the conjugative IncX3 plasmids, Tra_I-type of T4SS mostly in conjugative IncM2 plasmids, Trw-type of T4SS in conjugative IncN2 plasmids.
Conclusion
In this study, we analyzed the variants of blaNDM, replicon types, phylogenetic patterns, conjugative transfer modules, host STs, and geographical distributions of the 114 blaNDM-positive plasmids, from 3786 plasmids within 1346 complete whole genomes of E. coli from the GenBank database. Eight variants of blaNDM were found among the 114 blaNDM-positive plasmids, with blaNDM–5 and blaNDM–1 as the most dominant. The variant blaNDM–5 was mainly carried by the IncX3 plasmids and IncF plasmids in E. coli, the former were mainly geographically distributed in East Asia (especially in China) and the United States, and the latter were widely distributed all over the world, including East Asia, Southeast Asia, India, the United States, and some European countries. IncC plasmids were observed to be the predominant carriers of blaNDM–1 genes in E. coli, which were mainly geographically distributed in the United States and China. Other blaNDM–1-carrying plasmids also included IncM2 (mainly geographically distributed in the United Kingdom), IncN2 (distributed in Thailand), and IncHI1 (in Ghana and New Zealand). In addition, the overall picture of the conjugative and mobilizable blaNDM-positive plasmids in E. coli was described in our study. The eight representative plasmid patterns carrying blaNDM genes of E. coli was also validated with a larger data set (6054 plasmids of E. coli downloaded from the NCBI RefSeq database). This study provides important insights into the phylogeny and evolution of blaNDM-positive E. coli plasmids and further addresses their role in the acquisition and spread of resistance genes. However, the genetic diversity and characteristics of blaNDM -positive plasmids in other Enterobacteriaceae species need further study in the future.
Data Availability Statement
The original contributions presented in the study are included in the article/Supplementary Material, further inquiries can be directed to the corresponding author/s.
Author Contributions
ZLZ and ZLZ conceived the project. ZRZ and XBL analyzed all the data and wrote the manuscript. WL, GY, and WN performed data acquisition. MZ and LL provided some suggestions for manuscript writing. HG and XDL reviewed and edited the manuscript. All authors read and approved the final manuscript.
Funding
This work was supported financially by the grants from the State Key Development Program for Basic Research of China (Grant No. 2017YFA0205200), the National Natural Science Foundation of China (Grant Nos. 81901857 and 82002170), the Xiangshan Talent Project of Zhuhai People’s Hospital (Grant No. 2020XSYC-02), and the Natural Science Foundation of Guangdong Province of China (Grant No. 2021A1515010697).
Conflict of Interest
The authors declare that the research was conducted in the absence of any commercial or financial relationships that could be construed as a potential conflict of interest.
Publisher’s Note
All claims expressed in this article are solely those of the authors and do not necessarily represent those of their affiliated organizations, or those of the publisher, the editors and the reviewers. Any product that may be evaluated in this article, or claim that may be made by its manufacturer, is not guaranteed or endorsed by the publisher.
Supplementary Material
The Supplementary Material for this article can be found online at: https://www.frontiersin.org/articles/10.3389/fmicb.2021.729952/full#supplementary-material
Footnotes
- ^ https://www.ncbi.nlm.nih.gov/genome/browse/#!/prokaryotes/167/
- ^ https://ftp.ncbi.nih.gov/refseq/release/plasmid/
- ^ https://cge.cbs.dtu.dk/services/ResFinder/
- ^ https://cge.cbs.dtu.dk/services/PlasmidFinder/
- ^ http://www.stevekellylab.com/software/orthofinder
- ^ https://itol.embl.de/
- ^ https://bioinfo-mml.sjtu.edu.cn/oriTfinder/
- ^ https://bioinfo-mml.sjtu.edu.cn/oriTDB/index.php
- ^ https://cge.cbs.dtu.dk/services/MLST/
- ^ https://bioinfo-mml.sjtu.edu.cn/oriTDB/browse_oriT_type_p.php
- ^ https://bioinfo-mml.sjtu.edu.cn/oriTDB/browse_relaxase.php
- ^ https://bioinfo-mml.sjtu.edu.cn/oriTDB/browse_t4cp.php
- ^ https://db-mml.sjtu.edu.cn/SecReT4/browse_type.php
References
Albiger, B., Glasner, C., Struelens, M. J., Grundmann, H., and Monnet, D. L. (2015). Carbapenemase-producing Enterobacteriaceae in Europe: assessment by national experts from 38 countries, May 2015. Euro. Surveill. 20:pii=30062. doi: 10.2807/1560-7917.es.2015.20.45.30062
Ambrose, S. J., Harmer, C. J., and Hall, R. M. (2018). Evolution and typing of IncC plasmids contributing to antibiotic resistance in Gram-negative bacteria. Plasmid 99, 40–55. doi: 10.1016/j.plasmid.2018.08.001
Benson, D. A., Cavanaugh, M., Clark, K., Karsch-Mizrachi, I., Ostell, J., Pruitt, K. D., et al. (2018). GenBank. Nucleic Acids Res. 46, D41–D47. doi: 10.1093/nar/gkx1094
Bi, D., Liu, L., Tai, C., Deng, Z., Rajakumar, K., and Ou, H. Y. (2013). SecReT4: a web-based bacterial type IV secretion system resource. Nucleic Acids Res. 41, D660–D665. doi: 10.1093/nar/gks1248
Bortolaia, V., Kaas, R. S., Ruppe, E., Roberts, M. C., Schwarz, S., Cattoir, V., et al. (2020). ResFinder 4.0 for predictions of phenotypes from genotypes. J. Antimicrob. Chemother. 75, 3491–3500. doi: 10.1093/jac/dkaa345
Carattoli, A. (2011). Plasmids in Gram negatives: molecular typing of resistance plasmids. Int. J. Med. Microbiol. 301, 654–658. doi: 10.1016/j.ijmm.2011.09.003
Carattoli, A., and Hasman, H. (2020). PlasmidFinder and in silico pMLST: identification and typing of plasmid replicons in whole-genome sequencing (WGS). Methods Mol. Biol. 2075, 285–294. doi: 10.1007/978-1-4939-9877-7_20
de la Cruz, F., Frost, L. S., Meyer, R. J., and Zechner, E. L. (2010). Conjugative DNA metabolism in Gram-negative bacteria. FEMS Microbiol. Rev. 34, 18–40. doi: 10.1111/j.1574-6976.2009.00195.x
Emms, D. M., and Kelly, S. (2019). OrthoFinder: phylogenetic orthology inference for comparative genomics. Genome Biol. 20:238. doi: 10.1186/s13059-019-1832-y
Farhat, N., and Khan, A. U. (2020). Evolving trends of New Delhi Metallo-betalactamse (NDM) variants: a threat to antimicrobial resistance. Infect. Genet. Evol. 86:104588. doi: 10.1016/j.meegid.2020.104588
Göttig, S., Hamprecht, A. G., Christ, S., Kempf, V. A., and Wichelhaus, T. A. (2013). Detection of NDM-7 in Germany, a new variant of the New Delhi metallo-β-lactamase with increased carbapenemase activity. J. Antimicrob. Chemother. 68, 1737–1740. doi: 10.1093/jac/dkt088
Guducuoglu, H., Gursoy, N. C., Yakupogullari, Y., Parlak, M., Karasin, G., Sunnetcioglu, M., et al. (2018). Hospital outbreak of a colistin-resistant, NDM-1- and OXA-48-producing Klebsiella pneumoniae: high mortality from pandrug resistance. Microb. Drug Resist. 24, 966–972. doi: 10.1089/mdr.2017.0173
Han, R., Shi, Q., Wu, S., Yin, D., Peng, M., Dong, D., et al. (2020). Dissemination of carbapenemases (KPC, NDM, OXA-48, IMP, and VIM) among carbapenem-resistant Enterobacteriaceae isolated from adult and children patients in China. Front. Cell. Infect. Microbiol. 10:314. doi: 10.3389/fcimb.2020.00314
Hornsey, M., Phee, L., and Wareham, D. W. (2011). A novel variant, NDM-5, of the New Delhi metallo-β-lactamase in a multidrug-resistant Escherichia coli ST648 isolate recovered from a patient in the United Kingdom. Antimicrob. Agents Chemother. 55, 5952–5954. doi: 10.1128/aac.05108-11
Jiang, Y., Wang, Y., Hua, X., Qu, Y., Peleg, A. Y., and Yu, Y. (2020). Pooled plasmid sequencing reveals the relationship between mobile genetic elements and antimicrobial resistance genes in clinically isolated Klebsiella pneumoniae. Genomics Proteomics Bioinformatics 18, 539–548. doi: 10.1016/j.gpb.2020.12.002
Karlowsky, J. A., Lob, S. H., Kazmierczak, K. M., Badal, R. E., Young, K., Motyl, M. R., et al. (2017). In vitro activity of imipenem against carbapenemase-positive Enterobacteriaceae isolates collected by the SMART Global Surveillance Program from 2008 to 2014. J. Clin. Microbiol. 55, 1638–1649. doi: 10.1128/jcm.02316-16
Larsen, M. V., Cosentino, S., Rasmussen, S., Friis, C., Hasman, H., Marvig, R. L., et al. (2012). Multilocus sequence typing of total-genome-sequenced bacteria. J. Clin. Microbiol. 50, 1355–1361. doi: 10.1128/jcm.06094-11
Letunic, I., and Bork, P. (2016). Interactive tree of life (iTOL) v3: an online tool for the display and annotation of phylogenetic and other trees. Nucleic Acids Res. 44, W242–W245. doi: 10.1093/nar/gkw290
Li, X., Xie, Y., Liu, M., Tai, C., Sun, J., Deng, Z., et al. (2018). oriTfinder: a web-based tool for the identification of origin of transfers in DNA sequences of bacterial mobile genetic elements. Nucleic Acids Res. 46, W229–W234. doi: 10.1093/nar/gky352
Mouftah, S. F., Pál, T., Darwish, D., Ghazawi, A., Villa, L., Carattoli, A., et al. (2019). Epidemic IncX3 plasmids spreading carbapenemase genes in the United Arab Emirates and worldwide. Infect. Drug Resist. 12, 1729–1742. doi: 10.2147/idr.s210554
Nordmann, P., Poirel, L., Walsh, T. R., and Livermore, D. M. (2011). The emerging NDM carbapenemases. Trends Microbiol. 19, 588–595. doi: 10.1016/j.tim.2011.09.005
O’Leary, N. A., Wright, M. W., Brister, J. R., Ciufo, S., Haddad, D., McVeigh, R., et al. (2016). Reference sequence (RefSeq) database at NCBI: current status, taxonomic expansion, and functional annotation. Nucleic Acids Res. 44, D733–D745. doi: 10.1093/nar/gkv1189
Partridge, S. R., Kwong, S. M., Firth, N., and Jensen, S. O. (2018). Mobile genetic elements associated with antimicrobial resistance. Clin. Microbiol. Rev. 31, e00088–17. doi: 10.1128/cmr.00088-17
Pérez-Vázquez, M., Sola Campoy, P. J., Ortega, A., Bautista, V., Monzón, S., Ruiz-Carrascoso, G., et al. (2019). Emergence of NDM-producing Klebsiella pneumoniae and Escherichia coli in Spain: phylogeny, resistome, virulence and plasmids encoding blaNDM-like genes as determined by WGS. J. Antimicrob. Chemother. 74, 3489–3496. doi: 10.1093/jac/dkz366
Ramsay, J. P., and Firth, N. (2017). Diverse mobilization strategies facilitate transfer of non-conjugative mobile genetic elements. Curr. Opin. Microbiol. 38, 1–9.
Ravi, A., Valdés-Varela, L., Gueimonde, M., and Rudi, K. (2018). Transmission and persistence of IncF conjugative plasmids in the gut microbiota of full-term infants. FEMS Microbiol. Ecol. 94:fix158. doi: 10.1093/femsec/fix158
Rozwandowicz, M., Brouwer, M. S. M., Fischer, J., Wagenaar, J. A., Gonzalez-Zorn, B., Guerra, B., et al. (2018). Plasmids carrying antimicrobial resistance genes in Enterobacteriaceae. J. Antimicrob. Chemother. 73, 1121–1137. doi: 10.1093/jac/dkx488
Smillie, C., Garcillán-Barcia, M. P., Francia, M. V., Rocha, E. P., and de la Cruz, F. (2010). Mobility of plasmids. Microbiol. Mol. Biol. Rev. 74, 434–452. doi: 10.1128/mmbr.00020-10
Sugawara, Y., Akeda, Y., Hagiya, H., Sakamoto, N., Takeuchi, D., Shanmugakani, R. K., et al. (2019). Spreading patterns of NDM-producing Enterobacteriaceae in clinical and environmental settings in Yangon, Myanmar. Antimicrob. Agents Chemother. 63, e01924–18. doi: 10.1128/aac.01924-18
Wirth, T., Falush, D., Lan, R., Colles, F., Mensa, P., Wieler, L. H., et al. (2006). Sex and virulence in Escherichia coli: an evolutionary perspective. Mol. Microbiol. 60, 1136–1151. doi: 10.1111/j.1365-2958.2006.05172.x
Wu, W., and Feng, Y. (2019). NDM metallo-β-lactamases and their bacterial producers in health care settings. Clin. Microbiol. Rev. 32, e00115–18. doi: 10.1128/cmr.00115-18
Yong, D., Toleman, M. A., Giske, C. G., Cho, H. S., Sundman, K., Lee, K., et al. (2009). Characterization of a new metallo-beta-lactamase gene, blaNDM–1, and a novel erythromycin esterase gene carried on a unique genetic structure in Klebsiella pneumoniae sequence type 14 from India. Antimicrob. Agents Chemother. 53, 5046–5054. doi: 10.1128/aac.00774-09
Keywords: Escherichia coli, New Delhi metallo-β-lactamase, plasmid, conjugative, mobilizable
Citation: Zhang Z, Guo H, Li X, Li W, Yang G, Ni W, Zhan M, Lu L, Zhang Z, Li X and Zhou Z (2021) Genetic Diversity and Characteristics of blaNDM-Positive Plasmids in Escherichia coli. Front. Microbiol. 12:729952. doi: 10.3389/fmicb.2021.729952
Received: 24 June 2021; Accepted: 11 October 2021;
Published: 16 November 2021.
Edited by:
Ziad Daoud, Central Michigan University, United StatesReviewed by:
Haiquan Kang, Affiliated Hospital of Xuzhou Medical University, ChinaHua Zhou, Zhejiang University, China
Jun Sung Hong, Yonsei University College of Medicine, South Korea
Zhihai Liu, Qingdao Agricultural University, China
Bahareh Hajikhani, Shahid Beheshti University of Medical Sciences, Iran
Copyright © 2021 Zhang, Guo, Li, Li, Yang, Ni, Zhan, Lu, Zhang, Li and Zhou. This is an open-access article distributed under the terms of the Creative Commons Attribution License (CC BY). The use, distribution or reproduction in other forums is permitted, provided the original author(s) and the copyright owner(s) are credited and that the original publication in this journal is cited, in accordance with accepted academic practice. No use, distribution or reproduction is permitted which does not comply with these terms.
*Correspondence: Zhenlin Zhang, am51ZG9jdG9yQDE2My5jb20=; Xiaobin Li, eGlhb2JpbmxpQHNqdHUuZWR1LmNu; Zhiling Zhou, emhvdXpsQGFsaXl1bi5jb20=
†These authors have contributed equally to this work