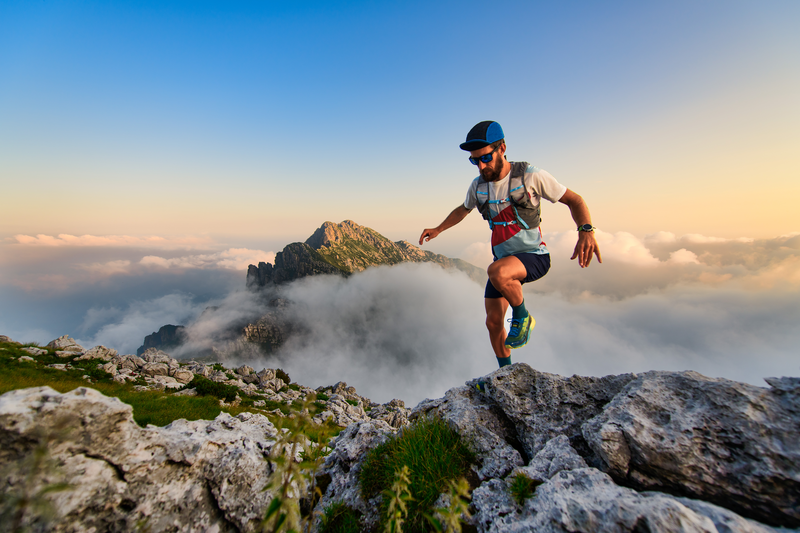
94% of researchers rate our articles as excellent or good
Learn more about the work of our research integrity team to safeguard the quality of each article we publish.
Find out more
ORIGINAL RESEARCH article
Front. Microbiol. , 04 October 2021
Sec. Virology
Volume 12 - 2021 | https://doi.org/10.3389/fmicb.2021.729914
This article is part of the Research Topic Influenza Viruses: Infection, Pathogenesis, and Host Responses View all 12 articles
Influenza neuraminidase (NA) is able to induce cross-subtype immunity and is considered as a promising target for the development of universal influenza vaccines. However, commercial influenza vaccines only induced low NA-specific immune responses due to the low amounts and the denatured conformation of NA proteins in current inactivated or split influenza vaccines. Here we investigated the protective efficacy of recombinant tetrameric and monomeric NA proteins to determine whether the conformation contributed to induce protective immunity. We found that H1N1PR8NA tetramer (NAtet) could provide complete homologous protection against A/PR8 (H1N1) virus infection in mice, while the protection of H1N1PR8NA monomer (NAmono) was moderate. Higher levels of NA-reactive binding and inhibition antibodies and less weight loss were observed in the H1N1PR8NAtet-vaccinated group. Similarly, H5N1VNNAtet immunization exhibited a preferable heterologous protection than H5N1VNNAmono, but neither H7N9SHNAtet nor H7N9SHNAmono vaccination showed heterosubtypic protection. We also compared the effect of three adjuvants, aluminum, 3′3′-cGAMP (cGAMP), and Poly(I:C), on the humoral response and protective efficacy induced by H1N1PR8NAtet. H1N1PR8NAtet protein adjuvanted with aluminum was observed to exhibited better capacity in inducing NA-specific humoral immunity and preventing weight loss than with cGAMP or Poly(I:C). In conclusion, our data demonstrate that tetrameric NA with natural conformation is required to induce protective anti-NA immunity. The NA tetramer could provide homologous protection and subtype-specific cross-protection. In addition, the aluminum adjuvant is preferable in recombinant NA protein vaccination.
The seasonal epidemics and less frequently global pandemics of influenza viruses result in high morbidity and mortality every year. Vaccination is the most effective way to prevent influenza circulation. The currently licensed vaccines, including inactivated and attenuated influenza vaccines, induce protection mainly by eliciting neutralizing antibodies (NAbs) against the major glycoprotein, hemagglutinin (HA), of influenza virus (Vogel and Manicassamy, 2020). Vaccine efficacy depends on the antigenic similarities of HA proteins between the vaccine and circulating strains. However, the continuous and extensive antigenic variation of HA protein allows influenza viruses to easily escape from the protection of vaccines. The vaccine components need to be updated yearly based on the prediction of circulating influenza strains. The mismatch of vaccine and circulating strains, as in the 2009–2010 and 2017–2018 influenza seasons, resulted in a significant increase of influenza-related morbidity and mortality. A universal vaccine may overcome the shortage of the current influenza vaccines and provide cross-protection against multiple influenza subtypes.
Neuraminidase (NA) protein is the second most abundant glycoprotein on the membrane of influenza virion. The native influenza NA protein is a tetramer with enzymatic activity (Saito et al., 1995; Wu et al., 2009; Da Silva et al., 2013) that can cleave off the terminal sialic acid from N-linked glycans to facilitate viral release and transmission (Krammer et al., 2018; Eichelberger and Monto, 2019). Serologic studies showed that individuals with higher NA-specific antibody titers were less likely to be infected by influenza virus with the same NA subtypes (Murphy et al., 1972; Monto and Kendal, 1973). NA inhibition (NAI) antibody titers were independently correlated with lower morbidity and decreased viral shedding in influenza-infected individuals (Couch et al., 2013; Stadlbauer et al., 2019). Although there are 11 different subtypes of NA proteins, N10 and N11 NA are unique to bats (Tong et al., 2012, 2013). The antigenic variation of NA protein is relatively low compared to that of HA protein (Couch et al., 2013; Stadlbauer et al., 2019). Furthermore, NA-specific monoclonal antibodies isolated from influenza-infected individuals provide cross-protection against multiple influenza virus strains (Chen et al., 2018; Stadlbauer et al., 2019; Yasuhara et al., 2019). Therefore, NA protein is considered a candidate for the development of universal influenza vaccines. Recent studies have shown that expressed or purified NA proteins could induce robust NA-based immunity and protect against influenza virus infection (Liu et al., 2015; Wohlbold et al., 2015; McMahon et al., 2020), indicating that the NA protein is immunogenic in both animal models and humans. In contrast, commercial inactivated and split influenza vaccines only induced low levels of NA-specific immune response (Wohlbold et al., 2015; Krammer et al., 2018). Several factors were suggested to contribute to the low immunogenicity of NA in inactivated and split influenza vaccines. First, the amount of NA proteins is relatively lower than HA in the vaccine formula (Sridhar et al., 2015; Wohlbold et al., 2015); second, NA seems to have immune subdominance to HA when both antigens were administered (Johansson et al., 1987; Krammer et al., 2018); and third, the NA conformation in the vaccine formula may be changed during vaccine manufacturing (McMahon et al., 2020).
In this paper, we investigated the factors that potentially affect the immune response and protective efficacy induced by NA protein in BALB/c mouse models. We compared the difference of the protective efficacy between tetrameric and monomeric NA proteins and investigated the humoral responses induced by tetrameric and monomeric NA proteins. We also assessed the influence of adjuvants on the humoral response and protective efficacy induced by H1N1PR8NAtet tetramer.
Madin Darby canine kidney (MDCK) and human embryonic kidney 293 (HEK293T) cells were obtained from the American Type Culture Collection and grown in complete high-glucose Dulbecco’s modified Eagle’s medium (DMEM, HyClone) supplemented with antibiotics (100 units/ml penicillin and 100 μg/ml streptomycin, HyClone) and 10% fetal bovine serum (FBS, Gibco). Expi293F (Thermo Fisher Scientific) cells were grown in SMM 293-TII expression medium (Sino Biological Inc.).
The influenza A virus (IAV) used in this study was the mouse-adapted strain A/Puerto Rico/8/1934 (H1N1). The IAV was propagated in MDCK cells in serum-free DMEM media in the presence of 1 μg/ml TPCK-trypsin (Sigma-Aldrich). The median tissue culture infective dose and median lethal dose (LD50) of viruses were calculated by the Reed and Munch method.
Recombinant NA proteins derived from A/Puerto Rico/8/1934 (H1N1), A/Shanghai/37T/2009 (H1N1), A/Hong Kong/16/68 (H3N2), A/Vietnam/1204 (H5N1), and A/Shanghai/4664T/2013 (H7N9)—referred to as H1N1PR8NA, H1N1p09NA, H3N2HKNA, H5N1VNNA, and H7N9SHNA, respectively—were expressed in Expi293F cells and purified by Ni-nitrilotriacetic acid (NTA) beads (GE Healthcare). Briefly, the NA ectodomains with additional N-terminal Igκ-light chain secretion sequence, followed by a hex-histidine tag (HHHHHH), a human vasodilator stimulating phosphoprotein (VASP) tetramerization domain (SSSDYSDLQRVKQELLEEVKKELQKVKEEIIEAFVQELRKRG), and a thrombin cleavage site (SLVPRGSPSRS) were constructed into eukaryotic expressing plasmid pcDNA3.1 to express NA tetramer (NAtet). The monomeric NA (NAmono) proteins were constructed in the same way as NAtet but without the VASP domain. Recombinant NA proteins were purified from the supernatant of transiently transfected Expi293F cells by Ni-NTA beads. The concentration of proteins was adjusted to 1 mg/ml with phosphate-buffered saline (PBS) and frozen at −80°C.
Recombinant NA proteins were then analyzed by Western blotting. Briefly, 2 μg of NA were mixed with 4× SDS-loading buffer containing 10% β-mercaptoethanol. The samples were heated for 10 min at 98°C and were afterward loaded on an SDS gradient gel (4–20% Precast Protein Improve Gels, Yeasen Biotechnology Inc.). The gel was run for 100 min at 120 V, and Western blotting transfer was performed. Following the transfer onto the nitrocellulose membrane, the membrane was blocked with TBS with 0.1% Tween 20 (TBS-T) containing 5% milk powder for 2 h at room temperature (RT). Then, mouse anti-HIS primary antibody (Younuoke Biotechnology Inc.) was added for 12 h at 4°C (1:1,000 dilution in TBS-T containing 1% milk). The membrane was washed three times with TBS-T after 12 h, and a secondary goat anti-mouse horse radish peroxidase (HRP) antibody (Jackson Immuno Research) was added for 1 h at RT (diluted 1:1,000 in PBS-T containing 1% milk). The membrane was then washed three times with TBS-T before it was visualized using Pierce Chemiluminescence (ECL) Western Blotting Substrate (as per the instructions of the manufacturer) on Tanon-5200 Chemiluminescent Imaging System (Tanon Science and Technology).
The extent of tetramerization and/or multimerization was investigated by cross-linking of NA with glutaraldehyde (Sigma-Aldrich). Briefly, 5 μg of NA was diluted in 25 μl of PBS in the presence of 0.3 mM of glutaraldehyde cross-linker. The mixture was incubated at RT for 5 min, and then glutaraldehyde was quenched by adding 1 M Tris–HCl buffer (pH 8.0) to a final concentration of 50 mM. Afterward, the protein samples were loaded on 4–20% SDS gradient gel. The gel was run for 60 min at 180 V and confirmed by Coomassie staining.
The enzymatic activity of recombinant NA proteins was determined by the cleavage of two specific substrates of NA, 2′-(4-methylumbelliferyl)-α-D-N-acetylneuraminic acid (MUNANA) (Job et al., 2018; Ju et al., 2018) and fetuin (Prevato et al., 2015; Biuso et al., 2019) as previously described, with minor modifications.
For the MUNANA-based enzymatic activity assay, twofold gradient diluted recombinant NA proteins that range from 0.0015625 to 1.6 μg/ml in morpholine ethanesulfonic acid (MES) buffer (32.5 mM MES and 2 mM CaCl2, pH 6.5) were incubated with 20 mM MUNANA (Sigma-Aldrich) at 37°C for 40 min. The reaction was stopped by adding a stopping buffer (0.2 M glycine and 0.2 M NaOH, pH 10.7), and the degree of fluorescence was detected by EnSight Multimode plate reader (PerkinElmer).
For the fetuin-based enzyme-linked lectin assay (ELLA), MaxiSorp Nunc-immuno 96-well plates were coated with 100 μl of fetuin (Sigma-Aldrich) at a concentration of 50 μg/ml and refrigerated at 4°C overnight. The plates were blocked with 200 μl blocking buffer (PBS containing 1% FBS and 5% dry milk) for 1 h at RT and washed six times with PBS-T solution. Following the blocking, 50 μl of the sample diluent [Dulbecco’s phosphate buffered saline (DPBS) with 1% bovine serum albumin and 0.5% Tween 20] and 50 μl of serially diluted recombinant NA protein or purified virus were then added to the fetuin-coated plates and mixed well. The plates were then incubated for 14–16 h at 37°C before being washed six times with PBS-T. After the extensive wash, the NA enzymatic activity was detected by horse-radish peroxidase-labeled peanut agglutinin (Sigma Aldrich) and developed with the 3,3′,5,5′-tetramethylbenzidine substrate (Sigma-Aldrich). The reaction was stopped by the addition of 2 N H2SO4 after 20 min of incubation. The optical density (OD) values were read at 450 nm on a Multiskan FC plate reader (Thermo Fisher Scientific).
Female BALB/c mice (6–8 weeks old) from the Laboratory Animal Center of Shanghai Public Health Clinical Center (SHPHCC) were used for all animal experiments. The protocols were reviewed and approved by the Ethics Committee of SHPHCC (approval no. 2019-A019-01/02).
To compare the immunogenicity and protective efficacy of tetrameric and monomeric NA proteins, the mice were intraperitoneally (i.p.) immunized with 20 μg H1N1PR8NAtet, H5N1VNNAtet, H7N9SHNAtet, H1N1PR8NAmono, H5N1VNNAmono, and H7N9SHNAmono, respectively. The antigens were diluted in 100 μl PBS and mixed with aluminum adjuvant (1:1). The mice were immunized with PBS as control. To investigate the influence of adjuvants on the immunogenicity of NA tetramers, the mice were intraperitoneally immunized with 20 μg H1N1PR8NAtet protein mixed with aluminum (i.p.), 3′3′-cGAMP (cGAMP; intradermally, i.d.) and Poly(I:C) (i.p.), respectively. Aluminum is a strong inducer of Th2 responses (Rudicell et al., 2019). cGAMP is a Th1 immune response inducer as an “ideal” adjuvant for cutaneous vaccination (Wang et al., 2016). The TLR3 agonist Poly(I:C) also promotes Th1-dominant immunity, and it is commonly used as intraperitoneal immune adjuvant (Moriyama et al., 2017). These three adjuvants were all purchased from InvivoGen. The mice were boosted with the same immunogen 2 weeks later.
Sera were collected 1 week after the final immunization to detect NA-specific antibodies. The mice were intranasally challenged with 5 LD50 of A/Puerto Rico/8/34 (H1N1) virus 2 weeks after the final immunization to evaluate the protective efficacy. The weight loss and survival rates of mice were monitored for 14 days after the challenge.
The NA-specific antibodies were measured by enzyme-linked immunosorbent assay (ELISA). To explore the immunogenicity of tetrameric and monomeric NA proteins, the sera samples which were collected 1 week after the final immunization were tested for NA-reactive antibodies by ELISA, including NA-specific binding antibodies to the respective immunogen, cross-subtype binding antibodies against NA of A/PR8 (H1N1), and NAI antibodies. The subtypes of NA-specific antibodies were also analyzed. Briefly, MaxiSorp Nunc-immuno 96-well plates (Thermo Fisher Scientific) were coated with 2 μg/ml NA proteins (100 μl/well) in carb/bicarb coating buffer (10 mM Na2CO3, 40 mM NaHCO3, and pH 9.6) at 4°C overnight. To detect the NA-specific antibodies, the respective immunogen to sera of immunized mice was coated. To detect the cross-binding activity of mice sera, the tetrameric NA protein of H1N1PR8NAtet was coated. The plates were washed three times with PBS-T (PBS containing 0.05% Tween 20) and blocked with blocking buffer (PBS containing 1% FBS and 5% dry milk) for 1 h at RT. The sera were fourfold serially diluted starting at 1:100 in disruption buffer (PBS containing 5% FBS, 2% BSA, and 1% Triton X-100). Then, 50 μl of diluted serum was added to each well and incubated for 1 h at RT. After three times of washing with PBS-T, bound antibodies were detected by horseradish peroxidase-labeled goat-anti mouse IgG antibody (Jackson Immuno Research) and substrate ABTS (Thermo Fisher Scientific). The OD was measured at 405 nm on a Multiskan FC plate reader (Thermo Fisher Scientific). The isotypes of NA-specific antibodies were determined by ELISA with Mouse Monoclonal Antibody Isotyping Reagents (Sigma-Aldrich) following the instructions of the manufacturer. The antibody titers were defined as the highest sera dilution at which the OD values were twice of those by the control sera.
The plates were coated and blocked as fetuin-based ELLA as described above. While the plates were blocked, pre-challenge mouse sera were fourfold serially diluted starting at 1:40 in PBS in a new U-bottom, 96-well plate. Then, the split A/PR8 (H1N1) virus was added to each well of the serially diluted serum plate, and the plates were incubated at 37°C for 1 h. The amount of split A/PR8 (H1N1) virus used in the NAI assay corresponded to 90% of the maximum signal. After incubation, 100 μl of the serum/virus mixture was added on the blocked fetuin-coated plates and incubated for 16–18 h at 37°C. The remainder of the NAI assay was performed as ELLA as described above. The values of test wells obtained from the plate reader were divided by the average value for virus-only control wells and then multiplied by a factor of 100 to obtain the NA activity. Percent inhibition was calculated by subtracting the NA activity from 100.
The survival rate was compared by log-rank test. Antibody titers, viral titers, and body weights among groups were compared by one- or two-way ANOVA. A P-value < 0.05 was considered significant. All the statistical analyses were performed by GraphPad Prism, version 8.00 (GraphPad Software, San Diego, CA, United States).
The NA tetramers and monomers were expressed using Expi293F cells and purified by Ni-NTA. The proteins were analyzed by Western blotting (Figures 1A,B) and cross-linking SDS-PAGE (Figures 1C,D). The bands of NA tetramers stabilized by the VASP domain were observed as monomers on Western blotting (Figure 1A), while they showed bands consistent with tetramers when cross-linked (Figure 1C). The monomeric NA proteins exhibited as monomers on Western blotting (Figure 1B) and showed some level on dimer or tetramer formation when cross-linked but was mostly monomeric (Figure 1D). The enzymatic activity of NA tetramers and monomers was characterized using two NA-specific substrates, MU-NANA and fetuin, respectively. These assays measured the amount of 4-methylumbelliferone cleaved by the influenza virus NA from the MUNANA or the amount of NA cleaved-fetuin from the intact fetuin. For the results of MUNANA-based enzymatic activity assays, as shown in Figures 1E,F, the NA tetramers exhibited enzymatic activity, while the NA monomers did not. The same results were also observed in fetuin-based enzymatic activity assays; the tetrameric NA showed high enzymatic activity, but the monomeric NA did not (Figures 1G,H). Taken together, these results suggest that the enzymatic activity depends on the tetrameric conformation of the NA proteins.
Figure 1. Expression, purification, and characterization of recombinant neuraminidase (NA) proteins. Tetrameric and monomeric NA proteins derived from A/Puerto Rico/8/1934 (H1N1), A/Shanghai/37T/2009 (H1N1), A/Hong Kong/16/68 (H3N2), A/Vietnam/1204 (H5N1), and A/Shanghai/4664T/2013 (H7N9) were expressed by the Expi293F cell expression system and purified by Ni-nitrilotriacetic acid (NTA). (A,B) Purified NA proteins were confirmed by Western blotting. (C,D) Cross-linking SDS-PAGE of tetrameric and monomeric recombinant NA proteins. (E,F) MUNANA-based enzymatic activity assays were used to determine the enzymatic activity of recombinant NA proteins. (G,H) Fetuin-based enzymatic activity assays were used to determine the enzymatic activity of recombinant NA proteins.
To investigate the protective efficacy of tetrameric NA vs. monomeric NA, the mice were immunized with NA tetramers or monomers as per the schedule shown in Figure 2A. As shown in Figures 2B–G, all the mice in the PBS control group showed signs of influenza, such as huddling or ruffled fur, from 2 to 3 days and died after the challenge with A/PR8 (H1N1) virus. The median survival days in the control group was 7 days. Vaccination with either H1N1PR8NAtet or H1N1PR8NAmono significantly protected the mice against the lethal dose of the A/PR8 (H1N1) influenza challenge (Figures 2B,C). All the H1N1PR8NAtet-immunized mice and 6/8 of H1N1PR8NAmono-immunized mice survived the influenza virus challenge (Figure 2B). Although the survival rates were not significantly different between the tetramer- and monomer-immunized mice, the mice immunized with H1N1PR8NAtet showed significantly less weight loss compared with those immunized with H1N1PR8NAmono (Figure 2C).
Figure 2. Protective efficacy of tetrameric neuraminidase (NA) vs. monomeric NA. (A) The experimental design for immunization and challenge studies. Six- to eight-week-old BALB/c mice (n = 8 in each group) were immunized twice at 2-week interval with 20 μg of H1N1PR8NAtet, H5N1VNNAtet, H7N9SHNAtet, H1N1PR8NAmono, H5N1VNNAmono, and H7N9SHNAmono proteins adjuvanted with aluminum (i.p.), respectively. Sera were collected at 1 week after the final immunization; then, the mice were challenged with 5 LD50 of A/PR8 (H1N1) virus intranasally (i.n.) at 2 weeks after the final immunization. (B–G) Survival rates (B,D,F) and weight loss (C,E,G) were monitored for 14 days post-infection.
The cross-subtype protection was evaluated in mice that were immunized with H5N1 and H7N9 NA by heterosubtypic challenge with A/PR8 (H1N1) virus. As shown in Figures 2D,E, immunization with H5N1 NA tetramer significantly improved the survival of mice post-challenge with the A/PR8 (H1N1) virus. Then, 75% (6/8) of the mice that were immunized with H5N1VNNAtet tetramer survived, while all the mice in the control group died. However, the protection induced by H5N1 NA monomer is significantly weaker than that induced by tetramer (p < 0.05, log-rank test), with only 37.5% (3/8) of H5N1VNNAmono-immunized mice surviving. No protection was observed in mice that were immunized with either H7N9SHNAtet or H7N9SHNAmono proteins (Figures 2E,F), indicating that protection induced by the NA protein is subtype-specific. Similar results were observed in tetrameric H1N1 and H3N2 NA proteins immunization. Tetrameric H1N1pdm09NA induced moderate cross-protection against A/PR8 (H1N1) virus infection while H3N2HKNA not (Supplementary Figure S2).
To explore the immunogenicity of tetrameric and monomeric NA proteins, the sera samples which were collected 1 week after the final immunization (Figure 2A) were tested for NA-reactive antibodies, including NA-specific binding antibodies to the respective immunogen, cross-subtype binding antibodies against NA of A/PR8 (H1N1), and NAI antibodies. The subtype of NA-specific antibodies was also analyzed. We firstly evaluated NA-specific binding antibodies against their respective immunogens by ELISA. As shown in Figure 3A, both of the H1N1 NA tetramer and monomer induced high levels of binding antibodies to the immunogens after two immunizations in mice. The H1N1 NA tetramer induced higher binding antibodies compared to H1N1 NA monomers (1:25,600 vs. 1:6,400). Similar results were observed between the groups of H5N1VNNAtet and H5N1VNNAmono (Figure 3B) as well as groups of H7N9SHNAtet and H7N9SHNAmono (Figure 3C). These results suggested that the NA tetramer with natural conformation has higher immunogenicity than the monomer.
Figure 3. Humoral response induced tetrameric and monomeric neuraminidase (NA) proteins. The experimental design for immunization and challenge studies was identical to that detailed in the legend of Figure 2A. Serum were collected at 1 week after the final immunization. (A–C) The sera of mice vaccinated, respectively, with H1N1PR8NAtet and H1N1PR8NAmono (A), H5N1VNNAtet and H5N1VNNAmono (B), and H7N9SHNAtet and H7N9SHNAmono (C) proteins were tested for NA-specific binding antibody levels to the respective immunogen via ELISA. (D–F) The same sera whose results are shown in panels (A–C) were tested for cross-binding activity to H1N1PR8NAtet protein via ELISA, respectively. (G–I) The same sera mentioned above were tested for NA inhibition (NAI) activity against NA of A/PR8 (H1N1) via enzyme-linked lectin assay (ELLA), respectively.
Then, we evaluated the cross-subtype binding antibodies against H1N1 PR8 NA induced by the H5N1 NA and H7N9 NA proteins. The cross-binding of sera from different mice groups against the H1N1PR8NAtet protein was tested by ELISA. Both H1N1 PR8 NA and H5N1 NA induced binding antibodies to H1N1PR8NAtet (Figures 3D,E). The antibody levels induced by NA tetramer were higher than those by the monomer as previously indicated. The sera from mice immunized with H7N9 NA tetramer weakly reacted with H1N1PR8NAtet, while the sera from mice immunized with H7N9 NA monomer did not react with H1N1PR8NAtet (Figure 3F). These results indicated that cross-binding antibodies are related to protection, while antibodies induced by NA proteins were mainly subtype-specific.
We also investigated the subtypes of NA-specific antibodies by isotyping ELISA assays. All tetrameric and monomeric NA proteins mainly induced IgG1 isotype of NA-specific antibodies (Supplementary Figure S1), indicating that the NA protein elicited a Th2-directed immune response.
Since it has been reported that the NA-induced protection was correlated with NAI antibodies (Couch et al., 2013; Memoli et al., 2016; Gilchuk et al., 2019; Stadlbauer et al., 2019; Vogel and Manicassamy, 2020), we evaluated the NAI activity of sera by ELLA. As shown in Figure 3G, sera from eight mice immunized with the H1N1PR8NAtet tetramer exhibited a higher NAI activity against the NA of A/PR8 (H1N1) than the six survivors that were immunized with H1N1PR8NAmono. In contrast, the NAI activity was barely detectable in sera from two deceased mice in this group (Figure 3G). The NAI activity against the NA of A/PR8 (H1N1) was only weakly detected in mice that were immunized with H5N1 NA tetramer and monomer (Figure 3H) and almost not detected in mice that were immunized with H7N9 NA proteins (Figure 3I), suggesting that NAI antibodies may be related to protection during influenza virus infection. Taken together, those results mentioned above indicated that NAtet exhibited better ability in inducing NA- specific-, cross- binding-, and NAI antibodies. Furthermore, NAtet proteins provide better homogeneous protection or cross-protection than NAmono.
We compared the effect of three adjuvants, aluminum, cGAMP, and Poly(I:C), on the protective efficacy and humoral responses induced by the H1N1PR8NAtet tetramer. The immunization and challenge schedule is shown in Figure 4A. We found that complete protection against homologous A/PR8 (H1N1) virus challenge was observed in groups of aluminum or cGAMP (Figures 4B,C). The protection efficacy in the Poly(I:C) group was lower than those in the aluminum and cGAMP groups, as indicated by both the lower survival rates and weight loss (Figures 4B,C). We then evaluated the antibody responses in mice with different adjuvants of groups. Aluminum-adjuvant H1N1PR8NAtet induced the highest NA-specific binding antibodies and NAI antibodies (Figures 4D,E). cGAMP-adjuvant H1N1PR8NAtet induced higher NA-specific binding antibodies than Poly(I:C)-adjuvant, but both adjuvants induced a similar level of NAI antibodies (Figures 4D,E). These results together suggested that tetrameric NA protein with natural conformation adjuvanted with aluminum elicited better protection than with cGAMP or Poly(I:C).
Figure 4. Effect of three adjuvants—aluminum, cGAMP, and Poly(I:C)—on the protective efficacy and humoral response induced by H1N1PR8NAtet tetramer. (A) Six- to eight-week-old BALB/c mice were immunized twice at 2-week interval with 20 μg of H1N1PR8NAtet protein adjuvanted with aluminum (n = 8, i.p.), cGAMP (n = 4, i.c.), or Poly(I:C) (n = 4, i.p.), respectively. The mice were immunized with phosphate-buffered saline (PBS) as control (n = 4, i.p.). Sera were collected at 1 week after the final immunization; then, the mice were challenged with 5 LD50 of A/PR8 (H1N1) virus intranasally (i.n.) at 2 weeks after the final immunization. (B,C) The survival rates (B) and weight loss (C) were monitored for 14 days post-infection. (D) The serum from individual mice in each experimental group was tested for neuraminidase (NA)-specific antibody levels against H1N1PR8NAtet protein via ELISA. (E) The NAI activity of mice serum against NA of A/PR8 (H1N1) was tested via enzyme-linked lectin assay (ELLA).
Influenza NA protein has been considered a potential target to develop universal vaccines that can provide cross-protection against different subtypes of influenza virus. The current commercial influenza vaccines are not efficient at inducing NA-specific protective immune response (Wohlbold et al., 2015; Chen et al., 2018; McMahon et al., 2020). Several NA-based vaccines, including recombinant NA proteins (Martinet et al., 1997; Bosch et al., 2010; Subathra et al., 2014; Liu et al., 2015; Wohlbold et al., 2015), DNA vaccines (Sandbulte et al., 2007), and virus-like particles (VLP) vaccines (Quan et al., 2012; Smith et al., 2017; Kim et al., 2019), have been evaluated in experimental animals and successfully provoked protection against influenza viruses. However, the mechanism of NA-induced protection has not been completely understood. Here we evaluated the immune response and protective efficacy of recombinant NA proteins. We found that the NA protein is highly immunogenic and induced protection against influenza viruses. Compared to the NA monomer, the NA tetramer is more immunogenic to induce higher NA-specific and cross-reactive biding antibodies, which are related to protection. The NA-reactive antibodies that bound to the native NA tetramers of the live virus might also play an important a role in inhibiting the viral release and spread of the infection. However, the protection is NA-subtype specific. H7N9SHNAtet tetramer could not provide heterosubtypic protection against A/PR8 (H1N1) virus infection. This finding is supported by the report that vaccination with the recombinant NA protein of A/PR8 (H1N1) could provide complete homologous protection against A/PR8 (H1N1) virus infection but not heterosubtypic protection against H3N2 virus (Wohlbold et al., 2015). Since there are 11 known NA subtypes, further studies may be required to explore an immune strategy, such as sequential or mixed immunization, to induce a broad immune response against all the 11 NA subtypes.
It was suggested that the protection induced by the NA protein was mediated by NAI antibodies (Couch et al., 2013; Memoli et al., 2016; Gilchuk et al., 2019; Stadlbauer et al., 2019; Vogel and Manicassamy, 2020). In this paper, we found that the protection induced by the NA protein was correlated with NAI antibodies. High NAI antibodies were detected in sera from the mice that survived the challenge, while NAI antibodies were undetectable in deceased mice. We also observed that NAI antibodies were strain-specific, while H5N1VNNAtet induced cross-protection against A/PR8 (H1N1) virus infection without provoking cross-reactive NAI antibodies, which probably depended on the differences among the epitopes around the active enzyme sites (Liu et al., 2015). However, H5N1VNNAtet induced high titers of subtype-specific binding antibodies to the NA protein of A/PR8 (H1N1) virus. Those cross-binding antibodies may potentially contribute to partial cross-protection. Further study is required to clarify whether the cross-protection was correlated with the NA-binding antibodies.
In addition, adjuvant is also an important consideration in NA protein-based vaccine design since we found that it worked on the immunity induced by the NA proteins. In contrast, we found that the mice of aluminum and cGAMP groups all survived the lethal influenza virus challenge, although the NAI antibody level of the aluminum group was slightly higher. We speculate that it may refer to the property of adjuvants or immune methods (i.p. vs. i.d.), but this still remains to be further determined. In short, adjuvant aluminum might be preferred for NA protein-based vaccination.
In conclusion, our data suggests that tetrameric NA provides better homologous protection against influenza virus infection, and it could also confer preferable subtype-specific cross-protection. NA-reactive binding and inhibition antibodies are related to protection. Furthermore, aluminum adjuvant is preferential in vaccination of recombinant NA protein than cGAMP and Poly(I:C). We hope that this information could be useful for influenza vaccine formulation and administration.
The original contributions presented in the study are included in the article/Supplementary Material, further inquiries can be directed to the corresponding authors.
The animal study was reviewed and approved by the Ethics Committee of Shanghai Public Health Clinical Center.
JH conceived and designed the experiments and supervised the project. XD, ML, and QZ expressed and purified the recombinant NA proteins. XD performed the MUNANA-based enzymatic activity assay and ELISA and ELLA assays. XD and QW performed the mice experiments. XD, JH, and FW analyzed the data and wrote the manuscript. All authors have read and agreed to the published version of the manuscript.
This work was supported by the National Natural Science Foundation of China (31771008 to JH), the National Major Science and Technology Projects of China (2017ZX10202102 to JH and 2018ZX10301403 to FW), the Hundred Talent Program of Shanghai Municipal Health Commission (2018BR08 to JH), and the Chinese Academy of Medical Sciences (2019PT350002 to JH).
The authors declare that the research was conducted in the absence of any commercial or financial relationships that could be construed as a potential conflict of interest.
All claims expressed in this article are solely those of the authors and do not necessarily represent those of their affiliated organizations, or those of the publisher, the editors and the reviewers. Any product that may be evaluated in this article, or claim that may be made by its manufacturer, is not guaranteed or endorsed by the publisher.
We are thankful for the help from the Laboratory Animal Center of Shanghai Public Clinical Center.
The Supplementary Material for this article can be found online at: https://www.frontiersin.org/articles/10.3389/fmicb.2021.729914/full#supplementary-material
Biuso, F., Palladino, L., Manenti, A., Stanzani, V., Lapini, G., Gao, J., et al. (2019). Use of lentiviral pseudotypes as an alternative to reassortant or Triton X-100-treated wild-type Influenza viruses in the neuraminidase inhibition enzyme-linked lectin assay. Influenza Other Respir. Viruses 13, 504–516. doi: 10.1111/irv.12669
Bosch, B. J., Bodewes, R., de Vries, R. P., Kreijtz, J. H. C. M., Bartelink, W., van Amerongen, G., et al. (2010). Recombinant soluble, multimeric HA and NA exhibit distinctive types of protection against pandemic Swine-Origin 2009 A(H1N1) influenza virus infection in ferrets. J. Virol. 84, 10366–10374. doi: 10.1128/JVI.01035-10
Chen, Y., Wohlbold, T. J., Zheng, N., Huang, M., Huang, Y., Neu, K. E., et al. (2018). Influenza infection in humans induces broadly cross-reactive and protective neuraminidase-reactive antibodies. Cell 173, 417–429. doi: 10.1016/j.cell.2018.03.030
Couch, R. B., Atmar, R. L., Franco, L. M., Quarles, J. M., Wells, J., Arden, N., et al. (2013). Antibody correlates and predictors of immunity to naturally occurring influenza in humans and the importance of antibody to the neuraminidase. J. Infect. Dis. 207, 974–981. doi: 10.1093/infdis/jis935
Da Silva, D. V., Nordholm, J., Madjo, U., Pfeiffer, A., and Daniels, R. (2013). Assembly of subtype 1 influenza neuraminidase is driven by both the transmembrane and head domains. J. Biol. Chem. 288, 644–653. doi: 10.1074/jbc.M112.424150
Eichelberger, M. C., and Monto, A. S. (2019). Neuraminidase, the forgotten surface antigen, emerges as an influenza vaccine target for broadened protection. J. Infect. Dis. 219, S75–S80. doi: 10.1093/infdis/jiz017
Gilchuk, I. M., Bangaru, S., Gilchuk, P., Irving, R. P., Kose, N., Bombardi, R. G., et al. (2019). Influenza H7N9 virus Neuraminidase-specific human monoclonal antibodies inhibit viral egress and protect from lethal influenza infection in mice. Cell Host Microbe 26, 715–728. doi: 10.1016/j.chom.2019.10.003
Job, E. R., Ysenbaert, T., Smet, A., Christopoulou, I., Strugnell, T., Oloo, E. O., et al. (2018). Broadened immunity against influenza by vaccination with computationally designed influenza virus N1 neuraminidase constructs. NPJ Vaccines 3:55. doi: 10.1038/s41541-018-0093-1
Johansson, B. E., Moran, T. M., and Kilbourne, E. D. (1987). Antigen-Presenting B cells and helper T cells cooperatively mediate intravirionic antigenic competition between influenza a virus surface glycoproteins. Proc. Natl. Acad. Sci. U.S.A. 84, 6869–6873. doi: 10.1073/pnas.84.19.6869
Ju, H., Zhang, J., Sun, Z., Huang, Z., Qi, W., Huang, B., et al. (2018). Discovery of C-1 modified oseltamivir derivatives as potent influenza neuraminidase inhibitors. Eur. J. Med. Chem. 146, 220–231. doi: 10.1016/j.ejmech.2018.01.050
Kim, K., Lee, Y., Park, S., Jung, Y., Lee, Y., Ko, E., et al. (2019). Neuraminidase expressing virus-like particle vaccine provides effective cross protection against influenza virus. Virology 535, 179–188. doi: 10.1016/j.virol.2019.07.008
Krammer, F., Fouchier, R. A. M., Eichelberger, M. C., Webby, R. J., Shaw-Saliba, K., Wan, H., et al. (2018). NAction! How can Neuraminidase-Based immunity contribute to better influenza virus vaccines? mBio 9:e02317-32. doi: 10.1128/mBio.02332-17
Liu, W., Lin, C., Tsou, Y., Jan, J., and Wu, S. (2015). Cross-reactive neuraminidase-inhibiting antibodies elicited by immunization with recombinant neuraminidase proteins of H5N1 and pandemic H1N1 influenza a viruses. J. Virol. 89, 7224–7234. doi: 10.1128/JVI.00585-15
Martinet, W., Saelens, X., Deroo, T., Neirynck, S., Contreras, R., Min, J. W., et al. (1997). Protection of mice against a lethal influenza challenge by immunization with yeast-derived recombinant influenza neuraminidase. Eur. J. Biochem. 247, 332–338. doi: 10.1111/j.1432-1033.1997.00332.x
McMahon, M., Strohmeier, S., Rajendran, M., Capuano, C., Ellebedy, A. H., Wilson, P. C., et al. (2020). Correctly folded - but not necessarily functional - influenza virus neuraminidase is required to induce protective antibody responses in mice. Vaccine 38, 7129–7137. doi: 10.1016/j.vaccine.2020.08.067
Memoli, M. J., Shaw, P. A., Han, A., Czajkowski, L., Reed, S., Athota, R., et al. (2016). Evaluation of antihemagglutinin and antineuraminidase antibodies as correlates of protection in an influenza A/H1N1 virus healthy human challenge model. mBio 7:e417. doi: 10.1128/mBio.00417-16
Monto, A. S., and Kendal, A. P. (1973). Effect of neuraminidase antibody on Hong Kong influenza. Lancet 1, 623–625. doi: 10.1016/S0140-6736(73)92196-X
Moriyama, M., Chino, S., and Ichinohe, T. (2017). Consecutive inoculations of influenza virus vaccine and poly(I:C) protects mice against homologous and heterologous virus challenge. Vaccine 35, 1001–1007. doi: 10.1016/j.vaccine.2017.01.025
Murphy, B. R., Kasel, J. A., and Chanock, R. M. (1972). Association of serum anti-neuraminidase antibody with resistance to influenza in man. N. Engl. J. Med. 286, 1329–1332. doi: 10.1056/NEJM197206222862502
Prevato, M., Ferlenghi, I., Bonci, A., Uematsu, Y., Anselmi, G., Giusti, F., et al. (2015). Expression and characterization of recombinant, tetrameric and enzymatically active influenza neuraminidase for the setup of an enzyme-linked lectin-based assay. PLoS One 10:e135474. doi: 10.1371/journal.pone.0135474
Quan, F., Kim, M., Lee, B., Song, J., Compans, R. W., and Kang, S. (2012). Influenza M1 VLPs containing neuraminidase induce heterosubtypic cross-protection. Virology 430, 127–135. doi: 10.1016/j.virol.2012.05.006
Rudicell, R. S., Garinot, M., Kanekiyo, M., Kamp, H. D., Swanson, K., Chou, T. H., et al. (2019). Comparison of adjuvants to optimize influenza neutralizing antibody responses. Vaccine 37, 6208–6220. doi: 10.1016/j.vaccine.2019.08.030
Saito, T., Taylor, G., and Webster, R. G. (1995). Steps in maturation of influenza a virus neuraminidase. J. Virol. 69, 5011–5017. doi: 10.1128/jvi.69.8.5011-5017.1995
Sandbulte, M. R., Jimenez, G. S., Boon, A. C., Smith, L. R., Treanor, J. J., and Webby, R. J. (2007). Cross-reactive neuraminidase antibodies afford partial protection against H5N1 in mice and are present in unexposed humans. PLoS Med. 4:e59. doi: 10.1371/journal.pmed.0040059
Smith, G. E., Sun, X., Bai, Y., Liu, Y. V., Massare, M. J., Pearce, M. B., et al. (2017). Neuraminidase-based recombinant virus-like particles protect against lethal avian influenza A(H5N1) virus infection in ferrets. Virology 509, 90–97. doi: 10.1016/j.virol.2017.06.006
Sridhar, S., Brokstad, K. A., and Cox, R. J. (2015). Influenza vaccination strategies: comparing inactivated and live attenuated influenza vaccines. Vaccines 3, 373–389. doi: 10.3390/vaccines3020373
Stadlbauer, D., Zhu, X., McMahon, M., Turner, J. S., Wohlbold, T. J., Schmitz, A. J., et al. (2019). Broadly protective human antibodies that target the active site of influenza virus neuraminidase. Science 366, 499–504. doi: 10.1126/science.aay0678
Subathra, M., Santhakumar, P., Narasu, M. L., Beevi, S. S., and Lal, S. K. (2014). Evaluation of antibody response in mice against avian influenza a (H5N1) strain neuraminidase expressed in yeast Pichia pastoris. J. Biosci. 39, 443–451. doi: 10.1007/s12038-014-9422-3
Tong, S. X., Li, Y., Rivailler, P., Conrardy, C., Alvarez Castillo, D. A., Chen, L. M., et al. (2012). A distinct lineage of influenza A virus from bats. Proc. Natl. Acad. Sci. U.S.A. 109, 4269–4274. doi: 10.1073/pnas.1116200109
Tong, S. X., Zhu, X. Y., Li, Y., Shi, M., Zhang, J., Bourgeois, M., et al. (2013). New world bats harbor diverse influenza A viruses. PLoS Pathog. 9:e1003657. doi: 10.1371/journal.ppat.1003657
Vogel, O. A., and Manicassamy, B. (2020). Broadly protective strategies against influenza viruses: universal vaccines and therapeutics. Front. Microbiol. 11:135. doi: 10.3389/fmicb.2020.00135
Wang, J., Li, P. Y., and Wu, M. X. (2016). Natural STING agonist as an “ideal” adjuvant for cutaneous vaccination. J. Invest. Dermatol. 136, 2183–2191. doi: 10.1016/j.jid.2016.05.105
Wohlbold, T. J., Nachbagauer, R., Xu, H., Tan, G. S., Hirsh, A., Brokstad, K. A., et al. (2015). Vaccination with adjuvanted recombinant neuraminidase induces broad heterologous, but not heterosubtypic, cross-protection against influenza virus infection in mice. mBio 6:e2556. doi: 10.1128/mBio.02556-14
Wu, Z. L., Ethen, C., Hickey, G. E., and Jiang, W. (2009). Active 1918 pandemic flu viral neuraminidase has distinct N-glycan profile and is resistant to trypsin digestion. Biochem. Biophys. Res. Commun. 69, 749–753. doi: 10.1016/j.bbrc.2008.12.139
Keywords: influenza virus, neuraminidase, protein, vaccine, cross-protection
Citation: Deng X, Wang Q, Liu M, Zheng Q, Wu F and Huang J (2021) Tetrameric Neuraminidase of Influenza A Virus Is Required to Induce Protective Antibody Responses in Mice. Front. Microbiol. 12:729914. doi: 10.3389/fmicb.2021.729914
Received: 24 June 2021; Accepted: 30 August 2021;
Published: 04 October 2021.
Edited by:
Dayan Wang, Chinese National Influenza Center, ChinaCopyright © 2021 Deng, Wang, Liu, Zheng, Wu and Huang. This is an open-access article distributed under the terms of the Creative Commons Attribution License (CC BY). The use, distribution or reproduction in other forums is permitted, provided the original author(s) and the copyright owner(s) are credited and that the original publication in this journal is cited, in accordance with accepted academic practice. No use, distribution or reproduction is permitted which does not comply with these terms.
*Correspondence: Fan Wu, d3VmYW5AZnVkYW4uZWR1LmNu; Jinghe Huang, amluZ2hlaHVhbmdAZnVkYW4uZWR1LmNu
Disclaimer: All claims expressed in this article are solely those of the authors and do not necessarily represent those of their affiliated organizations, or those of the publisher, the editors and the reviewers. Any product that may be evaluated in this article or claim that may be made by its manufacturer is not guaranteed or endorsed by the publisher.
Research integrity at Frontiers
Learn more about the work of our research integrity team to safeguard the quality of each article we publish.