- 1School of Life Sciences, Ludong University, Yantai, China
- 2Shandong Provincial Key Laboratory of Quality Safty Monitoring and Risk Assessment for Animal Products, Jinan, China
- 3Shandong Aquaculture Environmental Control Engineering Laboratory, Yantai, China
- 4Yantai Key Laboratory of Animal Pathogenetic Microbiology and Immunology, Yantai, China
- 5Yantai Research Institute for Replacing Old Growth Drivers with New Ones, Yantai, China
Fungi play an irreplaceable role in drug discovery in the course of human history, as they possess unique abilities to synthesize diverse specialized metabolites with significant medicinal potential. Trichoderma are well-studied filamentous fungi generally observed in nature, which are widely marketed as biocontrol agents. The secondary metabolites produced by Trichoderma have gained extensive attention since they possess attractive chemical structures with remarkable biological activities. A large number of metabolites have been isolated from Trichoderma species in recent years. A previous review by Reino et al. summarized 186 compounds isolated from Trichoderma as well as their biological activities up to 2008. To update the relevant list of reviews of secondary metabolites produced from Trichoderma sp., we provide a comprehensive overview in regard to the newly described metabolites of Trichoderma from the beginning of 2009 to the end of 2020, with emphasis on their chemistry and various bioactivities. A total of 203 compounds with considerable bioactivities are included in this review, which is worth expecting for the discovery of new drug leads and agrochemicals in the foreseeable future. Moreover, new strategies for discovering secondary metabolites of Trichoderma in recent years are also discussed herein.
Introduction
Trichoderma is a fungal genus that was first described in 1794 (Persoon, 1794). This genus is well adapted to various ecological niches and is ubiquitous in most types of soils, roots, and foliar environments. Trichoderma species are beneficial for their commercial enzymes, plant growth-accelerating abilities, and biocontrol of plant diseases, indicating their promising industrial, agricultural, and medicinal potential (Cai et al., 2013; Bhardwaj and Kumar, 2017; McMullin et al., 2017). Globally, Trichoderma has proved to achieve great success as effective biological control drugs (Keswani et al., 2014). Many Trichoderma species, such as T. harzianum, T. hamatum, T. asperellum, T. atroviride, T. koningii, T. virens, and T. viride, are lucratively used as potent biocontrol agents worldwide (Bhardwaj and Kumar, 2017). These fungal species exhibit outstanding biocontrol capability against pathogenic microorganisms either through indirect (scrambling for nutrients, changing the ambient conditions, stimulating plant growth and defense responses) or direct (mycoparasitism) mechanisms (Bhardwaj and Kumar, 2017). Moreover, in addition to ecological effects, it is well known that Trichoderma can produce secondary metabolites that not only participate in signal transduction but also go through communications with various organisms (Keswani et al., 2014; Zeilinger et al., 2016). It is also believed that the successes of Trichoderma as biocontrol drugs are, at least partially, due to their capacity to secrete abundant secondary metabolites (Zeilinger et al., 2016).
Fungi play an irreplaceable role in the drug discovery in the course of human history, as they possess unique abilities to synthesize diverse secondary metabolites with significant medical potential (Li X.Q. et al., 2020). The discovery of penicillin from the filamentous fungal species Penicillium was a milestone in pharmaceutical research (Fleming, 1929). Since then, chemical studies regarding fungal secondary metabolites have become a research hotspot (Zhang P. et al., 2020). A large number of fungal secondary metabolites have been discovered, many of which have potential as drug leads (Newman and Cragg, 2016). The fungal species belonging to Penicillium and Talaromyces are representative flora, with many secondary metabolites possessing intriguing chemical skeletons and bioactivities characterized from these species (Frisvad, 2014). For the genus Trichoderma, more than 1000 metabolites have been isolated from Trichoderma in recent years (Zeilinger et al., 2016). Accordingly, many reviews on various aspects of Trichoderma, not only for the chemical diversity of metabolites but also for the various bioactivities and their potential applications, have been published. Zeilinger et al. (2016) reviewed the selected Trichoderma-derived secondary metabolites and gave an all-round summary of genomic analysis and putative gene clusters involved in biosynthesis. Keswani et al. (2014) listed targeted metabolites of Trichoderma and pointed out the utilization potentiality in multifarious areas, especially in agriculture. As mentioned above, Trichoderma is a well-known biocontrol agent that is used globally. Since many Trichoderma species are some of the most prominent producers of anti-phytopathogenic secondary metabolites, Khan et al. (2020) exhibited 45 fungicidal secondary metabolites of Trichoderma sp. along with the structural overview, biosynthesis pathway, and action mechanism. Moreover, Reino et al. (2008) systematically summarized the metabolites of Trichoderma and their bioactivities. As of 2008, a total of 186 compounds and 269 references were cited, including a detailed study of the activities of biocontrol mechanisms (Reino et al., 2008). Herein, in order to update the relevant list of reviews of secondary metabolites of Trichoderma sp., we provide a comprehensive overview in regard to the newly described metabolites of Trichoderma from the beginning of 2009 to the end of 2020, with emphasis on their chemistry and various bioactivities. Moreover, new strategies for discovering secondary metabolites of Trichoderma in recent years have also been discussed.
Literature Search
To retrieve literature published up to 2020, an in-depth inspection was performed (Zhang P. et al., 2020). The key words of “Trichoderma” and “secondary metabolites” were used to search for related literatures in Web of Science, with the times pan from 2009 to 2020 (Supplementary Figure 1). Additionally, other platforms such as Crossref, Google Scholar, Elsevier, and Springer Link were also searched at the same time. The retrieved articles were categorized according to natural product chemistry. In all, 63 records in the context of natural product research were retained and assessed to present this review. A total of 203 compounds were found from Trichoderma from 2009 to 2020. It should be pointed out that some omissions were inevitable during the literature search. However, with the greatest effort, we have demonstrated almost all of the relevant research herein.
Chemical Diversity
Terpenoids
Trichothecene Sesquiterpenes
Trichothecenes, which are primarily produced by several genera of fungi, are sesquiterpene-based compounds possessing a tricyclic 12,13-epoxytrichothec-9-ene skeleton (Takahashi-Ando et al., 2020). Structurally, trichothecenes are classified into different families of nivalenols, neosolaniols, isotrichodermins, calonectrins, trichothecene, and trichobreols based on the substitution pattern. To date, more than 200 trichothecene derivatives have been discovered (Shi Z.Z. et al., 2020; Takahashi-Ando et al., 2020). The structures of trichothecenes isolated from Trichoderma species are listed in Figure 1. Eight newly discovered trichothecenes, trichodermarins G–N (1–8), and six known trichothecenes, trichodermol (9), trichodermin (10), trichoderminol (11), trichodermarin A (12) and trichodermarin B (13), and 2,4,12-trihydroxyapotrichothecene (14), were isolated from Trichoderma brevicompactum ADL-9-2, which was obtained as an endophyte of marine algae Chondria tenuissima (Shi Z.Z. et al., 2020). Trichodermarin N (8), featuring a 2’-N-acetylglucosaminyl moiety, represented the first aminoglycoside-bearing trichothecene. Chemical investigations of the marine fungus Trichoderma cf. brevicompactum TPU199 fermented with NaI afforded three new trichothecenes, trichobreols A–C (15–17) (Yamazaki et al., 2020a). Interestingly, 15 can be produced under the original culture conditions (seawater medium), whereas compounds 16 and 17 were found only under NaI-containing culture conditions. Additionally, isolation of the same fungus yielded two new trichothecenes, trichobreols D (18) and E (19) (Yamazaki et al., 2020b). Harzianums A (20) and B (21), two trichothecenes linked with octa-2,4,6-trienedioyl moiety, were isolated from the biofertilizer fungus T. brevicompactum (CGMCC19618) (Yin et al., 2020). From a marine fungus Trichoderma longibrachiatum, three trichothecenes, trichothecinol A (22), 8-deoxy-trichothecin (23), and trichothecinol B (24) were yielded (Du et al., 2020).
Carotane Sesquiterpenes
From the marine-derived fungus T. virens Y13-3, eight undescribed carotane sesquiterpenes, trichocarotins A–H (25–32), along with the known compounds CAF-603 (33), trichocarane B (34), 7-β-hydroxy CAF-603 (35), and trichocarane A (36), were discovered (Figure 2) (Shi et al., 2018a). Carotane sesquiterpenes are commonly found in plants. However, only approximately 10 compounds have been isolated from filamentous fungi, representing a rare class of fungal metabolites (Shi et al., 2018a).
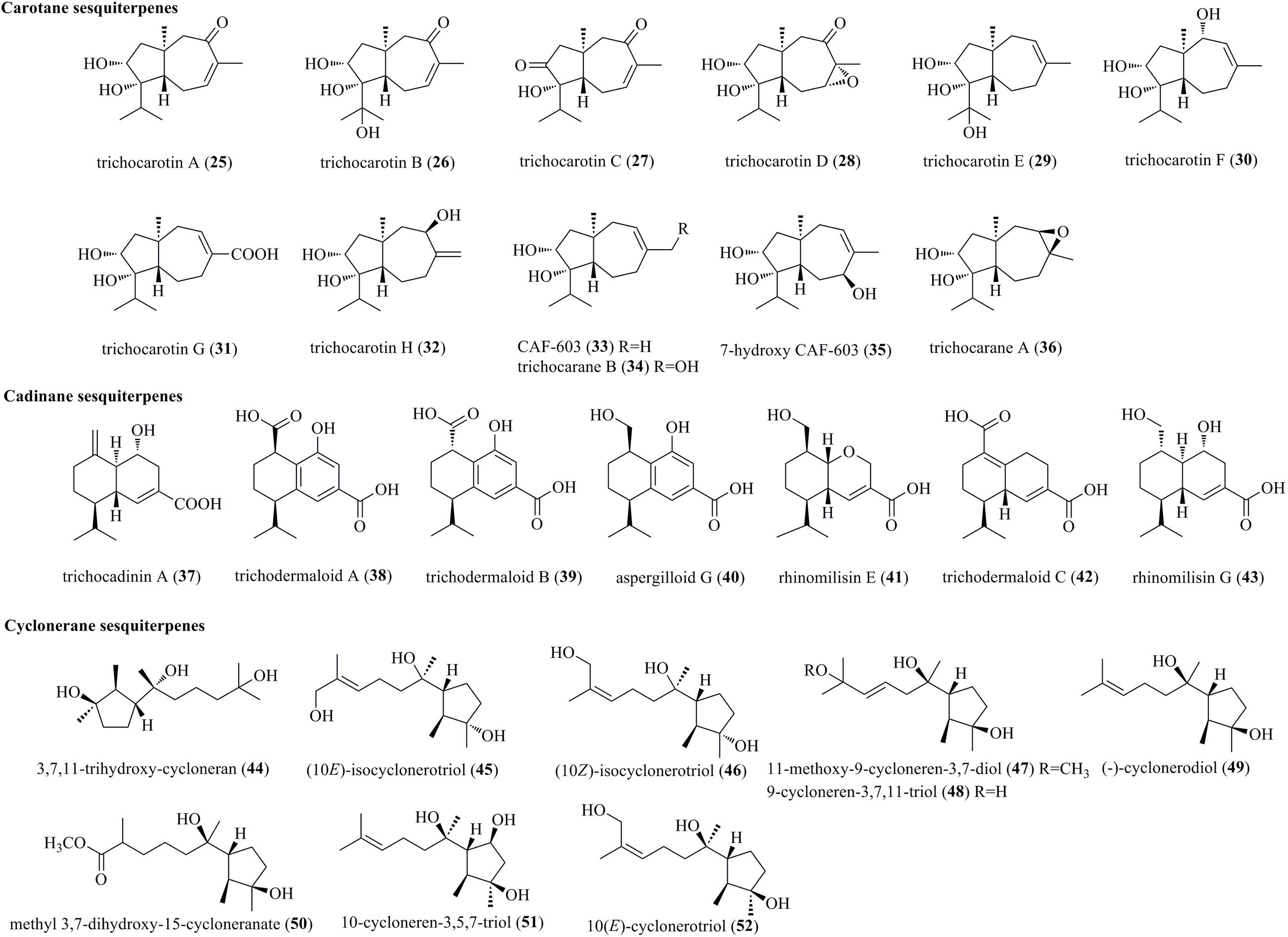
Figure 2. Carotane, cadinane, and cyclonerane sesquiterpenes produced by Trichoderma species (25–52).
Cadinane Sesquiterpenes
A new example of a cadinane-skeletoned sesquiterpene, trichocadinin A (37), containing a previously unrecognized site of an exocyclic olefin functionality at C-10, was obtained from the marine-derived fungus T. virens Y13-3 (Shi et al., 2018a). This is the first time to report cadinane sesquiterpenes from Trichoderma. three new cadinane-type sesquiterpenes, i.e., trichodermaloids A–C (38–40), and three known ones, i.e., aspergilloid G (41), rhinomilisin E (42), and rhinomilisin G (43), were characterized from the marine sponge-derived fungus Trichoderma sp. SM16 (Cui et al., 2020).
Cyclonerane Sesquiterpenes
Induced by a chemical epigenetic manipulation strategy, a new cyclonerane, 3,7,11-trihydroxy-cycloneran (44), was produced by the marine fungus T. harzianum (XS-20090075), which was isolated from soft corals (Shi T. et al., 2020). Cycloneranes having a monocyclic skeleton are reported to be produced from various fungal genera, such as Trichoderma, Aspergillus, Fusarium, Paecilomyces, and Trichothesium. Based on previous findings, the five-membered ring of all the isolated cyclonerane sesquiterpenes featured the same relative configuration (Liu X.H. et al., 2020). However, chromatographic separation of the marine fungus Trichoderma citrinoviride A-WH-20-3 yielded two undescribed cycloneranes, (10E)-isocyclonerotriol (45) and (10Z)-isocyclonerotriol (46), which were characterized as the first example with an isomerized ring in cycloneranes (Liu X.H. et al., 2020). New cycloneranes, 11-methoxy-9-cycloneren-3,7-diol (47), methyl 3,7-dihydroxy-15-cycloneranate (50), and 10-cycloneren-3,5,7-triol (51), as well as two structurally related cycloneranes, 9-cycloneren-3,7,11-triol (48) and (–)-cyclonerodiol (49), were obtained from T. harzianum X-5, an endophyte of the marine alga Laminaria japonica (Song et al., 2018). Biosynthetically, 47 and 50 may be produced through O-methylation during the fermentation process. Two previously reported cycloneranes, i.e., 10-cycloneren-3,5,7-triol (51) and 10(E)-cyclonerotriol (52), were obtained from T. longibrachiatum, an endophyte of the highly halophile Suaeda glauca (Du et al., 2020).
Drimane Sesquiterpenes
Chemical exploration of an endophyte Trichoderma sp. 1212-03 from Daedaleopsis tricolor yielded three new drimane sesquiterpenes, i.e., neomacrophorins I (53), II (54), and III (55) (Figure 3) (Hirose et al., 2014). They belong to macrophorins and drimane sesquiterpene-linked cyclohexenone epoxides but feature a hydroxyl in the drimene skeleton and a 5′,6′-α-epoxide in quinone functionality. Furthermore, six novel neomacrophorins, i.e., 3-deoxyneomacrophorin IV (56), 3-oxoneomacrophorins I (57) and II (58), neomacrophorin VII (59), 5′-epimacrophorin B (60), and 5′-deoxyneomacrophorin IV (61), as well as four novel premacrophorin congeners, i.e., premacrophorin III (62), premacrophorindiol (63), premacrophorintriols I (64), and II (65), were isolated from the same fungus (Nishiyama et al., 2019). These molecules possessed 2,3-epoxybenzoquinone (56 and 57) or 2,3-epoxybenzosemiquinol substructures (58–60 and 62–64). Premacrophorins 62–65 carried acyclic isoprenoid side chains biosynthetically derived from neomacrophorins in the early stage, rather than the common drimane skeleton.
Other Sesquiterpenes
Co-culture of the mangrove endophytic fungus Trichoderma sp. 307 and aquatic pathogenic bacterium Acinetobacter johnsonii B2 afforded two undescribed furan-type isoeremophilane sesquiterpenes, microsphaeropsisins B (66) and C (67) (Zhang et al., 2017). It is believed that both of them were derived from Trichoderma sp. rather than induced by the coculture. 8-Acoren-3,11-diol (68), trichoacorenol (69), and trichoacorenol B (70) were isolated from marine-derived T. harzianum X-5, and they were structurally characterized as acorane sesquiterpenes (Song et al., 2018). Trichodermene A (71), an unusual norsesquiterpene with a novel tricyclic-6/5/5-[4.3.1.01,6]-decane framework, was characterized to originate from T. longibrachiatum (Du et al., 2020). Two new sesquiterpenes, i.e., trichoderiols A (72) and B (73), were produced by T. atroviride S361, an endophyte of Cephalotaxus fortunei (Zheng et al., 2011). Compounds 72 and 73 were structurally characterized as 2-oxabicyclo[2,1]heptane derivatives. New cuparenes, i.e., trichocuparins A (74) and B (75), were obtained from T. brevicompactum ADL-9-2, which was isolated from marine algae C. tenuissima (Shi Z.Z. et al., 2020). Compounds 74 and 75 are rare cuparenes with a cyclopentylcyclohexane unit. Three new sesquiterpenes, i.e., Trichodones A–C (76–78), were isolated from T. asperellum residing in Panax notoginseng (Ding et al., 2012a). The new sesquiterpenes possess the same skeleton as juvabione. However, the diol groups in compounds 76–78 were different from those of known juvabione analogs. Fermentation of T. atroviride, an endophyte of Lycoris radiate, afforded a new sesquiterpene, atrichodermone C (79) (Zhou et al., 2017). Chemical study of the marine sponge-associated fungus T. harzianum LZDX-32-08 yielded two new structurally unique sesquiterpenes, i.e., harzianoic acids A (80) and B (81) (Li et al., 2019). Interestingly, 80 and 81 had a cyclobutane framework, which was biogenetically derived from an unusual isoprenoid pathway.
Harziane Diterpenes
Harziane diterpenes, typically possessing a 4/7/5/6-fused tetracyclic framework, have been reported exclusively from various Trichoderma species (Figure 4). Five undescribed harziane diterpenes, i.e., harzianols F–J (82–86), and three previously reported diterpenes, i.e., 3S-hydroxyharzianone (87), harziandione (88), and harzianol A (89), were isolated from the endophyte T. atroviride B7 of Colquhounia coccinea var. mollis (Li W.Y. et al., 2020). Deoxytrichodermaerin (90), an undescribed harziane lactone, was isolated from an endophyte T. longibrachiatum A-WH-20-2 of marine algae Laurencia okamurai (Zou et al., 2021). A new harziane diterpenoid, harzianone E (91), was obtained from the soft coral-sourced T. harzianum (XS-20090075) by chemical epigenetic manipulation strategy (Shi T. et al., 2020). A new harziane diterpene, 3R-hydroxy-9R,10R-dihydroharzianone (92), was produced by T. harzianum X-5 (Song et al., 2018). Two undescribed harzianes, i.e., (9R,10R)-dihydro-harzianone (93) and harzianelactone (94), were produced by Trichoderma sp. Xy24 from mangrove plant Xylocarpus granatum (Zhang et al., 2016). Chemical investigations on the marine fungus T. harzianum XS-20090075 yielded diverse harzianes, including two undescribed harziane lactones, i.e., harzianelactones A and B (95 and 96), as well as five new lactones, i.e., harzianones A–D (97–100) and harziane (101) (Zhao et al., 2019). Finally, a new diterpenoid lactone, trichodermaerin (102), was isolated from Trichoderma erinaceum derived from Acanthaster planci (Xie et al., 2013).
Other Diterpenes
Citrinovirin (103), a rare norditerpene, was produced by an endophyte T. citrinoviride cf-27 (Liang et al., 2016). Compound 103 possessed a perhydroazulene ring system, which was synthesized by a unique biogenetic pathway including demethylation, cyclization, oxidation, and SN2 reaction with Walden inversion. A new proharziane-type diterpene, 11R-methoxy-5,9,13-proharzitrien-3-ol (104), was characterized from the marine algicolous fungus T. harzianum X-5 (Song et al., 2018). 104 and harzianes were structurally related diterpenes. Harzianolic acid A (105), characterized as a novel chlorinated cleistanthane diterpenoid, was isolated from the marine fungal strain T. harzianum (XS-20090075) (Shi T. et al., 2020). Tricyclic diterpenoids categorized to cleistanthanes were reported from Trichoderma for the first time.
Cyclopeptides
Bioassay-guided fractionation of the plant endophytic fungus T. harzianum KZ-20 afforded four new cyclodepsipeptides belonging to the destruxin family, i.e., trichodestruxins A–D (106–109), and two previously reported derivative, i.e., destruxin E2 chlorohydrin (110) and destruxin A2 (111) (Figure 5) (Liu Z. et al., 2020). Destruxins represent rare cyclic hexadepsipeptides. Structurally, new compound 107 possessed hydroxy acid fragments of the 2,4,5-trihydroxypentanoic acid unit, while 106 and 108 had a β-methylproline moiety. Homodestcardin (112), trichomide B (113), and homodestruxin B (114), characterized as cyclohexadepsipeptides of the trichomide series, were produced by T. longibrachiatum (Du et al., 2020). Finally, cyclopeptides PF1022F (115) and halobacillin (116) were obtained from the endophyte T. asperellum (Ding et al., 2012a).
Diketopiperazines
The marine fungus Trichoderma sp. TPU199 is a producer of a series of diketopiperazines (117–127) (Figure 6) (Yamazaki et al., 2020a). Initially, this fungal strain was found to produce pretrichodermamide A (117), gliovirin (118), and trichodermamide A (119). 117 and 118 possessed an unusual epipolythiodiketopiperazine (ETP) skeleton. Then, this strain with sodium halides added to the culture medium afforded the halogenated gliovirin-type ETPs DC1149B (120), DC1149R (122), and iododithiobrevamide (123). Subsequently, chlorotrithiobrevamide (124), the first trisulfide derivative in the type of ETP, was characterized. Furthermore, a highly modified dipeptide, dithioaspergillazine A (125), was obtained after the long time cultivation. Finally, two undescribed ETPs, i.e., 5-epi-pretrichodermamide A (126) and 5-epi-trithiopretrichodermamide A (127), were characterized under NaI-containing culture conditions. Pretrichodermamide G (128) was established as a 1,2-oxazadecaline ETP, and it was identified from the endophyte T. harzianum of Zingiber officinale (Harwoko et al., 2021). A rare heterocyclic dipeptide, i.e., trichodermamide G (129), and a biogenetically related metabolite aspergillazin A (130) were produced by the marine-sourced T. harzianum D13 (Zhao et al., 2020). Notably, 129 and 130 were novel ETP derivatives with the sulfur bridge locating at different positions. Dehydroxymethylbis(dethio)bis(methylthio)gliotoxin (131) and (3S,6R)-6-(para-hydroxybenzyl)-1,4-dimethyl-3,6-bis(methylthio)piperazine-2,5-dione (132), which were structurally characterized as two undescribed sulfurated diketopiperazines, were produced by an algicolous isolate of T. virens Y13-3 (Shi et al., 2018b). The fungal strain T. asperellum A-YMD-9-2 from Gracilaria verrucosa produced an undescribed symmetric diketopiperazine, cyclo(L-5-MeO-Pro-L-5-MeO-Pro) (133) (Song et al., 2020).
Alkaloids and Other Nitrogen-Containing Compounds
Chemical survey of T. virens FKI-7573 generated an undescribed N-containing compound, i.e., trichothioneic acid (134) (Figure 7) (Miyano et al., 2020). 134 contained a heptelidic acid and an L-ergothioneine substructure. Ethyl 2-bromo-4-chloroquinoline-3-carboxylate (135) was produced by the soft coral-sourced T. harzianum (XS-20090075) in Czapek’s medium (Yu et al., 2021). 135 was the first halogenated quinoline derivative from Trichoderma. Trichoderamides A (136) and B (137), isolated as stereoisomers originating from the PKS-NRPS mixed pathway, were isolated from T. gamsii, an endophyte of P. notoginseng (Ding et al., 2015). Two rare pyridones, i.e., trichodins A (138) and B (139), were identified from Trichoderma sp. strain MF106 from the Greenland Seas (Wu et al., 2014). Harzianic acid (140), a nitrogen heterocyclic siderophore, was isolated from T. harzianum M10 (Vinale et al., 2013). 140 was identified as 2-hydroxy-2-[4-(1-hydroxy-octa-2,4-dienylidene)-1-methyl-3,5-dioxopyrrolidin-2-ylmethyl]-3-methyl-butyric acid. Atrichodermone A (141) was a unique compound with a dimeric cyclopentenone framework that was discovered from the endophytic fungal strain T. atroviride (Zhou et al., 2017). 141 was the first example of a 3-amino-5-hydroxy-5-vinyl-2-cyclopenten-1-one dimer. Two nitrogen-containing cyclonerane sesquiterpene derivatives, 5′-acetoxy-deoxycyclonerin B (142) and 5′-acetoxy-deoxycyclonerin D (143), were obtained from the marine fungus T. asperellum A-YMD-9-2 (Song et al., 2020).
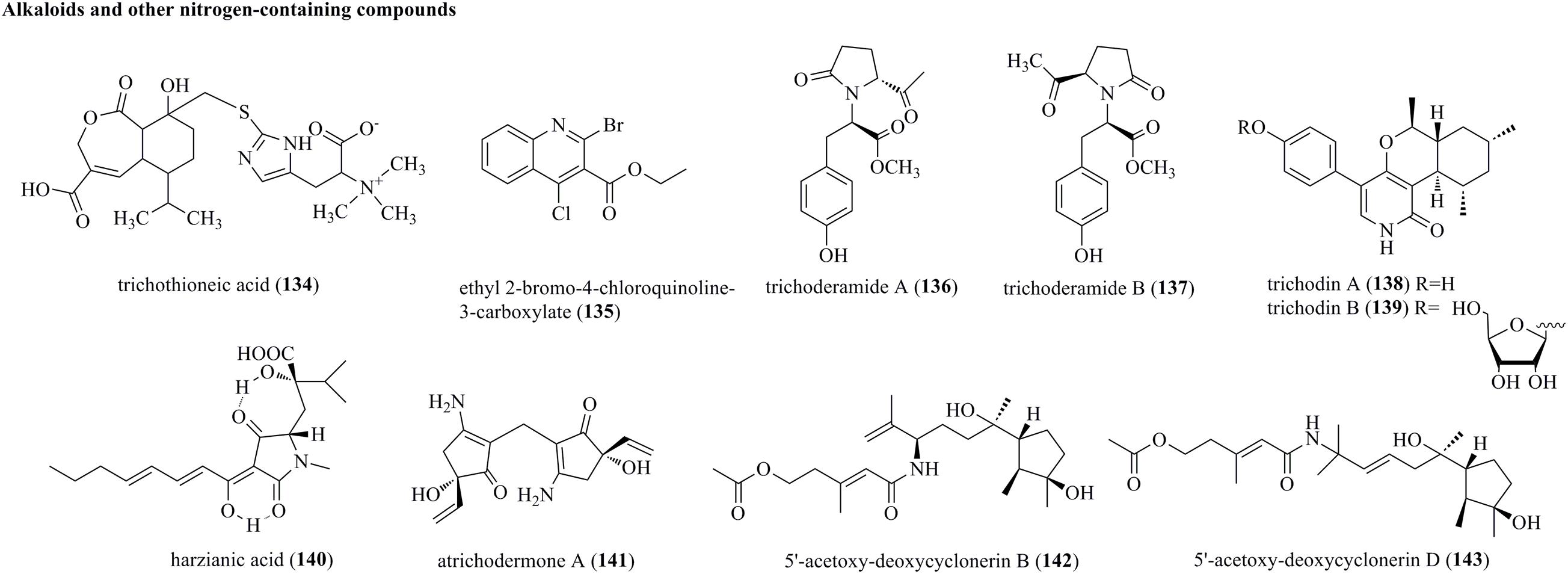
Figure 7. Alkaloids and other nitrogen-containing compounds produced by Trichoderma species (134–143).
Polyketides
Naphthalene Derivatives
An undescribed naphthalene, trichoharzin B (144), a natural product, methyl-trichoharzin (145), and the known trichoharzin (146) and eujavanicol A (147) were produced by the marine fungus T. harzianum XS-20090075 (Figure 8) (Yu et al., 2021). 144–146 were characterized as new polyketides with an alkylated decalin framework and esterified with a rare acyl group. Trichoharzianol (148) was identified from a fungal strain of T. harzianum F031 (Jeerapong et al., 2015). 148 was reported as a new decalin derivative bearing a 3-hydroxypropionyl moiety, a 1-methylpropyl moiety, and an acetonide moiety. Trichodermic acid A (149) and B (150) were isolated from an endophytic fungus T. spirale, and characterized as new octahydronaphthalene derivatives (Li et al., 2012).
Octaketides
Five new polyketides, ent-koninginin A (151), 1,6-di-epi-koninginin A (152), 15-hydroxykoninginin A (153), 10-deacetylkoningiopisin D (154), and koninginin T (155), along with two previously reported derivatives, koninginin L (156) and trichoketide A (157), were produced by Trichoderma koningiopsis QA-3 (Shi et al., 2017). Compounds 151–153 were characterized as tricyclic polyketides with an octahydrochromene skeleton. Koninginins I (158), J (159) and K (160), which were structurally characterized as new koninginin-type compounds, were produced by T. neokongii 8722 (Zhou et al., 2014). Chemical study of the marine-derived fungus T. koningii afforded five new polyketides, 7-O-methylkoninginin D (161) and trichodermaketones A–D (162–165) (Song et al., 2010). 162 and 163 represented unprecedented tricyclic polyketides having a bistetrafuran skeleton. Trichoketides A (166) and B (167), two undescribed octaketides, were isolated from Trichoderma sp. TPU1237 (Yamazaki et al., 2015). 166 and 167 were epimers at the α-position of the dihydrofuran ring. Finally, koninginins L (156) (herein reported as a new compound) and M (168) were isolated from solid fermentation of T. koningii 8662 (Lang et al., 2015). A series of koninginins were reported from Trichoderma species, but only in koninginins L (156) and M (168) was an oxygen bridge located between the C-10 and C-7 positions.
Cytochalasans
Cytochalasans are a kind of novel fungal metabolic products, with more than 100 cytochalasans being reported to date. These metabolites contain a polycyclic skeleton and an isoindole moiety, which was fused with one macrocyclic ring (Ding et al., 2012c). Two highly complicated pentacyclic cytochalasans, trichoderone A (169) and trichoderone B (170), together with three previously reported cytochalasans, aspochalasins D (171), J (172), and I (173), were obtained from T. gamsii from P. notoginseng (Figure 9) (Ding et al., 2012c). 169 possessed a highly functionalized 7/6/6/5/5 pentacyclic skeleton, while 170 contained the unusual 6/5/6/6/5 pentacyclic framework. Furthermore, two undescribed cytochalasans, trichalasins C (174) and D (175), as well as three known cytochalasans, aspochalasins D (171), M (176), and P (177), were obtained from the abovementioned strain T. gamsii (Ding et al., 2012b).
Other Polyketides
An undescribed cyclopentenone, atrichodermone B (178), was isolated from endophytic T. atroviride (Zhou et al., 2017). A newly discovered cyclopentenone, 5-hydroxycyclopeni cillone (179), was produced by the marine-sourced Trichoderma sp. HPQJ-34 (Fang et al., 2017). 179 possessed a 3-substituted 4,5-dihydroxy-2,5-dimethylcyclopent-2-enone skeleton. Two previously reported unsaturated lactones, xylogibloactones A (180) and B (181), were found from the marine fungus T. harzianum (XS-20090075) (Yu et al., 2021). Compounds 180 and 181 had a C9 polyketide framework with a γ-lactone moiety. Trichoderpyrone (182), a novel cyclopentenone-pyrone mixed polyketide, was produced by T. gamsii (Chen et al., 2017). 182 had two individual ring systems, i.e., a 3-aminocyclopent-2-en-1-one moiety and a 4-hydroxy-6-methyl-2H-pyran-2-one moiety, which were derived from a mixed biosynthetic pathway. Trichoderone (183), a new cyclopentenone, was obtained from the marine Trichoderma sp. (You et al., 2010). Compounds 184 and 185, characterized as two new isocoumarin derivatives with a butanetriol residue, were isolated from the endophytic fungus T. harzianum Fes1712 (Ding et al., 2019). Trichophenol A (186), which was identified as a new isocoumarin derivative with a 6,8-dihydroxyisocoumarin moiety, was isolated from T. citrinoviride A-WH-20-3 (Liu X.H. et al., 2020). Previously described metabolites, 5-hydroxy-3-hydroxymethyl-2-methyl-7-methoxychromone (187) and 4,6-dihydroxy-5-methylphthalide (188), were produced by the fungus T. harzianum F031 (Jeerapong et al., 2015). Two structurally new polyketides (189 and 190) with a 1-hydroxyhepta/methoxyhepta-3,5-dien-2-one moiety were produced in the transformant of Trichoderma afroharzianum (Ding et al., 2020). Two undescribed azaphilone derivatives, azaphilones D (191) and E (192), were obtained from dragonfly associated T. harzianum QTYC77 (Zhang S. et al., 2020). A new 10-membered lactone cremenolide (193), elucidated as but-2-enoic acid 7-acetoxy-6-hydroxy-2- methyl-10-oxo-5,6,7,8,9,10-hexahydro-2H-oxecin-5-yl ester, was isolated from cultural filtrates of Trichoderma cremeum (Vinale et al., 2016). Two undescribed polyketides, (3R,7R)-7-hydroxy-de-O-methyllasiodiplodin (194) and (3R)-5-oxo-de-O-methyllasiodiplodin (195), were isolated from the cocultivation of Trichoderma sp. 307 and A. johnsonii B2 (Zhang et al., 2017). An undescribed polyketide, nafuredin C (196), and the known nafuredin A (197), were produced by marine fungus T. harzianum D13 (Zhao et al., 2020).
Other Compounds
Two new sulfur compounds, designated thioporidiols A (198) and B (199), were produced by a culture broth of T. polypori FKI-7382 (Figure 10) (Matsuo et al., 2020). Both of them were determined to be C13 lipid structures with an N-acetylcysteine moiety. A chemical investigation of the endophytic fungus T. polyalthiae offered two diphenyl ethers, Violaceol I (200) and II (201). Notably, both of them were characterized from Trichoderma for the first time (Nuankeaw et al., 2020). Trichodenols A (202) and B (203), two new compounds with 4-(2-hydroxyethyl) phenol moieties, were isolated from an endophyte T. gamsii (Ding et al., 2015).
New Strategies for Discovering Secondary Metabolites of Trichoderma
Recent fungal genome sequencing indicated that the majority of biosynthetic gene clusters (BGCs) associated with secondary metabolites are cryptic (transcriptionally silent) or expressed at very low levels under general laboratory conditions (Ren et al., 2017). Therefore, despite a large number of secondary metabolites being characterized from Trichoderma, genome sequencing revealed that there were more BGCs than we discovered, especially in filamentous fungi. These findings suggested that those silent metabolic pathways urgently need to be stimulated, which may lead to the discovery of novel metabolites with attractive functions. To activate cryptic biosynthetic pathways, many innovative approaches, such as cultivation-based approaches, metabolomic profiling, and genome mining-based molecular approaches, have been developed in recent years. These new approaches were accomplished with various degrees of success. The following are typical examples of searching for secondary metabolites of Trichoderma induced by cultivation regulation, cocultivation, chemical epigenetic manipulation, and transcript regulation, as shown in Figure 11.
The marine-derived fungus Trichoderma sp. TPU199 was found to produce a series of diketopiperazines under different conditions (Figure 12) (Yamazaki et al., 2020a). Chemical investigations of this fungal strain under ordinary culture conditions led to the discovery of compounds 169–177. Then, this fungus produced the halogenated gliovirin-type ETPs 120 (Cl derivative of 117), 122 (Br derivative of 117), and 123 (I derivative of 177) when added with NaCl, NaBr, and NaI in culture medium, respectively. Moreover, TPU199 supplemented with DMSO yielded 124, a new trithio derivative of 120. A continuous study indicated that, with the long time cultivation, an undescribed modified dipeptide 125 was obtained. Finally, two undescribed ETPs 126 and 127 were characterized under NaI-containing culture conditions. It is undoubtedly proven that changing the culture conditions can activate cryptic metabolic pathways.
Microorganism coculture based on microbial interspecies competition is an efficient path to stimulate cryptic BGCs. Cocultivation of Trichoderma sp. 307 and pathogenic bacterium A. johnsonii B2 yielded two undescribed sesquiterpenes (66 and 67) and de-O-methyllasiodiplodin (194 and 195) (Zhang et al., 2017). HPLC analysis indicated that they were derived from Trichoderma sp. rather than by the coculture. Chemical epigenetic manipulation has also proven to be an effective method to activate cryptic BGCs. Therefore, it was applied to the marine fungal strain T. harzianum XS-20090075 to mine its potential to synthesize secondary metabolites (Shi T. et al., 2020). A histone deacetylase inhibitor, sodium butyrate at 10 μM, significantly changed its metabolic profile and gave rise to three undescribed terpenoids, including a cleistanthane 105, a harziane diterpenoid 91, and a cyclonerane sesquiterpenoid 44. Interestingly, harziane diterpenoids were the dominant metabolites from this fungal strain under ordinary culture conditions, indicating the production of harzianes as the dominant metabolic pathway. In this study, the production of harzianes was hampered due to chemical epigenetic manipulation. In contrast, the biosynthetic pathways of cleistanthanes and cycloneranes were successfully activated, leading to the isolation of the new cleistanthane diterpenoid 105 and a series of cyclonerane sesquiterpenoids (44 and other known cycloneranes). This is the first report of cleistanthane diterpenoids isolated from Trichoderma species. This study provided solid example to show that it is efficient to activate the silent genes of Trichoderma species by chemical epigenetic manipulation.
The transcriptional control has also proven to be an effective approach. To activate the chemical potential of the endophytic fungus T. afroharzianum, a laeA-like gene overexpression transformant was built (Ding et al., 2020). Further chemical investigation of this transformant successfully yielded two new antifungal polyketides (189 and 190). This study indicated that transcriptional control could be a considerable strategy in activating more secondary metabolites and enhancing the silent potential metabolism of Trichoderma species.
Biological Activities
The producing fungus, environmental source, and bioactivities of compounds 1–203 are listed in Table 1. As shown in Table 1, most compounds possess various moderate to potent biological activities. Among them, antimicrobial, antimicroalgal, and anticancer activities represent dominant bioactivities to assess the pharmacological potential of these natural products. Detailed descriptions of these metabolites with promising biological activities are described as follows.
Antimicrobial Activities
Isolated trichothecene derivatives 1–14 were assayed for antifungal activity against Botrytis cinerea, Cochliobolus miyabeanus, Fusarium oxysporum f. sp. cucumerium, F. oxysporum f. sp. niveum, and Phomopsis asparagi (Shi Z.Z. et al., 2020). Compounds 1–3 and 9–11 displayed promising antifungal activity with MICs of 4.0–64 μg/mL. Among them, 10 was the most active, while compounds 4, 5, 8, and 12–14 were inactive. Structure–activity relationships (SAR) among these trichothecenes indicated that the acetoxy and methyl functionalities (compound 10) were necessary, while the epoxide moiety and the ether linkage were other possibilities (Shi Z.Z. et al., 2020). Compounds 15–17 showed antifungal activities against Candida albicans and Cryptococcus neoformans, with MICs of 1.6–50 μg/mL (Yamazaki et al., 2020a). In the same way, compounds 18 and 19 were active, with MICs of 6.3, 12.5, and 25 μg/mL, respectively (Yamazaki et al., 2020b). Apparently, the diene group and 2’Z-configuration play an important role in antifungal activities. Trichothecenes 22 and 23 showed significant activities toward the soil-borne phytopathogens Colletotrichum lagenarium with an MIC value of 16 μg/mL, which was stronger than that of the positive control carbendazim (MIC, 32 μg/mL) (Du et al., 2020). Furthermore, both of them showed potency against carbendazim-resistant B. cinerea. In contrast, compared to those of 22 and 23, trichothecene congener 24 only showed weak effects, indicating that the hydroxyl substituted in 23 may enhance its antifungal activity. Trichothecenes are reported to possess promising antifungal, phytotoxic and cytotoxic activities. Trichoderma-derived trichothecenes were mainly focused on their antifungal activity in the literature above, which highlighted their potential as biocontrol agents. Drimane sesquiterpenes 53–55 were active against C. miyabeanus by inducing hyphal branching at 1.0 and 10 μg/mL (Hirose et al., 2014). The novel norsesquiterpene 71 showed potent ability against C. lagrnarium with an MIC of 8 μg/mL (Du et al., 2020). The new harziane diterpene harzianol I (85) exhibited potent effect on Staphylococcus aureus, Bacillus subtilis, and Micrococcus luteus, with EC50s of 7.7, 7.7, and 9.9 μg/mL, respectively (Li W.Y. et al., 2020). It seemed that substitutions at C-2 and/or C-3 of harzianes may decrease their antibacterial activity. The novel norditerpene 103 inhibited S. aureus with an MIC of 12.4 μg/mL (Liang et al., 2016). Cyclopeptides 115 and 116 displayed weak ability against Enterococcus faecium with IC50s of 7.30 and 5.24 μM and against S. aureus with IC50s of 19.02 and 14.00 μM, respectively (Ding et al., 2012a). The new pyridine trichodin A (138) was active against B. subtilis (IC50, 27.05 μM), Staphylococcus epidermidis (24.28 μM), and C. albicans (25.38 μM) (Wu et al., 2014). Trichoharzianol (148) displayed mild activity against Colletotrichum gloeosporioides, with an MIC value of 128 μg/mL (Jeerapong et al., 2015). Polyketides 151 and 157 showed moderate activity against Escherichia coli, Edwardsiella tarda, Vibrio anguillarum, and Vibrio parahaemolyticus, with MICs of 8–64 μg/mL (Shi et al., 2017). Trichodermaketone A (162) was inactive against C. albicans (MIC > 125 μg/mL). However, it was active at 125 μg/mL when treated with 0.05 μg/mL ketoconazole (Song et al., 2010). New isocoumarins 184 and 185 exhibited growth inhibitory activity against E. coli with an MIC of 32 μg/mL (Ding et al., 2019). Polyketides 189 and 190 exhibited selective antifungal activity toward B. cinerea, F. oxysporum, and C. lagenarium, with MICs of 8–32 μg/mL (Ding et al., 2020). The new azaphilone 191 displayed moderate effect on S. aureus and B. subtilis with disc diameters of the zone of inhibition of 7.3 and 7.0 mm (Zhang S. et al., 2020). The new 10-membered lactone 193 significantly inhibited F. oxysporum, B. cinerea, and Rhizoctonia solani (Vinale et al., 2016). Nafuredins 196 and 197 showed strong activity against Magnaporthe oryzae, with MICs of 8.63 and 17.4 μM, respectively (Zhao et al., 2020).
Antimicroalgal Activities
The antimicroalgal activity against marine phytoplankton (Amphidinium carterae, Heterocapsa circularisquama, Heterosigma akashiwo, and Prorocentrum donghaiense) of trichothecene derivatives 1–14 was evaluated. Notably, 10 featured the strongest effect, with IC50s of 1.7, 0.82, 0.91, and 1.4 μg/mL (Shi Z.Z. et al., 2020). Carotane sesquiterpenes 27–29, 32, and 36 exhibited strong activity against several phytoplankton, with IC50s of 0.24–1.2 μg/mL (Shi et al., 2018a). Proposed SAR study indicated that the carbonyl, hydroxyl, and the epoxy moiety play an important role in the antimicroalgal potency of carotenes. The cyclonerane sesquiterpene 45 was more active against Karlodinium veneficum than 46, with an IC50 of 8.1 μg/mL (Liu X.H. et al., 2020). It is interesting that, compared to their isomerized derivatives (10E)- and (10Z)-cyclonerotriol, the isomerization of the five-membered ring greatly suppressed their antimicroalgal activities (Liu X.H. et al., 2020). Cycloneranes 47–51 exhibited moderate to potent growth inhibition against (Chattonella marina, H. akashiwo, K. veneficum, and P. donghaiense with low μg/mL range (Song et al., 2018). Compound 47 potently inhibited C. marina with an IC50 of 0.66 μg/mL. The new acorane sesquiterpene 68 exhibited mild growth inhibition of C. marina (IC50, 2.8 μg/mL), while the new proharziane diterpene 104 potently inhibited with IC50s of 1.2–4.3 μg/mL (Song et al., 2018). Additionally, the new harziane lactone 90 possessed potent activity, with IC50s of 0.53–2.7 μg/mL (Zou et al., 2021). The new isocoumarin 186 was active against C. marina (IC50, 4.4 μg/mL), H. akashiwo (9.1 μg/mL), and P. donghaiense (5.9 μg/mL) (Liu X.H. et al., 2020).
Anticancer Activities
The cytotoxicities of cadinane sesquiterpenes 38–43 were evaluated against NCIH-460, NCI-H929, and SW620 cell lines (Cui et al., 2020). In contrast to the known compounds 41–43, the newly reported sesquiterpenes 38–40 showed more potent cytotoxicities, with IC50s of 6.8–12.7 μM. Neomacrophorin I (53) showed cytotoxicity against human adenocarcinoma cells (COLO 201) with an IC50 of 46 μg/mL (Hirose et al., 2014). Neomacrophorins 56 and 61 were cytotoxic toward COLO 201 with IC50s of 20.5 and 18.2 μg/mL, respectively (Nishiyama et al., 2019). From a structural point of view, the common substructure of 2′-cyclohexene-1′,4′-dione was critical for cytotoxicity. The new harziane diterpene harzianol I (85) was observed to exhibit moderate cytotoxicity against NCI-H1975 (IC50, 58.72 μM), HepG2 (60.88 μM), and MCF-7 (53.92 μM) cell lines (Li W.Y. et al., 2020). 93 showed selective cytotoxicity toward HeLa and MCF-7, with IC50s of 30.1 and 30.7 μM, respectively (Zhang et al., 2016). Cyclodepsipeptides 106–111 were active on HT-29, A549, and P388, with IC50s of 0.7–19.1 μM (Liu Z. et al., 2020). Cytochalasans 171 and 172 showed cytotoxicity on HeLa with IC50s of 5.72 and 27.4 μM, respectively, whereas 169, 170, and 173–177 were inactive (IC50 > 40.0μM) (Ding et al., 2012b, c). The rare polyketide 182 displayed weak only cytotoxic activity toward A549 (IC50, 16.9 μM), HepG2 (30.8 μM), and HeLa (33.9 μM) (Chen et al., 2017). Cyclopentenone 183 displayed potent cytotoxicities against A549, NCI-H460, MCF-7, MDA-MB-435, HeLa, and DU-145, whereas it was inactive toward the normal human lung fibroblast cell line (You et al., 2010). The selectivity index was more than 100, which was even more remarkable than that of cisplatin.
Phytotoxic Activities
In phytotoxicity assays, harzianums A (20) and B (21) decreased the shoot and root lengths of the dicot species Brassica chinensis and induced inhibitory effect of seed germination at 2 μg/mL (Yin et al., 2020). Moreover, 20 and 21 showed phytotoxicity against monocots, Oryza sativa and Echinochloa crusgalli, compared with the positive control 2,4-dichlorophenoxyacetic acid (a chlorophenoxy herbicide most commonly used worldwide). The results indicated that 20 and 21 possess potent herbicidal potential for dicotyledon and/or monocotyledon weeds. All of the isolated harzianes 95–101 exhibited potent phytotoxicity at 200 ppm (Zhao et al., 2019).
Other Activities
Cyclonerane sesquiterpenes 51 and 52 exhibited certain nematicidal activity against Meloidogyne incognita, with second-stage juvenile (J2s) lethal rates of 38.2 and 42.7% at 200 μg/mL (Du et al., 2020). Cyclodepsipeptides 112–114 also showed nematicidal activity against M. incognita (Du et al., 2020). New sesquiterpenes 72 and 73 showed potent NO scavenging effects, with IC50s of 15.3 and 9.1 μM, respectively (Zheng et al., 2011). Harzianoic acids 80 and 81 exerted potency to decrease the HCV RNA with EC50s of 24.5 and 20.4 μM, respectively (Li et al., 2019). Trichothioneic acid (134) showed OH radical-scavenging and singlet oxygen-quenching ability in a dose-dependent manner, which was equivalent to those of positive controls (Miyano et al., 2020). The activity of harzianic acid (140) as a plant growth promoter was evaluated (Vinale et al., 2013). Treatment with 100 or 10 μM 140 significantly affected seed germination at 4 and 5 times stronger than that of the blank control. Naphthalene derivatives 145 and 147 showed moderate antifouling potency with EC50s of 29.8 and 35.6 μg/mL, respectively (Yu et al., 2021). The new de-O-methyllasiodiplodin 194 and 195 showed strong α-glucosidase inhibitory activity with IC50s of 25.8 and 54.6 μM, respectively, which were higher than acarbose (703.8 μM) (Zhang et al., 2017).
Summary of the Studies
Chemical Structures
A total of 203 natural products were reported from Trichoderma from 2009–2020. Their chemical structures were classified into terpenoids (1–24 for trichothecene sesquiterpenes, 25–36 for carotane sesquiterpenes, 37–43 for cadinane sesquiterpenes, 44–52 for cyclonerane sesquiterpenes, 53–65 for drimane sesquiterpenes, 66–81 for other sesquiterpenes, 82–102 for harziane diterpenes, and 103–105 for other diterpenes), cyclopeptides (104–116), diketopiperazines (117–133), alkaloids and other nitrogen-containing compounds (134–143), polyketides (144–150 for naphthalene derivatives, 151–168 for octaketides, 169–177 for cytochalasans, and 178–197 for other polyketides), and other compounds (198–203) according to their putative biogenetic sources. As shown in Figure 13A, 39.9% of the metabolites reported were sesquiterpenes, followed by polyketides with 26.6%. Taking diterpenes into account, terpenoids accounted for 51.72% of the obtained compounds, which indicated that species belonging to Trichoderma are considerable producing strains of novel terpenoids. It should be pointed out that some terpenoids, such as harzianes, are isolated exclusively from Trichoderma species. This review described 21 harziane diterpenes produced by Trichoderma. Considering their intriguing structures and bioactivities, much more attention should be devoted to this type of terpenoid in subsequent chemical studies.
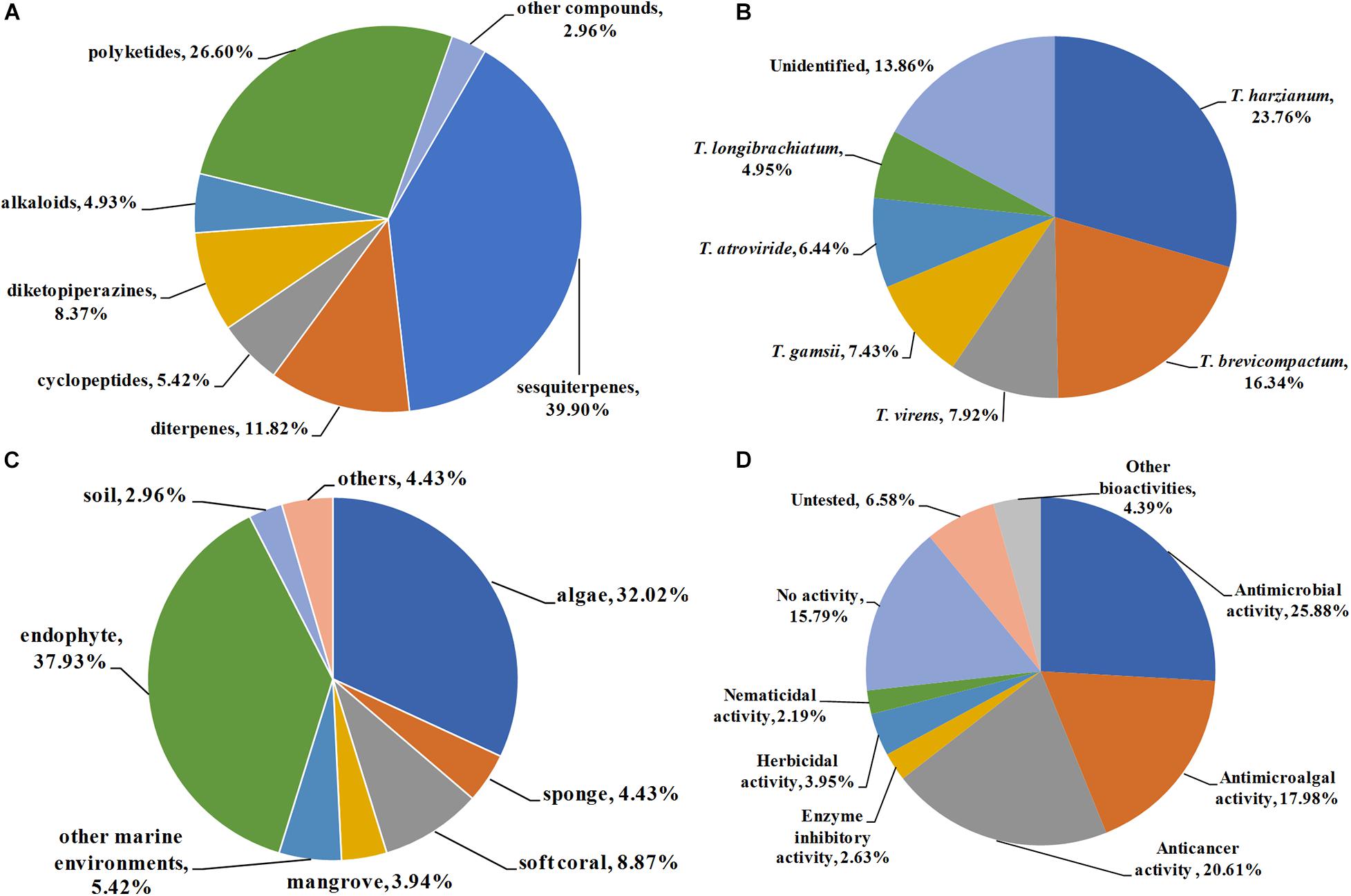
Figure 13. Quantification of this studies. (A) Chemical structures categories; (B) producing strains; (C) environment sources; and (D) bioactivity categories.
Producing Strains
The genus Trichoderma comprises more than 340 species. Some of them are used as biocontrol agents, while some of them are promising producers of enzymes for industrial purposes. On the other hand, some Trichoderma species possess the unique capacity to synthesize various secondary metabolites with potent biological activities. In this review, a total of 17 identified species, including T. harzianum, T. brevicompactum, T. virens, T. gamsii, T. atroviride, T. longibrachiatum, T. asperellum, T. koningiopsis, T. koningii, T. citrinoviride, T. neokongii, T. spirale, T. afroharzianum, T. polypore, T. polyalthiae, T. erinaceum, and T. cremeum, are reported as the producing strains of the described metabolites. Among them, T. harzianum and T. brevicompactum were the most prolific strains, with 48 (23.76%) and 33 (16.34%) metabolites identified, respectively (Figure 13B). The fungus T. harzianum is famous for widely used biocontrol agents, and it is also considered to be a promising producer of bioactive metabolic products. T. brevicompactum can synthesize trichothecene-type sesquiterpenoids with potent antifungal activity and high biotechnological value. Twenty-one novel trichothecenes (1–21) have been characterized from T. brevicompactum.
Environment Sources
The genus Trichoderma is widely distributed and has been isolated in soils, decaying wood, and endophytes in the inner tissue of host plants. Previous studies have mainly focused on terrestrial species of Trichoderma. However, Trichoderma from the marine environment are unexploited. It would be useful to examine marine-derived Trichoderma species since they may be induced to produce specific metabolites in hyperhaline environments. Accordingly, in recent years, increasing attention has been devoted to marine Trichoderma. As shown in Figure 13C, a total of 54.7% producing fungus were obtained from marine environments, including algae (32.02%), sponges (4.43%), soft corals (8.87), mangroves (3.94%), and other marine environments (5.42%, seawater, sediments), with 111 compounds characterized. Moreover, some fungi are obtained as endophytes from medicinal plants. Endophytic fungi, which harmoniously live in the inner tissues of their hosts without causing apparent diseases, are considered to be prolific sources of novel metabolites with remarkable pharmacological activities. It is estimated that 37.97% of these compounds were isolated from endophytic Trichoderma. From the above analysis, it can be concluded that marine environment and endophytes are more abundant sources of those productive strains.
Biological Activities
As discussed above, most of the presented compounds possess considerable biological activities, such as antimicrobial, antimicroalgal, anticancer, enzyme inhibitory, herbicidal, and nematicidal activities. Among them, antimicrobial (25.88%), anticancer (20.61%), and antimicroalgal (17.98%) activities were dominant in assessing the pharmacological potential of these metabolites (Figure 13D). It should be pointed out that a high proportion (73.40%) of the presented metabolites showed moderate to potent bioactivities. Even more importantly, a large number of them exhibit potent activities, which are higher than those of positive controls. For example, trichothecenes 22 and 23 showed higher antifungal effect on C. lagrnarium (MIC, 16 μg/mL) than the synthetic fungicide carbendazim (MIC, 32 μg/mL) (Du et al., 2020). Cyclopentenone 183 displayed potent cytotoxicities, whereas it was inactive toward the normal lung cell line (You et al., 2010). The selectivity index was even more remarkable than that of cisplatin, indicating 183 has high selective toxicity to cancer cell lines. Sesquiterpenes 72 and 73 showed potent NO scavenging effects (Zheng et al., 2011). These impressive bioactivities indicate that many of these compounds could be used as potential candidates for new drug discovery.
Conclusion
In the present review, we offer a detailed summary of recently isolated metabolites from Trichoderma from the beginning of 2009 to the end of 2020. As a result, a total of 203 metabolites are described herein, including their structural diversity and biological activities. Moreover, new strategies for discovering secondary metabolites of Trichoderma in recent years have also been discussed. Trichoderma has proven to be a treasure house of interesting secondary metabolites with medicinal importance. The biochemical studies of Trichoderma are untapped. Although a mass of metabolites have been isolated from Trichoderma species, the further excavation of those metabolites is worth expecting. By using new approaches to activate their silent gene clusters, including cultivation-based approaches, metabolomic profiling, and genome mining-based molecular approaches, an ever-increasing number of bioactive compounds will be obtained, which will be beneficial for the new drug discovery in the near future.
Author Contributions
J-LZ and W-LT wrote this manuscript. Q-RH, Y-ZL, M-LW, L-LJ, CL, XY, H-WZ, and G-ZC collected and reorganized the literature data. X-XZ supervised the research work and revised the manuscript. All authors reviewed the manuscript.
Funding
This work was financially supported by the National Key Research and Development Program of China (Grant Nos. 2016YFD0501010, 2017YFD0500806, and 2018YFD0501402), the Major Agricultural Applied Technological Innovation Projects of Shandong Province (to X-XZ), the Key Research and Development Plan of Yantai (Nos. 2020XDRH101, 2021YT06000060, 2021YT06000636, and 2018XSCC045), the Natural Science Foundation of Shandong Province (Nos. ZR201911120018 and ZR2014HL061), the Yantai Research Institute for Replacing Old Growth Drivers with New Ones (Grant No. 2020XJDN001), and the Innovation Team Project for Modern Agricultural Industrious Technology System of Shandong Province (SDAIT-11-10).
Conflict of Interest
The authors declare that the research was conducted in the absence of any commercial or financial relationships that could be construed as a potential conflict of interest.
Publisher’s Note
All claims expressed in this article are solely those of the authors and do not necessarily represent those of their affiliated organizations, or those of the publisher, the editors and the reviewers. Any product that may be evaluated in this article, or claim that may be made by its manufacturer, is not guaranteed or endorsed by the publisher.
Supplementary Material
The Supplementary Material for this article can be found online at: https://www.frontiersin.org/articles/10.3389/fmicb.2021.723828/full#supplementary-material
References
Bhardwaj, N., and Kumar, J. (2017). Characterization of volatile secondary metabolites from Trichoderma asperellum. J. Appl. Nat. Sci. 9, 954–959. doi: 10.31018/jans.v9i2.1303
Cai, F., Yu, G., Wang, P., Wei, Z., Fu, L., Shen, Q., et al. (2013). Harzianolide, a novel plant growth regulator and systemic resistance elicitor from Trichoderma harzianum. Plant Physiol. Biochem. 73, 106–113. doi: 10.1016/j.plaphy.2013.08.011
Chen, L., Niu, S. B., Li, L., Ding, G., Yu, M., Zhang, G. S., et al. (2017). Trichoderpyrone, a unique polyketide hybrid with a cyclopentenone-pyrone skeleton from the plant endophytic fungus Trichoderma gamsii. J. Nat. Prod. 80, 1944–1947. doi: 10.1021/acs.jnatprod.7b00190
Cui, J., Shang, R. Y., Sun, M., Li, Y. X., Liu, H. Y., Lin, H. W., et al. (2020). Trichodermaloids A-C, cadinane sesquiterpenes from a marine sponge symbiotic Trichoderma sp. SM16 fungus. Chem. Biodivers. 17:e2000036. doi: 10.1002/cbdv.202000036
Ding, G., Chen, A. J., Lan, J., Zhang, H., Chen, X., Liu, X., et al. (2012a). Sesquiterpenes and cyclopeptides from the endophytic fungus Trichoderma asperellum SAMUELS, LIECKF. & NIRENBERG. Chem. Biodivers. 9, 1205–1212. doi: 10.1002/cbdv.201100185
Ding, G., Chen, L., Chen, A., Tian, X., Chen, X., Zhang, H., et al. (2012b). Trichalasins C and D from the plant endophytic fungus Trichoderma gamsii. Fitoterapia 83, 541–544. doi: 10.1016/j.fitote.2011.12.021
Ding, G., Wang, H., Li, L., Chen, A. J., Chen, L., Chen, H., et al. (2012c). Trichoderones A and B: two pentacyclic cytochalasans from the plant endophytic fungus Trichoderma gamsii. Eur. J. Org. Chem. 2012, 2516–2519. doi: 10.1002/ejoc.201200053
Ding, G., Chen, L., Zhou, C., Hong-Mei, J., Liu, Y. T., Chang, X., et al. (2015). Trichoderamides A and B, a pair of stereoisomers from the plant endophytic fungus Trichoderma gamsii. J. Antibiot. 68, 409–413. doi: 10.1038/ja.2015.1
Ding, Z., Tao, T., Wang, L., Zhao, Y., Huang, H., Zhang, D., et al. (2019). Bioprospecting of novel and bioactive metabolites from endophytic fungi isolated from rubber tree ficus elastica leaves. J. Microbiol. Biotechnol. 29, 731–738. doi: 10.4014/jmb.1901.01015
Ding, Z., Wang, X., Kong, F. D., Huang, H. M., Zhao, Y. N., Liu, M., et al. (2020). Overexpression of global regulator talae1 leads to the discovery of new antifungal polyketides from endophytic fungus Trichoderma afroharzianum. Front. Microbiol. 11:622785. doi: 10.3389/fmicb.2020.622785
Du, F. Y., Ju, G. L., Xiao, L., Zhou, Y. M., and Wu, X. (2020). Sesquiterpenes and cyclodepsipeptides from marine-derived fungus Trichoderma longibrachiatum and their antagonistic activities against soil-borne pathogens. Mar. Drugs 18:165. doi: 10.3390/md18030165
Fang, F., Zhao, J., Ding, L., Huang, C., Naman, C. B., He, S., et al. (2017). 5-hydroxycyclopenicillone, a new β-amyloid fibrillization inhibitor from a sponge-derived fungus Trichoderma sp. HPQJ-34. Mar. Drugs 15:260. doi: 10.3390/md15080260
Fleming, A. (1929). On the antibacterial action of cultures of a Penicillium, with special reference to their use in the isolation of B. influenzae. Br. J. Exp. Pathol. 10, 226–236.
Frisvad, J. C. (2014). Taxonomy, chemodiversity, and chemoconsistency of Aspergillus, Penicillium, and Talaromyces species. Front. Microbiol. 5:773. doi: 10.3389/fmicb.2014.00773
Harwoko, H., Daletos, G., Stuhldreier, F., Lee, J., Wesselborg, S., Feldbrügge, M., et al. (2021). Dithiodiketopiperazine derivatives from endophytic fungi Trichoderma harzianum and Epicoccum nigrum. Nat. Prod. Res. 35, 257–265. doi: 10.1080/14786419.2019.1627348
Hirose, A., Maeda, H., Tonouchi, A., Nehira, T., and Hashimoto, M. (2014). Neomacrophorin I, II, and III, novel drimenyl cyclohexanes with hydroxylated butanoates from Trichoderma sp. 1212-03. Tetrahedron 70, 1458–1463. doi: 10.1016/j.tet.2013.12.087
Jeerapong, C., Phupong, W., Bangrak, P., Intana, W., and Tuchinda, P. (2015). Trichoharzianol, a new antifungal from Trichoderma harzianum F031. J. Agric. Food Chem. 63, 3704–3708. doi: 10.1021/acs.jafc.5b01258
Keswani, C., Mishra, S., Sarma, B. K., Singh, S. P., and Singh, H. B. (2014). Unraveling the efficient applications of secondary metabolites of various Trichoderma spp. Appl. Microbiol. Biotechnol. 98, 533–544. doi: 10.1007/s00253-013-5344-5
Khan, R. A., Najeeb, S., Hussain, S., Xie, B., and Li, Y. (2020). Bioactive secondary metabolites from Trichoderma spp. against phytopathogenic fungi. Microorganisms 8:817. doi: 10.3390/microorganisms8060817
Lang, B. Y., Li, J., Zhou, X. X., Chen, Y. H., Yang, Y. H., Li, X. N., et al. (2015). Koninginins L and M, two polyketides from Trichoderma koningii 8662. Phytochem. Lett. 11, 1–4. doi: 10.1016/j.phytol.2014.10.031
Li, B., Li, L., Peng, Z., Liu, D., Si, L., Wang, J., et al. (2019). Harzianoic acids A and B, new natural scaffolds with inhibitory effects against hepatitis C virus. Bioorg. Med. Chem. 27, 560–567. doi: 10.1016/j.bmc.2018.12.038
Li, D. L., Chen, Y. C., Tao, M. H., Li, H. H., and Zhang, W. M. (2012). Two new octahydronaphthalene derivatives from Trichoderma spirale, an endophytic fungus derived from Aquilaria sinensis. Helv. Chim. Acta 95, 805–809. doi: 10.1002/hlca.201100417
Li, W. Y., Liu, Y., Lin, Y. T., Liu, Y. C., Guo, K., Li, X. N., et al. (2020). Antibacterial harziane diterpenoids from a fungal symbiont Trichoderma atroviride isolated from Colquhounia coccinea var. mollis. Phytochemistry 170:112198. doi: 10.1016/j.phytochem.2019.112198
Li, X. Q., Xu, K., Liu, X. M., and Zhang, P. (2020). A systematic review on secondary metabolites of paecilomyces species: chemical diversity and biological activity. Planta Med. 86, 805–821. doi: 10.1055/a-1196-1906
Liang, X. R., Miao, F. P., Song, Y. P., Liu, X. H., and Ji, N. Y. (2016). Citrinovirin with a new norditerpene skeleton from the marine algicolous fungus Trichoderma citrinoviride. Bioorg. Med. Chem. Lett. 26, 5029–5031. doi: 10.1016/j.bmcl.2016.08.093
Liu, X. H., Hou, X. L., Song, Y. P., Wang, B. G., and Ji, N. Y. (2020). Cyclonerane sesquiterpenes and an isocoumarin derivative from the marine-alga-endophytic fungus Trichoderma citrinoviride A-WH-20-3. Fitoterapia 141:104469. doi: 10.1016/j.fitote.2020.104469
Liu, Z., Sun, Y., Tang, M., Sun, P., Wang, A., Hao, Y., et al. (2020). Trichodestruxins A-D: cytotoxic cyclodepsipeptides from the endophytic fungus Trichoderma harzianum. J. Nat. Prod. 83, 3635–3641. doi: 10.1021/acs.jnatprod.0c00808
Matsuo, H., Noguchi, Y., Miyano, R., Higo, M., Nonaka, K., Sunazuka, T., et al. (2020). Thioporidiols A and B: two new sulfur compounds discovered by molybdenum-catalyzed oxidation screening from Trichoderma polypori FKI-7382. Antibiotics 9:236. doi: 10.3390/antibiotics9050236
McMullin, D. R., Renaud, J. B., Barasubiye, T., Sumarah, M. W., and Miller, J. D. (2017). Metabolites of Trichoderma species isolated from damp building materials. Can. J. Microbiol. 63, 621–632. doi: 10.1139/cjm-2017-0083
Miyano, R., Matsuo, H., Mokudai, T., Noguchi, Y., Higo, M., Nonaka, K., et al. (2020). Trichothioneic acid, a new antioxidant compound produced by the fungal strain Trichoderma virens FKI-7573. J. Biosci. Bioeng. 129, 508–513. doi: 10.1016/j.jbiosc.2019.11.007
Newman, D. J., and Cragg, G. M. (2016). Natural products as sources of new drugs from 1981 to 2014. J. Nat. Prod. 79, 629–661. doi: 10.1021/acs.jnatprod.5b01055
Nishiyama, M., Maeda, H., Tonouchi, A., and Hashimoto, M. (2019). Neomacrophorin and premacrophorin congeners from Trichoderma sp. 1212-03. Tetrahedron 75, 2993–3000. doi: 10.1016/j.tet.2019.04.018
Nuankeaw, K., Chaiyosang, B., Suebrasri, T., Kanokmedhakul, S., Lumyong, S., and Boonlue, S. (2020). First report of secondary metabolites, Violaceol I and Violaceol II produced by endophytic fungus, Trichoderma polyalthiae and their antimicrobial activity. Mycoscience 61, 16–21. doi: 10.1016/j.myc.2019.10.001
Persoon, C. H. (1794). Neuer Versuch einer systematischen Einteilung der Schwämme. Racodium Römer’s Neues Magazin der Botanik. 1:123.
Reino, J. L., Guerrero, R. F., Hernández-Galán, R., and Collado, I. G. (2008). Secondary metabolites from species of the biocontrol agent Trichoderma. Phytochem. Rev. 7, 89–123. doi: 10.1007/s11101-006-9032-2
Ren, H., Wang, B., and Zhao, H. (2017). Breaking the silence: new strategies for discovering novel natural products. Curr. Opin. Biotechnol. 48, 21–27. doi: 10.1016/j.copbio.2017.02.008
Shi, T., Shao, C. L., Liu, Y., Zhao, D. L., Cao, F., Fu, X. M., et al. (2020). Terpenoids from the coral-derived fungus Trichoderma harzianum (XS-20090075) induced by chemical epigenetic manipulation. Front. Microbiol. 11:572. doi: 10.3389/fmicb.2020.00572
Shi, X. S., Wang, D. J., Li, X. M., Li, H. L., Meng, L. H., Li, X., et al. (2017). Antimicrobial polyketides from Trichoderma koningiopsis QA-3, an endophytic fungus obtained from the medicinal plant Artemisia argyi. RSC Adv. 7, 51335–51342. doi: 10.1039/C7RA11122C
Shi, Z. Z., Fang, S. T., Miao, F. P., Yin, X. L., and Ji, N. Y. (2018a). Trichocarotins A-H and trichocadinin A, nine sesquiterpenes from the marine-alga-epiphytic fungus Trichoderma virens. Bioorg. Chem. 81, 319–325. doi: 10.1016/j.bioorg.2018.08.027
Shi, Z. Z., Miao, F. P., Fang, S. T., Yin, X. L., and Ji, N. Y. (2018b). Sulfurated diketopiperazines from an algicolous isolate of Trichoderma virens. Phytochem. Lett. 27, 101–104. doi: 10.1016/j.phytol.2018.07.005
Shi, Z. Z., Liu, X. H., Li, X. N., and Ji, N. Y. (2020). Antifungal and antimicroalgal trichothecene sesquiterpenes from the marine algicolous fungus Trichoderma brevicompactum A-DL-9-2. J. Agric. Food Chem. 68, 15440–15448. doi: 10.1021/acs.jafc.0c05586
Song, F., Dai, H., Tong, Y., Ren, B., Chen, C., Sun, N., et al. (2010). Trichodermaketones A–D and 7-O-methylkoninginin D from the marine fungus Trichoderma koningii. J. Nat. Prod. 73, 806–810. doi: 10.1021/np900642p
Song, Y., Miao, F., Yin, X., and Ji, N. (2020). Three nitrogen-containing metabolites from an algicolous isolate of Trichoderma asperellum. Mar. Life Sci. Technol. 2, 155–160. doi: 10.1007/s42995-020-00030-6
Song, Y. P., Fang, S. T., Miao, F. P., Yin, X. L., and Ji, N. Y. (2018). Diterpenes and sesquiterpenes from the marine algicolous fungus Trichoderma harzianum X-5. J. Nat. Prod. 81, 2553–2559. doi: 10.1021/acs.jnatprod.8b00714
Takahashi-Ando, N., Matsui, K., Suzuki, T., Sadamatsu, K., Azuhata, H., Okada, A., et al. (2020). Trichothecene biosynthesis in different fungal genera: resistance mechanisms, pathway enzymes, and their product applications. JSM Mycotoxins 70, 67–74. doi: 10.2520/myco.70-2-3
Vinale, F., Nigro, M., Sivasithamparam, K., Flematti, G., Ghisalberti, E. L., Ruocco, M., et al. (2013). Harzianic acid: a novel siderophore from Trichoderma harzianum. FEMS Microbiol. Lett. 347, 123–129. doi: 10.1111/1574-6968.12231
Vinale, F., Strakowska, J., Mazzei, P., Piccolo, A., Marra, R., Lombardi, N., et al. (2016). Cremenolide, a new antifungal, 10-member lactone from Trichoderma cremeum with plant growth promotion activity. Nat. Prod. Res. 30, 2575–2581. doi: 10.1080/14786419.2015.1131985
Wu, B., Oesker, V., Wiese, J., Schmaljohann, R., and Imhoff, J. F. (2014). Two new antibiotic pyridones produced by a marine fungus, Trichoderma sp. strain MF106. Mar. Drugs 12, 1208–1219. doi: 10.3390/md12031208
Xie, Z. L., Li, H. J., Wang, L. Y., Liang, W. L., Liu, W., and Lan, W. J. (2013). Trichodermaerin, a new diterpenoid lactone from the marine fungus Trichoderma erinaceum associated with the sea star Acanthaster planci. Nat. Prod. Commun. 8, 67–68. doi: 10.1177/1934578X1300800116
Yamazaki, H., Saito, R., Takahashi, O., Kirikoshi, R., Toraiwa, K., Iwasaki, K., et al. (2015). Trichoketides A and B, two new protein tyrosine phosphatase 1B inhibitors from the marine-derived fungus Trichoderma sp. J. Antibiot. 68, 628–632. doi: 10.1038/ja.2015.44
Yamazaki, H., Takahashi, O., Kirikoshi, R., Yagi, A., Ogasawara, T., Bunya, Y., et al. (2020a). Epipolythiodiketopiperazine and trichothecene derivatives from the NaI-containing fermentation of marine-derived Trichoderma cf. brevicompactum. J. Antibiot. 73, 559–567. doi: 10.1038/s41429-020-0314-5
Yamazaki, H., Yagi, A., Takahashi, O., Yamaguchi, Y., Saito, A., Namikoshi, M., et al. (2020b). Antifungal trichothecene sesquiterpenes obtained from the culture broth of marine-derived Trichoderma cf. brevicompactum and their structure-activity relationship. Bioorg. Med. Chem. Lett. 30:127375. doi: 10.1016/j.bmcl.2020.127375
Yin, M., Fasoyin, O. E., Wang, C., Yue, Q., Zhang, Y., Dun, B., et al. (2020). Herbicidal efficacy of harzianums produced by the biofertilizer fungus, Trichoderma brevicompactum. AMB Express 10:118. doi: 10.1186/s13568-020-01055-x
You, J., Dai, H., Chen, Z., Liu, G., He, Z., Song, F., et al. (2010). Trichoderone, a novel cytotoxic cyclopentenone and cholesta-7, 22-diene-3β, 5α, 6β-triol, with new activities from the marine-derived fungus Trichoderma sp. J. Ind. Microbiol. Biotechnol. 37, 245–252. doi: 10.1007/s10295-009-0667-z
Yu, J. Y., Shi, T., Zhou, Y., Xu, Y., Zhao, D. L., and Wang, C. Y. (2021). Naphthalene derivatives and halogenate quinoline from the coral-derived fungus Trichoderma harzianum (XS-20090075) through OSMAC approach. J. Asian Nat. Prod. Res. 23, 250–257. doi: 10.1080/10286020.2020.1729752
Zeilinger, S., Gruber, S., Bansal, R., and Mukherjee, P. K. (2016). Secondary metabolism in Trichoderma–chemistry meets genomics. Fungal Biol. Rev. 30, 74–90. doi: 10.1016/j.fbr.2016.05.001
Zhang, L., Niaz, S. I., Khan, D., Wang, Z., Zhu, Y., Zhou, H., et al. (2017). Induction of diverse bioactive secondary metabolites from the mangrove endophytic fungus Trichoderma sp. (strain 307) by co-cultivation with Acinetobacter johnsonii (strain B2). Mar. Drugs 15:35. doi: 10.3390/md15020035
Zhang, M., Liu, J. M., Zhao, J. L., Li, N., Chen, R. D., Xie, K. B., et al. (2016). Two new diterpenoids from the endophytic fungus Trichoderma sp. Xy24 isolated from mangrove plant Xylocarpus granatum. Chin. Chem. Lett. 27, 957–960. doi: 10.1016/j.cclet.2016.02.008
Zhang, P., Wei, Q., Yuan, X., and Xu, K. (2020). Newly reported alkaloids produced by marine-derived Penicillium species (covering 2014-2018). Bioorg. Chem. 99:103840. doi: 10.1016/j.bioorg.2020.103840
Zhang, S., Sun, F., Liu, L., Bao, L., Fang, W., Yin, C., et al. (2020). Dragonfly-associated Trichoderma harzianum QTYC77 is not only a potential biological control agent of Fusarium oxysporum f. sp. cucumerinum but also a source of new antibacterial agents. J. Agric. Food Chem. 68, 14161–14167. doi: 10.1021/acs.jafc.0c05760
Zhao, D. L., Yang, L. J., Shi, T., Wang, C. Y., Shao, C. L., and Wang, C. Y. (2019). Potent phytotoxic harziane diterpenes from a soft coral-derived strain of the fungus Trichoderma harzianum XS-20090075. Sci. Rep. 9:13345. doi: 10.1038/s41598-019-49778-7
Zhao, D. L., Zhang, X. F., Huang, R. H., Wang, D., Wang, X. Q., Li, Y. Q., et al. (2020). Antifungal nafuredin and epithiodiketopiperazine derivatives from the mangrove-derived fungus Trichoderma harzianum D13. Front. Microbiol. 11:1495. doi: 10.3389/fmicb.2020.01495
Zheng, C. J., Sun, P. X., Jin, G. L., and Qin, L. P. (2011). Sesquiterpenoids from Trichoderma atroviride, an endophytic fungus in Cephalotaxus fortunei. Fitoterapia 82, 1035–1038. doi: 10.1016/j.fitote.2011.06.010
Zhou, P., Wu, Z., Tan, D., Yang, J., Zhou, Q., Zeng, F., et al. (2017). Atrichodermones A–C, three new secondary metabolites from the solid culture of an endophytic fungal strain, Trichoderma atroviride. Fitoterapia 123, 18–22. doi: 10.1016/j.fitote.2017.09.012
Zhou, X. X., Li, J., Yang, Y. H., Zeng, Y., and Zhao, P. J. (2014). Three new koninginins from Trichoderma neokongii 8722. Phytochem. Lett. 8, 137–140. doi: 10.1016/j.phytol.2014.03.004
Keywords: Trichoderma, secondary metabolites, chemical diversity, biological activity, bioactive compounds
Citation: Zhang J-L, Tang W-L, Huang Q-R, Li Y-Z, Wei M-L, Jiang L-L, Liu C, Yu X, Zhu H-W, Chen G-Z and Zhang X-X (2021) Trichoderma: A Treasure House of Structurally Diverse Secondary Metabolites With Medicinal Importance. Front. Microbiol. 12:723828. doi: 10.3389/fmicb.2021.723828
Received: 11 June 2021; Accepted: 28 June 2021;
Published: 23 July 2021.
Edited by:
Paola Angelini, University of Perugia, ItalyReviewed by:
Andreas Lazaros Chryssafidis, University of the State of Santa Catarina, BrazilWanping Chen, Georg-August-Universität Göttingen, Germany
Laith Khalil Tawfeeq Al-Ani, Universiti Sains Malaysia, Malaysia
Vivek Sharma, Chandigarh University, India
Copyright © 2021 Zhang, Tang, Huang, Li, Wei, Jiang, Liu, Yu, Zhu, Chen and Zhang. This is an open-access article distributed under the terms of the Creative Commons Attribution License (CC BY). The use, distribution or reproduction in other forums is permitted, provided the original author(s) and the copyright owner(s) are credited and that the original publication in this journal is cited, in accordance with accepted academic practice. No use, distribution or reproduction is permitted which does not comply with these terms.
*Correspondence: Xing-Xiao Zhang, zhangxingxiao@ldu.edu.cn
†These authors have contributed equally to this work