- 1College of Animal Science and Veterinary Medicine, Shenyang Agricultural University, Shenyang, China
- 2Laboratory of Gastrointestinal Microbiology, Jiangsu Key Laboratory of Gastrointestinal Nutrition and Animal Health, College of Animal Science and Technology, Nanjing Agricultural University, Nanjing, China
- 3Department of Laboratory Medicine and Pathobiology, University of Toronto, Toronto, ON, Canada
Alterations in mucosal microbiota and metabolites are critical to intestinal homeostasis and host health. This study used a combination of 16S rRNA gene sequencing and liquid chromatography-mass spectrometry (LC/MS) to investigate mucosal microbiota and their metabolic profiles in the ileum of Hu sheep fed different diets. Here, we randomly allocated 15 Hu sheep to three diets, a non-pelleted low-grain diet (control diet; CON), a non-pelleted high-grain diet (HG), and a pelleted high-grain diet (HP). After 60 days of treatment, ileal mucosal samples were collected for microbiome and metabolome analysis. The results of principal coordinate analysis and permutation multivariate analysis showed that there was a tendency for microbial differentiation between the CON and HG groups (P < 0.1), although no significant difference between the HG and HP groups was observed (P > 0.05). Compared with the CON diet, the HG diet decreased (P < 0.05) the abundance of some probiotic species (e.g., Sphingomonas and Candidatus Arthromitus) and increased (P < 0.05) the abundance of acid-producing microbiota (e.g., Succiniclasticum, Nesterenkonia, and Alloprevotella) in the ileal mucosa. Compared with the HG diet, the HP diet decreased (P < 0.05) the abundance of Alloprevotella and increased (P < 0.05) the abundance of Mycoplasma in the ileal mucosa. Furthermore, partial least squares discriminant analysis and orthogonal partial least-squared discriminant analysis indicated that different dietary treatments resulted in different metabolic patterns in the ileal mucosa of the CON, HG, and HP groups. The HG diet altered (VIP > 1 and P < 0.05) the metabolic patterns of amino acids, fatty acids, and nucleotides/nucleosides (such as increased amounts of ornithine, tyrosine, cis-9-palmitoleic acid, and adenosine) compared with the CON diet. However, 10 differential metabolites (VIP > 1 and P < 0.05; including tyrosine, ornithine, and cis-9-palmitoleic acid) identified in the HG group exhibited a diametrically opposite trend in the HP group, suggesting that the HP diet could partially eliminate the changes brought upon by the HG diet. Collectively, our findings demonstrate that different diets altered the ileal mucosal microbiota and metabolites and provide new insight into the effects of high-grain diets on the intestinal health of ruminant animals.
Introduction
Currently, a high-grain (HG) feeding is often used during the breeding of ruminant species as it improves production performance and has economic benefits. A series of studies have revealed fundamental knowledge on the effects of HG diets on the ruminant digestive tract (mainly the rumen and hindgut), including effects on microbial composition and function, metabolism, and epithelial gene expression (Plaizier et al., 2012; Liu et al., 2014; Plaizier et al., 2017; Wang et al., 2021). However, the application of HG diets while ensuring the health of ruminant animals is an important issue that needs to be resolved for the breeding of ruminants.
With the development of lamb breeding programs with increased productivity, feeding of the animals with pelleted concentrates has been promoted and implemented in many modern fattening systems (Islam et al., 2017). Recently, accumulating evidence has indicated that the application of pelleted concentrates is an effective method to avoid selective eating, reduce nutrition wastage during storage and feeding, save labor, and increase rearing profitability (Blanco et al., 2015; Zhong et al., 2018; Li et al., 2021b). Previous studies have demonstrated that pelleted HG diets improve rumen fermentation and amplify the use of simple sugars by rumen microbiota (Trabi et al., 2019), although information regarding the impact of pelleted HG diets on the small intestine is limited.
Intestinal mucosa physically separates the inimical intestinal contents from the host, and also severs as the interface for the crosstalk, such as chemical dialogue through metabolites, between microbiota and their host (Wells et al., 2011; Xie et al., 2013). Previous studies have shown that mucosal host-microbiota metabolic interactions are crucial to intestinal homeostasis and host health (Yuan et al., 2020). For instance, metabolic and microbial changes in the intestinal mucosa of humans with some diseases (such as inflammatory bowel disease) exhibit interdependent and co-directional relationships (McHardy et al., 2013; Ahmed et al., 2016); probiotics such as bifidobacteria and lactobacillus can inhibit the proliferation of pathogenic bacteria and regulate the intestinal immunity by directly adhering to the intestinal mucosa and their metabolites (He et al., 2001; Hammes and Hertel, 2006). For animals, various factors, especially diets, have important effects on the intestinal microbiota and its metabolites (Wang et al., 2019; Yao et al., 2021). Hence, understanding the different dietary effects on intestinal mucosal microbiota and metabolites would provide favorable information for the subsequent development of dietary probiotics in ruminants. However, limited information is currently available regarding the mucosal microbial and metabolic changes in the small intestine of ruminants under HG or pelleted HG diets.
In the present study, we used the combination of 16S rRNA gene sequencing and liquid chromatography-mass spectrometry (LC/MS) to investigate mucosal microbiota and their metabolic profiles in the ileum of Hu sheep fed low-grain, pelleted or non-pelleted high-grain diets. Our findings contribute to a deeper understanding of the impacts of high-grain diets, and improve our understanding of mucosal host-microbiota metabolic interactions.
Materials and Methods
Experimental Design, Animals and Diets
All animal experimentation was conducted in accordance with the Experimental Animal Welfare Ethics Committee of Shenyang Agricultural University. The present study was part of a series of studies designed to evaluate the effects of high-grain diets on the physiological health and microbial community of the gastrointestinal tract, with detailed experimental design, animals and diets described in previous studies (Trabi et al., 2019; Trabi et al., 2020). Briefly, a total of 15 male Hu-breed lambs, initial body weight (26.80 ± 0.32 kg), were randomly assigned to a control diet (non-pelleted low-grain diet; CON; n = 5), a non-pelleted high-grain diet (HG; n = 5), or a pelleted high-grain diet (HP; n = 5). The CON group were fed a control diet containing 30% mashed concentrate and 70% forage, while the HG group were fed a high-grain diet containing 70% mashed concentrate and 30% forage. The ingredients of the HP diet were same as the HG diet, but in pelleted form. The pelleting process was conducted using a pelleting machine (Jiangsu Muyang Group Co., Ltd., China) with a diameter of 3.2 mm. All sheep were placed in individual pens (1.2 m × 1.2 m), and experimental diets and water were supplied ad libitum throughout the 60-day experimental period. Ingredients and nutritional compositions of the experimental diets were presented in Supplementary Table 1.
Sample Collection
On day 60 of treatment, animals from each treatment group were euthanized by captive bolt stunning at 4–5 h after the morning feeding. Immediately after slaughter and dissection, ileum tissue was washed with ice cold sterile phosphate-buffered saline until no digesta was visible, then mucosal samples were scraped using sterile slides. Ileal mucosal samples were homogenized and mixed thoroughly, with a representative sample collected into sterile tubes, flash frozen in liquid nitrogen, and stored at −80°C until DNA extraction and metabolome measurement.
DNA Extraction, Illumina Sequencing, and Bioinformatics Analysis
Extraction of total genomic DNA from the ileal mucosa was performed following the standard protocol of the QIAamp DNA stool Mini Kit (QIAGEN, Hilden, Germany). The integrity and concentration of extracted DNA was measured using 1% agarose gel electrophoresis and a NanoDrop spectrophotometer (Nyxor Biotech, Paris, France). The hypervariable V3-V4 region in the bacterial 16S rRNA of all samples were targeted for PCR amplification using the bacterial universal primers 338F (5′-ACTCCTRCGGGAGGCAGCAG-3′) and 806R (5′-GGACTACCVGGGTATCTAAT-3′) (Mao et al., 2015). After amplification, PCR products from 3 replicates for each sample were mixed and then purified using the AxyPrep DNA Gel Extraction Kit (Axygen Biosciences, Union City, CA, United States). 16S rRNA sequencing was performed on an Illumina Miseq platform using standard protocols.
Raw sequence reads were demultiplexed and quality-filtered using Quantitative Insights into Microbial Ecology (QIIME) version 1.8.0-dev (Caporaso et al., 2010). Paired-end reads were merged using FLASH (version 1.2.7) (Magoc and Salzberg, 2011), with a minimum overlap of 10 bp and a maximal expected error of 0.2. Harvested sequences were clustered into OTUs at a similarity level of 97% using UPARSE (version 7.11) (Edgar, 2013) and chimeras were removed using UCHIME (Edgar et al., 2011). The taxonomic analysis on the OTU representative sequences was performed using the Ribosomal Database Project (RDP) classifier (Wang et al., 2007), and the SILVA database (version 119) was used for comparisons of the 16S bacteria (DeSantis et al., 2006). Richness and diversity of the microbial communities were reflected by indices such as Chao 1, Shannon index, and Simpson. Sequence data generated in the current research had been deposited into the Sequence Read Archive (SRA) database under accession no. PRJNA734004.
Liquid Chromatography-Mass Spectrometry (LC/MS) Analysis
Prior to LC-MS analysis, 50 mg of mucosa sample was transferred into a 1.5-mL Eppendorf tube, mixed with 800 μL methanol by ultrasonication for 90 s at 65 Hz at 4°C for 10 min, and then centrifuged at 12000 rpm at 4°C for 15 min. Finally, after a series of pretreatments, 200 μL of the supernatant and 5 μL DL-o-Chlorophenylalanine (0.14 mg/ml, as interior label) were transferred into the injection vial for the LC/MS analysis. Metabolomic analysis was conducted using a LC-MS system (Waters, UPLC; Thermo, Q Exactive) with a Waters Acquity UPLC HSS T3 column (100 mm × 2.1 mm, 1.8 μm). The column temperature was maintained at 40°C, and the flow rate was set to 0.3 mL/min. The mobile phase A was water with 0.05% (v/v) formic acid, and the mobile phase B was acetonitrile. During measurement, the injection volume of each sample was 6 μL, and the automatic injector temperature was set as 4°C.
The LC-MS system was equipped with both positive electrospray ionization (ESI+) and negative electrospray ionization (ESI-) modes. The measurement parameters for ESI+ mode were: Heater temperature 300°C; Sheath gas flow rate 45 arb, aux gas flow rate 15 arb, sweep gas flow rate 1 arb, spray voltage 3.0 KV, capillary temperature 350°C and S-Lens RF level 30%. Spray voltage and S-Lens RF level for the ESI- mode were set to 3.2 KV and 60%, with the other parameters the same as used for the ESI+ mode. Raw data was extracted and preprocessed using Compound Discoverer 2.0 software (Thermo), and normalized according to the interior label using Excel 2010 software. Harvested data included sample names, peak intensity, retention time (RT) and compound molecular weight, and metabolite identification was performed using the Kyoto Encyclopedia of Genes and Genomes Database2 and the online Human Metabolome Database3.
Statistical Analysis
All statistical analysis of the experimental data was performed using SPSS software (SPSS v. 20.0, SPSS Inc., Chicago, IL, United States). Differences among the microbial communities from the different experimental groups were detected by a principal coordinate analysis (PCoA) and the permutation multivariate analysis (PERMANOVA). Significance of the microbial differences at the phylum and genus level were identified using a Wilcoxon-Mann-Whitney U test. Featured OTUs in each experimental group were identified using a linear discriminant analysis effect size (LEfSe) analysis.
Normalized peak intensities of the metabolites were imported into SIMCA-P + 14.1 software (Umetrics, Umea, Sweden) for partial least squares discriminant analysis (PLS-DA) and orthogonal partial least-squared discriminant analysis (OPLS-DA). Metabolites at different abundances between groups were validated using the Variable importance in Projection (VIP) generated from PLS-DA and a Wilcoxon-Mann-Whitney U test (VIP > 1 and P < 0.05). Spearman rank correlation coefficients (r) between the differential metabolites and OTUs were calculated using a R program with a significance threshold of | r| > 0.6 and P < 0.05 adopted, and visualization of the correlation network was performed using Gephi 0.8.2 software4.
Results
General Sequencing Observations
In the present study, a total of 696,525 valid V3-V4 16S rRNA reads were obtained for further analysis after sequencing, with an average of 46,435 sequences per sample. Rarefaction curves of the ileal mucosal microbiota were conducted, and the results showed that the samples provided sufficient sequences to detect most of the microbial species (Supplementary Figure 1). Estimates of microbial richness and diversity showed that no significant differences were observed among the different dietary treatments (Supplementary Table 2). Results of the PCoA (Figures 1A,B) combined with PERMANOVA illustrated that there were certain microbial differences between the CON and HG groups (P = 0.082), with no significant differences between the HG and HP groups (P = 0.662).
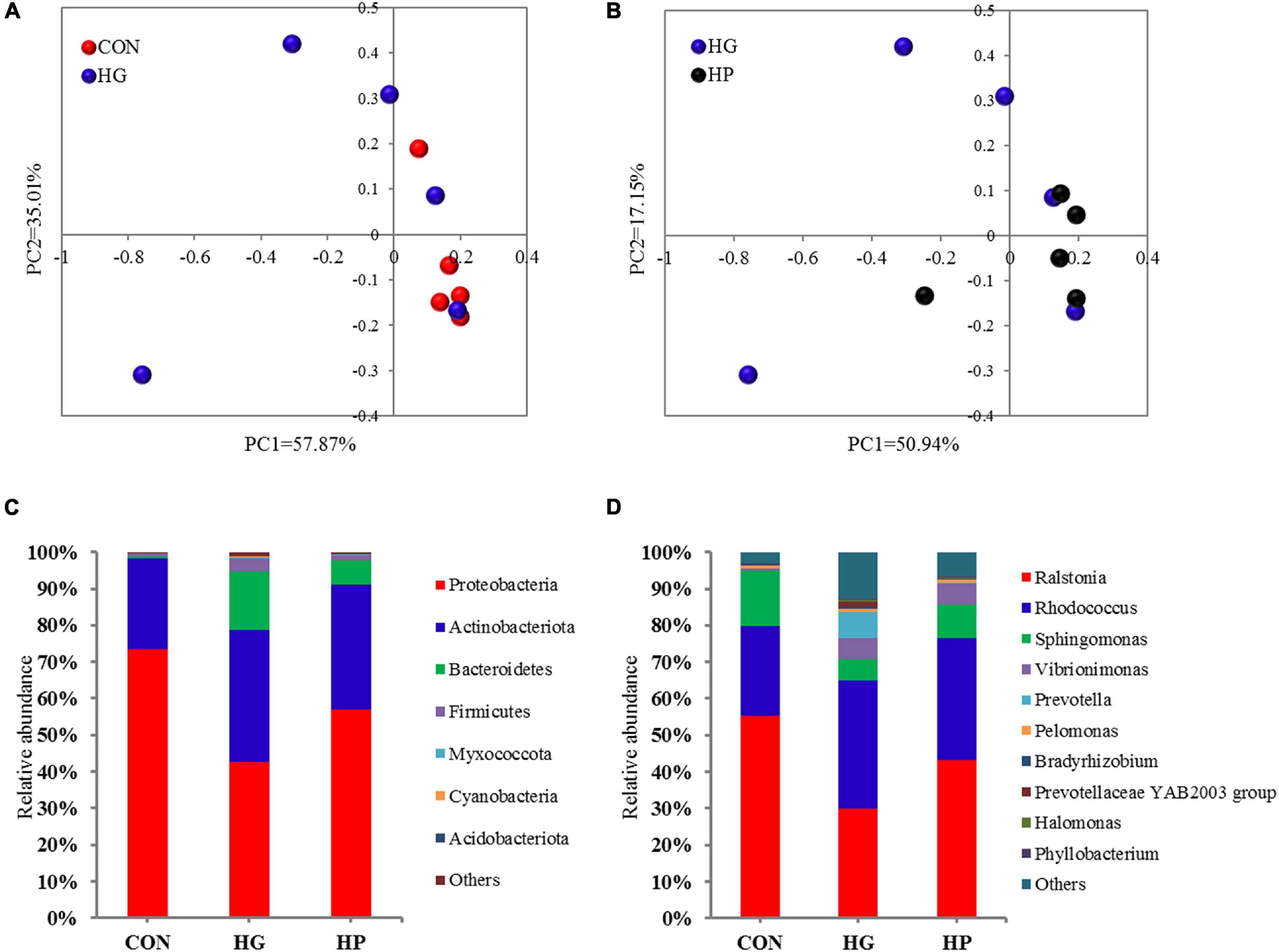
Figure 1. Taxonomic analysis of the microbial communities in the ileal mucosa of Hu sheep fed CON, HG, and HP diets. Principal coordinate analysis (PCoA) score plots of the samples from the CON and HG group (A) and the HG and HP group (B) based on the Bray-Curtis similarity metric. The composition of the ileal mucosal microbiota at the phylum (C) and genus (D) levels.
Alterations in Microbial Composition of the Ileal Mucosa After Different Dietary Treatments
To further evaluate the effects of different diets on ileal mucosal microbiota, we conducted a statistical analysis at different microbial taxonomic levels. At the phylum level (Figure 1C), the phyla Proteobacteria (average 57.80%), Actinobacteriota (average 31.46%), Bacteroidetes (average 7.74%), and Firmicutes (average 1.96%) occupied dominant positions for all three dietary groups. The relative abundance of Bacteroidetes tended to increase in the HG group compared to the CON group (P = 0.056), while the other phyla had no significant differences among the CON, HG, and HP groups (P > 0.05). At the genus level (Figure 1D), regardless of dietary treatment, Ralstonia (average 42.82%), Rhodococcus (average 30.87%), Sphingomonas (average 10.06), Vibrionimonas (average 3.97), and Prevotella (average 2.51%) were the most dominated genera in the ileal mucosal microbiota. As for genus-specific differences (Table 1), a significant decrease (P < 0.05) in the relative abundance of Sphingomonas, Candidatus Arthromitus, and [Eubacterium] coprostanoligenes group_norank, and a significant increase (P < 0.05) in the relative abundance of Succiniclasticum, Nesterenkonia, Alloprevotella, Labrys, and Paracoccus, were observed in the HG group compared with the CON group. In addition, a lower relative abundance of Alloprevotella (P < 0.05) and a higher relative abundance of Mycoplasma (P < 0.05) were observed in the HP group compared with the HG group.
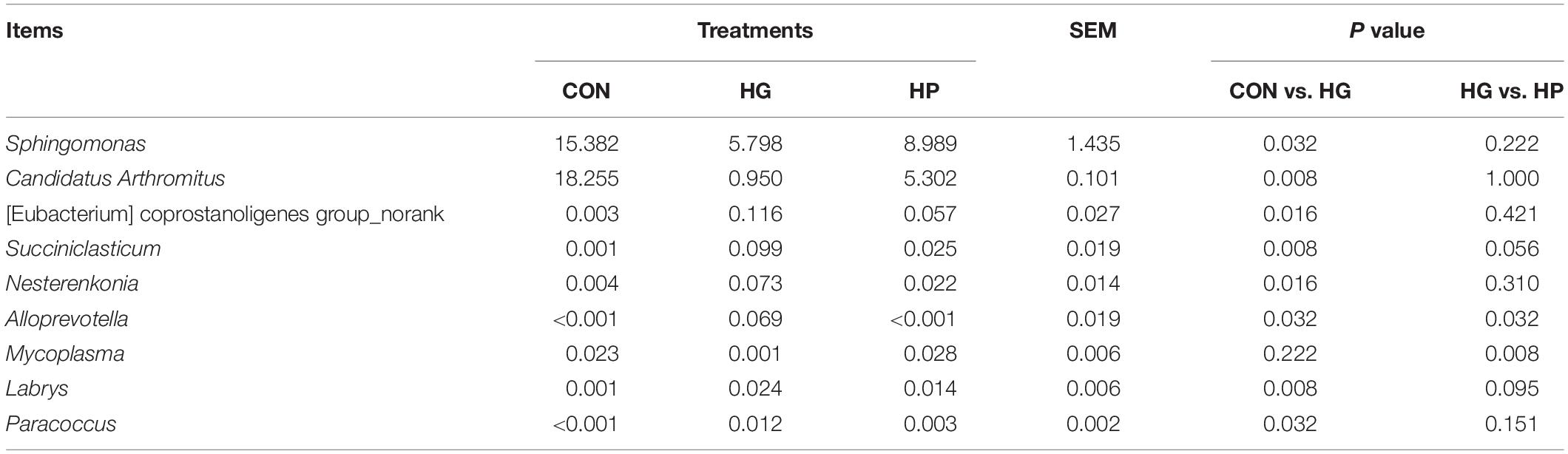
Table 1. Relative abundance of major genera (>0.01% in at least one group) in the ileal mucosal microbiota of Hu sheep fed CON, HG, and HP diets.
At the OTU level, a LEfSe analysis was performed to identify the featured microbial species in the different dietary treatments (Figure 2). Our results showed that 24 OTUs were significantly affected by dietary treatment (P < 0.05), and these OTUs accounted for 11.63, 0.09, and 0.50% of the total sequences in the CON, HG, and HP groups, respectively. Briefly, 14 OTUs (11 OTUs belonging to the genus Sphingomonas, 2 OTUs belonging to the genus Ralstonia, and 1 OTU belonging to the genus C. Arthromitus) were featured in the CON group (P < 0.05). For the HG group, OTU379 (S: Labrys neptuniae), OTU275 (S: Nesterenkonia sp.), and OTU652 (G: Rikenellaceae RC9 gut group) were the featured ileal mucosal microbiota (P < 0.05). Moreover, OTU42 (S: Bifidobacterium choerinum), OTU119 (G: Lachnospiraceae NK3A20 group), OTU659 and OTU1165 (S: Ralstonia solanacearum), OTU19 and OTU15 (G: Olsenella), and OTU147 (G: Vibrionimonas) were featured in the HP group (P < 0.05).
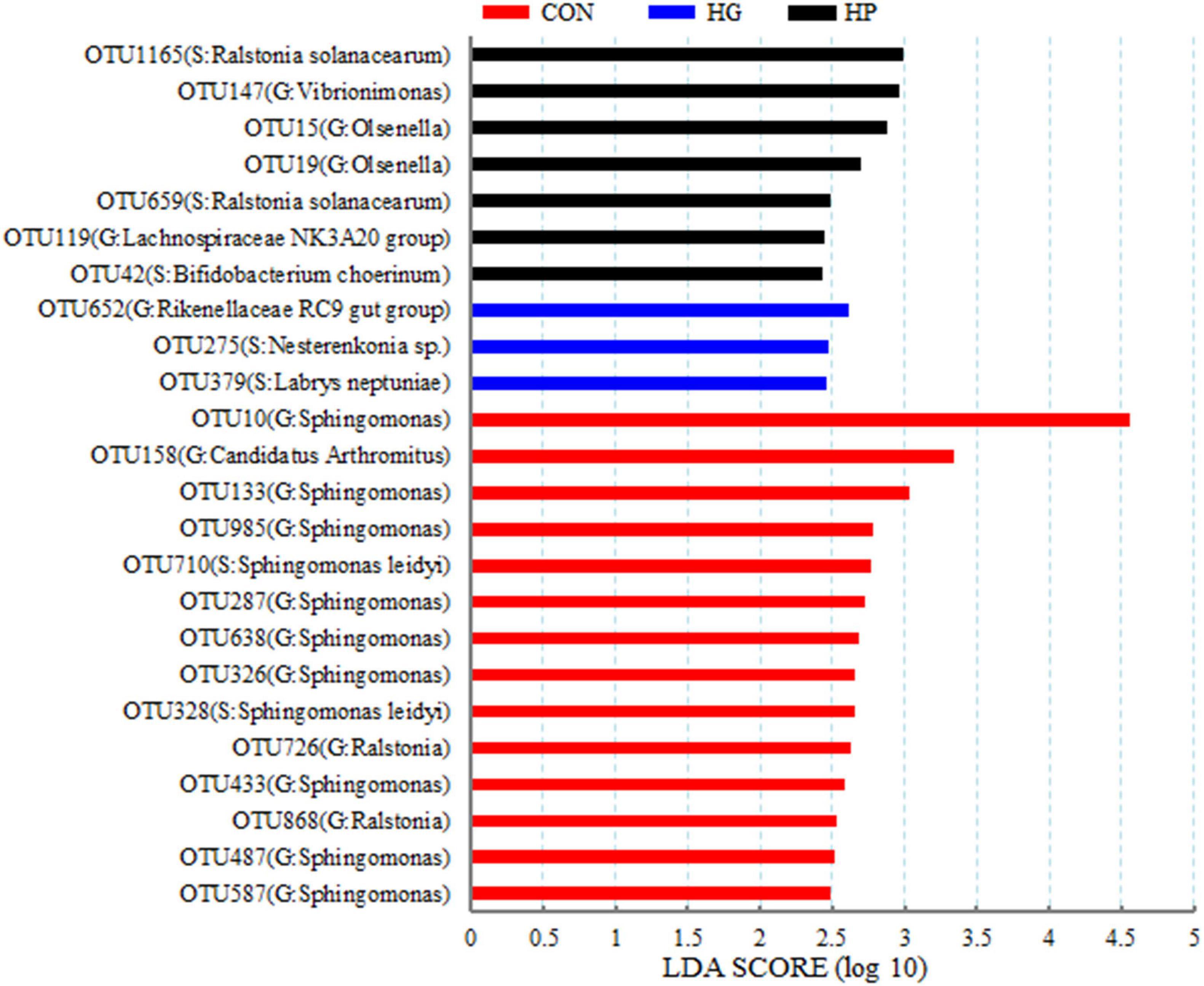
Figure 2. Linear discriminant analysis (LDA) scores of the microbial OTUs in the ileal mucosa of Hu sheep fed CON, HG, and HP diets.
Changes in the Metabolic Profiles of Ileal Mucosa After Different Dietary Treatments
To determine whether changes in microbial composition resulted in metabolic alterations, a LC-MS based metabolome analysis was used to reveal the metabolic profiles of the ileal mucosa with the different diets. After quality control and identification, a total of 238 reliable metabolites were found in the mucosal samples, which included organic acids, amino acids and their derivatives, fatty acids, sugars and their derivatives, amines, nucleoside, and others. PLS-DA and OPLS-DA score plots (Figure 3) of the identified metabolites in the mucosal samples revealed a clear separation between the CON and HG groups, as well as between the HG and HP groups, indicating that the different dietary treatments had a great impact on the mucosal metabolites. Moreover, the PLS-DA loading plots showed that the distribution of the important mucosal metabolites (VIP > 1.0). To assess the specific effects of the dietary treatments on mucosal metabolites, VIP values obtained from the PLS-DA analysis, combined with the statistical analysis, were used to screen for differential metabolites. In total, 47 metabolites (VIP > 1.0 and P < 0.05) were significantly affected by dietary treatments (Figure 4). In general, these results revealed that the major differential metabolites among the different dietary treatments were amino acids and their derivatives, fatty acids, and nucleosides/nucleotides, followed by amines, sugars and their derivatives, and others.
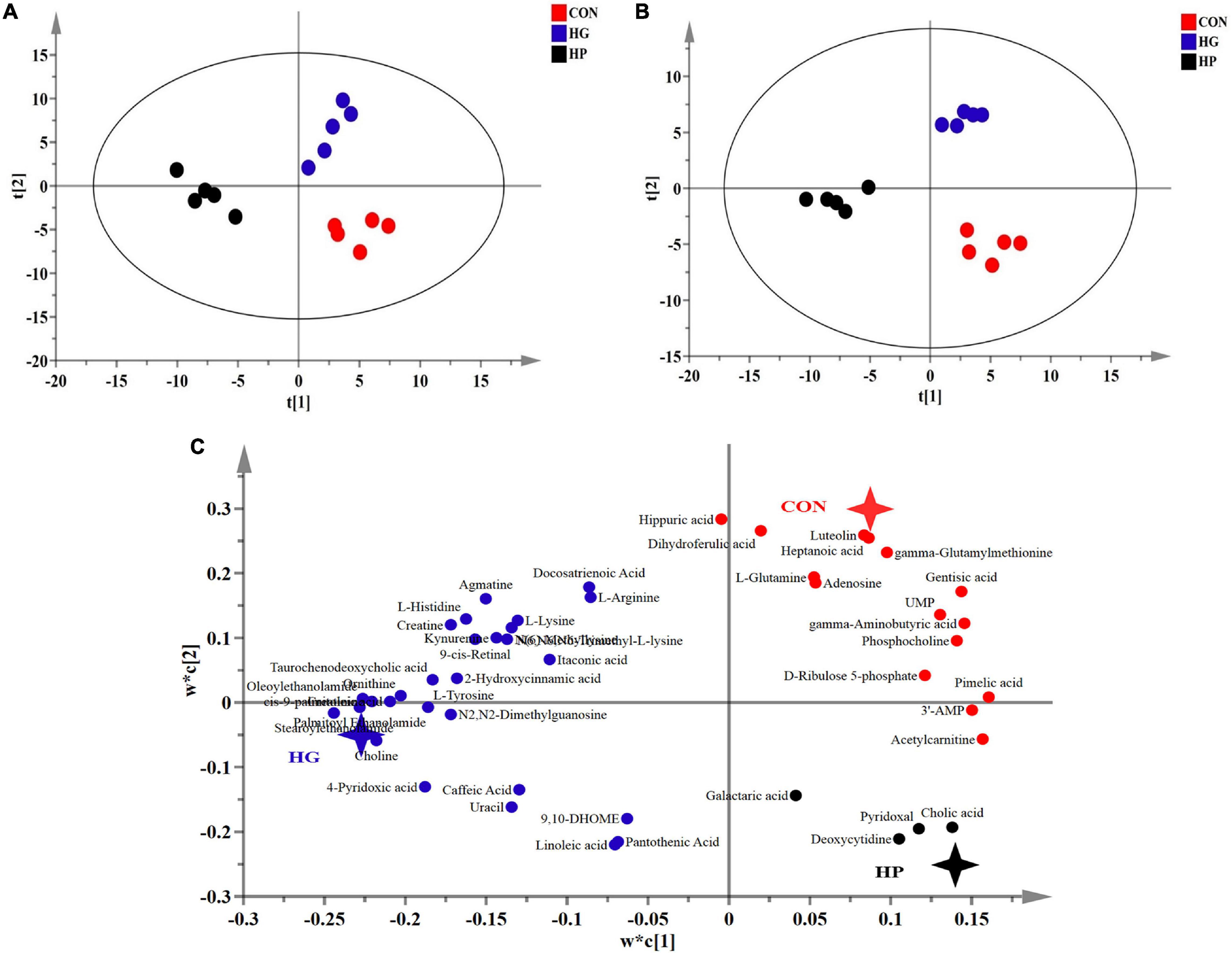
Figure 3. Partial least squares discriminant (PLS-DA) (A, score plots; C, loading plots) and orthogonal partial least squares discriminant (OPLS-DA; B) analysis of the ileal mucosal metabolites from Hu sheep fed CON, HG, and HP diets.
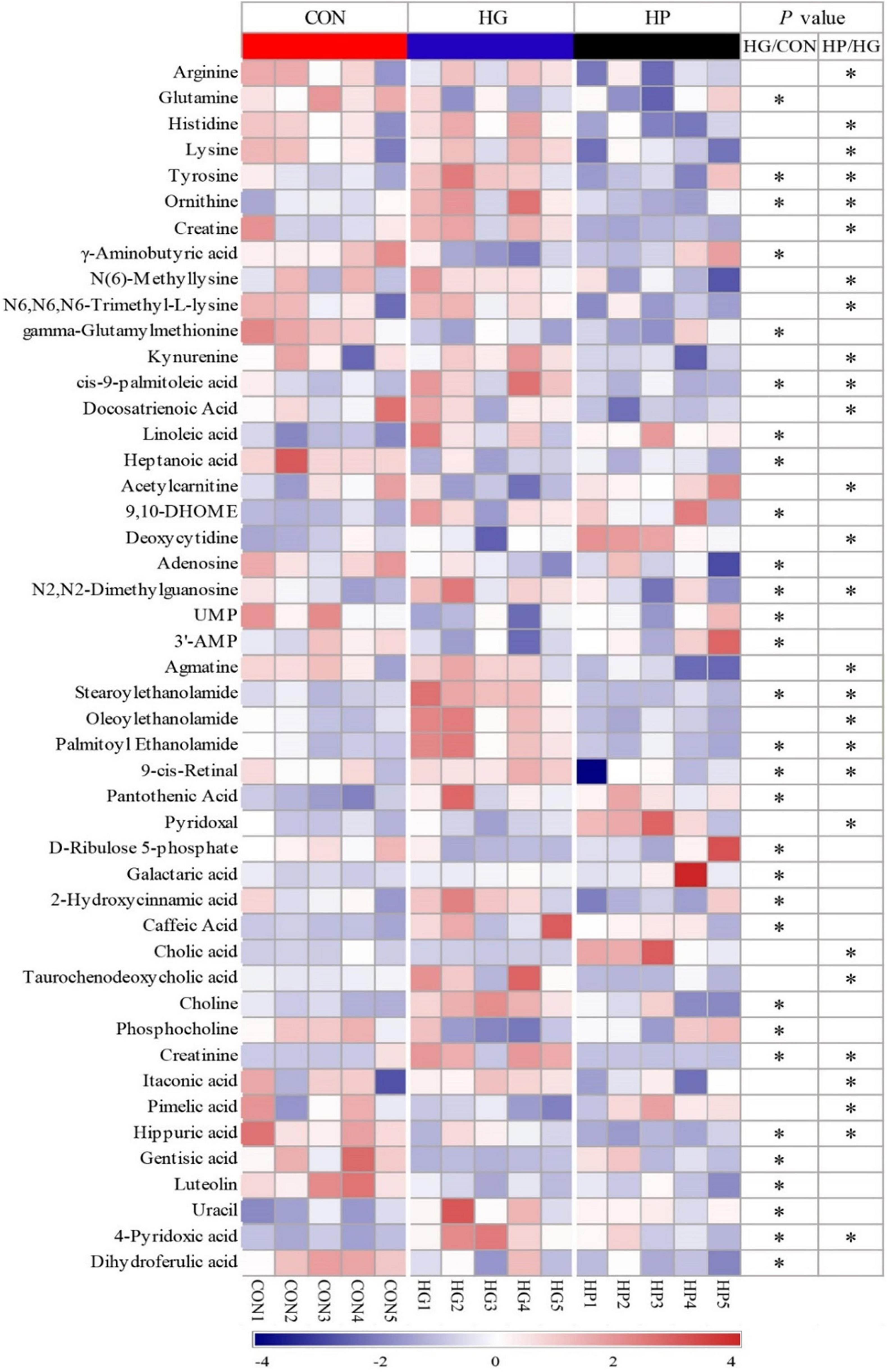
Figure 4. Heat-map visualizing of the differential metabolites identified in the ileal mucosal samples of sheep fed CON, HG, and HP diets.
For amino acids and their derivatives (Figure 4), the HG diet significantly increased (P < 0.05) the content of ornithine and tyrosine, while significantly decreasing (P < 0.05) the content of glutamine and γ-aminobutyric acid compared with the CON diet. HP diet significantly decreased (P < 0.05) the content of arginine, histidine, lysine, tyrosine, ornithine, creatine, N(6)-Methyllysine, and N6, N6, N6-Trimethyl-L-lysine compared with the HG diet. Additionally, HG diet significantly increased (P < 0.05) the content of stearoylethanolamide, and palmitoyl ethanolamide compared with the CON diet, while HP diet significantly decreased (P < 0.05) the content of agmatine, stearoylethanolamide, oleoylethanolamide, and palmitoyl ethanolamide compared with the HG diet. Moreover, a significant increase in the content of choline and a significant decrease in the content of phosphocholine were observed in the HG group compared with the CON diet.
For fatty acids, a significant increase (P < 0.05) in the content of cis-9-palmitoleic acid, linoleic acid, and 9,10-DHOME, and a significant decrease (P < 0.05) in the content of heptanoic acid, were observed in the HG group compared with the CON group. A significant decrease (P < 0.05) in the content of cis-9-palmitoleic acid and docosatrienoic acid were observed in the HP group compared with HG group. Meanwhile, among metabolites related to lipid digestion, HP diet substantially increased the content of cholic acid (FC = 30.28, P < 0.05) and decreased the content of taurochenodeoxycholic acid (FC = 0.13, P < 0.05) in the ileal mucosa compared with HG group. For nucleosides/nucleotides, HG diet significantly increased the content of N2,N2-dimethylguanosine, while decreasing (P < 0.05) the content of adenosine, UMP, and 3′-AMP compared with the CON diet. HP diet significantly increased (P < 0.05) the content of deoxycytidine, and significantly decreased (P < 0.05) the content of N2,N2-dimethylguanosine compared with the HG diet.
Interestingly, our results also found that a total of 11 differential metabolites were simultaneously identified in the HG group (compared with the CON group) and the HP group (compared with the HG group), with the fold change of these common differential metabolites presented in Figure 5. These results showed that, except for hippuric acid, the fold change of these 10 metabolites (tyrosine, ornithine, cis-9-palmitoleic acid, N2,N2-Dimethylguanosine, stearoylethanolamide, palmitoyl ethanolamide, 9-cis-Retinal, creatinine, 4-pyridoxic acid, and 2-hydroxycinnamic acid) in the HG and HP groups were exhibited a diametrically opposite directions. It seems that the HP diet partially eliminates the changes brought on by the HG diet.
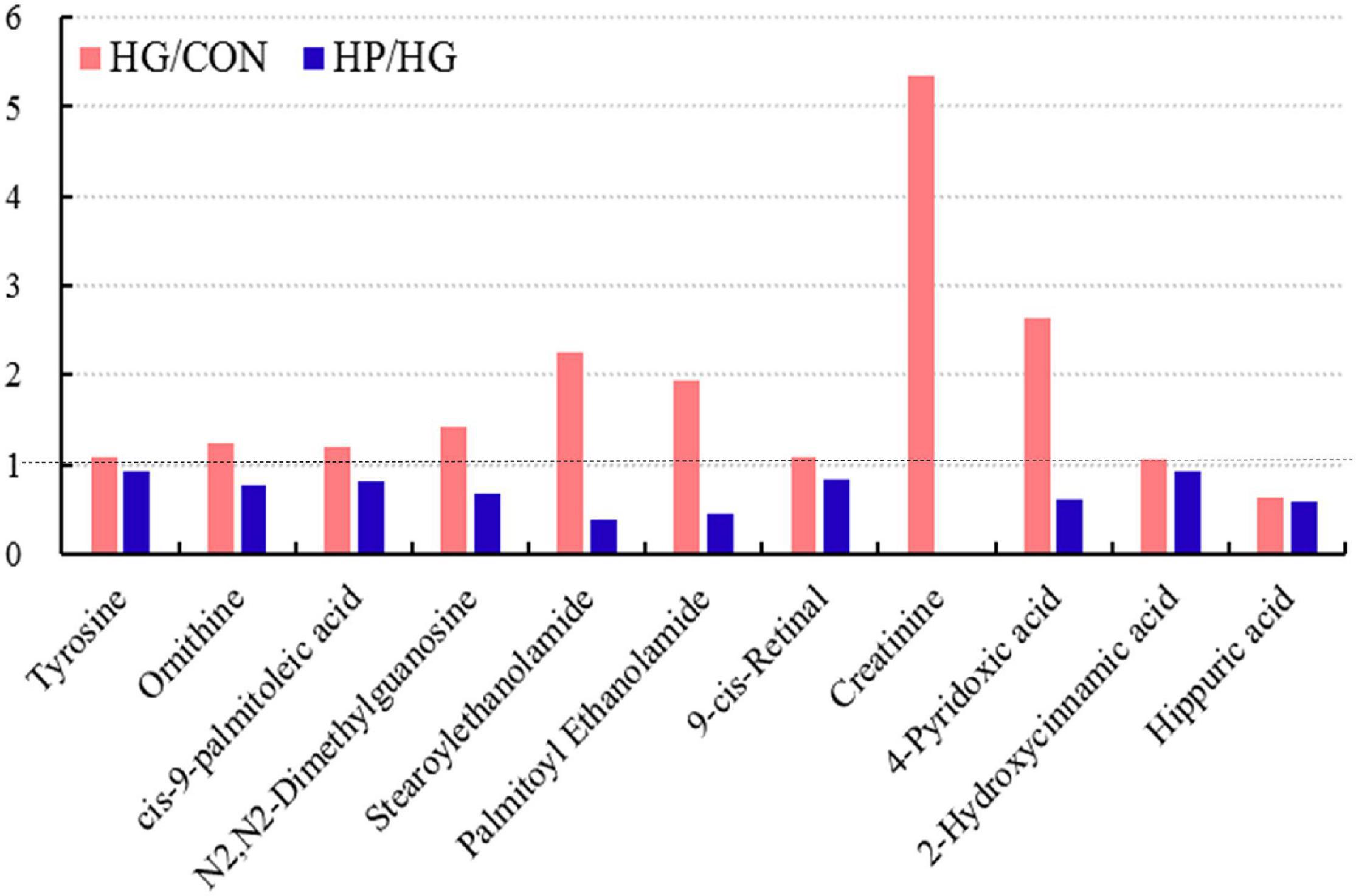
Figure 5. Common differential metabolites in the ileal mucosa of Hu sheep fed CON, HG, and HP diets.
Mucosal Host-Microbiota Metabolic Interactions in the Ileum
To explore the potential mucosal host-microbiota metabolic interactions in the ileum, a correlation network analysis was conducted using the disrupted microbial OTUs and the differential metabolites identified in the different dietary treatments (Figure 6). The results of this analysis demonstrated that there were complex connections between the intestinal mucosal microbiota and the metabolites. For example, the correlation network constructed using data from the CON and HG groups showed that OTU638 (G: Sphingomonas) was positively correlated with γ-aminobutyric acid, D-ribulose 5-phosphate, acetylcarnitine and docosatrienoic acid, and negatively correlated with N6,N6,N6-trimethyl-L-lysine, itaconic acid, lysine, histidine, agmatine, and 9-cis-retinal. The correlation network constructed using data from the HG and HP groups showed that OTU659 (S: R. solanacearum) was negatively correlated with 13 metabolites (such as oleoylethanolamide, tyrosine, creatine, lysine, and agmatine), while OTU275 (S: Nesterenkonia sp.) was positively correlated with eight metabolites (such as creatinine, 2-hydroxycinnamic acid, ornithine, and tyrosine).
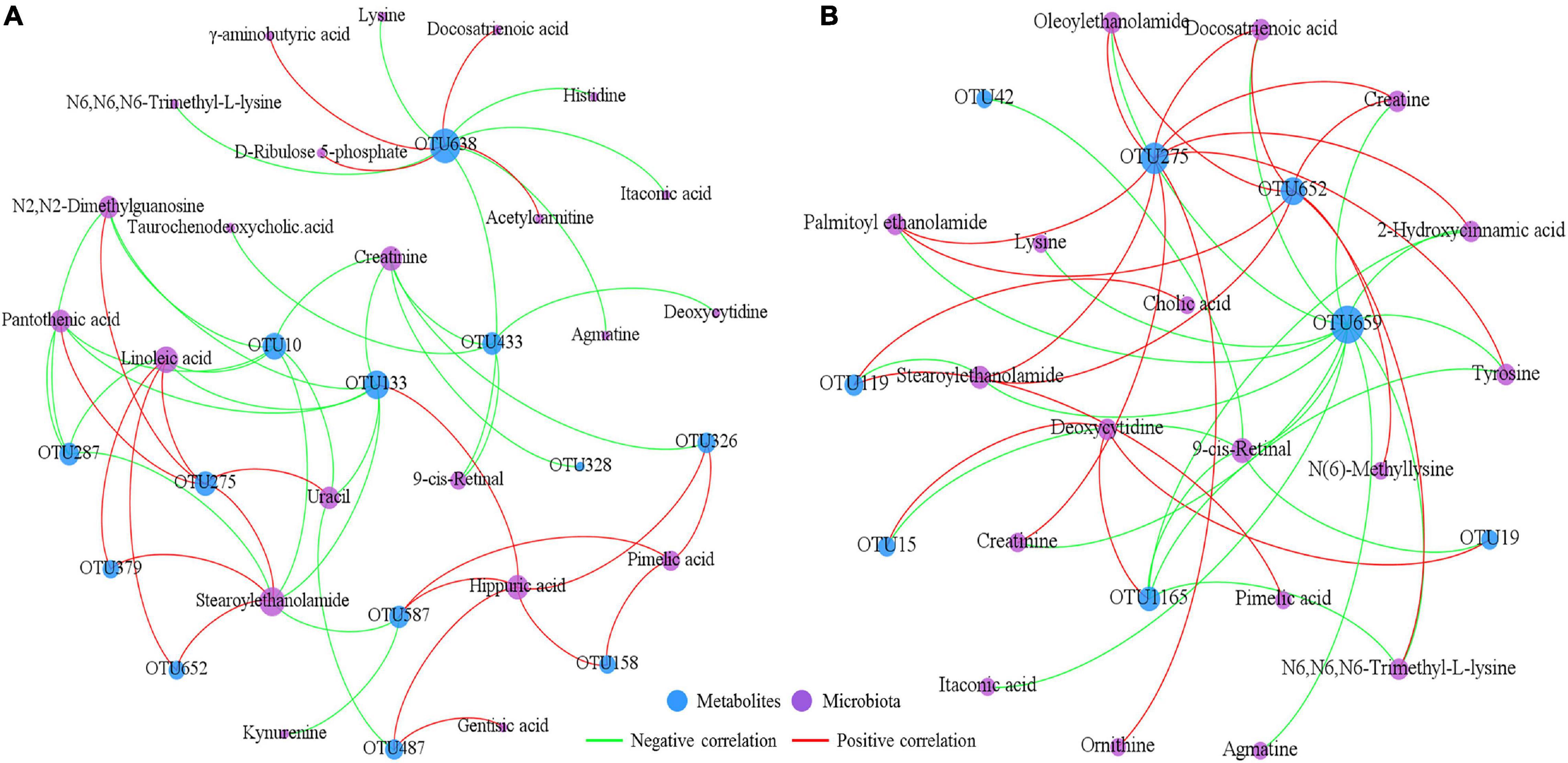
Figure 6. Correlation networks between the affected microbiota and metabolites in the ileal mucosa of Hu sheep between the CON and HG groups (A) and the HG and HP groups (B). Only correlation coefficients with P < 0.05 and the |r| > 0.6 were adopted to construct the correlation networks.
Discussion
Acting as the biological barrier for the intestine, mucosal microbiota play an important role in resisting invasion by pathogenic microbiota and by regulating host immunity (Ashida et al., 2012). Extensive studies have recently been conducted on mucosal microbiota and the remodeling effect of HG diet in the rumen and hindgut of ruminant animals (Chen et al., 2011; Ye et al., 2016; Wang et al., 2017; Zhang et al., 2017). However, studies on the mucosal microbiota of the ileum and the effects of HG or HP diets on them are limited. At present, 16S rRNA sequencing with an Illumina MiSeq Platform provides an efficient mean to study the intestinal microbiota (Rapin et al., 2017), and also has been widely used in ruminant researches (Li et al., 2018; Lin et al., 2020). Through sequencing, our results provide an overview of the microbial composition of the ileal mucosa of the Hu sheep. Consistent with other studies using 16S rRNA sequencing, our results found that the dominant bacterial phyla in ileal mucosal microbiota were Proteobacteria, Actinobacteriota, Bacteroidetes, and Firmicutes (Mao et al., 2015; Liu et al., 2019). As for genus level, our results indicated that Ralstonia, Rhodococcus, Sphingomonas, and Vibrionimonas dominated in ileal mucosal microbiota, while this result showed great differences from other studies (Mao et al., 2015; Liu et al., 2019). The reason for this discrepancy may be related to different dietary composition, animal age, and host species.
Microbial disturbance caused by the HG diets in the rumen has been confirmed by numerous studies (Zhang et al., 2017; Seddik et al., 2019), but the results of the studies on the effect of HG diet on the ileal microbiota in ruminants is inconsistent. Liu et al. (2019) reported that HG diets resulted no significant between-group differences in the composition of ileal mucosal microbiota in goats, while Jiao et al. (2018) revealed dissimilarities in the ileal mucosal microbiota between their HG and CON groups. In our present study, the results of PCoA and PERMANOVA analysis showed that there was a tendency for a microbial difference between the CON and HG groups, while no significant difference between the HG and HP groups was observed. Hence, the above results indicate that the impact of HG diet on the rumen is greater than that on the ileum, and more studies are needed to reveal the exact reason for the discrepancy.
To further evaluate the specific differences in the microbial communities between the different dietary treatments, we assessed microbial differences at the genus and OTU levels. Our results revealed that the relative abundance of the genus Sphingomonas, and several species (e.g., OTU587, OTU487, and OTU433) significantly declined in the ileal mucosal microbiota of the HG group compared with the CON group. Sphingomonas is a strictly aerobic commensal bacteria of the human and animal intestines, and existing evidence from studies suggests that Sphingomonas contributes to the degradation of several types of polysaccharides, such as cellulose (Birkholz and Kronenberg, 2015; Cui et al., 2020). Moreover, Sphingomonas spp. is used as a probiotic in intensive aquaculture, and is reported to have antagonistic effects on fish pathogens (Chaudhary and Qazi, 2014). Hence, the higher abundance of Sphingomonas in the CON group may be linked with its cellulose degradation activity and exerts a probiotic effects in the small intestine of the Hu sheep. Our results also found that the relative abundance of the genus C. Arthromitus and its species—OTU158 were significantly decreased in the ileal mucosal microbiota of the HG group compared with the CON group. C. Arthromitus is a Gram positive spore-forming segmented filamentous bacteria that colonizes the ileal mucosa of mammals (Caselli et al., 2010; Thompson et al., 2013). Previous studies showed that C. Arthromitus plays vital roles in the immune system maturation, defense against pathogenic infection, and maintenance of immune balance of the intestinal mucosa (Caselli et al., 2010; Schnupf et al., 2013). Hence, reduction of C. Arthromitus caused by the HG diets in the present study may be detrimental to intestinal mucosal health and leave the hosts more susceptible to infectious diseases.
In addition, results of the present study revealed that HG diets increased the relative abundance of some acid-producing microbiota, such as Succiniclasticum, Nesterenkonia, and Alloprevotella. Of these genera, Succiniclasticum spp. can convert succinate, produced by other microorganisms fermenting carbohydrates, to propionate (Van Gylswyk, 1995). Nesterenkonia strains have the ability to secrete amylase, and hence produce alcohols such as acetone, butanol, and ethanol, as well as acetate and butyrate under aerobic conditions (Ebrahimi et al., 2020). Members of Alloprevotella possess saccharolytic activity, and produce moderate amounts of acetate and major amounts of succinate as end products of fermentation (Downes et al., 2013). Thus, similar to the rumen, changes in theses acid-producing microbiota in the HG group is likely related to the increased levels of starch in their diet, and the altered microbial community may result an acidic microenvironment in the ileal mucosa. Interestingly, in contrast to the reduced abundance of Alloprevotella in the HG group, the HP group significantly decreased the relative abundance of this genus in the present study. As for other acid-producing microbiota, OTU42, belonging to B. choerinum, was found to be significantly enriched in the HP group, but not in the CON or HG groups. Bifidobacterium sp., an anaerobic probiotic species that colonizes animal intestines, plays an important role in inhibiting the proliferation of pathogenic bacteria and maintaining intestinal immune health (Wang et al., 2020). Members of Bifidobacterium can utilize a diverse range of dietary carbohydrates that evade rumen degradation and their presence in high numbers often has beneficial effects on the health of the intestine and the host (Pokusaeva et al., 2011; Bunesova et al., 2014).
To further understand the effects of HG and HP diets on the ileal mucosa, we conducted a LC-MS based metabolome analysis. Interestingly, contrary to the minor fluctuations of mucosal microbiota observed above, dietary treatments had large effects on mucosal metabolites. Our results showed that samples from the CON, HG, and HP groups were clearly separated on the PLS-DA and OPLS-DA scatter plots, indicating that the different dietary treatments resulted in different metabolic patterns in the ileal mucosa. For specifically affected metabolites, the HG diets increased the content of ornithine and tyrosine, and decreased the content of glutamine and γ-aminobutyric acid compared with the CON diet. The increased amino acids should be available to the nutrition of the host, but also increase potential risks for the intestines. In ruminants, ornithine and tyrosine are precursors of putrescine and tyramine, with these corresponding biogenic amines generated by decarboxylation of amino acids through microbial activity (Saleem et al., 2012). The higher content of ornithine and tyrosine may result in more accumulation of biogenic amines as is observed in the rumen (Xue et al., 2018), which might increase intestinal pathological processes such as cell apoptosis and inflammation (Linares et al., 2016; Zhang et al., 2017). However, due to the limitations of LC-MS technology, putrescine and tyramine were not detected in the present study, but other amines, including stearoylethanolamide palmitoyl ethanolamide, were observed to have a significant increase in the HG group. Simultaneously, contrary to the alterations in the HG group, the HP group significantly decreased the content of several amino acids (such as arginine, histidine, and ornithine) and amines (such as agmatine, stearoylethanolamide, and oleoylethanolamide) compared with the HG group. In addition, glutamine serves as important energy source for the intestinal mucosa, mediates multiple protective influences on intestinal homeostasis, such as promoting cell proliferation and differentiation, maintaining intestinal mucosal integrity, and protecting the physical barrier of the intestine (Rao and Samak, 2012). Moreover, as a nutritional regulatory factor, γ-aminobutyric acid can be converted into glutamine, and then exert its beneficial effects (Chen et al., 2015). Therefore, the reduced content of glutamine and γ-aminobutyric acid in HG groups may imply that the ileum is more fragile after feeding with the HG diet and can easily acquire damage to integrity and barrier function of the ileum. This speculation is supported by the study of Liu et al. (2019), who reported that HG diets caused downregulated mRNA expression of several tight junction genes (e.g., claudin-4, occludin, and ZO-1) in the ileal mucosa of the goat (Liu et al., 2019).
Besides amino acids and amines, results of our study on nucleotides/nucleosides also provided evidence that the HG diets were not conducive to intestinal health. In our results we found three nucleotides/nucleosides, adenosine, UMP, and ′-AMP, which had significantly decreased amounts in the ileal mucosa of the HG group compared with the CON group. Nucleotides and nucleosides have been reported to have multiple biological effects on intestinal health, such as enhancing cell proliferation and differentiation, improving intestinal morphology, and repairing tissue damage (Sato et al., 1999; Vieites et al., 2008; Rodríguez-Serrano et al., 2010). In addition, luteolin, a natural antioxidant, was observed to be significantly decreased in the HG group in our study. As a member of the flavonoid family, luteolin widely exists in many plants and has various beneficial anti-inflammatory and anti-oxidative functions (Li et al., 2021a). Previous studies have suggested that HG diets increase the potential risk of intestinal inflammation and damage to the mucosal barrier (Jiao et al., 2018; Liu et al., 2019), thus, decreased nucleotide/nucleoside and luteolin content might imply that HG diets weakened the ability of self-renewal, inhibiting inflammation, and self-repair.
Bile acids, synthesized in hepatocytes via oxidation of cholesterol and re-absorbed in the ileum after being modified by the intestinal microbiota, are critically important for lipid metabolism, regulating intestinal microbial balance, and promoting immune homeostasis (Jia et al., 2018). Interestingly, significant differences in the two primary bile acids (cholic acid and taurochenodeoxycholic acid) were observed in ileal mucosa between the HG and HP groups in the present study. A previous study demonstrated that HG diets altered the bacterial communities in the jejunum and significantly decreased the content of cholic acid in bile from cattle (Liu et al., 2020). Another study revealed that high rumen-degradable starch diets significantly increased the hepatic concentration of taurochenodeoxycholic acid in the liver compared with the control group (Shen et al., 2020). In the present study, the same trend (increased taurochenodeoxycholic acid and decreased cholic acid) was found in the ileal mucosa of the HG group, but it did not reach a statistically significant level. However, the opposite trend was observed in the ileal mucosa of the HP group. It is worth noting that, Bifidobacterium spp. has the ability to secrete bile salt hydrolases to deconjugate the amide bond between bile acids with glycine or taurine (Urdaneta and Casadesús, 2017), hence, the increased relative abundance of Bifidobacterium spp. is a reasonable explanation for the decreased levels of taurochenodeoxycholic acid in the HP group in the present study. Corresponding to the changes in the bile acids in the HP group, a significant decrease in two fatty acids (cis-9-palmitoleic acid and docosatrienoic acid) and a significant increase in acetylcarnitine were observed in the HP group in the present study. Acetylcarnitine serves as effective carriers to transport long-chain fatty acids into mitochondria for β-oxidation to meet the energy requirements of cells (Li et al., 2019). Therefore, decreased content of fatty acids in the HP group may be related to the effects of bile acids and acetylcarnitine in promoting lipid metabolism. The precise mechanisms for bile acids and lipid metabolism after feeding with HG and HP diets need additional systematic study.
Conclusion
In summary, the present study integrated microbiome and metabolome analyses to demonstrate that HG diets cause substrate-dependent alterations in the mucosal microbiota and metabolites. Ileal mucosal microbiota, such as the probiotics (Sphingomonas and C. Arthromitus) decreased and the acid-producing microbiota (Succiniclasticum, Nesterenkonia, and Alloprevotella) increased, and further alterations in the metabolism of amino acids, fatty acids and nucleotides/nucleosides were observed in the ileal mucosa of Hu sheep fed a HG diet. This mucosal host-microbiota metabolic interaction causes the ileal mucosa of the HG group to be more likely exposed to an unfriendly environment with increased risk of potential inflammation. However, HP diet seemed to partially reverse these changes caused by the HG diet. Our results help to better understand the effects of high-grain diets on health of ruminant species and their application in animal production.
Data Availability Statement
The datasets presented in this study can be found in online repositories. The names of the repository/repositories and accession number(s) can be found in the article/Supplementary Material.
Ethics Statement
The animal study was reviewed and approved by the Experimental Animal Welfare Ethics Committee of Shenyang Agricultural University.
Author Contributions
SZ, SM, and RZ designed the study. LL, FX, HM, and ZZ conducted the experiment and collected the samples. HM and ZZ conducted laboratory analyses. RZ analyzed the data and wrote the manuscript. ZW, SM, DI, and SZ revised the manuscript. All authors contributed to the article and approved the submitted version.
Funding
This work was funded by the National Natural Science Foundation of China (No. 32002214), the China Postdoctoral Science Foundation (2020M680985), the grant from the Educational Department of Liaoning Province (Climbing Scholar), and the Scientific Foundation for the Introduced Talent of Shenyang Agricultural University (No. 880416010).
Conflict of Interest
The authors declare that the research was conducted in the absence of any commercial or financial relationships that could be construed as a potential conflict of interest.
Publisher’s Note
All claims expressed in this article are solely those of the authors and do not necessarily represent those of their affiliated organizations, or those of the publisher, the editors and the reviewers. Any product that may be evaluated in this article, or claim that may be made by its manufacturer, is not guaranteed or endorsed by the publisher.
Supplementary Material
The Supplementary Material for this article can be found online at: https://www.frontiersin.org/articles/10.3389/fmicb.2021.718884/full#supplementary-material
Footnotes
References
Ahmed, I., Roy, B. C., Khan, S. A., Septer, S., and Umar, S. (2016). Microbiome, metabolome and inflammatory bowel disease. Microorganisms 4:20. doi: 10.3390/microorganisms4020020
Ashida, H., Ogawa, M., Kim, M., Mimuro, H., and Sasakawa, C. (2012). Bacteria and host interactions in the gut epithelial barrier. Nat. Chem. Biol. 8, 36–45. doi: 10.1038/nchembio.741
Birkholz, A. M., and Kronenberg, M. (2015). Antigen specificity of invariant natural killer T-cells. Biomed J. 38, 470–483. doi: 10.1016/j.bj.2016.01.003
Blanco, C., Giráldez, F. J., Prieto, N., Benavides, J., Wattegedera, S., Morán, L., et al. (2015). Total mixed ration pellets for light fattening lambs: effects on animal health. Animal 9, 258–266. doi: 10.1017/s1751731114002249
Bunesova, V., Vlkova, E., Rada, V., Killer, J., and Musilova, S. (2014). Bifidobacteria from the gastrointestinal tract of animals: differences and similarities. Benef. Microbes. 5, 377–388. doi: 10.3920/bm2013.0081
Caporaso, J. G., Kuczynski, J., Stombaugh, J., Bittinger, K., Bushman, F. D., Costello, E. K., et al. (2010). QIIME allows analysis of high-throughput community sequencing data. Nat. Methods 7, 335–336.
Caselli, M., Holton, J., Boldrini, P., Vaira, D., and Calò, G. (2010). Morphology of segmented filamentous bacteria and their patterns of contact with the follicle-associated epithelium of the mouse terminal ileum: implications for the relationship with the immune system. Gut. Microbes. 1, 367–372. doi: 10.4161/gmic.1.6.14390
Chaudhary, A., and Qazi, J. I. (2014). Probiotic antagonism of Sphingomonas sp. against Vibrio anguillarum exposed Labeo rohita fingerlings. Adv. Life Sci. 4, 156–165.
Chen, Y., Penner, G. B., Li, M., and Oba, M. (2011). Changes in bacterial diversity associated with epithelial tissue in the beef cow rumen during the transition to a high-grain diet. Appl. Environ. Microbiol. 77, 5770–5781. doi: 10.1128/aem.00375-11
Chen, Z., Xie, J., Hu, M., Tang, J., Shao, Z., and Li, M. (2015). Protective effects of γ-aminobutyric acid (GABA) on the small intestinal mucosa in heat-stressed Wenchang chicken. J. Anim. Plant Sci. 25, 78–87.
Cui, Z., Wu, S., Liu, S., Sun, L., Feng, Y., Cao, Y., et al. (2020). From maternal grazing to barn feeding during pre-weaning period: altered gastrointestinal microbiota contributes to change the development and function of the rumen and intestine of yak calves. Front. Microbiol. 11:485. doi: 10.3389/fmicb.2020.00485
DeSantis, T. Z., Hugenholtz, P., Larsen, N., Rojas, M., Brodie, E. L., Keller, K., et al. (2006). Greengenes, a chimera-checked 16S rRNA gene database and workbench compatible with ARB. Appl. Environ. Microbiol. 72, 5069–5072. doi: 10.1128/aem.03006-05
Downes, J., Dewhirst, F. E., Tanner, A. C., and Wade, W. G. (2013). Description of Alloprevotella rava gen. nov., sp. nov., isolated from the human oral cavity, and reclassification of Prevotella tannerae Moore et al. 1994 as Alloprevotella tannerae gen. nov., comb. nov. Int. J. Syst. Evol. Microbiol. 63:1214. doi: 10.1099/ijs.0.041376-0
Ebrahimi, E., Amiri, H., and Asadollahi, M. A. (2020). Enhanced aerobic conversion of starch to butanol by a symbiotic system of Clostridium acetobutylicum and Nesterenkonia. Biochem. Eng. J. 164:107752. doi: 10.1016/j.bej.2020.107752
Edgar, R. C. (2013). UPARSE: highly accurate OTU sequences from microbial amplicon reads. Nat. Methods 10, 996–998. doi: 10.1038/nmeth.2604
Edgar, R. C., Haas, B. J., Clemente, J. C., Quince, C., and Knight, R. (2011). UCHIME improves sensitivity and speed of chimera detection. Bioinformatics 27, 2194–2200. doi: 10.1093/bioinformatics/btr381
Hammes, W. P., and Hertel, C. (2006). The genera lactobacillus and carnobacterium. Prokaryotes 4, 320–403.
He, F., Ouwehand, A. C., Isolauri, E., Hashimoto, H., Benno, Y., and Salminen, S. (2001). Comparison of mucosal adhesion and species identification of bifidobacteria isolated from healthy and allergic infants. FEMS Immunol. Med. Microbiol. 30, 43–47. doi: 10.1111/j.1574-695x.2001.tb01548.x
Islam, R., Redoy, M., Shuvo, A., Sarker, M., Akbar, M., and Al-Mamun, M. (2017). Effect of pellet from total mixed ration on growth performance, blood metabolomics, carcass and meat characteristics of Bangladeshi garole sheep. Prog. Agric. 28, 222–229. doi: 10.3329/pa.v28i3.34659
Jia, W., Xie, G., and Jia, W. (2018). Bile acid–microbiota crosstalk in gastrointestinal inflammation and carcinogenesis. Nat Rev Gastro Hepat. 15:111. doi: 10.1038/nrgastro.2017.119
Jiao, J., Zhang, X., Wang, M., Zhou, C., Yan, Q., and Tan, Z. (2018). Linkages between epithelial microbiota and host transcriptome in the ileum during high-grain challenges: Implications for gut homeostasis in goats. J. Agric. Food Chem. 67, 551–561. doi: 10.1021/acs.jafc.8b05591
Li, B., Du, P., Du, Y., Zhao, D., Cai, Y., Yang, Q., et al. (2021a). Luteolin alleviates inflammation and modulates gut microbiota in ulcerative colitis rats. Life Sci. 269:119008. doi: 10.1016/j.lfs.2020.119008
Li, B., Sun, X., Huo, Q., Zhang, G., Wu, T., You, P., et al. (2021b). Pelleting of a total mixed ration affects growth performance of fattening lambs. Front Vet Sci. 8:629016. doi: 10.3389/fvets.2021.629016
Li, C., Wang, W., Liu, T., Zhang, Q., Wang, G., Li, F., et al. (2018). Effect of early weaning on the intestinal microbiota and expression of genes related to barrier function in lambs. Front. Microbiol. 9:1431. doi: 10.3389/fmicb.2018.01431
Li, S., Gao, D., and Jiang, Y. (2019). Function, detection and alteration of acylcarnitine metabolism in hepatocellular carcinoma. Metabolites 9:36. doi: 10.3390/metabo9020036
Lin, L., Wang, Y., Xu, L., Liu, J., Zhu, W., and Mao, S. (2020). Microbiome–host co-oscillation patterns in remodeling of colonic homeostasis during adaptation to a high-grain diet in a sheep model. Anim. Microbiome 2, 1–12.
Linares, D. M., del Rio, B., Redruello, B., Ladero, V., Martin, M. C., Fernandez, M., et al. (2016). Comparative analysis of the in vitro cytotoxicity of the dietary biogenic amines tyramine and histamine. Food Chem. 197, 658–663. doi: 10.1016/j.foodchem.2015.11.013
Liu, J., Liu, F., Cai, W., Jia, C., Bai, Y., He, Y., et al. (2020). Diet-induced changes in bacterial communities in the jejunum and their associations with bile acids in Angus beef cattle. Anim. Microbiome 2, 1–14.
Liu, J., Xu, T., Zhu, W., and Mao, S. (2014). High-grain feeding alters caecal bacterial microbiota composition and fermentation and results in caecal mucosal injury in goats. Br. J. Nutr. 112, 416–427. doi: 10.1017/s0007114514000993
Liu, J., Xue, C., Sun, D., Zhu, W., and Mao, S. (2019). Impact of high-grain diet feeding on mucosa-associated bacterial community and gene expression of tight junction proteins in the small intestine of goats. Microbiologyopen. 8:e00745.
Magoc, T., and Salzberg, S. L. (2011). FLASH: fast length adjustment of short reads to improve genome assemblies. Bioinformatics 27, 2957–2963. doi: 10.1093/bioinformatics/btr507
Mao, S., Zhang, M., Liu, J., and Zhu, W. (2015). Characterising the bacterial microbiota across the gastrointestinal tracts of dairy cattle: membership and potential function. Sci. Rep. 5:16116.
McHardy, I. H., Goudarzi, M., Tong, M., Ruegger, P. M., Schwager, E., Weger, J. R., et al. (2013). Integrative analysis of the microbiome and metabolome of the human intestinal mucosal surface reveals exquisite inter-relationships. Microbiome 1, 1–19.
Plaizier, J. C., Khafipour, E., Li, S., Gozho, G. N., and Krause, D. O. (2012). Subacute ruminal acidosis (SARA), endotoxins and health consequences. Anim. Feed Sci. Technol. 172, 9–21. doi: 10.1016/j.anifeedsci.2011.12.004
Plaizier, J. C., Li, S., Danscher, A. M., Derakshani, H., Andersen, P. H., and Khafipour, E. (2017). Changes in microbiota in rumen digesta and feces due to a grain-based subacute ruminal acidosis (SARA) challenge. Microb. Ecol. 74, 485–495. doi: 10.1007/s00248-017-0940-z
Pokusaeva, K., Fitzgerald, G. F., and van Sinderen, D. (2011). Carbohydrate metabolism in Bifidobacteria. Genes Nutr. 6, 285–306. doi: 10.1007/s12263-010-0206-6
Rao, R., and Samak, G. (2012). Role of glutamine in protection of intestinal epithelial tight junctions. J. Epithel. Biol. Pharmacol. 5:47. doi: 10.2174/1875044301205010047
Rapin, A., Pattaroni, C., Marsland, B. J., and Harris, N. L. (2017). Microbiota analysis using an Illumina MiSeq platform to sequence 16S rRNA genes. Curr. Protoc. Mouse Biol. 7, 100–129. doi: 10.1002/cpmo.29
Rodríguez-Serrano, F., Ríos, A., Marchal, J. A., Caba, O., Martínez-Amat, A., Prados, J., et al. (2010). Differentiation of intestinal epithelial cells mediated by cell confluence and/or exogenous nucleoside supplementation. Cells Tissues Organs. 191, 478–488. doi: 10.1159/000276593
Saleem, F., Ametaj, B., Bouatra, S., Mandal, R., Zebeli, Q., Dunn, S., et al. (2012). A metabolomics approach to uncover the effects of grain diets on rumen health in dairy cows. J. Dairy Sci. 95, 6606–6623. doi: 10.3168/jds.2012-5403
Sato, N., Nakano, T., Kawakami, H., and Idota, T. (1999). In vitro and in vivo effects of exogenous nucleotides on the proliferation and maturation of intestinal epithelial cells. J. Nutr. Sci. Vitaminol. 45, 107–118. doi: 10.3177/jnsv.45.107
Schnupf, P., Gaboriau-Routhiau, V., and Cerf-Bensussan, N. (2013). Host interactions with segmented filamentous bacteria: an unusual trade-off that drives the post-natal maturation of the gut immune system. Semin. Immunol. 25, 342–51. doi: 10.1016/j.smim.2013.09.001
Seddik, H., Xu, L., Wang, Y., and Mao, S. (2019). A rapid shift to high-grain diet results in dynamic changes in rumen epimural microbiome in sheep. Animal 13, 1614–1622. doi: 10.1017/s1751731118003269
Shen, J., Han, X., Zheng, L., Liu, S., Jin, C., Liu, T., et al. (2020). High rumen-degradable starch diet promotes hepatic lipolysis and disrupts enterohepatic circulation of bile acids in dairy goats. J Nutr. 150, 2755–2763. doi: 10.1093/jn/nxaa238
Thompson, C., Mikaelyan, A., and Brune, A. (2013). Immune-modulating gut symbionts are not “Candidatus Arthromitus”. Mucosal Immunol. 6, 200–201. doi: 10.1038/mi.2012.91
Trabi, E. B., Seddik, H., Xie, F., Lin, L., and Mao, S. (2019). Comparison of the rumen bacterial community, rumen fermentation and growth performance of fattening lambs fed low-grain, pelleted or non-pelleted high grain total mixed ration. Anim. Feed Sci. Technol. 253, 1–12. doi: 10.1016/j.anifeedsci.2019.05.001
Trabi, E. B., Seddik, H., Xie, F., Wang, X., Liu, J., and Mao, S. (2020). Effect of pelleted high-grain total mixed ration on rumen morphology, epithelium-associated microbiota and gene expression of proinflammatory cytokines and tight junction proteins in Hu sheep. Anim. Feed Sci. Technol. 263:114453. doi: 10.1016/j.anifeedsci.2020.114453
Urdaneta, V., and Casadesús, J. (2017). Interactions between bacteria and bile salts in the gastrointestinal and hepatobiliary tracts. Front. Med. 4:163. doi: 10.3389/fmed.2017.00163
Van Gylswyk, N. (1995). Succiniclasticum ruminis gen. nov., sp. nov., a ruminal bacterium converting succinate to propionate as the sole energy-yielding mechanism. Int. J. Syst. Evol. Microbiol. 45, 297–300. doi: 10.1099/00207713-45-2-297
Vieites, J. M., de la Torre, R., del, C. R. M., Torres, M. I, Sánchez-Pozo, A., Gil, A., et al. (2008). Exogenous nucleosides accelerate differentiation of rat intestinal epithelial cells. Br. J. Nutr. 99, 732–738. doi: 10.1017/s0007114507837457
Wang, J., Yu, X.-J., Bai, Y.-Y., Wang, P.-Z., and Liu, C.-Y. (2020). Effects of grazing and confinement on the morphology and microflora of the gastrointestinal tract of Small-tailed Han sheep. Livest Sci. 241:104208. doi: 10.1016/j.livsci.2020.104208
Wang, M., Li, Y., Gao, M., Song, L., Xu, M., Zhao, X., et al. (2021). Effects of subacute ruminal acidosis on colon epithelial morphological structure, permeability, and expression of key tight junction proteins in dairy goats. J. Dairy Sci. 104, 4260–4270. doi: 10.3168/jds.2020-18738
Wang, Q., Garrity, G. M., Tiedje, J. M., and Cole, J. R. (2007). Naive Bayesian classifier for rapid assignment of rRNA sequences into the new bacterial taxonomy. Appl. Environ. Microbiol. 73, 5261–5267. doi: 10.1128/aem.00062-07
Wang, W., Hu, H., Zijlstra, R. T., Zheng, J., and Gänzle, M. G. (2019). Metagenomic reconstructions of gut microbial metabolism in weanling pigs. Microbiome 7, 1–11. doi: 10.1007/978-1-4614-6418-1_752-1
Wang, Y., Xu, L., Liu, J., Zhu, W., and Mao, S. (2017). A high grain diet dynamically shifted the composition of mucosa-associated microbiota and induced mucosal injuries in the colon of sheep. Front. Microbiol. 8:2080. doi: 10.3389/fmicb.2017.02080
Wells, J. M., Rossi, O., Meijerink, M., and van Baarlen, P. (2011). Epithelial crosstalk at the microbiota–mucosal interface. Proc. Natl. Acad. Sci. U.S.A. 108, 4607–4614. doi: 10.1073/pnas.1000092107
Xie, G., Zhang, S., Zheng, X., and Jia, W. (2013). Metabolomics approaches for characterizing metabolic interactions between host and its commensal microbes. Electrophoresis 34, 2787–2798.
Xue, F., Pan, X., Jiang, L., Guo, Y., and Xiong, B. (2018). GC-MS analysis of the ruminal metabolome response to thiamine supplementation during high grain feeding in dairy cows. Metabolomics 14, 1–12.
Yao, J., Han, X., Lei, X., Yang, X., Shen, J., Zheng, L., et al. (2021). A metagenomic insight into the hindgut microbiota and their metabolites for dairy goats fed different rumen degradable starch. Front. Microbiol. 12:1179. doi: 10.3389/fmicb.2021.651631
Ye, H., Liu, J., Feng, P., Zhu, W., and Mao, S. (2016). Grain-rich diets altered the colonic fermentation and mucosa-associated bacterial communities and induced mucosal injuries in goats. Sci. Rep. 6, 1–13.
Yuan, C., Graham, M., Staley, C., and Subramanian, S. (2020). Mucosal microbiota and metabolome along the intestinal tract reveal a location-specific relationship. Msystems 5:3.
Zhang, R., Ye, H., Liu, J., and Mao, S. (2017). High-grain diets altered rumen fermentation and epithelial bacterial community and resulted in rumen epithelial injuries of goats. Appl. Microbiol. Biotechnol. 101, 6981–6992. doi: 10.1007/s00253-017-8427-x
Keywords: Hu sheep, high-grain diet, microbiota, metabolome, ileal mucosa, probiotics
Citation: Zhang R, Zhong Z, Ma H, Lin L, Xie F, Mao S, Irwin DM, Wang Z and Zhang S (2021) Mucosal Microbiota and Metabolome in the Ileum of Hu Sheep Offered a Low-Grain, Pelleted or Non-pelleted High-Grain Diet. Front. Microbiol. 12:718884. doi: 10.3389/fmicb.2021.718884
Received: 01 June 2021; Accepted: 29 July 2021;
Published: 26 August 2021.
Edited by:
Sharon Ann Huws, Aberystwyth University, United KingdomReviewed by:
Ravindra Pal Singh, National Agri-Food Biotechnology Institute, IndiaBabak Haghshenas, Kermanshah University of Medical Sciences, Iran
Copyright © 2021 Zhang, Zhong, Ma, Lin, Xie, Mao, Irwin, Wang and Zhang. This is an open-access article distributed under the terms of the Creative Commons Attribution License (CC BY). The use, distribution or reproduction in other forums is permitted, provided the original author(s) and the copyright owner(s) are credited and that the original publication in this journal is cited, in accordance with accepted academic practice. No use, distribution or reproduction is permitted which does not comply with these terms.
*Correspondence: Shuyi Zhang, c3poYW5nQHN5YXUuZWR1LmNu