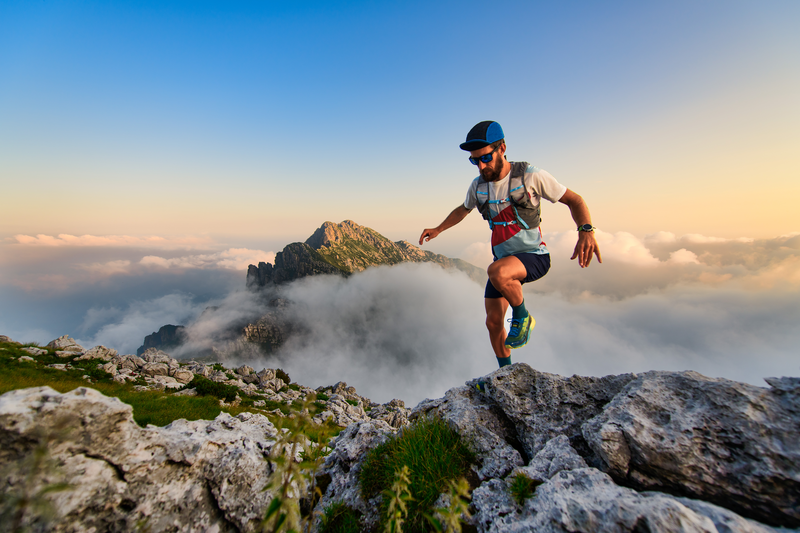
94% of researchers rate our articles as excellent or good
Learn more about the work of our research integrity team to safeguard the quality of each article we publish.
Find out more
REVIEW article
Front. Microbiol. , 27 July 2021
Sec. Antimicrobials, Resistance and Chemotherapy
Volume 12 - 2021 | https://doi.org/10.3389/fmicb.2021.712588
This article is part of the Research Topic Stem cell-derived exosome therapy of microbial diseases: from bench to bed View all 11 articles
Exosomes are nano-vesicles of endosomal origin inherited with characteristics of drug delivery and cargo loading. Exosomes offer a diverse range of opportunities that can be exploited in the treatment of various diseases post-functionalization. This membrane engineering is recently being used in the management of bacteria-associated diabetic foot ulcers (DFUs). Diabetes mellitus (DM) is among the most crippling disease of society with a large share of its imposing economic burden. DM in a chronic state is associated with the development of micro- and macrovascular complications. DFU is among the diabetic microvascular complications with the consequent occurrence of diabetic peripheral neuropathy. Mesenchymal stromal cell (MSC)-derived exosomes post-tailoring hold promise to accelerate the diabetic wound repair in DFU associated with bacterial inhabitant. These exosomes promote the antibacterial properties with regenerative activity by loading bioactive molecules like growth factors, nucleic acids, and proteins, and non-bioactive substances like antibiotics. Functionalization of MSC-derived exosomes is mediated by various physical, chemical, and biological processes that effectively load the desired cargo into the exosomes for targeted delivery at specific bacterial DFUs and wound. The present study focused on the application of the cargo-loaded exosomes in the treatment of DFU and also emphasizes the different approaches for loading the desired cargo/drug inside exosomes. However, more studies and clinical trials are needed in the domain to explore this membrane engineering.
Extracellular vesicles (EVs), including exosomes, apoptotic bodies, and microvesicles, are secreted by various cell types. EVs showed diverse characteristics in size, function, indigenous cargo, and secretion pathway (Raghav et al., 2021). Exosomes are small-sized EVs formed by the process of inward budding in early endosomes and later form multivesicular bodies (MVBs) of average 100-nm dimensions (Raghav et al., 2021). These later released into the extracellular matrix/environment to deliver their indigenous cargo/components fulfilling their fate (Raghav et al., 2021). Cellular exosomes release involves various steps, i.e., formation of early endosomes, followed by fusion of the MVBs containing intraluminal vesicles (ILVs), with the plasma membrane by exocytosis and release of exosomes in the extracellular space (Than et al., 2017). Exosomes are present in all bodily fluids secreted by cells, including blood (Lewis et al., 2018), urine (Cavallaro et al., 2019), plasma (Yan et al., 2019), breast milk (Adriano et al., 2021), saliva (Kurian et al., 2021), bile, synovial fluid, semen, amniotic fluid, ascites fluid (peritoneal cavity), and bronchoalveolar and gastrointestinal lavage fluid (Kumar et al., 2019). The exosomal indigenous cargo is mostly rich in proteins, lipids, sugars, and nucleic acids [messenger RNAs (mRNAs), microRNAs (miRNAs), and mitochondrial DNA (mtDNA), etc.] (Jan et al., 2021; Figure 1). Exosomes’ functions encompass an elaborative list depending on the origin of cell/tissue. Such functions include immune-modulatory, regeneration, antigen presentation programmed cell death (APPCD), inflammation, angiogenesis, and coagulation. The cargo imparts functionality to the exosomes for different cellular communications like paracrine, autocrine, endocrine, and/or juxtacrine signaling, while surface proteins provide identity to the exosomes for cargo delivery (Wei et al., 2021).
Figure 1. Schematic structure and contents of exosome. ATPase, adenosine triphosphatase; CD, cluster of differentiation; GAPDH, glyceraldehyde 3-phosphate dehydrogenase; HSP, heat shock protein; ICAM-1, intercellular adhesion molecule-1; LAM 1/2, lysosomal-associated membrane protein 1/2; MHC, major histocompatibility complex; miRNA, microRNA; mRNA, messenger RNA; MVB, multivesicular body; PGRL, PG regulatory-like protein; pgk1, phosphoglycerate kinase 1. [Adopted from Jan et al. (2021) distributed under the Creative Commons Attribution Licens].
Authors of past studies exploited the exosomes as delivery vehicles for drugs and other desired cargo of interest (Bertrand and Leroux, 2012; Lai and Breakefield, 2012; El Andaloussi et al., 2013). These inbuilt characteristics of exosomes allow for tailoring “cargo of interest” for therapeutics and imaging purpose with an additional feature of prolonged circulation time, specific target cell recognition due to the presence of cell surface markers, negligible toxicity, and immune tolerance. Exosomes can be manipulated with more than one type of deliverables like drugs, proteins, and coding/non-coding nucleic acids, simultaneously. However, further studies are required to evaluate whether there exists any sort of allogeneic immune rejection among exosomes from different donors and recipients (Zhuang et al., 2011; Lee et al., 2012).
In one of the recently published studies, the protective effect of adipocyte stem cell (ADSC)-derived exosomes was investigated in a diabetic animal in vitro model and found that exosomes promoted angiogenesis and proliferation of cells in the hyperglycemic environment (Li et al., 2018). The study showed a significant reduction in diabetic ulceration/wound area in the animal group receiving the exosomes from ADSCs overexpressing the Nrf2 factor (Li et al., 2018). The study laid the foundation that the exosomes can be exploited for the healing of diabetic foot ulcers (DFUs). An et al. (2021) showed the therapeutic role of mesenchymal stem cell (MSC)-derived exosomes in the treatment of diabetes-induced ulcers and lower limb ischemia.
Diabetic foot ulcers are a severe complication associated with diabetes mellitus (DM) that impose economic burden ranges from US$9 to US$13 billion in the United States, along with additional cost for the management of DM (Raghav et al., 2018). DFUs are the cause of various complications including peripheral neuropathy, deformity in the foot, and peripheral arterial diseases’ poor extremity perfusion (Noor et al., 2018). DFUs are characterized by the presence of bacterial pathogens that are responsible for wound microbiology and the development of the infection. Several microorganisms (fungi, aerobic, and anaerobic species) are responsible for the etiology of the DFUs, including Staphylococcus, Streptococcus, Proteobacteria, and Pseudomonas aeruginosa (Noor et al., 2015). In this review, first, we comprehensively focused on exosome biogenesis and factors affecting the biogenesis. In addition, we discussed the methods of isolation of exosomes and fabrication of the customized exosomes using various modification methods. This study discusses the idea that MSC-derived exosomes post-tailoring hold promise to accelerate the diabetic wound repair in DFU associated with bacterial inhabitant, along with the application of the cargo-loaded exosomes in the treatment of DFU, and this study also emphasizes the different approaches for loading the desired cargo/drug inside exosomes.
Biogenesis of exosomes is a constitutive mechanism that is initiated with plasma membrane inward invagination within cytosol generating early and late endosomes. These late endosomes further give rise to MVBs followed by ILV formation. It seems that during the ILV formation by inward budding, several essential proteins, growth factors, cytoskeleton components, nucleic acids, lipids, and other necessary cellular components get wrapped into it (Raghav et al., 2021). The key feature of biogenesis pathways includes internalization, fusion, and release (Figure 2). ILVs formed from MVBs fuse with the plasma membrane of the cells and released as exosomes into the extracellular environment by the mechanism of exocytosis.
Figure 2. Exosome biogenesis. Beginning from internalization of membrane proteins and lipid complexes by endocytosis, endocytotic vesicles are delivered to early endosomes, which fuse with each other resulting in formation of late endosomes/multivesicular bodies (MVB). [Adopted from Than et al. (2017) distributed under the Creative Commons Attribution License].
In one of the recently published studies, it was quoted that the budding of the exosomes and their sorting are either endosomal sorting complex required for transport (ESCRT)-dependent or -independent (Raghav et al., 2021). The ESCRT-mediated exosomes sorting process involves screening, identification, and sequestration of ubiquitinated proteins specific for endosomal proteins. This ESCRT-mediated mechanism showed an association between subunits I, II, and III of ESCRT that terminate the exosome budding process (Raghav et al., 2021). Moreover, the ESCRT-independent mechanism of exosome budding involves proteins and lipids such as tetraspanins and ceramides (Raghav et al., 2021). The exosomes play a crucial role in intercellular communication via the transfer of the biomolecules loaded within them. Their biogenesis mechanism is governed by various factors including ESCRT proteins, STAM1, VPS4, CHMP4, the Syndecan–syntenin–ALIX complex, nSMase2, CD9, and PLD2 (Gurunathan et al., 2021). Similar to the sorting mechanism, the exosome uptake process is mediated by either the clathrin-dependent or clathrin-independent events that involve micro-pinocytosis, phagocytosis, and lipid raft-mediated internalization. The exosomes are composed of several biomolecules including heat shock proteins, cell adhesion proteins, cell signaling proteins, tetraspanin membrane proteins, phosphatidylserine (PS), phosphatidic acid, sphingomyelin (SM), cholesterol, arachidonic acids, prostaglandins, and leukotrienes (Raghav et al., 2021). Besides these proteins and lipid components, exosomes are also rich in micro-RNAs, small nuclear RNAs, non-coding RNAs, long non-coding RNAs, piwi-interacting RNAs, rRNAs, and tRNAs (Raghav et al., 2021). Exosomes are considered to be the cocktail of these biomolecules that have therapeutic, diagnostic, and transmittance characteristics.
Exosomes can be derived from various cell types and all have diverse clinical characteristics, depending on the source of cells from which they are derived. The various sources of exosomes include the following:
Adipose tissue mesenchymal stem cells (ADMSCs) are abundantly distributed in the human body, compared to other exosome cell sources including umbilical cord mesenchymal stem cells (UCMSCs) and bone marrow mesenchymal stem cells (BMSCs). ADMSCs showed the highest degree of purification with high yield due to their abundance in nature (Tang et al., 2021). The extraction of ADMSCs is an easier and painless procedure, causing only a small episode of trauma (Tang et al., 2021). These cell-derived exosomes can be easily procured in clinics in the presence of plastic surgeons or medical aestheticians. ADMSC-derived exosomes require strict storage conditions, thereby possessing some restrictions in their clinical applications. Moreover, the lipid membrane structure is quite stable and has the properties to retain its contents for a long period and is therefore always a good choice for researchers (Tang et al., 2021).
The strict morphology of the adipose tissue is not fixed, and the primary source cells can be of any shape, either fusiform or circular. It was reported from in vitro cell culture observation that the primary cells get to adhere with other cells within 1–2 days of the cell culture (Tang et al., 2021). It was shown that after the fifth passage, these cells form a single layer, thereby showing a vortex or radial growth pattern (Tang et al., 2021). Later, their morphology changes to a single long spindle. The exosomes derived from ADMSCs have uniform cup shape morphology with average diameter ranges between 30 and 120 nm as evident from the scanning electron microscopy (Tang et al., 2021). The exosomes can be detected using flow cytometry, differential centrifugation, magnetic bead assay, and transmission electron microscopy. The presence of surface proteins CD9, CD10, CD13, CD29, CD44, CD63, CD73, CD90, CD105, enkephalin enzyme, and major histocompatibility complex MHC I molecules distinguishes these from other cell-derived exosomes. It is still a research lacuna that no single surface marker has been identified for these exosomes.
The umbilical cord has a placental origin and is involved in giving nourishment and nutrition to the fetus from the mother during pregnancy. Human-derived MSCs can be broadly classified into (i) human umbilical cord mesenchymal stem cells (hUC-MSCs), (ii) human umbilical cord perivascular MSCs (HUCPV-MSCs), (iii) human umbilical cord Wharton’s Jelly MSCs (HWJ-MSCs), and (iv) human amniotic membrane-derived MSCs (HA-MSCs) (Subra et al., 2010).
The morphology of the primary UCMSCs demonstrates a spindle shape with the absence of vortex growth. Later, the cells show vortex growth after day 1 of the direct adherent cell culture using the primary cell tissue mass culture method. Moreover, few cells during the fifth passage show vortex patterns from the second generation until the fifth generation (Tang et al., 2021). With the fifth passage, the cells become long, elongated, and fusiform with typical vortex growth. The exosomes derived from these primary cells show variation in size in the range between 30 and 100 nm, as revealed from electron microscopy (Tang et al., 2021). The exosome morphology exhibits a round or elliptical membranous structure with clear and distinct boundaries (Vohra et al., 2020). The UCMSCs exhibit cell-specific surface markers including CD29, CD44, C/73 (SH3), CD90 (Thy-1), and CD105 (SH2) and negative for CD11b, CD34, and CD45, while their extracted exosomes demonstrate CD9, CD63, and CD81 and the multivesicular biosynthesis-related protein ALIX (Tang et al., 2021).
The BMSCs can be isolated from bone marrow with the inbuilt advantage of low infection rate of pathogenic microorganisms, efficient and stable biological role, low immune rejection post-transplantation, and good survival rate in higher passages (Tan et al., 2020). These cells exhibit diverse size and shape and become adherent after 1–2 days of the cell culture seeding in the appropriate culture medium. The adherent cell shows round morphology as demonstrated by electron microscopy. These cells begin to colonize after 4–5 days of the culture exhibiting a single fusiform shape forming a vortex growth pattern usually at the fourth passage (Tang et al., 2021). The exosomes derived from BMSCs are uniform with a size range between 30 and 100 nm in diameter and having a cup-shaped morphology with clear and distinct boundaries (Tang et al., 2021).
Western blotting and flow cytometry analysis of the BMSC-derived exosomes show expression of CD9, CD63, CD81, HSP70, syntenin-1, and multi-vesicular biosynthesis-related protein TSG101 (Tang et al., 2021).
The following exosome isolation methods are currently available worldwide: microfluidics, differential centrifugation, precipitation, antibody affinity capture, ultrafiltration, flushing separation, magnetic bead-based capture, and size-exclusion chromatography (SEC).
Microfluidics provides highly efficient, precise control, and rapid methods for isolating the exosomes on a single chip with manipulated fluids at microscale levels. The basic principle of microfluidics is that it manipulates a small quantity of the fluid using specialized micro-dimension channels using capillary forces. Its manipulation characteristic with fluids in a micro/nanoscale environment makes it a highly preferred method of choice among researchers. The basic design of this method involves a single chip of a few square centimeters dimension with a scope of scaling up isolation and separation. This unique approach relies on interdisciplinary sciences that include physics, fluid chemistry, micro-processing, and bioengineering. In one published study, a microfluidics chip is coupled with acoustic, electrophoretic, and electromagnetic separations, which showed a fast and efficient way of exosome isolation and separation (Popovic et al., 2018). In another related study, the implication of silicon nanowires is engraved on the microchip pillar walls for trapping liposomes, and acoustic nanofiltration is used for isolation of exosomes within a size range of 100–1000 nm (Kurian et al., 2021).
One more study exhibited the use of viscoelastic microfluidics for the isolation and separation of the exosomes with an isolation efficiency of >80% and a purity degree of >90% (Kurian et al., 2021). Membrane of different pore sizes was also implicated for the separation of exosomes based on filtration using ExoTIC microfluidics chip (Lin et al., 2020). In another study, electric forces are applied along with a dialysis membrane of 30 nm pore size for the isolation of exosomes (Yang et al., 2017). Wu et al. (2017) used whole blood to isolate exosomes using the acoustic fluidics approach in combination with microfluidics. This system showed the unique feature of the cell removal module, which separates exosomes from microvesicles (Wu et al., 2017). The main advantages of this method are as follows: (i) it requires a lower amount of the sample volume, (ii) it is a time-saving approach, and (iii) it is a cost-saving and real-time process. The only disadvantage of this method is less sensitivity for the isolation of exosomes. So, a scale-up is required in this technology for the production of clinical-grade exosomes.
This is the most widely used method for the isolation of the exosomes (Momen-Heravi et al., 2013). Cell debris and apoptotic bodies shed exosomes during successive rounds of the centrifugation mechanism. This method is based on the density, size, and shape of the exosomes. This gold standard method for exosome isolation, however, exhibits low yield and insufficient purity due to similarity in sedimentation properties of the different types of EVs (Tauro et al., 2012; Witwer et al., 2013; Cvjetkovic et al., 2014; Lane et al., 2015).
The main advantages of this method include reduction of cost and contamination. Additionally, a large sample capacity can be easily handled with this technique followed by high yields of exosomes. In another study, researchers have added 30% sucrose in the first step and reported a high yield of the exosomes (Bajimaya et al., 2017). Moreover, the limitations of the present approach are that high-speed centrifugation can damage the exosomes and it needs a long runtime with labor-intensive work. In one of the studies, it was found that performing ultracentrifugation three times reduces the purity of the exosomes (Tang et al., 2021).
The precipitation of the exosomes depends on the principle of altering the solubility or dispersibility of the exosomes within a water-devoid medium. In this approach, the external solvent is implicated in the solution, which changes the polarity and solubility of the components present within the components, as a resultant, initiate the precipitation of desired molecules. It is a very simple approach for the isolation of the exosome. In one of the previously published studies, it was found that the precipitation approach is very effective in the separation of biological fluids (Maroto et al., 2017). Several commercial isolations and purification kits for exosomes are available, showing good yield and purity, including SerumTM, the Exo-Q and Exo-SpinTM blood cell purification kits, the mi-RCURY Exosome Separation Kit, the Exo Quick-TC ExosomeTM Precipitation Solution Kit, and the Total Exosome Isolation kit (Maroto et al., 2017; Zhao et al., 2017; Buschmann et al., 2018; Soares Martins et al., 2018). The advantages of the current methods are that they are easy to use, do not require sophisticated and specialized machines, do not put any harsh effect on exosomes, and can be used on large sample volumes. Some limitations of these methods include the co-precipitation of other contaminants like polymeric materials, proteins, and lipids and the fact that they additionally require a long runtime to complete the process.
This process is also called an immunomagnetic bead-based assay. It is a recently developed technology that uses ExoCAS-2 charge-based ion exchange and magnetic beads for the isolation of exosomes from biofluids (Kim and Shin, 2021). This ExoCAS-2 implicates polycationic polymer-functionalized and -coated magnetic beads. The sample before the magnetic separation is filtered to exclude the large size impurities present. The mechanism of separation of the exosomes involves the binding of negatively charged exosomes with the positively charged poly-L-lysine-coated cationic beads via electrostatic interactions (Kim and Shin, 2021). Following the process of incubation and continuous stirring, the nano-sized exosomes bind to the surface of the coated beads and later eluted using an elution buffer with different ionic strengths that disrupts the electrostatic interactions. This efficient exosome separation and isolation approach yields exosomes of high purity grade, but the limitations associated with this technology are that it cannot be used in clinics, it has a high cost, and the rate of unspecific binding during the binding process is higher.
This technique is based on the application of specific pore size diameter membranes for separation and isolation of the exosomes (Cheruvanky et al., 2007; Lobb et al., 2015; Konoshenko et al., 2018). This approach can be complementary with ultracentrifugation, although it can also be performed alone. Another improved version of ultrafiltration includes cross-flow filtration or tangential flow filtration (McNamara et al., 2018). This improvement helps in removing the protein contaminants from the exosomes containing samples if repeatedly passed from the exclusion filter of a defined diameter, thereby concentrating the exosomes. In one of the studies, it was claimed that a cellulose membrane with a pore size of 10 kDa is very efficient in the recovery of the exosomes using an ultrafiltration approach (Vergauwen et al., 2017). The advantages of ultrafiltration are that it does not require expensive equipment and consumes less time. The only associated limitation with the ultrafiltration method is exosome loss due to attaching with membranes as a result of shear stress and membrane clogging.
Size-exclusion chromatography depends on the separation of the exosomes’ molecules based on their size. The sample containing the exosomes is passed through the column consisting of the beads with variant pore size. Each molecule is passed through the individual beads based on their size. The small-size molecules show delayed elution from the column, as they have to traverse the complete length of the column. In one of the studies, it was found that exosomes have large hydrodynamic radii, passing through the column faster, as they do not show penetration inside the beads (Feng et al., 2014). In another, a single-step SEC using a Sepharose CL-2B column was used for isolation of exosomes with 75 nm diameter effectively from body fluids (Böing et al., 2014). This method allows minimal harm to the isolated exosomes compared to other precipitation-based methods. The SEC approach for isolating exosomes can efficiently remove the plasma proteins from the biological samples, as claimed by one of the studies (Gámez-Valero et al., 2016). In one of the studies, the authors have isolated clean and non-aggregated exosomes with a size range of 50–200 nm (Hong et al., 2016). It is also evident that SEC in conjugation with an ultracentrifugation approach can be efficiently used for the isolation of the exosomes from the biological fluids, compared to alone itself. The main advantages associated with SEC are that it can be used for the separation of the small and large molecules in biological fluids without altering the exosomal structure. The only limitation is the requirement of a long runtime.
Exosome-based delivery approaches showed promising benefits related to specificity, safety, and stability due to their inbuilt homing characteristics that exhibit effective delivery of desired cargo to specific target sites. Recent studies showed that exosomes can be used to deliver small interfering RNA (siRNA) or active pharmaceutical agents like drugs and vaccines to treat diseases (Aryani and Denecke, 2016). These nano-size envelopes tend to avoid phagocytosis and engulfment by lysosomes with a low immune response (Ha et al., 2016). Several tailoring approaches for modification of exosomes and loading of the desired cargo into the exosomes were studied, which can be broadly classified into two strategies: (i) exogenous tailoring of exosomes post isolation and (ii) endogenous tailoring during biogenesis of exosomes. Exogenous tailoring approaches can be further divided into an active and passive form; the active approach involves the sonication, extrusion, freeze–thaw cycles, electroporation, and chemical-based approach, while the passive form involves the incubation process. Moreover, the endogenous tailoring of exosomes involves the introduction of the cargo of interest into the cells producing exosomes, which commonly implies the application of transfecting cells with expression vectors as in genetic engineering for targeted therapy (Van der Meel et al., 2014). The following paragraphs provide a brief overview of the tailoring approach for modifications of exosomes.
This tailoring approach simply involves the incubation of exosomes with the desired interest of cargo, which can be referred to as passive loading. The potential difference created due to the interplay between the concentration of desired cargo inside and outside the exosomes drives the infusion of desired cargos through the lipid bilayer membrane of exosomes. In few cancer-related research studies, this method was used to load chemotherapeutic drugs like paclitaxel and doxorubicin into the exosomes and also to observe enhanced chemotherapeutic effects (Tian et al., 2014; Yang et al., 2015; Salarpour et al., 2019). This enhanced effect of drug-loaded exosomes is observed due to the ease of crossing the blood–brain barrier. In another study, exosomes loaded with enzymes were used in the treatment of Parkinson’s disease (Haney et al., 2015). In one of the studies, the authors have co-incubated curcumin with exosomes and found that it gets self-assembled with the lipid bilayer of exosomes due to the interplay of hydroscopic interactions. The curcumin encapsulated exosome not only increased the target specificity but also enhanced the anti-inflammatory property of curcumin (Sun et al., 2010). Though proven to be useful for modifying exosomes for their enhanced functionality with the desired cargo, this method sometimes affects the size of exosomes resulting in low yield, low entrapment, and uncontrollable drug loading. The present method is simple, cost-effective, and effective in transporting hydrophilic cargos efficiently into the exosomes.
Sonication provides an additional advantage of enhancing the loading of desired cargo inside the bilayer membrane of the exosomes. This approach utilizes sound waves generated from a sonicator machine to induce a shearing force effect upon the exosome membrane, which, in turn, increases the uptake of desired cargo inside the exosomes (Kim et al., 2016). Kim et al. (2016) successfully loaded paclitaxel and doxorubicin into the exosomes implicating this approach. It is believed that the sonication process decreases the micro-viscosity of the exosomal membrane that allows the passage of cargo inside (Kim et al., 2016). This cargo loading approach is healthy for biological molecules like small RNAs due to its high loading efficiency. Some limitations like the development of shearing forces, exosomal membrane deformation, heat generation during the sonication cycle, loss of exosomal surface proteins, and non-suitability for hydrophobic drug delivery are associated with this approach.
This tailoring method involves lipid bilayer membrane disruption of exosomes during extrusion through a small-size polycarbonate porous membrane. This reversible disruption in the membrane allows the entry of desired cargo of interest inside the exosomes (Haney et al., 2015). Le Saux et al. (2020) have reported that extrusion is an efficient and promising method for tailoring the exosomes and loading the desired cargo inside it for targeted delivery. In one previous study, exosomes were extruded with porphyrins (Fuhrmann et al., 2015). The extrusion mechanism reshapes and reforms the exosomal membrane extensively and thereby showed higher loading efficiency (Jamur and Oliver, 2010).
This tailoring approach involves freezing and subsequent thawing of the exosome sample in a vessel of desired cargo to be loaded. The mix is incubated at 37°C followed by rapid freezing at –80°C; the same steps were repeated several times depending on the efficiency of the system. It is always advisable to strictly monitor the freeze–thawing steps, because it may form the aggregates of these vesicles into large size particles (Haney et al., 2015; Le Saux et al., 2020). In one previously published study, catalase was loaded to exosomes using the freeze–thaw method (frozen at –80°C and thawed at RT) (Haney et al., 2015). It was also demonstrated that several freeze–thaw cycles resulted in a lipid dilution ratio that may be easily interpreted from fluorescence resonance energy transfer (FRET) assay (Sato et al., 2016). Though this method produces aggregates of exosomes with lower drug loading capacity compared to sonication or extrusion procedures, it is followed widely for cargo loading.
Tailoring of exosomes for loading cargo using electroporation is a commonly applied method that employs an electric field for cargo uptake. In the electric field, the phospholipid bilayer membrane is disrupted, thereby allowing the entry of hydrophilic compounds like small DNAs, miRNA, and siRNAs (Faruqu et al., 2018; Kobayashi et al., 2020; Lv et al., 2020; Orefice, 2020). A recent study has reported that exosomes tend to form aggregates during electroporation, although it did not affect the function of exosomes. However, certain refinements in the technique such as carrying out electroporation in an optimal buffer containing trehalose maintain the structural integrity of exosomes (Johnsen et al., 2016). In another study, fused exosomes were derived from αv-integrin-specific iRGD peptide with doxorubicin efficiently with electroporation and proved targeted tumor therapy (Gong et al., 2019). In one of the previously published studies, it was found that miRNA delivery to exosomes under mild electroporation protects miRNA from RNase degradation and showed efficient loading (Pomatto et al., 2019). In light of all the studies, it can be said that electroporation is a reliable method for cargo loading in exosomes that preserves the naïve cargo without compromising the structural integrity of exosomes.
Chemical transfection is preferably used to incorporate siRNA into exosomes under the influence of the transfection agent Lipofectamine 2000. Wahlgren et al. used a liposome-based transfection reagent to incorporate MAPK-1-siRNA into the exosomes by incubating at 37°C for 10 min (Wahlgren et al., 2012). This method of loading desired cargo into exosomes achieves relatively high transfection efficiency using lipids. Cationic transfection agents are the preferred choice of researchers considering their high degree of success. These chemical transfection reagents showed a high success rate in in vitro experiments; however, they have worse efficiency than electroporation. Immunogenicity and toxicity are some of the associated limitations of this approach.
Genetic engineering is another remarkable approach for the production of loaded exosomes with desired characteristics and functions. This approach involves transfection of the donor cells, thereby initiating the upregulation of specific genes, allowing the synthesis of specific gene-linked cargo-loaded exosomes during their biogenesis. The insertion of the desired “gene of interest” in the parent cell type is achieved by either viral/non-viral invasion/infection. The infection efficiency is optimized by the quantity and quality of the exosomal cargo. It is well reported that exosomes originate through the endosomal machinery of the cell membrane. Exosomal content reflects lineage and the original cell type; therefore, depending on the experimental requirement and/or therapeutic applications, the host cell selection should be performed. Genetic engineering for modification of the exosomal content from different cell types predominantly involves two types of viral vectors: (i) retroviral and (ii) adenoviral.
Jiang et al. (2020) observed the therapeutic effect of tumor necrosis factor (TNF)-stimulated gene-6 (TSG-6) modified MSC-derived exosomes in a wound model and found that tailoring of such exosomes prevents scar formation. In addition, several research studies demonstrated the therapeutic role of MSC-derived exosomes tailored with such methods carrying miRNA in improving treatment modalities (Xin et al., 2012). Wei et al. (2019) successfully engineered immature mouse dendritic cells, for exosome production, expressing Lamp2b fused to αγ integrin-specific iRGD peptide for breast cancer treatment in vitro. In one of the studies, engineered HEK293T was used for expression of Lamp2B along with a fragment of IL-3 and showed a reduction in tumor growth and was found to be effective in treating chronic myeloid leukemia (CML) (Bellavia et al., 2017). Rivoltini et al. (2016) transduced K562 cells with lentiviral human membrane TRAIL (TNF-Related Apoptosis-Inducing Ligand) for the production of TRAIL (+) exosomes. The authors reported apoptosis in cancer cells on treatment with TRAIL exosomes. Furthermore, the in vivo analysis revealed that engineered exosomes induced necrosis and vessel damage in melanoma tumor subjects (Rivoltini et al., 2016). In another study, exosomes enriched with miR-503 showed promising therapeutic potential for cancer treatment (Bovy et al., 2015).
“Omni Spirant” (patent pending) is a recently developed regenerative gene therapy for cystic fibrosis (CF) and involves the use of surface-engineered exosomes/bioengineered stem cell exosomes. The method involves mucus penetration of the exosomes and delivery of the gene therapy cargo for the effective treatment of CF (Health Europa, 2021). Bioengineering of cells for the production of engineered exosomes has gained significant attention in the past few years. However, further studies are mandatory for designing protocols with improved stability, drug solubility, and bioavailability, for the therapeutic application of engineered exosomes.
Mesenchymal stromal cell have a diverse role including multi-differentiation and immunomodulation that significantly contribute in reducing inflammation-related complications (Philipp et al., 2018). These MSCs show a contributory role in a paracrine manner mediating through secreted growth factors, cytokines, and exosomes (Phinney and Pittenger, 2017). One of the previously published studies quoted that MSC-mediated paracrine secretion promotes wound healing (Kourembanas, 2015). The advantage of using exosomes over cell-based therapies is that these vesicles may overcome the side effects associated with cell transplantation such as immune rejection. Pathogenesis of bacteria-associated DFUs is contributed by poor innervation and vascularization and chronic inflammation. In a recent study, it was observed that exosomes derived from MSCs inhibit M1 polarization and simultaneously promote M2 polarization that helps in the reduction of the inflammation (Cao et al., 2017). It is also found that these exosomes promote skin wound healing mediated by the regulation of M2 polarization (Cao et al., 2017). This dual nature of exosomes, i.e., anti-inflammatory and skin wound healing, can be explored in bacteria-associated DFUs.
Tailored MSC-derived exosomes possess promising result in the treatment of DFUs and diabetic wounds. In a recent study, exosomes derived after pre-treatment of MSCs with salidroside (glucoside of tyrosol) showed healing of diabetic wounds (Ariyanti et al., 2019). Similarly, fluoxetine and pretreated MSC exosomes managed diabetic neuropathy well (Abdelrahman et al., 2018).
It has been proved that these exosomes occupy the class of paracrine factor that mediates the therapeutic, tissue repair, and wound healing effects of MSCs (Joo et al., 2020). Several clinical trials showed the efficacy of BMSCs in the treatment of diabetic wound and ulcers (Table 1). In another research, tailored exosomes derived from pretreated BMSCs with atorvastatin (ATV) showed an acceleration in the healing of diabetic wound both in vivo and in vitro (Yu et al., 2020). It has been found that pretreated BMSCs with ATV secrete exosomes that activate the AKT/eNOS signaling mechanism that further initiates the angiogenesis of endothelial cells mediated through upregulation of miR-211-3p, thereby showing significant wound healing in the diabetic environment (Joo et al., 2020). In another study of exosome modification, it was found that exosomes derived from blue light-exposed human umbilical cord MSCs showed improved wound healing mediated through upregulation of MEF2C signaling (Yang et al., 2019).
Epidermal growth factor (EGF) and human adipose cell-derived stem cell exosome-loaded microcapsules integrated with collagen hydrogel can effectively show tissue regeneration and also restoration of blood perfusion in diabetic wounds (Cao et al., 2017). In the previously published literature, it has been found that adipose-derived MSC exosomes incorporated in freeze–thaw-based polypeptide-based hydrogel possess self-healing, antibacterial, and exosome release characteristics (Shen et al., 2016). These properties are useful in promoting wound healing by enhancing cell proliferation, neovascularization, re-epithelialization, and collagen remodeling at the wound site (Wang et al., 2019). In another recent tailoring approach, the cells are genetically engineered with transfection and co-culture to synthesize exosomes containing long non-coding RNA H19 that helps promote wound healing in DFU mediated by upregulation of PTEN through miRNA-152-3p (Li et al., 2020). Figure 3 demonstrates the paracrine effect of BMSCs in treatment of DFUs mediated via EVs. These tailoring approaches of exosomes may help provide promising results in the healing of DFUs associated with bacteria. The current work encourages the implication of differential centrifugation and ultracentrifugation method for isolation of EVs from spent media or any other sources. The reason for recommending these two methods is due to their low cost and easy installation in any lab/clinic. Moreover, the genetic engineering approach endogenous modification is suitable for modification of EVs if they are used for delivering genes of interest. The modified EVs can be easily used in the treatment of ulcers/wounds associated with the DM. For instance, DFUs associated with bacteria need antibacterial and regenerative therapy. EVs, if modified for gene delivery (for initiating regeneration of damaged skin) and drug (antibiotics/antibacterial), can fulfill the purpose of therapeutic intervention.
Figure 3. Mechanism of BM-MSCs for treatment of DFU. BM-MSCs can migrate and adhere via CCR7, ICAM1-, VCAM1-, and Akt- dependent mechanism and enhance angiogenesis through increasing VEGF, NGF, BDNF, VEGF-A, eNOS, and HIF. Cell proliferation of HUVECs and keratinocytes plays significant role in angiogenesis and reepithelialization, respectively. Keratinocyte function is improved by regulating IGF-1, EGF, MMP-2, MMP-9, TIMP-1, TIMP-2, and Erk signaling pathway. CCR7, C-C chemokine receptor type 7; ICAM1, intercellular adhesion molecule 1; VCAM1, vascular adhesion molecule 1; VEGF, vascular endothelial growth factor; NGF, nerve growth factor; BDNF, brain-derived neurotrophic factor; VEGF-A, vascular endothelial growth factor A; eNOS, endothelial nitric oxide synthase; HIF, hypoxia inducible factor; IGF-1, insulin-like growth factor 1; EGF, epidermal growth factor; MMP-2, matrix metalloproteinase-2; MMP-9, matrix metalloproteinase-9; TIMP-1, tissue inhibitor of metalloproteinase-1; and TIMP-2, tissue inhibitor of metalloproteinase-2. [Adopted from Cao et al. (2017) distributed under the Creative Commons Attribution Licens].
Diabetes mellitus is characterized by high blood glucose level and neuropathy that slow down the wound healing process. These slow-healing wounds are vulnerable to bacterial infections (Buch et al., 2019). These diabetic wounds and foot ulcers become chronic due to microbe habitat on the wound site (Bjarnsholt et al., 2008). This continuous growth of bacteria (both aerobes and anaerobes) on the wound site produces biofilm, which exhibits resistance toward antibiotics that in turn causes a problem in the treatment of these wounds (Shiau and Wu, 1998; Bridier et al., 2011). It has been observed that Staphylococcus aureus is among the most common bacteria that are prevalent in DFUs (Kalan et al., 2019). Moreover, other bacteria causing DFUs includes β-hemolytic streptococci, S. aureus, S. saprophyticus, S. epidermis, Streptococcus pyogenes, S. mutans, P. aeruginosa, Bacillus subtilis, Proteus species, Escherichia coli, and Klebsiella pneumoniae. The anaerobic bacteria include Peptostreptococcus species, anaerobic streptococci, Bacteroides fragilis, and Clostridium species (Lipsky et al., 2012; Richard et al., 2012; Kalan et al., 2019). Bacterial biofilms of diabetic wounds and DFUs are protected from various stresses, including antibiotics and immune responses. Biofilm production involves the uncontrolled growth of sessile and planktonic bacteria that grow continuously on themselves to form a layer that is termed biofilm. Treatment of biofilms is also a major health concern as emphasized by the World Health Organization (WHO), as it contributes to the development of antimicrobial resistance toward antibiotics. Clinicians and researchers are focusing on the promising alternative treatment approaches to the use of antibiotics in reducing bacterial infections. Natural sources such as plant-derived extracts, polyphenols, anti-sense RNA, and stem cell-derived exosomes might be the prospective alternative therapies to manage DFUs and diabetic wounds. Several emerging technologies identify the risk assessment associated with DFUs, including laser Doppler flowmetry, infrared thermography, ultrasound indentation tests (elastography), and plantar pressure and pressure gradient system (Lung et al., 2020). These technologies may be helpful in the screening of risk in DFUs, so that treatment approaches may be customized accordingly.
Extracellular vesicles are the key component of cell-to-cell communication that facilitates transfer of internalized cargo, including proteins, nucleic acids, and other biological factors. EVs are known to play an active role in pathological conditions like kidney injury, inflammatory disorders, wound healing, and regeneration, along with several therapeutic and diagnostic characteristics. A previously published study demonstrated that EVs possess antimicrobial peptides (AMPs) (Hiemstra et al., 2014). EVs were also reported to contain lysozyme C, dermcidin, mucin-1, calprotectin, and myeloperoxidase and to have a bactericidal effect. In one of the recent studies conducted in vitro on urinary exosomes, it was found that these exosomes showed a bactericidal effect against E. coli (Francisca et al., 2017). The same research concluded that nasal lavage fluid-derived exosomes showed defense against pathogens and allergens (Francisca et al., 2017).
In another study, it was found that EVs released from biliary and intestinal epithelium luminal contain AMPs along with LL-37 and hBD-2 that activate the toll-like receptor (TLR)-4 signaling cascade and contribute toward antimicrobial defense (Hu et al., 2013). In the past few years, MSC-derived EVs have been explored for therapeutic, diagnostic, and anti-inflammatory roles in several pre-clinical trials. In one of the published reports, it was found that MVs secreted by BMSCs are efficient in the treatment of acute lung injury (ALI) caused by E. coli endotoxins via transfer of keratinocyte growth factor (KGF) mRNA from the MVs to damaged lung endothelium and alveolar epithelium (Zhu et al., 2014). In another animal study conducted on a bacterial pneumonia mouse model, it was demonstrated that BMSC-extracted MVs showed significant survival and lessen the influx of inflammatory cells (Monsel et al., 2015). In another study, the antimicrobial effect of MSC-derived EVs was demonstrated, which is mediated by the transfer of mitochondria into the target cells that in turn increases the phagocytosis of macrophages (Islam et al., 2001). Several in vivo clinical trials demonstrated the antibacterial effect of MSC-derived EVs (Krasnodembskaya et al., 2010; Harman et al., 2017; Cortés-Araya et al., 2018). However, more studies and clinical trials are needed to establish the significant role of MSC-derived EVs as antimicrobial agent. This antimicrobial effect of EVs can be explored and serve as a prospective therapy for the treatment of diabetic wounds and DFUs.
Extracellular vesicles are known to be the safest therapeutic approach for both pre-clinical and clinical use. There were no signs of toxicity observed in previously published literature except that some human cell-derived EVs possess the potential to elicit an immune response, which is a positive sign for using EVs as cell-free therapeutic approach in DFUs (Zhu et al., 2017). In one study, C57BL/6 mice were given EVs for 3 weeks via intravenous and intraperitoneal administration, and no toxicity was observed with slight changes in expression of immune markers (Zhu et al., 2017). In another murine study, BMSC-derived engineered exosome (iExosomes) administration did not produce any toxicity and adverse immune reactions (Mendt et al., 2018). The engineered approaches for EVs mentioned in the present work suggest that EVs are a safe and non-toxic method for delivering cargo compared to cationic lipids, viral vectors, and polymer-based methods (Mendt et al., 2018). Moreover, long-term pre-clinical and clinical studies are needed to further evaluate the toxicological and immunological profile of engineered EVs (Table 2).
Extracellular vesicles are emerging as new therapeutics in the management of diseases, regeneration of tissue, and diagnostic markers. The heterogeneity and complexity with the ability of modification under a physiological and pathological environment make them interesting candidates for implication in the biological field. Exosomes have the potential to treat various diseases due to flexibility of loading diverse drugs and modifications. Exosomes can be used for detection, diagnosis, and treatment only because of their tendency of modification in the membrane. Moreover, MSC-derived exosomes are primarily exploited for regenerative medicine. Despite the fact that many advances in the modification approach of exosomes are currently being practiced; one of the most significant challenge with these vesicles is their inefficient production at a large scale for clinical use following GMP/GCP guidelines. MSC-derived exosomes are a rich source of AMPs along with other anti-bactericidal factors, which opens up the window of treating DFUs caused by microorganisms including S. aureus, S. saprophyticus, S. epidermis, S. pyogenes, S. mutans, P. aeruginosa, B. subtilis, Proteus species, E. coli, and K. pneumoniae. The potential bactericidal efficacy of the MSC-derived exosomes can be amplified through modification of cell conditioning medium and drug loading approach. AMP-encapsulated exosomes can be exploited further for clinical trials to treat DFUs associated with microbes. Notable EV-based management therapies promote wound/ulcer healing along with minimal scarring without ethical issues and conflicts. Future studies including pre-clinical and clinical trials are required to explore the therapeutic and anti-microbial effect of the MSC-derived exosomes. These EVs can be exploited in designing wound dressings that might be prospectively used in the treatment of DFUs associated with bacteria.
AR, PT, and KG are currently involved in COVID-19 testing duties.
AR, BM, SB, KG, QM, and AS are involved in manuscript writing, conceptualization, and data analysis. PT, G-BJ, PS, MS, and JA supervised and reviewed the manuscript. All the authors have read and agreed to the published version of the manuscript.
The authors declare that the research was conducted in the absence of any commercial or financial relationships that could be construed as a potential conflict of interest.
All claims expressed in this article are solely those of the authors and do not necessarily represent those of their affiliated organizations, or those of the publisher, the editors and the reviewers. Any product that may be evaluated in this article, or claim that may be made by its manufacturer, is not guaranteed or endorsed by the publisher.
AR and KG want to thank the Multidisciplinary Research Unit, Department of Health Research, Ministry of Health and Family Welfare, New Delhi, for providing financial assistance in the form of salary.
Abdelrahman, S. A., Samak, M. A., and Shalaby, S. M. (2018). Fluoxetine pretreatment enhances neurogenic, angiogenic and immunomodulatory effects of MSCs on experimentally induced diabetic neuropathy. Cell Tissue Res. 374, 83–97. doi: 10.1007/s00441-018-2838-6
Adriano, B., Cotto, N. M., Chauhan, N., Jaggi, M., Chauhan, S. C., and Yallapu, M. M. (2021). Milk exosomes: nature’s abundant nanoplatform for theranostic applications. Bioactive Mater. 6, 2479–2490. doi: 10.1016/j.bioactmat.2021.01.009
An, T., Chen, Y., Tu, Y., and Lin, P. (2021). Mesenchymal stromal cell-derived extracellular vesicles in the treatment of diabetic foot ulcers: application and challenges. Stem Cell Rev. Rep. 17, 369–378. doi: 10.1007/s12015-020-10014-9
Antimisiaris, S. G., Mourtas, S., and Marazioti, A. (2018). Exosomes and exosome-inspired vesicles for targeted drug delivery. Pharmaceutics 10:218. doi: 10.3390/pharmaceutics10040218
Ariyanti, A. D., Zhang, J., Marcelina, O., Nugrahaningrum, D. A., Wang, G., Kasim, V., et al. (2019). Salidroside-pretreated mesenchymal stem cells enhance diabetic wound healing by promoting paracrine function and survival of mesenchymal stem cells under hyperglycemia. Stem Cells Transl. Med. 8, 404–414. doi: 10.1002/sctm.18-0143
Aryani, A., and Denecke, B. (2016). Exosomes as a nanodelivery system: a key to the future of neuromedicine? Mol. Neurobiol. 53, 818–834. doi: 10.1007/s12035-014-9054-5
Bajimaya, S., Frankl, T., Hayashi, T., and Takimoto, T. (2017). Cholesterol is required for stability and infectivity of influenza A and respiratory syncytial viruses. Virology 510, 234–241. doi: 10.1016/j.virol.2017.07.024
Bellavia, D., Raimondo, S., Calabrese, G., Forte, S., Cristaldi, M., Patinella, A., et al. (2017). Interleukin 3-receptor targeted exosomes inhibit in vitro and in vivo chronic myelogenous leukemia cell growth. Theranostics 7, 1333–1345. doi: 10.7150/thno.17092
Bertrand, N., and Leroux, J. C. (2012). The journey of a drug-carrier in the body: an anatomo-physiological perspective. J. Controlled Release 161, 152–163. doi: 10.1016/j.jconrel.2011.09.098
Bjarnsholt, T., Kirketerp-Møller, K., Jensen, P. Ø, Madsen, K. G., Phipps, R., Krogfelt, K., et al. (2008). Why chronic wounds will not heal: a novel hypothesis. Wo und Repair Regen. 16, 2–10. doi: 10.1111/j.1524-475x.2007.00283.x
Böing, A. N., Van Der Pol, E., Grootemaat, A. E., Coumans, F. A., Sturk, A., and Nieuwland, R. (2014). Single-step isolation of extracellular vesicles by size-exclusion chromatography. J. Extracell. Ves. 3:10.3402/jev.v3.23430. doi: 10.3402/jev.v3.23430
Bovy, N., Blomme, B., Frères, P., Dederen, S., Nivelles, O., Lion, M., et al. (2015). Endothelial exosomes contribute to the antitumor response during breast cancer neoadjuvant chemotherapy via microRNA transfer. Oncotarget 6, 10253–10266. doi: 10.18632/oncotarget.3520
Bridier, A., Briandet, R., Thomas, V., and Dubois-B rissonnet, F. (2011). Resistance ofbacterial biofilms to disinfectants: a review. Biofouling 27, 1017–1032.4.
Buch, P. J., Chai, Y., and Goluch. (2019). Treating polymicrobial infections in chronic diabetic wounds. Clin. Microbiol. Rev. 32, 1–17. doi: 10.1007/978-1-4899-5968-3_1
Buschmann, D., Kirchner, B., Hermann, S., Märte, M., Wurmser, C., Brandes, F., et al. (2018). Evaluation of serum extracellular vesicle isolation methods for profiling miRNAs by next-generation sequencing. J. Extracell. Ves. 7:1481321. doi: 10.1080/20013078.2018.1481321
Cao, Y., Gang, X., Sun, C., and Wang, G. (2017). Mesenchymal stem cells improve healing of diabetic foot ulcer. J. Diab. Res. 2017:9328347. doi: 10.1155/2017/9328347
Cavallaro, S., Horak, J., Hååg, P., Gupta, D., Stiller, C., Sahu, S. S., et al. (2019). Label-free surface protein profiling of extracellular vesicles by an electrokinetic sensor. ACS Sens. 5, 1399–1408. doi: 10.1021/acssensors.9b00418
Cheruvanky, A., Zhou, H., Pisitkun, T., Kopp, J. B., Knepper, M. A., Yuen, P. S. T., et al. (2007). Rapid isolation of urinary exosomal biomarkers using a nanomembrane ultrafiltration concentrator. Am. J. Physiol. Renal Physiol. 292, F1657–F1661.
Cortés-Araya, Y., Amilon, K., Rink, B. E., Black, G., Lisowski, Z., et al. (2018). Comparison of antibacterial and immunological properties of mesenchymal stem/stromal cells from equine bone marrow, endometrium, and adipose tissue. Stem Cells Dev. 27, 1518–1525. doi: 10.1089/scd.2017.0241
Cvjetkovic, A., Lötvall, J., and Lässer, C. (2014). The influence of rotor type and centrifugation time on the yield and purity of extracellular vesicles. J. Extracell. Ves. 3:23111. doi: 10.3402/jev.v3.23111
El Andaloussi, S., Lakhal, S., Mäger, I., and Wood, M. J. (2013). Exosomes for targeted siRNA delivery across biological barriers. Adv. Drug Deliv. Rev. 65, 391–397. doi: 10.1016/j.addr.2012.08.008
Faruqu, F. N., Xu, L., and Al-Jamal, K. T. (2018). Preparation of exosomes for siRNA delivery to cancer cells. J. Vis. Exp. 142:10.3791/58814. doi: 10.3791/58814
Feng, Y., Huang, W., Wani, M., Yu, X., and Ashraf, M. (2014). Ischemic preconditioning potentiates the protective effect of stem cells through secretion of exosomes by targeting Mecp2 via miR-22. PLoS One 9:e88685. doi: 10.1371/journal.pone.0088685
Francisca, A.-M., Jimena, C., and Maroun, K. (2017). Antimicrobial activity of mesenchymal stem cells: current status and new perspectives of antimicrobial peptide-based therapies. Front. Immunol. 8:339. doi: 10.3389/fimmu.2017.00339
Fuhrmann, G., Serio, A., Mazo, M., Nair, R., and Stevens, M. M. (2015). Active loading into extracellular vesicles significantly improves the cellular uptake and photodynamic effect of porphyrins. J. Control. Release 205, 35–44. doi: 10.1016/j.jconrel.2014.11.029
Gámez-Valero, A., Monguió-Tortajada, M., Carreras-Planella, L., Beyer, K., and Borràs, F. E. (2016). Size-exclusion chromatography-based isolation minimally alters extracellular vesicles’ characteristics compared to precipitating agents. Sci. Rep. 6:33641.
Gong, C., Tian, J., Wang, Z., Gao, Y., Wu, X., Ding, X., et al. (2019). Functional exosome-mediated co-delivery of doxorubicin and hydrophobically modified microRNA 159 for triple-negative breast cancer therapy. J. Nanobiotechnol. 17:93.
Gurunathan, S., Kang, M. H., and Kim, J. H. (2021). A comprehensive review on factors influences biogenesis, functions, therapeutic and clinical implications of exosomes. Int. J. Nanomed. 16, 1281–1312. doi: 10.2147/ijn.s291956
Ha, D., Yang, N., and Nadithe, V. (2016). Exosomes as therapeutic drug carriers and delivery vehicles across biological membranes: current perspectives and future challenges. Acta Pharm. Sin B. 6, 287–296. doi: 10.1016/j.apsb.2016.02.001
Haney, M. J., Klyachko, N. L., Zhao, Y., Gupta, R., Plotnikova, E. G., He, Z., et al. (2015). Exosomes as drug delivery vehicles for Parkinson’s disease therapy. J. Control. Release 207, 18–30.
Harman, R. M., Yang, S., He, M. K., and Van de Walle, G. R. (2017). Antimicrobial peptides secreted by equine mesenchymal stromal cells inhibit the growth of bacteria commonly found in skin wounds. Stem Cell Res. Ther. 8:157.
Health Europa (2021). Developing a First-in-class Regenerative Gene Therapy for Cystic Fibrosis. Available online at: https://www.healtheuropa.eu/regenerative-gene-therapy-cystic-fibrosis/91009/ (accessed April 6, 2021)
Hiemstra, T. F., Charles, P. D., Gracia, T., Hester, S. S., Gatto, L., Al-Lamki, R., et al. (2014). Human urinary exosomes as innate immune effectors. J. Am. Soc. Nephrol. 25, 2017–2027. doi: 10.1681/asn.2013101066
Hong, C. S., Funk, S., Muller, L., Boyiadzis, M., and Whiteside, T. L. (2016). Isolation of biologically active and morphologically intact exosomes from plasma of patients with cancer. J. Extracell. Ves. 5:29289. doi: 10.3402/jev.v5.29289
Hu, G., Gong, A.-Y., Roth, A. L., Huang, B. Q., Ward, H. D., Zhu, G., et al. (2013). Release of luminal exosomes contributes to TLR4-mediated epithelial antimicrobial defense. PLoS Pathog 9:e1003261. doi: 10.1371/journal.ppat.1003261
Islam, D., Bandholtz, L., Nilsson, J., Wigzell, H., Christensson, B., Agerberth, B., et al. (2001). Downregulation of bactericidal peptides in enteric infections: a novel immune escape mechanism with bacterial DNA as a potential regulator. Nat. Med. 7, 180–185. doi: 10.1038/84627
Jamur, M. C., and Oliver, C. (2010). Permeabilization of cell membranes. Methods Mol. Biol. 588, 63–66. doi: 10.1007/978-1-59745-324-0_9
Jan, A. T., Rahman, S., Badierah, R., Lee, E. J., Mattar, E. H., Redwan, E. M., et al. (2021). Expedition into exosome biology: a perspective of progress from discovery to therapeutic development. Cancers 13:1157. doi: 10.3390/cancers13051157
Jiang, L., Zhang, Y., Liu, T., Wang, X., Wang, H., Song, H., et al. (2020). Exosomes derived from TSG-6 modified mesenchymal stromal cells attenuate scar formation during wound healing. Biochimie 177, 40–49. doi: 10.1016/j.biochi.2020.08.003
Johnsen, K. B., Gudbergsson, J. M., Skov, M. N., Christiansen, G., Gurevich, L., Moos, T., et al. (2016). Evaluation of electroporation-induced adverse effects on adipose-derived stem cell exosomes. Cytotechnology 68, 2125–2138. doi: 10.1007/s10616-016-9952-7
Joo, H. S., Suh, J. H., Lee, H. J., Bang, E. S., and Lee, J. M. (2020). Current knowledge and future perspectives on mesenchymal stem cell-derived exosomes as a new therapeutic agent. Int. J. Mol. Sci. 21:727. doi: 10.3390/ijms21030727
Kalan, L. R., Meisel, J. S., Loesche, M. A., Horwinski, J., Soaita, I., Chen, X., et al. (2019). Strain- and species-level vari-ation in the microbiome of diabetic wounds is associated with clinical outcomes and therapeutic efficacy. Cell Host Microbe 25, 641–655.e5.
Kim, H., and Shin, S. (2021). ExoCAS-2: rapid and pure isolation of exosomes by anionic exchange using magnetic beads. Biomedicines 9:28. doi: 10.3390/biomedicines9010028
Kim, M. S., Haney, M. J., Zhao, Y., Mahajan, V., Deygen, I., Klyachko, N. L., et al. (2016). Development of exosome-encapsulated paclitaxel to overcome MDR in cancer cells. Nanomedicine 12, 655–664. doi: 10.1016/j.nano.2015.10.012
Kobayashi, M., Sawada, K., Miyamoto, M., Shimizu, A., Yamamoto, M., Kinose, Y., et al. (2020). Exploring the potential of engineered exosomes as delivery systems for tumor-suppressor microRNA replacement therapy in ovarian cancer. Biochem. Biophys. Res. Commun. 527, 153–161. doi: 10.1016/j.bbrc.2020.04.076
Konoshenko, M. Y., Lekchnov, E. A., Vlassov, A. V., and Laktionov, P. P. (2018). Isolation of extracellular vesicles: general methodologies and latest trends. BioMed. Res. Int. 2018:8545347.
Kourembanas, S. (2015). Exosomes: vehicles of intercellular signaling, biomarkers, and vectors of cell therapy. Annu. Rev. Physiol. 77, 13–27. doi: 10.1146/annurev-physiol-021014-071641
Krasnodembskaya, A., Song, Y., Fang, X., Gupta, N., Serikov, V., Lee, J.-W., et al. (2010). Antibacterial effect of human mesenchymal stem cells is mediated in part from secretion of the antimicrobial peptide LL-37. Stem Cells 28, 2229–2238. doi: 10.1002/stem.544
Kumar, P. G., Aslam, K. M., Haseeb, Z., Kumar, S. S., Moh’d, K., Seema, S., et al. (2019). Comparative analysis of exosome isolation methods using culture supernatant for optimum yield, purity and downstream applications. Sci. Rep. 9:5335.
Kurian, T. K., Banik, S., Gopal, D., Chakrabarti, S., and Mazumder, N. (2021). Elucidating methods for isolation and quantification of exosomes: a review. Mol. Biotechnol. 63, 249–266. doi: 10.1007/s12033-021-00300-3
Lai, C. P., and Breakefield, X. O. (2012). Role of exosomes/microvesicles in the nervous system and use in emerging therapies. Front. Physiol. 3:228. doi: 10.3389/fphys.2012.00228
Lane, R. E., Korbie, D., Anderson, W., Vaidyanathan, R., and Trau, M. (2015). Analysis of exosome purification methods using a model liposome system and tunable-resistive pulse sensing. Sci. Rep. 5:7639.
Le Saux, S., Aarrass, H., Lai-Kee-Him, J., Bron, P., Armengaud, J., Miotello, G., et al. (2020). Post-production modifications of murine mesenchymal stem cell (mMSC) derived extracellular vesicles (EVs) and impact on their cellular interaction. Biomaterials 231:119675. doi: 10.1016/j.biomaterials.2019.119675
Lee, Y., Andaloussi, S. E., and Wood, M. J. (2012). Exosomes and microvesicles: extracellular vesicles for genetic information transfer and gene therapy. Hum. Mol. Genet. 21, R125–R134.
Lewis, J. M., Vyas, A. D., Qiu, Y., Messer, K. S., White, R., and Heller, M. J. (2018). Integrated analysis of exosomal protein biomarkers on alternating current electrokinetic chips enables rapid detection of pancreatic cancer in patient blood. ACS Nano 12, 3311–3320. doi: 10.1021/acsnano.7b08199
Li, B., Luan, S., Chen, J., Zhou, Y., Wang, T., Li, Z., et al. (2020). The MSC-derived exosomal lncRNA H19 promotes wound healing in diabetic foot ulcers by upregulating PTEN via MicroRNA-152-3p. Mol. Therapy-Nucleic Acids 19, 814–826. doi: 10.1016/j.omtn.2019.11.034
Li, X., Xie, X., Lian, W., Shi, R., Han, S., Zhang, H., et al. (2018). Exosomes from adipose-derived stem cells overexpressing Nrf2 accelerate cutaneous wound healing by promoting vascularization in a diabetic foot ulcer rat model. Exp. Mol. Med. 50, 1–14. doi: 10.1038/s12276-018-0058-5
Lin, S., Yu, Z., Chen, D., Wang, Z., Miao, J., Li, Q., et al. (2020). Progress in microfluidics-based exosome separation and detection technologies for diagnostic applications. Small (Weinheim an der Bergstrasse, Germany) 16, 1–18.
Lipsky, B. A., Berewdt, A. R., Cornia, P. B., Pile, J. C., Peters, E. Y., Armstrong, D. G., et al. (2012). Infectious diseases society of america: infectious disease society of america clinical practices guideline for the diagnosis and treatment of diabetic foot infections. Clin. Infect. Dis. 2012, e132–e173.
Lobb, R. J., Becker, M., Wen, S. W., Wong, C. S. F., Wiegmans, A. P., Leimgruber, A., et al. (2015). Optimized exosome isolation protocol for cell culture supernatant and human plasma. J. Extracell. Ves. 4:27031. doi: 10.3402/jev.v4.27031
Lung, C. W., Wu, F. L., Liao, F., Pu, F., Fan, Y., and Jan, Y. K. (2020). Emerging technologies for the prevention and management of diabetic foot ulcers. J. Tissue Viabil. 29, 61–68.
Lv, Q., Deng, J., Chen, Y., Wang, Y., Liu, B., and Liu, J. (2020). Engineered human adipose stem-cell-derived exosomes loaded with mir-21-5p to promote diabetic cutaneous wound healing. Mol. Pharm. 17, 1723–1733. doi: 10.1021/acs.molpharmaceut.0c00177
Maroto, R., Zhao, Y., Jamaluddin, M., Popov, V. L., Wang, H., Kalubowilage, M., et al. (2017). Effects of storage temperature on airway exosome integrity for diagnostic and functional analyses. J. Extracell. Ves. 6:1359478. doi: 10.1080/20013078.2017.1359478
McNamara, R. P., Caro-Vegas, C. P., Costantini, L. M., Landis, J. T., Griffith, J. D., Damania, B., et al. (2018). Large-scale, cross-flow based isolation of highly pure and endocytosis-competent extracellular vesicles. J. Extracell. Ves. 7:1541396. doi: 10.1080/20013078.2018.1541396
Mendt, M., Kamerkar, S., Sugimoto, H., Mcandrews, K. M., Wu, C. C., Gagea, M., et al. (2018). Generation and testing of clinical-grade exosomes for pancreatic cancer. JCI Insight 3, 1–22. doi: 10.1007/978-1-4939-6631-8_67-1
Menon, R., Dixon, C. L., Sheller-Miller, S., Fortunato, S. J., Saade, G. R., Palma, C., et al. (2019). Quantitative proteomics by SWATH-MS of maternal plasma exosomes determine pathways associated with term and preterm birth. Endocrinology 160, 639–650. doi: 10.1210/en.2018-00820
Momen-Heravi, F., Balaj, L., Alian, S., Mantel, P. Y., Halleck, A. E., Trachtenberg, A. J., et al. (2013). Current methods for the isolation of extracellular vesicles. Biol. Chem. 394, 1253–1262.
Monsel, A., Zhu, Y., Gennai, S., Hao, Q., Hu, S., Rouby, J.-J., et al. (2015). Therapeutic effects of human mesenchymal stem cell–derived microvesicles in severe pneumonia in mice. Am. J. RespirCrit. Care Med. 192, 324–336. doi: 10.1164/rccm.201410-1765oc
Noor, S., Raghav, A., Parwez, I., Ozair, M., and Ahmad, J. (2018). Molecular and culture based assessment of bacterial pathogens in subjects with diabetic foot ulcer. Diab. Metab. Syndr. 12, 417–421. doi: 10.1016/j.dsx.2018.03.001
Noor, S., Zubair, M., and Ahmad, J. (2015). Diabetic foot ulcer—a review on pathophysiology, classification and microbial etiology. Diab. Metab. Syndrome: Clin. Res. Rev. 9, 192–199. doi: 10.1016/j.dsx.2015.04.007
Orefice, N. S. (2020). Development of new strategies using extracellular vesicles loaded with exogenous nucleic acid. Pharmaceutics 12:705. doi: 10.3390/pharmaceutics12080705
Philipp, D., Suhr, L., Wahlers, T., Choi, Y. H., and Paunel-Görgülü, A. (2018). Preconditioning of bone marrow-derived mesenchymal stem cells highly strengthens their potential to promote IL-6-dependent M2b polarization. Stem Cell Res. Ther. 9:286.
Phinney, D. G., and Pittenger, M. F. (2017). Concise review: MSC-derived exosomes for cell-free therapy. Stem Cells 35, 851–858. doi: 10.1002/stem.2575
Pomatto, M. A. C., Bussolati, B., D’Antico, S., Ghiotto, S., Tetta, C., Brizzi, M. F., et al. (2019). Improved loading of plasma-derived extracellular vesicles to encapsulate antitumor miRNAs. Mol. Therapy: Methods Clin. Dev. 13, 1–12.
Popovic, M., Mazzega, E., Toffoletto, B., and de Marco, A. (2018). Isolation of anti-extra-cellular vesicle single-domain antibodies by direct panning on vesicle-enriched fractions. Microb Cell Factories 17:6.
Raghav, A., Khan, Z. A., Labala, R. K., Ahmad, J., Noor, S., and Mishra, B. K. (2018). Financial burden of diabetic foot ulcers to world: a progressive topic to discuss always. Therapeutic Adv. Endocrinol. Metab. 9, 29–31. doi: 10.1177/2042018817744513
Raghav, A., Khan, Z. A., Upadhayay, V. K., Tripathi, P., Gautam, K. A., Mishra, B. K., et al. (2021). Mesenchymal stem cell-derived exosomes exhibit promising potential for treating SARS-CoV-2-Infected patients. Cells 10:587. doi: 10.3390/cells10030587
Richard, J. L., Lavigne, J. P., and Sotto, A. (2012). Diabetes and foot infection: more than double trouble. Diab. Metab. Res. Rev. 28, 46–53. doi: 10.1002/dmrr.2234
Rivoltini, L., Chiodoni, C., Squarcina, P., Tortoreto, M., Villa, A., Vergani, B., et al. (2016). TNF-Related apoptosis-inducing ligand (TRAIL)-Armed exosomes deliver proapoptotic signals to tumor site. Clin. Cancer Res. 22, 3499–3512. doi: 10.1158/1078-0432.ccr-15-2170
Salarpour, S., Forootanfar, H., Pournamdari, M., Ahmadi-Zeidabadi, M., Esmaeeli, M., and Pardakhty, A. (2019). Paclitaxel incorporated exosomes derived from glioblastoma cells: comparative study of two loading techniques. Daru 27, 533–539. doi: 10.1007/s40199-019-00280-5
Sato, Y. T., Umezaki, K., Sawada, S., Mukai, S., Sasaki, Y., Harada, N., et al. (2016). Engineering hybrid exosomes by membrane fusion with liposomes. Sci. Rep. 6:21933.
Semreen, M. H., Alniss, H. Y., Mousa, M. K., El-Awady, R., Khan, F., and Al-Rub, K. A. (2018). Quantitative determination of doxorubicin in the exosomes of A549/MCF-7 cancer cells and human plasma using ultra performance liquid chromatography-tandem mass spectrometry. Saudi Pharm. J. 26, 1027–1034. doi: 10.1016/j.jsps.2018.05.011
Shen, Y. I., Cho, H., Papa, A. E., Burke, J. A., Chan, X. Y., Duh, E. J., et al. (2016). Engineered human vascularized constructs accelerate diabetic wound healing. Biomaterials 102, 107–119. doi: 10.1016/j.biomaterials.2016.06.009
Shiau, A. L., and Wu, C. L. (1998). The inhibitory effect of Staphylococcus epidermidis slime on the phagocytosis of murine peritoneal macrophages is interferon-independent. Microbiol. Immunol. 42, 33–40. doi: 10.1111/j.1348-0421.1998.tb01966.x
Soares Martins, T., Catita, J., Martins Rosa, I., da Cruz, E., Silva, O. A. B., and Henriques, A. G. (2018). Exosome isolation from distinct biofluids using precipitation and column-based approaches. PLoS One 13:e0198820. doi: 10.1371/journal.pone.0198820
Subra, C., Grand, D., Laulagnier, K., Stella, A., Lambeau, G., Paillasse, M., et al. (2010). Exosomes account for vesicle-mediated transcellular transport of activatable phospholipases and prostaglandins. J. Lipid Res. 51, 2105–2120. doi: 10.1194/jlr.m003657
Sun, D., Zhuang, X., Xiang, X., Liu, Y., Zhang, S., Liu, C., et al. (2010). A novel nanoparticle drug delivery system: the anti-inflammatory activity of curcumin is enhanced when encapsulated in exosomes. Mol. Ther. 18, 1606–1614. doi: 10.1038/mt.2010.105
Tan, S. H. S., Wong, J. R. Y., Sim, S. J. Y., Tjio, C. K. E., Wong, K. L., Chew, J. R. J., et al. (2020). Mesenchymal stem cell exosomes in bone regenerative strategies-a systematic review of preclinical studies. Mater. Today Biol. 7:100067. doi: 10.1016/j.mtbio.2020.100067
Tang, Y., Zhou, Y., and Li, H. J. (2021). Advances in mesenchymal stem cell exosomes: a review. Stem Cell Res. Ther. 12:71.
Tauro, B. J., Greening, D. W., Mathias, R. A., Ji, H., Mathivanan, S., Scott, A. M., et al. (2012). Comparison of ultracentrifugation, density gradient separation, and immunoaffinity capture methods for isolating human colon cancer cell line LIM1863-derived exosomes. Methods 56, 293–304. doi: 10.1016/j.ymeth.2012.01.002
Than, U. T. T., Guanzon, D., Leavesley, D., and Parker, T. (2017). Association of extracellular membrane vesicles with cutaneous wound healing. Int. J. Mol. Sci. 18:956. doi: 10.3390/ijms18050956
Tian, Y., Li, S., Song, J., Ji, T., Zhu, M., Anderson, G. J., et al. (2014). A doxorubicin delivery platform using engineered natural membrane vesicle exosomes for targeted tumor therapy. Biomaterials 35, 2383–2390. doi: 10.1016/j.biomaterials.2013.11.083
Van der Meel, R., Fens, M. H., Vader, P., van Solinge, W. W., Eniola-Adefeso, O., and Schiffelers, R. M. (2014). Extracellular vesicles as drug delivery systems: lessons from the liposome field. J. Control Release 195, 72–85. doi: 10.1016/j.jconrel.2014.07.049
Vergauwen, G., Dhondt, B., Van Deun, J., De Smedt, E., Berx, G., Timmerman, E., et al. (2017). Confounding factors of ultrafiltration and protein analysis in extracellular vesicle research. Sci. Rep. 7:2704.
Vohra, M., Sharma, A., Bagga, R., and Arora, S. K. (2020). Human umbilical cord-derived mesenchymal stem cells induce tissue repair and regeneration in collagen-induced arthritis in rats. J. Clin. Transl. Res. 6, 203–216.
Wahlgren, J., De, L., Karlson, T., Brisslert, M., Vaziri Sani, F., Telemo, E., et al. (2012). Plasma exosomes can deliver exogenous short interfering RNA to monocytes and lymphocytes. Nucleic Acids Res. 40:e130. doi: 10.1093/nar/gks463
Wang, C., Wang, M., Xu, T., Zhang, X., Lin, C., Gao, W., et al. (2019). Engineering bioactive self-healing antibacterial exosomes hydrogel for promoting chronic diabetic wound healing and complete skin regeneration. Theranostics 9, 65–76. doi: 10.7150/thno.29766
Wei, H., Chen, J., Wang, S., Fu, F., Zhu, X., Wu, C., et al. (2019). A nanodrug consisting of doxorubicin and exosome derived from mesenchymal stem cells for osteosarcoma treatment in vitro. Int. J. Nanomed. 14, 8603–8610. doi: 10.2147/ijn.s218988
Wei, H., Chen, Q., Lin, L., Sha, C., Li, T., Liu, Y., et al. (2021). Regulation of exosome production and cargo sorting. Int. J. Biol. Sci. 17, 163–177. doi: 10.7150/ijbs.53671
Witwer, K. W., Buzás, E. I., Bemis, L. T., Bora, A., Lässer, C., Lötvall, J., et al. (2013). Standardization of sample collection, isolation and analysis methods in extracellular vesicle research. J. Extracell. Ves. 2:20360. doi: 10.3402/jev.v2i0.20360
Wu, M., Ouyang, Y., Wang, Z., Zhang, R., Huang, P. H., Chen, C., et al. (2017). Isolation of exosomes from whole blood by integrating acoustics and microfluidics. Proc. Natl. Acad. Sci. U S A. 114, 10584–10589. doi: 10.1073/pnas.1709210114
Xin, H., Li, Y., Buller, B., Katakowski, M., Zhang, Y., Wang, X., et al. (2012). Exosome-mediated transfer of miR-133b from multipotent mesenchymal stromal cells to neural cells contributes to neurite outgrowth. Stem Cells 30, 1556–1564. doi: 10.1002/stem.1129
Yan, Z., Dutta, S., Liu, Z., Yu, X., Mesgarzadeh, N., Ji, F., et al. (2019). A label-free platform for identification of exosomes from different sources. ACS Sens. 4, 488–497. doi: 10.1021/acssensors.8b01564
Yang, F., Liao, X., Tian, Y., and Li, G. (2017). Exosome separation using microfluidic systems: size-based, immunoaffinity-based and dynamic methodologies. Biotechnol. J. 12, 1–8.
Yang, K., Li, D., Wang, M., Xu, Z., Chen, X., Liu, Q., et al. (2019). Exposure to blue light stimulates the proangiogenic capability of exosomes derived from human umbilical cord mesenchymal stem cells. Stem Cell Res. Ther. 10:358.
Yang, T., Martin, P., Fogarty, B., Brown, A., Schurman, K., Phipps, R., et al. (2015). Exosome delivered anticancer drugs across the blood-brain barrier for brain cancer therapy in Danio rerio. Pharm. Res. 32, 2003–2014. doi: 10.1007/s11095-014-1593-y
Yu, M., Liu, W., Li, J., Lu, J., Lu, H., Jia, W., et al. (2020). Exosomes derived from atorvastatin-pretreated MSC accelerate diabetic wound repair by enhancing angiogenesis via AKT/eNOS pathway. Stem Cell Res. Ther. 11:350.
Zhang, Y., Liu, Y., Liu, H., and Tang, W. H. (2019). Exosomes: biogenesis, biologic function and clinical potential. Cell Biosci. 9:19.
Zhao, L., Yu, J., Wang, J., Li, H., Che, J., and Cao, B. (2017). Isolation and identification of miRNAs in exosomes derived from serum of colon cancer patients. J. Cancer 8, 1145–1152. doi: 10.7150/jca.18026
Zhu, X., Badawi, M., Pomeroy, S., Sutaria, D. S., Xie, Z., Baek, A., et al. (2017). Comprehensive toxicity and immunogenicity studies reveal minimal effects in mice following sustained dosing of extracellular vesicles derived from HEK293T cells. J. Extracell. Ves. 6:1324730. doi: 10.1080/20013078.2017.1324730
Zhu, Y.-G., Feng, X.-M., Abbott, J., Fang, X.-H., Hao, Q., Monsel, A., et al. (2014). Human mesenchymal stem cell microvesicles for treatment of Escherichia coli endotoxin-induced acute lung injury in mice. Stem Cells 32, 116–125. doi: 10.1002/stem.1504
Keywords: exosomes, diabetes foot ulcers, diabetes mellitus, customized exosomes, bacterial infection
Citation: Raghav A, Tripathi P, Mishra BK, Jeong G-B, Banday S, Gautam KA, Mateen QN, Singh P, Singh M, Singla A and Ahmad J (2021) Mesenchymal Stromal Cell-Derived Tailored Exosomes Treat Bacteria-Associated Diabetes Foot Ulcers: A Customized Approach From Bench to Bed. Front. Microbiol. 12:712588. doi: 10.3389/fmicb.2021.712588
Received: 20 May 2021; Accepted: 16 June 2021;
Published: 27 July 2021.
Edited by:
Amin Tamadon, Bushehr University of Medical Sciences, IranReviewed by:
Mohammad Zubair, University of Tabuk, Saudi ArabiaCopyright © 2021 Raghav, Tripathi, Mishra, Jeong, Banday, Gautam, Mateen, Singh, Singh, Singla and Ahmad. This is an open-access article distributed under the terms of the Creative Commons Attribution License (CC BY). The use, distribution or reproduction in other forums is permitted, provided the original author(s) and the copyright owner(s) are credited and that the original publication in this journal is cited, in accordance with accepted academic practice. No use, distribution or reproduction is permitted which does not comply with these terms.
*Correspondence: Alok Raghav, YWxva2FsaWdAZ21haWwuY29t
†These authors have contributed equally to this work
‡Present address: Prashant Tripathi, COVID-19 Testing Laboratory, GSVM Medical College, Kanpur, India; Prem Singh, Multidisciplinary Research Unit, GSVM Medical College, Kanpur, India
Disclaimer: All claims expressed in this article are solely those of the authors and do not necessarily represent those of their affiliated organizations, or those of the publisher, the editors and the reviewers. Any product that may be evaluated in this article or claim that may be made by its manufacturer is not guaranteed or endorsed by the publisher.
Research integrity at Frontiers
Learn more about the work of our research integrity team to safeguard the quality of each article we publish.