- 1College of Animal Science and Technology, Hunan Agriculture University, Changsha, China
- 2Hunan Institute of Animal and Veterinary Science, Changsha, China
- 3Institute of Bast Fiber Crops, Chinese Academy of Agricultural Sciences, Changsha, China
- 4Shandong Lonct Enzymes Co., Ltd., Linyi, China
- 5Animal Husbandry and Fisheries Affairs Center, Huaihua, China
- 6Hunan Perfly Biotech Co., Ltd., Changsha, China
The present study investigated the effects of ferulic acid (FA) on the growth performance, serum cytokine profile, intestinal morphology, and intestinal microbiota in ducks at the growing stage. 300 female Linwu ducks at 28 days of age with similar body weights were randomly divided into five groups. Each group contained six replicates of 10 birds. The dietary treatments were corn-soybean-based diet supplemented with FA at the concentrations of 0 (control), 100, 200, 400, and 800 mg/kg diet. The results demonstrated that dietary FA at the levels of 200, 400, and 800 mg/kg increased the average daily gain (P = 0.01), 400 and 800 mg/kg FA increased the final body weight (P = 0.02), 100, 200, and 800 mg/kg FA increased the serum glutathione (P = 0.01), and 100, 400, and 800 mg/kg FA increased the glutathione peroxidase activities in birds (P < 0.01). Additionally, 200, 400, and 800 mg/kg dietary FA lowered the serum levels of interleukin-2 (P = 0.02) and interleukin-6 (P = 0.04). Moreover, the morphometric study of the intestines indicated that 400 mg/kg FA decreased the crypt depth in jejunum (P = 0.01) and caecum (P = 0.04), and increased the ratio of villus height to crypt depth in jejunum (P = 0.02). Significant linear and/or quadratic relationships were found between FA concentration and the measured parameters. 16S rRNA sequencing revealed that dietary FA increased the populations of genera Faecalibacterium, Paludicola, RF39, and Faecalicoccus in the cecum (P < 0.05), whereas decreased the populations of Anaerofilum and UCG-002 (P < 0.05). The Spearman correlation analysis indicated that phylum Proteobacteria were negatively, but order Oscillospirales, and family Ruminococcaceae were positively related to the parameters of the growth performance. Phylum Bacteroidetes, class Negativicutes and family Rikenellaceae were negatively associated with the parameters of the antioxidative capability. And phylum Cyanobacteria, Elusimicrobia, and Bacteroidetes, class Bacilli, family Rikenellaceae, and genus Prevotella were positively associated with the parameters of the immunological capability. Thus, it was concluded that the supplementations of 400 mg/kg FA in diet was able to improve the growth performance, antioxidative and immunological capabilities, intestinal morphology, and modulated the gut microbial construction of Linwu ducks at the growing stage.
Introduction
The intensive rearing system for poultry was widespread throughout the world. Better quantity and quality of poultry products were expected by breeding birds in indoor system with advanced technologies and management skills (Robins and Phillips, 2011). The intensive rearing system satisfied the increasing demands for the poultry product, but also brought many problems to the welfare of the birds, including the physiological and physical stresses (Lolli et al., 2010; Averós and Estevez, 2018), pathogenic infections (Zhu, 2011), and disturbance in the intestinal microbial construction (Wang S. et al., 2018). These problems might break the redox homeostasis and provoked immune response (Rehman et al., 2017; Lauridsen, 2018), leading to high mortality and lower productive efficiency (Averós and Estevez, 2018). In the past, antibiotic additives in poultry feed were commonly used in the intensive rearing system because they could effectively attenuate the negative influence of stress, and enhance the growth performance of birds (Castanon, 2007). However, the abuse of antibiotics led to the development of bacterial antibiotic-resistance and created huge pressure on the medication of bacterial infections in human and animal (Hayes et al., 2004). Starting from 2006, legislative bans on antibiotics in animal feed were issued worldwide gradually.
On this background, phytochemicals with characteristics of safety, multiple bioactivities and enhancement to animal growth became ideal alternations to the antibiotic additives in animal feed (Valenzuela-Grijalva et al., 2017). Ferulic acid (FA) is an active phenolic acid widely existing in the cell walls of plants, and it is the main active substance of Chinese herbs Chuangxiong (Ligusticum chuanxiong) and Danggui (Angelica sinensis) (Ou and Kwok, 2004). Previous researchers stated that FA possessed multiple bioactivities, such as anti-bacteria (Daglia, 2012), antioxidant (Mahmoud et al., 2020), anti-inflammatory (Lampiasi and Montana, 2016), anti-obesity (Wang et al., 2015), anti-tumor (Jinhua et al., 2018), and regulation of blood circulation (Zhou et al., 2017). Currently, FA was widely used in industries such as food preservation (Ou et al., 2005), cosmetics production (Gupta et al., 2020), and medication (Wang W. M. et al., 2018). A few studies demonstrated the antioxidative and growth promotional functions of FA and its derivatives in livestock, as supplement in the feed (Li et al., 2015; González-Ríos et al., 2016; Wang et al., 2019), but none referred to ducks. In the present study, we attempted to understand the effects of FA on the growth performance, serum cytokine profiles, intestine morphology, and intestinal microbiota composition in ducks.
Materials and Methods
The experimental procedures of this study were approved by Hunan Agricultural University Institutional Animal Care and Use Committee.
Experimental Design and Diets
Linwu duck is an important indigenous breed in South China, with the characteristics of fast growth, high feed efficiency, and unique meat flavor and texture. The market age for Linwu duck is 70 days and the average body weight at that time is about 1,750 g per duck. From the age of 28–54 days was the maximum growing stage for Linwu ducks, when they suffered the most by the immune and oxidative stresses. Therefore, Linwu ducks at 28 days of age were chosen for this study. A total of 300 female ducks were obtained from Hunan Shunhua Duck Industrial Development Company (Linwu, China) and housed in plastic plain netting cages with the dimension of 1.8 m × 1.2 m × 2 m (10 ducks/cage). Ducks were accessed to the water and feed freely. Birds were randomly divided into five groups with six replicates per group. And each replicate had 10 birds. The whole experiment period was 29 days.
Birds in group 1 were fed the basal diets, and in group 2–5 were fed basal diets supplemented with 100, 200, 400, and 800 mg/kg FA, respectively. The basal diet, which meets the nutritional requirements for growing ducks (National Research Council, 1994), was given in Table 1. FA (≥99%) was purchased from Shanghai Rhawn Chemical Technology Co., Ltd. (Shanghai, China).
Growth Performance
The birds were fasted for 12 h before weighting and sampling. On 29th day of the experiment, birds were weighted, and the total feed consumptions were summed up by cages (replicates). Average daily weight gain (ADG), average daily feed intake (ADFI) and ratio of feed to gain (F/G) were calculated for the whole experimental period.
Sample Collections
After weighting, one bird from each cage (6 birds per group) was randomly selected for sampling. Bloods were collected from the wing vein with vacuum blood collection tubes, and centrifuged at 3,000 × g for 10 min to obtain the serums. The birds were then sacrificed by bleeding in jugular vein and dissected for the duodenum, jejunal, ileal, and cecal tissue samples. Chyme in the cecum of each sampled duck were collected separately into 2 mL EP tubes, froze immediately in liquid nitrogen and stored at −80°C until analysis.
Serum Antioxidant and Inflammatory Biomarkers
The serum levels of antioxidant biomarkers including reduced glutathione (GSH), malonaldehyde (MDA), activities of superoxide dismutase (SOD) and glutathione peroxidase (GSH-Px), as well as the inflammatory biomarkers, such as immunoglobulin G (IgG), interlukin-1β (IL-1β), interlukin-6 (IL-6), and interleukin-2 (IL-2) were determined by the commercial assay kits (Nanjing Jiancheng Bioengineering Institute, Nanjing, China) with an automated fluorescence instrument (MultiskanTM SkyHigh, Thermo Fisher Scientific, Waltham, MA, United States).
Intestinal Morphology
The intestinal sections, including duodenum, jejunum, ileum, and cecum, were removed and emptied. 2 cm sections of intestinal tissues were collected and embedded in paraffin. A microtome (RM-2235, Leica microsystems AG, Hessen, Germany) was used to make 5 μm slices of the tissue samples, which were subsequently stained with hematoxylin and eosin. The slides were observed under a microscope (Olympus Van-Ox S, Opelco, Washington, DC, United States) and the proper microscopic fields were selected for the following diagnoses. Five readings of the villus height, crypt depth, intestinal wall thickness and mucosal thickness from each slide were determined by an image analysis system (Image-Pro, Media Cybernetics, Inc., Silver Springs, MD, United States). The ratios of villus height to crypt depth (VCR) were calculated as well.
Gut Microbiota Composition by 16S rRNA Gene Sequencing
Microbial community genomic DNA was extracted from cecum digesta samples using the E.Z.N.A. soil DNA kits (Omega Bio-tech, Norcross, GA, United States) according to the manufacturer’s instructions. The V3-V4 regions of the bacterial 16S rRNA gene were amplified using primer pairs 338F (5′-ACTCCTACGGGAGGCAGCAG-3′) and 806R (5′-GGACTACHVGGGTWTCTAAT-3′), and later purified using AxyPrep DNA Gel Extraction Kits (Axygen Bioscience, Union City, CA, United States). The purified amplicons were paired-end sequenced on an Illumina MiSeq PE300 platform (Illumina, San Diego, CA, United States). The raw reads in this study were uploaded to the National Center of Biotechnology Information (NCBI) Sequence Read Archive (SRA) database under accession number PRJNA723283.
Reads with length over 300 bp were kept for the following analysis. QIIME (version 1.17) software was used to filter the obtained sequences from the samples and the high-quality sequences were clustered into operational taxonomic units (OTUs) with a cut off of 97% similarity using UPARSE (version 7.1). Each OTU represented sequence was analyzed by Ramer–Douglas–Peucker (RDP) Classifier version 2.2 against the 16S rRND database.
The alpha and beta diversities of the cecal microbiota were estimated at genera level. Principal coordinate analysis (PCA) with analysis of similarities (ANOSIM) was carried out to determine the beta diversity of the bacteria. Statistical comparison of the relative abundance of the cecal microbiota was performed using one-way ANOVA to identify the differences in bacterial taxa among all groups, or using student’s t test between two groups. The spearman correlation analysis was applied to evaluate the relationship of microbial species with the measured parameters. Correlations were considered significantly different at P ≤ 0.05. The analysis procedures of cecal microbiota were processed on the free online platform of Majorbio Cloud Platform (Majorbio Bio-pharm Technology Co., Ltd., Shanghai, China).
Statistical Analysis
Cage was taken as experimental unit, and the homogeneity of variances of the data were tested before further analysis. One-way ANOVA followed by Duncan’s multiple range test were used to test the significant mean differences among groups. Polynomial orthogonal contrasts were applied to determine linearly and quadratically responses of measured parameters to the dietary FA concentration. All data analysis was performed in SPSS statistical program (SPSS25, IM Corp., Armonk, NY, United States). A probability of P < 0.05 was considered significant.
Results
Growth Performance
The initial BW, final BW, ADG, ADFI, and F/G of experimental ducks were shown in Table 2. The birds in Group 4 and 5 exhibited significantly higher final BW (P = 0.02) and ADG (P = 0.01) than the birds in Group 1. Group 3 showed the same trend, but the differences with Group 1 were not statistically significant. The F/G ratios in Group 3, 4, and 5 were decreased compared to Group 1 without statistical significance (P = 0.05). Significant linear (P < 0.05) and quadratic relationships (P < 0.05) were observed between final BW, ADG and F/G, and dietary FA level.
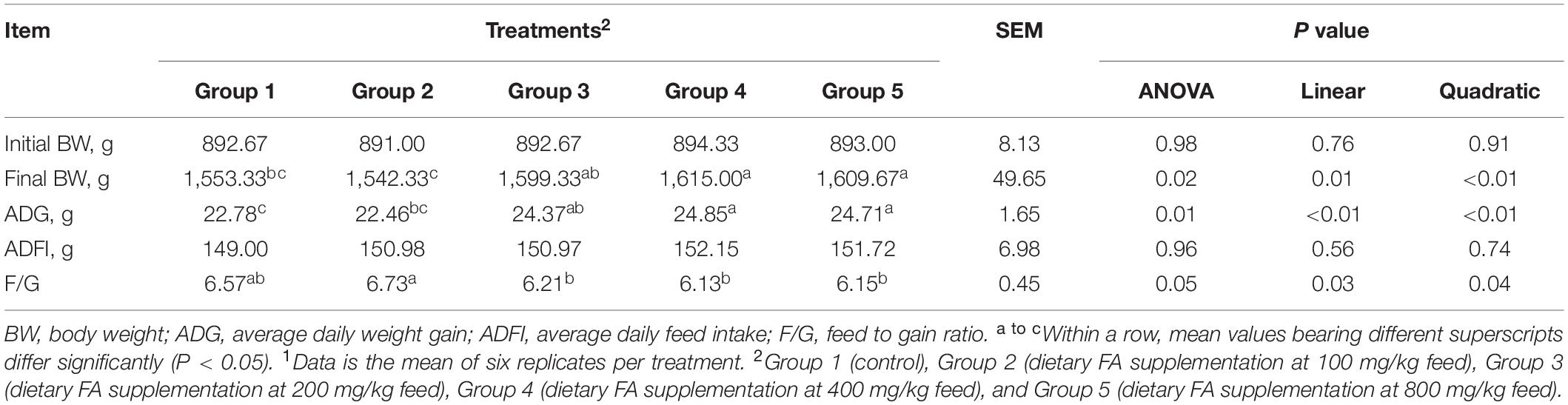
Table 2. Effect of different dietary FA supplementation on growth performance of experimental ducks1.
Serum Cytokines Profile: Oxidant and Antioxidant Status
The serum oxidant and antioxidant statuses were listed in Table 3. Serum levels of MDA (P = 0.52) and SOD activities (P = 0.22) among groups were similar, but significant differences were noticed in GSH levels (P = 0.01) and GSH-Px (P < 0.01) activities among groups. Serum levels of GSH in all groups except Group 4, and GSH-Px activities in all groups except Group 3 were significantly higher, compared to those in Group 1. Moreover, as the diet FA increased, the activities of GSH-Px increased linearly (P < 0.01) and quadratically (P < 0.01).
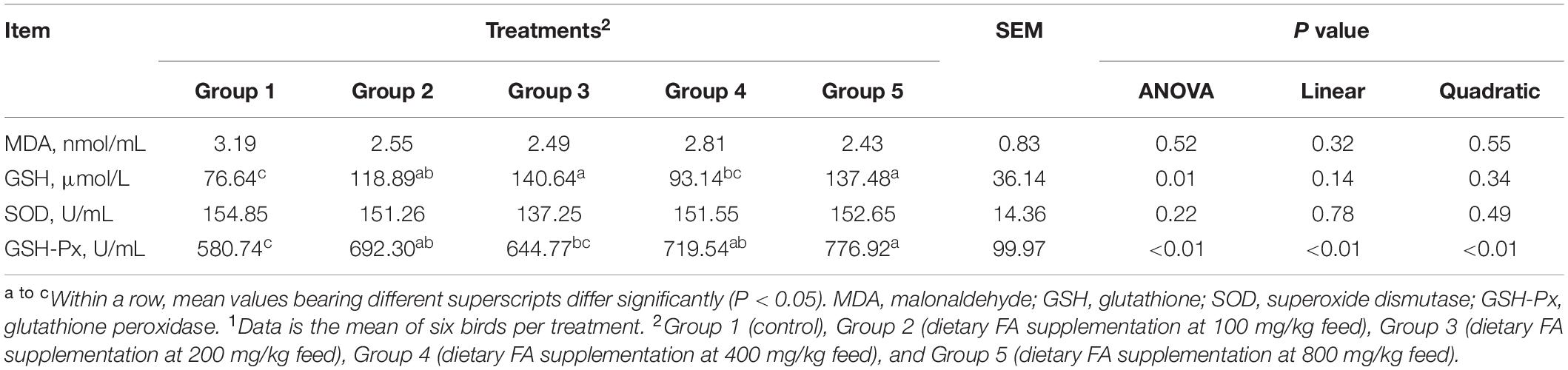
Table 3. Effect of different dietary FA supplementation on antioxidative parameters of experimental ducks1.
Serum Cytokine Profile: Immune Status
As demonstrated in Table 4, there were no significant differences in serum levels of IgG (P = 0.28) and IL-1β (P = 0.51) among groups. However, Group 3, 4, and 5 showed significant lower levels of IL-6 (P = 0.04) and IL-2 (P = 0.02) comparing to Group 1. Negative linear (P < 0.05) and quadratic (P < 0.05) relationships were found between dietary FA concentration and the IL-6, as well as the IL-2 levels.
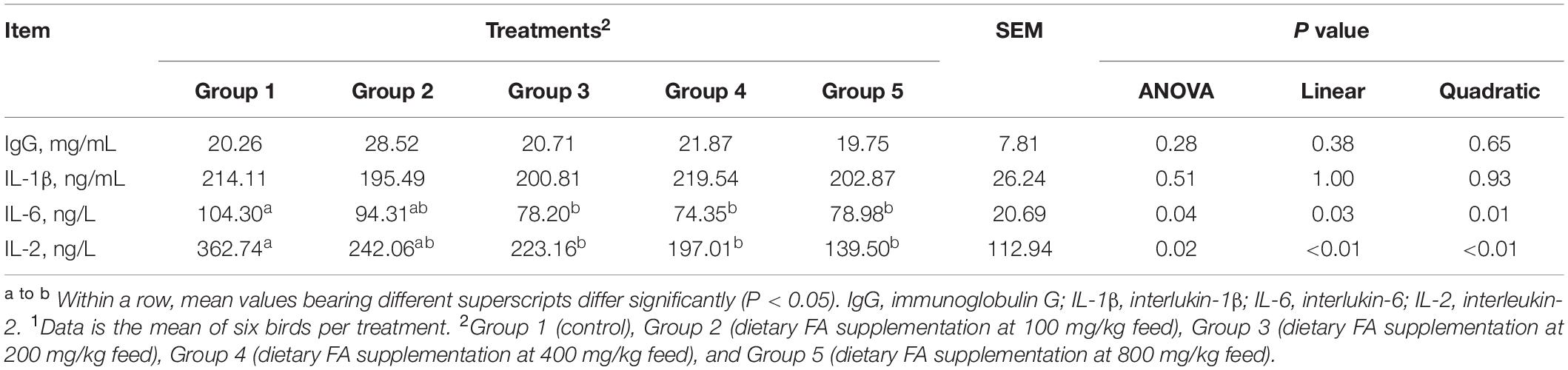
Table 4. Effect of different dietary FA supplementation on immunological parameters of experimental ducks1.
Intestinal Morphology
As shown in Table 5, compared to Group 1, Group 4 and 5 showed significantly decreased crypt depth in the jejunum (P = 0.01); Group 3, 4, and 5 showed significantly increased VCR in the jejunum (P = 0.02); and Group 4 showed significantly decreased crypt depth in the caecum (P = 0.04). Significant linear (P < 0.01) and quadratic (P < 0.01) relationships were noticed between dietary FA and the crypt depth (negative) and VCR (positive) in the jejunum; and significant quadratic but no linear relationship were found between the dietary FA and the crypt depth in the cecum (P < 0.05). In the representative images of jejunum and cecum tissues, it was noticeable that the intestinal mucosae in FA treated groups were more intact than those in Group 1 (Figure 1).
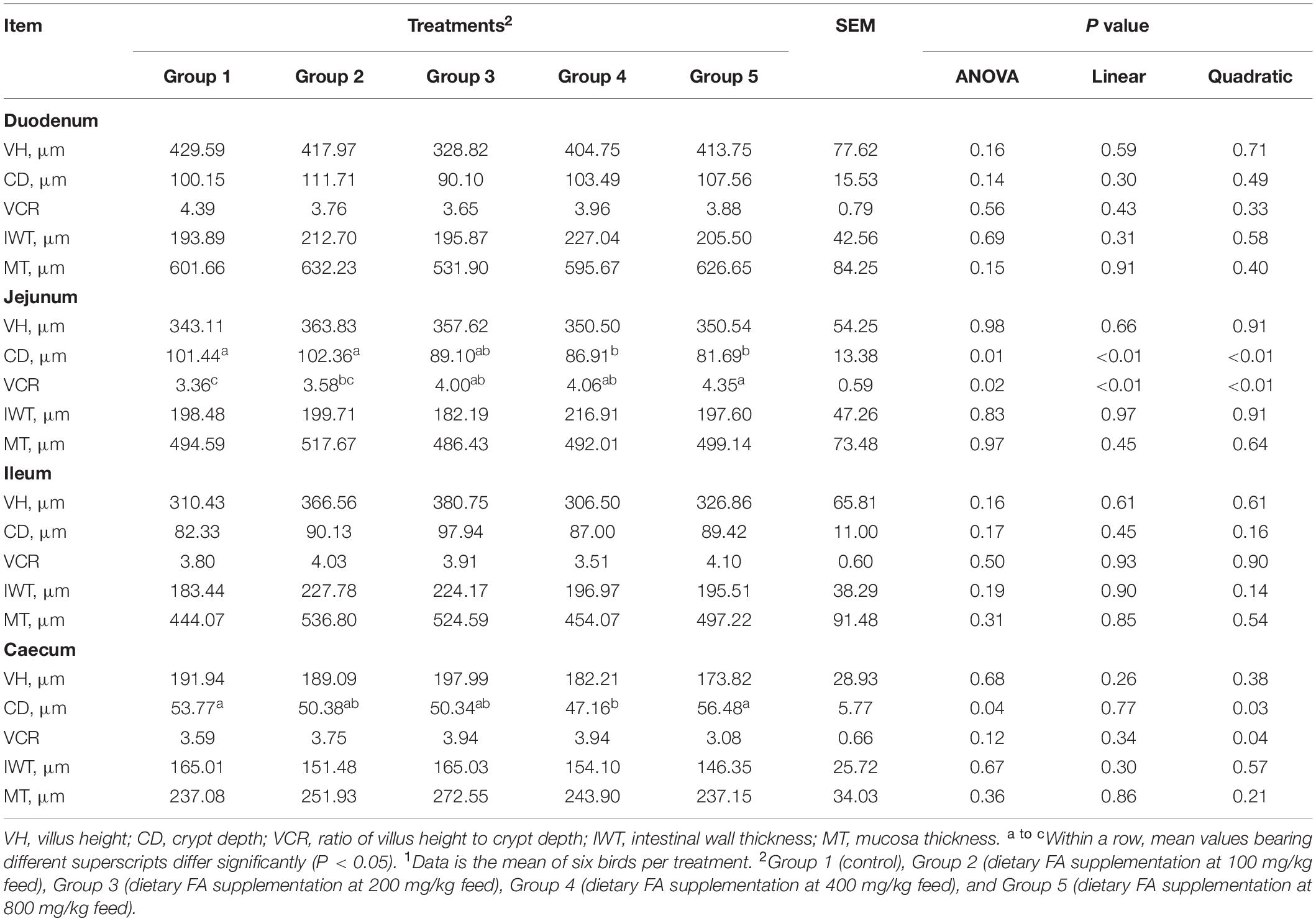
Table 5. Effect of different dietary FA supplementation on intestinal morphology of experimental ducks1.
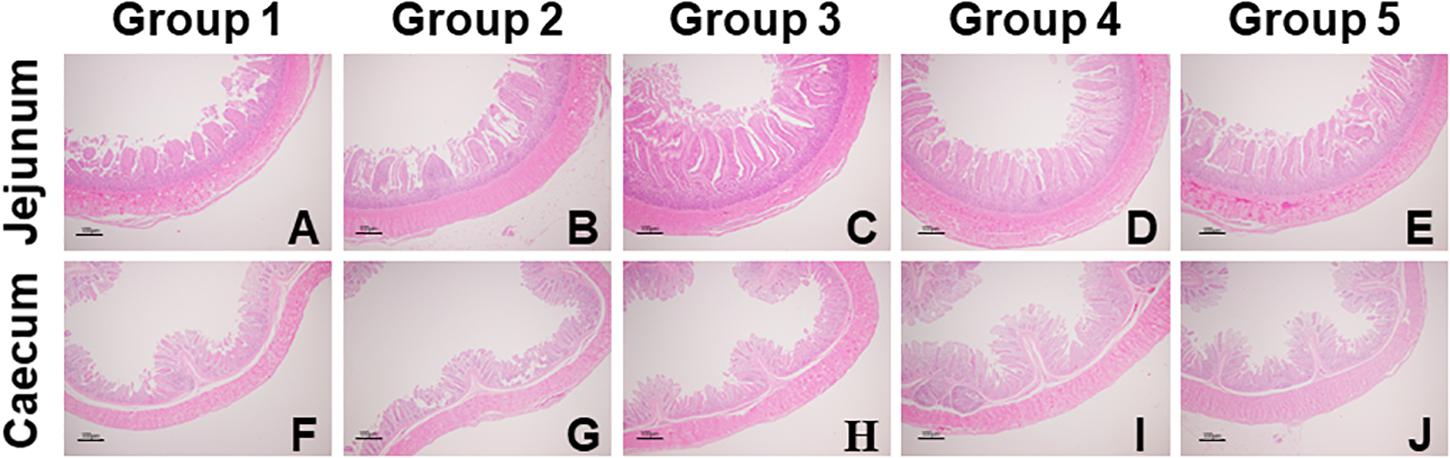
Figure 1. Representative images of jejunal (A–E) and cecal (F–J) morphology of experimental ducks in different groups (40 × magnification). Intestinal issue samples were processed with hematoxylin and eosin stain.
Modulation of Gut Microbiota
The compositions of cecal microbiota in all groups were analyzed by 16S rRNA sequencing. According to the α diversity result (Table 6), the cecal microbial abundances or diversities at the genera level were similar among groups (P > 0.05). PCA and ANOSIM further revealed no clear clustering patterns for cecal microbiota at genera level among groups (P = 0.40), as showed in Figure 2.
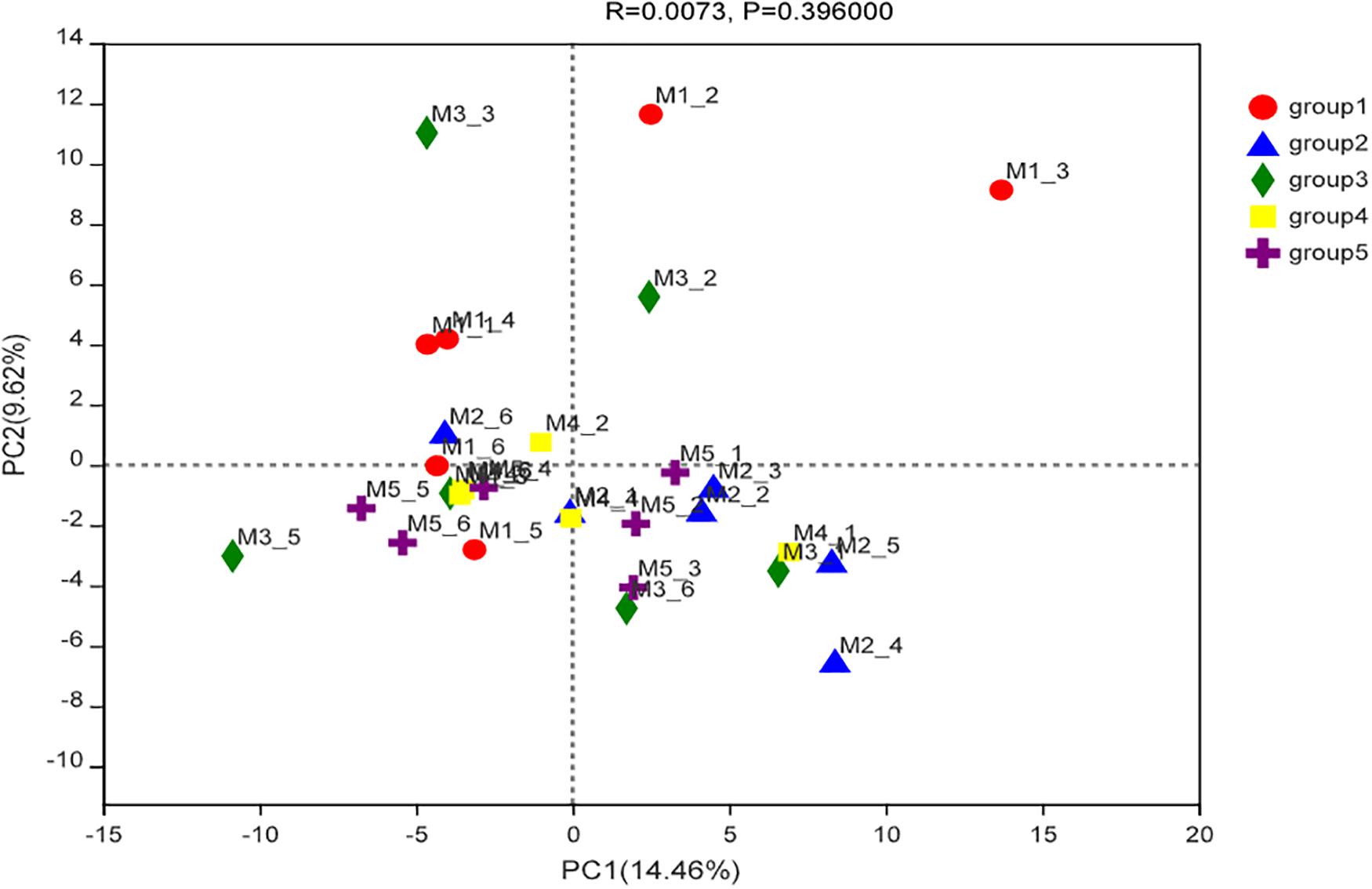
Figure 2. Principal component analysis ordinated plots of cecal microbial composition at the genera level of ducks in different groups.
The eight most predominant microbial phyla among groups were Firmicutes (43.3–48.7%), Bacteroidetes (40.5–47.8%), Spirochaetes (1.0–6.5%), Actinobacteriota (1.9–3.2%), Desulfobacterota (1.0–3.0%), Fusobacteriota (0.4–5.6%), Deferribacterota (0.1–3.7%), and Proteobacteria (0.9–1.7%) (Figure 3A). No significant differences in mean proportion were found in microbial communities at the phyla level among groups (P > 0.05). But at genera level, a total of eight microbial genera were found significantly different among (P < 0.05) groups (Figure 3B). Pair-wise comparisons with Student’s T test for the differences in microbial composition at genus level were further conducted between Group 1 and FA treated groups. Significant differences were found in proportions of Faecalibacterium, Paludicola, Anaerofilum, RF39, and Faecalicoccus between Group 1 and 2 (P < 0.05) (Figures 4A–E), Paludicola between Group 1 and 3 (P < 0.05) (Figure 4B), Faecalibacterium, RF39, and UCG-002 between Group 1 and 4 (P < 0.05) (Figures 4A,D,F), and Faecalicoccus and UCG-002 between Group 1 and 5 (P < 0.05) (Figures 4E,F).
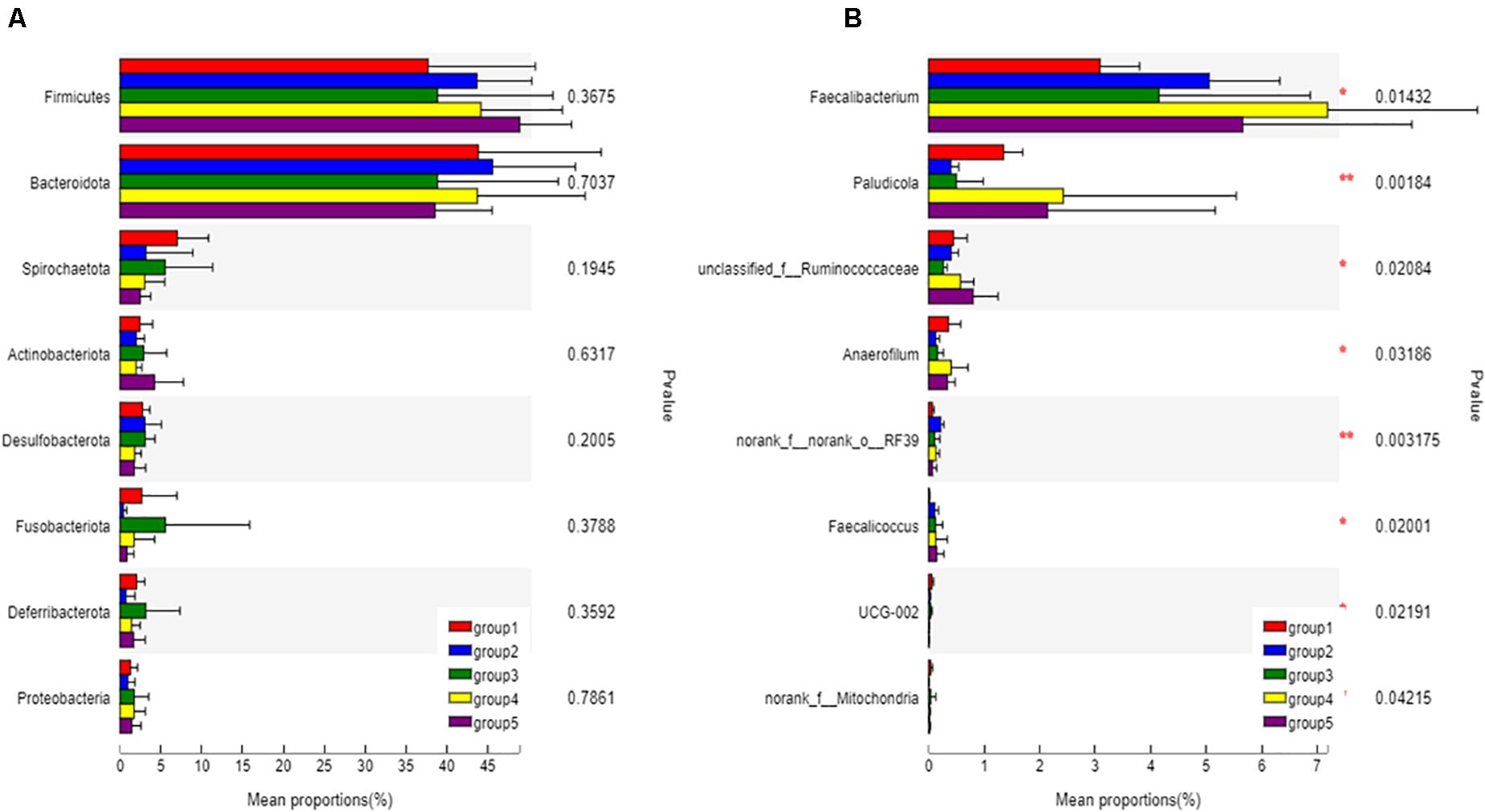
Figure 3. Classification and differences in cecal microbial composition of ducks in different groups. The eight most abundant bacteria at the phyla level (A) and bacteria with significant difference at genus level (B) among five groups were showed. Relative abundance of altered cecal microbiota levels were tested under ANOVA and Tukey post hoc analysis. Significant correlations were marked by *0.01 < P < 0.05, **0.001 < P ≤ 0.01.
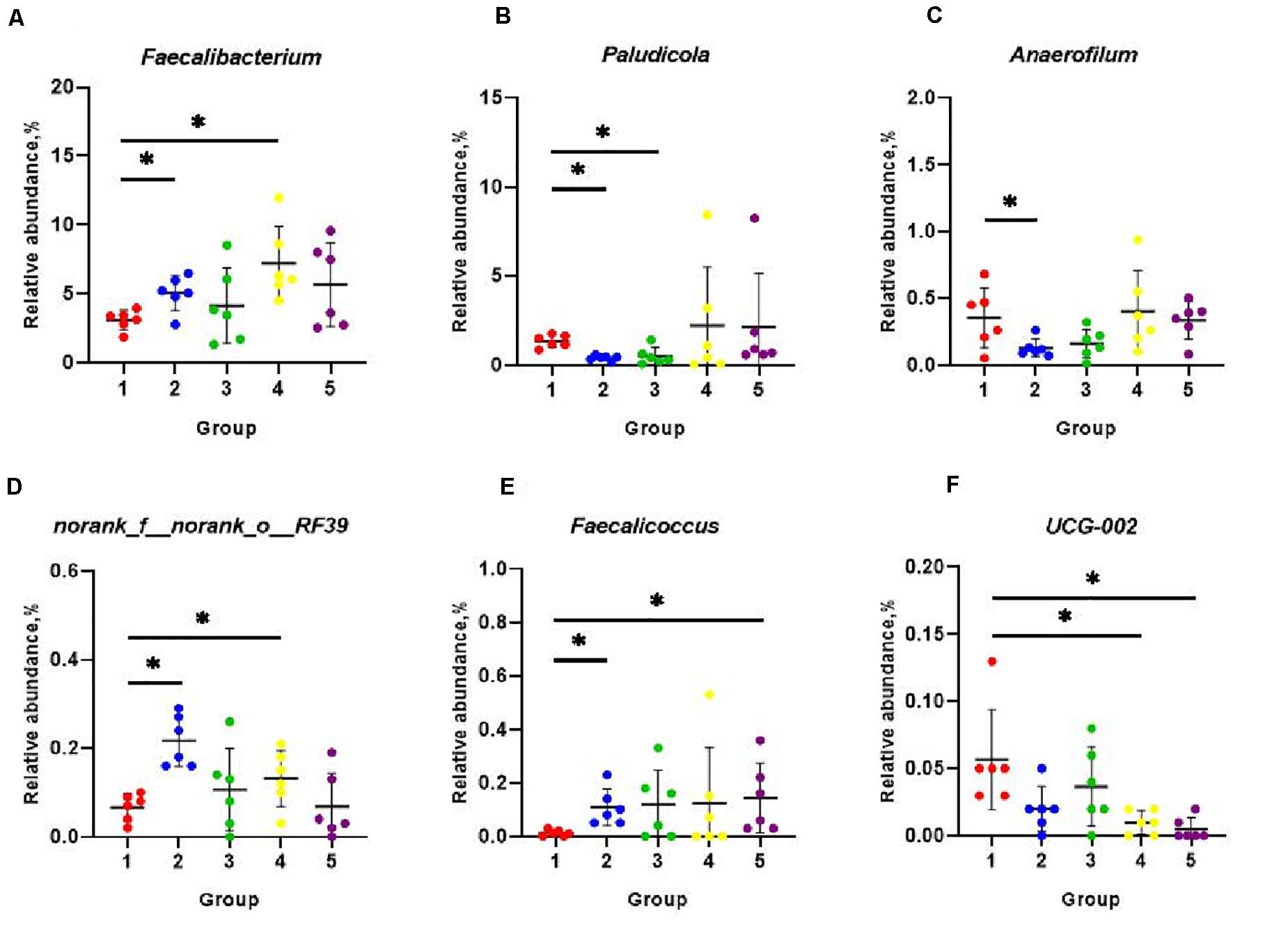
Figure 4. Classification and differences in cecal microbial composition at the genera level among five groups. The relative abundance of microbial genera that significantly altered included Faecalibacterium (A), Paludicola (B), Anaerofilum (C), norank_f_norank_o_RF39 (D), Faecalicoccus (E), and UCG-002 (F). Data were shown as means ± SD (n = 6), *P < 0.05 compared with Group 1.
The relationships between the cecal microbiota and the parameters relating to the growth performance, levels of cytokines representing the oxidative and inflammatory statuses were examined via Spearman’s correlation analysis. Data were showed in Figure 5. ADG was positively associated with the phylum Proteobacteria (P = 0.035), order Oscillospirales (P = 0.018), and family Ruminococcaceae (P = 0.006); and F/G was negatively associated with the phylum Proteobacteria (P = 0.002). Additionally, GSH level was negatively associated with the class Negativicutes (P = 0.036); and GSH-Px activity was negatively associated with the phylum Bacteroidetes (P = 0.013) and family Rikenellaceae (P = 0.003). Finally, IgG level was positively associated with the class Bacilli (P = 0.049); IL-1β level was positively associated with the phylum Cyanobacteria (P = 0.050); IL-2 level was positively associated with the phylum Elusimicrobia (P = 0.032); and IL-6 level was positively associated with phylum Bacteroidetes (P = 0.012), family Rikenellaceae (P = 0.013), and genus Prevotella (P = 0.017).
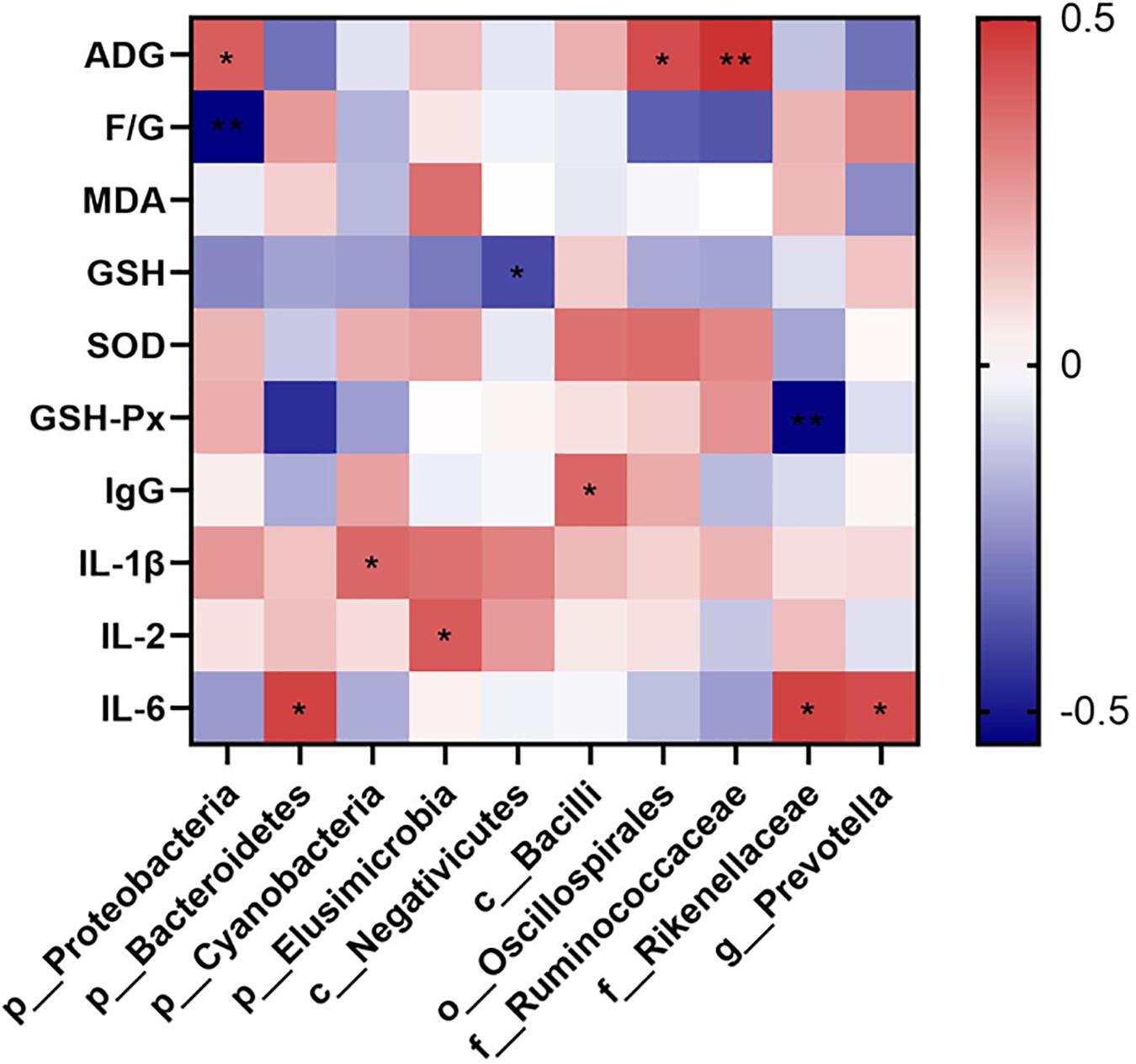
Figure 5. Spearman correlation heatmap of cecal microbiota and the measured parameters. The intensity of the colors demonstrated the degree of association (blue, negative correlation; red, positive correlation). Significant correlations marked by *0.01 < P ≤ 0.05, **0.001 < P ≤ 0.01. ADG, average daily weight gain; F/G, ratio of feed intake to weight gain; MDA, malonaldehyde; GSH, glutathione; SOD, superoxide dismutase; GSH-Px, glutathione peroxidase; IgG, immunoglobulin G; IL-1β, interlukin-1β; IL-6, interlukin-6; IL-2, interleukin-2.
Discussion
Stresses were the main factors that deteriorated the profits in poultry industry as the intensive system developing (Vizzier Thaxton et al., 2016). FA was reported to be able to ameliorate the oxidative stress by capturing reactive oxygen species (ROS) with its structure characteristics (Maurya and Devasagayam, 2010), and triggering the productions of the antioxidant enzymes through Nrf2-Keap1-ARE signaling pathway (Krajka-Kuźniak et al., 2015). Additionally, it was reported that FA could mediated the inflammation through IKK/NF-κB signaling pathway (Lampiasi and Montana, 2016). Some researchers utilized FA as feed additive in livestock farming and proved its beneficial effects. Wang et al. (2020) found that 0.05 and 0.45% FA supplemented in the diet improved the lipid profile and antioxidant capacity of the weaning piglets. González-Ríos et al. (2016) reported that steers fed diet with 6 mg/kg FA supplementation in the last 30 days of the finishing phase acquired better meat quality, and delayed lipid oxidation during storage. However, few papers were published relating to the effects of FA on poultry. In the present study, significant increase in ADG, and decrease in F/G ratio in ducks that fed diets supplemented with 200–800 mg/kg FA were detected during the growing stage (28–56 days of age). And the trends of changes were linear and quadratic related to the FA supplement rate. These results suggested promotional effects of FA on the growth performance of the ducks. As the writer’s knowledge, this study is the first time using pure FA as feed additive in poultry experiment. Previous researchers tried diets supplemented with propolis, sorghum or other materials, of which FA was considered as the main substances, with birds. Positive results were found with propolis that they improved the feed efficiency and ameliorated the effects of stresses on the weight gain (Seven, 2008; Hassan and Abdulla, 2011; Abdel-Rahman, 2013), which corroborated with our finding. However, birds offered sorghum-based diets showed decreased body weight gains (Truong et al., 2016). The variations in the effects of FA enriched materials on bird’s growth performance could partly be explained by the condensed tannins, other than the FA in the sorghum, negatively influenced energy utilization of starch, so that the weight gain of the birds decreased. It was also reported that FA in conjugated forms in plants might form complexes with debranched starch which caused the resistance to digestion (Hung et al., 2012).
In the present study, serum parameters related to the avian antioxidative status were investigated. Oxidative stress was mainly caused by the excessive accumulations of the ROS. In the organism, redox balance was modulated by the antioxidant defense system, consisting of enzymatic components such as SOD and GSH-Px, and non-enzymatic components such as GSH (Surai et al., 2019). Once the accumulation of ROS overpassed the scavenging capability of antioxidant defense system, the redox balance would break and the oxidative stress occurred (Nisar et al., 2013). MDA was formed by ROS degrading the polyunsaturated lipids in the organism, and was considered as a common biomarker for oxidative stress (Fu et al., 2013). The present study revealed the antioxidant effects of dietary FA, as it significantly improved the serum GSH levels and GSH-Px activities. Significant linear and quadratic relationships were noticed between the activities of GSH-Px and the rate of dietary FA. Similar results were found in multiple studies. The hepatic GSH contents and activities of SOD, catalase (CAT) and GSH-Px in methotrexate (MTX) induced rats were significantly alleviated after 15 days of oral supplements of 25 or 50 mg/kg FA (Mahmoud et al., 2020). It was also published that diets with 0.45% FA supplementation decreased the MDA contents, and increased the activities of CAT, total SOD and GSH-Px in the serums and the livers of the weaning pigs (Wang et al., 2020). Moreover, Wang et al. (2019) reported that 3-m-old lambs fed diet supplemented with 80 mg/kg FA showed higher plasma levels of GSH-Px, CAT and lower levels of MDA compared to the lambs fed basal diet.
Immunological stress was another notorious factor that deteriorated the animal performance (Lochmiller and Deerenberg, 2000). It usually occurred when animals were challenged by infectious agents, such as pathogenic organisms or environmental insults (Song et al., 2014). When immunological stress happened, both innate and adaptive systems in birds were triggered. Avian heterophils with strong phagocytic activities were firstly activated (Genovese et al., 2013). Later the basophils (Maxwell and Robertson, 1995), eosinophils (Maxwell, 1987), and dendritic cells (Ma S. et al., 2019) were involved as early modulators of inflammation and/or antigen presenters who initiated the adaptive immune responses. Lymphocyte cells in avian adaptive immune system were able to produce a diversity of antibodies, such as immunoglobulin M (IgM), IgG, and IgA, which served as the first line to fight against the intruders (Berghof et al., 2018). Simultaneously, the production of cytokines, such as IL-1β, IL-6, IL-2, were increased as chemical messengers that affected the proliferation, differentiation and activity of immune cells (Kaiser et al., 2005). In the present study, the addition of FA in diets significantly decreased the serum levels of IL-6 and IL-2, which outlined that the immunological status in the organism was ameliorated. Additionally, significant linear and quadratic relationships were noticed between the concentrations of dietary FA and the levels of the two cytokines. These results were partially consistent with previous findings in rats (Sadar et al., 2016; Mahmoud et al., 2020) and in vitro cell model (Cao et al., 2015), as FA attenuated not only the level of IL-6 but also IL-1β in the pathogenic circumstances.
Gastrointestinal tract (GIT) was essential for the growth of animals, and its integrity was a key factor for preventing pathogenic microorganism invasion and utilizing the nutrients (Choct, 2009). Villus height reflected the intestinal absorptive capacities of the nutrients and the crypt depth represented the colonization rate and the maturities of the crypt cells (Feng et al., 2017). The ratio of these two parameters was regarded as a general indicator for the intestinal function. Additionally, intestinal wall and mucosa were the foundations to GIT’s barrier function, of which the thickness was extremely critical. In our study, 200–800 mg/kg dietary FA supplementation linearly and quadratically decreased the crypt depth and increased the VCR of the jejunum, and 100–400 mg/kg dietary FA quadratically decreased the crypt depth of the caecum, demonstrating enhancing effects of dietary FA on the intestinal morphology in the ducks. This result was supported by previous research that 50 mg FA/kg body weight attenuated the effects of heat stress on rats’ intestinal permeability and microvilli structure (He et al., 2016). It was further reported that the beneficial effects of FA on the intestinal morphology and barrier function were possibly contributed to the activation of Nrf2/HO-1 signaling pathway, which was associated to the process of ROS elimination as well (He et al., 2018).
Microorganism inhabiting in the GIT were involved in the digestion of food, breakdown of toxins, stimulation of immune system, exclusion of pathogens and endocrine activities. The interaction between the microorganism and GIT influenced the stability of microbial communities, the animal’s health and the feed efficiency (Apajalahti et al., 2004). Cecum was the main location for the fermentation and digestion of complex substrates, so that it possesses the longest feed retention time and most diverse microorganism (Borda-Molina et al., 2018). In order to understand the effects of FA on the gut microbial environment, the chyme in the cecum were chosen in the present study for microbial community analysis. FA was reported to modulate gut microbiota composition in transverse aortic construction mice (Liu et al., 2019) and diabetic mice model (Ma y. et al., 2019; Huang et al., 2020). In present study, the differences of richness and diversity of cecal microbial community among groups were not statistically significant. The possible explanation to the inconsistencies might be the constructional and functional differences of the GIT between birds and mammals, as well as the sensitivities of the microbiome to FA in the diverse animal species. Previous papers illustrated that cecal bacterial microbiome of birds were usually dominated by members of the phyla Bacteroidetes and Firmicutes, followed by lower abundance of Proteobacteria, Actinobacteria and others (Costa et al., 2017; She et al., 2018; Xia et al., 2019). It was consistence with the cecal microbial construction in the present study. All groups in our study showed similar patterns of cecal microbial compositions at phyla level, but the changes in relative abundance of some bacteria at genera level were detected. Pair-wise comparison between the FA treated and untreated groups further suggested that different rates of dietary FA could alter the microbial compositions in the cecum. It was well documented that the intestinal microbiome closely interacted with the host through the exchange of nutrients, modulation of gut morphology and physiology, and maintenance of immune and redox homeostasis (Oakley et al., 2014; Pan and Yu, 2014; He et al., 2019)014). In the present study, the relative abundances of Faecalibacterium and Faecalicoccus were significantly increased with 400 and 800 mg/kg FA supplement, respectively. And the UCG-002 level was decreased in these two groups. Correspondingly, the ADGs were significantly higher in Group 4 (400 mg/kg FA) and 5 (800 mg/kg FA) compared to Group 1. Literatures indicated that species in Faecalibacterium were beneficial that improved the epithelial health and promoted the metabolite productions, especially butyrate and other SCFAs (Gangadoo et al., 2018), and Faecalicoccus belonged to family Erysipelotrichaceae, which was reported to be a major butyrate producer and considered more important in poultry cecum than those in human colon (Zhou et al., 2021). UCG-002 belongs to the family Oscillospiraceae, which was reported to be negatively related to the body weight gain of geese fed fermented feed (Yan et al., 2019). These studies were consistent with what we found, showing that the trends of changes in abundance of bacteria taxa mentioned above were correlative to the improvement in growth performance. Anaerofilum were published to be positively associated with the body weight gain in broilers fed dietary vitamins (Luo et al., 2013). But in the present study, we found inconsistent data regarding to the level of Anaerofilum and the body weight gains of the birds. Paludicola were a novel genus in the family Ruminococcaceae, whose function in the gut was unknown yet (Li et al., 2017).
The Spearman correlation analysis disclosed that phylum Proteobacteria, order Oscillospirales, and family Ruminococcaceae were associated with the ADG, and phylum Proteobacteria were associated with the F/G ratio, which demonstrated the strong influences of such bacteria on the growth performance in ducks. Similar results were reported previously regarding to those bacteria. Though phylum Proteobacteria included several pathogens, they could degrade the nutrients in the diet and provide suitable environmental support for symbiotic bacteria, and eventually promoted the growth of layers (Li et al., 2020). Shi et al. (2019) stated that species in order Oscillospirales, such as Oscillospira, were butyrate producers and negatively related to the F/G ratio in broilers under heat stress. Additionally, major members in family Ruminococcaceae were short-chain fatty acids producing bacteria, and they were outstanding for improving feed efficiency and enhancing health for the host (Wong et al., 2006). In the present study, class Negativicutes was found negatively associated with the GSH levels, and phylum Bacteroidetes and family Rikenellaceae were negatively associated with the GSH-Px activities. These species might be possible positive symbols to the levels of oxidative stress of the host. It was consistent with previous studies that the relative abundances of class Negativicutes were reduced in ducks with oxidative stress caused by heat (He et al., 2019), phylum Bacteroidetes were positively related to oxidative stress in rats fed high-fat diets (Ortega-Hernández et al., 2020), and relative abundance of family Rikenellaceae decreased after polysaccharides from pollen of Chinese wolfberry, which attenuated the oxidative stress in cyclophosphamide-treated mice (Zhao et al., 2020). Moreover, we found that phylum Bacteroidetes, family Rikenllaceae and genus Prevotella were positively associated with IL-6 levels; and class Bacilli, phylum Cyanobacteria, and phylum Elusimicrobia were positively associated with IgG, IL-1β, and IL-2 levels respectively. These findings potentially indicated the positive relationships of the relative abundances of these markers to the levels of immune stress of the host. Besides the roles of representative to the oxidative stress, phylum Bacteroidetes were reported to be implicated in immune regulations including activations of inflammation and autoimmune diseases (Gibiino et al., 2018), and family Rikenellaceae were proved to be positive associated with the arise of immune response induced by Pleurotus eryngii polysaccharide in mice (Ma et al., 2017). In vitro study using human monocyte-derived dendritic cells proved that genus Prevotella exhibited capacities to induce inflammatory mediators like IL-6 (Horewicz et al., 2013). Additionally, members of family bacilli, including the Gram-negative bacilli were among the most common causative agents of infectious diseases, and triggers of the inflammation (Dandachi et al., 2019). Phylum Cyanobacteria were reported to be immune suppressing (Gemma et al., 2016), but some members in Cyanobacteria, for example Microcystis aeruginosa, whose lipopolysaccharide could trigger human immune response (Moosová et al., 2019). Moreover, phylum Elusimicrobia were proved to be positively correlated to multiple proinflammatory cytokines and promoted the occurrences of immunological stress in colitis rats (Tao et al., 2017), which was in line with our finding.
Even so, massive works were still required to clarify the mechanism of the influences of FA on ducks. The obtained alternations of microbial species associated with the dietary FA should be carefully validated. The molecular pathway associated with the amelioration of oxidative and immunological stress by FA was needed to be explored. And the participation of microbiota in the stress modulation still required to be discussed.
Conclusion
In conclusion, dietary supplementation of FA altered the intestinal microbiota at the genera level, which associated with the beneficial effects on the growth performance, anti-oxidant and anti-inflammatory capabilities, intestinal morphology, and epithelia barrier functions in Linwu ducks at the growing stage. Quantity effects were obvious as the significant linear and quadratic relationships were noticed between diet FA levels and the measured parameters. Considering the cost and the efficacy of dietary FA as feed additives, we concluded that 400 mg/kg was the suggested supplementation rate of FA in diets for Linwu ducks during growing stage for the future use as feed additive.
Data Availability Statement
The datasets presented in this study can be found in online repositories. The names of the repository/repositories and accession number(s) can be found below: https://www.ncbi.nlm.nih.gov/, PRJNA723283.
Ethics Statement
The animal study was reviewed and approved by Hunan Agricultural University Institutional Animal Care and Use Committee.
Author Contributions
QD and XGH conceived of and designed the experiments. YL, XH, CL, and GJ performed the sampling. XH performed the serum index measurement. XZ conducted the tissue section. QL performed the 16S rRNA sequencing experiment. YLL, QL, SL, and LH analyzed the data. YL wrote the manuscript. QL contributed to refining the text. All authors contributed to the article and approved the submitted version.
Funding
This study was funded by the National Waterfowl Industrial Technology System of China (CARS-42-21), Innovation and Entrepreneurship Technology Investment Project of Hunan Province (2018Gk5009), and Major and Special Science and Technology Project of Changsha City (kq1902034).
Conflict of Interest
SL was employed by the company Shandong Lonct Enzymes Co., Ltd. YLL was employed by the company Hunan Perfly Biotech Co., Ltd.
The remaining authors declare that the research was conducted in the absence of any commercial or financial relationships that could be construed as a potential conflict of interest.
References
Abdel-Rahman, M. A. (2013). Effect of propolis as additive on some behavioural patterns, performance and blood parameters in muscovy broiler ducks. J. Adv. Vet. Res. 3, 64–68.
Apajalahti, J., Kettunen, A., and Graham, H. (2004). Characteristics of the gastrointestinal microbial communities, with special reference to the chicken. Worlds Poult. Sci. J. 60, 223–232. doi: 10.1079/WPS200415
Averós, X., and Estevez, I. (2018). Meta-analysis of the effects of intensive rearing environments on the performance and welfare of broiler chickens. Poult. Sci. 97, 3767–3785. doi: 10.3382/ps/pey243
Berghof, T. V. L., Arts, J. A. J., Bovenhuis, H., Lammers, A., Van der Poel, J. J., and Parmentier, H. K. (2018). Antigen-dependent effects of divergent selective breeding based on natural antibodies on specific humoral immune responses in chickens. Vaccine 36, 1444–1452. doi: 10.1016/j.vaccine.2018.01.063
Borda-Molina, D., Seifert, J., and Camarinha-Silva, A. (2018). Current perspectives of the chicken gastrointestinal tract and its microbiome. Comput. Struct. Biotechnol. J. 16, 131–139. doi: 10.1016/j.csbj.2018.03.002
Cao, Y., Zhang, Y., Qi, J., Liu, R., Zhang, H., and He, L. (2015). Ferulic acid inhibits h2o2-induced oxidative stress and inflammation in rat vascular smooth muscle cells via inhibition of the nadph oxidase and nf-κb pathway. Int. Immunopharmacol. 28, 1018–1025. doi: 10.1016/j.intimp.2015.07.037
Castanon, J. I. R. (2007). History of the use of antibiotic as growth promoters in european poultry feeds. Poult. Sci. 86, 2466–2471. doi: 10.3382/ps.2007-00249
Choct, M. (2009). Managing gut health through nutrition. Br. Poult. Sci. 50, 9–15. doi: 10.1080/00071660802538632
Costa, M. C., Bessegatto, J. A., Alfieri, A. A., Weese, J. S., and Oba, A. (2017). Different antibiotic growth promoters induce specific changes in the cecal microbiota membership of broiler chicken. PLoS One 12:e0171642. doi: 10.1371/journal.pone.0171642
Daglia, M. (2012). Polyphenols as antimicrobial agents. Curr. Opin. Biotechnol. 23, 174–181. doi: 10.1016/j.copbio.2011.08.007
Dandachi, I., Chaddad, A., Hanna, J., Matta, J., and Daoud, Z. (2019). Understanding the epidemiology of multi-drug resistant gram-negative bacilli in the middle east using a one health approach. Front. Microbiol. 10:1941. doi: 10.3389/fmicb.2019.01941
Feng, G. D., He, J., Ao, X., and Chen, D. W. (2017). Effects of maize naturally contaminated with aflatoxin b1on growth performance, intestinal morphology, and digestive physiology in ducks. Poult. Sci. 96, 1948–1955. doi: 10.3382/ps/pew420
Fu, J., Liu, C. P., Zhang, Z. W., Xing, M. W., and Xu, S. W. (2013). Influence of inflammatory pathway markers on oxidative stress induced by cold stress in intestine of quails. Res. Vet. Sci. 95, 495–501. doi: 10.1016/j.rvsc.2013.05.006
Gangadoo, S., Dinev, I., Chapman, J., Hughes, R. J., Van, T. T. H., Moore, R. J., et al. (2018). Selenium nanoparticles in poultry feed modify gut microbiota and increase abundance of faecalibacterium prausnitzii. Appl. Microbiol. Biotechnol. 102, 1455–1466. doi: 10.1007/s00253-017-8688-4
Gemma, S., Molteni, M., and Rossetti, C. (2016). Lipopolysaccharides in cyanobacteria: a brief overview. Adv. Microbiol. 06, 391–397. doi: 10.4236/aim.2016.65038
Genovese, K. J., He, H., Swaggerty, C. L., and Kogut, M. H. (2013). The avian heterophil. Dev. Comp. Immunol. 41, 334–340. doi: 10.1016/j.dci.2013.03.021
Gibiino, G., Lopetuso, L., Scaldaferri, F., Rizzatti, G., Binda, C., and Gasbarrini, A. (2018). Exploring bacteroidetes: metabolic key points and immunological tricks of our gut commensals. Digest. Liver Dis. 50, 635–639. doi: 10.1016/j.dld.2018.03.016
González-Ríos, H., Dávila-Ramírez, J. L., Peña-Ramos, E. A., Valenzuela-Melendres, M., Zamorano-García, L., Islava-Lagarda, T. Y., et al. (2016). Dietary supplementation of ferulic acid to steers under commercial feedlot feeding conditions improves meat quality and shelf life. Anim. Feed. Technol. 222, 111–121. doi: 10.1016/j.anifeedsci.2016.10.011
Gupta, K. M., Das, S., Chow, P. S., and Macbeath, C. (2020). Encapsulation of ferulic acid in lipid nanoparticles as antioxidant for skin: mechanistic understanding through experiment and molecular simulation. ACS Appl. Nano Mater. 3, 5351–5361. doi: 10.1021/acsanm.0c00717
Hassan, M. G., and Abdulla, T. A. (2011). The effect of propolis feed supplementation on hygiene and performance. Iraqi J. Vet. Sci. 25, 77–82. doi: 10.33899/ijvs.2011.5647
Hayes, J., English, L., Carr, L., Wagner, D., and Joseph, S. (2004). Multiple-antibiotic resistance of enterococcus spp. Isolated from commercial poultry production environments. Appl. Environ. Microbiol. 70, 6005–6011. doi: 10.1128/aem.70.10.6005-6011.2004
He, S., Guo, Y., Zhao, J., Xu, X., Song, J., Wang, N., et al. (2018). Ferulic acid protects against heat stress-induced intestinal epithelial barrier dysfunction in iec-6 cells via the pi3k/akt-mediated nrf2/ho-1 signaling pathway. Int. J. Hypertherm. 35, 112–121. doi: 10.1080/02656736.2018.1483534
He, S., Liu, F., Xu, L., Yin, P., Li, D., Mei, C., et al. (2016). Protective effects of ferulic acid against heat stress-induced intestinal epithelial barrier dysfunction in vitro and in vivo. PLoS One 11:e0145236. doi: 10.1371/journal.pone.0145236
Horewicz, V. V., Barbuto, J. A., Cury, P. R., and Carmo, J. P. (2013). Altered phenotype and function of dendritic cells in individuals with chronic periodontitis. Arch. Oral Biol. 58, 1208–1216. doi: 10.1016/j.archoralbio.2013.03.013
Huang, J. Q., Wu, M. S., Tao, G., Lu, M. W., and Song, Y. (2020). Feruloylated oligosaccharides and ferulic acid alter gut microbiome to alleviate diabetic syndrome. Food Res. Int. 137:109410. doi: 10.1016/j.foodres.2020.109410
Hung, P. V., Phat, N. H., and Phi, N. T. L. (2012). Physicochemical properties and antioxidant capacity of debranched starch-ferulic acid complexes. Starch Stärke 65, 382–389. doi: 10.1002/star.201200168
Jinhua, G., Hui, Y., Weikang, G., Ying, K., Lina, G., Qi, L., et al. (2018). The anticancer effects of ferulic acid is associated with induction of cell cycle arrest and autophagy in cervical cancer cells. Cancer Cell Int. 18:102. doi: 10.1186/s12935-018-0595-y
He, J., He, Y., Pan, D., Cao, J., Sun, Y., and Zeng, X. (2019). Associations of gut microbiota with heat stress-induced changes of growth, fat deposition, intestinal morphology, and antioxidant capacity in ducks. Front. Microbiol. 10:903. doi: 10.3389/fmicb.2019.00903
Kaiser, P., Poh, T. Y., Rothwell, L., Avery, S., and Young, J. R. (2005). A genomic analysis of chicken cytokines and chemokines. J. Interferon Cytokine Res. 25, 467–484. doi: 10.1089/jir.2005.25.467
Krajka-Kuźniak, V., Paluszczak, J., Szaefer, H., and Baer-Dubowska, W. (2015). The activation of the nrf2/are pathway in hepg2 hepatoma cells by phytochemicals and subsequent modulation of phase ii and antioxidant enzyme expression. J. Physiol. Biochem. 71, 227–238. doi: 10.1007/s13105-015-0401-4
Lampiasi, N., and Montana, G. (2016). The molecular events behind ferulic acid mediated modulation of il-6 expression in lps-activated raw 264.7 cells. Immunobiology 221, 486–493. doi: 10.1016/j.imbio.2015.11.001
Lauridsen, C. (2018). From oxidative stress to inflammation: redox balance and immune system. Poult. Sci. 98, 4240–4246. doi: 10.3382/ps/pey407
Li, S., Yan, C., Liu, T., Xu, C., Wen, K., Liu, L., et al. (2020). Research note: increase of bad bacteria and decrease of good bacteria in the gut of layers with vs. Without hepatic steatosis. Poult. Sci. 99, 5074–5078. doi: 10.1016/j.psj.2020.07.007
Li, Y., Zhang, L., Liu, L., Tian, Y., Liu, X., Li, W., et al. (2017). Paludicola psychrotolerans gen. Nov., sp. Nov., a novel psychrotolerant chitinolytic anaerobe of the family ruminococcaceae. Int. J. Syst. Evol. Microbiol. 67, 4100–4103. doi: 10.1099/ijsem.0.002260
Li, Y. J., Li, L., Li, J. L., Zhang, L., and Zhou, G. H. (2015). Effects of dietary supplementation with ferulic acid or vitamin e individually or in combination on meat quality and antioxidant capacity of finishing pigs. Asian Austrl. J. Anim. Sci. 28, 374–381. doi: 10.5713/ajas.14.0432
Liu, Z., Ma, Z., Zhang, H., Summah, B. S., Liu, H., An, D., et al. (2019). Ferulic acid increases intestinal lactobacillus and improves cardiac function in tac mice. Biomed. Pharmacother. 120:109482. doi: 10.1016/j.biopha.2019.109482
Lolli, S., Meluzzi, A., Barbieri, S., Vezzoli, G., and Ferrante, V. (2010). Rearing conditions on broiler welfare. Effect of rearing conditions and litter type on reactivity, plumage conditions and foot-pad lesions of broiler chickens. Zootec. Int. 4, 32–37.
Luo, Y. H., Peng, H. W., Wright, G. A. D., Bai, S. P., Ding, X. M., Zeng, Q. F., et al. (2013). Broilers fed dietary vitamins harbor higher diversity of cecal bacteria and higher ratio of clostridium, faecalibacterium, and lactobacillus than broilers with no dietary vitamins revealed by 16s RRNA gene clone libraries. Poult. Sci. 92, 2358–2366. doi: 10.3382/ps.2012-02935
Ma, G., Muinde, B., Zhao, L., Yang, W., and Hu, Q. (2017). In vivo fermentation of pleurotus eryngii polysaccharide and its effects on fecal microbiota composition and immune response. Food Funct. 8, 1810–1821. doi: 10.1039/C7FO00341B
Ma, S., Qiao, X., Xu, Y., Wang, L., and Li, Y. (2019). Screening and identification of a chicken dendritic cell binding peptide by using a phage display library. Front. Immunol. 10:1853. doi: 10.3389/fimmu.2019.01853
Ma, Y., Chen, K., Lv, L., Wu, S., and Guo, Z. (2019). Ferulic acid ameliorates nonalcoholic fatty liver disease and modulates the gut microbiota composition in high-fat diet fed apoe/mice. Biomed. Pharmacother. 113:108753. doi: 10.1016/j.biopha.2019.108753
Mahmoud, A. M., Hussein, O. E., Hozayen, W. G., Bin-Jumah, M. N., and El-Tawab, S. A. (2020). Ferulic acid prevents oxidative stress, inflammation, and liver injury via upregulation of nrf2/ho-1 signaling in methotrexate-induced rats. Environ. Sci. Pollut. Res. 27, 7910–7921. doi: 10.1007/s11356-019-07532-6
Maurya, D. K., and Devasagayam, T. P. A. (2010). Antioxidant and prooxidant nature of hydroxycinnamic acid derivatives ferulic and caffeic acids. Food Chem. Toxicol. 48, 3369–3373. doi: 10.1016/j.fct.2010.09.006
Maxwell, M. H. (1987). The avian eosinophil—a review. Worlds Poult. Sci. J. 43, 190–207. doi: 10.1079/WPS19870013
Maxwell, M. H., and Robertson, G. W. (1995). The avian basophilic leukocyte: a review. Worlds Poult. Sci. J. 51, 307–325. doi: 10.1079/WPS19950021
Moosová, Z., Šindlerová, L., Ambrùzová, B., Ambrožová, G., Vašíèek, O., Velki, M., et al. (2019). Lipopolysaccharides from microcystis cyanobacteria-dominated water bloom and from laboratory cultures trigger human immune innate response. Toxins (Basel) 11:218. doi: 10.3390/toxins11040218
National Research Council. (1994). Nutrient Requirements of Poultry, 9th ed. Washington, DC: The National Academies Press.
Nisar, N. A., Sultana, M., Waiz, H. A., Para, P. A., and Dar, S. A. (2013). Oxidative stress - threat to animal health and production. Int. J. Livestock Res. 3, 76–83.
Oakley, B. B., Lillehoj, H. S., Kogut, M. H., Kim, W. K., Maurer, J. J., Pedroso, A., et al. (2014). The chicken gastrointestinal microbiome. FEMS Microbiol. Lett. 360, 100–112. doi: 10.1111/1574-6968.12608
Ortega-Hernández, A., Martínez-Martínez, E., Gómez-Gordo, R., López-Andrés, N., and Cachofeiro, V. (2020). The interaction between mitochondrial oxidative stress and gut microbiota in the cardiometabolic consequences in diet-induced obese rats. Antioxidants 9:640. doi: 10.3390/antiox9070640
Ou, S., and Kwok, K. C. (2004). Ferulic acid: pharmaceutical functions, preparation and applications in foods. J.Sci. Food Agric. 84, 1261–1269. doi: 10.1002/jsfa.1873
Ou, S., Yong, W., Tang, S., Huang, C., and Jackson, M. G. (2005). Role of ferulic acid in preparing edible films from soy protein isolate. J. Food Eng. 70, 205–210. doi: 10.1016/j.jfoodeng.2004.09.025
Pan, D., and Yu, Z. (2014). Intestinal microbiome of poultry and its interaction with host and diet. Gut Microbes 5, 108–119. doi: 10.4161/gmic.26945
Rehman, M. S., Mahmud, A., Mehmood, S., Pasha, T. N., Hussain, J., and Khan, M. T. (2017). Blood biochemistry and immune response in aseel chicken under free range, semi-intensive, and confinement rearing systems. Poult. Sci. 96, 226–233. doi: 10.3382/ps/pew278
Lochmiller, R. L., and Deerenberg, C. (2000). Trade-offs in evolutionary immunology: Just what is the cost of immunity? Oikos 88, 87–98. doi: 10.1034/j.1600-0706.2000.880110.x
Robins, A., and Phillips, C. J. C. (2011). International approaches to the welfare of meat chickens. Worlds Poult. Sci. J. 67, 351–369. doi: 10.1017/S0043933911000341
Sadar, S. S., Vyawahare, N. S., and Bodhankar, S. L. (2016). Ferulic acid ameliorates tnbs-induced ulcerative colitis through modulation of cytokines, oxidative stress, inos, cox-2, and apoptosis in laboratory rats. Excli. J. 15, 482–499. doi: 10.17179/excli2016-393
Seven, P. T. (2008). The effects of dietary turkish propolis and vitamin c on performance, digestibility, egg production and egg quality in laying hens under different environmental temperatures. Asian Austrl. J. Anim. Sci. 21, 1164–1170. doi: 10.1016/j.applanim.2007.10.001
She, Y., Cai, H., and Liu, G. (2018). Effects of antibiotic on microflora in ileum and cecum for broilers by 16s rrna sequence analysis. Anim. Sci. J. 89, 1680–1691. doi: 10.1111/asj.13113
Shi, D., Bai, L., Qu, Q., Zhou, S., Yang, M., Guo, S., et al. (2019). Impact of gut microbiota structure in heat-stressed broilers. Poult. Sci. 98, 2405–2413. doi: 10.3382/ps/pez026
Song, C., Jiang, J., Han, X., Yu, G., and Pang, Y. (2014). Effect of immunological stress to neuroendocrine and gene expression in different swine breeds. Mol. Biol. Rep. 41, 3569–3576. doi: 10.1007/s11033-014-3219-1
Surai, P., Kochish, I., Fisinin, V., and Kidd, M. (2019). Antioxidant defence systems and oxidative stress in poultry biology: an update. Antioxidants 8:235. doi: 10.3390/antiox8070235
Tao, J. H., Duan, J. A., Jiang, S., Feng, N. N., and Ling, Y. (2017). Polysaccharides from chrysanthemum morifolium ramat ameliorate colitis rats by modulating the intestinal microbiota community. Oncotarget 8, 80790–80803. doi: 10.18632/oncotarget.20477
Truong, H. H., Neilson, K. A., Mcinerney, B. V., Khoddami, A., Roberts, T. H., Cadogan, D. J., et al. (2016). Comparative performance of broiler chickens offered nutritionally equivalent diets based on six diverse, ‘tannin-free’ sorghum varieties with quantified concentrations of phenolic compounds, kafirin, and phytate. Anim. Product. Sci. 57, 828–838. doi: 10.1071/AN16073
Valenzuela-Grijalva, N. V., Pinelli-Saavedra, A., Muhlia-Almazan, A., Domínguez-Díaz, D., and González-Ríos, H. (2017). Dietary inclusion effects of phytochemicals as growth promoters in animal production. J. Anim. Sci. Technol. 59:8. doi: 10.1186/s40781-017-0133-9
Vizzier Thaxton, Y., Christensen, K. D., Mench, J. A., Rumley, E. R., Daugherty, C., Feinberg, B., et al. (2016). Symposium: animal welfare challenges for today and tomorrow. Poult. Sci. 95:2198. doi: 10.3382/ps/pew099
Wang, O., Liu, J., Cheng, Q., Guo, X., and Wang, Y. (2015). Effects of ferulic acid and γ-oryzanol on high-fat and high-fructose diet-induced metabolic syndrome in rats. PLoS One 10:e0118135. doi: 10.1371/journal.pone.0118135
Wang, S., Chen, L., He, M., Shen, J., Li, G., Tao, Z., et al. (2018). Different rearing conditions alter gut microbiota composition and host physiology in shaoxing ducks. Sci. Rep. 8:7387. doi: 10.1038/s41598-018-25760-7
Wang, W. M., Peng, H., and Zhang, G. J. (2018). Specificity of ferulic acid in the chinese pharmacopoeia as a quality evaluation index for various chinese medicinal materials. China J. Tradition. Chin. Med. Pharm. 12, 407–409.
Wang, Y., Chen, X., Huang, Z., Chen, D., Yu, B., Yu, J., et al. (2020). Dietary ferulic acid supplementation improves antioxidant capacity and lipid metabolism in weaned piglets. Nutrients 12:3811. doi: 10.3390/nu12123811
Wang, Y., Wang, W., Wang, R., Meng, Z., Duan, Y., An, X., et al. (2019). Dietary supplementation of ferulic acid improves performance and alleviates oxidative stress of lambs in a cold envrionment. Can. J. Anim. Sci. 99, 705–712. doi: 10.1139/cjas-2018-0200
Wong, J. M. W., De Souza, R., Kendall, C. W. C., Emam, A., and Jenkins, D. J. A. (2006). Colonic health: fermentation and short chain fatty acids. J. Clin. Gastroenterol. 40, 235–243. doi: 10.1097/00004836-200603000-00015
Xia, Y., Kong, J., Zhang, G., Zhang, X., and Kong, Y. (2019). Effects of dietary supplementation with lysozyme on the structure and function of the cecal microbiota in broiler chickens. PLoS One 14:e0216748. doi: 10.1371/journal.pone.0216748
Yan, J., Zhou, B., Xi, Y., Huan, H., and Li, M. (2019). Fermented feed regulates growth performance and the cecal microbiota community in geese. Poult. Sci. 98, 4673–4684. doi: 10.3382/ps/pez169
Zhao, Y., Yan, Y., Zhou, W., Chen, D., and Cao, Y. (2020). Effects of polysaccharides from bee collected pollen of chinese wolfberry on immune response and gut microbiota composition in cyclophosphamide-treated mice. J. Funct. Foods 72:104057. doi: 10.1016/j.jff.2020.104057
Zhou, J., Wu, S., Qi, G., Fu, Y., Wang, W., Zhang, H., et al. (2021). Dietary supplemental xylooligosaccharide modulates nutrient digestibility, intestinal morphology, and gut microbiota in laying hens. Anim. Nutr. 7, 152–162. doi: 10.1016/j.aninu.2020.05.010
Zhou, Z., Xu, J., Zhao, W., Chen, X., Jin, Y., Tang, N., et al. (2017). Ferulic acid relaxed rat aortic, small mesenteric and coronary arteries by blocking voltage-gated calcium channel and calcium desensitization via dephosphorylation of erk1/2 and mypt1. Eur. J. Pharmacol. 815, 26–32. doi: 10.1016/j.ejphar.2017.10.008
Keywords: gut microbiota, ferulic acid, growth performance, serum cytokine, intestinal morphology, duck
Citation: Liu Y, Lin Q, Huang X, Jiang G, Li C, Zhang X, Liu S, He L, Liu Y, Dai Q and Huang X (2021) Effects of Dietary Ferulic Acid on the Intestinal Microbiota and the Associated Changes on the Growth Performance, Serum Cytokine Profile, and Intestinal Morphology in Ducks. Front. Microbiol. 12:698213. doi: 10.3389/fmicb.2021.698213
Received: 21 April 2021; Accepted: 09 June 2021;
Published: 13 July 2021.
Edited by:
Jia Yin, Hunan Normal University, ChinaReviewed by:
Xin Wu, Chinese Academy of Sciences (CAS), ChinaGuang-Hai Qi, Feed Research Institute, Chinese Academy of Agricultural Sciences, China
Xuemei Ding, Sichuan Agricultural University, China
Copyright © 2021 Liu, Lin, Huang, Jiang, Li, Zhang, Liu, He, Liu, Dai and Huang. This is an open-access article distributed under the terms of the Creative Commons Attribution License (CC BY). The use, distribution or reproduction in other forums is permitted, provided the original author(s) and the copyright owner(s) are credited and that the original publication in this journal is cited, in accordance with accepted academic practice. No use, distribution or reproduction is permitted which does not comply with these terms.
*Correspondence: Qiuzhong Dai, ZGFpcWl1emhvbmdAMTYzLmNvbQ==; Xingguo Huang, aHhnNjg5ODlAaHVuYXUuZWR1LmNu
†These authors have contributed equally to this work and share first authorship