- 1Beijing Advanced Innovation Center for Food Nutrition and Human Health, College of Food Science and Nutritional Engineering, China Agricultural University, Beijing, China
- 2Key Laboratory of Functional Dairy, Co-constructed by Ministry of Education and Beijing Municipality, College of Food Science and Nutritional Engineering, China Agricultural University, Beijing, China
- 3Department of Food Science, Beijing University of Agriculture, Beijing, China
- 4College of Food Science and Engineering, Ocean University of China, Qingdao, China
In Streptococcus thermophilus, EpsC is a polysaccharide co-polymerase which is involved in determining the chain length of EPS synthesized by the Wzx/Wzy-dependent pathway. Our previous study found that there was a positive correlation between transcription level of epsC and molecular weight of EPS in S. thermophilus 05-34. To further investigate the effects of EpsC on EPS biosynthesis, this gene was over-expressed in S. thermophilus 05-34 in this study. Reverse transcription qPCR and Western blotting confirmed the successful transcription and translation of epsC in 05-34, respectively. The yield of EPS was not affected by the over-expression of EpsC. Gas chromatography-mass spectrometry (GC-MS) showed that the monosaccharide composition was still composed of galactose and glucose in a molar ratio of 1.0:0.8, whereas high performance gel permeation chromatography (HPGPC) indicated that the molecular weight of EPS was increased from 4.62 × 105 Da to 9.17 × 105 Da by the over-expression of EpsC. In addition, S. thermophilus 05epsC which could produce higher molecular weight EPS improved the viscoelasticity and water-holding capacity of yogurt, but significantly reduced the level of syneresis in yogurt. In summary, these results indicated that homologous over-expression of EpsC in S. thermophilus could increase the molecular weight of EPS and improve the microrheological or physical properties of yogurt.
Introduction
Streptococcus thermophilus is a homofermentative facultative anaerobe, which has been widely used as a starter culture for the manufacture of dairy products, e.g., yogurt and cheese (Pega et al., 2017; Vieira et al., 2019). In yogurt, the presence of exopolysaccharides (EPS) produced by S. thermophilus has been reported to improve viscosity and texture, increase resistance to mechanical handling and prevent syneresis (Broadbent et al., 2003; Cui et al., 2017). Therefore, the in situ production of EPS by S. thermophilus has the potential to decrease the amount of added ingredients and stabilizers, such as dairy proteins, starch, pectin, and hydrocolloids (Zeidan et al., 2017). The replacement of these additives would lead to a clean label and a reduced production cost. Moreover, some EPSs produced by S. thermophilus have antibacterial, antioxidant, anti-inflammatory, and immunomodulating activities (Marcial et al., 2013; Li and Shah, 2014; Zhang et al., 2016). Consequently, EPS production is one of the most important and attractive properties of S. thermophilus strains.
Based on monosaccharide composition, the bacterial EPS are classified as homopolysaccharides (HoPS) and heteropolysaccharides (HePS). HoPS consist of only one type of monosaccharide. HePS are complex polymers with a backbone of repeating subunits that consists of three to eight monosaccharides, such as glucose, galactose, and rhamnose (Zeidan et al., 2017). HePS often differ by monosaccharide ratios, polymerization degree, charge, and linkages between units (Torino et al., 2015). The diverse EPS structures lead to the different functions of EPS, which are further connected to the rheological properties of dairy products. For example, high-molecular mass EPS produced by S. thermophilus can improve the viscosity of acidified milk (Faber et al., 1998; Mende et al., 2012). A stiff and linear EPS produced by S. thermophilus 2104 led to higher viscosity than other branched and flexible EPS (Gentès et al., 2011). In addition, anionic EPS led to higher viscosity and shear stability in stirred acid milk products (Mende et al., 2016). Therefore, a better understanding of the EPS structure–function relationship might provide new strategy for developing EPS with desirable characteristics and improving the rheological properties of dairy products.
The molecular structure of EPS has been proved to be regulated by a specific gene cluster (Zeidan et al., 2017). Generally, the eps cluster of S. thermophilus can be divided in three parts: the first region includes the regulatory genes of EPS production (epsA, epsB) and chain length determination genes (epsC, epsD); the second region is associated with biosynthesis of repeating units of EPS (epsE, epsI, and epsJ); the third region is responsible for polymerization and export of repeating units (epsH, epsK) (Zeidan et al., 2017; Wang et al., 2020). Notably, the chain length determination gene epsC and epsD are involved in polysaccharide assembly (Cui et al., 2017). When intracellular C-terminal portion of CpsC (an EpsC homolog) is deleted, long are produced by Streptococcus agalactiae (Toniolo et al., 2015). In Xanthomonas campestris, higher molecular weight xanthan is produced when the polysaccharide co-polymerase GumC (an EpsC homolog) is over-expressed (Galván et al., 2013). In addition, GumC over-expression increased xanthan viscosity. In our previous study, the transcription level of epsC showed a 2.7-fold up-regulation in S. thermophilus 05-34 under the optimal fermentation condition (Li et al., 2016). Meanwhile, the molecular weight of EPS was increased by nine times compared with that in the non-optimal fermentation condition. However, the relationship between EpsC expression level and molecular structure of EPS remains elusive in S. thermophilus. In this study, homologous over-expression was employed to investigate if the expression level of EpsC could affect the molecular weight of EPS and the microrheology of yogurt.
Materials and Methods
Bacterial Strains, Plasmids, and Growth Conditions
The bacterial strains and plasmids used in this study are listed in Table 1. Streptococcus thermophilus and Lactobacillus delbrueckii subsp. bulgaricus were grown under static conditions at 37°C in de Man-Rogosa-Sharpe (MRS) broth for 24 h. As a host for gene cloning, Lactococcus lactis was routinely grown at 30°C in M17 medium (Oxoid, Unipath, Basingstoke, United Kingdom) containing 0.5% wt/vol glucose (GM17) for 24 h. When necessary, medium was supplemented with 5 μg/mL chloramphenicol for S. thermophilus and Lc. lactis.
DNA Manipulation Techniques
Genomic DNA from S. thermophilus 05-34 was prepared by lysozyme pretreatment and the genomic DNA Extraction Kit (Tiangen, Beijing, China). Mini-prep plasmid isolations from Lc. lactis were performed using the E.Z.N.ATM Plasmid Mini Kit I (OMEGA Bio-tek Inc., Doraville, GA, United States). Standard PCR was carried out using Q5TM High-Fidelity DNA polymerase following the manufacturer’s instructions (NEB, Beijing, China). DNA digestion with restriction endonucleases and DNA ligation were performed according to the manufacturer’s instructions (NEB). The electroporation of Lc. lactis NZ9000 was preformed according to previously described procedures (Holo and Nes, 1989). Primers used in this study were designed using PRIMER V5 software (PREMIER Biosoft International, Palo Alto, CA) and synthesized by Sangon Biotech (Beijing, China). DNA sequencing was performed by Sangon Biotech and the results were further analyzed with the DNAMAN software package (Lynnon Biosoftware, Vaudreuil, QC, Canada).
Construction of the Recombinant Strain S. thermophilus 05epsC
The epsC was amplified from genomic DNA of S. thermophilus 05-34 using the primer pair: F-epsC: 5′-CATGCCATGGGGAATCAAGATAACAC-3′ and R-epsC 5′- CGCGAGCTCTTAAATTTTATCTGTATC-3′. Restriction sites NcoI and SacI used for subsequent cloning are underlined. The PCR product digested by NcoI and SacI was inserted into the corresponding sites of pSlpA-8148 at the downstream of PslpA promoter. Subsequently, the ligation mixture was transformed into Lc. lactis NZ9000 by electroporation. Lc. lactis transformants were selected on GM17 plates containing 5 μg/mL chloramphenicol. Plasmids were isolated from Lc. lactis transformants and further verified by DNA sequencing. Subsequently, the resulting plasmid pSlpA-epsC was transformed into S. thermophilus 05-34 by electroporation in a 0.2 cm cuvette at 1.5 kV, 25 mF, and 200 Ω (Marciset and Mollet, 1994). The recombinant strain was designated as S. thermophilus 05epsC. Moreover, plasmid pSlpA-epsC in S. thermophilus 05epsC was extracted and verified by DNA sequencing.
Transcriptional Analysis of epsC in S. thermophilus 05epsC
Streptococcus thermophilus 05epsC and the control strain S. thermophilus 05CK were grown in 10 mL MRS broth (initial pH 7.0) at 37°C for 30 h. Cells were harvested by centrifugation at 6,000 × g for 10 min. The total RNA was isolated using RNAprep pure cell/bacteria kit following the manufacture’s instruction (Tiangen, Beijing, China). Subsequently, 1 μg of total RNA was used as the template for reverse transcription. And cDNA was obtained using PrimeScript II 1st strand cDNA synthesis kit (Takara). Real-time quantitative PCR (qPCR) was performed using SuperReal PreMix Plus SYBR Green kit (Tiangen, Beijing, China) on LightCycler 480 real-time thermocycler (Roche Diagnostics, Meylan, France). The primers used for qPCR are listed in Supplementary Table 1. Primer specificity was assessed by examination of the melting curve at the end of amplification. Gene expressions were calculated by the 2–ΔΔCt method by using the 16S rRNA as the reference gene (Schmittgen and Livak, 2008).
Western Blotting Assay
To confirm the over-expression of epsC in S. thermophilus 05-34 by Western blotting, the epsC gene was amplified by PCR using primers F-epsC and R-epsCh6 (Supplementary Table 1), thereby introducing a 6× His tag coding sequence to the 3′-end. This amplicon was cloned into pSlpA-8148, and then transformed into S. thermophiles 05-34, resulting in the recombinant strain S. thermophilus 05epsCh6. S. thermophilus 05CK and 05epsCh6 were grown under static conditions at 37°C in MRS broth supplemented with 5 μg/mL chloramphenicol for 30 h prior to protein extraction. Protein extraction and Western blot were performed as previously described (Wang et al., 2020).
Isolation and Purification of EPS
For EPS isolation, overnight cultures of S. thermophilus 05epsC and 05CK were inoculated (2% v/v) into 1 L sterilized reconstituted skim milk medium (RSM, 10% w/v) and grown for 30 h at 37°C. The EPS extraction were performed as previously described (Qin et al., 2011). Briefly, cultures were heated at 100°C for 15 min and centrifuged at 6,000 g for 15 min to remove precipitates. And then proteins in the supernatant were precipitated by adding trichloroacetic acid (TCA) at final concentration of 4% (v/v). After 2 h incubation at 4°C, the supernatant was collected by centrifugation at 6,000 g for 15 min. To precipitate crude EPS, three volumes of cold absolute ethanol was mixed with one volume of supernatant. After 24 h incubation at 4°C, the pellet containing EPS was obtained by centrifugation at 6,000 g for 20 min. Crude EPS was dialyzed with a membrane of molecular weight cut-off (MWCO) 8–10 kDa and then lyophilized. The EPS was purified by a Sepharose CL-6B gel chromatography column (1.6 × 80 cm, Pharmacia, Piscataway, NJ, United States) as previously described (Li et al., 2016). Each 10 mL of elution was collected automatically. Elution curve was plotted by phenol-sulfuric acid assay (detected at 490 nm). The yield of EPS was quantified using the phenol-sulfuric method using glucose as a standard (Dubois et al., 1956). Concentration of protein and nucleic acid of purified fraction was determined spectroscopically at 280 and 260 nm, respectively.
Molecular Weight and Monosaccharide Composition of EPS
The average molecular weight (Mw) of EPS was measured by high performance gel permeation chromatography (HPGPC). The HPGPC system consisted of Agilent 1260 series HPLC system (Agilent, United States) and Shodex SUGAR KS series containing KS-804 and KS-805 columns (Shodex Co., Tokyo, Japan). The purified EPS was detected using refractive index detector (RI-2000, Waters, United States) according to the method which we used previously (Wang et al., 2020). Briefly, 40 μg purified EPS was eluted with 20 mM NaCl at a flow rate of 0.8 mL/min. Dextrans of known Mw (5,000, 11,600, 23,800, 48,600, 80,900, 148,000, 273,000, 409,800, and 667,800 Da, Sigma–Aldrich, St. Louis, MO, United States) were used to calibrate the Mw of sample. Data processing was performed with CirrusTM GPC software version 3.0 (Agilent Technologies, Santa Clara, CA, United States).
The monosaccharide composition was determined by gas chromatography coupled with mass spectrograph (GC-MS) as previously described (Li et al., 2016). Briefly, 2 mg purified EPS was hydrolyzed with 2 mL trifluoroacetic acid (TFA) at 120°C for 90 min. The hydrolysate was neutralized to pH 7.0 with 2 M NaOH and evaporated. The hydrolysate was reduced with 30 mg/mL NaBH4 for 8 h at room temperature. The excess NaBH4 was decomposed with acetic acid and removed by repeated evaporation to dryness with the addition 0.1% (v/v) methanol. Afterward, the dry hydrolysate was dissolved in 1 mL acetic anhydride, and then the mixture was incubated at 100°C for 60 min. After the reaction, 3 mL of methylbenzene was added and the product was dried with a rotary evaporator. A mixture of chloroform (1 mL) and deionized water (1 mL) was added to the sample, followed by vortexing. The organic phase was subsequently analyzed on a GC 7890A platform (Agilent Technologies, United States), equipped with an HP-5 column (30 m × 0.25 mm × 0.25 μm) and a mass spectrum (MS) analyzer. The conditions for GC-MS were used as follows: initial column temperature was set at 120°C with a rate of 3°C/min to reach 250°C and then held at 250°C for 5 min. Samples were injected into the column with N2 as the carrier gas at a flow rate of 1 mL/min. The following monosaccharides were derivatized as standards: rhamnose, fucose, arabinose, xylose, mannose, glucose, galactose. The monosaccharide composition of EPS was determined by comparison with the retention time of monosaccharide standards.
Microrheological Properties of Yogurt Produced by S. thermophilus 05epsC
The passive microrheology of yogurt was measured by diffusing-wave spectroscopy (DWS, LAB 6 Rheolaser MASTER, Formulaction SAS, I’Union, France). The production of yogurt was carried out as described previously (Qin et al., 2011). Ultra-high temperature treated (UHT) whole bovine milk was purchased from San Yuan Foods Company (Beijing, China) through a local distributor. This milk contained 3.1% fat, 2.9% protein, and 8.1% (w/w) non-fat milk solid. Non-EPS-producing Lb. delbrueckii subsp. bulgaricus 05-22 was used with S. thermophilus strains as starter cultures in this study. The experimental group yogurt was inoculated (1.5% v/v) with S. thermophilus 05epsC and Lb. delbrueckii subsp. bulgaricus 05-22 in a ratio of 1:1, while the control group was inoculated (1.5% v/v) with S. thermophilus 05CK and Lb. delbrueckii subsp. bulgaricus 05-22. Immediately after inoculation, 20 mL aliquots of the samples were transferred into optical glass cuvettes of LAB 6 Rheolaser MASTER. Gel formation was observed at 42°C. The observation and data collection were continued for over 6 h. The scatterer mobility, in terms of speed and displacement, enabled the mean square displacement (MSD) of the particles vs. decorrelation time to be plotted, which was directly related to the viscoelastic properties. Elasticity index (EI) and macroscopic viscosity index (MVI) were also calculated as described previously (Sun et al., 2018). The data, including MSD curve, EI and MVI, were collected and calculated using the software 1.4.0.0 incorporated in the instrument.
Physical Properties of Yogurt Produced by S. thermophilus 05epsC
The production of yogurt was carried out as described above. All batches were incubated at 42°C until pH 4.6 was reached. Acidity was measured by a titrimetric method with 0.1 mol/L NaOH as described previously (Qin et al., 2011). For water-holding capacity (WHC), 20 g sample were centrifuged at 500 g for 10 min at 4°C. The supernatant was then collected by pipette and weighed. The water holding capacity was determined in triplicate and calculated as follows: . WHC is the water holding capacity of the sample, MS is the supernatant after centrifugation and MY is the mass of the weighed yogurt. Whey separation was measured by weighing 100 g of yogurt into a funnel with one layer of 200 mesh nylon fabric. Whey was collected and weighed (% w/w) after 3 h at 6°C as described previously (Qin et al., 2011).
Statistical Analysis
Data were analyzed using GraphPad Prism 6 software for Windows (GraphPad Software, Inc., La Jolla, CA, United States). When two groups were compared, an unpaired Student t-test with Welch’s correction was used to calculate P-values.
Results
Homologous Over-Expression of EpsC
For homologous over-expression, the expected 693 bp epsC gene was amplified, purified and cloned into constitutive expression vector pSlpA-8148 to generate recombinant plasmid, designated as pSlpA-epsC. DNA sequencing showed that gene epsC in pSlpA-epsC was 100% identity to the epsC gene from S. thermophilus 05-34 (DIS_02530; GenBank Accession No. QFLC00000000). RT-qPCR revealed that the transcription level of epsC in S. thermophilus 05epsC was 38.04 ± 6.74 fold higher than that in the control strain S. thermophilus 05CK (Figure 1A). Western blotting assay with anti His-Tag mouse monoclonal antibody further confirmed the production of a 26-kDa protein in S. thermophilus 05epsCh6, while no corresponding band was observed in S. thermophilus 05CK (Figure 1B). These results indicated that gene epsC was successfully over-expressed in S. thermophilus 05-34.
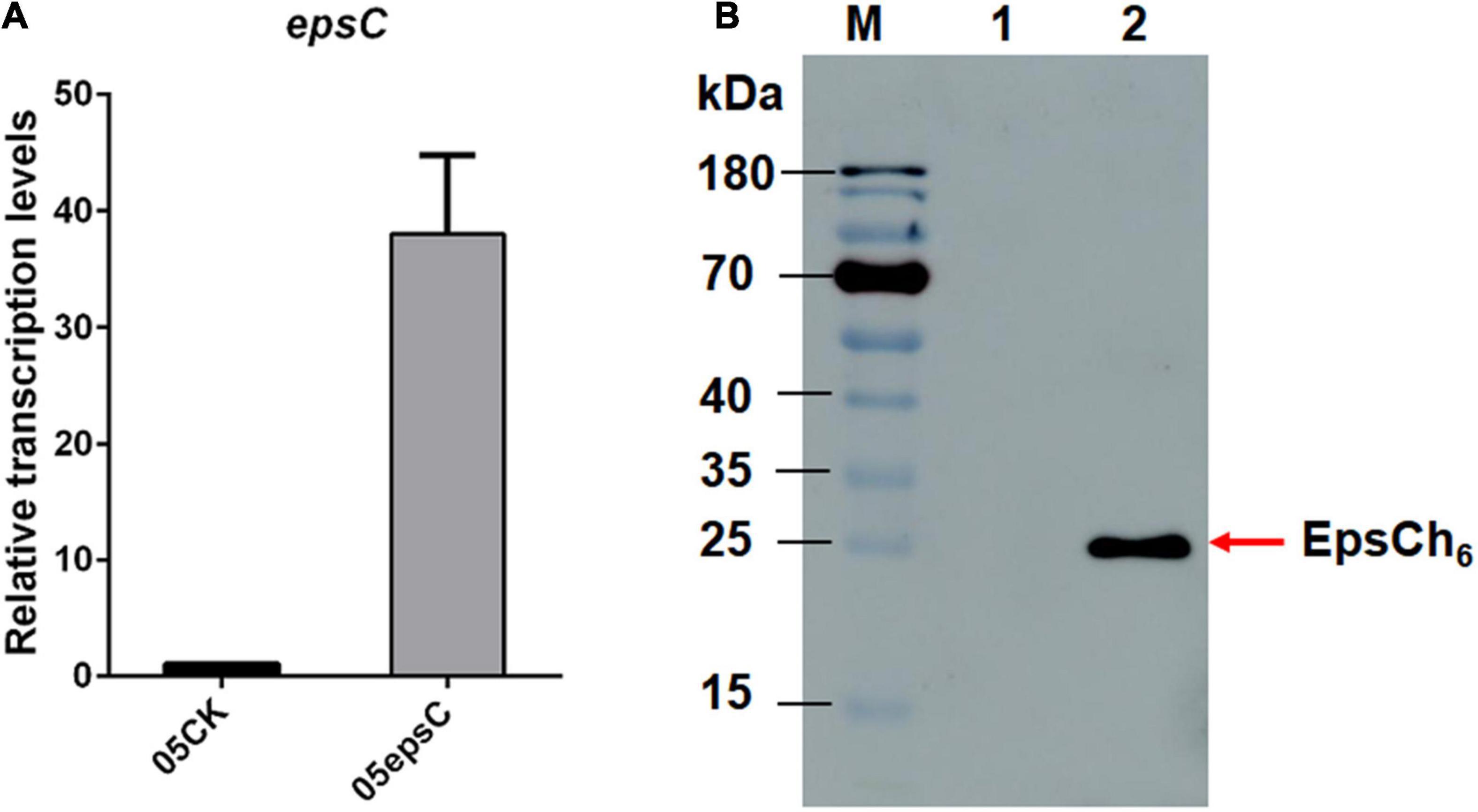
Figure 1. The over-expression of epsC in S. thermophilus 05-34. (A) RT-qPCR analysis of the transcription level of epsC in S. thermophilus 05epsC. The fold change calculated was relative to the transcript levels in S. thermophilus 05epsC compared with that in 05CK. They were normalized using 16S rRNA gene as an internal control. Data are reported as mean ± SD from three independent experiments. (B) Detection of the over-expression of EpsC in S. thermophilus by Western blotting. M: PageRuler Prestained Protein Ladder (Thermo Fisher Scientific, Waltham, MA, United States). Lane 1: Protein sample extracted from S. thermophilus 05CK; Lane 2: Protein sample extracted from S. thermophilus 05epsCh6.
Isolation and Purification of EPS Produced by S. thermophilus 05epsC
Streptococcus thermophilus 05epsC and 05CK were cultivated in 10% reconstituted skim milk (RSM) at 37°C for 30 h, respectively. Then EPS from RSM samples was isolated, purified and quantified. According to the elution profile of EPS from S. thermophilus 05epsC, there was only one single and relatively symmetrical peak at OD490 nm in fraction No. 7 (Supplementary Figure 2), while the peak of the elution profile in the control group appeared in fraction No. 9. These results suggested that over-expression of epsC in S. thermophilus 05-34 increased the average molecular weight of EPS. The ultraviolet spectrum of purified EPS fraction showed no absorption at 260 or 280 nm, indicating that there was no nucleic acids or protein contamination in EPS samples. Based on the glucose standard (Supplementary Figure 1), the yield of EPS produced by S. thermophilus 05epsC and 05CK was 85 ± 3 mg/L and 73 ± 9 mg/L, respectively. There is no significant difference in the yield of EPS between 05epsC and 05CK (P = 0.0936, Table 2).
Monosaccharide Composition and Molecular Weight of EPS Produced by S. thermophilus 05epsC
Based on the retention time of different monosaccharide standards, the monomer analysis by GC-MS indicated that the EPS produced by S. thermophilus 05epsC and 05CK were both composed of galactose and glucose in an approximate ratio of 1.0:0.8 (Figure 2). The average molecular weight of EPS produced by 05CK was 4.62 × 105 Da, which was determined by HPGPC. However, the average molecular weight of EPS produced by S. thermophilus 05epsC was increased to be 9.17 × 105 Da, which was 1.98-fold higher than that of 05CK (Figure 3). In this study, the yield and monosaccharide composition of EPS in 05-34 was not affected by over-expression of epsC.
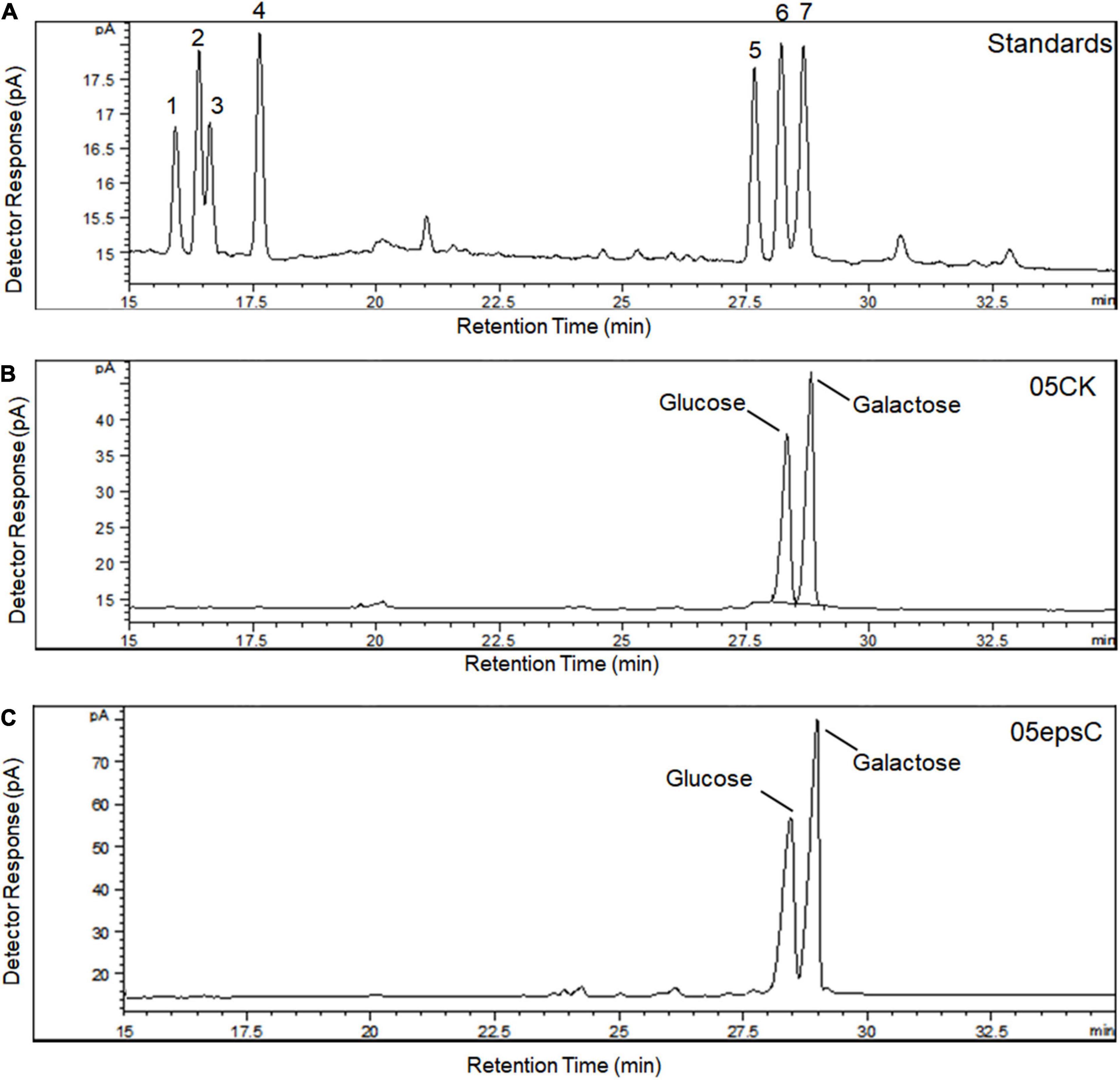
Figure 2. Monosaccharide composition of hydrolyzed EPS from S. thermophilus 05epsC and 05CK by GC-MS analysis. (A) Monosaccharide standards: (1) rhamnose; (2) fucose; (3) arabinose; (4) xylose; (5) mannose; (6) glucose; (7) galactose. (B) Hydrolyzed EPS from S. thermophilus 05CK. (C) Hydrolyzed EPS from S. thermophilus 05epsC.
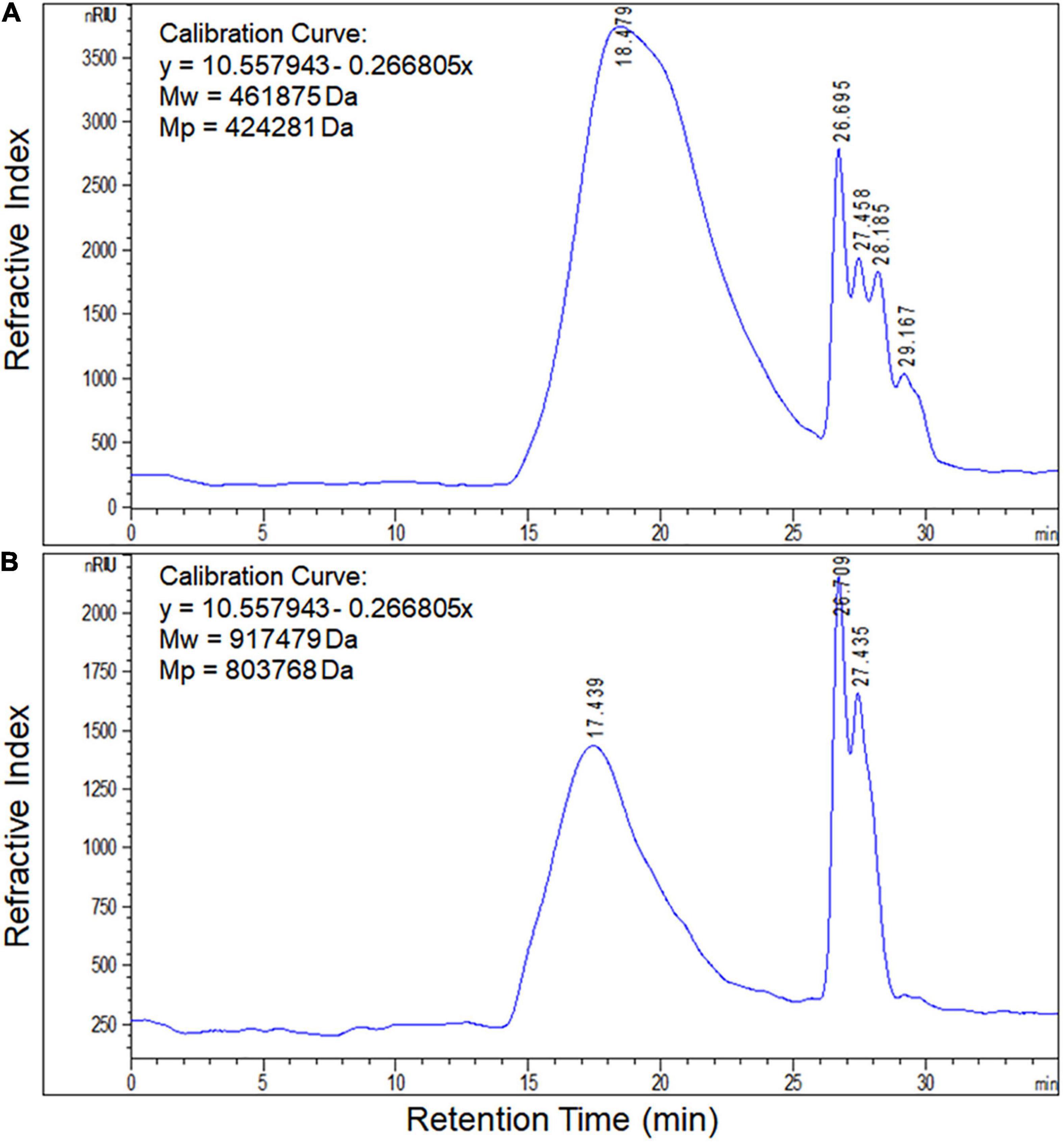
Figure 3. Molecular weight of EPS produced by S. thermophilus 05epsC (A) and 05CK (B). According to the linear regression equation drawn through dextran standards, the molecular weight of EPS samples was determined based on their retention time (X-axis) using gel permeation chromatography (GPC). Calibration curve was y = 10.557943 – 0.266805x. Mw, Average molecular weight; Mp, Peak molecular weight.
The Microrheology of Yogurt Fermented by S. thermophilus 05epsC
The entire fermentation of yogurt was monitored using DWS which has been widely applied to milk protein and polysaccharide mixed systems (Alexander et al., 2008). The time-dependent MSD profiles for all samples showed linear motion, indicating typical free diffusion of particles in the yogurts during fermentation (Figures 4A,B). After 4 h incubation, particles traveled less displacement distance in the yogurt made with S. thermophilus 05epsC than in the control during the same decorrelation time. For instance, when decorrelation time was 0.1 s after 6 h incubation, MSD of particles in the yogurt made with S. thermophilus 05epsC was 141 ± 1.41 nm2. Meanwhile, MSD of particles in the control was 235 ± 1.41 nm2 (Table 3). Particles traveled less displacement distance in the yogurt made with S. thermophilus 05epsC indicated that they have a lower particle speed and higher macroscopic viscosity (Tisserand et al., 2016). Therefore, the MVI of yogurt produced by S. thermophilus 05epsC was significantly higher than that of the control after 5 h incubation (Table 3). In addition, the elasticity index of yogurt produced by S. thermophilus 05epsC was significantly higher than that of the control after 4 h incubation (Figure 4C and Table 3). These results indicated that EPS with higher molecular weight produced by S. thermophilus 05epsC could increase the viscoelasticity of yogurt.
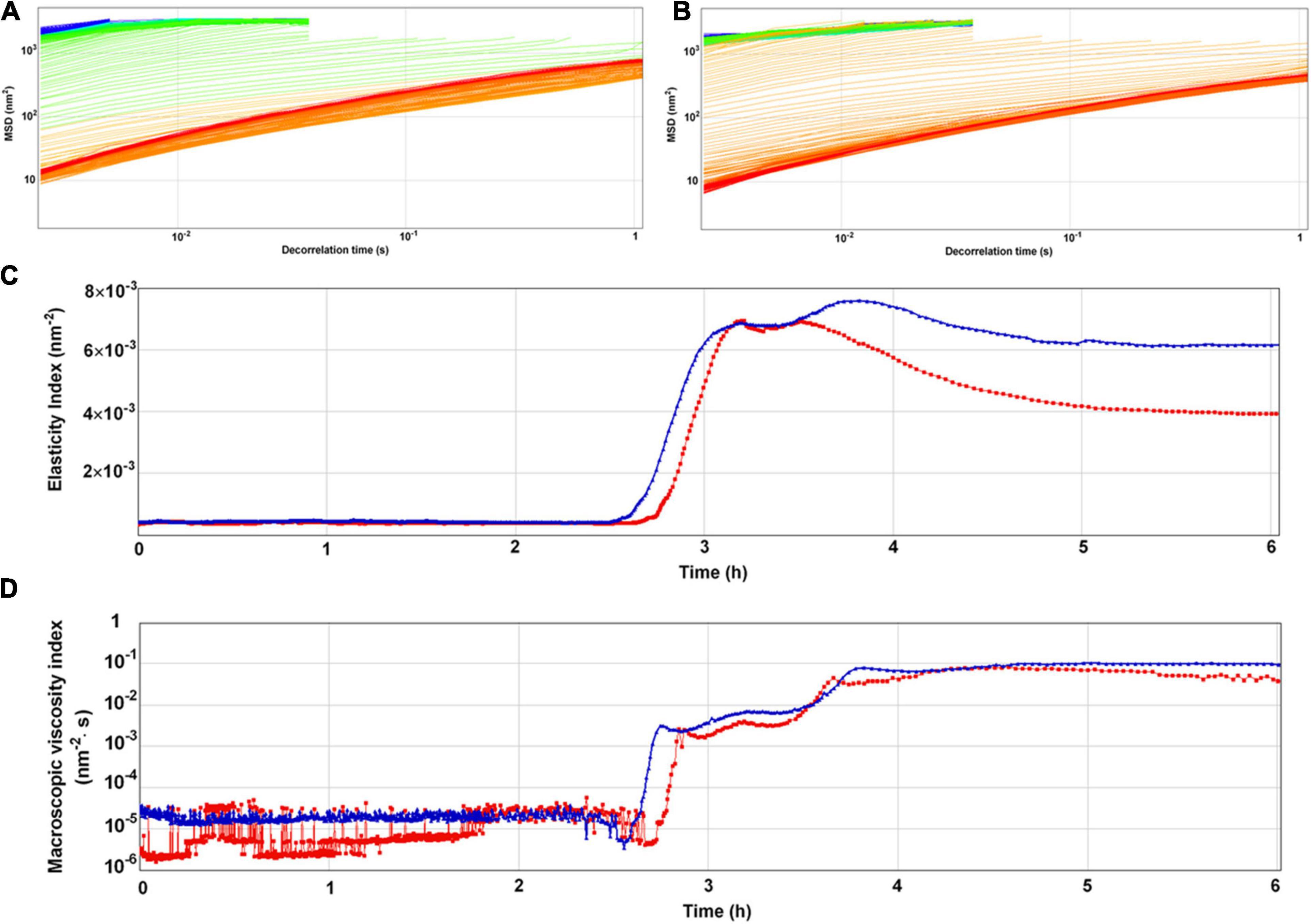
Figure 4. The representative mean square displacement (MSD) curves, elasticity index (EI), and macroscopic viscosity index (MVI) of yogurt produced by S. thermophilus 05epsC. (A) MSD of yogurt produced by S. thermophilus 05CK; (B) MSD of yogurt produced by 05epsC; (C) EI of yogurt produced by 05epsC and 05CK; (D) MVI of yogurt produced by 05epsC and 05CK; Blue triangle: yogurt produced by 05epsC; Red square: yogurt produced by 05CK.
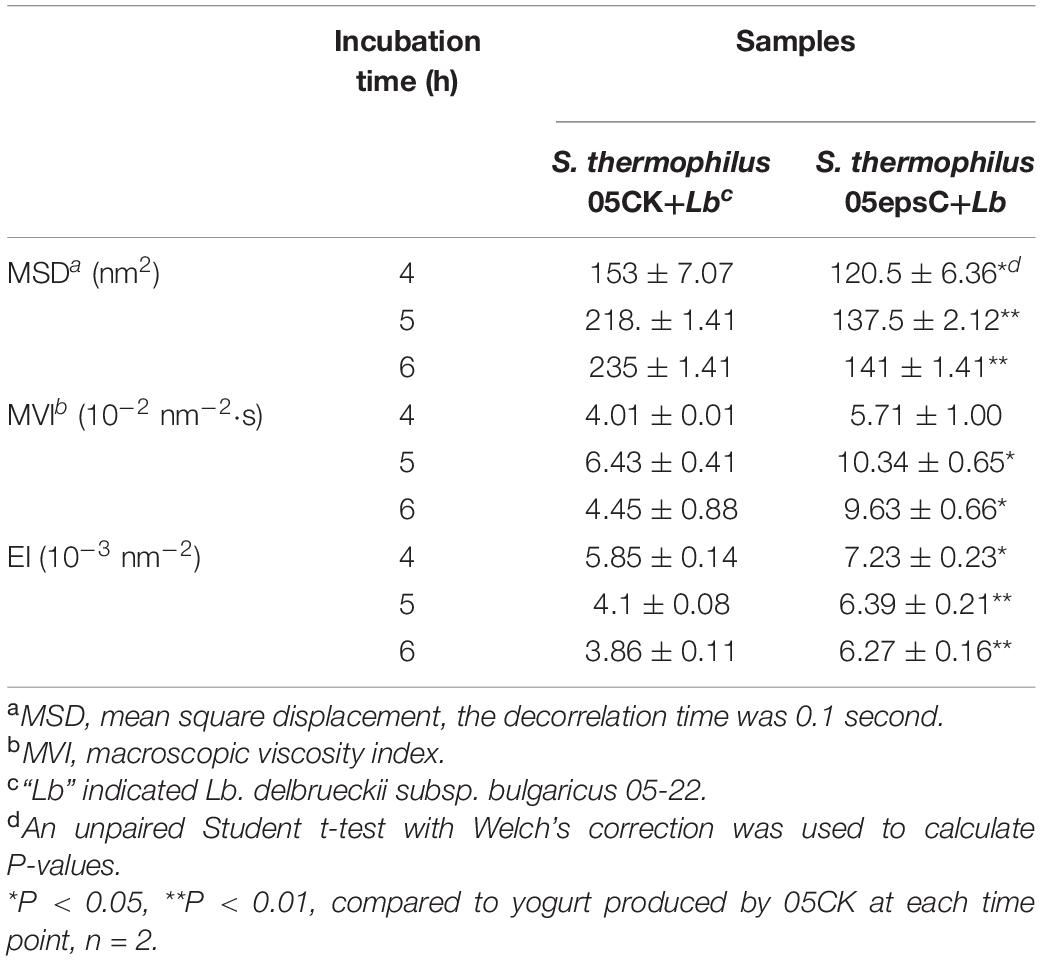
Table 3. The MSD, macroscopic viscosity index, and elasticity index of yogurt produced by S. thermophilus 05epsC or 05CK at 4, 5, and 6 h.
The Physical Properties of Yogurt Fermented by S. thermophilus 05epsC
The physical properties of yogurt after fermentation were also measured and listed in Table 4. There was no significant difference in titratable acidity between the experimental group and the control group (P > 0.05), which suggested that EPS produced by S. thermophilus 05epsC had no adverse effect on final acidity. However, the WHC of yogurt made with S. thermophilus 05epsC was slightly higher than that of the control group (P < 0.05). It is worth to note that the whey separation of yogurt made with S. thermophilus 05epsC was 36.70 ± 2.30% (w/w), which was significantly lower than that of the control group (40.80 ± 0.93% w/w). These results suggested that higher molecular weight EPS produced by S. thermophilus 05epsC could significantly reduce the level of syneresis in yogurt.
Discussion
In S. thermophilus, EpsC is a PCP which is involved in determining the chain length of EPS synthesized by the Wzx/Wzy-dependent pathway (Cui et al., 2017). Topological analysis with TMHMM program revealed that EpsC from S. thermophilus 05-34 is a cytoplasmic membrane protein and contains two transmembrane helices located in amino acid units 25–44 and 181–198 (Supplementary Figure 3A). Meanwhile, the region between amino acid units 48 and 180 was located out of the cell membrane, suggesting that nearly 60% of the protein is located on the outer membrane. Sequence alignment revealed that EpsC from S. thermophilus 05-34 showed 92.61% similarity at the amino acid level with tyrosine-protein kinase transmembrane modulator EpsC from S. thermophilus Sfi6. Secondary structure prediction of EpsC showed that it contains four beta strands and four alpha helices (Supplementary Figure 3B). The arrangement of secondary structure elements indicated that EpsC has a fold similar to the base domain of PCP family proteins, which could be involved in chain-length determination during polysaccharide biosynthesis (Morona et al., 2009). Our previous study indicated that there was a positive correlation between transcription level of epsC and molecular weight of EPS in S. thermophilus 05-34 (Li et al., 2016). In this study, we found that the average molecular weight of EPS increased from 4.62 × 105 Da to 9.17 × 105 Da after homologous over-expression of epsC in S. thermophilus 05-34. However, the yield and monosaccharide composition of EPS were not affected by over-expression of epsC. These results confirmed the role of EpsC in chain length determination during EPS biosynthesis.
Previous studies provided some evidence that viscosity of yogurt was relevant to the molecular weight of EPS produced by the S. thermophilus strains (Faber et al., 1998; Xu et al., 2021). For instance, the average molecular weight of EPS produced by S. thermophilus Sts and Rs was 3.7 × 103 kDa and 2.6 × 103 kDa, respectively. The viscosity of fermented milk produced by S. thermophilus Sts was 126 ± 15 s, which was 3.2-fold higher than that of fermented milk produced by Rs. These results gave a conclusion that EPS with higher molecular weight might contribute to the higher viscosity of products (Faber et al., 1998). Although EPS from these two strains have the same branched heptasaccharide repeating unit, the differences between S. thermophilus Sts and Rs in growth, fermentation rate or post-acidification might also affect the viscosity of stirred milk cultures. In this paper, S. thermophilus 05epsC and 05CK was derived from the same strain 05-34. The MVI of yogurt produced by S. thermophilus 05epsC was significantly higher than that of the control after 5 h incubation. Notably, the viscosity of yogurt can be represented by MVI (Rohart et al., 2016; Bai et al., 2020). The viscosity of fermented milk could be increased by the production of high molecular weight EPS (Hamet et al., 2015). In RSM, over-expression of EpsC increased the molecular weight of EPS, but did not affect the monosaccharide composition and yield. Both RSM and yogurt are milk-based medium and similar in nutritional composition. To further confirm the EpsC over-expression in yogurt, the transcription level of epsC in yogurt has been determined by RT-qPCR. The transcription level of epsC in S. thermophilus 05epsC in yogurt was 42.22 ± 7.13 fold higher than that in the control strain 05CK (Supplementary Figure 4). Therefore, the differences between the yogurt fermented by 05epsC and 05CK was mainly caused by the EPS with different molecular weight.
Generally, EPS can modify yogurt microstructure by preventing casein particles to form large aggregates (Han et al., 2016). Casein particles interconnect to form filaments with the bigger interspace, resulting in lower whey separation. In skim milk gels, the levels of syneresis were decreased by increasing the molecular weight of beta-glucan from 70 to 250 × 103 Da (Lazaridou et al., 2008). This is in consistent with our finding that higher molecular weight EPS produced by S. thermophilus 05epsC could significantly reduce the level of syneresis in yogurt. Therefore, we speculated that higher molecular weight EPS produced by S. thermophilus 05epsC may enhance the structural integrity of the protein-polysaccharide network and the entrapment of the whey in yogurt. To our knowledge, this is the first report about that over-expression of EpsC increased the molecular weight of EPS in S. thermophilus and improved the viscoelasticity and stability of yogurt. These findings will provide new insight into the structure-function relationship of EPS produced by S. thermophilus. And modulation of epsC expression could be a strategy for developing EPS with desirable molecular weight and improving the microrheology of dairy products.
Data Availability Statement
The original contributions presented in the study are included in the article/Supplementary Material, further inquiries can be directed to the corresponding author/s.
Author Contributions
YH and ZZ designed the study, wrote the manuscript, and revised the manuscript. SX and ZZ performed the experiments. HZ and HY analyzed and evaluated the data. All authors read and approved the final manuscript.
Funding
This work was supported by the National Key Research and Development Program of China (2018YFC1604303) and the National Natural Science Foundation of China (No. 21476250).
Conflict of Interest
The authors declare that the research was conducted in the absence of any commercial or financial relationships that could be construed as a potential conflict of interest.
Acknowledgments
We thank Sheng Yin (Beijing Technology & Business University) and Pengjie Wang (China Agricultural University) for the help on diffusing-wave spectroscopy.
Supplementary Material
The Supplementary Material for this article can be found online at: https://www.frontiersin.org/articles/10.3389/fmicb.2021.696222/full#supplementary-material
References
Alexander, M., Piska, I., and Dalgleish, D. G. (2008). Investigation of particle dynamics in gels involving casein micelles: a diffusing wave spectroscopy and rheology approach. Food Hydrocoll. 22, 1124–1134. doi: 10.1016/j.foodhyd.2007.06.004
Bai, M., Huang, T., Guo, S., Wang, Y., Wang, J., Kwok, L.-Y., et al. (2020). Probiotic Lactobacillus casei Zhang improved the properties of stirred yogurt. Food Biosci. 37:100718. doi: 10.1016/j.fbio.2020.100718
Broadbent, J. R., McMahon, D. J., Welker, D. L., Oberg, C. J., and Moineau, S. (2003). Biochemistry, genetics, and applications of exopolysaccharide production in Streptococcus thermophilus: a review. J. Dairy Sci. 86, 407–423. doi: 10.3168/jds.s0022-0302(03)73619-4
Cui, Y., Jiang, X., Hao, M., Qu, X., and Hu, T. (2017). New advances in exopolysaccharides production of Streptococcus thermophilus. Arch. Microbiol. 199, 799–809. doi: 10.1007/s00203-017-1366-1
De Ruyter, P., Kuipers, O. P., and De Vos, W. M. (1996). Controlled gene expression systems for Lactococcus lactis with the food-grade inducer nisin. Appl. Environ. Microbiol. 62, 3662–3667. doi: 10.1128/aem.62.10.3662-3667.1996
Dubois, M., Gilles, K. A., Hamilton, J. K., Rebers, P. T., and Smith, F. (1956). Colorimetric method for determination of sugars and related substances. Anal. Chem. 28, 350–356. doi: 10.1021/ac60111a017
Faber, E. J., Zoon, P., Kamerling, J. P., and Vliegenthart, J. F. (1998). The exopolysaccharides produced by Streptococcus thermophilus Rs and Sts have the same repeating unit but differ in viscosity of their milk cultures. Carbohyd. Res. 310, 269–276. doi: 10.1016/s0008-6215(98)00189-x
Galván, E. M., Ielmini, M. V., Patel, Y. N., Bianco, M. I., Franceschini, E. A., Schneider, J. C., et al. (2013). Xanthan chain length is modulated by increasing the availability of the polysaccharide copolymerase protein GumC and the outer membrane polysaccharide export protein GumB. Glycobiology 23, 259–272. doi: 10.1093/glycob/cws146
Gentès, M.-C., St-Gelais, D., and Turgeon, S. L. (2011). Gel formation and rheological properties of fermented milk with in situ exopolysaccharide production by lactic acid bacteria. Dairy Sci. Technol. 91:645. doi: 10.1007/s13594-011-0039-0
Hamet, M. F., Piermaria, J. A., and Abraham, A. G. (2015). Selection of EPS-producing Lactobacillus strains isolated from kefir grains and rheological characterization of the fermented milks. LWT Food Sci. Technol. 63, 129–135. doi: 10.1016/j.lwt.2015.03.097
Han, X., Yang, Z., Jing, X., Yu, P., Zhang, Y., Yi, H., et al. (2016). Improvement of the texture of yogurt by use of exopolysaccharide producing lactic acid bacteria. BioMed Res. Int. 2016:7945675.
Holo, H., and Nes, I. F. (1989). High-frequency transformation, by electroporation, of Lactococcus lactis subsp. cremoris grown with glycine in osmotically stabilized media. Appl. Environ. Microbiol. 55, 3119–3123. doi: 10.1128/aem.55.12.3119-3123.1989
Lazaridou, A., Vaikousi, H., and Biliaderis, C. (2008). Impact of mixed-linkage (1→ 3, 1→ 4) ß-glucans on physical properties of acid-set skim milk gels. Int. Dairy J. 18, 312–322. doi: 10.1016/j.idairyj.2007.08.005
Li, D., Li, J., Zhao, F., Wang, G., Qin, Q., and Hao, Y. (2016). The influence of fermentation condition on production and molecular mass of EPS produced by Streptococcus thermophilus 05-34 in milk-based medium. Food Chem. 197, 367–372. doi: 10.1016/j.foodchem.2015.10.129
Li, S., and Shah, N. P. (2014). Antioxidant and antibacterial activities of sulphated polysaccharides from Pleurotus eryngii and Streptococcus thermophilus ASCC 1275. Food Chem. 165, 262–270. doi: 10.1016/j.foodchem.2014.05.110
Marcial, G., Messing, J., Menchicchi, B., Goycoolea, F. M., Faller, G., de Valdez Graciela, F., et al. (2013). Effects of polysaccharide isolated from Streptococcus thermophilus CRL1190 on human gastric epithelial cells. Int. J. Biol. Macromol. 62, 217–224. doi: 10.1016/j.ijbiomac.2013.08.011
Marciset, O., and Mollet, B. (1994). Multifactorial experimental design for optimizing transformation: Electroporation of Streptococcus thermophilus. Biotechnol. Bioeng. 43, 490–496. doi: 10.1002/bit.260430609
Mende, S., Mentner, C., Thomas, S., Rohm, H., and Jaros, D. (2012). Exopolysaccharide production by three different strains of Streptococcus thermophilus and its effect on physical properties of acidified milk. Eng. Life Sci. 12, 466–474. doi: 10.1002/elsc.201100114
Mende, S., Rohm, H., and Jaros, D. (2016). Influence of exopolysaccharides on the structure, texture, stability and sensory properties of yoghurt and related products. Int. Dairy J. 52, 57–71. doi: 10.1016/j.idairyj.2015.08.002
Morona, R., Purins, L., Tocilj, A., Matte, A., and Cygler, M. (2009). Sequence-structure relationships in polysaccharide co-polymerase (PCP) proteins. Trends Biochem. Sci. 34, 78–84. doi: 10.1016/j.tibs.2008.11.001
Pega, J., Pérez, C. D., Rizzo, S., Rossetti, L., Díaz, G., Ruzal, S. M., et al. (2017). The incorporation of alpha-tocopherol and functional doses of phytosterol esters during cheesemaking does not affect DNA or mRNA dynamics of Streptococcus thermophilus and Lactococcus lactis throughout and after the end of ripening. LWT 84, 838–841. doi: 10.1016/j.lwt.2017.06.057
Qin, Q., Xia, B., Xiong, Y., Zhang, S., Luo, Y., and Hao, Y. (2011). Structural characterization of the exopolysaccharide produced by Streptococcus thermophilus 05−34 and its in situ application in yogurt. J. Food Sci. 76, C1226–C1230.
Rohart, A., Michon, C., Confiac, J., and Bosc, V. (2016). Evaluation of ready-to-use SMLS and DWS devices to study acid-induced milk gel changes and syneresis. Dairy Sci. Technol. 96, 459–475. doi: 10.1007/s13594-016-0281-6
Schmittgen, T. D., and Livak, K. J. (2008). Analyzing real-time PCR data by the comparative CT method. Nat. Prot. 3:1101. doi: 10.1038/nprot.2008.73
Sun, J., Ren, F., Chang, Y., Wang, P., Li, Y., Zhang, H., et al. (2018). Formation and structural properties of acid-induced casein–agar double networks: Role of gelation sequence. Food Hydrocoll. 85, 291–298.
Toniolo, C., Balducci, E., Romano, M. R., Proietti, D., Ferlenghi, I., Grandi, G., et al. (2015). Streptococcus agalactiae capsule polymer length and attachment is determined by the proteins CpsABCD. J. Biol. Chem. 290, 9521–9532. doi: 10.1074/jbc.m114.631499
Torino, M. I., Font de Valdez, G., and Mozzi, F. (2015). Biopolymers from lactic acid bacteria. Novel applications in foods and beverages. Front. Microbiol. 6:834.
Tisserand, C., Fleury, M., Brunel, L., Bru, P., and Meunier, G. (2012). “Passive microrheology for measurement of the concentrated dispersions stability,” in UK Colloids 2011, (Berlin: Springer), 101–105.
Vieira, P., Pinto, C. A., Lopes-da-Silva, J. A., Remize, F., Barba, F. J., Marszałek, K., et al. (2019). A microbiological, physicochemical, and texture study during storage of yoghurt produced under isostatic pressure. LWT 110, 152–157. doi: 10.1016/j.lwt.2019.04.066
Wang, G., Li, J., Xie, S., Zhai, Z., and Hao, Y. (2020). The N-terminal domain of rhamnosyltransferase EpsF influences exopolysaccharide chain length determination in Streptococcus thermophilus 05-34. PeerJ 8:e8524. doi: 10.7717/peerj.8524
Xu, Z., Guo, Q., Zhang, H., Xiong, Z., Zhang, X., and Ai, L. (2021). Structural characterisation of EPS of Streptococcus thermophilus S-3 and its application in milk fermentation. Int. J. Biol. Macromol. 178, 263–269. doi: 10.1016/j.ijbiomac.2021.02.173
Zeidan, A. A., Poulsen, V. K., Janzen, T., Buldo, P., Derkx, P. M., Øregaard, G., et al. (2017). Polysaccharide production by lactic acid bacteria: from genes to industrial applications. FEMS Microbiol. Rev. 41(Suppl._1), S168–S200.
Keywords: Streptococcus thermophilus, EpsC, homologous expression, molecular weight, yogurt, viscoelasticity
Citation: Zhai Z, Xie S, Zhang H, Yi H and Hao Y (2021) Homologous Over-Expression of Chain Length Determination Protein EpsC Increases the Molecular Weight of Exopolysaccharide in Streptococcus thermophilus 05-34. Front. Microbiol. 12:696222. doi: 10.3389/fmicb.2021.696222
Received: 16 April 2021; Accepted: 29 June 2021;
Published: 20 July 2021.
Edited by:
Marciane Magnani, Federal University of Paraíba, BrazilReviewed by:
Pradip Behare, National Dairy Research Institute (ICAR), IndiaJohn Renye, United States Department of Agriculture, United States
Pradeep Prasanna, University of Peradeniya, Sri Lanka
Maija Tenkanen, University of Helsinki, Finland
Copyright © 2021 Zhai, Xie, Zhang, Yi and Hao. This is an open-access article distributed under the terms of the Creative Commons Attribution License (CC BY). The use, distribution or reproduction in other forums is permitted, provided the original author(s) and the copyright owner(s) are credited and that the original publication in this journal is cited, in accordance with accepted academic practice. No use, distribution or reproduction is permitted which does not comply with these terms.
*Correspondence: Yanling Hao, haoyl@cau.edu.cn