- 1Joint R&D Center of Biotechnology, Retda, Yota Bio-Engineering Co., Ltd., Rizhao, China
- 2VBS Biotec SA, Mexico, Mexico
- 3State Key Laboratory of Biobased Material and Green Papermaking, Shandong Provincial Key Lab of Microbial Engineering, Qilu University of Technology (Shandong Academy of Science), Jinan, China
The identification and use of endophytic bacteria capable of triggering plant growth is an important aim in sustainable agriculture. In nature, plants live in alliance with multiple plant growth-promoting endophytic microorganisms. In the current study, we isolated and identified a new endophytic bacterium from a wild plant species Glyceria chinensis (Keng). The bacterium was designated as a Bacillus altitudinis strain using 16S rDNA sequencing. The endophytic B. altitudinis had a notable influence on plant growth. The results of our assays revealed that the endophytic B. altitudinis raised the growth of different plant species. Remarkably, we found transcriptional changes in plants treated with the bacterium. Genes such as maturase K, tetratricopeptide repeat-like superfamily protein, LOB domain-containing protein, and BTB/POZ/TAZ domain-containing protein were highly expressed. In addition, we identified for the first time an induction in the endophytic bacterium of the major facilitator superfamily transporter and DNA gyrase subunit B genes during interaction with the plant. These new findings show that endophytic B. altitudinis could be used as a favourable candidate source to enhance plant growth in sustainable agriculture.
Introduction
The use of fertilisers in agricultural practice to increase yields has had harmful effects on the environment and quality of the soil for a long time (Worlanyo and Jiangfeng, 2021). To minimise these negative impacts, the use of microorganisms located in the rhizosphere and in endophytic environments, such as bio-fertilisers, has received increased attention (Atieno et al., 2020). Beneficial plant–bacteria communities have been studied for many years (Pieterse et al., 2012; Zipfel and Oldroyd, 2017; Afzal et al., 2019; Ke et al., 2021; Song et al., 2021; Zhang et al., 2021). There are different bacteria in a direct interconnection with the plant cells; therefore, they might produce a direct beneficial effect (Hallmann et al., 1997). Different plant species are colonised by a great diversity of endophytic bacteria, such as Pseudomonas, Bacillus, Enterobacter, Burkholderia, and Azospirillum (Lodewyckx et al., 2002; Weyens et al., 2009).
The range of endophytic bacteria depends on the plant species and crop conditions (Zinniel et al., 2002; Ulrich et al., 2008). Endophytic bacteria colonise the intracellular and intercellular spaces of plant tissues and promote plant growth (Ulrich et al., 2008; Lugtenberg and Kamilova, 2009; Vacheron et al., 2013; Hardoim et al., 2015; Santoyo et al., 2016; Gouda et al., 2018). The procedures used by endophytic bacteria to boost plant growth constitute an essential step in the endophytic bacteria applications (Wani et al., 2015). However, the process by which endophytic bacteria enhance plant growth are not well understood (Hardoim et al., 2008; Marasco et al., 2012; Rashid et al., 2012; Bruto et al., 2014). Important evidence suggests that there is a relationship between genes involved in beneficial features (Bruto et al., 2014). The useful functions comprise the bacterial ability to enhance nutrient acquisition, fix nitrogen, acquire iron, and produce siderophores in plant species (Zhang et al., 2009; Hardoim et al., 2015; Zhou et al., 2016).
Furthermore, endophytic bacteria enhance plant growth through the production of different phytohormones. Phytohormones produced during endophytic colonisation process improved plant nutrient absorption and biomass (Gravel et al., 2007; Phetcharat and Duangpaeng, 2012; Shi et al., 2014). Plant growth regulators such as cytokines, ethylene, abscisic acid (ABA), gibberellins, and indole-3-acetic acid (IAA) are produced by endophytic bacteria during interactions with plants (Vacheron et al., 2013). Interestingly, IAA showed a crucial effect on plant growth and development processes. Modulating IAA is a notable hallmark through which the endophytic bacteria might strengthen plant growth. In addition, ethylene constitutes an essential plant hormone involved in different developmental and physiological processes (Sun et al., 2016). The enzyme 1-aminocyclopropane-1-carboxylate (ACC) deaminase precursor of ethylene is produced by endophytic bacteria (Sun et al., 2009). The hydrolysis of ACC improved plant growth under stress conditions (Santoyo et al., 2016). Plant growth-promoting endophytic bacteria displayed ACC deaminase activity (Nikolic et al., 2011; Zhang et al., 2011; Rashid et al., 2012). These bacteria can produce ACC deaminase to enhance plant growth. Mutation of the ACC deaminase gene compromised the canola growth (Sun et al., 2009). Moreover, diverse endophytic bacterial strains have the ability to release volatile organic compounds such as acetoin, pentadecane, 2,3-butanediol, 1 hexanol, and indole, which enhance plant growth (Ryu et al., 2003; Gutiérrez-Luna et al., 2010; Blom et al., 2011; Hernández-Calderón et al., 2018).
Plant endophytic bacteria have great potential to be used as bio-fertilisers. Although different types of bacterial species were identified from a broad plant species range, the action of these bacteria is commonly unstable under field conditions (Afzal et al., 2019). The poor understanding of the tricky events that control this alliance at the molecular level might be the reason. However, it is possible to obtain a better understanding through the evaluation of the both bacterial and plant genes expressed during the interaction (Afzal et al., 2019). The development of RNA-sequencing techniques has allowed the identification of transcripts involved in plant growth. However, few studies have investigated the endophytic bacterial diversity in unexplored wild plant species. Wild plant species survive in different environments and under diverse stress conditions. Identification of endophytic bacteria isolated from these species might allow the use of diverse mechanisms in these bacteria to promote plant growth (Afzal et al., 2019). The present study aimed to isolate, identify, and characterise endophytic bacteria from wild plant species and characterise their plant growth-promoting properties and the main molecular events involved in endophytic bacteria–plant interactions.
Materials and Methods
Isolation of Endophytic Bacterium
The samples were collected from wild plant species Glyceria chinensis (Keng) localised along the margin of the Fu Tuan River (35°20′17″N, 119°26′8″E) within 5 km1 of the coastal region of Rizhao City in Shandong Province (People’s Republic of China). The wild plant species was classified according to data on morphological traits from the Flora of China.2 This experimental study complies with Chinese national and local laws, and sample collection has been permitted by the Rizhao administration and Municipal Sciences and Technology Department [Collection information: South China Botanical Garden (IBSC) of the Chinese Academy of Sciences. Source: China Digital Plant Specimens Museum. Identifier: 0114164. Collector: Zhang Zhisong Acquisition number: 401467]. A total of 100 samples were collected randomly during the spring season. Firstly, the plant material (stems and roots) was rinsed with water. The samples were cut into small pieces under aseptic conditions. Each sample was surface sterilised with 70% ethanol for 1 min and immersed in a sodium hypochlorite solution (5%) during 1 min. Subsequently, the samples were washed in sterile distilled water during 1 min and dried on filter paper. After proper drying, pieces of plant parts were physically treated in a TissueLyser (Qiagen, Hilden, Germany) during 5 min in 1 ml of sterile water. The debris was decanted and 100 μl of the remaining water was incubated in Luria–Bertani (LB) agar medium (yeast extract, 5 g/L: peptone, 10 g/L; sodium chloride, 5 g/L; agar, 12 g/L; pH 7) at 37°C for 3 days. Parallel to the samples, the final wash solution of the surface sterilisation procedure was also spread plated onto the MS medium plate which served as a control. The bacteria were only isolated from processed samples internally. This was the criteria to classify as endophytes and not the surface contaminates. Bacterial colonies were selected based on growth rate, colony morphology, and pigmentation. Bacterial isolates were selected and purified by streaking procedure. These were incubated at 37°C. Pure cultures of the bacterial strains were maintained in 30% glycerol at −80°C.
Plant Materials and Growth Conditions
Arabidopsis thaliana ecotype Columbia plants were used in the experiments. First, surface-sterilised seeds were plated on Murashige and Skoog (0.5 × MS) basal media (Sigma Aldrich, St. Louis, MO, United States) supplemented with 1% w/v sucrose. The seeds were stored at 4°C in the dark for 2 days and transferred to a controlled growth room under a 16-h light/8-h dark photoperiod at 22°C. Small plants were transplanted to a mixture of soil composed of peat plugs and vermiculite in a 1:1 ratio during 14 days. In addition, Nicotiana tabacum, corn, and soybean seeds were germinated and the plants grown in pots (6”) containing sterilised black turf and rice husk (4:1) substrate and maintained in a controlled growth room at 23°C.
Identification of Endophytic Bacterium
The DNA from endophytic bacterium was extracted according to Sambrook et al. (1989). The 16S rRNA gene was used for molecular identification. A fragment of 16S rRNA gene was amplified by polymerase chain reaction (PCR) using the forward primer 27F 5′-AGAGTTTGATCCTGGCTCAG-3′ and reverse primer 1492R 5′-GGTTACCTTGTTACGACTT-3′. The amplification process was conducted in a Thermal Cycler T100 machine (Bio-Rad Life Science Research, Shanghai, People’s Republic of China) using a Taq PCR Master Mix Kit (Qiagen, Hilden, Germany). The PCR reaction was as follows: 95°C for 10 min; 35 cycles of 95°C for 30 s, 55°C for 1 min, and 72°C for 1.5 min; and final extension at 72°C for 10 min. The purified PCR fragment was sequenced using an ABI 3730 DNA sequencer (Applied Biosystems, ThermoFisher Scientific, Chino, CA, United States). The 16S rRNA gene fragment sequence (1,147 bp) was identified using BLASTN homology searches (Altschul et al., 1997). The National Center for Biotechnology Information (NCBI) GenBank, European Molecular Biology Laboratory–European Bioinformatics Institute (EMBL–EBI), and taxonomically united database of 16S rRNA EzBioCloud2 were used as databases (Yoon et al., 2017; De Los Santos Villalobos et al., 2019; Ha et al., 2019). Additionally, the full 16S rRNA gene (1,544 bp), glycosyl hydrolase lipoprotein, and SDR family oxidoreductase genes sequenced through RNA sequencing were used to an accurate identification. The phylogenetic tree was done using the neighbour-joining method and the full 16S rRNA (Saitou and Nei, 1987).
Greenhouse Experiments
Seeds pre-germinated from A. thaliana, N. tabacum, corn, and soybean were treated with endophytic bacterial fermentation twice weekly for 1 month. The selected bacterial strain was incubated in 100 ml of LB broth medium in a 250-ml Erlenmeyer flask with shaking (200 rpm) for 1 day at 37°C in the dark. The optical density (OD) from endophytic bacterial fermentation was adjusted to 1.0 (4.77 × 109 CFU/ml), and 30 ml of the fermentation was applied per pot. Each plastic pot received three pre-germinated seeds, and the plants were grown in a controlled growth room at 25°C and irrigated with water without any fertilisers. A completely randomised pot experiment with five replicates for each treatment was done to analyse the influence of the endophytic bacterium on the growing of different plant species. The substrate contained black turf and rice husks (4:1). The substrate was sterilised at 120°C during 20 min. Additionally, the roots of the plants inoculated with the endophytic bacterium used in the experiments were processed, and the bacterium was again isolated and classified as the original strain using the same protocol mentioned above. In Arabidopsis plants, the rosette diameter, leaf size, root size, and fresh weight parameters were evaluated after 1 month of treatment with endophytic bacterial fermentation. In addition, colony-forming units from endophytic bacteria were determined after 1 month of treatment. Plant size and fresh weight were evaluated in N. tabacum, corn, and soybean plants after 1 month of treatment. Data were analysed using GraphPad Prism software (La Jolla, CA, United States). Significant differences among the mean values were determined using t-test at p < 0.05. Five replicates were used for each treatment. The experiments were replicated twice.
Identification of New Genes Using RNA Sequencing
The Arabidopsis plants were treated with aliquots of 30 ml (4.77 × 109 CFU/ml) of endophytic bacterium. The bacterium was inoculated in the sterilised substrate around the plants (5-day-old). The colonisation of bacterium in the roots was verified using the same protocol used in the isolation of this strain from wild plant species on LB plates at different time points. The endophytic bacterium was well established 72 h post-inoculation. Leaves, stems, and roots from five plants were collected 72 h post-inoculation. Plants treated with water were used as control. Treatments and controls were repeated three times per group. The total RNA was extracted using the Qiagen RNeasy Midi Kit (Hilden, Germany), and the concentration of the total RNA was determined using spectrometry. The treatment and control samples were repeated three times per group. After extracting the total RNA, eukaryotic mRNA was enriched using oligo(dT) beads. The samples were sequenced using Illumina HiSeqTM 2000 (Personalbio Co., Shanghai, People’s Republic of China). High-quality reads were processed using the Perl script, and the differentially expressed genes were identified using the edgeR package.3 Genes with a fold change ≥2 were considered significant differentially expressed genes. Gene ontology (GO) and the Kyoto Encyclopedia of Genes and Genomes (KEGG) pathway enrichment analysis were used to characterise the differentially expressed genes. GO functional annotations were obtained from the non-redundant annotation results. In addition, the GO annotations were analysed using the Blast2GO software4 (Conesa et al., 2005). The RNA sequencing was done following these steps: (1) total RNA extraction; (2) total RNA quality test; (3) rRNA removal; (4) RNA fragmentation between 200 and 300 bp by ion disruption; (5) PCR: the first chain of cDNA was synthesised by random primers and reverse transcriptase; when the second chain of cDNA was synthesised, the library of chain-specific components was constructed, and dTTP was replaced by dUTP, which greatly improved the accuracy of the results. (6) PCR enrichment of library fragments: the size of the library ranged from 300 to 400 bp. (7) Library quality inspection: the size of the library was detected by Agilent 2100 Bioanalyzer, and the total concentration of the library was detected by fluorescence quantitative analysis. (8) Illumina sequencing: the best amount of sample was selected for PCR amplification. (9) Annealing of sequencing at the same time of synthesis: the prokaryote genetic analysis process firstly filtered the raw off-machine data to obtain a high-quality sequence, comparing the filtered sequence with the species reference genome. Based on the results of the comparison, the amount of expression of each gene was calculated. On this basis, the expression difference analysis, enrichment analysis, and cluster analysis of the sample were further carried out. The transcription structure of the sample was analysed and the manipulative substructure of the sample gene obtained. UTR, SNP, and InDel were obtained. HTSeq 0.6.1p25 was used to compare the read count values from each gene as the original expression of the gene. To make gene expression levels comparable between different genes and samples, we normalised the sequencing depth and gene length using fragments per kilobase of transcript per million (FPKM). FPKM calculates the number of fragments that two reads can compare to the same transcript. In the reference transcription group, we generally took into account the FPKM >1 when the gene was expressed. Pearson correlation coefficients were used to indicate the level of expression correlation between genes in the sample to test the reliability of the experiments and whether sample selection was reasonable. The level correlation of gene expression between samples was an important index to test the reliability of the experiments and whether sample selection was reasonable. The log2 fold change was calculated by dividing the FPKM values from the bacterium during interaction with Arabidopsis (SB001) and the bacterium without interaction with Arabidopsis (B001).
Results
Isolation and Identification of the Endophytic Bacterium Bacillus altitudinis
Six endophytic bacteria strains were isolated from wild plant species. The ability of the six isolated endophytic bacteria to colonise and persist in wild plant species was tested. Of these six, only one isolate (SB001) colonised this plant species at levels ranging from 9.40 × 102 CFU/cm fresh root compared with the other five strains, where the level was lower (Table 1). Based on the levels of colonisation, the isolated endophytic strain SB001 was selected for further assays.
The isolate SB001 showed a small rod-shaped structure and Gram-positive spore-forming bacterium distinctive of the genus Bacillus. The bacterium strain was identified using the partial (1,147 bp) and full (1,544 bp) 16S rRNA gene. Using the taxonomically united database of 16S rRNA in EzBioCloud, the full 16S rRNA was definitively identified as a top hit with Bacillus altitudinis with 100% similarity. Partial 16S rRNA sequences had an ambiguous 96.94% of similarity with B. altitudinis. Additionally, glycosyl hydrolase lipoprotein and SDR family oxidoreductase genes confirmed the identification of B. altitudinis (Supplementary Table 1). Considering the identification, the phylogenetic tree was constructed by comparing the full 16S rRNA gene sequences of the isolate SB001 with the reference strain sequences from the NCBI GenBank public database. Molecular analysis indicated that the isolated strain SB001 belongs to the genus Bacillus showing an identity percentage of 100% and E-value of 0.0 with B. altitudinis strain W3 (Figure 1).
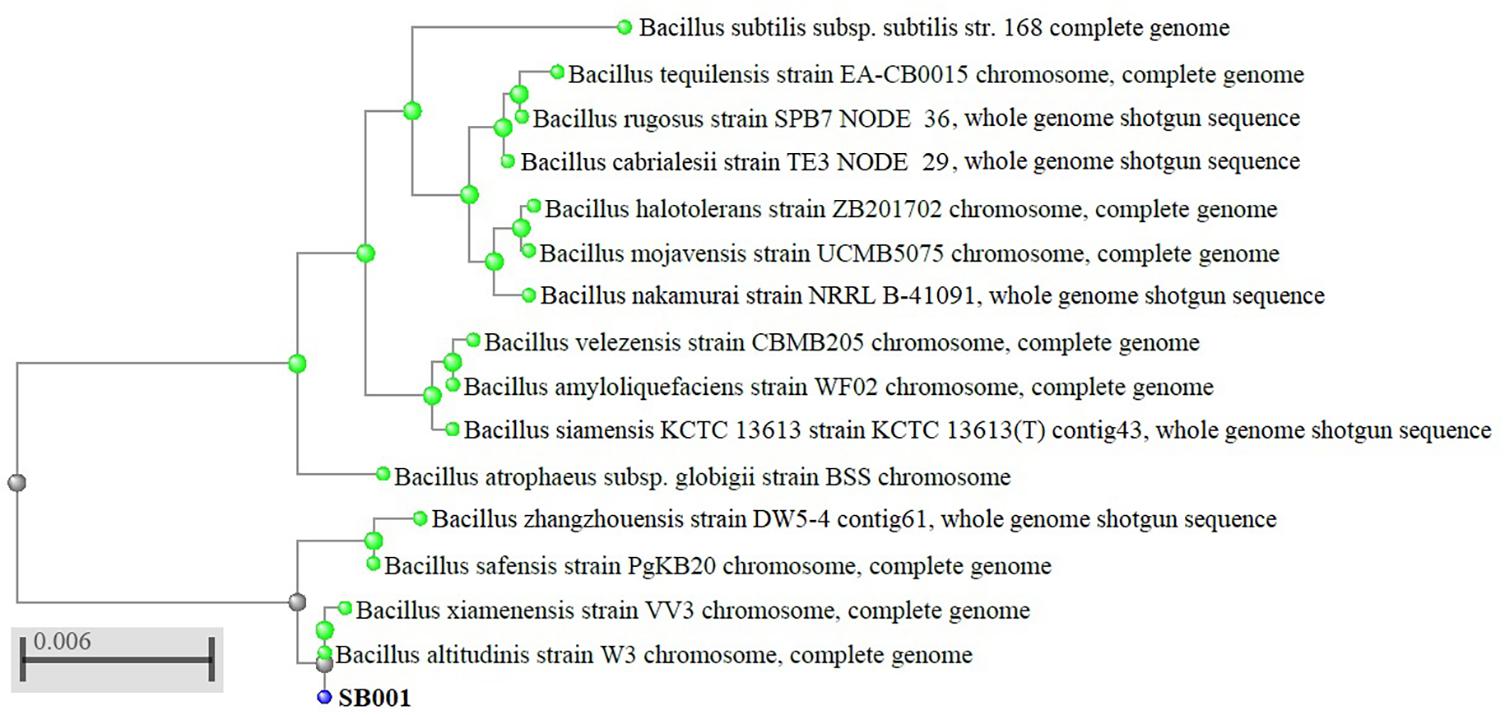
Figure 1. Phylogenetic tree of 16S rRNA sequences from endophytic bacterium strain (SB001) compared with representative members of Bacillus genus with more than 98% of identity.
Bacillus altitudinis Strain Promotes the Growth of Different Plant Species
The endophytic strain was also evaluated for plant growth promotion in different plant species. There was significant growth in Arabidopsis plants treated with the endophytic bacterium B. altitudinis (Figure 2A). The rosette diameter (Figure 2B), leaf size (Figure 2C), and fresh weight (Figure 2E) were considerably increased by inoculation with the B. altitudinis strain compared with the control in Arabidopsis plants at 20 days. However, endophytic bacteria did not influence root size (Figure 2D). Nevertheless, endophytic bacteria were suitably established in Arabidopsis roots (Figure 2F).
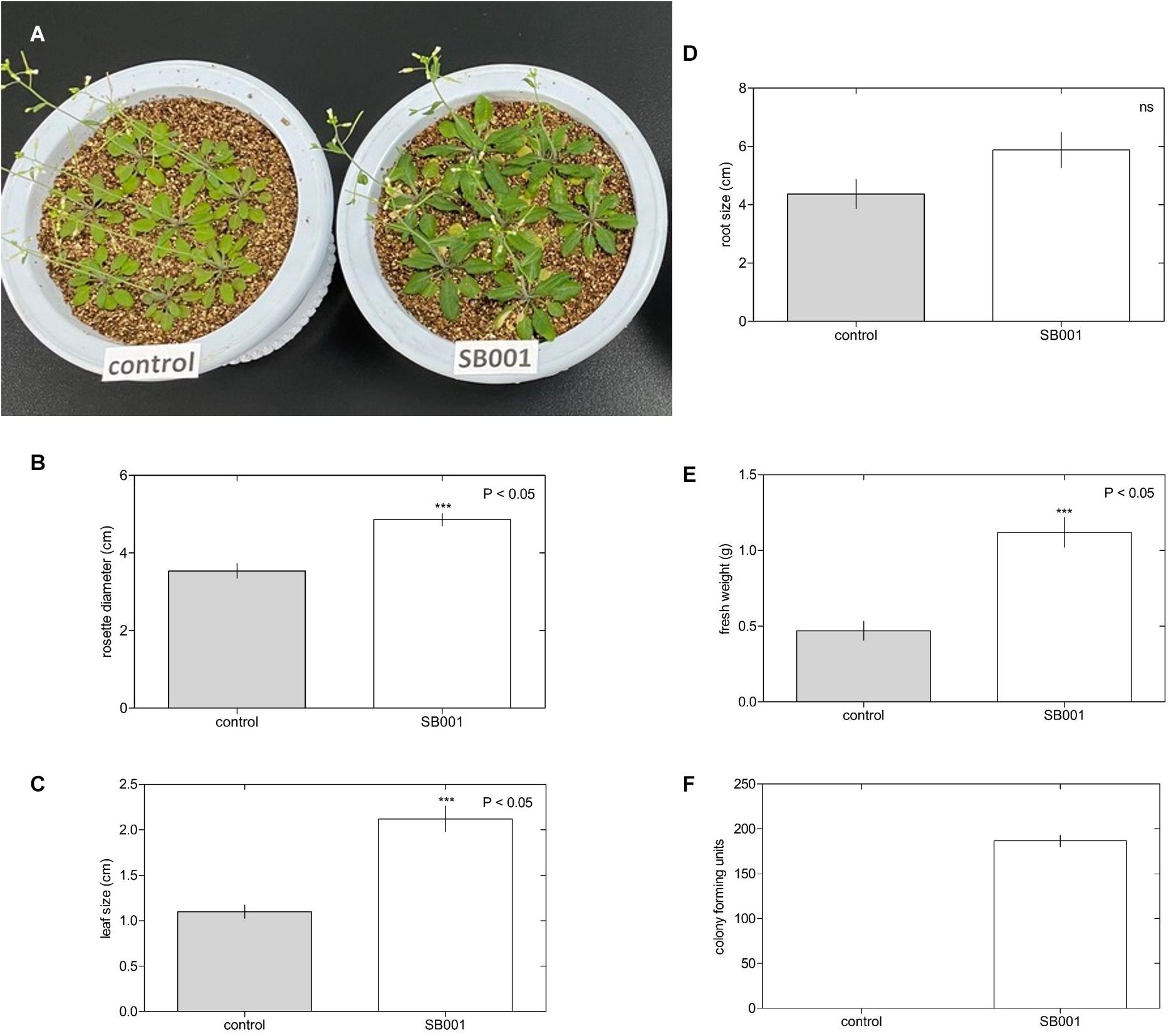
Figure 2. Endophytic bacterium B. altitudinis induces significant Arabidopsis plant growth. Plants (5-day-old) were mock-treated (control) or inoculated with 30 ml of B. altitudinis at 4.77 × 109 CFU/ml. (A) Phenotype of Arabidopsis plants mock-treated (control) and treated with B. altitudinis fermentations (SB001) at 20 days post-inoculation. Effect on the rosette diameter (B), leaf size (C), root size (D), and fresh weight (E) of Arabidopsis plants treated with B. altitudinis fermentations (SB001). (F) Colony-forming units were determined in Arabidopsis plants mock-treated (control) and treated with B. altitudinis fermentations (SB001). Each bar represents mean values with standard errors of two independent experiments (n = 10). ***P ≤ 0.001.
The B. altitudinis strain significantly stimulated the growth of N. tabacum plants (Figure 3A) and showed a substantial influence on plant size (Figure 3B) and fresh weight (Figure 3C) in N. tabacum plants. Inoculation with the B. altitudinis strain considerably enhanced the growth of maize plants with respect to non-inoculated plants (Figure 4A). Compared with the non-inoculated plants, the endophytic strain increased the plant size (Figure 4B) and fresh weight (Figure 4C) in inoculated plants. However, the endophytic strain had no significant influence on soybean plant growth (Figure 5). Overall, these results revealed that B. altitudinis improved the vegetative growth of different plant species following inoculation.
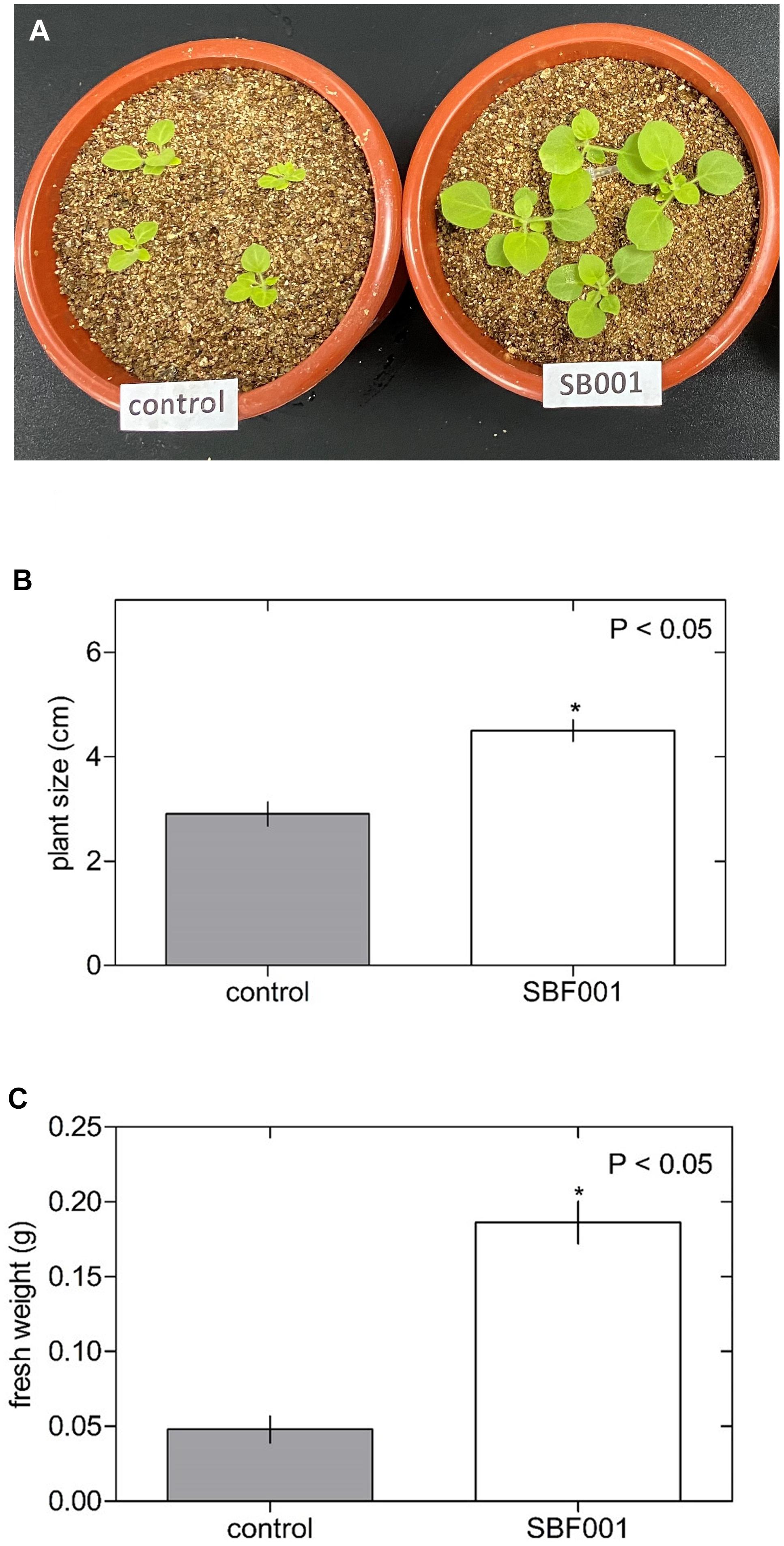
Figure 3. Endophytic bacterium B. altitudinis induces significant Nicotiana tabacum plant growth. Plants (5-day-old) were mock-treated (control) or inoculated with 30 ml of B. altitudinis at 4.77 × 109 CFU/ml. (A) Phenotype of N. tabacum plants mock-treated (control) and treated with B. altitudinis fermentations (SB001) at 20 days post-inoculation. Effect on plant size (B) and fresh weight (C) of N. tabacum plants mock-treated (control) and treated with B. altitudinis fermentations (SB001). Each bar represents mean values with standard errors of two independent experiments (n = 10). *P ≤ 0.05.
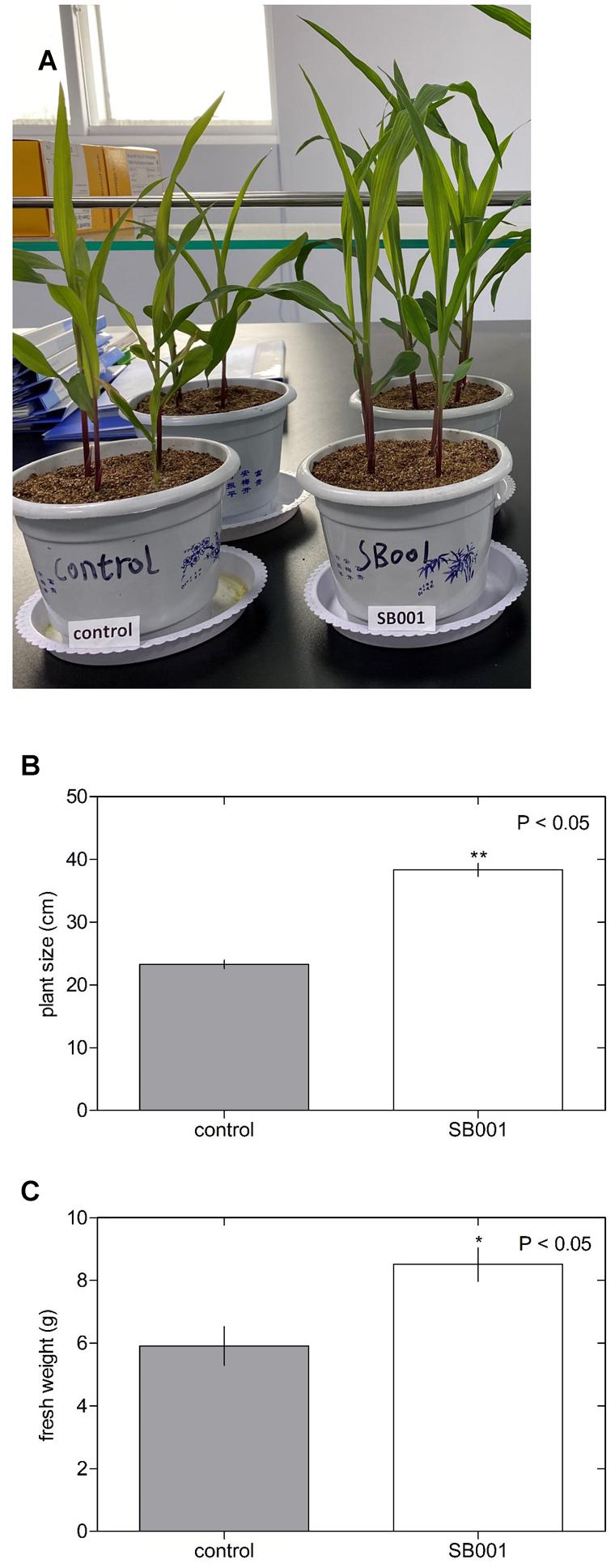
Figure 4. Endophytic bacterium B. altitudinis induces significant maize plant growth. Plants (5-day-old) were mock-treated (control) or inoculated with 30 ml of B. altitudinis at 4.77 × 109 CFU/ml. (A) Phenotype of maize plants mock-treated (control) and treated with B. altitudinis fermentations (SB001) at 20 days post-inoculation. Effect on plant size (B) and fresh weight (C) of maize plants mock-treated (control) and treated with B. altitudinis fermentations (SB001). Each bar represents mean values with standard errors of two independent experiments (n = 10). *P ≤ 0.05; **P ≤ 0.01.
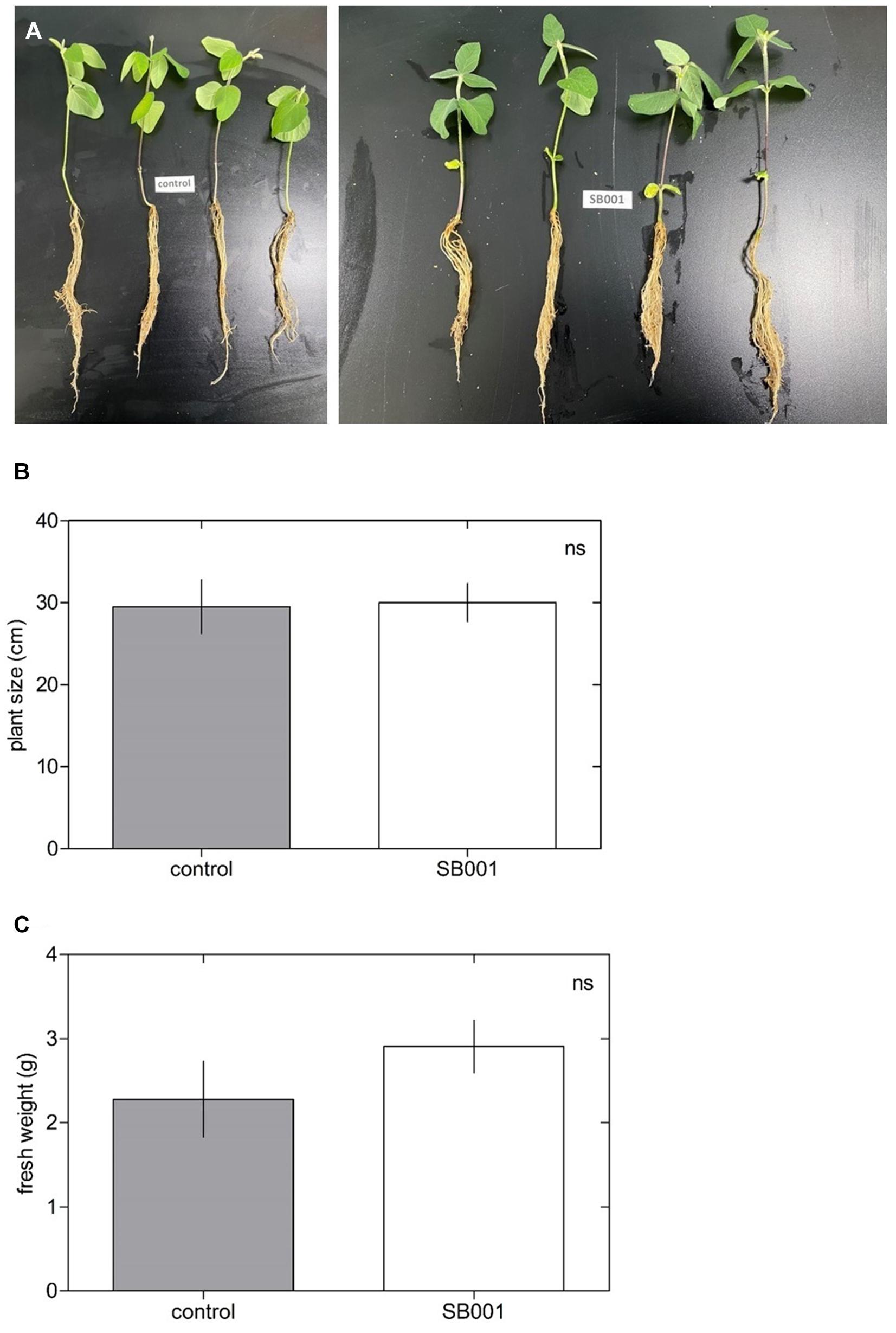
Figure 5. Endophytic bacterium B. altitudinis does not induce soybean plant growth. Plants (5-day-old) were mock-treated (control) or inoculated with 30 ml of B. altitudinis at 4.77 × 109 CFU/ml. (A) Phenotype of soybean plants mock-treated (control) and treated with B. altitudinis fermentations (SB001) at 20 days post-inoculation. Effect on plant size (B) and fresh weight (C) of soybean plants mock-treated (control) and treated with B. altitudinis fermentations (SB001). Each bar represents mean values with standard errors of two independent experiments (n = 10).
Bacillus altitudinis Induces Transcriptional Changes in Genes Involved in Plant Growth
RNA-seq was used to evaluate the interactions between B. altitudinis and Arabidopsis. To generate RNA-seq data sets, we prepared cDNA libraries from mock-inoculated and inoculated with the endophytic bacterium B. altitudinis from Arabidopsis plant RNA and sequenced them on two separate flow cells. The range of gene expression during the B. altitudinis–Arabidopsis interaction was determined. During the interaction, 27,628 transcripts were identified and annotated (Supplementary Table 2). From this, 7,379 new transcripts were annotated (Supplementary Table 3). The highest number of transcripts (33.57%) showed an expression level change of between 1- and 10-fold. The lowest number of transcripts was distributed at the highest expression level between >100-fold change (Figure 6). Analysis of RNA-seq data during the interaction revealed 368 differentially expressed transcripts, which included 256 upregulated and 112 downregulated transcripts (Figure 7). Among them, maturase K, tetratricopeptide repeat (TPR)-like superfamily protein, LOB domain-containing protein, and BTB/POZ and TAZ domain-containing proteins showed the highest gene differential expression during B. altitudinis–Arabidopsis interaction. Trihelix transcription factor (TF) ASR3, TF MYB90, and O-methyltransferase family proteins were significantly repressed during the interaction (Table 2 and Supplementary Table 4).
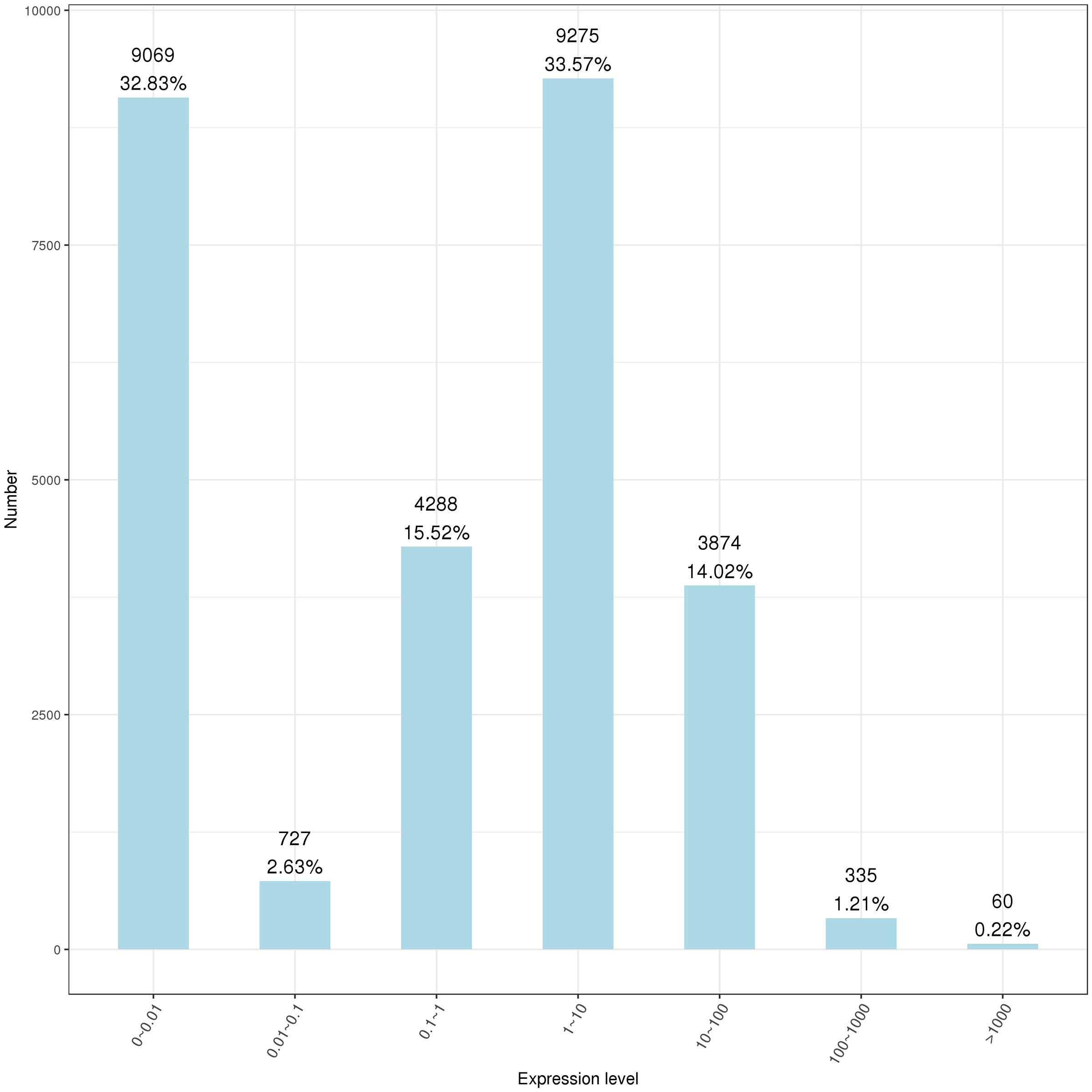
Figure 6. Statistics showing the range of plant gene expressions during the B. altitudinis–Arabidopsis interaction. Horizontal coordinates represent the range of expression measures, and ordinates represent the number of genes in each expression interval.
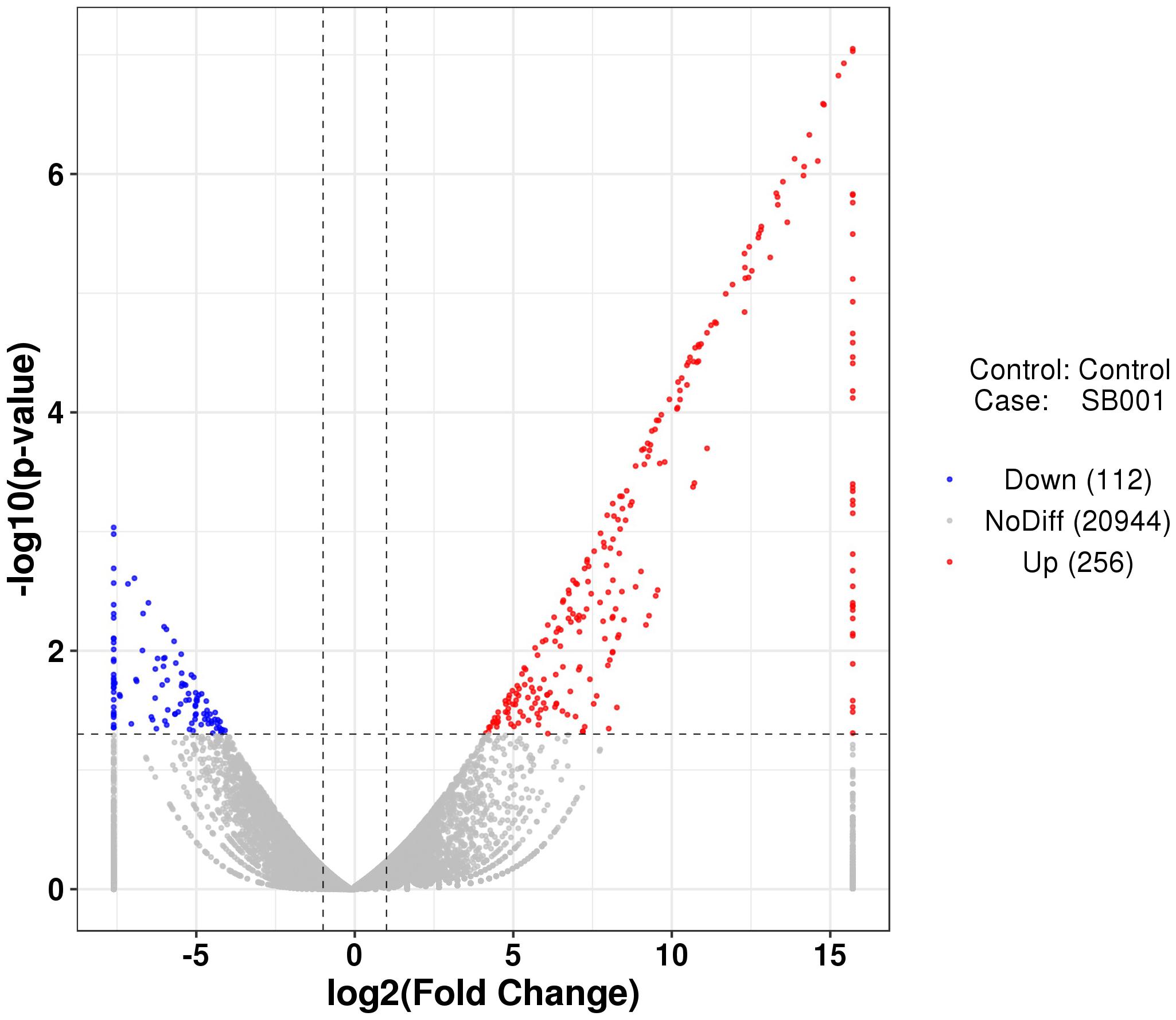
Figure 7. Volcanic diagrams of differential expression genes. The horizontal coordinates are log2 (fold change) and the ordinates are -log10 (p-value). The two vertical dashed lines are 2 times the difference threshold, and the horizontal dashed lines are p-value 0.05 thresholds. A red dot indicates the upregulated genes group, a blue dot indicates the downregulated genes group, and a grey dot represents a non-significant difference in the expression of the genes group. The scale was fixed considering the minimum and maximum log2 values (between -7.60 and 15.70) included in the Supplementary Table 4.
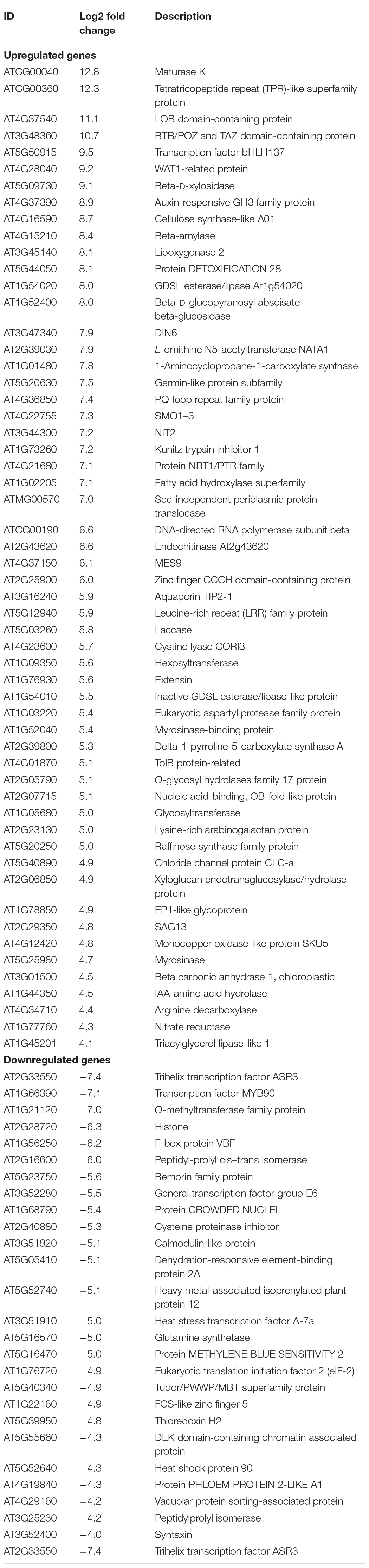
Table 2. Most important differentially expressed genes in Arabidopsis plants inoculated with B. altitudinis.
Differentially expressed transcripts were subjected to functional analyses. GO enrichment analysis was performed using the annotated difference gene to calculate the list of genes and the number of genes for each term. The transcripts with the largest increases in expression were NADH dehydrogenase (quinone), NADH dehydrogenase (ubiquinone) activity, NADH dehydrogenase, and oxidoreductase (Figure 8A). Based on the KEGG pathway, we categorised the most significant transcripts into nitrogen metabolism and oxidative phosphorylation pathways (Figure 8B and Supplementary Table 5). The expression profile of TF genes displays the upcoming transcription actions regulated by these genes. To determine their important roles, the TFs involved in the B. altitudinis–Arabidopsis interaction were analysed. The largest TF families detected in our study were basic helix-loop-helix (bHLH) DNA-binding superfamily protein, TF MYB13 (MYB), ethylene responsive factor (ERF), NAC domain-containing protein 1 (NAC), and zinc finger protein ZAT1 (C2H2), respectively (Figure 9).
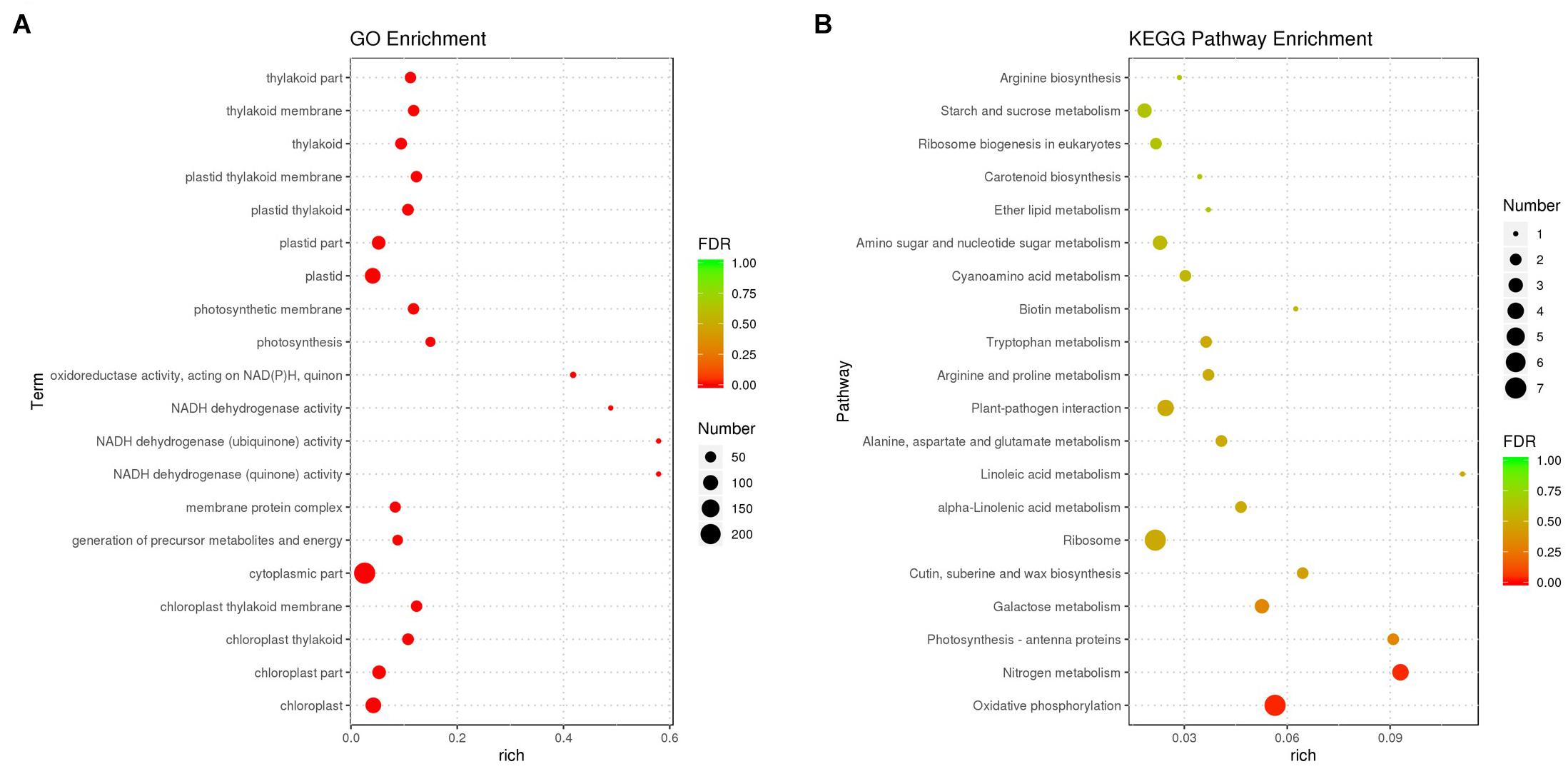
Figure 8. Gene ontology (GO) (A) and KEGG pathway-rich analysis (B). Based on the GO-rich and KEGG results, the extent of richness is measured by the rich factor, false discovery rate (FDR) values, and the number of genes that were collected into this GO term or KEGG pathway. Rich factor refers to the ratio of the number of different genes collected in the GO term or KEGG pathway to the number of genes annotated.
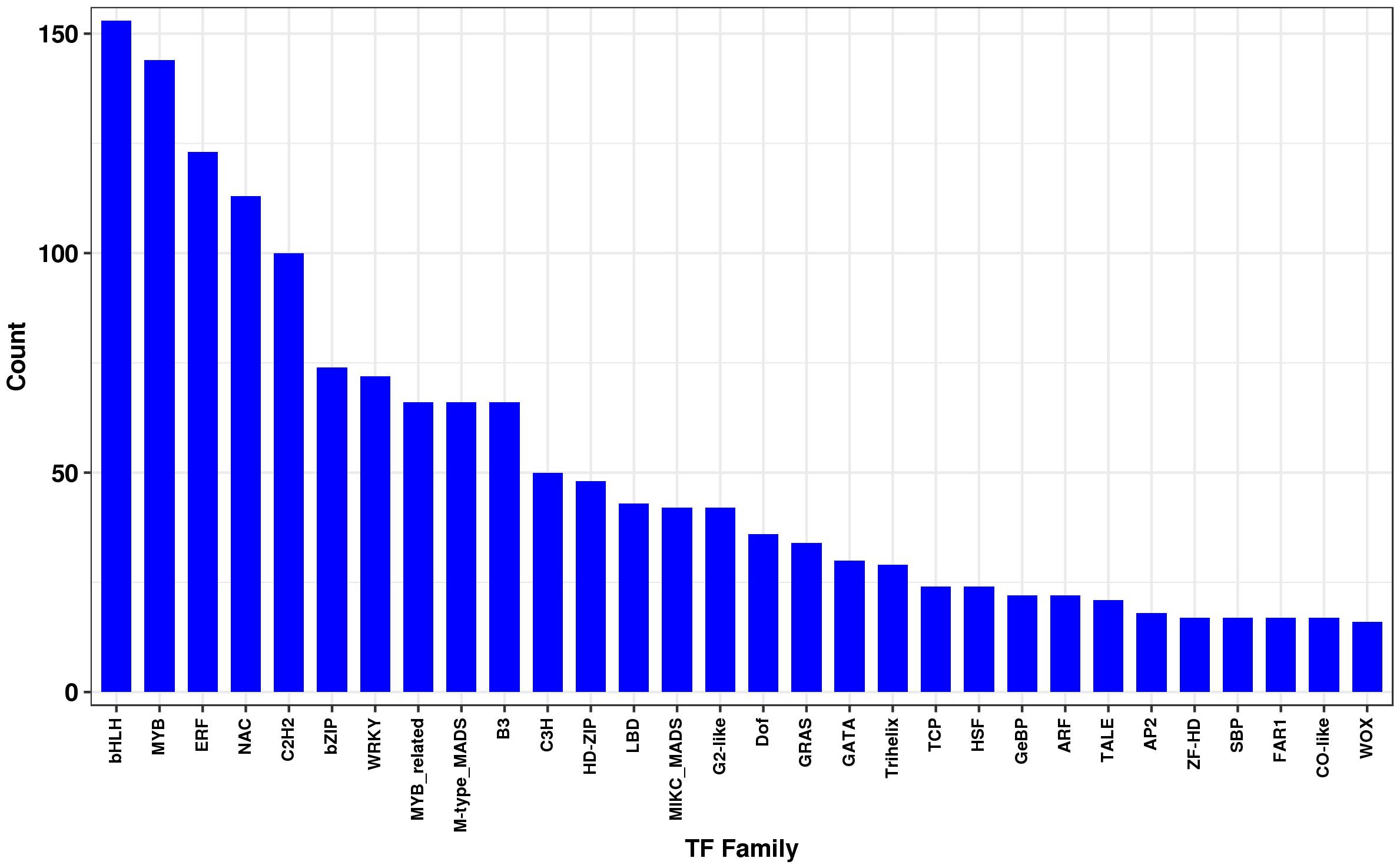
Figure 9. Transcription factor family. The horizontal coordinates are different transcription factor families, and the ordinates are the number of genes that fall into that transcription factor family.
During the interaction, a total of 3,413 genes were annotated from B. altitudinis. From these, genes with a log2 fold change between −1.2 and 5.3 were analysed. Twenty-two and 8 B. altitudinis genes were up- and down-regulated during the interaction with Arabidopsis plants, respectively. The rest of these genes were undefined, repeated, low significant fold change, or involved in the primary metabolism of the bacterium. The major facilitator superfamily (MFS) transporter, sucrose-6-phosphate hydrolase, phosphate ABC transporter, DNA gyrase subunit B, polysaccharide biosynthesis protein, serine phosphatase, and flagellar biosynthesis protein had the highest expression levels in B. altitudinis during the interaction with Arabidopsis plants. Moreover, elongation factors G, 2,3-butanediol dehydrogenase, dihydrolipoamide succinyltransferase, and proline dehydrogenase were highly downregulated in bacteria (Table 3 and Supplementary Table 6).
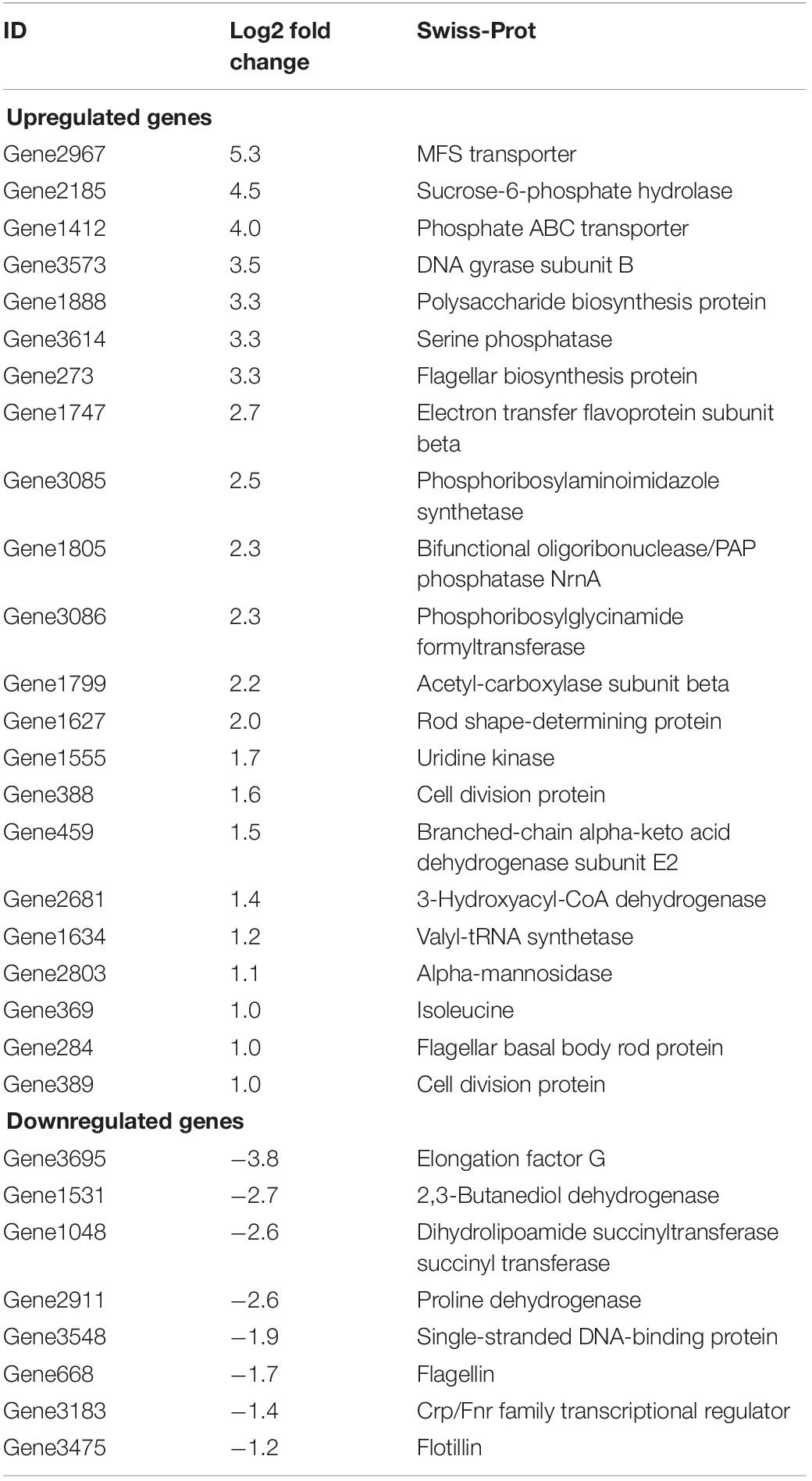
Table 3. Most important differentially expressed genes in B. altitudinis during the interaction with Arabidopsis plants.
Discussion
Different classes of microorganism have the potential to promote plant growth. The favourable effects of endophytic bacteria derived from the roots have been demonstrated. The significant reaction of these endophytic bacteria on plant growth has been achieved by both direct and indirect processes. Direct effects comprise the production of molecules that encourage plant growth (Goswami et al., 2016). In the current study, different endophytic bacteria strains were isolated from wild plant species. The SB001 strain was the most abundant in the inner tissues of the wild plant species. The strain was identified as B. altitudinis through morphological and molecular analyses.
Several plant growth promotion assays were conducted to determine whether SB001 could promote plant growth in different plant species under greenhouse conditions. Inoculated A. thaliana, N. tabacum, and maize plants exhibited growth improvement, and significant increases were observed for several growth variables, such as fresh weight and plant size, between the inoculated and non-inoculated control plants. However, this strain did not influence soybean growth. It is likely that the internal tissue environment of soybean roots is not suitable for the correct setting of the bacterium. Interestingly, B. altitudinis strain was well established in Arabidopsis roots, which constitutes important evidence of the ability of this strain to colonise internal tissues. Remarkably, this endophytic strain displayed vigorous plant growth-promoting effects in non-host plants. This result proposes that SB001 has the ability to efficiently enhance the growth of a broad range of plant species, and consequently, this might be used in applications under field conditions.
Earlier studies showed that several Bacillus species isolated from various hosts promote plant growth (Ashraf et al., 2004; Xie et al., 2014; Kuan et al., 2016; Pourbabaee et al., 2016; Radhakrishnan and Lee, 2016; Fouda et al., 2021). For example, B. insolitus, B. subtilis, and B. methylotrophicus increased the size and weight of shoots, leaves, and roots from diverse plant species (Ashraf et al., 2004; Radhakrishnan and Lee, 2016). Furthermore, B. subtilis and B. methylotrophicus induced the production of hormones such as IAA, cytokinins, gibberellic acid (GA), and spermidines to stimulate plant growth (Xie et al., 2014; Radhakrishnan and Lee, 2016; Fouda et al., 2021), whereas B. megaterium and B. methylotrophicus boosted endogenous proteins, amino acids, and minerals in plants (Kang et al., 2014; Radhakrishnan and Lee, 2016). Strain SB001 exhibited different plant growth-promoting properties, which were reflected in the enhanced vegetative growth of different plant species, although these properties under soil conditions need to be investigated. Due to its beneficial plant growth-promoting activities, the B. altitudinis strain may be used as a source of bio-fertiliser in sustainable agriculture.
Plant–harmless bacteria interactions have been studied for many years. However, the molecular events used and involved during the interaction are not well understood, which makes it difficult to use the advantages of these complex interactions under natural conditions. Endophytic bacteria positively impact plant growth and select beneficial bacterial colonisers (Hardoim et al., 2008; Marasco et al., 2012; Rashid et al., 2012). In this study, we conducted transcriptome sequencing of Arabidopsis–B. altitudinis interactions to identify and quantify the expression grades of transcripts. A large number of novel candidate genes related with primary and secondary metabolite biosynthesis were identified in our expressed sequence tag (EST) data set. The results show new understandings in the expression data of this endophytic bacterium, as well as a first insight into the gene expression patterns during Arabidopsis–B. altitudinis interaction. Moreover, the transcriptome information obtained in this study provides a significant contribution to gaining a suitable understanding of the molecular events implicated in the interaction.
Remarkably, GO and KEGG analyses showed significant changes, suggesting that the B. altitudinis strain had a considerable effect on the plant. Our data indicated that SB001 induces important pathways involved in plant growth. All the information about the molecular interactions in known metabolic and regulatory pathways are contained in the KEGG pathway database (Kanehisa and Goto, 2000). Interestingly, the maturase K (chloroplast-encoded splicing factor), TPR-like superfamily protein, LOB domain-containing protein, and BTB/POZ and TAZ domain-containing protein genes were highly induced for the first time during the Arabidopsis–B. altitudinis interaction.
Previously, maturase K gene expression was associated with developmental stage, etiolation, and regulation processes related with development and photosynthesis (Barthet and Hilu, 2007). Maturase K has influence in gene expression in plants and interferes with the proper expression of the photosynthetic mechanism (Qu et al., 2018). Indeed, the induction of the maturase K gene might have an effect on plant growth during the interaction, through an enhancing photosynthetic process. TPR proteins are important in plant hormone signalling, such as GA, cytokinin, and ethylene (Jacobsen et al., 1996; Wang et al., 2004; Greenboim-Wainberg et al., 2005; Yoshida et al., 2005). In addition, the TPR gene was a key positive regulator of ABA during Arabidopsis seedling development (Rosado et al., 2006).
Conversely, LOB domain-containing protein genes constitute a gene family that encodes plant-specific TFs that play key functions during plant growth (Zhang et al., 2020). These proteins are essential TFs that regulate plant organ development, photomorphogenesis (Mangeon et al., 2011), petiole development (Ge et al., 2014), hormone response (Zentella et al., 2007), and metabolism regulation (Rubin et al., 2009). Additionally, BTB/POZ and TAZ domain-containing protein genes are required during gametophyte development (Robert et al., 2009). This is an essential element of a signalling network that recognises and responds to diverse stimuli (Mandadi et al., 2009). Furthermore, the TF MYB90 is repressed during the interaction. Previously, it was found that heterologous expression of the TF AtMYB90 increased the anthocyanin production and inhibited the tomato plant growth (Li et al., 2018). The endophytic bacterium SB001 was capable of promoting growth through activation of important pathways implicated in plant growth, including the synthesis of phytohormones and TFs.
The TFs constitute crucial functional factors involved in the regulation of gene expression (Jiao et al., 2003). Numerous processes such as brassinosteroid signalling, ABA signalling, and axillary meristem formation are regulated by bHLH family members during seedling development (Zhao et al., 2012). A total of 153 transcripts were identified as bHLH TF proteins, and these constituted the largest TF family during the interaction. In addition, the bHLH proteins are a phytochrome interacting factor (Toledo-Ortiz et al., 2003).
The MYB proteins were involved in regulatory networks controlling development and metabolism of a diversity of plant species (Dubos et al., 2010). MYB115 and MYB118 had a key part in embryogenesis in Arabidopsis plants (Wang et al., 2009). Also, MYB38 and MYB18 regulate hypocotyl elongation in response to blue (Hong et al., 2008) and far-red light, respectively (Yang et al., 2009). Other MYB proteins, such as MYB58, MYB63, MYB85, MYB68, and MYB46, were related in the regulation of cell wall biosynthesis (Zhou et al., 2009). In this study, 144 putative MYB genes were detected during Arabidopsis seedling development.
The large NAC TF family was involved in a diversity of developmental events in Arabidopsis and soybean (Shamimuzzaman and Vodkin, 2013). This NAC TF family has the capacity to permit crosstalk between different pathways (Olsen et al., 2005). Furthermore, C2H2 zinc finger protein genes play a significant part in the development and differentiation (Razin et al., 2012). During the interaction, 100 putative C2H2 zinc finger genes were identified. In addition, ethylene responsive factor (ERF) TFs belong to the Apetala2/ERF) TF family involved in plant growth.
Interestingly, the MFS transporter and DNA gyrase subunit B protein genes were highly expressed in B. altitudinis during the interaction with the plant, which constitutes the first evidence of the expression of these types of genes in this species. The MFS transporter facilitates the transport of diverse molecules such as sugars, vitamins, amino acids, and hormones across cell membranes. These proteins are in different prokaryote and eukaryote organisms (Pao et al., 1998). It is likely that the high expression of this gene allows the transport of important hormones involved in plant growth. Silencing of the A. thaliana gyrase-A gene was embryo-lethal, whereas silencing of gyrase-B genes led to severely affected growth and development (Wall et al., 2004). We speculate that the overexpression of the gyrase gene in B. altitudinis might enhance plant growth.
Conclusion
Here, we report a new B. altitudinis endophytic strain isolated from wild plant species. Our finding revealed that the endophytic bacterium induced a key group of genes associated with plant growth-promoting traits. We demonstrated that this strain has a favourable reaction on plant growth. Remarkably, our results suggest that maturase K, TPR-like superfamily protein, LOB domain-containing protein, and BTB/POZ/TAZ domain-containing protein might be involved for the first time in this process. Furthermore, the MFS transporter and DNA gyrase subunit B genes expressed in B. altitudinis may be implicated in the observed effects. Future studies should evaluate the direct functions of these genes in plant growth during interactions. In addition, the potential beneficial effects of B. altitudinis strain under natural conditions need to be further investigated. In the long term, the new finding would allow the development of suitable and effective strategies in sustainable agriculture.
Data Availability Statement
The data presented in the study are deposited in the (SRX11022601) repository, accession number (PRJNA733570).
Author Contributions
OB-H, HX, and XG conceived the study. OB-H and DZ designed the experiments. DZ, HX, JG, RP, LD, XG, CB, and OB-H did the experiments. DZ and OB-H assisted in the data analysis. OB-H wrote the manuscript. All authors contributed to the article and approved the submitted version.
Funding
This study was supported by the Special Funds for Guiding Local Science and Technology Development of Central Government of Shandong Province (No. YDZX20193700004362).
Conflict of Interest
DZ, HX, JG, RP, LD, XG, and OB-H were employed by the company Yota Bio-Engineering Co., Ltd. CB was employed by the company VBS Biotec SA, Mexico.
The remaining author declare that the research was conducted in the absence of any commercial or financial relationships that could be construed as a potential conflict of interest.
Supplementary Material
The Supplementary Material for this article can be found online at: https://www.frontiersin.org/articles/10.3389/fmicb.2021.692313/full#supplementary-material
Supplementary Table 1 | Identification of endophytic bacterium.
Supplementary Table 2 | Annotation Arabidopsis–B. altitudinis interaction.
Supplementary Table 3 | New transcript annotation Arabidopsis-SB001 interaction.
Supplementary Table 4 | Arabidopsis vs. B. altitudinis up and down regulated genes during the interaction.
Supplementary Table 5 | KEGG enrichment Arabidopsis–B. altitudinis interaction.
Supplementary Table 6 | B. altitudinis bacterium.
Footnotes
- ^ https://www.ezbiocloud.net
- ^ http://www.iplant.cn/foc/
- ^ www.r-project.org/
- ^ www.blast2go.com
- ^ http://htseq.readthedocs.io
References
Afzal, I., Shinwari, Z. K., Sikandar, S., and Shahzad, S. (2019). Plant beneficial endophytic bacteria: mechanisms, diversity, host range and genetic determinants. Microbiol. Res. 221, 36–49. doi: 10.1016/j.micres.2019.02.001
Altschul, S. F., Madden, T. L., Schaffer, A. A., Zhang, J., Zhang, Z., Miller, W., et al. (1997). Gapped BLAST and PSIBLAST: a new generation of protein database search programs. Nucleic Acids Res. 25, 3389–3402. doi: 10.1093/nar/25.17.3389
Ashraf, M., Hasnain, S., Berge, O., and Mahmood, T. (2004). Inoculating wheat seedlings with exopolysaccharide-producing bacteria restricts sodium uptake and stimulates plant growth under salt stress. Biol. Fertil. Soils 40, 157–162. doi: 10.1007/s00374-004-0766-y
Atieno, M., Herrmann, L., Nguyen, H. T., Phan, H. T., Nguyen, N. K., Srean, P., et al. (2020). Assessment of biofertilizer use for sustainable agriculture in the Great Mekong Region. J. Environ. Manag. 275:111300. doi: 10.1016/j.jenvman.2020.111300
Barthet, M. M., and Hilu, K. W. (2007). Expression of matK: functional and evolutionary implications. Am. J. Bot. 94, 1402–1412. doi: 10.3732/ajb.94.8.1402
Blom, D., Fabbri, C., Connor, E. C., Schiestl, F. P., Klauser, D. R., Boller, T., et al. (2011). Production of plant growth modulating volatiles is widespread among rhizosphere bacteria and strongly depends on culture conditions. Environ. Microbiol. 13, 3047–3058. doi: 10.1111/j.1462-2920.2011.02582.x
Bruto, M., Prigent-Combaret, C., Muller, D., and Moenne-Loccoz, Y. (2014). Analysis of genes contributing to plant-beneficial functions in plant growth-promoting rhizobacteria and related proteobacteria. Sci. Rep. 4:6261. doi: 10.1038/srep06261
Conesa, A., Götz, S., García-Gómez, J. M., Terol, J., Talón, M., and Robles, M. (2005). Blast2GO: a universal tool for annotation, visualization and analysis in functional genomics research. Bioinformatics 2, 3674–3676. doi: 10.1093/bioinformatics/bti610
De Los Santos Villalobos, S., Robles, R. I., Parra Cota, F. I., Larsen, J., Lozano, P., and Tiedje, J. M. (2019). Bacillus cabrialesii sp. nov., an endophytic plant growth promoting bacterium isolated from wheat (Triticum turgidum subsp. durum) in the Yaqui Valley. Mexico Int. J. Syst. Evol. Microbiol. 69, 3939–3945. doi: 10.1099/ijsem.0.003711
Dubos, C., Stracke, R., Grotewold, E., Weisshaar, B., Martin, C., Lepiniec, L., et al. (2010). MYB transcription factors in Arabidopsis. Trends Plant Sci. 15, 573–581.
Fouda, A., Eid, A. M., Elsaied, A., El-Belely, E. F., Barghoth, M. G., Azab, E., et al. (2021). Plant growth-promoting endophytic bacterial community inhabiting the leaves of Pulicaria incisa (Lam.) DC inherent to arid regions. Plants 10:76. doi: 10.3390/plants10010076
Ge, L., Peng, J., Berbel, A., Madueño, F., and Chen, R. (2014). Regulation of compound leaf development by PHANTASTICA in Medicago truncatula. Plant Physiol. 164, 216–228. doi: 10.1104/pp.113.229914
Goswami, D., Thakker, J. N., and Dhandhukia, P. C. (2016). Portraying mechanics of plant growth promoting rhizobacteria (PGPR). Cogent Food Agric. 2, 1–19. doi: 10.1080/23311932.2015.1127500
Gouda, S., Kerry, R. G., Das, G., Paramithiotis, S., Shin, H. S., and Patra, J. K. (2018). Revitalization of plant growth promoting rhizobacteria for sustainable development in agriculture. Microbiol. Res. 206, 131–140. doi: 10.1016/j.micres.2017.08.016
Gravel, V., Antoun, H., and Tweddell, R. J. (2007). Growth stimulation and fruit yield improvement of greenhouse tomato plants by inoculation with Pseudomonas putida or Trichoderma atroviride: possible role of indole acetic acid (IAA). Soil Biol. Biochem. 39, 1968–1977. doi: 10.1016/j.soilbio.2007.02.015
Greenboim-Wainberg, Y., Maymon, I., Borochov, R., Alvarez, J., Olszewski, N., Ori, N., et al. (2005). Cross talk between gibberellin and cytokinin: the Arabidopsis GA response inhibitor SPINDLY plays a positive role in cytokinin signaling. Plant Cell 7, 92–102. doi: 10.1105/tpc.104.028472
Gutiérrez-Luna, F. M., López-Bucio, J., Altamirano-Hernández, J., Valencia-Cantero, E., Reyes de la Cruz, H., and Macías-Rodríguez, L. (2010). Plant growth-promoting rhizobacteria modulate root-system architecture in Arabidopsis thaliana through volatile organic compound emission. Symbiosis 51, 75–83. doi: 10.1007/s13199-010-0066-2
Ha, S. M., Kim, C. K., Roh, J., Byun, J. H., Yang, S. J., Choi, S. B., et al. (2019). Application of the whole genome-based bacterial identification system, TrueBac ID, in clinical isolates which were not identified with three MALDI-TOF M/S systems. Ann. Lab Med. 39, 530–536. doi: 10.3343/alm.2019.39.6.530
Hallmann, J., Quadt-Hallmann, A., Mahaffee, W. F., and Kloepper, J. W. (1997). Bacterial endophytes in agricultural crops. Can. J. Microbiol. 43, 895–914. doi: 10.1139/m97-131
Hardoim, P. R., Van Overbeek, L. S., Berg, G., Pirttila, A. M., Compant, S., Campisano, A., et al. (2015). The hidden world within plants: ecological and evolutionary considerations for defining functioning of microbial endophytes. Microbiol. Mol. Biol. Rev. 79, 293–320. doi: 10.1128/MMBR.00050-14
Hardoim, P. R., van Overbeek, L. S., and van Elsas, J. D. (2008). Properties of bacterial endophytes and their proposed role in plant growth. Trends Microbiol. 16, 463–471. doi: 10.1016/j.tim.2008.07.008
Hernández-Calderón, E., Aviles-Garcia, M. E., Castulo-Rubio, D. Y., Macías-Rodríguez, L., Ramírez, V. M., Santoyo, G., et al. (2018). Volatile compounds from beneficial or pathogenic bacteria differentially regulate root exudation, transcription of iron transporters, and defense signaling pathways in Sorghum bicolor. Plant Mol. Biol. 96, 291–304. doi: 10.1007/s11103-017-0694-5
Hong, S. H., Kim, H. J., Ryu, J. S., Choi, H., Jeong, S., Shin, J., et al. (2008). CRY1 inhibits COP1-mediated degradation of BIT1, a MYB transcription factor, to activate blue light-dependent gene expression in Arabidopsis. Plant J. 55, 361–371. doi: 10.1111/j.1365-313x.2008.03508.x
Jacobsen, S. E., Binkowski, K. A., and Olszewski, N. E. (1996). SPINDLY, a tetratricopeptide repeat protein involved in gibberellin signal transduction in Arabidopsis. Proc. Natl. Acad. Sci. U.S.A. 93, 9292–9296. doi: 10.1073/pnas.93.17.9292
Jiao, Y., Yang, H., Ma, L., and Sun, N. (2003). A genome-wide analysis of blue-light regulation of Arabidopsis transcription factor gene expression during seedling development. Plant Physiol. 133, 1480–1493. doi: 10.1104/pp.103.029439
Kanehisa, M., and Goto, S. (2000). KEGG: kyoto encyclopedia of genes and genomes. Nucleic Acids Res. 28, 27–30.
Kang, S. M., Radhakrishnan, R., You, Y. H., Joo, G. J., Lee, I. J., Lee, K. E., et al. (2014). Phosphate solubilizing Bacillus megaterium mj1212 regulates endogenous plant carbohydrates and amino acids contents to promote mustard plant growth. Indian J. Microbiol. 54, 427–433. doi: 10.1007/s12088-014-0476-6
Ke, J., Wang, B., and Yoshikuni, Y. (2021). Microbiome engineering: synthetic biology of plant-associated microbiomes in sustainable agriculture. Trends Biotechnol. 39, 244–261. doi: 10.1016/j.tibtech.2020.07.008
Kuan, K. B., Othman, R., Rahim, K. A., and Shamsuddin, Z. H. (2016). Plant growth-promoting rhizobacteria inoculation to enhance vegetative growth, nitrogen fixation and nitrogen remobilization of maize under greenhouse conditions. PLoS One 11:e0152478. doi: 10.1371/journal.pone.0152478
Li, N., Wu, H., Ding, Q., Li, H., Li, Z., Ding, J., et al. (2018). The heterologous expression of Arabidopsis PAP2 induces anthocyanin accumulation and inhibits plant growth in tomato. Funct. Integr. Genom. 18, 341–353. doi: 10.1007/s10142-018-0590-3
Lodewyckx, C., Vangronsveld, J., Porteous, F., Moore, E. R. B., and Taghavi, S. (2002). Endophytic bacteria and their potential applications. Crit. Rev. Plant Sci. 21, 583–606.
Lugtenberg, B., and Kamilova, F. (2009). Plant growth-promoting rhizobacteria. Annu. Rev. Microbiol. 63, 541–556. doi: 10.1146/annurev.micro.62.081307.162918
Mandadi, K. K., Misra, A., Ren, S., and McKnight, T. D. (2009). BT2, a BTB protein, mediates multiple responses to nutrients, stresses, and hormones in Arabidopsis. Plant Physiol. 150, 1930–1939. doi: 10.1104/pp.109.139220
Mangeon, A., Bell, E. M., Lin, W. C., Jablonska, B., and Springer, P. S. (2011). Misregulation of the LOB domain gene DDA1 suggests possible functions in auxin signalling and photomorphogenesis. J. Exp. Bot. 62, 221–233. doi: 10.1093/jxb/erq259
Marasco, R., Rolli, E., Ettoumi, B., Vigani, G., Mapelli, F., Borin, S., et al. (2012). A drought resistance-promoting microbiome is selected by root system under desert farming. PLoS One. 7:e48479. doi: 10.1371/journal.pone.0048479
Nikolic, B., Schwab, H., and Sessitsch, A. (2011). Meta genomic analysis of the 1-aminocyclopropane- 1-carboxylate deaminase gene (acdS) operon of an uncultured bacterial endophyte colonizing Solanum tuberosum L. Arch. Microbiol. 193, 665–676. doi: 10.1007/s00203-011-0703-z
Olsen, A. N., Ernst, H. A., Leggio, L. L., and Skriver, K. (2005). NAC transcription factors: structurally distinct, functionally diverse. Trends Plant Sci. 10, 79–87. doi: 10.1016/j.tplants.2004.12.010
Pao, S. S., Paulsen, T. I, and Saier, M. H. Jr. (1998). Major facilitator superfamily. Microbiol. Mol. Biol. Rev. 62, 1–34.
Phetcharat, P., and Duangpaeng, A. (2012). Screening of endophytic bacteria from organic rice tissue for indole acetic acid production. Proc. Eng. 32, 177–183. doi: 10.1016/j.proeng.2012.01.1254
Pieterse, C. M., Van der Does, D., Zamioudis, C., León-Reyes, A., and Van Wees, S. C. (2012). Hormonal modulation of plant immunity. Annu. Rev. Cell Dev. Biol. 28, 489–521. doi: 10.1146/annurev-cellbio-092910-154055
Pourbabaee, A. A., Bahmani, E., Alikhani, H. A., and Emami, S. (2016). Promotion of wheat growth under salt stress by halotolerant bacteria containing ACC deaminase. J. Agric. Sci. Technol. 18, 855–864.
Qu, Y., Legen, J., Arndt, J., Henkel, S., Hoppe, G., Thieme, C., et al. (2018). Ectopic transplastomic expression of a synthetic MatK gene leads to cotyledon-specific leaf variegation. Front. Plant Sci. 4:1453. doi: 10.3389/fpls.2018.01453
Radhakrishnan, R., and Lee, I. J. (2016). Gibberellins producing Bacillus methylotrophicus KE2 supports plant growth and enhances nutritional metabolites and food values of lettuce. Plant Physiol. Biochem. 109, 181–189. doi: 10.1016/j.plaphy.2016.09.018
Rashid, S., Charles, T. C., and Glick, B. R. (2012). Isolation and characterization of new plant growth-promoting bacterial endophytes. Appl. Soil Ecol. 61, 217–224. doi: 10.1016/j.apsoil.2011.09.011
Razin, S. V., Borunova, V. V., Maksimenko, O. G., and Kantidze, O. L. (2012). Cys2His2 zinc finger protein family: classification, functions, and major members. Biochemistry. 77, 217–226. doi: 10.1134/s0006297912030017
Robert, H. S., Quint, A., Brand, D., Vivian-Smith, A., and Offringa, R. (2009). BTB and TAZ domain scaffold proteins perform a crucial function in Arabidopsis development. Plant J. 58, 109–121. doi: 10.1111/j.1365-313X.2008.03764.x
Rosado, A., Schapire, A. L., Bressan, R. A., Harfouche, A. L., Hasegawa, P. M., Valpuesta, V., et al. (2006). The Arabidopsis tetratricopeptide repeat-containing protein TTL1 is required for osmotic stress responses and abscisic acid sensitivity. Plant Physiol. 142, 1113–1126. doi: 10.1104/pp.106.085191
Rubin, G., Tohge, T., Matsuda, F., Saito, K., and Scheible, W. R. (2009). Members of the LBD family of transcription factors repress anthocyanin synthesis and affect additional nitrogen responses in Arabidopsis. Plant Cell 21, 3567–3584. doi: 10.1105/tpc.109.067041
Ryu, C. M., Farag, M. A., Hu, C. H., Reddy, M. S., Wei, H. X., Pare, P. W., et al. (2003). Bacterial volatiles promote growth in Arabidopsis. Proc. Natl. Acad. Sci. U.S.A 100, 4927–4932. doi: 10.1073/pnas.0730845100
Saitou, N., and Nei, M. (1987). The neighbor-joining method: a new method for reconstructing phylogenetic trees. Mol. Biol. Evol. 4, 406–425.
Sambrook, J., Fritschi, E. F., and Maniatis, T. (1989). Molecular Cloning: A Laboratory Manual. New York, NY: Cold Spring Harbor Laboratory Press.
Santoyo, G., Moreno-Hagelsieb, G., Orozco-Mosqueda Mdel, C., and Glick, B. R. (2016). Plant growth-promoting bacterial endophytes. Microbiol. Res. 183, 92–99. doi: 10.1016/j.micres.2015.11.008
Shamimuzzaman, M., and Vodkin, L. (2013). Genome-wide identification of binding sites for NAC and YABBY transcription factors and co-regulated genes during soybean seedling development by ChIP-Seq and RNA-Seq. BMC Genomics 14:77. doi: 10.1186/1471-2164-14-477
Shi, Y., Yang, H., Zhang, T., Sun, J., and Lou, K. (2014). Illumina-based analysis of endophytic bacterial diversity and space-time dynamics in sugar beet on the north slope of Tianshan Mountain. Appl. Microbiol. Biotechnol. 98, 6375–6385. doi: 10.1007/s00253-014-5720-9
Song, S., Liu, Y., Wang, N. R., and Haney, C. H. (2021). Mechanisms in plant-microbiome interactions: lessons from model systems. Curr. Opin. Plant Biol. 2:102003. doi: 10.1016/j.pbi.2021.102003
Sun, L., Wang, X., and Li, Y. (2016). Increased plant growth and copper uptake of host and nonhost plants by metal-resistant and plant growth-promoting endophytic bacteria. Int. J. Phytoremed. 18, 494–501. doi: 10.1080/15226514.2015.1115962
Sun, Y., Cheng, Z., and Glick, B. R. (2009). The presence of a1-aminocyclopropane-1-carboxylate (ACC) deaminase deletion mutation alters the physiology of the endophytic plant growth-promoting bacterium Burkholderia phytofirmans PsJN. FEMS Microbiol. Lett. 296, 131–136. doi: 10.1111/j.1574-6968.2009.01625.x
Toledo-Ortiz, G., Huq, E., and Quail, P. H. (2003). The Arabidopsis basic/helix-loop-helix transcription factor family. Plant Cell. 15, 1749–1770. doi: 10.1105/tpc.013839
Ulrich, K., Ulrich, A., and Ewald, D. (2008). Diversity of endophytic bacterial communities in poplar grown under field conditions. FEMS Microbiol. Ecol. 63, 169–180. doi: 10.1111/j.1574-6941.2007.00419.x
Vacheron, J., Desbrosses, G., Bouffaud, M. L., Touraine, B., Moenne-Loccoz, Y., Muller, D., et al. (2013). Plant growth-promoting rhizobacteria and root system functioning. Front. Plant Sci. 4:356. doi: 10.3389/fpls.2013.00356
Wall, M. K., Mitchenall, L. A., and Maxwell, A. (2004). Arabidopsis thaliana DNA gyrase is targeted to chloroplasts and mitochondria. Proc. Natl. Acad. Sci. U.S.A. 101, 7821–7826. doi: 10.1073/pnas.0400836101
Wang, K. L., Yoshida, H., Lurin, C., and Ecker, J. R. (2004). Regulation of ethylene gas biosynthesis by the Arabidopsis ETO1 protein. Nature 428, 945–950. doi: 10.1038/nature02516
Wang, X., Niu, Q. W., Teng, C., Li, C., Mu, J., Chua, N. H., et al. (2009). Overexpression of PGA37/MYB118 and MYB115 promotes vegetative-to-embryonic transition in Arabidopsis. Cell Res. 19, 224–235. doi: 10.1038/cr.2008.276
Wani, Z. A., Ashraf, N., Mohiuddin, T., and Riyaz-Ul-Hassan, S. (2015). Plant-endophyte symbiosis, an ecological perspective. Appl. Microbiol. Biotechnol. 99, 2955–2965. doi: 10.1007/s00253-015-6487-3
Weyens, N., van der Lelie, D., Taghavi, S., and Vangronsveld, J. (2009). Phytoremediation: Plant-endophyte partnerships take the challenge. Curr. Opin. Biotech. 20, 248–254. doi: 10.1016/j.copbio.2009.02.012
Worlanyo, A. S., and Jiangfeng, L. (2021). Evaluating the environmental and economic impact of mining for post-mined land restoration and land-use. J. Environ. Manag. 279, 111623. doi: 10.1016/j.jenvman.2020.111623
Xie, S., Wu, H. J., Zang, H., Wu, L., Zhu, Q., and Gao, X. (2014). Plant growth promotion by spermidine-producing Bacillus subtilis OKB105. Mol. Plant Microbe Interact. 27:6556663. doi: 10.1094/MPMI-01-14-0010-R
Yang, S. W., Jang, I. C., Henriques, R., and Chua, N. H. (2009). FAR-RED ELONGATED HYPOCOTYL1 and FHY1-LIKE associate with the Arabidopsis transcription factors LAF1 and HFR1 to transmit phytochrome A signals for inhibition of hypocotyl elongation. Plant Cell 21, 1341–1359. doi: 10.1105/tpc.109.067215
Yoon, S. H., Ha, S. M., Kwon, S., Lim, J., Kim, Y., Seo, H., et al. (2017). Introducing EzBioCloud: a taxonomically united database of 16S rRNA and whole genome assemblies. Int. J. Syst. Evol. Microbiol. 67, 1613–1617. doi: 10.1099/ijsem.0.001755
Yoshida, H., Nagata, M., Saito, K., Wang, K. L., and Ecker, J. R. (2005). Arabidopsis ETO1 specifically interacts with and negatively regulates type 2 1-aminocyclopropane-1-carboxylate synthases. BMC Plant Biol. 5:14. doi: 10.1186/1471-2229-5-14
Zentella, R., Zhang, Z. L., Park, M., Thomas, S. G., Endo, A., Murase, K., et al. (2007). Global analysis of DELLA direct targets in early gibberellin signaling in Arabidopsis. Plant Cell 19, 3037–3057. doi: 10.1105/tpc.107.054999
Zhang, H., Sun, Y., Xie, X., Kim, M. S., Dowd, S. E., and Pare, P. W. (2009). A soil bacterium regulates plant acquisition of iron via deficiency inducible mechanisms. Plant J. 58, 568–577. doi: 10.1111/j.1365-313x.2009.03803.x
Zhang, J., Cook, J., Nearing, J. T., Zhang, J., Raudonis, R., Glick, B. R., et al. (2021). Harnessing the plant microbiome to promote the growth of agricultural crops. Microbiol. Res. 245:126690. doi: 10.1016/j.micres.2020.126690
Zhang, Y., Li, Z., Ma, B., Hou, Q., and Wan, X. (2020). Phylogeny and functions of LOB domain proteins in plants. Int. J. Mol. Sci. 21:2278. doi: 10.3390/ijms21072278
Zhang, Y. F., He, L. Y., Chen, Z. J., Wang, Q. Y., Qian, M., and Sheng, X. F. (2011). Characterization of ACC deaminase-producing endophytic bacteria isolated from copper-tolerant plants and their potential in promoting the growth and copper accumulation of Brassica napus. Chemosphere 83, 57–62. doi: 10.1016/j.chemosphere.2011.01.041
Zhao, H., Li, X., and Ma, L. (2012). Basic helix-loop-helix transcription factors and epidermal cell fate determination in Arabidopsis. Plant Signal Behav. 7, 1556–1560. doi: 10.4161/psb.22404
Zhou, C., Guo, J., Zhu, L., Xiao, X., Xie, Y., Zhu, J., et al. (2016). Paenibacillus polymyxa BFKC01 enhances plant iron absorption via improved root systems and activated iron acquisition mechanisms. Plant Physiol. Biochem 105, 162–173. doi: 10.1016/j.plaphy.2016.04.025
Zhou, J., Lee, C., Zhong, R., and Ye, Z. H. (2009). MYB58 and MYB63 are transcriptional activators of the lignin biosynthetic pathway during secondary cell wall formation in Arabidopsis. Plant Cell 21, 248–266. doi: 10.1105/tpc.108.063321
Zinniel, D. K., Lambrecht, P., Harris, N. B., Feng, Z., Kuczmarski, D., Higley, P., et al. (2002). Isolation and characterization of endophytic colonizing bacteria from agronomic crops and prairie plants. Appl. Environ. Microbiol. 68, 2198–2208. doi: 10.1128/aem.68.5.2198-2208.2002
Keywords: endophytic bacteria, Bacillus altitudinis, plant growth, transcriptome, Glyceria chinensis
Citation: Zhang D, Xu H, Gao J, Portieles R, Du L, Gao X, Borroto Nordelo C and Borrás-Hidalgo O (2021) Endophytic Bacillus altitudinis Strain Uses Different Novelty Molecular Pathways to Enhance Plant Growth. Front. Microbiol. 12:692313. doi: 10.3389/fmicb.2021.692313
Received: 08 April 2021; Accepted: 26 May 2021;
Published: 25 June 2021.
Edited by:
Maurizio Ruzzi, University of Tuscia, ItalyReviewed by:
Tyler Helmann, United States Department of Agriculture (USDA), United StatesSergio de los Santos Villalobos, Instituto Tecnológico de Sonora (ITSON), Mexico
Copyright © 2021 Zhang, Xu, Gao, Portieles, Du, Gao, Borroto Nordelo and Borrás-Hidalgo. This is an open-access article distributed under the terms of the Creative Commons Attribution License (CC BY). The use, distribution or reproduction in other forums is permitted, provided the original author(s) and the copyright owner(s) are credited and that the original publication in this journal is cited, in accordance with accepted academic practice. No use, distribution or reproduction is permitted which does not comply with these terms.
*Correspondence: Orlando Borrás-Hidalgo, Ym9ycmFzb3JsYW5kb0B5YWhvby5jb20=