- 1Faculdade de Ciências Agrárias e Veterinárias (FCAV), Universidade Estadual Paulista (UNESP), Jaboticabal, Brazil
- 2Fundo de Defesa da Citricultura (Fundecitrus), Araraquara, Brazil
- 3Empresa Brasileira de Pesquisa Agropecuária (Embrapa), Cruz das Almas, Brazil
- 4Instituto de Biología Molecular y Celular de Plantas (IBMCP), Consejo Superior de Investigaciones Científicas (CSIC), Universidad Politécnica de Valencia (UPV), Valencia, Spain
Huanglongbing is a highly destructive citrus disease associated with “Candidatus Liberibacter asiaticus” (Las), a phloem−limited and non-culturable bacterium, naturally transmitted by the psyllid Diaphorina citri. Although diverse approaches have been used to understand the molecular mechanisms involved in the pathogen–host interaction, such approaches have focused on already infected and/or symptomatic plants, missing early events in the initial days post-inoculation. This study aimed to identify the time course of Las multiplication and whole-plant colonization immediately following inoculation by infected psyllids feeding for 2 days. Thus, the experimental approach was to track Las titers after psyllid inoculation in new shoots (NS) of Citrus × sinensis (susceptible), Murraya paniculata (partially resistant), and Bergera koenigii (fully resistant). Soon after psyllid removal, Las titers dropped until the 10–12th days in all three species. Following this, Las titers increased exponentially only in C. × sinensis and M. paniculata, indicating active bacterial multiplication. In C. × sinensis, Las reached a stationary phase at ∼5 log Las cells/g of tissue from the 40th day onward, while in M. paniculata, Las increased at a lower rate of up to ∼3 log Las cells/g of tissue between the 40th and 60th days, decreasing gradually thereafter and becoming undetectable from the 160th day onward. In B. koenigii, Las titers decreased from the start and remained undetectable. In C. × sinensis, an average of 2.6 log of Las cells/g of tissue was necessary for Las to move out of 50% of the NS in 23.6 days and to colonize the rest of the plant, causing a successful infection. Conversely, the probability of Las moving out of the NS remained below 50% in M. paniculata and zero in B. koenigii. To our knowledge, this is the first study on Las dynamics and whole-plant colonization during the earliest stages of infection. Identification of critical time-points for either successful multiplication or Las resistance may help to elucidate initial events of Las–host interactions that may be missed due to longer sampling intervals and at later stages of infection.
Introduction
Huanglongbing (HLB) is the most severe citrus disease worldwide (Bové, 2006). In Asia and America, the unculturable α-proteobacteria, phloem-limited “Candidatus Liberibacter asiaticus” (Las) is associated with HLB (Gottwald, 2010). It is transmitted in the field by the Asian citrus psyllid Diaphorina citri (Kuwayama) (Sternorrhyncha: Liviidae) (Capoor et al., 1967). As there is no known cure for this disease, HLB control in Brazil is based on the use of healthy plants from certified nurseries, elimination of symptomatic plants in the groves, and inspection and rigorous control of the insect vector (Bassanezi et al., 2020). However, these strategies may be economically and environmentally unsustainable and inefficient for the management of HLB, whose incidence continues to increase, and it is currently estimated to be present in more than 20% of symptomatic trees in the citrus belt of São Paulo and Minas Gerais (Fundecitrus, 2020). In Florida, United States, the citrus industry relies on the production of more than 80% of the orchards that are Las-infected (Singerman and Useche, 2016). Leaves from affected trees show typical HLB symptoms such as asymmetrical chlorosis called blotchy mottle, enlarged veins, and intense canopy defoliation (Bové, 2006). The evolution of symptoms in Las-positive plants is rapid once they appear, and as the disease severity increases, the yield and fruit quality decrease (Gottwald, 2010). HLB-affected fruits are smaller, lighter, and highly acidic and have a reduced Brix ratio (Bassanezi et al., 2009).
Diaphorina citri acquires Las when they feed on the phloem sap from infected trees by inserting their stylets into the sieve elements. Las infects psyllids in a propagative and circulative manner (Inoue et al., 2009; Ammar et al., 2016; Canale et al., 2017). After a minimum latent period of 2–3 weeks, infected psyllids can transmit the bacterium to healthy plants by releasing it directly into the phloem when feeding (Canale et al., 2017). Electrical penetration graph (EPG) experiments have shown that 8 h is usually sufficient for the psyllid to come in contact with the phloem (Bonani et al., 2010; Wu et al., 2016; Carmo-Souza et al., 2020), but the efficiency of Las transmission is strongly dependent on the developmental stage of the plant tissue in which the insect settles as well as the environmental conditions (Raiol-Junior et al., 2017; Lopes and Cifuentes-Arenas, 2021; Pandey et al., 2021). The nature of insect saliva effectors capable of counteracting host defenses, similar to those shown for other phloem-feeding insects (Hogenhout and Bos, 2011), is largely unknown for D. citri. Since Las is released into the phloem, it is notable that symptoms appear in infected plants after the bacterium has already reached high titers, in a time period ranging from 4 to 8 months after inoculation under experimental conditions (Lopes et al., 2009) and to more than a year under field conditions (Gottwald, 2010). However, little is known about citrus host–Las interactions before the onset of symptoms, especially during the initial days and weeks after psyllid inoculation. Pandey and Wang (2019) detected Las as early as 2 days after D. citri fed on new shoots (NS), but the subsequent population growth was not monitored. It has been reported that Las moves through the phloem pores in an elongated form and adheres to the plasma membrane in sieve plates through unknown extracellular dark materials (Achor et al., 2020). Las preferentially invades new tissues during development (Raiol-Junior et al., 2021a) and irregularly colonizes different parts of the plant (Tatineni et al., 2008; Li et al., 2009), which makes the selection of the tissue used for study and/or diagnostics very important (Tatineni et al., 2008; Johnson et al., 2014; Raiol-Junior et al., 2021a). Moreover, losses in the root system caused by Las occur before the appearance of symptoms in the canopy (Johnson et al., 2014). Therefore, the first steps of the vector–plant–pathogen interaction are challenging to study. In addition, most previous studies were conducted using only susceptible Citrus species.
Although susceptibility to Las varies among Citrus species and varieties (Folimonova et al., 2009), full resistance has already been described in species that are sexually and/or graft-compatible with Citrus (Alves et al., 2021) as well as in species that are phylogenetically more distant within the family Rutaceae, subfamily Aurantioideae (Ramadugu et al., 2016; Beloti et al., 2018; Cifuentes-Arenas et al., 2019). Murraya paniculata (L.) Jack and Bergera koenigii L. plants, known as orange jasmine and curry leaf, respectively, are commonly used as ornamental plants in tropical and subtropical regions of the world (Swingle and Reece, 1967). Both these species are good hosts for D. citri (Damsteegt et al., 2010; Teck et al., 2011; Westbrook et al., 2011), being much more attractive to psyllid females as compared to sweet orange under laboratory and field conditions (Beloti et al., 2017; Tomaseto et al., 2019). However, low suitability and immunity to Las have been reported in M. paniculata and B. koenigii, respectively (Damsteegt et al., 2010; Beloti et al., 2018; Cifuentes-Arenas et al., 2019). The role of D. citri as a Las vector and the first stages of Las infection in the phloem of the two plant species remain unexplored.
We studied the time course of Las multiplication in the susceptible host Citrus × sinensis ‘Valencia’, in the transient host M. paniculata, and in the fully resistant B. koenigii to better understand the initial bacterial dynamics between the delivery of Las into the phloem by adults psyllids, bacterial multiplication at the first stages post-inoculation, and the time taken for Las to get out from the NS and spread through the rest of the plant. The use of a well-controlled challenge-inoculation system using infective psyllids allowed us to replicate experiments with consistent results, leading to the establishment of the earliest events of Las infection and whole-plant colonization. Understanding Las progression and defense responses triggered by resistant species may help to develop bacterial control strategies.
Materials and Methods
Plant Material and Growth Conditions
One-year-old plantlets of Citrus × sinensis (L.) Osbeck ‘Valencia’ sweet orange grafted on ‘Rangpur’ lime (Citrus × limonia Osbeck) rootstock and 1-year-old seedlings of M. paniculata (orange jasmine) and B. koenigii (curry leaf) were used. All plants were grown in 300-ml conical tubes (6.5 × 5.9 cm, upper × lower diameter; 16 cm, height), filled with coconut fiber, and irrigated twice a week. Prior to the experimental use, the C. × sinensis plantlets were 20 to 30 cm above the substrate level, and M. paniculata and B. koenigii were 20–40 cm above the substrate level. Rearing of Las-infected Diaphorina citri and studies on the time course of Las multiplication were carried out inside a controlled environmental room (CER) using shelves where the light source was 20 to 30 cm above the plants. The daily temperature varied from 24°C to 27°C, relative humidity ranged between 55% and 78%, and photoperiod was set to 12 h of light at 300 μmol m–2 s–1 of photosynthetically active radiation and 12 h of darkness. At the end of the longest sampling time (see below) for up to 12 months, plants were transferred from CER to a greenhouse in which the mean daily air temperature varied between 18.5°C and 34.4°C, and illumination was natural.
Rearing of Las-Infective Psyllids and Inoculation Procedure
A methodology adapted from Lopes and Cifuentes-Arenas (2021) was used for insect rearing and inoculation purposes. Las-positive, fully symptomatic, and 2-year-old plants of C. × sinensis grafted on ‘Swingle’ citrumelo (Citrus × paradisi Macfad. × Poncirus trifoliata L. Raf.) growing in 4.7-l pots were used for rearing Las-infective D. citri insects. The Ct value of Las in these source plants was 20.7 ± 0.33 (equivalent to ca. 6.2 ± 0.1 log of Las cells/g of tissue). Las infection in C. × sinensis plants was confirmed by qPCR (Li et al., 2006). Plants were lightly pruned to stimulate the growth of NS, and when they reached the V2 stage of development (Cifuentes-Arenas et al., 2018), four Las-negative 10- to 20-day-old psyllids were confined per shoot for oviposition for 7 days. The Las-negative mixed sex psyllids were obtained from a colony kept at Fundecitrus, reared on healthy M. paniculata, adapting the methodology proposed by Skelley and Hoy (2004). The adult psyllids were then removed from the plants, and the plants remained inside the CER to allow for the eggs to hatch and nymphs to develop into first-generation adults (F1). Once F1 adults began to emerge, a random sample of three insects per source plant was taken at 5 days postemergence to confirm Las presence by qPCR, and the rest were maintained in the same plants up to 10–15 days before being used (Figure 1).
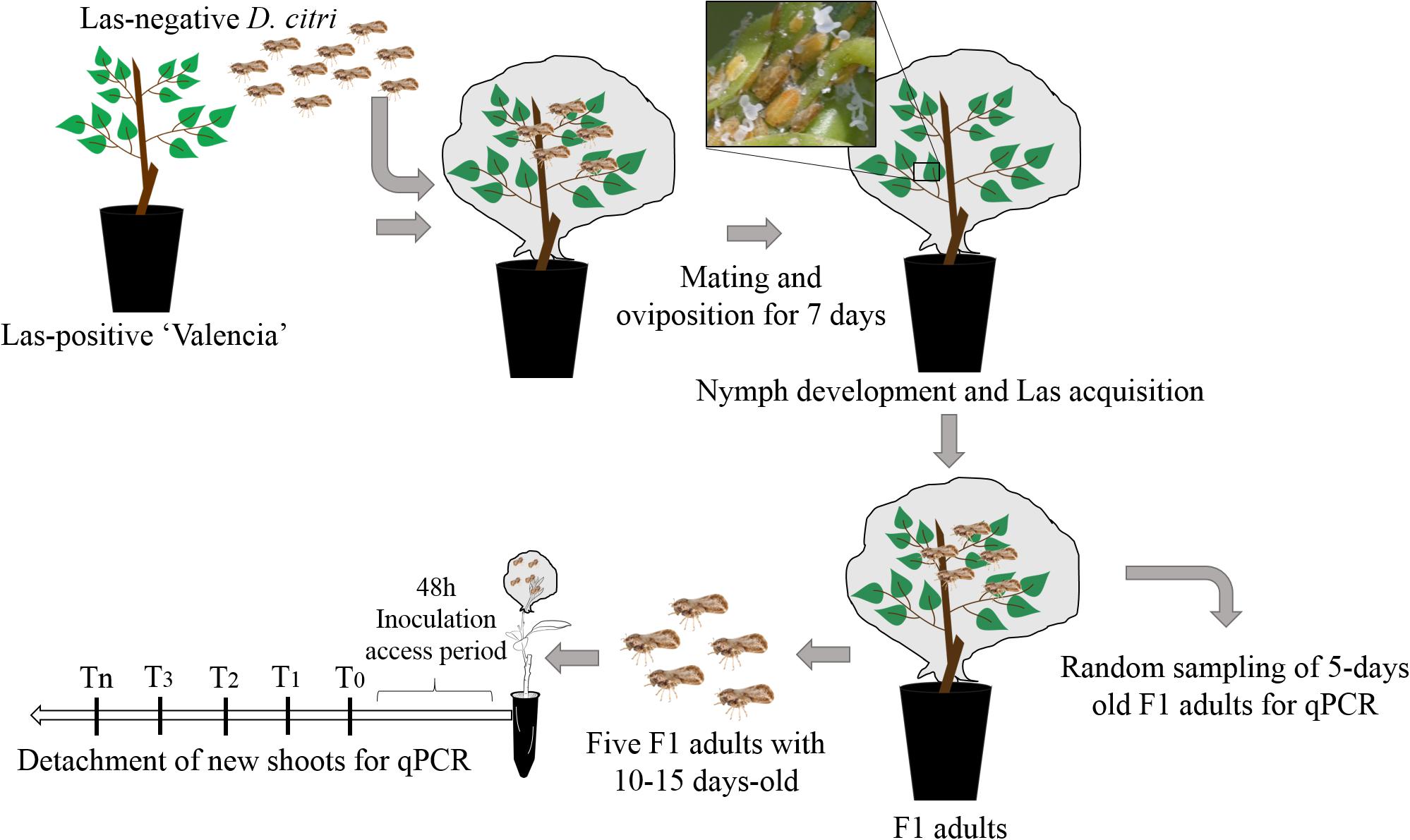
Figure 1. Experimental procedures for “Candidatus Liberibacter asiaticus” transmission by Diaphorina citri.
The C. × sinensis plantlets and the M. paniculata and B. koenigii seedlings were pruned at ∼15 cm above the substrate level to force NS growth until they reached stage V3 of development 18 to 23 days post-pruning (Cifuentes-Arenas et al., 2018). In M. paniculata and B. koenigii, V3 shoots were characterized by the presence of one partially unfurled and one or more tender expanding leaflets (Figure 2).
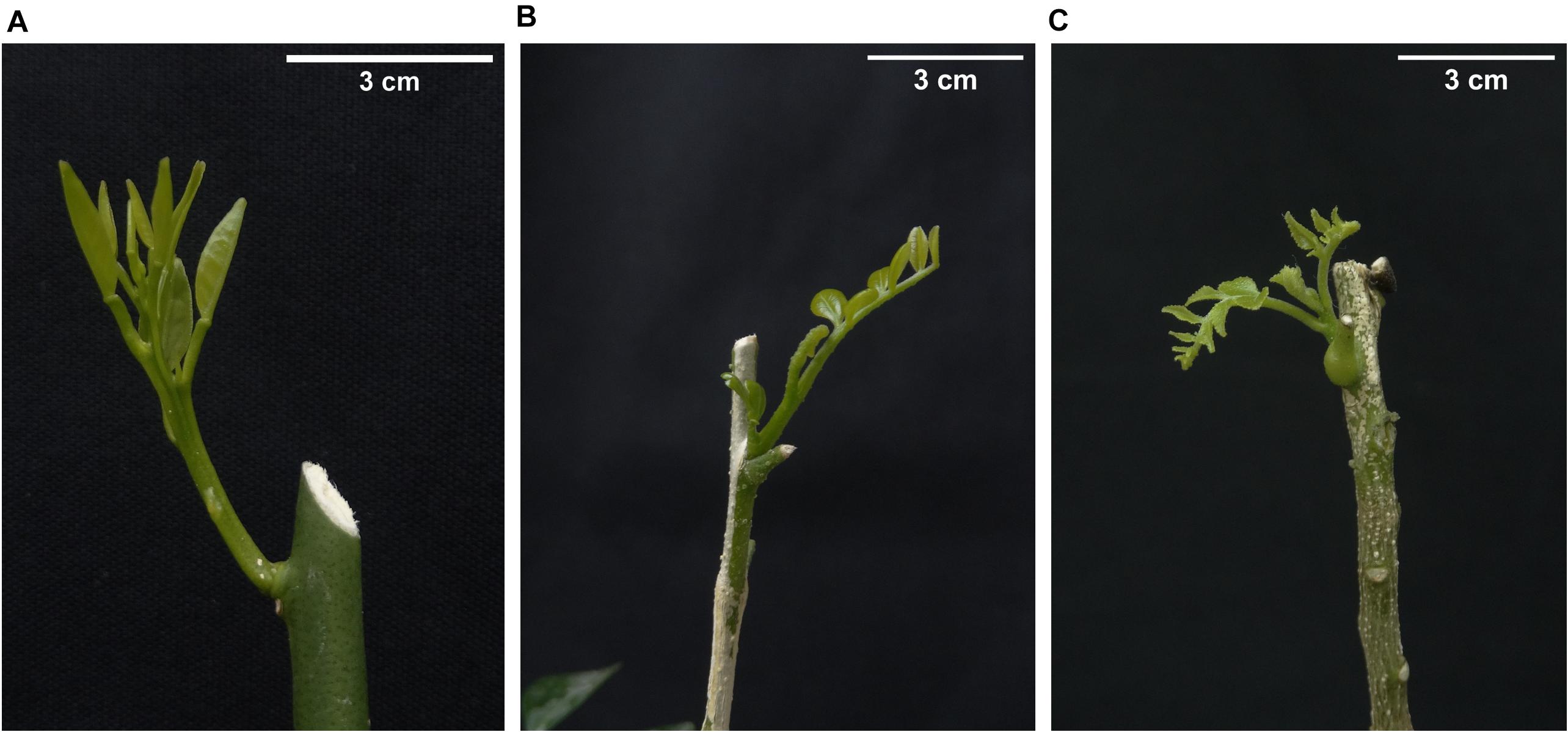
Figure 2. Aspect of new shoots in (A) Citrus × sinensis, (B) Murraya paniculata, and (C) Bergera koenigii. Such shoot stages were used for “Candidatus Liberibacter asiaticus” challenge Diaphorina citri-mediated inoculation experiments.
Five 10–15-day-old Las-exposed adults were confined to a single V3 NS per plant for 48 h of inoculation access period (IAP). The adults were then removed and stored at −20°C. Subsequently, random-stratified samplings were performed, where one of the five adult insects from each of three randomly selected plants per NS detaching time (see below) for qPCR analysis was selected. The plants were then sprayed with insecticide (Abamectin EC 7.2 g of active ingredient per 1 l of water) to eliminate any psyllid eggs or nymphs present. This experiment was repeated twice with 70, 100, and 41 individuals of C. × sinensis, M. paniculata, and B. koenigii, respectively, in the first replication, and 95, 106, and 70 individuals in the second replication. As a control group, 8–10 plants of each species in both experimental replicates were exposed to Las-negative adults of similar age and conditions as described above.
Time Course of Las Multiplication and Whole Plant Colonization
To determine the population dynamics of Las multiplication after psyllid feeding, 5–10 plants per genotype were randomly selected at each time point, and the NS on which the Las-positive adults fed was detached from time 0 (day of psyllid removal) and at variable times post-IAP. Each plant was used at a single time point. For C. × sinensis, sampling was performed at 0, 5, 10, 15, 25, 40, 55, and 70 days and 0, 5, 10, 15, 18, 20, 30, 40, 65, and 95 days post-IAP in the first and second experiment replicates, respectively. For M. paniculata, sampling was performed at 0, 5, 10, 15, 25, 40, 55, 70, 95, 110, and 180 days and 0, 5, 10, 15, 20, 25, 30, 40, 65, 95, and 170 days post-IAP in the first and second experiment replicates, respectively. For B. koenigii, sampling was performed at 0, 5, 10, 15, 30, 60, and 95 days and 0, 5, 10, 15, 20, 34, and 55 days post-IAP in the first and second experiment replicates, respectively. NS was analyzed to verify the presence of Las by qPCR. In the first sampling intervals, the whole NS on which the Las-positive adults fed was used for processing, but as they grew, only a proportion of the tissue was necessary; it was ensured that tissue pieces were randomly sampled from all parts (tip to base) of the NS on which the Las-positive adults fed. At the end of the longest sampling time (180 days), the plants were transferred from the CER to a greenhouse to complete up to 12 months post-IAP. Then, these plants were sampled again, selecting four to five leaves randomly distributed in the whole canopy. The entire leaves were processed to detect Las presence using qPCR; thus, the successful establishment of the infection was verified.
DNA Extraction and qPCR
Tissue samples (0.3 g) were processed as described by Teixeira et al. (2005) using a TissueLyser II system (Qiagen, Valencia, CA, United States) at 45 Hz for 30 s in a 2.0-ml microtube containing 5-mm steel beads. For psyllid DNA extraction, one insect and a 3-mm steel bead were used in a 1.5-ml microtube. Total DNA was extracted using the cetyltrimethylammonium bromide (CTAB) extraction buffer as mentioned in Murray and Thompson (1980), with adaptations. Briefly, for C. × sinensis, M. paniculata, and D. citri DNA extraction, 1.5 ml of CTAB buffer was used (2% CTAB; 1.4 M NaCl; 2% PVP 10000; 0.5 M EDTA pH 8; 1 M Tris–HCl pH 8; 0.2% β-mercaptoethanol), incubated at 65°C for 30 min, and then centrifuged for 10 min at 12000 × g. The supernatant was transferred to a new 1.5-ml microtube, followed by extraction with chloroform/isoamyl alcohol (24:1). For B. koenigii, to avoid oxidation of phenolic compounds after sampling, 0.3 g of the tissue was immediately cooled with liquid nitrogen and stored at −80°C until DNA extraction. The tissue trituration was done with 350 μl of a modified CTAB buffer (2% CTAB; 1.4 M NaCl; 2% PVP 10000; 0.5 M EDTA pH 8; 1 M Tris–HCl pH 8; 5% β-mercaptoethanol) and then 1.15 ml of this buffer was added, incubated at 60°C for 20 min, and centrifuged for 10 min at 12000 × g. The supernatant was transferred to a new 1.5-ml microtube, followed by two extractions with phenol/chloroform/isoamyl alcohol (25:24:1) and one with chloroform/isoamyl alcohol (24:1). In both extractions, DNA precipitation was performed with 0.6 V isopropanol, washed twice with 70% ethanol, and resuspended in 50 μl of Milli-Q® water. DNA quality was checked using a NanoDrop (Thermo Fisher Scientific, Massachusetts, EUA) (Desjardins and Conklin, 2010).
For real-time polymerase chain reactions (qPCR) and the identification of the 16S rRNA of Las, 1.0 μl of total DNA (100 ng/μl), TaqMan® PCR Master Mix (1×) (Invitrogen, Carlsbad, CA, United States), and HLBas primer/probe (0.5 μM/0.2 μM) were used in a StepOnePlus thermocycler (Applied Biosystems, California, USA) as described by Li et al. (2006). To estimate Las multiplication in time-course experiments, qPCR analysis was performed in triplicate, and for Las colonization and Las detection in the whole plant, qPCR analyses were performed only once. The mitochondrial gene cytochrome oxidase (COX) and an insect wingless (Wg) gene region were used in all samples as internal controls for the quality of plants and psyllid DNA, respectively (Li et al., 2006; Manjunath et al., 2008). In addition, negative and positive citrus leaf and psyllid samples were included during DNA extraction and qPCR assays. Las was quantified based on the linear relationship between Ct and the 16S rRNA log, according to Lopes et al. (2013). As Ct values higher than 34.0 (below 0.9 log Las cells/g of tissue) produce variable results (Lopes et al., 2013), samples were considered Las-positive when the qPCR cycle threshold (Ct) was lower than 34.0, suspected to be positive when Ct values were between 34.0 and 36.0, and negative when Ct > 36 and/or when undetermined.
Data Analysis
Data on adult survival at the time of psyllid removal were analyzed using a chi-square test by adjusting a binomial generalized linear model (GLM). To determine if there were significant differences in Las titers between the groups of psyllids used to inoculate the three plant species (in each repetition of the experiment and between time points within each species), a two-way ANOVA with interactions was first performed considering the species and the repetition of the experiment as a factor with three and two levels, respectively. Subsequently, the possible differences between the time points within each species were analyzed by performing a one-way ANOVA, considering each shoot detachment time point as a treatment. These analyses were performed by adjusting a Gaussian GLM. Similarly, to compare the initial bacterial load in NS between species at the time of psyllid removal, a two-way ANOVA (plant species × experiment repetition) was carried out. When significant differences were found, the means were separated using Tukey’s post hoc test.
To analyze the time course of Las population multiplication in NS after psyllid removal, data on Las titer were submitted to nonlinear regressions to compare several growth models. The best model was selected based on the overall model significance and R2. For this, data consisted of log values estimated over the Ct values (including those above 34) according to Lopes et al. (2013). An arbitrary value of 39.9999 was assigned to the qPCR results that gave “undetermined” outputs.
Data on new shoot growth were subjected to nonlinear regression to adjust the Gompertz growth model (Tjørve and Tjørve, 2017). To analyze the probability of successful infection after 48 h of psyllid IAP and subsequent detachment of NS at different time intervals, data on Las-positive leaf samples (Ct < 34.0) taken 1 year after the start of the experiments were subjected to a logistic regression by adjusting a binomial GLM (Peng et al., 2002).
Results
Adult survival after 48 h of IAP was not significantly different between plant species [χ2(2, 2403) = 466.81; p > 0.05]. On an average, psyllid survival after the confinement period was 99.1%, 99.6%, and 96.6% in the first experiment and 95.8, 97.4, and 99.4% in the second experiment replicates on C. × sinensis ‘Valencia’, M. paniculata, and B. koenigii, respectively (Supplementary Table 1). Las titers in psyllids did not differ between plant species on which they were confined [F(2,158) = 1.4863; p > 0.05] or between replicates [F(1,158) = 0.1535; p > 0.05], and there was no significant interaction [F(2,158) = 2.6445; p > 0.05] between these two factors (Supplementary Table 2). At different time points after NS detachment, there was no difference in Las titers in psyllids used in C. × sinensis [F(12,53) = 0.9967; p > 0.05], M. paniculata [F(14,64) = 1.7563; p > 0.05], and B. koenigii [F(10,38) = 1.7061; p > 0.05]. The averages of Las titers varied from 5.33 to 5.98 log (min. = 1.94; max. = 6.94; Figure 3). The amount of initial bacterial load injected by the adult psyllids after 48 h of IAP was not influenced by the plant species [F(2,50) = 0.003; p > 0.05] or by the repetition of the experiment [F(1,50) = 1.542; p > 0.05]. The interaction between the two factors was not significant [F(2,50) = 3.220; p = 0.05]. Details on Ct and log values of each plant and time of evaluation are shown in Supplementary Tables 3, 4.
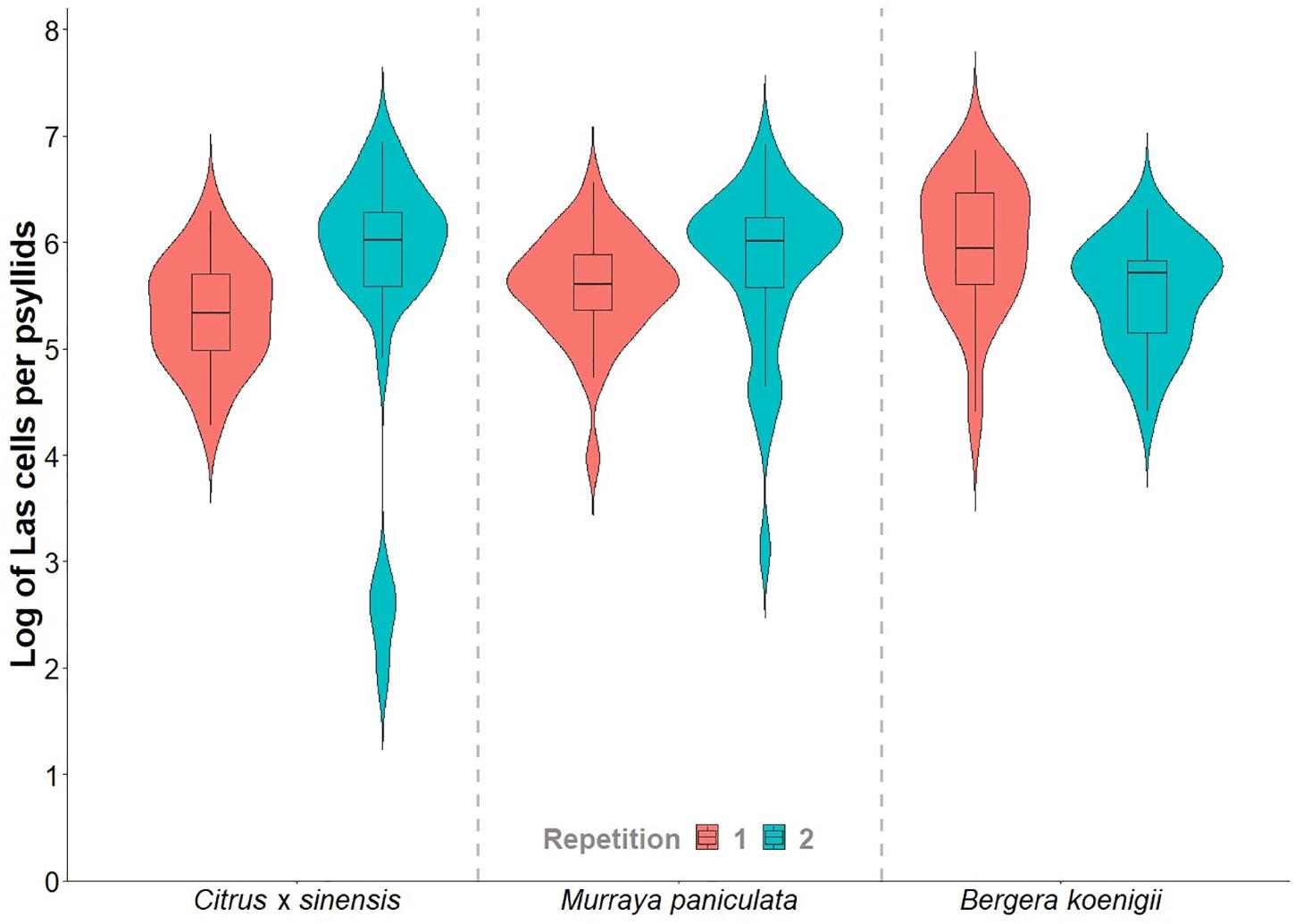
Figure 3. Violin plots of the “Candidatus Liberibacter asiaticus” titer in a subsample of 15- to 20-day-old Diaphorina citri adults reared on Las-positive Citrus × sinensis plants and confined during 48 h of inoculation access period (IAP) in new shoots of healthy Citrus × sinensis, M. paniculata, and B. koenigii.
The data on Las titers in C. × sinensis and M. paniculata adjusted well to the Lorentzian growth model (Table 1 and Supplementary Figure 1). This model describes two contrasting situations for both species in both replicates (Figure 4). At time 0 (the day of psyllid removal after 48 h of IAP), 10/10 and 7/10 C. × sinensis plants had Ct values lower than 34.0, in the first and second replicates, respectively. The average Las titer in NS at time 0 was 2.91 ± 0.16 log cell/g of tissue. After this, there was an initial decrease in Las titers soon after psyllid removal until the 10–12th days, up to ∼2.0 log cell/g of tissue. Subsequently, Las multiplied exponentially, reaching ∼90% of its final population after ∼40 days (Figure 4). A stationary phase was then observed, and the bacterial titer remained stable (∼4.5–5 log cell/g of tissue) until the last evaluation (70 and 95 days post-IAP in experiment replicates 1 and 2, respectively) with 80%–100% Las-positive plants.
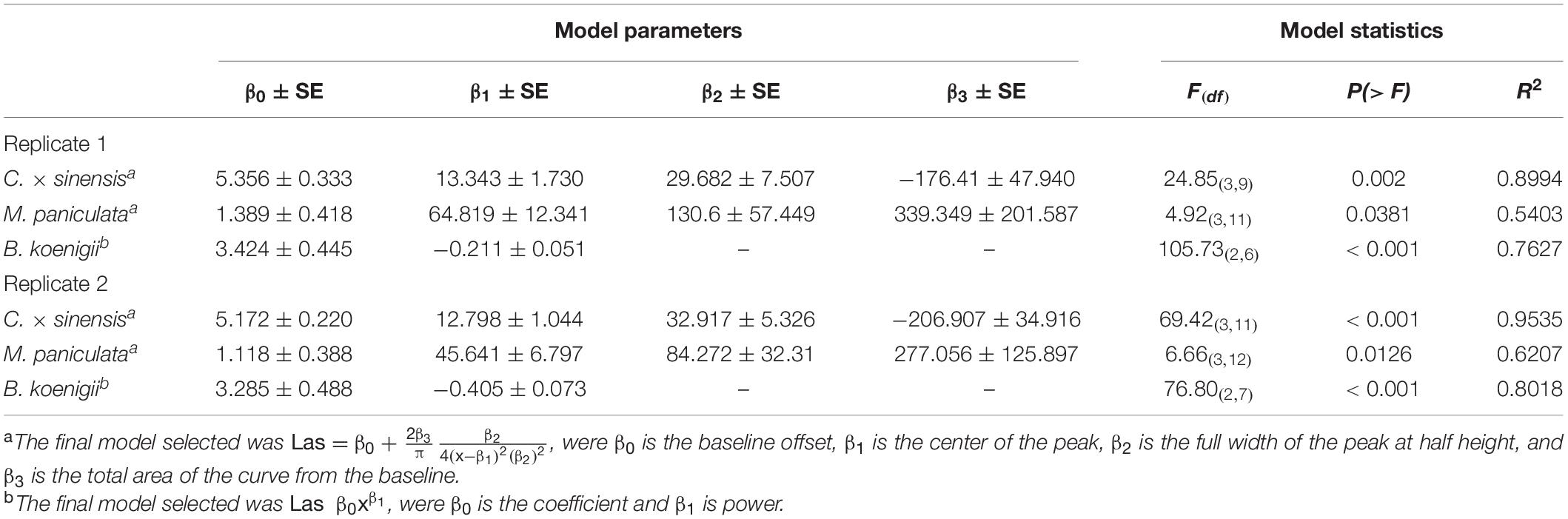
Table 1. Nonlinear regression models describing the time course of “Candidatus Liberibacter asiaticus” population dynamics in new shoots after 48 h of Las-exposed Diaphorina citri confinement at 25°C ± 2°C.
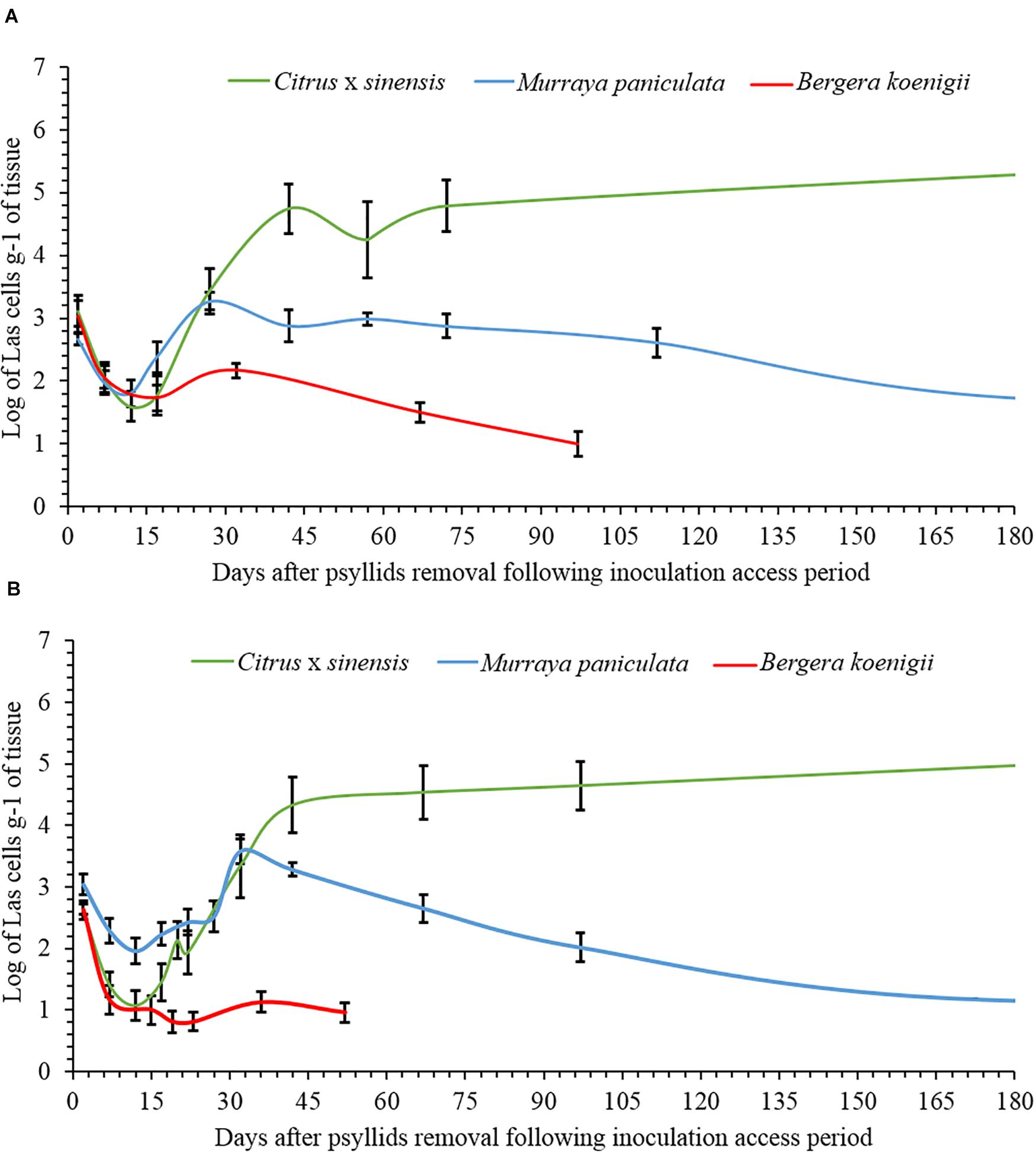
Figure 4. Evolution of the population dynamics of “Candidatus Liberibacter asiaticus” over time in new shoots of Citrus × sinensis, Murraya paniculata, and Bergera koenigii, following a 48-h period of Las-exposed Diaphorina citri confinement in experiment replicates 1 (A) and 2 (B).
Moreover, 9/10 and 10/10 M. paniculata plants were Las-positive at time 0 in the first and second experiment replicates, with an average titer of 2.90 ± 0.10 log cell/g of tissue. The initial decrease in Las titer up to the 10–12th days was less severe than that in C. × sinensis, with an average titer of ∼2.5 log cell/g of tissue. The subsequent exponential increase was also less pronounced, and Las reached a maximum titer between the 40th and 60th days, with a population similar to that of the day of psyllid removal (∼3 log cell/g of tissue). After reaching this maximum, Las titer decreased slowly until it reached undetectable levels from the ∼180th and 170th days onward in the first and second experiment replicates, respectively (Figure 4).
In B. koenigii, the best model describing Las population progress was the negative exponential model (Table 1 and Supplementary Figure 1). At time 0, 6/8 and 6/10 B. koenigii plants showed Ct values lower than 34.0, in the first and second replicates, respectively. The average of the Las titer in NS at time 0 was 2.93 ± 0.15 log cells/g of tissue, but after this, a fast reduction in Las titer following psyllid removal was observed, reaching undetectable levels from the 30th and 10th days onward in the first and second replicates, respectively (Figure 4).
For the three species, the Gompertz model described sufficiently well the growth of NS (Table 2), with very active growth from 15 to approximately 45 days after pruning. The maximum rate of NS growth was found in C. × sinensis at days 25–30 in both experimental replicates, followed by B. koenigii at days 33–35 also in both experimental replicates. M. paniculata presented the lowest growth rate, reaching the maximum rate at days 20 and 23 in replicates 1 and 2, respectively (Figure 5 and Supplementary Table 5).
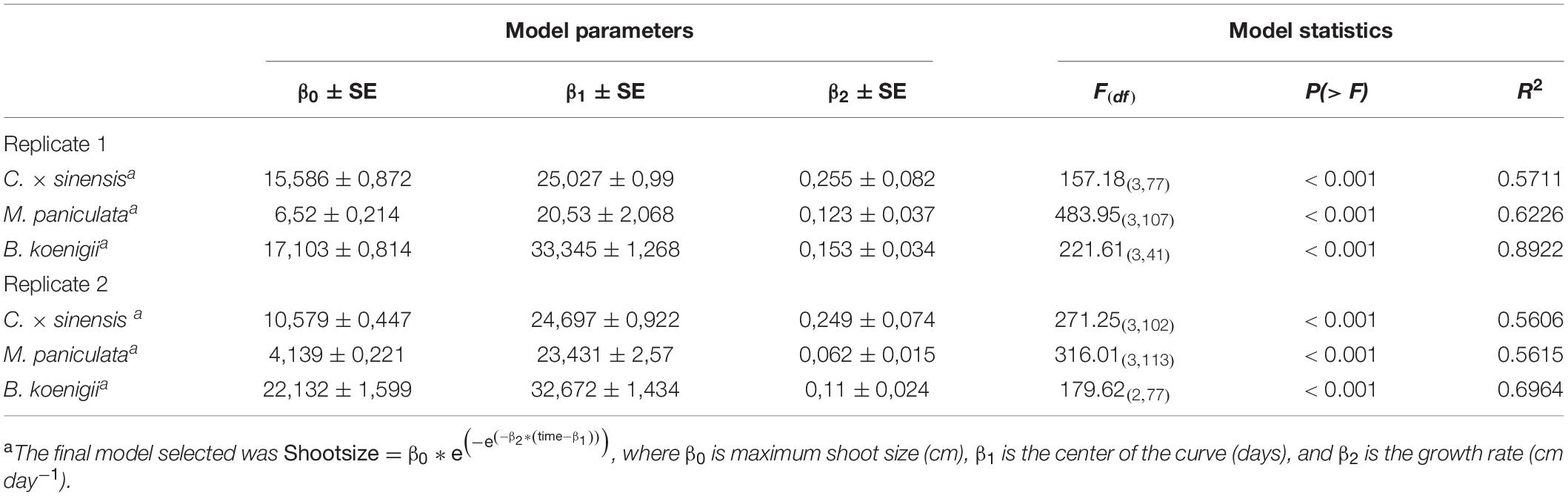
Table 2. Parameters of the Gompertz growth model of new shoots of Citrus × sinensis, Murraya paniculata, and Bergera koenigii.
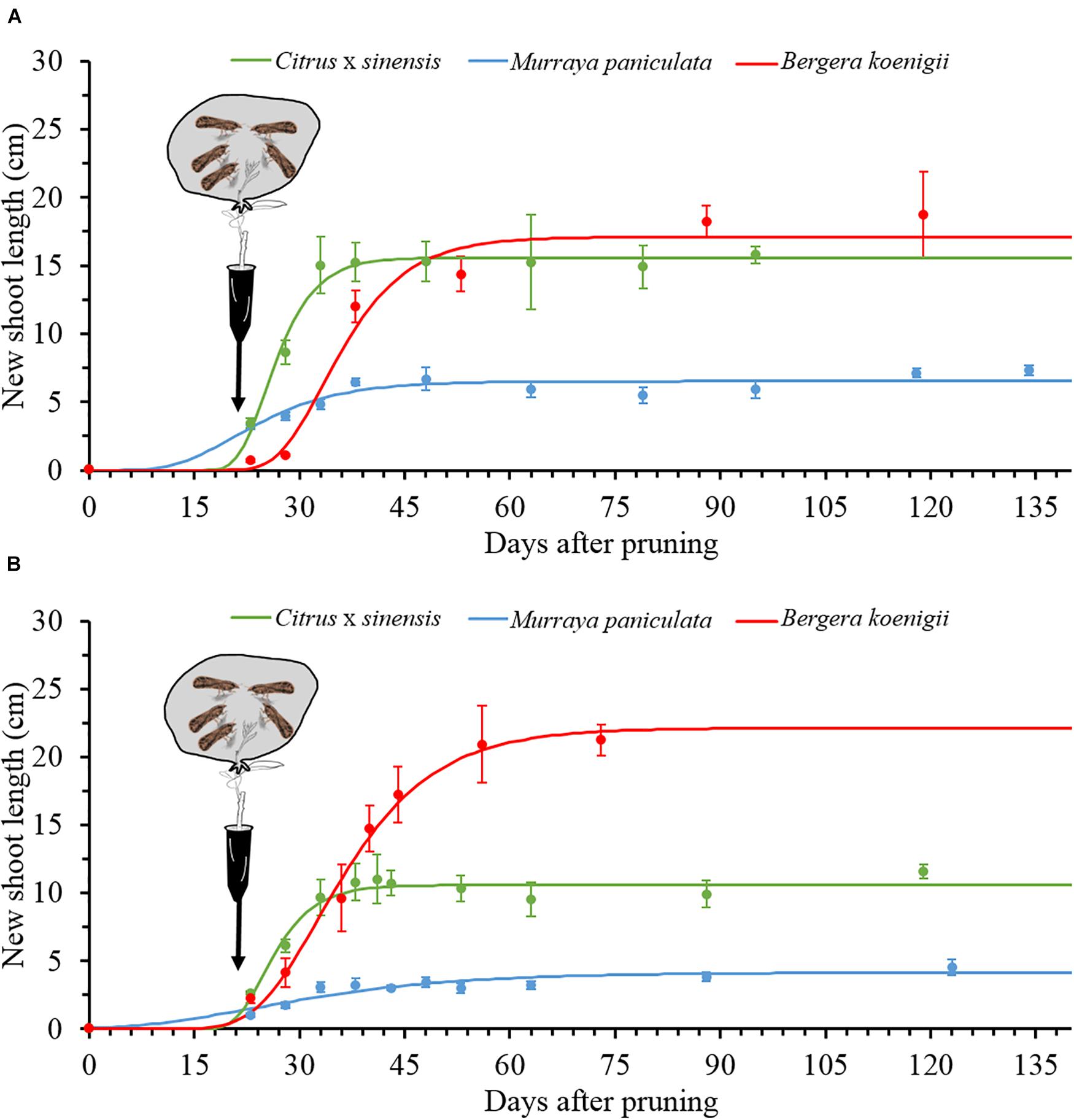
Figure 5. The Gompertz models for new shoot growth of Citrus × sinensis, M. paniculata, and B. koenigii in an acclimatized room at 25°C (black arrows indicate the day of psyllid confinement for a 48-h inoculation access period (IAP), (see section “Materials and Methods” for details), in experiment replicates 1 (A) and 2 (B).
In C. × sinensis, the time after 48 h of IAP in which NS was detached significantly affected the probability of a successful infection in the whole plant, evaluated by qPCR 1 year after the initial insect confinement (Table 3), indicating that Las was restricted to the NS during the first weeks post-inoculation. In C. × sinensis, the mean time for the NS to begin to serve as source of Las for the rest of the plant and thus to establish a successful infection was 21.8 days (∼15 to 30 days) in the first experiment replicate and 25.3 (∼18 to 33 days) in the second replicate. For M. paniculata, the results indicated that Las was almost unable to achieve whole-plant stable infections in the host (Figure 6), as only 18 out of 100 and 10 out of 106 seedlings in the first and second experiment replicates, respectively, were infected out of the NS at 12 months after psyllid inoculation, and such Las-positive plants were distributed randomly along the time points evaluated in both replicates (Supplementary Table 4). In B. koenigii, Las could not establish successful infections regardless of the NS detachment time.
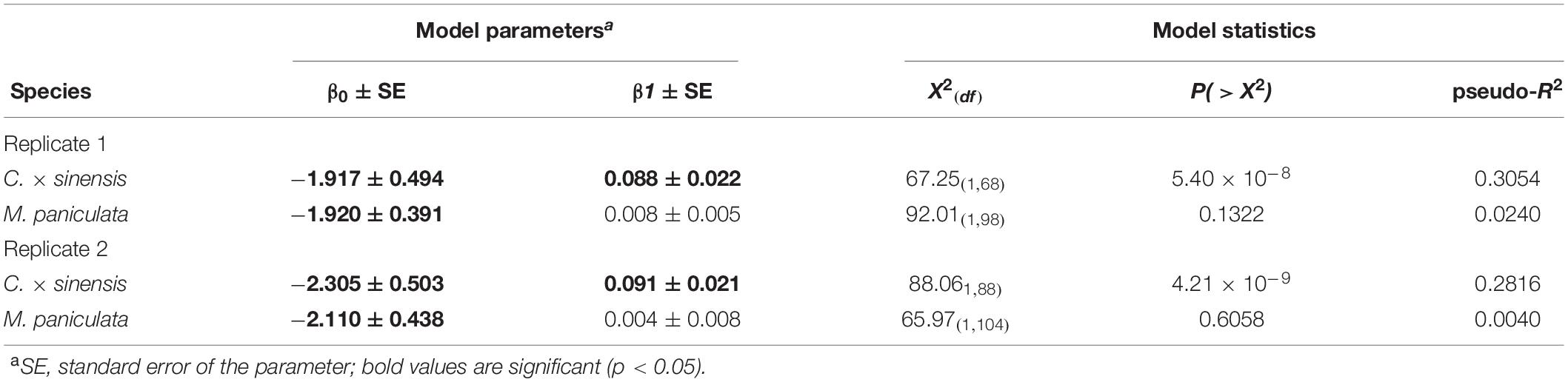
Table 3. Logistic models for the probability of a successful infection after 48 h of Las-positive inoculation by Diaphorina citri adults onto new shoots.
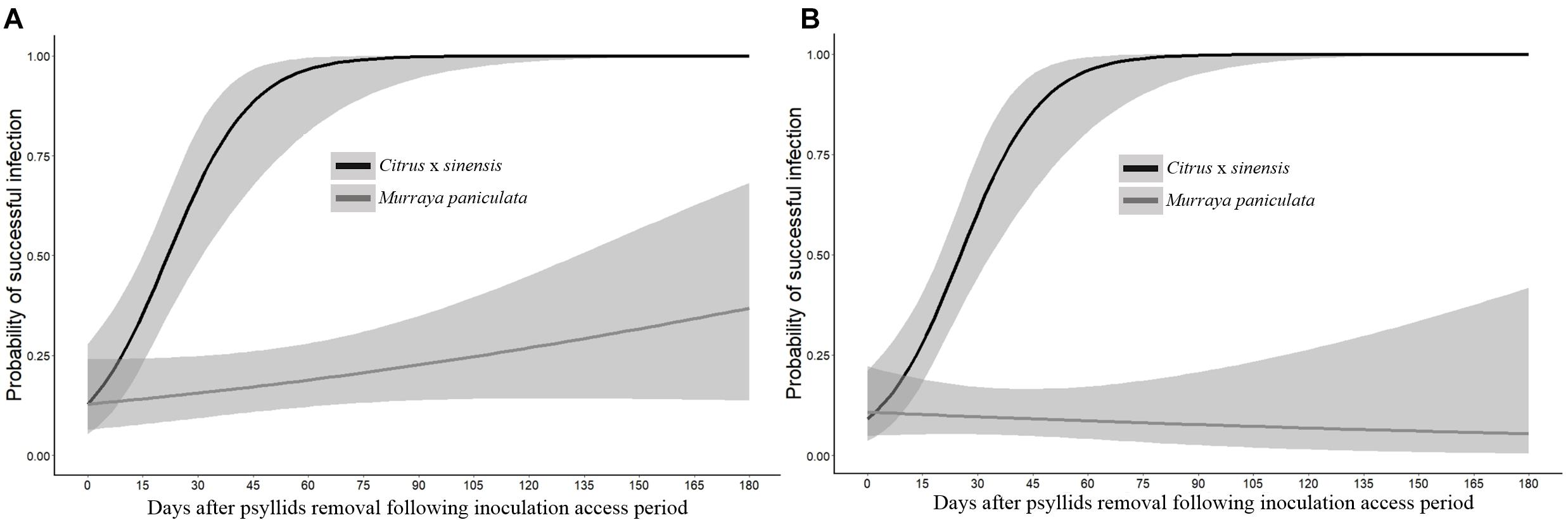
Figure 6. Probability of occurrence of a successful infection of the whole plant of Citrus × sinensis and M. paniculata after an initial 48 h of Las-exposed psyllid confinement and subsequent removal of new shoots at different time intervals in experiment replicates 1 (A) and 2 (B).
Discussion
In this study, we investigated the earliest steps of D. citri–Las–host interactions, which resulted in the establishment of either bacterial infection in the susceptible C. × sinensis ‘Valencia’ host or in resistance to Las in M. paniculata and B. koenigii. These two latter species were considered among the most suitable D. citri hosts among the Rutaceae, because volatile and visual cues made them more attractive than Citrus (Beloti et al., 2017; Tomaseto et al., 2019). The number of adult psyllids settled on the seedlings, number of eggs laid, and nymphs developed were also higher in M. paniculata and B. koenigii than in Citrus in host choice assays (Teck et al., 2011). However, we did not find differences among these three host plants in the ability of infected psyllids to inoculate Las into them, because Las showed similar titers at time 0 in NS of the three plant species, after the infected insects fed on them for 48 h. Although it has been reported that Las infection negatively affects D. citri performance (Ghanim et al., 2016) and induces important transcriptome reprogramming in the insect midgut (Yu et al., 2020), most infected D. citri individuals used in our experiments remained alive after 48 h of IAP and were efficient in transmitting Las to the three hosts. Morphological, developmental, and chemical differences among the three Rutaceae species (Beloti et al., 2018; Cifuentes-Arenas et al., 2019; Tomaseto et al., 2019) did not influence the ability of psyllids to overcome physical barriers and access sieve elements through their stylets to release the bacterium at relatively high titers. However, it is possible that Las acquisition by the psyllids may differ between these three host plants, whether due to the above differences or for other reasons including different Las titers in these hosts.
Once released into the phloem, bacterial titers decreased during the first 10–12 days after inoculation, reaching very low concentrations in the three species investigated. Such bacterial titer decline may be attributed, at least in part, to a dilution effect due to active NS growth a few days after pruning at the V2–V3 developmental stages in C. × sinensis, according to Cifuentes-Arenas et al. (2018). However, there was a clear lag between Las titer fall and NS enlargement, which occurred from 15 to 45 days after pruning. Moreover, Las decline was similar in the three species and quite comparable in the two replicates, while NS development followed slightly differing patterns in the two replicates, especially for C. × sinensis and B. koenigii (Figures 4, 5). Las has a reduced genome size and lacks genes involved in type 3 and most part of type 2 and 4 secretion systems, as well as those required for life in vitro (Duan et al., 2009). Indeed, it is only able to metabolize some sugars, which makes it dependent on phloem sap as a carbon source (Fagen et al., 2014), as it occurs in many other phloem-restricted bacteria (Huang et al., 2020). It is possible that Las needs some time to adapt to the new phloem environment to sequester metabolites from the host required to live and multiply. On the other hand, such initial bacterial titer decline does not occur when Liberibacter crescens strain BT-1, the only cultured wild-type strain of the Liberibacter genus, is grown in vitro under optimal nutritional and environmental conditions (Sena-Vélez et al., 2019). This suggests that the phloem does not provide the necessary metabolites to Las so fast and/or they need to be transformed for use by bacterial cells, as it occurs with sugars for spiroplasmas (André et al., 2005). Killiny (2016) proposed that the M. paniculata and B. koenigii phloem sap has poor nutrient qualities, which may be associated with the limited or lack of Las multiplication in these two species observed in later time-course phases, but not during the first 12 days after inoculation, because the bacterial titer decrease was similar in C. × sinensis, an excellent Las host.
Alternatively, bacterial titer decrease may be caused as a consequence of defense responses induced by plant cells to either psyllid damage through herbivore-associated molecular patterns or to Las through microbe-associated molecular patterns (Huang et al., 2020). Although it is unclear whether the latter occurs in phloem sieve elements (Jiang et al., 2019), it has been recently found that Las can attach and move into nucleated cells associated with the phloem, at least in seed coat phloem tissue (Achor et al., 2020), which would likely activate immune cell responses. Moreover, such a drastic and consistent decline in bacterial titers in different plant species at a few days post-inoculation may explain, at least in part, the relatively low efficiency of Las inoculation and the frequent failures in attempts to inoculate Las into citrus plants through psyllids under controlled conditions (Pelz-Selinski et al., 2010; Ammar et al., 2016; Canale et al., 2017). Therefore, Las should be able to overcome such a lag, probably caused by the presence of physical and chemical obstacles, to remain alive and proceed with the infection process. It is known that the Las genome encodes a functional salicylic acid hydroxylase capable of degrading salicylic acid to neutralize plant defenses (Li et al., 2017). Moreover, the Las prophage gene SC2-gp095 encodes a ROS-scavenging peroxidase, which may assist Las in evading plant defense responses (Jain et al., 2015).
After the decrease phase, the Las concentration increased exponentially until it reached a titer of approximately 5 log Las cells/g of NS tissue at 45 days, which was maintained stationary in the susceptible C. × sinensis host. Other studies conducted with already Las-infected plants, inoculated by grafting, obtained similar results in terms of stationary phase titers in susceptible citrus genotypes (Hilf and Luo, 2018; Raiol-Junior et al., 2021a). However, in our study, there was a lag of approximately 15–30 days for Las to reach a plateau of 5 log Las cells/g of tissue compared to the previous studies, which may be due to differences in the initial inoculum titers (budwoods vs. psyllids), a dose–effect already reported (Raiol-Junior et al., 2017), which seems to lose relevance once Las is established (Cifuentes-Arenas et al., 2019). An average of 23.6 (16.5–31.5) days of post-IAP was required for Las to move out of 50% of the NS and colonize the rest of the plant, causing a successful infection, that is, for the initially infected shoot to serve as a source of Las for the rest of the C. × sinensis plant. This occurred several months before Las-secreted proteins may be suitable for interaction with host cell enzymes to induce disease symptoms (Clark et al., 2018; Pang et al., 2020), including conspicuous callose deposition on sieve plates and consequent leaf mottling in infected C. × sinensis plants (Kim et al., 2009; Achor et al., 2010). Other phloem responses arising just after Las phloem translocation from infected tissues, but much earlier than the expression of symptoms, remain poorly understood.
In M. paniculata, Las population dynamics were similar to those of C. × sinensis up to the 30th day. Las reached a semi-stationary phase for about 15–30 days, and then bacterial titers declined progressively to become undetectable at 180 days onward. Moreover, Las translocation from the NS to the rest of the plant was rare and uneven. In previous controlled experiments with M. paniculata, the earliest samplings were taken from the second to fourth months post-IAP (Damsteegt et al., 2010; Cifuentes-Arenas et al., 2019). However, the continuous decrease in Las titers to undetectable levels confirms previous consideration of M. paniculata as a transient host for Las (Damsteegt et al., 2010; Cifuentes-Arenas et al., 2019). Even at low titers, the transitory presence of Las in M. paniculata (Damsteegt et al., 2010; Walter et al., 2012; Cifuentes-Arenas et al., 2019) suggests that this species may be a suitable host for Las spread. However, Las transmission from M. paniculata to C. × sinensis by D. citri was observed at very low frequencies (less than 1%) in controlled experiments and only when environmental and plant developmental conditions were highly favorable for Las infection (Cifuentes-Arenas et al., 2019). Indeed, both the transient nature of Las infection and its low titers when the bacterium remains detectable in this host explain the 43% reduction in HLB incidence in a sweet orange orchard when M. paniculata was used as a trap crop fence to efficiently attract and control the D. citri population and its spread (Tomaseto et al., 2019).
In contrast, Las could not be established in NS of B. koenigii, as shown previously by Beloti et al. (2018). However, we were able to detect Las multiplication in B. koenigii NS in one of the experiment replicates, indicating that under specific, highly suitable conditions, the bacterium could attain low but clearly detectable concentrations in the inoculated shoots, although only occasionally. Moreover, the probability of Las moving out of the NS was null in the B. koenigii. Whether M. paniculata and B. koenigii phloem sap are unsuitable for Las survival (Killiny, 2016) and/or their cells activate efficient defense responses to hold bacterial infections remains to be further investigated. Nevertheless, B. koenigii is more efficient than M. paniculata in limiting Las. Considering the different responses of Las infection between Murraya paniculata and Bergera koenigii, their close phylogenetic relationships, and the wide diversity of Murraya and Bergera species found in Asia (Nguyen et al., 2019), the interactions of the two genera with Las is worth investigating, as they seem to represent hosts at the frontier between resistance and susceptibility to the bacterium.
This is the first study to investigate the early population dynamics of Las in three species with contrasting susceptibility/resistance responses to this bacterium, by simulating the natural Las inoculation system using infective D. citri and evaluating the presence of Las in NS by qPCR at different short time intervals post-IAP. Using this controlled and reproducible system, we were able to expose all the plants to similar initial inoculum pressure, as they received the same initial bacterial load injected into the phloem by psyllids and the environmental conditions were adequate to maximize the chances of successful infection occurrence (Raiol-Junior et al., 2021b). Our results allowed us to identify the key time points of Las growth in plant genotypes with different responses to Las infection. This information could be essential to investigating whether or when plant cell defense responses are activated after wounding and bacterial release into the phloem by D. citri, as well as how Las is able to evade such responses in C. × sinensis but not in B. koenigii and only partially in M. paniculata. It may also help to identify suitable targets to be knocked out or overexpressed biotechnologically with the aim of creating HLB-resistant varieties.
Data Availability Statement
The original contributions presented in the study are included in the article/Supplementary Material, further inquiries can be directed to the corresponding author.
Author Contributions
MA, LP, and JF conceptualized the work. MA, LP, JF, and J-CA designed the work. MA, J-CA, and LR-J collected the data. J-CA performed the statistical analysis. MA, J-CA, LR-J, JF, and LP contributed to interpretation, drafting the article, and critical revision of the manuscript. All authors contributed to the article and approved the submitted version.
Funding
This study was financed in part by Fundecitrus, grant no. 817526 from the European Union H2020 Innovation Action Program and Project PID2019-104569RB-I00 from the AEI-Spain, the Coordenação de Aperfeiçoamento de Pessoal de Nível Superior - Brazil (CAPES) - Finance Code 001. MA is recipient of a Ph.D. fellowship from CAPES. JF is recipient of a fellowship grant (#312089/2019-8) from Conselho Nacional de Desenvolvimento Científico e Tecnológico – Brazil (CNPq).
Conflict of Interest
The authors declare that the research was conducted in the absence of any commercial or financial relationships that could be construed as a potential conflict of interest.
Acknowledgments
We thank André Luís Sanches from Fundecitrus for his help with grafting for propagation and plant maintenance, as well as Berta Alquézar and Jose Gadea from the Universidad Politécnica de Valencia and Nelson A. Wulff and Silvio A. Lopes from Fundecitrus for their invaluable comments during the design of this work. We also thank Fernanda Benedito and Priscila Alves from Fundecitrus for their assistance with DNA extraction and qPCR analysis.
Supplementary Material
The Supplementary Material for this article can be found online at: https://www.frontiersin.org/articles/10.3389/fmicb.2021.683923/full#supplementary-material
References
Achor, D. S., Etxeberria, E., Wang, N., Folimonova, S. Y., Chung, K. R., and Albrigo, L. G. (2010). Sequence of anatomical symptom observations in Citrus affected with Huanglongbing disease. Plant Pathol. J. 9, 56–64. doi: 10.3923/ppj.2010.56.64
Achor, D. S., Welker, S., Ben-Mahmoud, S., Wang, C., Folimonova, S. Y., Dutt, M., et al. (2020). Dynamics of Candidatus Liberibacter asiaticus movement and sieve-pore plugging in Citrus sink cells. Plant Physiol. 188, 882–891. doi: 10.1104/pp.19.01391
Alves, M. N., Lopes, S. A., Raiol-Junior, L. L., Wulff, N. A., Girardi, E. A., Ollitrault, P., et al. (2021). Resistance to ‘Candidatus Liberibacter asiaticus,’ the Huanglongbing associated bacterium, in sexually and/or graft-compatible Citrus relatives. Front. Plant Sci. 11:617664. doi: 10.3389/fpls.2020.617664
Ammar, E.-D., Ramos, J. E., Hall, D. G., Dawson, W. O., and Shatters, R. G. Jr. (2016). Acquisition, replication and inoculation of Candidatus Liberibacter asiaticus following various acquisition periods on Huanglongbing-infected Citrus by nymphs and adults of the Asian Citrus Psyllid. PLoS ONE 11:e0159594. doi: 10.1371/journal.pone.0159594
André, A., Maucourt, M., Moing, A., Rolin, D., and Renaudin, J. (2005). Sugar import and phytopathogenicity of Spiroplasma citri: Glucose and fructose play distinct roles. MPMI 18, 33–42. doi: 10.1094/MPMI-18-0033
Bassanezi, R. B., Lopes, S. A., Miranda, M. P., Wulff, N. A., Volpe, H. X. L., and Ayres, A. J. (2020). Overview of citrus huanglongbing spread and management strategies in Brazil. Trop. Plant Pathol. 45, 251–264. doi: 10.1007/s40858-020-00343-y
Bassanezi, R. B., Montesino, L. H., and Stuchi, E. S. (2009). Effects of huanglongbing on fruit quality of sweet orange cultivars in Brazil. Eur. J. Plant Pathol. 125, 565–572. doi: 10.1007/s10658-009-9506-3
Beloti, V. H., Alves, G. R., Coletta-Filho, H. D., and Yamamoto, P. T. (2018). The Asian Citrus psyllid host Murraya koenigii is immune to Citrus huanglongbing pathogen ‘Candidatus Liberibacter asiaticus’. Phytopathology 108, 1089–1094. doi: 10.1094/PHYTO-01-18-0012-R
Beloti, V. H., Santo, F., Alves, G. R., Bento, J. M. S., and Yamamoto, P. T. (2017). Curry leaf smells better than citrus to females of Diaphorina citri (Hemiptera: Liviidae). Arthropod-Plant Interact. 11, 709–716. doi: 10.1007/s11829-017-9524-6
Bonani, J. P., Fereres, A., Garzo, E., Miranda, M. P., Appezato-Da-Gloria, B., and Lopes, J. R. S. (2010). Characterization of electrical penetration graphs of the Asian citrus psyllid, Diaphorina citri, in sweet orange seedlings. Entomol. Exp. Appl. 134, 35–49. doi: 10.1111/j.1570-7458.2009.00937.x
Bové, J. M. (2006). Huanglongbing: a destructive, newly-emerging, century-old disease of citrus. J. Plant Pathol. 88, 7–37. doi: 10.4454/jpp.v88i1.828
Canale, M. C., Tomaseto, A. F., Haddad, M. L., Coletta-Filho, H. D., and Lopes, J. R. S. (2017). Latency and persistence of ‘Candidatus Liberibacter asiaticus’ in its psyllid vector, Diaphorina citri (Hemiptera: Liviidae). Phytopathology 107, 264–272. doi: 10.1094/PHYTO-02-16-0088-R
Capoor, S., Rao, D., and Viswanath, S. (1967). Diaphorina citri Kuway., a vector of the greening disease of citrus in India. Indian J. Agric. Sci. 37, 572–579.
Carmo-Souza, M., Garcia, R. B., Wulff, N. A., Fereres, A., and Miranda, M. P. (2020). Drench application of systemic insecticides disrupts probing behavior of Diaphorina citri (Hemiptera: Liviidae) and inoculation of Candidatus Liberibacter asiaticus. Insects 11, 314–325. doi: 10.3390/insects11050314
Cifuentes-Arenas, J. C., Beattie, G. A. C., Peña, L., and Lopes, S. A. (2019). Murraya paniculata and Swinglea glutinosa as short-term transient hosts of ‘Candidatus Liberibacter asiaticus’ and implications for the spread of huanglongbing. Phytopathology 109, 2064–2073. doi: 10.1094/PHYTO-06-19-0216-R
Cifuentes-Arenas, J. C., de Goes, A., de Miranda, M. P., Beattie, G. A. C., and Lopes, S. A. (2018). Citrus flush shoot ontogeny modulates biotic potential of Diaphorina citri. PLoS One 13:e0190563. doi: 10.1371/journal.pone.0190563
Clark, K., Franco, J. Y., Schwizer, S., Pang, Z., Hawara, E., Liebrand, T. W. H., et al. (2018). An effector from the Huanglongbing-associated pathogen targets citrus proteases. Nature communications 9, 1718. doi: 10.1038/s41467-018-04140-9
Damsteegt, V. D., Postnikova, E. N., and Stone, A. L. (2010). Murraya paniculata and related species as potential hosts and inoculum reservoirs of ‘Candidatus Liberibacter asiaticus’, causal agent of Huanglongbing. Plant Dis. 94, 528–533. doi: 10.1094/PDIS-94-5-0528
Desjardins, P., and Conklin, D. (2010). NanoDrop microvolume quantitation of nucleic Acids. J. Vis. Exp. 45, e2565. doi: 10.3791/2565
Duan, Y., Zhou, L., Hall, D. G., Li, W., Doddapaneni, H., Lin, H., et al. (2009). Complete genome sequence of Citrus Huanglongbing bacterium, ‘Candidatus Liberibacter asiaticus’ obtained through metagenomics. MPMI 22, 1011–1020.
Fagen, J. R., Leonard, M. T., Coyle, J. F., McCullough, C. M., Davis-Richardson, A. G., Davis, M. J., et al. (2014). Liberibacter crescens gen. nov., sp. nov., the first cultured member of the genus Liberibacter. Int. J. Syst. Evol. Microbiol. 64, 2461–2466. doi: 10.1099/ijs.0.063255-0
Folimonova, S. Y., Robertson, C. J., Gamsey, S. M., and Dawson, W. O. (2009). Examination of the responses of different genotypes of citrus to huanglongbing (citrus greening) under different conditions. Phytopathology 23, 1346–1354. doi: 10.1094/PHYTO-99-12-1346
Fundecitrus. (2020). Doenças: Greening/HLB. Available online at: https://www.fundecitrus.com.br/levantamentos/greening (accessed March 21, 2020).
Ghanim, M., Fattah-Hosseini, S., Levy, A., and Cilia, M. (2016). Morphological abnormalities and cell death in the Asian citrus psyllid (Diaphorina citri) midgut associated with Candidatus Liberibacter asiaticus. Sci. Rep. 6, 33418. doi: 10.1038/srep33418
Gottwald, T. R. (2010). Current epidemiological understanding of citrus Huanglongbing. Annu. Rev. Phytopathol 48, 119–139. doi: 10.1146/annurev-phyto-073009-114418
Hilf, M. E., and Luo, W. (2018). Dynamics of ‘Candidatus Liberibacter asiaticus’ colonization of new growth of citrus. Phytopathology 108, 1165–1171. doi: 10.1094/PHYTO-12-17-0408-R
Hogenhout, S. A., and Bos, J. I. (2011). Effector proteins that modulate plant–insect interactions. Curr. Opin. Plant Biol. 14, 422–428. doi: 10.1016/j.pbi.2011.05.003
Huang, W., Reyes-Caldas, P., Mann, M., Seifbarghi, S., Kahn, A., Almeida, R. P. P., et al. (2020). Bacterial vector-borne plant diseases: unanswered questions and future directions. Mol. Plant. 13, 1379–1393. doi: 10.1016/j.molp.2020.08.010
Inoue, H., Ohnish, J., Ito, T., Tomimur, K., Miyata, S., and Ashihara, W. (2009). Enhanced proliferation and efficient transmission of Candidatus Liberibacter asiaticus by adult Diaphorina citri after acquisition feeding in the nymphal stage. Ann. Appl. Biol. 155, 29–36. doi: 10.1111/j.1744-7348.2009.00317.x
Jain, M., Fleites, L. A., and Gabriel, D. W. (2015). Prophage-encoded peroxidase in ‘Candidatus Liberibacter asiaticus’ is a secreted effector that suppresses plant defenses. Mol. Plant Microbe Interact. 12, 1330–1337. doi: 10.1094/MPMI-07-15-0145-R
Jiang, Y., Zhang, C.-X., Chen, R., and He, S. Y. (2019). Challenging battles of plants with phloem-feeding insects and prokaryotic pathogens. PNAS. 116, 23390–23397. doi: 10.1073/pnas.1915396116
Johnson, E. G., Wu, J., Bright, D. B., and Graham, J. H. (2014). Association of ‘Candidatus Liberibacter asiaticus’ root infection, but not phloem plugging with root loss on huanglongbing-affected trees prior to appearance of foliar symptoms. Plant Pathol. 63, 290–298. doi: 10.1111/ppa.12109
Killiny, N. (2016). Metabolomic comparative analysis of the phloem sap of curry leaf tree (Bergera koenegii), orange jasmine (Murraya paniculata), and Valencia sweet orange (Citrus sinensis) supports their differential responses to Huanglongbing. Plant signal. behav. 11, e1249080. doi: 10.1080/15592324.2016.1249080
Kim, J.-S., Sagaram, U. S., Burns, J. K., Li, J.-L., and Wang, N. (2009). Response of sweet orange (Citrus sinensis) to ‘Candidatus Liberibacter asiaticus’ Infection: Microscopy and Microarray Analyses. Phytopathology 99, 50–57. doi: 10.1094/PHYTO-99-1-0050
Li, J., Pang, Z., Trivedi, P., Zhou, X., Ying, X., Jia, H., et al. (2017). ‘Candidatus Liberibacter asiaticus’ encodes a functional salicylic acid (SA) hydroxylase that degrades SA to suppress plant defenses. MPMI 30, 620–630. doi: 10.1094/MPMI-12-16-0257-R
Li, W., Hartung, J. S., and Levy, L. (2006). Quantitative real-time PCR for detection and identification of Candidatus Liberibacter species associated with citrus huanglongbing. J. Microbiol. Methods 66, 104–115. doi: 10.1016/j.mimet.2005.10.018
Li, W., Levy, L., and Hartung, J. S. (2009). Quantitative distribution of “Candidatus Liberibacter asiaticus” in citrus plants with citrus huanglongbing. Phytopathology 99, 139–144. doi: 10.1094/PHYTO-99-2-0139
Lopes, S. A., and Cifuentes-Arenas, J. C. (2021). A protocol for successful transmission of ‘Candidatus Liberibacter asiaticus’ from citrus to citrus using Diaphorina citri. Phytopathology doi: 10.1094/PHYTO-02-21-0076-R [Epub ahead of print].
Lopes, S. A., Frare, G. F., Bertolni, E., Cambra, M., Fernandes, N. G., Ayres, A. J., et al. (2009). Liberibacters associated with citrus huanglongbing in Brazil: ‘Candidatus Liberibacter asiaticus’ is heat tolerant, ‘Ca. L. americanus’ is heat sensitive. Plant Dis. 3, 257–262. doi: 10.1094/PDIS-93-3-0257
Lopes, S. A., Luiz, F. Q. B. Q., Martins, E. C., Fassini, C. G., Barbosa, J. C., and Beattie, G. A. C. (2013). Candidatus Liberibacter asiaticus titers in citrus and acquisition rates by Diaphorina citri are decreased by higher temperature. Plant Dis. 97, 1563–1570. doi: 10.1094/PDIS-11-12-1031-RE
Manjunath, K. L., Halbert, S. E., Ramadugu, C., Weeb, S., and Lee, R. F. (2008). Detection of ‘Candidatus Liberibacter asiaticus’ in Diaphorina citri and its importance in the management of Citrus Huanglongbing in Florida. Phytopathology 98, 387–396. doi: 10.1094/PHYTO-98-4-0387
Murray, M. G., and Thompson, W. F. (1980). Rapid isolation of high molecular weight plant DNA. Nucleic Acids Res. 8, 4321–4325. doi: 10.1093/nar/8.19.4321
Nguyen, C. H., Beattie, A. C., Haigh, A. M., Astuti, I. P., Mabberley, D. J., Weston, P. H., et al. (2019). Molecular differentiation of the Murraya paniculata Complex (Rutaceae: Aurantioideae: Aurantieae). BMC Evol. Biol. 19:236. doi: 10.1186/s12862-019-1555-4
Pandey, S. S., Vasconcelos, F. N. C., and Wang, N. (2021). Spatiotemporal dynamics of Candidatus Liberibacter asiaticus colonization inside citrus plant and Huanglongbing disease development. Phytopathology doi: 10.1094/PHYTO-09-20-0407-R [Epub ahead of print].
Pandey, S. S., and Wang, N. (2019). Targeted early detection of Citrus Huanglongbing causal agent ‘Candidatus Liberibacter asiaticus’ before symptom expression. Phytopathology 109, 952–959. doi: 10.1094/PHYTO-11-18-0432-R
Pang, Z., Zhang, L., Coaker, G. L., Ma, W., He, S. Y., and Wang, N. (2020). Citrus CsACD2 is a target of Candidatus Liberibacter asiaticus in Huanglongbing disease. Plant Physiol. 184, 792–805. doi: 10.1104/pp.20.00348
Pelz-Selinski, K. S., Brlansky, R. H., Ebert, T. A., and Rogers, M. E. (2010). Transmission parameters for Candidatus Liberibacter asiaticus by Asian Citrus Psyllid (Hemiptera: Psyllidae). J. Econ. Entomol. 103, 1531Ð1541. doi: 10.1603/EC10123
Peng, C.-Y. J., Le, K. L., and Ingersoll, G. M. (2002). An introduction to Logistic regression analysis and reporting. J. Educ. Res. 96, 3–14. doi: 10.1080/00220670209598786
Raiol-Junior, L. L., Baia, A. D. B., Luiz, F. Q. B. F., Fassini, C. G., Marques, V. V., and Lopes, S. A. (2017). Improvement in the excised citrus leaf assay to investigate inoculation of ‘Candidatus Liberibacter asiaticus’ by the Asian Citrus Psyllid Diaphorina citri. Plant Dis 101, 409–413. doi: 10.1094/PDIS-08-16-1093-RE
Raiol-Junior, L. L., Cifuentes-Arenas, J. C., Carvalho, E. V., Girardi, E. A., and Lopes, S. A. (2021a). Evidence that ‘Candidatus Liberibacter asiaticus’ moves predominantly towards new tissue growth in citrus plants. Plant Dis. 105, 34–42. doi: 10.1094/PDIS-01-20-0158-RE
Raiol-Junior, L. L., Cifuentes-Arenas, J. C., Cunniffe, N. J., Turgeon, R., and Lopes, S. A. (2021b). Modelling ‘Candidatus Liberibacter asiaticus’ movement within citrus plants. Phytopathology doi: 10.1094/PHYTO-12-20-0559-R [Epub ahead of print].
Ramadugu, C., Keremane, M. L., Halbert, S. E., Duan, Y. P., Roose, M. L., Stover, E., et al. (2016). Long-term field evaluation reveals huanglongbing resistance in Citrus relatives. Plant Dis. 9, 1858–1869. doi: 10.1094/PDIS-03-16-0271-RE
Sena-Vélez, M., Holland, S. D., Aggarwal, M., Cogan, N. G., Jain, M., Gabriel, D. W., et al. (2019). Growth dynamics and survival of Liberibacter crescens BT-1, an important model organism for the citrus Huanglongbing pathogen “Candidatus Liberibacter asiaticus.”. Appl. Environ. Microbiol. 85, e1656–e1619. doi: 10.1128/AEM.01656-19
Singerman, A., and Useche, P. (2016). Impact of Citrus greening on Citrus operations in Florida. Available online at: https://edis.ifas.ufl.edu/fe983#:~{}:text=Summary,an%20increased%20cost%20of%20production (accessed March 09, 2021).
Skelley, L. H., and Hoy, M. A. (2004). A synchronous rearing method for the asian citrus psyllid and its parasitoids in quarantine. Biol. Control. 29, 14–23. doi: 10.1016/S1049-9644(03)00129-4
Swingle, W. T., and Reece, C. (1967). “The botany of Citrus and its wild relatives,” in The Citrus Industry, eds W. Reuther, H. J. Webber, and L. D. Batchelor (Berkeley, CA: University of California Press), 191–430.
Tatineni, S., Sagaram, U. S., Gowda, S., Robertson, C. J., Dawson, W. O., Iwanami, T., et al. (2008). In planta distribution of ‘Candidatus Liberibacter asiaticus’ as revealed by polymerase chain reaction (PCR) and real-time PCR. Phytopathology 98, 592–599. doi: 10.1094/PHYTO-98-5-0592
Teck, S. L. X., Fatimah, A., Beattie, A., Heng, R. K. J., and King, W. S. (2011). Influence of host plant species and flush growth stage on the Asian Citrus Psyllid, Diaphorina citri Kuwayama. Am. J. Agric. Biol. Sci. 6, 536–543. doi: 10.3844/ajabssp.2011.536.543
Teixeira, D. C., Danet, J. L., Eveillard, S., Martins, E. C., Junior, W. C. J., Yamamoto, P. T., et al. (2005). Citrus huanglongbing in São Paulo State, Brazil: PCR detection of the ‘Candidatus’ Liberibacter species associated with the disease. Mol. Cell. Probes 19, 173–179. doi: 10.1016/j.mcp.2004.11.002
Tjørve, K. M. C., and Tjørve, E. (2017). The use of Gompertz models in growth analyses, and new Gompertz-model approach: An addition to the unified-richards family. PLoS One 12:e0178691. doi: 10.1371/journal.pone.0178691
Tomaseto, A. F., Marques, R. N., Fereres, A., Zanardi, O. A., Volpe, H. X. L., Alquézar, B., et al. (2019). Orange jasmine as a trap crop to control Diaphorina citri. Sci. Rep. 9, 2070. doi: 10.1038/s41598-019-38597-5
Walter, A. J., Duan, Y. P., and Hall, D. G. (2012). Titers of ‘Ca. Liberibacter asiaticus’ in Murraya paniculata and Murraya-reared Diaphorina citri are much lower than in Citrus and Citrus-reared psyllids. Hortscience 47, 1449–1452. doi: 10.21273/HORTSCI.47.10.1449
Westbrook, C. J., Hall, D. G., Stover, E., Duan, Y. P., and Lee, R. F. (2011). Colonization of Citrus and Citrus-related germplasm by Diaphorina citri (Hemiptera: Psyllidae). Hortscience 46, 997–1005. doi: 10.21273/HORTSCI.46.7.997
Wu, T., Lou, X., Xu, C., Wu, F., Qureshil, W. A., and Cen, Y. (2016). Feeding behavior of Diaphorina citri and its transmission of ‘Candidatus Liberibacter asiaticus’ to citrus. Entomol. Exp. Appl 161, 104–111. doi: 10.1111/eea.12496
Keywords: HLB, Las multiplication, Citrus spp., Murraya paniculata, Bergera koenigii, bacterial growth, qPCR
Citation: Alves MN, Cifuentes-Arenas JC, Raiol-Junior LL, Ferro JA and Peña L (2021) Early Population Dynamics of “Candidatus Liberibacter asiaticus” in Susceptible and Resistant Genotypes After Inoculation With Infected Diaphorina citri Feeding on Young Shoots. Front. Microbiol. 12:683923. doi: 10.3389/fmicb.2021.683923
Received: 22 March 2021; Accepted: 29 April 2021;
Published: 09 June 2021.
Edited by:
Lukasz Lech Stelinski, University of Florida, United StatesReviewed by:
Greg McCollum, Horticultural Research Laboratory (USDA-ARS), United StatesCopyright © 2021 Alves, Cifuentes-Arenas, Raiol-Junior, Ferro and Peña. This is an open-access article distributed under the terms of the Creative Commons Attribution License (CC BY). The use, distribution or reproduction in other forums is permitted, provided the original author(s) and the copyright owner(s) are credited and that the original publication in this journal is cited, in accordance with accepted academic practice. No use, distribution or reproduction is permitted which does not comply with these terms.
*Correspondence: Leandro Peña, lpenya@fundecitrus.com.br