- 1Grupo de Ecología y Medio Ambiente, Departamento de Agronomía, Biotecnología y Alimentación, Universidad Pública de Navarra, Pamplona, Spain
- 2Centro Jerónimo de Ayanz, Institute on Innovation & Sustainable Development in Food Chain, Pamplona, Spain
- 3Instituto de Recursos Naturales y Agrobiología de Salamanca (CSIC), Salamanca, Spain
The plant microbiome is likely to play a key role in the resilience of communities to the global climate change. This research analyses the culturable fungal mycobiota of Brachypodium rupestre across a sharp gradient of disturbance caused by an intense, anthropogenic fire regime. This factor has dramatic consequences for the community composition and diversity of high-altitude grasslands in the Pyrenees. Plants were sampled at six sites, and the fungal assemblages of shoots, rhizomes, and roots were characterized by culture-dependent techniques. Compared to other co-occurring grasses, B. rupestre hosted a poorer mycobiome which consisted of many rare species and a few core species that differed between aerial and belowground tissues. Recurrent burnings did not affect the diversity of the endophyte assemblages, but the percentages of infection of two core species -Omnidemptus graminis and Lachnum sp. -increased significantly. The patterns observed might be explained by (1) the capacity to survive in belowground tissues during winter and rapidly spread to the shoots when the grass starts its spring growth (O. graminis), and (2) the location in belowground tissues and its resistance to stress (Lachnum sp.). Future work should address whether the enhanced taxa have a role in the expansive success of B. rupestre in these anthropized environments.
Introduction
The study of the plant microbiome is a powerful tool that contributes to the understanding and prediction of the resilience of plant communities to global change (Vandenkoornhuyse et al., 2015). In extreme environments, where ecosystems are fragile and very sensitive to changes, the plant microbiota is crucial to understand the adaptive capacity of plant communities. Low temperatures, soil acidity, low rates of mineralization, and low nutrient availability are some of the common traits of artic and alpine ecosystems, where the study of the composition and function of the plant–microbial consortium has been addressed in the last decades (Li et al., 2012; Poosakkannu et al., 2014; Rudgers et al., 2014; Bråthen et al., 2015; Kotilínek et al., 2017).
Current research reports that for a particular ecosystem, the composition and diversity of the fungal microbiome of a plant host depends on its potential for carbon provision (plant abundance and biomass), on the fungal propagule availability, and on environmental factors, mainly climate, rather than on the taxonomy of the plant host (Higgins et al., 2014; Ranelli et al., 2015; Glynou et al., 2016; Kivlin et al., 2019). The first factor, potential for carbon provision, gives support to the resource-diversity hypothesis, the highest plant carbon determines the highest guest diversity (Wang et al., 2020), and this effect is modulated by the richness and diversity of the neighboring host plants and their own fungal populations (Hiiesalu et al., 2017; Vannier et al., 2020). Regarding the climate, the historical and current precipitation regime is known to play a key role in fungal endophyte composition (Lau et al., 2013; Liu et al., 2017), and its consequences in a scenario of climate change are a growing subject of study in recent years (Giauque and Hawkes, 2016; Koide et al., 2017; Slaughter et al., 2018).
In addition to the climate change, plant communities in some cold environments are being affected by other significant mechanisms of change (Pauchard et al., 2009). Many high altitude grasslands undergo profound changes due to the disruption of the historical regime of disturbances that created and preserved them (Lasanta-Martínez et al., 2005; Komac et al., 2013). Fire and herbivory are two crucial disturbances shaping the landscape of natural grasslands worldwide (Archibald et al., 2005; Anderson and Hoffman, 2007). Extensive grazing by livestock in highlands has sharply declined in Europe in the last decades. Grazing is considered the major driver of plant guild composition and diversity in grassland communities (Milchunas et al., 1988; Canals and Sebastia, 2000; Frank, 2005; Eldridge and Delgado-Baquerizo, 2018). Grazing has profound effects on soils, promoting root exudation and carbon flow exchange to the rhizosphere (Dawson et al., 2000; Hamilton and Frank, 2001), enhancing soil microbial biomass and activating the soil biogeocycles (Bardgett and Wardle, 2003; Shaw et al., 2016), increasing and redistributing nutrients availability (Augustine et al., 2003; Liu et al., 2017) and affecting soil aeration by compaction (Jing et al., 2014). Through these effects on plants and soils, grazing may affect the structure of the fungal microbiome of plants (Schulz and Boyle, 2005; Wang et al., 2020).
Regarding fire, some high-altitude areas are currently experiencing an intensified regime of anthropic fires to reduce shrub encroachment and biomass build-ups caused by the relaxation of grazing activities (Köhler et al., 2005; Múgica et al., 2018). The effects of prescribed fires on soil properties have been documented in a range of contrasted habitats (Alcañiz et al., 2018), including high-altitude grasslands (in the Pyrenees, Armas-Herrera et al., 2016; San Emeterio et al., 2016). Although prescribed burnings do not reach the high temperatures of wildfires, their short and mid-term effects (thermal shocking, aboveground plant combustion, ash deposition, nutrient mineralization…) may drive profound changes in the plant community composition if the fire regime increases in recurrence (Uys et al., 2004). Regarding microbial soil communities, even low-intensity fires may depress soil microbial biomass (Múgica et al., 2018), with complex consequences on the C and N cycles (Soong and Cotrufo, 2015; Shaw et al., 2016; Pellegrini et al., 2020). The composition of soil fungal communities has been documented to be disrupted by fires as well (Artz et al., 2009; Egidi et al., 2016; Semenova-Nelsen et al., 2019). Few studies have addressed the effects of fire on plant fungal assemblages (Bellgard et al., 1994; Eom et al., 1999; Mataix-Solera et al., 2002), although changes in species richness have been detected in foliar endophytes of burned trees (Huang et al., 2016), and specific plant-fungal mutualisms and pyrophilous fungal species have been described (Baynes et al., 2012; Raudabaugh et al., 2020).
The western Pyrenees encompass large areas affected by the decoupling of traditional fire and grazing practices. As a result of decreasing herbivore pressure and increasing burnings, a native perennial grass is expanding. Brachypodium rupestre (Host) Roem. & Schult (Schippmann, 1991; Schippmann and Jarvis, 1988) (=B. pinnatum subsp. rupestre (Host) Schübl. & Martens according to some authors), (Aizpuru et al., 1999), dominates grasslands and causes a severe loss of sympatric species (Canals et al., 2014; Canals, 2019), a phenomenon also observed in other European mountain ranges (Buckland et al., 2001; Holub et al., 2012; Tardella et al., 2018). In this natural setting, many questions arise concerning the response of the plant holobiont to the changed disturbance regime. To what extent the composition of the B. rupestre mycobiome responds to the altered disturbance regime? Does the species harbor a specific fire-adapted mycobiome in frequently burnt areas? Or on the contrary, is there sufficient inertia to maintain a similar mycobiome in a common climatic environment (cold temperatures, high rainfall and humidity), independently of the current disturbance regime? Since taxon-specific endophytes (such as Epichloë sp.) may confer a higher adaptive advantage in stressful situations than broadly distributed non-systemic endophytes (Hill et al., 1989; Malinowski and Belesky, 2000; Pereira et al., 2019; Harrison and Griffin, 2020), is the mycobiome of the plant responding to these expectations?
The main objective of this research was to characterize the culturable fungal endophyte community of B. rupestre across a well-defined gradient of disturbance. The gradient encompassed sites with different burning recurrences and levels of herbivory, which have led to different patterns of grassland structure and diversity. We also studied the fungal endophyte community of the aerial tissues of the most frequent companion species of B. rupestre in grasslands, Festuca rubra and Agrostis capillaris. The purpose was to estimate whether the major diversity of coexisting plant species (and potential fungal propagules) in diverse grasslands had a positive influence in the fungal assemblage of B. rupestre.
Materials and Methods
Study Site and Sampling Design
The study area was located in the Aezkoa Valley in the western Pyrenees (43°3′ N1°13′ W; 800–1900 m.a.s.l.), and occupies an area of 198 Km2. Because of the influence of the Atlantic ocean (55 km away in a straight line) the weather is cold and snowy during winter, and mild and foggy during summer. The mean annual temperature is 9.3°C and mean annual precipitation is 1856 mm, according to data collected during 1989–2019 at the nearest climatic station, Irabia at 822 m.a.s.l.1. Soils are mainly derived from sandstones and calcareous clays, acidic with high organic matter and loamy or clay-loamy textures. The landscape is a mosaic of beech forests, gorse shrublands dominated by Ulex gallii Planch and Erica vagans L., and grasslands dominated by perennial grasses such as Festuca rubra gr., Agrostis capillaris L., Brachypodium rupestre (Host) Roem. & Schult, Danthonia decumbens (L.) and Avenula sulcata (J. Gay ex Boiss) Dumort. Other species are Galium saxatile L., Potentilla erecta (L.) Räeusch, Potentilla montana Brot., Hypochaeris radicata L., and the legume Trifolium repens L. The area is part of the European Protected Areas Network (Natura 2000) and was declared Special Area of Conservation (Roncesvalles-Selva de Irati, code ES0000126) in 2011 (Figure 1).
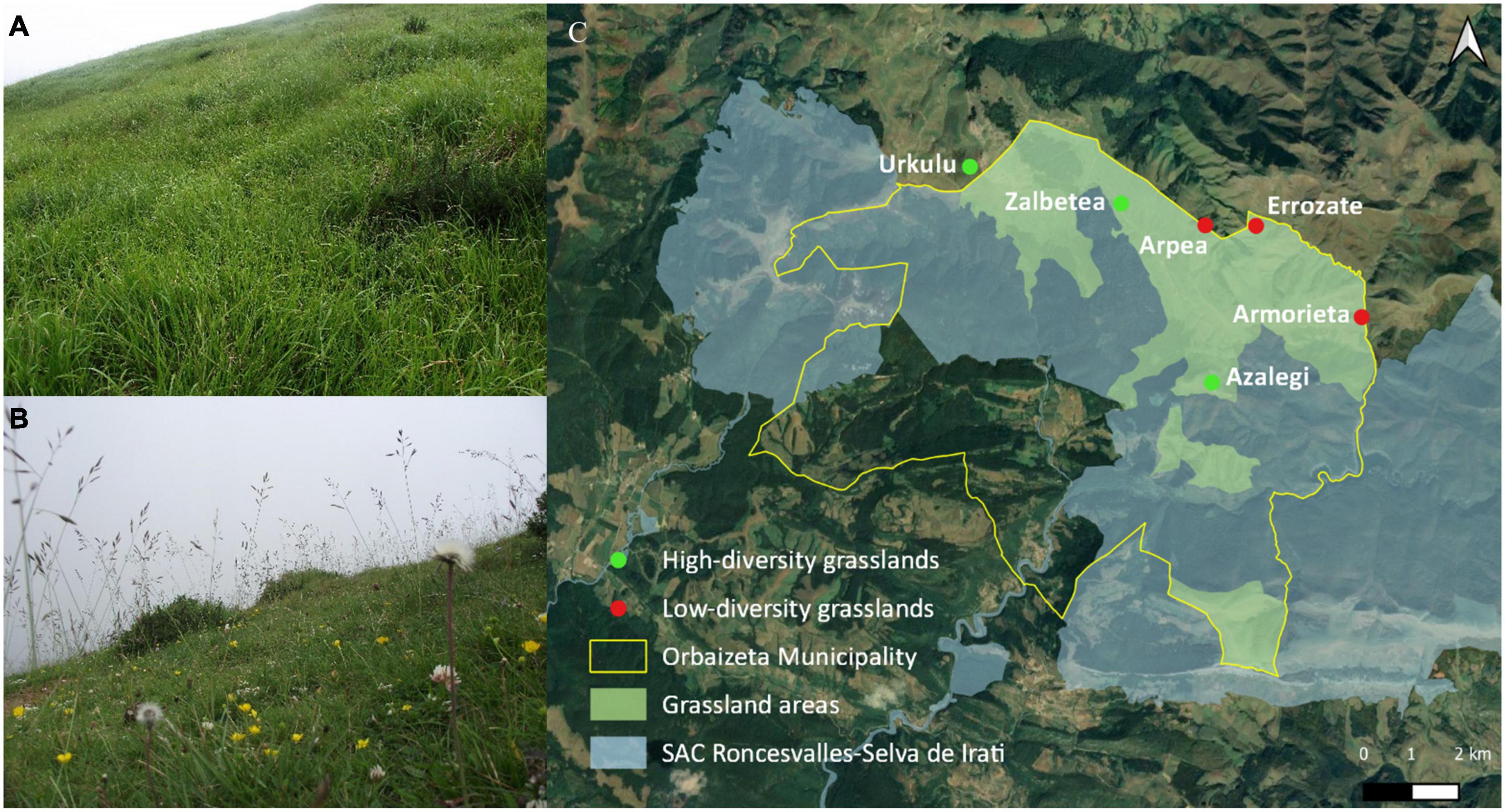
Figure 1. Locations selected for collecting plants. Red points represent low-diversity grasslands and green points high-diversity grasslands (C). Pictures of the Brachypodium rupestre invaded (A) and non-invaded (B) areas.
High-altitude grasslands are extensively grazed from May to October by sheep, cows and horses. Domestic herbivores have been present in the area since the Neolithic, as numerous prehistoric pastoral remains indicate. Where an appropriate extensive summer grazing exists, grasslands constitute high-diversity communities that are burned every 6–7 years by the bush-to-bush traditional practice to control localized shrub resprouting (San Emeterio et al., 2016). However, in the last decades, the number of grazers has sharply declined due to socio-economical changes experienced in the valleys, and the use of repeated winter burnings to control the build-up of ungrazed biomass has increased (Durán et al., 2020). Nowadays, many areas are burned recurrently, every 1–2 years (Canals et al., 2017). Due to the frequent burnings and the lack of grazing, the grasslands are far less diverse, favored by the expansion and dominance of B. rupestre, which grows in dense and tall clumps. Consequently, the current open landscape is a combination of grassland communities with different degrees of cover by B. rupestre, which accurately reflects the level of herbivory and the burning recurrence.
Based on the cartographic information provided by the Management Plan of the area (Ferrer and Canals, 2008), we selected six grassland communities where B. rupestre was present at different coverages: three areas had above 75% cover and three areas under 25% cover (Table 1 and Figure 1). The detailed floristic communities of the area are available in Durán et al., 2020. At each location, 40 turfs (400 cm3) of B. rupestre (including shoots, rhizomes, roots and rhizosphere) were collected in summer 2018. We established a distance of ca 30 m among collected plants to avoid clonal individuals (Baba et al., 2012). In the three areas of diverse grasslands, 40 turfs of Festuca rubra and 40 turfs of Agrostis capillaris were also collected. Sampling points were georeferenced and the sampling grid covered ca 2.5 ha per location (Supplementary Figure 1). In total, 480 turfs were collected, placed in seedbed trays and transported to the UPNA laboratory. The plants in the turfs were processed for the isolation of fungi from shoots, rhizomes and roots in the same week.
Isolation and Identification of Fungi
We isolated fungi from shoots, rhizomes and roots of B. rupestre and from shoots of F. rubra and A. capillaris. Leaf sheaths and stems were cut into fragments of ca 5 mm, and surface-disinfected by immersion in a solution of 20% commercial bleach (1% active chlorine) containing 0.02% Tween 80 (v:v) for 10 min, and finally rinsed with sterile water. The fragments of rhizomes and roots were surface-sterilized with the same bleach-Tween 80 solution, but then treated with an aqueous solution of 70% ethanol for 30 s, and a final rinse with sterile water. About 10–12 tissue fragments of the same individual were plated in a Petri dish with potato dextrose agar (PDA) containing 200 mg/L of chloramphenicol to avoid the growth of endophytic bacteria. Petri dishes, kept at room temperature and ambient light, were checked daily for mycelium growth during 5 weeks. When mycelium emerged from a tissue fragment, a small amount was transferred to a new Petri dish to obtain a culture.
Isolated fungi were grouped into morphotypes according to their morphological characteristics (colony color, exudates, growth type, and mycelium appearance). One or more isolates of each morphotype were genotyped for taxonomic purposes. To do this, a small amount of mycelium was scratched from the isolate culture and its DNA extracted using the Phire Plant Direct PCR Kit (Thermo Fisher Scientific). The ITS1, 5.8s and ITS2 regions were amplified using ITS4 and ITS5 primers (White et al., 1990). PCR amplification was done at 98°C for 5 min, followed by 35 cycles of 95°C for 5 s, 54°C for 5 s, 72°C for 20 s, and a final phase of 72°C for 1 min. Amplicons were purified (Favor PrepTM Plant Genomic DNA Extraction Mini Kit, Favorgen) and sequenced by the Sanger method at an external sequencing service (STABvida).
Because the range of intraspecific variation in ITS sequences is unknown for most fungal species (Taylor et al., 2000), DNA sequences were clustered using the CD_HIT program (Li and Godzik, 2006; Huang et al., 2010) and those with 97% or more similarity were considered to belong to the same taxon. A representative sequence of each cluster was selected and used to search the database of the ITS region from fungi type and reference material (Schoch et al., 2014) at the National Center for Biotechnology Information (NCBI) using the BLAST algorithm. In addition, the UNITE database of fungal nucleotide sequences was used as a complement for sequences without type specimens in NCBI. We also used the database FUNGuild (Nguyen et al., 2016) to get information on the ecological guild of each taxon, and to estimate for their possible functional roles.
Data Analyses
To evaluate the efficiency of our sampling effort for measuring species richness, species accumulation curves were estimated with all the species, and also excluding singletons, species that appeared only once (Vegan package, Oksanen et al., 2017). We calculated the incidence of all endophyte species within each type of tissue (shoots, rhizomes and roots), grassland type (low and high-diversity) and host plant species, and determined richness and Shannon and Simpson diversity indexes (Vegan package, Oksanen et al., 2017). Differences in species richness and diversity indexes of B. rupestre endophytes were analyzed using two-way ANOVA with tissue and grassland type as fixed factors, and permutation tests was used to compare diversity indexes of B. rupestre, F. rubra, and A. capillaris shoots (Coin package, Hothorn et al., 2008).
Venn diagrams were used to represent the taxa shared among grassland types, plant tissues and grass species (Euler package, Chen and Boutros, 2011). Frequencies were calculated from the matrix of presence/absence of isolates and permutational analyses of variance (PERMANOVA) were used to evaluate the variability of the fungal endophyte assemblages of B. rupestre between grassland types and among plant tissues. For that purpose, Adonis function was used (Vegan package, Oksanen et al., 2017). Distances were calculated using Bray–Curtis dissimilarities, set the number of permutations to 9999 and constrained the permutations within each location. Since PERMANOVA analyses are very sensitive to heterogeneity of multivariate dispersions, homogeneous dispersion between treatment groups was tested using the betadisper function.
To identify which species were characteristic of a particular tissue and grassland type, the indicator species tests in the Labsdv package was used (Roberts, 2019). The indicator value of a species (indval) measures the fidelity and relative abundance of the species in a particular situation (Dufrêne and Legendre, 1997). General Linear Mixed Models (GLMM) was used to determine whether the indicator species for a particular grassland type presented differences in the probability of incidence among grassland types. The grassland type was included as the fixed factor, the location as the random factor, using a binomial distribution. In addition, GLMM was used to analyze whether the probability of incidence of some mycobiome core species from the aboveground tissues presented differences among host plant species. Host plant species was included as the fixed factor, location as the random factor, using a binomial distribution. GLMM’s were done using the lme4 package (Bates et al., 2015).
Results
Isolation and Identification of Fungal Endophytes
We plated ca 10000 tissue fragments of B. rupestre (shoots, rhizomes and roots), F. rubra (shoots) and A. capillaris (shoots) in 960 culture media plates (10–12 fragments per plate). We obtained 1151 isolates (ca 190 isolates per location) which were classified into a total of 95 morphotypes. One or more isolates of each morphotype were sequenced, obtaining 116 sequences. After the sequence clustering process, 61 different sequence types remained, which were identified into 53 different taxa using the NCBI and UNITE databases. Twenty-two of them were classified to species rank, 20 to genus, 6 to family, 3 to order, and the remaining two to class rank (Supplementary Table 1 contains the complete list of taxa identified and their accession number).
Diversity of the B. rupestre Mycobiome
Fungi were isolated from 58% of the B. rupestre plants in low-diversity grasslands and from 43% in high-diversity grasslands (Table 2). Endophyte incidence varied among tissues: 37% of shoots, 52% of rhizomes and 64% of roots harbored fungi. Forty-five different taxa were identified in B. rupestre. Endophyte species richness in B. rupestre shoots, rhizomes and roots were 9, 25, and 26 taxa, respectively. Endophyte species richness in B. rupestre growing in low-diversity grasslands ranged from 9 to 22, and from 12 to 18 taxa in high-diversity grasslands (Table 2). Roots and rhizomes shared 23.9% of the species, whereas shoots with rhizomes and with roots shared 8.7 and 6.5%, respectively, all tissues had only 6.5% of all species in common (Figure 2). B. rupestre plants in low and high-diversity grasslands shared 39.1% of the species.
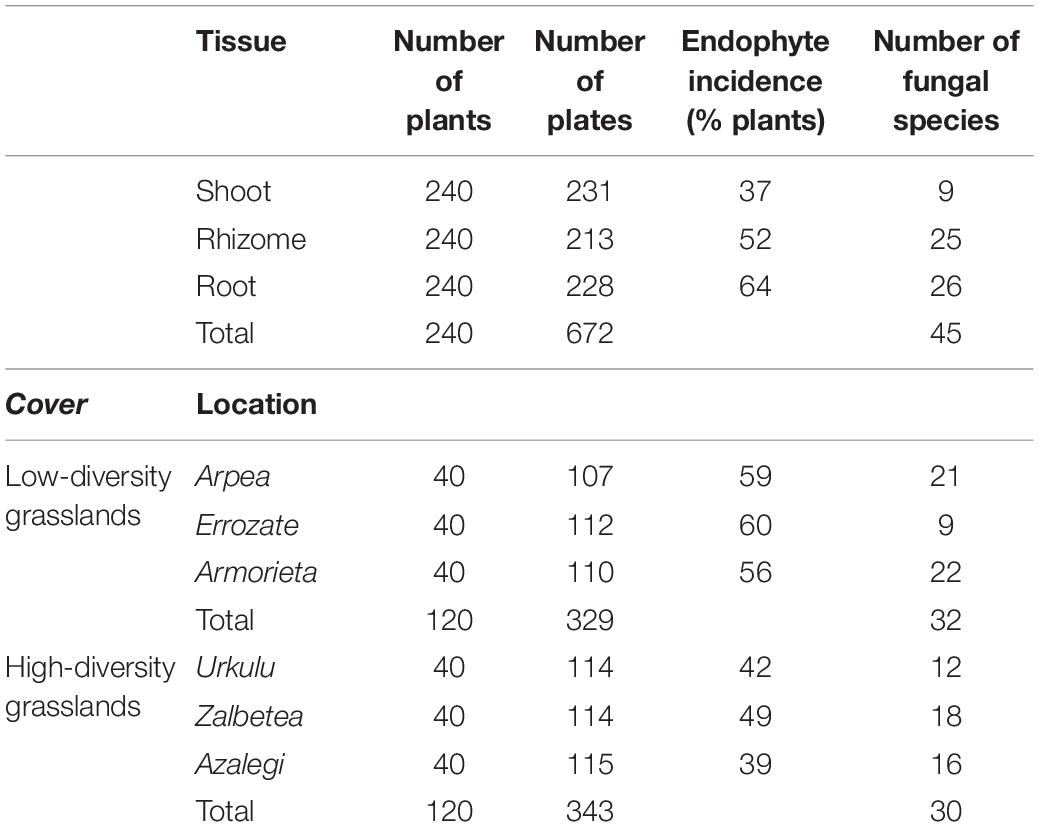
Table 2. Number of plants collected, final plates (taking into account the contaminations), fungal endophyte incidence (% plants), and number of fungal species in Brachypodium rupestre, all of that according to tissue and location.
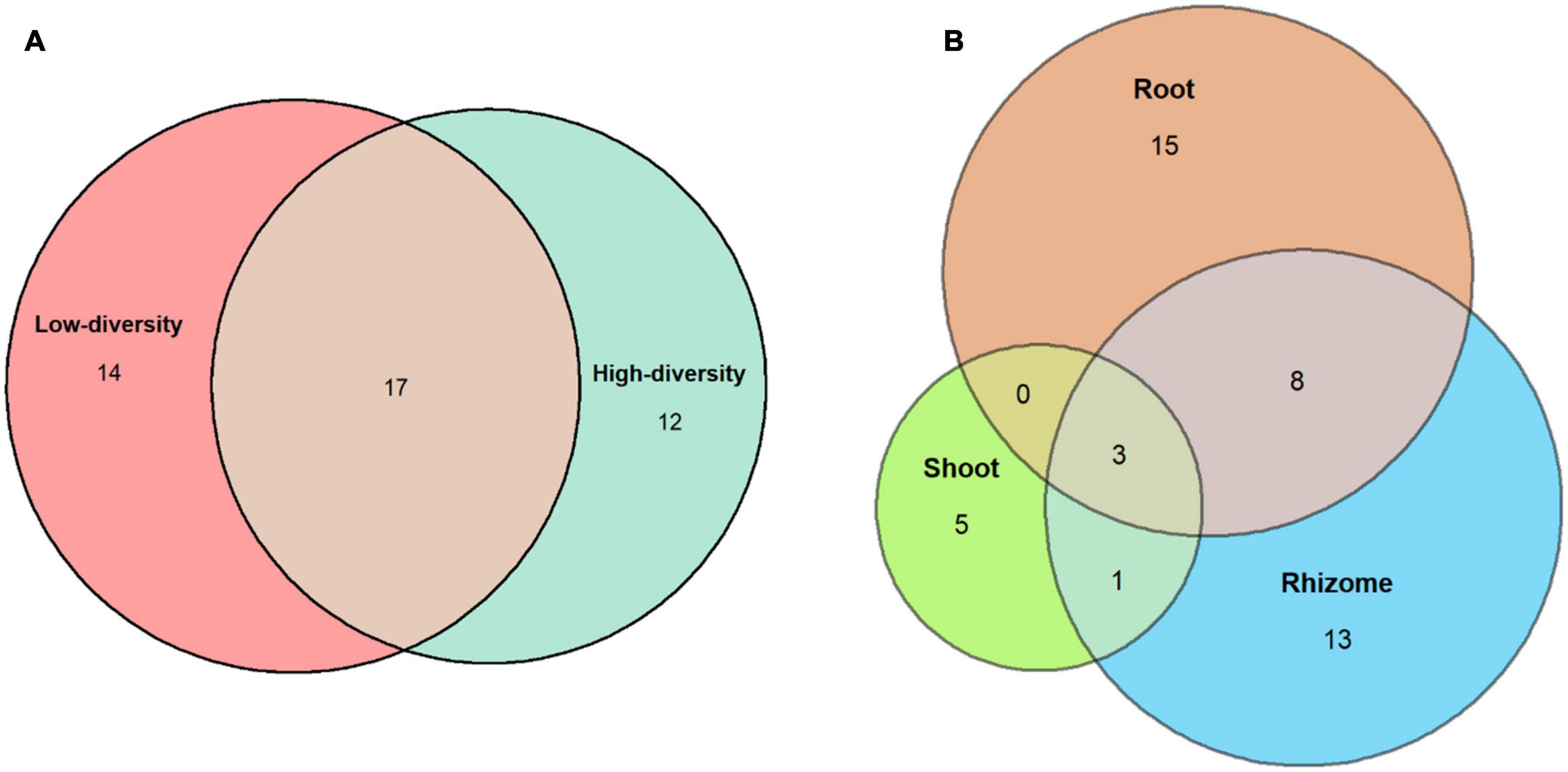
Figure 2. Venn’s diagrams indicating the number of shared fungal species across grassland types (A) and tissues (B).
The most frequent species were Albotricha sp., Lachnum sp. B, Omnidemptus graminis, and Mollisiaceae sp. (Table 3). Omnidemptus graminis was more frequent in shoots than in rhizomes and absent in roots, whereas Albotricha sp., Lachnum sp. B, and Mollisiaceae sp. were more frequent in belowground tissues than in shoots. In addition to the Mollisiaceae sp. taxon, other dark septate endophytes (DSE) such as Cadophora sp., Microdochium bolleyi, Microdochium neoqueenslandicum, and Periconia sp. were found, although with a low incidence. Members of the Clavicipitaceae family, such as Metapochonia bulbillosa, Metarhizium carneum, and Epichlöe typhina in shoots and rhizomes also occurred. The frequencies of the remaining species were under 2% (Supplementary Table 2). Three trophic types (pathotroph, saprotroph, and symbiotroph) were found in the mycobiome of B. rupestre. The most common guilds were plant pathogens, endophytes and undefined saprotrophs. However, a remarkable number of taxa were animal pathogens (Clonostachys rosea, Tolypocladium album, and Trichoderma koningii are described as entomopathogens, Metapochonia bulbillosa and Sarocladium strictum as nematophagous). Fungi with antimicrobial activity (Fusarium circinatum, Glarea sp., Nemania sp., and Penicillium ortum) and species with a high source of bioactive compounds (Acremonium sp., Gaeumannomycella sp., and Lachnum sp.) also occurred (Supplementary Table 1).
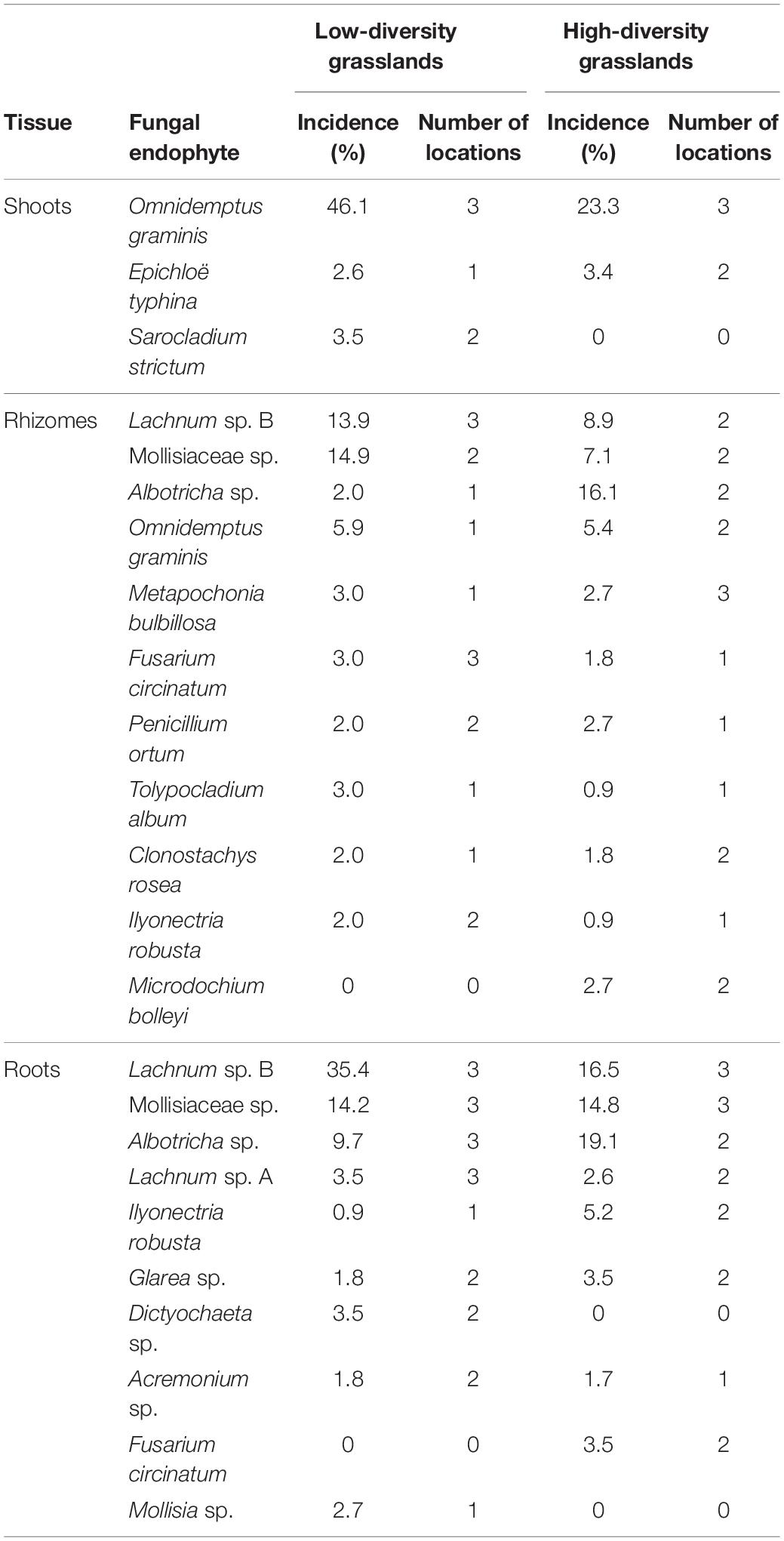
Table 3. Incidence in Brachypodium rupestre plants of the most abundant fungal endophyte taxa, differentiated by tissues and number of locations where they were present.
The species-accumulation curves including all the endophyte taxa were non-asymptotic (Figure 3), but when singletons were excluded the curves reached a plateau, suggesting that an increase in the sampling effort would reveal few common taxa, and mostly rare species. Therefore, a sampling effort of 100 plants is adequate to flatten the curves of the different tissues when excluding the singletons (Figures 3B–D). A greater sampling effort would be needed to include all the tissues (Figure 3A), although low-diversity grasslands need more plants than high-diversity grasslands to characterize their endophyte richness (Figures 3E,F).
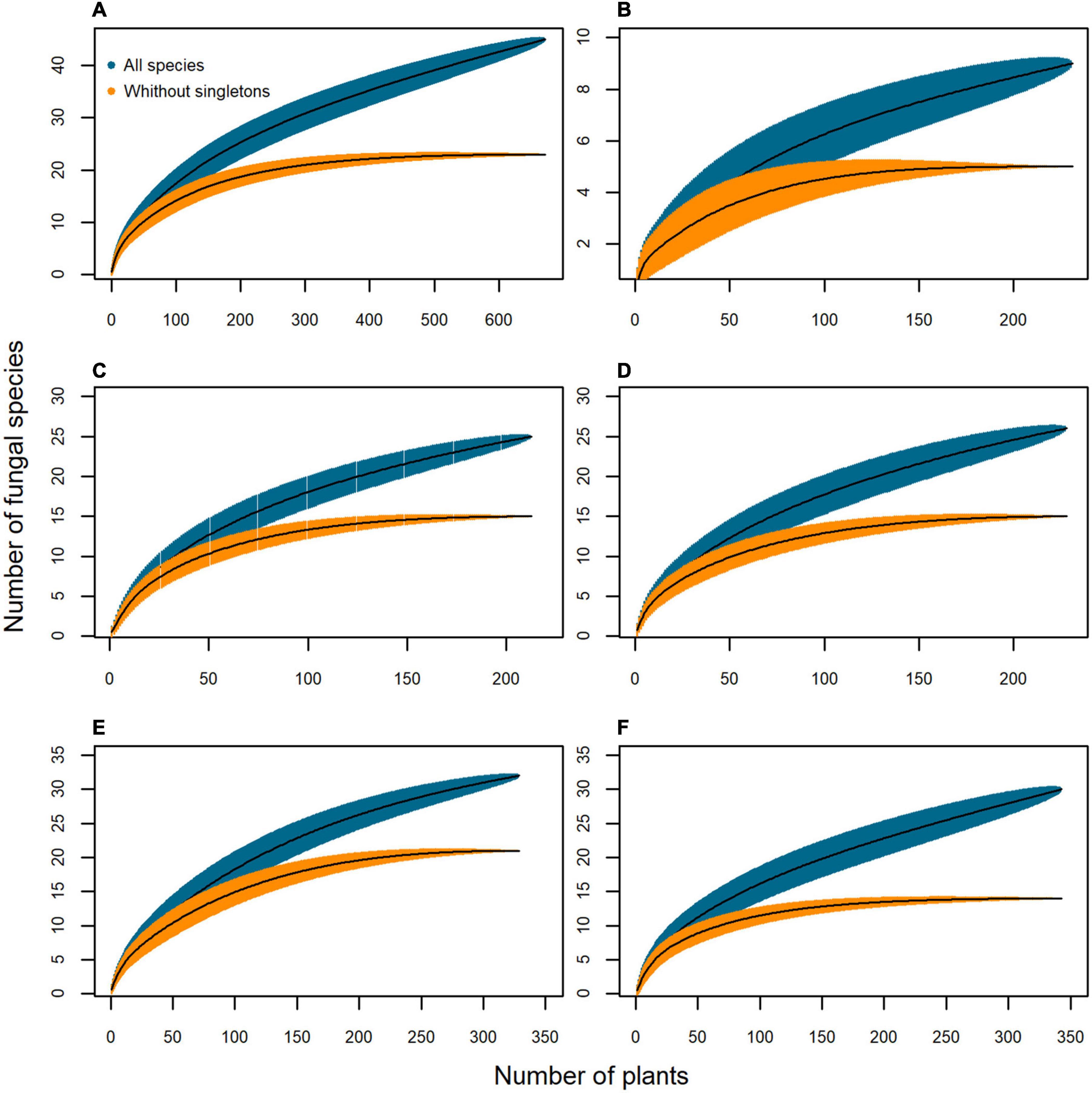
Figure 3. Species-accumulation curves of the mycobiota isolated from Brachypodium rupestre according to plant tissue and grassland type. Black line, based estimator of the total number of species; shaded zone, standard deviation; dark blue, all species included; orange, excluding singleton species. (A) All tissues, (B) Shoots, (C) Rhizomes, (D) Roots, (E) Low-diversity grasslands, (F) High-diversity grasslands.
Species richness and diversity indexes were significantly different among tissues (F = 10.319, p < 0.001; F = 18.336, p < 0.001; F = 24.114, p < 0.001; for richness, Shannon and Simpson, respectively). Shoots showed consistently lower diversity values than rhizomes or roots in both types of grasslands (Figure 4). No significant differences in diversity were found between low and high-diversity grasslands (F = 0.210, p > 0.05; F = 0.180, p > 0.05; F = 0.311, p > 0.05; for richness, Shannon and Simpson, respectively).
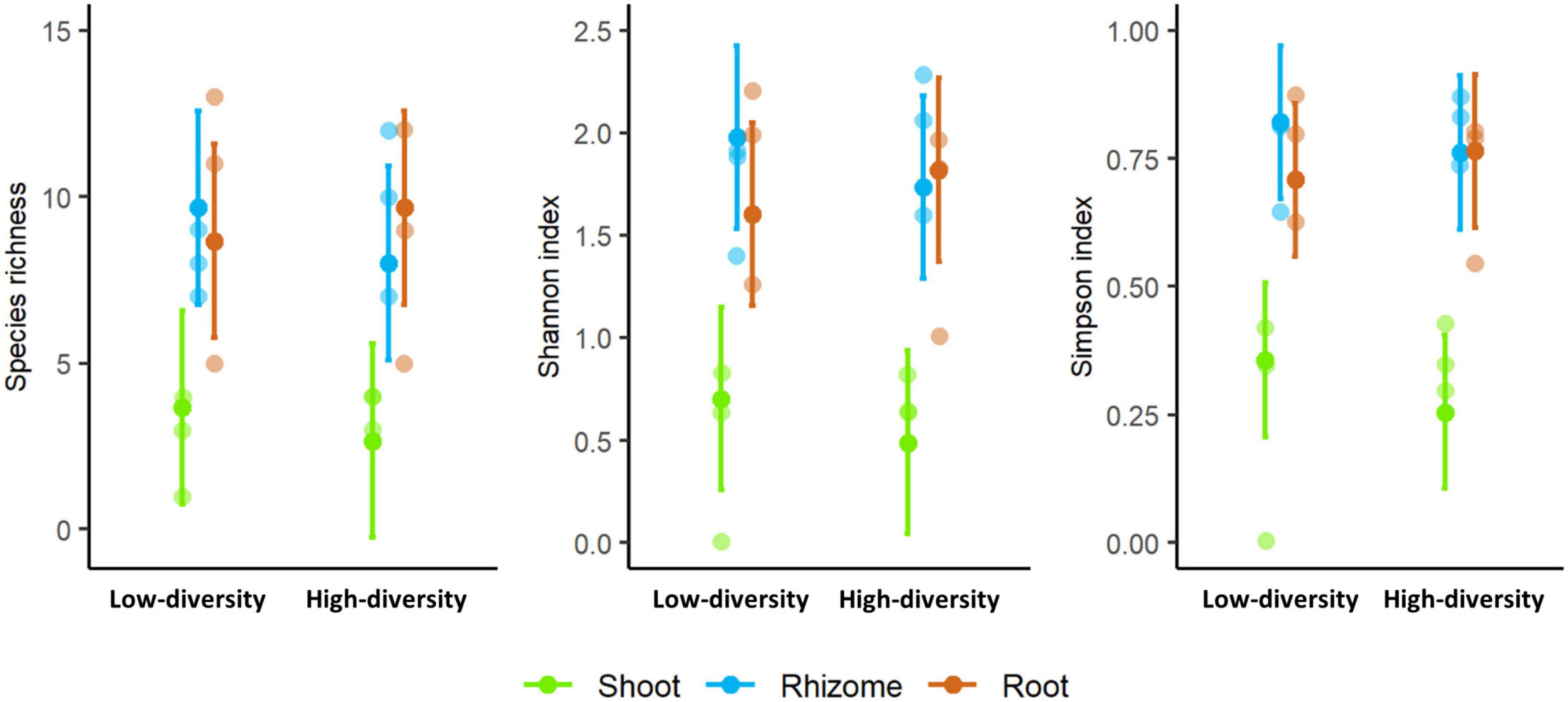
Figure 4. Species richness and diversity indexes (Shannon and Simpson) of the mycobiome of shoots, rhizomes, and roots of Brachypodium rupestre in low and high-diversity grasslands.
Distribution Patterns of the B. rupestre Mycobiome
A homogeneous dispersion was found within different tissues (Beta-disper; F2,15 = 2.195, p = 0.146) and grassland types (Beta-disper; F1,16 = 0.028, p = 0.870), contrasting with the significant effects on community assemblages. PERMANOVA analysis showed that tissue and grassland type had a significant effect on the endophytic community of B. rupestre plants (both p < 0.001). Tissue explained ca 50% of the variance of the model (r2 = 0.508), whereas grassland type explained ca 5% (r2 = 0.052). The NMDS (Non-Metric Multidimensional Scaling) plot discriminated the assemblages according to the tissues and included the indicator species (Figure 5). Omnidemptus graminis (p = 0.001) and Sarocladium strictum (p = 0.032) were indicators of shoots. Metapochonia bulbillosa (p = 0.003), Clonostachys rosea (p = 0.011), and Tolypocladium album (p = 0.009) were indicators of rhizomes. And Lachnum sp. B (p = 0.001), Albotricha sp. (p = 0.001), Mollisiaceae sp. (p = 0.001), Lachnum sp. A (p = 0.008), Acremonium sp. (p = 0.021), and Dictyochaeta sp. (p = 0.024) were indicators of roots. Regarding the grassland type, Albotricha sp. (p = 0.001) was an indicator of high-diversity grasslands and Lachnum sp. B (p = 0.004) and Omnidemptus graminis (p = 0.003) of low-diversity grasslands.
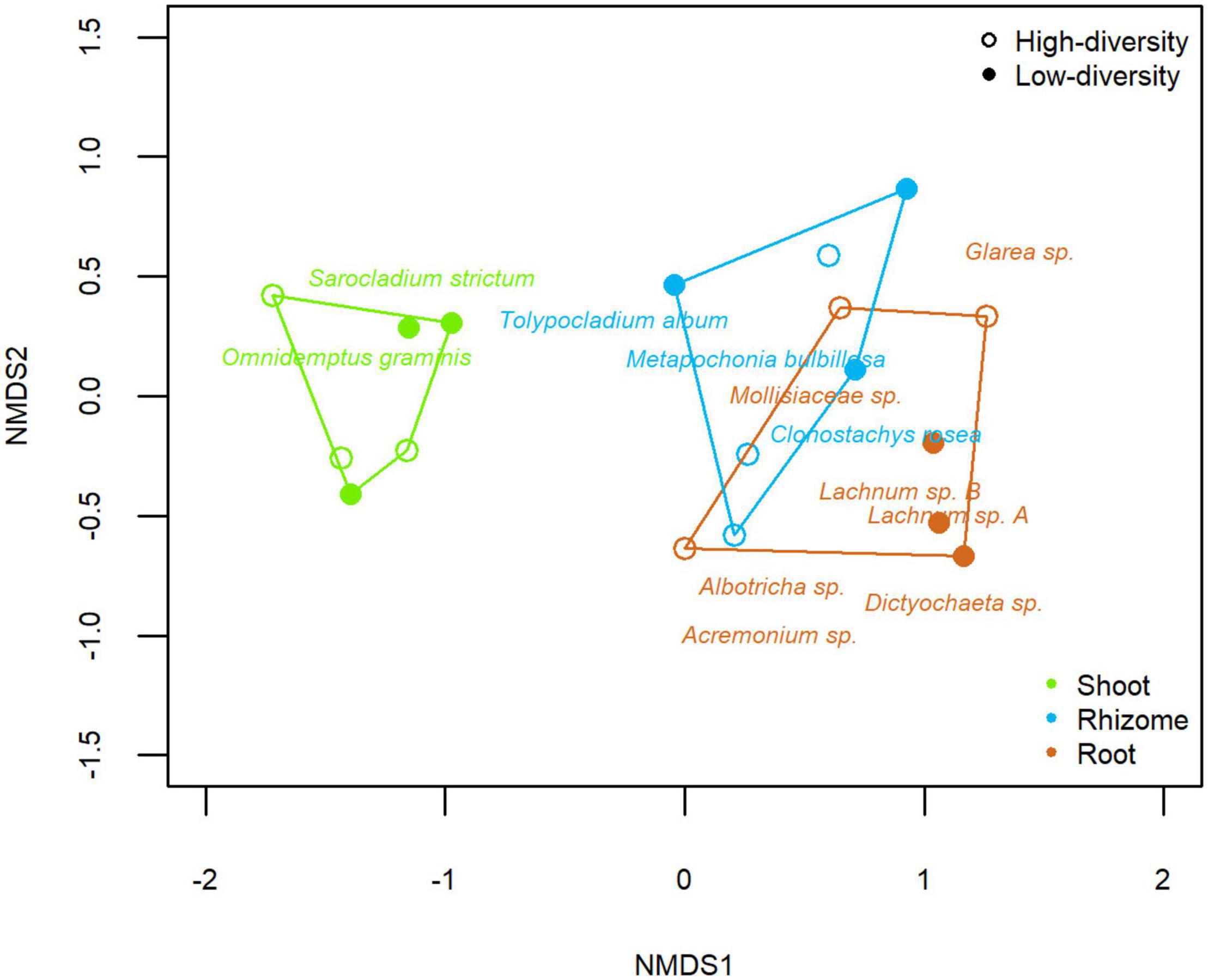
Figure 5. Non-metric multidimensional scaling (NMDS) of the fungal endophyte community composition of tissues of Brachypodium rupestre from low and high-diversity grasslands.
GLMM’s showed that B. rupestre plants from low-diversity grasslands had a greater probability of having their roots infected by Lachnum sp. B (LRT = 4.5719, p = 0.032) and their shoots by Omnidemptus graminis (LRT = 4.679, p = 0.030) than plants from high-diversity grasslands (Figure 6). Regarding Albotricha sp., the patterns were not so clear and its probability of incidence in B. rupestre roots was not significantly different between grassland types (LRT = 0.2849, p = 0.594, Figure 6).
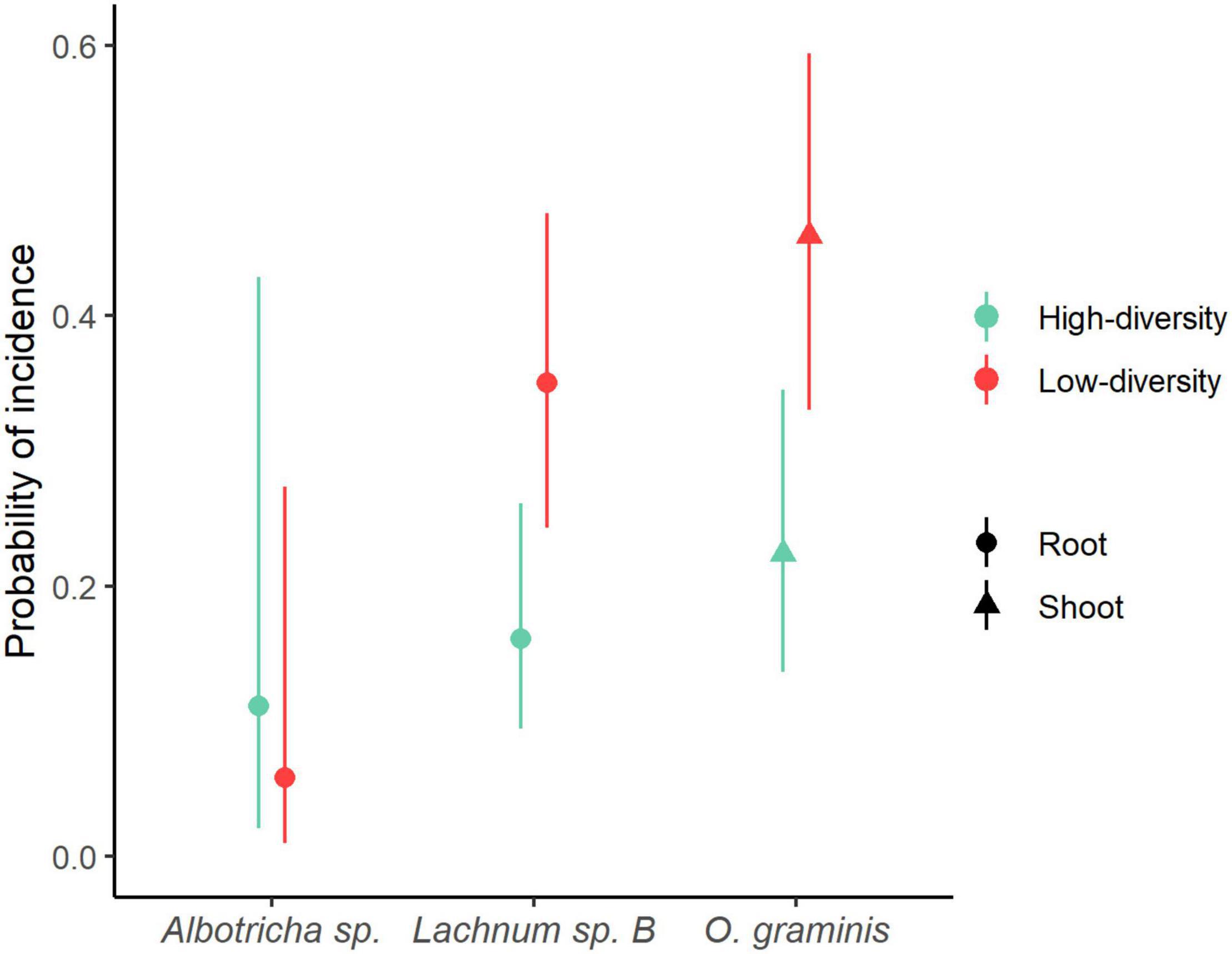
Figure 6. Probability of incidence of fungal indicator species of Brachypodium rupestre calculated with GLMM.
Aboveground Endophyte Assemblages of the Most Common Grasses in High-Diversity Grasslands
The incidence of endophytes varied among the shoots of the three grass species, ranging from 25% in B. rupestre and A. capillaris to 38% in F. rubra. For each plant species, a strong variability of endophyte incidence among locations was detected (Table 4). Eight taxa not observed in the B. rupestre survey were isolated from F. rubra or A. capillaris (Supplementary Table 1).
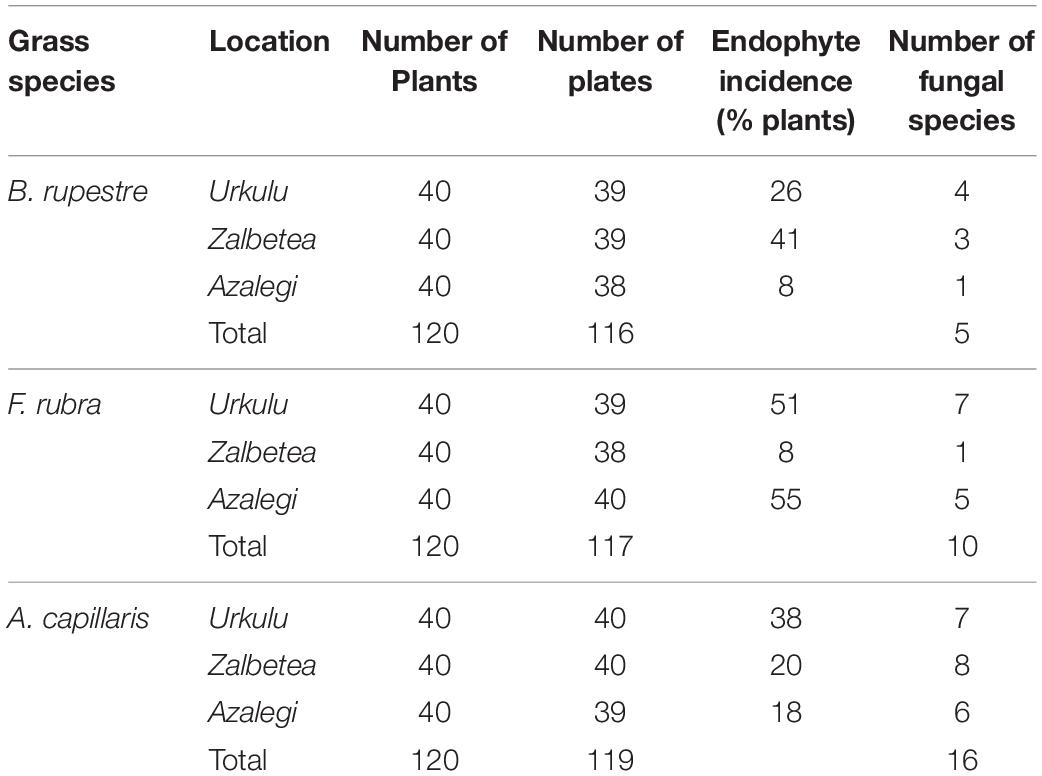
Table 4. Number of plants collected, number of final plates (taking into account the contaminations), fungal endophyte incidence (% plants), and number of fungal taxa per location for each grass species from high-diversity grasslands in aboveground tissues.
A total of 23 taxa were isolated from the shoots of B. rupestre, F. rubra and A. capillaris (Supplementary Table 3). Endophyte species richness in B. rupestre, F. rubra, and A. capillaris shoots were 5, 10, and 16, respectively (Table 4). F. rubra and A. capillaris shared more endophyte species between them than B. rupestre with either (Figure 7). The three grasses had two endophyte species in common, Lachnum sp. B and Omnidemptus graminis. The most frequent endophyte species in B. rupestre shoots were Omnidemptus graminis and Epichloë typhina, in F. rubra Epichlöe festucae and Mollisiaceae sp., and in A. capillaris Lachnum sp. B, Epichloë baconii and Mollisiaceae sp. (Table 5).
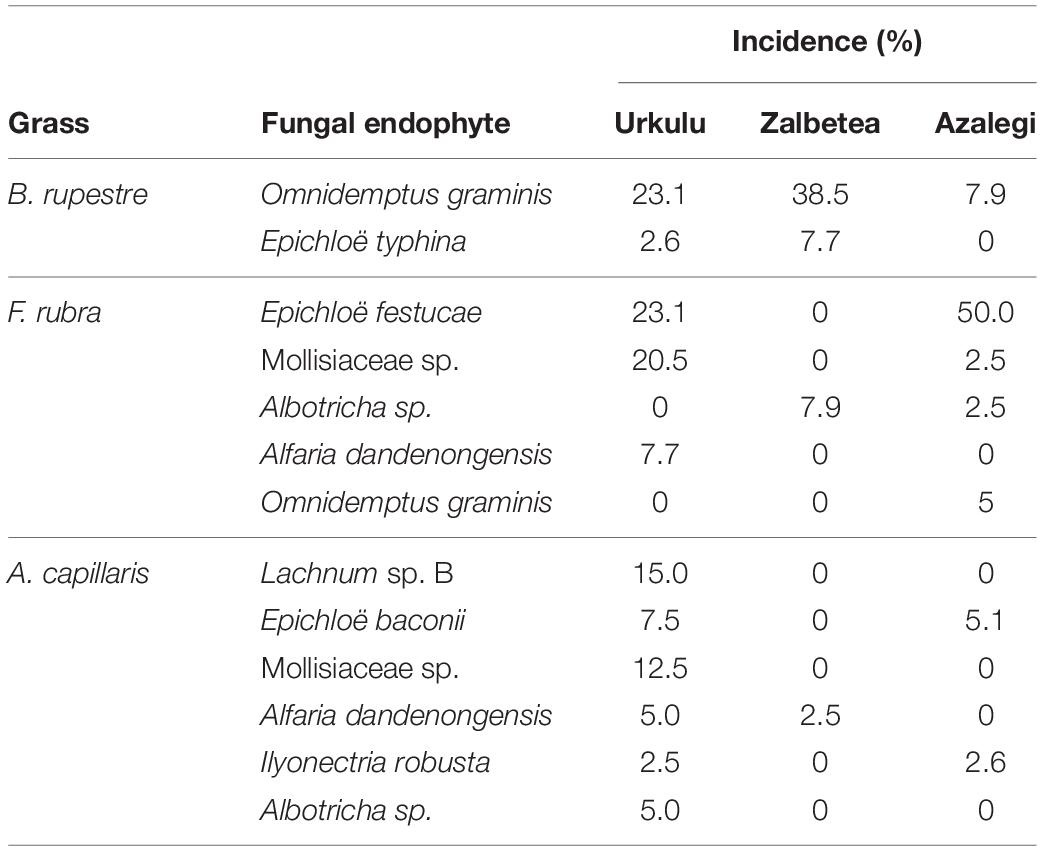
Table 5. Percentage of incidence of dominant fungal endophyte species in shoots of three representative grasses of high-diversity grasslands.
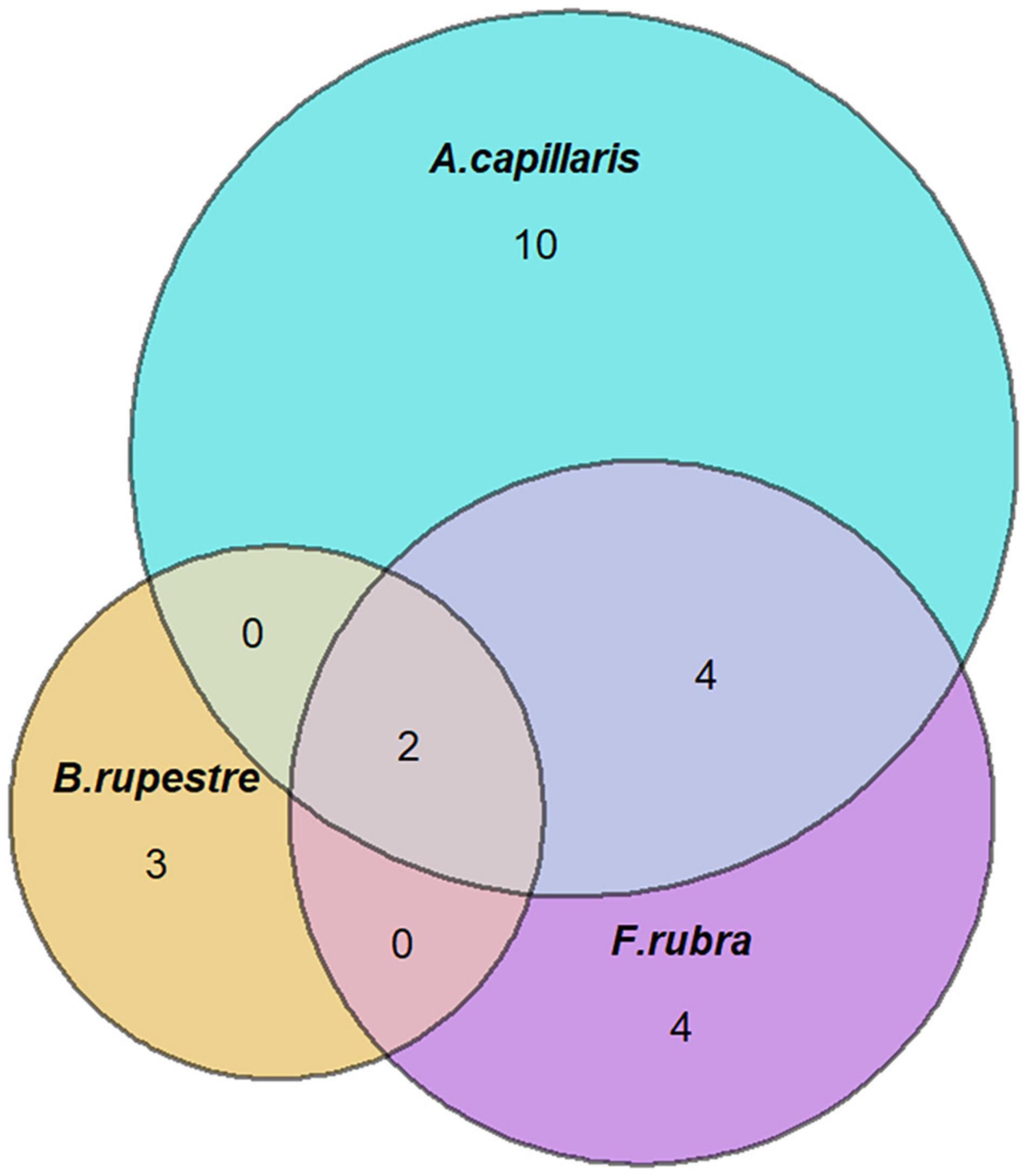
Figure 7. Venn’s diagrams indicating the number of shared fungal endophyte species among grass species.
When including all endophyte species, the species-accumulation curves of the three host grasses were non-asymptotic (Figure 8), but when removing singletons the curves flattened with a sampling effort of 50 plants for B. rupestre and 60 plants for F. rubra and A. capillaris in high-diversity grasslands (Figure 8).
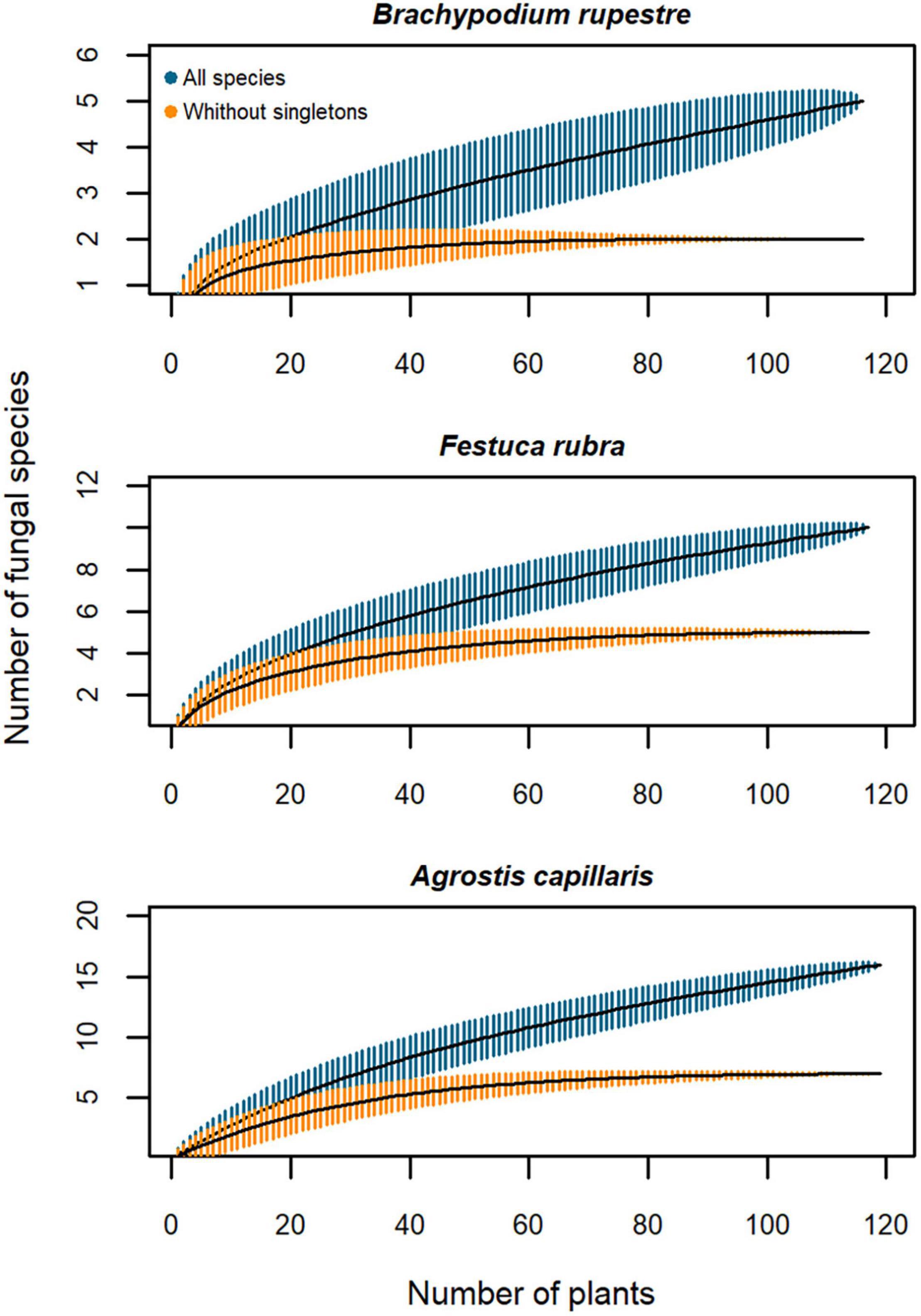
Figure 8. Species-accumulation curves of fungal endophytes from Brachypodium rupestre, Festuca rubra and Agrostis capillaris shoots from high-diversity grasslands. Black line, based estimator of the total number of species; shaded zone, standard deviation; dark blue, all species included; orange, excluding singleton species.
The mycobiome of A. capillaris appeared to be more rich and diverse (averaged per site richnessAC = 7.0, ShannonAC = 1.85, SimpsonAC = 0.83) than that of F. rubra and B. rupestre (averaged richnessFR = 4.3, ShannonFR = 0.76, SimpsonFR = 0.36; averaged richnessBR = 2.7, ShannonBR = 0.49, SimpsonBR = 0.26). However, the high variability among locations and the size of the sample (Figure 9) did not allow to detect significant differences in richness and diversity indexes among grasses in the permutations tests (richness, t = 1.7876, p = 0.1737; Shannon, t = 2.0697, p = 0.09614; Simpson, t = 2.0193, p = 0.1076).
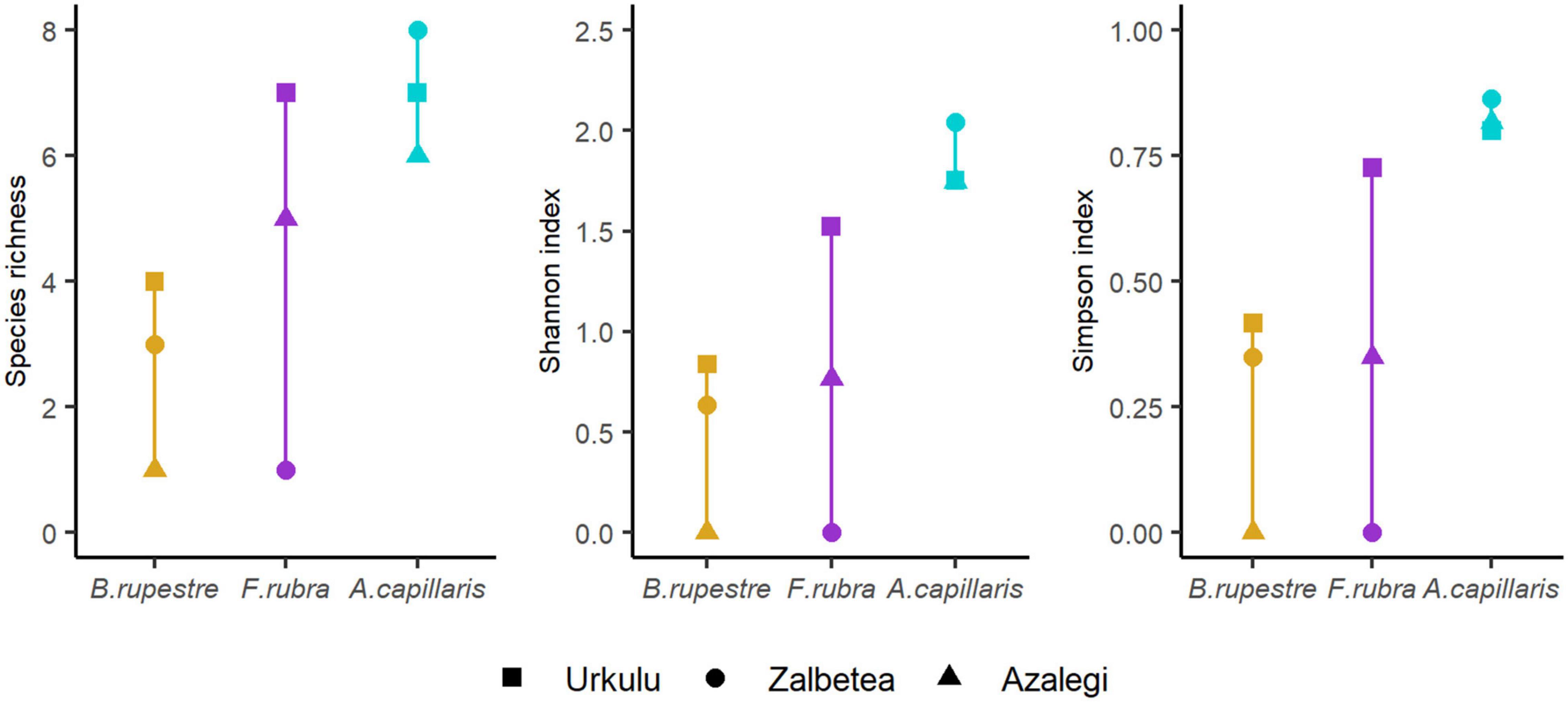
Figure 9. Species richness and diversity indexes (Shannon, Simpson) of the mycobiome of shoots from three representative grasses of high-diversity grasslands from three locations.
Omnidemptus graminis and Epichloë typhina were species indicators of B. rupestre (p = 0.001; p = 0.008), whereas Epichloë festucae was a species indicator of F. rubra (p = 0.001) and Epichloë baconii of A. capillaris (p = 0.009). The GLMM showed that the probability of incidence of Omnidemptus graminis significantly varied among species (LRT = 38.194, p < 0.001), and had a higher probability of incidence in B. rupestre, despite its presence in the other grasses (Figure 10). On the contrary, Epichloë species were specific of each grass (E. typhina in B. rupestre, E. festucae in F. rubra and E. baconii in A. capillaris), but the probability of Epichloë infection was higher in F. rubra than in the rest of grasses (LRT = 34.581, p < 0.001; Figure 10).
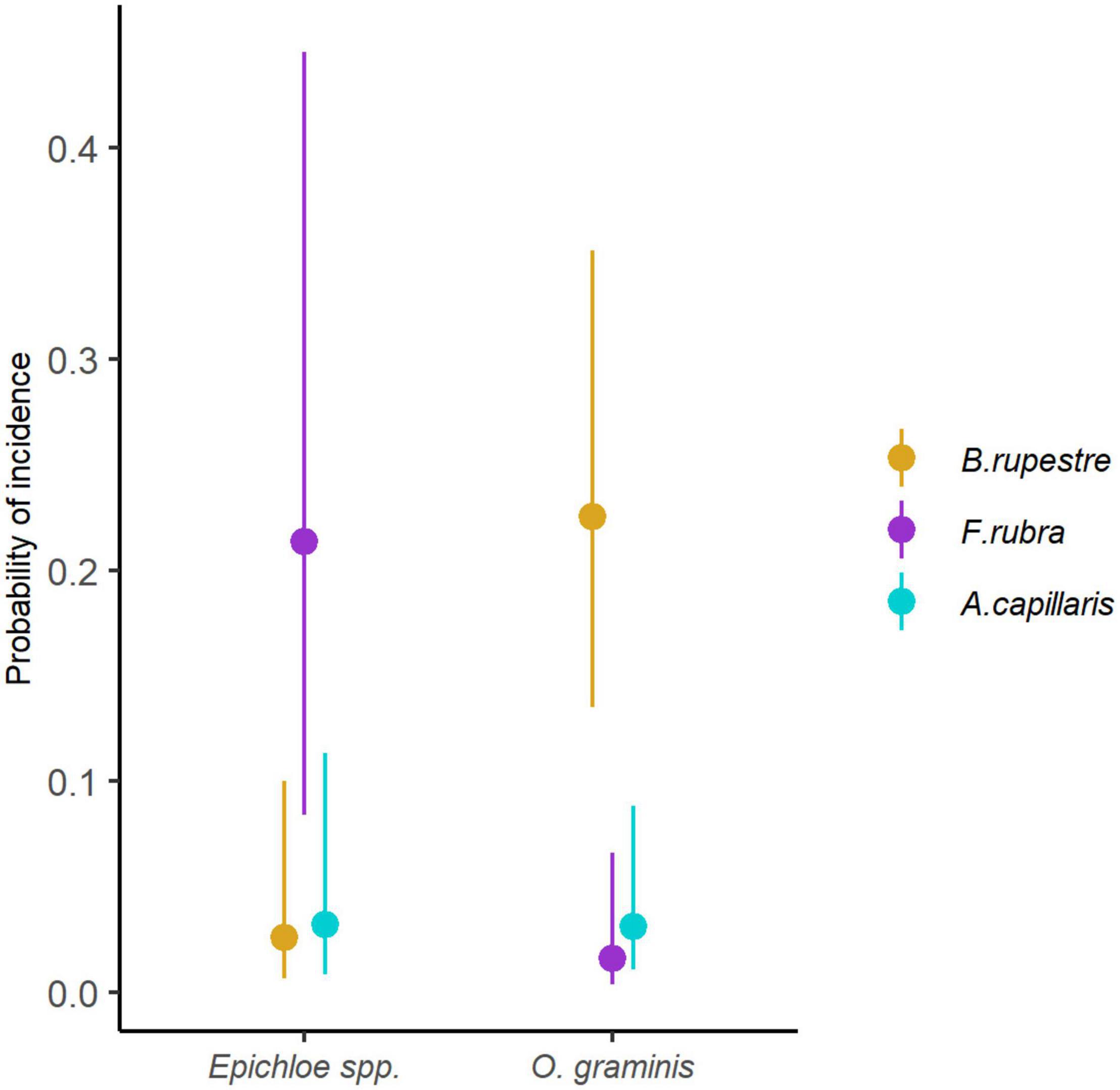
Figure 10. Probability of incidence of Epichloë spp. and Omnidemptus graminis in the aboveground tissues of the most common grasses of high-diversity grasslands.
Discussion
Shoot Fungal Endophyte Assemblages of the Most Common Grasses
The richness and diversity of the mycobiomes from this survey was relatively low compared to other studies in grasses (Sánchez-Márquez et al., 2008, 2010; Pereira et al., 2019). The study of fungal endophytes is highly method-dependent (Hyde and Soytong, 2008). In this research, we applied a conservative protocol, with along sterilization process -i.e., 10 min vs. 6 min in Pereira et al. (2019)-, and discarding the mycelia growing in the first day, which might influence the final counts of species (Burgdorf et al., 2014).
Despite the low richness reported, interesting patterns emerged from the shoot assemblages of the grasses. B. rupestre hosted the poorest mycobiome, half of that present in F. rubra, and one third of that of A. capillaris. Only two of 23 endophyte species identified were common to all grasses (Omnidemptus graminis and Lachnum sp. B). Recent studies indicate that plant identity influences foliar fungal assemblages more than expected from previous literature (Kivlin et al., 2019). Since the three grasses are sympatric and share an identical abiotic environment (i.e., climate, soil, location), other factors might define their mycobiomes. Plant abundance and capacity for carbon provision (B. rupestre develops more biomass but it is generally less abundant in diverse grasslands than the other grasses), potential interactions among fungal species (i.e., Omnidemptus graminis, which is abundant in B. rupestre, can induce host defense against other fungi (Schulz and Boyle, 2005; Constantin et al., 2020) and the different degree of herbivory (B. rupestre is less palatable to livestock than A. capillaris and F. rubra), may play a definite role in the structure of fungal assemblages.
Regarding systemic endophytes, the three grasses were infected by species of the genus Epichloë. E. typhina, E. baconii, and E. festucae are highly specific for B. rupestre, A. capillaris, and F. rubra, respectively (Leuchtmann et al., 2014; Saikkonen et al., 2016). Epichloë species are known to produce long-term, systemic infections and have a narrow range of hosts, limited to a genus or to related genera (Schardl et al., 2009; Schirrmann et al., 2015). In the area of study, the incidence of Epichloë was variable depending on species and locations, and symbioses with E. festucae were by far the most abundant (up to 50% incidence in one location) compared to E. typhina and E. baconii (less than 8% incidence in all locations). This is in accordance with previous research showing that the incidence of Epichloë-grass infections change depending on the associations (Leuchtmann and Schardl, 1998). E. festucae mainly reproduces asexually by vertical transmission to seeds, while in E. typhina and E. baconii seed transmission is absent (Leuchtmann et al., 2014). B. sylvaticum, a species close to B. rupestre, is very common in forest undergrowth, and displays high levels of infection by E. sylvatica, a seed-transmitted species (Meijer and Leuchtmann, 1999; Brem and Leuchtmann, 2001). The results suggest that the capacity of systemic endophytes to infect host seeds and transmit vertically, which is the most common reproductive mechanism in Epichloë species, leads to higher levels of incidence than in sexual, horizontally transmitted Epichloë species. This makes sense because in vertically transmitted endophytes reproductive fitness is intimately tied to that of their hosts (Saikkonen et al., 2002). As a consequence, mutualisms are expected to be more successful and intense in these situations. Of the three Epichloë species identified, E. festucae has been extensively studied for its capacity to establish successful symbioses in the most extreme environments (Vázquez-de-Aldana et al., 2013b; Zabalgogeazcoa et al., 2013; Leinonen et al., 2019; Pereira et al., 2019), and for the variety of advantages that confers to the host plant (i.e., tolerance to stress, resistance to herbivores, plant fitness) (Malinowski and Belesky, 2000; Zaurov et al., 2001).
Fungal Tissue Assemblages and Core Mycobiome of B. rupestre
Roots and rhizomes of B. rupestre displayed different endophyte assemblages than aboveground tissues, had the greatest percentages of fungal infection (Figure 5), and a significantly high richness and diversity of taxa. Previous studies have shown that belowground tissues have greater endophyte diversity than aerial tissues, and this could be related to the level of tissue exposition to fungal inoculum (Sánchez-Márquez et al., 2010; Harrison and Griffin, 2020). In B. rupestre, as in many perennial grasses, most aboveground tissues die and renew annually, while belowground tissues such as rhizomes, survive and consequently have a larger time frame for fungal reinfection.
As in other grass species, the mycobiome of B. rupestre was constituted by a few core species and many rare species (Comby et al., 2016; Ofek-Lalzar et al., 2016; Pereira et al., 2019; Sun et al., 2020). Only two species were found in more than 20% of the B. rupestre plants and in most locations, Omnidemptus graminis in shoots and rhizomes and Lachnum sp. B in roots and rhizomes. O. graminis is a member of the Magnaporthaceae that has been described recently (Hernández-Restrepo et al., 2019). Although some Magnaporthaceae strains have shown plant-growth promoting activity (Yuan et al., 2010; Changyeol et al., 2017), the best known members of this family are pathogens of grasses, associated to roots (Gaeumannomyces graminis and Magnaporthe poae) and shoots (Magnaporthe oryzae) (Illana et al., 2013). M. oryzae is a hemibiotrophic fungus that causes the rice blast disease. This species switches from a biotrophic growth phase -it feeds from the host plant without killing its cells- in early infection to a necrotrophic stage (Kankanala et al., 2007). According to Talbot et al. (1997), the switch between phases may be triggered by the lack of nutrients within the host cell. In this survey, O. graminis was present in asymptomatic plants, suggesting at least the occurrence of a biotrophic phase of unknown duration. As many other fungal endophytes, this species may have a latent saprobic lifestyle (Vázquez-de-Aldana et al., 2013a).
Lachnum is a large genus within the Hyaloscyphaceae family with more than 250 species described and distributed in a wide range of habitats and host species (Wu and Su, 2007; Nagao, 2008). Most Lachnum species are latent saprophytes, that may grow as endophytes in roots of perennial grasses (Sánchez-Márquez et al., 2010; Pereira et al., 2019) and form ericoid mycorrhiza coils in some Ericaceae (Walker et al., 2011). Many Lachnum sp. are bioactive, producing a wide range of biologically active compounds (Shan et al., 1997; Ondeyka et al., 2009; McMullin et al., 2017; Zong et al., 2017).
In addition to the core species Omnidemptus graminis and Lachnum sp., the culturable mycobiota of B. rupestre was characterized by a remarkable number of taxa with recognized defensive activities, such as entomopathogenic fungi (Tolypocladium album and Sarocladium strictum), (Gera Hol et al., 2007; Quesada-Moraga et al., 2014; El-Sayed et al., 2020), nematophagous fungi (Metapochonia bulbillosa and Clonostachys rosea) (Sankaranarayanan et al., 1997; Ownley et al., 2010; Manzanilla-López and Lopez-Llorca, 2017), and fungi with antifungal activity (Fusarium circinatum, Penicillium ortum, Lachnum sp., Glarea sp., Trichoderma sp., Trichoderma koningii and Nemania sp.) (Ondeyka et al., 2009; Youssar et al., 2011; Zhang et al., 2014; Mousa et al., 2015; Kornsakulkarn et al., 2017). The known defensive functions of this group of microorganisms may confer a decisive advantage to B. rupestre, which may use them for its own protection, as predators of its pathogens and pests, according to the bodyguard hypothesis (Elliot et al., 2000). To what extent this particular defensive assemblage plays a key role in the success and expansion of B. rupestre with regard to other grasses is a matter of interest that needs further research.
Fungal Assemblages in B. rupestre Associated to Specific Grasslands
Low and high-diversity grasslands, generated by a distinctive disturbance regime, had similar fungal richness and diversity (Figure 4), but different endophyte assemblages (Figure 2 and PERMANOVA analysis). Differences were mostly due to the different probability of incidence of the core taxa (Figure 6). B. rupestre in low-diversity grasslands had a greater probability of incidence of Omnidemptus graminis and Lachnum sp. B than in high-diversity grasslands, and the two grassland types only shared 36% of the total fungal taxa identified. Considering that we sampled 120 turfs per grassland type, and that we did not reach the asymptote in the species accumulation curves (Figure 3), a more exhaustive sampling effort would be necessary to draw conclusions on a specific cohort of rare taxa per grassland type. With regard to the systemic endophyte E. typhina, no significant differences in the probability of incidence were found between grassland types. In consequence, the highest degree of incidence of the two core taxa, O. graminis (in shoots and rhizomes) and Lachnum sp. B (in roots and rhizomes), in low-diversity, recurrently burned grasslands was the most sound result of this part of the study.
From the spectrum of core species infecting B. rupestre, O. graminis was only found in shoots and rhizomes, which indicates its affinity for both tissues, which are anatomically similar (de Kroon and Knops, 1990), and different from roots (Table 3). When isolated and cultured in plates, we observed rapid mycelial growth of O. graminis compared to the rest of species. We hypothesize that fires destroy the aerial mycelium of O. graminis, together with the aboveground biomass of the plant, but the fungus remains in the rhizome reservoir. Since fire is applied in winter time in moist soils, the temperatures reached in the top soil are low (at 1 cm deep, soil temperature rise 9–10°C and no change is measured at 5 cm depth – data unpublished), and the function of rhizomes remains unaffected. Consequently, recolonization of the aboveground tissues by the rhizome mycelium of O. graminis can occur rapidly, paralleling the regrowth of the plant, and conferring a decisive initial advantage to this fungal species.
Regarding Lachnum sp. B, the increased incidence of this fungal species in frequently burnt areas paralleled a consistent decrease of Albotricha sp. in roots and rhizomes (Table 3). These two endophytic taxa constitute a large part of the belowground mycobiome of B. rupestre and belong to the same family, Hyaloscyphaceae. The close phylogeny of both genera and the polyphyly of the Lachnum group has been demonstrated in genetic studies (Ye and Zhuang, 2003; Hosoya et al., 2010). The pattern of increased Lachnum sp. and decreased Albotricha sp. infection in the most burned sites, may indicate a negative interaction between both species, or a contrasting response among them to the fire disturbance. The capacity of Lachnum sp. to cope with environmental stress, by producing sclerotia and adopting latent forms, and its growth in belowground structures, which are less affected by fire than aboveground structures, may help to explain its success. In particular, in temperate shrublands close to the region of study, ascocarps of Lachnum pygmaeum have been observed on charred wood and roots of Ulex europaeus (unpublished results). It is known that the thermal shock produced by fire may enhance fungal fecundity and activate the development of sexual structures in some pyrophilous fungi (Raudabaugh et al., 2020). Also, fungal biomass may be increased by fires, as demonstrated for the pyrophilous fungi Morchella sp. infecting the grass Bromus tectorum (Baynes et al., 2012). To what extent the particular Lachnum sp. B identified in the area of study is a pyrophilous fungus merits additional study.
Although the incidences of O. graminis and Lachnum sp. B are favored in such anthropized fire-prone habitats, the extent to which these endophytes confer particular advantages to its host, B. rupestre, is unknown. Further experimental research is needed to evaluate whether O. graminis and/or Lachnum sp. B infected plants perform better after fires than non-infected plants and whether the relationship in such a disrupted environment relies in a mutualistic relationship. In this research, the systemic E. typhina did not display significant differences in incidence among grasslands, and its percentage of infection was low at all sites. Previous research on Epichloë endophytes of grasses did not find evidence of a mutualistic relationship associated to fire (Faeth et al., 2002; Hall et al., 2014), contrary to grazing intensities that have been positively related to the abundance of vertically transmitted Epichloë producers of toxic metabolites (Vázquez-de-Aldana et al., 2010; Hume et al., 2020).
Conclusion
The perennial tall-grass B. rupestre had a moderately diverse endophytic mycobiome consisting of a few core species and many rare species in assemblages that differed between aerial and belowground tissues. Recurrent grassland burnings, which eliminate the aerial biomass of the grass every 1–2 years, did not affect the richness and the diversity of the fungal community in B. rupestre, but the percentages of infection of two core taxa, Omnidemptus graminis and Lachnum sp. B, were significantly modified. The results indicate that although in frequently burnt areas the same core species of diverse grasslands subsist, Omnidemptus graminis and Lachnum sp. B are singularly benefited due to the proposed following mechanisms: (1) the capacity to survive belowground in rhizomes during the winter, and to spread rapidly to the shoots when the plant starts its spring growth (O. graminis), and (2) the location in belowground tissues (Lachnum sp. B) and the higher resistance to stress than other core root fungi, such as the related-taxon Albotricha sp. Following steps should address whether these two core taxa benefit the expansive success of B. rupestre in these antrophized, fire-prone environments, as well as to determine whether the cohort of less abundant fungi with well-defined defensive functions play a role too.
Data Availability Statement
The datasets presented in this study can be found in online repositories. The names of the repository/repositories and accession number(s) can be found in the article/Supplementary Material.
Author Contributions
IZ and BV introduced the training in culturable techniques for fungal identification to MD. MD and LS designed the experiments and analyzed the data. MD, LS, and LM collected the plants and isolated the fungi. MD and IZ identified the fungi. MD and RC wrote the manuscript. LS, IZ, and BV reviewed the manuscript. MD and RC got the projects that supported financially the research. All authors contributed to the article and approved the submitted version.
Funding
MD and LM got funding through two UPNA’s doctorate scholarships. The research was supported by three projects financed by Ecoembes-SEO/Birdlife (Libera 2017), “la Caixa” Foundation and CAN foundation (LCF/PR/PR13/51080004), and the Spanish Ministry of Science and Innovation and FEDER funds (AGL2016-76035-C2-1R).
Conflict of Interest
The authors declare that the research was conducted in the absence of any commercial or financial relationships that could be construed as a potential conflict of interest.
Acknowledgments
We are very grateful to B. Soret, C. Paredero, and I. Maquirriain for offering technical support in the laboratory tasks. We thank the Aezkoa Valley Council for its interest and willingness to process the permissions necessary for sampling in the study sites.
Supplementary Material
The Supplementary Material for this article can be found online at: https://www.frontiersin.org/articles/10.3389/fmicb.2021.679729/full#supplementary-material
Footnotes
References
Aizpuru, I., Aseginolaza, C., Uribe-Echebarría, P. M., Urrutia, P., and Zorrakin, I. (1999). Claves Ilustradas de la Flora del País Vasco y Territorios Limítrofes. Vitoria-Gasteiz: Servicio Central de Publicaciones del Gobierno Vasco.
Alcañiz, M., Outeiro, L., Francos, M., and Úbeda, X. (2018). Effects of prescribed fires on soil properties: a review. Sci. Total Environ. 61, 944–957. doi: 10.1016/j.scitotenv.2017.09.144
Anderson, P. M. L., and Hoffman, M. T. (2007). The impacts of sustained heavy grazing on plant diversity and composition in lowland and upland habitats across the Kamiesberg mountain range in the Succulent Karoo, South Africa. J. Arid Environ. 70, 686–700. doi: 10.1016/j.jaridenv.2006.05.017
Archibald, J. K., Mort, M. E., Crawford, D. J., and Kelly, J. K. (2005). Life history affects the evolution of reproductive isolation among species of Coreopsis (Asteraceae). Evolution 59, 2362–2369. doi: 10.1111/j.0014-3820.2005.tb00946.x
Armas-Herrera, C. M., Martí, C., Badía, D., Ortiz-Perpiñá, O., Girona-García, A., and Porta, J. (2016). Immediate effects of prescribed burning in the central pyrenees on the amount and stability of topsoil organic matter. CATENA 147, 238–244. doi: 10.1016/j.catena.2016.07.016
Artz, R. R. E., Reid, E., Anderson, I. C., Campbell, C. D., and Cairney, J. W. G. (2009). Long term repeated prescribed burning increases evenness in the basidiomycete laccase gene pool in forest soils. Fems Microbiol. Ecol. 67, 397–410. doi: 10.1111/j.1574-6941.2009.00650.x
Augustine, D. J., McNaughton, S. J., and Frank, D. A. (2003). Feedbacks between soil nutrients and large herbivores in a managed savanna ecosystem. Ecol. Appl. 13, 1325–1337. doi: 10.1890/02-5283
Baba, W., Kurowska, M., Kompala-Baba, A., Wilczek, A., Dlugosz, J., and Szarejko, I. (2012). Genetic diversity of populations of Brachypodium pinnatum (L.) P. Beauv.: expansive grass in a fragmented landscape. Polish J. Ecol. 60, 31–40.
Bardgett, R. D., and Wardle, D. A. (2003). Herbivore-mediated linkages between aboveground and belowground communities. Ecology 84, 2258–2268. doi: 10.1890/02-0274
Bates, D., Mächler, M., Bolker, B. M., and Walker, S. C. (2015). Fitting linear mixed-effects models using lme4. J. Stat. Softw. 67, 1–48. doi: 10.18637/jss.v067.i01
Baynes, M., Newcombe, G., Dixon, L., Castlebury, L., and O’Donnell, K. (2012). A novel plant-fungal mutualism associated with fire. Fungal Biol. 116, 133–144. doi: 10.1016/j.funbio.2011.10.008
Bellgard, S. E., Whelan, R. J., and Muston, R. M. (1994). The impact of wildfire on vesicular-arbuscular mycorrhizal fungi and their potential to influence the re-establishment of post-fire plant communities. Mycorrhiza 4, 139–146. doi: 10.1007/BF00203532
Bråthen, K. A., Jahiri, X., Jusdado, J. G. H., Soininen, E. M., and Jensen, J. B. (2015). Fungal endophyte diversity in tundra grasses increases by grazing. Fungal Ecol. 17, 41–51. doi: 10.1016/j.funeco.2015.05.002
Brem, D., and Leuchtmann, A. (2001). Epichloë grass endophytes increase herbivore resistance in the woodland grass Brachypodium sylvaticum. Oecologia 126, 522–530. doi: 10.1007/s004420000551
Buckland, S. M., Thompson, K., Hodgson, J. G., and Grime, J. P. (2001). Grassland invasions: effects of manipulations of climate and management. J. Appl. Ecol. 38, 301–309. doi: 10.1046/j.1365-2664.2001.00603.x
Burgdorf, R. J., Laing, M. D., Morris, C. D., and Jamal-Ally, S. F. (2014). A procedure to evaluate the efficiency of surface sterilization methods in culture-independent fungal endophyte studies. Braz. J. Microbiol. 45, 977–983. doi: 10.1590/S1517-83822014000300030
Canals, R. M. (2019). Landscape in motion: revisiting the role of key disturbances in the preservation of mountain ecosystems. Geogr. Res. Lett. 45, 515–531. doi: 10.18172/cig.3634
Canals, R. M., Pedro, J., Rupérez, E., and San-Emeterio, L. (2014). Nutrient pulses after prescribed winter fires and preferential patterns of N uptake may contribute to the expansion of Brachypodium pinnatum (L.) P. Beauv. in highland grasslands. Appl. Veg. Sci. 17, 419–428. doi: 10.1111/avsc.12088
Canals, R. M., San Emeterio, L., Durán, M., and Múgica, L. (2017). Plant-herbivory feedbacks and selective allocation of a toxic metal are behind the stability of degraded covers dominated by Brachypodium pinnatum in acidic soils. Plant Soil 415, 373–386. doi: 10.1007/s11104-016-3153-1
Canals, R. M., and Sebastia, M. T. (2000). Soil nutrient fluxes and vegetation changes on molehills. J. Veg. Sci. 11, 23–30. doi: 10.2307/3236771
Changyeol, L., Kim, S., Li, W., Bang, S., Lee, H., Lee, H., et al. (2017). Bioactive secondary metabolites produced by an endophytic fungus Gaeumannomyces sp. JS0464 from a maritime halophyte Phragmites communis. J. Antibiot. 70, 737–742. doi: 10.1038/ja.2017.39
Chen, H., and Boutros, P. C. (2011). VennDiagram: a package for the generation of highly-customizable Venn and Euler diagrams in R. BMC Bioinform. 12:35. doi: 10.1186/1471-2105-12-35
Comby, M., Lacoste, S., Baillieul, F., Profizi, C., and Dupont, J. (2016). Spatial and temporal variation of cultivable communities of co-occurring endophytes and pathogens in wheat. Front. Microbiol. 7:403. doi: 10.3389/fmicb.2016.00403
Constantin, M. E., Vlieger, B. V., Takken, F. I., and Rep, M. (2020). Diminished pathogen and enhanced endophyte colonization upon coinoculation of endophytic and pathogenic Fusarium strains. Microorganisms 8:544. doi: 10.3390/microorganisms8040544
Dawson, L. A., Mayes, R. W., Elston, D. A., and Smart, T. S. (2000). Root hydrocarbons as potential markers for determining species composition. Plant Cell Environ. 23, 743–750. doi: 10.1046/j.1365-3040.2000.00592.x
de Kroon, H., and Knops, J. (1990). Habitat exploration through morphological plasticity in two chalk grassland perennials. Oikos 59, 39–49. doi: 10.2307/3545120
Dufrêne, M., and Legendre, P. (1997). Species assemblages and indicator species: the need for a flexible asymmetrical approach. Ecol. Monogr. 67, 345–366. doi: 10.2307/2963459
Durán, M., Canals, R. M., Sáez, J. L., Ferrer, V., and Lera-López, F. (2020). Disruption of traditional land use regimes causes an economic loss of provisioning services in high-mountain grasslands. Ecosyst. Servic. 46:101200. doi: 10.1016/j.ecoser.2020.101200
Egidi, E., McMullan-Fisher, S., Morgan, J. W., May, T., Zeeman, B., and Franks, A. E. (2016). Fire regime, not time-since-fire, affects soil fungal community diversity and composition in temperate grasslands. FEMS Microbiol. Lett. 363, 1–11. doi: 10.1093/femsle/fnw196
Eldridge, D. J., and Delgado-Baquerizo, M. (2018). Functional groups of soil fungi decline under grazing. Plant Soil 426, 51–60. doi: 10.1007/s11104-018-3617-6
Elliot, S. L., Sabelis, M. W., Janssen, A., van der Geest, L. P. S., Beerling, E. A. M., and Fransen, J. (2000). Can plants use entomopathogens as bodyguards? Ecol. Lett. 3, 228–235. doi: 10.1046/j.1461-0248.2000.00137.x
El-Sayed, A. S. A., Moustafa, A. H., Hussein, H. A., El-Sheikh, A. A., El-Shafey, S. N., Fathy, N. A. M., et al. (2020). Potential insecticidial activity of Sarocladium strictum, an endophyte of Cynanchum acutum, against Spodoptera littorales, a polyphagous insect pest. Biocatal. Agric. Biotechnol. 24:101524. doi: 10.1016/j.bcab.2020.101524
Eom, A. H., Hartnett, D. C., Wilson, G. W. T., and Figge, D. A. H. (1999). The effect of fire, mowing and fertilizer amendment on arbuscular mycorrhizas in tallgrass prairie. Am. Midl. Nat. 142, 55–70.
Faeth, S. H., Haase, S. M., Sackett, S. S., Sullivan, T. J., Remington, R. K., and Hamilton, C. E. (2002). Does fire maintain symbiotic, fungal endophyte infections in native grasses? Symbiosis 32, 211–228.
Ferrer, V., and Canals, R. M. (2008). Proyecto de Ordenación de los Recursos Pascícolas Forestales del Monte Aezkoa n° 1 del CUP. Pamplona: Consultoría Belardi & Universidad Pública de Navarra.
Frank, D. A. (2005). The interactive effects of grazing ungulates and aboveground production on grassland diversity. Oecologia 143, 629–634. doi: 10.1007/s00442-005-0019-2
Gera Hol, W. H., de la Peña, E., Moens, M., and Cook, R. (2007). Interaction between a fungal endophyte and root herbivores of Ammophila arenaria. Basic Appl. Ecol. 8, 500–509. doi: 10.1016/j.baae.2006.09.013
Giauque, H., and Hawkes, C. V. (2016). Historical and current climate drive spatial and temporal patterns in fungal endophyte diversity. Fungal Ecol. 20, 108–114. doi: 10.1016/j.funeco.2015.12.005
Glynou, K., Ali, T., Buch, A.-K., Kia, S. H., Ploch, S., Xia, X., et al. (2016). The local environment determines the assembly of root endophytic fungi at a continental scale. Environ. Microbiol. 18, 2418–2434. doi: 10.1111/1462-2920.13112
Hall, S. L., McCulley, R. L., Barney, R. J., and Phillips, T. D. (2014). Does fungal endophyte infection improve tall fescue’s growth response to fire and water limitation? PLoS One 9:e0086904. doi: 10.1371/journal.pone.0086904
Hamilton, E. W., and Frank, D. A. (2001). Can plants stimulate soil microbes and their own nutrient supply? Evidence from a grazing tolerant grass. Ecology 82, 2397–2402.
Harrison, J. G., and Griffin, E. A. (2020). The diversity and distribution of endophytes across biomes, plant phylogeny and host tissues: how far have we come and where do we go from here? Environ. Microbiol. 22, 2107–2123. doi: 10.1111/1462-2920.14968
Hernández-Restrepo, M., Bezerra, J. D. P., Tan, Y. P., Wiederhold, N., Crous, P. W., Guarro, J., et al. (2019). Re-evaluation of Mycoleptodiscus species and morphologically similar fungi. Persoonia 42, 205–227. doi: 10.3767/persoonia.2019.42.08
Higgins, K. L., Arnold, A. E., Coley, P. D., and Kursar, T. A. (2014). Communities of fungal endophytes in tropical forest grasses: highly diverse host- and habitat generalists characterized by strong spatial structure. Fungal Ecol. 8, 1–11. doi: 10.1016/j.funeco.2013.12.005
Hiiesalu, I., Bahram, M., and Tedersoo, L. (2017). Plant species richness and productivity determine the diversity of soil fungal guilds in temperate coniferous forest and bog habitats. Mol. Ecol. 26, 4846–4858. doi: 10.1111/mec.14246
Hill, N. S., Stringer, W. C., Rottinghaus, G. E., Belesky, D. P., Parrott, W. A., and Pope, D. D. (1989). Influence of endophyte and water regime upon tall fescue accessions. I. Growth characteristics. Ann. Bot. 63, 495–503. doi: 10.1093/oxfordjournals.aob.a087775
Holub, P., Tuma, I., and Fiala, K. (2012). The effect of nitrogen addition on biomass production and competition in three expansive tall grasses. Environ. Pollut. 170, 211–216. doi: 10.1016/j.envpol.2012.07.007
Hosoya, T., Sasagawa, R., Hosaka, K., Gi-Ho, S., Hirayama, Y., Yamaguchi, K., et al. (2010). Molecular phylogenetic studies of Lachnum and its allies based on the Japanese material. Mycoscience 51, 170–181. doi: 10.1007/s10267-009-0023-1
Hothorn, T., Van De Wiel, M. A., Hornik, K., and Zeileis, A. (2008). Implementing a class of permutation tests: the coin package. J. Stat. Softw. 28, 1–23. doi: 10.18637/jss.v028.i08
Huang, Y. L., Devan, M. M. N., U’Ren, J. M., Furr, S. H., and Arnold, A. E. (2016). Pervasive effects of wildfire on foliar endophyte communities in montane forest trees. Microb. Ecol. 71, 452–468. doi: 10.1007/s00248-015-0664-x
Huang, Y. L., Niu, B. F., Gao, Y., Fu, L. M., and Li, W. Z. (2010). CD-HIT Suite: a web server for clustering and comparing biological sequences. Bioinformatics 26, 680–682. doi: 10.1093/bioinformatics/btq003
Hume, D. E., Stewart, A. V., Simpson, W. R., and Johnson, R. D. (2020). Epichloë fungal endophytes play a fundamental role in New Zealand grasslands. J. Roy. Soc. N. Z. 20, 279–298. doi: 10.1080/03036758.2020.1726415
Illana, A., Rodriguez-Romero, J., and Sesma, A. (2013). “major plant pathogens of the magnaporthaceae family genomics of soil- and plant-associated fungi,” in Genomics of Soil- and Plant-Associated Fungi, eds B. A. Horwitz, P. K. Mukherjee, M. Mukherjee, and C. P. Kubicek (Berlin: Springer Berlin Heidelberg), 45–88. doi: 10.1007/978-3-642-39339-6_4
Jing, Z., Cheng, J., Su, J., Bai, Y., and Jin, J. (2014). Changes in plant community composition and soil properties under 3-decade grazing exclusion in semiarid grassland. Ecol. Eng. 64, 171–178. doi: 10.1016/j.ecoleng.2013.12.023
Kankanala, P., Czymmek, K., and Valent, B. (2007). Roles for rice membrane dynamics and plasmodesmata during biotrophic invasion by the blast fungus. Plant Cell 19, 706–724. doi: 10.1105/tpc.106.046300
Kivlin, S. N., Kazenel, M. R., Lynn, J. S., Lee Taylor, D., and Rudgers, J. A. (2019). Plant identity influences foliar fungal symbionts more than elevation in the colorado rocky mountains. Microb. Ecol. 78, 688–698. doi: 10.1007/s00248-019-01336-4
Köhler, B., Gigon, A., Edwards, P. J., Krüsi, B., Langenauer, R., Lüscher, A., et al. (2005). Changes in the species composition and conservation value of limestone grasslands in Northern Switzerland after 22 years of contrasting managements. Perspect. Plant Ecol. Evol. Syst. 7, 51–67. doi: 10.1016/j.ppees.2004.11.003
Koide, R. T., Ricks, K. D., and Davis, E. R. (2017). Climate and dispersal influence the structure of leaf fungal endophyte communities of Quercus gambelii in the eastern Great Basin. USA. Fungal Ecol. 30, 19–28. doi: 10.1016/j.funeco.2017.08.002
Komac, B., Alados, C. L., Bueno, C. G., and Gomez, D. (2013). Spatial patterns of species distributions in grazed subalpine grasslands. Plant Ecol. 212, 519–529.
Kornsakulkarn, J., Saepua, S., Suvannakad, R., Supothina, S., Boonyuen, N., Isaka, M., et al. (2017). Cytotoxic tropolones from the fungus Nemania sp. BCC30850. Tetrahedron 73, 3505–3512. doi: 10.1016/j.tet.2017.05.030
Kotilínek, M., Hiiesalu, I., Košnar, J., Šmilauerová, M., Šmilauer, P., Altman, J., et al. (2017). Fungal root symbionts of high-altitude vascular plants in the Himalayas. Sci. Rep. 7, 1–14. doi: 10.1038/s41598-017-06938-x
Lasanta-Martínez, T., Vicente-Serrano, S. M., and Cuadrat-Prats, J. M. (2005). Mountain mediterranean landscape evolution caused by the abandonment of traditional primary activities: a study of the spanish central pyrenees. Appl. Geogr. 25, 47–65. doi: 10.1016/j.apgeog.2004.11.001
Lau, M. K., Arnold, A. E., and Johnson, N. C. (2013). Factors influencing communities of foliar fungal endophytes in riparian woody plants. Fungal Ecol. 6, 365–378. doi: 10.1016/j.funeco.2013.06.003
Leinonen, P. H., Helander, M., Vázquez-de-Aldana, B. R., Zabalgogeazcoa, I., and Saikkonen, K. (2019). Local adaptation in natural European host grass populations with asymmetric symbiosis. PLoS One 14:e0215510. doi: 10.1371/journal.pone.0215510
Leuchtmann, A., Bacon, C. W., Schardl, L., White, J. F., and Tadych, M. (2014). Nomenclatural realignment of Neotyphodium species with genus Epichloë. Mycologia 106, 202–215. doi: 10.3852/13-251
Leuchtmann, A., and Schardl, C. L. (1998). Mating compatibility and phylogenetic relationships among two new species of Epichloë and other congeneric European species. Mycol. Res. 102, 1169–1182. doi: 10.1017/S0953756298006236
Li, H. Y., Shen, M., Zhou, Z. P., Li, T., Wei, Y. L., and Lin, L. B. (2012). Diversity and cold adaptation of endophytic fungi from five dominant plant species collected from the Baima Snow Mountain. Southwest China. Fungal Divers. 54, 79–86. doi: 10.1007/s13225-012-0153-1
Li, W., and Godzik, A. (2006). Cd-hit: a fast program for cluestering and comparing large sets of protein or nucleotide sequences. Bioinformatics Appl. Note 22, 1658–1659. doi: 10.1093/bioinformatics/btl158
Liu, C., Wang, L., Song, X., Chang, Q., Frank, D. A., Wang, D., et al. (2017). Towards a mechanistic understanding of the effect that different species of large grazers have on grassland soil N availability. J. Ecol. 106, 357–366. doi: 10.1111/1365-2745.12809
Malinowski, D., and Belesky, P. (2000). Adaptations of endophyte-infected cool-season grasses to environmental stresses: mechanisms of drought and mineral stress tolerance. Crop Sci. 40, 923–940. doi: 10.2135/cropsci2004.0523
Manzanilla-López, R., and Lopez-Llorca, L. V. (2017). “Perspectives in sustainable nematode management through Pochonia chlamydosporia applications for root and rhizosphere health,” in Sustainability in Plant Crop Protection, eds R. Peshin and A. Dhawan (Berlin: Springer), doi: 10.1007/978-3-319-99768-1
Mataix-Solera, J., Navarro-Pedreno, J., Guerrero, C., Gomez, I., Marco, B., and Mataix, J. (2002). “Effects of an experimental fire on soil microbial populations in a Mediterranean environment,” in Man and Soil at the Third Millennium. Proceedings International Congress of the European Society for Soil Conservation, Vol. 2, eds J. L. Rubio, R. P. C. Morgan, S. Asins, and V. Andreu (Valencia), 1607–1613. Geoforma Ediciones.
McMullin, D. R., Green, B. D., Prince, N. C., Tanney, J. B., and Miller, D. J. (2017). Natural products of Picea endophytes from the acadian forest. J. Nat. Prod. 80, 1475–1483. doi: 10.1021/acs.jnatprod.6b01157
Meijer, G., and Leuchtmann, A. (1999). Multistrain infections of the grass Brachypodium sylvaticum by its fungal endophyte Epichloë sylvatica. New Phytol. 141, 355–368. doi: 10.1046/j.1469-8137.1999.00332.x
Milchunas, D. G., Sala, O. E., and Lauenroth, W. K. (1988). A generalized model of the effects of grazing by large herbivores on grassland community structure. Am. Nat. 132, 87–106.
Mousa, W. K., Schwan, A., Davidson, J., Strange, P., Liu, H., Zhou, T., et al. (2015). An endophytic fungus isolated from finger millet (Eleusine coracana) produces anti-fungal natural products. Front. Microbiol. 6:1157. doi: 10.3389/fmicb.2015.01157
Múgica, L., Canals, R. M., and San Emeterio, L. (2018). Changes in soil nitrogen dynamics caused by prescribed fires in dense gorse lands in SW Pyrenees. Sci. Total Environ. 639, 175–185. doi: 10.1016/j.scitotenv.2018.05.139
Nagao, H. (2008). Discomycetes on decayed tree fern. Lachnum lanariceps and Lachnum oncospermatum new to Japan. Mycoscience 49, 403–406. doi: 10.1007/S10267-008-0438-0
Nguyen, N. H., Song, Z., Bates, S. T., Branco, S., Tedersoo, L., Menke, J., et al. (2016). FUNGuild: An open annotation tool for parsing fungal community datasets by ecological guild. Fungal Ecol. 20, 241–248. doi: 10.1016/j.funeco.2015.06.006
Ofek-Lalzar, M., Gur, Y., Ben-Moshe, S., Sharon, O., Kosman, E., Mochli, E., et al. (2016). Diversity of fungal endophytes in recent and ancient wheat ancestors Triticum dicoccoides and Aegilops sharonensis. FEMS Microbiolo. Ecol. 92:fiw152. doi: 10.1093/femsec/fiw152
Oksanen, J., Blanchet, F. G., Friendly, M., Kindt, R., Legendre, P., McGlinn, D., et al. (2017). Vegan: Community Ecology Package. R Package Version 2.4-3. Available online at: https://CRAN.R-project.org/package=vegan (accessed April 10, 2019).
Ondeyka, J., Harris, G., Zink, D., Basilio, A., Vicente, F., Bills, G., et al. (2009). Isolation, structure elucidation, and biological activity of virgineone from Lachnum virgineum using the genome-wide Candida albicans fitness test. J. Nat. Prod. 72, 136–141. doi: 10.1021/np800511r
Ownley, B. H., Gwinn, K. D., and Vega, F. E. (2010). Endophytic fungal entomopathogens with activity against plant pathogens: ecology and evolution. BioControl 55, 113–128. doi: 10.1007/s10526-009-9241-x
Pauchard, A., Kueffer, C., Dietz, H., Daehler, C. C., Alexander, J., Edwards, P. J., et al. (2009). Ain’t no mountain high enough: plant invasions reaching new elevations. Front. Ecol. Environ. 7:479–486. doi: 10.1890/080072
Pellegrini, A. F. A., McLauchlan, K. K., Hobbie, S. E., Mack, M. C., Marcotte, A. L., Nelson, D. M., et al. (2020). Frequent burning causes large losses of carbon from deep soil layers in a temperate savanna. J. Ecol. 108, 1426–1441. doi: 10.1111/1365-2745.13351
Pereira, E., Vázquez de Aldana, B. R., San Emeterio, L., and Zabalgogeazcoa, Í (2019). A survey of culturable fungal endophytes from Festuca rubra subsp. pruinosa, a grass from marine cliffs, reveals a core microbiomes. Front. Microbiol. 9:3321. doi: 10.3389/fmicb.2018.03321
Poosakkannu, A., Nissinen, R., and Kytöviita, M.-M. (2014). Culturable endophytic microbial communities in the circumpolar grass, Deschampsia flexuosa in a sub-Arctic inland primary succession are habitat and growth stage specific. Environ. Micribiol. Rep. 7, 111–122. doi: 10.1111/1758-2229.12195
Quesada-Moraga, E., Herrero, N., and Zabalgogeazcoa, Í (2014). “Entomopathogenic and nematophagous fungal endophytes,” in Advances in Endophytic Research, eds V. Verma and A. Gange (New Delhi: Springer), doi: 10.1007/978-81-322-1575-2_4
Ranelli, L. B., Hendricks, W. Q., Lynn, J. S., Kivlin, S. N., and Rudgers, J. A. (2015). Biotic and abiotic predictors of fungal colonization in grasses of the Colorado Rockies. Divers. Distrib. 21, 962–976. doi: 10.1111/ddi.12310
Raudabaugh, D. B., Matheny, P. B., Hughes, K. W., Iturriaga, T., Sargent, M., and Miller, A. N. (2020). Where are they hiding? Testing the body snatchers hypothesis in pyrophilous fungi. Fungal Ecol. 43:100870. doi: 10.1016/j.funeco.2019.100870
Roberts, D. W. (2019). Labdsv: Ordination and Multivariate Analysis for Ecology. R Package Version 1.8-0.
Rudgers, J. A., Kivlin, S. N., Whitney, K. D., Price, M. V., Waser, N. M., and Harte, J. (2014). Responses of high-altitude graminoids and soil fungi to 20 years of experimental warming. Ecology 95, 1918–1928. doi: 10.1890/13-1454.1
Saikkonen, K., Ion, D., and Gyllenberg, M. (2002). The persistence of vertically transmitted fungi in grass metapopulations. Proc. R. Soc. Lond. B 269, 1397–1403. doi: 10.1098/rspb.2002.2006
Saikkonen, K., Young, C. A., Helander, M., and Schardl, C. L. (2016). Endophytic Epichloë species and their grass hosts: from evolution to applications. Plant Mol. Biol. 90, 665–675. doi: 10.1007/s11103-015-0399-6
San Emeterio, L., Múgica, L., Ugarte, M. D., Goicoa, T., and Canals, R. M. (2016). Sustainability of traditional pastoral fires in highlands under global change: effects on soil function and nutrient cycling. Agric. Ecosyst. Environ. 235, 155–163. doi: 10.1016/j.agee.2016.10.009
Sánchez-Márquez, S., Bills, G. F., Acuña, L. D., and Zabalgogeazcoa, I. (2010). Endophytic mycobiota of leaves and roots of the grass Holcus lanatus. Fungal Divers. 41, 115–123.
Sánchez-Márquez, S., Bills, G. F., and Zabalgogeazcoa, I. (2008). Diversity and structure of the fungal endophytic assemblages from two sympatric coastal grasses. Fungal Divers. 33, 87–100.
Sankaranarayanan, C., Hussaini, S. S., Kumar, P. S., and Prasad, R. D. (1997). Nematicidal effect of fungal filtrates against root-knot nematodes. J. Biol. Control 11, 37–41. doi: 10.18311/jbc/1997/7575
Schardl, C. L., Balestrini, R., Florea, S., Zhang, D., and Scott, B. (2009). “Epichloë endophytes: clavicipitaceous symbionts of grasses,” in Plant Relationships. The Mycota (A Comprehensive Treatise on Fungi as Experimental Systems for Basic and Applied Research), Vol. 5, ed. H. B. Deising (Berlin: Springer Berlin Heidelberg), 275–306. doi: 10.1007/978-3-540-87407-2_15
Schippmann, U. (1991). Revision der europäischen arten der gattung Brachypodium palisot de beauvois (Poaceae). Boissiera 45, 1–250.
Schippmann, U., and Jarvis, C. E. (1988). Typification of three Linnean names associated with the genus Brachypodium (Poaceae). Taxon 37, 158–164. doi: 10.2307/1220950
Schirrmann, M. K., Zoller, S., Fior, S., and Leuchtmann, A. (2015). Genetic evidence for reproductive isolation among sympatric Epichloë endophytes as inferred from newly developed microsatellite markers. Microb. Ecol. 70, 51–60. doi: 10.1007/s00248-014-0556-5
Schoch, C. L., Robbertse, B., Robert, V. Vu, D., Cardinali, G., Iriny, L., et al. (2014). Finding needles in haystacks: linking scientific names, reference specimens and molecular data for Fungi. Database (Oxford) 2014:bau061. doi: 10.1093/database/bau061
Schulz, B., and Boyle, C. (2005). The endophytic continuum. Mycol. Res. 109, 661–686. doi: 10.1017/S095375620500273X
Semenova-Nelsen, T. A., Platt, W. J., Patterson, T. R., Huffman, J., and Sikes, B. A. (2019). Frequent fire reorganizes fungal communities and slows decomposition across a heterogeneous pine savanna landscape. New Phytol. 224, 916–927. doi: 10.1111/nph.16096
Shan, R., Stadler, M., Anke, H., and Sterner, O. (1997). Naphthalenone and phthalide metabolites from Lachnum papyraceum. J. Nat. Prod. 60, 804–805. doi: 10.1021/np970145s
Shaw, E. A., Denef, K., de Tomasel, C., Cotrufo, M. F., and Wall, D. H. (2016). Fire affects root decomposition, soil food web structure, and carbon flow in tallgrass prairie. SOIL 2, 199–210. doi: 10.5194/soil-2-199-2016
Slaughter, L. C., Nelson, J. A., Carlisle, E., Bourguignon, M., Dinkind, R. D., Phillips, T. D., et al. (2018). Climate change and Epichloë coenophiala association modify belowground fungal symbioses of tall fescue host. Fungal Ecol. 31, 37–46. doi: 10.1007/s00248-016-0828-3
Soong, J. L., and Cotrufo, M. F. (2015). Annual burning of a tallgrass prairie inhibits C and N cycling in soil, increasing recalcitrant pyrogenic organic matter storage while reducing N availability. Glob. Change Biol. 21, 2321–2333. doi: 10.1111/gcb.12832
Sun, X., Kosman, E., Sharon, O., Ezrati, S., and Sharon, A. (2020). Significant host- and environment-dependent differentiation among highly sporadic fungal endophyte communities in cereal crops-related wild grasses. Environ. Microbiol. 22, 3357–3374. doi: 10.1111/1462-2920.15107
Talbot, N. J., McCafferty, H. R. K., Ma, M., Moore, K., and Hamer, J. E. (1997). Nitrogen starvation of the rice blast fungus Magnaporthe grisea may act as an environmental cue for disease symptom expression. Physiol. Mol. Plant Pathol. 50, 179–195. doi: 10.1006/pmpp.1997.0081
Tardella, F. M., Malatesta, L., Goia, I. G., and Catorci, A. (2018). Effects of long-term mowing on coenological composition and recovery routes of a Brachypodium rupestre-invaded community: insight into the restoration of sub-Mediterranean productive grasslands. Rend. Lincei Sci. Fis. Nat. 29, 329–341.
Taylor, J. W., Jacobson, D. J., Kroken, S., Kasuga, T., Geiser, D. M., Hibbett, D. S., et al. (2000). Phylogenetic species recognition and species concepts in fungi. Fungal Genet. Biol. 31, 21–32.
Uys, R. G., Bond, W. J., and Everson, T. M. (2004). The effect of different fire regimes on plant diversity in southern African grasslands. Biol. Conserv. 118, 489–499. doi: 10.1016/j.biocon.2003.09.024
Vandenkoornhuyse, P., Quaiser, A., Duhamel, M., Le Van, A., and Dufresne, A. (2015). The importance of the microbiome of the plant holobiont. New Phytol. 206, 1196–1206. doi: 10.1111/nph.13312
Vannier, N., Bittebiere, A. K., Mony, C., and Vandenkoornhuyse, P. (2020). Root endophytic fungi impact host plant biomass and respond to plant composition at varying spatio-temporal scales. Fungal Ecol. 44:100907. doi: 10.1016/j.funeco.2019.100907
Vázquez-de-Aldana, B. R., Bills, G., and Zabalgogeazcoa, I. (2013a). Are endophytes an important link between airborne spores and allergen exposure? Fungal Divers. 60, 33–42. doi: 10.1007/s13225-013-0223-z
Vázquez-de-Aldana, B. R., García-Ciudad, A., García-Criado, B., Vicente-Tavera, S., and Zabalgogeazcoa, I. (2013b). Fungal endophyte (Epichloë festucae) alters the nutrient content of Festuca rubra regardless of water availability. PLoS One 8:e84539. doi: 10.1371/journal.pone.0084539
Vázquez-de-Aldana, B. R., Zabalgogeazcoa, I., Rubio de Casas, R., García-Ciudad, A., and García-Criado, B. (2010). Relationships between the genetic distance of Epichloë festucae isolates and the ergovaline and peramine contents of their Festuca rubra hosts. Ann. Appl. Biol. 156, 51–61. doi: 10.1111/j.1744-7348.2009.00360.x
Walker, J. F., Aldrich-Wolfe, L., Riffel, A., Barbare, H., Simpson, N. B., Trowbridge, J., et al. (2011). Diverse Helotiales associated with the roots of three species of Arctic Ericaceae provide no evidence for host specificity. New Phytol. 191, 515–527. doi: 10.1111/j.1469-8137.2011.03703.x
Wang, Y., Liu, L., Yang, J., Duan, Y., Luo, Y., Taherzadeh, M. J., et al. (2020). The diversity of microbial community and function varied in response to different agricultural residues composting. Sci. Total Environ. 715:136983. doi: 10.1016/j.scitotenv.2020.136983
White, T. J., Bruns, T., Lee, S., and Taylor, J. (1990). “Amplificacition and direct sequencing of fungal ribosomal RNA genes for phylogenetics,” in PCR Protocols: A Guide to Methods and Applications, ed. M. A. Innis (San Diego, CA: Academic Press), 315–322.
Wu, M., and Su, Y. (2007). Lachnum alnifolium,a foliicolous discomycetes new to Taiwan. Fung. Sci. 22, 85–89.
Ye, M., and Zhuang, W.-Y. (2003). New taxa of Lachnum (Helotiales. Hyaloscyphaceae) from temperate China. Nova Hedwigia 76, 443–450. doi: 10.1127/0029-5035/2003/0076-0443
Youssar, L., Grüning, B. A., Erxleben, A., Günther, S., and Hüttel, W. (2011). Genome sequence of the fungus Glarea lozoyensis: the first genome sequence of a species from the Helotiaceae family. Eukaryotic Cell 11:250. doi: 10.1128/EC.00142-12
Yuan, Z., Lin, F., Zhang, C., and Kubicek, C. P. (2010). A new species of Harpophora (Magnaporthaceae) recovered from healthy wild rice (Oryza granulata) roots, representing a novel member of a beneficial dark septate endophyte. FEMS Microbiol. Lett. 307, 94–101. doi: 10.1111/j.1574-6968.2010.01963.x
Zabalgogeazcoa, I., Gundel, P., Helander, M., and Saikkonen, K. (2013). Non-systemic fungal endophytes in Festuca rubra plants infected by Epichloë festucae in subarctic habitats. Fungal Divers. 60, 25–32.
Zaurov, D. E., Bonos, S., Murphy, J. A., Richardson, M., and Belanger, F. C. (2001). Endophyte infection can contribute to aluminum tolerance in fine fescues. Crop Sci. 41, 1981–1984. doi: 10.2135/cropsci2001.1981
Zhang, Q., Zhang, J., Yang, L., Zhang, L., Jiang, D., Chen, W., et al. (2014). Diversity and biocontrol potential of endophytic fungi in Brassica napus. Biol. Control 72, 98–108. doi: 10.1016/j.biocontrol.2014.02.018
Keywords: Brachypodium rupestre, disturbances, fire recurrence, culturable endophytes, Omnidemptus graminis, Lachnum sp., Epichloë typhina
Citation: Durán M, San Emeterio L, Múgica L, Zabalgogeazcoa I, Vázquez de Aldana BR and Canals RM (2021) Disruption of Traditional Grazing and Fire Regimes Shape the Fungal Endophyte Assemblages of the Tall-Grass Brachypodium rupestre. Front. Microbiol. 12:679729. doi: 10.3389/fmicb.2021.679729
Received: 12 March 2021; Accepted: 18 May 2021;
Published: 11 June 2021.
Edited by:
Daniela Minerdi, University of Turin, ItalyReviewed by:
George Newcombe, University of Idaho, United StatesDebdulal Banerjee, Vidyasagar University, India
Copyright © 2021 Durán, San Emeterio, Múgica, Zabalgogeazcoa, Vázquez de Aldana and Canals. This is an open-access article distributed under the terms of the Creative Commons Attribution License (CC BY). The use, distribution or reproduction in other forums is permitted, provided the original author(s) and the copyright owner(s) are credited and that the original publication in this journal is cited, in accordance with accepted academic practice. No use, distribution or reproduction is permitted which does not comply with these terms.
*Correspondence: María Durán, maria.duran@unavarra.es