- 1College of Food Science and Engineering, Heilongjiang Bayi Agricultural University, Daqing, China
- 2Heilongjiang Engineering Research Center for Coarse Cereals Processing and Quality Safety, Daqing, China
- 3Key Laboratory of Agro-Products Processing and Quality Safety of Heilongjiang Province, Daqing, China
- 4National Coarse Cereals Engineering Research Center, Heilongjiang Bayi Agricultural University, Daqing, China
BS Sufu is a fermented food that is made by mixed black soybeans and soybeans. Microbial communities and metabolites play an important role for the final product. We characterized microbial diversity of BS Sufu during fermentation by high-throughput DNA sequencing. Meanwhile, volatile compounds were investigated by solid-phase microextraction (SPME) coupled with gas chromatography–mass spectrometry (GC-MS). The results showed that bacterial diversity was higher than that of fungi in BS Sufu. We found the existence of bacterial and fungal core communities, including Enterococcus, Enterobacter, Rhizopus, and Monascus. Network analysis indicated that bacterial and fungal communities maintain positive and negative interactions, which are important to shape the resident microbial communities in Sufu. In addition, 17 free amino acids (FAAs) were detected at the post-fermentation stage, and umami amino acid mainly contributed to taste of BS Sufu. Furtherly, a total of 79 volatile constituents in BS Sufu, including nine alcohols, 31 esters, and four aldehydes, form synergistically the unique odor of Sufu. Additionally, the correlations between microbiota and metabolites were analyzed. Our results suggested that these microbial taxa and metabolites contribute to the taste and flavor of BS Sufu. This study provided information for analysis of BS Sufu at different fermentation periods in terms of the microbial diversity and metabolites, and this information was important to understand the properties of mixed soybeans Sufu.
Introduction
Sufu is a traditional fermented soybean food in China that shares similar shapes and fermentation mechanisms with cheese, but the taste and flavor are different (Wan H. et al., 2020). Sufu is normally consumed as a flavor enhancer and appetizer owing to its characteristic flavor, pleasant taste, and nutritional value in China and other Asian countries (Xie et al., 2018). Based on the strain used as a starter, Sufu can be classified into three different types, including mold-fermented Sufu, bacteria-fermented Sufu, and naturally fermented Sufu (Feng et al., 2013). The nutrition and taste of Sufu are affected by many factors, for example, the type of raw materials and the production process. Current studies on Sufu mainly focus on optimization of technological conditions, determination of flavor components, and microbial analysis during fermentation process, but there are few studies on the production of Sufu with various beans as raw materials. The content of protein and essential amino acids (EAAs) in black beans is higher than that of soybeans, and the content of phenolic substances is very rich (Meng et al., 2020). Therefore, we decided to use mixed black beans and soybeans (BS) as raw materials to make Sufu by processing technology of mold-fermented Sufu with minor modifications, which can promote nutrition diversification of traditional Sufu.
Sufu is produced under open or semi-open conditions via the actions of various microbes, including starter and indigenous microorganisms, which play important roles in flavor generation (Huang et al., 2018). At present, high-throughput sequencing (HTS) provides a reliable method to comprehensively describe the dynamics of microbial communities, and these studies have expanded our knowledge of the microbial community structure in Sufu. Recent published data indicate that Sufu microbiota showed a prevalence of bacteria genus belonging to Lactococcus, Streptococcus, Enterobacter, and Acinetobacter (Wan H. F. et al., 2020); and fungi such as Trichosporon and Aspergillus were also described. In addition, microbial abundances are mostly correlated with chemical characteristics, such as salinity, ethanol content, and environmental factor. Moreover, metabolomic approaches, such as gas chromatography (GC), mass spectrometry (MS), and high-performance liquid chromatography (HPLC) have been applied to determine the metabolite profiles of Sufu (Moy and Chou, 2010). However, few systematic studies have evaluated the correlation on the microbial compositions and metabolites of Sufu at different fermentation stages; so far, there is no study on the characteristics of mixed beans Sufu. Accordingly, in this study, we aimed to explore the diversity and composition of the microbial community, the profile of metabolites, including amino acids and flavor compounds, and the correlation between microbes and metabolites in BS Sufu. The results will help us to improve understanding of the role of microorganisms in the production of Sufu, which will provide a certain reference for research and development of a new type of Sufu.
Materials and Methods
BS Sufu Preparation and Sample Collection
The isolated strains Rhizopus microsporus SE-3 and Rhizopus oryzae CD-1 (derived from spontaneous fermented Sufu in our previous study) combined with Actinomucor elegans CCIC3093 (China Center of Industrial Culture Collection) of 1:1:1 ratio were used as the starter for inoculation and fermentation. Black beans (Jihei 6) and soybeans (Heihe 43) (Jiansanjiang, Heilongjiang, China) of 1:1 ratio were used as raw materials to prepare Sufu, which is called BS Sufu. Simply, pehtze is prepared by inoculating mixed strain on the surface of tofu cubes, then the pehtze is salted for 24 h, and the salted pehtze is dispensed into wide-mouthed bottles and ripened in the dressing mixture for 60 days. The dressing mixture of BS Sufu mainly consists of food-grade monascus pigment, yellow rice wine, high-concentration liquor, and other spices (Supplementary Figure 1). Sufu samples were collected periodically at different stages of fermentation: pehtze (BS_M), salted pehtze (BS_Y), and post-fermentation 10 days (BS_10), 30 days (BS_30), and 60 days (BS_60). The samples were collected and stored at −80°C.
Free Amino Acid Detection of BS Sufu
Free amino acids (FAAs) were detected using Amino Acid Analyzer (Model L-8900, Hitachi, Tokyo, Japan). The sample was extracted with 0.01 mol/L of HCl ultrasonically for 5 min and centrifuged at 4,000 rpm for 10 min; then the supernatant was added with 2%∼4% sulfosalicylic acid (Solarbio, Beijing, China), centrifuged, and filtered by a 0.22-μm filter for measurement. The chromatographic conditions were as follows: 2622#PH ion exchange chromatography column (4.5 × 60 mm), column temperature (57°C), flow rate (0.4 ml/min), and injection volume (20 μl).
Volatile Flavor Composition Analysis of BS Sufu Through Headspace Solid-Phase Microextraction–Gas Chromatography–Mass Spectrometry
Individual samples (5 g) were mashed and placed into 20-ml headspace vial. Then, the vial was sealed after adding isoamyl phenylacetate (Solarbio, Beijing, China) and heated in water bath at 60°C for 20 min. After that, the solid-phase microextraction needle (57348-U, Supelco, Bellefonte, Pennsylvania, United States) was inserted into the vial to extract for 30 min (the needle being aged at 250°C before use) and immediately inserted into the GC inlet, followed by 3 min of desorption. Subsequently, the GC-MS analysis of volatile compounds was performed on Agilent 7890A-5975C GC-MS equipped with HP-5MS chromatographic column (60 m × 250 μm × 0.25 μm). High-purity helium (purity > 99.999%) was used as the carrier gas at a constant flow of 1.5 ml/min, and the inlet temperature was set at 250°C. Oven starting temperature was 50°C, programmed at 5°C/min to 100°C for 2 min, programmed at 4°C/min to 140°C for 1 min, programmed at 4°C/min to 180°C for 2 min, and finally programmed to 250°C at 5°C/min and held at 250°C for 5 min. The conditions of MS were ion source temperature 230°C, quadrupole temperature 150°C, electron ionization (EI) 70 eV, and full scan 35∼550 da.
DNA Extraction and High-Throughput Sequencing
Total genomic DNA was extracted using an Ezup Column Genomic DNA Purification Kit (Sangon Biotech, Shanghai, China), according to the manufacturer’s protocol. The quality and quantity of the extracted DNA were assessed by the ratios of 260 nm/280 nm and 260 nm/230 nm with a NanoDrop spectrophotometer (A360, AOE, Shanghai, China). The V3–V4 region of the bacterial 16S rRNA gene and ITS1 region of the fungi were amplified using TransStart Fastpfu DNA Polymerase (TransGen, Beijing, China). Then PCR products were purified using the SanPerp Column PCR Product Purification Kit (Sangon Biotech, Shanghai, China). The 16S rRNA and ITS1 gene amplicons were sequenced using Illumina HiSeq deep sequencing (Majorbio, Shanghai, China).
Bioinformatics Analysis
The double-end sequence data obtained by HiSeq sequencing were spliced or merged into a sequence tag, and quality control filtering was performed on the quality of reads and the effect of the merging. Then, the stitching sequence obtained is the original tag data. The read sequences were aligned with species annotation databases through the UCHIME Algorithm. Then, the chimera sequence was identified and removed to obtain the final valid data. Usearch software was used to cluster the tags at a similarity level of 97% to obtain operational taxonomic units (OTUs) (Hang et al., 2014). Alpha diversity was evaluated using the Mothur v.1.11.0 program, and Kyoto Encyclopedia of Genes and Genomes (KEGG) function of 16S RNA gene was annotated by Tax4Fun and Silva database. Ultimately, redundancy analysis (RDA) was performed using Canoco software to reflect the relationship between the core microorganisms and flavor compounds (Barka, 2019).
Statistics Analysis
SPSS 22.0 was used to calculate the significance of difference by ANOVA; the results were expressed as the mean ± SD.
Sequence Accession
The datasets (raw sequences) generated and analyzed during this study have been submitted to National Center for Biotechnology Information (NCBI) Sequence Read Archive (SRA) repository with accession number PRJNA693985 and can be accessed through the following link: http://www.ncbi.nlm.nih.gov/sra/PRJNA693985.
Results
Free Amino Acid Composition and Content of BS Sufu
Seventeen FAAs were detected in BS Sufu at the post-fermentation stage (Table 1). The content of all FAAs reached the highest in BS_60, except for ARG. FAAs have the characteristics of low taste threshold and strong taste ability. According to the taste characteristics of FAAs, they are grouped into three categories, including umami amino acids (UAAs), sweet amino acids (SAAs), and bitter amino acids (BAAs). The content of total SAAs and UAAs accounted for 62.5%, and total BAAs accounted for 37.5%. The content of seven EAAs was relatively stable, maintaining about 37% in post-fermentation stages. The taste intensity is affected by both the content of the taste substance and the threshold value. TAV is the ratio between the concentration of the taste substance and its threshold value. The value (TAV > 1) can be considered as the food taste contribution: the greater the TAV value, the greater the contribution of FAAs to taste. In the BS_10 stage, FAAs with TAV > 1 included ASP, GLU, ALA, VAL, and HIS; FAAs in the BS_30 stage includes ASP, GLU, PHE, ALA, VAL, LYS, and HIS; the BS_60 stage includes ASP, GLU, PHE, ALA, VAL, MET, ILE, TYR, LYS, and HIS. In total, the kinds of BAAs were significantly higher than those of UAAs and SAAs, but the TAV value of UAAs was significantly higher than that of other FAAs, indicating that UAAs contribute to the taste of BS Sufu.
Volatile Flavor Composition Analysis of BS Sufu
A total of 79 volatile constituents in BS Sufu at the post-fermentation stage, including nine alcohols, 31 esters, four aldehydes, seven ketones, two acids, eight olefins, seven alkanes, three ethers, and eight other organic compounds, form synergistically the unique odor of Sufu (Table 2). These volatile compounds in three fermentation stages were different. Compared with BS_30, BS_60 had fewer kinds of flavor compounds, but the content was higher. For example, in the BS_60 stage, the total amount of alcohols and esters compounds was significantly higher than that of the BS_30 stage. Esters accounted for the highest proportion in all compounds identified of BS Sufu, which mainly contribute to the complex flavor of BS Sufu. The relative content of ethyl caproate, isoamyl phenylacetate, ethyl palmitate, ethyl linoleate, and ethyl alcohol was higher than that of other flavor compounds. The relative content of ethyl alcohol and phenethyl alcohol was higher among the alcohol components. In addition, the aldehyde, ketone, alkane, and olefin compounds were also identified, but the content was extremely low, which contributes less to the flavor of BS Sufu.
Microbial Community Dynamics of BS Sufu During Fermentation
After sequencing and quality control, the clean reads of all samples were clustered, revealing 346 and 168 OTUs of bacteria and fungi at 97% similarity level, respectively. Furthermore, alpha diversity was analyzed (Table 3). The Shannon index of bacteria was generally higher than that of fungi (P < 0.05), which proved that bacterial diversity was higher than that of fungi in BS Sufu. At the end of fermentation, the Shannon index of bacteria and fungi were reduced. Likewise, abundance-based coverage estimator (ACE) index indicated that the abundance of bacterial species was significantly higher than that of fungi. The coverage of samples at different fermentation stages is greater than 99%, indicating that sequencing has a large coverage of species, and showed that the sequencing depth was sufficient, which proved the reliability of the sequencing results and could represent the true composition of microorganisms in BS Sufu.
Bacterial community dynamics were evaluated based on changes in relative abundance at phylum and genus levels (Figures 1A,B). Firmicutes, Proteobacteria, and Bacteroides were the main phyla, which exist in the whole fermentation process. At the genus level, the abundances of Enterococcus and Enterobacter were higher. At the stage of post-fermentation, the abundance of Acinetobacter decreased from 23.91% to 5.74%, while the abundance of Enterobacter and Enterococcus increased to 19.92% and 53.67%, respectively. During the whole post-fermentation process, the abundance of Enterococcus was the highest, the abundance of Bacillus increased, and Acinetobacter was almost undetectable.
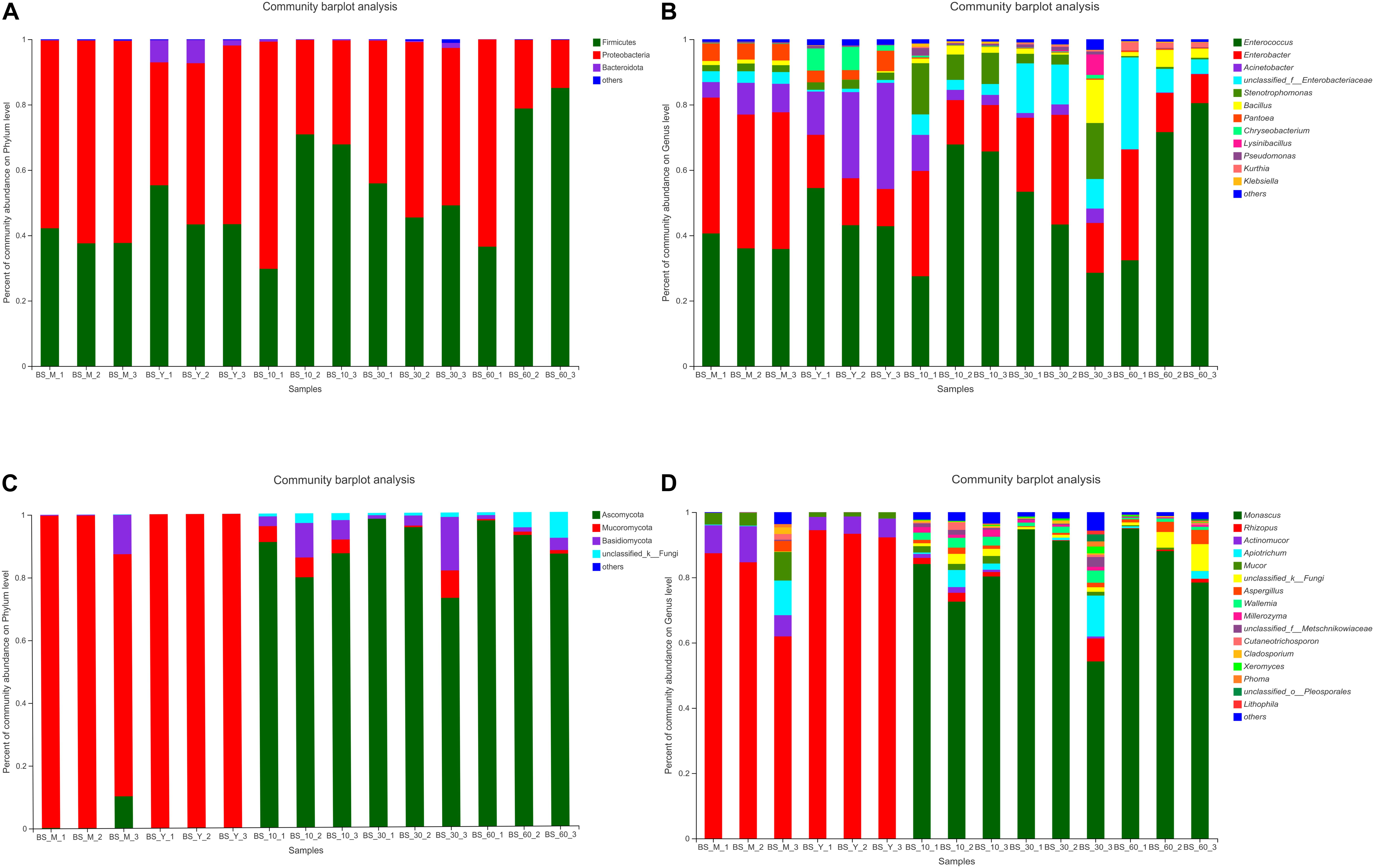
Figure 1. Species distribution and abundance of BS Sufu in different fermentation stages. The relative abundance of each taxon was defined as the percentage of the same taxon to the corresponding total sequences for each sample. Phylum [(A), bacteria; (C), fungi]; genus [(B), bacteria; (D), fungi]. Some classes with abundances of less than 1% were summarized as “other.”
The phylum of fungi included Ascomycota, Mucoromycota, and Basidiomycota (Figure 1C). The Mucoromycota was mainly concentrated in the pre-fermentation stage, but the Ascomycota and Basidiomycota were concentrated in the post-fermentation stage. The distribution of fungi genus is shown in Figure 1D. The abundance of Rhizopus in the pre-fermentation stage is 77.96% in the BS_M stage and 93.35% in the BS_Y stage, while the abundances of Mucor and Actinomucor declined in the post-fermentation stage. In general, the diversity of fungi elevated in the post-fermentation stage; for instance, Wallemia, Millerozyma, and Xeromyces begin to appear, and the abundance of Monascus was the highest in entire post-fermentation process. In summary, BS Sufu had significantly more bacterial species than fungi during the pre-fermentation stage, but the identified fungal species are slightly higher than bacteria during the post-fermentation stage.
Comparison of Microbial Communities in BS Sufu at Different Fermentation Stages
The Lefse analysis was performed to uncover differentially abundant biomarkers by an Linear discriminant analysis (LDA) score (log 10) > 4 (Supplementary Figure 2). The unclassified_Bacteria was significantly enriched in BS_M, with an LDA value of 4.8. Acinetobacter (5.1) and Chryseobacterium (4.5) were significantly enriched in BS_Y, which contributed to the difference between BS_Y and other samples. Only the LDA score of Pseudomonas (3.4) at BS_10 was calculated, indicating a little contribution to the difference. Stenotrophomonas (4.7) was significantly enriched in BS_30; Enterobacteriaceae and Kurthia were significantly enriched in BS_60, demonstrating that their abundance was different from that of other species. Compared with bacteria, the LDA results of fungi were more unambiguous. Actinomucor (4.8) had a greater impact on the difference between BS_M and other samples. The significantly enriched species in BS_Y was Rhizopus, and significantly enriched species in BS_10 was Wallemia. Compared with other fermentation stages, there are obviously more enriched species in the BS_60 stage; nevertheless, only Monascus had a greater contribution to sample differences.
Network Inference of Species in BS Sufu
The relationship between BS Sufu microorganisms based on the relevant network diagram was studied, and the correlation coefficient in different species was calculated (Figure 2). The correlation network diagram of genus level revealed that there were significant interactions between different genera of the BS microorganisms. In the bacterial network diagram, Acinetobacter and Enterobacteriaceae had a significantly positive correlation, and the clustering coefficient was 0.504. The number of genera connected to Enterobacteriaceae and Staphylococcus was the largest (degree = 12) (degree represents the number of one node connected to other node), followed by Weissella, but the clustering coefficients of Weissella were small (0.348), and Pseudomonas and Lactococcus had the largest clustering coefficients of 0.669. There was an obviously positive correlation between Lactococcus and other bacterial genera. The clustering coefficient indicated the importance of this genus in the entire microbial environment.
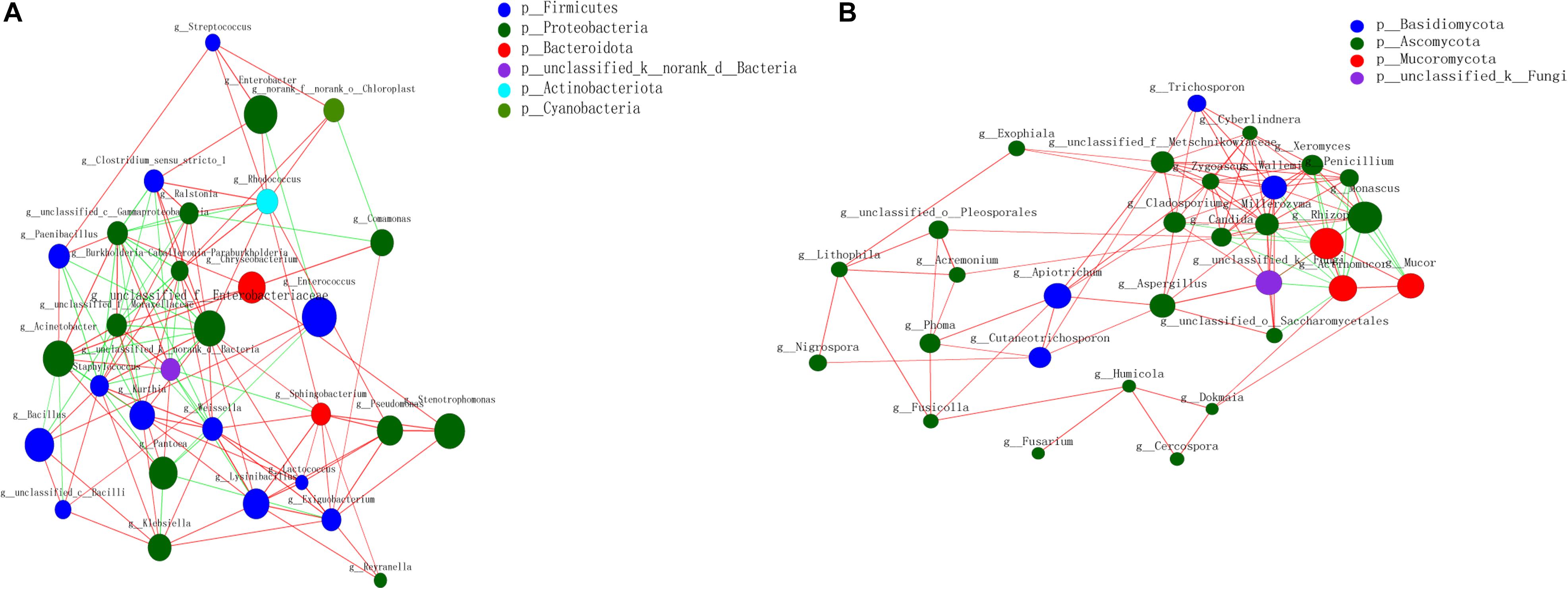
Figure 2. Horizontal correlation network diagram of BS Sufu bacteria (A) and fungi (B). Color and size of the nodes represent relative abundance of different microbiota genus, respectively. Red indicates positive correlation, and green indicates negative correlation. The thickness of the line indicates the size of the correlation coefficient. p, phylum; g, genus.
In the fungi network diagram, Mucor has the biggest positive correlation with Actinomucor and Rhizopus in the pre-fermentation stage. The number of fungi genera interconnected with Millerozyma was the largest (degree = 15). Interestingly, Monascus added in the post-fermentation process is positively correlated with Rhizopus and Mucor. In addition, Zygosaccharomyces had a significantly positive correlation with other genera.
Correlation Analysis of Microbial Diversity and Metabolites of BS Sufu
Bacteria Pearson correlation heat map (Figure 3A) indicated that all FAAs were positively correlated with Kurthia, unclassified_Enterobacteriaceae, Staphylococcus, Weissella, and Klebsiella. Besides, all FAAs had obviously negative correlation with Acinetobacter, Gammaproteobacteria, Paenibacillus, and Streptococcus. The fungal correlation is shown in Figure 3B. All amino acids had an obviously positive correlation with Monascus, unclassified_Saccharomycetales, Wallemia, Millerozyma, and Xeromyces. Furthermore, Aspergillus and Zygoascus were also positively correlated with GLU, SER, PRO, etc.
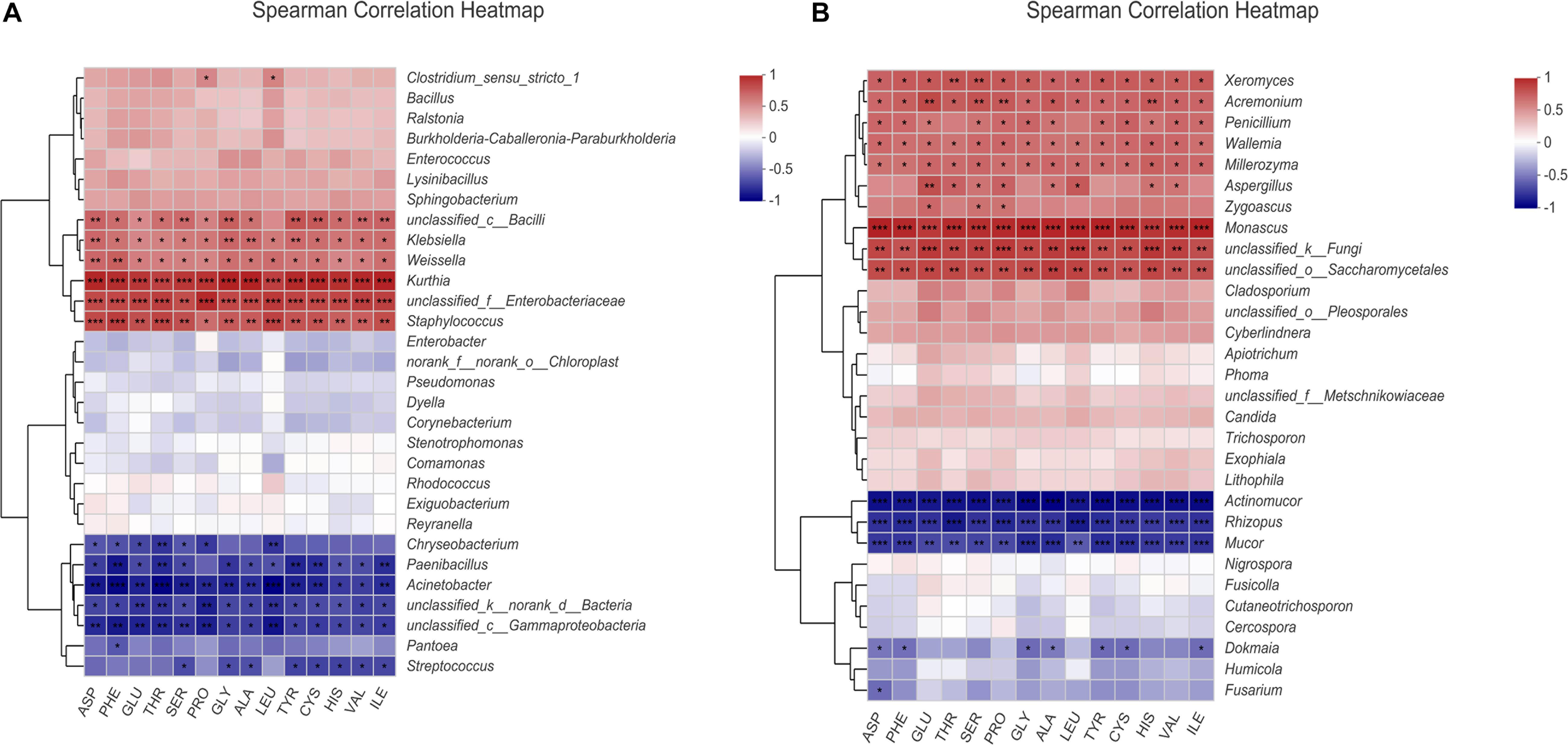
Figure 3. Heat map of the correlation between amino acids and microorganisms of BS Sufu: (A) bacteria and (B) fungi. The figure shows 14 free amino acids with high content; * represents the correlation between free amino acids and species (***P < 0.001, **P < 0.01, *P < 0.05). Colors closer to red mean stronger positive correlation; colors close to blue means stronger negative correlation.
Redundancy analysis revealed the correlation between dominant genus and flavor compounds (Figure 4). Enterococcus had a significantly positive correlation with esters, alcohols, ketones, alkanes, and ethers. Stenotrophomonas and Bacillus were significantly positively correlated with organic acids and aldehydes. Enterobacter and unclassified-Enterobacteriaceae were positively correlated with organic acids but negatively correlated with the other types of compounds. Unclassified-fungi had a significantly positive correlation with alcohols, esters, ketones, and ethers, which were concentrated in the end of post-fermentation. Rhizopus and Apiotrichum were positively related with organic acids and aldehydes, but the two substances were concentrated in the early stage of post-fermentation. Monascus was also positively correlated with alcohols, ethers, and ketones and negatively correlated with esters and organic acids.
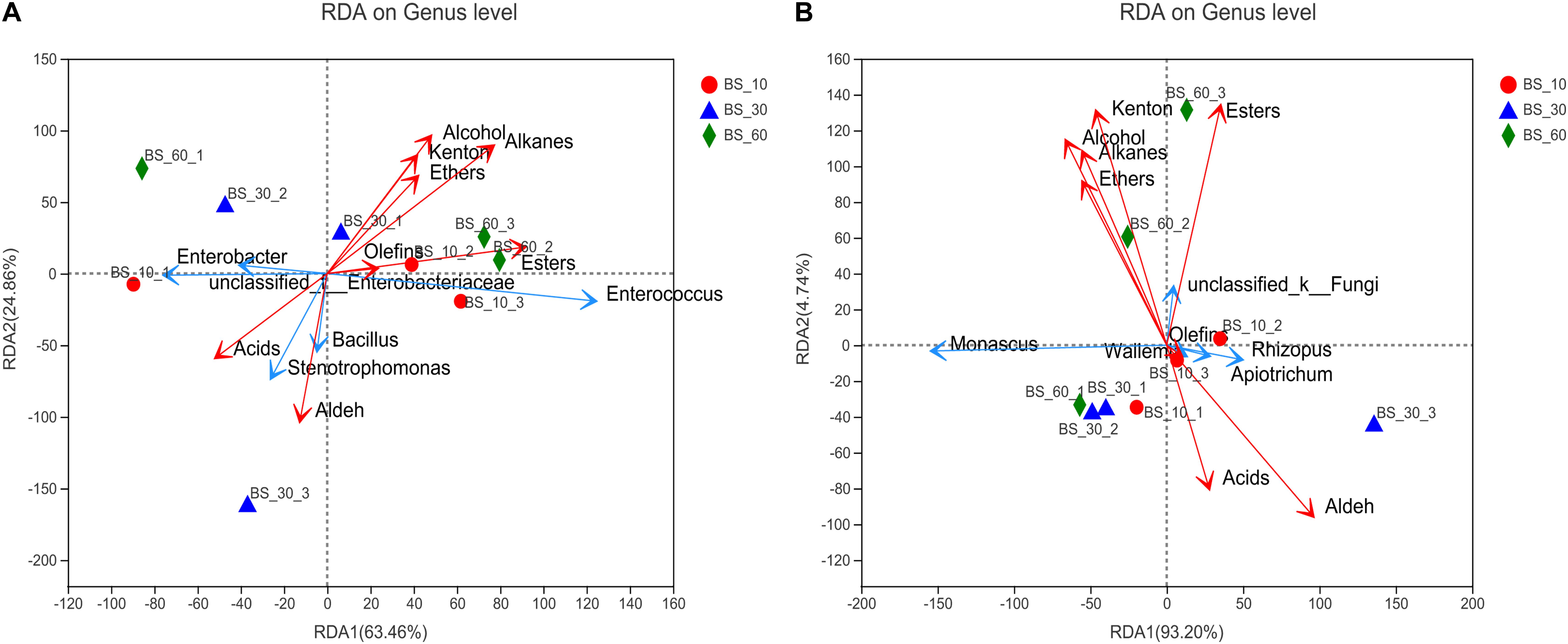
Figure 4. Redundancy analysis (RDA) of the correlation between flavor compounds and microorganisms of BS Sufu: (A) bacteria and (B) fungi. The blue arrow represents the species; the red arrow represents flavor compounds. The longer the arrow, the stronger the correlation.
Functional Prediction of Bacteria Gene
As shown in Figure 5, the function of bacterial genes was mostly annotated in metabolism (59.9%), genetic information processing (15.1%), environmental information processing (18.9%), cellular processes (2.8%), human diseases (2.5%), and biological systems (0.7%). Among them, carbohydrate metabolism accounted for 25.8% of metabolism, lipids 6.7%, amino acids 21.4%, and metabolism of terpenoids and polyketides 3.9%. The carbohydrate metabolism was more abundant in BS_M, BS_30, and BS_60. The amino acid metabolism was enriched in BS_Y, BS_10, and BS_60. In general, the genes involved in carbohydrate metabolism were more than those in amino acid metabolism. The function prediction showed that majority of the functional information of bacterial community in BS Sufu was meaningful.
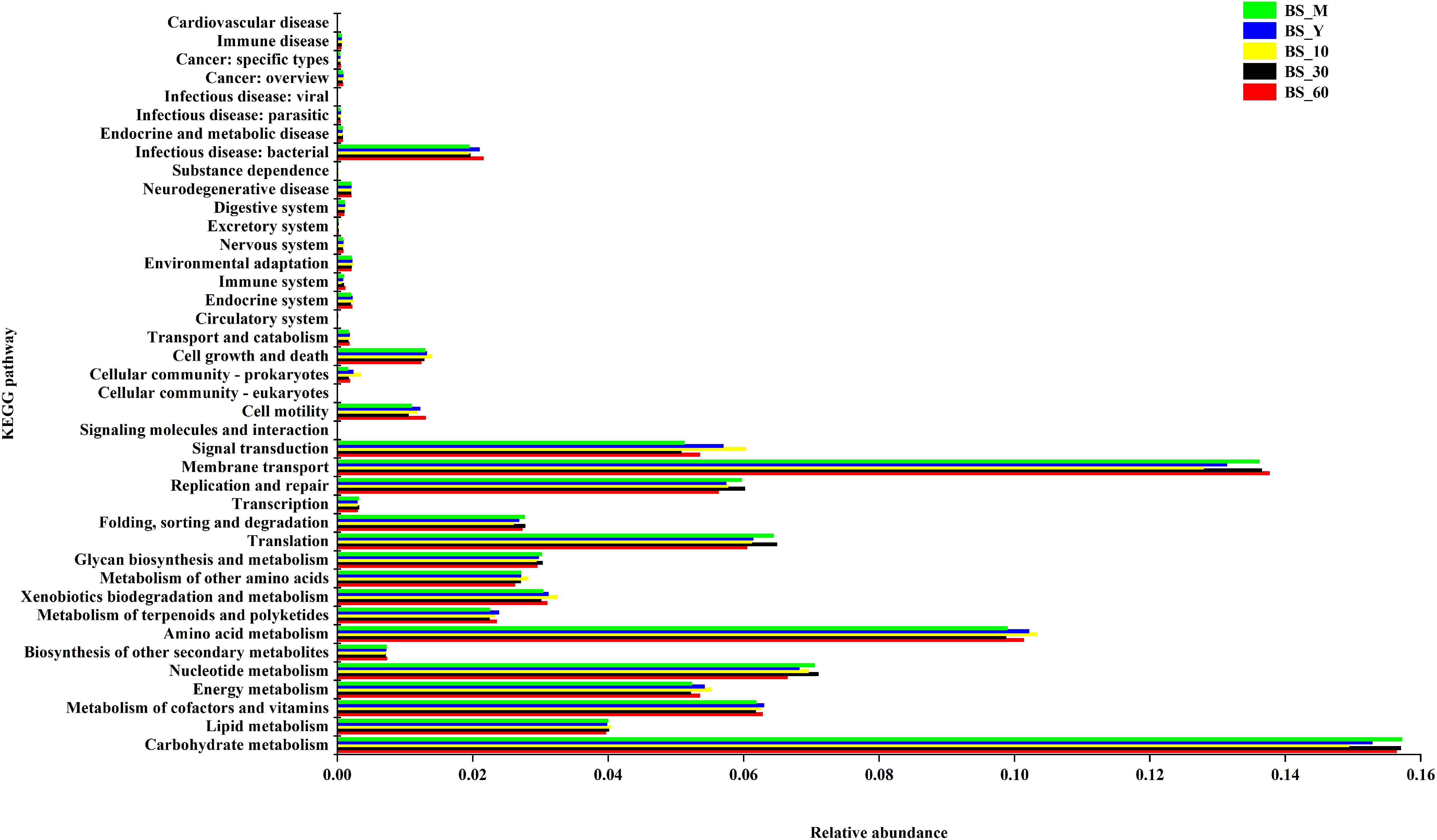
Figure 5. Kyoto Encyclopedia of Genes and Genomes (KEGG) function annotation of bacteria in BS Sufu.
Discussion
The fermentation process of Sufu is complicated and affected by various factors (Liu et al., 2018). As a fermented food rich in protein, protein degradation and amino acid metabolism are extremely key in the formation of flavor substances. The content of FAAs depends on the ratio of their generation and degradation (Tang et al., 2018). ARG has the function of anti-tumor and regulating immunity (Hui et al., 2017; Mondanelli et al., 2017). LYS can increase the absorption and accumulation of calcium, accelerate bone growth, enhance immunity, and promote development (Korompokis et al., 2016). PHE can synthesize important neurotransmitters and hormones to improve memory in the body (Kumar et al., 2017; Souza et al., 2017). LEU can improve the recovery ability of muscle fibers and make thinking more agile; MET is involved in fat reduction and detoxification, as well as the synthesis of choline; THR has the function of enhancing immunity and transforming certain amino acids to achieve balance (Zhang et al., 2017). FAAs not only give BS Sufu a unique taste but also make the BS Sufu possess a certain physiological effect. The total amount of FAAs increases with the extension of BS Sufu fermentation time. In addition, through comparative studies, we found that the types and contents of various FAAs in BS Sufu are not significantly different from those of previous studies (Xu et al., 2019). However, due to the different fermentation strains used, there are certain differences in the enzyme systems during the fermentation process, which will lead to differences in the total amount of amino acids.
We identified 79 flavor constituents in BS Sufu. Ji et al., identified 35 volatile compounds in nine Sufu (Ji, 2020). Since the flavor components in Sufu are affected by raw materials, production technology, fermentation microorganisms, and environmental conditions, we believe that the effects of raw materials (black beans and soybeans) and microorganisms (three strains) make the BS Sufu rich in flavor substance diversity and higher content. Lipid oxidation gives a large number of volatile compounds or aroma precursors, which can subsequently react with amino acids derived from proteolysis to form the characteristic aroma of Sufu. The esters are the most common volatile compounds detected in BS Sufu. There are 31 kinds of ester, and their content increased with the extension of fermentation time. Esters are mainly synthesized by two pathways. One is the reaction of acyl-CoA with the corresponding higher alcohols under the action of ester synthase, and the other is the formation of organic acids and higher alcohols through esterification, but the synthesis speed of this pathway is very slow. Besides, acetyl-CoA is the precursor for the synthesis of fatty substances. Therefore, there is a certain competitive relationship between ester metabolism and lipid metabolism; but during the fermentation of BS Sufu, the abundance of carbohydrate metabolism is much higher than that of lipid metabolism (Figure 5). So, acetyl-CoA is mainly used for ester metabolism. Studies have also shown that hexanal can generate hexanol under the action of alcohol dehydrogenase, hexanol can generate hexyl acetate, and hexanal can be oxidized to hexanoic acid and can further generate esters, such as methyl hexanoate (Egea et al., 2014). Esters usually have a special aroma (Moy et al., 2012). The relative content of ethyl caprylate is 8.67% with brandy flavor, being mainly used for flavoring and spices. Ethyl phenylacetate is often found in liquor with a noticeable rose fragrance and honey-like smell. Ethyl myristate has the scent of iris oil, which is generated by esterification of myristic acid and ethanol. Ethyl caproate has a strong fruity and wine aroma and has the aroma of pineapple and banana (Kai et al., 2013). In addition, some esters also have very good pharmacological effects, such as linoleic acid ethyl ester, which has the same effect as linoleic acid in lowering cholesterol and blood lipid and can prevent or reduce atherosclerosis. Moreover, the side effect of ethyl linoleate is much lower than that of linoleic acid. BS_60 sample is rich in long-chain esters such as ethyl stearate, methyl palmitate, and ethyl linoleate. These esters improve the flavor of BS Sufu as well as health benefits. There are nine kinds of alcohols detected in BS Sufu. After 60 days of fermentation, the relative content of alcohols is significantly reduced, which may be the result of esterification or oxidation of alcohols. Alcohols often have botanical, aromatic, and earthy odors. Although their thresholds are high, they can form esters with organic acids to facilitate the formation of Sufu flavor (Wu et al., 2009). Several kinds of acids, aldehydes, and ketones were detected in BS Sufu. The aldehydes possess aromatic characteristics such as fresh fragrance, fruity and nutty fragrance, and low flavor threshold (Qi et al., 2012). Ketones are generally formed by fat degradation and oxidation reactions (Jonsdottir et al., 2008). The other volatile components detected in BS Sufu are mainly furans and pyrazines. Among them, 2-pentylfuran is mainly derived from the oxidation of linoleic acid or 2,4-decadienal, with a low threshold value and has a bean aroma (Lu et al., 2014).
The bacterial diversity of BS Sufu is higher than that of fungi. The composition of dominant bacteria in BS Sufu at different fermentation stages has a certain similarity, yet the composition of dominant fungi is quite different. Enterococcus and Enterobacter are present in the whole fermentation process, and their abundance is relatively high. Enterococcus with higher abundance was also found in Jiajiang Sufu (Wan H. F. et al., 2020). Similarly, Enterobacter and Enterococcus are widely present in various fermented foods, such as soy sauce, fruit juice, and tea. The abundance of the two genera in BS Sufu is relatively high, but we have measured the total number of coliforms, and the result is <3.0 MPN/g, which is lower than the national standard, indicating that the Sufu products are safe. Previous reports have shown that Lactococcus, Acinetobacter, Tetragenococcus, Pseudomonas, and Lactobacillus are the dominant bacteria and crucial contributors during the production of fermented foods, such as cheese, Sufu, soy sauce, liquor, and tea (Tanaka et al., 2012; Liu and Qiao, 2019). Similarly, Lactobacillus, Acinetobacter, and Pseudomonas were detected in BS Sufu. Acinetobacter and Chryseobacterium are abundantly enriched in BS_Y, but the amounts are significantly reduced in post-fermentation. Acinetobacter is also abundant in other fermented foods (Li et al., 2017; Huang et al., 2018). Previous studies have indicated that Acinetobacter is well known for its capacity to secrete esterolytic enzymes and is positively related to flavor compounds (Hassan et al., 2010), particularly esters.
Lefse difference analysis showed that Lactobacillus and Streptococcus were enriched in BS_M samples. Lactobacillus was often used in cheese fermentation (Zhang X. X. et al., 2020) and existed in other fermented products (Li et al., 2020). Streptococcus had a strong ability to metabolize carbohydrates (Gefei et al., 2020). The genus is enriched in the pre-fermentation stage, which may be related to carbohydrates and protein metabolism. The Pantoea is higher during the pre-fermentation period, and 43% of Pantoea is distributed in the salted pehtze (Figure 1). Pantoea can produce volatile compounds such as p-hydroxyphenylethyl alcohol, methyl 4-hydroxybenzeneacetate, and certain antibacterial properties (Mingxi et al., 2012).
Compared with bacteria, the fungal community composition is quite different. The main genera in the pre-fermentation stage are the inoculated Rhizopus and Mucor. Inoculated strain requires the accumulation of a large amount of protease and other enzymes for fermentation (Guiliang et al., 2020). Rhizopus and Mucor can secrete a large amount of protease to decompose large molecular proteins. In BS-Y, Rhizopus rose to 93%, which indicated that Rhizopus has a strong resistance to high salt environments. In the post-fermentation stage, Monascus is the dominant genus because Monascus pigment is added. Millerozyma is a yeast that can degrade bio-amine amines and has a certain heat resistance. It is often found in fermented wines (Jiangang et al., 2018) and also used to degrade bio-amines purposefully during the fermentation of soy sauce (Cheng et al., 2019). Wallemia is a salt-tolerant fungus with metabolites that have an antibacterial activity, and its fermentation products are mainly pyrrolidones and sesquiterpenoids.
Kurthia has a significantly positive correlation with almost all amino acids (P < 0.001). Current research shows that Kurthia can consume glucose to produce lactic acid and can produce ALA, GLU, and GLY through autotrophic pathways (Fang et al., 2015). In addition, Staphylococcus and Enterobacteriaceae also showed a significantly positive correlation with all amino acids, which indicated their important roles in the production and transformation of amino acids in Sufu fermentation. Enterobacteriaceae comprises various genera at the taxonomic level, appearing dominantly in fermented foods, such as doenjang, Sufu, and soy sauce, which is essential for the production of EAAs and volatile compounds (Kim et al., 2009; Devanthi et al., 2018). All amino acids have a clearly positive correlation with Monascus (Figure 3), which proves that Monascus not only provides dyes in the post-fermentation stage but also plays a prominent role during the formation of amino acids. Mucor and Rhizopus are inoculated for pre-fermentation of BS Sufu; the enzymes produced by the two molds can act directly on protein of materials involved in the production of amino acids (Zhang Y. T. et al., 2020). In addition, Bacillus has obviously a positive correlation with organic acids and aldehyde compounds (Figure 3). Bacillus can produce highly active extracellular enzymes, such as protease, amylase, and glucanase. These complex enzyme systems provide a key impetus for the formation of primary and secondary metabolites. So Bacillus can produce unique characteristic flavors during fermentation process, such as spicy, sweet, honey, acetic, aromatic, ethereal, and creamy (Quan et al., 2005). Therefore, Bacillus played a role in the production of volatile compounds during fermentation of BS Sufu. Stenotrophomonas can use nitrogen sources to produce peroxidase and lignolytic enzymes during growth, which can decompose macromolecular substances such as cellulose (Olajuyigbe et al., 2018). The microorganisms that metabolize aromatic amino acids and form ester flavors in the post-fermentation stage mainly include Staphylococcus, Enterococcus, Lactobacillus, and Pseudomonas. Monascus also has a certain correlation with amino acids, esters, alcohols, etc., while Rhizopus and Apiotrichum have a certain positive correlation with acids and ketone compounds.
Some research indicated that the number of genes in glycolysis/gluconeogenesis pathway is the most abundant in fermented foods, followed by citric acid cycle and oxidative phosphorylation (Procopio et al., 2013). In addition, carbohydrate and amino acid metabolism accounted for the highest proportion, followed by lipid and other metabolism. It proved that Enterococcus, Enterobacter, and other microorganisms with high abundance participate in the production of metabolites through different pathways. Although ester compounds have the highest correlation with Enterococcus, the flavor formation of BS Sufu is the result of joint action of all microorganisms in the fermentation system.
Conclusion
In summary, we examined the microbial community structure and flavor compounds of BS Sufu at different fermentation stages by coupling HTS and GC-MS. The microbial community and metabolites in BS Sufu at different fermentation stages are significantly different. The correlation between dominant microorganism and metabolites is extremely complex, which contributes to the taste and flavor of BS Sufu. Our work provides insights into the role of microbial communities in the production of mixed soybean Sufu, and this information is important to develop a new type of Sufu.
Data Availability Statement
The datasets (raw sequences) generated and analyzed during this study has been submitted to NCBI Sequence Read Archive (SRA) repository with accession number PRJNA693985 and can be access through following links: http://www.ncbi.nlm.nih.gov/sra/PRJNA693985.
Author Contributions
DY and LX designed the experiments, performed most of the experiments, and wrote the manuscript. MW and XW performed the statistical analysis. KW and ZL provided valuable advice on experiments design and data analysis tools. DZ was the coordinator and guarantor, overseeing all aspects of this study. All authors read and approved the final manuscript.
Funding
This work was supported by grants from the National Key Research and Development Project (2018YFE0206300), Research Team Project of Natural Science Foundation of Heilongjiang Province (TD2020C003), Advantage and Characteristic of Discipline of Coarse Production and Processing in Heilongjiang Province [Grant No. (2018)4], Heilongjiang Natural Science Foundation Project (LH2019C049), and Innovative Talents Project of Heilongjiang Bayi Agricultural University (No. CXRC2017010).
Conflict of Interest
The authors declare that the research was conducted in the absence of any commercial or financial relationships that could be construed as a potential conflict of interest.
Acknowledgments
The authors are grateful to Majorbio Biotech Co., Ltd. (Shanghai, China) for the support with the bioinformatics analysis.
Supplementary Material
The Supplementary Material for this article can be found online at: https://www.frontiersin.org/articles/10.3389/fmicb.2021.665826/full#supplementary-material
Supplementary Figure 1 | The schematic diagram of BS Sufu production process.
Supplementary Figure 2 | The microbial communities of BS Sufu were analyzed using the Lda effect size algorithm to determine the optimal characteristic taxa and rank them according to the effect size. Lda scores identified the size of differentiation; the score threshold was 4.0. Bacteria: (A,C); Fungi: (B,D).
References
Barka, G. D. (2019). Evaluation of microbial communities in peels of Brazilian tropical fruits by amplicon sequence analysis. Braz. J. Microbiol. 50, 739–748. doi: 10.1007/s42770-019-00088-0
Cheng, S. M., Lan, X., Xu, Y., Wang, D. F., and Ranran, M. (2019). Biogenic amine degrading bacteria within soy sauce fermentation. Food Ferm. Industr. 45, 129–134.
Devanthi, P. V. P., Linforth, R., Onyeaka, H., and Gkatzionis, K. (2018). Effects of co-inoculation and sequential inoculation of Tetragenococcus halophilus and Zygosaccharomyces rouxii on soy sauce fermentation. Food Chem. 240, 1–8. doi: 10.1016/j.foodchem.2017.07.094
Egea, M. B., Pereira-Netto, A. B., Cacho, J., Ferreira, V., and Lopez, R. (2014). Comparative analysis of aroma compounds and sensorial features of strawberry and lemon guavas (Psidium cattleianum Sabine). Food Chem. 164, 272–277. doi: 10.1016/j.foodchem.2014.05.028
Fang, W., Guo, M. W., Ruan, Z. W., Xue, H., Wang, L. F., Tian, G. Z., et al. (2015). Multilocus sequence analysis of the genus Kurthia, and a description of Kurthia populi sp. nov. Int. J. Syst. Evol. Microbiol. 65, 3788–3793. doi: 10.1099/ijsem.0.000494
Feng, Z., Gao, W., Ren, D., Chen, X., and Li, J. J. (2013). Evaluation of bacterial flora during the ripening of Kedong sufu, a typical Chinese traditional bacteria−fermented soybean product. J. Sci. Food Agric. 93, 1471–1478. doi: 10.1002/jsfa.5918
Gefei, L., Yali, Q., Yanjiao, Z., Cong, L., Hongyu, C., Jiahui, S., et al. (2020). Metabolic profiles of carbohydrates in Streptococcus thermophilus during pH-controlled batch fermentation. Front. Microbiol. 11:1131–1141. doi: 10.3389/fmicb.2020.01131
Guiliang, L. T., Min, H., Xue, Y. L., Zi, Q. P., Mei, L., Lin, L., et al. (2020). High-Throughput sequencing and metabolomics reveal differences in bacterial diversity and metabolites between red and white sufu. Front. Microbiol. 11:758. doi: 10.3389/fmicb.2020.00758
Hang, J., Desai, V., Zavaljevski, N., Yang, Y., and Lin, X. J. M. (2014). 16S rRNA gene pyrosequencing of reference and clinical samples and investigation of the temperature stability of microbiome profiles. Microbiome. 2:31. doi: 10.1186/2049-2618-2-31
Hassan, A. E., Tripti, R., and Datta, M. (2010). An alkaline lipase from organic solvent tolerant Acinetobacter sp. EH28: application for ethyl caprylate synthesis. Bioresour. Technol. 101, 3628–3634. doi: 10.1016/j.biortech.2009.12.107
Huang, X., Yu, S., Han, B., and Chen, J. (2018). Bacterial community succession and metabolite changes during sufu fermentation. LWT-Food Sci. Technol. 97, 537–545. doi: 10.1016/j.lwt.2018.07.041
Hui, J., Song, G., and Dan, X. (2017). Arginine deiminase expressed in vivo, driven by human telomerase reverse transcriptase promoter, displays high hepatoma targeting and oncolytic efficiency. Oncotarge. 8, 37694–37704. doi: 10.18632/oncotarget.17032
Ji, X. (2020). Investigation of the volatile components in commercial sufu (Chinese fermented soybean curd) based on HS−SPME/GC−MS combined with multivariate statistical analysis. J. Food Process Preserv. 44, 1–8. doi: 10.1111/jfpp.14309
Jiangang, Y., Chang, S., Xiao, D., Jiaxiu, G., Qi, Z., and Suyi, Z. (2018). Analysis of yeast succession during the fermentation of luzhou-flavor liquor and its effect on the formation of selected flavor components. Food Sci. 39, 166–172. doi: 10.7506/spkx1002-6630-201818026
Jonsdottir, R., Olafsdottir, R., Chanie, E., and Haugen, J. E. J. F. C. (2008). Volatile compounds suitable for rapid detection as quality indicators of cold smoked salmon (Salmo salar). Food Chem. 109, 184–195. doi: 10.1016/j.foodchem.2007.12.006
Kai, Y. D., Xiao, H. H., and Wei, D. B. (2013). Effect of storage conditions on aromatic components of litchi wine. Food Sci. 34, 304–307. doi: 10.7506/spkx1002-6630-201312063
Kim, T. W., Lee, J. H., Kim, S. E., Park, M. H., Chang, H. C., and Kim, H. Y. (2009). Analysis of microbial communities in doenjang, a Korean fermented soybean paste, using nested PCR-denaturing gradient gel electrophoresis. Int. J. Food Microbiol. 131, 265–271. doi: 10.1016/j.j.ijfoodmicro.2009.03.001
Korompokis, K., Östman, E., and Dougkas, A. (2016). The impact of liquid preloads varying in macronutrient content on postprandial kinetics of amino acids relative to appetite. Proc. Nutr. Soc. 75, 511–520. doi: 10.1016/j.appet.2016.08.099
Kumar, T. A., Tripti, K., Anshika, T., Mohd, S., Tayyaba, A., Manoj, K., et al. (2017). Selective phenylalanine to proline substitution for improved antimicrobial and anticancer activities of peptides designed on phenylalanine heptad repeat. Acta Biomater. 57, 170–186. doi: 10.1016/j.actbio.2017.05.007
Li, X. Y., Gao, J., Li, Y. J., Wang, Z. L., Zhang, Z. X., and Sang, Y. X. (2020). Analysis of the relationship between bacterial community composition and changes in organic acids during natural fermentation of mixed fruits. Food Science 41, 61–68. doi: 10.7506/spkx1002-6630-20191015-141
Li, Z., Rui, J., Li, X., Li, J., Dong, L., Huang, Q., et al. (2017). Bacterial community succession and metabolite changes during doubanjiang-meju fermentation, a Chinese traditional fermented broad bean (Vicia faba L.) paste. Food Chem. 218, 534–542. doi: 10.1016/j.foodchem.2016.09.104
Liu, J., Han, B., Deng, S., Sun, S., and Chen, J. (2018). Changes in proteases and chemical compounds in the exterior and interior of sufu, a Chinese fermented soybean food, during manufacture. LWT Food Sci. Technol. 87, 210–216. doi: 10.1016/j.lwt.2017.08.047
Liu, S., and Qiao, J. (2019). bacterial diversity of anshun sufu, a traditional fermented tofu in guizhou province of China. Tra. Tianjin Univers. 25, 497–503. doi: 10.1007/s12209-019-00198-8
Lu, J., Ping, L., Li, Z. Z., and Zhen, M. C. (2014). Changes in volatile flavor compounds in sufu during fermentation. Food Sci. 35, 175–179. doi: 10.7506/spkx1002-6630-201416034
Meng, S., Wang, W., and Longkui, C. (2020). Soluble dietary fibers from black soybean hulls: physical and enzymatic modification, structure, physical properties, and cholesterol binding capacity. J. Food Sci. 85, 1668–1674. doi: 10.1111/1750-3841.15133
Mingxi, S., Gang, C., and Wen, L. (2012). Isolation and structure identification of chemical constituents from marine bacterium Pantoea agglomerans. Chin. J. Medi. Chem. 22, 127–130.
Mondanelli, G., Ugel, S., Grohmann, U., and Bronte, V. (2017). The immune regulation in cancer by the amino acid metabolizing enzymes ARG and IDO. Curr. Opin. Pharmacol. 35, 30–39. doi: 10.1016/j.coph.2017.05.002
Moy, Y. S., and Chou, C. C. (2010). Changes in the contents of sugars and organic acids during the ripening and storage of sufu, a traditional Oriental fermented product of soybean cubes. J. Agric. Food Chem. 58, 12790–12793. doi: 10.1021/jf1033653
Moy, Y. S., Lu, T. J., and Chou, C. C. (2012). Volatile components of the enzyme-ripened sufu, a Chinese traditional fermented product of soy bean. J. Biosci. Bioeng. 113, 196–201. doi: 10.1016/j.jbiosc.2011.09.021
Olajuyigbe, F. M., Fatokun, C. O., and Oyelere, O. M. (2018). Biodelignification of some agro-residues by Stenotrophomonas sp. CFB-09 and enhanced production of ligninolytic enzymes. Bio. Agric. Biotech. 15, 120–130. doi: 10.1016/j.bcab.2018.05.016
Procopio, S., Krause, D., Hofmann, T., and Becker, T. (2013). Significant amino acids in aroma compound profiling during yeast fermentation analyzed by PLS regression. LWT Food Sci. Technol. 51, 423–432. doi: 10.1016/j.lwt.2012.11.022
Qi, Y. M., Sun, J. Y., Chen, H. T., Sun, B. G., and Xu, X. L. (2012). Analysis of volatile Compounds in sauced beef essence prepared by maillard reaction. Food Sci. 33, 199–202.
Quan, C. S., Liu, Q., Tian, W. J., Kikuchi, J., and Fan, S. D. (2005). Biodegradation of an endocrine-disrupting chemical, di-2-ethylhexyl phthalate, by Bacillus subtilis No. 66. Appl. Microbiol. Biotechnol. 66, 702–710. doi: 10.1007/s00253-004-1683-6
Souza, L. C. D., Provensi, G., and Vullo, D. (2017). Carbonic anhydrase activation enhances object recognition memory in mice through phosphorylation of the extracellular signal-regulated kinase in the cortex and the hippocampus. Neuropharmacology 118, 148–156. doi: 10.1016/j.neuropharm.2017.03.009
Tanaka, Y., Watanabe, J., and Mogi, Y. (2012). Monitoring of the microbial communities involved in the soy sauce manufacturing process by PCR-denaturing gradient gel electrophoresis. Food Microbiol. 31, 100–106. doi: 10.1016/j.fm.2012.02.005
Tang, K. X., Shi, T., and Gänzle, M. (2018). Effect of starter cultures on taste-active amino acids and survival of pathogenic Escherichia coli in dry fermented beef sausages. Eur. Food Res. Technol. 244, 2203–2212.
Wan, H., Liu, T., Su, C., Ji, X., Wang, L., Zhao, Y., et al. (2020). Evaluation of bacterial and fungal communities during the fermentation of Baixi sufu, a traditional spicy fermented bean curd. J. Sci. Food Agric. 100, 1448–1457. doi: 10.1002/jsfa.10151
Wan, H. F., Wang, L. P., Zhao, Y., and Li, L. (2020). Analysis of microorganisms in two kinds of Jiajiang sufu based on high-throughput sequencing. J. Chin. Inst. Food Sci.Technol. 20, 278–285. doi: 10.16429/j.1009-7848.2020.08.034
Wu, H. Y., Xie, W. C., Yang, X. H., and Yang, L. (2009). SPME-GC-MS analysis of volatile components in cured golden thread (Nemipterus virgatus) Meat. Food Sci. 30, 278–281.
Xie, C., Zeng, H., Wang, C., Xu, Z., and Qin, L. (2018). Volatile flavour components, microbiota and their correlations in different sufu, a Chinese fermented soybean food. J. Appl. Microbiol. 125, 1761–1773. doi: 10.1111/jam.14078
Xu, Q., Xu, X. Q., and Zheng, G. J. (2019). Amino acid composition and nutritional evaluation of different varieties of sufu. Food Fermen. Sci. Technol. 55, 122–128.
Zhang, Q., Chen, X., Eicher, S. D., Ajuwon, K. M., and Applegate, T. J. (2017). Effect of threonine on secretory immune system using a chicken intestinal ex vivo model with lipopolysaccharide challenge. Poult. Sci. 96, 3043–3051. doi: 10.3382/ps/pex111
Zhang, X. X., Hao, X. Y., Wang, H. X., Li, X. D., Lu, L., and Massounga, B. A. (2020). The effects of Lactobacillus plantarum combined with inulin on the physicochemical properties and sensory acceptance of low-fat Cheddar cheese during ripening. Int. Dairy J. 115, 1–30. doi: 10.1016/j.idairyj.2020.104947
Keywords: BS Sufu, microbial community succession, metabolite, high-throughput DNA sequencing, volatile flavor component
Citation: Yao D, Xu L, Wu M, Wang X, Wang K, Li Z and Zhang D (2021) Microbial Community Succession and Metabolite Changes During Fermentation of BS Sufu, the Fermented Black Soybean Curd by Rhizopus microsporus, Rhizopus oryzae, and Actinomucor elegans. Front. Microbiol. 12:665826. doi: 10.3389/fmicb.2021.665826
Received: 09 February 2021; Accepted: 17 May 2021;
Published: 25 June 2021.
Edited by:
S. R. Joshi, North-Eastern Hill University, IndiaReviewed by:
Qinglong Wu, Baylor College of Medicine, United StatesLi Li, South China University of Technology, China
Copyright © 2021 Yao, Xu, Wu, Wang, Wang, Li and Zhang. This is an open-access article distributed under the terms of the Creative Commons Attribution License (CC BY). The use, distribution or reproduction in other forums is permitted, provided the original author(s) and the copyright owner(s) are credited and that the original publication in this journal is cited, in accordance with accepted academic practice. No use, distribution or reproduction is permitted which does not comply with these terms.
*Correspondence: Dongjie Zhang, YnluZHpkakAxMjYuY29t