- 1Department of Microbiology and Immunology, Faculty of Pharmacy, October University for Modern Sciences and Arts (MSA), Giza, Egypt
- 2Department of Microbiology and Immunology, Faculty of Pharmacy, Cairo University, Cairo, Egypt
The composition and metabolic functions of oral microbiota are affected by many factors including smoking leading to several health problems. Cigarette smoking is associated with changes in oral microbiota composition and function. However, it is not known if the depletion of certain bacterial genera and species is due to specific toxins in cigarette smoke, or indirectly due to competition for colonization with smoking-enriched bacteria. Therefore, the aim of this study was to determine the effect of cigarette smoking on the microbial prevalence and polycyclic aromatic hydrocarbons (PAHs) biodegradation of selected enriched and depleted oral bacteria from oral microbiota of smokers compared to that in non-smokers. Samples of oral rinse from smokers and non-smokers were collected (n = 23, 12 smokers and 11 non-smokers) and screened for oral bacterial strains of Streptococcus mutans, Lactobacillus spp., and Veillonella spp. Comparing counts, S. mutans, V. tobetsuensis, and V. dispar showed higher counts in smokers compared to non-smokers while the Lactobacillus spp. were higher in non-smokers. Lactobacillus fermentum was prevalent in smokers, representing 91.67% of the total Lactobacillus spp. isolates. The biodegradation potential of anthracene; a representative of PAHs of collected isolates, in single and mixed cultures, was assayed with anthracene as the sole source of carbon using 2,6-dichlorophenol indophenol (2,6-DCPIP) as indicator. S. mutans isolates recovered from smokers showed higher degradation of anthracene compared to those recovered from non-smokers. The anaerobic anthracene biodegradation activity of V. parvula isolates from non-smokers was the highest among all isolates of the three recovered genera from the same subject. The anthracene biodegradation potential of Lactobacillus spp. was variable. Combinations of isolated bacteria in co-cultures showed that Lactobacillus spp. interfered with anthracene biodegradation ability along with the viable counts of S. mutans and Veillonella spp. In conclusion, oral dysbiosis due to cigarette smoking was observed not only due to changes in oral bacterial relative abundance but also extended to bacterial functions such as anthracene biodegradation tested in this study. Microbe–microbe interactions changed the anthracene biodegradation potential and growth of the microbial mixture compared to their corresponding single isolates, and these changes differ according to the constituting bacteria.
Introduction
Microorganisms particularly bacteria heavily colonize the human mouth. It has been shown that this microbial community in the human mouth is the second most complex in the body after that of the colon with over 600 different types of microbes present in the human mouth (Dewhirst et al., 2010). The bacterial community of the mouth is dominated by five phyla Firmicutes, Bacteroidetes, Proteobacteria, Actinobacteria and Fusobacteria. Members of these bacterial phyla are involved in a wide variety of functions, and many are important in maintaining oral and systemic health (Dewhirst et al., 2010). Firmicutes constitutes 33.2% of oral microbiota, and it is mainly composed of three major classes, Bacilli, Erysipelotrichia, and Clostridia (Dewhirst et al., 2010). From the Bacilli, Streptococcus genus has the most dominant bacterial species in the oral cavity representing 19.2% of the total oral microbiota (Al-Mudallal et al., 2008). Oral Streptococci produce adhesive molecules allowing them to successfully colonize different sites in the mouth. S. mutans is among the most acidiuric oral Streptococcus species leading to excessive acidification of the oral environment and it is directly associated with the occurrence of dental caries (Abranches et al., 2018). Veillonella genus (Class Clostridia) is gram negative strict anaerobic cocci and it is one of the most common oral bacterial species that represents 8.6% of the total oral microbiota. Veillonella spp. has been reported to play a significant role as early colonizers in biofilm formation and in facilitating the biofilm and dental plaque formation by other species. Members of Lactobacillus (Class Erysipelotrichia) are diverse gram-positive bacilli that are aerotolerant anaerobes. Lactobacillus has been reported as one of the most common oral species linked to dental caries due to their ability to ferment a wide range of carbohydrates and to survive in low pH (Badet and Thebaud, 2008).
Disturbance of oral microbiota are associated with periodontitis and dental caries. Moreover, there is increasing evidence of a role for oral microbiota disturbance in systemic diseases of the lungs, digestive tract and cardiovascular system (Belda-Ferre et al., 2012). There are many factors that lead to oral dysbiosis such as environmental exposures through diet, living conditions and habits that could favor the growth of a particular microbial phyla or genera in the oral cavity. One particular habit is tobacco smoking, as cigarette smoke creates an environment favoring strict or facultative anaerobes. This may result in the loss of beneficial bacterial species leading to pathogen colonization and eventually to disease. Other deleterious effects of cigarette smoke are increased acidity of saliva, alteration in bacterial adherence to mucosal surfaces and impairment of host immunity (Bizzarro et al., 2013; Kato et al., 2016; Yu et al., 2017; Stewart et al., 2018; Vallès et al., 2018; Huang and Shi, 2019). Tobacco smoke is classified as potential carcinogen for oral cavity and pharynx by the International Agency of Research in Cancer (IARC) (IARC, 2020). Tobacco smoke contains over 7,000 different chemicals including polycyclic aromatic hydrocarbons (PAHs) (Talhout et al., 2011).
PAHs are products of incomplete combustion and are known carcinogenic chemicals postulated to play a potential role in cigarette smoking carcinogenesis. There are sixteen PAHs in tobacco smoke classified by the U.S food and drug administration (FDA) as harmful and potentially harmful constituents (HPHCs) (Hecht, 2011; Lawal, 2017), among which anthracene is one of the important priority PAH pollutant. Owing to its simple structure, it is used as a model PAH in different studies to determine the factors affecting the bioavailability, biodegradation and rate of microbial degradation of PAHs in the environment (Karale et al., 2015). PAHs are highly lipophilic, so after human exposure through inhalation or ingestion, their bioavailability is significant and detectable levels of PAHs appear in almost all the human body internal organs (Moorthy et al., 2015). Microorganisms exhibit capabilities for PAHs biodegradation by their metabolic pathways by using these PAHs as carbon sources, they show an ecofriendly behavior to help in PAHs biodegradation by applying two modes of action; the aerobic biodegradation and the anaerobic biodegradation processes (Haritash and Kaushik, 2009).
Dysbiosis in oral microbiota associated with smoking is evident in studies comparing smokers to non-smokers at the phylum level. These studies showed that there is a significant depletion of Proteobacteria, Bacteroidetes and Fusobacteria and enrichment of Firmicutes and Actinobacteria in smokers compared to non-smokers (Wu et al., 2016; Vallès et al., 2018). At the genus level, there is an increased abundance of Streptococcus spp. in current smokers, they are facultative or obligate anaerobes and generally acid tolerant, which may explain their success in the smoking environment. It was also proved that even low concentration of cigarette’s nicotine significantly enhances the growth, biofilm formation and metabolic activities of cariogenic bacteria as S. mutans. (Huang et al., 2012). Additionally, there is an increase in the anaerobic Veillonella genus and Actinobacteria operational taxonomic unit (OTUs). Conversely, aerobes such as Neisseria and Corynebacterium are depleted in smokers. Interestingly, there is a depletion of certain anaerobic OTUs in smokers as well, including V. parvula, Leptotrichia spp., and Peptostreptococcus spp. It is possible that these bacteria were depleted due to specific toxicants in cigarette smoke, or indirectly due to competition for colonization with smoking enriched bacteria (Wu et al., 2016; Vallès et al., 2018). The depletion of certain xenobiotic biodegradation pathways in current smokers suggests important functional losses with potential health consequences, as the oral bacteria are first to come into contact with cigarette smoke as it enters the human body, and may play an important role in degrading the accompanying toxic compounds (Wu et al., 2016).
Although the biodegradation of PAHs by soil bacteria isolated from contaminated sites is well studied but PAHs biodegradation by human microbiota in particular the oral ones is nascent. Moreover, previous studies dealing with human microbiota and cigarette smoking were observational or in silico and did not show if the changes of oral microbial prevalence in smokers is due to direct effects of toxicants in cigarettes or due to indirect effect of oral microbe-microbe interaction. Hence the current study was carried out to understand the effect of anthracene (one of the cigarette toxicant) on selected oral bacteria and to determine the effect of anthracene on degradation potential of these bacteria and their viability either in single or mixed cultures (co-cultures) to determine the effect of microbe-microbe interaction. The selected bacteria were S. mutans, Veillonella spp. and Lactobacillus spp. belonging to the most dominant oral phylum Firmicutes that represents 33% of the total oral microbiota.
Materials and Methods
Participants
Sixteen smokers and fourteen non-smokers were enrolled in this study and provided oral rinse samples after giving their informed consent following the approval of the Research Ethics committees of the Faculty of Pharmacy at October University for Modern Sciences and Arts (MSA) with approval ID (M2/EC2/2016MS) and Faculty of Pharmacy, Cairo University with approval ID MI (1722). Participants recruited in this study were asked to fill in a questionnaire embodying information about smoking status (never/former/current smoker), tobacco consumption, smoking duration, smoking dose. All participants were chosen to be >18 years of age, and all participants with the following criteria were excluded: (1) recent antibiotic treatment or steroidal drug use (<2 weeks); (2) clinical signs of periodontal disease; (3) underlying chronic or systemic disease; (4) smoking of e-cigarettes, water pipes and all other smoking tools other than cigarettes; (5) ceased smoking; (6) pregnant or breast feeding for female participants (Ghannoum et al., 2010; Gaudet et al., 2013; Vallès et al., 2018). Smoker subjects were smoking ≥ 5 cigarettes/day for at least 1 year till time of sample collection and they were further classified according to the number of daily cigarettes consumption into light, intermediate and heavy smokers for those who consume <10, 10–20, and >20 cigarettes/day, respectively (Lohse et al., 2016).
Standard Bacterial Strains
Standard bacterial strains used in the study were Veillonella parvula ATCC 10790, V. dispar ATCC 17748, S. mutans ATCC 25175, Lactobacillus salivarius ATCC 11741, and Bacillus subtilus ATCC 6051.
Collection of Oral Rinse
For oral rinse samples’ collection, each participant was asked to refrain from eating and drinking for one hour prior to sample collection, rinse mouth for 60 s with 10 ml sterile saline, then spill saline wash into 50 ml sterile tube to be immediately transferred on ice for processing in the microbiology laboratory following the procedure of Vallès et al. (2018).
Enumeration and Isolation of S. mutans, Lactobacillus spp., and Veillonella spp. From Concentrated Oral Rinse
Each oral rinse sample was then centrifuged at 1,900×g for 10 min, the supernatant was discarded and the pellets were resuspended in 2.5 ml saline to produce a concentrated homogenous suspension (Latimer et al., 2015). Bacterial suspensions were diluted 10-fold serial dilutions and aliquots were plated on custom-made media: Tryptone Yeast Extract Cystine with Sucrose and Bacitracin (TYCSB) (Wan et al., 2002), Lactobacillus Selective agar (LBS) (Coeuret et al., 2003) and Veillonella selective agar medium (Djais et al., 2017) for isolation of S. mutans, Lactobacillus spp., and Veillonella spp., respectively. Bacteria were incubated anaerobically at 37°C for 48 h for the enumeration of S. mutans (Al-Mudallal et al., 2008) and Lactobacillus spp., while the slowly growing Veillonella spp. required incubation for 72 h (Igarashi et al., 2009). After anaerobic incubation, colonies with characteristic appearance of each bacteria were counted and selected for further identification by Gram staining, followed by molecular identification for each species. Isolates were preserved in glycerol stock at −20°C.
Biochemical and Molecular Identification of Bacterial Isolates
We combined multiple molecular and biochemical approaches to identify the genus and species to give more credibility to species-level identification, which usually cannot be achieved by sequencing the SSU rRNA alone. Isolated bacteria were categorized into Gram positive or negative after staining by Gram stain and examination under microscope. S. mutans was biochemically identified by catalase production, dextran production, sugar fermentation and tolerance to high concentration of sodium chloride. Colonies growing on selective media for S. mutans and Veillonella spp. were grown in brain heart infusion (BHI) broth while, those for Lactobacillus spp. were cultured in De Man, Rogosa and Sharpe (MRS) broth for 48 h under anaerobic conditions at 37°C. Genomic DNA extraction was done using GeneJET Genomic DNA Purification kit (Thermo Fisher Scientific Surrey, United Kingdom) according to the instruction of manufacturer.
Lactobacillus were identified by amplification and sequencing of the 16s rRNA gene following the method of Weisburg et al. (1991) at the Macrogen sequencing facility (Macrogen, South Korea).
Identification of oral S. mutans was confirmed by the PCR amplification of the glucosyl transferase (gtfB) gene responsible for the production of extracellular glucans that play a major role in dental plaque following the method of Oho et al. (2000).
The colonies showing typical morphology on Veillonella selective agar were first identified at the genus level using the method of Arif et al. (2008). Species of oral Veillonella were identified using species-specific primers following the method of Mashima et al. (2016). All primers used in this study were synthesized by Invitrogen (Surrey, United Kingdom) and are listed in Table 1.
The thermal cycler used was Biometra thermal cycler (Hamburg, Germany). PCR products were detected by agarose gel electrophoresis (1.5% w/v) using Tris Acetate EDTA (TAE) as the running buffer and gel loading buffer (Pelt-Verkuil et al., 2008).
Determination of Anthracene Biodegradation Ability of Bacterial Isolates by 2, 6-Dichlorophenol Indophenol (2, 6-DCPIP) Colorimetric Assay
S. mutans and Veillonella spp. were grown in brain heart infusion (BHI) broth while, Lactobacillus spp. was cultured in MRS broth for 48 h under anaerobic conditions at 37°C. The cultures were then centrifuged at 1,900×g for 10 min and the supernatant was discarded. The pellet was washed twice with 0.9% sterile saline to remove any traces of the medium and resuspended in 0.9% sterile saline to produce a culture with turbidity equivalent to OD of 1 at 600 nm which corresponds approximately to the turbidity of a homogenous suspension of bacteria of 5 × 109cfu/ml (Karale et al., 2015).
The ability of the isolates to utilize anthracene substrate can be measured by observing the disappearance of the blue color of the 2,6-DCPIP with time (Karale et al., 2015). The 2,6-DCPIP assay was carried out according to the method of Chowdhury et al. (2017) with modifications. The assay was carried out to evaluate the capability of single or combined isolates for the biodegradation of anthracene. Assay was done on individual bacterial isolates from smokers and non-smokers and also in three replicates of the respective standard strains including: S. mutans, V. parvula, V. dispar, and L. salivarius, also different combinations of bacterial isolates including different co-cultures of duos mixtures (1:1 v/v) of S. mutans and Veillonella spp., S. mutans and Lactobacillus spp., Lactobacillus spp., and Veillonella spp. and triples mixtures (1:1:1 v/v/v) of S. mutans, Veillonella spp. and Lactobacillus spp. The capability of the whole oral rinse (consortium) from selected smokers (S 11 and 12) and non-smokers participants (NS 10 and 11) to degrade anthracene was also tested.
A volume of 2.4 ml bacterial suspension (O.D.1 at 600 nm) was used to inoculate the assay mixture containing 22.5 ml Fe free W medium, 1.5 ml FeCl3 6.H2O solution (150 μg/l), 1.5 ml of 2,6-DCPIP solution (50 μg/l) followed by addition of 0.25 ml of anthracene (1 mg/ml in dichloromethane). The reaction mixture was anaerobically incubated at 37°C for 8 days. Anthracene biodegradation ability was indicated by change in the color of the medium from blue to colorless (Karale et al., 2015). Three controls were simultaneously run: a positive control with B. subtilus (one of the highest anthracene biodegrader microorganisms), a negative control without bacterial suspension and another negative control without anthracene. Negative controls were done to assure that there is no significant spontaneous reduction in 2, 6-DCPIP color.
Aliquots from the assay mixture were pipetted at 0, 1, 2, 7, and 8 days over a period of 8 days of incubation. Aliquots were centrifuged at 7,700×g for 15 min to avoid the interference between bacterial growth turbidity and indicator’s color. The anthracene biodegradation was estimated by measuring the change in absorbance of the DCPIP indicator. Absorbance readings were converted to concentrations using a standard curve. A standard curve for DCPIP was constructed using different concentrations from 244X10–8 (Absorbance = 0) to 5X10–3 g/L (Absorbance = 2.55), with correlation coefficient (r = 0.9984). The equation Y = 501×−0.0071 was obtained from the curve, y represents the OD of DCPIP of each × indicates the corresponding concentration in mg/ml (Supplementary Figure 1). The percentage of remaining DCPIP color intensity was calculated by comparing the change in color intensity after a given time with the initial value based on Chowdhury et al., 2017 equation:
The growth of the bacteria was monitored during the assay by drop plate technique on selective medium for each genus (Esteban Florez et al., 2016).
Statistical Analysis
Statistical analysis was performed using GraphPad Prism 5.01 (GraphPad software Inc., CA, United States). The results were presented as median and error ± interquartile range. Kruskal Wallis and multiple comparisons using Dunn’s corrections were used to compare the prevalence of S. mutans, Lactobacillus spp., and Veillonella spp. in smokers and non-smokers subjects and viable counts of the three microorganisms in anthracene biodegradation study where p < 0.05 was considered to be statistically significant. Data of the anthracene biodegradation by the three microorganisms and their mixtures were statistically analyzed by Mann-Whitney U-test and multiple comparisons using Holm Sidak’s corrections where p < 0.05 was also considered to be statistically significant.
Results
Demographic Data on Study’s Participants
Applying selection criteria mentioned in materials and methods section, 27 from the two genders out of the 30 participants were found eligible for including and handling their oral rinses’ samples in the study and 3 samples were excluded due to local oral disease (severe periodontitis) or systemic disease (diabetes). The average ages of smoker and non-smoker participants was 21–40 and 25–30 years old, respectively, and the average duration of smoking was 1–15 years. The average cigarette’s consumption was 6–60 cigarettes/day (Supplementary Table 1). The fourteen smoker participants were divided according to cigarettes consumption into heavy smokers (n = 5), intermediate smokers (n = 7) and light smokers (n = 2). One of the limitations of the current study was the small sample size, but it was appropriate for proper statistical testing.
Prevalence of S. mutans, Lactobacillus spp., and Veillonella spp. in Smokers and Non-smokers Groups
From the 27 samples, 4 were neglected due to failure in isolation of the three required bacterial genus from the same participant and a total of 69 bacterial strains were isolated from 23 oral rinses. Isolates recovered on selective media were preliminary identified by microscopic examination revealing Gram positive cocci bacteria with chain arrangement, Gram negative cocci or diplococci cells arranged in masses or short chains and Gram-positive rod-shaped cells for S. mutans, Veillonella spp. and Lactobacillus spp., respectively. Biochemically, isolates of S. mutans were identified by being devoid of catalase enzyme, tolerant to 4% NaCl, their ability to produce dextran and to ferment sugars (namely: mannitol, sorbitol, sucrose, and inulin). Further molecular identification was carried out for confirmation. Molecular detection of gtfB gene in colonies growing on TYCSB confirmed their identification as S. mutans with 100% prevalence in samples obtained from all smoker and non-smokers groups (Supplementary Figure 2). Sequencing of the 16s rRNA gene of colonies growing on Lactobacillus Selective agar (LBS) was used to identify various species, and according to the similarity score defined previously by Sabat et al. (2017), bacteria were assigned to a species or genus with similarity score between 90 and 99% (Table 2). Sequencing of the 16s rRNA gene revealed that L. fermentum was the prevalent species in smokers 11/12 (91.67%) while in non-smokers it represented 2/11(18.18%) of samples (Figure 1). However, in the non-smokers participants, L. salivarius was detected in 36.6 % of samples. V. parvula was the prevalent strain (100%) in non-smokers samples, while V. tobetsuensis was detected in 10/12 (83.3%) of smokers’ samples (Figure 1 and Supplementary Figure 3).
Enumeration of S. mutans, Lactobacillus spp., and Veillonella spp. in Smokers and Non-smokers Groups
Results showed that S. mutans was the most prevalent bacteria in smokers’ samples, followed by Veillonella spp., then Lactobacillus spp. A significant increase (P < 0.001) between the viable counts of Veillonella spp. in smokers compared to non-smokers participants was detected. Among smokers, S. mutans showed higher viable counts than Veillonella spp. and Lactobacillus spp., respectively, while in non-smokers S. mutans viable counts was higher than Lactobacillus spp. and Veillonella spp., respectively (Figure 2).
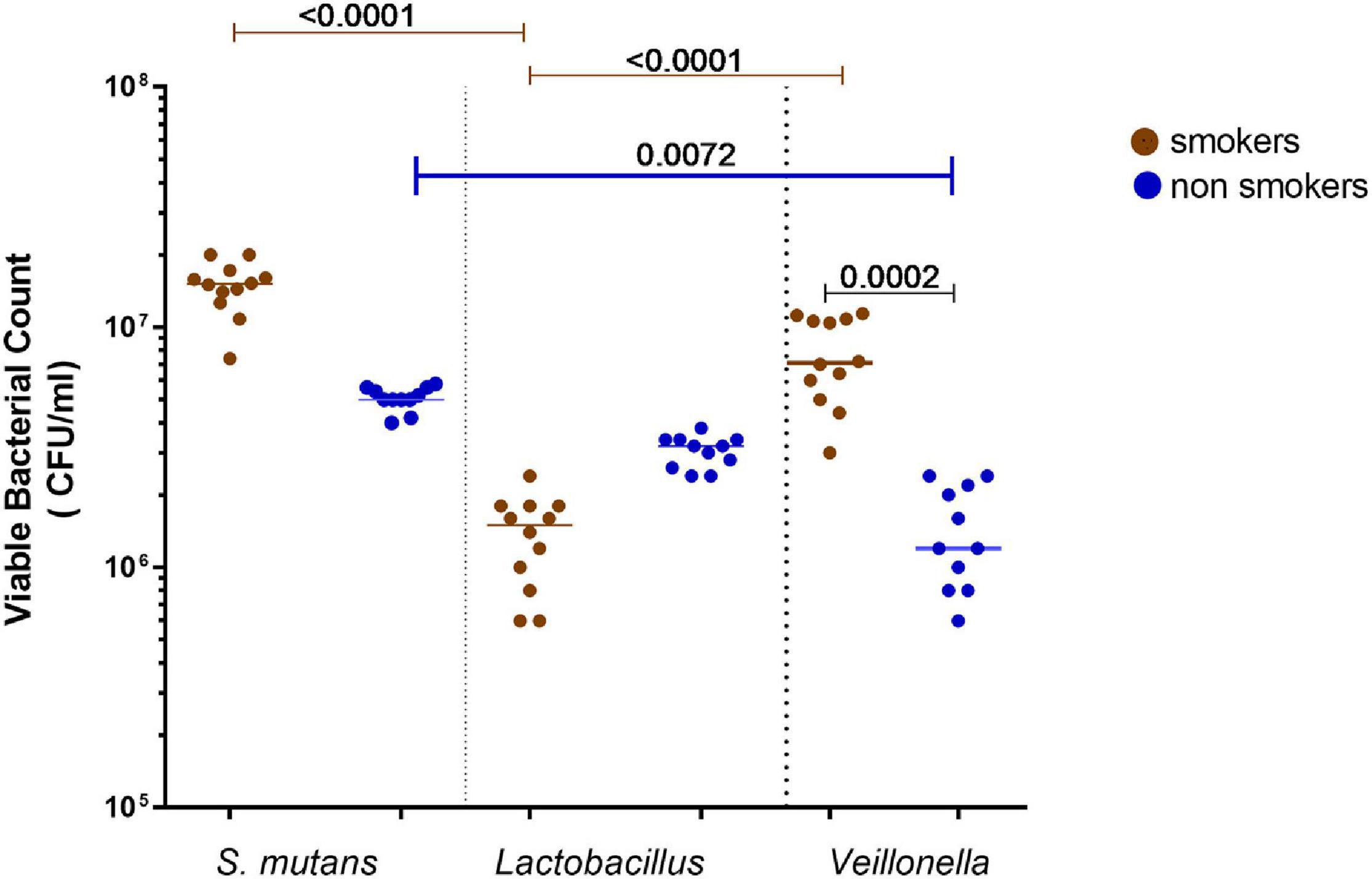
Figure 2. Total viable bacterial counts of isolated identified Streptococcus mutans, Lactobacillus spp., and Veillonella spp. from oral rinse of smokers (n = 12) and non- smokers (n = 11). Statistical differences calculated using Kruskal-Wallis followed by multiple comparisons using Dunn’s corrections. Data presented as median ± interquartile range, where p < 0.05 was considered to be statistically significant.
Determination of Anthracene Biodegradation by Oral Bacterial Isolates From Smokers and Non-smokers Measured by 2, 6-Dichlorophenol Indophenol (DCPIP) Assay
The results of preliminary estimation of anthracene biodegradation was determined by the ability of the bacterial isolates to reduce the blue color of DCPIP indicator in a period of 8 days. V. parvula being a highly reducer of blue color of DCPIP indicator after 8 days thus possessed the highest potential of anthracene biodegradation (Figure 3).
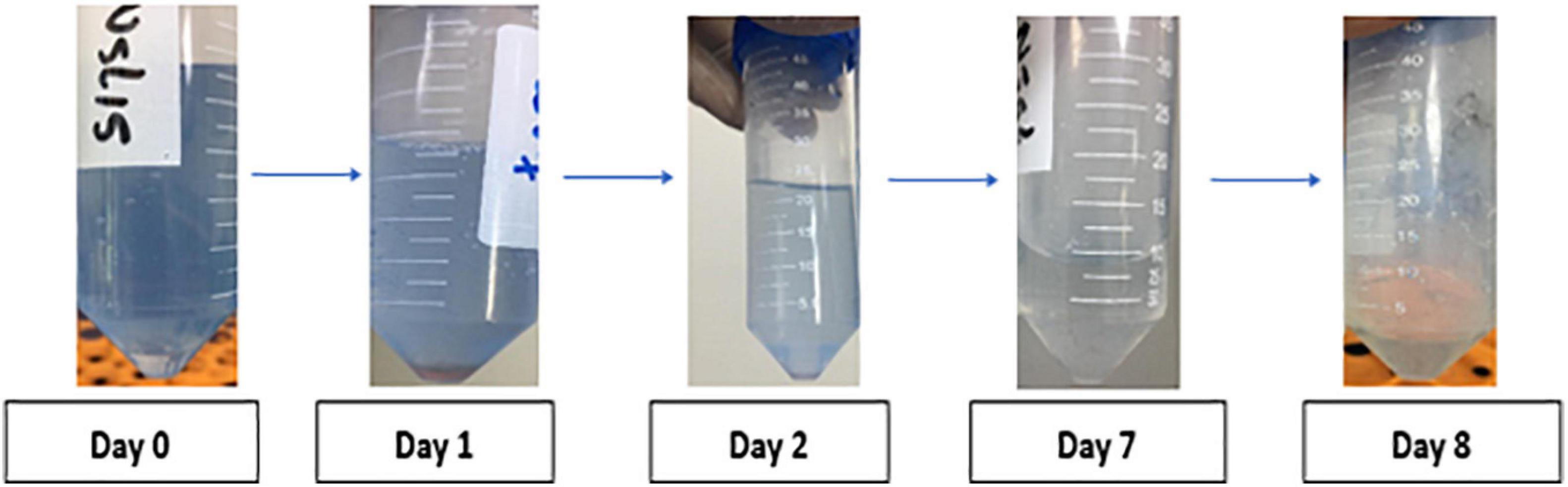
Figure 3. DCPIP assay of a representative smoker sample showing dye discoloration. during anthracene biodegradation over a period of 8 days in a representative smoker sample.
Culture dependent method of isolation made it impossible in some cases to compare results of anthracene degradation by the same species isolated from smokers and non-smoker group. This was mainly due to the fact that not all the species were isolated from both groups mainly due to depletion of some species in one group than the other. Results of anthracene biodegradation by individual bacterial isolates from smokers and non-smokers using 2,6-DCPIP colorimetric assay, compared to relevant standard strains showed that S. mutans isolated from smokers had significantly higher anthracene biodegradation ability than that from non-smokers and S. mutans ATCC 25175 at days 7 and 8 (p < 0.0001) (Supplementary Figure 4A). Anthracene biodegradation of V. parvula isolated from non-smokers was significantly higher than Veillonella spp. isolated from smokers, starting from day 1 to the end of the assay at day 8 (p < 0.0001). Moreover, V. parvula isolated from non-smokers showed significant differences in anthracene biodegradation than V. parvula ATCC 10790 and V. dispar ATCC 17748 (p < 0.0001) except at days 7 and 8 where no significant difference between V. parvula from non-smokers and V. parvula ATCC 10790 (Supplementary Figure 4B). For Lactobacillus spp. isolated from smokers and non-smokers, the results showed that at day 1, the anthracene biodegradation ability of smoker’s isolates was significantly higher (p = 0.0384) than non-smokers isolates, while at day 8, the ability of non-smokers isolates to degrade anthracene was significantly higher than isolates from smokers and L. salivarius ATCC 11741 (p = 0.0062) (Supplementary Figure 4C).
From previous results, the differences in anthracene biodegradation capability of bacterial species isolated from smokers and non-smokers was significant at the 8th day of reaction. Therefore, next comparison of data was represented as DCPIP color intensity % after 8 days of incubation using the 2, 6- DCPIP assay. V. parvula of non-smokers showed relatively higher anthracene biodegradation (median = 66.1%) than other isolates, while V. dispar of smokers (Figures 4A,C) showed relatively low ability to degrade anthracene (median = 44%) (Figure 4C). Non-smokers isolates of S. mutans (median = 44%) showed lower anthracene biodegradation ability than V. parvula, L. gasseri, L. salivarius, L. rhamnosus, and L. plantarum. For smokers’ subjects, the degradation potential of anthracene was 58, 53.7% for L. plantarum and L. fermentum, respectively, which is higher than S. mutans (median = 54.25%). Results of comparison of anthracene degradation by 2,6-DCPIP assay by individual bacterial isolates from smokers and non-smokers showed that S. mutans isolated from smokers (median = 54.25%) had higher anthracene degradation ability than that from non-smokers (Figure 4A). Lactobacillus spp. isolated from non-smokers including L. gasseri, L. salivarius, and L. plantarum, showed higher anthracene degradation potential than L. fermentum isolated from smokers’ samples (Figure 4B).
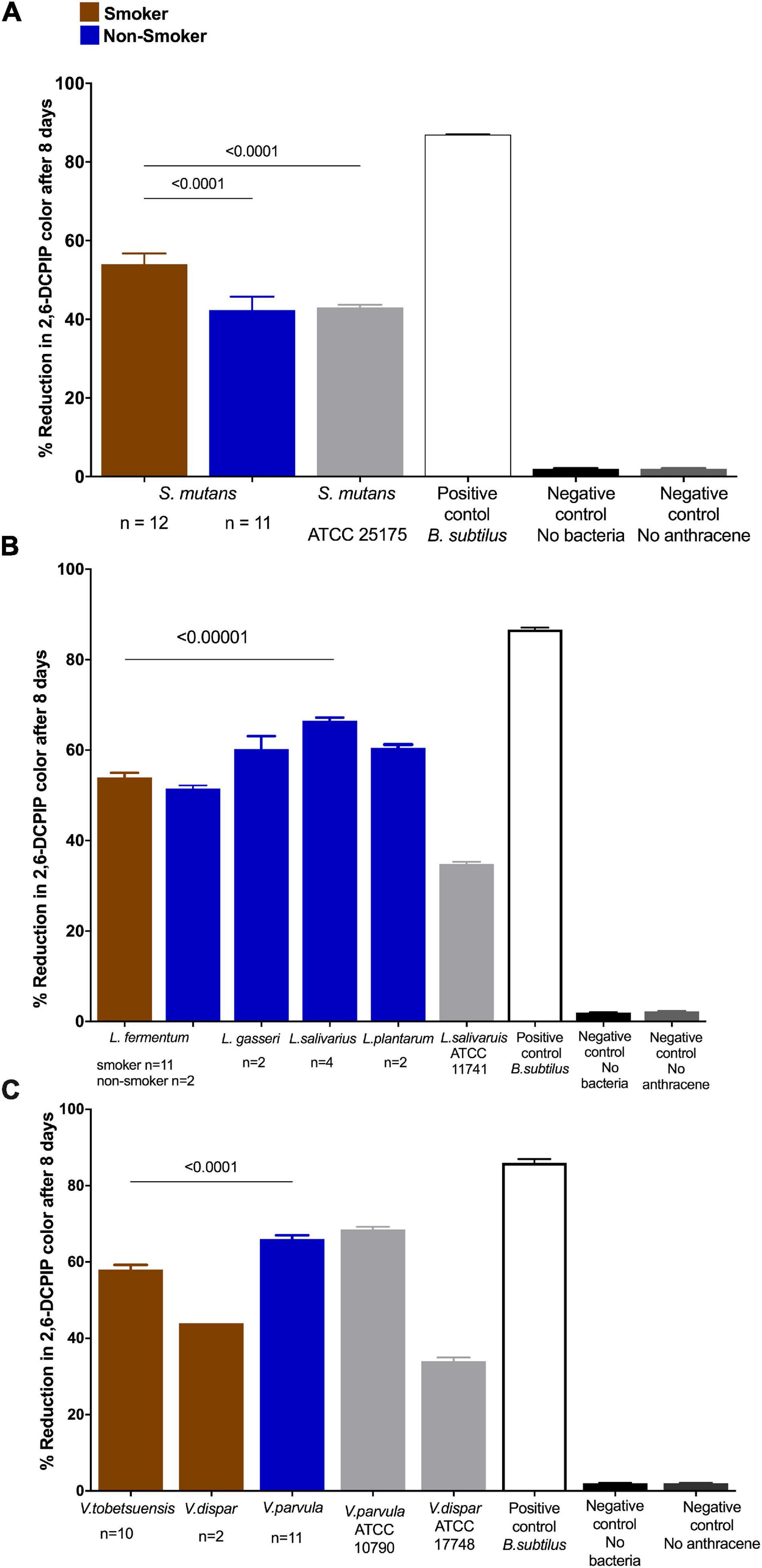
Figure 4. Anthracene biodegradation after 8 days in smokers and non-smokers compared to standard strains, positive and negative controls. Biodegradation of anthracene by (A) S. mutans; (B) Lactobacillus spp.; and (C) Veillonella spp. represented as color intensity (%) of DCPIP. Statistical differences calculated using Mann-Whitney U-test followed by multiple comparisons using Holm Sidak’s corrections. Data presented as median ± interquartile range, where p < 0.05 was considered to be statistically significant. Bacillus subtilis (positive control one of the highest anthracene biodegrading microorganisms) and negative controls (no anthracene or no bacteria) were significantly different from the isolated species and ATCC strains. ****p < 0.00001.
Next, we sought to assess the growth and anthracene degradation by various bacteria isolated from non-smokers and smokers. These bacteria were different as for instance, V. parvula was only isolated from non-smokers while it was depleted in smokers according to our results and in accordance with previous studies (Dalié et al., 2010; Wu et al., 2016). Changes in isolates viable counts during anthracene biodegradation were measured during a time of incubation of 10 days in a representative smoker participant and another from non-smoker participant to check the pattern of bacterial growth during the use of anthracene as a sole source of carbon and to confirm that anthracene biodegradation is reflected by an increase in bacterial viability. Acclimatized S. mutans with higher anthracene degradation showed higher growth than non-acclimatized S. mutans isolated from non-smokers (Figures 5A,B). L. salivarius showed higher level than L. fermentum in anthracene biodegradation potential which is reflected as higher viable bacterial counts (Figures 5C,D). V. parvula isolated from non-smoker participant showed higher degradation than V. tobetsuensis in the time period corresponding to lag and log phase of bacterial growth but after 8 days the degradation was nearly equivalent to each other. V. parvula showed higher viable counts than V. tobetusensis in the stationary phase (Figures 5E,F).
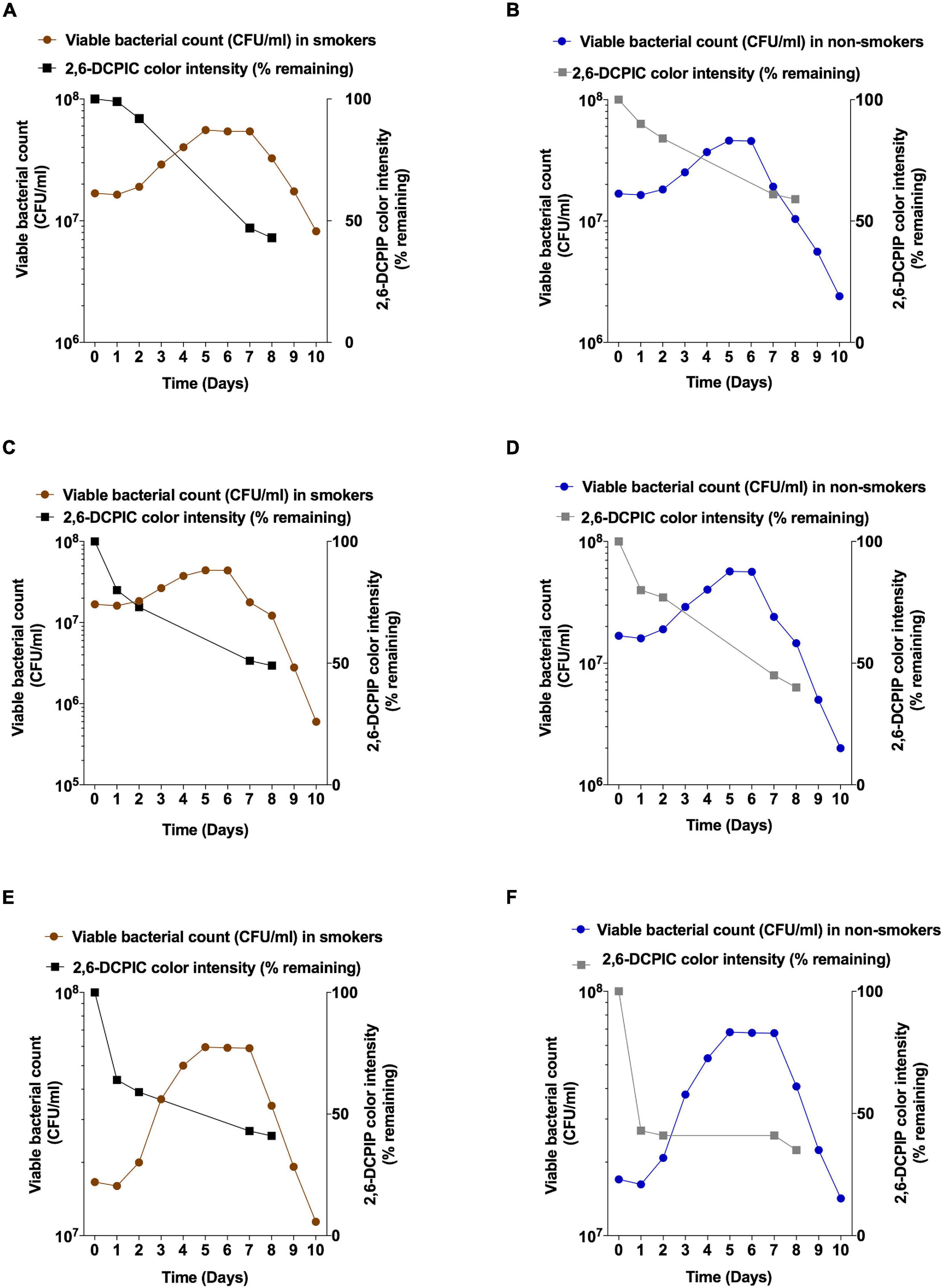
Figure 5. Viable counts and anthracene biodegradation by selected bacteria isolated from representative smoker and non-smoker participant over a period of 10 days. S. mutans from (A) smokers and (B) non-smokers (C) L. fermentum from smoker; (D) L. salivarius from non-smoker; (E) V. tobetsuensis from smoker; (F) V. parvula from non-smoker.
Effect of Microbe-Microbe Interaction on Anthracene Biodegradation and Viable Bacterial Counts During DCPIP Assay
Co-cultures of duo and triple mixtures containing V. dispar showed lower biodegradation potential compared to other mixtures (Figure 6A). Duo mixtures containing L. salivarius with either S. mutans or Veillonella spp. showed lower biodegradation potential than mixtures containing S. mutans and Veillonella spp. in both smokers and non-smokers groups (Figures 6A,B and Supplementary Figures 5A–C). In triple mixtures, it was found that no viable cells were detected for Lactobacillus spp. after 8 days of incubation in presence of anthracene as sole source of carbon, and on the other hand Veillonella spp. viable counts were increased significantly (P < 0.001) after 8 days of incubation under the same conditions (Figures 7A,B). In consortium assay, the results showed that there was a significant increase in anthracene biodegradation ability by rinses from smokers compared to non-smokers starting from day 1 to the end of the assay at day 8 (Figure 6C and Supplementary Figure 5E).
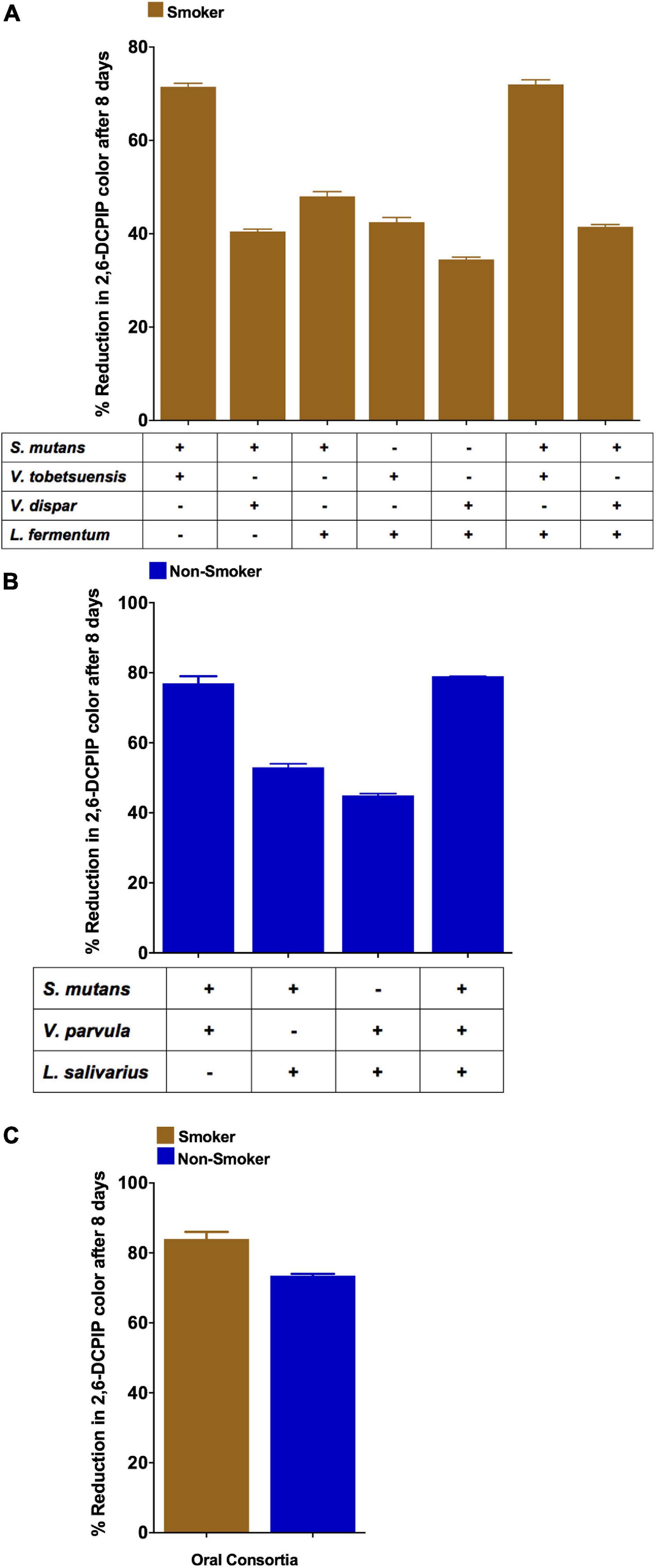
Figure 6. Anthracene biodegradation represented as DCPIP color intensity % by S. mutans, Lactobacillus spp. and Veillonella spp. mixtures after 8 days in smokers and non-smokers. (A) Duos and triples mixtures from smokers; (B) Duos and triples mixtures from non-smokers; (C) Consortium from representative smokers and non-smokers.
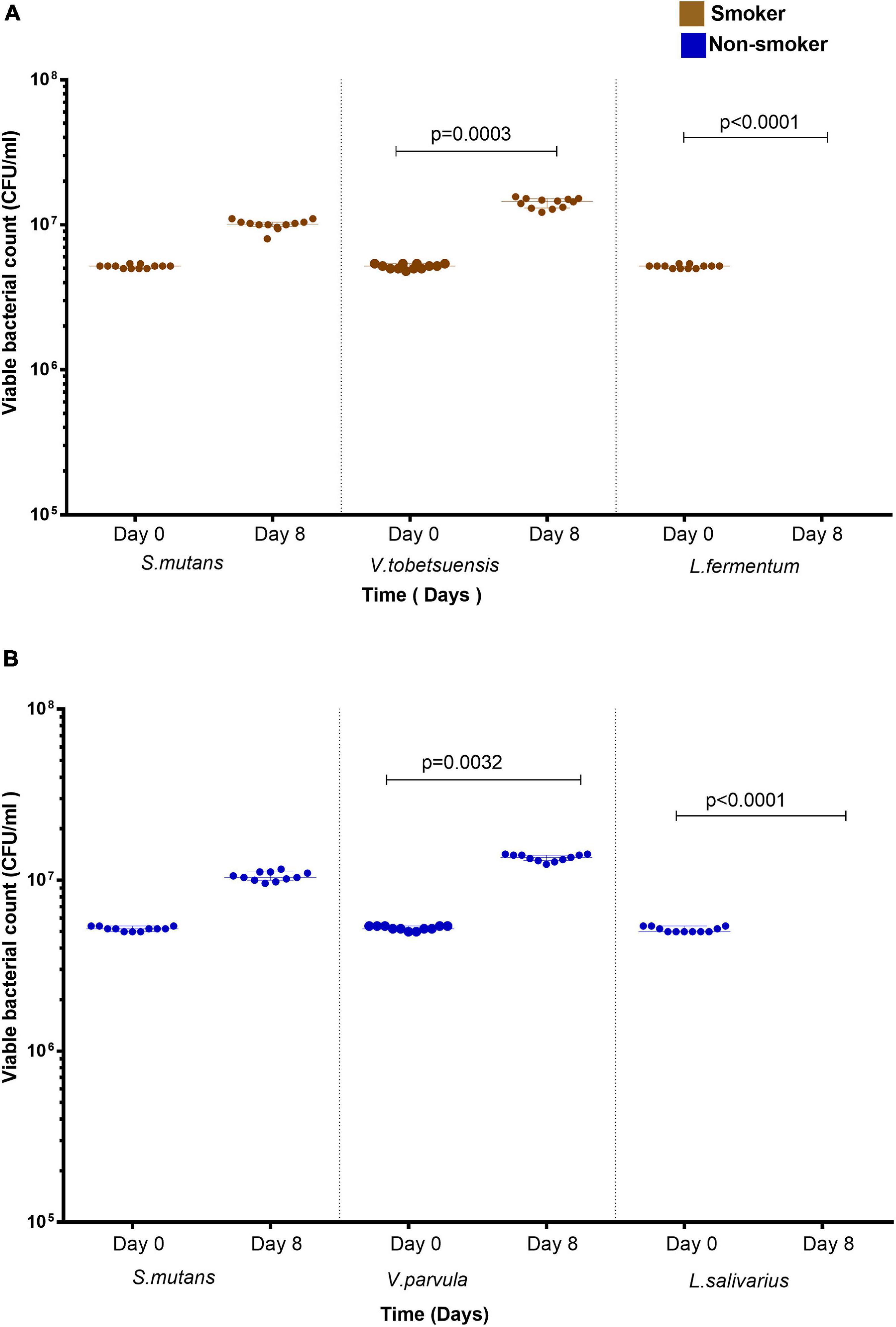
Figure 7. Viable bacterial count of S. mutans, Lactobacillus spp., and Veillonella spp. triple assay in presence of anthracene as a sole carbon source at day 0 and day 8. (A) smokers and (B) non-smokers. For Lactobacillus spp., no colonies was detected at day 8. Statistical differences calculated using Kruskal-Wallis followed by multiple comparisons using Dunn’s corrections. Data presented as median ± interquartile range, where p < 0.05 was considered to be statistically significant.
Discussion
Many factors such as smoking may affect both the composition and the function of oral microbiota. Cigarette smoke is one of the causes of dysbiosis, i.e. microbial imbalance or maladaptation of oral microbiota. This alteration in microbial profile by cigarette smoke could be attributed to different factors including direct contact with extensive microbes in the cigarette itself leading to acquisition and colonization by pathogenic bacteria. The oral microbial ecology may be also altered by cigarette smoke due to the direct effect with its released xenobiotics and toxicants on oral microbiota (Talhout et al., 2011). Other deleterious effects of cigarette smoking include suppression of immunity, increase of bacterial biofilm formation, depletion of local oxygen, decrease of salivary flow rate as well as lowering of pH of the oral cavity (Huang and Shi, 2019).
Cigarette smokers inhale many toxicants including more than 500 different polycyclic aromatic hydrocarbons (PAHs) compounds being identified in cigarette smoke (Hecht, 2011; Lawal, 2017). Polycyclic aromatic hydrocarbons, including anthracene, are classified as a major class of environmental genotoxins (Lawal, 2017). Screening of literature indicated a shortage of study and outcomes for the biodegradation of anthracene as an important member of PAHs by oral isolates. Therefore, this study can be considered innovative for comparing anthracene biodegradation by oral bacterial isolates from smokers and non-smokers, namely S. mutans, Veillonella spp., and Lactobacillus spp. Wu et al. (2016) suggested that cigarette smoking may affect the capability of oral microbiota to degrade PAHs, where abundances of PAHs biodegradation pathways were significantly altered in current smokers compared to never smokers. Therefore, the current study aimed to understand the mechanism by which selected bacterial members of oral microbiota are affected by exposure to cigarette smoking. The selected bacteria were S. mutans, Veillonella spp., and Lactobacillus spp. belonging to the most dominant oral phylum Firmicutes that represents 33% of the total oral microbiota. The selected bacteria were studied in regard to their counts and their ability to degrade one of the smoking hazardous compounds namely PAHs represented by anthracene using culture-dependent approach. Cigarette smoking was found to alter the relative abundance of the studied bacteria.
Enumeration of characteristic colonies of each genus on its selective medium revealed an increase in the prevalence of S. mutans in smokers compared to non-smokers. Such result as published by Wu et al. (2019), assumed a close relationship between the components of cigarettes particularly nicotine and the growth of cariogenic bacteria such as S. mutans. Cigarette smoking has been associated with an increased prevalence of dental caries where heavy smokers have significantly higher number of decayed, missing and filled teeth (DMFT) (Vellappally et al., 2007). S. mutans contributes to caries formation through acid production which damage dental enamel. The ability of S. mutans to synthesize the exopolysaccharides glucan and fructan by the glucosyl transferase (gtfs) and fructosyl transferases (ftf) genes, respectively, enables the bacteria-teeth binding and hence plaque formation (Wasfi et al., 2018). It was proved that nicotine significantly enhances biofilm formation and metabolic activities of S. mutans even at low concentration (Huang et al., 2012). In our study, S. mutans adapted to cigarette toxicant, as anthracene, isolated from smokers oral rinse showed higher biodegradation potential than those isolated from non-smokers and that was reflected in higher prevalence of this species in smoker samples. The reason behind the significant anthracene biodegradation potential of S. mutans in smokers compared to non-smokers could be explained with the theory of microbial adaption to xenobiotics. S. mutans isolated from anthracene polluted environment were found to be among high anthracene degradation genera (Karale et al., 2015). Microorganisms could adapt to the presence of xenobiotics by different mechanisms including :random mutations, increasing the resistance to toxic xenobiotics, mutations that enhance the ability of bacteria to degrade xenobiotics, acquiring genes coding for catabolic enzymes from other microorganisms by plasmids, transposons, integrons or phages (Haiser and Turnbaugh, 2013). All the previously mentioned mechanisms could explain the reason for the high abundance of S. mutans in smokers compared to non-smokers.
Concerning the genus Lactobacillus, no significant difference was detected between its salivary counts in smokers and non-smokers in agreement with a study done by Wu et al. (2016), but at the species level prevalence was significantly different. The prevalence of salivary L. fermentum was higher in smokers comprising about 92% of collected isolates compared to non-smokers (18.1%). In contrast to the high anthracene biodegradation pattern of S. mutans isolated from smokers, a substantial increase in biodegradation was observed with isolates from non-smokers’ samples rather than smokers’ ones. The low prevalence of high biodegrader Lactobacillus spp. in smokers may results from the rapid saturation of the enzymes responsible for anthracene biodegradation by anthracene itself in isolates recovered from smokers as mentioned by Wu et al. (2016). Therefore, the high prevalence of L. fermentum in smokers could be explained by the presence of a fully operational glutathione producing system in this species that removes reactive oxygen species (ROS) from the oral environment thus protecting the bacteria from the oxidative effect of cigarette smoking (Sardaro et al., 2019). The glutathione system allows this species to directly detoxify and eliminate ROS as hydrogen peroxide, hydroxyl radicals and lipid peroxyl radicals giving it a maximum protection against oxidative stress in smokers’ oral environment. The variation in the ability of different Lactobacillus spp. to reduce PAHs among different species as seen in the current study is conforming with the results of Yousefi et al. (2018). Different lactic acid producing bacteria have the ability to reduce xenobiotics and PAHs by two different mechanisms. The first one is by detoxification to less toxic metabolites which occurs when the presence of PAHs induces the production of aromatic ring deoxygenase enzyme known as one of the most common PAHs degrader enzymes (Abou-Arab et al., 2015). The second mechanism is by adsorption of these toxicants on the cell wall through physical interactions. It has been reported that the anionic functional groups present in the peptidoglycan layer, teichoic acids and teichuronic acids of gram positive bacteria are responsible for the bacterial toxic compound’s adsorption capacity (Yousefi et al., 2018) and that the proteins and carbohydrates present in the cell wall of lactic acid bacteria have an important role in toxins adsorption (Dalié et al., 2010).
As for the genus Veillonella, the study indicated a significant abundance of this genus in smokers compared to non-smokers, which is a result also verified by Wu et al. (2016) and Vallès et al. (2018). This finding suggests that cigarette smoking might have an impact on the physiology of the oral cavity creating an environment that favors the growth of strict anaerobic bacteria such as Veillonella spp. This is not surprising because one of the potential mechanisms by which cigarette smoking alters the oral microbial ecology is by oxygen depletion (Kilian et al., 2016; Yu et al., 2017; Huang and Shi, 2019). PAHs biodegradation by Veillonella spp. was not previously studied, but Dixit (2015) has mentioned Veillonella among the major anaerobic bacterial genera that are capable of degrading xenobiotics compounds. Gibson and Harwood (2002) reported that aromatic compounds such as PAHs can serve as electron acceptors to allow the anaerobic bacteria to transfer electrons to these compounds during anaerobic biodegradation process.
Identification of Veillonella to the species level showed that there was a significant increase in prevalence of V. dispar in smokers compared to non-smokers in accordance with the study of Moon et al. (2015) who found that V. dispar is one of the seven species occupying > 1% of the whole oral subgingival microbiota of smokers. PAHs biodegradation potential was not correlated with the ability of the Veillonella spp. to tolerate smoking stress in oral environment and this was concluded from the prevalence pattern of different Veillonella spp. in smokers and non-smokers samples. Comparing the biodegradation capability of Veillonella spp. isolates, a significant high anthracene biodegradation ability by V. parvula from non-smokers’ samples as compared to V. tobetsuensis and V. dispar isolated from smokers. The high PAH biodegradation potential by V. parvula may be the reason for the depletion of this species in smokers by rapid saturation of the degradation enzymes with toxic PAH leading to its death. It is also possible that the depletion of V. parvula is directly due to specific toxicant in cigarette smoke (Wu et al., 2016). A transcriptomic study of V. dispar and V. parvula, isolated from oral samples, showed a significant up-regulation of V. dispar genes which encode for oxidoreductase enzymes involved in the use of NAD+ and NADP+ as electron acceptors when compared to V. parvula, this might explain the increase in the prevalence of V. dispar in smokers due to the resistance of this species to the increase in ROS resulting from cigarette smoking by its oxidoreductase enzymes (Do et al., 2015). V. dispar was associated with few cases of infective endocarditis (Hillier et al., 2011) and the prevalence of this species in smokers might be one of the factors that enhance smokers’ associated increased risk of infective endocarditis (Strom et al., 2000; Huang and Shi, 2019).
The effect of microbe-microbe interactions on anthracene biodegradation ability along with its influence on viable bacterial count was studied. We tested several combinations of the oral isolates and the combinations giving the highest biodegradation potential with highest viable cells number were the co-cultures of S. mutans and Veillonella spp. except V. dispar. These results of anthracene biodegradation in mixture could be explained by the high biodegradation potential of S. mutans and Veillonella spp. in single cultures among collected isolates while V. dispar showed the lowest degradation potential (35%). The increased number of S. mutans and Veillonella spp. in co-culture was noticed by Kara et al. (2006) and Mashima and Nakazawa (2014) and when they found that the thickness of biofilms formed by S. mutans, in the presence of all the six oral Veillonella spp. was significantly higher than those formed of single Streptococcus spp. culture except for the mixture of S. mutans and V. dispar which is characterized by reduced biofilm formation by S. mutans. They suggested that the difference in mechanisms of biofilm formation, communication and coaggregation between the oral Streptococcus spp. and Veillonella spp. differs according to the species in mixed culture. Several studies reported that genera of Streptococcus and Veillonella are among the members of resident oral microbiota and they are both early colonizers of dental biofilm (Egland et al., 2004; Palmer et al., 2006; Liu et al., 2011, 2019; Mashima and Nakazawa, 2015).
Liu et al. (2011) mentioned that V. parvula is considered as a “friend” to S. mutans as they have been frequently detected in association in dental caries. Communities of both genera are considered as metabolic and co-aggregation partners in the human oral biofilm and that Veillonella spp. are not capable to colonize the dental surface without the aid of Streptococcus spp. during the early stages of oral biofilm formation (Palmer et al., 2006). The mutual relationship between Streptococcus spp. and Veillonella spp. is based on mutual benefits between the two genera where Veillonella spp. utilize short chains organic acids such as lactate produced by Streptococcus spp. for growth because Veillonella spp. is non-saccharolytic which make this genus unable to catabolize glucose or other sugars and rely on the fermentation of lactate and other organic acids to propionic and acetic acids (Mashima and Nakazawa, 2015). The lactate utilization by Veillonella spp. accelerates the glycolytic rate of Streptococcus spp. due to the removal of the lactate end product. Veillonella spp. also generate diffusible signal leading to upregulation of Streptococcus spp. amylase gene (amyB) which in turn increases the production of fermentable glucose and generates more lactate favoring the conditions for growth of Veillonella (Egland et al., 2004). Veillonella spp. relieve the microenvironment acidic pressure promoting the growth of S. mutans and resulting in balance between acid production and acid consumption, eventually leads to a constant pH, moreover it may lead to a less cariogenic environment in vivo. Microorganisms could adapt to the presence of xenobiotics by many mechanisms mentioned earlier, which may explain the increase in anthracene biodegradation ability by the consortium of smokers compared to that of non-smokers (Haiser and Turnbaugh, 2013).
In several studies, Lactobacillus spp. were found to play a beneficial role in inhibiting the growth of some cariogenic bacteria such as S. mutans (del Carmen Ahumada et al., 2001; Sookkhee et al., 2001; Simark-Mattsson et al., 2007; Badet and Thebaud, 2008; Rossoni et al., 2018). L. casei, L. plantarum, L. rhamnosus, L. salivarius, L. fermentum, and L. paracasei were found to exert the greatest antimicrobial activity against S. mutans (Koll-Klais et al., 2005; Teanpaisan et al., 2011). L. fermentum supernatant revealed the presence of antimicrobial compounds mainly organic acids, hydrogen peroxides and bacteriocins (Rodrigues et al., 2020). Phosphoric acid was produced by L. fermentum and it has strong antimicrobial properties against S. mutans (Prado et al., 2015), the bacteriocin detected in culture supernatant showed a broad spectrum of antimicrobial activity against gram positive bacteria such as S. mutans by formation of pores in the cytoplasmic membrane resulting in energy loss and alteration in the activity of important enzymes in the target bacteria (Mokoena, 2017). L. fermentum and its culture supernatant have the ability to decrease S. mutans adherence by inhibiting the production of water insoluble glucan by S. mutans, in addition to the production of biosurfactants which interfere with the adhesion of S. mutans to surfaces (Salehi et al., 2011). Overnight exposure of S. mutans to Lactobacillus spp. spent culture supernatant (SCT) showed an overall significant reduction in the expression of genes involved in virulence of S. mutans in planktonic and biofilm forming cells such as atpD and aguD genes which are acid tolerance genes helping S. mutans to tolerate acidity of the plaque environment and survival under acidic conditions, so downregulation of these genes can lead to bacteriostasis and eventual death (Wasfi et al., 2018).
Conclusion
Cigarette smoking related oral dysbiosis was not limited to changes in oral bacterial counts but it extended to bacterial anthracene biodegradation tested in this study. Smoking participants’ oral rinse contained higher levels of viable S. mutans, V. tobetsuensis, and V. dispar and less of V. parvula compared to non-smoking participants. Although there was no difference observed in the total prevalence of Lactobacillus spp. between smokers and non-smokers; L. fermentum was significantly higher in smokers compared to non-smokers which might be due to the presence of fully operational glutathione system helping the bacteria to resist the oxidative stress resulting from cigarette smoking. Cigarette smoking affected S. mutans not only by changing its viable counts but also its anthracene biodegradation ability. Streptococcus mutans from smokers had higher anthracene biodegradation than non-smokers. Microbe –microbe interaction could account for changes in the anthracene biodegradation potential and the viable count of the microbial mixture compared to their corresponding single isolates and these changes differ according to the constituting genera. Singlets of S. mutans and Veillonella spp. showed higher anthracene biodegradation potential and viable counts than their co-cultures with Lactobacillus spp., suggesting that microbe-microbe interaction also affected anthracene biodegradation ability and not cigarettes toxicants only. The highest anthracene biodegradation ability was observed in the mixture of V. parvula with S. mutans indicating synergistic effect occurring in their co-culture.
Data Availability Statement
The original contributions presented in the study are included in the article/Supplementary Material, further inquiries can be directed to the corresponding author/s.
Ethics Statement
The studies involving human participants were reviewed and approved by the Research Ethics Committees (REC) of faculty of Pharmacy in October University for Modern Sciences and Arts (MSA) and Cairo University. The patients/participants provided their written informed consent to participate in this study.
Author Contributions
SM, NA, and RW conceptualized the work. HM performed the experiments. SM, NA, RW, and HM analyzed the results. All authors edited the manuscript and contributed to the article and approved the submitted version.
Conflict of Interest
The authors declare that the research was conducted in the absence of any commercial or financial relationships that could be construed as a potential conflict of interest.
Supplementary Material
The Supplementary Material for this article can be found online at: https://www.frontiersin.org/articles/10.3389/fmicb.2021.661509/full#supplementary-material
References
Abou-Arab, A. A. K., Salim, A.-B., Maher, R. A., El-Hendawy, H. H., and Awad, A. A. (2015). Persistence of some lactic acid bacteria as affected by polycyclic aromatic hydrocarbons. J. Microbiol. Exp. 2, 159–164. doi: 10.15406/jmen.2015.02.00062
Abranches, J., Zeng, L., Kajfasz, J. K., Palmer, S. R., Chakraborty, B., Wen, Z. T., et al. (2018). Biology of oral streptococci. Microbiol. Spectr. 6:10.1128/microbiolspec.GPP3-0042-2018. doi: 10.1128/microbiolspec.GPP3-0042-2018
Al-Mudallal, N. H., Al-Jumaily, E. F., Muhimen, N. A., and Al-Shaibany, A. A.-W. (2008). Isolation and identification of mutan’s streptococci bacteria from human dental plaque samples. Al Nahrain J. Sci. 11, 98–105.
Arif, N., Do, T., Byun, R., Sheehy, E., Clark, D., Gilbert, S. C., et al. (2008). Veillonella rogosae sp. nov., an anaerobic, Gram-negative coccus isolated from dental plaque. Int. J. Syst. Evolu. Microbiol. 58(Pt. 3):581.
Badet, C., and Thebaud, N. (2008). Ecology of lactobacilli in the oral cavity: a review article. Open Microbiol. J. 2, 38–48. doi: 10.2174/1874285800802010038
Belda-Ferre, P., Alcaraz, L. D., Cabrera-Rubio, R., Romero, H., Simón-Soro, A., Pignatelli, M., et al. (2012). The oral metagenome in health and disease. Multidiscipl. J. Microbial. Ecol. 6, 46–56. doi: 10.1038/ismej.2011.85
Bizzarro, S., Loos, B. G., Laine, M. L., Crielaard, W., and Zaura, E. (2013). Subgingival microbiome in smokers and non-smokers in periodontitis: an exploratory study using traditional targeted techniques and a next-generation sequencing. J. Clin. Periodontol. 40, 483–492. doi: 10.1111/jcpe.12087
Chowdhury, R., Dhar, K., Ahamed, F., Alam, S., and Uddin, M. (2017). Biodegradation of anthracene and phenanthrene by bacteria isolated from oil-contaminated soil of Bangladesh. Chem. Ecol. 33, 843–855. doi: 10.1080/02757540.2017.1382481
Coeuret, V., Dubernet, S., Bernardeau, M., Gueguen, M., and Vernoux, J. P. (2003). Isolation, characterisation and identification of lactobacilli focusing mainly on cheeses and other dairy products. Lait 83, 269–306.
Dalié, D. K. D., Deschamps, A. M., and Richard-Forget, F. (2010). Lactic acid Bacteriapotential for control of mould growth and mycotoxins: a review. Food Control. 21, 370–380. doi: 10.1016/j.foodcont.2009.07.011
del Carmen Ahumada, M.a, López, M.a.E, Colloca, M.a.E, and Nader-Macıìas, M.a.E (2001). Lactobacilli isolation from dental plaque and saliva of a group of patients with caries and characterization of their surface properties. Anaerobe 7, 71–77. doi: 10.1006/anae.2001.0372
Dewhirst, F. E., Chen, T., Izard, J., Paster, B. J., Tanner, A. C. R., Yu, W.-H., et al. (2010). The human oral microbiome. J. Bacteriol. 192, 5002–5017. doi: 10.1128/jb.00542-10
Dixit, A. (2015). An environmental cleanup strategy - microbial transformation of xenobiotic compounds. Int. J. Curr. Microbiol. Appl. Sci. 4, 429–461.
Djais, A., Theodorea, C., Gunawan, H., and Auerkari, E. (2017). The sensitivity of selective veillonella medium as confirmed by gram staining and veill-rpoB polymerase chain reaction. J. Int. Dental Med. Res. 10, 764–768.
Do, T., Sheehy, E. C., Mulli, T., Hughes, F., and Beighton, D. (2015). Transcriptomic analysis of three Veillonella spp. present in carious dentine and in the saliva of caries-free individuals. Front. Cell. Infect. Microbiol. 5:25. doi: 10.3389/fcimb.2015.00025
Egland, P. G., Palmer, R. J. Jr., and Kolenbrander, P. E. (2004). Interspecies communication in Streptococcus gordonii-veillonella atypica biofilms: signaling in flow conditions requires juxtaposition. Proc. Natl. Acad. Sci. U.S.A. 101, 16917–16922. doi: 10.1073/pnas.0407457101
Esteban Florez, F. L., Hiers, R. D., Smart, K., Kreth, J., Qi, F., Merritt, J., et al. (2016). Real-time assessment of Streptococcus mutans biofilm metabolism on resin composite. Dent. Mater. 32, 1263–1269. doi: 10.1016/j.dental.2016.07.010
Gaudet, M., Gapstur, S., Sun, J., Diver, W., Hannan, L., and Thun, M. (2013). Active smoking and breast cancer risk: original cohort data and meta-analysis. J. Natl. Cancer Instit. 105, 515–525. doi: 10.1093/jnci/djt023
Ghannoum, M. A., Jurevic, R. J., Mukherjee, P. K., Cui, F., Sikaroodi, M., Naqvi, A., et al. (2010). Characterization of the oral fungal microbiome (mycobiome) in healthy individuals. PLoS Pathog. 6:e1000713. doi: 10.1371/journal.ppat.1000713
Gibson, J., and Harwood, C. (2002). Metabolic diversity in aromatic compound utilization by anaerobic microbes. Ann. Rev. Microbiol. 56, 345–369. doi: 10.1146/annurev.micro.56.012302.160749
Haiser, H. J., and Turnbaugh, P. J. (2013). Developing a metagenomic view of xenobiotic metabolism. Pharmacol. Res. 69, 21–31. doi: 10.1016/j.phrs.2012.07.009
Haritash, A. K., and Kaushik, C. P. (2009). Biodegradation aspects of polycyclic aromatic hydrocarbons (PAHs): a review. J. Hazardous Mater. 169, 1–15. doi: 10.1016/j.jhazmat.2009.03.137
Hecht, S. S. (2011). “Tobacco smoke carcinogens and lung cancer,” in Chemical Carcinogenesis, ed. T. M. Penning (Totowa, NJ: Humana Press), 1194–1210.
Hillier, D. I., Ballal, M. S., Sanger, R., and Qazzafi, Z. (2011). Veillonella dispar and streptococcus bovis septic arthritis in a native shoulder joint. Shoulder Elbow 3, 219–221. doi: 10.1111/j.1758-5740.2011.00134.x
Huang, C., and Shi, G. (2019). Smoking and microbiome in oral, airway, gut and some systemic diseases. J. Trans. Med. 17:225. doi: 10.1186/s12967-019-1971-7
Huang, R., Li, M., and Gregory, R. (2012). Effect of nicotine on growth and metabolism of Streptococcus mutans. Eur. J. Oral Sci. 120, 319–325. doi: 10.1111/j.1600-0722.2012.00971.x
IARC. (2020). IARC Monographs on the Identification of Carcinogenic Hazards to Humans [Online]. Switzerland: World Health Organization.
Igarashi, E., Kamaguchi, A., Fujita, M., Miyakawa, H., and Nakazawa, F. (2009). Identification of oral species of the genus veillonella by polymerase chain reaction. Oral Microbiol. Immunol. 24, 310–313. doi: 10.1111/j.1399-302X.2009.00513.x
Kara, D., Luppens, S., and ten cate, J. (2006). Differences between single- and dual-species biofilms of Streptococcus mutans and veillonella parvula in growth, acidogenicity and susceptibility to chlorhexidine. Eur. J. Oral Sci. 114, 58–63. doi: 10.1111/j.1600-0722.2006.00262.x
Karale, M., Kadam, T., Bhosale, H., and Maske, K. (2015). Characterization of anthracene degrading bacteria from drug industry effluent polluted soil. Scholars Res. Library Arch. Appl. Sci. Res. 7, 16–22.
Kato, I., Vasquez, A. A., Moyerbrailean, G., Land, S., Sun, J., Lin, H. S., et al. (2016). Oral microbiome and history of smoking and colorectal cancer. J. Epidemiol. Res. 2, 92–101. doi: 10.5430/jer.v2n2p92
Kilian, M., Chapple, I. L., Hannig, M., Marsh, P. D., Meuric, V., Pedersen, A. M., et al. (2016). The oral microbiomean update for oral healthcare professionals. Br. Dent. J. 221, 657–666. doi: 10.1038/sj.bdj.2016.865
Koll-Klais, P., Mandar, R., Leibur, E., Marcotte, H., Hammarstrom, L., and Mikelsaar, M. (2005). Oral lactobacilli in chronic periodontitis and periodontal health: species composition and antimicrobial activity. Oral Microbiol. Immunol. 20, 354–361. doi: 10.1111/j.1399-302X.2005.00239.x
Latimer, J., Munday, J. L., Buzza, K. M., Forbes, S., Sreenivasan, P. K., and McBain, A. J. (2015). Antibacterial and anti-biofilm activity of mouthrinses containing cetylpyridinium chloride and sodium fluoride. BMC Microbiol. 15:169.
Lawal, A. (2017). Polycyclic aromatic hydrocarbons. Rev. Cogent Environ. Sci. 3:1339841. doi: 10.1080/23311843.2017.1339841
Liu, J., Wu, C., Huang, I. H., Merritt, J., and Qi, F. (2011). Differential response of Streptococcus mutans towards friend and foe in mixed-species cultures. Microbiology 157, 2433–2444. doi: 10.1099/mic.0.048314-0
Liu, S., Chen, M., Wang, Y., Zhou, X., Peng, X., Ren, B., et al. (2019). Effect of Veillonella parvula on the physiological activity of Streptococcus mutans. Arch. Oral Biol. 109:104578. doi: 10.1016/j.archoralbio.2019.104578
Lohse, T., Rohrmann, S., Bopp, M., and Faeh, D. (2016). Heavy smoking is more strongly associated with general unhealthy lifestyle than obesity and underweight. PLoS One 11:e0148563. doi: 10.1371/journal.pone.0148563
Mashima, I., and Nakazawa, F. (2014). The influence of oral Veillonella species on biofilms formed by Streptococcus species. Anaerobe 28, 54–61. doi: 10.1016/j.anaerobe.2014.05.003
Mashima, I., and Nakazawa, F. (2015). The interaction between Streptococcus spp. and Veillonella tobetsuensis in the early stages of oral biofilm formation. J. Bacteriol. 197, 2104–2111. doi: 10.1128/JB.02512-14
Mashima, I., Theodorea, C. F., Thaweboon, B., Thaweboon, S., and Nakazawa, F. (2016). Identification of Veillonella Species in the tongue biofilm by using a novel one-step polymerase chain reaction method. PLoS One 11:e0157516. doi: 10.1371/journal.pone.0157516
Mokoena, M. P. (2017). Lactic acid bacteria and their bacteriocins: classification, biosynthesis and applications against uropathogens: a mini-review. Molecules 22:1255. doi: 10.3390/molecules22081255
Moon, J.-H., Lee, J.-H., and Lee, J.-Y. (2015). Subgingival microbiome in smokers and non-smokers in korean chronic periodontitis patients. Mol. Oral Microbiol. 30, 227–241. doi: 10.1111/omi.12086
Moorthy, B., Chu, C., and Carlin, D. J. (2015). Polycyclic aromatic hydrocarbons: from metabolism to lung cancer. Toxicol. Sci. Offi. J. Soc. Toxicol. 145, 5–15. doi: 10.1093/toxsci/kfv040
Oho, T., Yamashita, Y., Shimazaki, Y., Kushiyama, M., and Koga, T. (2000). Simple and rapid detection of Streptococcus mutans and Streptococcus sobrinus in human saliva by polymerase chain reaction. Oral Microbiol. Immunol. 15, 258–262. doi: 10.1034/j.1399-302x.2000.150408.x
Palmer, R., Diaz, P., and Kolenbrander, P. (2006). Rapid succession within the veillonella population of a developing human oral biofilm in situ. J. Bacteriol. 188, 4117–4124. doi: 10.1128/JB.01958-05
Pelt-Verkuil, E. V., Belkum, A. V., and Hays, J. P. (2008). Principles and Technical Aspects of PCR Amplification. Berlin: Springer.
Prado, M., Silva, E.J.N.L.d, Duque, T. M., Zaia, A. A., Ferraz, C. C. R., and Almeida, J.F.A.d, et al. (2015). Antimicrobial and cytotoxic effects of phosphoric acid solution compared to other root canal irrigants. J. Appl. Oral Sci. 23, 158–163. doi: 10.1590/1678-775720130691
Rodrigues, J.Z.d.S, Passos, M. R., Silva de Macêdo Neres, N., Almeida, R. S., Pita, L. S., Santos, I. A., et al. (2020). Antimicrobial activity of Lactobacillus fermentum TcUESC01 against Streptococcus mutans UA159. Microb. Pathog. 142:104063. doi: 10.1016/j.micpath.2020.104063
Rossoni, R. D., Velloso, M.d.S, de Barros, P. P., de Alvarenga, J. A., and Santos, J.D.d, Santos Prado, A.C.C.d, et al. (2018). Inhibitory effect of probiotic Lactobacillus supernatants from the oral cavity on Streptococcus mutans biofilms. Microbial. Pathog. 123, 361–367. doi: 10.1016/j.micpath.2018.07.032
Sabat, A. J., van Zanten, E., Akkerboom, V., Wisselink, G., van Slochteren, K., de Boer, R. F., et al. (2017). Targeted next-generation sequencing of the 16S-23S rRNA region for culture-independent bacterial identification - increased discrimination of closely related species. Sci. Rep. 7:3434. doi: 10.1038/s41598-017-03458-6
Salehi, R., Kermanshahi, R. K., and Eslami, G. (2011). The anti-biofouling effect of Lactobacillus fermentum-derived biosurfactant against Streptococcus mutans. Biofouling 27, 385–392. doi: 10.1080/08927014.2011.575458
Sardaro, N., Della Vella, F., Incalza, M. A., Di Stasio, D., Lucchese, A., Contaldo, M., et al. (2019). Oxidative stress and oral mucosal diseases: an overview. Vivo 33, 289–296. doi: 10.21873/invivo.11474
Simark-Mattsson, C., Emilson, C. G., Hakansson, E. G., Jacobsson, C., Roos, K., and Holm, S. (2007). Lactobacillus-mediated interference of mutans streptococci in caries-free vs. caries-active subjects. Eur. J. Oral Sci. 115, 308–314. doi: 10.1111/j.1600-0722.2007.00458.x
Sookkhee, S., Chulasiri, M., and Prachyabrued, W. (2001). Lactic acid bacteria from healthy oral cavity of thai volunteers: inhibition of oral pathogens. J. Appl. Microbiol. 90, 172–179. doi: 10.1046/j.1365-2672.2001.01229.x
Stewart, C. J., Auchtung, T. A., Ajami, N. J., Velasquez, K., Smith, D. P., and De La Garza, R. II, et al. (2018). Effects of tobacco smoke and electronic cigarette vapor exposure on the oral and gut microbiota in humans: a pilot study. PeerJ 6:e4693. doi: 10.7717/peerj.4693
Strom, B. L., Abrutyn, E., Berlin, J. A., Kinman, J. L., Feldman, R. S., Stolley, P. D., et al. (2000). Risk factors for infective endocarditis. Circulation 102, 2842–2848. doi: 10.1161/01.CIR.102.23.2842
Talhout, R., Schulz, T., Florek, E., van Benthem, J., Wester, P., and Opperhuizen, A. (2011). Hazardous compounds in tobacco smoke. Int. J. Environ. Res. Public Health 8, 613–628. doi: 10.3390/ijerph8020613
Teanpaisan, R., Piwat, S., and Dahlén, G. (2011). Inhibitory effect of oral Lactobacillus against oral pathogens. Lett. Appl. Microbiol. 53, 452–459. doi: 10.1111/j.1472-765X.2011.03132.x
Vallès, Y., Inman, C., Peters, B., Ali, R., Wareth, L., Alsafar, H., et al. (2018). Types of tobacco consumption and the oral microbiome in the united arab emirates healthy future (UAEHFS) pilot study. Sci. Rep. 8:11327. doi: 10.1038/s41598-018-29730-x
Vellappally, S., Fiala, Z., Smejkalova, J., Jacob, V., and Shriharsha, P. (2007). Influence of tobacco use in dental caries development. Central Eur. J. Public Health 15, 116–121.
Wan, A., Seow, W. K., Walsh, L., and Bird, P. (2002). Comparison of five selective media for the growth and enumeration of streptococcus mutans. Austr. Dental J. 47, 21–26. doi: 10.1111/j.1834-7819.2002.tb00298.x
Wasfi, R., Abd El-Rahman, O. A., Zafer, M. M., and Ashour, H. M. (2018). Probiotic Lactobacillus sp. inhibit growth, biofilm formation and gene expression of caries-inducing Streptococcus mutans. J. Cell. Mol. Med. 22, 1972–1983. doi: 10.1111/jcmm.13496
Weisburg, W. G., Barns, S. M., Pelletier, D. A., and Lane, D. J. (1991). 16S ribosomal DNA amplification for phylogenetic study. J. Bacteriol. 173, 697–703. doi: 10.1128/jb.173.2.697-703.1991
Wu, J., Li, M., and Huang, R. (2019). The effect of smoking on caries-related microorganisms. Tobacco Indu. Dis. 17:105913. doi: 10.18332/tid/105913
Wu, J., Peters, B., Dominianni, C., Zhang, Y., Pei, Z., Yang, L., et al. (2016). Cigarette smoking and the oral microbiome in a large study of American adults. ISME J. 10:37. doi: 10.1038/ismej.2016.37
Yousefi, M., Shariatifar, N., Ebrahimi, M., Mortazavian, A., Mohammadi, A., Khorshidian, N., et al. (2018). In vitro removal of polycyclic aromatic hydrocarbons by lactic acid bacteria. J. Appl. Microbiol. 126, 954–964. doi: 10.1111/jam.14163
Keywords: oral microbiota, smoking, polycyclic aromatic hydrocarbons biodegradation, microbial interactions, anthracene
Citation: Moussa HA, Wasfi R, Abdeltawab NF and Megahed SA (2021) High Counts and Anthracene Degradation Ability of Streptococcus mutans and Veillonella parvula Isolated From the Oral Cavity of Cigarette Smokers and Non-smokers. Front. Microbiol. 12:661509. doi: 10.3389/fmicb.2021.661509
Received: 30 January 2021; Accepted: 31 May 2021;
Published: 28 June 2021.
Edited by:
Adam Rosenthal, DuPont, United StatesReviewed by:
Eric Matson, University of Wisconsin–Oshkosh, United StatesEthan Solomon, IFF, United States
Copyright © 2021 Moussa, Wasfi, Abdeltawab and Megahed. This is an open-access article distributed under the terms of the Creative Commons Attribution License (CC BY). The use, distribution or reproduction in other forums is permitted, provided the original author(s) and the copyright owner(s) are credited and that the original publication in this journal is cited, in accordance with accepted academic practice. No use, distribution or reproduction is permitted which does not comply with these terms.
*Correspondence: Nourtan F. Abdeltawab, bm91cnRhbi5hYmRlbHRhd2FiQHBoYXJtYS5jdS5lZHUuZWc=