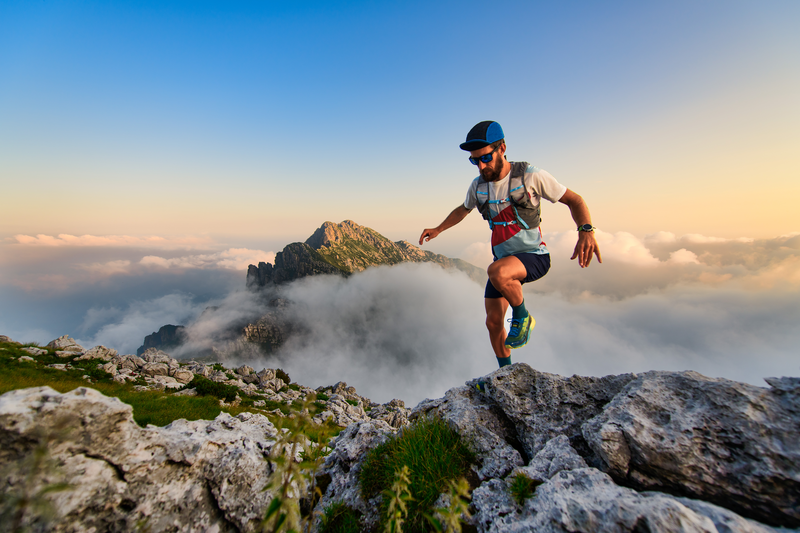
95% of researchers rate our articles as excellent or good
Learn more about the work of our research integrity team to safeguard the quality of each article we publish.
Find out more
MINI REVIEW article
Front. Microbiol. , 22 June 2021
Sec. Systems Microbiology
Volume 12 - 2021 | https://doi.org/10.3389/fmicb.2021.655620
This article is part of the Research Topic Actinobacteria: Recent Trends in Genomics, Omics Study and Discovery of Novel Natural Products View all 7 articles
The presence of secondary metabolite biosynthetic gene clusters (BGCs) makes actinobacteria well-known producers of diverse metabolites. These ubiquitous microbes are extensively exploited for their ability to synthesize diverse secondary metabolites. The extent of their ability to synthesize various molecules is yet to be evaluated. Current advancements in genome sequencing, metabolomics, and bioinformatics have provided a plethora of information about the mechanism of synthesis of these bioactive molecules. Accessing the biosynthetic gene cluster responsible for the production of metabolites has always been a challenging assignment. The genomic approach developments have opened a new gateway for examining and manipulating novel antibiotic gene clusters. These advancements have now developed a better understanding of actinobacterial physiology and their genetic regulation for the prolific production of natural products. These new approaches provide a unique opportunity to discover novel bioactive compounds that might replenish antibiotics’ exhausted stock and counter the microbes’ resistance crisis.
Actinobacteria are an omnipresent group of bacteria that play an essential part in recycling complicated organic matter in the soil. They are of great interest for researchers as they are versatile producers of diverse metabolites with several biotechnological applications. Genome mining tools could help the researchers to determine the BGCs of secondary metabolites compounds like herboxidiene, paulomycin, sceliphrolactam, bagremycin, and humidimycin produced from Streptomyces (Lee et al., 2020). Actinobacteria synthesize several secondary metabolites with various biological activities (Passari et al., 2017). Several phenolic compounds (catechin, kaempferol, chebulagic acid, chlorogenic acid, Asiatic acid, ferulic acid, arjunic acid, gallic acid, and boswellic acid) and paclitaxel, an anticancer compound were detected and quantified by Streptomyces species as reported by Passari et al. (2017). The majority of bioactive compounds from actinobacteria discovered to date have been derived from Streptomyces sp. It is estimated that Streptomyces genome can synthesize 100,000 antimicrobial metabolites from which only a speck has been identified (Doroghazi et al., 2014). For decades, actinobacteria have been intensively exploited by industrial discovery programs for novel natural products (NPs), to derive antibiotics currently in clinical use (Genilloud, 2018). Despite this, the development and spread of multi-drug resistance among pathogens have made it difficult to treat the infections resulting in significant health concerns. The lack of therapeutic solutions for the rising disorders by resistant pathogens in the clinic requires a new approach to responding to novel antibiotics. Tapping into the actinobacterial genomes and exploiting rare actinobacteria with unexpected genes might be an answer to the ever-rising need of novel antibiotics (Ward and Allenby, 2018). The rare actinobacteria which are of non-streptomycetes category, isolated from diverse habitats have efficiency for the discovery of novel bioactive compounds. The draft genome of Saccharomonospora sp. CNQ490 has revealed 19 unexplored BGCs exhibiting its diverse metabolic capacity. These actinobacteria can be a promising source for drug discovery (Luzhetskyy et al., 2007; Thumar et al., 2010; Hug et al., 2018). Particularly, non-streptomycetes belonging to genera, such as Micromonospora, Nocardia, Actinomadura, Actinoplanes, Streptoverticilllium, and Saccharopolyspora have been reported to produce unique antibiotics with various biological activities (Hug et al., 2018), such as abyssomicins (Bister et al., 2004) and proximicins (Fiedler et al., 2008) from Verrucosispora strains. Subsequently, approximately 2,250 new bioactive secondary metabolites have been discovered from rare actinobacteria according to 2005 information (Genilloud et al., 2011; Subramani and Aalbersberg, 2013).
In the past decade, the failure to unveil the full potential of the natural product (NP) producing actinobacteria was due to the lack of understanding of their genome and expression of biosynthetic gene clusters (BGCs). The advent of rapid and economical sequencing technologies has revealed that BGCs in actinobacteria are in more significant numbers than the already discovered molecules synthesized by them (Schorn et al., 2016; Tokovenko et al., 2016). The evolution of genomics and bioinformatics tools has exponentially increased the understanding of useful genetic information that can be exploited to discover bioactive compounds (Machado et al., 2015; Weber and Kim, 2016; Albarano et al., 2020; Figure 1). The genome sequencing of Streptomyces has revealed that they contain BGC in large numbers. Streptomyces clavuligerus was found to have 58 BGCs, Streptomyces bottropensis have 21 BGCs, whereas S. avermitilis contain 30 BGCs (Zhang et al., 2017). Actinobacterial genomes are sequenced rapidly since 2013 for the hunt for novel bioactive NP discovery. Till recently, a total of 1,749 Streptomyces genomes have been deposited as of the 6th of February 2020 and are available in the RefSeq database. The 1,749 Streptomyces genomes contains 867 contig level, 646 scaffold level, 36 chromosome level, whereas 200 complete genomes are included (Lee et al., 2020).
The current review explicates the recent advancements in actinobacterial genomic research. It gives an insight into the different technologies currently employed in genomics for NP discovery, along with challenges and research gaps that need to be filled for better outcomes.
A plethora of actinobacteria genomes has been sequenced and submitted to databases by different researchers. RefSeq database contains 1,749 Streptomyces genomes (Lee et al., 2020). Researchers started mining genomes successfully and isolated potential metabolites from the available genetic data based on these available databases. Bioinformatics tools like antiSMASH, PRISM, ClustScan, and CLUSEAN have revolutionized the researchers’ genome mining experience and have acquainted us with the untapped potential of the actinobacteria. Over time, the actinobacterial strain collection from different habitats has increased, resulting in massive genetic data enhancement. This has resulted in an overwhelming discovery of novel metabolites in the past 10 years. Initially, genome mining was accomplished by detecting and exploiting the central enzymes of secondary metabolite production’s biosynthetic pathways. These enzymes include polyketide synthases (PKSs), non-ribosomal peptide synthetases (NRPs). Analyzing the massive database of actinobacterial genomics requires specialized bioinformatics tools.
Later, various computational tools like antiSMASH and PRISM were developed that could identify partially or complete BCGs. These tools efficiently compared the user-submitted genomes with the available BCGs database and provided a structural prediction of secondary metabolites. The methods used for structural prediction followed certain basic rules which involved consideration of substrate specificity of catalytic domain of PKS and NRPS module for developing the backbone of metabolite structure. It was followed by identification of the tailoring domains through which the further cyclization of compound was predicted and later mapped with the available databases to provide an idea of secondary structure produced by unknown BGCs. The prediction accuracy completely depends on the algorithm and available databases. Presently, the database comprises of limited number of experimentally validated genes. The incorrect gene annotations have hampered the accuracy in gene mining and prediction of structure of NPs and lead to spread of false functional role of the gene (Salzberg, 2019). Despite the advancements in structural predictions over time, lack of information on tailoring enzymes and assignment of related BGCs as hybrid BGCs still require validations and improvements. Certain bioactive compounds like streptoketides from Streptomyces sp. Tu6314, atratumycin from S. atratus, nybomycin from S. albus has been discovered using antiSMASH based genome mining (Sun et al., 2019; Qian et al., 2020). Bioinformatics tools like MultiGeneBlast can be employed to identify BGCs within any genomic database like MIBiG, which comprises of known gene clusters (Medema and Fischbach, 2015). Specific tools like NaPDoS (Natural Product Domain Seeker) are used to identify distinct antibiotic gene clusters within the genome.
The recent advancements in next-generation sequencing (NGS) and genome mining have revealed that actinobacteria have a potential of synthesizing enormous compounds. The Streptomyces sp. within the group actinobacteria harbor linear chromosomes and have the largest genome among bacteria ranging from 6 to 12 Mb (Tidjani et al., 2019; Nicault et al., 2020). They are responsible for the production of two-third of the total antibiotics that are in clinical use today (Takahashi and Nakashima, 2018; Van Bergeijk et al., 2020). The production of these bioactive compounds depends on the presence of genes that encode the enzymes essential for synthesizing these compounds. These genes are arranged in groups known as BGCs in microbial genomes (Cimermancic et al., 2014).
Different studies have revealed that actinobacterial genomes are a storehouse of the plethora of antibiotics (Salwan and Sharma, 2020; Silva et al., 2020). Streptomyces are not the only source for the production of bioactive compounds, but they are prolific producers and therefore the genus is the greatest in the prokaryotes and also contains wide numbers of 20–30 BGCs for secondary metabolites, which include PKSs and NRPs. Total, 1346 BGCs detected by antiSMASH in the 39 streptomycete genomes in the antiSMASH database (https://antismash-db.secondarymetabolites.org/; accessed March 2018) (Ward and Allenby, 2018). Earlier, the discovery of BGC was based entirely on bioactive screening, which involves the laborious procedure of construction and screening the genome libraries. The advent of whole-genome sequencing methods revolutionized the detection of BGCs. Streptomyces coelicolor, before its genome sequencing, was known to produce four metabolites (Gomez-Escribano and Bibb, 2011; Hoskisson and Van Wezel, 2019). The whole-genome sequencing revealed the presence of 18 BGC, which were utterly incognito (Berdy, 2012). Since then, novel methods have been developed to detect BGCs using whole-genome databases and were termed genome mining (Albarano et al., 2020; Nicault et al., 2020; Van Bergeijk et al., 2020). The approach of genome mining enables prediction of BGCs within actinobacterial genome data by using bioinformatics tools like antiSMASH. Once the gene clusters are predicted they are made to express under laboratory conditions for the production of different novel compounds. Certain novel compounds were discovered recently using the genome mining approach, including humidimycin, isolated from Streptomyces humidus (Sánchez-Hidalgo et al., 2020), pentaminomycin from Streptomyces cacaoi (Kaweewan et al., 2020), pentamycin from Streptomycin sp. S816 (Zhou et al., 2019), salinosporamide A from marine actinobacteria Salinospora tropica (Pérez and Fenical, 2017), and abyssomicin C from a marine Verrucosispora (Freundlich et al., 2010; Table 1). Implementing a genome mining-based approach in Streptomyces curacoi resulted in the discovery of oxazole, methyloxazole, and thiazole ring containing macrocyclic compound named Curacozole (Kaweewan et al., 2020).
Even after genome mining and discovering unexpressed BGCs in actinobacteria, utilizing it for NP discovery remains a tedious challenge. Streptomyces coelicolor being the most studied actinobacteria, still harbors undiscovered biosynthetic pathways. These actinobacteria carried out the discovery of rare amicetin by inactivating the biosynthetic genes of streptomycin and streptothricin (Zhou et al., 2019). Programming a genome for the production of NPs is the biggest challenge in discovering novel bioactive compounds. Another challenge that needs to be considered is that even after choosing a BGC for desired NP production, its expression within the host or laboratory conditions remains poor (Lee et al., 2019). The actinobacteria produce metabolites by multi-enzyme catalytic pathways, which are encoded by BGCs. The biotic and abiotic stresses strictly regulate these gene clusters’ expression in their thriving natural habitat. Therefore, only a speck of metabolites is produced within the controlled environment of laboratories. To harness the full potential of actinobacteria for synthesis of diverse compounds; we need to understand the natural stimulus required to induce the production of NPs. The complete biosynthetic potential of actinobacteria in such a scenario can be explored by developing tools to detect complete BGCs, especially those that remain silent in laboratory conditions.
One of the critical steps in genome mining studies is the prediction of NP structures from the genome. Bioinformatics tools like antiSMASH (Blin et al., 2019) and PRISM (Skinnider et al., 2017) are available that ensures the structural novelty of the NPs. Still, another challenge is the precise prediction of these structures, as these tools are based on our current understanding of BGC knowledge. The prediction of these structures is based on the algorithm and the information within the available databases. The lack of genomic data affects the predictive accuracy of the NP structure. There exist 16 different tailoring pathways that combine to form the complex structure of erythromycin. The lack or improper prediction of any tailoring step may result in its inaccurate system (Amarasinghe et al., 2020).
Most of the BGCs are silent or “cryptic,” which require advanced approaches like genome editing, genome engineering, or metabolic engineering for expression. The available datasets are point out that there is a plethora of uncharacterized BGC in nature whose potential is yet to be characterized (Lee et al., 2020). The hybrid clusters of PKSs, NRPs have been the current research array as an existing lot of available antibiotics have majorly become inefficacious against potential pathogens. The products formed by the combination of clusters can be crucial and might counter the problem of infections caused by resistant microbes by providing novel bioactive molecules. The production of virginiamycin and oxazolomycin has been reported recently, resulting from PKS/NRPS hybrid (Sharma and Thakur, 2020).
The bottlenecks in the biosynthetic pathways restrict the natural production of metabolites in high titers. There are different targeted approaches that are designed for the activation of specific BGCs in actinobacteria which includes heterologous expression, promoter exchange and BGC regulator manipulation. Heterologous expression of BGCs has become a common approach for NP discovery (Ren et al., 2017). This strategy requires a suitable host that can be genetically mutated and is fast growing. Among Streptomyces species, S. coelicolor (Zhou et al., 2012), S. avermitilis (Komatsu et al., 2010), S. albus have been genetically engineered for the expression of desired metabolites (Liu et al., 2018). S. coelicolor A(3)2 is the most commonly used heterologous host for expression and production of different classes of NPs like novobiocin (Eustáquio et al., 2005), salinomycin (Yin et al., 2015).
Within the natural environment, the actinobacterial BGCs get expressed at a much better scale than that in laboratory conditions. The expression of silent or poorly expressed gene clusters can be regulated using transcriptional activators. The transcription mechanism of BGCs involves specific gene clusters comprising of activators and repressors, which can activate or suppress any biosynthetic pathway. Apart from activating the cryptic genes, transcriptional regulators can also upscale the production of actinobacterial metabolites (Doroghazi et al., 2014). The presence PSRs genes offer possibility of activating BGCs by suppressing the repressor or upscale production of metabolite or by over-expression of activator (Baral et al., 2018). The discovery of antibiotic argimycin P using Streptomyces argillaceus was possible only after inactivation of TetR transcriptional repressor gene arpRII (Ye et al., 2018). Similarly, the transcription regulation for overexpression of orf22 and orf42 in Streptomyces fungicidicus upscaled the production of enduracidins by 4-fold (Chen et al., 2019). Different approaches for activating the silent BGCs include optimization of organism’s growth media, which is a hit and trial approach—altering various sources of nutrition influences the organism’s metabolome, thus activating their BGCs. This approach has enabled the discovery of novel thiopeptide antibiotic TP-1161 from Nocardiopsis sp. Since actinobacteria dwell in soil, it is reported that addition of rare earth elements like scandium and lanthanum can enhance the production of antibiotic to multifold (Xia et al., 2020). Other techniques include the addition of elicitors, metabolic engineering, and ribosome engineering. Practically, γ-butyrolactones can be used as chemical elicitors for the production of antibiotics like virginiamycin, showdomycins in actinobacteria. Using ribosomal engineering, S. coelicolor A3(2) yielded 1.63 g/L of actinorhodin which is 180-fold higher than wild type strain (Sharma and Thakur, 2020).
The activation method of cryptic BGCs includes expression in heterologous hosts, promoter exchange, and genome editing using CRISPR (Li et al., 2018). The most commonly employed strategy is the isolation of BGC from the native environment and its heterologous expression within the host. This BGC activation strategy is most successful as the host can be domesticated in the characterized environment and is genetically stable.
The novel BCGs identified and chosen to discover NP pose hurdles in expression within the standard fermentation process. The majority of the BCG are silent or cryptic, and heterologous expression is the only possible solution for the expression of these gene clusters. This expression is carried out in three significant steps: identifying BGC in the actinobacterial genome, cloning and expression in a heterologous host, and product isolation. There lies many constrains in heterologous expression of selective metabolite production. The major limitation is that only a few organisms can be used a model for heterologous expression (Demain, 2014). Apart from that mandatory knowledge of bias codons, substrate specificity and metabolic regulation of host should be available (Luo et al., 2015). The probability of expression of native promoters is always a question. Moreover, the expressed product may prove to be toxic for the heterologous host (Helfrich et al., 2014). Maintaining a fine tuning between the expression and production of metabolite should be in check. Evading these shortcomings lead to low recombination efficiency.
Within the natural environment, the actinobacterial genes are expressed better than its laboratory cultivation. Specific genes become cryptic or silent once actinobacteria are cultured on an artificial medium. To express these silent genes, a better understanding of actinobacterial interaction with the biotic and abiotic components of its environment is requisite (Lee et al., 2020).
Over a brief period, CRISPR/Cas9 genome editing tool has been a great breakthrough in manipulating different organisms’ genetic makeup (Tong et al., 2015). Streptococcus thermophilus which is widely used for making cheese and yogurts was often infected with virus which effected the quality of these food products. The alteration in genetic makeup using CRISPR sequences made S. thermophilus immune toward viral attacks. Huang et al. (2015) utilized CRISPR/Cas9 for removing BGCs of various lengths from the most widely investigated actinobacteria S. coelicolor. This tool is useful in engineering a host for heterologous expression and the production of NPs. Through the RNA guided DNA editing technology of CRISPR/Cas9 the BGCs of different length from S. coelicolor was removed and its heterologous expression was carried out in native host for the production of various novel compounds. Using CRISPR/Cas9 genes and BGCs of Streptomyces pyogenes were deleted, and site-directed mutagenesis was performed, which gradually enhanced the secondary metabolite production (Zeng et al., 2015). This technology was also utilized to activate and express silent BGCs in Streptomyces sp. (Zhang et al., 2017). This tool is highly efficient, and its combination with other existing technology can result in discovering novel compounds.
CRISPR-Cas9 mediated promoter knockout strategy is widely employed for activation of silent BGCs within actinobacteria. The novel amexanthomycins A-J were discovered only after knocking out rifampicin promoter genes in Amycolatopsis mediterranei S6991 (Hug et al., 2018). The native promoter within S. albus was replaced by a strong constitutive promoter using CRISPR-Cas9 (Li et al., 2018). This modification in the genome gradually enhanced metabolites’ biosynthesis, and novel compounds like type II polyketides were obtained using this technique (Zhang et al., 2017). The successful production of auroramycin, a type I PKS was carried out using S. roseosporus NRRL 15998 by CRISPR-Cas9 based promoter knock in of KasOp promoter to activate the silent BGCs (Lim et al., 2018). A similar approach was carried out in S. venezuelae, S. lividans, and S. viridochromogenesto discover NPs (Jia et al., 2017). The promoter knockout technique is useful in the activation and expression of silent BGCs. CRISPR-Cas9 is highly efficient in actinobacterial genome editing and has revolutionized the genome engineering technology to discover new uncharacterized compounds.
The genetic potential for the discovery of bioactive molecules by actinobacteria can be determined via genome mining. Sequencing actinomycete genomes provide useful information for inventing novel antimicrobial agents. The sequencing of rare actinobacteria genomes guided with bioinformatics analysis will open the door for scientists to explore the biochemical pathways and, consequently, discover novel bioactive molecules. This approach would contribute to more discoveries of natural antibiotics and therefore promote the pharmaceutical industry.
The discovery of NPs by harnessing the genomic potential of actinobacteria is making significant progress with time. Still, the rate of discovery of novel bioactive compounds is lesser than the pace by which microbes are attaining resistance. To enhance the pace screening of BGCs and determining their novelty at the earliest is necessary. Moreover, a multidisciplinary approach involving microbiologists, computational biologists, and chemists needs to collaborate to increase the discovery of NPs from actinobacterial sources. The new environmental niches should be approached for isolation of novel actinobacteria, which might provide novel BCGs to discover NPs.
Various researchers reported that actinobacteria especially genus Streptomyces and rare actinobacteria are well-known to produce wide number of bioactive compounds (Barka et al., 2016; Genilloud, 2017; Li et al., 2019a). In the last 10 years, a number of novel metabolic engineering and synthetic biology strategies have been established to exploit the development potential of actinobacteria, including dynamic metabolic control, BGC amplification, pathway refactoring, and genome-minimized Streptomyces chassis (Beites and Mendes, 2015; Tan and Liu, 2017; Li et al., 2019b). We anticipate that NP discovery and development will be accelerated rapidly by refactoring and amplifying entire biosynthetic pathways in combination with powerful heterologous expression platforms. It is very much essential to search diverse heterologous hosts and universal refactoring methods to trigger silent BGCs or for the production of secondary metabolites.
TS, AP, AJ, AH, and AA: writing original draft. SB, VG, and EA: writing-review and editing. All authors contributed to the article and approved the submitted version.
The authors declare that the research was conducted in the absence of any commercial or financial relationships that could be construed as a potential conflict of interest.
We would like to extend their sincere appreciation to the Deanship of Scientific Research at King Saud University for funding this research group NO (RGP-271). AP was supported by the postdoctoral scientist grant number IN202216 from DGAPA, UNAM. AP is also thankful to CONACYT (Grant No. 979132) for receiving Sistema Nacional de Investigadores (SNI) level I. We are thankful to CA Shalu Agrawal, Delhi, India for the critical reading and the language editing of the manuscript.
Albarano, L., Esposito, R., Ruocco, N., and Costantini, M. (2020). Genome mining as new challenge in natural products discovery. Mar. Drugs 18:199. doi: 10.3390/md18040199
Amarasinghe, S. L., Su, S., Dong, X., Zappia, L., Ritchie, M. E., and Gouil, Q. (2020). Opportunities and challenges in long-read sequencing data analysis. Genome Biol. 21:30. doi: 10.1186/s13059-020-1935-5
Asolkar, R. N., Kirkland, T. N., Jensen, P. R., and Fenical, W. (2010). Arenimycin, an antibiotic effective against rifampin-and methicillin-resistant Staphylococcus aureus from the marine actinomycete Salinispora arenicola. J. Antibiot. 63, 37–39. doi: 10.1038/ja.2009.114
Axenov-Gibanov, D., Voytsekhovskaya, I. V., Tokovenko, B. T., Protasov, E. S., Gamaiunov, S. V., Rabets, Y. V., et al. (2016). Actinobacteria isolated from an underground lake moonmilk speleothem from the biggest conglomeratic karstic cave in Siberia as sources of novel biologically active compounds. PLoS One 11:e0149216. doi: 10.1371/journal.pone.0149216
Baral, B., Akhgari, A., and Metsa-Ketela, M. (2018). Activation of microbial secondary metabolite pathways: avenues and challenges. Synth. Syst. Biotechnol. 3, 163–178. doi: 10.1016/j.synbio.2018.09.001
Barka, E. A., Vatsa, P., Sanchez, L., Gaveau-Vaillant, N., Jacquard, C., Klenk, H. P., et al. (2016). Taxonomy, physiology, and natural products of actinobacteria. Microbiol. Mol. Biol. Rev. 80, 1–43. doi: 10.1128/MMBR.00019-15
Beites, T., and Mendes, M. V. (2015). Chassis optimization as a cornerstone for the application of synthetic biology-based strategies in microbial secondary metabolism. Front. Microbiol. 6:906. doi: 10.3389/fmicb.2015.00906
Berdy, J. (2012). Thoughts and facts about antibiotics: where we are now and where we are heading. J. Antibiot. 65, 385–395. doi: 10.1038/ja.2012.27
Bister, B., Bischoff, D., Strobele, M., Riedlinger, J., Reicke, A., Wolter, F., et al. (2004). Abyssomicin C—A polycyclic antibiotic from a marine Verrucosispora strain as an inhibitor of the p-aminobenzoic acid/tetrahydrofolate biosynthesis pathway. Angew. Chem. Int. Ed. Engl. 43, 2574–2576. doi: 10.1002/anie.200353160
Blin, K., Shaw, S., Steinke, K., Villebro, R., Ziemert, N., Lee, S. Y., et al. (2019). antiSMASH 5.0:updates to the secondary metabolite genome mining pipeline. Nucleic Acids Res. 47, W81–W87. doi: 10.1093/nar/gkz310
Chen, Y. W., Liu, X. C., Lv, F. X., and Li, P. (2019). Characterization of three regulatory genes involved in enduracidin biosynthesis and improvement of enduracidin production in Streptomyces fungicidicus. J. Appl. Microbiol. 127, 1698–1705. doi: 10.1111/jam.14417
Cimermancic, P., Medema, M. H., Claesen, J., Kurita, K., Brown, L. C. W., Mavrommatis, K., et al. (2014). Insights into secondary metabolism from a global analysis of prokaryotic biosynthetic gene clusters. Cell 158, 412–421. doi: 10.1016/j.cell.2014.06.034
Demain, A. L. (2014). Importance of microbial natural products and the need to revitalize their discovery. J. Ind. Microbiol. Biotechnol. 41, 185–201. doi: 10.1007/s10295-013-1325-z
Derewacz, D. K., McNees, C. R., Scalmani, G., Covington, C. L., Shanmugam, G., Marnett, L. J., et al. (2014). Structure and stereochemical determination of hypogeamicins from a cave-derived actinomycete. J. Nat. Prod. 77, 1759–1763. doi: 10.1021/np400742p
Dias, D. A., Urban, S., and Roessner, U. (2012). A historical overview of natural products in drug discovery. Metabolites 2, 303–336. doi: 10.3390/metabo2020303
Doroghazi, J. R., Albright, J. C., Goering, A. W., Ju, K. S., Haines, R. R., Tchalukov, K. A., et al. (2014). A roadmap for natural product discovery based on large-scale genomics and metabolomics. Nat. Chem. Biol. 10, 963–968. doi: 10.1038/nchembio.1659
Eustáquio, A. S., Gust, B., Galm, U., Li, S. M., Chater, K. F., and Heide, L. (2005). Heterologous expression of novobiocin and clorobiocin biosynthetic gene clusters. Appl. Environ. Microbiol. 71, 2452–2459. doi: 10.1128/aem.71.5.2452-2459.2005
Fiedler, H. P., Bruntner, C., Riedlinger, J., Bull, A. T., Knutsen, G., Goodfellow, M., et al. (2008). Proximicin A, B and C, novel aminofuran antibiotic and anticancer compounds isolated from marine strains of the actinomycete Verrucosispora. J. Antibiot. 61, 158–163. doi: 10.1038/ja.2008.125
Freundlich, J. S., Lalgondar, M., Wei, J.-R., Swanson, S., Sorensen, E. J., Rubin, E. J., et al. (2010). The abyssomicin C family as in vitro inhibitors of Mycobacterium tuberculosis. Tuberculosis 90, 298–300. doi: 10.1016/j.tube.2010.08.002
Genilloud, O. (2017). Actinomycetes: still a source of novel antibiotics. Nat. Prod. Rep. 34, 1203–1232. doi: 10.1039/c7np00026j
Genilloud, O. (2018). Mining actinomycetes for novel antibiotics in the omics era: are we ready to exploit this new paradigm? Antibiotics (Basel) 7:85. doi: 10.3390/antibiotics7040085
Genilloud, O., Gonzalez, I., Salazar, O., Martin, J., Tormo, J. R., and Vicente, F. (2011). Current approaches to exploit actinomycetes as a source of novel natural products. J. Ind. Microbiol. Biotechnol. 38, 375–389. doi: 10.1007/s10295-010-0882-7
Gomez-Escribano, J. P., and Bibb, M. J. (2011). Engineering Streptomyces coelicolor for heterologous expression of secondary metabolite gene clusters. Microb. Biotechnol. 4, 207–215. doi: 10.1111/j.1751-7915.2010.00219.x
Helfrich, E. J., Reiter, S., and Piel, J. (2014). Recent advances in genome based polyketide discovery. Curr. Opin. Biotechnol. 29, 107–115. doi: 10.1016/j.copbio.2014.03.004
Hoskisson, P. A., and Van Wezel, G. P. (2019). Streptomyces coelicolor. Trends Microbiol. 27, 468–469. doi: 10.1016/j.tim.2018.12.008
Huang, H., Zheng, G., Jiang, W., Hu, H., and Lu, Y. (2015). One-step high-efficiency CRISPR/Cas9-mediated genome editing in Streptomyces. Acta Biochim. Biophys. Sin. (Shanghai) 47, 231–243. doi: 10.1093/abbs/gmv007
Hug, J. J., Bader, C. D., Remskar, M., Cirnski, K., and Muller, R. (2018). Concepts and methods to access novel antibiotics from actinomycetes. Antibiotics (Basel) 7:44. doi: 10.3390/antibiotics7020044
Janardhan, A., Kumar, A. P., Viswanath, B., Saigopal, D. V. R., and Narasimha, G. (2014). Production of bioactive compounds by actinomycetes and their antioxidant properties. Biotechnol. Res. Int. 2014:217030. doi: 10.1155/2014/217030
Jia, H., Zhang, L., Wang, T., Han, J., and Tang, H. (2017). Development of a CRISPR/Cas9-mediated gene-editing tool in Streptomyces rimosus. Microbiology 163, 1148–1155. doi: 10.1099/mic.0.000501
Jiang, L., Pu, H., Xiang, J., Su, M., Yan, X., Yang, D., et al. (2018). Huanglongmycin A-C, cytotoxic polyketides biosynthesized by a putative type II polyketide synthase from Streptomyces sp. CB09001. Front. Chem. 6:254. doi: 10.3389/fchem.2018.00254
Kaweewan, I., Hemmi, H., Komaki, H., and Kodani, S. (2020). Isolation and structure determination of a new antibacterial peptide pentaminomycin C from Streptomyces cacaoi subsp. cacaoi. J. Antibiot. 73, 224–229. doi: 10.1038/s41429-019-0272-y
Komatsu, M., Uchiyama, T., Ōmura, S., Cane, D. E., and Ikeda, H. (2010). Genome-minimized Streptomyces host for the heterologous expression of secondary metabolism. Proc. Natl. Acad. Sci. U.S.A. 107, 2646–2651. doi: 10.1073/pnas.0914833107
Lee, N., Hwang, S., Kim, J., Cho, S., Palsson, B., and Cho, B. (2020). Genome mining approaches for the identification of secondary metabolite biosynthetic gene clusters in Streptomyces. Comput. Struct. Biotechnol. 18, 1548–1556. doi: 10.1016/j.csbj.2020.06.024
Lee, N., Hwang, S., Lee, Y., Cho, S., Palsson, B., and Cho, B. K. (2019). Synthetic biology tools for novel secondary metabolite discovery in Streptomyces. J. Microbiol. Biotechnol. 29, 667–686. doi: 10.4014/jmb.1904.04015
Li, L., Liu, X., Jiang, W., and Lu, Y. (2019a). Recent advances in synthetic biology approaches to optimize production of bioactive natural products in actinobacteria. Front. Microbiol. 10:2467. doi: 10.3389/fmicb.2019.02467
Li, L., Liu, X., Wei, K., Lu, Y., and Jiang, W. (2019b). Synthetic biology approaches for chromosomal integration of genes and pathways in industrial microbial systems. Biotechnol. Adv. 37, 730–745. doi: 10.1016/j.biotechadv.2019.04.002
Li, L., Wei, K., Zhemg, G., Liu, X., Chen, S., Jiang, W., et al. (2018). CRISPR-Cpf1 assisted multiplex genome editing and transcriptional repression in Streptomyces. Appl. Environ. Microbiol. 84, e00827–18.
Lim, Y. H., Wong, F. T., Yeo, W. L., Ching, K. C., Lim, Y. W., Heng, E., et al. (2018). Auroramycin: a potent antibiotic from Streptomyces roseosporus by CRISPR-Cas9 activation. Chem. Bio. Chem. 19, 1716–1719. doi: 10.1002/cbic.201800266
Liu, R., Deng, Z., and Liu, T. (2018). Streptomyces species ideal chassisfor natural product discovery and overexpression. Metab. Eng. 50, 74–84. doi: 10.1016/j.ymben.2018.05.015
Luo, Y., Li, B. Z., Liu, D., Zhang, L., Chen, Y., Jia, B., et al. (2015). Engineered biosynthesis of natural products in heterologous hosts. Cem. Soc. Rev. 44, 5265–5290. doi: 10.1039/c5cs00025d
Luzhetskyy, A., Pelzer, S., and Bechthold, A. (2007). The future of natural products as a source of new antibiotics. Curr. Opin. Investig. Drugs 8, 608–613.
Machado, H., Sonnenschein, E. C., Melchiorsen, J., and Gram, L. (2015). Genome mining reveals unlocked bioactive potential of marine Gram-negative bacteria. BMC Genomics 16:158. doi: 10.1186/s12864-015-1365-z
Medema, M. H., and Fischbach, M. A. (2015). Computational approaches to natural product discovery. Nat. Chem. Biol. 11, 639–648. doi: 10.1038/nchembio.1884
Nicault, M., Tidjani, A. R., Gauthier, A., Dumarcay, S., Gelhaye, E., and Bontemps, C. (2020). Mining the biosynthetic potential for specialized metabolism of a Streptomyces soil community. Antibiotics 9:271. doi: 10.3390/antibiotics9050271
Nithya, K., Muthukumar, C., Biswas, B., Alharbi, N. S., Kadaikunnan, S., and Khaled, J. M. (2017). Desert actinobacteria as a source of bioactive compounds production with a special emphases on Pyridine-2,5-diacetamide a new pyridine alkaloid produced by Streptomyces sp. DA3-7. Microbiol. Res. 207, 116–133. doi: 10.1016/j.micres.2017.11.012
Passari, A. K., Mishra, V. K., Singh, G., Singh, P., Kumar, B., Gupta, V. K., et al. (2017). Insights into the functionality of endophytic actinobacteria with a focus on their biosynthetic potential and secondary metabolites production. Sci. Rep. 7:11809. doi: 10.1038/s41598-017-12235-4
Pérez, L. B., and Fenical, W. (2017). Accessing Marine Microbial Diversity for Drug Discovery Microbial Resources. Amsterdam: Elsevier, 169–187.
Qian, Z., Bruhn, T., D’Agostino, P. M., Herrmann, A., Haslbeck, M., Antal, N., et al. (2020). Discovery of the streptoketides by direct cloning and rapid heterologous expression of a cryptic PKS II gene cluster from Streptomyces sp. Tu 6314. J. Org. Chem. 85, 664–673. doi: 10.1021/acs.joc.9b02741
Ren, H., Wang, B., and Zhao, H. (2017). Breaking the silence: new strategies for discovering novel natural products. Curr. Opin. Biotechnol. 48, 21–27. doi: 10.1016/j.copbio.2017.02.008
Rodríguez Estévez, M., Gummerlich, N., Myronovskyi, M., Zapp, J., and Luzhetskyy, A. (2020). Benzanthric acid, a novel metabolite from Streptomyces albus Del14 expressing the nybomycin gene cluster. Front. Chem. 7:896. doi: 10.3389/fchem.2019.00896
Salwan, R., and Sharma, V. (2020). Molecular and biotechnological aspects of secondary metabolites in actinobacteria. Microbiol. Res. 231:126374. doi: 10.1016/j.micres.2019.126374
Salzberg, S. L. (2019). Next generation genome annotation: we still struggle to get it right. Genome Biol. 20:92.
Sánchez-Hidalgo, M., Martín, J., and Genilloud, O. (2020). Identification and heterologous expression of the biosynthetic gene cluster encoding the lasso peptide humidimycin, a caspofungin activity potentiator. Antibiotics 9:67. doi: 10.3390/antibiotics9020067
Schorn, M. A., Alanjary, M. M., Aguinaldo, K., Korobeynikov, A., Podell, S., Patin, N., et al. (2016). Sequencing rare marine actinomycete genomes reveals high density of unique natural product biosynthetic gene clusters. Microbiology (Reading, England) 162, 2075–2086. doi: 10.1099/mic.0.000386
Sharma, P., and Thakur, D. (2020). Antimicrobial biosynthetic potential and diversity of culturable soil actinobacteria from forest ecosystems of Northeast India. Sci. Rep. 10:4104. doi: 10.1038/s41598-020-60968-6
Silva, L. J., Crevelin, E. J., Souza, D. T., Lacerda-Junior, G. V., de Oliveira, V. M., Gois Ruiz, A. L. T., et al. (2020). Actinobacteria from Antarctica as a source for anticancer discovery. Sci. Rep. 10:13870. doi: 10.1038/s41598-020-69786-2
Skinnider, M. A., Merwin, N. J., Johnston, C. W., and Magarvey, N. A. (2017). PRISM 3: expanded prediction of natural product chemical structures from microbial genomes. Nucleic Acids Res. 45, W49–W54. doi: 10.1093/nar/gkx320
Subramani, R., and Aalbersberg, W. (2013). Culturable rare actinomycetes: diversity, isolation and marine natural product discovery. Appl. Microbiol. Biotechnol. 97, 9291–9321. doi: 10.1007/s00253-013-5229-7
Sugiura, T., Ariyoshi, Y., Negoro, S., Nakamura, S., Ikegami, H., and Takada, M. (2005). Phase I/II study of amrubicin, a novel 9-aminoanthracycline, in patients with advanced non-small-cell lung cancer. Investig. New Drugs 23, 331–337. doi: 10.1007/s10637-005-1441-3
Sun, C., Yang, Z., Zhang, C., Liu, Z., He, J., Liu, Q., et al. (2019). Genome mining of Streptomyces atratus SCSIO ZH16: discovery of atratumycin and identification of its biosynthetic gene cluster. Org. Lett. 21, 1453–1457. doi: 10.1021/acs.orglett.9b00208
Takahashi, Y., and Nakashima, T. (2018). Actinomycetes, an inexhaustible source of naturally occurring antibiotics. Antibiotics 7:45. doi: 10.3390/antibiotics7020045
Tan, G. Y., and Liu, T. (2017). Rational synthetic pathway refactoring of natural products biosynthesis in actinobacteria. Metab. Eng. 39, 228–236. doi: 10.1016/j.ymben.2016.12.006
Thumar, J. T., Dhulia, K., and Singh, S. P. (2010). Isolation and partial purification of an antimicrobial agent from halotolerant alkaliphilic Streptomyces aburaviensis strain Kut-8. World J. Microbiol. Biotechnol. 26, 2081–2087. doi: 10.1007/s11274-010-0394-7
Tidjani, A. R., Lorenzi, J. N., Toussaint, M., van Dijk, E., Naquin, D., and Lespinet, O. (2019). Massive gene flux drives genome diversity between sympatric streptomyces conspecifics. mBio 10:e01533-19. doi: 10.1128/mBio.01533-19
Tokovenko, B., Rebets, Y., and Luzhetskyy, A. (2016). Automating assessment of the undiscovered biosynthetic potential of actinobacteria. bioRxiv [Preprint]. doi: 10.1101/036087
Tong, Y., Charusanti, P., Zhang, L., Weber, T., and Lee, S. Y. (2015). CRISPR-Cas9 based engineering of actinomycetal genomes. ACS Synth. Biol. 4, 1020–1029. doi: 10.1021/acssynbio.5b00038
Van Bergeijk, D. A., Terlouw, B. R., Medema, M. H., and van Wezel, G. P. (2020). Ecology and genomics of Actinobacteria: new concepts for natural product discovery. Nat. Rev. Microbiol. 18, 546–558. doi: 10.1038/s41579-020-0379-y
Ward, A. C., and Allenby, N. E. (2018). Genome mining for the search and discovery of bioactive compounds: the Streptomyces paradigm. FEMS Microbiol. Lett. 365:fny240. doi: 10.1093/femsle/fny240
Weber, T., and Kim, H. U. (2016). The secondary metabolite bioinformatics portal: computational tools to facilitate synthetic biology of secondary metabolite production. Synthetic Syst. Biotechnol. 1, 69–79. doi: 10.1016/j.synbio.2015.12.002
Wu, C., Zhu, H., van Wezel, G. P., and Choi, Y. H. (2016). Metabolomics-guided analysis of isocoumarins production by Streptomyces species MBT76 and biotransformation of flavonoids and phenylpropanoids. Metabolomics 12:90.
Wu, H., Liu, W., Shi, L., Si, K., Liu, T., Dong, D., et al. (2017). Comparative genomic and regulatory analysis of netamycin production of Streptomyces lydicus A02. Sci. Rep. 7:9114.
Wu, R. Y., Yanq, L. M., Yokoi, T., and Lee, K. H. (1988). Neihumicin, a new cytotoxic antibiotic from Micromonospora neihuensis. I. The producing organism, fermentation, isolation and biological properties. J. Antibiot. (Tokyo) 41, 481–487. doi: 10.7164/antibiotics.41.481
Xia, H., Li, X., Li, Z., Zhan, X., Mao, X., and Li, Y. (2020). The application of regulatory cascades in Streptomyces: yield enhancement and metabolite mining. Front. Microbiol. 11:406.
Ye, S., Brana, A. F., Gonzalez-Sabin, J., Moris, F., Olano, C., Salas, J. A., et al. (2018). New insights into the biosynthesis pathway of polyketide alkaloid argimycins P in Streptomyces argillaceus. Front. Microbiol. 9:252.
Yin, J., Hoffmann, M., Bian, X., Tu, Q., Yan, F., Xia, L., et al. (2015). Direct cloning and heterologous expression of the salinomycin biosynthetic gene cluster from Streptomyces albus DSM41398 in Streptomyces coelicolor A3(2). Sci. Rep. 5:15081. doi: 10.1038/srep15081
Zeng, H., Wen, S., Xu, W., He, Z., Zhai, G., Liu, Y., et al. (2015). Highly efficient editing of the actinorhodin polyketide chain length factor gene in Streptomyces coelicolor M145 using CRISPR/Cas9-CodA (sm) combined system. Appl. Microbiol. Biotechnol. 99, 10575–10585. doi: 10.1007/s00253-015-6931-4
Zhang, M. M., Wong, F. T., Wang, Y., Luo, S., Lim, Y. H., Heng, E., et al. (2017). CRISPR-Cas9 strategy for activation of silent Streptomyces biosynthetic gene clusters. Nat. Chem. Biol. 13, 607–609. doi: 10.1038/nchembio.2341
Zhou, M., Jing, X., Xie, P., Chen, W., Wang, T., Xia, H., et al. (2012). Sequential deletion of all the polyketide synthase and non ribosomal peptide synthatase ribosomal gene clusters and a 900 kb subtelomeric sequence of the linear chromosome of Streptomyces coelicolor. FEMS Microbiol. Lett. 333, 169–179. doi: 10.1111/j.1574-6968.2012.02609.x
Keywords: actinobacteria, genomics, antibiotics, secondary metabolites, biosynthetic gene cluster
Citation: Singh TA, Passari AK, Jajoo A, Bhasin S, Gupta VK, Hashem A, Alqarawi AA and Abd_Allah EF (2021) Tapping Into Actinobacterial Genomes for Natural Product Discovery. Front. Microbiol. 12:655620. doi: 10.3389/fmicb.2021.655620
Received: 19 January 2021; Accepted: 31 May 2021;
Published: 22 June 2021.
Edited by:
Byung-Kwan Cho, Korea Advanced Institute of Science and Technology, South KoreaReviewed by:
Juan F. Martin, Universidad de León, SpainCopyright © 2021 Singh, Passari, Jajoo, Bhasin, Gupta, Hashem, Alqarawi and Abd_Allah. This is an open-access article distributed under the terms of the Creative Commons Attribution License (CC BY). The use, distribution or reproduction in other forums is permitted, provided the original author(s) and the copyright owner(s) are credited and that the original publication in this journal is cited, in accordance with accepted academic practice. No use, distribution or reproduction is permitted which does not comply with these terms.
*Correspondence: Ajit Kumar Passari, YWppdC5wYXNzYXJpMjJAaWliaW9tZWRpY2FzLnVuYW0ubXg=; Sheetal Bhasin, c2hlZXRhbHJiaGFzaW5AZ21haWwuY29t
Disclaimer: All claims expressed in this article are solely those of the authors and do not necessarily represent those of their affiliated organizations, or those of the publisher, the editors and the reviewers. Any product that may be evaluated in this article or claim that may be made by its manufacturer is not guaranteed or endorsed by the publisher.
Research integrity at Frontiers
Learn more about the work of our research integrity team to safeguard the quality of each article we publish.