- 1Faculty of Agriculture and Natural Resources, University of Phayao, Muang Phayao, Thailand
- 2Department of Biology, Faculty of Science, Chiang Mai University, Chiang Mai, Thailand
- 3Research Center of Microbial Diversity and Sustainable Utilization, Chiang Mai University, Chiang Mai, Thailand
- 4Academy of Science, The Royal Society of Thailand, Bangkok, Thailand
Gummy stem blight and wilt are known to cause enormous losses to the global production of muskmelon (Cucumis melo). In this study, the potential of endophytic fungi isolated from leaves of Siam weed (Chromolaena odorata) was investigated for the inhibition of mycelial growth of Stagonosporopsis cucurbitacearum and Fusarium equiseti. Twenty-one fungal isolates were obtained. The results indicated that a fungal isolate UP-L1I3 displayed the highest percentage in terms of inhibition of the mycelial growth of F. equiseti and S. cucurbitacearum at 90.80 and 81.60%, respectively. Consequently, this isolate was selected for its potential ability to promote plant growth and control gummy stem blight and wilt in muskmelon seedlings. Morphological and multilocus phylogenetic analyses revealed that the isolate UP-L1I3 was a new species that has been described herein as Trichoderma phayaoense. Pathogenicity test confirmed that F. equiseti and S. cucurbitacearum were the cause of gummy stem blight and wilt disease in muskmelon seedlings, respectively. However, no disease symptoms were observed in seedlings inoculated with T. phayaoense. It was found that T. phayaoense could be used preventively in muskmelon seedlings that were inoculated with F. equiseti and S. cucurbitacearum, which could then reduce the impact on the disease severity index. T. phayaoense was also effective in improving plant development by increasing plant height, as well as shoot and root dry weight values. Moreover, T. phayaoense could effectively increase weight, diameter, and the circumference and total soluble solid of fruit without having a negative effect on fruit quality parameters. Additionally, T. phayaoense was able to tolerate a commonly applied fungicide (metalaxyl) in recommended dosages for field applications.
Introduction
Muskmelon (Cucumis melo L.) is one of the most popular edible fruits in the world. This fruit is rich in nutrients, minerals, and several other health-bioactive compounds that are beneficial to humans (Ismail et al., 2010; Parle and Singh, 2011). In 2018, the largest producer of melons (including muskmelons and cantaloupes) is produced in China at 1.27 million tonnes with global production at 2.73 million tonnes (FAOSTAT, 2020). Indonesia is the Southeast Asia’s leading melon producer, followed by the Lao People’s Democratic Republic and the Philippines. Nowadays, muskmelon production is an important economic activity in Thailand and the production area continues to increase. On the other hand, the incidence and severity of certain diseases have also increased when plantings occur at unsuitable locations. Plant pathogenic microorganisms (bacteria, fungi, and viruses) are known to cause diseases in muskmelons under the field before they are harvested, during harvesting and during storage, all of which can cause considerable yield loses (Walcott et al., 2004; Malik et al., 2010; Kehinde, 2013). Fungi in the genera Alternaria, Colletotrichum, Cladosporium, Fusarium, Penicillium, Phytophthora, and Stagonosporopsis have been reported to cause a range of leaf, fruit, stem, and/or root diseases in muskmelons (Li et al., 2015; Garampalli et al., 2016; Ghuffar et al., 2018). Gummy stem blight disease caused by Stagonosporopsis cucurbitacearum (synonym = Phoma cucurbitacearum and Didymella bryoniae), and wilt caused by Fusarium oxysporum f. sp. melonis, are important diseases associated with muskmelons and have been known to cause significant losses in yield and quality of this fruit (Gomez and Tello, 2000; Perchepied and Pitrat, 2004; Li et al., 2015; Garampalli et al., 2016). Other diseases that may pose a serious problem in muskmelons are anthracnose, powdery mildew, downy mildew, and necrotic spot virus (Kishi, 1966; Kristkova et al., 2009). Chemical fungicides are commonly used for the control of fungal pathogens. However, these chemical fungicides are known to be hazardous to farmers’ and consumers’ health, and environment (Lo, 2010; Yang, 2011). Many researchers have been interested in application of beneficial microorganisms, especially biological control agents in replacing chemical fungicides that can support the sustainability of the agriculture, produce safe food, and reduce environmental pollution (Cal et al., 2009; Wackett, 2013; Gava and Pinto, 2016).
Endophytic fungi are characterized by their ability to asymptomatically colonize in tissues of plants (Bacon and White, 2000; Rodriguez et al., 2009). Endophytic fungi benefit the host plant by enhancing growth, development, adaption, and stress tolerance (Carroll, 1988; Bacon and White, 2000; Rodriguez et al., 2009; Padhi et al., 2015). Interestingly, endophytic fungi have been shown to benefit their host by conferring protection against certain diseases resulting in reduced levels of infection, as well as suppression of the plant’s growth and/or a reduction in the amount of pathogens (Bacon and White, 2000; Gao et al., 2010; Selim et al., 2012). Several endophytic fungi, such as the Cladosporium, Colletotrichum, Fusarium, Penicillium, Pestalotiopsis, and Trichoderma species, have been used as attractive options for the management of some plant diseases (Bacon and White, 2000; Gao et al., 2010; Gava and Pinto, 2016). During our investigation of endophytic fungi in the Siam weed [Chromolaena odorata (L.) R. M. King & H. Rob.], we found Trichoderma to be a species of particular interest. Consequently, we have described this new species as Trichoderma phayaoense in this present paper. The abilities of this fungus as a potential biological agent to control S. cucurbitacearum and Fusarium equiseti has been noted in both in vitro and in vivo experiments. These pathogens are known to cause devastating gummy stem blight diseases and wilt in muskmelons, respectively. The in vivo potential of this fungus on plant growth was evaluated. Moreover, the ability of T. phayaoense to tolerate some commercial forms of fungicides, pesticides, and herbicides in the solid culture was investigated. The finding from this research study will be used to develop T. phayaoense as a biocontrol agent, which may then be used to replace the chemical fungicides that are currently being used.
Materials and Methods
Isolation of Endophytic Fungi
Endophytic fungi were isolated from the healthy leaves of Siam weed (C. odorata) collected from a native forest located on the campus of the University of Phayao, Phayao Province, Northern Thailand (19°1'42.6'' N, 99°53'38.9'' E) in May of 2015. Leaves were washed in running tap water for 15 min. The isolation of endophytic fungi was followed the method described by Nuangmek et al. (2008) with some modifications. The samples were then cut into small pieces (5 mm × 5 mm), then surface-disinfected by soaking in 75% ethanol for 30 s, 2% sodium hypochlorite solution for 3 min and 95% ethanol for 30 s. The sterilized samples were placed on potato dextrose agar (PDA; CONDA®, Spain) supplemented with 0.05% streptomycin sulfate and 0.03% rose Bengal in Petri plates. The isolation plates were incubated at 25°C in darkness until fungal growth was initiated. The tips of the fungal hyphae were aseptically removed and transferred to PDA. Each pure fungal isolate was kept on PDA slants for future use.
Fungal Pathogens
Two fungal pathogens, S. cucurbitacearum SDBR-CMU292 (Nuangmek et al., 2018) and F. equiseti SDBR-UP-PA002 (Nuangmek et al., 2019), were used in this study. Both fungal strains were obtained from the Sustainable Development of Biological Resources (SDBR) Laboratory, Faculty of Science, Chiang Mai University. Fungal cultures were cultivated on PDA plate and grown in darkness for 1 week at 25°C.
In vitro Test of Isolated Endophytic Fungi Against Mycelial Growth of S. cucurbitacearum and F. equiseti
Dual culture assay was used to test for inhibitory effects of the 21 isolates of endophytic fungi against F. equiseti SDBR-UP-PA002 and S. cucurbitacearum SDBR-CMU292 following the method described by Arnold et al. (2000). An agar plug (5 mm in diameter) of each endophytic fungus was cut from a 5-day-old colony on a PDA and was placed on one side of that PDA plate. A mycelial plug of pathogen was cut from a 5-day-old colony on PDA and was placed on the opposing side of the PDA plate. Plates were sealed with Parafilm M® (Sigma-Aldrich, United States) and then incubated at 25°C for 5 days. A mycelial plug of the pathogen was placed alone in the same manner on PDA plates serve as a control. The radial growth of pathogen colonies was measured. The percentage inhibition was calculated as has been previously described by Nuangmek et al. (2008) according to the following formula: [(A−B)/A] × 100 where A represents the radial growth of pathogens in the control and B represents the radial growth of pathogens after treatment. Three replicates were completed for each treatment, and experiments were repeated twice. The effective fungal isolate against the mycelial growth of both fungal pathogens was selected and used in future experiments.
Identification of Selected Endophytic Fungus
Morphological Studies
The morphological characteristics were used to initially identify the selected endophytic fungus. Colony characteristics e.g., mycelial density, mycelial texture, and pigment production on different were recorded (Shah et al., 2012; Chaverri et al., 2015; Jaklitsch and Voglmayr, 2015; Chen and Zhuang, 2017). Furthermore, a light compound microscope (Olympus CX51, Japan) was used to examine the micromorphological characteristics. Size, shape, and structure data associated with the relevant anatomical features were measured at least 50 measurements of each structure.
Molecular Studies
The identification of a selected fungus was confirmed by molecular phylogenetic analysis. Genomic DNA was extracted from fungal cultured on PDA for 3 days, using FavoPrep® DNA Extraction Mini Kit (Taiwan). The internal transcribed spacers (ITS) was amplified with primers ITS4/ITS5 (White et al., 1990), the second largest subunit of RNA polymerase II (rbp2) genes was amplified by primers fRPB2-5f/fRPB2-7cr (Lui et al., 1999) and the translation elongation factor 1-alpha (tef-1) gene was amplified using primers EF1-728F/EF1-986R (Carbone and Kohn, 1999). The amplification program was conducted by running for 35 cycles for all gene regions. The initial denaturation at 95°C for 5 min, denaturation at 95°C for 30 s, annealing at 52°C for 30 s (ITS), 54°C for 45 s (rbp2), 52°C for 1 min (tef-1), extension at 72°C for 1 min, and a final cycle at 72°C for 10 min. The amplified PCR products were then checked by electrophoresis on 1% agarose gel stained with ethidium bromide and detected under UV light. The products were purified using NucleoSpin Gel and PCR Clean-up Kit (Macherey-Nagel, Germany), and then sent to a commercial sequencing provider (1ST BASE Company, Kembangan, Malaysia). The obtained sequences were subjected to BLASTn search in GenBank.1
For phylogenetic analysis, the sequences obtained from this study and from previous studies, along with the sequences obtained from the GenBank database, were used (Supplementary Table S1). Multiple sequence alignment was carried out using MUSCLE (Edgar, 2004). The finalized alignment of a combined ITS, rbp2, and tef-1 alignments in this study were submitted to TreeBASE under the study ID 27012. Trichoderma ceramicum and T. parestonicum were used as the outgroup. A phylogenetic tree was constructed using maximum likelihood (ML) and Bayesian inference (BI) methods. The best substitution models for ML and BI analyses were estimated by Akaike Information Criterion (AIC) in jModeltest 2.1.10 (Darriba et al., 2012). ML analysis was carried out on RAxML v7.0.3 under the GTR+I+G model with 1,000 bootstrap replications (Stamatakis, 2006). BI analysis was conducted with MrBayes v3.2.6 (Ronquist et al., 2012) to evaluate the posterior probabilities (PP) by Markov chain Monte Carlo sampling (MCMC). Markov chains were run for 1 million generations and trees were sampled every 100th generation and 10,000 trees were obtained. The first 2,000 trees representing the burning phase of the analyses were discarded. The remaining 8,000 trees were then used to calculate PP in the majority rule consensus tree. Bootstrap support (BS) and PP values greater than or equal to 70% and 0.95, respectively, of each branch were considered to be significantly supported (Felsenstein, 1985; Alfaro et al., 2003). The phylogenetic species were delimited in this study according to the genealogical concordance phylogenetic species recognition (GCPSR) criterion (Taylor et al., 2000). Using this method, phylogenetic species were recognized as genealogically exclusive under GCPSR if they were concordantly supported by multiple independent loci.
Pathogenicity Test
For the pathogenicity test, selected endophytic fungus, S. cucurbitacearum SDBR-CMU292 and F. equiseti SDBR-UP-PA002, were evaluated for their ability to act as causal agents of diseases in muskmelons. Seeds of muskmelons (C. melo var. inodorus) were soaked in sterilized distilled water overnight. Commercial soil (Sunantha Nursery Company, Thailand) was used with a pH value of 6.5–6.8. The soil was sterilized at 121°C for 60 min. After cooling for 24 h, the sterilized soil was added into each 7 cm × 7.5 cm pot. Seeds were then placed in each pot. The experiment was conducted in a greenhouse (30 ± 2°C) located at the Faculty of Agriculture and Natural Resources, University of Phayao from February to March in 2018. The temperature, relative humidity, and maximum daily-light intensity ranged from 30 to 34°C, 60 to 75%, and 13,500 to 57,000 lux, respectively. Water was added every other day. After 2 weeks, seedlings were transferred into each 14 cm × 19 cm pot containing sterilized soil. Seedlings were then continuously grown for 1 week and used in the pathogenicity test. A conidial suspension of each fungus was prepared following the method described by Li and Brewer (2016). Fungal cultured on PDA for 2 weeks was flooded with a sterile 0.05% (v/v) Tween 80 and 0.85% (w/v) NaCl aqueous solution. The conidial suspension was filtrated through two layers of sterilized cheesecloth. The conidia concentration was adjusted by using a hemocytometer to a final concentration of approximately 1 × 106 conidia/ml. Inoculation was done by addition of 10 ml conidial suspension onto the soil surface around the base of the seedlings. Seedlings treated with sterile distilled water served as control. Disease symptoms were observed. Ten replicates per each treatment were generated. All treatments were repeated twice. The re-isolation of fungi from any lesions that developed on inoculated plants was performed by the single spore isolation method as described by Choi et al. (1999) in order to complete Koch’s postulates.
Plant Growth Promotion and Control of Gummy Stem Blight and Wilt in Muskmelon With a Selected Endophytic Fungus in Greenhouse
Seedlings
Seedlings of muskmelons were prepared following the method described above. One two-week-old seedling was transferred into each plastic bag (20.32 cm × 33.02 cm) containing sterilized commercial soil. Seedlings were continuously grown for 2 weeks and were then used in this experiment.
Fungal Inoculum Preparation
A selected endophytic fungus and fungal pathogens (S. cucurbitacearum SDBR-CMU292 and F. equiseti SDBR-UP-PA002) were cultivated on PDA. A conidial suspension of each fungus was prepared following the method described above. The final conidial concentration was adjusted to 1 × 106 conidia/ml before being used.
Experimental Design
Six treatments were tested to establish the effectiveness of the selected endophytic fungus on controlling gummy stem blight and wilt in muskmelons under greenhouse conditions (Supplementary Table S2). Fifteen milliliters of conidial suspension of a selected endophytic fungus were poured onto soil surface around seedlings. Sterile distilled water was used as a control. After 1 week of inoculation with a selected endophytic fungus, 10 ml of conidial suspension of each pathogenic fungus was added onto soil surface around seedlings. All treatments were conducted in a greenhouse from March to May in 2018. The temperature, relative humidity, and maximum daily-light intensity ranged from 32 to 36°C, 60 to 70%, and 14,500 to 59,000 lux, respectively. Water was added every other day. The symptoms of gummy stem blight and wilt were observed after 5 weeks of incubation. The disease severity index (DSI) for each pathogen was evaluated. For F. equiseti, the DSI was applied by following the description of Boughalleb et al. (2007) with some modifications to the scales: 0 = healthy; 1 = slight yellowing of leaves with slight rot pivot and lateral roots and crown rot; 2 = significant yellowing in leaves with or without wilting, stunting of plants, severe rot at the pivot and lateral roots, significant rot, and browning of vessels in the stem; and 3 = death of the plant. The DSI of S. cucurbitacearum was applied followed the method described by Dalcin et al. (2017) with slight modifications to the scales: 0 = healthy; 1 = plants with less than 1% of damaged leaf area; 2 = plants between 1 and 5% of damaged leaf area; 3 = plants between 6 and 25% damaged leaf area; 4 = pants between 26 and 50% of damaged leaf area; 5 = plants showing more than 50% of damaged leaf area. An experiment was conducted as a completely randomized design (CRD) with 10 replications each. All treatments were repeated twice.
Measurement of Plant Growth and Fruit Production
Plant height, the number of leaves, the dry weight of the shoot (including the leaves) and the dry weight of the roots were recorded during incubation periods. After 5 weeks of incubation, the fruit of the plants reached the maturity stage for harvesting. In this study, the number of fruits for all plants was set at one fruit per plant. Fruit weight, diameter of the fruit, circumference of the fruit, and fruit quality parameters (total soluble solid, total titratable acidity, and fruit firmness values) were measured.
Fungicide, Herbicide, and Insecticide Tolerance of Selected Endophytic Fungus
Three commercial fungicides, metalaxyl (Metalaxyl 35 W®, Thailand), pyraclostrobin (Headline®, Thailand), and copper hydroxide (Funguran-OH®, Thailand), one insecticide, carbamate (Carbaryl 85®, Thailand), and one herbicide, paraquat dichloride (Grammoxone®, Thailand) were tested in this experiment. The aqueous stock solution of metalaxyl, pyraclostrobin, copper hydroxide, carbamate, and paraquat dichloride were prepared at 35000, 55000, 75,000, 85,000, and 1,000 ppm, respectively, according the method described by Suwannarach et al. (2015). The recommended field dosages of metalaxyl, pyraclostrobin, copper hydroxide, carbamate, and paraquat dichloride were 500, 500, 1,000, 1,000, and 6.25 ppm, respectively. The aqueous stock solution of each compound added to a sterilize PDA at final concentrations of half recommended dosages to quintuple the recommended dosage. The surface of the test media was covered by a sterile cellophane disc, and the mycelial plug (5 mm in diameter) of the selected endophytic fungus was placed onto the test media. The plates were incubated at 25°C in darkness. After 2 weeks of incubation, the cellophane disc was removed and dried at 60°C for 48 h. Then, mycelium dry weights were measured. The tolerance index (TI) was calculated following the formula described by Fomina et al. (2005). TI values at 0% indicated a lethal effect and lower than 50% indicated a growth inhibition effect. Four replicates were made for each treatment.
Statistical Analysis
The data were analyzed by one-way analysis of variance (ANOVA) using SPSS program version 16.0 for Windows. The significant differences (p ≤ 0.05) between the mean values of each treatment were determined using Duncan’s multiple range test.
Results
Inhibitory Effects of Endophytic Fungi Against Fungal Pathogens
Twenty-one fungal isolates were recovered from the leaves of the Siam weed. The results showed that the fungal isolate UP-L1I3 showed the highest degree of percentage for the inhibition of mycelial growth of F. equiseti SDBR-UP-PA002 and S. cucurbitacearum SDBR-CMU292 at 90.80 and 81.60%, respectively, by dual culture assay (Table 1; Figure 1). This observation was informed by measurements of growth. An overgrowth of fungal isolate UP-L1I3 on both fungal pathogens was observed. It could be explained that the inhibition effect was parasitism. Therefore, the isolate UP-L1I3 was selected and used for future experiments. This fungal isolate was deposited at SDBR Laboratory and Thailand Bioresource Research Center (TBRC) under number SDBR-CMU349 and TBRC10852, respectively.
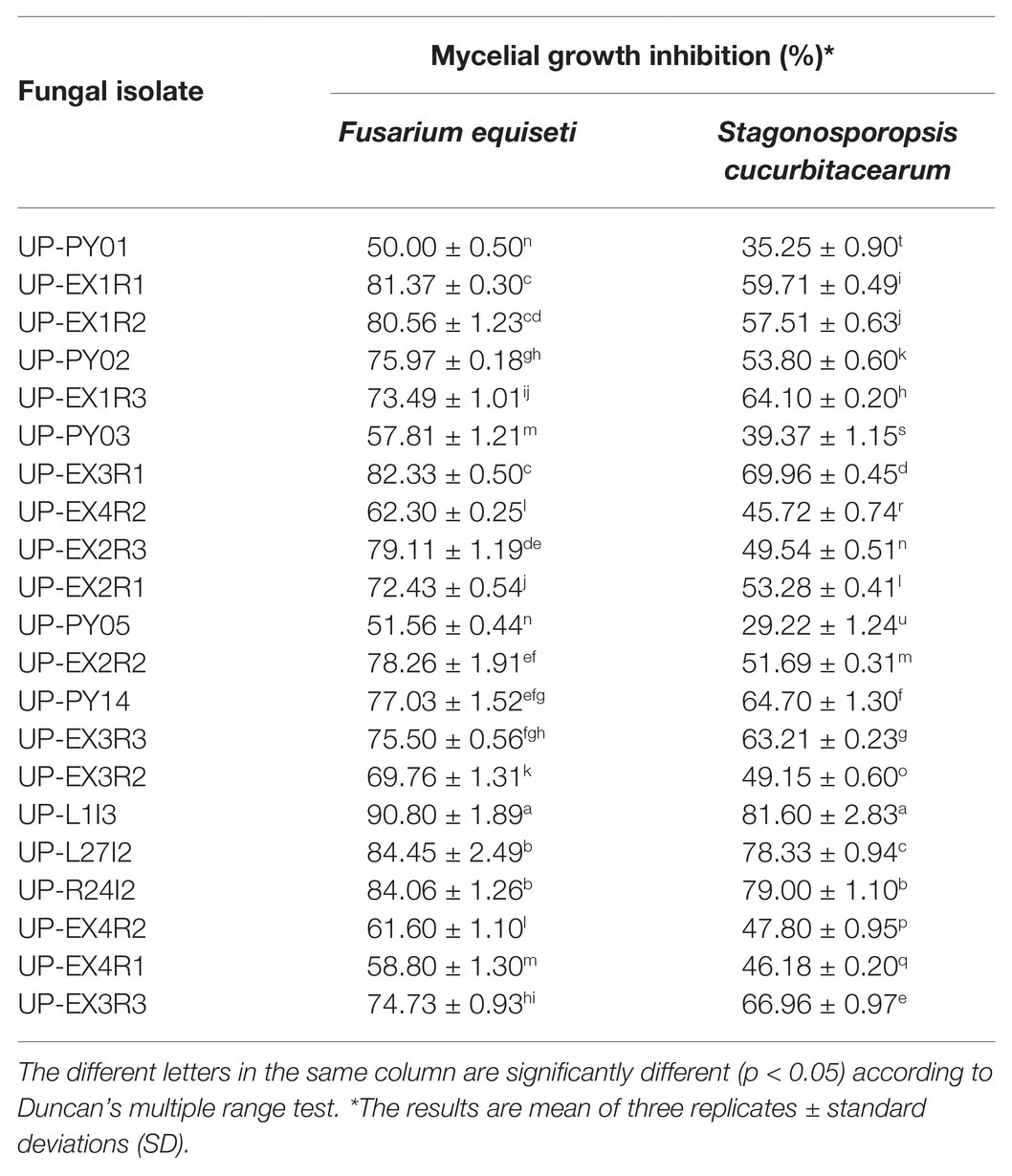
Table 1. Mycelial growth inhibition of 21 fungal isolates against F. equiseti and S. cucurbitacearum assessed by dual culture assay.
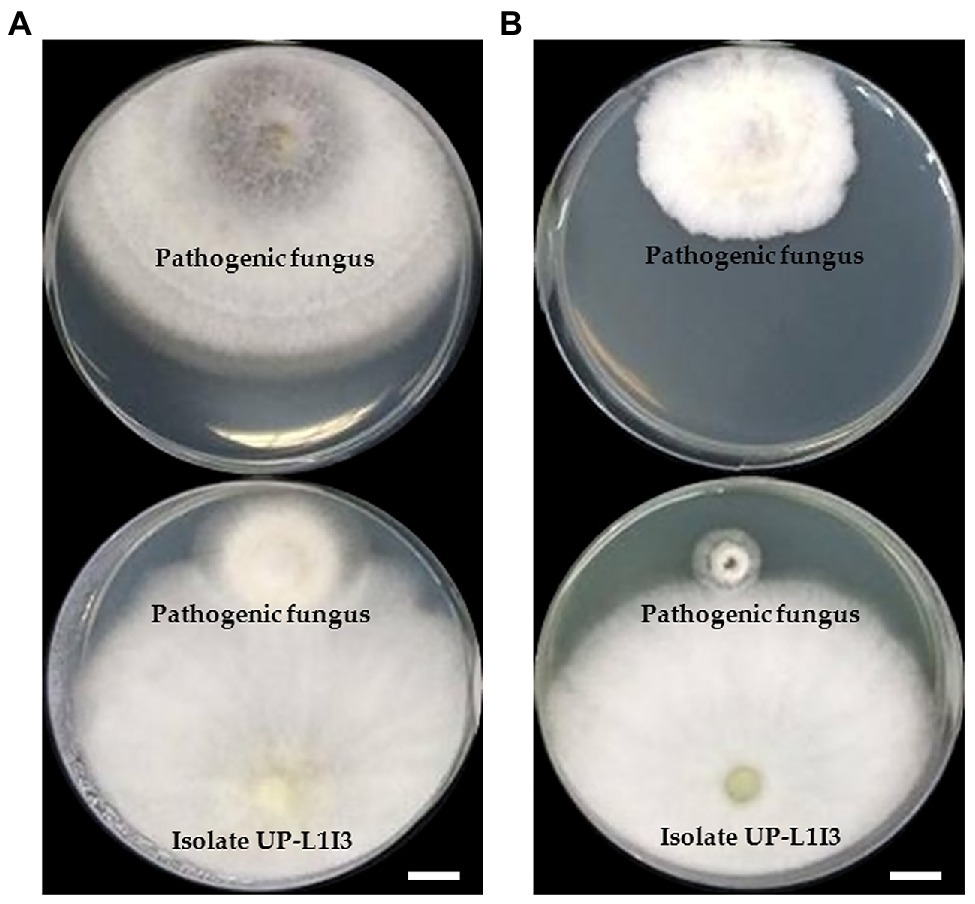
Figure 1. Dual culture assay of an endophytic fungus isolate UP-L1I3 against mycelia growth of Fusarium equiseti (A) and Stagonosporopsis cucurbitacearum (B) on potato dextrose agar (PDA) after 5 day of incubation at 25°C in darkness. The upper plate was a control plate, and the lower pate was an experimental plate. Scale bars = 10 mm.
Identification of Selected Endophytic Fungus
Morphological Observation
Fungal colonies of isolate UP-L1I3 on corn meal dextrose agar (CMD), PDA, and synthetic nutrient-poor agar (SDN) were reached 32–33, 30–34, and 30–32 mm in diameter at 25°C for 3 days, respectively. The conidia production was observed in all agar media. The fungal isolate UP-L1I3 produced conidiophores often on long main axis, ampulliform to lageniform phialie (3.2–3.3 length/wide ratio), smooth globose to subglobose with rarely broadly ellipsoid conidia and chlamydospores. The fungal isolate UP-L1I3 was initially identified as belonging to the Trichoderma harzianum species complex based on the morphological observations (Gams and Meyer, 1998; Samuels, 2006; Chaverri et al., 2015). Therefore, molecular methods were applied to confirm the identification of this fungal isolate.
Phylogenetic Results
The sequences of ITS, rbp2, and tef-1 obtained from fungal isolate UP-L1I3 (SDBR-CMU349) were deposited in GenBank database under the accession numbers MT995122, MW002073, and MW002074, respectively (Supplementary Table S1). The combined ITS, rpb2, and tef-1 sequence dataset consisted of 58 taxa, while the aligned dataset was comprised of 3,031 characters including gaps (ITS: 1–608, rpb2: 609–1,693, and tef-1: 1694–3,031). The average SD of the split frequencies of BI analysis was 0.030270. ML analysis revealed the proportion of invariable sites and a gamma distribution with shape parameters for the rates of nucleotide substitution among the variable sites at 0.7570 and 0.5560, respectively. The tree with a final log likelihood value of −14876.59 was obtained. The phylogram of ML and BI analyses were similar in topology. Therefore, we have only presented the phylogram obtained from ML analysis (Figure 2). Our phylogenetic tree was constructed concordantly and was supported by previous studies (Chaverri et al., 2015; Jaklitsch and Voglmayr, 2015; Chen and Zhuang, 2017). A phylogram assigned the fungal isolate UP-L1I3 as a new species which described herein as T. phayaoense into the Harzianum calde. Trichoderma phayaoense was clearly distinguished from other Trichoderma species in the Harzianum clade and formed a sister taxon to Trichoderma lixii with high bootstrap (99%) and posterior probability (1.0) supports. According to the genealogical concordance phylogenetic species recognition criterion of Taylor et al. (2000), T. phayaoense, in this study, should be recognized as belonging to an independent phylogenetic species.
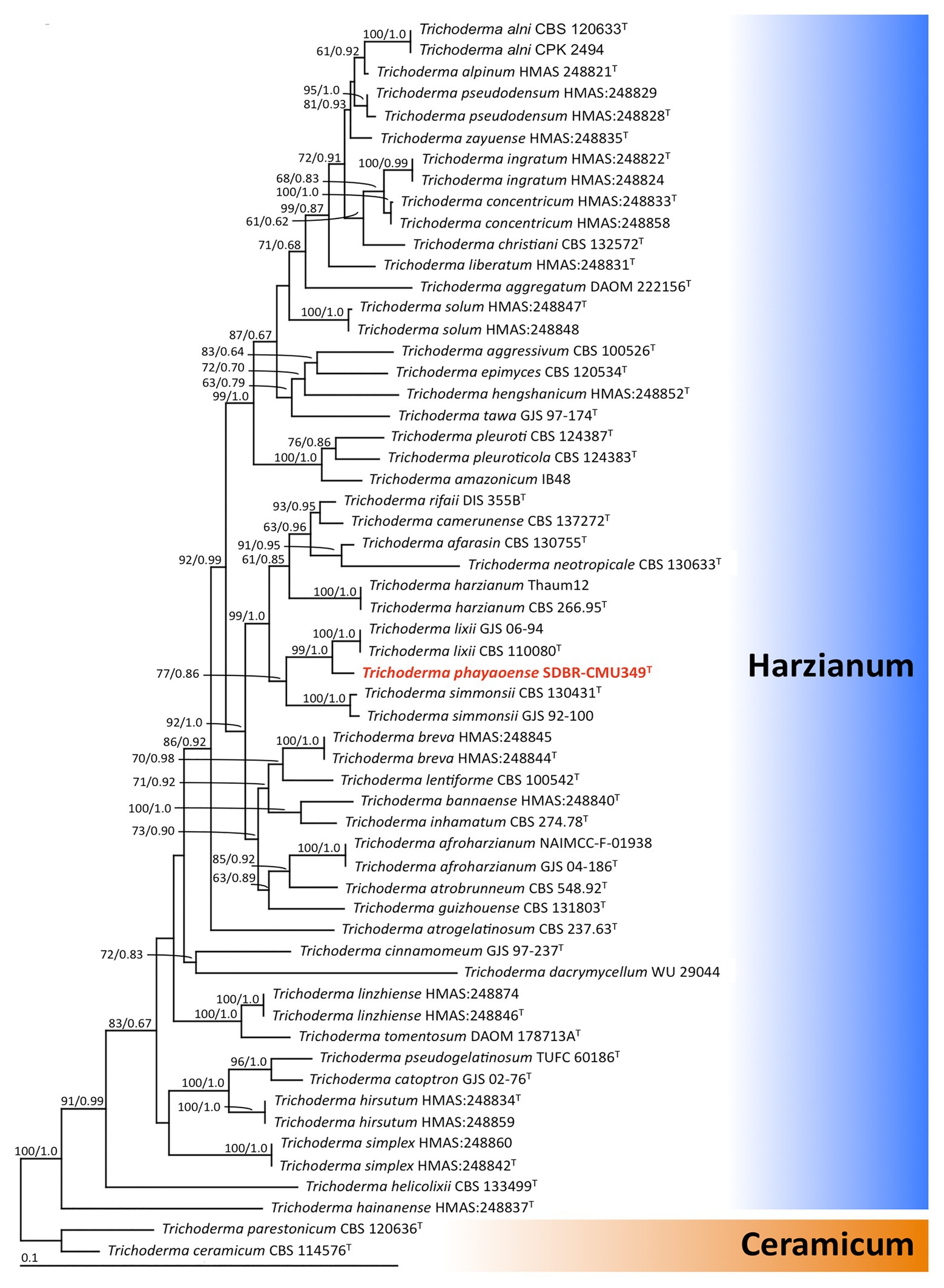
Figure 2. The phylogenic tree obtained by maximum likelihood analysis of the combined ITS, rpb2, and tef-1 genes of 58 specimens of Trichoderma. Trichoderma ceramicum, and Trichoderma parestonicum were used as an outgroup. The set of numbers above the nodes are BS value (left) and PP value (right) expressed values above 50% and 0.95, respectively. Superscription “T” means the type species and the fungal species obtained in this study is in red.
Taxonomic Description
Trichoderma phayaoense W. Nuangmek & N. Suwannarach sp. nov. Figure 3.
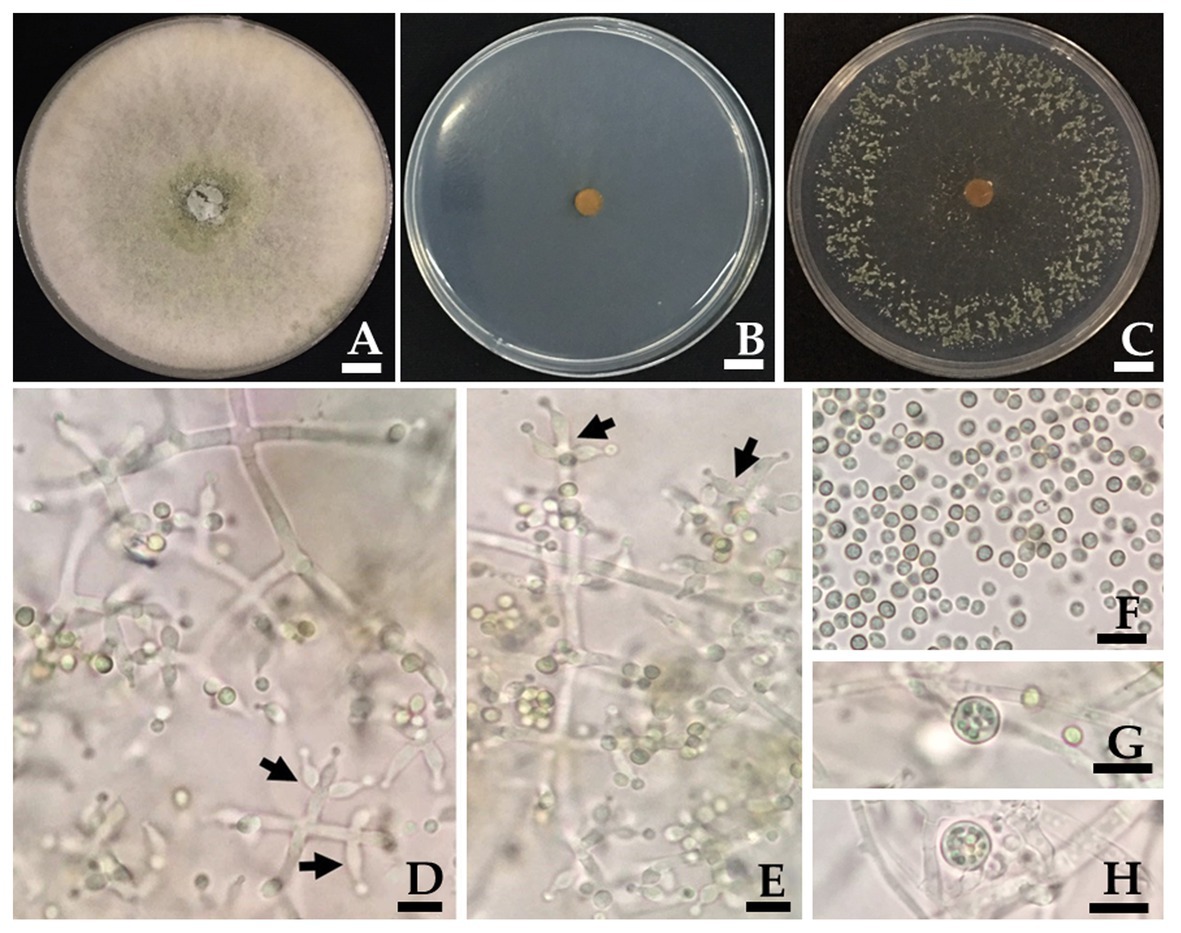
Figure 3. Trichoderma phayaoense SDBR-CMU349 (holotype). Colony on PDA (A), corn meal dextrose agar (CMD; B) and SNA (C) after 5 days of incubation at 25°C in darkness. (D,E) Phialide (arrows point), (F) Conidia, and (G,H) Chlamydospores. Scale bars (A–C) = 10 mm, (D–H) = 10 μm.
MycoBank no.: 837510.
Etymology: “phayaoense” referring to Phayao Province, Thailand, which the new fungus was isolated from C. odorata.
Holotype: THAILAND. Phayao Province, Mueang District, (19°1'42.6''N, 99°53'38.9508''E), isolated from C. odorata in deciduous dipterocarp forest of The University of Phayao, May 2015, W. Nuangmek, dried cultures: SDBR-CMU349; ex-type living culture: TBRC10852.
Gene sequences: MT995122 (ITS), MW002073 (rpb2), and MW002074 (tef-1).
Culture characteristics: Fungal colonies on PDA, CMD and SDN reached 30–34, 30–32, and 32–33 mm in diameter at 25°C for 3 days, respectively, while mycelium covered the plates after 5 days of incubation. Colonies on PDA were circular, dense, aerial hyphae abundant but loosely disposed, forming a white mat, becoming floccose to granular in the center and turning pale yellowish green (Figure 3A). Colonies on CMD were circular, flat, homogeneous, and mycelium loose (Figure 3B). Colonies on SNA were circular but not dense, surface becoming downy due to numerous long aerial hyphae except in the center (Figure 3C). No distinct odor, while no diffusing pigment was observed in all agar media. Conidiation began after 3 days in all agar media and were numerous and effused on aerial hyphae. Conidiophores symmetrical, trichoderma-like, often with a long main axis up to 150 μm, side branches short with no rebranching. Phialides formed solitary, paired or in whorls of 3, ampulliform to lageniform, 6.7–9.8 × 2.0–3.0 μm, 3.2–3.3 length/wide ratio, 1.0–1.5 μm wide at the base (n = 50; Figures 3D,E). Conidia green, smooth, globose to subglobose, rarely broadly ellipsoid, 2.0–3.9 × 2.0–2.9 μm (n = 50), and 1.0–1.3 length/wide ratio (Figures 3D–F). Chlamydospores were only observed in PDA and were terminal and intercalary of hyphae, globose, or pyriform 5.9–7.8 × 4.9–7.8 μm (n = 50; Figures 3G,H).
Habitat: Isolated from leaves of C. odorata in deciduous dipterocarp forest.
Geographic distribution: Know only from Thailand.
Morphologically, T. phayaoense is characterized by its developed conidiophores and its often long main axis. Furthermore, it possesses ampulliform to lageniform phialie with 3.2–3.3 length/width ratio. It is characterized as smooth globose to subglobose with rarely broadly ellipsoid conidia and the presence of chlamydospores. Thus, these morphological characteristics support its placement within the T. harzianum complex (Gams and Meyer, 1998; Samuels, 2006; Chaverri et al., 2015). The characteristics of the colonies of T. phayaoense are similar to those of Trichoderma afarasin, T. harzianum, Trichoderma lentiforme, T. lixii, and Trichoderma simmonsii. The size of the conidia and phaialde, and the degrees of chlamydospore production and geographic distribution of T. phayaoense, were also compared to other related Trichoderma species (Table 2). Chlamydospores were observed in cultures of T. phayaoense and were similar to those of T. afarasin, T. harzianum, and T. simmonsii (Blanchette, 1991; Shah et al., 2012; Chaverri et al., 2015). However, the width of the phialide of T. phayaoense was narrower than that of other related species. The phialide length of T. afarasin (5.3–6.0 μm), T. lentiforme (5.3–5.5 μm), T. lixii (5.3–6.0 μm), and T. simmonsii (6.1–6.5 μm) was distinguishable from our new species (6.7–9.8 μm; Chaverri et al., 2015). Moreover, the width of conidia of T. phayaoense (2.0–2.9 μm) was found to be narrower than T. lixii (3.1–3.2 μm) and T. simmonsii (3.0–3.1 μm wide; Chaverri et al., 2015). Multi-locus phylogenetic analysis confirmed that T. phayaoense clearly separated it from other Trichoderma species within the Harzianum calde. Consequently, it forms a sister taxon to T. lixii (Figure 2). Trichoderma lixii differs from T. phayaoense by the absence of chlamydospores and the different phialide and conidia sizes of T. phayaoense and T. lixii that have been mentioned above (Chaverri et al., 2015).
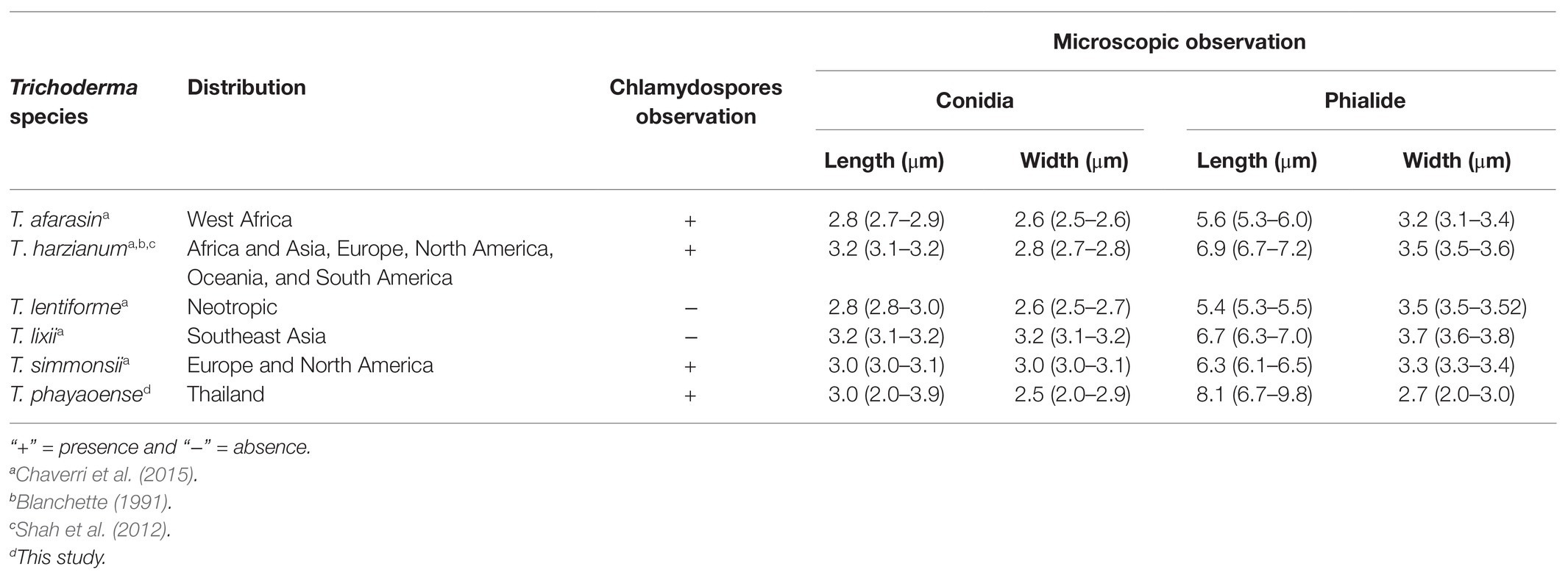
Table 2. Distribution, chlamydospores observation, and microscopic observation of Trichoderma phayaoense with the closely related species.
Pathogenicity Test
It was found that no disease symptoms were observed on the seedlings inoculated with T. phayaoense and the control seedlings. Therefore, T. phayaoense was determined to be non-pathogenic to the muskmelon plant. However, disease symptoms of gummy stem blight developed on the inoculated seedlings after 2 weeks of incubation. The initial symptoms of the gummy stem blight appeared on the stems by brown to dark brown spots and develop into elliptical- to oblong-shaped lesions. Subsequently, stem cankers developed in the cortical tissue, water soaked, and the presence of brown gummy exudates formed on the surface. Cankers expanded, while wilted leaves of the vines became blighted and desiccated. Additionally, initial symptoms of wilt were observed after 2 weeks of incubation, wherein leaves turned yellow or dull green and became brown and dry followed by the death of the lateral branches. A vascular brown discoloration appeared on the stem. Fusarium equiseti and S. cucurbitacearum were also isolated from the lesions of wilt and gummy stem blight, respectively.
Plant Growth Promotion and Control of Gummy Stem Blight and Wilt in Muskmelon With T. phayaoense
Six treatments were tested to establish the effectiveness of the selected endophytic fungus on controlling gummy stem blight and Fusarium wilt in muskmelons under greenhouse conditions. The disease severity index (DSI) of each treatment is shown in Table 3. After 5 weeks in a greenhouse, both conidial inoculations of F. equiseti (T4) and S. cucurbitacearum (T5) were found to produce the highest DSI values. In contrast, there was no lesion development on the seedling control (T0) and on the seedlings treated with T. phayaoense (T3). Furthermore, T. phayaoense significantly decreased DSI values in terms of both wilt and gummy stem blight of the seedlings (T1 and T2). After 5 weeks, muskmelon seedlings from each experiment were measured for shoot height, the number of leaves, the dry weight of shoots, and the dry weight of roots. The shoot height, number of leaves, shoot dry weight, and root dry weight of the seedlings in F. equiseti (T4) and S. cucurbitacearum (T5) treatments were significantly lower than in all other treatments (Figure 4). It was found that the T. phayaoense treatment (T3) did increase shoot height, shoot dry weight, and root dry weight when compared to the control (T0). It was also found that muskmelon seedlings treated with T. phayaoense and inoculated with F. equiseti (T1) and S. cucurbitacearum (T2) displayed increases in shoot height, shoot dry weight, and root dry weight when compared to the control.
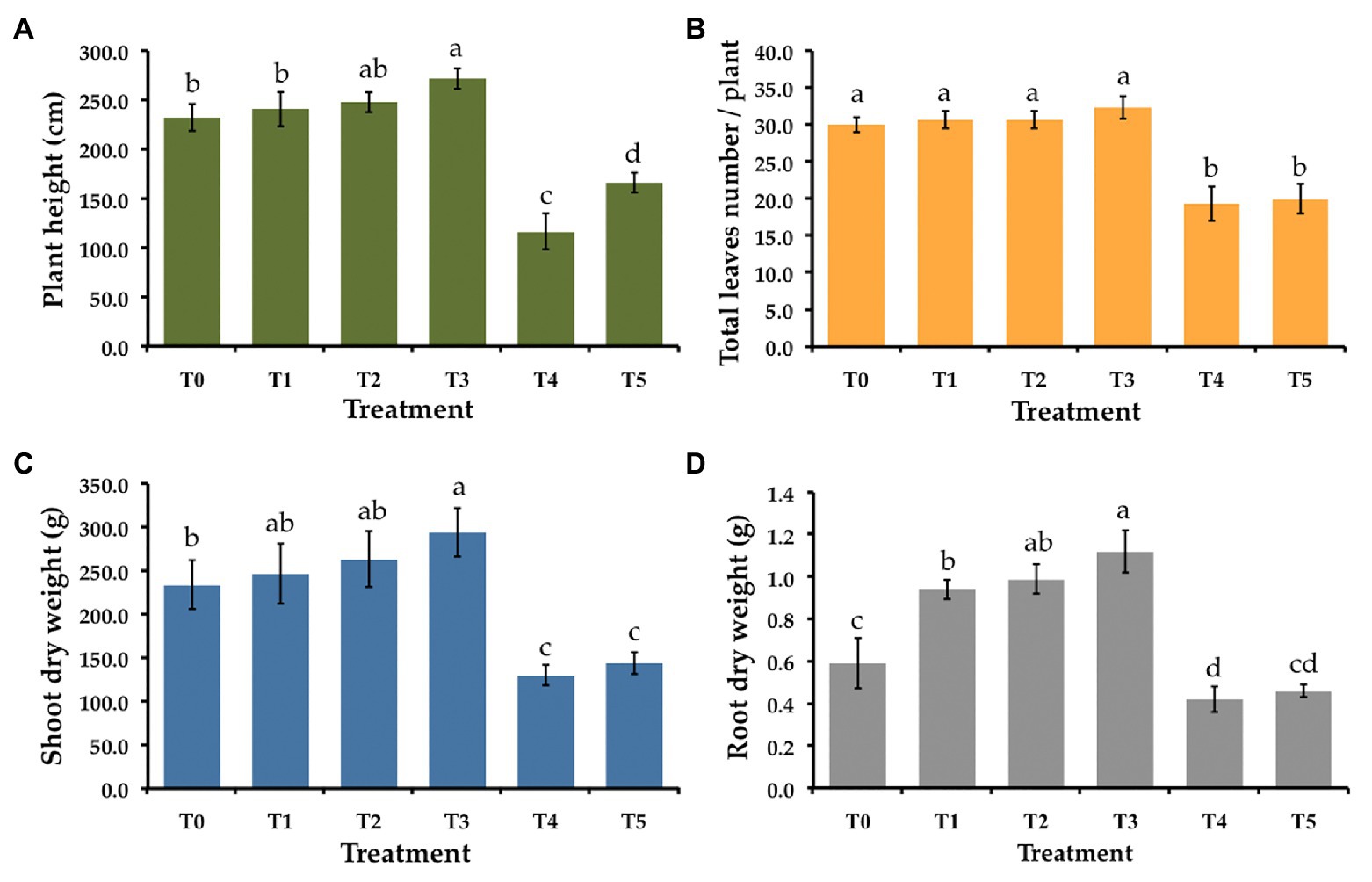
Figure 4. Effect of T. phayaoense, F. equiseti, and S. cucurbitacearum treatments on the growth of muskmelon plant. (A) Plant height, (B) total leaves number per plant, (C) shoot dry weight, and (D) root dry weight. Error bars represent standard deviation of the mean. Different letter indicate the significantly different at p ≤ 0.05.
Weight, diameter, circumference, total solid soluble values, total titratable acidity, and fruit firmness of all muskmelon fruits in each treatment were measured and the data are shown in Figure 5. The value of weight, diameter, and circumference of fruits in both F. equiseti (T4) and S. cucurbitacearum (T5) treatments were significantly lower than in other treatments. The results indicated that the fruits receiving the T. phayaoense treatment (T3) displayed higher values in terms of weight, diameter, and circumference when compared to the control (T0). Additionally, the fruits of the seedlings treated with T. phayaoense and inoculated with F. equiseti (T1) and S. cucurbitacearum (T2) displayed higher values in terms of weight, diameter, and circumference when compared to the control (T0), but these values were not found to be significantly different. The result showed that the fruits receiving the T. phayaoense treatment (T3) have the highest value of total soluble solid. Notably, total titratable acidity and fruit firmness in all treatments were not found to be significantly different.
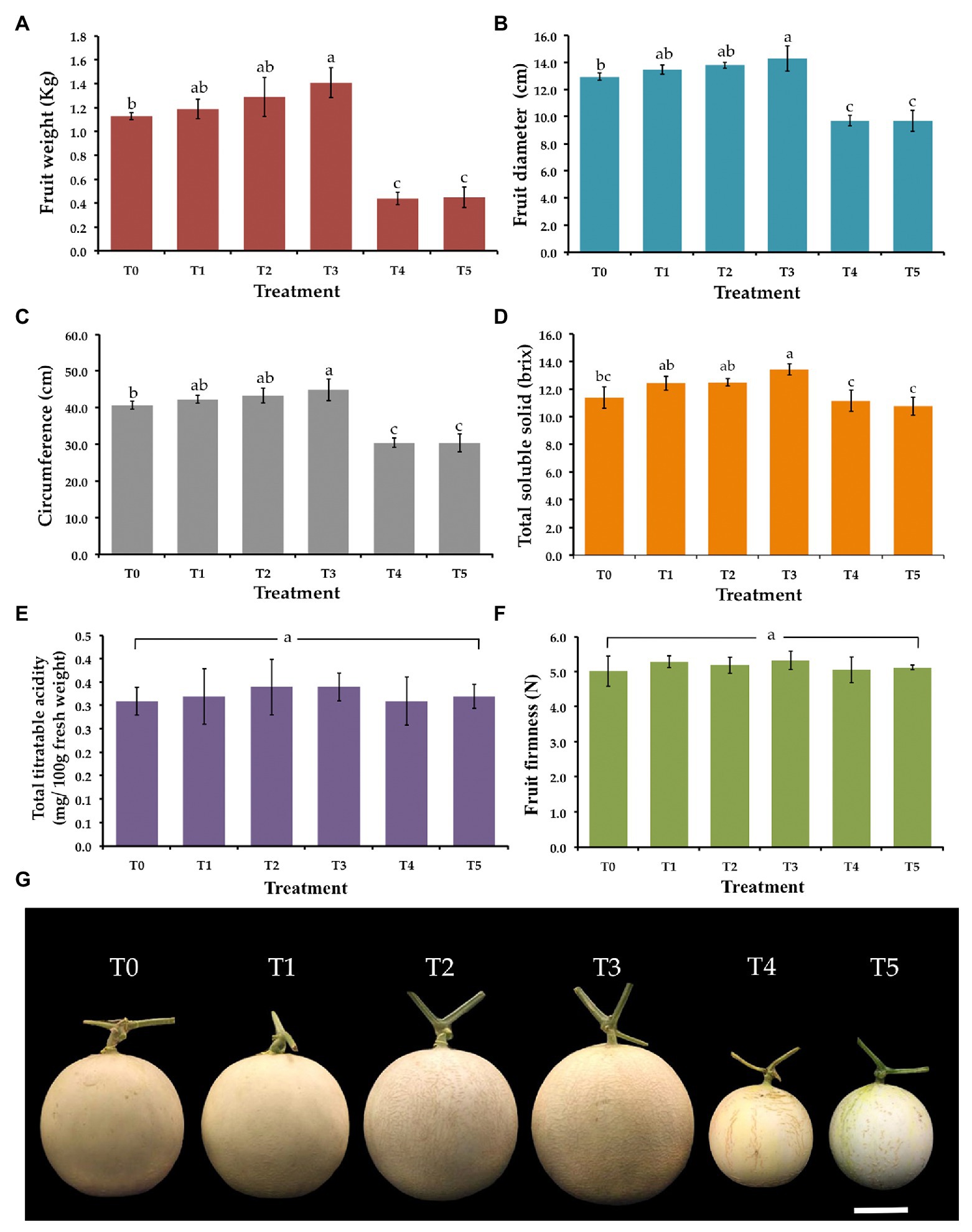
Figure 5. Effect of T. phayaoense, F. equiseti, and S. cucurbitacearum treatments on the fruit of muskmelon. (A) Fruit weight, (B) fruit diameter, (C) circumference, (D) total soluble solids, (E) total titrable acidity, (F) fruit firmness, and (G) muskmelon fruits in each treatment. Error bars represent SD of the mean. Error bars represent standard deviation of the mean. Different letter indicate the significantly different at p ≤ 0.05. Scale bar = 5 cm.
Fungicide, Herbicide, and Insecticide Tolerance of T. phayaoense
The fungicide, herbicide, and insecticide tolerance capabilities of T. phayaoense were reported in terms of a TI value and are showed in Figure 6. It was found that the TI value decreased when the concentration of all chemical compounds increased. The result indicated that the growth of T. phayaoense was inhibited (TI value < 50%) by pyraclostrobin and carbamate in all tested dosages. It was found that this fungus was tolerant (IT value > 50%) in recommended dosages of metalaxyl and in half-recommended dosages of copper hydroxide and paraquat dichloride.
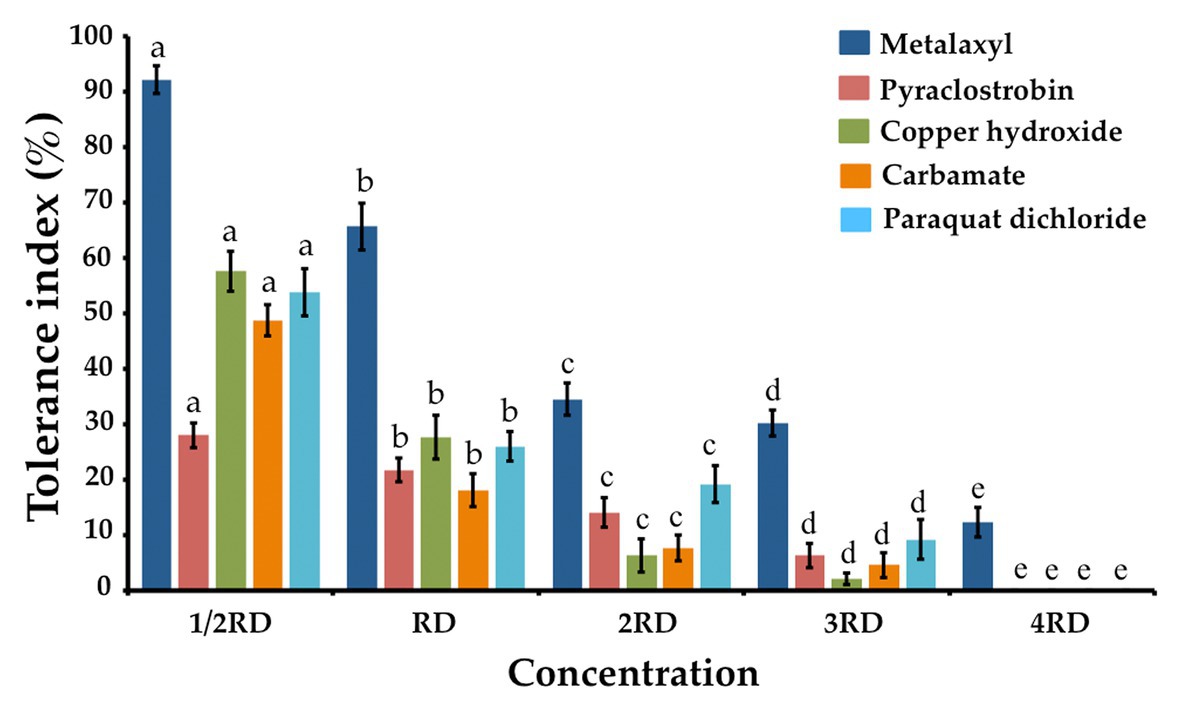
Figure 6. Tolerance fungicide, herbicides and insecticide index of T. phayaoense. Data were means of four replicates. Error bars represent SD of the mean. Different letter indicate the significantly different at p ≤ 0.05. 1/2 RD, RD, 2RD, 3RD, and 4RD indicate the half recommended dosage, recommended dosage, double recommended dosage, triple recommended dosage and quadruple recommended dosage, respectively.
Discussion
This study constitutes an investigation of the potential ability of endophytic fungi obtained from Siam weed leaves collected from Northern Thailand in the control of gummy stem blight and wilt diseases in muskmelon seedlings. The fungal isolate UP-L1I3 was selected and used based on the determination that this isolate displayed the highest percentage values for inhibition of the mycelial growth of F. equiseti and S. cucurbitacearum. The present study has identified the fungal isolate UP-L1I3 as a new endophytic fungal species of Trichoderma, which has been described herein as T. phayaoense based on a combination of its morphological characteristics and an extensive multilocus (ITS, rbp2, and tef-1 genes) phylogenetic analysis (Chaverri et al., 2015; Jaklitsch and Voglmayr, 2015; Chen and Zhuang, 2017). Several previous studies have reported that the Trichoderma species have been found on a wide variety of substrates and have been isolated from rotting plant material, soil, and other organisms (Samuels, 2006; Jaklitsch, 2009; Jaklitsch and Voglmayr, 2015; Chen and Zhuang, 2017). Recently, it has been recognized as one of the most commonly isolated species of endophytes in many plants (Samuels, 2006; Jaklitsch, 2009; Chaverri and Samuels, 2013; Thapa et al., 2020). In this study, T. phayaoense could reduce the degree of mycelial growth of S. cucurbitacearum and F. equiseti in the dual culture assay through the parasitism effect. These results are similar to those of previous studies (Ru and Di, 2012; Sánchez-García et al., 2017; Chounhary and Ashraf, 2019; Haque et al., 2020) which reported that the Trichoderma species could inhibit the mycelial growth of several plant pathogenic fungi by the parasitism, antifungal production, and a competition for space and nutrients. Additionally, the inhibition efficiency was varied among Trichoderma species and strains, as well as being dependent upon the species of fungal plant pathogens (Hermosa et al., 2012; Ru and Di, 2012; Dawidziuk et al., 2016; Bunbury-Blanchette and Walker, 2019; Haque et al., 2020).
Many diseases that are caused by fungi can infect and damage of the melons plants (including cantaloupes and muskmelons) during the growing season, the harvesting process and storage, all of which can result in significant losses in the yield and quality of this fruit crop (Gomez and Tello, 2000; Walcott et al., 2004; Ghuffar et al., 2018; Li et al., 2015). Gummy stem blight disease caused by S. cucurbitacearum on a number of melon plants is distributed in many countries throughout the world (Li et al., 2015; Garampalli et al., 2016; Nuangmek et al., 2018). In the United States, gummy stem blight disease on cantaloupes caused by S. citrull has been previously reported (Stewart et al., 2015). In addition, Fusarium wilt is an important disease that is associated with muskmelons. Generally, F. oxysporum f. sp. melonis is known to be a causal agent of this disease (Punja et al., 2001; Perchepied and Pitrat, 2004; Garampalli et al., 2016). Fusarium oxysporum f. sp. niveum and F. solani have been reported as causal agents of melon wilt disease (Egel and Martyn, 2007; González et al., 2020). However, no incidence of wilt disease from F. equiseti had been reported in muskmelons. The symptoms of this disease caused by F. equiseti in muskmelons observed in the current study are similar to those that are known to be caused by Fusarium pathogens. Therefore, F. equiseti could be considered as one of the causal agents of wilt disease in muskmelons.
Trichoderma species have been widely known aspect of biological control agents that can serve as a multifaceted mechanism (in competition for nutrients and space, and mycoparasitism; Hyder et al., 2007; Schuster and Schmoll, 2010; Thapa et al., 2020). This study found that T. phayaoense could inhibit the mycelial growth of S. cucurbitacearum and F. equiseti and reduce the impact of the disease on the disease severity index for gummy stem blight and wilt disease in muskmelon seedlings. This determination is similar to the findings of other previous reports. Similarly, previous studies have determined that Trichoderma species (e.g., T. akoningii, T. asperlloides, T. atroviride, T. harzianum, T. lentiforme, T. longibrachiatum, and T. virens) successfully controlled various fungal plant pathogens including the fungal genera Alternaria, Cladosporium, Fusarium, Phytophthora, and Stagonosporopsis (Puyam, 2016; Ghazanfar et al., 2018; Sood et al., 2020; Thapa et al., 2020). Furthermore, Utkhede and Koch (2004) and Patel et al. (2017) found that T. harzanum could significantly reduce the effects of gummy stem blight disease caused by S. cucurbitacearum in cucurbit plants under both greenhouse and field conditions. Moreover, Gava and Pinto (2016) found that T. harzianum, T. koningii, T. polysporum, and T. viride showed a degree of efficiency in the control of melon wilt disease caused by F. oxysporum f. sp. melonis, which could then lead to increases in fruit production. A study conducted by Martínez-Medina et al. (2014) on T. harzianum, T. ghanense, and T. hamatum reported on their antagonistic activity to F. oxysporum f. sp. melonis in vitro, and their biocontrol activity against Fusarium wilt on melon plants in field experiments. In this present study, the inoculation of T. phayaoense could be effective in improving plant development (increasing plant height and dry weight values of shoots and roots) and the number of fruit attributes (weight, diameter, circumference, and total soluble solids) of muskmelons. These results were similar to those of Fernondo et al. (2018) who found that T. saturnisporum enhanced the growth of melon seedlings, as well as increased fruit productivity and quality when compared with the control. Moreover, previous studies have reported that Trichoderma spp. could promote the growth of bitter gourds, bottle gourds, cucumbers, peppers, rice, and tomato seedlings (Chagas et al., 2015; Kotasthane et al., 2015; Marin-Guirao et al., 2016; Herrera-Parra et al., 2017; Uddin et al., 2018). Additionally, Trichoderma spp. can produce phytohormones [indole-3-acetic acid (IAA) or auxin analogues, abscisic acid, and gibberellin], siderophores, and other plant growth promoting substances (e.g., 6-pentyl-α-pyrone, cyclonerodiol, harzianolide, and koninginins; Contreras-Cornejo et al., 2009; Vinale et al., 2012; Martínez-Medina et al., 2014; Zhao and Zhang, 2015; Zúñiga-Silgado and Vargas, 2016; Jaroszuk-Ściseł et al., 2019). Moreover, several previous studies have shown that Trichoderma spp. can solubilize several plant nutrients, especially phosphate, into an available form for plant that can improve plant growth (Chagas et al., 2015; Zhao and Zhang, 2015; França et al., 2017; Bononi et al., 2020).
The degrees of tolerance to fungicide, herbicide, and insecticide microorganisms indicate the capability of this species to effectively degrade those fungicides, herbicides, and insecticides. It was also found that tolerance activities varied in different plants depending on the types of compounds being used as well as the fungal isolates being employed (Ortiz-Hernández and Sánchez-Salinas, 2010; Chaparro et al., 2011; Singh et al., 2012; Mohiddin and Khan, 2013). An increase in the concentrations of chemical compounds decreased both fungal growth and TI values, which was in accordance with previous findings (Suwannarach et al., 2015; Boat et al., 2018; Karaoglu et al., 2018). In this study, T. phayaoense could tolerate the selected fungicide (metalaxyl) in the field at recommended dosages. Similar to our results, previous studies have shown that T. atroviride, T. harzianum, T. koningii, and T. pseudokoningii have been acknowledged for their ability to tolerate fungicides, herbicides, and insecticides (Khan and Shahzad, 2007; Chaparro et al., 2011; Singh et al., 2012; Bhale and Rajkonda, 2015; da Silva et al., 2018). Additionally, the biocontrol agents of T. harzianum and T. virens could tolerate different insecticide compounds and could display varying degrees of tolerance (Wedajo, 2015). Therefore, information on the effects of fungicides, herbicides, and insecticides on biocontrol agents would be beneficial to a range of important in vivo applications.
Conclusion
Many researchers have been an increased interest in application of beneficial microorganisms for the sustainable agriculture in last decade. In this study, a new endophytic fungus, T. phayaoense, was isolated from the leaves of the Siam weed. Findings indicated the presence of the controlling muskmelon pathogens, F. equiseti and S. cucurbitacearum, in an in vitro investigation. A pathogenicity test confirmed that T. phayaoense could serve as a non-pathogen on muskmelon seedlings. Furthermore, T. phayaoense could prevent gummy stem blight and Fusarium wilt in muskmelon seedlings and could also be effective in improving plant and fruit development. Moreover, this fungus was found to be able to tolerate metalaxyl in field applications at recommended dosages. Thus, it is possible that T. phayaoense could be used as a plant growth promotion and biological control agent to manage gummy stem blight and wilt in muskmelons. It could also be demonstrated that the use of chemical fungicides can be replaced by this fungus. Further studies, the optimal techniques of inoculum production will be investigated, and its ability to produce chitinase, β-glucosidase, β-N-acetyl-D-hexosaminidase, and plant growth promotion substances will be determined. The toxicity assays are also necessary in laboratory, and clinical tests are needed in future studies to fully understand the profile of this fungus. Additionally, field trials will need to be conducted in the future.
Data Availability Statement
The original contributions presented in the study are publicly available. This data can be found in NCBI’s GenBank with accession numbers: MT995122, MW002073, and MW002074.
Author Contributions
WN, WA, and NS: conceptualization. WA, NS, and JK: methodology, software, formal analysis, investigation, data curation, and writing-original draft. WN, WA, NS, and SL: validation. WN and WA: resources. WN, WA, JK, NS, and SL: writing-review and editing. SL: supervision. All authors read, revised, and approved the final manuscript.
Funding
This work was supported by the financial support provided from Thailand Science Research and Innovation (RTA5880006), and partially supported by Chiang Mai University, Thailand.
Conflict of Interest
The authors declare that the research was conducted in the absence of any commercial or financial relationships that could be construed as a potential conflict of interest.
Acknowledgments
We are gratefully acknowledge to Russell Kirk Hollis for the English correction of this manuscript.
Supplementary Material
The Supplementary Material for this article can be found online at: https://www.frontiersin.org/articles/10.3389/fmicb.2021.634772/full#supplementary-material
Supplementary Table S1 | Details of sequences used in molecular phylogenetic analysis.
Supplementary Table S2 | Treatment details in this study.
Footnotes
References
Alfaro, M. E., Zoller, S., and Lutzoni, F. (2003). Bayes or bootstrap? A simulation study comparing the performance of Bayesian Markov Chain Monte Carlo sampling and bootstrapping in assessing phylogenetic confidence. Mol. Biol. Evol. 20, 255–266. doi: 10.1093/molbev/msg028
Arnold, A. E., Maynard, Z., Gilbert, G. S., Coley, P. D., and Kursar, T. A. (2000). Are tropical fungal endophytes hyperdiverse? Ecol. Lett. 3, 267–274. doi: 10.1046/j.1461-0248.2000.00159.x
Bhale, U. N., and Rajkonda, J. N. (2015). Compatibility of fungicides and antagonistic activity of Trichoderma spp. against plant pathogens. BioSci. Method 6, 1–9. doi: 10.5376/bm.2015.06.0003
Blanchette, R. (1991). Delignification by wood-decay fungi. Annu. Rev. Phytopathol. 29, 281–403. doi: 10.1146/annurev.py.29.090191.002121
Boat, M. A. B., Iacomi, B., Sameza, M. L., and Boyom, F. F. (2018). Fungicide tolerance and effect of environmental conditions on growth of Trichoderma spp. with antagonistic activity against Sclerotinia sclerotiorum causing white mold of common bean (Phaseolus vulgaris). Int. J. Innov. Appro. Agric. Res. 2, 226–243. doi: 10.29329/ijiaar.2018.151.8
Bononi, L., Chiaramonte, J. B., Pansa, C. C., Moitinho, N. V., and Melo, I. S. (2020). Phosphorus-solubilizing Trichoderma spp. from Amazon soils improve soybean plant growth. Sci. Rep. 10:2858. doi: 10.1038/s41598-020-59793-8
Boughalleb, N., Tarchoun, N., El-Mbarki, A., and El-Mahjoub, M. (2007). Resistance evaluation of nine cucurbit rootstocks and grafted watermelon (Citrullus lanatus L.) varieties against Fusarium wilt and Fusarium crown and root rot. J. Plant Sci. 2, 102–107. doi: 10.3923/jps.2007.102.107
Bunbury-Blanchette, A. L., and Walker, A. K. (2019). Trichoderma species show biocontrol potential in dual culture and greenhouse bioassays against Fusarium basal rot of onion. Biol. Control 130, 127–135. doi: 10.1016/j.biocontrol.2018.11.007
Cal, A. D., Sztejnberg, A., Sabuquillo, P., and Melgarejo, P. (2009). Management Fusarium wilt on melon and watermelon by Penicillium oxalicum. Biol. Control 51, 480–486. doi: 10.1016/j.biocontrol.2009.08.011
Carbone, I., and Kohn, L. M. (1999). A method for designing primer sets for speciation studies in filamentous ascomycetes. Mycologia 91, 553–556. doi: 10.1080/00275514.1999.12061051
Carroll, G. (1988). Fungal endophytes in stems and leaves: from latent pathogen to mutualistic symbiont. Ecology 69, 2–9. doi: 10.2307/1943154
Chagas, L. F. B., Junior, A. F. C., de Carvalho, M. R., Miller, L. D., and Colonia, B. S. O. (2015). Evaluation of the phosphate solubilization potential of Trichoderma strains (Trichoplus JCO) and effects on rice biomass. J. Soil Sci. Plant Nutr. 15, 794–804. doi: 10.4067/S0718-95162015005000054
Chaparro, A. P., Carvajal, L. H., and Orduz, S. (2011). Fungicide tolerance of Trichoderma asperelloides and T. harzianum strains. Agric. Sci. 2, 301–307. doi: 10.4236/as.2011.23040
Chaverri, P., Branco-Rocha, F., Jaklitsch, W., Gazis, R., Degenkolb, T., and Samuels, G. J. (2015). Systematics of the Trichoderma harzianum species complex and the re-identification of commercial biocontrol strains. Mycologia 107, 558–590. doi: 10.3852/14-147
Chaverri, P., and Samuels, G. J. (2013). Evolution of habitat preference and nutrition mode in a cosmopolitan fungal genus with evidence of interkingdom host jumps and major shifts in ecology. Evolution 7, 2823–2837. doi: 10.1111/evo.12169
Chen, K., and Zhuang, W. Y. (2017). Discovery from a large-scaled survey of Trichoderma in soil of China. Sci. Rep. 7:9090. doi: 10.1038/s41598-017-07807-3
Choi, Y. W., Hyde, K. D., and Ho, W. W. H. (1999). Single spore isolation of fungi. Fungal Divers. 3, 29–38.
Chounhary, A., and Ashraf, S. (2019). Utilizing the combined antifungal potential of Trichoderma spp. and organic amendments against dry root rot of mungbean. Egypt J. Biol. Pest. Control 29:83. doi: 10.1186/s41938-019-0187-8
Contreras-Cornejo, H. A., Macias-Rodriguez, L., Cortes-Penagos, C., and Lopez-Bucio, J. (2009). Trichoderma virens, a plant beneficial fungus, enhances biomass production and promotes lateral root growth through an auxin-dependent mechanism in Arabidopsis. Plant Physiol. 149, 1579–1592. doi: 10.1104/pp.108.130369
da Silva, M. A. F., de Moura, K. E., de Moura, K. E., Salomao, D., and Patricio, F. R. A. (2018). Compatibility of Trichoderma isolates with pesticides used in lettuce crop. Summa Phytopathol. 44, 137–142. doi: 10.1590/0100-5405/176873
Dalcin, M. S., Tschoeke, P. H., Aguiar, R. W. S., Fidelis, R. R., Didonet, J., and Santos, G. R. (2017). Severity of gummy stem blight on melon in relation to cultivars, use of fungicides and growing season. Hortic. Bras. 35, 483–489. doi: 10.1590/s0102-053620170403
Darriba, D., Taboada, G. L., Doallo, R., and Posada, D. (2012). jModelTest 2: more models, new heuristics and parallel computing. Nat. Methods 9:772. doi: 10.1038/nmeth.2109
Dawidziuk, A., Popiel, D., Kaczmarek, J., Strakowska, J., and Jedryczka, M. (2016). Optimal Trichoderma strains for control of stem canker of brassicas: molecular basis of biocontrol properties and azole resistance. BioControl 61, 755–768. doi: 10.1007/s10526-016-9743-2
Edgar, R. C. (2004). MUSCLE: multiple sequence alignment with high accuracy and high throughput. Nucleic Acids Res. 32, 1792–1797. doi: 10.1093/nar/gkh340
Egel, D. S., and Martyn, R. D. (2007). Fusarium wilt of watermelon and other cucurbits. Plant Health Instr. 10:1094. doi: 10.1094/PHI-I-2007-0122-01
FAOSTAT (2020). Food and Agriculture Data. Available at: http://www.fao.org/faostat/en/#home (Accessed September 25, 2020).
Felsenstein, J. (1985). Confidence intervals on phylogenetics: an approach using bootstrap. Evolution 39, 783–791. doi: 10.1111/j.1558-5646.1985.tb00420.x
Fernondo, D., Milagrosa, S., Francisco, C., and Francisco, M. (2018). Biostimulant activity of Trichoderma saturnisporum in melon (Cucumis melo). HortScience 53, 810–815. doi: 10.21273/HORTSCI13006-18
Fomina, M. A., Alexander, I. J., Colpaert, J. V., and Gadd, G. M. (2005). Solubilization of toxic metal minerals and metal tolerance of mycorrhizal fungi. Soil Biol. Biochem. 37, 851–866. doi: 10.1016/j.soilbio.2004.10.013
França, D. V. C., Kupper, K. C., Magri, M. M. R., Gomes, T. M., and Rossi, F. (2017). Trichoderma spp. isolates with potential of phosphate solubilization and growth promotion in cherry tomato. Pesqui. Agropecu. Trop. 47, 360–368. doi: 10.1590/1983-40632017v4746447
Gams, W., and Meyer, W. (1998). What exactly is Trichoderma harzianum? Mycologia 90, 904–915. doi: 10.2307/3761332
Gao, F., Dai, C., and Liu, X. (2010). Mechanisms of fungal endophytes in plant protection against pathogens. Afr. J. Agric. Res. 4, 1346–1351.
Garampalli, R., Mangala, G., Li, H. X., and Brewer, T. (2016). Two Stagonosporopsis species identified as causal agents of gummy stem blight epidemics of gherkin cucumber (Cucumis sativus) in Karnataka, India. Eur. J. Plant Pathol. 145, 507–512. doi: 10.1007/s10658-015-0841-2
Gava, C. A. T., and Pinto, J. M. (2016). Biocontrol of melon wilt caused by Fusarium oxysporum Schlect f. sp. melonis using seed treatment with Trichoderma spp. and liquid compost. Biol. Control 97, 13–20. doi: 10.1016/j.biocontrol.2016.02.010
Ghazanfar, M. U., Raza, M., Raza, W., and Qamar, M. I. (2018). Trichoderma as potential biocontrol agent, its exploitation in agriculture: a review. Plant Prot. 2, 109–135.
Ghuffar, S., Irshad, G., Naz, F., Rosli, H. B., Hyder, S., Mehmood, N., et al. (2018). First report of two Penicillium spp. causing postharvest fruit rot of grapes in Pakistan. Plant Dis. 104:1037. doi: 10.1094/PDIS-10-17-1616-PDN
Gomez, V. J., and Tello, J. C. (2000). Las semillas de melón (Cucumis melo L.) portadoras de diversos patotipos de Fusarium oxysporum f. sp. melonis. Bol. Sanid. Veg. Plagas 26, 35–45.
González, V., Armijos, E., and Garcés-Claver, A. (2020). Fungal endophytes as biocontrol agents against the main soil-borne diseases of melon and watermelon in Spain. Agronomy 10:820. doi: 10.3390/agronomy10060820
Haque, Z., Iqbal, M. S., Ahmad, A., Khan, M. S., and Prakash, J. (2020). Molecular characterization of Trichoderma spp. isolates by internal transcribed spacer (ITS) region sequencing technique and ITS use as a biocontrol agent. Open Biotechnol. J. 14, 70–77. doi: 10.2174/1874070702014010070
Hermosa, R., Viterboo, A., Chet, I., and Monte, E. (2012). Plant-beneficial effects of Trichoderma and of its genes. Microbiology 158, 17–25. doi: 10.1099/mic.0.052274-0
Herrera-Parra, E., Cristobal-Zapata, J., and Ramos-Zappta, J. (2017). Trichoderma strains as growth promoters in Capsicum annuum and as biocontrol agents in Meloidogyne incognita. Chil. J. Agric. Res. 77, 318–324. doi: 10.4067/S0718-58392017000400318
Hyder, S., Inam-ul-Haq, M., Bibi, S., Malik, A. H., Ghuffar, S., and Iqbal, S. (2007). Novel potential of Trichoderma spp. as biocontrol agent. J. Entomol. Zool. Stud. 5, 214–222.
Ismail, H. I., Chan, K. W., Mariod, A. A., and Ismail, M. (2010). Phenolic content and antioxidant activity of cantaloupe (Cucumis melo) methanolic extracts. Food Chem. 119, 643–647. doi: 10.1016/j.foodchem.2009.07.023
Jaklitsch, W. M. (2009). European species of Hypocrea I. the green-spored species. Stud. Mycol. 63, 1–91. doi: 10.3114/sim.2009.63.01
Jaklitsch, W. M., and Voglmayr, H. (2015). Biodiversity of Trichoderma (Hypocreaceae) in southern Europe and Macaronesia. Stud. Mycol. 80, 1–87. doi: 10.1016/j.simyco.2014.11.001
Jaroszuk-Ściseł, J., Tyśkiewicz, R., Nowak, A., Ozimek, E., Majewska, M., Hanaka, A., et al. (2019). Phytohormones (auxin, gibberellin) and ACC deaminase in vitro synthesized by the mycoparasitic Trichoderma DEMTkZ3A0 strain and changes in the level of auxin and plant resistance markers in wheat seedlings inoculated with this strain conidia. Int. J. Mol. Sci. 20:4923. doi: 10.3390/ijms20194923
Karaoglu, S. A., Bozdeveci, A., and Pehlivan, N. (2018). Characterization of local Trichoderma spp. as potential bio-control agents, screening of in vitro antagonistic activities and fungicide tolerance. J. Biol. Chem. 46, 247–261. doi: 10.15671/HJBC.2018.233
Kehinde, I. A. (2013). Characteristic symptoms of melon diseases caused by fungi in south western Nigeria. Afr. J. Agric. Res. 8, 5791–5801. doi: 10.5897/AJAR10.1108
Khan, M. O., and Shahzad, S. (2007). Screening of Trichoderma species for tolerance to fungicide. Pak. J. Bot. 39, 945–951.
Kishi, K. (1966). Necrotic spot of melon, a new virus disease. Ann. Phytopathol. Soc. Japan 32, 138–144.
Kotasthane, A., Agrawal, T., Kushwah, R., and Rahatkar, O. V. (2015). In-vitro antagonism of Trichoderma spp. against Sclerotium rolfsii and Rhizoctonia solani and their response towards growth of cucumber, bottle gourd and bitter gourd. Eur. J. Plant Pathol. 141, 523–543. doi: 10.1007/s10658-014-0560-0
Kristkova, E., Lebeda, A., and Sedlakova, B. (2009). Species spectra, distribution and host range of cucurbit powdery mildews in the Czech Republic, and in some other European and middle eastern countries. Phytoparasitica 37, 337–350. doi: 10.1007/s12600-009-0045-4
Li, X., and Brewer, T. (2016). Spatial genetic structure and population dynamics of gummy stem blight fungi within and among watermelon fields in the Southeastern United States. Phytopathology 106, 900–908. doi: 10.1094/PHYTO-01-16-0006-R
Li, P. F., Ren, R. S., Yao, X. F., Xu, J. H., Babu, B., Paret, M. L., et al. (2015). Identification and characterization of the causal agent of gummy stem blight from muskmelon and watermelon in East China. J. Phytopathol. 163, 314–319. doi: 10.1111/jph.12277
Lo, C. C. (2010). Effect of pesticides on soil microbial community. J. Environ. Sci. Health B 45, 348–359. doi: 10.1080/10934520903467873
Lui, Y. J., Whelen, S., and Hall, B. D. (1999). Phylogenetic relationships among ascomycetes: evidence from an RNA polymerase II subunit. Mol. Biol. Evol. 16, 1799–1808. doi: 10.1093/oxfordjournals.molbev.a026092
Malik, A. H., Mansoor, S., Iram, S., Briddon, R. W., and Zarfar, Y. (2010). Several disease of melon in northwest frontier province is associated with simultaneous infection of two RNA viruses. Pak. J. Bot. 42, 361–367.
Marin-Guirao, J. J., Rodriguez-Romera, P., Lupion-Rodriguez, B., Camacho-Ferre, F., and Tello-Marquina, J. C. (2016). Effect of Trichoderma on horticultural seedlings’ growth promotion depending on inoculum and substrate type. J. Appl. Microbiol. 121, 1095–1102. doi: 10.1111/jam.13245
Martínez-Medina, A., Alguacil, M. D. M., Pascual, J. A., and Wees, S. C. M. (2014). Phytohormone profiles induced by Trichoderma isolates correspond with their biocontrol and plant growth-promoting activity on melon plants. J. Chem. Ecol. 40, 804–815. doi: 10.1007/s10886-014-0478-1
Mohiddin, F. A., and Khan, M. R. (2013). Tolerance of fungal and bacterial biocontrol agents to six pesticides commonly used in the control of soil borne plant pathogens. Afr. J. Agric. Res. 8, 5331–5334. doi: 10.5897/AJAR11.677
Nuangmek, W., Aiduang, W., Suwannarach, N., Kumla, J., and Lumyong, S. (2018). First report of gummy stem blight caused by Stagonosporopsis cucurbitacearum on cantaloupe in Thailand. Can. J. Plant Pathol. 40, 306–311. doi: 10.1080/07060661.2018.1424038
Nuangmek, W., Aiduang, W., Suwannarach, N., Kumla, J., and Lumyong, S. (2019). First report of fruit rot on cantaloupe caused by Fusarium equiseti in Thailand. J. Gen. Plant Pathol. 85, 295–300. doi: 10.1007/s10327-019-00841-1
Nuangmek, W., McKenzie, E. H. C., and Lumyong, S. (2008). Endophytic fungi from wild banana (Musa acuminata Colla) works against anthracnose disease caused by Colletotrichum musae. Res. J. Microbiol. 3, 368–374. doi: 10.3923/jm.2008.368.374
Ortiz-Hernández, M. L., and Sánchez-Salinas, E. (2010). Biodegradation of the organophosphate pesticide tetrachlorvinphos by bacteria isolated from agricultural soils in México. Rev. Int. Contam. Ambient 26, 27–38.
Padhi, L., Mohanta, Y. K., and Panda, K. (2015). Endophytic fungi with great promises: a review. J. Adv. Pharm. Educ. Res. 3, 152–170.
Patel, M., Solanki, V. A., Rakholia, K. B., and Khunt, M. D. (2017). Efficacy of biocontrol agents against Didymella bryoniae under field conditions. Trends Biosci. 10, 8547–8551. doi: 10.1007/978-3-030-22304-5_13
Perchepied, L., and Pitrat, M. (2004). Polygenic inheritance of partial resistance to Fusarium oxysporum f. sp. melonis race 1.2 in melon. Phytopathology 94, 1331–1336. doi: 10.1094/PHYTO.2004.94.12.1331
Punja, Z. K., Parker, M., and Elmhirst, J. F. (2001). Fusarium wilt of field-grown muskmelon in British Columbia. Can. J. Plant Pathol. 23, 403–410. doi: 10.1080/07060660109506961
Puyam, A. (2016). Advent of Trichoderma as a bio-control agent- a review. J. Nat. Appl. Sci. 8, 1100–1109. doi: 10.31018/jans.v8i2.927
Rodriguez, R. J., White, J. F. J., Arnold, A. E., and Redman, R. S. (2009). Fungal endophytes: diversity and functional roles. New Phytol. 182, 314–330. doi: 10.1111/j.1469-8137.2009.02773.x
Ronquist, F., Teslenko, M., Van der Mark, P., Ayres, D. L., Darling, A., Höhna, S., et al. (2012). MrBayes 3.2: efficient Bayesian phylogenetic inference and model choice across a large model space. Syst. Biol. 61, 539–542. doi: 10.1093/sysbio/sys029
Ru, Z., and Di, W. (2012). Trichoderma spp. from rhizosphere soil and their antagonism against Fusarium sambucinum. Afr. J. Biotechnol. 11, 4180–4186. doi: 10.5897/AJB11.3426
Samuels, G. J. (2006). Trichoderma: systematics, the sexual state and ecology. Phytopathology 96, 195–206. doi: 10.1094/PHYTO-96-0195
Sánchez-García, B. M., Espinosa-Huerta, E., Vollordo-Pineda, E., Rodriguez-Guerra, R., and Mora-Aviles, M. A. (2017). Trichoderma spp. native strains molecular identification and in vitro antagonistic evaluation of root phitopathogenic fungus of the common bean (Phaseolus vulgaris L.) cv. Montcalm. Agrociencia 51, 63–79.
Schuster, A., and Schmoll, M. (2010). Biology and biotechnology of Trichoderma. Appl. Microbiol. Biotechnol. 87, 787–799. doi: 10.1007/s00253-010-2632-1
Selim, K. A., El-Beih, A. A., AbdEl-Rahman, T. M., and El-Diwany, A. I. (2012). Biology of endophytic fungi. Curr. Res. Environ. Appl. Mycol. 2, 31–82. doi: 10.5943/cream/2/1/3
Shah, S., Nasreen, S., and Sheikh, P. A. (2012). Cultural and morphological characterization of Trichoderma spp. associated with green mold disease of Pleurotus spp. in Kashmir. Res. J. Microbiol. 7, 139–144. doi: 10.3923/jm.2012.139.144
Singh, V. P., Srivastava, S., Shrivastava, S. K., and Singh, H. B. (2012). Compatibility of different insecticides with Trichoderma harzianum under in vitro condition. Plant Pathol. J. 11, 73–76. doi: 10.3923/ppj.2012.73.76
Sood, M., Kapoor, D., Kumar, V., Sheteiwy, M. S., Ramakrishnan, M., Landi, M., et al. (2020). Trichoderma: the “secrets” of a multitalented biological agent. Plan. Theory 9:762. doi: 10.3390/plants9060762
Stamatakis, A. (2006). RAxML-VI-HPC: maximum likelihood based phylogenetic analyses with thousands of taxa and mixed models. Bioinformatics 22, 2688–2690. doi: 10.1093/bioinformatics/btl446
Stewart, E., Turner, N., and Brewer, T. (2015). Evolutionary history and variation in host range of three Stagonosporopsis species causing gummy stem blight of cucurbits. Fungal Biol. 199, 370–382. doi: 10.1016/j.funbio.2014.12.008
Suwannarach, N., Kumla, J., Matsui, K., and Lumyong, S. (2015). Characterization and efficacy of Muscodor cinnamomi in promoting plant growth and controlling Rhizoctonia root rot in tomatoes. Biol. Control 90, 25–233. doi: 10.1016/j.biocontrol.2015.05.008
Taylor, J. W., Jacobson, D. J., Kroken, S., Kasuga, T., Geiser, D. M., Hibbett, D. S., et al. (2000). Phylogenetic species recognition and species concepts in fungi. Fungal Genet. Biol. 31, 21–32. doi: 10.1006/fgbi.2000.1228
Thapa, S., Rai, N., Limbu, A. K., and Joshi, A. (2020). Impact of Trichoderma sp. in agriculture: a mini-review. J. Biol. Today's World 9:227. doi: 10.35248/2322-3308.20.09.225
Uddin, M. N., ur Rahman, U., Khan, W., Uddin, N., and Muhammad, M. (2018). Effect of Trichoderma harzianum on tomato plant growth and its antagonistic activity against Phythium ultimum and Phytopthora capsici. Egypt J. Biol. Pest Co. 28:32. doi: 10.1186/s41938-018-0032-5
Utkhede, R. S., and Koch, C. A. (2004). Evaluation of biological and chemical treatments for control of gummy stem blight on cucumber plants grown hydroponically in greenhouses. BioControl 49, 109–117. doi: 10.1023/B:BICO.0000009394.75429.a7
Vinale, F., Sivasithamparam, K., Ghisalberti, E. L., and Ruocco, M. (2012). Trichoderma secondary metabolites that affect plant metabolism. Nat. Prod. Commun. 7, 1545–1550. doi: 10.1177/1934578X1200701133
Wackett, L. P. (2013). Microbial biocontrol agents. Microb. Biotechnol. 6, 443–444. doi: 10.1111/1751-7915.12065
Walcott, R., Fessehaie, A., and Castro, A. (2004). Differences in pathogenicity between two genetically distinct groups of Acidovorax avenae subsp. citrulli on cucurbit hosts. J. Phytopathol. 152, 277–285. doi: 10.1111/j.1439-0434.2004.00841.x
Wedajo, B. (2015). Compatibility studies of fungicides with combination of Trichoderma species under in vitro conditions. Virol. Mycol. 4:2. doi: 10.4172/2161-0517.1000149
White, T. J., Burns, T., Lee, S., and Taylor, J. (1990). “Amplification and direct sequencing of fungal ribosomal RNA genes for phylogenetics” in PCR protocols, a guide to methods and application. eds. M. A. Innis, D. H. Gelfand, J. J. Sninsky, and T. J. White (San Diego, USA: Academic Press), 315–322.
Yang, C. (2011). Fungicide: modes of action and possible impact on nontarget microorganisms. ISRN Ecol. 8:130289. doi: 10.5402/2011/130289
Zhao, L., and Zhang, Y. (2015). Effects of phosphate solubilization and phytohormone production of Trichoderma asperellum Q1 on promoting cucumber growth under salt stress. J. Integr. Agric. 14, 1588–1597. doi: 10.1016/S2095-3119(14)60966-7
Keywords: biocontrol, cucurbit, fungal endophyte, fungal disease, plant growth promotion
Citation: Nuangmek W, Aiduang W, Kumla J, Lumyong S and Suwannarach N (2021) Evaluation of a Newly Identified Endophytic Fungus, Trichoderma phayaoense for Plant Growth Promotion and Biological Control of Gummy Stem Blight and Wilt of Muskmelon. Front. Microbiol. 12:634772. doi: 10.3389/fmicb.2021.634772
Edited by:
Samantha Chandranath Karunarathna, Chinese Academy of Sciences, ChinaReviewed by:
Abdullah M. Al-Sadi, Sultan Qaboos University, OmanShahzad Munir, Yunnan Agricultural University, China
Copyright © 2021 Nuangmek, Aiduang, Kumla, Lumyong and Suwannarach. This is an open-access article distributed under the terms of the Creative Commons Attribution License (CC BY). The use, distribution or reproduction in other forums is permitted, provided the original author(s) and the copyright owner(s) are credited and that the original publication in this journal is cited, in accordance with accepted academic practice. No use, distribution or reproduction is permitted which does not comply with these terms.
*Correspondence: Nakarin Suwannarach, c3V3YW4uNDYyQGdtYWlsLmNvbQ==; c3V3YW5fNDYxQGhvdG1haWwuY29t