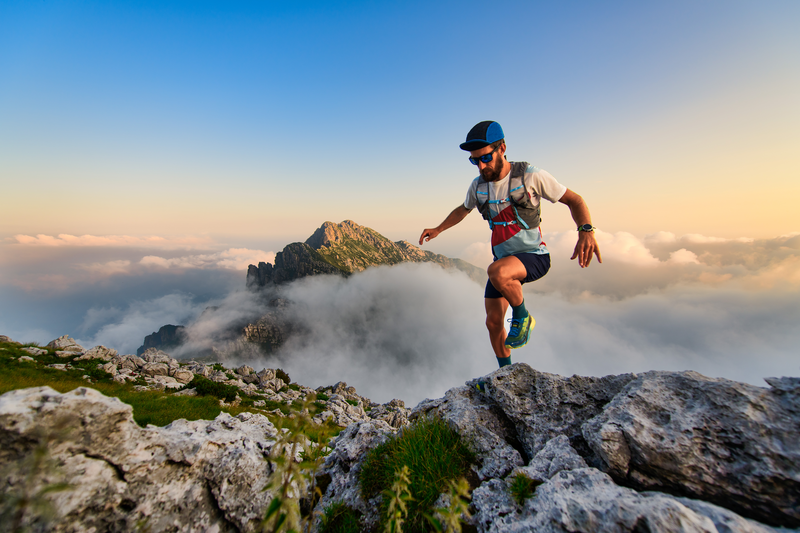
95% of researchers rate our articles as excellent or good
Learn more about the work of our research integrity team to safeguard the quality of each article we publish.
Find out more
ORIGINAL RESEARCH article
Front. Microbiol. , 26 February 2021
Sec. Microbiotechnology
Volume 12 - 2021 | https://doi.org/10.3389/fmicb.2021.631096
This article is part of the Research Topic New Microbial Inoculants for Enhancing Fermentation Quality of Silage View all 17 articles
To better understand the mechanism underlying the citric acid (CA)-regulated silage fermentation, we investigated the bacterial community and fermentation quality of king grass (KG) ensiled without (CK) or with Lactobacillus plantarum (L), CA and the combination of L and CA (CAL). The bacterial community was characterized by using the 16Sr DNA sequencing technology. The L and CA treatments altered the silage bacterial community of KG, showing reduced bacterial diversity, while the abundance of desirable genus Lactobacillus was increased, and the abundances of undesirable genus Dysgonomonas and Pseudomonas were decreased. The additives also significantly raised the lactic acid content, dropped the pH, and reduced the contents of acetic acid, propionic acid, and ammonia-N in ensiled KG (P < 0.01). Besides, the combination treatment was more effective on silage fermentation with the highest pH and lactic acid content, while the contents of acetic acid, propionic acid, and ammonia-N were the lowest (P < 0.01). Moreover, CAL treatment exerted a notable influence on the bacterial community, with the lowest operational taxonomic unit (OTU) number and highest abundance of Lactobacillus. Furthermore, the bacterial community was significantly correlated with fermentation characteristics. These results proved that L and CA enhanced the KG silage quality, and the combination had a beneficial synergistic effect.
As a Pennisetum grass species, king grass (Pennisetum purpureum Schumacher × P. glaucum (Linnaeus) R. Brown, KG) is widely distributed in the tropical and subtropical regions worldwide (Zhao et al., 2019). KG is a multifunctional plant, and it is extensively used in ecological environmental protection, bioenergy industry, and animal husbandry (Li et al., 2014; Li M. et al., 2019). KG vigorously grows in the summer or rainy season. However, its growth lags in the winter or drought season, resulting in biomass shortages (Li et al., 2014). Due to its seasonal harvest, KG should be properly preserved to provide a continuous supply. Ensiling is an approach for the long-term preservation of green forage. However, it is hard to make high-quality silage since KG or other Pennisetum grass has a higher lignin content and a lower content of soluble carbohydrates (Li et al., 2014; Desta et al., 2016).
The fermentation quality of silage mainly depends on the microbial community and its metabolites. Therefore, further study on the composition of silage microbial communities can provide a valuable scientific basis to enhance fermentation quality (Guo et al., 2018). Previous studies have investigated the silage microbial community composition of many forages, such as Alfalfa, corn, Napier grass, and so on (Guo et al., 2018; Xu et al., 2019; Yuan et al., 2019). Besides, the mechanism underlying the additive-improved fermentation quality has been analyzed, and various additives can enhance the silage fermentation quality in different ways (Guo et al., 2018; Xu et al., 2019; Yuan et al., 2019; Li F. et al., 2020).
Nevertheless, few reports have investigated the microbial composition of KG silage, and the mechanism underlying the response to additives remains unclear. Citric acid (CA) is a type of antioxidant, and it is also a safe additive used in the food industry (Li M. et al., 2020). Besides, CA can be used as a carbohydrate source to provide energy to lactic acid bacteria (LAB) and accelerate their growth (Li et al., 2016). Therefore, CA is considered as an ideal and novel silage additive to enhance the fermentation quality (Li et al., 2016; Ke et al., 2017, 2018; He et al., 2019b; Li M. et al., 2020; Lv et al., 2020). Our previous study has found that CA improves the silage quality though increasing the abundances of lactic acid-producing microorganisms, Paenibacillus and Bacillus (Li M. et al., 2020). Meanwhile, Lv et al. (2020) have reported that the addition of CA can increase the abundances of Pediococcus and Lactobacillus, while the abundances of Enterobacter, Escherichia-Shigella, and Pantoea are decreased, leading to enhanced Amomum villosum silage quality. However, the impacts of CA on the diversity of KG silage microorganisms remain largely unexplored.
In the present study, we hypothesized that LAB and CA had beneficial effects on the fermentation and microbes of KG silage, and their mixture might be shown a potential synergetic effect. Therefore, we aimed to assess the effects of LAB and CA on the microbial diversity and fermentation property of KG silage.
King grass (Reyan No. 4) was grown in the experimental base of the Chinese Academy of Tropical Agricultural Sciences (109°58′E, 19°52′N). The first cut KG of the vegetative stage (approximately 1.5–1.8 m height) was harvested and chopped into about 2-cm pieces by grass shredding machine (Donghong No. 1, Donghong Mechanical Equipment Co., Ltd., China). Four different treatments were conducted in our current study as follows: control (no additive, CK), Lactobacillus plantarum (L), CA, and the combination of L. plantarum and CA (CAL). According to the manufacturer’s guidelines, the additives were dissolved in sterile distilled water and then mixed thoroughly with the grass. An equal amount of sterile distilled water was added to the control group. Every treatment was carried out in triplicate. The application rate of L. plantarum (Snow Brand Seed Co., Ltd., Japan) and CA (Sinopharm Chemical Reagent Co., Ltd., China) was 1.0 × 105 colony-forming units (cfu)/g of fresh matter (FM) and 5 g/kg of FM, respectively. Briefly, 200 g of KG was blended with additives, and the mixture was placed in plastic bags (35 cm × 12 cm × 5 cm; Guozhong Packing Co., Ltd., Haikou, China). A total of 60 bags (four treatments × five ensiling durations × three replicates) were prepared and stored at normal temperature (25–30°C). Three bags were used to determine the chemical composition and organic acid on days 1, 3, 7, 14, and 30. The microbial community was determined after 30 days of fermentation.
Specimens were heated at 65°C for 72 h to determine the dry matter (DM) content, and dried materials were ground for chemical analysis. Crude protein (CP) and ether extract (EE) were determined according to the Guidelines of the Association of Official Analytical Chemists (AOAC 1990). Neutral detergent fiber (NDF) and acid detergent fiber (ADF) were determined as previously described by Van Soest et al. (1991). Water soluble carbohydrate (WSC) was determined according to a previously described method (Murphy, 1958). The fermentation quality of silage was determined using distilled water extracts. Briefly, 50 g wet silage was blended with 200 mL distilled water, followed by incubation at 4°C for 24 h and then filtration. The pH and concentrations of lactic acid, acetic acid, propionic acid, butyric acid, and ammonia-N were measured as previously established (Li et al., 2014). Microbial counts were analyzed using the plate count method on MRS agar, Violet Red Bile agar, and Rose Bengal agar as previously described (Li P. et al., 2019).
The above-mentioned extracts were used for the molecular analysis of the microbiota. Briefly, 20 mL filtrate was centrifuged at 12,000 g/min for 5 min, and the sediment was collected from the bottom. Microbial DNA was isolated from silage specimens with the E.Z.N.A®. soil DNA Kit (Omega Bio-Tek, Norcross, GA, United States) according to the manufacturer’s instructions. The concentration and purity of extracted DNA were assessed by NanoDrop 2000 UV-vis spectrophotometer (Thermo Fisher Scientific, Wilmington, DE, United States), and DNA integrity was confirmed by electrophoresis on 1% agarose gel. Primers 338F (5′-ACTCCTACGGGAGGCAGCAG-3′) and 806R (5′-GGACTACHVGGGTWTCTAAT-3′) were adopted to amplify the V3–V4 hypervariable regions of the bacterial 16S rRNA gene using a thermocycler PCR system (GeneAmp 9700, ABI, United States). After PCR products were purified and quantified, next-generation sequencing was carried out using Illumina MiSeq 2500 platform (Illumina, Inc., San Diego, CA, United States), and paired-end reads of 250 bp were generated.
The assembly of tags was carried out using filtered reads according to the principles as follows: overlap between paired-end reads should be more than 10 bp and less than 2% mismatch. The unique tags were obtained by removing redundant tags using software MOTHUR (Schloss et al., 2009). The abundance was then determined using the resultant unique tags. The high-quality reads were grouped into operational taxonomic units (OTUs) defined at a similarity of 97%. Diversity metrics were determined using the core-diversity plug-in within QIIME21 (Callahan et al., 2016). The microbial diversity within an individual sample was assessed using the alpha diversity indices, including observed OTUs, Chao1 richness estimator, Shannon diversity index, and Faith’s phylogenetic diversity index. Beta diversity was analyzed to assess the structural variation of microbiota across specimens, and then principal component analysis (PCA) was conducted (Vázquez-Baeza et al., 2013). Appropriate methods were employed to identify the bacterial strains with different abundances among samples and groups (Segata et al., 2011). Unless otherwise specified above, parameters used in the analysis were set as default. The heat map function of the R software2 and genus information for the Pennisetum sinese silage were used to generate a heat map. The data were analyzed using the free online BMKCloud Platform.3 The sequencing data were submitted to the National Center for Biotechnology Information Sequence Read Archive database under the BioProject accession number of PRJNA556187.
The impacts of additives (L. plantarum and CA), ensiling duration, and their interactions were investigated by two-way analysis of variance using general linear model (GLM) procedure of SAS 9.3 software (SAS Institute Inc., Cary, NC, United States). Significant differences were compared using Duncan’s multiple range tests, and P < 0.05 was considered statistically significant.
Table 1 shows the chemical and microbial compositions of fresh KG. The contents of DM, EE, CP, NDF, ADF, and WSC were 152.8, 60.2, 91.2, 765.3, 496.9, and 72.1 g/kg, respectively. Meanwhile, the number of LAB, yeast, and mold in fresh KG was 4.22, 2.78 and 3.04 cfu/g, respectively.
Table 2 shows the chemical composition dynamics of KG silages during 30 days of ensiling, which was reduced when the ensiling duration was prolonged. The DM content of the CK group was the lowest compared with the other groups on day 30 (P < 0.05). The CP content in the CK and L groups was lower compared with the CA and CAL groups on days 14 and 30 (P < 0.05). The contents of NDF and ADF showed a similar reducing trend, although it was not significantly different from the CK group. Meanwhile, lower contents of NDF and ADF were found in the CA and CAL groups on day 30 (P < 0.05). Additionally, the ensiling duration (D) and additive treatment (T) remarkably affected the contents of DM, NDF, and ADF (P < 0.01), and the CP content was only influenced by D (P < 0.01). Besides, we also found a significant interaction between D and T for the contents of DM, NDF, and ADF (P < 0.01).
Table 3 illustrates the fermentation characteristic dynamics of KG silage. The pH in all groups was dramatically reduced (P < 0.05) during the 7 days of fermentation, while the lowest pH was found on day 30. Meanwhile, the highest and lowest pH on day 30 were found in the CK group and CAL group (P < 0.05), respectively. The lactic acid content in all groups was remarkably elevated during the fermentation, while the highest lactic acid content was found in the CAL group on day 30 (P < 0.05). The acetic acid content of the CA and CAL groups was lower compared with the CK and L groups on day 30 (P < 0.05). In the present study, the content of acetic acid in the CK and L groups was greater compared with the CA and CAL groups, which led to the pH raised in the CK and L groups accordingly. The propionic acid content in all groups was not significantly changed during ensiling, and the lowest content was found in the CAL group on day 30 (P < 0.05). Butyric acid was not detected in all groups, indicating that KG was well preserved. The ammonia-N content in all groups was dramatically increased (P < 0.05) after 3 or 7 days of fermentation, while the highest and lowest ammonia-N contents were found in the CK group and CAL groups (P < 0.05), respectively.
A total of 958,785 raw reads and 910,947 raw tags were generated, after an average of 70, 971 clean tags and 68,123 effective tags was obtained in each silage sample.
Table 4 and Figure 1 show the α-diversity of the bacterial community of silages. Additive treatment affected the Ace, Chao 1, Shannon, and Simpson indices of bacterial diversity (Table 4). For community richness comparison, the Shannon index was lower, and the Simpson index was higher in the additive-treated groups compared with the CK group. A total of 249 OTUs were detected, with the highest number of OTUs found in the CK group (234), and the lowest number found in the CAL group (195) (Figure 1). Venn analysis exhibited that the additive treatment resulted in 181 common OTUs, and there were 31, 3, and 2 special OTUs in the CK, CA, and L groups, respectively. The PCA was employed to examine the correlations among the community structures of the silage bacterial community. A clear separation and difference of bacterial communities were found in four groups (Figure 2), suggesting that the bacterial composition was changed with different additive treatments. Therefore, we drew a conclusion based on the α-diversity and β-diversity that the LAB and CA treatments could affect the bacterial diversity and community structure of KG silage.
Figure 1. Venn analysis of OTUs for KG silage (CK, control; L, Lactobacillus plantarum; CA, Citric acid; CAL, Citric acid + Lactobacillus plantarum).
Figure 2. Beta-diversity of the bacterial community of KG silage (CK, control; L, Lactobacillus plantarum; CA, Citric acid; CAL, Citric acid + Lactobacillus plantarum).
Figure 3A describes the bacterial community at the phylum level. Firmicutes and Proteobacteria were dominant in all groups, Bacteroidetes was sub-dominant in the CK and CA groups, and Cyanobacteria was sub-dominant in the L group. The silage bacterial community was shifted upon the additives, the abundance of Firmicutes in additive treatment groups was increased, while the abundances of Proteobacteria and Bacteroidetes were decreased (except for the CA group) compared with the CK group. To further investigate the effects of additives on the bacterial community during ensiling, we examined the bacterial structures of KG silage at the genus level (Figure 3 B). Lactobacillus was predominant in the four groups. The sub-dominant microbes, in turn, were Dysgonomonas, Morganella, and Pseudomonas in the CK group, Gluconacetobacter in the L group, Pseudomonas and Gluconacetobacter in the CA group, and Pseudomonas in the CAL group. The abundance of Lactobacillus was increased along with the additive treatments, while the abundances of Dysgonomonas and Pseudomonas were decreased (except for the CA group). Particularly, CA treatment promoted the abundance of Lactobacillus, especially for the combination group, which accounted for as high as 95% of the total population.
Figure 3. The bacterial community and abundance at the phylum (A) and genus (B) levels in KG silage (CK, control; L, Lactobacillus plantarum; CA, Citric acid; CAL, Citric acid + Lactobacillus plantarum).
The linear discriminant analysis (LDA) effect size (LEfSe) method was used to assess the differences in microbial community between four groups and explore the specific bacterial in each group (LDA score >4.0). Figure 4 shows that CA exerted a dramatic impact on the microbial community. Lactobacillus paraplantarum and Lactobacillus brevis were the most abundant species in the CK group, and Lachnospiraceae and Ruminococcaceae were the most abundant families in the CA group, which could be the biomarkers of different treatments.
Figure 4. Comparison of microbial variations using the LEfSe online tool for KG silage (CK, control; CA, Citric acid).
To better understand the silage fermentation process, we also assessed the correlation between the fermentation characteristics and the bacterial community of KG silage (Figure 5). The lactic acid was positively correlated with genera lactobacilli, Weissella, and Enterobacter (P < 0.05), while it was negatively correlated with genera Delftia, Gemmobacter, Azospirillum, and Comamonas (P < 0.05). The acetic acid was positively associated with genera Ralstonia (P < 0.001), while it was negatively associated with genera Delftia, Gemmobacter, Brevundimonas, Sphingobacterium, and Azospirillum (P < 0.01). The propanoic acid was highly and positively correlated with genera Ralstonia (P < 0.001), but negatively associated with genera Delftia, Brevundimonas, and Comamonas (P < 0.05). The pH was negatively correlated with genera Lactobacillus, Weissella, Delftia, Azospirillum, and Clostridium (P < 0.05). Moreover, the ammonia-N content was positively correlated with genera Lactobacillus and Ralstonia (P < 0.05), while it was negatively associated with genus Delftia (P < 0.05).
Figure 5. Correlation of the bacterial community and silage fermentation (CK, control; L, Lactobacillus plantarum; CA, Citric acid; CAL, Citric acid + Lactobacillus plantarum; LA, Lactic acid; AA, Acetic acid; PA, Propionic acid; BA, Butyric acid; NH3-N, Ammonia-N). *P < 0.05, **P < 0.01 and ***P < 0.001.
Compared with the previous reports, the DM of KG was lower, while the contents of EE, CP, NDF, and ADF were higher (Li et al., 2014; Li M. et al., 2019; Xu et al., 2019). Recently, Li D. et al. (2019); Li P. et al. (2019) have found that the contents of DM and CP are 239 and 133 g/kg in KG, respectively, which are much higher compared with the above-mentioned studies. The differences in forage quality may be caused by cultivation, climatic conditions, soil fertility, growth period, and harvest time. The WSC content is a key factor affecting the fermentation quality. The WSC content in KG was 72.1 g/kg, which met the minimum requirement (60–80 g/kg DM) for well-conserved silage (Smith, 1962). However, the tropical forages normally have coarse and stemmy structures and high fiber fraction, which can cause the bad quality of silage (Yu et al., 2011; Li et al., 2017). Some previous studies have confirmed that KG ensiled alone can not achieve high fermentation quality (Li et al., 2014; Li M. et al., 2019). The well-preserved silage needs an LAB number of more than 5.0 log10 cfu/g during ensiling (Cai et al., 1998). The lower LAB number and higher yeast and mold contents indicated that more LAB was required for preparing KG silage. Moreover, the undesirable yeast and mold could be inhibited by the low pH, leading to the wide application of organic acid in forage silage (Guo et al., 2018; He et al., 2019b). Therefore, it might be helpful to achieve higher silage quality by rapidly reducing pH and promoting fermentation through adding CA and L. plantarum.
In the present study, the CA and CAL groups contained higher CP content. This finding might be explained as the effect of CA, which could inhibit protein hydrolysis (Ke et al., 2018). The CA treatment dramatically reduced the contents of NDF and ADF in KG. However, the effect of CA on ADF and NDF was contrary to the report of Ke et al. (2018) in alfalfa silage. The divergence might be related to the different types of forage that KG in this study was Gramineae plant, while the alfalfa was Leguminosae plant, which contained a higher amount of structural fibers. This probably impacted the effect of CA on ADF and NDF during ensiling. Meanwhile, the positive effect of CA on forage chemical composition has been also reported in previous studies (Li et al., 2016; Ke et al., 2017, 2018; He et al., 2019b). These results suggested that a combination of L. plantarum and CA could improve the feeding value of KG to some extent.
Silage pH plays an important role in the evaluation of fermentation property, and silage with pH 4.2 or lower would be considered as well-fermented silage (Edwards and McDonald, 1978). In this study, the pH value of the additive-treated groups was close to or below 4.2, which could ensure good preservation of KG silage. Li et al. (2016) and He et al. (2019b) have shown a similar pH of CA-treated silage. In contrast, Ke et al. (2017, 2018) have shown a higher pH value in CA-treated alfalfa silage, which is even higher than 5.0, and such discrepancy may be attributed to the different application rates. The lactic acid in silage is the dominant fermentation product, which is another important evaluation index of silage quality. The addition of CA directly decreased the pH, which could inhibit harmful microorganisms and promote the reproduction and growth of LAB, leading to increased lactic acid content. Such finding was consistent with the previous data, indicating that CA can increase the lactic acid content, while different supplementation levels have various effects (Ke et al., 2017; He et al., 2019b). However, some studies have demonstrated the different effect. Li et al. (2016) and He et al. (2019b) have reported that CA treatment reduces lactic acid content in alfalfa and Neolamarckia cadamba leaf silage. Zhao et al. (2004) and Seo et al. (2007) have shown that released CA can be used by some yeast strains as a carbon and energy sources, and the growth of yeasts may be more competitive than LAB when an appropriate level of CA is applied, resulting in the lower lactic acid content. Therefore, the optimal amount of CA in different silage materials needs to be further studied. As the main metabolite of Acetobacter fermentation in silage, acetic acid is also a crucial index for the evaluation of silage quality. The higher acetic acid concentration always leads to a higher pH, which benefits the undesirable microorganism Clostridia, leading to reduced silage quality (Zheng et al., 2017). The ammonia-N content in silage often reflects the breakdown efficiency of protein to peptide. The comparatively low ammonia-N content in additive-treated silage could be attributed to the effect of lower pH values, indicating that the activity of protease was inhibited and preservation of more nutrients. The above-mentioned results indicated that the addition of L. plantarum and CA in the ensiling process could promote the fermentation quality, and the combination treatment more efficiently enhanced the fermentation. He et al. (2019b) have reported the profitable associated effects of L. plantarum and CA combination treatment on alfalfa silage. Similarly, Guo et al. (2018) have also found that the combination of hexanoic acid and L.plantarum can improve the fermentation characteristics compared with the single treatment in Napier grass silage.
In our study, the CK group showed the high Shannon, Ace, and Chao1 indices, as well as the low Simpson index mean high bacterial diversity. These results implied that additive treatment could reduce bacterial diversity. It could be explained that the LAB and CA treatment decreased the pH, inhibited the growth of harmful microorganisms, and promoted the growth of LAB species. When LAB became the dominant species, the bacterial diversity was decreased. Similar data have been reported by Ni et al. (2017) and (Wang et al., 2019a,b) in soybean, Moringa oleifera leaves and Morus alba leaves. Meanwhile, PCA indicated that a clear separation and difference of bacterial communities were found in different groups of KG silage, suggesting that the bacterial composition was changed in the ensiling process with different additive treatments. This could be explained by that CAs could provide additional substrates to LAB and accelerate its growth, while the addition of L. plantarum also increased desirable microorganisms.
In the present study, Firmicutes and Proteobacteria were the top two dominant phyla in KG silage, which was similar to previous studies (Xu et al., 2017; Dong et al., 2019). However, the total abundances of the two phyla were increased from approximately 80% to more than 95% after fermentation (Liu et al., 2019; Wang et al., 2019b). Such elevation might be attributed to different silage materials and treatments. Normally, as the major bacterial strain with desirable functions, the Lactobacillus is dominant in well-preserved forage silage, because it is responsible for driving lactic fermentation during ensiling (Bao et al., 2016; Ni et al., 2017, 2018; Guan et al., 2018; Liu et al., 2019). CA treatment also promoted the abundance of Lactobacillus, especially for the combination group, which accounted for as high as 95% of the total population. It could be attributed to that the addition of CAs could provide substrates and supplement energy to Lactobacillus, which was beneficial for its propagation. Besides, the acidic environment formed by the addition of CA also inhibited the undesirable microbes, which might be conducive to the growth of Lactobacillus. Similar data have also been found by Lv et al. (2020) that the addition of CA can increase Pediococcus and Lactobacillus, resulting in enhanced silage quality of Amomum villosum. However, our previous study has found that CA improves the silage quality through raising the abundances of Paenibacillus and Bacillus, and other studies have also shown that Lactobacillus is not the dominant bacterial strain in organic acid-treated silage (He et al., 2019a, 2020; Li M. et al., 2020). Therefore, the type of organic acids and the proportion of additives may affect the bacterial community. In contrast, Dysgonomonas is a facultative anaerobe, Pseudomonas is an aerobic bacterium, and both of them are undesirable microorganisms and can reduce the fermentation quality (Hofstad et al., 2000; Dunière et al., 2013). Dysgonomonas is rarely reported in silage, and its effect and mechanism in silage fermentation need to be further studied. Pseudomonas spp. is considered an undesirable bacterial strain for silage, because it may be associated with the production of biogenic amines, leading to decreased protein content and nutritional value (Dunière et al., 2013). In the present work, the LAB additive treatment markedly reduced the abundance of Pseudomonas, which was harmful to the silage quality and nutrient preservation. Similar findings have been reported in corn stover, alfalfa, M. oleifera leaves, and red clover (Xu et al., 2017; Ogunade et al., 2018; Dong et al., 2019; Wang et al., 2019b). Gluconacetobacter is widely used in the production of vinegar and wine, which is a type of acetic acid-producing bacterial strain, and can consume ethanol and sugar substances (Du et al., 2018; Huang et al., 2018). The higher abundance of Gluconacetobacter resulted in more acetic acid, leading to raised pH and impaired fermentation quality.
Silage fermentation is a very complex biological process involving a variety of microorganisms, and such a process produces many different metabolites during ensiling. Lactobacillus is the core microorganism during the ensiling process, Weissella is also widely distributed in silage, and both of them produce lactic acid and determine the silage quality (Cai et al., 1998). Similar to our findings, previous studies have reported that the concentration of lactic acid is positively correlated with genera Lactobacillus and Weissella in silage (Xu et al., 2017; Guan et al., 2018; Ogunade et al., 2018; Yang et al., 2019). Meanwhile, Enterobacter converts lactic acid to acetic acid and other organic acids, so it is positively correlated with lactic acid (Ostling and Lindgren, 2010). The ammonia-N is created by the synthetic effect of plant enzymes and microbial activity, and the reduction of silage ammonia-N was attributed to the rapid acidification of LAB. Therefore, Lactobacillus was sensitive to lower pH, contributing to the high correlation coefficients between ammonia-N and Lactobacillus. Our results were consistent with the other studies (Ogunade et al., 2018; Ren et al., 2019).
The addition of LAB or CA significantly raised lactic acid content, decreased pH, and reduced contents of acetic acid, propionic acid, and ammonia-N in ensiled KG. The LAB and CA treatment also altered the bacterial community of KG silage, which reduced bacterial diversity. However, such treatments increased the abundance of desirable Lactobacillus, but decreased the abundance of undesirable Dysgonomonas and Pseudomonas. Besides, their combination treatment displayed a beneficial synergistic impact on silage fermentation, and notable influence on the bacterial community. Furthermore, the bacterial community was significantly correlated with fermentation characteristics. Collectively, our results confirmed that LAB, CA, and their combination exerted beneficial effects on KG silage fermentation.
The datasets presented in this study can be found in online repositories. The names of the repository/repositories and accession number(s) can be found below: https://catalog.data.gov/dataset/sequence-read-archive-sra, PRJNA556187.
ML, XZ, YC, and RL did the experimental design work. ML, XZ, YC, RL, and JT conducted the experiments. ML, XZ, YC, RL, JT, and HZ analyzed the data. ML, XZ, and YC wrote the manuscript. All authors read and approved the manuscript.
This study was funded by the NSF of Hainan Province (No. 319QN308), NSFC (No. 31860680), CATAS (No. 1630032017033), the Ministry of Agriculture and Rural Affairs of the People’s Republic of China (Nos. 2130109 and 12200012), and Integrated Demonstration of Key Techniques for the Industrial Development of Featured Crops in Rocky Desertification Areas of Yunnan-Guangxi-Guizhou Provinces.
The authors declare that the research was conducted in the absence of any commercial or financial relationships that could be construed as a potential conflict of interest.
Association of Official Analytical Chemists (AOAC) (1990). Official Methods of Analysis, 15th Edn. Arlington, VA: AOAC
Bao, W., Mi, Z., Xu, H., Zheng, Y., Kwok, L. Y., Zhang, H., et al. (2016). Assessing quality of Medicago sativa silage by monitoring bacterial composition with single molecule, real-time sequencing technology and various physiological parameters. Sci. Rep. 6:28358.
Cai, Y., Benno, Y., Ogawa, M., Ohmomo, S., Kumai, S., and Nakase, T. (1998). Influence of Lactobacillus spp. from an inoculant and of Weissella and Leuconostoc spp. from forage crops on silage fermentation. Appl. Environ. Microbiol. 64, 2982–2987. doi: 10.1128/aem.64.8.2982-2987.1998
Callahan, B. J., Mcmurdie, P. J., Rosen, M. J., Han, A. W., Johnson, A. J. A., and Holmes, S. P. (2016). DADA2: high-resolution sample inference from illumina amplicon data. Nat. Methods 13, 581–583. doi: 10.1038/nmeth.3869
Desta, S. T., Yuan, X. J., Li, J. F., and Shao, T. (2016). Ensiling characteristics, structural and nonstructural carbohydrate composition and enzymic digestibility of Napier grass ensiled with additives. Bioresour. Technol. 221, 447–454. doi: 10.1016/j.biortech.2016.09.068
Dong, Z., Li, J., Chen, L., Wang, S., and Shao, T. (2019). Effects of freeze–thaw event on microbial community dynamics during red clover ensiling. Front. Microbiol. 10:1559.
Du, R., Zhao, F., Peng, Q., Zhou, Z., and Han, Y. (2018). Production and characterization of bacterial cellulose produced by Gluconacetobacter xylinus isolated from Chinese persimmon vinegar. Carbohydr. Polym. 194, 200–207. doi: 10.1016/j.carbpol.2018.04.041
Dunière, L., Sindou, J., Chaucheyras-Durand, F., Chevallier, I., and Thévenot-Sergentet, D. (2013). Silage processing and strategies to prevent persistence of undesirable microorganisms. Anim. Feed Sci. Technol. 182, 1–15. doi: 10.1016/j.anifeedsci.2013.04.006
Edwards, R. A., and McDonald, P. (1978). “The chemistry of silage,” in Fermentation of Silage-a Review, ed. M. E. McCullough (West Des Moines IA: NFIA,), 27–60.
Guan, H., Yan, Y., Li, X., Li, X., Shuai, Y., Feng, G., et al. (2018). Microbial communities and natural fermentation of corn silages prepared with farm bunker-silo in Southwest China. Bioresour. Technol. 265, 282–290. doi: 10.1016/j.biortech.2018.06.018
Guo, X. S., Ke, W. C., Ding, W. R., Ding, L. M., Xu, D. M., Wang, W. W., et al. (2018). Profiling of metabolome and bacterial community dynamics in ensiled Medicago sativa inoculated without or with Lactobacillus plantarum or Lactobacillus buchneri. Sci. Rep. 8:357.
He, L., Chen, N., Lv, H., Wang, C., Zhou, W., Chen, X., et al. (2020). Gallic acid influencing fermentation quality, nitrogen distribution and bacterial community of high-moisture mulberry leaves and stylo silage. Bioresour. Technol. 295:122255. doi: 10.1016/j.biortech.2019.122255
He, L., Wang, C., Xing, Y., Zhou, W., Pian, R., Yang, F., et al. (2019a). Dynamics of proteolysis, protease activity and bacterial community of Neolamarckia cadamba leaves silage and the effects of formic acid and Lactobacillus farciminis. Bioresour. Technol. 294:122127. doi: 10.1016/j.biortech.2019.122127
He, L., Wang, C., Zhou, W., Zhang, Q., and Chen, X. (2019b). Effect of additives on fermentation characteristics, nutrient values and in vitro fermentation profile of Neolamarckia cadamba leaf silage. S. Afr. J. Anim. Sci. 49, 644–653. doi: 10.4314/sajas.v49i4.6
Hofstad, T., Olsen, I., Eribe, E. R., Falsen, E., Collins, M. D., and Lawson, P. A. (2000). Dysgonomonas gen. nov. to accommodate Dysgonomonas gadei sp. nov., an organism isolated from a human gall bladder, and Dysgonomonas capnocytophagoides (formerly CDC group DF-3). Int. J. Syst. Evol. Microbiol. 50, 2189–2195. doi: 10.1099/00207713-50-6-2189
Huang, Z. R., Hong, J. L., Xu, J. X., Li, L., Guo, W. L., and Pan, Y. Y. (2018). Exploring core functional microbiota responsible for the production of volatile flavour during the traditional brewing of Wuyi Hong Qu glutinous rice wine. Food Microbiol. 76, 487–496. doi: 10.1016/j.fm.2018.07.014
Ke, W. C., Ding, W. R., Xu, D. M., Ding, L. M., Zhang, P., Li, F. D., et al. (2017). Effect of addition of malic or citric acids on fermentation quality and chemical characteristics of alfalfa silage. J. Dairy Sci. 100, 8958–8966. doi: 10.3168/jds.2017-12875
Ke, W., Ding, W., Xu, D., Shah, M. N., Zhang, P., and Guo, X. (2018). Influences of addition of malic acid or citric acid, Lactobacillus plantarum and their mixtures on fermentation quality, proteolysis and fatty acid composition of ensiled alfalfa. Arch. Anim. Nutr. 6, 492–502. doi: 10.1080/1745039x.2018.1510156
Li, D., Ni, K., Zhang, Y., Lin, Y., and Yang, F. (2019). Fermentation characteristics, chemical composition and microbial community of tropical forage silage under different temperatures. Asian-Australas J. Anim. Sci. 32, 665–674. doi: 10.5713/ajas.18.0085
Li, F., Ke, W., Ding, Z., Bai, J., Zhang, Y., Xu, D., et al. (2020). Pretreatment of Pennisetum sinese silages with ferulic acid esterase-producing lactic acid bacteria and cellulase at two dry matter contents: fermentation characteristics, carbohydrates composition and enzymatic saccharification. Bioresour. Technol. 295:122261. doi: 10.1016/j.biortech.2019.122261
Li, M., Zhang, L., Zhang, Q., Zi, X., Lv, R., Tang, J., et al. (2020). Impacts of citric acid and malic acid on fermentation quality and bacterial community of cassava foliage silage. Front. Microbiol. 11:595622.
Li, M., Zhou, H., Zi, X., and Cai, Y. (2017). Silage fermentation and ruminal degradation of stylo prepared with lactic acid bacteria and cellulase. Anim. Sci. J. 88, 1531–1537. doi: 10.1111/asj.12795
Li, M., Zi, X., Tang, J., Zhou, H., and Cai, Y. (2019). Silage fermentation, chemical composition and ruminal degradation of king grass, cassava foliage and their mixture. Grassland Sci. 64, 210–215. doi: 10.1111/grs.12235
Li, M., Zi, X., Zhou, H., Hou, G., and Cai, Y. (2014). Effects of sucrose, glucose, molasses and cellulase on fermentation quality and in vitro gas production of king grass silage. Anim. Feed Sci. Technol. 197, 206–212. doi: 10.1016/j.anifeedsci.2014.06.016
Li, P., Ji, S., Hou, C., Tang, H., Wang, Q., and Shen, Y. (2016). Effects of chemical additives on the fermentation quality and N distribution of alfalfa silage in south of China. Anim. Sci. J. 87, 1472–1479. doi: 10.1111/asj.12600
Li, P., Zhang, Y., Gou, W., Cheng, Q., Bai, S., and Cai, Y. (2019). Silage fermentation and bacterial community of bur clover, annual ryegrass and their mixtures prepared with microbial inoculant and chemical additive. Anim. Feed Sci. Technol. 247, 285–293. doi: 10.1016/j.anifeedsci.2018.11.009
Liu, B., Huan, H., Gu, H., Xu, N., Shen, Q., and Ding, C. (2019). Dynamics of a microbial community during ensiling and upon aerobic exposure inlactic acid bacteria inoculation-treated and untreated barley silages. Bioresour. Technol. 273, 212–219. doi: 10.1016/j.biortech.2018.10.041
Lv, H., Pian, R., Xing, Y., Zhou, W., Yang, F., Chen, X., et al. (2020). Effects of citric acid on fermentation characteristics and bacterial diversity of Amomum villosum silage. Bioresour. Technol. 307:123290. doi: 10.1016/j.biortech.2020.123290
Murphy, R. P. (1958). A method for the extraction of plant samples and the determination of total soluble carbohydrates. J. Sci. Food Agric. 9, 714–717. doi: 10.1002/jsfa.2740091104
Ni, K., Wang, F., Zhu, B., Yang, J., Zhou, G., Pan, Y., et al. (2017). Effects of lactic acid bacteria and molasses additives on the microbial community and fermentation quality of soybean silage. Bioresour. Technol. 238, 706–715. doi: 10.1016/j.biortech.2017.04.055
Ni, K., Zhao, J., Zhu, B., Su, R., Pan, Y., Ma, J., et al. (2018). Assessing the fermentation quality and microbial community of the mixed silage of forage soybean with crop corn or sorghum. Bioresour. Technol. 265, 563–567. doi: 10.1016/j.biortech.2018.05.097
Ogunade, I. M., Jiang, Y., Pech Cervantes, A. A., Kim, D. H., Oliveira, A. S., Vyas, D., et al. (2018). Bacterial diversity and composition of alfalfa silage as analyzed by Illumina MiSeq sequencing: effects of Escherichia coli. O157:H7 and silage additives. J. Dairy Sci. 101, 2048–2059. doi: 10.3168/jds.2017-12876
Ostling, C., and Lindgren, S. (2010). Influences of enterobacteria on the fermentation and aerobic stability of grass silages. Grass Forage Sci. 50, 41–47. doi: 10.1111/j.1365-2494.1995.tb02292.x
Ren, F., He, R., Zhou, X., Gu, Q., Xia, Z., Liang, M., et al. (2019). Dynamic changes in fermentation profiles and bacterial community composition during sugarcane top silage fermentation: a preliminary study. Bioresour. Technol. 285:121315. doi: 10.1016/j.biortech.2019.121315
Schloss, P. D., Westcott, S. L., Ryabin, T., Hall, J. R., Hartmann, M., and Hollister, E. B. (2009). Introducing mothur: open-source, platform-independent, community-supported software for describing and comparing microbial communities. Appl. Environ. Microbiol. 75, 7537–7541. doi: 10.1128/aem.01541-09
Segata, N., Abubucker, S., Goll, J., and Schuber, A. M. (2011). Metagenomic biomarker discovery and explanation. Genome Biol. 12:R60.
Seo, S. H., Rhee, C. H., and Park, H. D. (2007). Degradation of malic acid by Issatchenkia orientalis KMBL 5774, an acidophilic yeast strain isolated from Korean grape wine pomace. J. Microbiol. 45, 521–527.
Smith, L. H. (1962). Theoretical carbohydrate requirement for alfalfa silage production. Agron J. 54, 291–293. doi: 10.2134/agronj1962.00021962005400040003x
Van Soest, P. J., Robertson, J. B., and Lewis, B. A. (1991). Symposium: carbohydrate methodology, metabolism, and nutritional implications in dairy cattle. methods for dietary fiber, neutral detergent fiber, and nonstarch polysaccharides in relation to animal nutrition. J. Dairy Sci. 74, 3583–3597. doi: 10.3168/jds.s0022-0302(91)78551-2
Vázquez-Baeza, Y., Meg, P., and Antonio, G. (2013). EMPeror: a tool for visualizing high-throughput microbial community data. GigaScience 2:16.
Wang, Y., Chen, X., Wang, C., He, L., Zhou, W., Yang, F., et al. (2019a). The bacterial community and fermentation quality of mulberry (Morus alba) leaf silage with or without Lactobacillus casei and sucrose. Bioresour. Technol. 293:122059. doi: 10.1016/j.biortech.2019.122059
Wang, Y., He, L., Xing, Y., Zhou, W., Pian, R., and Yang, F. (2019b). Bacterial diversity and fermentation quality of Moringa oleifera leaves silage prepared with lactic acid bacteria inoculants and stored at different temperatures. Bioresour. Technol. 284, 349–358. doi: 10.1016/j.biortech.2019.03.139
Xu, D., Ding, W., Ke, W., Li, F., Zhang, P., and Guo, X. (2019). Modulation of metabolome and bacterial community in whole crop corn silage by inoculating homofermentative Lactobacillus plantarum and Heterofermentative Lactobacillus buchneri. Front. Microbiol. 9:3299.
Xu, Z., He, H., Zhang, S., and Kong, J. (2017). Effects of inoculants Lactobacillus brevis and Lactobacillus parafarraginis on the fermentation characteristics and microbial communities of corn stover silage. Sci. Rep. 7:13614.
Yang, L., Yuan, X., Li, J., Dong, Z., and Shao, T. (2019). Dynamics of microbial community and fermentation quality during ensiling of sterile and nonsterile alfalfa with or without Lactobacillus plantarum inoculant. Bioresour. Technol. 275, 280–287. doi: 10.1016/j.biortech.2018.12.067
Yu, Z., Naoki, N., and Guo, X. S. (2011). Chemical changes during ensilage and in sacco degradation of two tropical grasses: rhodesgrass and guineagrass treated with cell wall-degrading enzymes. Asian Austral. J. Anim. Sci. 24, 214–221. doi: 10.5713/ajas.2011.10170
Yuan, X. J., Li, J. F., Dong, Z. H., and Shao, T. (2019). The reconstitution mechanism of napier grass microiota during the ensiling of alfalfa and their contributions to fermentation quality of silage. Bioresour. Technol. 297:122391. doi: 10.1016/j.biortech.2019.122391
Zhao, J., Xia, B., Meng, Y., Yang, Z., Pan, L., Zhou, M., et al. (2019). Transcriptome analysis to shed light on the molecular mechanisms of early responses to cadmium in roots and leaves of king grass (Pennisetum americanum × P. purpureum). Int. J. Mol. Sci. 20:2532. doi: 10.3390/ijms20102532
Zhao, Y. P., Du, L. X., Liu, L. L., and Wang, E. Y. (2004). Screening yeast degrading citric acid in Hawthorn fruit juice and its degrading characteristics. Acta Microbiol. Sin 44, 235–239.
Keywords: king grass, Lactobacillus plantarum, citric acid, silage fermentation, bacterial community
Citation: Zi X, Li M, Chen Y, Lv R, Zhou H and Tang J (2021) Effects of Citric Acid and Lactobacillus plantarum on Silage Quality and Bacterial Diversity of King Grass Silage. Front. Microbiol. 12:631096. doi: 10.3389/fmicb.2021.631096
Received: 19 November 2020; Accepted: 08 February 2021;
Published: 26 February 2021.
Edited by:
Fuyu Yang, China Agricultural University, ChinaReviewed by:
Hao Guan, Southwest Minzu University, ChinaCopyright © 2021 Zi, Li, Chen, Lv, Zhou and Tang. This is an open-access article distributed under the terms of the Creative Commons Attribution License (CC BY). The use, distribution or reproduction in other forums is permitted, provided the original author(s) and the copyright owner(s) are credited and that the original publication in this journal is cited, in accordance with accepted academic practice. No use, distribution or reproduction is permitted which does not comply with these terms.
*Correspondence: Mao Li, bGltYW9obkAxNjMuY29t; Yeyuan Chen, Y2hlbnl5MTk2MkAxMjYuY29t
†These authors have contributed equally to this work
Disclaimer: All claims expressed in this article are solely those of the authors and do not necessarily represent those of their affiliated organizations, or those of the publisher, the editors and the reviewers. Any product that may be evaluated in this article or claim that may be made by its manufacturer is not guaranteed or endorsed by the publisher.
Research integrity at Frontiers
Learn more about the work of our research integrity team to safeguard the quality of each article we publish.