- Hainan Key Laboratory for Sustainable Utilization of Tropical Bioresource, College of Tropical Crops, Hainan University, Haikou, China
The rubber tree (Hevea brasiliensis) is a tropical perennial crop for the primary source of natural rubber. Colletotrichum gloeosporioides from Hevea brasiliensis (C. gloeosporioides Hb) and Colletotrichum acutatum from Hevea brasiliensis (C. acutatum Hb) are the causal agents of rubber tree anthracnose and lead to serious loss of natural rubber production. Inoculation tests showed that C. gloeosporioides Hb possessed higher pathogenicity than C. acutatum Hb to the rubber tree. Genomic analysis revealed that an unknown gene, named CgNPG1 (a Novel Pathogenic Gene 1), was presented in the genome of C. gloeosporioides Hb but not identified in C. acutatum Hb. CgNPG1 was predicted to encode a small secretory protein without any conserved domain. To investigate the functions of CgNPG1 in C. gloeosporioides Hb and in C. acutatum Hb, the gene deletion and overexpression mutants were generated. The phenotype analysis showed that deletion of CgNPG1 led to changed conidia morphology, decreased mycelial growth, conidiation, conidia germination rate, appressorium formation rate, and pathogenicity of C. gloeosporioides Hb to the rubber tree. Meanwhile, heterogeneous expression of CgNPG1 in C. acutatum Hb significantly changed the conidia morphology and improved the mycelial growth rate, conidiation, conidia germination rate, appressorium formation rate, and the pathogenicity of C. acutatum Hb to the rubber tree. Consistently, CgNPG1 increased the expression level of CaCRZ1 and CaCMK1 in C. acutatum Hb. These data suggested that CgNPG1 contributed to mycelial growth, conidiation, the development of invasive structures, and the pathogenicity of Colletotrichum to the rubber tree, which might be related to the modulation of CaCRZ1 and mitogen-activated protein kinase CMK1. Our results provided new insight into CgNPG1 in regulating growth and pathogenicity of the Colletotrichum spp.
Introduction
Colletotrichum as asexual genus is classified into fungi imperfecti, coelomycetes (Dean et al., 2012). As one of the most common and important genus of filamentous phytopathogenic fungi, Colletotrichum caused anthracnose on a wide variety of herbaceous and woody plants in tropical, subtropical, and temperate climates, resulting in serious economic losses (Cannon et al., 2012; Dean et al., 2012; Crouch et al., 2014; Baroncelli et al., 2016). Most Colletotrichum species feed on hemibiotrophy, exhibiting initially a brief biotrophic phase with large intracellular primary hyphae and later a destructive, necrotrophic phase with narrower secondary hyphae, which ramify throughout the host tissue (Münch et al., 2008; Dean et al., 2012). Among these Colletotrichum species, Colletotrichum acutatum, and Colletotrichum gloeosporioides are frequently mentioned and reported because of early taxonomic confusion (Dean et al., 2012).
Although multiple inoculation investigations have demonstrated that most isolates of Colletotrichum spp. are relatively non-host-specific, there are still some differences in host and tissue pathogenicity between species (Peres et al., 2005; de Souza et al., 2013). For example, C. acutatum isolates from pine were non-pathogenic or weakly pathogenic to lupine and vice versa, and the isolates from fruits were not pathogenic on either pine or lupine (Lardner et al., 1999; Peres et al., 2005). In plant pathogens, host specificity is often attributed to pathogen virulence factors, including specialized secondary metabolites (SSM), toxin, cell wall-degrading enzymes, and small-secreted protein (SSP) effectors (Stukenbrock and McDonald, 2007; Brunner et al., 2013; Poppe et al., 2015; Buiate et al., 2017). Recently, several reports have revealed that horizontal gene transfer (HGT) and horizontal chromosome transfer (HCT) between plant pathogenic fungi affect their host range and virulence (Mehrabi et al., 2011; Jaramillo et al., 2014).
Mitogen-activated protein (MAP) kinase signal transduction pathways were involved in fungal pathogenesis (Dickman and Yarden, 1999), and some virulence-involved transcription factors (TFs) played important roles in fungal infection to plant (Dickman and Yarden, 1999; Son et al., 2011). For example, MAP kinase Cpmk2 from ascomycete Claviceps purpurea and mps1 from Magnaporthe grisea contributed to conidiation and virulence (Xu et al., 1998; Mey et al., 2002). MoCRZ1 from rice blast fungus Magnaporthe oryzae was essential for cell wall integrity, conidiation, pathogenicity, and Ca2+-dependent vegetative growth (Choi et al., 2009). The infection process of Colletotrichum includes germination, formation of melanized appressoria, appressorial penetration, and subsequent invasive growth in host plants. In C. lagenarium, CMK1 regulated conidial germination, appressorium formation, and pathogenicity (Takano et al., 2000). In C. gloeosporioides, CgCrzA as the homolog of CRZ1, was involved in regulating cell wall integrity and infection-related morphogenesis. Deletion of the CgCRZA in C. gloeosporioides showed severe cell wall integrity defects and inhibited the vegetative growth, conidiation, appressorial formation rate, and lost pathogenicity to plant hosts (Wang et al., 2020). These data suggested that both CMK1 and CRZ1 in Colletotrichum were involved in fungal development and pathogenesis.
The rubber tree (Hevea brasiliensis) is a tropical perennial crop for natural rubber; however, rubber latex production is seriously affected by rubber tree anthracnose (Pu et al., 2007; Cao et al., 2017), which are caused by C. gloeosporioides and C. acutatum (Brown and Soepena, 1994; Saha et al., 2002). In China, C. gloeosporioides was considered as the only pathogen of rubber tree anthracnose at first, until C. acutatum was first reported as a causal agent of rubber tree anthracnose in Yunnan Province in 2008 and in Hainan in 2010 (Zhang et al., 2008; Li et al., 2010). Further research revealed that both C. gloeosporioides and C. acutatum can induce typical water-soaked, darker, and circular lesions on rubber tree leaves, but the colony growth rate of C. gloeosporioides is significantly higher than that of C. acutatum (Cao et al., 2017). So far, little is known about the pathogenic mechanism of C. gloeosporioides and C. acutatum to rubber trees. In this study, C. gloeosporioides Hb and C. acutatum Hb were isolated from the leaves of rubber trees infected with anthracnose in Hainan province, and the following analysis showed that C. gloeosporioides Hb possesses significantly higher pathogenicity than that of C. acutatum Hb to rubber tree leaves. In order to explain the difference of pathogenicity to the rubber tree, the specific genes of C. gloeosporioides Hb, but not in C. acutatum Hb, were screened, especially CgNPG1 (a Novel Pathogenic Gene of C. gloeosporioides). Further, the biological functions of CgNPG1 have been characterized through construction of CgNPG1 deletion mutant in C. gloeosporioides Hb and CgNPG1 heterologous expression in C. acutatum Hb. These results not only extend our understanding of the pathogenesis of C. gloeosporioides Hb to the rubber tree but also provide novel insight into the host specificity mechanism of C. gloeosporioides Hb and C. acutatum Hb to the rubber tree.
Materials and Methods
Fungal Strains and Culture Conditions
Colletotrichum gloeosporioides from Hevea brasiliensis and Colletotrichum acutatum from Hevea brasiliensis strains were isolated from the leaves of Hevea brasiliensis with anthracnose in Hainan province. Both strains were grown on potato dextrose agar (PDA) at 28°C in the dark.
In silico Analysis of CgNPG1
The amino acid sequence of CgNPG1 was deduced by DNASTAR software. Prediction of signal peptides was performed online by SignalP 5.0 analysis tool1. Prediction of conserved domain and motif were performed by Motif Scan2. The bootstrap neighbor-joining phylogenetic tree was constructed using Clustal X 2.0 and MEGA X.
Vector Constructions
For the construction of the gene deletion vector, the 5′ and 3′ flanking region nucleotides of the CgNPG1 were amplified from genomic DNA and ligated into the vector pCB1532 carrying the acetolactate synthase gene (SUR) cassette from M. oryzae conferred resistance to chlorimuron ethyl (a sulfonylurea herbicide) (Supplementary Figure 1A). For construction of the complementation vector, the open read frame of CgNPG1 was amplified from cDNA, fused with the 3 X FLAG coding sequence, and cloned into the vector harboring the promoter of ToxA, the terminator of nos, and the hygromycin phosphotransferase gene (HPH) (Supplementary Figure 2A).
Transformation of Colletotrichum gloeosporioides and Colletotrichum acutatum
Protoplast preparation and transformation were carried out as described in our previous work (Wang et al., 2018). The deletion mutants were analyzed by two round PCR analyses, which were diagnostic tests for homologous integration of the 5′ part and 3′ part. Then the correct transformants were purified by single conidia isolations and analyzed by Southern blot. For Southern blot analysis, genomic DNA of wild type and the deletion mutant was extracted and digested with HindIII, and the upstream flanking region of CgNPG1 was amplified and used as probe. Another PCR was conducted for screening existence of CgNPG1. The complementation mutant of C. gloeosporioides Hb and the heterogeneous expression mutant of C. acutatum Hb were identified by PCR analysis, and the CgNPG1 expression level of the mutants was estimated by RT-PCR.
RNA Isolation, cDNA Synthesis, and qRT-PCR
RNA was extracted from the mycelium of C. gloeosporioides Hb and C. acutatum Hb using the CTAB-LiCl method (Yang et al., 2020). The contaminating DNA was eliminated using RNase-free DNase, and the first-strand cDNA was synthesized using Revert Aid First Strand cDNA Synthesis Kit (Thermo Fisher). Quantitative RT-PCR analysis was performed with the LightCycler 96 System (Roche). The beta-tubulin-1 (β-tub1) gene was used as an endogenous control for normalization. Relative expression levels of target genes were estimated using the 2–ΔΔ Ct method.
Fungal Growth Assay
For the vegetate growth assay, 5-mm-diameter disks of mycelium together with agar were inoculated on fresh potato dextrose agar medium and cultured for 9 days. The diameters of the colonies were recorded, and the growth rates were calculated. The experiment was repeated three times. Statistical analysis was performed by SPSS software (version 20), with P < 0.05 as statistically significant.
Conidiation and Appressorium Formation Assay
For the conidiation assay, conidia were harvested from the strains growing on PDA medium for 8 days and inoculated into 50-ml liquid CMC medium to the final concentration of 104/ml, respectively. Then all samples were cultured at 28°C, 150 rpm, and the conidia numbers were calculated under a microscope after incubation for 2 days.
For appressorium formation assays, 20-μl drops of conidial suspensions were placed on nylon membrane and incubated at 28°C. After 4 and 8 h post-incubation, the percentages of conidial germination and appressorium formation were determined under a microscope, respectively. The experiment was repeated three times, and at least 100 conidia were detected per replicate.
Pathogenicity Test
For the pathogenicity test, conidia suspension was used to inoculate the rubber tree variety 7–33–97 leaves at the “light green” stage. Conidia were harvested from mycelium grown on potato dextrose agar medium for 12 days in a 28°C incubator, washed with double-distilled H2O, filtered through a filter membrane (Miracloth, Millipore), and resuspended in a solution of 5% Sabouraud Maltose Broth (Difco) to a final concentration of 2 × 105 conidia/ml. Then 10 μl of the conidial suspensions were inoculated onto the wounded rubber tree leaves. The inoculated leaves were kept in a moist chamber at 28°C under natural illumination for 4 days, and the disease symptoms were scored. Each treatment contained three replicates of nine leaves, and the entire experiment was repeated three times. Statistical analysis was performed by SPSS software (version 20), with P < 0.05 as statistically significant.
Results
Pathogenicity Difference of Colletotrichum gloeosporioides Hb and Colletotrichum acutatum Hb on Rubber Tree Leaves
Colletotrichum gloeosporioides from Hevea brasiliensis and Colletotrichum acutatum from Hevea brasiliensis were isolated and characterized from rubber trees in Hainan province of China. When the detached “light green” rubber tree leaves from variety 73–3–97 were inoculated with C. gloeosporioides Hb and C. acutatum Hb, respectively, the necrotic lesions were obviously observed on the 4th day (Figure 1A). The mean size of the disease lesion was about 1.3 cm in C. gloeosporioides Hb, while that of C. acutatum Hb was only about 0.4 cm (Figure 1B), suggesting that C. gloeosporioides Hb possesses higher pathogenicity to the rubber tree than C. acutatum Hb.
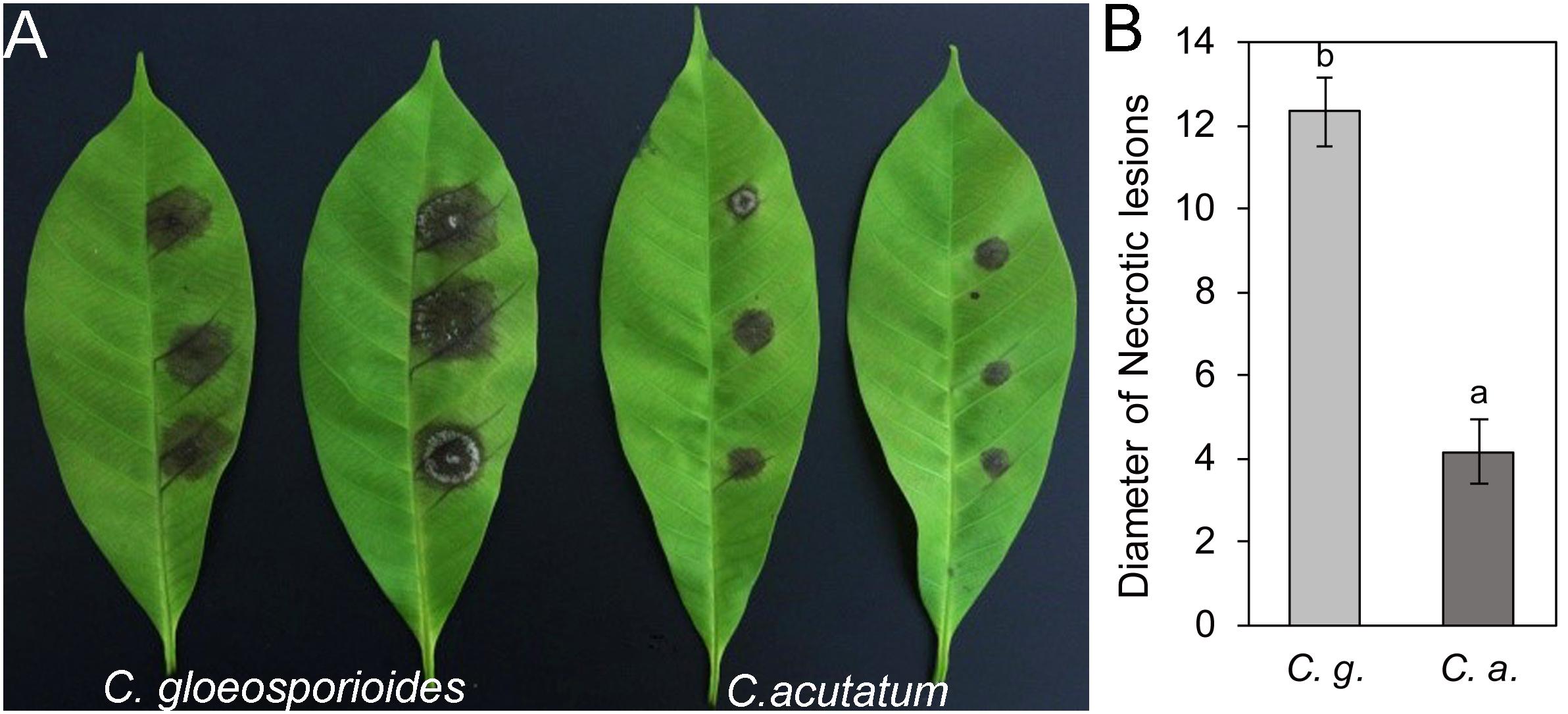
Figure 1. Pathogenicity assay of Colletotrichum gloeosporioides Hb and Colletotrichum acutatum Hb on rubber tree leaves. (A) Disease symptoms of rubber-tree leaves at 3 days post-inoculation with C. gloeosporioides Hb and C. acutatum Hb mycelium. (B) Lesion diameters at 3 day post-inoculation. Error bars indicated standard deviation, and columns with different letters indicate significant difference (P < 0.05).
Cloning and Analysis of Specific Pathogenic Genes of Colletotrichum gloeosporioides Hb
Genome sequencing of C. gloeosporioides Hb and C. acutatum Hb was produced by Illumina Hiseq2000. Both of the genome sequences have been uploaded to the NCBI database (Bioproject ID: PRJAN690880). In order to reveal the mechanism of the different pathogenicities of C. gloeosporioides Hb and C. acutatum Hb to the rubber tree, the genes encoding secretory proteins were predicted. Among these genes, three genes encoded proteins with no putative conserved domains and were specific to C. gloeosporioides Hb than to C. acutatum Hb. One of them was named CgNPG1 (a Novel Pathogenic Gene of C. gloeosporioides Hb). CgNPG1 contains a 435-bp open reading frame encoding a polypeptide of 144 amino acids with a molecular weight of approximately 15.0 kDa and a theoretical pI of 4.28. The CgNPG1 protein contained 10 cysteines, accounting for 6.9% of the total number of amino acids. Signal peptide was predicted at the N-terminal of 1 to 16 amino acids (Supplementary Figure 1). In addition, the transmembrane domain and any known conserved domains were not found in the CgNPG1 protein. In addition, the protein sequences of CgNPG1 and some highly homologous protein sequences of representative hemibiotrophic fungi, necrotrophic fungi, and oomycetes from Blastp were used to generate a phylogenetic tree. Phylogenetic tree analysis showed that the homologous proteins of CgNPG1 widely exist in hemibiotrophic fungi, necrotrophic fungi, and oomycetes, and CgNPG1 is the closest, homologous to Colletotrichum and oomycetes (Figure 2).
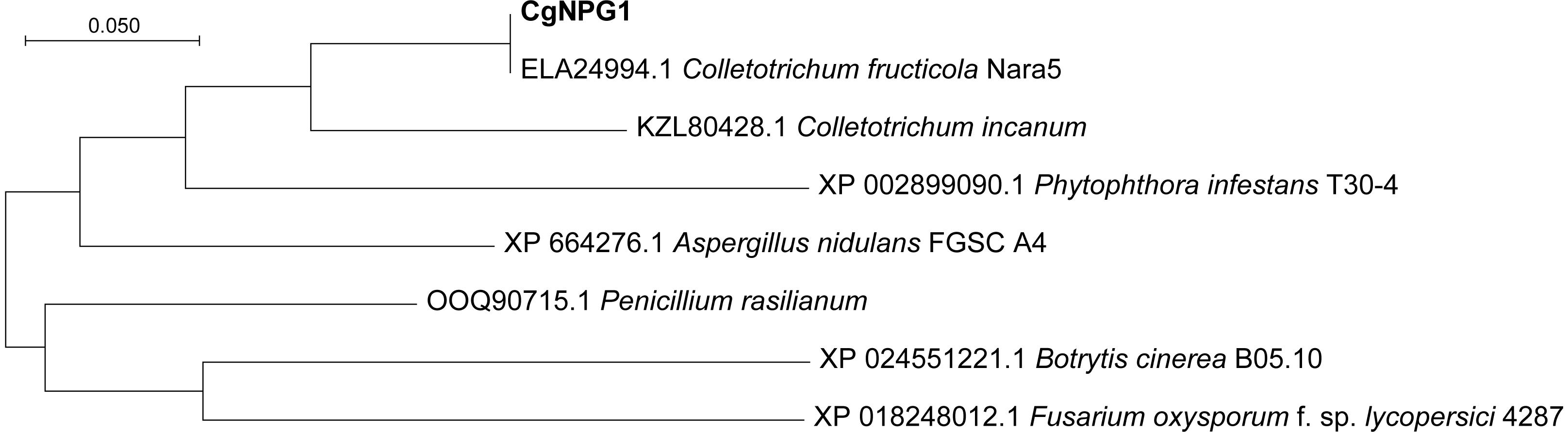
Figure 2. Phylogenetic analysis of a Novel Pathogenic Gene 1 (CgNPG1). Phylogenetic analysis of CgNPG1 and another seven orthologs by neighbor-joining methods using MEGA X software.
Generation of CgNPG1 Deletion and Complementary Mutant in Colletotrichum gloeosporioides, and Heterogeneous Expression Mutant in Colletotrichum acutatum
To investigate the functions of CgNPG1 in C. gloeosporioides Hb, the CgNPG1 gene deletion and complementation mutants were generated. In addition, the CgNPG1 heterogeneous expression mutant of C. acutatum Hb was generated to investigate its roles on C. acutatum Hb. The CgNPG1 deletion mutant of C. gloeosporioides Hb (Cg-ΔNPG1) was generated by homologous recombination strategy (Supplementary Figure 2A). PCR diagnostic analysis showed that CgNPG1 was successfully deleted from the C. gloeosporioides Hb genome (Supplementary Figure 2B). Southern blot assays revealed that the CgNPG1 had only one copy and was deleted in the Cg-ΔNPG1 mutant (Supplementary Figure 2C). The complementation strain (Cg-ResΔNPG1) was generated by expressing CgNPG1 driven by a ToxA promoter in the Cg-ΔNPG1 mutant (Supplementary Figure 3A). PCR and semi-quantitative RT-PCR analysis showed that CgNPG1 was restored in the complementary strain (Supplementary Figures 3B,C). The complementary vector of CgNPG1 was used to transform C. acutatum Hb to generate the heterogeneous expression mutant (Ca-NPG1). PCR and semiquantitative RT-PCR analysis showed that CgNPG1 was successfully integrated into the genome and expressed in C. acutatum Hb (Supplementary Figure 4).
CgNPG1 Contributes to Colony Growth of Colletotrichum gloeosporioides Hb and Colletotrichum acutatum Hb
Growth analysis showed that the deletion of CgNPG1 obviously decreased the colony growth rate in C. gloeosporioides Hb, with the colony diameter decreased to 6.5 cm in Cg-ΔNPG1 in comparison to 8 cm in Cg-WT at 8 days after inoculation. Meanwhile, the Cg-ΔNPG1 showed different colonial morphology with more aerial hyphae compared with Cg-WT. In addition, complementation of CgNPG1 restored the growth rate of Cg-ΔNPG1 (Figure 3). Heterologous expression of CgNPG1 significantly promoted the colony growth rate in C. acutatum Hb (Figure 4). These results suggested that CgNPG1 contributed to the vegetative growth of C. gloeosporioides Hb and C. acutatum Hb.
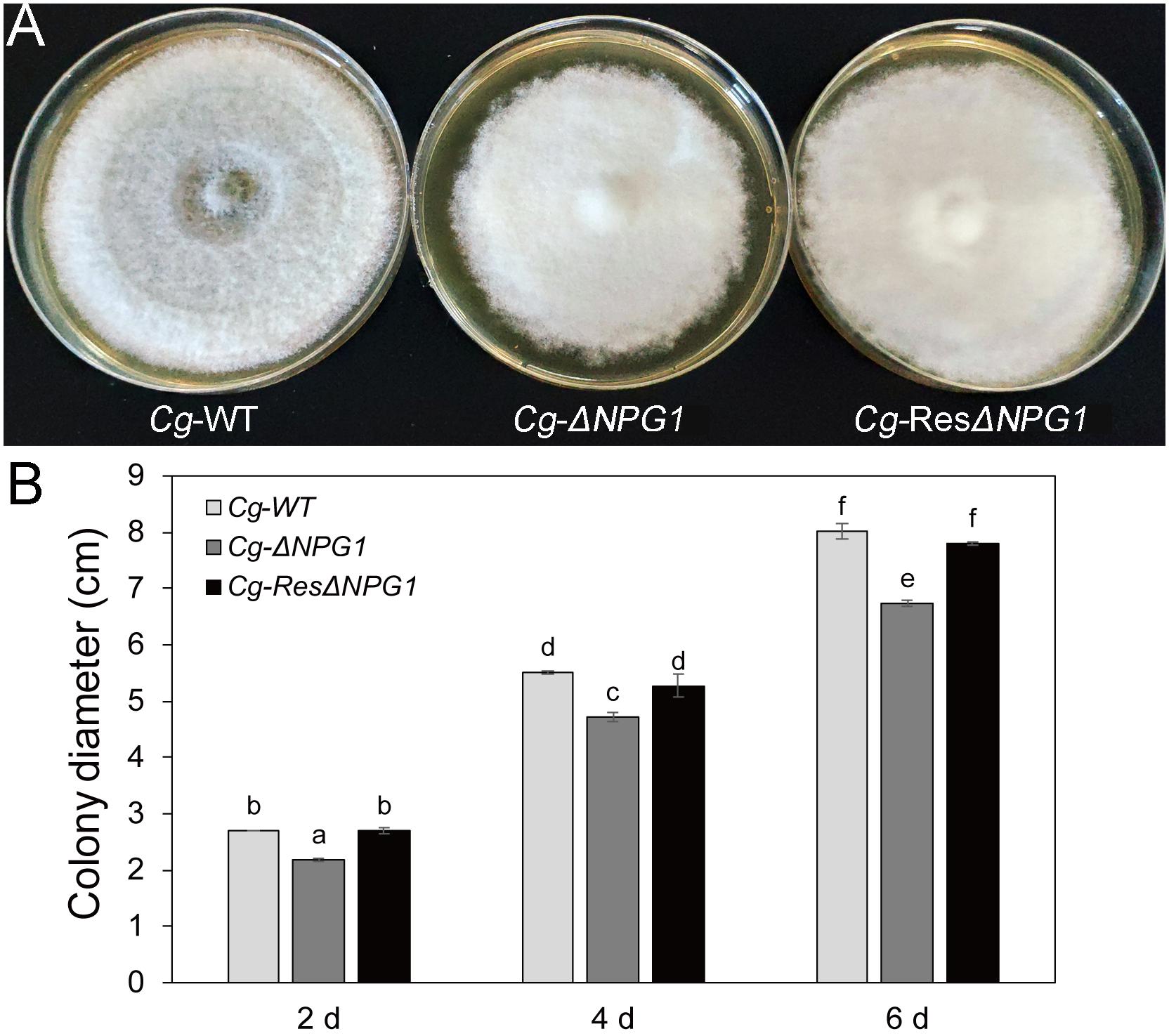
Figure 3. Growth rate assays of CgNPG1 deletion and complementation mutants of C. gloeosporioides Hb. (A) Colonial morphology of wild type strain (Cg-WT), the CgNPG1 deletion mutant (Cg-ΔNPG1), and complementation mutant (Cg-ResΔNPG1) after culture for 8 day on potato dextrose agar. (B) Colony diameters at 2, 4, and 6 day post-inoculation. Error bars indicated standard deviation, and columns with different letters indicate significant difference (P < 0.05).
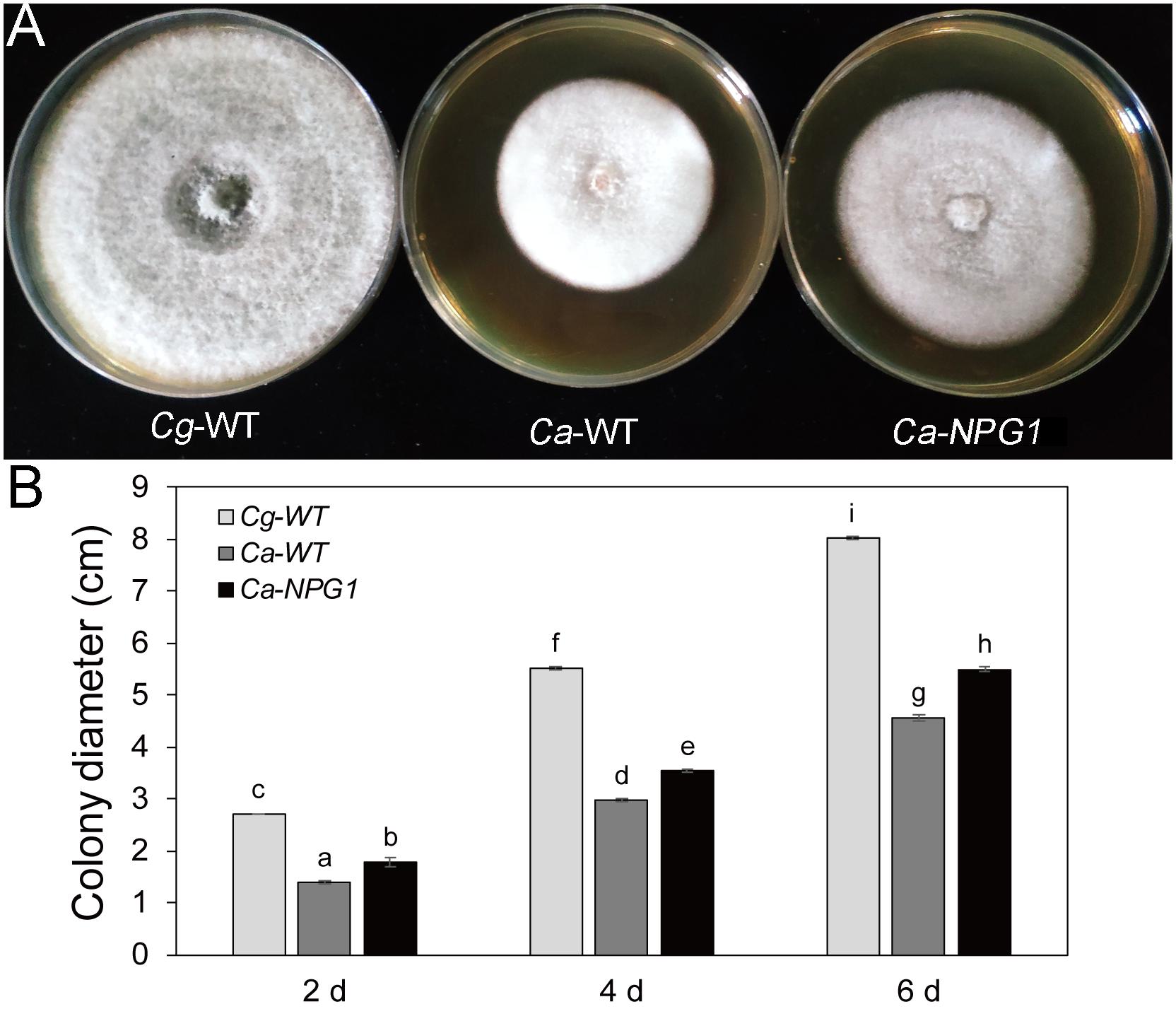
Figure 4. Growth rate assays of C. acutatum Hb strains expressing CgNPG1. (A) Colonial morphology of C. gloeosporioides Hb wild type strain (Cg-WT), C. acutatum Hb wild type strain (Ca-WT), and the CgNPG1 heterologous expression mutant (Ca-NPG1). (B) Colony diameters at 2, 4, and 6 day post-inoculation. Error bars indicated standard deviation, and columns with different letters indicate significant difference (P < 0.05).
CgNPG1 Contributes to Conidia Morphology and Conidiation of C. gloeosporioides Hb and C. acutatum Hb
The morphological observation showed that the Conidia of Cg-ΔNPG1 were significantly shorter than that of Cg-WT and complemented strains Cg-ResΔNPG1, and heterologous expression of CgNPG1 in C. acutatum Hb caused more than 65% of conidia to become blunt round at both ends and fatter compared with Ca-WT (Figure 5A). Conidiation assay showed that the conidia production of ΔCgNPG1 was drastically reduced to less than half of Cg-WT and Cg-ResΔNPG1 (Figure 5B), and heterologous expression of CgNPG1 in C. acutatum Hb doubled the conidia yield of Ca-WT (Figure 5C).
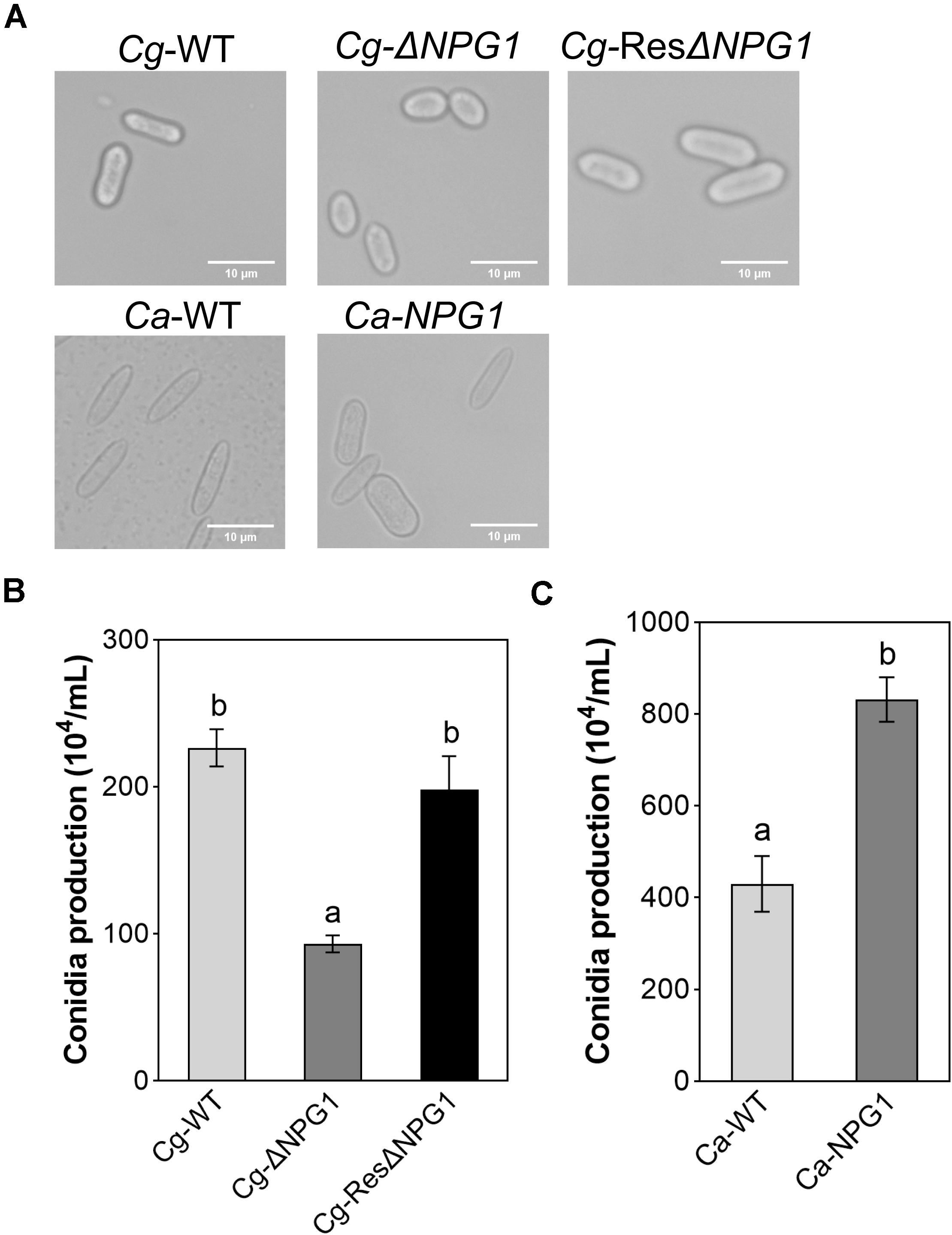
Figure 5. Conidia morphology observation and conidiation assay of CgNPG1 deletion mutant, CgNPG1 complementation mutant, and CgNPG1 heterologous expression mutant. (A) Conidial morphology of Cg-WT, Cg-ΔNPG, Cg-ResΔNPG1, Ca-WT, and Ca-NPG1. (B) The number of conidia developed by the Cg-WT, Cg-ΔNPG, Cg-ResΔNPG1. (C) The number of conidia developed by the Ca-WT and Ca-NPG1. Error bars indicated standard deviation, and columns with different letters indicate significant difference (P < 0.05).
CgNPG1 Is Involved in Conidia Germination and Appressorium Formation
The processes of conidia germination and appressorium formation was observed and counted on nylon membrane. In Cg-WT and Cg-ResΔNPG1, more than 80% of the conidia germinated after 4 h of post-incubation, compared with less than 30% in Cg-ΔNPG1 (Figures 6A,B). In the Cg-ΔNPG1mutant, over 70% of conidia failed to form abnormal appressoria after 8 h of post-incubation, compared with Cg-WT and Cg-ResΔNPG1 strains, which formed normal appressoria (Figures 6A,D). Additionally, after 4 h of post-incubation, the conidia germination rate of Ca-NPG1 strain was about 90%, which was significantly higher than that of wild type Ca-WT that was about 80% (Figure 6C). After 8 h of post-incubation, the appressorium information rate of Ca-NPG1 strain was more than 95%, which was significantly higher than that of the wild type Ca-WT strain, which was nearly 80% (Figure 6E). These data indicated that CgNPG1 was involved in the invasive structure development of C. gloeosporioides Hb, including conidia germination and normal appressorium formation, and heterologous expression of CgNPG1 in C. acutatum Hb promoted conidia germination and appressorium formation.
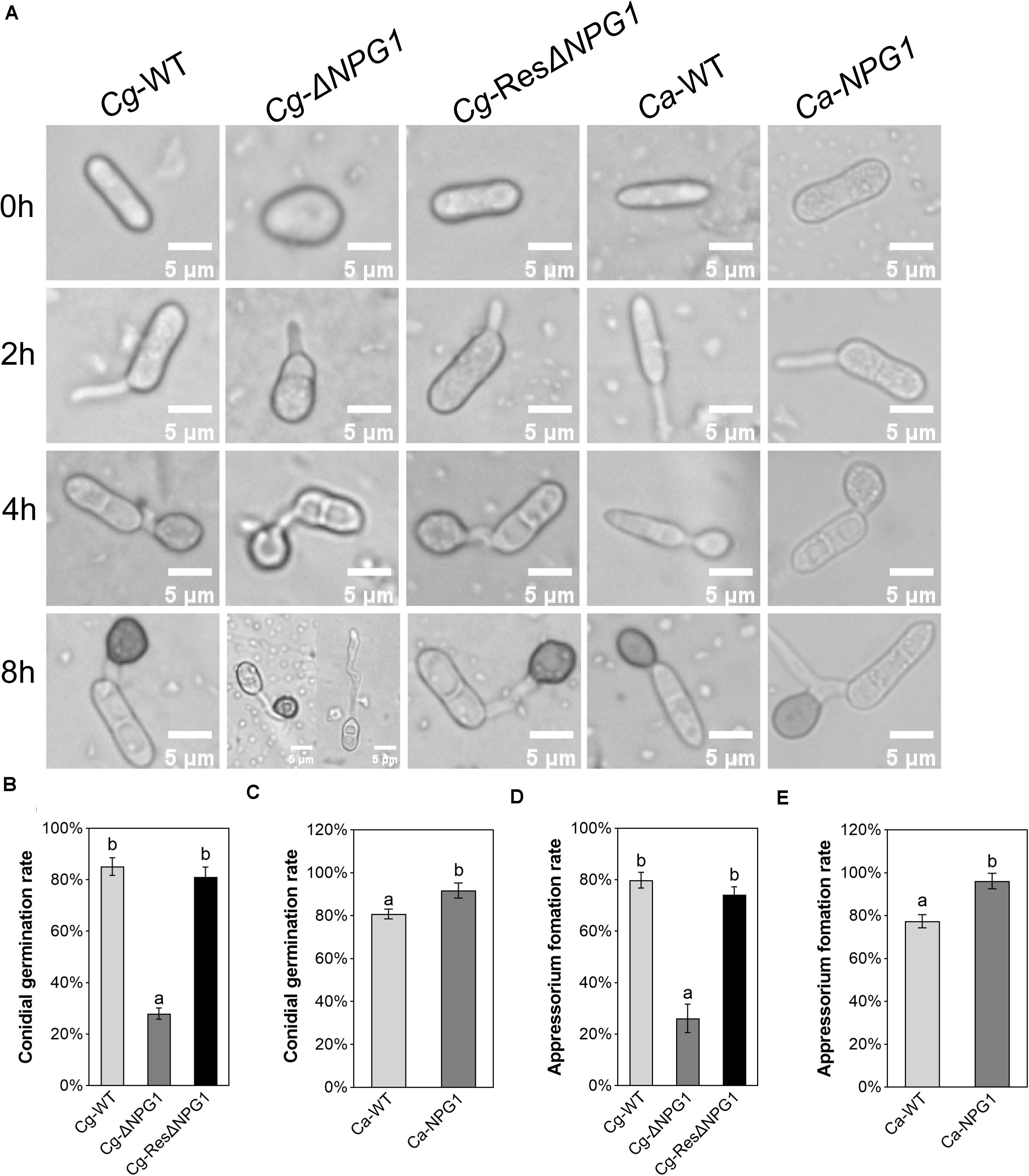
Figure 6. Conidial germination and appressorium formation assay of CgNPG1 deletion mutant and CgNPG1 heterologous expression mutant. (A) Appressorium development progress of Cg-WT, Cg-ΔNPG, Cg-ResΔNPG1, Ca-WT, and Ca-NPG1. (B) Conidia germination rates of Cg-WT, Cg-ΔNPG, Cg-ResΔNPG1 at 4 h time intervals. (C) Conidia germination rates of Ca-WT and Ca-NPG1 at 4 h time intervals. (D) Appressorium formation rates of Cg-WT, Cg-ΔNPG, Cg-ResΔNPG1 at 8 h time intervals. (E) Appressorium formation rates of Ca-WT and Ca-NPG1 at 8 h time intervals. Error bars indicated standard deviation, and columns with different letters indicate significant difference (P < 0.05).
CgNPG1 Is Required for the Virulence of Colletotrichum gloeosporioides Hb and Increases the Virulence of Colletotrichum acutatum Hb on Rubber Tree
Detached rubber tree leaves were used for pathogenicity analysis. After inoculation for 4 day, both Cg-ΔNPG1 and Cg-ResΔNPG1 caused typical anthracnose symptoms as well as Cg-WT (Figure 7A), but the mean lesion diameter caused by Cg-ΔNPG1 (only 0.9 cm) was obviously smaller than that caused by Cg-WT (over 1.4 cm) (Figure 7B). Meanwhile, Cg-ResΔNPG1 basically restored the pathogenicity of Cg-ΔNPG1, with a lesion diameter of 1.3 cm after inoculation at 4 days. Interestingly, heterologous expression of CgNPG1 in C. acutatum Hb significantly increase the pathogenicity of C. acutatum Hb to rubber tree leaves, with lesion diameters of 1.3 cm in Ca-NPG1; in contrast, the mean lesion diameter of Ca-WT was only 0.4 cm (Figures 7C,D). These data demonstrated that CgNPG1 play important roles in the pathogenicity of C. gloeosporioides Hb and C. acutatum Hb to rubber tree.
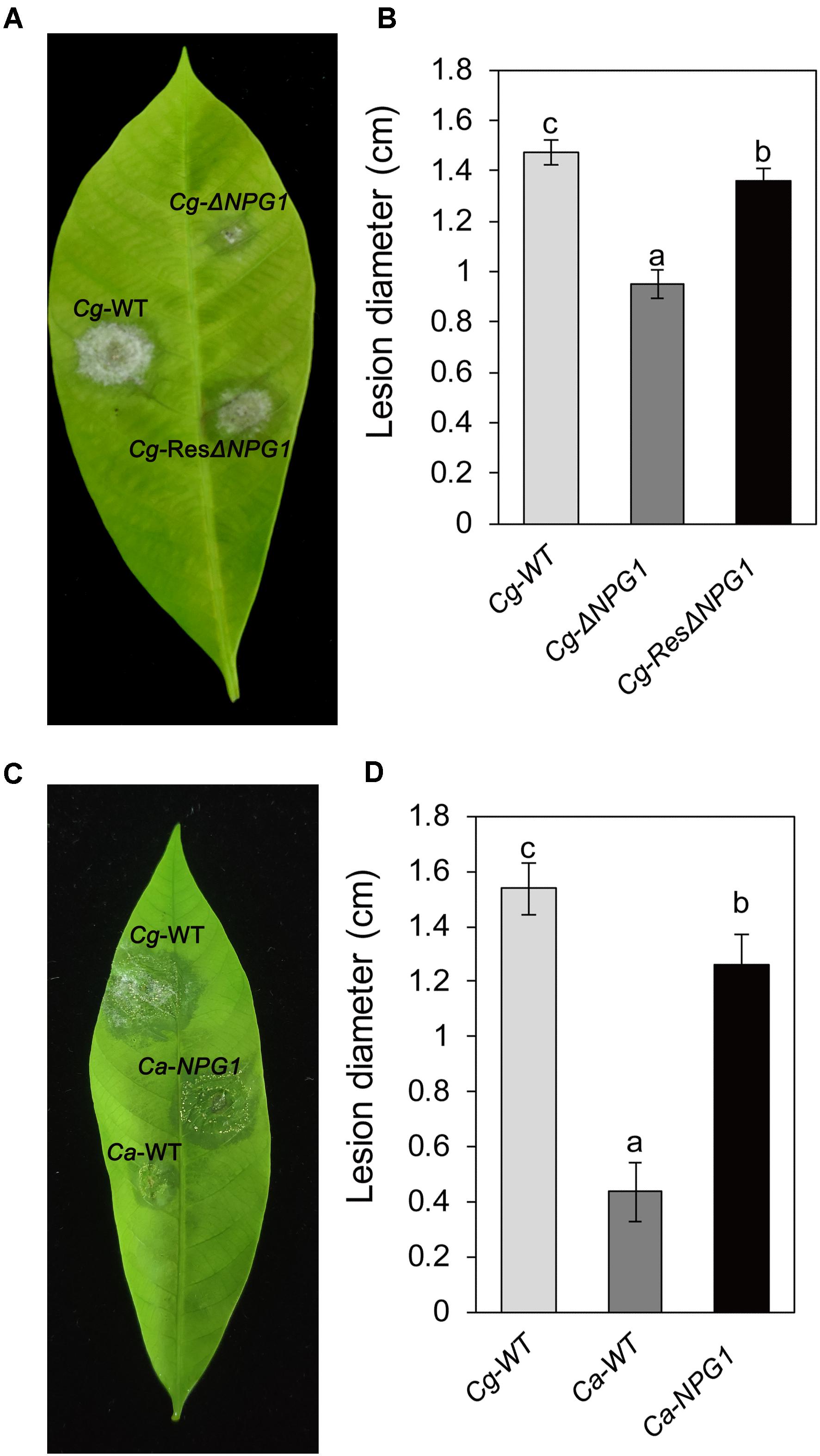
Figure 7. Pathogenicity assay of CgNPG1 deletion mutant and CgNPG1 heterologous expression mutant on rubber tree leaves. (A) Disease symptoms of rubber tree leaves at 4 days post-inoculation with conidia of C. gloeosporioides Hb wild type (Cg-WT), the CgNPG1 deletion mutant (Cg-ΔNPG1), and complementation mutant (Cg-ResΔNPG1) strains. (B) Lesion diameters at 4 day post-inoculation with conidia of Cg-WT, Cg-ΔNPG1, and Cg-ResΔNPG1 strains. (C) Disease symptoms of rubber tree leaves at 4 days post-inoculation with conidia of C. gloeosporioides Hb wild type (Cg-WT), C. acutatum Hb wild type (Ca-WT), and the CgNPG1 heterologous expression mutant (Ca-NPG1) strains. (D) Lesion diameters at 4 day post-inoculation with conidia of Cg-WT, Ca-WT, and Ca-NPG1 strains. Error bars indicated standard deviation, and columns with different letters indicate significant difference (P < 0.05).
CgNPG1 Increased the Expression of CaCRZ1 and CaCMK1 in Colletotrichum acutatum Hb
CRZ1 and CMK1 act as regulators of the upstream signals to control the growth, conidiation, invasive structures development, and pathogenicity in fungal pathogens (Mey et al., 2002; Wang et al., 2020). Here, heterologous expression of CgNPG1 in C. acutatum Hb obviously upregulated the transcription of CaCRZ1 and CaCMK1, with the expression level of CaCRZ1 increased to twofold and CaCMK1 to 1.3-fold (Figure 8), indicating that the effect of CgNPG1 on C. acutatum Hb might be related to the modulation of CaCRZ1 and CaCMK1.
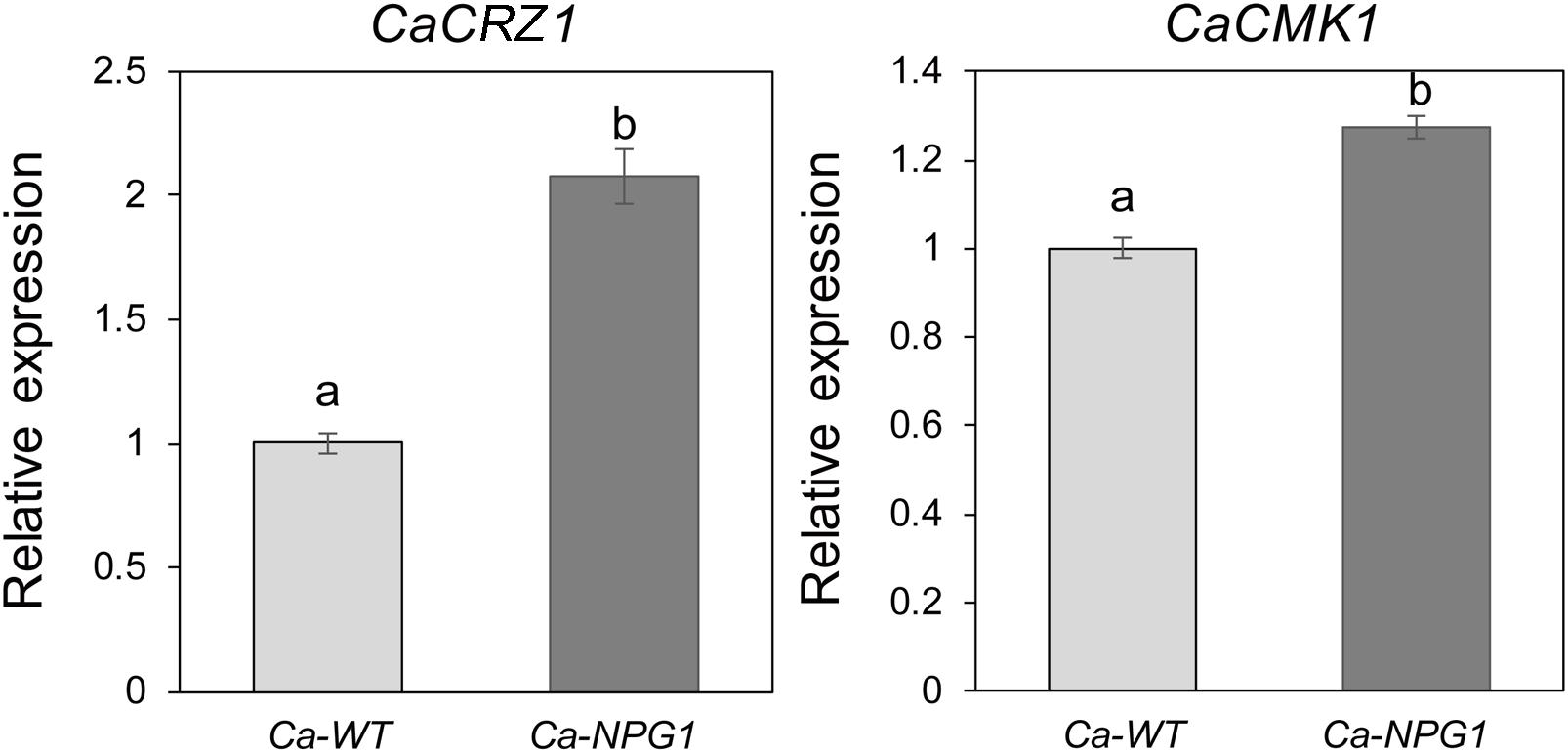
Figure 8. Quantitative RT-PCR analysis. Relative expression levels of CaCRZ1 and CaCMK1 were estimated using the 2− ΔΔ Ct method with beta-tubulin 1 as the reference gene. Error bars indicated standard deviation, and columns with different letters indicate significant difference (P < 0.05).
Discussion
Colletotrichum spp. is one of the most common and important genera of plant pathogenic fungi, causing anthracnose on important staple food crops (Dean et al., 2012). Although both C. gloeosporioides and C. acutatum could cause typical anthracnose on rubber tree (Hevea brasiliensis) (Zhang et al., 2008; Li et al., 2010), there is virulence difference between the two pathogens. Consistently, the anthracnose caused by C. gloeosporioides Hb was more severe than that by C. acutatum Hb, suggesting that C. gloeosporioides Hb possesses higher pathogenicity. For the same race of plant pathogens, individual pathogenic isolates possesses different abilities to infect just one or a few host species, and these different isolates are conventionally merged into infraspecific assemblages, known as formae speciales (ff. spp.). Different isolates of the same formae speciales could be further separated into different virulence groups. Genomic analysis revealed that the weakly virulent isolates often lack some virulence factors, including TFs, effectors, cell wall-degrading enzymes, and toxins (Stukenbrock and McDonald, 2007; de Vega-Bartol et al., 2011; Brunner et al., 2013; Poppe et al., 2015; Buiate et al., 2017). Notably, the presence of particular small secreted proteins is associated with the determination of host range in some phytopathogenic fungi, including Alternaria spp., Cochliobolus spp., and Fusarium spp. (Ito et al., 2004; Houterman et al., 2008; Bhadauria et al., 2015). Therefore, the objective of this study was to investigate whether there are special virulence factors that contribute to the virulence difference between C. gloeosporioides Hb and C. acutatum Hb, especially CgNPG1 as a potential specific pathogenic factor in C. gloeosporioides Hb. Although no conserved domains was identified in CgNPG1, phylogenetic tree analysis showed that the homologous proteins of CgNPG1 widely existed in plant pathogens, including the most common fungal pathogens and oomycetes (Phytophthora infestans), and human pathogens (Aspergillus nidulans and Penicillium brasilianum) (Figure 2). CgNPG1 contained 144 amino acids, of which 10 cysteines accounted for 6.9% and did not have any transmembrane domain except a signal peptide at the N-terminal of 1 to 16 amino acids (Supplementary Figure 1). Usually, the SSPs classified as effectors had the following characteristics: the sequence length of ≤300 amino acids and ≥4 cysteines, and function as key infection factors in suppressing host defense responses and modulating its physiology (Feldman et al., 2020). In our study, CgNPG1 matched the above sequence characteristics and effects in mycelial growth, conidiation, invasive structure development, and pathogenesis (Figures 3–7). Moreover, the prediction results by EeffectorP1.0 software showed that CgNPG1 was an effector. Based on the above data, CgNPG1 could be considered as an effector.
To investigate the function of CgNPG1, its nucleotide sequence was deleted from the genome of C. gloeosporioides Hb and heterogeneously expressed in C. acutatum Hb. In vitro test showed that mycelial growth of Cg-ΔNPG1 mutant on PDA medium decreased slightly but was still comparable to that of the wild type, and growth rate recovered to the wild type level in the restored transformants (Figure 3). Heterogeneous expression of CgNPG1 in C. acutatum Hb obviously increased the colony growth rate (Figure 4). Leaf inoculation tests showed that deletion of CgNPG1 in C. gloeosporioides Hb resulted in significant decreased pathogenicity. Although Cg-ΔNPG1 mutant could infect the leaves and develop necrotic lesions, but the lesion diameters decreased by about 50% compared to that of the WT and the restored transformants (Figures 7A,B). Heterogeneous expression of CgNPG1 in C. acutatum Hb significantly increased the pathogenicity of C. acutatum Hb to the rubber tree (Figures 7C,D), with the disease severity of Ca-NPG1 increased by about 60% compared with the Ca-WT strain. Interestingly, the deletion of CgNPG1 in C. gloeosporioides Hb decreased the conidiation and changed the conidia morphology, and heterogeneous expression of CgNPG1 in C. acutatum Hb increased the conidiation and also changed the morphology. In addition, we also found that the deletion of CgNPG1 could affect conidia germination and appressorium formation (Figure 6). Since CgNPG1 was required for the mycelial growth, conidiation, conidia morphology, and germination, and pathogenicity of C. gloeosporioides Hb and C. acutatum Hb, the deletion of CgNPG1 in C. acutatum Hb might contribute to the poor pathogenicity to the rubber tree, at least partially.
Specialization to a new host is possible through advantageous mutations, vertical inheritance, and gene transfer events. Gene transfer (HGT and HCT) is associated with host specificity (Jaramillo et al., 2014). In Fusarium oxysporum, transfer of lineage-specific chromosomes between strains by HCT could even convert a non-pathogenic strain into a pathogen (Ma et al., 2010). For HGT, the candidate transferred genes are those constantly subject to gene duplication and gene loss. In Pezizomycotina, although no evidence of a burst of HGT events coinciding with major geological events was found, HGT appears to be occurring at a steady rate during their evolution (Jaramillo et al., 2014). Between two vegetative incompatible biotypes of C. gloeosporioides, it has been proved that HCT can occur during co-cultivation under laboratory conditions and most likely in nature (Masel et al., 1996; He et al., 1998). Moreover, 11 HGT events from bacteria into Colletotrichum or their ancestors have been identified, and some of the genes play roles in virulence (Jaramillo et al., 2014). In our case, to explore the possibility of HGT event in Colletotrichum, we constructed the phylogenetic relationships among the fungi close to C. gloeosporioides based on their 28S ribosomal sequences (Supplementary Figure 5). Referring to the phylogenetic tree, none homolog of NPG1 was further found in these close organisms other than Colletotrichum. However, the high similarity was found between NPG1 genes in Colletotrichum and Phytophthora (Supplementary Figure 6). Collectively, the results indicated the occurrence of HGT events.
Perception of environmental signals initiates intracellular signal transduction pathways and promote infection-related morphogenesis in phytopathogens. Ca2+-calmodulin signal transduction pathways controls a wide array of processes in cell growth and development (Berridge et al., 2003) through the mediation of transcription factors (Cyert, 2003). As a major component of Ca2+ signaling pathway, MoCRZ1 was involved in growth, conidiation, and pathogenicity (Choi et al., 2009). As CRZ1 homolog in C. gloeosporioides s.s. strain SMCG1#C, CgCrzA was also involved in vegetative growth, conidiation, appressorial formation rate, and lost pathogenicity to plant hosts (Wang et al., 2020). In addition, MAP kinase cascades are also an important regulatory system in cells (Dean, 1997). In C. lagenarium, CMK1 regulates the conidiation, appressoria formation, and pathogenic growth (Takano et al., 2000). Here, our results showed that the mutant heterogeneously expressing CgNPG1 (Ca-NPG1) exhibited changed conidia morphology (Figure 5A), enhanced mycelia growth rate (Figure 4), conidiation (Figure 5C), conidia germination rate, appressorial formation rate (Figures 6C,E), and pathogenicity to rubber trees (Figures 7C,D). These results suggested that the function of CgNPG1 was similar to the function of CgCrzA and CMK1. Besides that, our results also showed that heterogeneous expression of CgNPG1 significantly increased the expression level of CaCRZ1 and induced the transcription of CaCMK1 in C. acutatum Hb (Figure 8), suggesting that the regulation of CgNPG1 on C. acutatum Hb might be related to CaCRZ1 and CaCMK1.
Taken together, these data suggested that C. gloeosporioides Hb-specific CgNPG1 played important roles in controlling mycelial growth, conidiation, and the development of invasive structures, such as conidia morphology and germination, appressorium formation, and pathogenicity.
Data Availability Statement
The original contributions presented in the study are included in the article/Supplementary Material, further inquiries can be directed to the corresponding author.
Author Contributions
CL carried out most of the experiments and analyzed the data. BZ carried out the complementation experiments and the data assay. YZ cloned the CgNPG1 gene. HY completed the bioinformatics analysis of HGT. CL, BA, and HL wrote the manuscript. CH revised the manuscript. All authors read and approved the final manuscript.
Funding
This study was supported by grants from the National Natural Science Foundation of China (Grant Nos. 31860478 and 32060591).
Conflict of Interest
The authors declare that the research was conducted in the absence of any commercial or financial relationships that could be construed as a potential conflict of interest.
Supplementary Material
The Supplementary Material for this article can be found online at: https://www.frontiersin.org/articles/10.3389/fmicb.2021.629387/full#supplementary-material
Supplementary Figure 1 | Nucleotide sequence and deduced amino acid sequence of CgNPG1. The predicted signal peptide is shaded by medium gray.
Supplementary Figure 2 | Generation and molecular confirmation of CgNPG1 deletion mutant (Cg-ΔNPG1). (A) The gene deletion strategy. (B) Diagnostic PCR analysis for correct integration of recombinant fragment into the CgNPG1 locus. (C) Southern blot analysis of wild type and Cg-ΔNPG1 mutant.
Supplementary Figure 3 | Generation and molecular confirmation of CgNPG1 complementation mutant (Cg-ResΔNPG1). (A) The diagram of complementation vector. (B) Diagnostic PCR analysis for integration of CgNPG1 into the genome of the CgNPG1 deletion mutant. (C) Semi-quantitative RT-PCR analysis of CgNPG1 expression level in Cg-ΔNPG1 and Cg-ResΔNPG1.
Supplementary Figure 4 | Generation and molecular identification of mutant with heterogenous Expression of CgNPG1 in C. acutatum Hb (Ca-NPG1). (A) Diagnostic PCR analysis for integration of CgNPG1 open read frame into the genome of C. acutatum Hb. (B) Semi-quantitative RT-PCR analysis of CgNPG1 expression level in Ca-NPG1strains.
Supplementary Figure 5 | Phylogenetic tree of top 600 fungi with close relationships to C. gloeosporioides Hb utilizing 28 s sequences (Ribosomal Database Project, Release 11, http://rdp.cme.msu.edu).
Supplementary Figure 6 | Phylogenetic tree of top 10 sequences with close relationships to CgNPG1.
Footnotes
References
Baroncelli, R., Amby, D. B., Zapparata, A., Sarrocco, S., Vannacci, G., Floch, G. L., et al. (2016). Gene family expansions and contractions are associated with host range in plant pathogens of the genus Colletotrichum. BMC Genomics 17:555. doi: 10.1186/s12864-016-2917-6
Berridge, M. J., Bootman, M. D., and Roderick, H. L. (2003). Calcium signaling: dynamics, homeostasis and remodeling. Nat. Rev. Mol. Cell Biol. 4, 517–529. doi: 10.1038/nrm1155
Bhadauria, V., Maclachlan, R., Pozniak, C., and Banniza, S. (2015). Candidate effectors contribute to race differentiation and virulence of the lentil anthracnose pathogen Colletotrichum lentis. BMC Genomics 16:628. doi: 10.1186/s12864-015-1836-2
Brown, A., and Soepena, H. (1994). Pathogenicity of Colletotrichum acutatum and C. gloeosporioide on leaves of Hevea spp. Mycol. Res. 98, 264–266. doi: 10.1016/S0953-7562(09)80453-X
Brunner, P. C., Torriani, S. F. F., Croll, D., Stukenbrock, E. H., and McDonald, B. A. (2013). Coevolution and life cycle specialization of plant cell wall degrading enzymes in a hemibiotrophic pathogen. Mol. Biol. Evol. 30, 1337–1347. doi: 10.1093/molbev/mst041
Buiate, E. A. S., Xavier, K. V., Moore, N., Torres, M. F., Farman, M. L., Schardl, C. L., et al. (2017). A comparative genomic analysis of putative pathogenicity genes in the host-specific sibling species Colletotrichum graminicola and Colletotrichum sublineola. BMC Genomics 18:67. doi: 10.1186/s12864-016-3457-9
Cannon, P. F., Damm, U., Johnston, P. R., and Weir, B. S. (2012). Colletotrichum- current status and future directions. Stud. Mycol. 73, 181–213. doi: 10.3114/sim0014
Cao, X., Xu, X., Che, H., West, S. J., and Luo, D. (2017). Distribution and fungicide sensitivity of Colletotrichum species complexes from rubber tree in Hainan, China. Plant Dis. 101, 1774–1780. doi: 10.1094/PDIS-03-17-0352-RE
Choi, J., Kim, Y., Kim, S., Park, J., and Lee, Y. H. (2009). MoCRZ1, a gene encoding a calcineurin-responsive transcription factor, regulates fungal growth and pathogenicity of Magnaporthe oryzae. Fungal Genet. Biol. 46, 243–254. doi: 10.1016/j.fgb.2008.11.010
Crouch, J., O’Connell, R., Gan, P., Buiate, E., Torres, M. F., Beirn, L., et al. (2014). “The genomics of Colletotrichum,” in Genomics of Plant-Associated Fungi: Monocot Pathogens, eds R. A. Dean, A. Lichens-Park, and C. Kole. Berlin: Springer, 69–102. doi: 10.1007/978-3-662-44053-7_3
Cyert, M. (2003). Calcineurin signaling in Saccharomyces cereviside: how yeast go crazy in response to stress. Biochem. Biophys. Res. Commun. 311, 1143–1150. doi: 10.1016/S0006-291X(03)01552-3
de Souza, A., Delphino Carboni, R. C., Wickert, E., De Macedo, L. E. G., and de Goes, A. (2013). Lack of host specificity of Colletotrichum spp. isolates associated with anthracnose symptoms on mango in Brazil. Plant Pathol. 62, 1038–1047. doi: 10.1111/ppa.12021
de Vega-Bartol, J. J., Martín-Dominguez, R., Ramos, B., García-Sánchez, M. A., and Díaz-Mínguez, J. M. (2011). New virulence groups in Fusarium oxysporum f. sp. phaseoli: the expression of the gene coding for the transcription factor FTF1 correlates with virulence. Phytopathology 101, 470–479. doi: 10.1094/PHYTO-09-10-0252
Dean, R., Kan, J. A. V., Pretorius, Z. A., Hammond-Kosack, K. E., Di Pietro, A., Spanu, P. D., et al. (2012). The top 10 fungal pathogens in molecular plant pathology. Mol. Plant Pathol. 13, 414–430. doi: 10.1111/j.1364-3703.2012.00822.x
Dean, R. A. (1997). Signal pathways and appressorium morphogenesis. Annu. Rev. Phytopathol. 35, 211–234. doi: 10.1146/annurev.phyto.35.1.211
Dickman, M. B., and Yarden, O. (1999). Serine/threonine protein kinases and phosphatases in filamentous fungi. Fungal Genet. Biol. 26, 99–117. doi: 10.1006/fgbi.1999.1118
Feldman, D., Yarden, O., and Hadar, Y. (2020). Seeking the roles for fungal small-secreted proteins in affecting saprophytic lifestyles. Front. Microbiol. 11:455. doi: 10.3389/fmicb.2020.00455
He, C., Rusu, A. G., Poplawski, A. M., Irwin, J. A. G., and Manners, J. M. (1998). Transfer of a supernumerary chromosome between vegetatively incompatible biotypes of the fungus Colletotrichum gloeosporioides. Genetics 150, 1459–1466. doi: 10.1017/S0016672398003528
Houterman, P. M., Cornelissen, B. J. C., and Rep, M. (2008). Suppression of plant resistance gene-based immunity by a fungal effector. PLoS Pathog. 4:e1000061. doi: 10.1371/journal.ppat.1000061
Ito, K., Tanaka, T., Hatta, R., Yamamoto, M., and Tsuge, T. (2004). Dissection of the host range of the fungal plant pathogen Alternaria alternata by modification of secondary metabolism. Mol. Microbiol. 52, 399–411. doi: 10.1111/j.1365-2958.2004.04004.x
Jaramillo, V. D. A., Sukno, S. A., and Thon, M. R. (2014). Identification of horizon- tally transferred genes in the genus Colletotrichum reveals a steady tempo of bacterial to fungal gene transfer. BMC Genomics 16:2. doi: 10.1186/1471-2164-16-2
Lardner, R., Johnston, P. R., Plummer, K. M., and Pearson, M. N. (1999). Morphological and molecular analysis of Colletotrichum acutatum sensu lato. Mycol. Res. 103, 275–285. doi: 10.1017/S0953756298007023
Li, J. F., Liu, X. B., Cai, J. M., Lin, C. H., and Huang, G. X. (2010). Identification and the internal transcribed spacer regions analysis of pathogen causing rubber anthracnose. Chin. Agricul. Sci. Bull. 26, 221–226.
Ma, L. J., van der Does, H. C., Borkovich, K. A., Coleman, J. J., Daboussi, M., Di Pietro, A., et al. (2010). Comparative genomics reveals mobile pathogenicity chromosomes in Fusarium. Nature 464, 367–373. doi: 10.1038/nature08850
Masel, A. M., He, C. Z., Poplawski, A. M., Irwin, J. A. G., and Manners, J. M. (1996). Molecular evidence for chromosome transfer between biotypes of Colletotrichum gloeosporioides. Mol. Plant Microbe Interact. 9, 339–348. doi: 10.1094/MPMI-9-0339
Mehrabi, R., Bahkali, A. H., Abd-Elsalam, K. A., Moslem, M., M’Barek, S. B., Gohari, A. M., et al. (2011). Horizontal gene and chromosome transfer in plant pathogenic fungi affecting host range. FEMS Microbiol. Rev. 35, 542–554. doi: 10.1111/j.1574-6976.2010.00263.x
Mey, G., Held, K., Scheffe, R. J., Tenberge, K. B., and Tudzynski, P. (2002). CPMK2, an SLT2-homologous mitogen-activated protein (MAP) kinase, is essential for pathogenesis of Claviceps purpurea on rye: evidence for a second conserved pathogenesis-related MAP kinase cascade in phytopathogenic fungi. Mol. Microbiol. 46, 305–318. doi: 10.1046/j.1365-2958.2002.03133.x
Münch, S., Lingner, U., Floss, D. S., Ludwing, N., Sauer, N., and Desing, H. B. (2008). The hemibiotrophic lifestyle of Colletotrichum species. Plant Physiol. 165, 41–51. doi: 10.1016/j.jplph.2007.06.008
Peres, N. A., Adaskaveg, J. E., and Correll, J. C. (2005). Life styles of Colletotrichum acutatum. Plant Dis. 89, 784–796. doi: 10.1094/PD-89-0784
Poppe, S., Dorsheimer, L., Happel, P., and Stukenbrock, E. H. (2015). Rapidly evolving genes are key players in host specialization and virulence of the fungal wheat pathogen Zymoseptoria tritici (Mycosphaerella graminicola). PLoS Pathog. 11:e1005055. doi: 10.1371/journal.ppat.1005055
Pu, J. J., Zhang, X., Qi, Y. X., Xie, Y. X., Zhang, H. Q., and Zhang, H. (2007). First record of Corynespora leaf fall disease of Hevea rubber tree in China. Australas. Plant Dis. Notes 2, 35–36. doi: 10.1071/DN07017
Saha, T., Kumar, A., Ravindran, M., Jacob, C. K., Roy, B., and Nazeer, M. A. (2002). Identification of Colletotrichum acutatum from rubber using random amplified polymorphic DNAs and ribosomal DNA polymorphisms. Mycol. Res. 106, 215–221. doi: 10.1017/S0953756201005342
Son, H., Seo, Y. S., Min, K., Park, A. R., Lee, J., Jin, J. M., et al. (2011). A phenome-based functional analysis of transcription factors in the cereal head blight fungus, Fusarium graminearum. PLoS Pathog. 7:e1002310. doi: 10.1371/journal.ppat.1002310
Stukenbrock, E. H., and McDonald, B. A. (2007). Geographical variation and positive diversifying selection in the host-specific toxin SnToxA. Mol. Plant Pathol. 8, 321–332. doi: 10.1111/j.1364-3703.2007.00396.x
Takano, Y., Kikuchi, T., Kubo, Y., John, E. H., Mise, K., and Furusawa, I. (2000). The Colletotrichum lagenarium MAP kinase gene CMK1 regulates diverse aspects of fungal pathogenesis. Mol. Plant Microbe Interact. 13, 374–383. doi: 10.1094/MPMI.2000.13.4.374
Wang, P., Li, B., Pan, Y., Zhang, Y., Li, D., and Huang, L. (2020). Calcineurin-responsive transcription factor CgCrzA is required for cell wall integrity and infection-related morphogenesis in Colletotrichum gloeosporioides. Plant Pathol. J. 36, 385–397. doi: 10.5423/PPJ.OA.04.2020.0071
Wang, Q., An, B., Hou, X., Guo, Y., Luo, H., and He, C. (2018). Dicer-like proteins regulate the growth, conidiation, and pathogenicity of Colletotrichum gloeosporioides from Hevea brasiliensis. Front. Microbiol. 8:2621. doi: 10.3389/fmicb.2017.02621
Xu, J. R., Staiger, C. J., and Hamer, J. E. (1998). Inactivation of the mitogen-activated protein kinase Mps1 from the rice blast fungus prevents penetration of host cells but allows activation of plant defense responses. Proc. Natl. Acad. Sci. U. S. A. 95, 12713–12718. doi: 10.1073/pnas.95.21.12713
Yang, J., Wang, Q., Luo, H., He, C., and An, B. (2020). HbWRKY40 plays an important role in the regulation of pathogen resistance in Hevea brasiliensis. Plant Cell Rep. 39:1095-1107. doi: 10.1007/s00299-020-02551-x
Keywords: Colletotrichum gloeosporioides, Colletotrichum acutatum, CgNPG1, mycelial growth, conidiation, pathogenicity
Citation: Liang C, Zhang B, Zhou Y, Yin H, An B, Lin D, He C and Luo H (2021) CgNPG1 as a Novel Pathogenic Gene of Colletotrichum gloeosporioides From Hevea brasiliensis in Mycelial Growth, Conidiation, and the Invasive Structures Development. Front. Microbiol. 12:629387. doi: 10.3389/fmicb.2021.629387
Received: 14 November 2020; Accepted: 02 February 2021;
Published: 08 March 2021.
Edited by:
Yong Wang, Guizhou University, ChinaReviewed by:
Zhibo Zhao, Guizhou University, ChinaOded Yarden, Hebrew University of Jerusalem, Israel
Copyright © 2021 Liang, Zhang, Zhou, Yin, An, Lin, He and Luo. This is an open-access article distributed under the terms of the Creative Commons Attribution License (CC BY). The use, distribution or reproduction in other forums is permitted, provided the original author(s) and the copyright owner(s) are credited and that the original publication in this journal is cited, in accordance with accepted academic practice. No use, distribution or reproduction is permitted which does not comply with these terms.
*Correspondence: Hongli Luo, aGxsdW9AaGFpbmFudS5lZHUuY24=
†These authors have contributed equally to this work