- 1Department of Global Health and Infection, Brighton and Sussex Medical School, Brighton, United Kingdom
- 2Department of Microbiology, Soba University Hospital, University of Khartoum, Khartoum, Sudan
- 3Faculty of Medical Laboratories, Microbiology Department, Ibn Sina University, Khartoum, Sudan
- 4Bioscience Research Institute, Ibn Sina University, Khartoum, Sudan
- 5College of Health Sciences, Medical Laboratory Sciences Program, Gulf Medical University, Ajman, United Arab Emirates
- 6Institute for Medical Microbiology, Immunology and Hygiene, University of Cologne, Cologne, Germany
- 7German Centre for Infection Research, Partner Site Bonn-Cologne, Cologne, Germany
Carbapenem resistant Acinetobacter baumannii (CRAb) is an important global pathogen contributing to increased morbidity and mortality in hospitalized patients, due to limited alternative treatment options. Nine international clonal (IC) lineages have been identified in many countries worldwide, however, data still lacks from some parts of the world, particularly in Africa. We hereby present the molecular epidemiology of MDR A. baumannii from four hospitals in Khartoum, Sudan, collected from 2017 to 2018. Forty-two isolates were whole-genome sequenced, and subsequent molecular epidemiology was determined by core genome MLST (cgMLST), and their resistomes identified. All isolates had an array of diverse antibiotic resistance mechanisms conferring resistance to multiple classes of antibiotics. We found a predominance (88%) of IC2 (with the intrinsic OXA-66 and acquired OXA-23), and some with NDM-1. IC2 isolates were sub-divided into 4 STs separated by 5 to 431 allelic differences, and with evidence of seven transmission clusters. Isolates belonging to IC1, IC5, and IC9 were also identified. These data illustrate that MDR IC2 A. baumannii are widely distributed in Khartoum hospitals and are in possession of multiple antibiotic resistance determinants.
Introduction
Acinetobacter baumannii is an important nosocomial pathogen that causes a variety of infections including but not limited to ventilator-associated pneumonia and bloodstream infection (Peleg et al., 2008). Treatment options have been compromised by the emergence of multi-drug resistant (MDR) isolates. The prevalence of MDR A. baumannii in hospitals has put the organism on the “ESKAPE” pathogens list: an acronym developed by the Infectious Diseases Society of America for a group of MDR nosocomial pathogens with limited remaining treatment options (Boucher et al., 2009). A. baumannii is also considered as priority 1 (“critical”) on the World Health Organization list of priority organisms for research and development of new antibiotics (WHO, 2017). Of particular concern is carbapenem resistance, as carbapenems are considered the drugs of last resort in treating A. baumannii infections, and resistance is commonly attributed to acquired carbapenemases (Poirel and Nordmann, 2006).
For the investigation of local outbreaks, pulsed field gel electrophoresis (PFGE) has been the traditional typing method, but given the global distribution of A. baumannii, current typing methods must be reproducible and portable, both of which PFGE lacks. Multi-locus sequence typing (MLST) is a relatively easy method to perform but it lacks the resolution for outbreak investigation (Higgins et al., 2017). The advent of relatively cheap whole-genome sequencing (WGS) technology gives the opportunity to perform high resolution typing and the ability to compare whole genomes rather than only a few loci. WGS also allows for the identification of antimicrobial resistance determinants, both intrinsic and acquired, and can aid in our understanding of the circulating resistome.
Molecular epidemiological studies have assigned nine major International Clones (IC1-9) of A. baumannii, the most widespread of which is IC2 commonly harboring the acquired carbapenemase OXA-23 (Higgins et al., 2010a; Zarrilli et al., 2013; Hamidian and Nigro, 2019; Müller et al., 2019; Tomaschek et al., 2019). However, there is a significant lack of epidemiological and genomic data from low- and middle-income countries (LMICs), mainly due to limited research infrastructure and resources. Given that antimicrobial resistance does not respect borders, there is therefore an urgent need to support local initiatives in LMICs to generate epidemiological and genomic data in order to assess the burden of infectious diseases, and track resistance globally (Okeke et al., 2005).
This study aimed to explore the molecular epidemiology and antimicrobial resistance mechanisms using WGS of carbapenem resistant A. baumannii (CRAb) isolated from patients in Khartoum State in Sudan.
Materials and Methods
Bacterial Strains and Antimicrobial Susceptibility
A total of 71 consecutive A. baumannii isolates were collected between 2017 and 2018 from hospitalized patients in four different hospitals in Khartoum, Sudan: Soba University Hospital (n = 37), Ibrahim Malik Hospital (n = 3), The Military Hospital (n = 16), and Al Baraha Hospital (n = 16). These were isolated from a variety of samples including blood, urine, sputum, wound, cerebrospinal fluid, and catheter tips (Table 1). Isolates were initially identified at the clinical microbiology laboratories of the hospitals using conventional methods. For the purpose of this study, all isolates were confirmed as A. baumannii by the gyrB multiplex PCR method and MALDI-TOF prior to whole genome sequencing (WGS) (Higgins et al., 2010b). Presence of OXA and metallo- carbapenemase-encoding genes was determined by PCR (OXA-23, -40,-51, -58, -143, -235, and NDM, IMP, VIM, GIM, KPC, and GES) (Woodford et al., 2006; Ellington et al., 2007; Higgins et al., 2013).
Antimicrobial susceptibility (AST) was initially performed by disk-diffusion at the clinical microbiology laboratories and interpreted according to CLSI guidelines. Upon species confirmation, the minimum inhibitory concentration (MIC) was determined by MICRONAUT-GN, an automated microtiter broth dilution susceptibility testing system (Merlin Diagnostika, Germany). Colonies from a pure overnight subculture were used to prepare a 0.5-McFarland-standard suspension in 0.9% saline as recommended by the manufacturer (Merlin Diagnostika, Germany). This system allows the determination of the MIC for a panel of antibiotics with two concentrations (mg/L) based on the EUCAST MIC breakpoints for sensitivity (S) or resistance (R) (EUCAST, 2020b): meropenem (MER), gentamicin (GEN), amikacin (AMK), trimethoprim-sulfamethoxazole (SXT), ciprofloxacin (CIP), and colistin (COL) in a single microtitre plate. After the addition of the bacterial suspension and rehydration of the antibacterial agents, the plates were incubated at 37°C for 18–24 h. Bacterial growth in the wells was monitored photometrically at 620 nm, and a density of >50% above the cutoff value for the growth control. The results were also observed visually for turbidity to confirm the results. Additionally, MIC for imipenem (IMP) was performed by broth microdilution (BMD) method according to the EUCAST guidelines V2, 2020 (EUCAST, 2020a). Quality control strains Escherichia coli ATCC 25922 and Pseudomonas aeruginosa ATCC 27853 were used in all AST experiments.
Whole Genome Sequencing and Molecular Typing
Total DNA was extracted from the bacterial isolates using the MagAttract HMW DNA Kit (Qiagen, Hilden, Germany) according to the manufacturer’s instruction. Sequencing libraries were prepared using the Nextera XT library prep kit (Illumina GmbH, Munich, Germany) for a 250-bp paired-end sequencing run on an Illumina MiSeq platform. Genomes were assembled de novo using Velvet v1.1.04. Molecular epidemiology of the isolates was investigated by core-genome MLST (cgMLST) based on a core genome of 2,390 alleles using Ridom < cps:sup > ® < /cps:sup > SeqSphere+ v. 7.2.3 (Higgins et al., 2017).
Multi-locus sequence typing was performed on the pubMLST database https://pubmlst.org/abaumannii/ to identify sequence types (STs) for both the Pasteur and Oxford schemes. Antimicrobial resistance determinants were identified using the Resfinder software v3.2 https://cge.cbs.dtu.dk/services/ResFinder/. Beta-lactamases were identified using the online beta-lactamase database1 (Naas et al., 2017).
All assembled genomes were submitted to GenBank under BioProject number PRJNA628907. All accession numbers are listed in Table 1.
Results
Of the 71 isolates initially collected, a total of 42 isolates were confirmed as A. baumannii by gyrB, presence of blaOXA–51–like, and MALDI-TOF, and subsequently included in this study. The remaining isolates were other Gram negative organisms misidentified as A. baumannii and excluded from the study.
Antimicrobial susceptibility results showed that all isolated were MDR (Table 1) and that 95% of the isolates (n = 40) were CRAb, with an MIC for imipenem ranging from 16 to 256 mg/L (Table 2). Resistome analysis revealed that blaOXA–23 was the most prevalent carbapenemase, present in 39 isolates (92%), with three isolates co-harboring blaOXA–58 (Table 2). Other β-lactamases detected included NDM-1 (n = 10), GES-11 (n = 2), CTX-M-15 (n = 1), OXA-1 (n = 1), TEM-1D (n = 30) and TEM-199 (n = 1). Multiple aminoglycoside modifying enzymes were identified: armA, aadA1, aad-B-like, aac(3)-Ia-like, aph(3′)-VIa-like, aph(3′)-Ia, aph(6)-Id, aph(3″)-Ib, aph(3′)-Ic, strA, strB, and aac(6′)-Ib-like. Genes contributing to macrolide resistance mphE and msrE were present in 33 isolates, some of which co-localized in a gene cassette with aminoglycoside resistance genes. Twenty-five isolates possessed tet(A), tet(B) or tet(39) contributing to tetracycline and erythromycin resistance, found on the same contigs as aminoglycoside modifying enzymes. All isolates had the chromosomally encoded ADC cephalosporinase.
The isolates belonged to seven distinct STs according to the Pasteur scheme: ST1, ST2, ST85, ST570, ST584, ST600, ST602, and ST632, which belonged to IC1, IC2, IC5, IC9, and a singleton. Table 1 lists the STs identified in both the Pasteur and Oxford schemes. OXA-66, OXA-69, OXA-91, OXA-94, and OXA-51 were the intrinsic OXA-51-like enzymes identified in the study (Table 2).
Molecular epidemiology by cgMLST confirmed that IC2 with the intrinsic OXA-66 and acquired OXA-23 was the major clonal lineage found across the four hospitals in Khartoum (n = 37, 88%). As seen in Figure 1, IC2 is sub-divided into 4 STs (ST-2, −570, −600, and −632) separated by 5–431 allelic differences. Within IC2, we found evidence of seven transmission clusters (TC) (defined at ≤10 allelic differences between isolates) one of which included two Pasteur STs (ST-632 and ST-2), including two inter-hospital transmissions (Figure 1). The largest transmission cluster (TC-1, n = 20) comprises two Pasteur STs: ST-2 and ST-632 (single-locus variants at the rpoB allele), and two Oxford STs: ST-195 and ST-208 (single locus variants at the gpi allele).
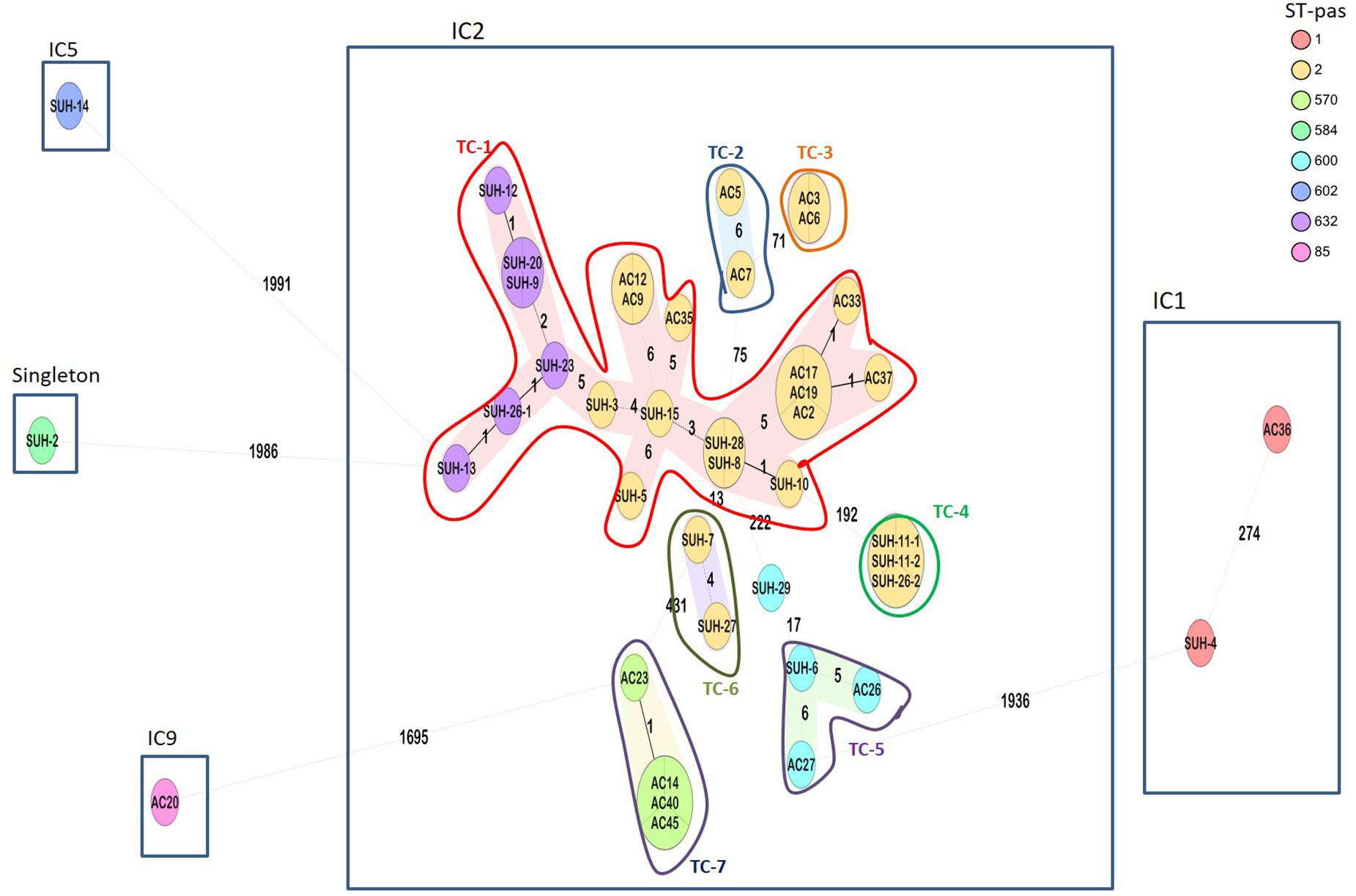
Figure 1. Ridom SeqSphere+ minimum spanning tree (MST) for 42 samples based on 2,390 alleles. Isolates grouped by color indicating the different STs (Pasteur scheme). Eight different STs were identified, belonging to IC1, IC2, IC5, IC9, and a singleton. Numbers between the nodes indicate the number of allelic differences. Shaded nodes represent transmission clusters (TC), and are numbered TC1-7.
Two isolates belonging to IC1 (ST-1) and harboring OXA-69 were from two different hospitals (Soba and the Military Hospital), separated by >200 allelic differences, and with distinct resistomes. The two isolates shared the presence of aminoglycoside modifying enzymes aadA1 and aac(3)-Ia, and sul1. Isolate SUH-4 was carbapenem resistant, and harbored OXA-23, NDM-1, and additional aminoglycoside modifying enzymes aadB-like, aph(3′)VIa, aph(3′)Ic, strA, strB, and dfrA1 contributing to trimethoprim resistance. Isolate AC-36, on the other hand, was carbapenem susceptible.
The remaining isolates SUH-14 (OXA-91, ST-602) and AC-20 (OXA-94, ST-85) belonged to IC5 and IC-9, respectively, while SUH-2 (OXA-98, ST-584) was a singleton not belonging to an IC.
These data also gave an indication of the local inter-hospital transmissions. Soba University Hospital had a large diversity of isolates belonging to IC1, IC2, IC5 and the singleton ST-584. As seen in Figure 1, the majority of these isolates were part of a large transmission cluster (TC-1) that included an isolate from the Ibrahim Malik hospital, as well as having three other smaller transmission clusters (TC-4, TC-5, and TC-6), of which TC-4 comprised three identical isolates, two of which were from the Ibrahim Malik hospital. Isolates from the other two hospitals, Al-Baraha and the Military Hospital, in this study were part of transmission clusters TC-2, TC-3, and TC-7, but with no inter-hospital spread.
With regards to carbapenem-resistance, all but three isolates possessed blaOXA–23 which was the sole carbapenemase in 26 isolates. In addition to blaOXA–23, two isolates had blaGES–11, eight blaNDM–1, two blaOXA–58, and one had both blaNDM–1 and blaOXA–58 (Table 2 and Supplementary Figure 2). One isolate had only NDM-1. There were two carbapenem susceptible isolates, one of which was positive for the ESBLs CTX-M-15 and blaOXA–1. The plasticity of the isolates is demonstrated by them being identical by cgMLST, but differing in their resistomes as for of isolate SUH-8 differing from SUH-28 by having an additional blaNDM–1.
Three isolates from Ibrahim Malik Hospital all fall within IC2 (ST-2) but one of which was separated from the other two by >192 allelic differences. The Military Hospital, on the other hand, had isolates belonging to IC1, IC2, and IC9. A single cluster represented isolates from Al-Baraha Hospital (ST-570) belonging to IC2 and all co-harbored OXA-23 and NDM-1.
Discussion
We hereby present the molecular epidemiology of carbapenem-resistant A. baumannii collected from patients from Khartoum, Sudan. A total of 71 isolates from four different hospitals were collected in 2017–2018, out of which 42 were confirmed as A. baumannii. Routine identification in clinical microbiology laboratories in Sudan relies primarily on phenotypic methods in combination with simple biochemical methods, which are not always reliable for accurate bacterial species identification, and 29 isolates (40%) were excluded in the study due to misidentification. Similar findings were reported in a study in Sudan on Klebsiella pneumoniae where 40% of isolates were misidentified (Osman et al., 2020). These data highlight an urgent need to improve diagnostic facilities across LMICs such as Sudan, in order to obtain accurate information of the local burden of infections and resistance, and to select optimal treatment options. However, restrictions in funding and capacity pose great limitations in implementing molecular diagnosis in clinical microbiology laboratories. Genomic data from Sudan is scarce, despite the indicative high burden of MDR in hospitals (Hamdan et al., 2015). One study that has performed WGS on an A. baumannii isolate from Khartoum, identified it as STOXF164, with OXA-91 as the intrinsic OXA-51-like enzyme (Mohamed et al., 2019), however, the genome of this isolate had over 2,000 allelic differences when compared to our isolates (data not shown).
Core-genome MLST analysis revealed seven transmission clusters, with a predominance of IC2-OXA-23 accounting for 88% of the isolates across Khartoum Hospitals. This is in accordance with previously published data showing that IC2-OXA-23 is the most successfully disseminating clone globally (Hamidian and Nigro, 2019), however, there are regional variation such as IC5 and IC7 being more predominant in South and Central America (Higgins et al., 2010a). IC2 in Sudan is composed of four sequence types (STPAS2, STPAS570, STPAS600, and STPAS632). The IC2 isolates were further delineated by cgMLST showing that the ST2 isolates were in some cases more distant than to other STs. For example >190 allelic differences are found between isolates within ST2 (SUH-10 and SUH-11), whereas SUH-3 and SUH-23 which belong to ST2 and ST632, respectively, only have 5 allelic differences. A recent comparison of the two MLST schemes suggested that the Pasteur scheme was more stable than the Oxford scheme (Gaiarsa et al., 2019); while this might be true in most cases, these data from Sudan highlight that a reliance on only 7-loci can lead to a false sense of strain divergence, when in fact, isolates differ in five alleles out of a total 2,390, one of which is the rpoB MLST allele. Furthermore, the seven transmission clusters, included inter-hospital spread of two clones (TC-1 and TC-4), which was not apparent from their STs. Similarly, isolates belonging to ST1 have >200 allelic differences between them. These findings illustrate the low resolution of traditional 7-loci typing, particularly for local outbreak and transmission investigations.
Although transmission clusters are clearly evident (Figure 1), and despite the relatively conserved dissemination of carbapenemases in the current study, there are some differences, particularly in acquired genes such as NDM-1. For example, SUH-28 and SUH-8 were identical by cgMLST and both had OXA-23, but SUH-28 harbored additional NDM-1 and OXA-58. Furthermore, NDM-1 was present in only three of the four ST-600 isolates. Relying on characterisation of the resistome for determining clonality can be misleading and illustrate the genome plasticity of MDR A. baumannii. It is therefore important that discriminatory genomic typing methods are applied in combination with the resistome, clinical and epidemiological data to obtain an accurate picture of outbreaks and transmission links. As A. baumannii is an emerging nosocomial pathogen with extended antibiotic resistance, it’s important to note the availability of online resources and databases offering rapid typing and phylogenetic relatedness to use when investigating local and global outbreaks, which is increasingly important in a globalized community.
Three isolates (SUH-2, AC20 and AC36) did not harbor OXA-23, but on the other hand harbored other β-lactamases: CTX-M-15, OXA-1, and/or NDM-1. To our knowledge, this is the first report of OXA-1 in A. baumannii. OXA-1 is commonly found in Enterobacteriaceae, and frequently co-carried with CTX-M-15, as also present in our data (Sugumar et al., 2014).
Our study complements previous studies on the successful dissemination and possible endemicity of specific STs and resistance determinants across North Africa and the Middle East. ST-85 (IC9) is a common clone in Africa and the Middle East as it has been reported as a major clone spreading the NDM-1 gene in Tunisia, among Syrian refugees in Lebanon and Turkey, in France from patients with a history of hospitalization in Algeria, Tunisia and Egypt (Bonnin et al., 2013; Jaidane et al., 2018; Salloum et al., 2018), and recently Spain in a strain harboring NDM-6 (Xanthopoulou et al., 2020). ST6, a single locus variant of ST85, has also been identified among Lebanese patients (Rafei et al., 2014). A single isolate from the Military Hospital was identified as ST-85 harboring OXA-94 and NDM-1 similar to the isolates from Tunisia and Lebanon. With Oxford MLST scheme, this isolate (AC20) was assigned to ST-1089OXF, which has also been reported in Egypt (Al-Hassan et al., 2019; Al-Hassan and Al-Madboly, 2020). Two isolates, SUH-14 and SUH-7, assigned to ST602 (IC5) and ST-2 (IC2), respectively, were positive for GES-11 ESBL. GES-11 has been identified in a Belgian outbreak and was found associated with travel to Egypt, Turkey, and Gaza, thereby indicating a possible geographic dissemination (Moubareck et al., 2009; Bogaerts et al., 2010). Travel and medical tourism are among the major contributors to the acceleration of spread of resistance globally (Ostholm-Balkhed et al., 2013; Tatem, 2014).
Conclusion
Our study illustrates that CRAb IC2 A. baumannii are widely distributed in Khartoum hospitals and are in possession of multiple antibiotic resistance determinants. We are also able to confirm the spread of specific clones, such as IC9 (ST-85) globally, and across the Middle East and North Africa region specifically. Despite advances in genomic technology, and the relative cheap price of conducting WGS on bacterial genomes, it remains a challenge to implement routine molecular typing methods in Sudan, particularly due to the need of advanced technology and expertise. It is, however, essential to support local efforts to obtain epidemiological data on the burden of resistance in major hospital-acquired infections such as those caused by A. baumannii. For local outbreak investigations, molecular epidemiology must be combined with patient clinical and demographic data in order to track transmission and resistance.
Data Availability Statement
The data presented in the study are deposited in GenBank, under BioProject PRJNA628907. All accession numbers are listed in Table 1.
Author Contributions
LA-H, HE, EO, DC, and PH contributed to the design of the experiments. HE, EO, SA, and KE were responsible for the collection of isolates from the hospitals. LA-H, HE, EO, DC, and JW performed the experiments. LA-H, HE, EO, JW, HS, and PH analyzed and interpreted the data. LA-H, HE, and PH wrote the manuscript. All authors contributed to the article and approved the submitted version.
Conflict of Interest
The authors declare that the research was conducted in the absence of any commercial or financial relationships that could be construed as a potential conflict of interest.
Funding
HE was funded by an ESCMID observership ID 1507 and the International Research Partnerships and Networks Fund from the University of Sussex (IRPNF3-003). PH was supported by the German Research Council (DFG) – FOR2251 (www.acinetobacter.de). LA-H and EO were supported by the International Development Challenges Fund (IDCF1-007) from the University of Sussex.
Acknowledgments
Many thanks to the medical and technical staff of Medical Microbiology Department in Soba University Hospital, Military Hospital, Al-Baraha Hospital, and undergraduate students Awab Hassan, Amna Mohammed, Zeinab Mustafa at Africa International University for their help in strain and data collection.
Supplementary Material
The Supplementary Material for this article can be found online at: https://www.frontiersin.org/articles/10.3389/fmicb.2021.628736/full#supplementary-material
Supplementary Figure 1 | Ridom SeqSphere+ minimum spanning tree (MST) for 42 samples based on 2,390 alleles, Numbers between the nodes indicate the number of allelic differences. Isolates grouped based on the hospital they were collected from.
Supplementary Figure 2 | Ridom SeqSphere+ minimum spanning tree (MST) for 42 samples based on 2,390 alleles. Color of nodes indicate the different β-lactamases they harbor. Numbers between the nodes indicate the number of allelic differences. OXA-23 is the main carbapenemase. NDM-1 is present in 10 isolates representing IC1, IC2, and IC9. Several isolates harbor multiple β-lactamases.
Footnotes
References
Al-Hassan, L., Zafer, M. M., and El-Mahallawy, H. (2019). Multiple sequence types responsible for healthcare-associated Acinetobacter baumannii dissemination in a single centre in Egypt. BMC Infect. Dis. 19:829. doi: 10.1186/s12879-019-4433-1
Al-Hassan, L. L., and Al-Madboly, L. A. (2020). Molecular characterisation of an Acinetobacter baumannii outbreak. Infect. Prev. Pract. 2:100040. doi: 10.1016/j.infpip.2020.100040
Bogaerts, P., Naas, T., El Garch, F., Cuzon, G., Deplano, A., Delaire, T., et al. (2010). GES extended-spectrum β-lactamases in Acinetobacter baumannii isolates in Belgium. Antimicrob. Agents Chemother. 54, 4872–4878. doi: 10.1128/AAC.00871-10
Bonnin, R. A., Cuzon, G., Poirel, L., and Nordmann, P. (2013). Multidrug-resistant Acinetobacter baumannii clone. France. Emerg. Infect. Dis. 19, 822–823. doi: 10.3201/eid1905.121618
Boucher, H. W., Talbot, G. H., Bradley, J. S., Edwards, J. E., Gilbert, D., Rice, L. B., et al. (2009). Bad bugs, no drugs: no ESKAPE! an update from the infectious diseases Society of America. Clin. Infect. Dis. 48, 1–12. doi: 10.1086/595011
Ellington, M. J., Kistler, J., Livermore, D. M., and Woodford, N. (2007). Multiplex PCR for rapid detection of genes encoding acquired metallo-β-lactamases. J. Antimicrob. Chemother. 59, 321–322. doi: 10.1093/jac/dkl481
EUCAST (2020a). EUCAST Reading Guide for Broth Microdilution (v2) 2 March 2020. Available online at: https://www.eucast.org/ast_of_bacteria/mic_determination/ (accessed September 1, 2020)
EUCAST (2020b). Testing Breakpoint Tables for Interpretation of MICs and Zone Diameters (v10.0). Available online at: https://eucast.org/clinical_breakpoints/ (accessed September 1, 2020).
Gaiarsa, S., Biffignandi, G. B., Esposito, E. P., Castelli, M., Jolley, K. A., Brisse, S., et al. (2019). Comparative analysis of the two Acinetobacter baumannii multilocus sequence typing (MLST) schemes. Front. Microbiol. 10:930. doi: 10.3389/fmicb.2019.00930
Hamdan, H. Z., Kubbara, E., Adam, A. M., Hassan, O. S., Suliman, S. O., and Adam, I. (2015). Urinary tract infections and antimicrobial sensitivity among diabetic patients at Khartoum, Sudan. Ann. Clin. Microbiol. Antimicrob. 14:26. doi: 10.1186/s12941-015-0082-4
Hamidian, M., and Nigro, S. J. (2019). Emergence, molecular mechanisms and global spread of carbapenem-resistant Acinetobacter baumannii. Microb. Genomics 5:e000306. doi: 10.1099/mgen.0.000306
Higgins, P. G., Dammhayn, C., Hackel, M., and Seifert, H. (2010a). Global spread of carbapenem-resistant Acinetobacter baumannii. J. Antimicrob. Chemother. 65, 233–238. doi: 10.1093/jac/dkp428
Higgins, P. G., Lehmann, M., Wisplinghoff, H., and Seifert, H. (2010b). gyrB multiplex PCR to differentiate between acinetobacter calcoaceticus and Acinetobacter genomic species 3. J. Clin. Microbiol. 48, 4592–4594. doi: 10.1128/JCM.01765-10
Higgins, P. G., Pérez-Llarena, F. J., Zander, E., Fernández, A., Bou, G., and Seifert, H. (2013). OXA-235, a novel class D β-lactamase involved in resistance to carbapenems in Acinetobacter baumannii. Antimicrob. Agents Chemother. 57, 2121–2126. doi: 10.1128/AAC.02413-12
Higgins, P. G., Prior, K., Harmsen, D., and Seifert, H. (2017). Development and evaluation of a core genome multilocus typing scheme for whole-genome sequence-based typing of Acinetobacter baumannii. PLoS One 12:e0179228. doi: 10.1371/journal.pone.0179228
Jaidane, N., Naas, T., Oueslati, S., Bernabeu, S., Boujaafar, N., Bouallegue, O., et al. (2018). Whole-genome sequencing of NDM-1-producing ST85 Acinetobacter baumannii isolates from Tunisia. Int. J. Antimicrob. Agents 52, 916–921. doi: 10.1016/j.ijantimicag.2018.05.017
Mohamed, S. B., Hassan, M., Munir, A., Kambal, S., Abdalla, N. I., Hamad, A., et al. (2019). Whole-genome sequence of Acinetobacter baumannii strain NUBRI-A, isolated from a hospitalized patient in Khartoum, Sudan. Microbiol. Resour. Announc. 8, 9–10. doi: 10.1128/MRA.00542-19
Moubareck, C., Brémont, S., Conroy, M.-C., Courvalin, P., and Lambert, T. (2009). GES-11, a novel integron-associated GES variant in Acinetobacter baumannii. Antimicrob. Agents Chemother. 53, 3579–3581. doi: 10.1128/AAC.00072-09
Müller, C., Stefanik, D., Wille, J., Hackel, M., Higgins, P. G., and Seifert, H. (2019). “Molecular epidemiology of carbapenem-resistant Acinetobacter baumannii clinical isolates and identification of the novel international clone IC9: results from a worldwide surveillance study (2012 – 2016),” in Proceedings of the Abstract Book of the 29th European Congress of Clinical Microbiology and Infectious Diseases, P0947, Amsterdam.
Naas, T., Oueslati, S., Bonnin, R. A., Dabos, M. L., Zavala, A., Dortet, L., et al. (2017). Beta-lactamase database (BLDB)–structure and function. J. Enzyme Inhib. Med. Chem. 32, 917–919. doi: 10.1080/14756366.2017.1344235
Okeke, I. N., Laxminarayan, R., Bhutta, Z. A., Duse, A. G., Jenkins, P., O’Brien, T. F., et al. (2005). Antimicrobial resistance in developing countries. Part I: recent trends and current status. Lancet Infect. Dis. 5, 481–493. doi: 10.1016/S1473-3099(05)70189-4
Osman, E. A., El-Amin, N., Adrees, E. A. E., Al-Hassan, L., and Mukhtar, M. (2020). Comparing conventional, biochemical and genotypic methods for accurate identification of Klebsiella pneumoniae in Sudan. Access Microbiol. 2:acmi000096. doi: 10.1099/acmi.0.000096
Ostholm-Balkhed, A., Tärnberg, M., Nilsson, M., Nilsson, L. E., Hanberger, H., and Hällgren, A. (2013). Travel-associated faecal colonization with ESBL-producing Enterobacteriaceae: incidence and risk factors. J. Antimicrob. Chemother. 68, 2144–2153. doi: 10.1093/jac/dkt167
Peleg, A. Y., Seifert, H., and Paterson, D. L. (2008). Acinetobacter baumannii: emergence of a successful pathogen. Clin. Microbiol. Rev. 21, 538–582. doi: 10.1128/CMR.00058-07
Poirel, L., and Nordmann, P. (2006). Carbapenem resistance in Acinetobacter baumannii: mechanisms and epidemiology. Clin. Microbiol. Infect. 12, 826–836. doi: 10.1111/j.1469-0691.2006.01456.x
Rafei, R., Dabboussi, F., Hamze, M., Eveillard, M., Lemarié, C., Gaultier, M. P., et al. (2014). Molecular analysis of Acinetobacter baumannii strains isolated in lebanon using four different typing methods. PLoS One 9:e115969. doi: 10.1371/journal.pone.0115969
Salloum, T., Tannous, E., Alousi, S., Arabaghian, H., Rafei, R., Hamze, M., et al. (2018). Genomic mapping of ST85 blaNDM-1 and blaOXA-94 producing Acinetobacter baumannii isolates from syrian civil war victims. Int. J. Infect. Dis. 74, 100–108. doi: 10.1016/j.ijid.2018.07.017
Sugumar, M., Kumar, K. M., Manoharan, A., Anbarasu, A., and Ramaiah, S. (2014). Detection of OXA-1 β-lactamase gene of Klebsiella pneumoniae from blood stream infections (BSI) by conventional PCR and in-silico analysis to understand the mechanism of OXA mediated resistance. PLoS One 9:e91800. doi: 10.1371/journal.pone.0091800
Tatem, A. J. (2014). Mapping population and pathogen movements. Int. Health 6, 5–11. doi: 10.1093/inthealth/ihu006
Tomaschek, F., Higgins, P. G., Stefanik, D., Wisplinghoff, H., Seifert, H., Hatcher, J., et al. (2019). Head-to-Head comparison of two multi-locus sequence typing (MLST) schemes for characterization of Acinetobacter baumannii outbreak and sporadic isolates. PLoS One 11:e0153014. doi: 10.1371/journal.pone.0153014
WHO (2017). Global Priority List of Antibiotic-Resistant Batceria to Guide Research, Discovery, and Development of New Antibiotics. Geneva: WHO, 7.
Woodford, N., Ellington, M. J., Coelho, J. M., Turton, J. F., Ward, M. E., Brown, S., et al. (2006). Multiplex PCR for genes encoding prevalent OXA carbapenemases in Acinetobacter spp. Int. J. Antimicrob. Agents 27, 351–353. doi: 10.1016/j.ijantimicag.2006.01.004
Xanthopoulou, K., Urrutikoetxea-Gutiérrez, M., Vidal-Garcia, M., Diaz de Tuesta del Arco, J. L., Sánchez-Urtaza, S., Wille, J., et al. (2020). First report of New Delhi Metallo-β-Lactamase-6 (NDM-6) in a clinical Acinetobacter baumannii isolate from Northern Spain. Front. Microbiol. 11:589253. doi: 10.3389/fmicb.2020.589253
Keywords: antibiotic resistance, cgMLST, OXA, NDM-1, Sudan, international clones
Citation: Al-Hassan L, Elbadawi H, Osman E, Ali S, Elhag K, Cantillon D, Wille J, Seifert H and Higgins PG (2021) Molecular Epidemiology of Carbapenem-Resistant Acinetobacter baumannii From Khartoum State, Sudan. Front. Microbiol. 12:628736. doi: 10.3389/fmicb.2021.628736
Received: 12 November 2020; Accepted: 10 February 2021;
Published: 26 February 2021.
Edited by:
Paolo Visca, Roma Tre University, ItalyCopyright © 2021 Al-Hassan, Elbadawi, Osman, Ali, Elhag, Cantillon, Wille, Seifert and Higgins. This is an open-access article distributed under the terms of the Creative Commons Attribution License (CC BY). The use, distribution or reproduction in other forums is permitted, provided the original author(s) and the copyright owner(s) are credited and that the original publication in this journal is cited, in accordance with accepted academic practice. No use, distribution or reproduction is permitted which does not comply with these terms.
*Correspondence: Leena Al-Hassan, bC5hbC1oYXNzYW5AYnNtcy5hYy51aw==
†These authors have contributed equally to this work