- 1Department of Horticulture, College of Agriculture, The Key Laboratory of Characteristics of Fruit and Vegetable Cultivation and Utilization of Germoplasm Resources of the Xinjiang Production and Construction Crops, Shihezi University, Shihezi, China
- 2Shihezi Academy of Agricultural Sciences, Shihezi, China
Fertilizer practices can significantly impact the fruit quality and microbial diversity of the orchards. The fungi on the surface of fruits are essential for fruit storability and safety. However, it is not clear whether fertilization affects the fungal diversity and community structure on the surface of grape berries. Here, grape quality and the fungal diversity on the surface of grapes harvested from three fertilizer treatments were analyzed shortly after grape picking (T0) and following 8 days of storage (T1). The study involved three treatments: (1) common chemical fertilizer for 2 years (CH); (2) increased organic fertilizer and reduced chemical fertilizer for 1 year (A.O); and (3) increased organic fertilizer and reduced chemical fertilizer for 2 years (B.O). The application of increased organic fertilizer and reduced chemical fertilizer increased the soluble solids content (SSC) of the grape berries and decreased the pH of the grape juice. A total of 827,947 high-quality fungal sequences were recovered and assigned to 527 operational taxonomic units. Members of the Ascomycota phylum were dominant in all samples and accounted for 94.41% of the total number of detected sequences, followed by the Basidiomycota (5.05%), and unidentified fungi (0.54%). Alpha and beta diversity analyses revealed significantly different fungal populations in the three fertilizer treatments over the two time periods. The fungal diversity and richness on the grape berry surface in the B.O and A.O treatments were higher than those in the CH treatment. Among the detected fungi, the B.O treatments were mainly Pichia, Aureobasidium, and Candida genera, while the CH treatments were Botrytis, Aspergillus, and Penicillium. Moreover, significant differences were revealed between the two assessment times (T0 and T1). The samples from the T0 timepoint had higher fungal richness and diversity than the samples from T1 timepoint. Increasing organic fertilizer usage in grape management could improve grape quality and went on to increase the fungal diversity, as well as the relative abundance (RA) of beneficial fungi on grape berry surfaces. The correlation analysis suggested that the pH of the grape juice was significantly negatively correlated with fungal diversity parameters.
Introduction
Increases in global grain and fruit yields in recent decades have been largely dependent on heavy investments in fertilizer (Geng et al., 2019). However, fertilizer can harm marine, freshwater, and terrestrial ecosystems (Tilman et al., 2011). Soil conditions with excess nutrients may result in potential damages to the environment, causing widespread environmental problems (García-Díaz et al., 2017). Excessive fertilizer application aggravated the decline in soil organic matter (SOM) and fertility (Lv et al., 2020; Wu et al., 2020). Therefore, in addition to yields, agricultural production needs to take environmental sustainability into account. Organic fertilizers are often used as basal fertilizer to ensure that SOM and micronutrients play unique roles during grapevine growth and development (Zhao et al., 2019). Organic fertilizer can affect soil moisture-holding capacity by increasing soil infiltration and minimizing soil evaporation (Moreno-Jiménez et al., 2019). Although organic fertilizers are more eco-friendly, they have low nutrient concentrations and nutrient release is too slow to support grape production in a short time (Xiao et al., 2017). The nutrient contents of chemical fertilizers are higher than that of organic ones, but they easily to cause environmental pollution and soil degradation (Song et al., 2020). The partial replacement of chemical fertilizers with organic fertilizers has proven to be a beneficial approach to sustain soil fertility compared to applying chemical or organic fertilizers alone (Song et al., 2020). Studies have shown that the use of inorganic-organic compound fertilizers can not only reduce the use of chemical fertilizer but also improve the efficiency and sustainability of agricultural ecosystems in the long term (Geng et al., 2019).
Numerous studies have found that the combination of chemical fertilizer and organic fertilizer can improve the quality of peaches (Sharma et al., 2017), pears (Wei et al., 2012), grapes (Zhao et al., 2020), and others. Organic fertilizer application increased the soluble sugar content of bananas (Zhang et al., 2020), decreased the titratable acid content of pears (Wei et al., 2012), and significantly affected the pH of the grape juice (Zhao et al., 2020). Through the modification of soil physicochemical properties, fertilization has been found to influence the soil microbial biomass and community composition (Liu et al., 2010). Similar studies also found that the use of organic fertilizer not only affected soil physical properties but also enhanced soil microbial diversity (Hao et al., 2008). Many studies have shown that organic fertilization had positive effects on soil microbial richness and diversity (Zhang et al., 2012; Geng et al., 2019). By contrast, chemical fertilization was bad for microbial diversity (Luan et al., 2020). Plant surfaces host diverse and abundant microbial communities, which are related to specific functions that may affect plant productivity and health (Angeli et al., 2019). Microbial communities associated with grape leaves, flowers, and berries shared a greater proportion of taxa with the soil communities, suggesting that soil may serve as a microbial reservoir (Zarraonaindia et al., 2015). Ascomycota, Basidiomycota, Chytridiomycota, Blastocladiomycota, and Glomeromycota were main fungal phylum in the soil; Ascomycota and Basidiomycota were main fungal phylum on the grape berry surface. There were 24.63% of the fungal genera which were common to soil and on the grapes surface (Morrison-Whittle et al., 2017). Martins et al. (2013) also found about 50% of the bacteria genera that were common to soil and grapes. Microbial community structure on the grape surface is mainly controlled by the cultivation environments (Abdelfattah et al., 2016a; Kecskemeti et al., 2016). The microbiota on the surface of grapes are important for the development of plant diseases and might also impact fruit maturity (Buchholz et al., 2018). Barata et al. (2012) identified over 50 bacterial species on grape berries, mainly belonging to two groups, Proteobacteria and Firmicutes. The bacterial community on the surface of grape berry changed with the ripening of the grapes (Renouf et al., 2005). The majority of research has been focused on the bacterial communities, but fungi are also important (Escribano-Viana et al., 2018). Fungi can profoundly influence plant function, health, and development, through a wide range of interactions acting as biocontrol agents or as pathogens (Oliveira et al., 2018). Some fungi are vital for plant health as well as fruit quality and yield (Ding et al., 2019). Previous studies have mostly focused on the pathogenic fungi causing grape diseases, including Botrytis cinerea (Chen et al., 2019), Penicillium expansum (Jurick 2nd et al., 2020; Luciano-Rosario et al., 2020), and Aspergillus japonicus (Yan and Liu, 2019), the causal agents of grapevine powdery mildew, blue mold, and bitter rot, respectively (Lorenzini et al., 2018). Moreover, researchers have also identified saprophytic molds, such as Cladosporium sp., Aspergillus sp., and Penicillium sp., that could produce mycotoxins, which were indirectly responsible for food spoilage and directly responsible for grape rot (Martins et al., 2014). Several Botryosphaeriaceae species were known to occur worldwide, causing dieback, canker, and fruit rot on various hosts (Akila et al., 2020). Grape black rot is caused by Guignardia bidwellii and was the grapevine disease attributed to a botryosphaeriaceous fungus (Onesti et al., 2017). Macrophoma rot is caused by Botryosphaeria dothidea (Úrbez-Torres, 2011; Xu et al., 2015). Both Botryosphaeria kuwatsukai and B. dothidea are the main causal agents for apple ring rot in China and Japan (Xu et al., 2015). Plant-associated fungi, such as Aureobasidium pullulans (Lorenzini and Zapparoli, 2019) and Candida xylopsoci (Gao et al., 2019), have also been suggested to have a positive interaction with their host plants. In the production of organic apples and pears, A. pullulans is used as a biocontrol agent for fire blight protection (Temple et al., 2020). Utilization of A. pullulans was shown to be successful in controlling against both P. expansum and Penicillium digitatum (Agirman and Erten, 2020). A better understanding of these interactions may provide novel opportunities to develop creative biocontrol methods against plant pathogens (Sebastien et al., 2015). Contemporary studies dealing with the microbiome in grapes and the winemaking process have focused on the effects of vineyard locations and grape varieties (Shen et al., 2020). Subsequently, varieties, management methods, and eco-geographic factors were taken into account to explain their possible impacts on the microbiome associated with grapes (Bokulich et al., 2014). Fungi associated with the winemaking process and grape musts have been extensively documented (Escribano-Viana et al., 2018; Oliveira et al., 2018), however, few studies have concentrated on the fungal community composition on the table grape berry surface under different fertilizer practices.
Xinjiang is an area with a long history of viticulture, with a planting area of 142,900 ha (Huang, 2019). Located in arid regions in Xinjiang, the orchard environment is fragile, and the long-term unscientific use of chemical fertilizer results in an increased frequency of fungal diseases such as powdery mildew (Erysiphe necator) and downy mildew (Plasmopara viticola) in vineyards. Previous studies mainly focused on the effects of fertilization on the growth of grapes (Liu et al., 2016) and the soil environment (Zhang et al., 2018; Cheng et al., 2020). The fungi on the surface of the grape berries are essential for the quality and storage of grape berries, which are also very important for people’s food safety. However, there is no report on the effects of different fertilization modes on grape berry surface fungal communities. The paper aimed to investigate the grape quality and the fungal diversity on the grape berry surface under three fertilizer treatments at two postharvest timepoints to provide a theoretical basis for improving the ecological environment and promoting the sustainable production of grapes in arid areas.
Materials and Methods
Experimental Site and Experimental Design
The experimental site was located in the grape standard experimental orchard (lat. 45°19'N, long. 86°03'E) of Shihezi Agricultural Science Research Institute in Xinjiang. The experimental zone is classified as temperate and arid, with a continental climate. The site’s annual precipitation, annual evaporation, and mean annual temperature are 0.23 m, 1.34 m, and 7.8°C, respectively (Tao et al., 2018). The meteorological data (daily temperature and daily rainfall) recorded during the experiment are shown in Supplementary Figure S1. The soil was sandy loam soil. The basic soil properties before the beginning of the experiment were as follow: pH, 8.04; SOM, 32.23 g kg−1; total nitrogen (TN), 1.26 g kg−1; alkali-hydrolysed nitrogen (AN), 50.13 mg kg−1; total phosphorus (TP), 0.89 g kg−1; available phosphorus (AP), 42.95 mg kg−1; available potassium (AK), 121.05 mg kg−1; and conductivity, 2.10 ms m−1. The soil pH, SOM, TN, TP, TK, AN, AK, and AP was measured as described (Bao, 2000).
These experiments used table grapes (Vitis vinifera L.) from one cultivar (Summer Black) that is widely planted in Xinjiang. Each treatment was repeated three times and randomly assigned to the experimental units. The grape trellis was a V-shaped scaffold (Figure 1) placed between the vines. In the spring, organic fertilizer was applied as the base fertilizer, 0.25 m deep (unilateral fertilization, and on one side of the grape line to avoid mutual influence between the grape plants) and 0.30 m away from the central trunk of the grape vine. Chemical fertilizers (drip application) were applied during the growing season. A total of 11 irrigations were carried out during the whole growth period, and the irrigation interval was 7–10 days. The three fertilization treatments had the same irrigation dates and irrigation times. The three treatments were: (1) common chemical fertilizer for 2 years (CH); (2) increased organic fertilizer and reduced chemical fertilizer for 1 year (A.O); and (3) increased organic fertilizer and reduce chemical fertilizer for 2 years (B.O). The study used a randomized complete block designed with three replicates of the three treatments. Rotten cattle manure was used as organic fertilizer containing 2.48% nitrogen, 1.79% phosphorus, and 1.67% potassium. See Table 1 for the application amount. The amount of chemical fertilizer applied in the CH treatment was determined according to the amount of fertilizer used by local fruit farmers on the vineyard.
Sample Preparation
Grape bunches were chosen to represent the same aspect (orientation to prevailing wind and sun) and position (proximity to the middle of the row), and samples were randomly collected from each vine at 11 a.m. on August 20, 2018. Then, samples were transported to the laboratory, and healthy bunches were selected based on color uniformity and absence of blemishes or disease. The first nine samples (three replicates × three treatments) were analyzed for grape quality and fungal communities on the grape berry surface shortly after grape picking. The other samples of the three fertilizer treatments (five clusters per fertilizer treatment) were packed into three cardboard boxes and stored at room temperature (~20°C) for 8 days. Table grapes can be stored for 7–12 days at room temperature and some table grapes are sold during this period (Zhu, 2020). Eighteen samples (three replicates × three treatments × two timepoints) of microbial DNA were collected from the grape surfaces. Each treatment consisted of three replications with 50 fresh grape berries per replicate. The microbial samples were gathered by wiping or swabbing each grape with a pre-moistened cotton swab. Swab samples were collected and stored at −80°C before microbial DNA extraction. The wiped grape berries were then used to assess the quality parameters of the grape berries.
Determination of Quality Parameters of Grape Berries
The pH was determined using a Mettler Toledo FE20 Desktop pH Meter (Mettler Toledo Instruments Co. Ltd., Shanghai, China). The SSC was measured with a Pocket Brix-acidity Meter (Atago, PALBX/ACID 5, Tokyo, Japan). Titratable acid content was determined using potentiometric titration with 0.1 N NaOH up to pH = 8.2 and expressed as percentage of tartaric acid (Youssef et al., 2015). The total anthocyanin content (TAC) in berries was determined by the pH differential method (Yang et al., 2020). The berry firmness was performed with a texture analyzer (TA). XT plus (Stable Micro Systems, Surrey, England), analyzing the equatorial position of 15 berries with pedicels per plot (Youssef et al., 2015).
Extraction of Genomeice DNA and PCR Amplification
The MoBio Power Water® DNA Isolation kit (MoBio Laboratories, Inc., Carlsbad, CA, United States) was used to extract microbial genomic DNA from the swabs. The NC 2000 spectrophotometer (Thermo Scientific Fisher, Waltham, MA, United States) and 1.0% agarose gel electrophoresis were used to determine the concentration and molecular size of the extracted DNA, respectively. Then, the DNA extractions were stored at −80°C until further use. Grape berries were analyzed for fungal communities shortly after grape picking, which was referred to as T0. Grape samples stored for 8 days, were referred to as T1. A total of 18 diluted DNA samples were submitted to Shanghai Personal Biotechnology Co., Ltd (Shanghai, China) for internal transcribed spacer (ITS) sequencing using the Illumina HiSeq sequencing platform. The specific pairs were ITS25F (5'-GGAAGTAAAAGTAACAAGG-3') and ITS1R (5'-GCTGCGTTCTTCATGC-3'). The PCR reaction contained 5 U of DNA polymerase (Pyrobest TaKaRa, Japan), 15 pmol of each primer, 2.5 mM of dNTP mixture, 10 μl of 10x Buffer II, and 40 ng of template DNA for a total volume of 25 μl. The PCR amplification series was performed using an ABI 9600 instrument under method of Hao et al. (2016). The obtained ITS1 amplicons were sequenced on the Illumina MiSeq platform at Shanghai Personal Biotechnology Co., Ltd (Shanghai, China).
PCR Product Purification and Library Preparation
According to the concentrations of the PCR products, PCR products were mixed at equal density ratios and then purified using a Qiagen Gel Extraction Kit (Qiagen Company in Germany; Zetsche et al., 2017). The TruSeq® DNA PCR-Free Sample Preparation Kit (Illumina, United States) was used to generate sequencing libraries, and then index codes were added (Noguerol-Pato et al., 2014). The library quality was evaluated using the Qubit@ 2.0 Fluorometer (Thermo Scientific) and an Agilent Bioanalyzer 2100 system (Kao et al., 2016). Finally, the library was sequenced on the Illumina HiSeq 2500 platform and 250 bp paired-end reads were generated.
Bioinformatic Analyses
After completing high-throughput sequencing, paired-end reads were obtained, which were assigned to samples based on their unique barcodes, and then truncated by cutting off the barcode and primer sequences. Using FLASH V1.2.7 spliced the paired-end reads (El-Ashram and Suo, 2017), filtered according to the QIIME V1.7.0 quality control process, and termed the high-quality clean tags. The tags were compared to the reference database using the UCHIME algorithm to detect chimeric sequences, and then removed the chimeric sequences (Li et al., 2018). Finally, we obtained the effective tags. Alpha diversity was evaluated using the QIIME suite of programmes, including the Simpson index, ACE, Shannon diversity, Chao1 richness, and observed species (Marsh et al., 2013). Principal coordinate analysis (PCoA) based on weighted calculations was used to analyze the differences in the community structure of the different treatments and timepoints (Horn et al., 2017). We used flower charts and Venn diagrams to explore the specific and common operational taxonomic units (OTUs) among the different treatments or timepoints. The microbial communities on the grape berry surfaces from the different treatments/timepoints were further compared using analysis of similarities (Anosim) and analysis of molecular variance (Amova). Amova was used to assess the significance of the fungal community structures among the different treatments. After basic analysis, Origin 2019 and R Studio (version 2.15.3) were used to draw figures (Hayden and Beman, 2016).
Statistical Analysis
SPSS 20.0 software was used to test the significance of the differences in the quality and fungal diversity of grapes, and the data were expressed as the means ± SD of triplicates.
Result
The Quality Parameters of Grape Berries
The SSC, pH of the grape juice, and TAC significantly increased after 8 days of storage in grapes from all three fertilizer treatments. At both timepoints, the SSC was significantly higher with the organic fertilizer treatments (A.O and B.O) relative to the chemical fertilizer treatment (CH); the value of the B.O sample was 14.21% higher than that of the CH sample, and the value of the A.O sample increased by 11.76% compared with the CH samples from T1 timepoint (Figure 2A). Of the three treatments, the titratable acid content was the highest in grapes from the B.O treatment at both the T0 and T1 timepoints (Figure 2B). Figure 2C shows the effect of the fertilizer treatments on the pH of the grape juice. At both the T0 and T1 timepoints, the pH of the grape juice in the A.O samples was significantly higher than that in the B.O samples. As seen in Figure 2D, at both timepoints, the TAC of the B.O and A.O samples was higher than that of the CH sample. The TAC in the T1.B.O and T1.A.O samples increased by 72.48 and 53.32%, respectively, compared with the T1.CH samples. Figure 2E shows that the firmness of the grape flesh from the three fertilizer treatments decreased during the storage period. At both timepoints, the firmness of grape flesh in the CH samples was the highest, while the B.O samples were the lowest. The firmness of the grape flesh in the CH samples increased by 8.87 and 9.85% compared with A.O and B.O samples, respectively, at the T0 timepoint.
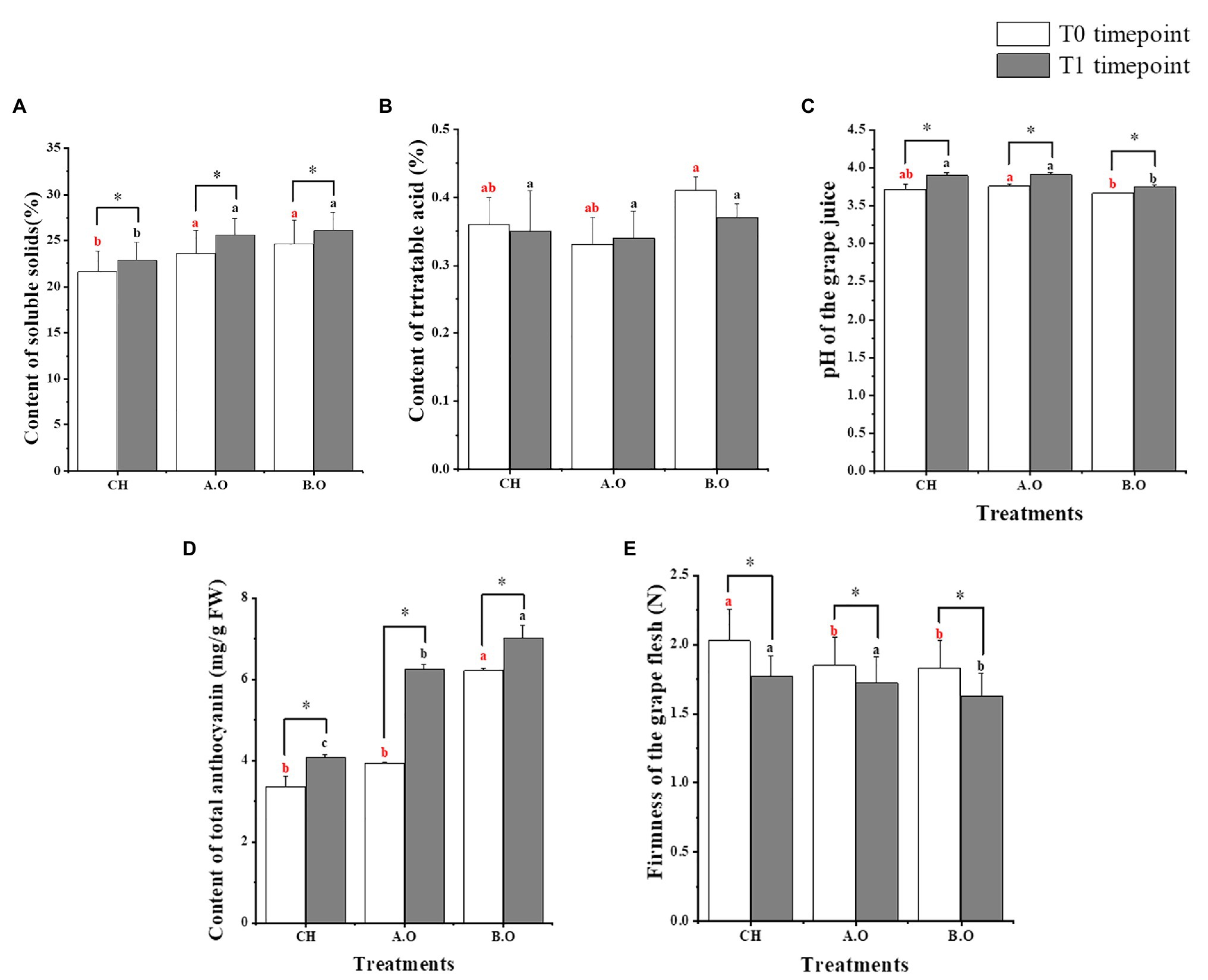
Figure 2. Quality parameters of grape berries. (A) Soluble solids content (SSC) of grape berries. (B) Titratable acid contents. (C) The pH of the grape juice. (D) Total anthocyanins contents. (E) Firmness of the grape flesh. Values presented are the means ± SD (n ≥ 3). Vertical bars indicate the SD of the means. Different letters indicate significant differences among treatments from the same timepoint using Duncan’s test (p < 0.05). ∗ indicates a significant difference between the T0 and T1 timepoint samples of the same treatment using a one-tailed Student’s t-test (p < 0.05).
ITS-Based Fungal Sequencing Data and Fungal Diversity
A total of 848,903 fungal ITS sequences passed quality control. The total number of reads obtained was 415,995 in the grape samples obtained at T0 timepoint and 432,909 in the samples at the T1 timepoint. Then, the high-quality sequences were clustered into OTUs at 97% sequence identity. After filtering rare OTUs, a total of 827,947 sequences were clustered into 527 identified OTUs (Supplementary Table S1).
The observed species and Shannon and ACE indexes of the three fertilizer treatment samples were significantly higher at the T0 timepoint than those at the T1 timepoint. At the T0 timepoint, the Shannon index of the B.O and A.O samples was significantly increased by 14.38 and 11.71%, respectively, compared to the CH samples. The observed species and ACE index of the B.O samples were the highest of three fertilizer treatments. At the T1 timepoint, the Shannon index of the B.O samples was higher than that of the A.O and CH samples, and the difference between the B.O samples and the CH samples was significant. The observed species and ACE index were significantly higher in the B.O samples relative to the A.O and CH samples (Table 2).
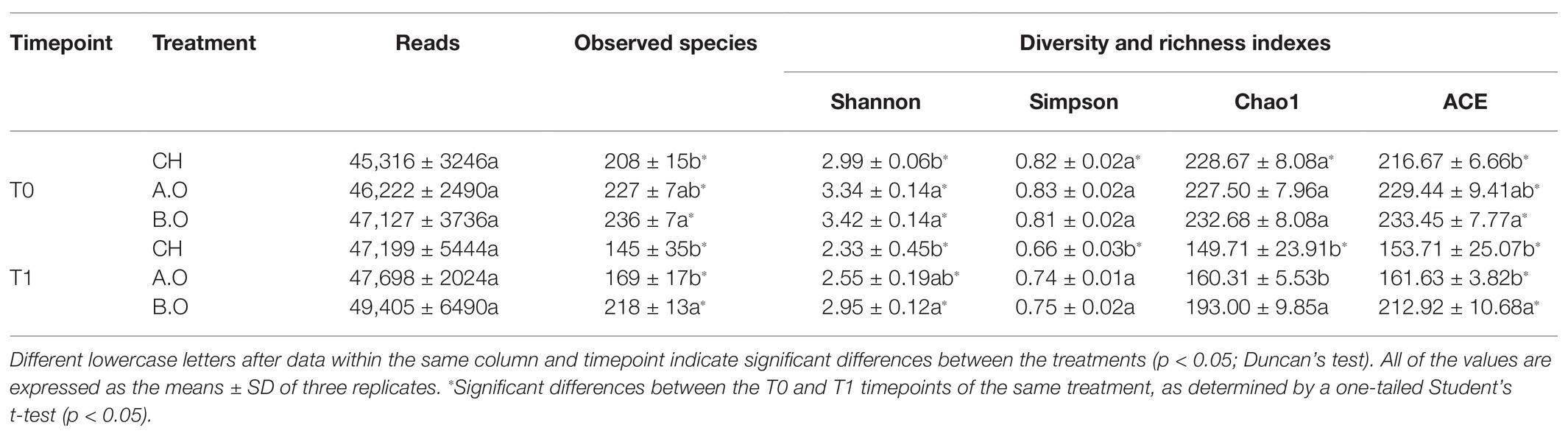
Table 2. Richness and diversity indexes of the fungal communities on the grape berry surfaces following the three treatments across the two timepoints.
Overall Characteristics of the Fungal Community
The percentages and distributions of the predominant fungi at different classification levels (phylum, class, order, family, genus, and species) are shown in Figure 3. Ascomycota was the most abundant phylum, accounting for 94.41% of the total sequences. We also detected Basidiomycota as a minor phylum (5.05%). Dominant classes included Saccharomycetes (48.47%), Dothideomycetes (16.63%), and Tremellomycetes (3.65%). Dominant orders were Saccharomycetales (48.47%), Pleosporales (13.46%), Capnodiales (2.63%), and Helotials (2.57%). Dominant families were Pichiaceae (13.01%), Sclerotiniaceae (2.57%), and Davidiellaceae (2.03%). A total of 99 genera were detected in grape surface samples from the T0 timepoint, and 83 genera were detected in the grape surface samples from the T1 timepoint. The predominant genera of samples from the T0 timepoint were Botrytis (3.55%), Cladosporium (2.72%), Cryptococcus (1.68%), Pichia (1.06%), Aspergillus (0.98%), and Aureobasidium (0.73%). Correspondingly, the predominant genera of samples from the T1 timepoint were Pichia (21.26%), Issatchenkia (3.07%), Botryis (1.58%), Cladosporium (1.34%), Cryptococcus (1.17%), Aureobasidium (0.39%), and Aspergillus (0.32%; Table 3). Most of these highly detected genera included multiple species. A total of nine species Cryptococcus were detected, primarily C. albidus, C. cyanovorans, C. heimaeyensis, and C. heveanensis. Aspergillus was detected with nine species, mainly of A. japonicus, A. pipers, A. cibarius, A. subversicolor, and A. ochraceus. Two species of Aureobasidium, A. pullulans and A. microstictum were highly detected in all samples. A total of seven species of Penicillium were detected primarily P. spinulosum, P. cinnamopurpureum, and P. bialowiezense. Only one species each of Pichia, Issatchenkia, Botrytis, and Cladosporium were detected, P. kluyveri, I. terricola, B. caroliniana, and C. grevilleae, respectively (Supplementary Table S1).
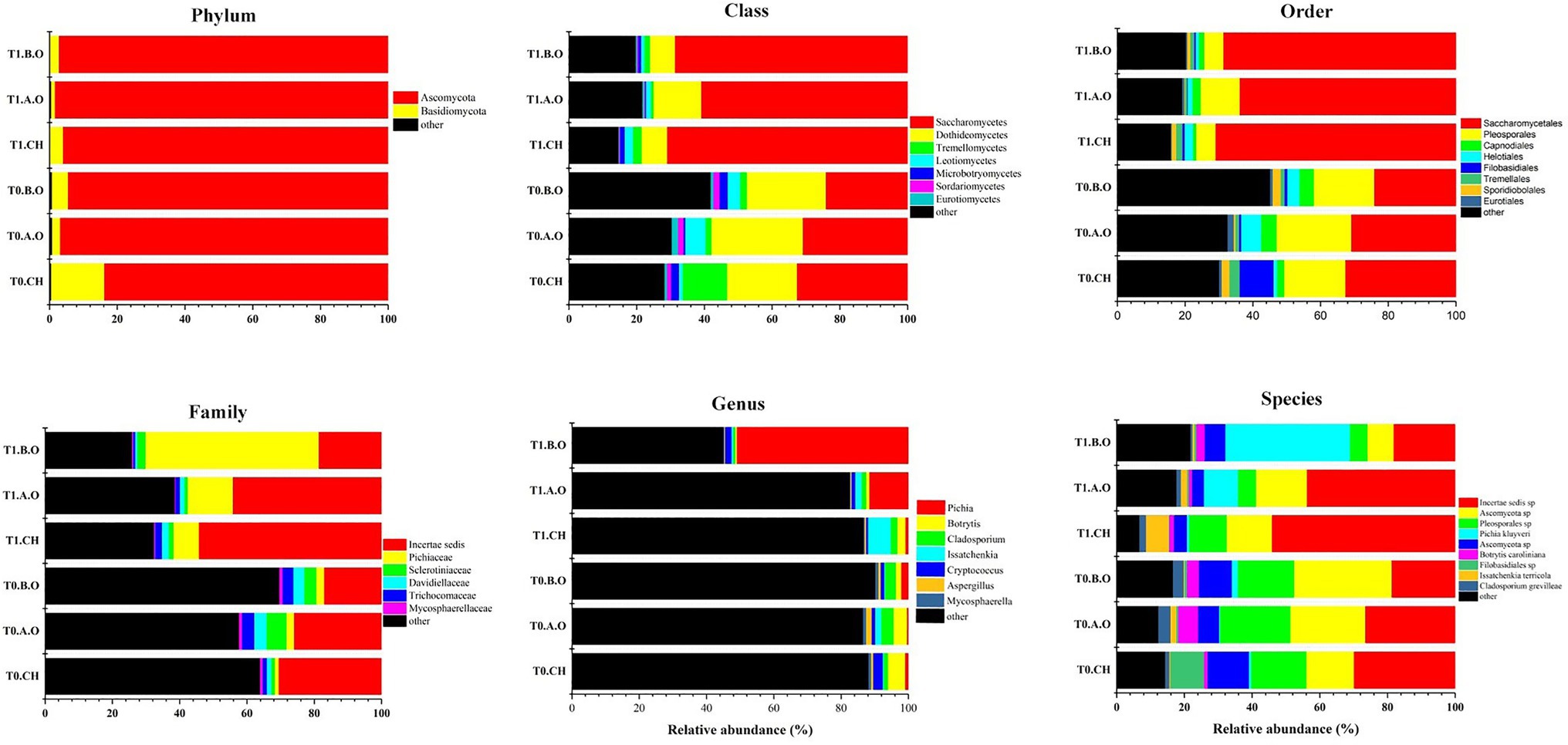
Figure 3. Composition of predominant fungi (abundance ≥ 0.5%) at different taxonomic levels (phylum, class, order, family, genus, and species) from the three fertilizer treatments at the T0 and T1 timepoints.
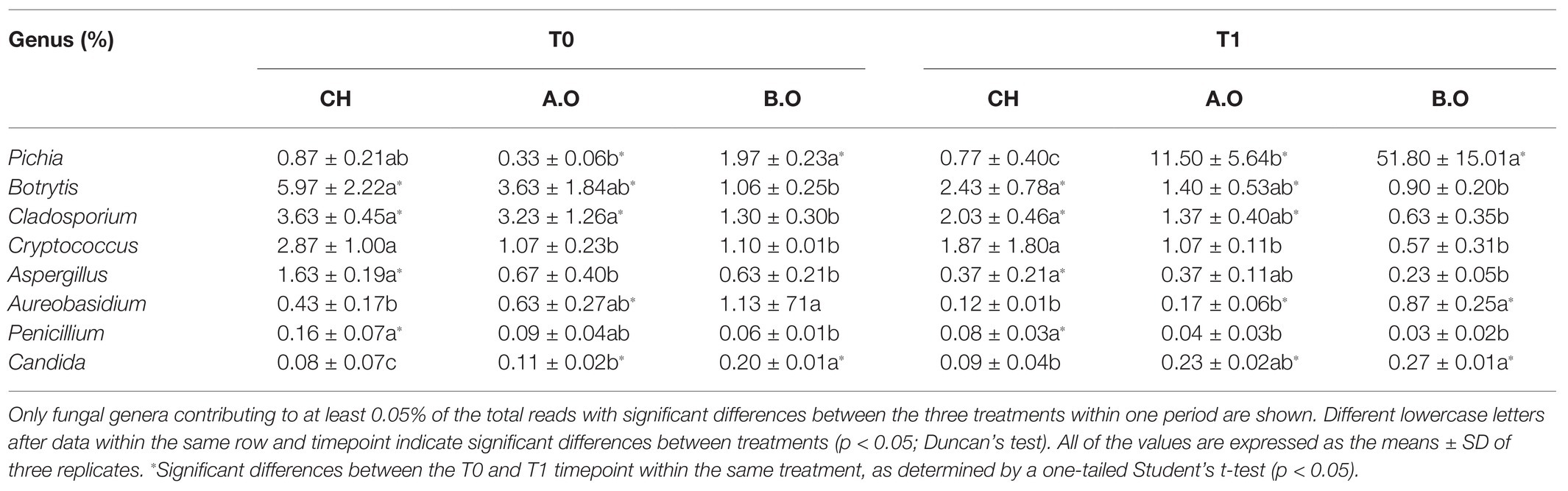
Table 3. Effects of storage time and fertilizer treatment on the fungal communities on the grape berry surface at the genus level.
Fungal Community Variation
The beta diversity results of the weighted UniFrac PCoA showed significant fungal compositional differences between samples from the T0 and T1 timepoints (Figure 4). The weighted UniFrac PCoA clustered the samples into two groups; the grape surface fungal community compositions from the T1.B.O, T1.A.O, and T0.CH samples grouped together, while the other treatments were scattered (Figure 4). PC1 contributed 73.55% and PC2 contributed 13.70%.
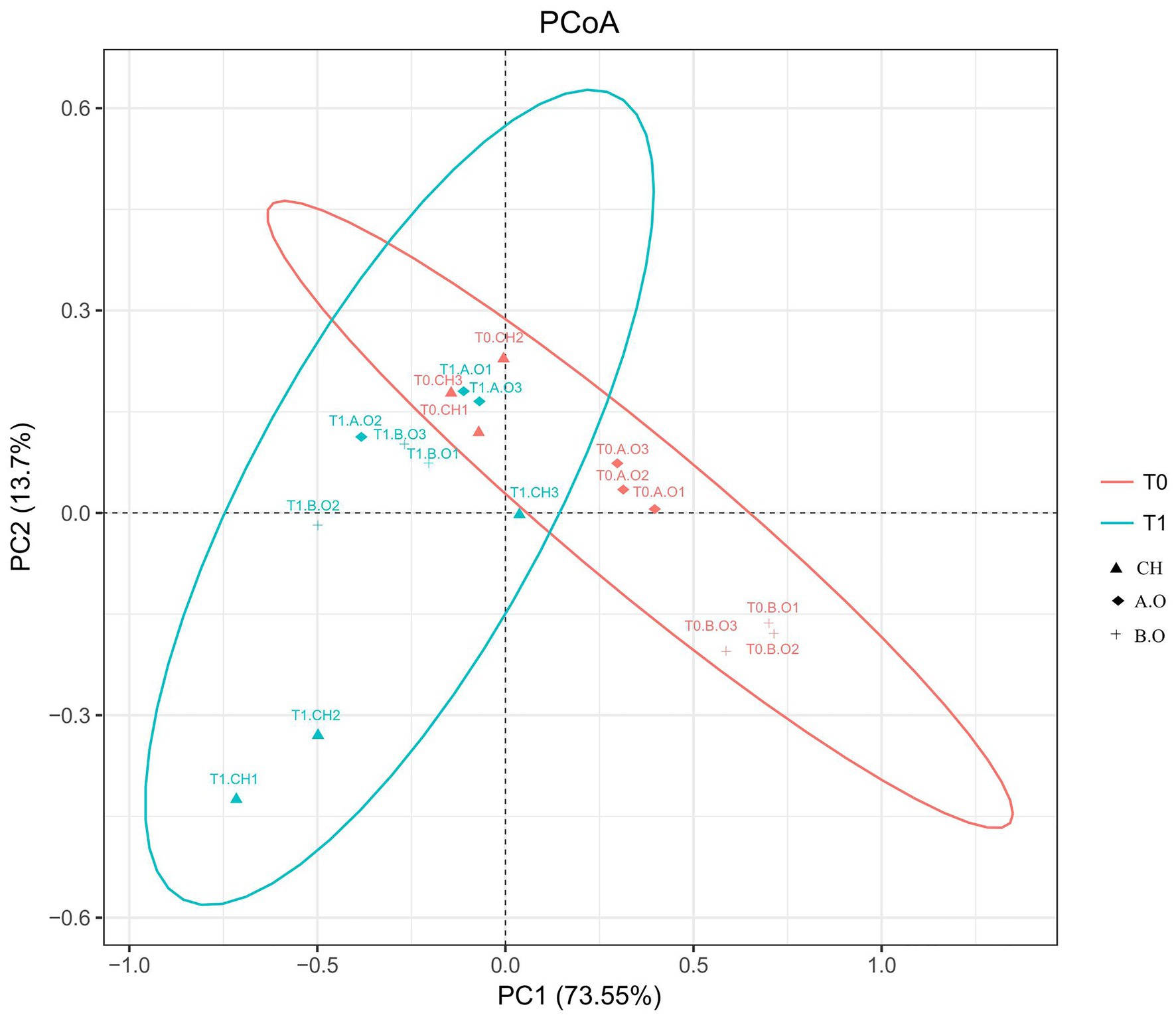
Figure 4. Comparison of fungal communities on the grape berry surface. A principal coordinate analysis (PCoA) based on weighted UniFrac distances was generated with operational taxonomic units (OTUs) (at 97% similarity) present in the three fertilizer treatments. Red colored oval represents samples from the T0 timepoint. Blue colored oval represents samples from the T1 timepoint.
Core Fungal Communities on the Surface of Grape Berries
A total of 157 fungal OTUs were shared by all samples of the three treatments at the T0 and T1 timepoints (Figure 5A). There were more OTUs in the samples from the T0 timepoint (472 OTUs) than that of the samples from T1 timepoint (390 OTUs). From the total number of reads at the T0 timepoint, 345 OTUs were identified in the CH treatment, while 323 and 346 OTUs were identified in the A.O and B.O treatments (Figure 5B), respectively. A total of 225 fungal OTUs were shared by all the samples at T0 timepoint. For the T1 timepoint, 262, 288, and 237 OTUs were identified in B.O, A.O, and CH treatments (Figure 5C), respectively, and 157 fungal OTUs were shared by all the samples at the T1 timepoint. There were significantly more OTUs in the three fertilizer treatments at the T0 timepoint than at the T1 timepoint. The structure of the core fungal community was significantly different between the three treatments at both the T0 and T1 timepoints (Figures 5D,E). The dominant species of the core fungal communities of the samples at the T0 timepoint were Ascomycota sp., Pleosporales sp., Filobasidiales sp., and Botrytis caroliniana, while those of the samples at the T1 timepoint were Pichia kluyveri, Ascomycota sp., Pleosporales sp., and Saccharomycetales sp.
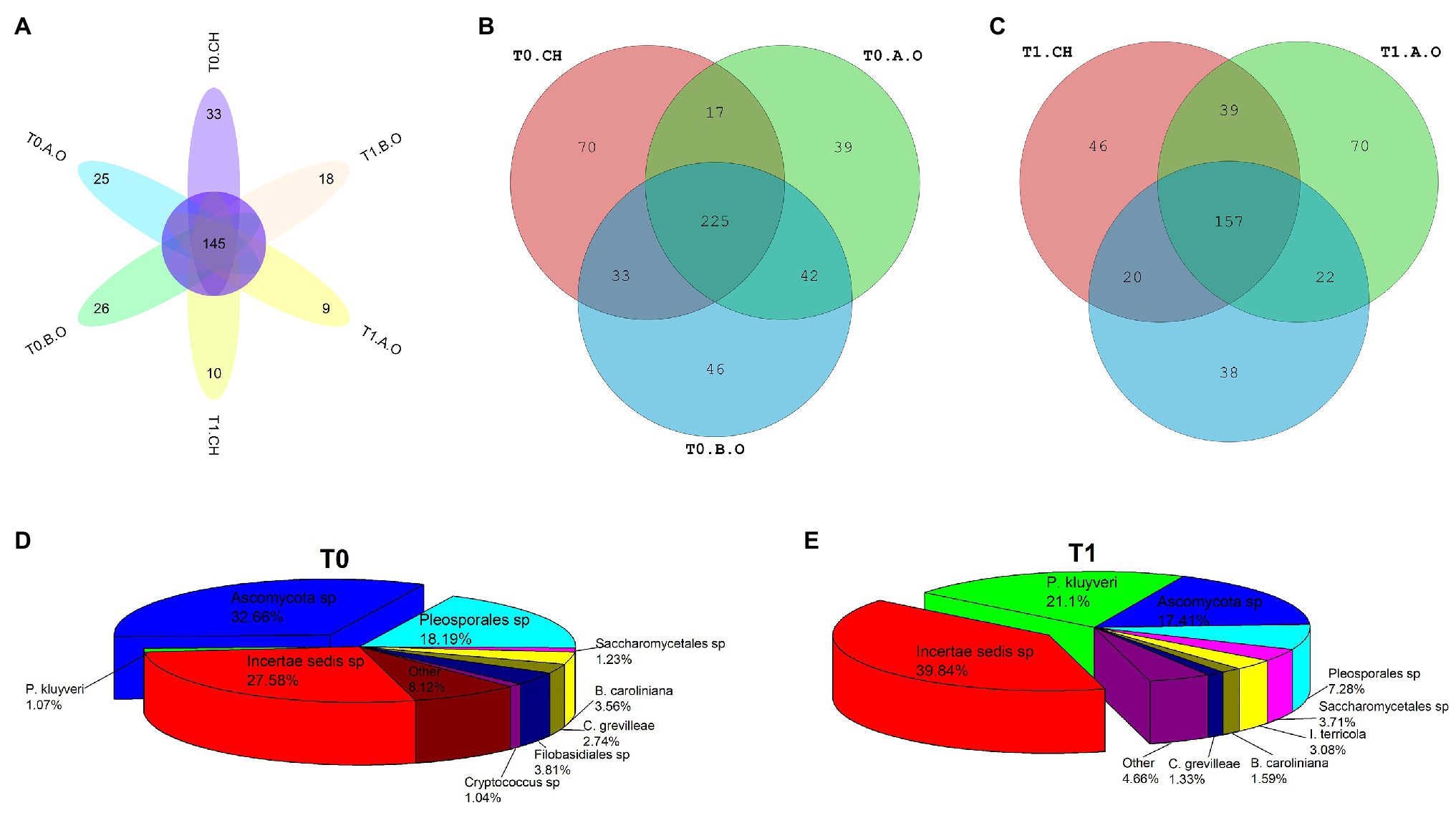
Figure 5. The exclusive and common fungal OTUs on the grape berry surface. (A) Flower chart of the three fertilizer treatments at the T0 and T1 timepoints; (B) Venn diagram of the three fertilizer treatments at the T0 timepoint; (C) Venn diagram of the three fertilizer treatments at the T1 timepoint; (D) Composition of the core fungal community of the three fertilizer treatments at the T0 timepoint; and (E) Composition of the core fungal community of the three fertilizer treatments at the T1 timepoint.
The Fungal Communities at the Genus Level
At the genus level, the relative abundances (RAs) of Pichia and Candida in the organic fertilizer treatments significantly increased after 8 days of storage. By contrast, the RAs of Botrytis, Cladosporium, Cryptococcus, Aspergillus, Aureobasidium, and Penicillium in the three fertilizer treatments decreased during the storage period (Table 3). The RAs of Botrytis in the T0.CH and T0.A.O samples significantly decreased by 59.30 and 61.43%, respectively, compared with the T1.CH and T1.A.O samples. The RAs of Aureobasidium and Candida were the highest of three fertilizer treatments in the B.O samples at both timepoints. The RA of Aureobasidium in the T0.B.O samples was 162.79% higher than that in the T0.CH samples. The RAs of Botrytis, Cladosporium, and Penicillium in the T0.CH samples were significantly higher than those in T0.B.O samples. The RA of Botrytis in T0.B.O samples was 82.42% lower than that in the T0.CH samples. The RAs of Cryptococcus and Aspergillus were significantly higher in the T0.CH samples relative to the T0.B.O and T0.A.O samples. The RA of Aspergillus in the T0.CH samples increased by 61.35 and 58.90% compared to the T0.B.O and T0.A.O samples, respectively (Table 3).
The Fungal Communities at the Species Level
The dominant fungi at species level were Ascomycota sp., Pleosporales sp., and P. kluyver at both timepoints (Figures 5D,E). However, the RAs of these fungi within the fungal community changed. Table 4 shows that the RA of P. kluyver in all three treatments at the T1 timepoint was higher than that at the T0 timepoint, and the same was true for I. terricola and B. caroliniana. The RA of P. kluyver in the T1.A.O and T1.B.O samples was significantly increased by 3509.52 and 1976.68%, respectively, compared to the T0.A.O and T0.B.O samples (Table 4). By contrast, the RAs of Filobasidiales sp., A. pullulans, C. xylopsoci, and A. japonicas in the three fertilizer treatments decreased during the storage period. Aureobasidium pullulans and C. xylopsoci RAs were the highest of three fertilizer treatments in the B.O samples at both timepoints. Aureobasidium pullulans, Filpbasidiales sp., and C. xylopsoci RAs were the lowest of three fertilizer treatments in the CH samples at both timepoints. The A. pullulans RA in the T0.B.O samples increased by 464.29% compared with the T0.CH samples. The abundance of A. pullulans in the T1.B.O samples was 687.50% higher than that in the T1.CH samples. The B. caroliniana and A. japonicus RAs in the T0.CH samples were significantly higher than those in the T0.B.O samples (p < 0.05). The B. caroliniana RA in the T0.B.O samples decreased by 59.73% compared with the T0.CH samples. The I. terricola RA was significantly higher in the T0.CH samples relative to the T0.B.O and T0.A.O samples. The I. terricola RA in the T0.CH samples increased by 89.15 and 85.27% compared to the T0.A.O and T0.B.O samples, respectively (Table 4).
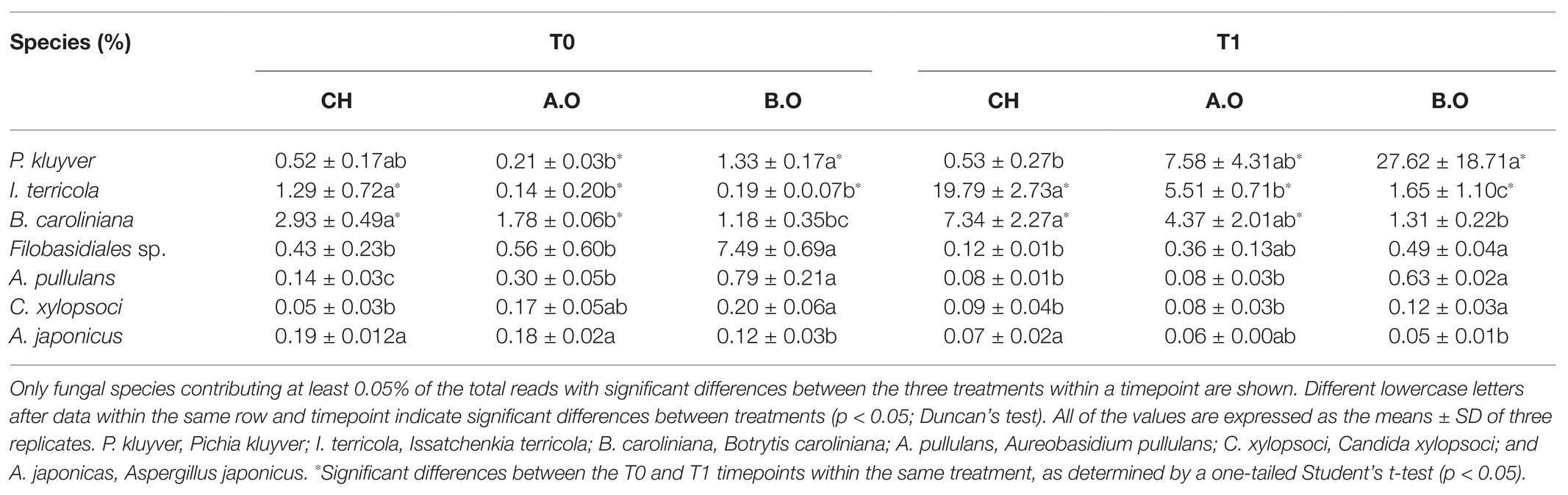
Table 4. Effects of storage time and fertilizer treatment on the fungal communities on the grape berry surface at the species level.
Association Networks Among Fungal Species
Fungal co-occurrence networks among the most abundant fungal species (RA > 0.1%) are shown in Figures 6, 7. A total of 82 interaction pairs related to 25 species were obtained from the T0 timepoint, P. kluyveri (four linkages), I. terricola (four linkages), B. caroliniana (eight linkages), Filobasidiales sp. (eight linkages), A. pullulans (four linkages), C. xylopsoci (four linkages), and A. japonicus (four linkages) exhibited higher degrees of linkage with other species (Figure 6). The species P. kluyveri cooperated with A. pullulans and competed with I. terricola. Aureobasidium pullulans competed with A. japonicus and I. terricola. Botrytis caroliniana competed with C. xylopsoci and Filobasidiales sp. Candida xylopsoci cooperated with Filobasidiales sp. and Cryptococcus victoriae.
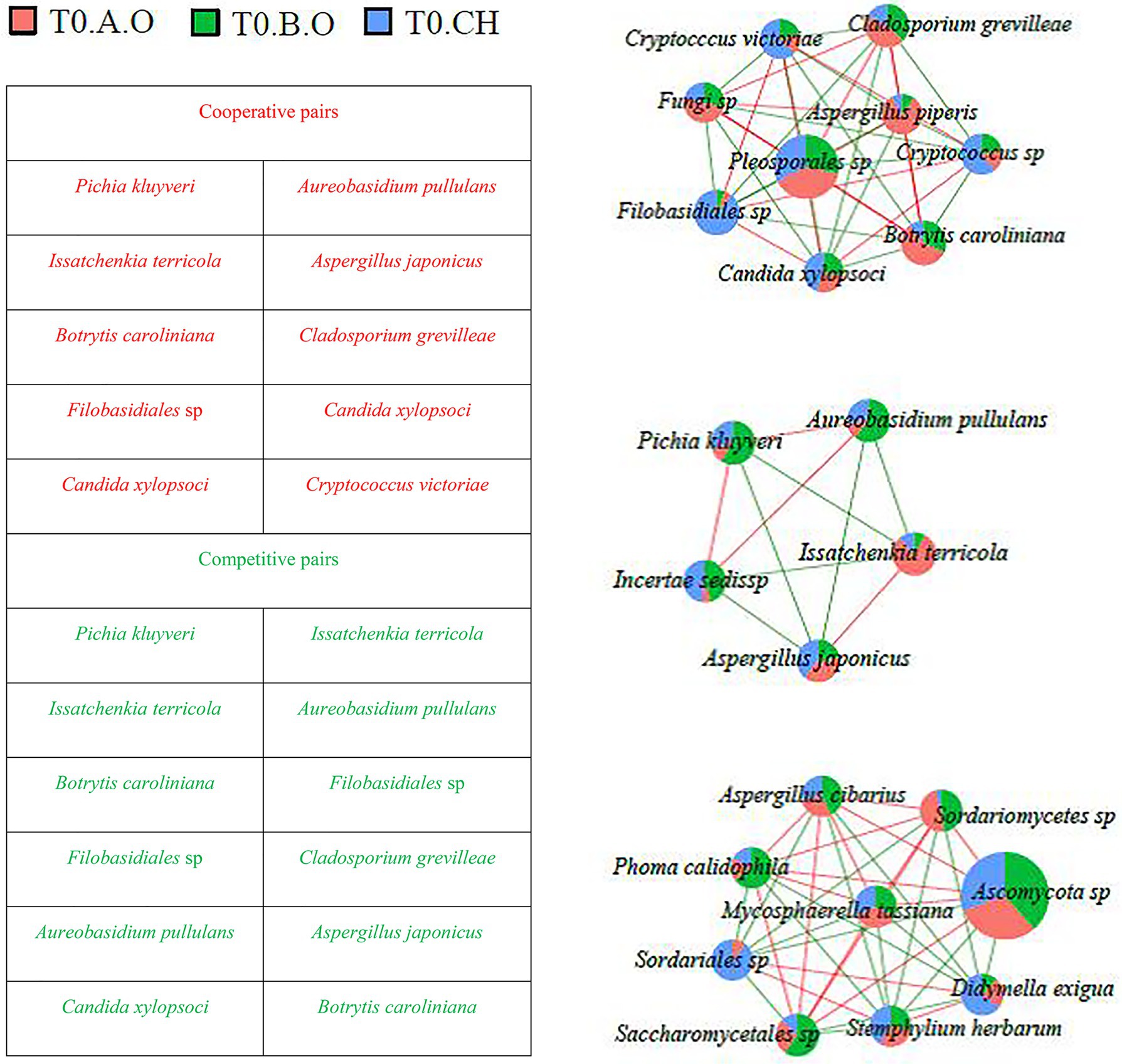
Figure 6. Network diagram of dominant species in the T0 timepoint samples (RA > 0.1%) showing cooperative and competitive associations. Green represents a competitive association and red represents a cooperative association.
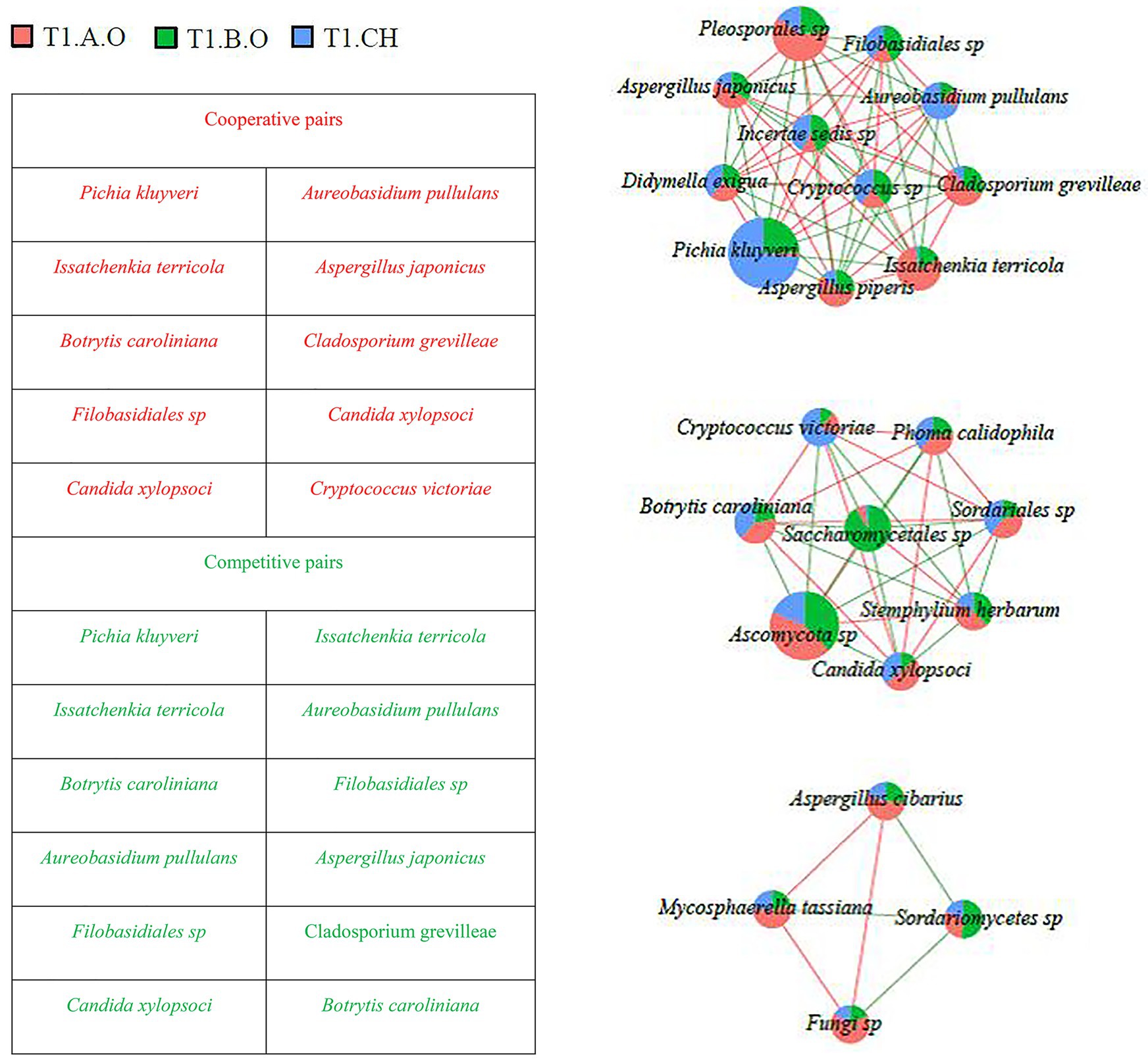
Figure 7. Network diagram of dominant species in the T1 timepoint samples (RA > 0.1%) showing cooperative and competitive associations. Green represents a competitive association and red represents a cooperative association.
As shown in Figure 7, a total of 89 interaction pairs related to 26 species were obtained from the T1 timepoint; P. kluyveri (10 linkages), I. terricola (10 linkages), B. caroliniana (seven linkages), Filobasidiales sp. (10 linkages), A. pullulans (10 linkages), C. xylopsoci (seven linkages), and A. japonicus (10 linkages) exhibited higher degrees of linkage with other species. At the T1 timepoint, the species Filobasidiales sp. was observed to cooperate with Pichia kluyveri and Candida xylopsoci, whereas Filobasidiales sp. was observed to compete with I. terricola. Aspergillus japonicus cooperated with I. terricola and Cryptococcus sp., whereas A. japonicus competed with P. kluyveri and A. pullulans. Issatchenkia terricola competed with Filobasidiales sp. and A. pullulans.
Correlation Analysis Between the Fungal Diversity on the Grape Berry Surface and Quality Parameters of Grape Berries
The Pearson correlation analysis was used to verify the correlations between the experimental indicators, such as firmness, pH, SSC, titratable acid, anthocyanin, and the Shannon, Simpson, Chao1, and ACE indexes, and the indicators of correlation are listed in Figure 8. The firmness of the grape flesh showed a positive correlation with the Chao1, Simpson, ACE, and Shannon indexes, as well as with titratable acid. The firmness of the grape flesh showed a significant negative correlation with anthocyanin and SSC. Moreover, the pH of the grape juice showed a significant negative correlation with the ACE, Chao1, Simpson, and Shannon indexes. Likewise, anthocyanin of grapes had a significant positive correlation with SSC. Additionally, titratable acid showed a positive correlation with the ACE, Chao1, Simpson, and Shannon indexes, and a negative correlation was observed between pH and titratable acid.
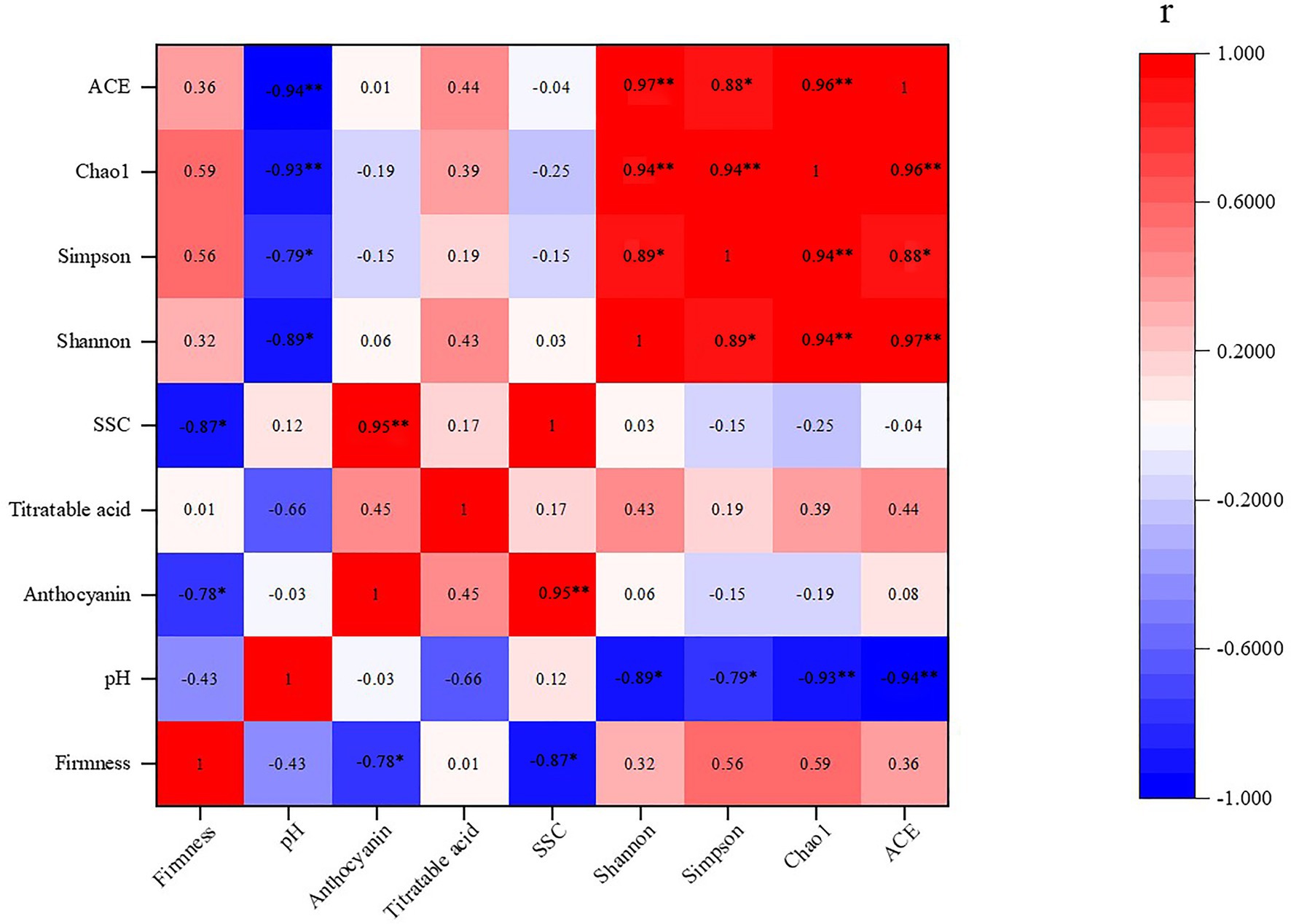
Figure 8. Correlation between the fungal diversity on the grape berry surface and quality parameters of the grape berries. ∗, ∗∗ indicate significant correlations at p < 0.05 and 0.01, respectively.
Discussion
Organic farming is important to maintain or even improve soil quality (Hondebrink et al., 2017). Although organic farming is more eco-friendly and can improve the quality of grapes, it cannot meet the requirements for high grape yields in a short period. In recent years, to maintain high yields and protect the environment, the replacement of chemical fertilizers with organic ones has received more and more attention (Geng et al., 2019). In this study, we showed that the SSC of grape berries treated with organic fertilizer was higher than that of control grapes, and grape berries produced higher TAC with organic fertilizer (Figure 2). Furthermore, increased application of organic fertilizer along with reduced chemical fertilizer for 2 years induced the most significant improve the contents in the SSC and TAC of grape compared to the control grapes from T0 and T1 timepoints.
Fertilizer practices can have an impact on microbial diversity. Agricultural practices affect the community structure of phyllosphere fungal communities associated with Carménère grapevines. Grapes under organic agricultural management had more fungal species than grapes under conventional agricultural management (Castaneda et al., 2018). There are significant differences in the microbial community compositions on the surface of conventional and organic grapes (Leff and Fierer, 2013). Moreover, significant differences in fungal populations were observed in conventional and organic apples. Several unique taxa have been exclusively detected in organic apples (Abdelfattah et al., 2016a). We observed different level of fungal diversity in the fungal taxonomies obtained from the three fertilizer treatments at two timepoints (Figure 3). Also, we found that the Simpson and ACE indexes of the three fertilizer treatments at the T0 timepoint were significantly higher than those of the three fertilizer treatments at the T1 timepoint (Table 2). These results indicated that samples of the three fertilizer treatments at T0 timepoint shared a more diverse of fungal community than samples of the three fertilizer treatments at the T1 timepoint. These results indicate that room temperature storage reduces fungal diversity on the grape berry surface. Furthermore, the Simpson and ACE indexes of A.O and B.O samples (increased organic fertilizer and reduced chemical fertilizer for 1 and 2 years, respectively) were both higher than the CH samples (application of common chemical fertilizer for 2 years). These results indicate that increasing the application of organic fertilizer and reducing the application of chemical fertilizer in the grape rhizosphere can increase the fungal diversity on the grape berry surface. The majority of vine-associated microbial assemblages originate in the soil, and their distribution reflects the influence of vineyard management (Zarraonaindia et al., 2015). Morrison-Whittle et al. (2017) found 24.63% of the fungal genera which were common to soil and on the grape surface. This result was maybe due to the increased organic matter content in the soil that occurred through the application of organic fertilizer, thereby improving the soil properties and increasing the soil fungal diversity. Aboveground (leaves, flowers, and grapes) fungal communities shared a greater proportion of taxa with soil communities (Zarraonaindia et al., 2015), so the fungal diversity on the grape berry surface increased.
Ascomycota was the most highly detected phylum in this study (94.41%). The high percentage of Ascomycota coincided with results from other studies of Kyoho grape berries (80.24%; Abdelfattah et al., 2016b) and wine grape (77.00%; Carmichael et al., 2017). Gao et al. (2019) found that the main genera of four different wine grape cultivars were Aureobasidium, Botrytis, Alternaria, Cladosporium, Mucor, Hanseniaspora, and Cryptococcus, but their abundances in the different cultivars changed significantly. Sclerotinia sp., Cladosporium sp., A. pullulans, and A. alternate were the dominant fungal species in study of Kecskemeti et al. (2016). In our study, the predominant genera of the samples from the T0 timepoint were Botrytis, Cladosporium, Cryptooccus, Pichia, and Aspergillus. Correspondingly, the predominant genera of the samples from the T1 timepoint were Pichia, Issatchenkia, Botryis, Cladosporium, and Cryptococcus. The dominant genera of the three fertilizer treatments were almost unchanged between the T0 and T1 timepoints, but their abundances changed significantly. The components of the core fungal communities were significantly different between samples from the T0 and T1 timepoints (Figures 5D,E), indicating that the epiphytic fungal community on the grape berry surface at the T0 timepoint was significantly different from that of T1 timepoint.
Different fungal communities on the fruit surface can affect the fruit safety. Peri-urban apples samples carried potential pathogenic risks, whereas rural apple maintained more diverse fungal communities on their surfaces (Shen et al., 2018). We analyzed the dominant genera and species of the three fertilizer treatments from the T0 and T1 timepoints with RAs greater than 0.05%. We found that the RAs of Aureobasidium and Candida in the B.O samples were significantly higher than that of the CH samples at both timepoints. The RAs of Aureobasidium and Candida in the A.O samples were also higher than that of the CH samples at both timepoints, but the difference was not significant (Table 3). The epiphytic fungi living in plant tissues, particularly Aureobasidium (Ferreira-Pinto et al., 2006), Candida (Mewa-Ngongang et al., 2019), and Pichia (Mewa-Ngongang et al., 2019), play essential roles in disease control. Aureobasidium pullulans was used as a biocontrol agent against B. cinerea on grapes (Wang et al., 2018a). Certain fungal genera enriched in the B.O and A.O samples have been reported to exhibit protective functions. Therefore, the soil environment by organic fertilizer may provide certain advantages in maintaining healthier fungal communities on grape surfaces. Botrytis, Cladosporium, Cryptococcus, Aspergillus, and Penicillium were enriched in the CH samples. Significantly, several of these genera have been reported to exhibit pathogenic features and may be related with fruit decay. Specifically, Penicillium and Aspergillus are wildly reported to cause fruit deterioration and mycotoxin contamination. Botrytis causes gray mold on grapevines and strawberries (Haidar et al., 2016; Dowling et al., 2017). Penicillium causes grape losses (Platania et al., 2012; Parafati et al., 2015). Cladosporium was most common and important fungal agent of grape bunch rot (Mahdian and Zafari, 2016). Aspergillus japonicus is an important black Aspergillus species that may cause grape black rot (Xanthopoulou et al., 2018; Apud et al., 2019). So we suggested that CH samples carried more potential microbiological risks. These results indicated that the increased application of organic fertilizer and reduced application of chemical fertilizer to grapes could increase the RAs of beneficial fungal species on the grape surface. Increased organic fertilizer and reduced chemical fertilizer for 2 years had the most significant effect on increasing the beneficial fungal species on the grape surface.
Inter-species interactions are extremely important in shaping fungal dynamics (Shen et al., 2018). The network analysis results are shown in Figures 6, 7 demonstrate potential intra-species relationships. We observed that A. pullulans was competitive with I. terricola and A. japonicas. Aureobasidium pullulans was cooperative with P. kluyveri and C. xylopsoci. Candida xylopsoci competed with B. caroliniana. The fungal species with biological control activity cooperated against the pathogenic fungal species (Shen et al., 2018). pH significantly affected the fungal community structure, which was similar to the results of soil fungal and archaeal community structures (Wang et al., 2019) and soil bacterial community structures (Wang et al., 2018b) in an ongoing antimony mine area. The pH of the grape juice was significantly positively correlated with the Shannon, Simpson, Chao1, and ACE indexes (Figure 8). The Shannon, Simpson, Chao1, and ACE indexes were positively correlated with firmness and titratable acidity, but the correlations were not significant. Consequently, the pH of the grape juice can be regarded as a key factor affecting the fungal richness and diversity on the grape berry surface. In our past study, we found that increasing the application of organic fertilizer can significantly affect the pH of the grape juice (Zhao et al., 2020). Ren et al. (2019) also found that the fungal community structure of Pinggu peach trees was significantly correlated with pH.
In summary, increasing the application of organic fertilizer to the root zone of grapes decreased the pH of the grape juice and increased the soluble solids of the grapes, and went on to increase the fungal diversity and RAs of P. kluyver, A. pullulans, and C. xylopsoci (beneficial fungi) on the surface of the grape berries at T0 and T1 timepoints. The positive effect of increased organic fertilizer and reduced chemical fertilizer for 2 years was the most significant. Moreover, significant differences were revealed between the two assessment times (T0 and T1). After 8 days of storage at room temperature, the fungal diversity on the surface of the grape berries significantly decreased. The correlation analysis suggested that the pH of the grape juice was significantly negatively correlated with fungal diversity parameters.
Data Availability Statement
The datasets generated can be found in the NCBI SRA under the Bioproject: ID PRJNA678874.
Author Contributions
LW, ZL, and KY designed the study. LW performed the experiments and was involved with writing the manuscript. JF, HL, and KY contributed to revising the manuscript. FZ and BZ participated in lab work and helped with data analysis. All authors contributed to the article and approved the submitted version.
Funding
This work was supported by the National Key Research and Development Program (2018YFD1000200), the Natural Science Foundation of China (31760550), and the Transformation Project of Scientific and Technological Achievements of the Xinjiang Production and Construction Corps (2020BA006).
Conflict of Interest
The authors declare that the research was conducted in the absence of any commercial or financial relationships that could be construed as a potential conflict of interest.
Acknowledgments
We are very grateful to Xiaoxia Sun for excellent technical support.
Supplementary Material
The Supplementary Material for this article can be found online at: https://www.frontiersin.org/articles/10.3389/fmicb.2021.628503/full#supplementary-material
References
Abdelfattah, A., Wisniewski, M., Droby, S., and Schena, L. (2016a). Spatial and compositional variation in the fungal communities of organic and conventionally grown apple fruit at the consumer point-of-purchase. Hortic. Res. 3:16047. doi: 10.1038/hortres.2016.47
Abdelfattah, A., Wisniewski, M., Li Destri Nicosia, M. G., Cacciola, S. O., and Schena, L. (2016b). Metagenomic analysis of fungal diversity on strawberry plants and the effect of management practices on the fungal community structure of aerial organs. PLoS One 11:e0160470. doi: 10.1371/journal.pone.0160470
Agirman, B., and Erten, H. (2020). Biocontrol ability and action mechanisms of Aureobasidium pullulans GE17 and Meyerozyma guilliermondii KL3 against Penicillium digitatum DSM2750 and Penicillium expansum DSM62841 causing postharvest diseases. Yeast 37, 437–448. doi: 10.1002/yea.3501
Akila, B.-T., Eddine, M. A., Wassila, A.-M., Milan, Š., Jana, Č., Robert, P., et al. (2020). Lasiodiplodia mitidjana sp. nov. and other Botryosphaeriaceae species causing branch canker and dieback of Citrus sinensis in Algeria. PLoS One 15:e0232448. doi: 10.1371/journal.pone.0232448
Angeli, D., Sare, A. R., Jijakli, M. H., Pertot, I., and Massart, S. (2019). Insights gained from metagenomic shotgun sequencing of apple fruit epiphytic microbiota. Postharvest Biol. Technol. 153, 96–106. doi: 10.1016/j.postharvbio.2019.03.020
Apud, G. R., Aredes-Fernandez, P. A., Kritsanida, M., Grougnet, R., and Sampietro, D. A. (2019). Antifungal activity of Bignoniaceae plants on Aspergillus carbonarius and Aspergillus niger. Nat. Prod. Res. 34, 2656–2659. doi: 10.1080/14786419.2018.1548453
Barata, A., Malfeito-Ferreira, M., and Loureiro, V. (2012). The microbial ecology of wine grape berries. Int. J. Food Microbiol. 153, 243–259. doi: 10.1016/j.ijfoodmicro.2011.11.025
Bokulich, N. A., Thorngate, J. H., Richardson, P. M., and Mills, D. A. (2014). Microbial biogeography of wine grapes is conditioned by cultivar, vintage, and climate. Proc. Natl. Acad. Sci. U. S. A. 111, E139–E148. doi: 10.1073/pnas.1317377110
Buchholz, F., Kostic, T., Sessitsch, A., and Mitter, B. (2018). The potential of plant microbiota in reducing postharvest food loss. Microb. Biotechnol. 11, 971–975. doi: 10.1111/1751-7915.13252
Carmichael, P. C., Siyoum, N., Chidamba, L., and Korsten, L. (2017). Characterization of fungal communities of developmental stages in table grape grown in the northern region of South Africa. J. Appl. Microbiol. 123, 1251–1262. doi: 10.1111/jam.13577
Castaneda, L. E., Miura, T., Sanchez, R., and Barbosa, O. (2018). Effects of agricultural management on phyllosphere fungal diversity in vineyards and the association with adjacent native forests. PeerJ 6:e5715. doi: 10.7717/peerj.5715
Chen, X., Wang, Y., Gao, Y., Gao, T., and Zhang, D. (2019). Inhibitory abilities of Bacillus isolates and their culture filtrates against the gray mold caused by Botrytis cinerea on postharvest fruit. Plant Pathol. J. 35, 425–436. doi: 10.5423/PPJ.OA.03.2019.0064
Cheng, H., Zhang, D., Huang, B., Song, Z., Ren, L., Hao, B., et al. (2020). Organic fertilizer improves soil fertility and restores the bacterial community after 1,3-dichloropropene fumigation. Sci. Total Environ. 738:140345. doi: 10.1016/j.scitotenv.2020.140345
Ding, S., Li, N., Cao, M. M., Huang, Q. F., Chen, G. P., Xie, S. Y., et al. (2019). Diversity of epiphytic fungi on the surface of Kyoho grape berries during ripening process in summer and winter at Nanning region, Guangxi, China. Fungal Biol. 123, 283–289. doi: 10.1016/j.funbio.2018.11.014
Dowling, M. E., Hu, M. J., and Schnabel, G. (2017). Identification and characterization of Botrytis fragariae isolates on strawberry in the United States. Plant Dis. 101, 1769–1773. doi: 10.1094/PDIS-03-17-0316-RE
El-Ashram, S., and Suo, X. (2017). Exploring the microbial community (microflora) associated with ovine Haemonchus contortus (macroflora) field strains. Sci. Rep. 7:70. doi: 10.1038/s41598-017-07623-9
Escribano-Viana, R., Lopez-Alfaro, I., Lopez, R., Santamaria, P., Gutierrez, A. R., and Gonzalez-Arenzana, L. (2018). Impact of chemical and biological fungicides applied to grapevine on grape biofilm, must, and wine microbial diversity. Front. Microbiol. 9:59. doi: 10.3389/fmicb.2018.00059
Ferreira-Pinto, M. M., Moura-Guedes, M. C., Barreiro, M. G., Pais, I., Santos, M. R., and Silva, M. J. (2006). Aureobasidium pullulans as a biocontrol agent of blue mold in "Rocha" pear. Commun. Agric. Appl. Biol. Sci. 71, 973–978.
Gao, F. F., Chen, J. L., Xiao, J., Cheng, W. D., Zheng, X. J., Wang, B., et al. (2019). Microbial community composition on grape surface controlled by geographical factors of different wine regions in Xinjiang, China. Food. Res. Int. 122, 348–360. doi: 10.1016/j.foodres.2019.04.029
García-Díaz, A., Bienes, R., Sastre, B., Novara, A., Gristina, L., and Cerdà, A. (2017). Nitrogen losses in vineyards under different types of soil groundcover. A field runoff simulator approach in Central Spain. Agric. Ecosyst. Environ. 236, 257–267. doi: 10.1016/j.agee.2016.12.013
Geng, Y., Cao, G., Wang, L., and Wang, S. (2019). Effects of equal chemical fertilizer substitutions with organic manure on yield, dry matter, and nitrogen uptake of spring maize and soil nitrogen distribution. PLoS One 14:e0219512. doi: 10.1371/journal.pone.0219512
Haidar, R., Deschamps, A., Roudet, J., Calvo-Garrido, C., Bruez, E., Rey, P., et al. (2016). Multi-organ screening of efficient bacterial control agents against two major pathogens of grapevine. Biol. Control 92, 55–65. doi: 10.1016/j.biocontrol.2015.09.003
Hao, X. H., Liu, S. L., Wu, J. S., Hu, R. G., Tong, C. L., and Su, Y. Y. (2008). Effect of long-term application of inorganic fertilizer and organic amendments on soil organic matter and microbial biomass in three subtropical paddy soils. Nutr. Cycl. Agroecosyst. 81, 17–24. doi: 10.1007/s10705-007-9145-z
Hao, D. C., Song, S. M., Mu, J., Hu, W. L., and Xiao, P. G. (2016). Unearthing microbial diversity of Taxus rhizosphere via MiSeq high-throughput amplicon sequencing and isolate characterization. Sci. Rep. 6:22006. doi: 10.1038/srep22006
Hayden, C. J., and Beman, J. M. (2016). Microbial diversity and community structure along a lake elevation gradient in Yosemite National Park, California, USA. Environ. Microbiol. 18, 1782–1791. doi: 10.1111/1462-2920.12938
Hondebrink, M. A., Cammeraat, L. H., and Cerda, A. (2017). The impact of agricultural management on selected soil properties in citrus orchards in eastern Spain: a comparison between conventional and organic citrus orchards with drip and flood irrigation. Sci. Total Environ. 581-582, 153–160. doi: 10.1016/j.scitotenv.2016.12.087
Horn, S., Hempel, S., Verbruggen, E., Rillig, M. C., and Caruso, T. (2017). Linking the community structure of arbuscular mycorrhizal fungi and plants: a story of interdependence? ISME J. 11, 1400–1411. doi: 10.1038/ismej.2017.5
Jurick, W. M. 2nd, Peng, H., Beard, H. S., Garrett, W. M., Lichtner, F. J., Luciano-Rosario, D., et al. (2020). Blistering1 modulates Penicillium expansum virulence via vesicle-mediated protein secretion. Mol. Cell. Proteomics 19, 344–361. doi: 10.1074/mcp.RA119.001831
Kao, C. M., Liao, H. Y., Chien, C. C., Tseng, Y. K., Tang, P., Lin, C. E., et al. (2016). The change of microbial community from chlorinated solvent-contaminated groundwater after biostimulation using the metagenome analysis. J. Hazard. Mater. 302, 144–150. doi: 10.1016/j.jhazmat.2015.09.047
Kecskemeti, E., Berkelmann-Lohnertz, B., and Reineke, A. (2016). Are epiphytic microbial communities in the carposphere of ripening grape clusters (Vitis vinifera L.) different between conventional, organic, and biodynamic grapes? PLoS One 11:e0160852. doi: 10.1371/journal.pone.0160852
Leff, J. W., and Fierer, N. (2013). Bacterial communities associated with the surfaces of fresh fruits and vegetables. PLoS One 8:e59310. doi: 10.1371/journal.pone.0059310
Li, J., Hu, W., Huang, X., and Xu, Y. (2018). Investigation of yeast population diversity and dynamics in spontaneous fermentation of vidal blanc icewine by traditional culture-dependent and high-throughput sequencing methods. Food Res. Int. 112, 66–77. doi: 10.1016/j.foodres.2018.06.011
Liu, H. T., Wang, Y. W., Huang, W. D., and Lei, M. (2016). Response of wine grape growth, development and the transfer of copper, lead, and cadmium in soil-fruit system to sludge compost amendment. Environ. Sci. Pollut. Res. Int. 23, 24230–24236. doi: 10.1007/s11356-016-7676-z
Liu, E., Yan, C., Mei, X., He, W., Bing, S. H., Ding, L., et al. (2010). Long-term effect of chemical fertilizer, straw, and manure on soil chemical and biological properties in Northwest China. Geoderma 158, 173–180. doi: 10.1016/j.geoderma.2010.04.029
Lorenzini, M., Simonato, B., Favati, F., Bernardi, P., Sbarbati, A., and Zapparoli, G. (2018). Filamentous fungi associated with natural infection of noble rot on withered grapes. Int. J. Food Microbiol. 272, 83–86. doi: 10.1016/j.ijfoodmicro.2018.03.004
Lorenzini, M., and Zapparoli, G. (2019). Yeast-like fungi and yeasts in withered grape carposphere: characterization of Aureobasidium pullulans population and species diversity. Int. J. Food Microbiol. 289, 223–230. doi: 10.1016/j.ijfoodmicro.2018.10.023
Luan, H., Gao, W., Huang, S., Tang, J., Li, M., Zhang, H., et al. (2020). Substitution of manure for chemical fertilizer affects soil microbial community diversity, structure and function in greenhouse vegetable production systems. PLoS One 15:e0214041. doi: 10.1371/journal.pone.0214041
Luciano-Rosario, D., Keller, N. P., and Jurick, W. M. 2nd (2020). Penicillium expansum: biology, omics, and management tools for a global postharvest pathogen causing blue mould of pome fruit. Mol. Plant Pathol. 21, 1391–1404. doi: 10.1111/mpp.12990
Lv, F., Song, J., Giltrap, D., Feng, Y., Yang, X., and Zhang, S. (2020). Crop yield and N2O emission affected by long-term organic manure substitution fertilizer under winter wheat-summer maize cropping system. Sci. Total Environ. 732:139321. doi: 10.1016/j.scitotenv.2020.139321
Mahdian, S., and Zafari, D. (2016). First report of alternaria cucurbitae causing bunch rot on grape in Iran. J. Plant Pathol. 98:185.
Marsh, A. J., O'Sullivan, O., Hill, C., Ross, R. P., and Cotter, P. D. (2013). Sequencing-based analysis of the bacterial and fungal composition of kefir grains and milks from multiple sources. PLoS One 8:e69371. doi: 10.1371/journal.pone.0069371
Martins, G., Lauga, B., Miot-Sertier, C., Mercier, A., Lonvaud, A., Soulas, M. -L., et al. (2013). Characterization of epiphytic bacterial communities from grapes, leaves, bark and soil of grapevine plants grown, and their relations. PLoS One 8:e73013. doi: 10.1371/journal.pone.0073013
Martins, G., Vallance, J., Mercier, A., Albertin, W., Stamatopoulos, P., Rey, P., et al. (2014). Influence of the farming system on the epiphytic yeasts and yeast-like fungi colonizing grape berries during the ripening process. Int. J. Food Microbiol. 177, 21–28. doi: 10.1016/j.ijfoodmicro.2014.02.002
Mewa-Ngongang, M., Plessis, H. W. D., Ntwampe, S. K. O., Chidi, B. S., Hutchinson, U. F., Mekuto, L., et al. (2019). The use of Candida pyralidae and Pichia kluyveri to control spoilage microorganisms of raw fruits used for beverage production. Foods 8:454. doi: 10.3390/foods8100454
Moreno-Jiménez, E., Plaza, C., Saiz, H., Manzano, R., Flagmeier, M., and Maestre, F. T. (2019). Aridity and reduced soil micronutrient availability in global drylands. Nat. Sustain. 2, 371–377. doi: 10.1038/s41893-019-0262-x
Morrison-Whittle, P., Lee, S. A., and Goddard, M. R. (2017). Fungal communities are differentially affected by conventional and biodynamic agricultural management approaches in vineyard ecosystems. Agric. Ecosyst. Environ. 246, 306–313. doi: 10.1016/j.agee.2017.05.022
Noguerol-Pato, R., Torrado-Agrasar, A., Gonzalez-Barreiro, C., Cancho-Grande, B., and Simal-Gandara, J. (2014). Influence of new generation fungicides on Saccharomyces cerevisiae growth, grape must fermentation and aroma biosynthesis. Food Chem. 146, 234–241. doi: 10.1016/j.foodchem.2013.09.058
Oliveira, M., Arenas, M., Lage, O., Cunha, M., and Amorim, M. I. (2018). Epiphytic fungal community in Vitis vinifera of the portuguese wine regions. Lett. Appl. Microbiol. 66, 93–102. doi: 10.1111/lam.12826
Onesti, G., González-Domínguez, E., and Rossi, V. (2017). Production of pycnidia and conidia by Guignardia bidwellii, the causal agent of grape black rot, as affected by temperature and humidity. Phytopathology 107, 173–183. doi: 10.1094/PHYTO-07-16-0255-R
Parafati, L., Vitale, A., Restuccia, C., and Cirvilleri, G. (2015). Biocontrol ability and action mechanism of food-isolated yeast strains against Botrytis cinerea causing post-harvest bunch rot of table grape. Food Microbiol. 47, 85–92. doi: 10.1016/j.fm.2014.11.013
Platania, C., Restuccia, C., Muccilli, S., and Cirvilleri, G. (2012). Efficacy of killer yeasts in the biological control of Penicillium digitatum on tarocco orange fruits (Citrus sinensis). Food Microbiol. 30, 219–225. doi: 10.1016/j.fm.2011.12.010
Ren, F., Dong, W., and Yan, D. H. (2019). Organs, cultivars, soil, and fruit properties affect structure of endophytic mycobiota of pinggu peach trees. Microorganisms 7:322. doi: 10.3390/microorganisms7090322
Renouf, V., Claisse, O., and Lonvaud-Funel, A. (2005). Understanding the microbial ecosystem on the grape berry surface through numeration and identification of yeast and bacteria. Aust. J. Grape Wine Res. 11, 316–327. doi: 10.1111/j.1755-0238.2005.tb00031.x
Sebastien, M., Margarita, M. M., and Haissam, J. M. (2015). Biological control in the microbiome era: challenges and opportunities. Biol. Control 89, 98–108. doi: 10.1016/j.biocontrol.2015.06.003
Sharma, S., Sharma, S. D., and Kumar, P. (2017). Response of nectarines to organic fertilization under the rain-fed ecosystem of Northwest Himalayas. J. Plant Nutr. 40, 2014–2025. doi: 10.1080/01904167.2017.1346118
Shen, Y., Nie, J., Kuang, L., Zhang, J., and Li, H. (2020). DNA sequencing, genomes and genetic markers of microbes on fruits and vegetables. Microb. Biotechnol. 14, 323–362. doi: 10.1111/1751-7915.13560
Shen, Y., Nie, J., Li, Z., Li, H., Wu, Y., Dong, Y., et al. (2018). Differentiated surface fungal communities at point of harvest on apple fruits from rural and peri-urban orchards. Sci. Rep. 8:2165. doi: 10.1038/s41598-017-17436-5
Song, H., Wang, J., Zhang, K., Zhang, M., Hui, R., Sui, T., et al. (2020). A 4-year field measurement of N2O emissions from a maize-wheat rotation system as influenced by partial organic substitution for synthetic fertilizer. J. Environ. Manag. 263:110384. doi: 10.1016/j.jenvman.2020.110384
Tao, R., Wakelin, S. A., Liang, Y., Hu, B., and Chu, G. (2018). Nitrous oxide emission and denitrifier communities in drip-irrigated calcareous soil as affected by chemical and organic fertilizers. Sci. Total Environ. 612, 739–749. doi: 10.1016/j.scitotenv.2017.08.258
Temple, T. N., Thompson, E. C., Uppala, S., Granatstein, D., and Johnson, K. B. (2020). Floral colonization dynamics and specificity of Aureobasidium pullulans strains used to suppress fire blight of pome fruit. Plant Dis. 104, 121–128. doi: 10.1094/PDIS-09-18-1512-RE
Tilman, D., Balzer, C., Hill, J., and Befort, B. L. (2011). Global food demand and the sustainable intensification of agriculture. Proc. Natl. Acad. Sci. U. S. A. 108, 20260–20264. doi: 10.1073/pnas.1116437108
Úrbez-Torres, J. R. (2011). The status of Botryosphaeriaceae species infecting grapevines. Phytopathol. Mediterr. 50, S5–S45.
Wang, X., Glawe, D. A., Kramer, E., Weller, D., and Okubara, P. A. (2018a). Biological control of Botrytis cinerea: interactions with native vineyard yeasts from Washington state. Phytopathology 108, 691–701. doi: 10.1094/PHYTO-09-17-0306-R
Wang, N., Wang, A., Xie, J., and He, M. (2019). Responses of soil fungal and archaeal communities to environmental factors in an ongoing antimony mine area. Sci. Total Environ. 652, 1030–1039. doi: 10.1016/j.scitotenv.2018.10.300
Wang, N., Zhang, S., and He, M. (2018b). Bacterial community profile of contaminated soils in a typical antimony mining site. Environ. Sci. Pollut. Res. 25, 141–152. doi: 10.1007/s11356-016-8159-y
Wei, S., Zhang, Y., Wang, H., Wang, H., Gao, H., and Wang, S. (2012). Effect of organic fertilizer and bagging on fruit flavor quality of Yali pear. Plant Nutr. Fer. Sci. 18, 1269–1276.
Wu, L., Jiang, Y., Zhao, F., He, X., Liu, H., and Yu, K. (2020). Increased organic fertilizer application and reduced chemical fertilizer application affect the soil properties and bacterial communities of grape rhizosphere soil. Sci. Rep. 10:9568. doi: 10.1038/s41598-020-66648-9
Xanthopoulou, A., Ganopoulos, I., Tryfinopoulou, P., Panagou, E. Z., Osanthanunkul, M., Madesis, P., et al. (2018). Rapid and accurate identification of black aspergilli from grapes using high-resolution melting (HRM) analysis. J. Sci. Food Agric. 99, 309–314. doi: 10.1002/jsfa.9189
Xiao, L., Sun, Q., Yuan, H., and Lian, B. (2017). A practical soil management to improve soil quality by applying mineral organic fertilizer. Acta Geochim. 36, 198–204. doi: 10.1007/s11631-017-0139-5
Xu, C., Wang, C., Ju, L., Zhang, R., Biggs, A. R., Tanaka, E., et al. (2015). Multiple locus genealogies and phenotypic characters reappraise the causal agents of apple ring rot in China. Fungal Divers. 71, 215–231. doi: 10.1007/s13225-014-0306-5
Yan, L., and Liu, Y. (2019). Insights into the mechanism and enantioselectivity in the biosynthesis of ergot alkaloid cycloclavine catalyzed by Aj_EasH from Aspergillus japonicus. Inorg. Chem. 58, 13771–13781. doi: 10.1021/acs.inorgchem.9b01168
Yang, B., He, S., Liu, Y., Liu, B., Ju, Y., Kang, D., et al. (2020). Transcriptomics integrated with metabolomics reveals the effect of regulated deficit irrigation on anthocyanin biosynthesis in cabernet sauvignon grape berries. Food Chem. 314:126170. doi: 10.1016/j.foodchem.2020.126170
Youssef, K., Roberto, S. R., Chiarotti, F., Koyama, R., Hussain, I., and de Souza, R. T. (2015). Control of Botrytis mold of the new seedless grape ‘BRS Vitoria’ during cold storage. Sci. Hortic. 193, 316–321. doi: 10.1016/j.scienta.2015.07.026
Zarraonaindia, I., Owens, S. M., Weisenhorn, P., West, K., Hampton-Marcell, J., Lax, S., et al. (2015). The soil microbiome influences grapevine-associated microbiota. MBio 6, e02527–e02614. doi: 10.1128/mBio.02527-14
Zetsche, B., Heidenreich, M., Mohanraju, P., Fedorova, I., Kneppers, J., DeGennaro, E. M., et al. (2017). Multiplex gene editing by CRISPR-Cpf1 using a single crRNA array. Nat. Biotechnol. 35, 31–34. doi: 10.1038/nbt.3737
Zhang, J., Balkovic, J., Azevedo, L. B., Skalsky, R., Bouwman, A. F., Xu, G., et al. (2018). Analyzing and modelling the effect of long-term fertilizer management on crop yield and soil organic carbon in China. Sci. Total Environ. 627, 361–372. doi: 10.1016/j.scitotenv.2018.01.090
Zhang, J., Li, B., Zhang, J., Christie, P., and Li, X. (2020). Organic fertilizer application and mg fertilizer promote banana yield and quality in an Udic Ferralsol. PLoS One 15:e0230593. doi: 10.1371/journal.pone.0230593
Zhang, Q., Shamsi, I. H., Xu, D., Wang, G., Lin, X., Jilani, G., et al. (2012). Chemical fertilizer and organic manure inputs in soil exhibit a vice versa pattern of microbial community structure. Appl. Soil Ecol. 57, 1–8. doi: 10.1016/j.apsoil.2012.02.012
Zhao, Z., Chu, C., Zhou, D., Sha, Z., and Wu, S. (2019). Soil nutrient status and the relation with planting area, planting age and grape varieties in urban vineyards in Shanghai. Heliyon 5:e02362. doi: 10.1016/j.heliyon.2019.e02362
Zhao, F., Jiang, Y., He, X., Liu, H., and Yu, K. (2020). Increasing organic fertilizer and decreasing drip chemical fertilizer for two consecutive years improved the fruit quality of ‘summer black’ grapes in arid areas. HortScience 55, 196–203. doi: 10.21273/HORTSCI14488-19
Keywords: organic fertilizer, chemical fertilizer, fungal diversity, beneficial fungi, grape
Citation: Wu L, Li Z, Zhao F, Zhao B, Phillip FO, Feng J, Liu H and Yu K (2021) Increased Organic Fertilizer and Reduced Chemical Fertilizer Increased Fungal Diversity and the Abundance of Beneficial Fungi on the Grape Berry Surface in Arid Areas. Front. Microbiol. 12:628503. doi: 10.3389/fmicb.2021.628503
Edited by:
Jia Liu, Chongqing University of Arts and Sciences, ChinaReviewed by:
Asha Janadaree Dissanayake, University of Electronic Science and Technology of China, ChinaYanfang Wang, Shandong Agricultural University, China
Copyright © 2021 Wu, Li, Zhao, Zhao, Phillip, Feng, Liu and Yu. This is an open-access article distributed under the terms of the Creative Commons Attribution License (CC BY). The use, distribution or reproduction in other forums is permitted, provided the original author(s) and the copyright owner(s) are credited and that the original publication in this journal is cited, in accordance with accepted academic practice. No use, distribution or reproduction is permitted which does not comply with these terms.
*Correspondence: Kun Yu, yukun410@163.com
†These authors have contributed equally to this work