- 1State Key Laboratory of Plateau Ecology and Agriculture, Qinghai University, Xining, China
- 2College of Agriculture and Animal Husbandry, Qinghai University, Xining, China
- 3National Research Center for Protozoan Diseases, Obihiro University of Agriculture and Veterinary Medicine, Obihiro, Japan
- 4The Collaboration Unit for Field Epidemiology of State Key Laboratory for Infectious Disease Prevention and Control, Jiangxi Provincial key Laboratory of Animal-origin and Vector-borne Diseases, Nanchang Center for Disease Control and Prevention, Nanchang, China
- 5Department of Internal Medicine and Infectious Diseases, Faculty of Veterinary Medicine, Mansoura University, Mansoura, Egypt
- 6Transboundary Animal Diseases Research Center, Joint Faculty of Veterinary Medicine, Kagoshima University, Kagoshima, Japan
Toxoplasmosis is a zoonotic parasitic disease caused by the obligate intracellular protozoa Toxoplasma gondii, which threatens a range of warm-blooded mammals including humans. To date, it remains a challenge to find safe and effective drug treatment or vaccine against toxoplasmosis. In this study, our results found that the development of a mutant strain based on gene disruption of dense granule protein 9 (gra9) in type II PLK strain decreased parasite replication in vivo, severely attenuated virulence in mice, and significantly reduced the formation of cysts in animals. Hence, we developed an immunization scheme to evaluate the protective immunity of the attenuated strain of Δgra9 in type II PLK parasite as a live attenuated vaccine against toxoplasmosis in the mouse model. Δgra9 vaccination-induced full immune responses characterized by significantly high levels of pro-inflammatory cytokine interferon gamma (IFN-γ) and interleukin-12 (IL-12), maintained the high T. gondii-specific immunoglobulin G (IgG) level, and mixed high IgG1/IgG2a levels. Their levels provided the complete protective immunity which is a combination of cellular and humoral immunity in mouse models against further infections of lethal doses of type I RH, type II PLK wild-type tachyzoites, or type II PLK cysts. Results showed that Δgra9 vaccination proved its immunogenicity and potency conferring 100% protection against acute and chronic T. gondii challenges. Together, Δgra9 vaccination provided safe and efficient immune protection against challenging parasites, suggesting that PLK:Δgra9 is a potentially promising live attenuated vaccine candidate.
Introduction
Toxoplasmosis is a zoonotic parasitic disease caused by the obligate intracellular protozoan Toxoplasma gondii (Montoya and Liesenfeld, 2004; Saadatnia and Golkar, 2012). T. gondii has the ability to infect all nucleated cells, and thus, has a broad host range of warm-blooded mammals, including humans (Loh et al., 2019). One-third of the global population is estimated to have T. gondii infection, most of which are asymptomatic in immunocompetent people, but causes severe complications in immunocompromised individuals and pregnant women (Tenter et al., 2000; Innes, 2010; Robert-Gangneux and Darde, 2012; Le Roux et al., 2020). Additionally, reproductive problems, i.e., abortion and stillbirth, induced by T. gondii infection in livestock presents a grave challenge to the animal industry (Robert-Gangneux and Darde, 2012; Wang et al., 2019). The ensuing public health problems and agricultural economic losses necessitate the search for and development of safe and effective drug treatments and vaccines against toxoplasmosis in humans and animals.
Despite unsparing research efforts in recent decades, treatment and vaccine options against toxoplasmosis are still limited, owing to the unique characteristics of T. gondii. For instance, Toxoplasma infection has multiple routes of transmission in humans or animals. One route is ingestion of raw or undercooked meat containing tissue cysts with bradyzoites which transmits the parasite to humans. Although a combination of pyrimethamine and sulfadiazine or other compounds has been used to treat active toxoplasmosis in animals or humans (Alday and Doggett, 2017; Dunay et al., 2018), there is no significant therapeutic efficiency on the bradyzoite residing within tissue cysts. Furthermore, T. gondii develops complex population structures, in which North America and Europe strains are classified into three major clonal lineages, type I, II, and III (Howe and Sibley, 1995; Loh et al., 2019). The composition of these complex strains will inevitably bring new challenges to the control of toxoplasmosis because of the different proliferative ability and degree of pathogenicity in mouse models. Therefore, due to the current unsatisfactory status in drug treatment of toxoplasmosis, such as the inability of eliminating tissue cysts, the development of a vaccine to control Toxoplasma infections caused by different strains and contracted through multiple routes has been a priority.
Several studies on T. gondii vaccines have been done and reported in animal models. Although compared with nucleic acid vaccines (Li et al., 2015; Zhang et al., 2015; Lu et al., 2017) and recombinant protein vaccines (El Bissati et al., 2014; Tanaka et al., 2014; Wang et al., 2014a,b), the higher protection against acute or chronic T. gondii infection provided by live attenuated vaccines (Fox and Bzik, 2015; Lagal et al., 2015; Abdelbaset et al., 2017; Wang et al., 2017, 2018; Xia et al., 2018b; Yang et al., 2019; Liang et al., 2020) was highlighted both in short- and long-term vaccination using different strains in the mouse model. However, the safeness of these mutants still needs to be tested in animal models. With the advent of the genomic era, the widespread application of the CRISPR/Cas9 system has permitted precise and efficient genetic manipulations in T. gondii, such as gene editing and gene deletion resulting in attenuated strains which can be functionally selected (Shen et al., 2017). Advantages of this system facilitate the development of a live attenuated vaccine with reduced virulence but retaining its ability for limited replication in order to induce an immune response, which is considered as the ideal vaccine for resisting toxoplasmosis (Wang et al., 2019).
Dense granule proteins (GRAs) play major functions within the structural formation of the parasitophorous vacuole (PV) and the cyst wall of T. gondii (Guevara et al., 2019). One of the GRAs, the dense granule protein 9 (GRA9), has been characterized in T. gondii. Recent studies reported that GRA9 was investigated as one of the intravacuolar-network-associated GRAs during cyst development in vitro, and loss of gra9 in type II Prugniaud (Pru) strain induced severe defects in the development of chronic-stage cysts in vivo (Fox et al., 2019; Guevara et al., 2019). More so, our previous study revealed that disruption of gra9 gene in T. gondii type II PLK strain significantly reduced the growth of tachyzoites in vitro (Guo et al., 2019). In the current study, we confirmed that the development of a mutant strain based on gene disruption of gra9 in type II PLK strain decreased parasite replication in vivo, severely attenuated virulence in mice, and significantly reduced the formation of cysts in animals. These suggest that Δgra9 could be considered a vaccine candidate. Hence, we developed an immunization scheme to evaluate the protective immunity of the attenuated strain of Δgra9 in type II PLK parasite as a live attenuated vaccine against toxoplasmosis in the mouse model. Results showed that Δgra9 vaccination proved its immunogenicity and potency with 100% protection against acute and chronic T. gondii challenge infections.
Materials and Methods
Animals and Parasite Strains
The recommendations in the Guide for the Care and Use of Laboratory Animals of Obihiro University of Agriculture and Veterinary Medicine, Japan were strictly followed. The protocol of this study was approved by the Committee on the Ethics of Animal Experiments at the Obihiro University of Agriculture and Veterinary Medicine, Japan (permission numbers: 190246). Six-week-old female BALB/c mice were purchased from Clea Japan. All animals were housed in the animal facility of the National Research Center for Protozoan Diseases, Obihiro University of Agriculture and Veterinary Medicine, with adequate temperature (25 ± 2°C) and luminosity (12-h light and 12-h dark) under specific pathogen-free conditions, and free access to food and water. All animals were used at least one week after habituation.
All procedures of pathogen experiments were carried out according to the guidelines of Obihiro University of Agriculture and Veterinary Medicine (permission number: 2018728). In this study, T. gondii type I (RH strain with hypoxanthine-xanthine-guanine phosphoribosyl transferase deficiency), and type II (PLK, which is a clone of ME49 strain) strain (Kirkman et al., 2001) were used. Mutant PLK:Δgra9 attenuated strain with green fluorescent protein (GFP) was generated and cultured in our laboratory, which has been passed for more than 250 generations (Guo et al., 2019). All parasites were cultured in human foreskin fibroblast (HFF) cells maintained in Dulbecco’s modified Eagle’s medium (DMEM, Sigma), as previously described (Li et al., 2020).
Bradyzoite Differentiation in vitro of Δgra9 Strain
Toxoplasma gondii PLK wild-type (WT) or PLK:Δgra9 parasites were cultured in RPMI 1640 medium supplemented with 50 mM HEPES and 1% fetal bovine serum, pH 8.2, ambient CO2 for 4 days, as previously described (Xia et al., 2018a). The parasites (2 × 105 per well) forced to egress were allowed to invade cell monolayer (1 × 105 per well) for 3 h, then washed, and each culture was allowed to grow under bradyzoite-inducing conditions for 24, 48, 72, and 96 h, as described above. Subsequently, samples were fixed by 4% paraformaldehyde. After permeabilizing with 0.3% Triton X-100/phosphate-buffered saline (PBS), samples were incubated with rabbit anti-SAG1 polyclonal antibody diluted to 1:500. The Alexa Fluor 594-conjugated goat anti-rabbit IgG (Life Technologies, Inc., United States) and Dolichos biflorus Agglutinin, FITC Conjugate (DBA-FITC) (Vector Laboratories, United States) were used to detect primary antibody and cyst wall. Samples were examined using the All-in-one Fluorescence Microscope (BZ-900, Keyence, Japan). Conversion rates were counted in at least 100 vacuoles, wherein DBA-FITC (green) was used for cyst wall staining and anti-SAG1 (red) antibody was used for tachyzoite marker. All assays were conducted in triplicate and repeated at least three times.
Mutant Δgra9 Parasite Infection Tests in Mice
To determine the virulence of PLK:Δgra9 in animals, six BALB/c mice were injected with a lethal dose (freshly egressed tachyzoites, 1 × 105 per mouse) by intraperitoneal injection (i.p.). Daily observations of body weight and clinical signs were noted. Clinical scores ranged from 0 to 10, denoting presence of no signs or all signs, respectively. Evaluated clinical signs included hunching, piloerection, worm-seeking, behavior, ptosis, sunken eyes, ataxia, the latency of movement, deficient evacuation and touch reflexes, and lying on belly (Leesombun et al., 2016). Surviving mice were monitored for 30 days and blood was drawn at day 30 to confirm infection by an ELISA. Tissues were collected to determine parasite burdens through an examination of TgB1 gene by quantitative PCR (qPCR). T. gondii PLK strain was used as control.
To test the cyst formation in animals, 103 tachyzoites of PLK:Δgra9 was used to infect four 7-week-old female BALB/c mice by i.p. Mice were monitored for 35 days, and sera from the blood samples were tested to confirm infection by ELISA. Fresh brain cysts were isolated from each positive mouse brain homogenates, and the number of cysts was estimated by DBA-FITC staining, as previously described (Huskinson-Mark et al., 1991). T. gondii PLK WT strain was used as control.
To test the difference in immune response between PLK and PLK:Δgra9-infected mice, the different doses of parasites including 103, 104, or 105 were used to infect mice and clinical signs or T. gondii-specific IgG levels were noted. Moreover, sera of 103 T. gondii PLK or PLK:Δgra9-injected mice as above, were collected at day 7 or 30 post-infection to determine T. gondii-specific IgG levels using 0.5 μg/ml soluble PLK tachyzoite antigens coated by ELISA assay, and cytokine productions such as interleukin 12 (IL-12), interleukin 10 (IL-10), and interferon-gamma (IFN-γ) were tested using ELISA kits (Thermo Fisher Scientific, United States) according to the manufacturer’s recommendations. Furthermore, the difference in cytokine productions by splenocytes after T. gondii antigen stimulation was determined in infected mice with PLK or PLK:Δgra9 parasite at day 35 post-infection, as follows.
Vaccination of Mice and Immunogenicity Measurements
Mice were either vaccinated once with 103 freshly harvested PLK:Δgra9 tachyzoites or mock-vaccinated in a total of 200 μl PBS i.p. 30 and 70 days post-infection (dpi), then, sera were collected to test total T. gondii-specific IgG and IgG subclasses (IgG1 and IgG2a) levels. Briefly, the 96-well ELISA plates were coated with 0.5 μg/ml soluble tachyzoite antigens of PLK parasites diluted in coating buffer (0.05 M Carbonate-Bicarbonate, pH 9.6) and incubated at 4°C overnight. The ELISA plates were washed by PBS-T (0.05% Tween-20) three times, and then blocked with 3% BSA, then washed once. Collected sera were diluted by 1:50 and incubated for 1 h at 37°C. The plates were washed with PBS-T six times, the HRP conjugated goat anti-mouse IgG, IgG1 and IgG2a secondary antibodies were added and incubated for another 1 h at 37°C. After washing six times, ABTS (2, 2′-Azinobis [3-ethylbenzothiazoline-6-sulfonic acid]-diammonium salt) substrate was used to develop the reaction and measure the results at OD 415 nm. All samples were analyzed three times. Meanwhile, cytokine productions IL-12, IL-10, and IFN-γ were determined using ELISA kits according to the manufacturer’s recommendations, as above.
Cytokine Productions of Splenocytes by T. gondii Antigens Stimulation After Vaccination
Vaccinated mouse spleens were isolated to test stimulating cytokine productions of splenocytes at 70 days post-vaccination (dpv), and unvaccinated mouse spleens were also collected to use as controls. In brief, the splenocytes were washed three times with RPMI 1640 (Sigma, United States), and hemolyzed in a lysing buffer (0.83% NH4Cl and 0.01 M Tris-HCl, pH 7.2) for 5 min, then washed with RPMI 1640. The viability of the splenocytes was determined by trypan blue staining. A total of 3 × 105 viable splenocytes each well of 96-well cell culture plates were plated and cultured in RPMI 1640 supplemented with 20% FBS maintained 24 h. The final concentration of 50 μg/ml of T. gondii soluble antigens (TSA) of PLK parasites were used to stimulate cytokine productions for 3 days. Then supernatants from each well were harvested for cytokine level measurements, as above. For negative and positive controls, the same number of splenocytes was also plated into 96-well cell culture plates at the same time and stimulated with RPMI 1640 with 20% FBS only or 5 μg/ml concanavalin A (Sigma, United States) for 3 days, respectively. Each spleen-harvested splenocytes were plated in at least three wells for TSA, negative and positive control, and each supernatant sample was tested three times.
Protective Immunity Against T. gondii Challenges
BALB/c mice were vaccinated with 103 tachyzoites of PLK:Δgra9 through i.p. At 70 dpv, mice were challenged with 103 type I RH or 105 type II PLK tachyzoites by i.p., or 50 PLK cysts by oral administration (six mice for each group). Unvaccinated mice with same ages and numbers were used as control and infected with the same doses and routes. All challenged mice were monitored for another 30 days for tachyzoite-challenge or 35 days for cyst-challenge infections to record daily body weights, clinical signs, and survival rates in detail. Meanwhile, at 7 days tachyzoite post-challenges or 14 days cyst post-challenges, peritoneal fluid and serum samples of all experimental mice were collected to test cytokine productions. Parasite burdens were examined in peritoneal fluids during acute challenges at day 7 post-challenges. Furthermore, for chronic infection, the number of cysts in survival mouse brains was detected as above at 35 days cyst post-challenges.
Passive Immunization Test of Δgra9-Vaccinated Sera
BALB/c mice were infected with 105 type II PLK tachyzoites through i.p. At the day 0 and 3 post-infection, the 100 μl sera from naïve mice or the day 70 after PLK:Δgra9-vaccinated per mouse were administered into WT parasite infected mice by intraperitonea injection, which includes four mice for naïve sera as negative control, and five mice for PLK:Δgra9-vaccinated sera as test group. Survival rates were recorded. Parasite burdens were examined in peritoneal fluids at day 5 or 7 post-infection by qPCR as follows to evaluate parasite growth under passive immunization.
DNA Isolation and Quantitative PCR (qPCR) Detection of Parasite Burdens in Infected Mice
DNA was extracted from the tissues or peritoneal fluid of parasite-challenged mice by DNeasy Blood & Tissue Kit (Qiagen, Germany), according to the manufacturer’s instructions. The 50 ng DNA was then amplified with primers specific to the T. gondii B1 gene (forward primer 5′-AAC GGG CGA GTA GCA CCT GAG GAG-3′ and reverse primer 5′-TGG GTC TAC GTC GAT GGC ATG ACA AC-3′) by qPCR, as previously described (Li et al., 2020). A standard curve was constructed using 10-fold serial dilutions of T. gondii DNA extracted from 105 parasites; thus, the curve ranged from 0.01 to 10,000 parasites. The parasite number was calculated from the standard curve.
Statistical Analysis
To graph and analyze the data, GraphPad Prism 7 software (GraphPad Software Inc., United States) was used. In this study, statistical analyses were performed using unpaired Student’s t-test, Tukey’s Multiple Comparison Test, and one-way ANOVA plus Tukey-Kramer post hoc analysis. Data represent the mean ± Standard Error of Mean. Survival curves were generated using the Kaplan–Meier method and statistical comparisons were made by the log-rank method. A P value < 0.05 was considered statistically significant.
Results
Δgra9 Generation in Type II PLK Strain Markedly Reduces Cyst Formation in vitro and in vivo
Our previous study revealed that (Guo et al., 2019). To see whether a single mutant of Δgra9 affects bradyzoite differentiation from tachyzoite, WT or Δgra9 (with GFP) tachyzoites were cultured under bradyzoite-inducing conditions to count the conversion rates. Both strains formed cysts, as cysts were stained by DBA-FITC (green) (Figure 1A). However, compared with the cyst differentiation rate of WT parasites, Δgra9 reduced by 74.19, 94.04, 63.07, and 41.52% at 24, 48, 72, and 96 h post-induction, respectively (Figure 1B). In vivo, with the dose of 103 tachyzoites infections, no mortalities were recorded until day 35 in mice infected with parasites either strains (data not shown), while as shown in Figure 1C, the cyst number in the brain of mice with Δgra9 infection significantly reduced by 73.68% compared with that of the PLK. Collectively, the loss of gra9 in type II PLK strain, did not abolish, but markedly reduced cyst formation in vitro and in vivo.
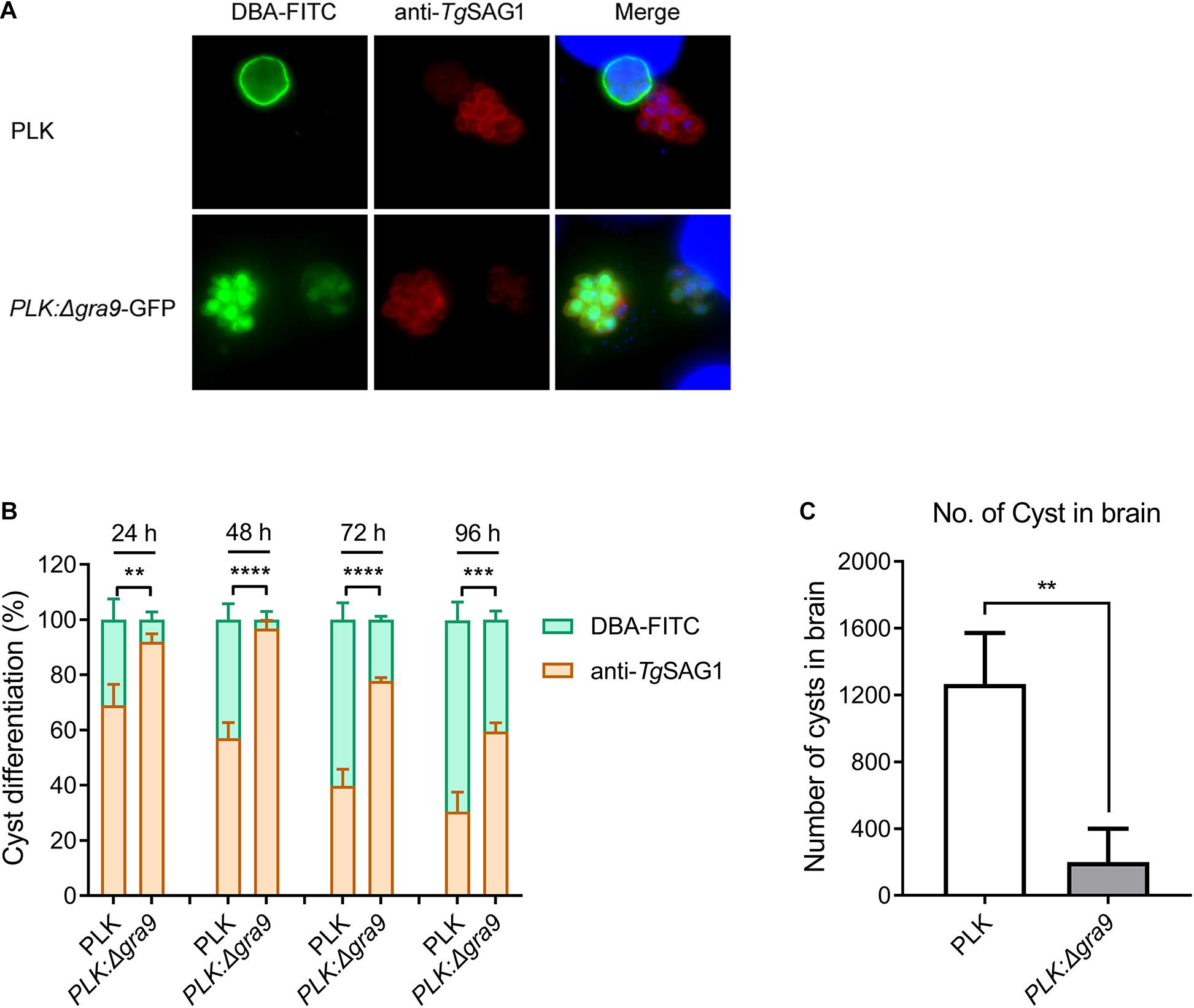
Figure 1. Disruption of gra9 in type II PLK strain reduces cyst formation in vitro and in vivo. (A) The bradyzoite differentiation from wild-type or Δgra9 with GFP tachyzoites under bradyzoite-inducing conditions in vitro. DBA-FITC (green) was used for cyst wall staining and anti-SAG1 (red) antibody was used for tachyzoite marker. Both strains formed cysts, as cysts were stained by DBA-FITC. The blue fluorescence showed the nuclear DNA staining by DAPI. (B) Conversion rates in vitro. Bradyzoite differentiation rates were counted in at least 100 vacuoles at 24, 48, 72, and 96 post-induced hours. The data are presented as the mean ± SEM of at least three independent experiments (**P < 0.01; ***P < 0.001; ****P < 0.0001; one-way ANOVA plus Dunnett’s multiple comparisons test). (C) The cyst number in the mouse brains of Δgra9 infection. The dose of 103 WT or Δgra9 tachyzoites were injected each mouse and were monitored for 35 days, and then the number of cysts in survival mouse brains were determined (n = 4; **P < 0.01; Student’s t-test).
Δgra9 Generation in Type II PLK Strain Severely Attenuates Virulence in Mice
To evaluate the virulence of Δgra9, a lethal dose of 105 parasites was infected into mice by i.p., with body weight and clinical signs monitored for 30 days. The parental PLK strain caused 100% mortality (Figure 2A) with severe body weight losses (Figure 2B) and clinical symptoms (Figure 2C), whereas infection with the mutant strain surprisingly proved to be unfatal to mice (Figure 2A), indicating that Δgra9 generation in type II PLK strain severely attenuated virulence in mice.
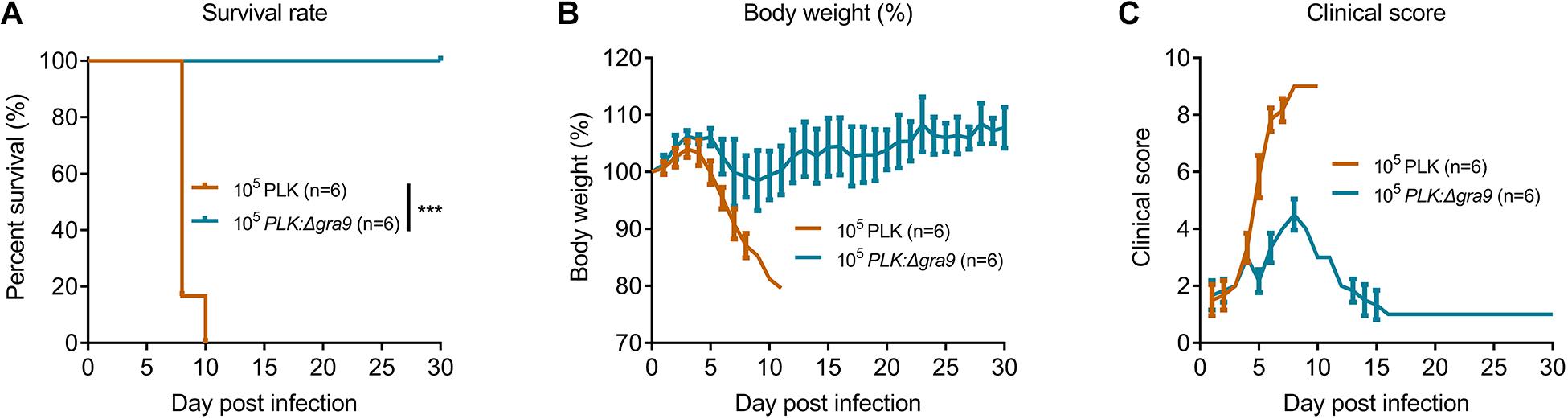
Figure 2. Δgra9 generation in PLK strain severely attenuates the virulence in mice. A lethal dose of 105 parasites of wild-type or Δgra9 were infected into female BALB/c mice by intraperitoneal injection (n = 6), and mice were monitored for 30 days to note daily observations of body weight and clinical signs. (A) Survival rates (***P < 0.001; Log-rank (Mantel-Cox) test). (B) Body weight%. (C) Clinical scores. The scores varied from 0 (no signs) to 10 (all signs).
Δgra9 Infection Shows Different Cytokine Productions and Relatively High T. gondii-Specific IgG Levels Compared With Wild-Type Infection in Mice
Lethal type II strain infections are associated with extremely elevated pro-inflammatory cytokine levels in the serum, including IFN-γ and IL-12 (Mordue et al., 2001). In order to elucidate the differences in immune response caused by infection with Δgra9 or WT strains, mice were infected with a non-lethal dose of 103 parasites. Sera were collected at 7 or 30 dpi to measure the changes in immune response. Levels of pro-inflammatory cytokines IFN-γ and IL-12, as well as anti-inflammatory cytokine IL-10, were significantly elevated at 7 and 30 dpi in both Δgra9 and WT infections compared with control (Supplementary Figures S1A–C). However, Δgra9-infected mice showed 39.17–54.05% lower serum cytokine levels compare with WT infections at 7 dpi, although not statistically significant (Supplementary Figures S1A–C). This suggests that loss of gra9 in PLK parasites led to milder cytokine productions resulting in complete mouse survival. Conversely, similarly high T. gondii-specific IgG levels were induced at 30 dpi after either PLK or mutant parasite infection (Supplementary Figure S1D). At the cellular level, cytokine productions by splenocytes after T. gondii antigen stimulation was determined at 35 dpi, found that high levels of cytokine IFN-γ and IL-10 in Δgra9-infected splenocytes were induced quickly, which were slightly higher than the WT (Supplementary Figures S1E,F). Together, these results suggest that Δgra9 infection induces balanced cytokine productions and relatively high T. gondii-specific IgG levels compared with WT infection in mice, which relates to the severely attenuated virulence of Δgra9 strain.
Δgra9 Vaccination Induces Full T. gondii-Specific Immune Response
The above findings reveal the fact that Δgra9 strain attenuated acute virulence and affected the formation of cysts. To evaluate the potential as a good vaccine of this mutant strain and test the immunogenicity derived from Δgra9 vaccination, we designed an immunization scheme within 105 days using mouse models (Figure 3A). At the outset, the chosen immune dose was 103 Δgra9 tachyzoites each mouse by i.p. Results of our preliminary experiments showed that the lowly clinical score was observed in 103 Δgra9-infected mice, whereas 104 and 105 Δgra9-infected or 103, 104, and 105 PLK-infected mice showed severe clinical signs (Supplementary Figure S2). At 30 dpi, induced T. gondii-specific IgG in sera of 103, 104, and 105 Δgra9- or 103 and 104 PLK-infected mice were of similarly high levels (Supplementary Figure S3). These represent similar immunogenicity and suggest that Δgra9 vaccination of 103 tachyzoites was a safe and effective immune dose. After vaccination of 103 Δgra9 tachyzoites, the immunogenicity of Δgra9 was tested through detection of specific anti-T. gondii IgG and IgG subclasses (IgG1 and IgG2a) levels in vaccinated mouse sera at 30 and 70 dpv. Unvaccinated (naïve) mice were used as control. The ELISA results showed that Δgra9 vaccination induced a significantly higher T. gondii-specific IgG level at 30 dpv, and maintained a similarly high level at 70 dpv (Figure 3B). Nest study was to test the levels of IgG subclasses, the results showed that compared to unvaccinated mice, the level of IgG2a in vaccinated mice was significantly higher at 30 and 70 dpv (Figure 3B). To test IgG1 level in mice, although the level was lower at 30 dpv compared with 70 dpv, it was also increased to significantly higher in the vaccinated mice both 30 and 70 dpv than unvaccinated mice (Figure 3B). These suggest that Δgra9 vaccination elicits a mixed Th1/Th2 immune response both 30 and 70 dpv. Opposite to the stable IgG levels, cytokine levels changed over time. Relatively higher levels of pro-inflammatory IFN-γ and IL-12, or anti-inflammatory IL-10 were recorded from vaccinated mice compared with unvaccinated mice at 30 dpv, while levels decreased at 70 dpv (Figures 3C–E). Collectively, these results reveal that Δgra9 vaccination provided a benign humoral and cellular immune response in mice and proved to induce effective immunogenicity.
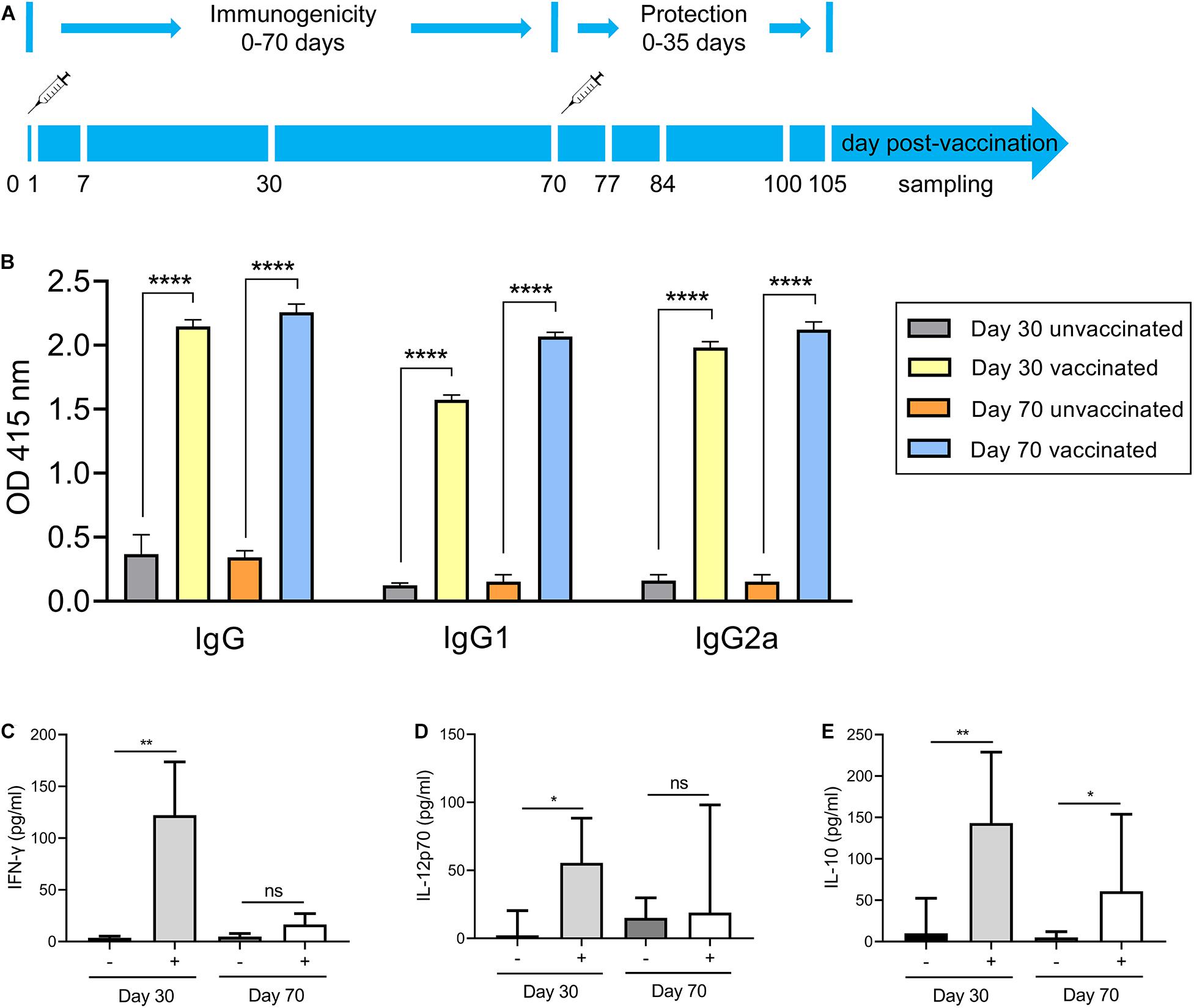
Figure 3. Immunization scheme and study design. (A) Immunization scheme. The immune dose of 103 Δgra9 tachyzoites each mouse was injected into female BALB/c mice (n = 6 each group) by intraperitoneal injection and that day was designated as 0 day post-vaccination. The immunogenicity of Δgra9 vaccination was tested via the detection of specific anti-T. gondii IgG levels and cytokine productions in sera at 30 and 70 dpv. At 70 dpv, vaccinated or unvaccinated mice were secondly challenged by acute or chronic T. gondii infection to assess the protection. Within the vaccination and challenge period of total 105 days, the daily body weights, clinical signs, and survival rates of all mice were recorded in detail, and peritoneal fluid or serum samples were collected to evaluate immune response at the limited sampling periods as shown in (A). (B) The specific anti-T. gondii IgG and IgG subclasses (IgG1 and IgG2a) levels in vaccinated mice at 30 or 70 dpv. Unvaccinated naïve mice with the same ages were used as control (****P < 0.0001; Student’s t-test). (C–E) Cytokine productions in sera at 30 and 70 dpv. The levels of IFN-γ (C), IL-12p70 (D), or IL-10 (E) were determined by ELISA kits. –, unvaccinated mice; +, vaccinated mice (*P < 0.05; **P < 0.01; ns, not significant; Student’s t-test).
To assess the immunological memory in Δgra9 vaccinated mice, splenocytes were harvested from vaccinated or unvaccinated mice at 70 dpv, and stimulated with total Toxoplasma soluble antigen (TSA) prepared from fresh wild-type (PLK) tachyzoites. As shown in Figures 4A,B, the significantly high levels of pro-inflammatory cytokine IFN-γ, as well as anti-inflammatory cytokine IL-10, were stimulated by TSA compared with no stimulation or no vaccination. Interestingly, although the significantly decreased levels of stimulated IFN-γ (16,787.5 pg/ml) and IL-10 (5,347.5 pg/ml) at 70 dpv were observed compared with their levels of IFN-γ (207,667.7 pg/ml) and IL-10 (10,210.4 pg/ml) at 30 dpv in the Δgra9 vaccinated splenocytes (Supplementary Figures S1E,F), there were obviously high levels at both time points. These suggest that Δgra9 vaccination could activate the ability of the immune cells to quickly and specifically recognize the Toxoplasma antigen to produce corresponding immune cytokines in short-term and long-term immunization.
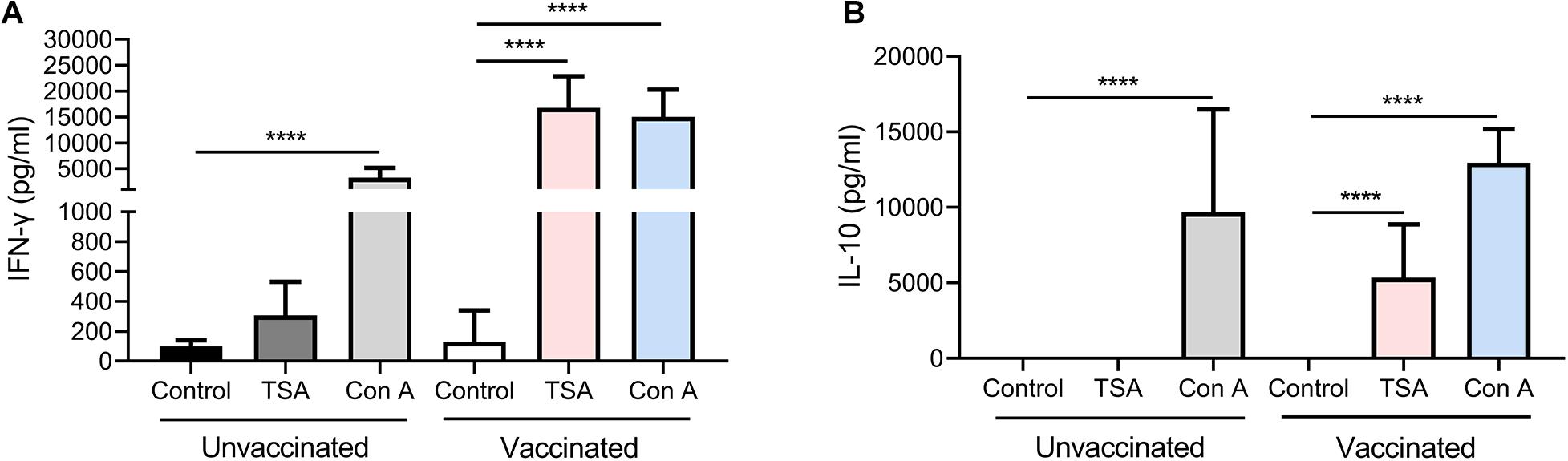
Figure 4. Δgra9 vaccination activates the ability of the splenocytes to rapidly and specifically recognize the Toxoplasma antigen to induce high-level cytokines, compared with unvaccinated. Immunological memory of mice in Δgra9 vaccination was evaluated at 70 dpv via stimulated splenocytes by total Toxoplasma soluble antigen resulting in the production of cytokines IFN-γ (A) or IL-10 (B). RPMI 1640 with 20% FBS only or 5 μg/ml concanavalin A were used as negative or positive controls, respectively. The data are presented as the mean ± SEM of at least three repeats each sample (****P < 0.0001; Student’s t-test).
Δgra9 Vaccination Confers Potent for Protection Against Acute and Chronic T. gondii Challenges
Based on the findings that Δgra9 has strong immunogenicity and immunological memory, we then preformed the second challenges with 103 type I (RH) or 105 type II (PLK) tachyzoites by i.p. or 50 cysts (PLK) by oral administration to vaccinated mice at 70 dpv. All challenged mice were monitored for another 30 days for tachyzoite or 35 days for cyst infections, and recorded daily body weights, clinical signs, and survival rates. For both RH (Figure 5A) and PLK (Figure 5B) tachyzoite challenges, Δgra9 vaccination elicited strong protection following 100% survival rates of mice and no obvious signs or body weight changes were observed during the 30-day challenge period (Supplementary Figure S4). However, for unvaccinated mice challenged with the lethal dose of RH or PLK tachyzoites, the clinical signs and body weight changes were initially observed at day 4 of RH or day 2 of PLK infections until the signs developed to most severe at 6-8 days (Supplementary Figure S4), resulting in 100% mortality rates for unvaccinated mice within 8 days post-challenges (Figures 5A,B). As expected, all Δgra9-vaccinated mice survived when infected with 50 cysts (PLK strain), whereas only 50% of unvaccinated mice survived (Figure 5C). While the 50 cysts-challenges to vaccinated or unvaccinated mice led to decreased body weights (%) in both groups during the whole period of 35 days, clinical signs were observed only in unvaccinated mice starting from day 8 post-challenge infection (Supplementary Figure S4). Altogether, Δgra9 vaccination confers strong protective immunity against acute and chronic toxoplasmosis.
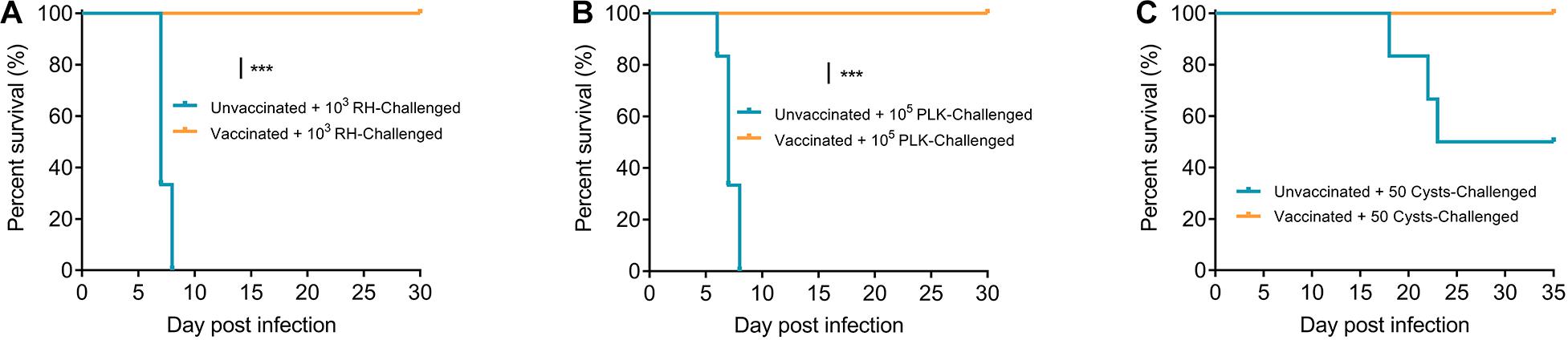
Figure 5. Δgra9 vaccination protects mice against T. gondii infections. Vaccinated or unvaccinated mice were challenged with 103 type I RH (A) or 105 type II PLK (B) tachyzoites by intraperitoneal injection or 50 PLK cysts (C) by oral administration at 70 dpv, and monitored for another 30 days for tachyzoite or 35 days for cyst infections to note survival rates (***P < 0.001; Log-rank (Mantel-Cox) test).
Δgra9 Vaccination Rapidly Clears Challenging Parasites and Blocks Cyst Formation in New Challenges
To further understand how Δgra9 vaccination provided strong protection in mice suffering both acute and chronic T. gondii challenges, peritoneal fluids and sera in challenged mice were collected at 7 days tachyzoite post-challenges or 14 days cyst post-challenges (time-points when we observed the most serious signs in unvaccinated mice) to determine cytokine productions, as well as parasite burdens in peritoneal fluids. In naïve mice, RH infections resulted in rapid proliferation with 87,305 parasites in 50 ng DNA, whereas PLK infections caused higher parasite burdens of 2.74 × 107 at 7 days post-challenge infection (Figure 6A). However, we could not detect any parasites using a qPCR test in both RH- and PLK- challenged Δgra9-vaccinated mice, suggesting that Δgra9 vaccination promoted the activity to rapidly clear infecting parasites. Meanwhile, for chronic toxoplasmosis, parasites were not detected in any mice peritoneal fluid at 14 dpi (data not shown), but markedly reduced number of cysts in vaccinated survival mouse brain was noted by day 35 compared with unvaccinated mice (Figure 6B), which are similar to that level of cyst formation of vaccinated but no cyst challenged mice (Figure 1C). This suggests that Δgra9 vaccination blocks cyst formation in new challenged cysts.
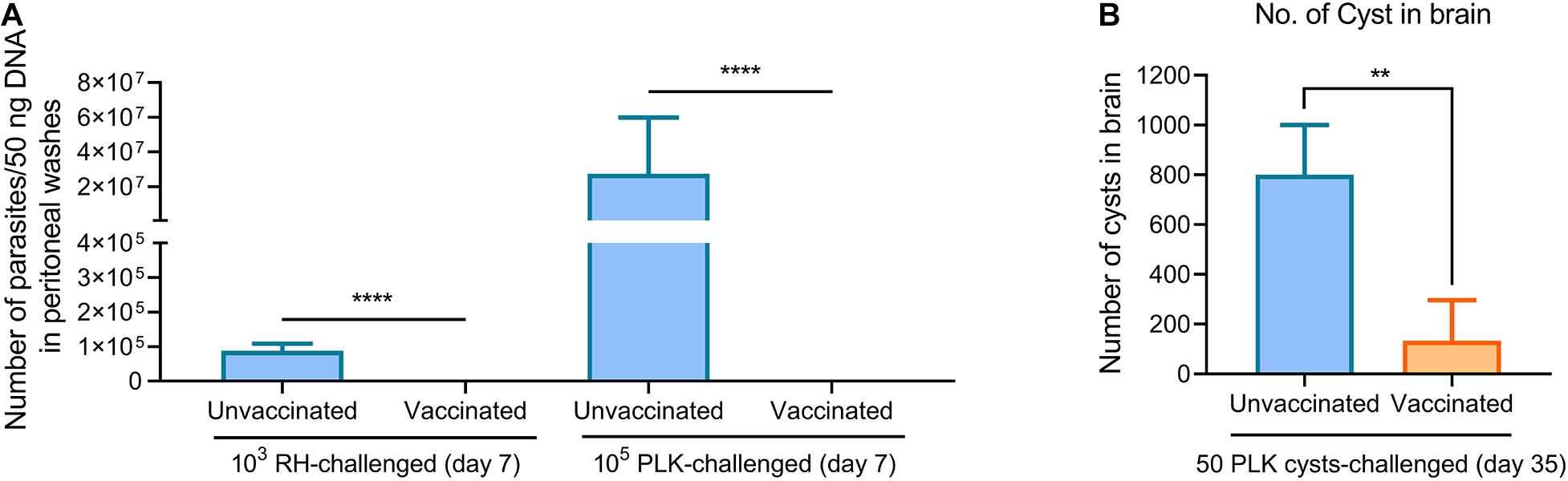
Figure 6. Δgra9 vaccination rapidly clears challenging parasites and blocks cyst formation in new challenges. (A) Parasite burdens in peritoneal fluids of RH or PLK tachyzoites-challenged vaccinated mice. A quantitative PCR of the TgB1 gene using 50 ng extracted DNA from peritoneal fluids was used to confirm parasite proliferation in vaccinated mice at 7 days post-challenge infection, compared with unvaccinated challenged mice (****P < 0.0001; Student’s t-test). (B) No. of cyst in brain in PLK cysts-challenged vaccinated mice. At day 35 post-challenges, the brains were isolated from survival mice of 50 cysts challenges to estimate cyst numbers by DBA-FITC staining (**P < 0.01; Student’s t-test).
Subsequently, the immune responses were also tested during the limited time-points, levels of cytokines (IFN-γ, IL-12, and IL-10) in both peritoneal fluid and serum were substantially induced in unvaccinated mice relative to the extremely low levels in Δgra9-vaccinated mice, especially the IFN-γ levels (Figure 7A). More importantly, all mice remained with high levels of T. gondii-specific IgG (Figure 7B). Taken together, Δgra9 vaccination provided efficient and safe immune protection to kill challenging parasites, resulting in host survival.
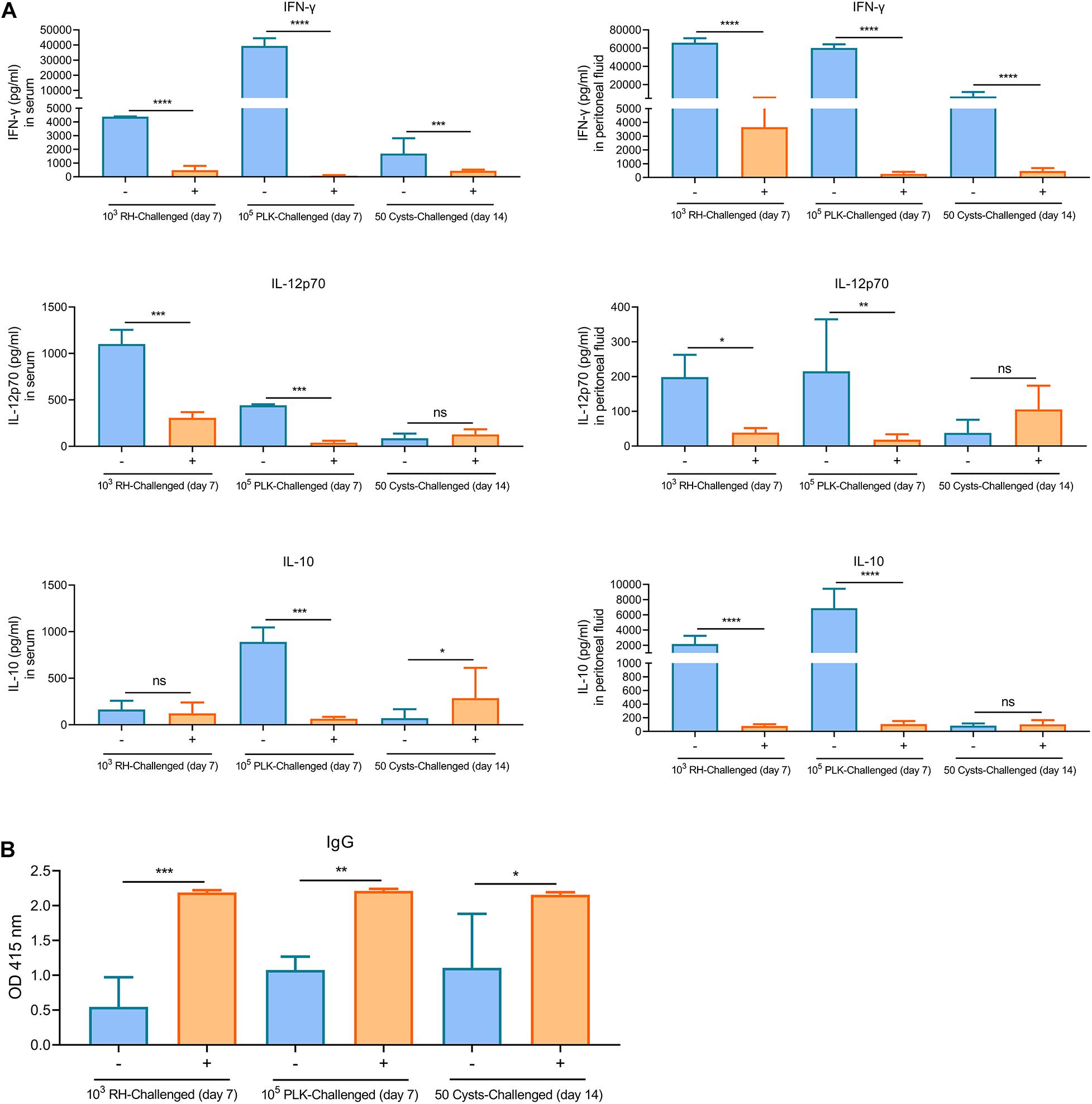
Figure 7. Δgra9 vaccination provides safe and effective immune protection. The peritoneal fluids and sera in challenged mice were collected at 7 days tachyzoite post-challenges or 14 days cyst post-challenges to determine cytokine productions and T. gondii specific IgG, compared with unvaccinated but secondly challenged mouse samples. (A) IFN-γ, IL-12p70, or IL-10 levels in serum or peritoneal fluid samples. –, unvaccinated; +, vaccinated (*P < 0.05; **P < 0.01; ***P < 0.001; ****P < 0.0001; ns, not significant; Student’s t-test). (B) The levels of T. gondii specific IgG. IgG levels in vaccinated challenged or unvaccinated challenged mice at 7 days tachyzoite post-challenges or 14 days cyst post-challenges were determined by an ELISA test. –, unvaccinated, +, vaccinated (*P < 0.05; **P < 0.01; ***P < 0.001; Student’s t-test).
A Special Protection Against T. gondii Infection Is Provided by the Sera of Δgra9-Vaccinated Mice
Δgra9 vaccination induced a significantly high T. gondii-specific IgG level with low cytokine levels at 70 dpv in mouse sera as shown above. In this study, the sera from PLK:Δgra9-vaccinated mice were administered into parasites infected mice with lethal dose, survival rates were recorded and parasite burdens were determined in peritoneal fluids at day 5 and 7 post-infection to evaluate parasite growth under passive immunization. The results showed that vaccinated sera gave 40% survival rates (n = 5, Figure 8A) and led to significantly lower parasite burdens in peritoneal fluids of WT parasites-infected mice both 5 and 7 dpi, compared with naïve sera (Figure 8B). As shown in Figure 8B, the numbers of parasite in control mice at 5 or 7 day post-infection were resulted in 5 or 4 times of mice injected with vaccinated sera, respectively. These suggest that the sera of Δgra9-vaccinated mice with high IgG and low cytokine levels are able to reduce parasite propagation in mice.
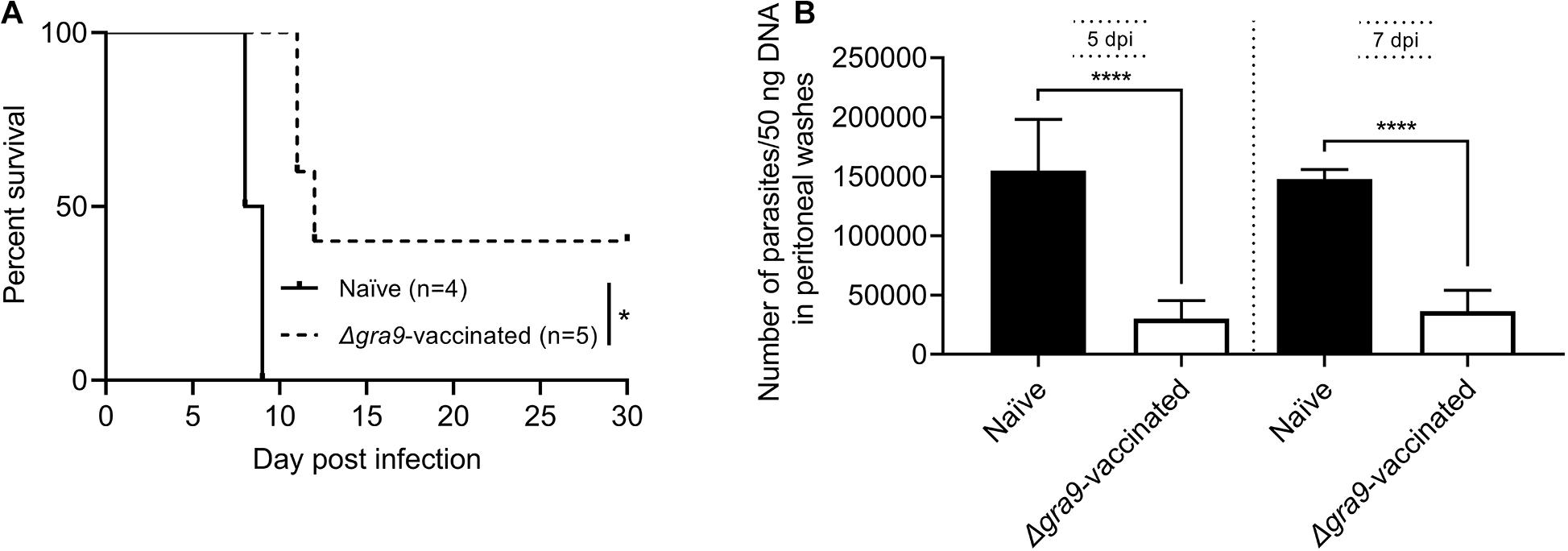
Figure 8. The sera from Δgra9-vaccinated mice are able to reduce parasite propagation in mice. The sera from PLK:Δgra9-vaccinated mice were administered into 105 PLK parasites infected mice at 0 and 3 day post-infection, survival rates were noted and parasite burdens were determined in peritoneal fluids at day 5 and 7 post-infection to evaluate parasite growth under passive immunization. The four mice for naïve sera as negative control, and five mice for PLK:Δgra9-vaccinated sera as test group. (A) Survival rates (*P < 0.05; Log-rank (Mantel-Cox) test). (B) Parasite burdens (****P < 0.0001; Student’s t-test).
Discussion
In recent years, active immunization is considered to be the ideal and long-term strategy to induce the host immune response against acute and chronic T. gondii infections (Loh et al., 2019). One means is to develop live attenuated vaccines, a whole parasite-based vaccine, which is the live strain with reduced replication and attenuated virulence but retaining the ability to induce an immune response against a variety of wild-type strain and multiple stages of parasite infections (Wang et al., 2017, 2018; Xia et al., 2018b; Yang et al., 2019; Liang et al., 2020). In this study, PLK:Δgra9 vaccination induced a high level of immune response and resisted the infections of tachyzoites from type I or type II strains and cysts from type II strain.
Our previous study reported that the generation of mutant Δgra9 strain in PLK parasites not only significantly reduced the growth of tachyzoites in vitro but also changed replication in mice resulting in severely attenuated virulence (Guo et al., 2019), which consistent with the current result of 100% survival rate in mice during challenge infection with 105 Δgra9 parasites which have been passed for more than 250 generations in culture cells. This suggests that the attenuated Toxoplasma strain did not revert back to virulent form in animals at least 250 cell passages under confirmed loss of gra9 gene. Importantly, GRAs have been investigated with playing the major structural roles within the PV and the cyst wall of T. gondii (Guevara et al., 2019). Current results demonstrate that loss of gra9 in type II strain (PLK), reduced the formation of cysts in vitro and in vivo, which corroborates gra9 protein as one of the intravacuolar-network-associated dense GRAs involved during cyst development (Fox et al., 2019; Guevara et al., 2019). To test the virulence of cysts, we isolated and injected 50 cysts of Δgra9 were into three mice which resulted in no mice deaths (data not shown), suggesting the possibility that the loss of gra9 in type II PLK strain might reduce the virulence of cysts, although there was limited little number of mouse model. Δgra9 strain’s inability to form cysts or significantly reduce cyst formation and the lack of virulence from the type II parasites are good indicators that the produced strain could be utilized as a candidate vaccine. On the other hand, compared with the severe immune response after wild-type parasite infection, Δgra9-infected mice produced moderate immune response which represents a balance between inducing pro-inflammatory and anti-inflammatory cytokines, denoting its safety as a live attenuated vaccine. Furthermore, it is worthy of attention that the chosen immune dose was 103 Δgra9 tachyzoites each mouse, since mice with 103 Δgra9 infection observed the high specific anti-T. gondii IgG levels and no significantly clinical symptoms compared with naïve mice, suggesting that the vaccination with 103 Δgra9 tachyzoites was effective and safe immune dose.
In the two phases of the entire experiment, the generation of immunogenicity and the second challenge of protection, we recorded in detail the changes in the levels of cytokines and antibodies in mice. Compared with unimmunized mice, the vaccinated mice showed significantly higher levels of the pro-inflammatory cytokines IFN-γ and IL-12 and the anti-inflammatory cytokine IL-10 at 30 dpv and dropped to low levels at 70 dpv. In the subsequent challenge infection, cytokine levels of vaccinated mice at 7 days post-challenge infection surprisingly remained at similar levels as before being challenged, suggesting the second challenges did not cause severe immune responses in vaccinated mice. Importantly, the specific anti-T. gondii IgG was maintained at a similarly high level throughout the process. While to test the levels of IgG subclasses, the results confirmed that both IgG1 and IgG2a in vaccinated mice was significantly higher levels at challenged time-point compared with unvaccinated mice. These suggest that Δgra9 vaccination elicits a mixed Th1/Th2 immune response at this time-point. On the other hand, the passive immunization test was observed that the sera from vaccinated mice could reduce parasite propagation. Hence, we consider that the Δgra9 vaccination-induced full protective immunity was a cellular immunity-based immune response combined with humoral immunity. At 70 dpv, Δgra9 vaccination induced a Th1-biased inflammatory response to produce IFN-γ and IL-12 which are two pro-inflammatory cytokines crucial for activation of cell-mediated immunity against T. gondii infection (Pifer and Yarovinsky, 2011; Hunter and Sibley, 2012; Yarovinsky, 2014). Meanwhile, the anti-inflammatory cytokine IL-10 was also highly produced suggesting that the balanced level of cytokines was maintained in Δgra9-vaccinated mice. This balance was also proved by the splenocytes stimulation test, which rapidly and specifically produced significantly high levels of pro-inflammatory cytokine IFN-γ and anti-inflammatory cytokine IL-10. When vaccinated mice are secondly challenged with wild-type parasites, the immune system rapidly recognizes and produces high levels of IFN-γ and other pro-inflammatory cytokines to activate cellular immune responses, and simultaneously, the high level of T. gondii-specific IgG antibodies hinders the parasite attachment and invasion to host cells and activates the classical complement pathway to clear secondary infections efficiently in cooperation (Sayles et al., 2000; Spellberg and Edwards, 2001; Pifer and Yarovinsky, 2011; Hunter and Sibley, 2012). These are consistent with our results that Δgra9 vaccination rapidly blocks challenging parasite tachyzoites and new cyst formation.
In the present mouse models, noteworthy is the lack of any obvious signs or body weight changes in vaccinated mice during challenge periods, signifying that infecting parasites are strongly inhibited from replication and killed quickly by the rapid immune response during tachyzoite challenges. This might also explain why the increased levels of cytokine productions were not observed at day 7 post-challenge. In contrast, we noted significantly high parasite burden and the high levels of pro-inflammatory or anti-inflammatory cytokines with severe clinical symptoms and significant weight loss resulting in 100% mortality in naïve mice challenge infected with a lethal dose of tachyzoites. On the other hand, the Δgra9-vaccination-induced full immune response was not only observed against type I RH and type II PLK wild-type tachyzoite acute infections but also against type II cyst challenge and reduction of cyst formation. Interestingly, IFN-γ was cytokine which had significantly different levels between vaccinated and unvaccinated mice at day 14 post-cyst-challenge, suggesting that IFN-γ is also central to the development of immunity against T. gondii cyst infection, consistent with Δgra9-vaccination-induced immune response for protection of cyst challenge.
Conclusion
In conclusion, the present study demonstrated that loss of gra9 in type II PLK strain dramatically attenuated virulence and significantly reduced the formation of cysts in animals. Δgra9 vaccination effectively elicited immune responses which conferred absolute protection from subsequent lethal type I RH, type II PLK wild-type tachyzoites or type II PLK cysts challenge infections in mice, suggesting that the mutant Δgra9 of T. gondii type II PLK strain is a potential live-attenuated vaccine candidate against acute and chronic toxoplasmosis. However, the current study is limited to the mouse model; thus, the effectiveness and safety should likewise be extensively investigated in animals of veterinary and economic importance, including but not limited to definitive hosts cats, susceptible sheep and pigs and other meat-producing animals. Future studies should assess its effectiveness against other Toxoplasma strains, particularly infections with local endemic strains.
Data Availability Statement
The raw data supporting the conclusions of this article will be made available by the authors, without undue reservation.
Ethics Statement
The animal study was reviewed and approved by the Committee on the Ethics of Animal Experiments at the Obihiro University of Agriculture and Veterinary Medicine, Japan (permission numbers: 190246).
Author Contributions
JL and XX conceived and designed the experiments. JL, EG, HG, ML, YL, and SJ performed the experiments. JL, IZ, YG, and WZ analyzed the data and wrote the manuscript. PA, MR, MT, BB, AR, and TM critically revised the manuscript. All authors read and approved the final version of the manuscript.
Funding
This study was supported by a Grant-in-Aid for Scientific Research (19K22354) from the Ministry of Education, Culture, Sports and Technology, Japan.
Conflict of Interest
The authors declare that the research was conducted in the absence of any commercial or financial relationships that could be construed as a potential conflict of interest.
Acknowledgments
We would like to thank all the people who were involved in making this project a success.
Supplementary Material
The Supplementary Material for this article can be found online at: https://www.frontiersin.org/articles/10.3389/fmicb.2021.619335/full#supplementary-material
Supplementary Figure 1 | Δgra9 infection induces mild cytokine productions and relatively high T. gondii-specific IgG levels in mice compared with Wild-type PLK infection. A non-lethal dose of 103 parasites was chosen to infect BALB/c mice, and the sera were collected at day 7 or 30 post-infection to measure immune response. Naïve mouse sera were used as controls. The levels of pro-inflammatory cytokines IFN-γ (A) and IL-12p70 (B) or anti-inflammatory cytokine IL-10 (C) were assessed by an ELISA test (n = 4; ∗P < 0.05; ∗∗P < 0.01; ∗∗∗P < 0.001; ****P < 0.0001, Control vs parental or Δgra9 at 7 dpi; #P < 0.05, control vs parental or Δgra9 at 30 dpi; one-way ANOVA plus Tukey-Kramer post hoc analysis). (D) The T. gondii-specific IgG levels were measured at day 7 or 30 post-infection compared with naïve mice (****P < 0.0001; Student’s t-test). (E,F) Cytokine productions by splenocytes after T. gondii antigen stimulation. At 35 dpi, the splenocytes were harvested from parental or Δgra9 infected mouse spleens, and 3 × 105 viable splenocytes were cultured in vitro and stimulated by T. gondii antigen to induce cytokine IFN-γ (E) or IL-10 (F) productions. For the negative and positive controls, the same number of splenocytes was cultured at same time and stimulated with RPMI 1640 with 20% FBS only or 5 μg/ml concanavalin A, respectively (****P < 0.0001; Student’s t-test).
Supplementary Figure 2 | Clinical symptoms of mice during parasite infection. The different dose of 103, 104, and 105Δ gra9- or 103, 104, and 105 PLK-parasites were injected into mice, and clinical signs of infected mice were noted within 30 days post-infection. The clinical scores varied from 0 (no signs) to 10 (all signs). No-infected mice were used as control.
Supplementary Figure 3 | T. gondii-specific IgG in sera from Toxoplasma infected-mice. The different dose of 103, 104, and 105 Δgra9- or 103 and 104 PLK-parasites were injected into mice, at 30 dpi, induced T. gondii-specific IgG in sera were detected by ELISA tests. No-infected sera and PBS only were used as control (****P < 0.0001; Student’s t-test).
Supplementary Figure 4 | Δgra9 vaccination protects mice against T. gondii infections. Vaccinated or unvaccinated mice were challenged with 103 type I RH or 105 type II tachyzoites by intraperitoneal injection or 50 PLK cysts by oral administration at 70 dpv, and monitored for another 30 days for tachyzoite or 35 days for cyst infections to note daily body weights (A–C) and clinical signs (D–F) in detail.
References
Abdelbaset, A. E., Fox, B. A., Karram, M. H., Abd Ellah, M. R., Bzik, D. J., and Igarashi, M. (2017). Lactate dehydrogenase in Toxoplasma gondii controls virulence, bradyzoite differentiation, and chronic infection. PLoS One 12:e0173745. doi: 10.1371/journal.pone.0173745
Alday, P. H., and Doggett, J. S. (2017). Drugs in development for toxoplasmosis: advances, challenges, and current status. Drug Des. Devel. Ther. 11, 273–293.
Dunay, I. R., Gajurel, K., Dhakal, R., Liesenfeld, O., and Montoya, J. G. (2018). Treatment of toxoplasmosis: historical perspective, animal models, and current clinical practice. Clin. Microbiol. Rev. 31:e0057-17. doi: 10.1128/CMR.00057-17
El Bissati, K., Zhou, Y., Dasgupta, D., Cobb, D., Dubey, J. P., Burkhard, P., et al. (2014). Effectiveness of a novel immunogenic nanoparticle platform for Toxoplasma peptide vaccine in HLA transgenic mice. Vaccine 32, 3243–3248. doi: 10.1016/j.vaccine.2014.03.092
Fox, B. A., and Bzik, D. J. (2015). Nonreplicating, cyst-defective type II Toxoplasma gondii vaccine strains stimulate protective immunity against acute and chronic infection. Infect. Immun. 83, 2148–2155. doi: 10.1128/IAI.02756-14
Fox, B. A., Guevara, R. B., Rommereim, L. M., Falla, A., Bellini, V., Pétre, G., et al. (2019). Toxoplasma gondii parasitophorous vacuole membrane-associated dense granule proteins orchestrate chronic infection and GRA12 underpins resistance to host gamma interferon. mBio 10:e0589-19.
Guevara, R. B., Fox, B. A., Falla, A., and Bzik, D. J. (2019). Toxoplasma gondii intravacuolar-network-associated dense granule proteins regulate maturation of the cyst matrix and cyst wall. mSphere 4:e0487-19. doi: 10.1128/mSphere.00487-19
Guo, H., Gao, Y., Jia, H., Moumouni, P. F. A., Masatani, T., Liu, M., et al. (2019). Characterization of strain-specific phenotypes associated with knockout of dense granule protein 9 in Toxoplasma gondii. Mol. Biochem. Parasitol. 229, 53–61.
Howe, D. K., and Sibley, L. D. (1995). Toxoplasma gondii comprises three clonal lineages: correlation of parasite genotype with human disease. J. Infect. Dis. 172, 1561–1566. doi: 10.1093/infdis/172.6.1561
Hunter, C. A., and Sibley, L. D. (2012). Modulation of innate immunity by Toxoplasma gondii virulence effectors. Nat. Rev. Microbiol. 10, 766–778. doi: 10.1038/nrmicro2858
Huskinson-Mark, J., Araujo, F. G., and Remington, J. S. (1991). Evaluation of the effect of drugs on the cyst form of Toxoplasma gondii. J. Infect. Dis. 164, 170–171. doi: 10.1093/infdis/164.1.170
Innes, E. A. (2010). A brief history and overview of Toxoplasma gondii. Zoonoses Public Health 57, 1–7. doi: 10.1111/j.1863-2378.2009.01276.x
Kirkman, L. A., Weiss, L. M., and Kim, K. (2001). Cyclic nucleotide signaling in Toxoplasma gondii bradyzoite differentiation. Infect. Immun. 69, 148–153.
Lagal, V., Dinis, M., Cannella, D., Bargieri, D., Gonzalez, V., Andenmatten, N., et al. (2015). AMA1-deficient Toxoplasma gondii parasites transiently colonize mice and trigger an innate immune response that leads to long-lasting protective immunity. Infect. Immun. 83, 2475–2486. doi: 10.1128/IAI.02606-14
Le Roux, D., Djokic, V., Morisse, S., Chauvin, C., Doré, V., Lagrée, A. C., et al. (2020). Evaluation of immunogenicity and protection of the Mic1-3 knockout Toxoplasma gondii live attenuated strain in the feline host. Vaccine 38, 1457–1466.
Leesombun, A., Boonmasawai, S., Shimoda, N., and Nishikawa, Y. (2016). Effects of extracts from Thai Piperaceae plants against infection with Toxoplasma gondii. PLoS One 11:e0156116. doi: 10.1371/journal.pone.0156116
Li, J., Guo, H., Galon, E. M., Gao, Y., Lee, S. H., Liu, M., et al. (2020). Hydroxylamine and carboxymethoxylamine can inhibit Toxoplasma gondii growth through an aspartate aminotransferase-independent pathway. Antimicrob. Agents Chemother. 64:e01889-19.
Li, X. Z., Wang, X. H., Xia, L. J., Weng, Y. B., Hernandez, J. A., Tu, L. Q., et al. (2015). Protective efficacy of recombinant canine adenovirus type-2 expressing TgROP18 (CAV-2-ROP18) against acute and chronic Toxoplasma gondii infection in mice. BMC Infect. Dis. 15:114. doi: 10.1186/s12879-015-0815-1
Liang, Q. L., Sun, L. X., Elsheikha, H. M., Cao, X. Z., Nie, L. B., Li, T. T., et al. (2020). RHΔgra17Δnpt1 strain of Toxoplasma gondii elicits protective immunity against acute, chronic and congenital toxoplasmosis in mice. Microorganisms 8:E352.
Loh, F. K., Nathan, S., Chow, S. C., and Fang, C. M. (2019). Vaccination challenges and strategies against long-lived Toxoplasma gondii. Vaccine 37, 3989–4000. doi: 10.1016/j.vaccine.2019.05.083
Lu, G., Zhou, J., Zhou, A., Han, Y., Guo, J., Song, P., et al. (2017). SAG5B and SAG5C combined vaccine protects mice against Toxoplasma gondii infection. Parasitol. Int. 66, 596–602.
Montoya, J. G., and Liesenfeld, O. (2004). Toxoplasmosis. Lancet 363, 1965–1976. doi: 10.1016/S0140-6736(04)16412-X
Mordue, D. G., Monroy, F., La Regina, M., Dinarello, C. A., and Sibley, L. D. (2001). Acute toxoplasmosis leads to lethal overproduction of Th1 cytokines. J. Immunol. 167, 4574–4584.
Pifer, R., and Yarovinsky, F. (2011). Innate responses to Toxoplasma gondii in mice and humans. Trends Parasitol. 27, 388–393. doi: 10.1016/j.pt.2011.03.009
Robert-Gangneux, F., and Darde, M. L. (2012). Epidemiology of and diagnostic strategies for toxoplasmosis. Clin. Microbiol. Rev. 25, 264–296.
Saadatnia, G., and Golkar, M. (2012). A review on human toxoplasmosis. Scand. J. Infect. Dis. 44, 805–814. doi: 10.3109/00365548.2012.693197
Sayles, P. C., Gibson, G. W., and Johnson, L. L. (2000). B cells are essential for vaccination-induced resistance to virulent Toxoplasma gondii. Infect. Immun. 68, 1026–1033.
Shen, B., Brown, K., Long, S., and Sibley, L. D. (2017). Development of CRISPR/Cas9 for efficient genome editing in Toxoplasma gondii. Methods Mol. Biol. 1498, 79–103. doi: 10.1007/978-1-4939-6472-7_6
Spellberg, B., and Edwards, J. E. Jr. (2001). Type 1/type 2 immunity in infectious diseases. Clin. Infect. Dis. 32, 76–102. doi: 10.1086/317537
Tanaka, S., Kuroda, Y., Ihara, F., Nishimura, M., Hiasa, J., Kojima, N., et al. (2014). Vaccination with profilin encapsulated in oligomannose-coated liposomes induces significant protective immunity against Toxoplasma gondii. Vaccine 32, 1781–1785. doi: 10.1016/j.vaccine.2014.01.095
Tenter, A. M., Heckeroth, A. R., and Weiss, L. M. (2000). Toxoplasma gondii: from animals to humans. Int. J. Parasitol. 30, 1217–1258.
Wang, H. L., Pang, M., Yin, L. T., Zhang, J. H., Meng, X. L., Yu, B. F., et al. (2014a). Intranasal immunisation of the recombinant Toxoplasma gondii receptor for activated C kinase 1 partly protects mice against T. gondii infection. Acta. Trop. 137, 58–66.
Wang, H. L., Zhang, T. E., Yin, L. T., Pang, M., Guan, L., Liu, H. L., et al. (2014b). Partial protective effect of intranasal immunization with recombinant Toxoplasma gondii rhoptry protein 17 against toxoplasmosis in mice. PLoS One 9:e108377. doi: 10.1371/journal.pone.0108377
Wang, J. L., Elsheikha, H. M., Zhu, W. N., Chen, K., Li, T. T., Yue, D. M., et al. (2017). Immunization with Toxoplasma gondii GRA17 deletion mutant induces partial protection and survival in challenged mice. Front. Immunol. 8:730. doi: 10.3389/fimmu.2017.00730
Wang, J. L., Li, T. T., Elsheikha, H. M., Chen, K., Cong, W., Yang, W. B., et al. (2018). Live attenuated Pru:Δcdpk2 strain of Toxoplasma gondii protects against acute, chronic, and congenital toxoplasmosis. J. Infect. Dis. 218, 768–777.
Wang, J. L., Zhang, N. Z., Li, T. T., He, J. J., Elsheikha, H. M., and Zhu, X. Q. (2019). Advances in the development of anti-Toxoplasma gondii vaccines: Challenges, opportunities, and perspectives. Trends Parasitol. 35, 239–253.
Xia, N., Yang, J., Ye, S., Zhang, L., Zhou, Y., Zhao, J., et al. (2018a). Functional analysis of Toxoplasma lactate dehydrogenases suggests critical roles of lactate fermentation for parasite growth in vivo. Cell Microbiol. 20:10.
Xia, N., Zhou, T., Liang, X., Ye, S., Zhao, P., Yang, J., et al. (2018b). A lactate fermentation mutant of Toxoplasma stimulates protective immunity against acute and chronic toxoplasmosis. Front. Immunol. 9:1814. doi: 10.3389/fimmu.2018.01814
Yang, W. B., Wang, J. L., Gui, Q., Zou, Y., Chen, K., Liu, Q., et al. (2019). Immunization with a live-attenuated RH:ΔNPT1 strain of Toxoplasma gondii induces strong protective immunity against toxoplasmosis in mice. Front. Microbiol. 10:1875. doi: 10.3389/fmicb.2019.01875
Yarovinsky, F. (2014). Innate immunity to Toxoplasma gondii infection. Nat. Rev. Immunol. 14, 109–121. doi: 10.1038/nri3598
Zhang, N. Z., Xu, Y., Wang, M., Petersen, E., Chen, J., Huang, S. Y., et al. (2015). Protective efficacy of two novel DNA vaccines expressing Toxoplasma gondii rhomboid 4 and rhomboid 5 proteins against acute and chronic toxoplasmosis in mice. Expert. Rev. Vacc. 14, 1289–1297. doi: 10.1586/14760584.2015.1061938
Keywords: Toxoplasma gondii, toxoplasmosis, live attenuated vaccine, PLK:Δgra9, protective immunity
Citation: Li J, Galon EM, Guo H, Liu M, Li Y, Ji S, Zafar I, Gao Y, Zheng W, Adjou Moumouni PF, Rizk MA, Tumwebaze MA, Benedicto B, Ringo AE, Masatani T and Xuan X (2021) PLK:Δgra9 Live Attenuated Strain Induces Protective Immunity Against Acute and Chronic Toxoplasmosis. Front. Microbiol. 12:619335. doi: 10.3389/fmicb.2021.619335
Received: 20 October 2020; Accepted: 19 February 2021;
Published: 11 March 2021.
Edited by:
Hong-Juan Peng, Southern Medical University, ChinaReviewed by:
Si-Yang Huang, Yangzhou University, ChinaDong-Hui Zhou, Fujian Agriculture and Forestry University, China
Copyright © 2021 Li, Galon, Guo, Liu, Li, Ji, Zafar, Gao, Zheng, Adjou Moumouni, Rizk, Tumwebaze, Benedicto, Ringo, Masatani and Xuan. This is an open-access article distributed under the terms of the Creative Commons Attribution License (CC BY). The use, distribution or reproduction in other forums is permitted, provided the original author(s) and the copyright owner(s) are credited and that the original publication in this journal is cited, in accordance with accepted academic practice. No use, distribution or reproduction is permitted which does not comply with these terms.
*Correspondence: Xuenan Xuan, gen@obihiro.ac.jp