- 1State Key Laboratory of Food Nutrition and Safety, Key Laboratory of Industrial Fermentation Microbiology, Ministry of Education, Tianjin Industrial Microbiology Key Laboratory, College of Biotechnology, Tianjin University of Science & Technology, Tianjin, China
- 2Tianjin Engineering Research Center of Microbial Metabolism and Fermentation Process Control, Tianjin, China
- 3Key Laboratory of Wuliangye-flavor Liquor Solid-state Fermentation, China National Light Industry, Yibin, China
Baijiu is a traditional distilled beverage in China with a rich variety of aroma substances. 2,3,5,6-tetramethylpyrazine (TTMP) is an important component in Baijiu and has the function of promoting cardiovascular and cerebrovascular health. During the brewing of Baijiu, the microorganisms in jiuqu produce acetoin and then synthesize TTMP, but the yield of TTMP is very low. In this work, 2,3-butanediol dehydrogenase (BDH) coding gene BDH1 and another BDH2 gene were deleted or overexpressed to evaluate the effect on the content of acetoin and TTMP in Saccharomyces cerevisiae. The results showed that the acetoin synthesis of strain α5-D1B2 was significantly enhanced by disrupting BDH1 and overexpressing BDH2, leading to a 2.6-fold increase of TTMP production up to 10.55 mg/L. To further improve the production level of TTMP, the α-acetolactate synthase (ALS) of the pyruvate decomposition pathway was overexpressed to enhance the synthesis of diacetyl. However, replacing the promoter of the ILV2 gene with a strong promoter (PGK1p) to increase the expression level of the ILV2 gene did not result in further increased diacetyl, acetoin and TTMP production. Based on these evidences, we constructed the diploid strains AY-SB1 (ΔBDH1:loxP/ΔBDH1:loxP) and AY-SD1B2 (ΔBDH1:loxP-PGK1p-BDH2-PGK1t/ΔBDH1:loxP-PGK1p-BDH2-PGK1t) to ensure the fermentation performance of the strain is more stable in Baijiu brewing. The concentration of TTMP in AY-SB1 and AY-SD1B2 was 7.58 and 9.47 mg/L, respectively, which represented a 2.3- and 2.87-fold increase compared to the parental strain. This work provides an example for increasing TTMP production in S. cerevisiae by genetic engineering, and highlight a novel method to improve the quality and beneficial health attributes of Baijiu.
Introduction
Baijiu is an alcoholic beverage that is widely consumed in China (Du et al., 2011). Cereals (sorghum corn, rice, wheat, peas, and millet) and fermentation starter are the main raw materials for brewing Baijiu. The fermentation starter of Baijiu is also known as jiuqu, including daqu, xiaoqu, fuqu, and other jiuqu. Baijiu brewed from different jiuqu will have significant differences in content of aroma and flavor substances. Therefore, Baijiu is classified into five types according to its aroma: strong aroma type, light aroma type, soy sauce aroma type, sweet honey aroma type, and miscellaneous aroma type (Wang et al., 2014). Esters and alcohols are the main flavor substances and their content will affect the quality of Baijiu. Although the kinds of pyrazine are lower than these two volatile flavor compounds, pyrazine is also one of the important aroma compounds in Baijiu (Liu and Sun, 2018). Fan et al. (2007) identified 27 pyrazines in Baijiu by gas chromatography-mass spectrometry (GC-MS), including 2,5-dimethylpyrazine, 2,3,5-trimethylpyrazine, and 2,3,5,6-tetramethylpyrazine (TTMP).
2,3,5,6-Tetramethylpyrazine was originally extracted from the herb of Chuan qiong (Ligusticum wallichii), a traditional Chinese medicinal plant (Xiao et al., 2006). TTMP has been previously found to have a beneficial effect on cardiovascular and cerebrovascular health (Xiao et al., 2018; Chen et al., 2020). Moreover, recent studies have shown that TTMP has a positive therapeutic effect on several diseases, including hepatocellular carcinoma and spinal cord injury, and especially with regards to heart toxicity caused by ethanol (Shin et al., 2013; Cao et al., 2015). During Baijiu fermentation, TTMP may be produced via the microbial metabolism and acetoin is the precursor of TTMP (Meng et al., 2016; Xu et al., 2018). First, acetoin is used as a substrate to react with ammonium (or ammonia) to form α-hydroxyimine (Xiao et al., 2014). Subsequently, α-hydroxyimine is converted to 2-amino-3-butanone, which condense to form TTMP spontaneously (Xu et al., 2018; Zhang et al., 2019). It has been reported that the content of TTMP also increased with a gradually increased concentration of acetoin, suggesting that acetoin is a precursor of TTMP (Rizzi, 1988; Chen et al., 2010; Zhu et al., 2010; Meng et al., 2020).
In terms of Saccharomyces cerevisiae, pyruvate is converted into α-acetolactate by α-acetolactate synthase (ALS), which is encoded by the ILV2 gene (YMR108W) (Brat et al., 2012). In S. cerevisiae,α-acetolactate is decarboxylated into diacetyl spontaneously when the cells are cultured in an aerobic environment (Dulieu and Poncelet, 1999). Diacetyl is then reduced into acetoin by 2,3-butanediol dehydrogenase (BDH), which is encoded by the BDH1 gene (YAL060W) (Ehsani et al., 2009; Kim et al., 2013; Wess et al., 2019). Acetoin is converted into 2,3-butanediol under the control of BDH. The BDH1 gene plays an important role in the synthesis of acetoin and 2,3-butanediol. The BDH2 gene (YAL061W) is adjacent to BDH1, Bdh2p is 51% similar to the Bdh1p (González et al., 2010; Li et al., 2017). The overexpression of the BDH2 gene improved the tolerance of yeast cells to vanillin (Ishida et al., 2016, 2017). Although the function of BDH in the synthesis of 2,3-butanediol has been extensively studied, its effect on TTMP production has not been reported yet (Kim et al., 2013; Choi et al., 2016; Yang and Zhang, 2018).
2,3,5,6-Tetramethylpyrazine has been proved to be effective in the treatment of a variety of diseases and beneficial to human health (Hao et al., 2013). However, the content of TTMP in Baijiu is low, so it is interesting to increase the concentration of TTMP in Baijiu. This study is the first to genetically S. cerevisiae engineer strains to increase the content of TTMP in Baijiu. The biosynthetic pathway of TTMP and metabolic regulation strategy is shown in Figure 1. In α-type haploid strain, BDH coding gene BDH1 and another gene BDH2 were deleted or overexpressed to elucidate their role in TTMP production. Then, recombinant diploid strains were constructed to ensure the fermentation performance during Baijiu brewing. The results demonstrated that overexpression of BDH2 and deletion of BDH1 can effectively increase the accumulation of acetoin and then enhance the content of TTMP. The concentrations of ester and higher alcohol produced by the diploid strains were not affected by the genetic modification. In addition, we found that overexpression of the ALS coding gene ILV2 had no significant effect on the production of acetoin and TTMP. Overall, the diploid strains showed significant potential for industrial applications.
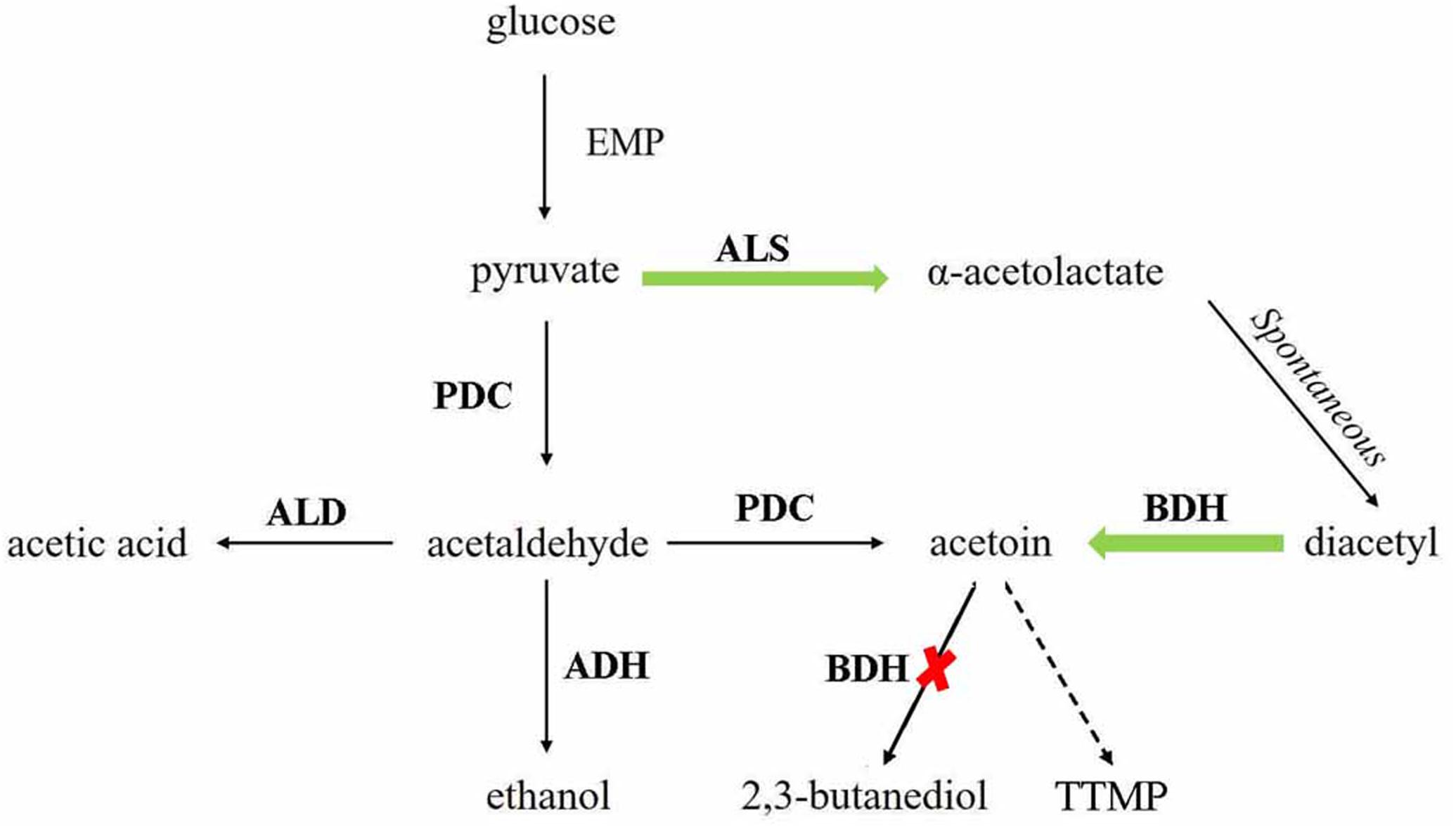
Figure 1. TTMP and acetoin pathway construction in Saccharomyces cerevisiae. The green arrows indicate the enhanced pathways. The red cross mark indicates the disrupted pathways. ALS, α-acetolactate synthase, encoded by ILV2; PDC, pyruvate decarboxylase, encoded by PDC1, PDC5, and PDC6; ALD, acetaldehyde dehydrogenase, encoded by ALD6; ADH, alcohol dehydrogenase, encoded by ADH1, ADH3, and ADH5; BDH, 2,3-butanediol dehydrogenase, encoded by BDH1.
Materials and Methods
Strains and Medium
The strains and plasmids used in this study are listed in Table 1. S. cerevisiae was grown in YPD medium at 30°C. Then, 1,000 μg/mL G418 (Promega, Madison, WI, United States) was added to the YPD medium to select the right transformants. The YPG medium (10 g/L yeast extract, 20 g/L peptone, 20 g/L galactose) was used to eliminate the KanMX resistance gene, as the selection marker for the yeast strain. Escherichia coli was grown in LB medium at 37°C. Ampicillin (100 μg/mL) was added to the LB medium as required. The YPD, LB, and YPG mediums were mixed with 2% agar powder for plating.
Construction of Recombinant Strains
The primers used in this study are listed in Supplementary Table 1. The plasmid pUG6 was used to obtain the loxP-KanMX-loxP fragment, with the KanMX gene used as the selection marker for the yeast strain. To construct the BDH1 gene deletion strain (α5-TB1) and BDH2 gene deletion strain (α5-TB2), the plasmid pUG6 was used as the template to obtain the KanMX-B1 and KanMX-B2 fragments using the primers B1C-U/B1C-D and B2C-U/B2C-D, respectively. Homologous recombination was used to assemble the fragments into the parental strain α5.
The ILV2 expression cassette was composed of the homologous sequence fragments of the ILV2 gene, ILV2A and ILV2B, the PGK1 promoter and terminator, the ILV2 gene, and the KanMX gene. The cassette containing the ILV2 gene was under the control of the PGK1 promoter and PGK1p terminator. The strain α5-VI2 was obtained as follows: the ILV2A-loxP-KanMX-loxP-PGK1p and PGK1t-ILV2B fragments were obtained through PCR using the primers L1-F/L1-R and L2-F/L2-R, respectively, from the plasmid YEPI2KPB1. The fragment of the ILV2 gene was obtained using the primers ILV2-F/ILV2-R from the α5 yeast genome.
The construction of the α5-VB1, α5-VB2, and α5-D1B2 strain was similar to that of α5-ILV2. The recombinant fragments and corresponding PCR primers of the constructed strains are listed in Supplementary Table 2. The α5 yeast strain was transformed using lithium acetate/PEG according to the standard protocol (Gietz and Woods, 2002). The transformant cells were grown on a YPD-G418 plate for 48 h at 30°C. The recombinant strains were then verified using PCR with different primers. Finally, the resistance gene KanMX of the recombinant strains α5-TB1, α5-VB1, α5-TB2, α5-VB2, α5-D1B2, and α5-VI2 were removed using the Cre/loxP procedure.
Construction of the Diploid Recombinants
To obtain diploid recombinant strains, the a-type and α-type haploid strains were hybridized. These two type strains were cultured in test tubes until the logarithmic growth phase in YPD medium. They were then hybridized in fresh YPD medium for 8 h. The diploid recombinants were tested with MAT-F/MAT-a/MAT-α as primers.
The a-type strain a8 was hybridized with α-type haploid recombinant strain α5-TB1 and α5-D1B2 to obtain the diploid recombinant strains AY-B1 and AY-D1B2, respectively. The KanMX-B1S fragment was amplified using PCR with the primers B1C-2U/B1C-2D from the plasmid pUG6. The B12A-KanMX-PGKp-BDH2-PGKt-B12B fragment was amplified using the primers B12C-U/B12C-D from the α5-D1B2 strain. The KanMX-B1S and B12A-KanMX-PGK1p-BDH2-PGK1t-B12B fragments were transferred into the AY-B1 and AY-D1B2 strains to delete the BDH1 gene of the a8 strain, respectively. The resulting diploid strains were verified by PCR using the corresponding primers. The KanMX gene of the resulting diploid strains was eliminated using the Cre/loxP procedure, to obtain the AY-SB1 and AY-SD1B2 strains.
Fermentation Experiments
Corn-semi-solid medium was used to simulate industrial Baijiu fermentation (Li W. et al., 2017). The corn-semi-solid medium was prepared by gelatinizing a mixture of 60 g of corn flour and 130 mL of water at 65°C for 20 min in a 250-mL conical bottle. Then the mixture was liquefied at 90°C for 90 min with thermostable α-amylase (10 U/g corn weight, 2 × 105 U/mL) and subsequently saccharified at 60°C for 30 min with a saccharifying enzyme (150 U/g corn weight, 10 × 105 U/mL). The resulting medium was cooled to 30°C at room temperature. The strains were cultured in a test tube with 8° Bx medium (6 mL) for 24 h. The culture was then added to 12° Bx medium (54 mL) for 16 h. Finally, 15 mL of the strain culture was inoculated into corn-semi-solid fermentation medium for 4 days. The content of residual sugar was measured using Fehling’s reagent.
GC and HPLC Analysis
The contents of flavor substances (esters, higher alcohols, diacetyl, and TTMP) were detected by gas chromatography (GC) using an Agilent 7890C GC (Agilent, Palo Alto, CA, United States) equipped with an G4513A autosampler, injector, and flame ionization detector (FID) (Cui et al., 2018). HP-INNOWax polyethylene glycol (30 m × 320 μm, i.e., 0.5 μm coating thickness) was used for separation. N-Butyl acetate was used as the internal standard. An internal calibration curve was established utilizing authentic standards to detected the content of the compound. The chemicals were purchased from Merck (Darmstadt, Germany).
High-performance liquid chromatography (HPLC) was used to measure the production of acetoin and 2,3-butanediol. The fermentation broth was diluted to an appropriate concentration and filtered using a 0.22-μm filter. Agilent 1260HPLC equipped with a Bio-Rad HPX-87H column was used, according to the detection method reported by Li P. et al. (2018).
Real-Time Quantitative PCR
The transcript levels of the BDH1, BDH2, and ILV2 genes in the strains were detected using quantitative real-time PCR (RT-qPCR). The ACT1 gene was used as the reference gene. The primers ACT1-F/ACT1-R, BDH1-F/BDH1-R, BDH2-F/BDH2-R, and ILV2-F/ILV2-R were used to amplify the ACT1, BDH1, BDH2, and ILV2 genes, respectively. Yeast Processing Reagent (Takara Biotechnol, Dalian, China) was used to extract yeast total RNA, and then reverse transcribed using a PrimeScriptTM RT reagent Kit with gDNA Eraser (Perfect Real Time) (Takara Biotechnol, Dalian, China). TB Green PreMix Ex Taq II (Tli RNAseH Plus) (Takara Biotechnol, Dalian, China) was used to detect changes in gene expression levels by RT-qPCR. The PCR amplification program includes 95°C pre-denaturation 30 s, 95°C denaturation 5 s, 60°C annealing polymerization 30 s, melting curve stage 15 s, 60°C 1 min. Real-time PCR was performed using a StepOnePlus real-time PCR system (Applied Biosystems/Thermo Fisher Scientific, Foster City, CA, United States). The 2–ΔΔCt method was used for quantitative analysis.
Enzyme Assays
The enzyme activities of BDH and ALS were determined as previously described (Calam et al., 2016; Murashchenko et al., 2016). The protein concentration was determined using a TaKaRa Bradford Protein Assay Kit (Takara Biotechnology, Dalian, China). The ALS reaction mixture contained 40 mM pyruvate, 1 mM thiamine diphosphate, 1 mM MgCl2, 10 μM FAD, and 100 mM potassium phosphate buffer (pH 7.0). The enzyme activity unit was defined as the amount of acetoin catalyzed by 1 mg enzyme within 1 min under reaction conditions. The BDH reaction mixture contained 10 mM acetoin, 0.1 mM NADH, and 100 mM potassium phosphate buffer (pH 7.0). Acetoin and NADH were used as substrates to measure the activity of BDH. The BDH enzyme unit was defined as 1 μmol of NAD+ produced from NADH per min.
Determination of the Growth Curve
The method used to calculate the growth curve was obtained from Li W. et al. (2018). Briefly, the yeast strain was grown in YPD medium for 12 h up until the stationary phase. Then, the yeast culture was inoculated into fresh YPD medium and cultured in a shaking flask for 24 h. The optical density (OD600) was measured every 1 h to obtain the data needed to draw the growth curve.
Statistical Analysis
Data are provided as the mean ± standard error. The differences between the recombinant strains and host strain were analyzed using Student’s t-test. A p-value <0.05 was considered statistically significant.
Results and Discussion
Effects of BDH1 Deletion or Overexpression on Production of TTMP in Saccharomyces cerevisiae
The BDH1 gene encodes BDH and plays an important role in the synthesis of 2,3-butanediol and acetoin (Kim et al., 2013). To determine the effect of BDH1 gene on TTMP production, we constructed BDH1 deletion strain α5-TB1 and BDH1 overexpression strain α5-VB1. The TTMP content in the strain α5-TB1 was 1.94-fold higher than that of strain α5 (Table. 2). As the precursor, acetoin production by strain α5-TB1 was 3.77-fold greater than that of α5. Meanwhile, 2,3-butanediol produced by strain α5-TB1 was reduced by 77.33%. The recombinant strain α5-VB1 yielded a 74.85% decrease in acetoin compared to the parental strain. Hence, the production of TTMP by recombinant strain α5-VB1 decreased slightly. The production of 2,3-butanediol by α5-VB1 was increased to 2,702.16 mg/L, which was 1.44-fold greater than that generated by α5. Moreover, deletion or overexpression of BDH1 gene did not affect the fermentation performance (ethanol production, CO2 emission, residual sugar, and growth curve) of the strain (Table. 3 and Supplementary Figure 1A).
The expression of BDH1 at the transcriptional level in strains α5-VB1 and α5-TB1 was also measured by RT-qPCR. As shown in Figure 2A, the relative expression of the BDH1 gene in the recombinant α5-VB1 strain was 16.38-fold that of α5. In strain α5-TB1, the BDH1 relative expression level was approximately zero. The expression of BDH2 in α5-VB1 and α5-TB1 were 0.45- and 0.72-fold that of α5. Compared with the parental α5 strain, the level of BDH enzyme activity in the α5-VB1 strain were significantly improved. As shown in Figure 3, the specific activity of BDH in the parental strain α5 at 25°C was 0.264 U/mg. The activity of BDH in the recombinant α5-VB1 and α5-TB1 strains was 1.32 and 0.027 U/mg, respectively. This result is consistent with the transcription assay shown in Figure 2A. In our study, the enzyme activity of BDH was almost reduced to zero after knocking out the BDH1 gene. This indicates that the BDH1 gene is the main BDH encoding gene, which supports the conclusion that the expression of BDH2 gene is not related to BDH activity (González et al., 2001, 2010).
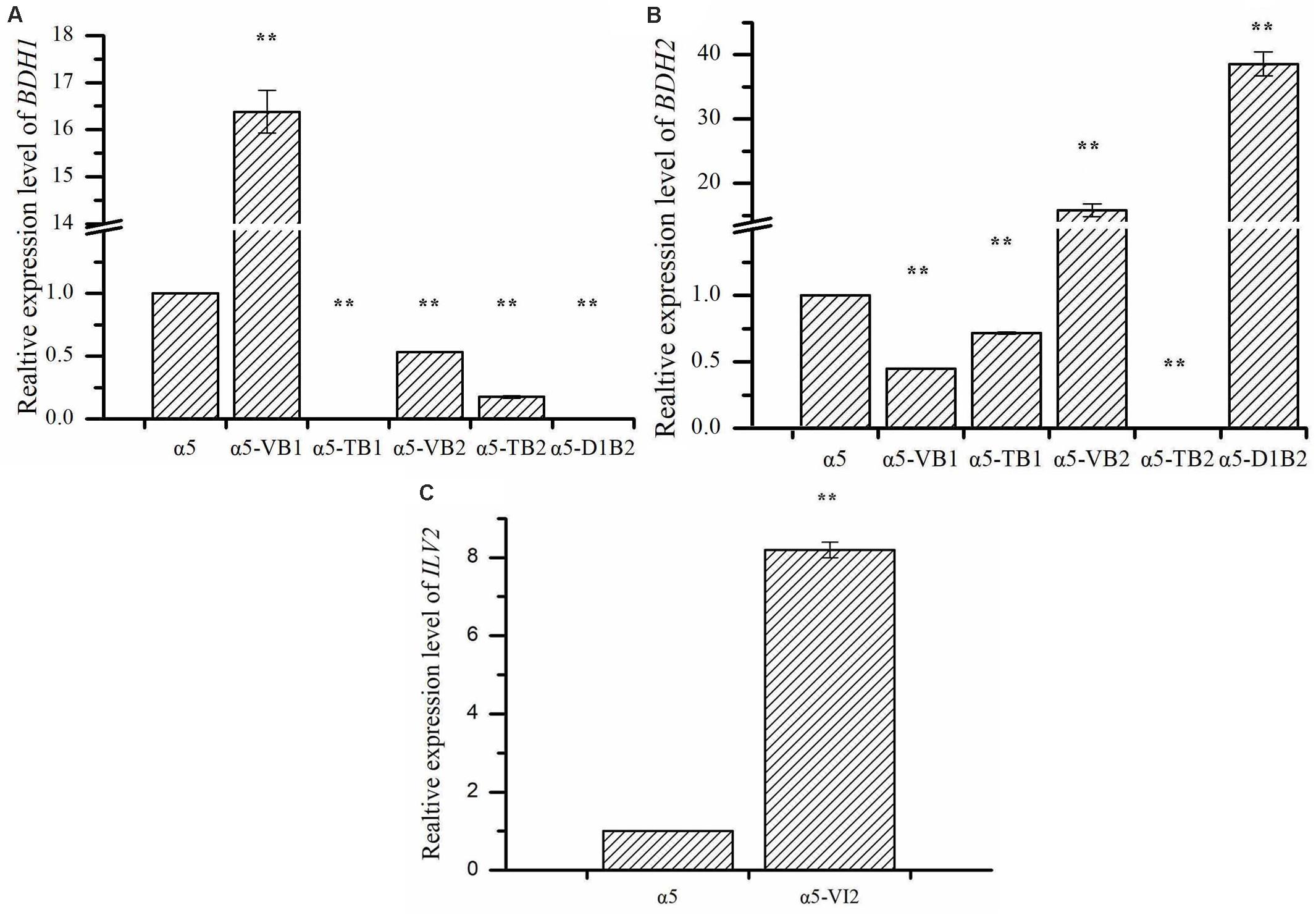
Figure 2. The relative expression levels of BDH1, BDH2, and ILV2. (A) The relative expression of BDH1 in strain α5, α5-VB1, α5-TB1, α5-VB2, α5-TB2, and α5-D1B2. (B) The relative expression of BDH2 in strain α5, α5-VB1, α5-TB1, α5-VB2, α5-TB2, and α5-D1B2. (C) The relative expression of ILV2 in strain α5 and α5-VI2. Data represent the mean of three independent biological replicates. Error bars represent the SD of the average values. Statistical significance is denoted as **P < 0.01 and **P < 0.05.
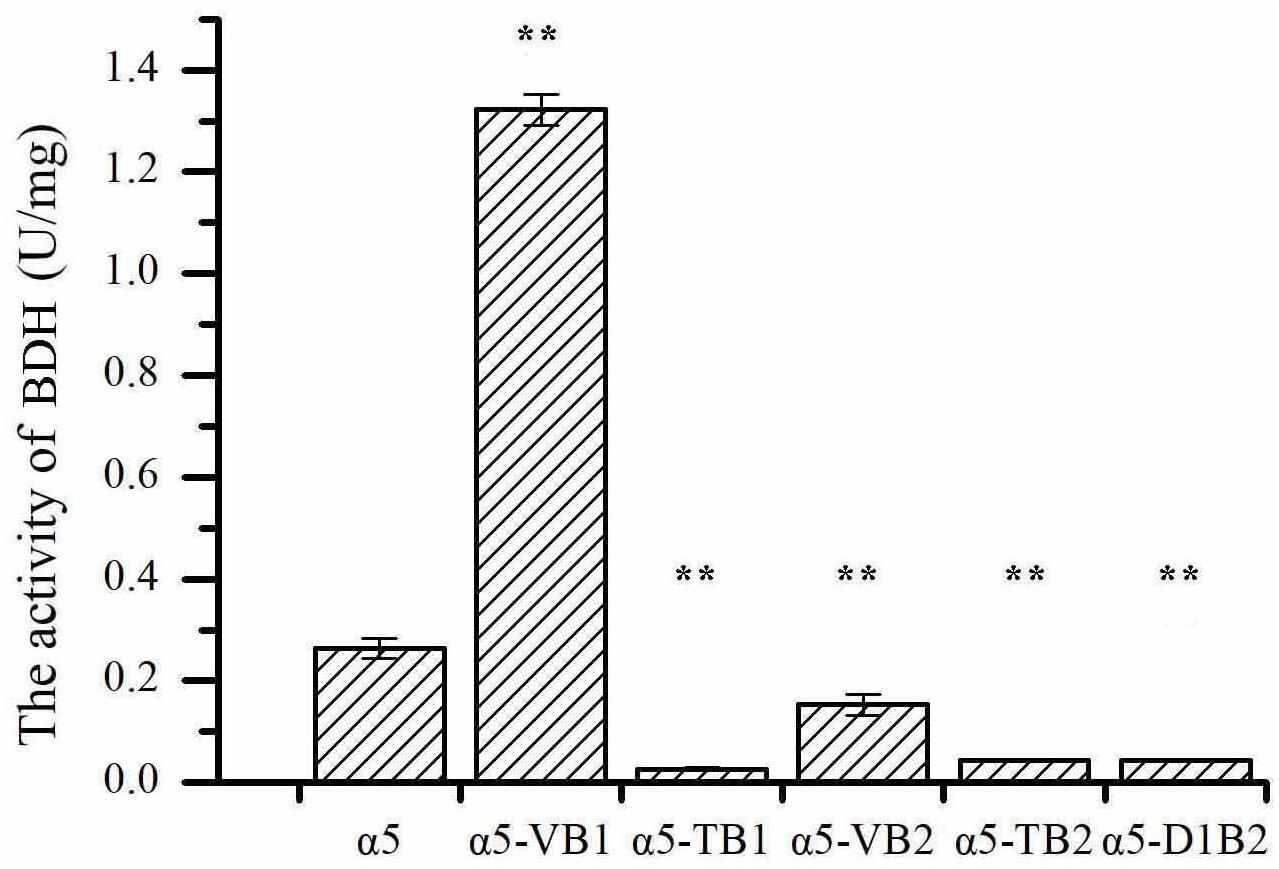
Figure 3. The specific activities of 2,3-butanediol dehydrogenase (BDH). Data represent the mean of three independent biological replicates. Error bars represent the SD of the average values. Statistical significance is denoted as **P < 0.01 and **P < 0.05.
In the present work, YAL060W gene was considered to encode BDH, namely BDH1 (González et al., 2000, 2001). Many subsequent reports focused on the regulating the production of glycerin, isobutanol, and 2,3-butanediol in laboratory strain of S. cerevisiae (Ehsani et al., 2009; Kim et al., 2014; Wess et al., 2019). However, the Bdh1p activity of industrial S. cerevisiae strain may be different from that of laboratory strain. The results of Kuang et al. (2020) demonstrated that the reduction of acetaldehyde, glycolaldehyde and furfural by Bdh1p from industrial strain was better than that of Bdh1p from laboratory strain. Therefore, we further deleted and overexpressed BDH1 gene in industrial strain α5, resulting in strain α5-TB1 and α5-VB1, respectively. The production of acetoin indicates that the deletion of BDH1 gene is beneficial to the synthesis of acetoin. After knocking out the BDH1 gene, the relative expression level of the BDH1 gene and the enzyme activity of the BDH decreased, which resulted in the pyruvate flux was successfully redirected toward acetoin pathway (Bae et al., 2016). With the accumulation of acetoin, the TTMP produced during the fermentation of Baijiu has also increased, which is consistent with the previous research conclusions (Hao et al., 2013; Zhang et al., 2019; Zhong et al., 2020).
Many reports have focused on reducing or eliminating the production of glycerol and ethanol by knocking out the ADH1-5, PDC1, PDC5, GPD1, and GPD2 genes, which redirect metabolic flux to 2,3-butanediol or acetoin for high-yield production (Kim et al., 2013; Kim and Hahn, 2015; Bae et al., 2016). However, although the content of acetoin in S. cerevisiae strain with ADH1-5 gene deletion was increased, the yield of ethanol was significantly reduced, which was unfavorable for Baijiu fermentation. Moreover, the simultaneous knockout of PDC1 and PDC5 genes would affect the fermentation performance of the engineered strain (Oud et al., 2012). In addition, the introduction of acetoin biosynthetic pathway (ALS and α-acetolactate decarboxylase) from Bacillus subtilis into S. cerevisiae can also significantly increase the concentration of acetoin (Kim and Hahn, 2015; Jia et al., 2017). However, the insertion of heterogeneous genes may pose a hidden danger to the safety of the strain (Gibson et al., 2018). Therefore, these strategies are not suitable for the construction of high-yield TTMP-producing Baijiu industrial strain.
Effects of BDH2 Deletion or Overexpression on Production of TTMP in Saccharomyces cerevisiae
The amino acid sequence of Bdh2p is 51% identical to that of Bdh1p, but the effect of Bdh2p on the production of acetoin and TTMP have not been reported yet. We determined the effect of BDH2 overexpression or deletion on TTMP production in S. cerevisiae. The concentration of TTMP in the α5-TB2 (BDH2 deletion) and α5-VB2 (BDH2 overexpression) strains was 4.12 and 5.48 mg/L, respectively (Table. 2). The recombinant strain α5-TB2 yielded a 16.07% decrease in acetoin compared with the initial α5 strain. Overexpression of the BDH2 gene increased the production of acetoin by 1.75-fold compared with the original strain. The production of 2,3-butanediol by the α5-TB2 and α5-VB2 strains was 10.62 and 18.75% decrease compared to that produced by α5, respectively. The deletion or overexpression of BDH2 gene did not have an impact on fermentation performance in Baijiu fermentation (Table 3 and Supplementary Figure 1A).
The expression of BDH2 at the transcriptional level in α5-TB2 and α5-VB2 was also measured by RT-qPCR. The BDH2 relative expression level in α5-TB2 was approximately zero. The expression of BDH2 in α5-VB2 was 15.84-fold higher than that of α5 (Figure 2B). Under the control of PGK1p promoter, the relative expression level of BDH2 gene was increased significantly. Moreover, the BDH1 gene expression level and BDH activity were detected in strains α5-TB2 and α5-VB2. As shown in Figure 2A, the relative expression of the BDH1 gene in the recombinant α5-VB2 and α5-TB2 strains was 0.53- and 0.17-fold that of α5. The BDH enzyme activity was also decreased significantly. Obviously, the decrease of BDH enzyme activity of strain α5-VB2 and α5-TB2 was closely related to the expression level of BDH1 gene. This means that overexpression or deletion of BDH2 gene affected the transcription level of BDH1 gene firstly, and then decreased the enzyme activity of BDH. Therefore, the enzyme activity of BDH depends on the transcription level of BDH1 gene, and has no obvious relationship with BDH2 gene.
Many recent reports focus on the use of BDHs gene to regulate yeast tolerance to vanillin and acetaldehyde. Ishida et al. (2017) found that BDH2 gene overexpression improved the tolerance of S. cerevisiae to vanillin, and this effect was stronger than that of overexpression of BDH1. In reduction of acetaldehyde, the activities of Bdh2p were significantly higher than Bdh1p (Kuang et al., 2020). However, few literatures have reported that the overexpression of BDH2 gene can improve the synthesis of acetoin and TTMP. In our study, we confirmed that overexpression of BDH2 could reduce the content of diacetyl, thereby increasing the production of acetoin and TTMP. As shown in Figure 2, we found that knocking out or overexpressing the BDH1 gene will reduce the expression level of the BDH2 gene. Similarly, the expression level of BDH1 gene was also reduced to different degrees in BDH2 deletion or overexpression strains. In the study of González et al. (2010), the same phenomenon occurred when the BDH1 or BDH2 gene was deleted. Due to the changes in the transcription level of BDH1 and BDH2 genes, the activity of BDH enzymes was also regulated to different degrees. Based on these data, we speculate that there is a potential association between the Bdh1p and Bdh2p in S. cerevisiae. But the functions of Bdh1p and Bdh2p were different: Bdh1p was related to the synthesis of 2,3-butanediol, while Bdh2p was related to the synthesis of acetoin. Under the catalysis of Bdh2p, diacetyl is converted into acetoin, increasing the concentration of TTMP. Hence, the diacetyl content produced by the strain α5-VB2 was lower than that of parental strain α5 and the yield of acetoin increased by 1.74-fold. In contrast, the acetoin production was decreased due to the deletion of BDH2 gene in the α5-TB2 strain. If the main enzyme that converts diacetyl to acetoin is Bdh1p, then the content of acetoin should be increased, but this is contrary to our experimental results. In our study, the acetoin content produced by strain α5-VB1 (BDH1 overexpression) decreased by 74.85% compared with the strain α5, while the production of 2,3-butanediol increased significantly. Therefore, the main function of Bdh1p is related to the synthesis of 2,3-butanediol. However, the specific mechanism between Bdh1p and Bdh2p is still unclear and needs further research. It is the first report that overexpression of the BDH2 gene can increase the concentration of TTMP produced in Baijiu fermentation.
Increasing Production of TTMP by BDH1 Deletion and BDH2 Overexpression in Saccharomyces cerevisiae
Based on the results of TTMP productions in the strains α5-TB1, α5-VB1, α5-TB2, and α5-VB2, BDH1 gene deletion and BDH2 gene overexpression may improve the content of TTMP. Therefore, we constructed the recombinant strain α5-D1B2. The constructed α5-D1B2 strain with a 4.88-fold increase in acetoin production and a 2.63-fold increase in TTMP titer compared to the parental strain (Table. 2). Owing to the combined action of the BDH1 deletion and BDH2 overexpression, the final concentrations of diacetyl and 2,3-butanediol were markedly decreased. The fermentation performance of the α5-D1B2 strain was similar to that of parental strain (Table 3 and Supplementary Figure 1A).
Due to the deletion of BDH1 gene, the relative expression level of BDH1 was almost zero (Figure 2A). As shown in Figure 2B, the expression of BDH2 in α5-D1B2 were 38.56-fold that of α5. The expression level of BDH2 gene was higher than that of single overexpression of BDH2 gene (strain α5-VB2). It seems that the expression level of BDH2 gene is influenced by BDH1 gene, which confirmed our hypothesis that the functions of Bdh1p and Bdh2p are closely related. These changes demonstrated that the combination of BDH1 gene deletion and BDH2 gene overexpression could increase the concentration of TTMP significantly, which was the most effective strategy in this study.
No Influence of ILV2 Overexpression on Production of TTMP in Saccharomyces cerevisiae
In S. cerevisiae, α-acetolactate is decarboxylated into diacetyl spontaneously. This means that the strategy of enhancing the “α-acetolactate – diacetyl” pathway by genetic engineering could not be used to increase the content of diacetyl. Since pyruvate produced α-acetolactate under the catalysis of ALS, we focused on enhancing this pathway to increase the content of diacetyl (Espinosa-Cantú et al., 2018). In order to determine the accumulation effect of increasing the expression level of ALS on diacetyl production, thereby increasing the content of acetoin and TTMP, we improved the catalytic reaction of the α-acetolactate biosynthetic pathway by overexpressing the ILV2 gene, resulting in strain α5-VI2. As shown in Table. 2, the diacetyl content in the parental strain α5 and recombinant strain α5-VI2 was 30.85 and 34.06 mg/L, respectively. Except that the content of diacetyl was slightly increased, no other metabolites were affected by ILV2 overexpression. As shown in Table 3 and Supplementary Figure 1A, the performances of strain α5-VI2 did not change significantly in comparison to the parental strain α5.
The relative expression level of ILV2 gene was determined by RT-PCR. As shown in Figure 2C, the relative expression level of ILV2 was increased significantly, which was 8.21-fold higher than that of the parental strain a5. As shown in Figure 4, the specific activities of ALS in the α5 and α5-VI2 strains were 0.0111 and 0.0121 U/mg, respectively. The overexpression of ILV2 gene had little effect on the activity of the ALS. Further promoting of α-acetolactate biosynthesis by overexpressing ILV2 did not significantly improve the concentration of TTMP.
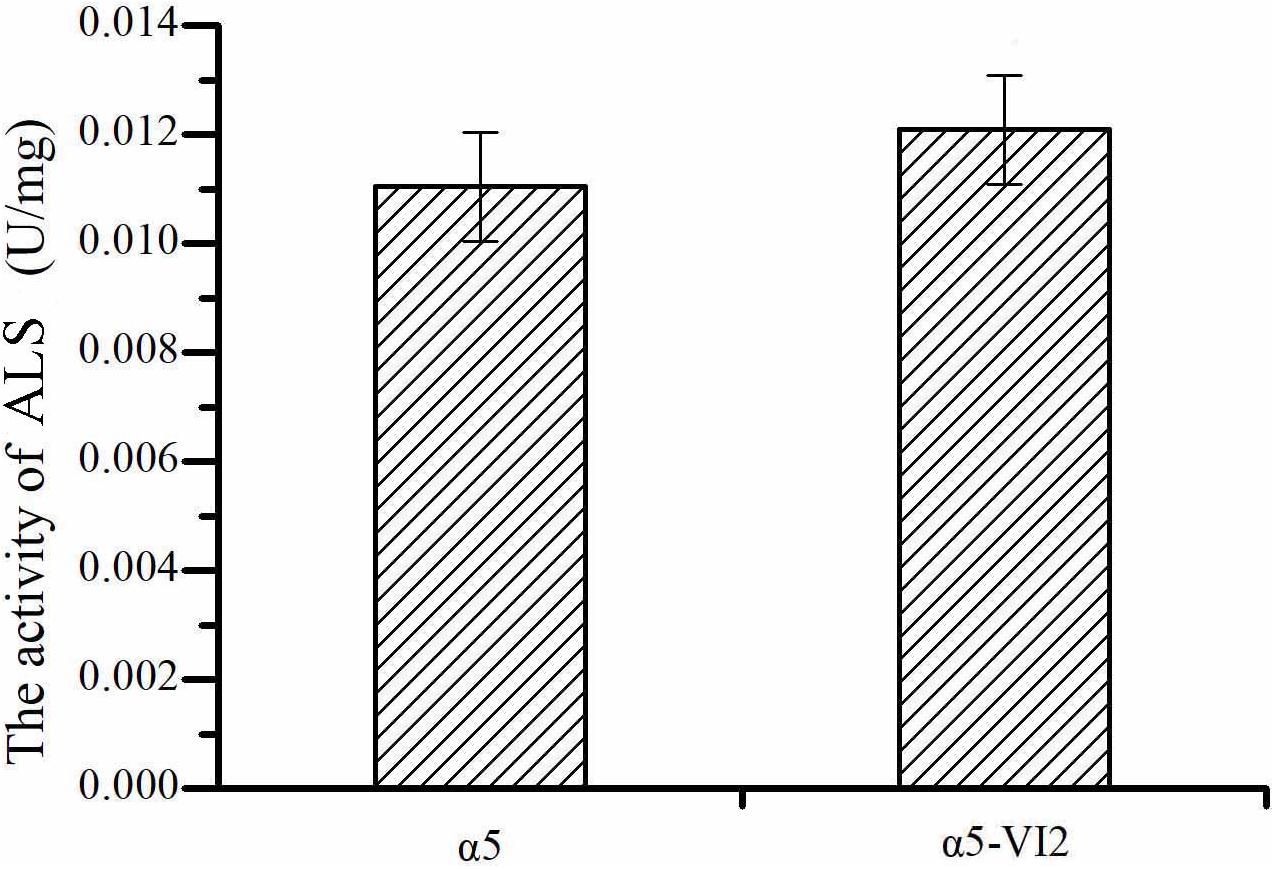
Figure 4. The activities of α-acetolactate synthase (ALS). Data represent the mean of three independent biological replicates. Error bars represent the SD of the average values.
During the fermentation of beer and wine, high concentration of diacetyl was often considered to affect quality of alcoholic beverages (Bartowsky and Henschke, 2004; Humia et al., 2019). In previous studies, we tried to regulate the content of diacetyl by controlling the expression level of ILV2 gene. Shi et al. (2016) reduced the diacetyl content during beer fermentation by 17.83% by knocking out the ILV2 gene in brewer’s yeast strain. Meanwhile, the content of diacetyl was decreased by 61.93% in recombinant brewer’s yeast, which deleting ILV2 gene and overexpressing acetohydroxyacid reductoisomerase encoding gene ILV5 (Shi et al., 2017). These results indicated that the expression of ILV2 gene is related to diacetyl biosynthesis. However, in this study, the biosynthesis of diacetyl needs to be improved and then increased the content of acetoin.
The ILV2 gene overexpression slightly increased diacetyl production, but had no significant influence on the content of TTMP, acetoin, 2,3-butanediol. Although the expression level of the ILV2 gene increased, it did not significantly affect the activity of the ALS. It has been known that ALS consists of two subunits, the catalytic subunit (Ilv2p) and the regulatory subunit (Ilv6p). A previous study revealed that Ilv2p activity is negatively regulated by the regulatory subunit Ilv6, which may be one of the reasons for affecting the enzyme activity of Ilv2p (Dasari and Kölling, 2011; Takpho et al., 2018). Diacetyl production was also regulated by the expression level of ILV6 gene. Li P. et al. (2018) reduced the expression level of ILV6 gene in Saccharomyces uvarum by 40% and then diacetyl production was reduced by 25.71%. In lager brewers’ yeast with single and double deletion of ILV6, the ILV6 expression was reduced by 13% and 40%, respectively, and the concentration of diacetyl was significantly reduced. However, the enzyme activity of ALS had not been significantly reduced, which was similar to our experimental results (Duong et al., 2011). It has been reported that the ILV6 transcription level of native strain with high diacetyl production is higher than that of native strain with low production (Gibson et al., 2015). Meanwhile, overexpression of the ILV6 gene of S. cerevisiae not only enhanced the content of diacetyl, but also increased the transcription level of ILV2 gene. These results suggest that an effective strategy to increase ALS enzyme activity may be to simultaneously overexpress the genes encoding the regulatory and catalytic subunits, which are ILV6 and ILV2 genes.
Moreover, the acetohydroxyacid reductoisomerase (Ilv5p) catalyzes the degradation of α-acetolactate to produce dihydroxy isovalerate, hence the content of diacetyl is also regulated by Ilv5p (Omura, 2008; van Bergen et al., 2016; Shi et al., 2017). In our study, the diacetyl content of strains overexpressing the ILV2 gene was only slightly increased, which may be related to the decomposition of a part of α-acetolactate by Ilv5p. Furthermore, the conversion of α-acetolactate to diacetyl is a rate-limiting step in S. cerevisiae (Kim et al., 2017). This may mean that α-acetolactate needs a large amount of accumulation to significantly increase the concentration of diacetyl. However, α-acetolactate is toxic to S. cerevisiae cells and affects the growth performance of the strain (Park and Hahn, 2019). In addition, too high content of diacetyl results in an unwanted buttery flavor, the balance of Baijiu flavor will be broken and the quality of Baijiu will also be decreased. In general, the strategy of promoting the synthesis of acetoin and TTMP by excessively increasing the content of diacetyl is not entirely ideal for Baijiu. Therefore, it is necessary to precisely control the concentration of α-acetolactate and diacetyl to maintain the balance between diacetyl and Baijiu flavor.
Production of TTMP by Recombinant Diploid Strains
The TTMP content of haploid strains α5-TB1 (BDH1 deletion) and α5-D1B2 (BDH1 deletion and BDH2 overexpression) significantly increased compared with the parental strain α5. In order to investigate the fermentation performance of the strain during Baijiu brewing, four diploid strains (AY-B1, AY-SB1, AY-D1B2, and AY-SD1B2) were constructed based on strains α5-TB1 and α5-D1B2. The production of TTMP and acetoin by diploid strains was investigated by simulating alcohol fermentation experimentally. The data obtained by GC and HPLC are shown in Table. 4. The content of TTMP and acetoin in the parental AY15 strain were 3.29 and 581.13 mg/L, respectively. Compared to the AY15 strain, the AY-B1 strain (BDH1 single-allele-deletion diploid) yielded a 10.94 and 24.07% increase in TTMP and acetoin production, respectively. The AY-SB1 strain (BDH1 double-allele-deletion diploid) yielded a 130.39% increase in TTMP and a 271.57% increase in acetoin.
The diploid strain AY-D1B2 represents BDH1 single-allele-deletion and BDH2 single-allele-overexpression. The production of TTMP and acetoin by the AY-D1B2 strain was 4.48 and 909.73 mg/L, respectively. Strain AY-D1B2 yielded a 36.17% increase in TTMP and a 56.54% increase in acetoin. The diploid strain AY-SD1B2 was BDH1 double-allele-deletion and BDH2 double-allele-overexpression. The TTMP and acetoin content represented a 2.87- and 4.97-fold increase compared to strain AY15. As shown in Table. 3 and Supplementary Figure 1B, the fermentation performance of the AY-B1, AY-SB1, AY-D1B2, and AY-SD1B2 were similar to that of parental strain AY15. The production of acetoin and TTMP produced by AY-SD1B2 was the highest among the four diploid strains, while the double-allele-deletion of BDH1 and the double-allele-overexpression of BDH2 had the greatest impact on acetoin and TTMP production. In addition, regardless of whether this was the result of the deletion or overexpression of BDH1 and BDH2, the content of acetoin was positively correlated with TTMP production.
Diploid strains of S. cerevisiae are widely used for fermentation in the food industry, including bread making and wine brewing. The diploid strain of S. cerevisiae is used as a stable source of fermentation in Baijiu brewing. To ensure the fermentation performance of the strain, it is necessary to hybridize the haploid strains into diploid strains. Diploid strains AY-SB1 (BDH1 double-allele-deletion) and AY-SD1B2 (BDH1 double-allele-deletion and BDH2 double-allele-overexpression) were found to produce high levels of acetoin, which resulted in increased TTMP production. Moreover, compared with the original strain AY15, there were no significant differences regarding the growth curve, the fermentation performance, or the production of esters or higher alcohols. It is worth noting that the BDH1 double-allele-deletion and BDH2 double-allele-overexpression were the most efficient in terms of increased TTMP production. This study provides an important reference for the application genetic engineering to S. cerevisiae in order to increase the yield of TTMP in Baijiu and other alcoholic beverages, thereby improving their beneficial health attributes.
Production of Ester and Higher Alcohol by Recombinant Diploid Strains
To analyze the effects of the modification of BDH1 and BDH2 genes on the flavor substances, we detected the contents of esters and higher alcohols in diploid strains. The production of ester and higher alcohol was detected as a result of corn-semi-solid medium fermentation. As shown in Figure 5, the production of ethyl acetate by the AY15, AY-B1, AY-SB1, AY-D1B2, and AY-SD1B2 strains was 24.51, 19.44, 23.87, 27.98, and 24.92 mg/L, respectively. The AY-D1B2 strain showed a 23.71% decrease in the production of 2-phenylethanol. There were no significant changes in terms of the content of other higher alcohols in the fermentation samples of AY-B1, AY-SB1, AY-D1B2, and AY-SD1B2. These results indicated that the modification of BDH1 and BDH2 did not affect the flavor substance content produced by the diploid strain.
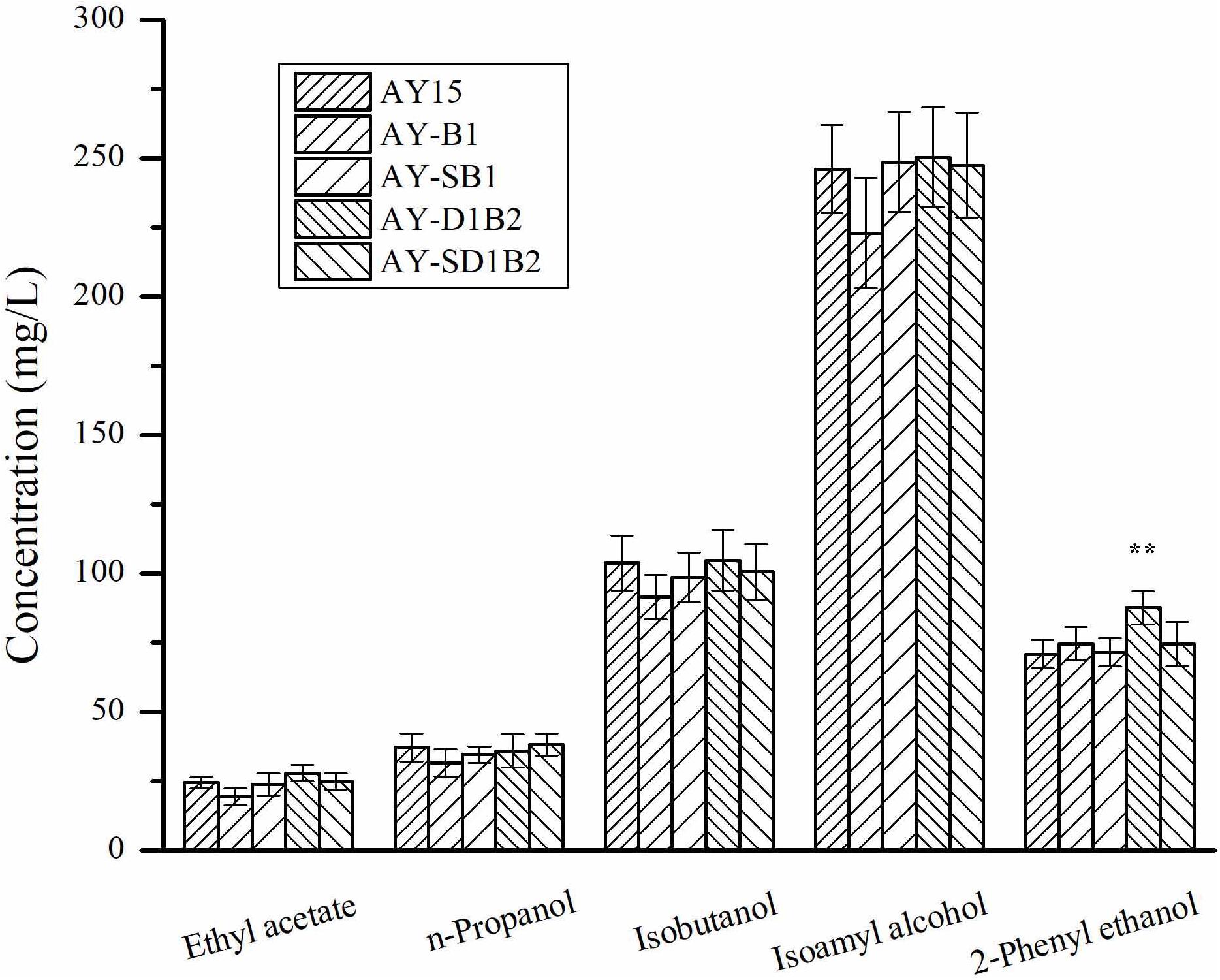
Figure 5. The content of esters and higher alcohols in diploid strains. Data represent the mean of three independent biological replicates. Error bars represent the SD of the average values. Statistical significance is denoted as **P < 0.01 and **P < 0.05.
Conclusion
2,3,5,6-Tetramethylpyrazine is a type of pyrazine that has a nutty aroma, and is a key flavor compound of Baijiu (Zhu et al., 2007). During Baijiu fermentation, acetoin is converted to TTMP spontaneously rather than as an enzymatic reaction, which is one of the reasons for the low TTMP content in Baijiu (Xiao et al., 2014; Zhang et al., 2019). In this study, we focus on regulating the expression levels of BDH1 and BDH2 genes to control the content of acetoin and TTMP. The results proved that knockout of BDH1 or overexpression of BDH2 can promote the synthesis of acetoin, and then increase the concentration of TTMP. In our research, knocking out BDH1 and overexpressing BDH2 in S. cerevisiae is the best strategy to increase the concentration of TTMP. It is important that the fermentation performance and the content of esters and higher alcohols produced by diploid strains (AY-B1, AY-SB1, AY-D1B2, and AY-SD1B2) with high TTMP production were not negatively affected. This study provides an important reference for the application genetic engineering to S. cerevisiae in order to increase the yield of TTMP in Baijiu and other alcoholic beverages, thereby improving their beneficial health attributes.
Data Availability Statement
The original contributions presented in the study are included in the article/Supplementary Material, further inquiries can be directed to the corresponding author.
Author Contributions
D-YC, D-GX, and C-YZ conceived and designed research. D-YC, Y-NW, and P-PF conducted experiments. L-CL, XL, and C-YZ analyzed data. D-YC and S-JC wrote the manuscript. D-GX, L-CL, and C-YZ revised and reviewed the manuscript. All authors read and approved the manuscript.
Funding
The current study was financially supported by the National Key Research and Development Program of China (2016YFD0400500), National Natural Science Foundation of China (31571809), the Key Laboratory of Wuliangye-flavor Liquor Solid-state Fermentation, China National Light Industry (No. 2019JJ005), Public Service Platform Project for Selection and Fermentation Technology of Industrial Microorganisms (17PTGCCX00190) and the Innovative Research Team of Tianjin Municipal Education Commission (TD13-5013). The Project Supported by the Foundation (No. 2018KF001) of Key Laboratory of Industrial Fermentation Microbiology of Ministry of Education and Tianjin Key Lab of Industrial Microbiology (Tianjin University of Science & Technology).
Conflict of Interest
The authors declare that the research was conducted in the absence of any commercial or financial relationships that could be construed as a potential conflict of interest.
Supplementary Material
The Supplementary Material for this article can be found online at: https://www.frontiersin.org/articles/10.3389/fmicb.2020.596306/full#supplementary-material
Supplementary Figure 1 | Growth curve of haploid strains and diploid strains.
Supplementary Table 1 | Primers used in this study.
Supplementary Table 2 | The recombinant fragments and corresponding PCR primers.
References
Bae, S. J., Kim, S., and Hahn, J. S. (2016). Efficient production of acetoin in Saccharomyces cerevisiae by disruption of 2,3-butanediol dehydrogenase and expression of NADH oxidase. Sci. Rep. 6:27667. doi: 10.1038/srep27667
Bartowsky, E. J., and Henschke, P. A. (2004). The ‘buttery’ attribute of wine–diacetyl–desirability, spoilage and beyond. Int. J. Food Microbiol. 96, 235–252. doi: 10.1016/j.ijfoodmicro.2004.05.013
Brat, D., Weber, C., Lorenzen, W., Bode, H. B., and Boles, E. (2012). Cytosolic re-localization and optimization of valine synthesis and catabolism enables increased isobutanol production with the yeast Saccharomyces cerevisiae. Biotechnol. Biofuels 5:65. doi: 10.1186/1754-6834-5-65
Calam, E., González-Roca, E., Fernández, M. R., Dequin, S., Parés, X., Virgili, A., et al. (2016). Enantioselective synthesis of vicinal (R,R)-diols by Saccharomyces cerevisiae butanediol dehydrogenase. Appl. Environ. Microbiol. 82, 1706–1721. doi: 10.1128/aem.03717-15
Cao, J., Miao, Q., Miao, S., Bi, L., Zhang, S., Yang, Q., et al. (2015). Tetramethylpyrazine (TMP) exerts antitumor effects by inducing apoptosis and autophagy in hepatocellular carcinoma. Int. Immunopharmacol. 26, 212–220. doi: 10.1016/j.intimp.2015.03.028
Chen, J. C., Chen, Q. H., Guo, Q., Ruan, S., Hui, R., He, G. Q., et al. (2010). Simultaneous determination of acetoin and tetramethylpyrazine in traditional vinegars by HPLC method. Food Chem. 122, 1247–1252.
Chen, Y., Lu, W., Yang, K., Duan, X., Li, M., Chen, X., et al. (2020). Tetramethylpyrazine, a promising drug for treatment of pulmonary hypertension. Br. J. Pharmacol. 177, 2743–2764. doi: 10.1111/bph.15000
Choi, E. J., Kim, J. W., Kim, S. J., Seo, S. O., Lane, S., Park, Y. C., et al. (2016). Enhanced production of 2,3-butanediol in pyruvate decarboxylase-deficient Saccharomyces cerevisiae through optimizing ratio of glucose/galactose. Biotechnol. J. 11, 1424–1432. doi: 10.1002/biot.201600042
Cui, D. Y., Zhang, Y., Xu, J., Zhang, C. Y., Li, W., and Xiao, D. G. (2018). PGK1 promoter library for the regulation of acetate ester production in Saccharomyces cerevisiae during Chinese Baijiu fermentation. J. Agric. Food Chem. 66, 7417–7427. doi: 10.1021/acs.jafc.8b02114
Dasari, S., and Kölling, R. (2011). Cytosolic localization of acetohydroxyacid synthase Ilv2 and its impact on diacetyl formation during beer fermentation. Appl. Environ. Microbiol. 77, 727–731. doi: 10.1128/aem.01579-10
Du, H., Fan, W., and Xu, Y. (2011). Characterization of geosmin as source of earthy odor in different aroma type Chinese liquors. J. Agric. Food Chem. 59, 8331–8337. doi: 10.1021/jf201171b
Dulieu, C., and Poncelet, D. (1999). Spectrophotometric assay of α-acetolactate decarboxylase. Enzyme Microb. Technol. 25, 537–542. doi: 10.1016/S0141-0229(99)00079-4
Duong, C. T., Strack, L., Futschik, M., Katou, Y., Nakao, Y., Fujimura, T., et al. (2011). Identification of Sc-type ILV6 as a target to reduce diacetyl formation in lager brewers’ yeast. Metab. Eng. 13, 638–647. doi: 10.1016/j.ymben.2011.07.005
Ehsani, M., Fernández, M. R., Biosca, J. A., Julien, A., and Dequin, S. (2009). Engineering of 2,3-butanediol dehydrogenase to reduce acetoin formation by glycerol-overproducing, low-alcohol Saccharomyces cerevisiae. Appl. Environ. Microbiol. 75, 3196–3205. doi: 10.1128/aem.02157-08
Espinosa-Cantú, A., Ascencio, D., Herrera-Basurto, S., Xu, J., Roguev, A., and Krogan, N. J. (2018). Protein moonlighting revealed by noncatalytic phenotypes of yeast enzymes. Genetics 208, 419–431. doi: 10.1534/genetics.117.300377
Fan, W., Xu, Y., and Zhang, Y. (2007). Characterization of pyrazines in some Chinese liquors and their approximate concentrations. J. Agric. Food Chem. 55, 9956–9962. doi: 10.1021/jf071357q
Gibson, B., Krogerus, K., Ekberg, J., Monroux, A., Mattinen, L., Rautio, J., et al. (2015). Variation in α-acetolactate production within the hybrid lager yeast group Saccharomyces pastorianus and affirmation of the central role of the ILV6 gene. Yeast 32, 301–316. doi: 10.1002/yea.3026
Gibson, B., Vidgren, V., Peddinti, G., and Krogerus, K. (2018). Diacetyl control during brewery fermentation via adaptive laboratory engineering of the lager yeast Saccharomyces pastorianus. J. Ind. Microbiol. Biotechnol. 45, 1103–1112. doi: 10.1007/s10295-018-2087-4
Gietz, R. D., and Woods, R. A. (2002). Transformation of yeast by lithium acetate/single-stranded carrier DNA/polyethylene glycol method. Methods Enzymol. 350, 87–96. doi: 10.1016/s0076-6879(02)50957-5
González, E., Fernández, M. R., Larroy, C., Parés, X., and Biosca, J. A. (2001). Characterization and functional role of Saccharomyces cerevisiae 2,3-butanediol dehydrogenase. Chem. Biol. Interact. 130-132, 425–434. doi: 10.1016/s0009-2797(00)00282-9
González, E., Fernández, M. R., Larroy, C., Solà, L. S., Pericàs, M. A., Parés, X., et al. (2000). Characterization of a (2R,3R)-2,3-butanediol dehydrogenase as the Saccharomyces cerevisiae YAL060W gene product disruption and induction of the gene. J. Biol. Chem. 275, 35876–35885. doi: 10.1074/jbc.M003035200
González, E., Fernández, M. R., Marco, D., Calam, E., Sumoy, L., Parés, X., et al. (2010). Role of Saccharomyces cerevisiae oxidoreductases Bdh1p and Ara1p in the metabolism of acetoin and 2,3-butanediol. Appl. Environ. Microbiol. 76, 670–679. doi: 10.1128/AEM.01521-09
Gueldener, U., Heinisch, J., Koehler, G. J., Voss, D., and Hegemann, J. H. (2002). A second set of loxP marker cassettes for Cre-mediated multiple gene knockouts in budding yeast. Nucleic Acids Res. 30:e23. doi: 10.1093/nar/30.6.e23
Hao, F., Wu, Q., and Xu, Y. (2013). Precursor supply strategy for tetramethylpyrazine production by Bacillus subtilis on solid-state fermentation of wheat bran. Appl. Biochem. Biotechnol. 169, 1346–1352. doi: 10.1007/s12010-012-0083-0
Humia, B. V., Santos, K. S., Barbosa, A. M., Sawata, M., Mendonça, M. D. C., and Padilha, F. F. (2019). Beer molecules and its sensory and biological properties: a review. Molecules 24:1568. doi: 10.3390/molecules24081568
Ishida, Y., Nguyen, T., and Izawa, S. (2017). The yeast ADH7 promoter enables gene expression under pronounced translation repression caused by the combined stress of vanillin, furfural, and 5-hydroxymethylfurfural. J. Biotechnol. 252, 65–72. doi: 10.1016/j.jbiotec.2017.04.024
Ishida, Y., Nguyen, T. T., Kitajima, S., and Izawa, S. (2016). Prioritized expression of BDH2 under bulk translational repression and its contribution to tolerance to severe vanillin stress in Saccharomyces cerevisiae. Front. Microbiol. 7:1059. doi: 10.3389/fmicb.2016.01059
Jia, X., Liu, Y., and Han, Y. (2017). A thermophilic cell-free cascade enzymatic reaction for acetoin synthesis from pyruvate. Sci. Rep. 7:4333. doi: 10.1038/s41598-017-04684-8
Kim, S., and Hahn, J. S. (2015). Efficient production of 2,3-butanediol in Saccharomyces cerevisiae by eliminating ethanol and glycerol production and redox rebalancing. Metab. Eng. 31, 94–101. doi: 10.1016/j.ymben.2015.07.006
Kim, S. J., Kim, J. W., Lee, Y. G., Park, Y. C., and Seo, J. H. (2017). Metabolic engineering of Saccharomyces cerevisiae for 2,3-butanediol production. Appl. Microbiol. Biotechnol. 101, 2241–2250. doi: 10.1007/s00253-017-8172-1
Kim, S. J., Seo, S. O., Jin, Y. S., and Seo, J. H. (2013). Production of 2,3-butanediol by engineered Saccharomyces cerevisiae. Bioresour. Technol. 146, 274–281. doi: 10.1016/j.biortech.2013.07.081
Kim, S. J., Seo, S. O., Park, Y. C., Jin, Y. S., and Seo, J. H. (2014). Production of 2,3-butanediol from xylose by engineered Saccharomyces cerevisiae. J. Biotechnol. 192, 376–382. doi: 10.1016/j.jbiotec.2013.12.017
Kuang, X., Ouyang, Y., Guo, Y., Li, Q., Wang, H., Abrha, G. T., et al. (2020). New insights into two yeast BDHs from the PDH subfamily as aldehyde reductases in context of detoxification of lignocellulosic aldehyde inhibitors. Appl. Microbiol. Biotechnol. 104, 6679–6692. doi: 10.1007/s00253-020-10722-9
Li, P., Gao, Y., Wang, C., Zhang, C. Y., Guo, X., and Xiao, D. (2018). Effect of ILV6 deletion and expression of aldB from Lactobacillus plantarum in Saccharomyces uvarum on diacetyl production and wine flavor. J. Agric. Food Chem. 66, 8556–8565. doi: 10.1021/acs.jafc.8b02356
Li, P., Guo, X., Shi, T., Hu, Z., Chen, Y., Du, L., et al. (2017). Reducing diacetyl production of wine by overexpressing BDH1 and BDH2 in Saccharomyces uvarum. J. Ind. Microbiol. Biotechnol. 44, 1541–1550. doi: 10.1007/s10295-017-1976-2
Li, W., Chen, S. J., Wang, J. H., Zhang, C. Y., Shi, Y., Guo, X. W., et al. (2018). Genetic engineering to alter carbon flux for various higher alcohol productions by Saccharomyces cerevisiae for Chinese Baijiu fermentation. Appl. Microbiol. Biotechnol. 102, 1783–1795. doi: 10.1007/s00253-017-8715-5
Li, W., Wang, J. H., Zhang, C. Y., Ma, H. X., and Xiao, D. G. (2017). Regulation of Saccharomyces cerevisiae genetic engineering on the production of acetate esters and higher alcohols during Chinese Baijiu fermentation. J. Ind. Microbiol. Biotechnol. 44, 1–12. doi: 10.1007/s10295-017-1907-2
Liu, H., and Sun, B. (2018). Effect of fermentation processing on the flavor of Baijiu. J. Agric. Food Chem. 66, 5425–5432. doi: 10.1021/acs.jafc.8b00692
Meng, W., Ding, F., Wang, R. M., and Wang, T. F. (2020). Enhanced production of tetramethylpyrazine in Bacillus licheniformis BL1 through aldC over-expression and acetaldehyde supplementation. Sci. Rep. 10:3544. doi: 10.1038/s41598-020-60345-3
Meng, W., Xiao, D., and Wang, R. (2016). Enhanced production of tetramethylpyrazine in Bacillus licheniformis BL1 by bdhA disruption and 2,3-butanediol supplementation. World J. Microbiol. Biotechnol. 32:46. doi: 10.1007/s11274-015-1992-1
Murashchenko, L., Abbas, C., Dmytruk, K., and Sibirny, A. (2016). Overexpression of the truncated version of ILV2 enhances glycerol production in Saccharomyces cerevisiae. Yeast 33, 463–469. doi: 10.1002/yea.3161
Omura, F. (2008). Targeting of mitochondrial Saccharomyces cerevisiae Ilv5p to the cytosol and its effect on vicinal diketone formation in brewing. Appl. Microbiol. Biotechnol. 78, 503–513. doi: 10.1007/s00253-007-1333-x
Oud, B., Flores, C. L., Gancedo, C., Zhang, X., Trueheart, J., Daran, J. M., et al. (2012). An internal deletion in MTH1 enables growth on glucose of pyruvate-decarboxylase negative, non-fermentative Saccharomyces cerevisiae. Microb. Cell Fact. 11:131. doi: 10.1186/1475-2859-11-131
Park, S. H., and Hahn, J. S. (2019). Development of an efficient cytosolic isobutanol production pathway in Saccharomyces cerevisiae by optimizing copy numbers and expression of the pathway genes based on the toxic effect of α-acetolactate. Sci. Rep. 9:3996. doi: 10.1038/s41598-019-40631-5
Rizzi, G. P. (1988). Formation of pyrazines from acyloin precursors under mild conditions. J. Agric. Food Chem. 36, 349–352. doi: 10.1021/jf00080a026
Shi, T. T., Li, P., Chen, S. J., Chen, Y. F., Guo, X. W., and Xiao, D. G. (2017). Reduced production of diacetyl by overexpressing BDH2 gene and ILV5 gene in yeast of the lager brewers with one ILV2 allelic gene deleted. J. Ind. Microbiol. Biotechnol. 44, 397–405. doi: 10.1007/s10295-017-1903-6
Shi, T. T., Li, P., Zhou, Z., and Xiao, D. G. (2016). Diacetyl content reduction in industrial brewer’s yeast through ILV2 disruption and BDH1 expression. Eur. Food Res. Technol. 242, 919–926. doi: 10.1007/s00217-015-2598-4
Shin, J. W., Moon, J. Y., Seong, J. W., Song, S. H., Cheong, Y. J., Kang, C., et al. (2013). Effects of tetramethylpyrazine on microglia activation in spinal cord compression injury of mice. Am. J. Chin. Med. 41, 1361–1376. doi: 10.1142/s0192415x13500912
Takpho, N., Watanabe, D., and Takagi, H. (2018). High-level production of valine by expression of the feedback inhibition-insensitive acetohydroxyacid synthase in Saccharomyces cerevisiae. Metab. Eng. 46, 60–67. doi: 10.1016/j.ymben.2018.02.011
van Bergen, B., Cyr, N., Strasser, R., Blanchette, M., Sheppard, J. D., and Jardim, A. (2016). α,β-Dicarbonyl reduction is mediated by the Saccharomyces Old Yellow Enzyme. FEMS Yeast Res. 16:fow059. doi: 10.1093/femsyr/fow059
Wang, X., Fan, W., and Xu, Y. (2014). Comparison on aroma compounds in Chinese soy sauce and strong aroma type liquors by gas chromatography–olfactometry, chemical quantitative and odor activity values analysis. Eur. Food Res. Technol. 239, 813–825. doi: 10.1007/s00217-014-2275-z
Wess, J., Brinek, M., and Boles, E. (2019). Improving isobutanol production with the yeast by successively blocking competing metabolic pathways as well as ethanol and glycerol formation. Biotechnol. Biofuels 12:173. doi: 10.1186/s13068-019-1486-8
Xiao, Z., Hou, X., Xin, L., Xi, L., and Zhao, J. (2014). Accelerated green process of tetramethylpyrazine production from glucose and diammonium phosphate. Biotechnol. Biofuels 7:106. doi: 10.1186/1754-6834-7-106
Xiao, Z., Zhao, L., Tian, L., Wang, L., and Zhao, J. Y. (2018). GC-FID determination of tetramethylpyrazine and acetoin in vinegars and quantifying the dependence of tetramethylpyrazine on acetoin and ammonium. Food Chem. 239:726. doi: 10.1016/j.foodchem.2017.07.015
Xiao, Z. J., Xie, N. Z., Liu, P. H., Hua, D. L., and Xu, P. (2006). Tetramethylpyrazine production from glucose by a newly isolated Bacillus mutant. Appl. Microbiol. Biotechnol. 73, 512–518. doi: 10.1007/s00253-006-0491-6
Xu, Y., Jiang, Y., Li, X., Sun, B., Teng, C., Yang, R., et al. (2018). Systematic characterization of the metabolism of acetoin and its derivative ligustrazine in Bacillus subtilis under micro-oxygen conditions. J. Agric. Food Chem. 66, 3179–3187. doi: 10.1021/acs.jafc.8b00113
Yang, Z., and Zhang, Z. (2018). Production of (2R, 3R)-2,3-butanediol using engineered Pichia pastoris: strain construction, characterization and fermentation. Biotechnol. Biofuels 11:35. doi: 10.1186/s13068-018-1031-1
Zhang, L., Cao, Y., Tong, J., and Xu, Y. (2019). An alkylpyrazine synthesis mechanism involving l-threonine-3-dehydrogenase describes the production of 2,5-dimethylpyrazine and 2,3,5-trimethylpyrazine by Bacillus subtilis. Appl. Environ. Microbiol. 85, e1807–e1819. doi: 10.1128/aem.01807-19
Zhong, H., Shen, J., Meng, Z., Zhao, J. Y., and Xiao, Z. (2020). Tetramethylpyrazine production from edible materials by the probiotic Bacillus coagulans. Prep. Biochem. Biotechnol. 50, 935–942. doi: 10.1080/10826068.2020.1774777
Zhu, B. F., Xu, Y., and Fan, W. L. (2010). High-yield fermentative preparation of tetramethylpyrazine by Bacillus sp. using an endogenous precursor approach. J. Ind. Microbiol. Biotechnol. 37, 179–186. doi: 10.1007/s10295-009-0661-5
Keywords: Saccharomyces cerevisiae, 2, 3, 5, 6-tetramethylpyrazine, acetoin, Baijiu, BDH1, BDH2
Citation: Cui D-Y, Wei Y-N, Lin L-C, Chen S-J, Feng P-P, Xiao D-G, Lin X and Zhang C-Y (2020) Increasing Yield of 2,3,5,6-Tetramethylpyrazine in Baijiu Through Saccharomyces cerevisiae Metabolic Engineering. Front. Microbiol. 11:596306. doi: 10.3389/fmicb.2020.596306
Received: 19 August 2020; Accepted: 28 October 2020;
Published: 26 November 2020.
Edited by:
Xucong Lv, Fuzhou University, ChinaReviewed by:
Yi Wang, Auburn University, United StatesChoowong Auesukaree, Mahidol University, Thailand
Liaoyuan Zhang, Fujian Agriculture and Forestry University, China
Copyright © 2020 Cui, Wei, Lin, Chen, Feng, Xiao, Lin and Zhang. This is an open-access article distributed under the terms of the Creative Commons Attribution License (CC BY). The use, distribution or reproduction in other forums is permitted, provided the original author(s) and the copyright owner(s) are credited and that the original publication in this journal is cited, in accordance with accepted academic practice. No use, distribution or reproduction is permitted which does not comply with these terms.
*Correspondence: Cui-Ying Zhang, cyzhangcy@tust.edu.cn