- 1Key Laboratory of Coastal Environmental Processes and Ecological Remediation, Yantai Institute of Coastal Zone Research, Chinese Academy of Sciences, Yantai, China
- 2College of Resources and Environment, University of Chinese Academy of Sciences, Beijing, China
- 3Department of Microbiology and Environmental Toxicology, University of California, Santa Cruz, Santa Cruz, CA, United States
- 4Center for Ocean Mega-Science, Chinese Academy of Sciences, Qingdao, China
- 5National Engineering Laboratory for Efficient Utilization of Soil and Fertilizer Resources, College of Resources and Environment of Shandong Agricultural University, Taian, China
Chemotaxis is essential for the competitiveness of motile bacteria in complex and harsh environments. The localization of chemotactic proteins in the cell is critical for coordinating a maximal response to chemotactic signals. One chemotaxis protein with a well-defined subcellular localization is the phosphatase CheZ. CheZ localizes to cell poles by binding with CheA in Escherichia coli and other enteric bacteria, or binding with a poorly understood protein called ChePep in epsilon-Proteobacteria. In alpha-Proteobacteria, CheZ lacks CheA-binding sites, and its cellular localization remains unknown. We therefore determined the localization of CheZ in the alpha-Proteobacteria Azorhizobium caulinodans ORS571. A. caulinodans CheZ, also termed as CheZAC, was found to be located to cell poles independently of CheA, and we suspect that either the N-terminal helix or the four-helix bundle of CheZAC is sufficient to locate to cell poles. We also found a novel motif, AXXFQ, which is adjacent to the phosphatase active motif DXXXQ, which effects the monopolar localization of CheZAC. This novel motif consisting of AXXFQ is conserved in CheZ and widely distributed among Proteobacteria. Finally, we found that the substitution of phosphatase active site affects the polar localization of CheZAC. In total, this work characterized the localization pattern of CheZ containing a novel motif, and we mapped the regions of CheZAC that are critical for its polar localization.
Introduction
In harsh and complex environments, bacteria must adapt and respond to external changes quickly. Chemotaxis systems are one-way bacteria have envolved to do this. Chemotaxis enables bacteria to regulate their motility in response to environmental signals. The chemotaxis pathway has been well studied in Escherichia coli. External signals or nutrient molecules are sensed by chemoreceptors. Upon binding with attractant signals, conformational changes of chemoreceptors inhibit the autokinase activity of the associated histidine kinase CheA. In the presents of a repellent signal, CheA can phosphorylate the response regulator CheY, and CheY-P diffuses and binds with the flagellar motor proteins FliM and FliN, causing the flagella to change rotational direction from counterclockwise to clockwise (Szurmant and Ordal, 2004). The phosphatase CheZ promotes the intrinsic dephosphorylation of CheY-P to terminate the signal transduction (Blat and Eisenbach, 1994; Silversmith et al., 2008; Silversmith, 2010).
The spatial organization of chemotaxis proteins is critical for bacterial chemotaxis to adapt to environments. Chemotaxis proteins are localized to cellular poles using multiple strategies, including the nucleoid occlusion, Tol/Pal complex, membrane curvature, and protein-protein interactions (Laloux and Jacobs-Wagner, 2014). Transmembrane E. coli chemoreceptors maintain polar localization through the Tol/Pal complex, strong membrane curvature, or nucleoid exclusion (Santos et al., 2014; Neeli-Venkata et al., 2016; Saaki et al., 2018) The Tol/Pal complex is a conserved component of bacterial cell envelope, which is involved in the maintenance of cell wall integrity (Bernadac et al., 1998). Other chemotaxis proteins including CheA, CheW, CheY, and CheZ locate to cellular poles based on the interaction with other chemotaxis proteins (Sourjik and Berg, 2000). CheA and CheW can bind to chemoreceptor forming polar chemotaxis complexes (Pinas et al., 2016), and the localization of CheZ and CheY depends on the presence of CheA in E. coli (Sourjik and Berg, 2000).
CheZ is encoded in around 40% of bacterial genomes (Wuichet and Zhulin, 2010), and the localization mechanism of CheZ has been well studied in E. coli. E. coli CheZ, termed as CheZEC, locates to cellular poles with the help of CheA-short (CheAs), a short form of CheA lacking the first 97 amino residues of full length CheA, called CheA-long. CheZEC interacts with CheA using a small region of amino acids with most interactions coming from the apical hairpin loop consisting of two aromatic residues, Phe-97 and Trp-98 (Cantwell and Manson, 2009). For CheAs, two hydrophobic residues Leu-123 and Leu-126 in the N-terminus of CheA are responsible for CheZEC interactions (Cantwell et al., 2003; Hao et al., 2009).
Azorhizobium caulinodans ORS571 is a rhizobium belonging to alpha-Proteobacteria uses chemotaxis for plant colonization. It fixes nitrogen with the host Sesbania rostrata by forming stem or root nodules (Dreyfus et al., 1983). A. caulinodans ORS571 has only one chemotaxis pathway including one gene cluster (cheA, cheW, cheY2, cheB, and cheR) and two orphan genes (cheY1 and cheZ) (Jiang et al., 2016). Deletion of one or several genes within the A. caulinodans ORS571 chemotaxis cluster reduces or abolishes the chemotaxis of A. caulinodans ORS571, confirming the role of these genes in chemotaxis (Liu W. et al., 2018; Liu X. et al., 2018). Deletion of cheZ causes A. caulinodans non-chemotactic, while in contrast to other chemotaxis proteins which are important for host plant colonization, CheZ plays negative roles on early colonization (Liu et al., 2019). We previously found that a soluble heme-binding chemotaxis protein in A. caulinodans locates at the cell poles with the help of CheA (Jiang et al., 2016). However, it has been reported that CheZ proteins in alpha- and delta-Proteobacteria lack the sequences responsible for CheAs binding (Wuichet et al., 2007), and the localization of CheZ in alpha-Proteobacteria remains unknown.
In the present study, we reported the localization pattern of CheZ in A. caulinodans ORS571 and mapped regions of CheZAC that are sufficient for polar localization by constructing various truncated mutants of CheZAC. Furthermore, a novel motif in CheZAC, which is conserved among Proteobacteria, was found to be involved in the regulation of monopolar CheZ localization.
Results
Bioinformatics Analysis Shows that CheZAC Lacks Canonical Sites Involved in CheZEC Polar Localization
The structure of CheZEC consists of four regions, including an N-terminal helix (residues 1–34) of unknown functions, a four-helix bundle formed from a dimer of two hairpin structures (residues 35–168), a linker (residues 169–199), and a C-terminal helix (residues 199–214) (Zhao et al., 2002; Silversmith, 2005). Because the amino acid sites involved in the CheZEC polar localization were well studied, we first aligned the CheZ amino acid sequences from E. coli (CheZEC) and A. caulinodans (CheZAC) using an EMBOSS Needle program (Madeira et al., 2019). Then, we modeled the structure of CheZAC using online server SWISS-MODEL (Waterhouse et al., 2018) and Jpred4 (Drozdetskiy et al., 2015).
An alignment of CheZAC and CheZEC proteins showed significant similarity (33.9%) between them and both of them have conserved phosphatase active sites (Asp 165 and Gln 169 in CheZAC) (Figure 1A), which is consistent with our previous report (Liu X. et al., 2018). Structure modeling results (Figures 1A,B) suggested CheZAC also has the N-terminal helix (residues 1–86 in CheZAC), four-helix bundle hairpin (residues 87–195 in CheZAC), the linker (residues 196–225 in CheZAC), and the C-terminal helix (residues 225–236 in CheZAC). Interestingly, the N-terminal helix in CheZAC is substantially longer (∼50 residues) than CheZEC, while the four-helix bundle hairpin (∼25 residues) in CheZAC is substantially shorter than CheZEC (Figure 1). And, the gaps in the alignment are similar in size on either side of the hairpin turn (residues ∼140), which is consistent with a shorter bundle (Figures 1A,B). Remaining of residues from 70 to 133 including the tip of hairpin (residues ∼100) is sufficient for polar localization of CheZEC (Cantwell and Manson, 2009). The similarity of the hairpin tip between CheZAC and CheZEC indicates that the hairpin tip might be also employed by CheZAC to bind potential localization partner proteins. However, a conserved motif D(D/E)WF (residues 95–98) (Cantwell et al., 2003), which is important for CheZEC polar localization, was not found in CheZAC (Figure 1A).
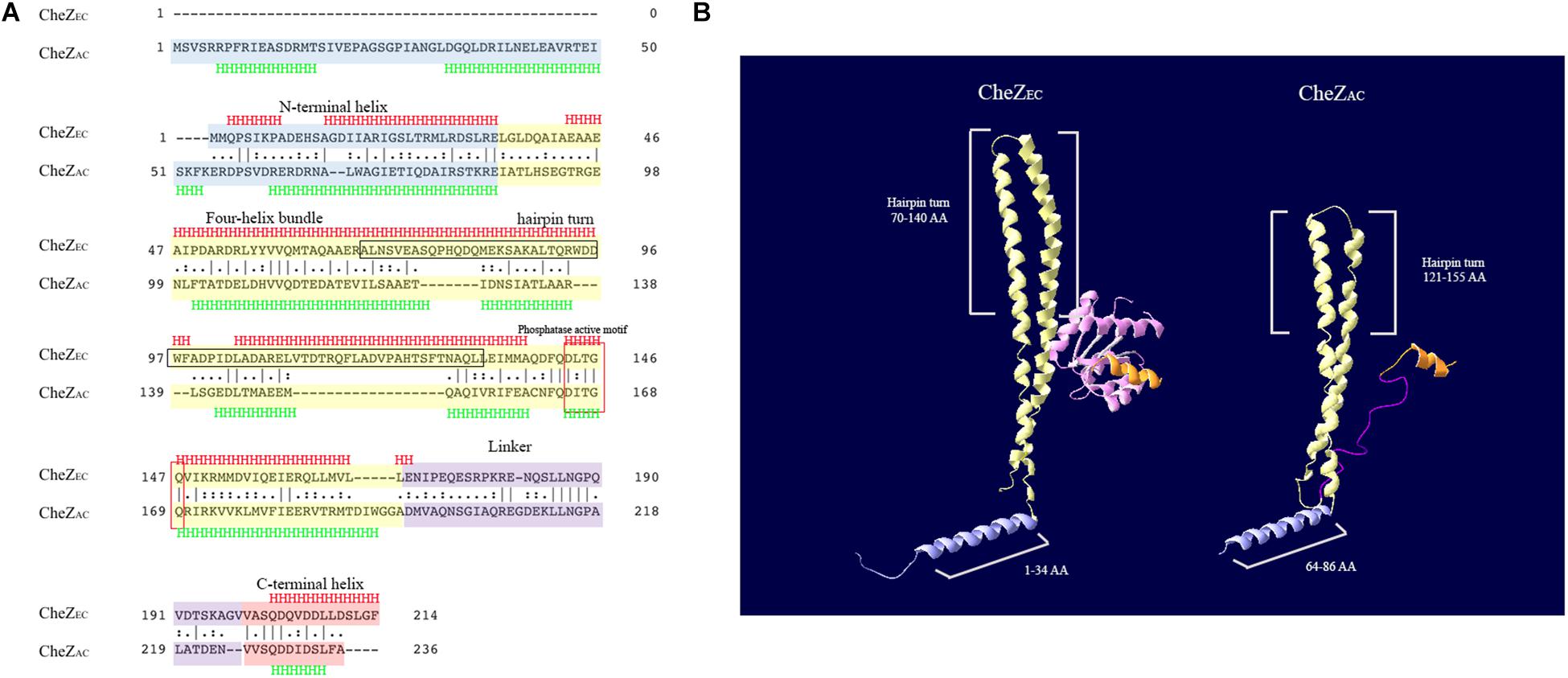
Figure 1. Bioinformatics analysis of CheZEC and CheZAC. (A) The alignment of CheZEC and CheZAC was generated using the default settings of EMBOSS Needle software from EMBL. The N-terminal helix, four-helix bundle, linker, and C-terminus of CheZs were marked with blue, yellow, purple, and red color, respectively. The region containing hairpin turn, which is sufficient for localization of CheZEC, was marked with black frame. The secondary structure was predicted with Jpred4 program. (B) Structure overview of CheZEC (1KMI) and CheZAC. The N-terminal helix, four-helix bundle, linker, and C-terminus of CheZs were recolored as above. CheY (marked with pink) was shown in the structure of CheZEC and the linker region was not shown in CheZEC. The structure model of CheZAC was produced by SWISS-MODEL program, and the first 50 amino acids (involved in its N-terminal helix) were not shown.
In E. coli, the polar localization of CheZ is achieved by binding with a short form of CheA (CheAEC), which begins at Met-98 of full length CheAEC (Cantwell et al., 2003; Hao et al., 2009). There is only one CheA protein encoded in A. caulinodans genome, termed as CheAAC. When we made a pairwise sequence alignment of CheAEC and CheAAC, the absence of cognate CheAEC Met-98 in CheAAC suggests that CheAAC does not have a short form of CheA (Supplementary Figure S1). These results suggest that CheZAC may not locate to cell poles or locate to cell poles with a different mechanism from CheZEC.
Characteristics of CheZ Localization in A. caulinodans ORS571
To study the subcellular localization of CheZ in A. caulinodans cells, we designed a C-terminal GFP fusion to CheZAC. To avoid artifacts related overexpression, the expression of the fusion gene was controlled by the native promoter of cheZ (Liu X. et al., 2018). When the CheZAC fusion was expressed in the cheZ mutant strain, the chemotactic behavior of the cheZ null mutant was partially complemented to wild-type levels that contains a control vector pBBR2GFP, indicating the CheZ-GFP retains function (Figure 2A). Another evidence showing that CheZ-GFP functions is that the presence of CheZ-GFP restores ΔcheZ flagella rotating to wild-type level. Wild type and ΔcheZ complemental strains both rotate their flagella between clockwise and counter-clockwise, while ΔcheZ with or without pBBR2GFP always shows counter-clockwise rotation (Unpublished data).
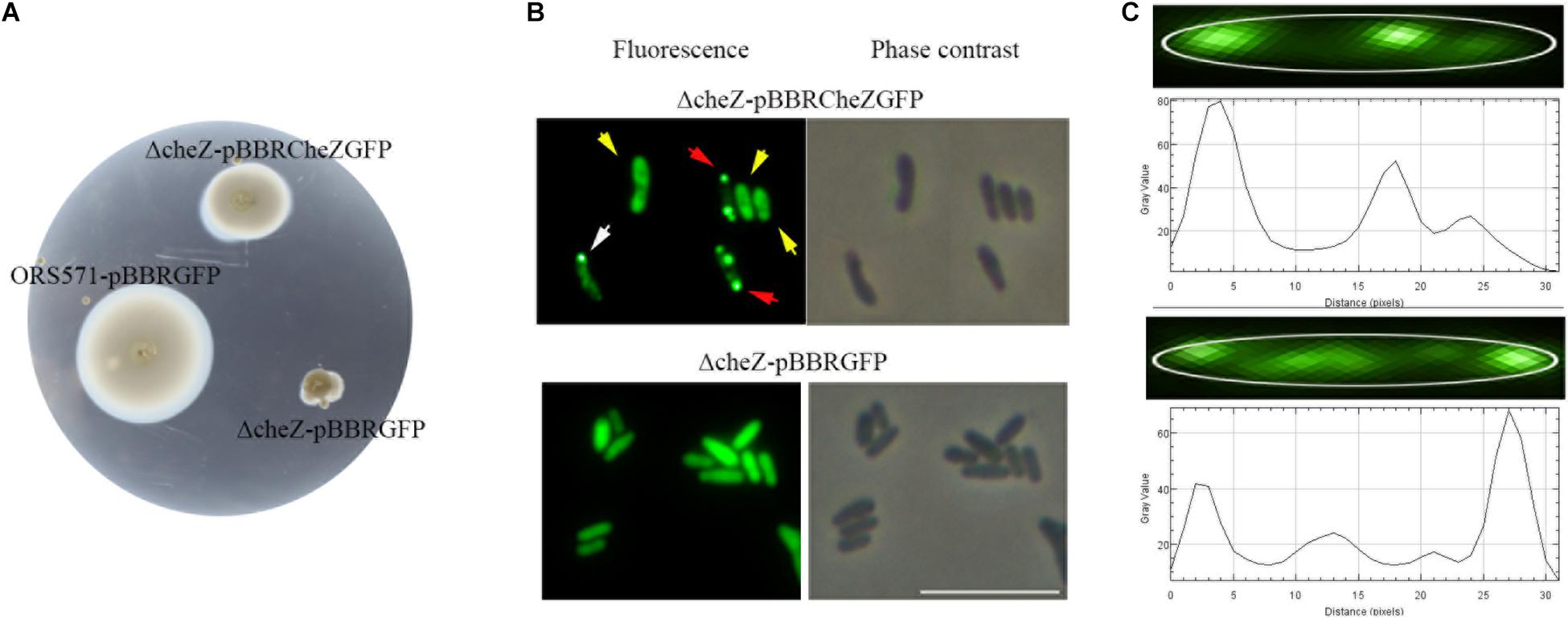
Figure 2. Cellular localization of CheZ in A. caulinodans ORS571. The plasmid pBBR2CheZ-GFP containing native promoter can restore the chemotaxis deficiency of cheZ mutant (A). The sublocalization of CheZAC is heterogenous including diffuse (indicated with yellow arrows), monopolar (indicated with white arrows), and bipolar (red arrows) localization (B). The spatial distribution of CheZ clusters (C), and the edge of cell body was outlined with white line. The gray value peaks correspond to the GFP spots of CheZ clusters. The length of the cell matched with the length of the x-axis. The scale bar in panel B represents 10 μm.
We next determined the spatial distribution of CheZ-GFP by fluorescence microscopy. The CheZ-GFP fusion showed three unique localization patterns (Figure 2B). We manually determined and quantified these localization patterns using ImageJ, comparing the brightness of polar foci with that of cell body (see Materials and Methods). About 60% of cells demonstrated diffuse CheZ-GFP localization, 30% of cells CheZ-GFP localized to both cell poles, and 10% of cells showed monopolar localization (Figure 3B). The percent of cells with polar localized CheZ in A. caulinodans are significantly lower than that in E. coli, in which CheZEC shows polar localization in 85% of the cells (Blat and Eisenbach, 1996). Except polar and diffuse localized pattern, there were also many lateral clusters of CheZ-GFP (Figure 2C). When analyzing the distance from each lateral cluster to the pole of cell, the position of each lateral cluster distributed along the cell body with a period corresponding to the 1/2 or 1/4 of the cell length (Figure 2C).
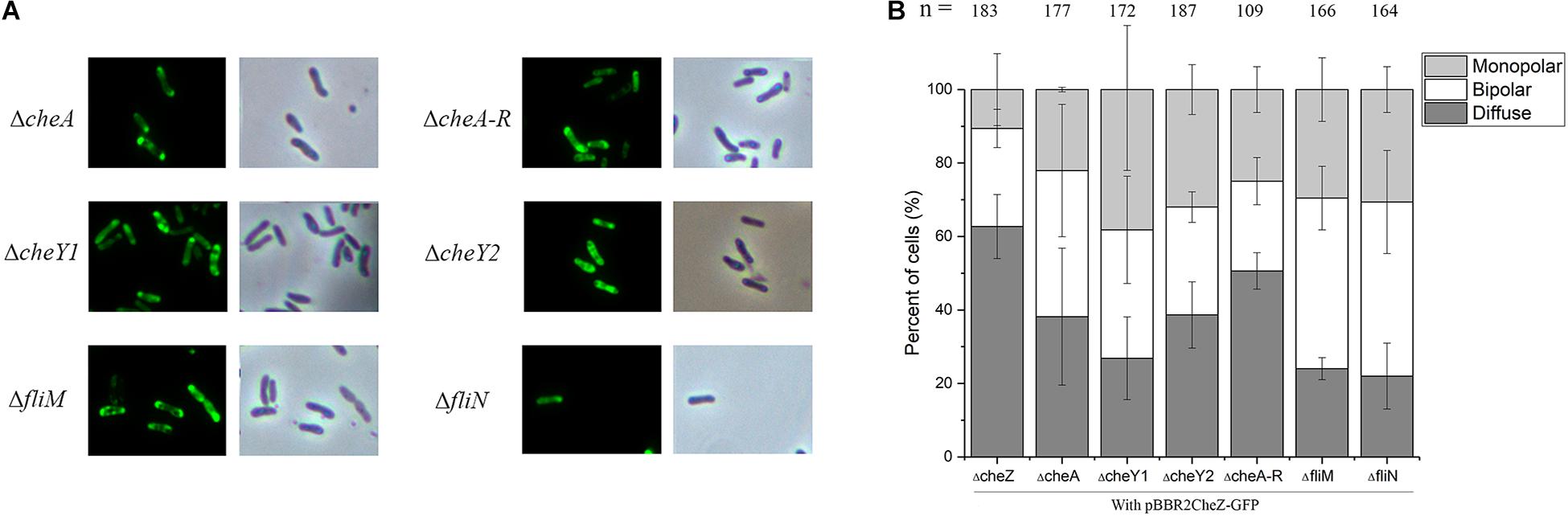
Figure 3. The localization pattern of CheZ in cheA, cheA-R, cheY1, cheY2, fliM, and fliN mutant. The full-length CheZ including its upstream promoter was fused to GFP, and the plasmid containing CheZ-GFP was introduced into each mutant. (A) Representative images of CheZAC localization in each strain. (B) Quantification of cells ratios showed different localized pattern of CheZAC. Values are shown as the means and standard deviations from at least three independent experiments.
The Polar Localization of CheZAC Is Independent of Chemotaxis and Flagellar Proteins
Numerous studies have shown that chemotaxis protein can form a polar cluster to better adapt to environmental conditions. To study whether the polar localization of CheZAC is dependent on CheA or other chemotaxis proteins, we examined the localization patterns of CheZAC in different backgrounds lacking different chemotaxis proteins. Consistent with our bioinformatics analysis, deletion of cheA does not alter the cellular localization of CheZAC (Figure 3). We then tested the localization pattern of CheZAC in the following chemotaxis mutants, ΔcheY1, ΔcheY2, or ΔcheA-R clusters (including cheA, cheY2, cheW, cheB, and cheR) (Liu W. et al., 2018; Liu et al., 2020). Interestingly, CheZAC maintains polar localization in both the ΔcheY1, ΔcheY2, and ΔcheA-R mutants backgrounds (Figure 3).
Next, we tested if flagellar proteins affect CheZAC polar localization. FliM and FliN are two flagellar motor components and interact with CheY either directly or indirectly (Delalez et al., 2014). Deletion of either one makes A. caulinodans non-flagellated and non-motile (Shen et al., 2018), however, neither of them abolished the polar localization of CheZAC (Figure 3). These results indicate that CheZAC is localized to the cell poles independent of chemotaxis or flagellar proteins.
N-terminal Helical Regions Are Sufficient for CheZAC Polar Localization
Although there is low conservation between E. coli and A. caulinodans CheZ, the C-terminal sequences including CheY-P binding region and phosphatase active sites are conserved (Blat and Eisenbach, 1996; Zhao et al., 2002; Wuichet et al., 2007; Silversmith, 2010; Liu X. et al., 2018). The N-terminal helix, whose function remains unknown, and the middle four-helix bundle hairpin of CheZ, which is required for localization in E. coli, are variable (Lertsethtakarn and Ottemann, 2010). To map the region sufficient for polar localization, various portions of the N-terminal helix (residues 1–86), and middle four-helix bundle regions (residues 87–195) of CheZAC were fused to GFP (Figure 4A). CheZΔ51-236, containing a portion of N-terminal helix of CheZAC, failed to localize to the cell poles (Figures 4B,C). Surprisingly, CheZΔ71-236, containing nearly all regions of the N-terminal helix can locate to cellular poles, though the number of cells with bipolar localized CheZAC decreased from 30 to 5% compared to full-length CheZAC (Figures 4B,C). Because the four-helix bundle, especially its hairpin tip, is essential for the polar localized pattern of CheZEC (Hao et al., 2009), we made a longer truncated mutant containing a portion of the four-helix bundle CheZΔ140-236. Intriguingly, CheZΔ140-236 can localize to cell poles, however, the cell ratio showed polar localized pattern decreased no more than 5%. When the remaining residues extended from 1–139 to 1–169, including almost all the region of N-terminal helix and four-helix bundle, the CheZΔ170-236 mutant can locate to mono- or bi-polar poles in cells above 70% (Figure 4). These results suggest that the role of N-terminal helix and four-helix bundle on the polar localization of CheZAC is different from that of CheZEC. The N-terminal helical region is sufficient for polar localization of CheZAC, and the four-helix bundle is involved in the regulation of CheZ polar localization.
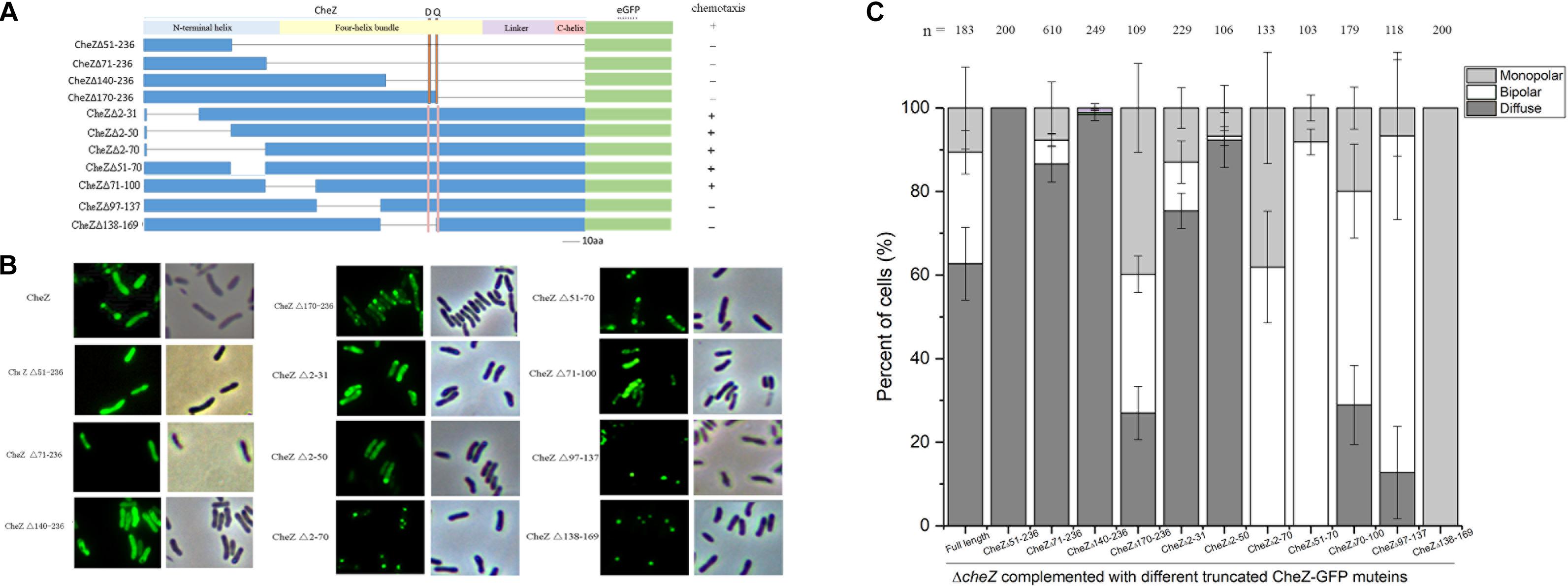
Figure 4. The regions of CheZ involved in the polar localization. The overview of the deleted region in CheZAC and their effects on chemotaxis (A). The truncated CheZ proteins were fused to green fluorescent protein at the carboxyl-terminal ends. The letter “D” and “Q” above the figure indicate the position of the conserved phosphatase motif, DXXXQ, of CheZ. The representative images and quantification of the localized patches of various truncated CheZ in ΔcheZ background (B,C). The “+” and “-” in panel A means strain containing cognate CheZ derivatives can or cannot restore chemotaxis. Values are expressed as means ± standard deviation from at least three independent experiments.
To further determine the regions of CheZ that are responsible for the polar localization in A. caulinodans, CheZAC proteins with various deletions at the N-terminal helix and four-helix bundle were fused to GFP (Figure 4A). All the fusion proteins were introduced into the cheZ mutant and expressed with the native promoter. When part of the CheZAC N-terminal helix was deleted, including residues from 2 to 31 or from 2 to 50 (termed as CheZΔ2-31 and CheZΔ2-50), the truncated mutant maintained polar localization, though the polar localized CheZAC decreased from 40 to 25, and 10%, respectively (Figures 4B,C). Unexpectedly, deletions of N-terminal helical regions from residues 2–70 (CheZΔ2-70) or 51–70 (CheZΔ51-70) did not abolish the polar localization of CheZAC (Figures 4B,C). These results suggest that the region from residues 2–70 might not be the sole region sufficient for polar localization of CheZAC. We further tested the polar localized pattern of mutants lacking part of the four-helix bundle hairpin, CheZΔ71-100, CheZΔ97-137, and CheZΔ138-169, and we found that all of them remained the polar location of CheZAC. These results suggest that CheZAC might be anchored to cell poles via multiple motifs. Interestingly, CheZΔ138-169 not only maintains polar localization, but also shows 100% monopolar localized pattern (Figure 4).
Mining for a Novel Motif Involved in the Regulation of CheZAC Localization
We next sought to identify what protein or residues are responsible for the unique monopolar localization pattern of CheZΔ138-169. When CheZΔ138-169 was introduced into various chemotaxis and flagella mutants, it always maintained nearly 100% monopolar localization (Supplementary Figure S2). These results suggest that the role of residues from 138 to 169 on the localization of CheZAC is not affected by other chemotaxis or flagellar proteins.
To find potential conserved sites required for the monopolar localized pattern of CheZ, we analyzed twenty-five amino acid sequences of CheZ proteins from alpha-Proteobacteria which are closely related to A. caulinodans (Figure 5A). Within residues 138–169, two conserved features were found. One is the conserved phosphatase motif (DXXXQ) (Lertsethtakarn and Ottemann, 2010), and the other is an uncharacterized conserved motif (ACNFQ), which is close to the phosphatase motif (Figures 5B,C). Interestingly, deletion of the ACNFQ motif (CheZΔ158-164) was sufficient to cause the monopolar localized pattern of CheZAC (Figure 5D). To further investigate whether the monopolar localization resulted from the deletion of ACNFQ, three point-directed mutants, A160R, C161A, and F163L, were constructed successfully. The localization pattern of CheZ_C161A and CheZ_F163L were similar with that of wild-type CheZAC, indicating these residues are not required. CheZ_A160R showed different localization that was nearly 100% bipolar (Figure 5E). These results suggest that the novel motif ACNFQ is involved in the monopolar localization of CheZAC and conserved site A160 within the motif might contribute more to the function.
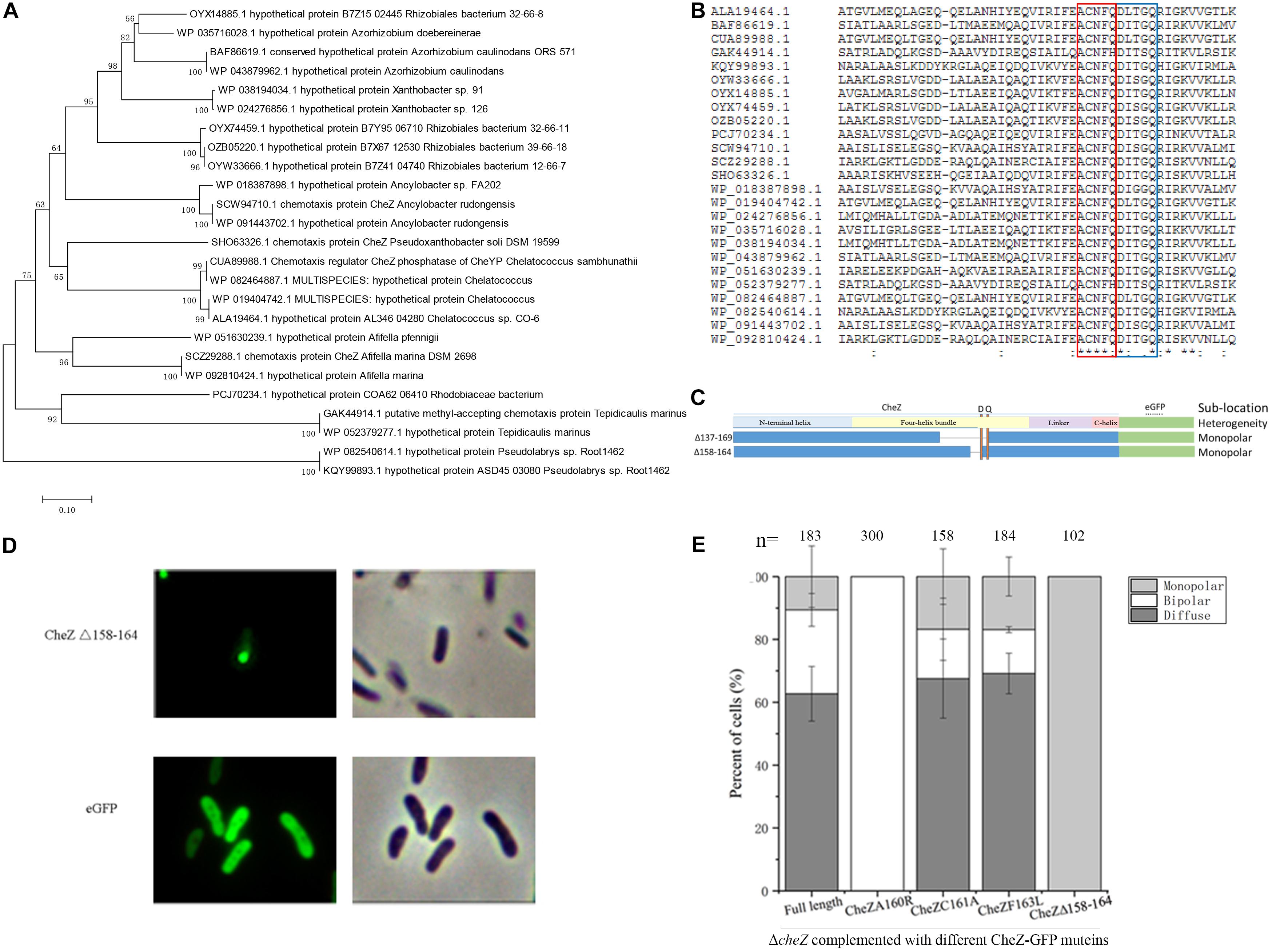
Figure 5. Identification of a novel motif and the effect of some conserved sites of CheZ on polar localization. The phylogenetic tree constructed by twenty-five amino acid sequences of CheZ from species that are closely related to A. caulinodans in alpha-Proteobacteria (A). Multiple alignment of CheZ protein sequences (B). The position of “ACNFQ” and “DXXXQ” motifs are marked with red and blue boxes, respectively. The construction of a motif (ACNFQ) deleted CheZ mutant (C). The letter “D” and “Q” indicate the position of conserved phosphatase motif DXXXQ. The localization pattern of the novel-motif-deleted mutant (D). The roles of other conserved sites on CheZ sublocalization (E). The “-” means strain containing cognate CheZ derivatives cannot restore chemotaxis.
The AXXFQ Motif Is Conserved in Proteobacteria
CheZ distributes broadly among alpha-, beta-, gamma-, delta-, and epsilon-Proteobacteria (Wuichet et al., 2007). Although the degree of identity and similarity between these CheZ proteins are low, the catalytic residues in phosphatase active motif are highly conserved among them (Wuichet et al., 2007). To determine the distribution of the novel motif ACNFQ in Proteobacteria, the representative CheZ sequences from each class (alpha-, beta-, gamma-, delta-, and epsilon- Proteobacteria) were selected for alignment. All these CheZ proteins have the novel ACNFQ motif close to phosphatase sites (Figure 6A), although the second and third amino acids in the motif are variable among Proteobacteria, which was renamed as AXXFQ motif. We then used more than 200 representative sequences from each class to align and build a WebLogo of the conserved region consensus sequences (Crooks et al., 2004). The glutamine residue (Q164 in A. caulinodans) near phosphatase sites DXXXQ is the most conserved site among different Proteobacteria (Figure 6B). Alanine and phenylalanine residues (A160 and F163 in A. caulinodans) are the second conserved sites (Figure 6B). In epsilon-Proteobacteria, there is a tyrosine residue instead of phenylalanine (Figure 6B). Considering both tyrosine and phenylalanine have a benzene ring, this might be a conservative substitution. These results showed that the novel motif AXXF(Y)Q is widely distributed and conserved among Proteobacteria.
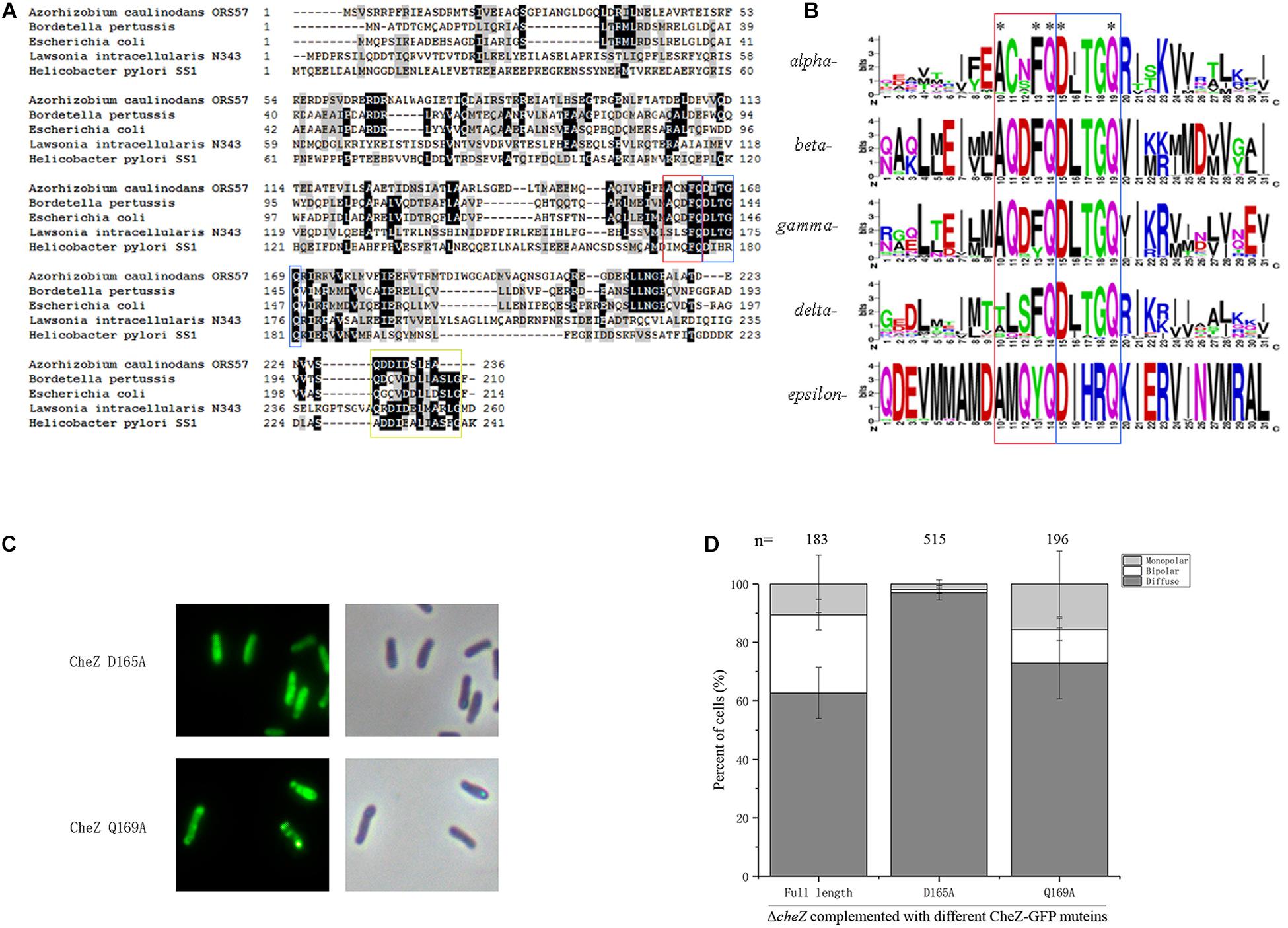
Figure 6. The distribution of AXXFQ motif among Proteobacteria and the effect of two key phosphatase active sites, CheZ_D165A and CheZ_Q169A, on CheZ localization. Alignment of five CheZ sequences from representative strains belonging to different Proteobacteria (A). Logos of the novel motif built with more than 1800 amino acid sequences among CheZs in Proteobacteria (B). The position of “ACNFQ” and “DXXXQ” motifs are marked with red and blue boxes, respectively in panels A,B. The yellow box in panel A means the position of conserved C-terminal region involved in CheY binding. Representative images of localization of CheZ_D165A and CheZ_Q169A (C). Quantification of localization patterns of CheZ_D165A and CheZ_Q169A (D). Data are means ± standard deviation from at least three independent experiments.
Phosphatase Active Sites Affect CheZAC Location
The proximity between these two motifs (AXXFQ and DXXXQ) led us to assess if the localization pattern could be affected by phosphatase active sites. To investigate the role of phosphatase activity on the subcellular localization of CheZAC, site-directed mutants of D165A and Q169A, both critical for the phosphatase activity of CheZAC (Zhao et al., 2002), were fused to GFP. CheZ_Q169A showed a small increase in diffuse localization, and D165A caused an obvious decrease of polar localization (Figures 6C,D), suggesting the subcellular localization of CheZAC might be affected by phosphatase active sites. Because the localization of CheZAC is affected by phosphatase active sites, in turn, the role of different regions affecting localization on chemotactic behavior were assessed. Five truncated mutants, CheZΔ2-31, CheZΔ2-50, CheZΔ2-70, CheZΔ51-70, and CheZΔ71-100, restored the chemotaxis of cheZ mutant (Figure 4A and Supplementary Figure S3), suggesting that the N-terminus of CheZAC is not essential for its phosphatase activity.
Discussion
The location of CheZ to cell poles can improve the sensitivity to chemotactic stimuli. In E. coli, CheZ_F98S, a CheZEC variant that abolished localization, showed a decreased chemotactic response to external signals (Cantwell et al., 2003; Vaknin and Berg, 2004). Furthermore, the spatial distribution of chemotactic proteins, including CheZ, provides a region for specialized functions which are similar as the membrane-bound organelles in eukaryotic cells (Maddock and Shapiro, 1993). Three localization patterns of CheZ were found in A. caulinodans, diffuse, bipolar, and monopolar, indicating the localization pattern of CheZ in A. caulinodans is more complex than that in E. coli (Sourjik and Berg, 2000; Cantwell et al., 2003). The ratio of cells that demonstrated polar localization of CheZAC is much lower than that of CheZEC, indicating the role of CheZAC localization may be different between them. Similar to CheZ in E. coli, the localization of CheZ at lateral body of A. caulinodans cells showed a typical periodic distribution, and this phenomenon may be interpreted by a “stochastic nucleation model” (Greenfield et al., 2009; Saaki et al., 2018).
For many bacteria, CheZ locates near cell poles where CheY-P is generated, and the phosphatase activity of CheZ is 5- to 10-folds higher at the position (Vaknin and Berg, 2004). The enhanced phosphatase activity of CheZ ensures that peritrichously located flagellar motors experience a uniform concentration of CheY-P, which is critical for the coordinated regulation of flagellar motility (Cluzel et al., 2000; Lipkow et al., 2005; Ringgaard et al., 2011). We determined CheZAC can still locate to cellular poles despite lacking a CheA binding site (Wuichet et al., 2007) or other chemotaxis or flagellar proteins. The localization of a remote CheZ ortholog in Helicobacter pylori has been studied (Lertsethtakarn and Ottemann, 2010; Lertsethtakarn et al., 2015). And, in contrast to E. coli CheZ, H. pylori CheZ localization is independent of CheA or other typical chemotaxis proteins, but dependent on ChePep, a novel chemotaxis protein distributed among epsilon-Proteobacteria (Howitt et al., 2011; Lertsethtakarn et al., 2015). Because the genes encoding homologs of ChePep were not found in A. caulinodans genome, we suspect that there might be other partner proteins that contribute CheZ to localization clusters.
The four-helix bundle of CheZEC, especially the tip of the hairpin, is responsible for polar localization in E. coli. In this work, we found that the N-terminal helix is sufficient for the polar localization of CheZAC. The sequence conservation of N-terminus of CheZ between E. coli and A. caulinodans is low, and interestingly, deletion of the N-terminal helix, CheZAC still remained the polar location, indicating more than one region is sufficient for its polar localization. However, the reason why CheZAC has two independent regions that are sufficient for polar localization is unknown.
CheZΔ138-169 results in monopolar localization. In this study, a conserved motif AXXF(Y)Q which is close to the phosphatase active motif DXXXQ was found to be responsible for the unique monopolar localized pattern of CheZAC. Although AXXF(Y)Q is conserved among Proteobacteria, its role in localization and chemotaxis had not been studied. We speculate the high level of polar localization of CheZ in E. coli and H. pylori under common conditions may mask the observation of the role of AXXF(Y)Q on localization changes (Sourjik and Berg, 2000; Cantwell et al., 2003; Lertsethtakarn et al., 2015). The biological significance of the polar localization is that each daughter cell can inherit a CheZ after cell division (Jones and Armitage, 2015; Mauriello et al., 2018). For example, the location of chemotactic proteins transfers from monopolar to bipolar clusters in Vibrio cholerae before cell division (Ringgaard et al., 2011). The unipolar localization of CheZΔ138-169 or CheZΔ158-164 indicates that one daughter cell cannot inherit CheZAC, and the residues 138–169 might be involved in the dissociation between CheZAC and its binding partners for localization. In E. coli, the polar localization of CheZEC can be improved by the interaction with CheA at the chemotaxis signaling complex (Wang and Matsumura, 1996; Vaknin and Berg, 2004). These results further suggest that there may be other proteins that recruit CheZ to the clusters and/or affect the catalysis activity of CheZ, as seen in H. pylori (Lertsethtakarn and Ottemann, 2010; Howitt et al., 2011; Lertsethtakarn et al., 2015).
In this study, we mapped the critical regions sufficient for CheZAC localization and assessed the role of regions in the N-terminal helix and four-helix bundle of CheZAC on both localization changes and chemotaxis. Furthermore, a novel and widespread motif affecting monopolar localization of CheZAC was identified, which might be also important for the modulation of CheZ polar localization in other Proterobacteria.
Materials and Methods
Bacterial Strains and Growth Conditions
Azorhizobium caulinodans ORS571, its derivatives, and E. coli strains are listed in Table 1. A. caulinodans strains were grown in TY media at 37°C. E. coli strains were cultured in Luria broth (Luria et al., 1960) at 37°C.
Generation of CheZ Variants
To construct CheZ variants, a fragment including cheZ gene and its native promoter was amplified by polymerase chain reaction (PCR). Then an egfp gene encoding enhanced GFP was amplified from pEGFP-N1. The two fragments were linked by overlap extension, as previously described (Ho et al., 1989). Next, the resulting construct CheZ-GFP fusion was cloned into a broad-host-range plasmid pBBR1MCS-2 (Kovach et al., 1995), and the pBBR1-CheZ-GFP was used as a temple to construct other CheZ variants. Both the truncated mutants such as CheZΔ2-31-GFP and site-directed mutants such as CheZC161A-GFP were constructed by overlap extension PCR as described by Ho et al. (1989). All the CheZ variants were introduced into the cheZ mutant strain by triparental conjugation using a helper plasmid pRK2013 (Ditta et al., 1980). Primer pairs used in the construction are listed in Table 2.
Microscopy and Data Analysis
After growing in TY solid medium for overnight with shaking, cells with GFP fusion were used for observation. Agarose pads were used to immobile bacteria as described by Meier and Scharf (2009). Images were taken by an Olympus DP73 camera on an Olympus BX53 system fluorescence microscope with a 100 × objective and controlled by a cellSens Dimension 1.7 imaging software (Olympus Inc.,). A space between 505 to 550 nm filter was used to detect fluorescence signals. The images analyzing spatial distribution of CheZ were processed by ImageJ1 as described by Thiem et al. (2007). Distribution of CheZAC was manually enumerated and classified into three types (diffuse, bipolar, and monopolar localization). CheZAC cells with monopolar or bipolar localization showed obvious bright spots at one end or both ends of cell. When the brightness in the whole cell distribute evenly, the localization of CheZAC in these cells is counted as diffuse. ImageJ was used to quantify the brightness at different regions of cells. Experiments were repeated at least three times, and for each sample at least 100 cells were counted.
Soft Agar Plate Assay
The chemotactic behavior of cheZ mutant derivative strains was assessed using soft agar plate assay, as previously described (Miller et al., 2009). Overnight bacterial cultures were washed with chemotaxis buffer at least two times and then adjusted to OD600 of 0.6. Five microliter of cells was dropped in the center of 0.3% soft agar plate. After culturing for 3 days at 37°C, the chemotactic rings on soft agar plate were counted. Ten mM sodium lactate was used as sole carbon source. Experiments were repeated at least three times.
Sequence Alignment and Analysis
CheZ sequences from different Proteobacteria classes were selected from Mist 2.22 (Ulrich and Zhulin, 2010) and NCBI database3. The amino acid sequences alignment was performed by an EMBOSS Needle program4. Secondary and tertiary structure of CheZ proteins were predicted using online server SWISS-MODEL5 and Jpred46. The phylogenetic tree was established using MEGA7 software (Kumar et al., 2016). The multiple sequences of CheZ were aligned by T-coffee7 (Notredame et al., 2000) and BioEdit (Alzohairy, 2011). Hundreds of CheZ sequences selected from NCBI database from each class of Proteobacteria were aligned by MEGA7, then the constructing file was put into Jalview (Waterhouse et al., 2009) to produce a graphical representation, and the region including phosphatase active sties was put into WebLogo (Crooks et al., 2004).
Data Availability Statement
The raw data supporting the conclusions of this article will be made available by the authors, without undue reservation.
Author Contributions
XL and ZX conceived and designed the experiments, analyzed the data, prepared the figures and tables, and wrote the manuscript. XL, YL, and XD carried out the experiments. KJ helped with the improvement and revision of the manuscript. All authors approved the submission for publication.
Funding
This work was financed by the NSFC-Shandong Joint Fund Key Projects (U1806206) and the National Natural Science Foundation of China (31870020).
Conflict of Interest
The authors declare that the research was conducted in the absence of any commercial or financial relationships that could be construed as a potential conflict of interest.
Acknowledgments
We thank Robert B. Bourret, Karen M. Ottemann, Shuai Hu, and John S. Parkinson for helpful and insightful comments on the manuscript.
Supplementary Material
The Supplementary Material for this article can be found online at: https://www.frontiersin.org/articles/10.3389/fmicb.2020.585140/full#supplementary-material
Supplementary Figure 1 | The alignment of CheAEC and CheAAC was generated using the default settings of EMBOSS Needle software from EMBL. The beginning Met of the short form of CheAEC was marked with black frame.
Supplementary Figure 2 | The localization pattern of CheZΔ138-169 in cheA, cheA-R, cheY1, cheY2, fliM, and fliN mutant. The mutein CheZΔ138-169 was fused to GFP, and CheZΔ138-169GFP including its own promoter were inserted into pBBR2 and then were transformed into each mutant strain.
Supplementary Figure 3 | The representative images of chemotactic rings formed by cheZ mutant containing different CheZ derivatives fused to GFP. Ten mM sodium lactate was used as sole carbon source. The cognate results of each strain also shown in Figures 4A and 5A.
Footnotes
- ^ https://imagej.nih.gov/ij/
- ^ https://mistdb.com/
- ^ https://www.ncbi.nlm.nih.gov/
- ^ https://www.ebi.ac.uk/Tools/psa/emboss_needle/
- ^ https://swissmodel.expasy.org/
- ^ http://www.compbio.dundee.ac.uk/jpred/
- ^ https://www.ebi.ac.uk/Tools/msa/tcoffee/
References
Alzohairy, A. M. (2011). BioEdit: an important software for molecular biology. Gerf. Bull. Biosci. 2, 60–61.
Bernadac, A., Gavioli, M., Lazzaroni, J. C., Raina, S., and Lloubes, R. (1998). Escherichia coli tol-pal mutants form outer membrane vesicles. J. Bacteriol. 180, 4872–4878. doi: 10.1128/jb.180.18.4872-4878.1998
Blat, Y., and Eisenbach, M. (1994). Phosphorylation-dependent binding of the chemotaxis signal molecule CheY to its phosphatase, CheZ. Biochemistry 33, 902–906. doi: 10.1021/bi00170a008
Blat, Y., and Eisenbach, M. (1996). Conserved C-terminus of the phosphatase CheZ is a binding domain for the chemotactic response regulator CheY. Biochemistry 35, 5679–5683. doi: 10.1021/bi9530447
Cantwell, B. J., Draheim, R. R., Weart, R. B., Nguyen, C., Stewart, R. C., and Manson, M. D. (2003). CheZ phosphatase localizes to chemoreceptor patches via CheA-short. J. Bacteriol. 185, 2354–2361. doi: 10.1128/jb.185.7.2354-2361.2003
Cantwell, B. J., and Manson, M. D. (2009). Protein domains and residues involved in the CheZ/CheAS interaction. J. Bacteriol. 191, 5838–5841. doi: 10.1128/jb.00280-09
Cluzel, P., Surette, M., and Leibler, S. (2000). An ultrasensitive bacterial motor revealed by monitoring signaling proteins in single cells. Science 287, 1652–1655. doi: 10.1126/science.287.5458.1652
Crooks, G. E., Hon, G., Chandonia, J. M., and Brenner, S. E. (2004). WebLogo: a sequence logo generator. Genome Res. 14, 1188–1190. doi: 10.1101/gr.849004
Delalez, N. J., Berry, R. M., and Armitage, J. P. (2014). Stoichiometry and turnover of the bacterial flagellar switch protein FliN. mBio 5, e001216–14.
Ditta, G., Stanfield, S., Corbin, D., and Helinski, D. R. (1980). Broad host range DNA cloning system for gram-negative bacteria: construction of a gene bank of Rhizobium meliloti. Proc. Natl. Acad. Sci. U.S.A. 77, 7347–7351. doi: 10.1073/pnas.77.12.7347
Dreyfus, B. L., Elmerich, C., and Dommergues, Y. R. (1983). Free-living Rhizobium strain able to grow on N2 as the sole nitrogen source. Appl. Environ. Microbiol. 45, 711–713. doi: 10.1128/aem.45.2.711-713.1983
Drozdetskiy, A., Cole, C., Procter, J., and Barton, G. J. (2015). JPred4: a protein secondary structure prediction server. Nucleic. Acids Res. 43, W389–W394. doi: 10.1093/nar/gkv332
Greenfield, D., Mcevoy, A. L., Shroff, H., Crooks, G. E., Wingreen, N. S., Betzig, E., et al. (2009). Self-organization of the Escherichia coli chemotaxis network imaged with super-resolution light microscopy. PLoS Biol. 7:e1000137. doi: 10.1371/journal.pbio.1000137
Hao, S., Hamel, D., Zhou, H., and Dahlquist, F. W. (2009). Structural basis for the localization of the chemotaxis phosphatase CheZ by CheAS. J. Bacteriol. 191, 5842–5844. doi: 10.1128/jb.00323-09
Ho, S. N., Hunt, H. D., Horton, R. M., Pullen, J. K., and Pease, L. R. (1989). Site-directed mutagenesis by overlap extension using the polymerase chain reaction. Gene 77, 51–59. doi: 10.1016/0378-1119(89)90358-2
Howitt, M. R., Lee, J. Y., Lertsethtakarn, P., Vogelmann, R., Joubert, L. M., Ottemann, K. M., et al. (2011). ChePep controls Helicobacter pylori Infection of the gastric glands and chemotaxis in the Epsilonproteobacteria. mBio 2:e0098-11.
Jiang, N., Liu, W., Li, Y., and Xie, Z. (2016). Comparative genomic and protein sequence analyses of the chemotaxis system of Azorhizobium caulinodans. Acta Microbiol. Sin. 56, 1256–1265.
Jones, C. W., and Armitage, J. P. (2015). Positioning of bacterial chemoreceptors. Trends Microbiol. 23, 247–256. doi: 10.1016/j.tim.2015.03.004
Kovach, M. E., Elzer, P. H., Hill, D. S., Robertson, G. T., Farris, M. A., and Roop, R. M. II, et al. (1995). Four new derivatives of the broad-host-range cloning vector pBBR1MCS, carrying different antibiotic-resistance cassettes. Gene 166, 175–176. doi: 10.1016/0378-1119(95)00584-1
Kumar, S., Stecher, G., and Tamura, K. (2016). MEGA7: molecular evolutionary genetics analysis version 7.0 for bigger datasets. Mol. Biol. Evol. 33, 1870–1874. doi: 10.1093/molbev/msw054
Laloux, G., and Jacobs-Wagner, C. (2014). How do bacteria localize proteins to the cell pole? J. Cell Sci. 127, 11–19. doi: 10.1242/jcs.138628
Lertsethtakarn, P., Howitt, M. R., Castellon, J., Amieva, M. R., and Ottemann, K. M. (2015). Helicobacter pylori CheZ(HP) and ChePep form a novel chemotaxis-regulatory complex distinct from the core chemotaxis signaling proteins and the flagellar motor. Mol. Microbiol. 97, 1063–1078. doi: 10.1111/mmi.13086
Lertsethtakarn, P., and Ottemann, K. M. (2010). A remote CheZ orthologue retains phosphatase function. Mol. Microbiol. 77, 225–235. doi: 10.1111/j.1365-2958.2010.07200.x
Lipkow, K., Andrews, S. S., and Bray, D. (2005). Simulated diffusion of phosphorylated CheY through the cytoplasm of Escherichia coli. J. Bacteriol. 187, 45–53. doi: 10.1128/jb.187.1.45-53.2005
Liu, W., Bai, X., Li, Y., Min, J., Kong, Y., and Hu, X. (2020). CheY1 and CheY2 of Azorhizobium caulinodans ORS571 regulate chemotaxis and competitive colonization with the host plant. Appl. Environ. Microbiol. 86:AEM.599-AEM.520.
Liu, W., Sun, Y., Shen, R., Dang, X., Liu, X., Sui, F., et al. (2018). A Chemotaxis-like pathway of Azorhizobium caulinodans controls flagella-driven motility, which regulates biofilm formation, exopolysaccharide biosynthesis, and competitive nodulation. Mol. Plant Microbe Interact. 31, 737–749.
Liu, X., Liu, W., Sun, Y., Xia, C., Elmerich, C., and Xie, Z. (2018). A cheZ-like gene in Azorhizobium caulinodans is a key gene in the control of chemotaxis and colonization of the host plant. Appl. Environ. Microbiol. 84:AEM.1827-AEM.1817.
Liu, X., Xie, Z., and Zhang, H. (2019). Inactivation of the phosphatase CheZ alters cell-surface properties of Azorhizobium caulinodans ORS571 and symbiotic association with Sesbania rostrata. Mol. Plant Microbe Interact 32, 1547–1556.
Luria, S. E., Adams, J. N., and Ting, R. C. (1960). Transduction of lactose-utilizing ability among strains of E. coli and S. dysenteriae and the properties of the transducing phage particles. Virology 12, 348–390. doi: 10.1016/0042-6822(60)90161-6
Maddock, J. R., and Shapiro, L. (1993). Polar location of the chemoreceptor complex in the Escherichia coli cell. Science 259, 1717–1723. doi: 10.1126/science.8456299
Madeira, F., Park, Y. M., Lee, J., Buso, N., Gur, T., Madhusoodanan, N., et al. (2019). The EMBL-EBI search and sequence analysis tools APIs in 2019. Nucl. Acids Res. 47, W636–W641.
Mauriello, E. M. F., Jones, C., Moine, A., and Armitage, J. P. (2018). Cellular targeting and segregation of bacterial chemotaxis systems. FEMS Microbiol. Rev. 42, 462–476.
Meier, V. M., and Scharf, B. E. (2009). Cellular localization of predicted transmembrane and soluble chemoreceptors in Sinorhizobium meliloti. J. Bacteriol. 191, 5724–5733. doi: 10.1128/jb.01286-08
Miller, L. D., Russell, M. H., and Alexandre, G. (2009). Diversity in bacterial chemotactic responses and niche adaptation. Adv. Appl. Microbiol. 66, 53–75. doi: 10.1016/s0065-2164(08)00803-4
Neeli-Venkata, R., Startceva, S., Annila, T., and Ribeiro, A. S. (2016). Polar localization of the serine chemoreceptor of Escherichia coli is nucleoid exclusion-dependent. Biophys. J. 111, 2512–2522. doi: 10.1016/j.bpj.2016.10.024
Notredame, C., Higgins, D. G., and Heringa, J. (2000). T-Coffee: a novel method for fast and accurate multiple sequence alignment. J. Mol. Biol. 302, 205–217. doi: 10.1006/jmbi.2000.4042
Pinas, G. E., Frank, V., Vaknin, A., and Parkinson, J. S. (2016). The source of high signal cooperativity in bacterial chemosensory arrays. Proc. Natl. Acad. Sci. U.S.A. 113, 3335–3340. doi: 10.1073/pnas.1600216113
Ringgaard, S., Schirner, K., Davis, B. M., and Waldor, M. K. (2011). A family of ParA-like ATPases promotes cell pole maturation by facilitating polar localization of chemotaxis proteins. Genes Dev. 25, 1544–1555. doi: 10.1101/gad.2061811
Saaki, T. N. V., Strahl, H., and Hamoen, L. W. (2018). Membrane curvature and the Tol-Pal complex determine polar localization of the chemoreceptor Tar in E. coli. J. Bacteriol. 200:e00658-17.
Santos, T. M., Lin, T. Y., Rajendran, M., Anderson, S. M., and Weibel, D. B. (2014). Polar localization of Escherichia coli chemoreceptors requires an intact Tol-Pal complex. Mol. Microbiol. 92, 985–1004. doi: 10.1111/mmi.12609
Shen, R., Liu, W., Sun, Y., Li, R., and Xie, Z. (2018). Function analysis of flagellar genes fliN and FliM in Azorhizobium caulinodans ORS571. Acta Microbiol. Sin. 58, 882–892.
Silversmith, R. E. (2005). High mobility of carboxyl-terminal region of bacterial chemotaxis phosphatase CheZ is diminished upon binding divalent cation or CheY-P substrate. Biochemistry 44, 7768–7776. doi: 10.1021/bi0501636
Silversmith, R. E. (2010). Auxiliary phosphatases in two-component signal transduction. Curr. Opin. Microbiol. 13, 177–183. doi: 10.1016/j.mib.2010.01.004
Silversmith, R. E., Levin, M. D., Schilling, E., and Bourret, R. B. (2008). Kinetic characterization of catalysis by the chemotaxis phosphatase CheZ. Modulation of activity by the phosphorylated CheY substrate. J. Biol. Chem. 283, 756–765. doi: 10.1074/jbc.m704400200
Sourjik, V., and Berg, H. C. (2000). Localization of components of the chemotaxis machinery of Escherichia coli using fluorescent protein fusions. Mol. Microbiol. 37, 740–751. doi: 10.1046/j.1365-2958.2000.02044.x
Szurmant, H., and Ordal, G. W. (2004). Diversity in chemotaxis mechanisms among the bacteria and archaea. Microbiol. Mol. Biol. Rev. 68, 301–319. doi: 10.1128/mmbr.68.2.301-319.2004
Thiem, S., Kentner, D., and Sourjik, V. (2007). Positioning of chemotaxis clusters in E. coli and its relation to cell division. Embo J. 26, 1615–1623. doi: 10.1038/sj.emboj.7601610
Ulrich, L. E., and Zhulin, I. B. (2010). The MiST2 database: a comprehensive genomics resource on microbial signal transduction. Nucl. Acids Res. 38, D401–D407.
Vaknin, A., and Berg, H. C. (2004). Single-cell FRET imaging of phosphatase activity in the Escherichia coli chemotaxis system. Proc. Natl. Acad. Sci. U.S.A. 101, 17072–17077. doi: 10.1073/pnas.0407812101
Wang, H., and Matsumura, P. (1996). Characterization of the CheAS/CheZ complex: a specific interaction resulting in enhanced dephosphorylating activity on CheY-phosphate. Mol. Microbiol. 19, 695–703. doi: 10.1046/j.1365-2958.1996.393934.x
Waterhouse, A., Bertoni, M., Bienert, S., Studer, G., Tauriello, G., Gumienny, R., et al. (2018). SWISS-MODEL: homology modelling of protein structures and complexes. Nucleic. Acids Res. 46, W296–w303. doi: 10.1093/nar/gky427
Waterhouse, A. M., Procter, J. B., Martin, D. M., Clamp, M., and Barton, G. J. (2009). Jalview Version 2–a multiple sequence alignment editor and analysis workbench. Bioinformatics 25, 1189–1191. doi: 10.1093/bioinformatics/btp033
Wuichet, K., Alexander, R. P., and Zhulin, I. B. (2007). Comparative genomic and protein sequence analyses of a complex system controlling bacterial chemotaxis. Methods Enzymol. 422, 1–31.
Wuichet, K., and Zhulin, I. B. (2010). Origins and diversification of a complex signal transduction system in prokaryotes. Sci. Signal. 3:ra50. doi: 10.1126/scisignal.2000724
Keywords: chemotaxis, CheZ, cellular localization, Azorhizobium caulinodans, rhizobia
Citation: Liu X, Liu Y, Johnson KS, Dong X and Xie Z (2020) Protein Residues and a Novel Motif Involved in the Cellular Localization of CheZ in Azorhizobium caulinodans ORS571. Front. Microbiol. 11:585140. doi: 10.3389/fmicb.2020.585140
Received: 19 July 2020; Accepted: 16 November 2020;
Published: 07 December 2020.
Edited by:
Hai-Lei Wei, Institute of Agricultural Resources and Regional Planning (CAAS), ChinaReviewed by:
Lindsey O’Neal, University of Washington, United StatesTetsuhiro Ogawa, The University of Tokyo, Japan
Copyright © 2020 Liu, Liu, Johnson, Dong and Xie. This is an open-access article distributed under the terms of the Creative Commons Attribution License (CC BY). The use, distribution or reproduction in other forums is permitted, provided the original author(s) and the copyright owner(s) are credited and that the original publication in this journal is cited, in accordance with accepted academic practice. No use, distribution or reproduction is permitted which does not comply with these terms.
*Correspondence: Zhihong Xie, emh4aWVAeWljLmFjLmNu