- Department of Microbiology, Institute of Biomedical Sciences, University of São Paulo, São Paulo, Brazil
Pseudomonas aeruginosa and Candida spp. are biofilm-forming pathogens commonly found colonizing medical devices, being mainly associated with pneumonia and bloodstream infections. The coinfection by these pathogens presents higher mortality rates when compared to those caused by a single microbial species. This study aimed to evaluate the antibiofilm activity of echinocandins and polymyxin B (PMB) against polymicrobial biofilms of carbapenem-resistant (CR) Pseudomonas aeruginosa and Candida spp. (C. albicans, C. parapsilosis, C. tropicalis, and C. glabrata). In addition, we tested the antimicrobial effect on their planktonic and monomicrobial biofilm counterparties. Interestingly, beyond inhibition of planktonic [minimum inhibitory concentration (MIC) = 0.5 μg/ml] and biofilm [minimum biofilm inhibitory concentration (MBIC)50 ≤ 2–8 μg/ml] growth of P. aeruginosa, PMB was also effective against planktonic cells of C. tropicalis (MIC = 2 μg/ml), and polymicrobial biofilms of CR P. aeruginosa with C. tropicalis (MBIC50 ≤ 2 μg/ml), C. parapsilosis (MBIC50 = 4–16 μg/ml), C. glabrata (MBIC50 = 8–16 μg/ml), or C. albicans (MBIC50 = 8–64 μg/ml). On the other hand, while micafungin (MFG) showed highest inhibitory activity against planktonic (MIC ≤ 0.008–0.5 μg/ml) and biofilm (MBIC50 ≤ 2–16 μg/ml) growth of Candida spp.; caspofungin (CAS) displays inhibitory activity against planktonic cells (MIC = 0.03–0.25 μg/ml) and monomicrobial biofilms (MBIC50 ≤ 2–64 μg/ml) of Candida spp., and notably on planktonic and monomicrobial biofilms of CR P. aeruginosa (MIC or MBIC50 ≥ 64 μg/ml). Particularly, for mixed biofilms, while CAS reduced significantly viable cell counts of CR P. aeruginosa and Candida spp. at ≥32 and ≥ 2 μg/ml, respectively; PMB was effective in reducing viable cells of CR P. aeruginosa at ≥2 μg/ml and Candida spp. at ≥8 μg/ml. Similar reduction of viable cells was observed for CAS (32–64 μg/ml) combined with PMB (2 μg/ml). These findings highlight the potential of PMB and CAS for the treatment of polymicrobial infections caused by Candida spp. and critical priority CR P. aeruginosa.
Introduction
Biofilms are commonly defined as complex systems, comprising consortia of bacteria and/or fungi adhered to biotic or abiotic surfaces and lodged in a three-dimensional extracellular polymeric matrix (EPM) constituted mainly by secreted polysaccharides and proteins (Bandara et al., 2010; Deveau et al., 2018; Ibrahim et al., 2018). In this regard, most microorganisms are organized into biofilms, instead of living as planktonic cells, since biofilms offer protection against environmental, chemical, and mechanical stresses (Boudarel et al., 2018). Biofilm-derived-cells are phenotypically different from their planktonic counterparts; in fact, in the former, the reduction of microbial growth and metabolism can lead to the evasion of the immune system and increased resistance to antimicrobial agents (Ibrahim et al., 2018).
In hospital settings, microbial biofilms are relevant sources of infectious diseases, indeed, device-associated biofilm infections pose a considerable risk for patients (Desai et al., 2014; Ibrahim et al., 2018). In this regard, Pseudomonas aeruginosa and Candida spp. are among the most commonly found biofilm-forming pathogens and their biofilms are considered a risk factor for bacteremia/candidaemia, contributing to increased mortality rate (Ramage et al., 2005; Tumbarello et al., 2007; Vitkauskienė et al., 2010; Li et al., 2018; Lee et al., 2020).
Polymicrobial infections involving P. aeruginosa and Candida spp. have been described in wound infection, chronic lung disease, pneumonia associated with mechanical ventilation, and bloodstream infections (Klotz et al., 2007; Peleg et al., 2010; Trejo-Hernandez et al., 2014; Rodrigues et al., 2017).
Polymicrobial biofilms significantly alter treatment therapies and patient outcomes (Peters et al., 2012). For the treatment of Candida biofilms, only echinocandins and liposomal formulation of amphotericin B have been recommended (Pappas et al., 2016); however, the echinocandins are considered the first-line drug to treat candidemia related with biofilm formation. These antifungal agents exhibit non-competitive inhibition of the β-(1,3)-D-glucan synthetase enzyme, which catalyzes the polymerization of UDP-glucose to β-(1,3)-D-glucan, the main component of the fungal cell wall and biofilm EPM of Candida spp. (Denning, 2003). On the other hand, there are no specific recommendations for the treatment of P. aeruginosa biofilms. Polymyxins [polymyxin E (colistin) and polymyxin B (PMB)] are positively charged peptides used in the antimicrobial therapy, as a last option to treat infections caused by multidrug-resistant (MDR) Gram-negative bacteria, including carbapenem-resistant (CR) P. aeruginosa, which has become a serious health threat worldwide due to the limited options available for its treatment (Pogue et al., 2020).
The detection of β-(1,3)-D-glucan in the serum of patients suffering from P. aeruginosa infections has arisen an interest in evaluating the effect of echinocandins on this bacterial pathogen (Bazzi et al., 2013). In this respect, some studies have revealed the presence of β-(1,3)-D-glucan in P. aeruginosa as a periplasmic glucan polymer, and as an additional component of biofilm EPM (Lequette et al., 2007; Coulon et al., 2010; Beaudoin et al., 2012; Bazzi et al., 2013). Moreover, previous studies had demonstrated inhibitory effects of echinocandins on bacterial biofilms formed by Staphylococcus aureus and P. aeruginosa (Bazzi et al., 2013; Siala et al., 2016; Rasheed et al., 2018). Therefore, the in vitro activity of echinocandins and PMB against polymicrobial biofilms is worthy of investigation. In this study, we evaluated the activity of echinocandins and PMB, alone or in combination, against mixed biofilms of Candida spp. and CR P. aeruginosa.
Materials and Methods
Microorganisms
Clinical and environmental isolates of carbapenem-sensitive and CR P. aeruginosa (PAO1, ATCC 15442, ATCC 27853, 48-1997A, 141, 151, and 564-FC; Toleman et al., 2002; Fontes et al., 2011; Turano et al., 2016), and clinical isolates of Candida spp. (C. albicans SC5314, C. paraposilosis ATCC 22019, C. tropicalis ATCC 200956, and C. glabrata ATCC 2001) were used in this study. All isolates were maintained in brain heart infusion (BHI) broth with 20% glycerol at −80°C. Candida spp. and P. aeruginosa were recovered on Sabouraud dextrose and MacConkey agar, respectively, for 24 h at 37°C. Additionally, yeasts and bacteria were subcultured in Sabouraud dextrose or trypticase soy broths, respectively, for 24 h at 37°C, under agitation (150 rpm). All culture mediums were purchased from Becton, Dickinson and Company (New Jersey, United States).
Antimicrobial Susceptibility Testing of Planktonic Cells
Antifungal drugs [i.e., caspofungin (CAS) and micafungin (MFG)] and PMB antibiotic were purchased from Sigma-Merck (Darmstadt, Germany), and stock solutions were prepared in dimethyl sulfoxide. Initially, the antimicrobial susceptibility profile of planktonic P. aeruginosa cells was determined by the disk diffusion method (Supplementary Table S1). Additionally, minimum inhibitory concentrations (MICs) of PMB and echinocandins were performed by broth microdilution methods, following CLSI guidelines for bacteria and fungi (Clinical and Laboratory Standards Institute, 2017, 2020). After broth microdilution testing, minimum microbicidal concentrations (MMCs) were determined as previously described (Freitas et al., 2018).
Antimicrobial Susceptibility of Monomicrobial and Mixed Biofilms Formed by Candida spp. and Carbapenem-Resistant Pseudomonas aeruginosa
For the formation of polymicrobial biofilms, 50 μl of Candida spp. suspension at 1 × 107 colony forming unit (CFU)/ml were incubated simultaneously with 50 μl of P. aeruginosa (PAO1, 151, and 48-1997A strains) suspension at 1 × 108 CFU/ml, in RPMI 1640 medium buffered with 0.16 M MOPS (Sigma-Merck), using a 96-well flat sterile microplate. Monomicrobial biofilms were formed using 50 μl of each fungal or bacterial inoculum in wells containing 50 μl of medium. After 24 h of incubation at 37°C and under agitation (150 rpm), the monomicrobial or polymicrobial biofilms were washed with PBS twice and treated with several concentrations of CAS, MFG, or PMB (2–128 μg/ml) diluted in RPMI 1640 buffered with 0.16 M MOPS. Untreated biofilms and wells, containing only medium, were included in the assay, as microbial growth and sterility controls, respectively. In addition, the combination of CAS (32 or 64 μg/ml) with PMB (2 μg/ml) was tested against polymicrobial biofilms formed by Candida spp. and P. aeruginosa 151 strain. Microplates were incubated at 37°C, for 24 h at 150 rpm, the supernatant was removed, the biofilm washed in PBS twice, and the total biomass and cell viability were determined as described below. Assays were performed in quadruplicate in three independent experiments.
Total Biomass Quantification
The total biomass (sessile cells plus EPM) of bacterial, fungal, and mixed biofilms was quantified using the violet crystal (VC) staining assay (Freitas et al., 2018). After the incubation period, biofilms were washed and fixed with 100 μl of methanol for 15 min, and then stained with 100 μl of 0.1% VC for 15 min. VC excess was removed and microplates were washed with distilled water and dried. The total biomass quantification was performed by adding 100 μl of 33% (v/v) glacial acetic acid as a CV solvent. Optical density (O.D.) at 590 nm of dissolved CV was measured in a microplate reader (Epoch 2 model, Biotek, Winooski, United States). The lowest concentrations of antimicrobials inhibiting 50% minimum biofilm inhibitory concentration (MBIC50) and 90% (MBIC90) of monomicrobial and polymicrobial biofilms were determined by calculating the inhibition percentage following the formula: 100 – (treated O.D. × 100/untreated O.D.), after subtraction of medium control O.D. Finally, MBIC50 and MBIC90 values were considered as a modal average from all results obtained.
Viable Cell Count
The cell viability from polymicrobial biofilms after treatments with CAS or PMB (2, 8, 32, and 128 μg/ml) alone or in combination (32 or 64 μg/ml CAS plus 2 μg/ml PMB), compared with untreated biofilms, was evaluated by viable cell count assays. In brief, biofilms were washed and 100 μl of PBS was added to each well to remove the sessile cells by scraping and rinsing using a tip. The cells were transferred to a sterile microtube, sonicated at 42 KHz in an ultrasonic bath for 3 min, and serial dilutions (1:10) in PBS were performed and plated in a selective media (i.e., Cetrimide agar for P. aeruginosa and Sabouraud dextrose agar with 50 μg/ml chloramphenicol for Candida spp.) for CFU counting. Log CFU/ml values were calculated, and the final results were expressed as average and SD (Qu et al., 2016).
Statistical Analysis
Statistical analyzes were performed using the GraphPad Prism v. 8 software (GraphPad, La Jolla, CA, United States), and values of p ≤ 0.05 were considered statistically significant.
Results and Discussion
Antimicrobial susceptibility of planktonic bacterial mode of growth revealed that three P. aeruginosa strains (151, 141, and 48-1997A) exhibited a MDR profile (Magiorakos et al., 2012; Supplementary Table S1). In this regard, the MDR phenotype and carbapenem resistance was associated with SPM-1 metallo-β-lactamase production (Toleman et al., 2002; Fontes et al., 2011; Turano et al., 2016). Otherwise, P. aeruginosa strains ATCC 27853, ATCC 15442, and PAO1 were susceptible to all antibacterial tested, whereas 564-FC strain displays resistance to amikacin and ciprofloxacin (Supplementary Table S1). All P. aeruginosa strains were susceptible to PMB, whereas Candida spp. were susceptible to echinocandins (Table 1).
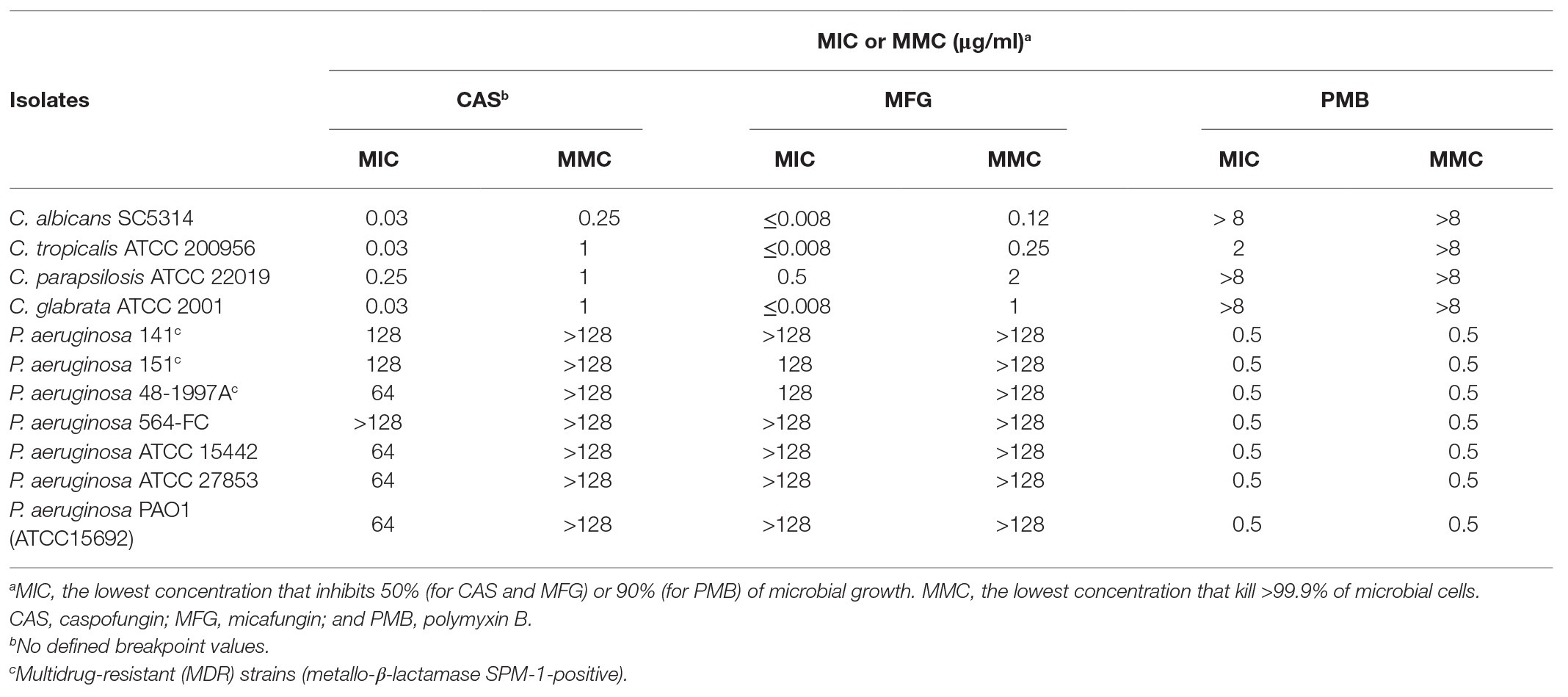
Table 1. Values of minimum inhibitory concentration (MIC) and minimum microbicidal concentration (MMC) of caspofungin (CAS), micafungin (MFG), and polymyxin B (PMB) against planktonic cells of Candida spp. and Pseudomonas aeruginosa.
Polymyxin B inhibited only the planktonic growth of C. tropicalis ATCC 200956 at 2 μg/ml (Table 1). Interestingly, C. tropicalis ATCC 200956 isolate was previously described as resistant to AMB that has cross-resistance to azoles (Forastiero et al., 2013). On the other hand, among the echinocandins evaluated on planktonic bacterial cells, CAS showed bacteriostatic action on six P. aeruginosa strains, at 64–128 μg/ml, including CR MDR isolates 141, 151, and 48-1997A; whereas MFG showed inhibitory effects against CR P. aeruginosa 151 and 48-1997A strains, at 128 μg/ml (Table 1). Then, the selection of P. aeruginosa isolates was based on MDR profiles or susceptibility to clinically available antibiotics (MDR: 151 and 48-1997A, and susceptible: PAO1) for evaluation of antibiofilm effect of CAS and MFG against mono- and polymicrobial biofilms formed with Candida spp.
Monomicrobial biofilms of P. aeruginosa (PAO1, 151, and 48-1997A) were susceptible to PMB with MBIC50 ranging from ≤2 to 8 μg/ml (Table 2). However, only CAS, at 64 μg/ml, was able to inhibit P. aeruginosa 151 biofilm (Table 2). Previous studies have shown that MFG requires high concentrations (10 mg/ml) to have antibiofilm effect against P. aeruginosa isolates (Bazzi et al., 2013; Kissoyan et al., 2016) corroborating with our results which even higher concentration tested we did not observe any biofilm inhibition (Table 2).
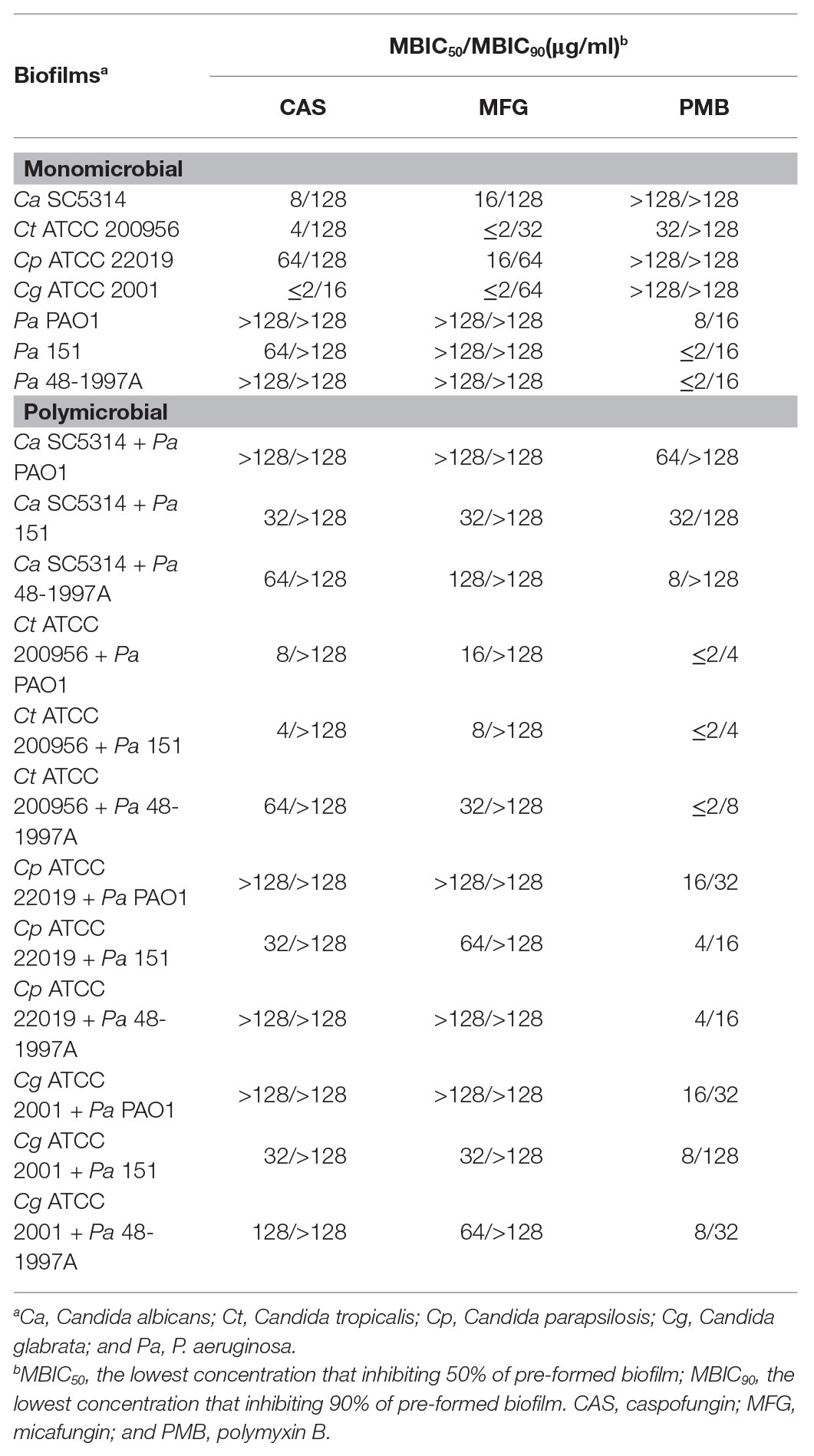
Table 2. Minimum biofilm inhibitory concentrations (MBICs) of antimicrobials for monomicrobial and polymicrobial biofilms formed by Candida spp. and Pseudomonas aeruginosa.
As previously reported in the literature the echinocandins had a strong activity on Candida spp. biofilms but lesser than those observed on the planktonic cells corroborating with our findings (MBIC50 from ≤2 to 64 μg/ml vs. MIC ≤1 μg/ml; Tables 1, 2; Vila et al., 2016); whereas PMB was active only for C. tropicalis but at higher concentration (32 μg/ml; Table 2).
Candida-bacteria interactions are not a rare occurrence, and several studies suggest that P. aeruginosa and C. albicans interact with each other in the human body and in surfaces and frequently the coinfection leads to higher pathogenicity and mortality rates (Hogan and Kolter, 2002; Peleg et al., 2010; Trejo-Hernandez et al., 2014; Rodrigues et al., 2017). Although, in vitro studies have shown that P. aeruginosa forms a dense biofilm on C. albicans filaments and kills the fungus, P. aeruginosa neither binds to nor kills yeast-form C. albicans, even after prolonged incubation (Hogan and Kolter, 2002; Allison et al., 2016). In this study, we observed that C. albicans viability is totally preserved up to 2 days of interaction, and only on the 3rd day of fungus-bacterium interaction there was a reduction of fungal viability (Supplementary Figure S1). Therefore, antifungals and antibacterial treatments were performed on preformed 24-h mixed biofilms of Candida spp. and CR P. aeruginosa.
Our data showed that the polymicrobial biofilms formed by fungus-bacterium interaction were inhibited by antimicrobials CAS, MFG, and PMB at MBIC50 values ≥ MBIC50 values for monomicrobial biofilms (Table 2) corroborating with literature data that described the polymicrobial biofilms are more tolerant than their monomicrobial counterparties (Peleg et al., 2010). PMB was effective against polymicrobial biofilms of P. aeruginosa with C. tropicalis (MBIC50 ≤ 2 μg/ml), C. parapsilosis (MBIC50 = 4–16 μg/ml), C. glabrata (MBIC50 = 8–16 μg/ml), or C. albicans (MBIC50 = 8–64 μg/ml). Both echinocandins (CAS, 4 ≥ 128 μg/ml; MFG, 8 ≥ 128 μg/ml) reduced the total biomass of polymicrobial biofilms, especially for those formed by Candida spp. and P. aeruginosa strain 151, which were inhibited at the lowest MBIC values (Table 2). Therefore, additionally to the total biomass data, the cell viabilities of Candida spp. and P. aeruginosa (151 strain) from mixed biofilms were assessed after treatments with CAS or PMB.
There are several methods to evaluate the inhibition of biofilm formation besides VC staining (total biomass), such as XTT assay (metabolic activity of prokaryotic and eukaryotic cells) and CFU counting (cell viability), which are complementary and correlated (Mukherje et al., 2005; Wilson et al., 2017). Although XTT assay has been considered a gold standard method to evaluate the antibiofilm activity of compounds (Scudiero et al., 1988; Mukherje et al., 2005; Sabaeifard et al., 2014), in this study, we have used CFU counting to evaluate the viability of fungal and bacterial cells from single or mixed biofilms of Candida spp. and P. aeruginosa, since CFU counting allows to differentiate quantitatively (CFUs) fungus and bacterium viability inside of mixed biofilms by using selective culture media. In fact, CFU counting method has been often used in studies of inter-kingdom mixed biofilms (Qu et al., 2016; Santos et al., 2016; Kim et al., 2018).
Caspofungin, at concentrations ≥2 μg/ml, significantly reduced in up to 5 log, the number of viable counts of all Candida spp. in a dose-dependent manner, reaching undetectable levels at 128 μg/ml (p < 0.001, Figure 1), whereas CAS at concentrations ≥32 μg/ml decreased 2 log, the number of viable cells of P. aeruginosa 151 (p < 0.05, Figure 1). Notably, P. aeruginosa 151 – a CR and MDR strain – was inhibited by the echinocandins, both in planktonic growth mode or in monomicrobial and mixed biofilms (Tables 1, 2). The antibiotic PMB, at concentrations ≥2 μg/ml, reduced significantly the viability of P. aeruginosa 151, and at ≥8 μg/ml this reduction achieves undetectable levels in all mixed biofilms (p < 0.001, Figure 1). PMB at ≥8 μg/ml was also effective in reducing the viability of C. glabrata and C. parapsilosis in mixed biofilms with P. aeruginosa 151 (Figures 1F,H). Interestingly, mixed biofilms formed by hyphae/pseudohyphae-forming species (C. albicans and C. tropicalis) were susceptible only at concentrations ≥32 μg/ml PMB (Figures 1B,D).
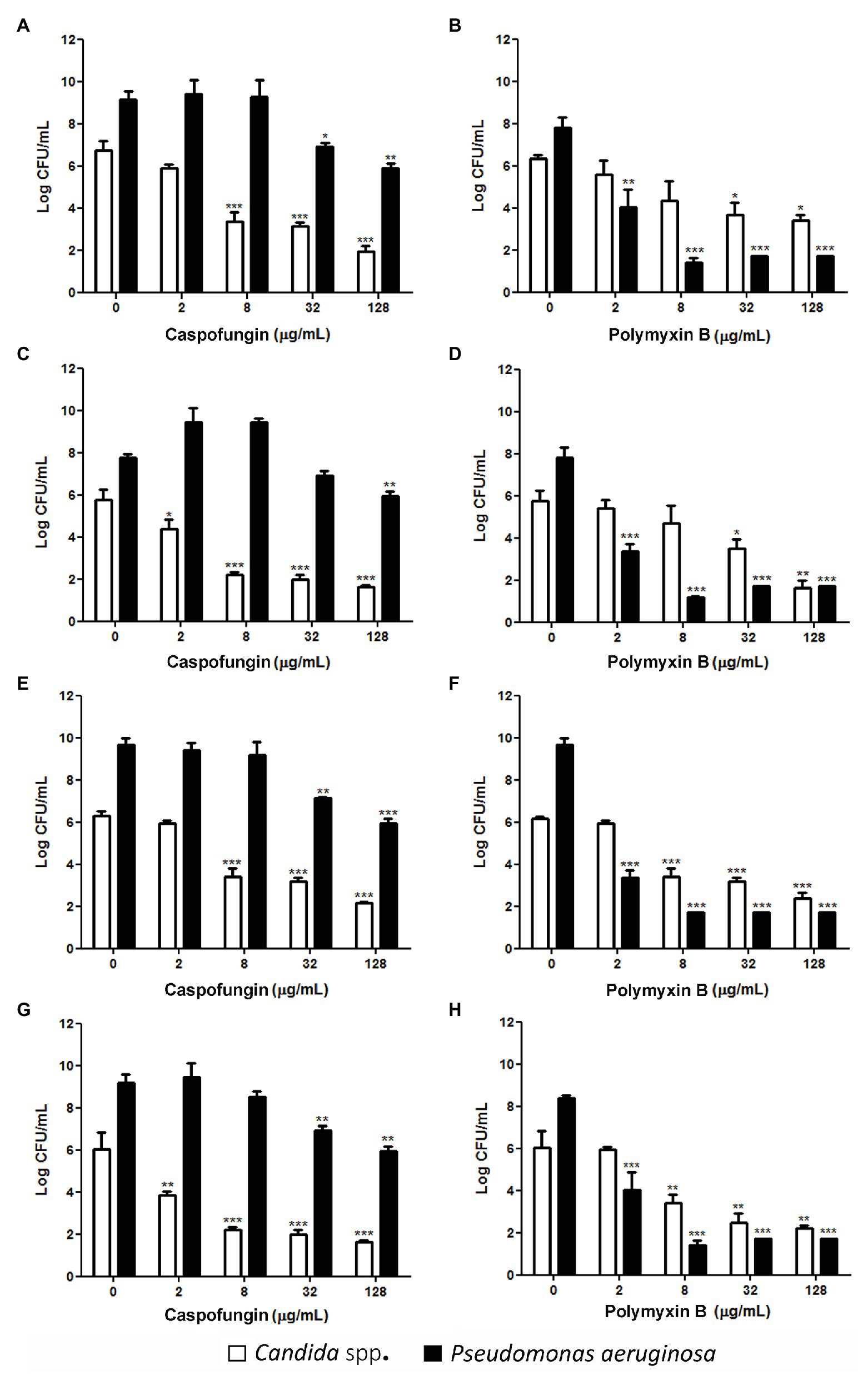
Figure 1. Count of viable cells of Candida spp. and P. aeruginosa 151 from polymicrobial biofilms after treatment with CAS or PMB. (A) and (B): C. albicans SC5314; (C) and (D): C. tropicalis ATCC 200956; (E) and (F): C. parapsilosis ATCC 22019; and (G) and (H): C. glabrata ATCC 2001. The results refer to the mean ± SD of three independent experiments in duplicate. * p < 0.05, ** p < 0.01, and *** p < 0.001 when compared with the respective untreated cells (group 0; on-way ANOVA with Dunnett’s posttest). Detection limit is 2 log colony forming unit (CFU)/ml.
Although echinocandins are known to be effective for the treatment of Candida biofilms, little is known about its effects on mixed biofilms formed by Candida spp. and bacterial pathogens. In fact, while antibacterial activity of echinocandins has been restricted to the evaluation of MFG against P. aeruginosa and S. aureus (Bazzi et al., 2013; Kissoyan et al., 2016; Lown et al., 2016; Rasheed et al., 2018), CAS has only been evaluated against S. aureus, so far (Siala et al., 2016; Moore et al., 2020). Interestingly, in this study, we report for the first time that CAS alone was able to inhibit and reduce the viability of P. aeruginosa, and this antibacterial activity was extended to critical priority MDR and carbapenemase-producing P. aeruginosa lineages belonging to the international sequence type ST277, which is endemic in Brazilian hospitals (Nascimento et al., 2016). In this regard, CAS inhibits 1,3-β-D-glucan synthesis in Candida spp.; therefore, since P. aeruginosa also has 1,3-β-D-glucan in its cell wall (Mennink-Kersten et al., 2008), the in vitro activity against planktonic and biofilm-forming P. aeruginosa could be expected.
The antifungal properties of polymyxins (PMB and colistin) on C. albicans and other fungal species have been previously described (Ogita et al., 2009; Zhai et al., 2010; Yousfi et al., 2019; Bidaud et al., 2020), but this activity has been directed against planktonic cells. In this study, while MIC values for planktonic Candida spp. were similar to those obtained in previous studies, we have observed additional inhibitory activity against Candida biofilms. The action of PMB on fungal cells is poorly understood, and some studies suggest effects on the fungal membrane (Zhai et al., 2010; Yousfi et al., 2019). This explanation could be plausible if we consider that polymyxins binds to the outer membrane of Gram-negative bacteria, where it complexes avidly with lipopolysaccharides showing an outer membrane-disorganizing action (Ayoub Moubareck, 2020). This primary damage in the outer membrane allows polymyxins to achieve the cytoplasmic membrane, where it causes leakage of cytoplasmic contents leading to bacterial death. Thus, it is evident that the membrane permeability changes immediately on contact with the drug. However, since activity of polymyxins is antagonized by Mg2+ and Ca2+ could be assumed that part of the action of this antibiotic is also due to the competitively displacing Mg2+ or Ca2+ from the negatively charged phosphate groups of membrane lipids (Ayoub Moubareck, 2020). Therefore, once cationic polymyxins interact with negatively charged moieties at the outer microbial cell structure, the negative charge of the fungal wall, conferred by mannoproteins that harbor phosphate groups (Ruiz-Herrera et al., 2006; Yousfi et al., 2019; Garcia-Rubio et al., 2020), would allow the coverage of fungal cells by polymyxins generating a positive charge on the cell surface with eventual disruption leading to antimicrobial effects. Interestingly, besides confer a negative charge to the cells, fungal glycoproteins containing abundant phosphodiester linkages provide hydrophilic or hydrophobic properties to the cell surface (Ruiz-Herrera et al., 2006; Yousfi et al., 2019), which could favor interaction with amphipathic polymyxin molecules. However, further studies should be conducted to clarify the exact mechanism of polymyxins against Candida spp.
The reduction of microbial viability of mixed biofilms after treatments with CAS or PMB is a relevant finding. The mean PMB maximum serum concentration (Cmax) at steady-state ranges from ~2 to 14 μg/ml after intravenous administration of clinically used dose (Avedissian et al., 2019), while CAS achieves 7–9 μg/ml after intravenous administration of recommended doses in adult patients (Groll et al., 2011). Then, concentrations ≥2 μg/ml of CAS or PMB were able to reduce significantly the viability of fungal and bacterial cells, respectively, from biofilms and achieving to undetectable levels at higher drug concentrations (Figure 1).
Here, we also evaluated the combination of CAS (32 or 64 μg/ml) with PMB (2 μg/ml) on mixed biofilms formed by P. aeruginosa strain 151 and Candida spp. (C. albicans, C. tropicalis, or C. glabrata; Figure 2). Both combinations were able to significantly reduce the total biomass of mixed biofilms, but mainly for those formed with C. glabrata ATCC 2001 (reduction of ca. 70%; Figure 2C). Moreover, a significant reduction of 1–2 logs in the viable fungal cells counting occurred in all fungus-bacterium interactions; however, for P. aeruginosa it occurred only when associated with C. tropicalis (Figure 2B). Although the antibiofilm effect of combinations of CAS with PMB was similar to the data obtained when monotherapies were used on mixed biofilms, the drug combinations are an important strategy to eradicate the bacterium-fungus biofilms, and then further studies should be conducted.
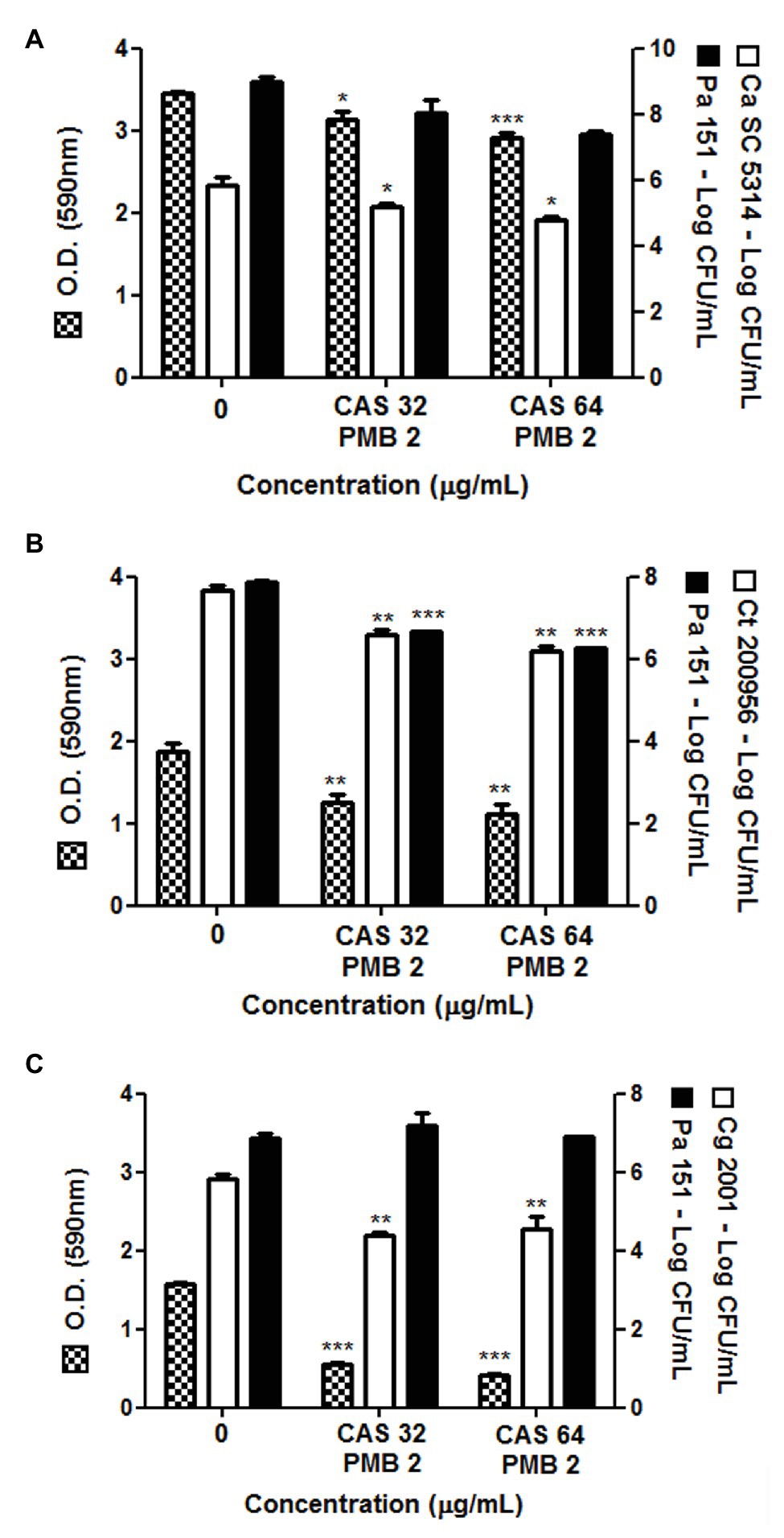
Figure 2. Total biomass and cell viability of pre-formed polymicrobial biofilms by P. aeruginosa 151 strain with different species of Candida treated with the combination of PMB (2 μg/ml) with CAS (32 or 64 μg/ml). Mixed biofilm of P. aeruginosa 151 formed with (A): C. albicans SC5314, (B): C. tropicalis ATCC 200956, and (C): C. glabrata ATCC 2001. The results refer to the mean ± SD of three independent experiments in duplicate. * p < 0.05, ** p < 0.01, and *** p < 0.001 when compared with the respective untreated biofilms (group 0; on-way ANOVA with Dunnett’s posttest). Detection limit for count of viable cells is 2 log CFU/ml.
Synergistic effect of PMB combined with membrane-targeting antifungals (i.e., azoles and polyenes) or CAS, against only planktonic yeasts, has been showed (Moneib, 1995; Zhai et al., 2010; Adams et al., 2016; Rodrigues et al., 2017; Liu et al., 2019; Yousfi et al., 2019). Previous works described synergistic effect of PMB combined with azole agents and also combined with CAS against Candida spp., actuating as a facilitating agent for antifungals entry into the cell (Adams et al., 2016; Yousfi et al., 2019). In contrast, it has been suggested that echinocandin-mediated weakening of cell wall facilitates colistin targeting of membrane, which in turn enhance the antifungal activity of echinocandins (Zeidler et al., 2013).
Our work shows for the first time the combination of PMB with a conventional antifungal (CAS) against mixed biofilms formed by P. aeruginosa and Candida spp. CAS combined or not with PMB was able to disrupt the mono- and polymicrobial mature biofilms of P. aeruginosa and Candida spp. reducing the total biomass, as well as microbial viability. The combined use of echinocandins with another antibacterial agent could facilitate the penetration of this drug into deeper biofilm layers due to reduction of EPM produced by Candida cells. In addition, echinocandins are capable of non-competitively inhibiting the glycosyltransferase encoded by the ndvB gene, which acts in the production of the bacterial β-(1,3)-D-glucan (Beaudoin et al., 2012) presents in P. aeruginosa cell wall and EPM from biofilms contributing to mixed biofilm susceptibility (Bazzi et al., 2013). In this regard, combined treatment of MFG with ceftazidime, levofloxacin, ciprofloxacin, or aztreonam increases the survival rates of mice infected with P. aeruginosa (Kissoyan et al., 2016). In addition, CAS combined with fluoroquinolones acts as facilitator agent for penetration of the antibacterials into the S. aureus biofilms (Siala et al., 2016), as well as anidulafungin, another echinocandin agent, acts synergistically when combined with tigecycline against in vivo intra-abdominal mixed biofilms formed by C. albicans and S. aureus (Rogiers et al., 2018). Therefore, the activity of echinocandins on fungi and bacteria can be an important advantage in the treatment of polymicrobial infections in which Candida species are involved.
Conclusion
Echinocandins, especially CAS, and PMB displayed in vitro activity against polymicrobial biofilms, reducing the total biomass and cell viability of Candida spp. and P. aeruginosa, including CR strains. Our results support the potential use of these antimicrobial agents to treat polymicrobial biofilm-mediated infections.
Data Availability Statement
The original contributions presented in the study are included in the article/Supplementary Material, further inquiries can be directed to the corresponding author.
Author Contributions
LF and BF performed the experiments, analyzed the results, and drafted the manuscript. KI and NL designed the experiments and wrote the manuscript. All authors contributed to the article and approved the submitted version.
Funding
This study was supported by Fundação de Amparo a Pesquisa do Estado de São Paulo (FAPESP, grant 2017/19374-9), Conselho Nacional de Desenvolvimento Científico e Tecnológico (CNPq, grant405556/2018-7), and Coordenação de Aperfeiçoamento de Pessoal de Nível Superior (CAPES). LF was a CAPES fellow and BF was a FAPESP fellow (finance code 001 and 2017/26076-4, respectively). KI and NL are research fellows of the CNPq (grants 303373/2019-9 and 312249/2017-9, respectively).
Conflict of Interest
The authors declare that the research was conducted in the absence of any commercial or financial relationships that could be construed as a potential conflict of interest.
Acknowledgments
We thank the National Institute for Quality and Safety Control (INCQS, Fiocruz, Rio de Janeiro/RJ, Brazil) for kindly providing the ATCC standard strains of Candida spp. and Dr. Márcia de Souza Carvalho Melhem from Adolfo Lutz Institute (IAL, São Paulo/SP, Brazil) for Candida albicans SC5314 strain.
Supplementary Material
The Supplementary Material for this article can be found online at: https://www.frontiersin.org/articles/10.3389/fmicb.2020.573263/full#supplementary-material
References
Adams, E. K., Ashcraft, D. S., and Pankey, G. A. (2016). In vitro synergistic activity of caspofungin plus polymyxin B against fluconazole-resistant Candida glabrata. Am J Med Sci 351, 265–270. doi: 10.1016/j.amjms.2015.12.014
Allison, D. L., Willems, H. M. E., Jayatilake, J. A. M. S., Bruno, V. M., Peters, B. M., and Shirtliff, M. E. (2016). Candida-bacteria interactions: their impact on human disease. Microbiol. Spectr. 4, 1–26. doi: 10.1128/microbiolspec.VMBF-0030-2016
Avedissian, S. N., Liu, J., Rhodes, N. J., Lee, A., Pais, G. M., Hauser, A. R., et al. (2019). A review of the clinical pharmacokinetics of polymyxin B. Antibiotics 8:31. doi: 10.3390/antibiotics8010031
Ayoub Moubareck, C. (2020). Polymyxins and bacterial membranes: a review of antibacterial activity and mechanisms of resistance. Membranes 10:181. doi: 10.3390/membranes10080181
Bandara, H. M. H. N., Yau, J. Y. Y., Watt, R. M., Jin, L. J., and Samaranayake, L. P. (2010). Pseudomonas aeruginosa inhibits in-vitro Candida biofilm development. BMC Microbiol. 10:125. doi: 10.1186/1471-2180-10-125
Bazzi, W., Sabra, A., Zahreddine, L., Khairallah, M. T., Baroud, M., Hadj, U., et al. (2013). The inhibitory effect of micafungin on biofilm formation by Pseudomonas aeruginosa. Biofouling 29, 909–915. doi: 10.1080/08927014.2013.816299
Beaudoin, T., Zhang, L., Hinz, A. J., Parr, C. J., and Mah, T. F. (2012). The biofilms-specific antibiotic resistance gene ndvB is important for expression of ethanol oxidation genes in Pseudomonas aeruginosa biofilms. J. Bacteriol. 194, 3128–3136. doi: 10.1128/JB.06178-11
Bidaud, A. L., Djenontin, E., Botterel, F., Chowdhary, A., and Dannaoui, E. (2020). Colistin interacts synergistically with echinocandins against Candida auris. Int. J. Antimicrob. Agents 55:105901. doi: 10.1016/j.ijantimicag.2020.105901
Boudarel, H., Mathias, J. D., Blaysat, B., and Grédiac, M. (2018). Towards standardized mechanical characterization of microbial biofilms: analysis and critical review. NPJ Biofilms Microbiomes 4:17. doi: 10.1038/s41522-018-0062-5
Clinical and Laboratory Standards Institute (2017). Reference method for broth dilution antifungal susceptibility testing of yeasts. CLSI standard M27. 4th Edn. Wayne, PA: CLSI
Clinical and Laboratory Standards Institute (2020). Performance standards for antimicrobial susceptibility testing; twenty-nine informational supplement. CLSI document M100-S30. Wayne, PA: CLSI.
Coulon, C., Vinogradov, E., Filloux, A., and Sadovckaya, I. (2010). Chemical analysis of cellular and extracellular carbohydrates of a biofilm-forming strain Pseudomonas aeruginosa PA14. PLoS One 5:e14220. doi: 10.1371/journal.pone.0014220
Denning, D. W. (2003). Echinocandin antifungal drugs. Lancet 362:1142. doi: 10.1016/S0140-6736(03)14472-8
Desai, J. V., Mitchell, A. P., and Andes, D. R. (2014). Fungal biofilms, drug resistance, and recurrent infection. Cold Spring Harb.Perspect. Med. 4:a019729. doi: 10.1101/cshperspect.a019729
Deveau, A., Bonito, G., Uehling, J., Paoletti, M., Becker, M., Bindschedler, S., et al. (2018). Bacterial-fungal interactions: ecology, mechanisms and challenges. FEMS Microbiol. Rev. 42, 335–352. doi: 10.1093/femsre/fuy008
Fontes, L. C., Neves, P. R., Oliveira, S., Silva, K. C., Hachich, E. M., Sato, M. I. Z., et al. (2011). Isolation of Pseudomonas aeruginosa coproducing metallo-β-lactamase SPM-1 and 16S rRNA methylase RmtD1 in an urban river. Antimicrob. Agents Chemother. 55, 3063–3064. doi: 10.1128/AAC.00138-11
Forastiero, A., Mesa-Arango, A. C., Alastruey-Izquierdo, A., Alcazar-Fuoli, L., Bernal-Martinez, L., Pelaez, T., et al. (2013). Candida tropicalis antifungal cross-resistance is related to different azole target (Erg11p) modifications. Antimicrob. Agents Chemother. 57, 4769–4781. doi: 10.1128/AAC.00477-13
Freitas, A. L. D., Kaplum, V., Rossi, D. C. P., Silva, L. B. R., Melhem, M. S. C., Taborda, C. P., et al. (2018). Proanthocyanidin polymeric tannins from Stryphnodendron adstringens are effective against Candida spp. isolates and for vaginal candidiasis treatment. J. Ethnopharmacol. 216, 184–190. doi: 10.1016/j.jep.2018.01.008
Garcia-Rubio, R., de Oliveira, H. C., Rivera, J., and Trevijano-Contador, N. (2020). The fungal cell wall: Candida, Cryptococcus, and Aspergillus species. Front. Microbiol. 10:2993. doi: 10.3389/fmicb.2019.02993
Groll, A. H., Schrey, D., and Walsh, T. J. (2011). “Echinocandins” in Essentials of clinical mycology. eds. C. A. Kauffman, P. G. Pappas, J. D. Sobel, and W. E. Dismukes (New York: Springer), 95–112.
Hogan, D. A., and Kolter, R. (2002). Pseudomonas-Candida interactions: an ecological role for virulence factors. Science 296, 2229–2232. doi: 10.1126/science.1070784
Ibrahim, O., Overman, J., Arndt, K. A., and Dover, J. (2018). Filler nodules: inflammatory or infectious? A review of biofilms and their implications on clinical practice. Dermatol. Surg. 44, 53–60. doi: 10.1097/DSS.0000000000001202
Kim, D., Liu, Y., Benhamou, R. I., Sanchez, H., Simón-Soro, A., Li, Y., et al. (2018). Bacterial-derived exopolysaccharides enhance antifungal drug tolerance in a cross-kingdom oral biofilm. ISME J. 12, 1427–1442. doi: 10.1038/s41396-018-0113-1
Kissoyan, K. A. B., Bazzi, W., Hadi, U., and Matar, G. M. (2016). The inhibition of Pseudomonas aeruginosa biofilm formation by micafungin and the enhancement of antimicrobial agent effectiveness in BALB/c mice. Biofouling 32, 779–786. doi: 10.1080/08927014.2016.1199021
Klotz, S. A., Chasin, B. S., Powell, B., Gaur, N. K., and Pilke, P. N. (2007). Polymicrobial bloodstream infections involving Candida species: analysis of patients and review of the literature. Diagn. Microbiol. Infect. Dis. 59, 401–406. doi: 10.1016/j.diagmicrobio.2007.07.001
Lee, C. H., Chen, Y. C., Chen, I. L., Chen, F. J., and Chien, C. C. (2020). Impact of biofilm production by Candida species and antifungal therapy on mortality of patients with candidemia. Mycoses 63, 1382–1391. doi: 10.1111/myc.13179
Lequette, Y., Rollet, E., Delangle, A., Greenbergi, E. P., and Bohin, J. P. (2007). Linear osmoregulated periplasmic glucans are encoded by the opgGH locus of Pseudomonas aeruginosa. Microbiology 153, 3255–3263. doi: 10.1099/mic.0.2007/008953-0
Li, W. -S., Chen, Y. -C., Kuo, S. -F., Chen, F. -J., and Lee, C. -H. (2018). The impact of biofilm formation on the persistence of Candidemia. Front. Microbiol. 9:1196. doi: 10.3389/fmicb.2018.01196
Liu, Y., Wang, W., Yan, H., Wang, D., Zhang, M., and Sun, S. (2019). Anti-Candida activity of existing antibiotics and their derivatives when used alone or in combination with antifungals. Future Microbiol. 14, 899–915. doi: 10.2217/fmb-2019-0076
Lown, L., Peters, B. M., Walraven, C. J., Noverr, M. C., and Lee, S. A. (2016). An optimized lock solution containing micafungin, ethanol and doxycycline inhibits Candida albicans and mixed C. albicans—Staphyloccoccus aureus biofilms. PLoS One 11:e0159225. doi: 10.1371/journal.pone.0159225
Magiorakos, A. P., Srinivasan, A., Carey, R. B., Carmeli, Y., Falagas, M. E., Giske, C. G., et al. (2012). Multidrug-resistant, extensively drug-resistant and pandrug-resistant bacteria: an international expert proposal for interim standard definitions for acquired resistance. Clin. Microbiol. Infect. 18, 268–281. doi: 10.1111/j.1469-0691.2011.03570.x
Mennink-Kersten, M. A., Ruegebrink, D., and Verweij, P. E. (2008). Pseudomonas aeruginosa as a cause of 1,3-beta-D-glucan assay reactivity. Clin. Infect. Dis. 46, 1930–1931. doi: 10.1086/588563
Moneib, N. A. (1995). In-vitro activity of commonly used antifungal agents in the presence of rifampin, polymyxin B and norfloxacin against Candida albicans. J. Chemother. 7, 525–529. doi: 10.1179/joc.1995.7.6.525
Moore, J. A., Meakin, M., Earl, M. H., Kummer, T. M., McAleer, J. P., and Long, T. E. (2020). Effects of caspofungin, tolcapone and other FDA-approved medications on MRSA susceptibility to vancomycin. J. Glob. Antimicrob. Resist. 22, 283–289. doi: 10.1016/j.jgar.2020.03.014
Mukherje, P., Zhou, G., Munyon, R., and Ghannoum, M. A. (2005). Candida biofilm: a well-designed protected environment. Med. Mycol. 43, 191–208. doi: 10.1080/13693780500107554
Nascimento, A. P., Ortiz, M. F., Martins, W. M., Morais, G. L., Fehlberg, L. C., Almeida, L. G., et al. (2016). Intraclonal genome stability of the metallo-β-lactamase SPM-1-producing Pseudomonas aeruginosa ST277, an endemic clone disseminated in Brazilian hospitals. Front. Microbiol. 7:1946. doi: 10.3389/fmicb.2016.01946
Ogita, A., Konishi, Y., Borjihan, B., Fujita, K., and Tanaka, T. (2009). Synergistic fungicidal activities of polymyxin B and ionophores, and their dependence on direct disruptive action of polymyxin B on fungal vacuole. J. Antibiot. 62, 81–87. doi: 10.1038/ja.2008.13
Pappas, P. G., Kauffman, C. A., Andes, D. R., Clancy, A. J., Marr, K. A., Ostrosky-Zeichner, L., et al. (2016). Clinical practice guideline for the management of candidiasis: 2016 update by the infectious diseases society of America. Clin. Infect. Dis. 62, e1–e50. doi: 10.1093/cid/civ933
Peleg, A. Y., Hogan, D. A., and Mylonakis, E. (2010). Medically important bacterial-fungal interactions. Nat. Rev. Microbiol. 8, 340–349. doi: 10.1038/nrmicro2313
Peters, B. B., Jabra-Rizk, M. A., O’May, G. A., Costerton, W., and Shirtliff, E. (2012). Polymicrobial interactions: impact on pathogenesis and human disease. Clin. Microbiol. Rev. 25, 193–213. doi: 10.1128/CMR.00013-11
Pogue, J. M., Jones, R. N., Bradley, J. S., Andes, D. R., Bhavnani, S. M., Drusano, G. L., et al. (2020). Polymyxin susceptibility testing and interpretive breakpoints: recommendations from the United States committee on antimicrobial susceptibility testing (USCAST). Antimicrob. Agents Chemother. 64, e01495–e01419. doi: 10.1128/AAC.01495-19
Qu, Y., Locock, K., Verma-Gaur, J., Hay, I. D., Meagher, L., and Traven, A. (2016). Searching for new strategies against polymicrobial biofilm infections: guanylated polymethacrylates kill mixed fungal/bacterial biofilms. J. Antimicrob. Chemother. 71, 413–421. doi: 10.1093/jac/dkv334
Ramage, G., Saville, S. P., Thomas, D. P., and López-Ribot, J. L. (2005). Candida biofilms: an update. Eukaryot. Cell 4, 633–638. doi: 10.1128/EC.4.4.633-638.2005
Rasheed, S., Kissoyan, K., Hadi, U., El Sabban, M., and Matar, G. (2018). Assessing the effect of micafungin on Pseudomonas aeruginosa biofilm formation using confocal microscopy and gene expression. J. Infect. Dev. Ctries. 12:8S. doi: 10.3855/jidc.10091
Rodrigues, M. E., Lopes, S. P., Pereira, C. R., Azevedo, N. F., Lourenço, A., Henriques, M., et al. (2017). Polymicrobial ventilator-associated pneumonia: fighting in vitro Candida albicans-Pseudomonas aeruginosa biofilms with antifungal-antibacterial combination therapy. PLoS One 12:e0170433. doi: 10.1371/journal.pone.0170433
Rogiers, O., Holtappels, M., Siala, W., Lamkanfi, M., Van Bambeke, F., Lagrou, K., et al. (2018). Anidulafungin increases the antibacterial activity of tigecycline in polymicrobial Candida albicans/Staphylococcus aureus biofilms on intraperitoneally implanted foreign bodies. J. Antimicrob. Chemother. 73, 2806–2814. doi: 10.1093/jac/dky246
Ruiz-Herrera, J., Elorza, M. V., Valentín, E., and Sentandreu, R. (2006). Molecular organization of the cell wall of Candida albicans and its relation to pathogenicity. FEMS Yeast Res. 6, 14–29. doi: 10.1111/j.1567-1364.2005.00017.x
Sabaeifard, P., Abdi-Ali, A., Soudi, M. R., and Dinarvand, R. (2014). Optimization of tetrazolium salt assay for Pseudomonas aeruginosa biofilm using microtiter plate method. J. Microbiol. Methods 105, 134–140. doi: 10.1016/j.mimet.2014.07.024
Santos, J. D., Piva, E., Vilela, S. F. G., Jorge, A. O. C., and Junqueira, J. C. (2016). Mixed biofilms formed by C. albicans and non-albicans species: a study of microbial interactions. Braz. Oral Res. 30:e23. doi: 10.1590/1807-3107BOR-2016.vol30.0023
Scudiero, D. A., Showmaker, R. H., Paull, K. D., Monks, A., Tierney, S., Nofziger, T. H., et al. (1988). Evaluation of a soluble tetrazolium/formazan assay for cell growth and drug sensitivity in culture using human and other tumor cell lines. Cancer Res. 48, 4827–4833.
Siala, W., Kucharíková, S., Vleugels, J., Tulkens, P. M., Mingeot-Leclercq, M. P., Van Dijck, P., et al. (2016). The antifungal caspofungin increases fluoroquinolone activity against Staphylococcus aureus biofilms by inhibiting N-acetylglucosamine transferase. Nat. Commun. 7:13286. doi: 10.1038/ncomms13286
Toleman, M. A., Simm, A. M., Murphy, T. A., Gales, A. C., Biedenbach, D. J., Jones, R. N., et al. (2002). Molecular characterization of SPM-1, a novel metallo-β-lactamase isolated in Latin America: report from the SENTRY antimicrobial surveillance programme. J. Antimicrob. Chemother. 50, 673–679. doi: 10.1093/jac/dkf210
Trejo-Hernandez, A., Andrade-Domínguez, A., Hernández, M., and Encarnación, S. (2014). Interspecies competition triggers virulence and mutability in Candida albicans-Pseudomonas aeruginosa mixed biofilms. ISME J. 8, 1974–1988. doi: 10.1038/ismej.2014.53
Tumbarello, M., Posteraro, B., Trecarichi, E. M., Fiori, B., Rossi, M., Porta, R., et al. (2007). Biofilm production by Candida species and inadequate antifungal therapy as predictors of mortality for patients with candidemia. J. Clin. Microbiol. 45, 1843–1850. doi: 10.1128/JCM.00131-07
Turano, H., Gomes, F., Medeiros, M., Oliveira, S., Fontes, L., Sato, M. I., et al. (2016). Presence of high-risk clones of OXA-23-producing Acinetobacter baumannii (ST79) and SPM-1-producing Pseudomonas aeruginosa (ST277) in environmental water samples in Brazil. Diagn. Microbiol. Infect. Dis. 86, 80–82. doi: 10.1016/j.diagmicrobio.2016.06.005
Vila, T., Ishida, K., Seabra, S. H., and Rozental, S. (2016). Miltefosine inhibits Candida albicans and non-albicans Candida spp. biofilms and impairs the dispersion of infectious cells. Int. J. Antimicrob. Agents 48, 512–520. doi: 10.1016/j.ijantimicag.2016.07.022
Vitkauskienė, A., Skrodenienė, E., Dambrauskienė, A., Macas, A., and Sakalauskas, R. (2010). Pseudomonas aeruginosa bacteremia: resistance to antibiotics, risk factors, and patient mortality. Medicina 46, 490–495. doi: 10.3390/medicina46070071
Wilson, C., Lukowicz, R., Merchant, S., Valquier-Flynn, H., Caballero, J., Sandoval, J., et al. (2017). Quantitative and qualitative assessment methods for biofilm growth: a mini-review. Res. Rev. J. Eng. Technol. 6, 1–42.
Yousfi, H., Ranque, S., Rolain, J. M., and Bittar, F. (2019). In vitro polymyxin activity against clinical multidrug-resistant fungi. Antimicrob. Resist. Infect. Control 8:66. doi: 10.1186/s13756-019-0521-7
Zeidler, U., Bougnoux, M. E., Lupan, A., Helynck, O., Doyen, A., Garcia, Z., et al. (2013). Synergy of the antibiotic colistin with echinocandin antifungals in Candida species. J. Antimicrob. Chemother. 68, 1285–1296. doi: 10.1093/jac/dks538
Keywords: antifungal, echinocandin, micafungin, polymicrobial biofilm, antibiofilm effect, resistance
Citation: Fernandes L, Fortes BN, Lincopan N and Ishida K (2020) Caspofungin and Polymyxin B Reduce the Cell Viability and Total Biomass of Mixed Biofilms of Carbapenem-Resistant Pseudomonas aeruginosa and Candida spp. Front. Microbiol. 11:573263. doi: 10.3389/fmicb.2020.573263
Edited by:
Miguel Cacho Teixeira, University of Lisbon, PortugalReviewed by:
Maurizio Sanguinetti, Catholic University of the Sacred Heart, ItalyMariana Henriques, University of Minho, Portugal
Copyright © 2020 Fernandes, Fortes, Lincopan and Ishida. This is an open-access article distributed under the terms of the Creative Commons Attribution License (CC BY). The use, distribution or reproduction in other forums is permitted, provided the original author(s) and the copyright owner(s) are credited and that the original publication in this journal is cited, in accordance with accepted academic practice. No use, distribution or reproduction is permitted which does not comply with these terms.
*Correspondence: Kelly Ishida, ishidakelly@usp.br; ishidakelly@gmail.com