- 1Enzyme and Microbial Technology Research Centre, Faculty of Biotechnology and Biomolecular Sciences, Universiti Putra Malaysia, UPM Serdang, Selangor, Malaysia
- 2Department of Microbiology, Faculty of Biotechnology and Biomolecular Sciences, Universiti Putra Malaysia, UPM Serdang, Selangor, Malaysia
- 3Department of Biochemistry, Faculty of Biotechnology and Biomolecular Sciences, Universiti Putra Malaysia, UPM Serdang, Selangor, Malaysia
The world today is in a quest for new means of environmental remediation as the methods currently used are not sufficient to halt the damage. Mostly, a global direction is headed toward a shift from traditional chemical-based methods to a more ecofriendly alternative. In this context, biocatalysis is seen as a cost-effective, energy saving, and clean alternative. It is meant to catalyze degradation of recalcitrant chemicals in an easy, rapid, green, and sustainable manner. One already established application of biocatalysis is the removal of dyes from natural water bodies using enzymes, notably oxidoreductases like laccases, due to their wide range of substrate specificity. In order to boost their catalytic activity, various methods of enhancements have been pursued including immobilization of the enzyme on different support materials. Aside from increased catalysis, immobilized laccases have the advantages of higher stability, better durability against harsh environment conditions, longer half-lives, resistance against protease enzymes, and the ability to be recovered for reuse. This review briefly outlines the current methods used for detoxification and decolorization of dye effluents stressing on the importance of laccases as a revolutionary biocatalytic solution to this environmental problem. This work highlights the significance of laccase immobilization and also points out some of the challenges and opportunities of this technology.
Introduction
Throughout the last century, several industries have inflicted a high demand for synthetic dyes. An estimate of 700,000 tons of different synthetic dyes are produced annually (Holkar et al., 2016; Bilal et al., 2017; Katheresan et al., 2018). Those dyes have stable chemical structures that make them resistant to degradation by heat, light, or water (Lu et al., 2012a,b; Singh and Gupta, 2020). Some even form water-soluble complexes that are toxic to human, animal, and marine life (Bilal et al., 2019). Moreover, hazardous chemicals used in the dye industry, like corrosive acids, hydrogen peroxide, and caustic soda, are abundantly found in dye wastewater (Katheresan et al., 2018). Major industries as leather and textile are guilty of discarding untreated used dyes in waste water. Other high dye-demanding fields, including electroplating, paper, pulp, tannery, plastic, pharmaceutical, and cosmetic industries (Husain, 2010), are also responsible of causing irreparable damage to the ecosystem through the way they release their wastes containing dyes into clean water bodies (Holkar et al., 2016; Katheresan et al., 2018; Shakerian et al., 2020).
The conventional chemical processes currently used are effective in dye degradation; nevertheless, they lead to the production of toxic intermediate products (Bilal et al., 2017, 2019; Rasheed et al., 2019). Consequently, utilizing technologies that are effective, cheap, and environmentally friendly are being highly favored by governments and dye manufacturers (Ashrafi et al., 2013; Sharma et al., 2018; Deska and Kończak, 2019; Shakerian et al., 2020). Accordingly, biocatalysis is considered a clean process of dye degradation. Various biocatalysts like oxidoreductases are used in degrading hazardous compounds, including phenolic pollutants, and natural or synthetic dye wastes (Bilal et al., 2018, 2019). Laccase is one widely used oxidoreductase catalyst of interest due to its catalysis potential of different dyes (Bilal et al., 2019). As a matter of fact, new sources for fungal and bacterial laccases have been increasingly demanded to degrade dye effluents, or most commonly known as dye waste (Dauda and Erkurt, 2020; Jeon and Park, 2020; Joshi et al., 2020; Singh and Gupta, 2020).
In the past decade, scientists have been working on the development of novel immobilization methods and support materials for laccases to improve their performance and reusability. This improvement is due to the noteworthy enhancement in pH and thermal stability profile range, as well as the capability of working under a wider range of environmental conditions (Dai et al., 2016; Ba and Vinoth Kumar, 2017; Skoronski et al., 2017; Ali et al., 2020).
The aim of this review is to highlight the recent and distinctive application of immobilized laccases in the degradation of dyes in waste water, and how enzyme immobilization enhanced its biocatalysis activity. Finally, limitations and future opportunities of using immobilized laccase are discussed.
Recent Dye Removal Methods
Dyes are complex unsaturated organic molecules that are able to absorb light and give color, through reflecting the fraction of light not absorbed by the dye. They are categorized based on their chromophore structure, particle charge after dissolution, color index number, and industrial application as summarized in Supplementary Table 1 (Hunger, 2004; Yagub et al., 2014; Zhou et al., 2019; Benkhaya et al., 2020). Dyes and metal ions are the most prevalent detrimental materials found in dye wastewater that are very harmful to water and soil (Gosavi and Sharma, 2014; Holkar et al., 2016). Attention has been drawn lately toward remediation of dye wastewater for reuse, due to scarcity of clean natural water sources. An effective dye removal method ideally does its task rapidly, cost effectively, and without producing secondary contaminants (Rodríguez-Couto et al., 2009; Katheresan et al., 2018). The current established dye removal methods are classified into three main categories: physical, oxidation, and biological methods.
Physical
The first physical dye removal method is the coagulation (flocculation) method. This method is mainly used for good removal of disperse dyes (Liang et al., 2014; Yeap et al., 2014), but it has the disadvantage of increased generation of sludge volume (Crini and Lichtfouse, 2019). Another physical method is adsorption, which has higher efficiency in discoloring more types of dyes than the coagulation method (Jadhav and Srivastava, 2013). It is also considered a cheap method of water remediation if low cost adsorbents, like polymeric resins and bentonite clay, are used, but this is not a cost-effective method as the adsorbent is usually used once, generating sludge, with no chance of regeneration for future use (Gupta et al., 2011). Third is the filtration method, where techniques like reverse osmosis and ultrafiltration are used to restore the effluent dye for commercial reuse, but the constant problems of these techniques are the high cost of filtration membranes and their maintenance (Holkar et al., 2016; Katheresan et al., 2018).
Oxidation
This is an easily applied method for dye degradation; hence, it is the most commonly used, starting with advanced oxidation processes, where they can oxidize a wide range of chemicals, including organic and inorganic compounds found in wastewater, but it has the limitation of forming a precipitating sludge (Babuponnusami and Muthukumar, 2014). Then there are the chemical oxidation processes that are very powerful and can break down even the chemical structures of dyes with double bonds and complex aromatic rings using ozone (O3) molecules (Asghar et al., 2015). Nevertheless, using these molecules releases toxic secondary products as well as being expensive to purchase (Holkar et al., 2016). Finally, there are the synergistic hybrid advanced oxidation processes. It is a combination of the two previous methods, which is advised to be used when synergistic oxidative decolorization effect is desired (Holkar et al., 2016). However, it still has the disadvantages of the previous two methods.
Biological
This method is the most preferred nowadays for degrading, detoxifying, and remediation of recalcitrant dyes from factory effluents. Its mechanism is based on the adaptability of the selected microorganisms and the strength of the biological enzymes either secreted directly from microorganisms or free enzymes (Solís et al., 2012). Preference of biological methods over physical and oxidation practices is attributed to their environmental benefits as there is no production of hazardous byproducts as well as less sludge formation. Moreover, it is a cost-effective method, which makes it a better candidate to be used in industrial scale (Hayat et al., 2015). Up to this point, many microorganisms and their enzymes have been studied and tested for their potential ability in degrading dyes found in wastewater (D’Souza-Ticlo et al., 2009; Wikee et al., 2019). Apart from the enzyme source, those enzymes degrade dyes through biocatalytic oxidation of their chromophores; hence, the increased interest in studying proprieties of different biocatalysts lately (Chapman et al., 2018). A number of research reports have documented the high efficiency of using enzymes in dye removal (Chiong et al., 2016; Yang X. et al., 2016; Katheresan et al., 2018; Kashefi et al., 2019b).
As promising as using enzymes commercially may seem, they still have their limitations like low stability and lack of recovery decreasing their potential for reuse. Also, rapid loss of catalytic activity may occur in case of altering their favorable operating conditions. Hence, they usually fail to perform under harsh industrial conditions. Therefore, new methods are being developed to enhance the durability of enzymes through immobilization. This is particularly crucial when intracellular enzymes are desired to be used in a cell free system. Nowadays, the usage of enzymes immobilized on solid carriers is gaining popularity more than the free enzyme, as immobilization stabilizes the protein structure giving it longer shelf-life, resistance against proteases, thermal and pH stability, and repeatability of use, thus, reducing operational cost, which makes a good candidate for commercial and industrial use (Dodor et al., 2004; Fernández-Fernández et al., 2013; Nair et al., 2013; Li et al., 2014; Sun et al., 2015; Bilal et al., 2019; Deska and Kończak, 2019).
Immobilized Laccase in Removal of Dyes
One enzyme of interest that has been around for some time, is laccase. Scientists have regained interest in it due to its promising catalytic and physiochemical properties, making it the perfect candidate for bioremediation processes (Rao et al., 2014; Legerska et al., 2016). Laccase is a monomeric, dimeric, or a tetrameric glycoprotein, which oxidizes a broad spectrum of phenolic and non-phenolic substrates (Giardina et al., 2010). By now, many laccases have been discovered, and as a family, they show different structures and functions depending on their source as they can be widely found in eukaryotes as well as prokaryotes (Deska and Kończak, 2019); including higher plants, bacteria, insects, fungi, and recently mammals (Giardina et al., 2010; Janusz et al., 2020). As for the mechanism of action of laccases, they catalyze three types of reactions using oxygen atom and releasing water molecule: (i) direct oxidation of phenolic substrates, (ii) indirect oxidation of non-phenolic substrates with high redox potential in the presence of a natural or synthetic low molecular weight mediator (Breen and Singleton, 1999; Agrawal et al., 2018), and (iii) coupling reactions with reactive intermediate radicals formed during direct oxidation (Polak and Jarosz-Wilkolazka, 2012). The active site of laccases, where biocatalysis occurs, has three copper centers/types/domains; (1) blue copper center (type I), (2) normal copper center (type II), and (3) coupled binuclear copper centers (type 3) (Messerschmidt and Huber, 1990; Solomon et al., 1992; Dwivedi et al., 2011).
A great number of research articles have reported the increased biocatalytic performance of laccase for a longer stretch of time when immobilized. As a general definition, to immobilize an enzyme is when the soluble form of the enzyme is attached to a solid support or, most recently, through formation of aggregates. In this context, different ways of immobilization include adsorption on beads or a matrix, covalent binding to a solid support, entrapment or encapsulation in polymers, and crosslinking as illustrated in Supplementary Figure 1 (Shrivastava et al., 2012; Datta et al., 2013; Sirisha et al., 2016). Whichever method is chosen, one point ought to be taken into consideration: no immobilization method should influence the enzymatic conformation so that the activity shall not be affected (Shaheen et al., 2017). Other factors to bear in mind while considering the different immobilization methods shall be more elaborated in Supplementary Data 1. A simple proposed mechanism of dye degradation by immobilized laccase is illustrated in Figure 1 with the aid of the crystal structure of laccase from Thermus thermophilus HB27 (PDB code: 5JRR). In the figure, the dye structure, substrate, is being oxidized by only oxygen in the presence of laccase as a catalyst. When the dye is oxidized, it loses an electron that moves from one Cu2+ atom to another inside the catalytic site, until it eventually reduces oxygen to release water. More information about laccase-catalyzed descoloration of dyes and the exact oxidized products have been reported in literature (Zille et al., 2005; Yang et al., 2015; Kagalkar et al., 2015).
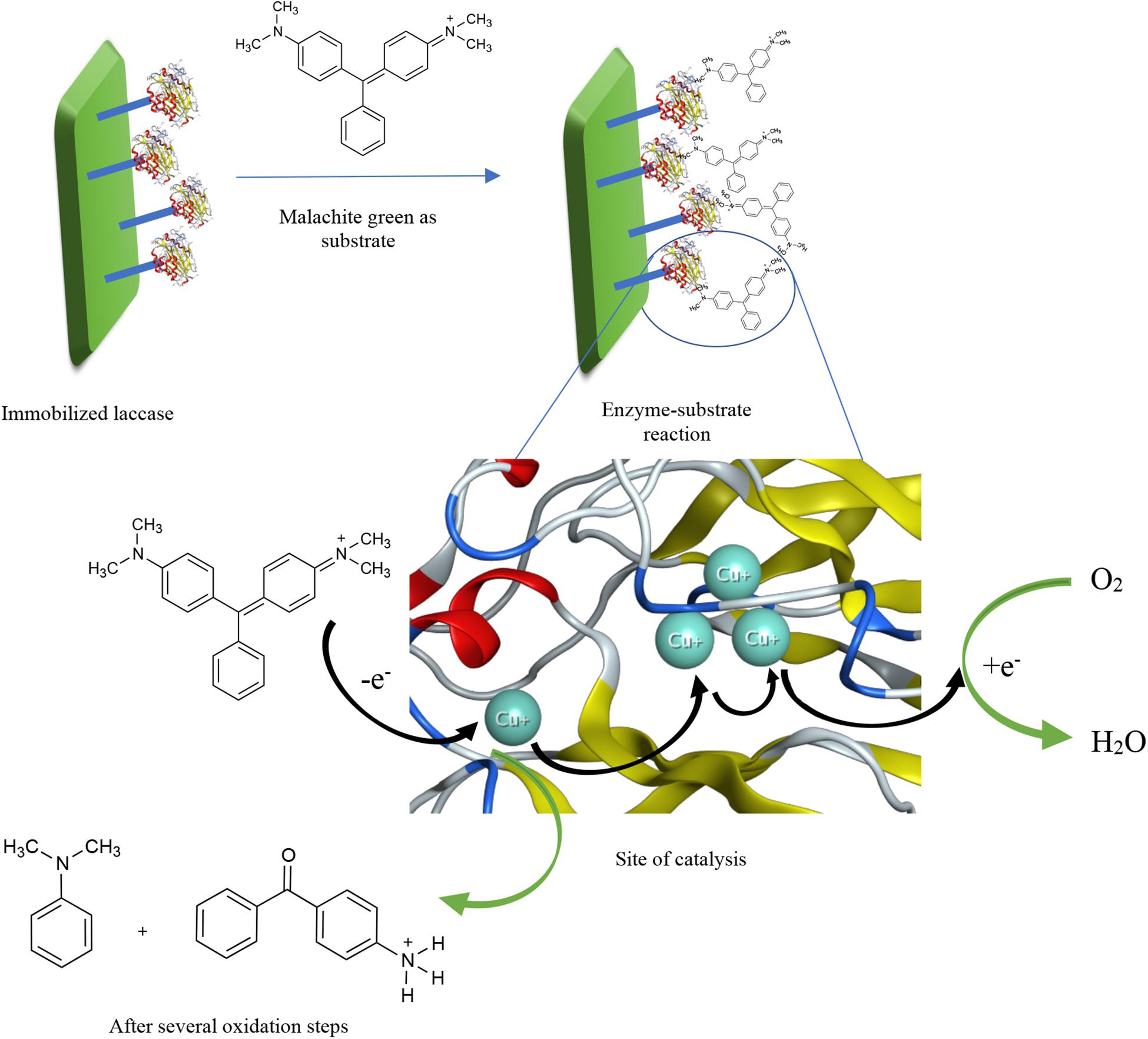
Figure 1. A proposed mechanism of dye degradation by immobilized laccase with the aid of the crystal structure of laccase from Thermus thermophilus HB27 (PDB code: 5JRR).
Immobilized laccases proved to be worthy challengers for the effective decolorization, degradation, and removal of dyes (Bilal et al., 2019). For example, laccase from the Cerrena sp. strain HYB07 was immobilized by preparing cross-linked enzyme aggregates (CLEAs) of the enzyme to degrade Remazol Brilliant Blue Reactive dye. Almost 90% of the dye was eliminated from the solution in just 40 min without the help of a redox mediator (Yang J. et al., 2016). In another study, Arica et al. (2017) demonstrated the immobilization of laccase on fibrous polymer-grafted polypropylene chloride film, where three dyes, namely, Procion Green H4G, Brilliant Blue G, and Crystal Violet, were tested for removal by both the free and immobilized enzyme. The immobilized laccase gave better dye degradation results than the free one (Arica et al., 2017).
In addition to the improved biocatalytic properties, immobilized laccase shows notable storage stability and repeated use capability, while maintaining satisfactory efficiency (Deska and Kończak, 2019). In a study, by Ma et al. (2018), genipin-activated chitosan beads were introduced as a highly stable laccase biocatalyst from Trametes pubescens. The resulting immobilized laccase reached to a decolorization of 77.49% of Acid Black 172 dye. Moreover, the carrier-supported laccase displayed excellent reuse potential with > 55% of remaining activity reserved after 11 cycles of constant use. It also showed high storage stability retaining over 57.14% of its original activity after 30 days of storage at 4°C (Ma et al., 2018).
Also, increased pH stability is a notable characteristic of the immobilized laccase as stated by Wen et al. (2019), when the enzyme was physically adsorbed on kaolinite for the removal of malachite green dye. The immobilized biocatalyst exhibited outstanding stability over a broad pH range from 3 to 6, with the lowest relative activity being 60% at pH 5.5 and the highest is 100% at pH 4.5. The authors justified this enhanced pH tolerance to a relative stable proton production on the surface of support and assured that it could retain the activity of laccase (Wen et al., 2019).
Furthermore, immobilized catalysts often show remarkable improved thermal steadiness than free enzymes. A recent report presented a novel support carrier; laccase covalently bound to crosslinked graphene oxide–zeolite nanocomposites. The obtained catalytic system was exploited for the degradation of Direct Red 23 dye. Notably, the immobilized laccase was very stable at 80°C and preserved 84% of its initial catalytic activity, unlike its free counterpart, which could only retain 18%. The author attributed this thermal protection to the covalent bonding between laccase and supporting carrier (Mahmoodi and Saffar-Dastgerdi, 2020).
Many literature reports confirm that using immobilized laccases is a favorable technology in the treatment of dye effluents. Several recent reports regarding this matter are listed in Table 1.
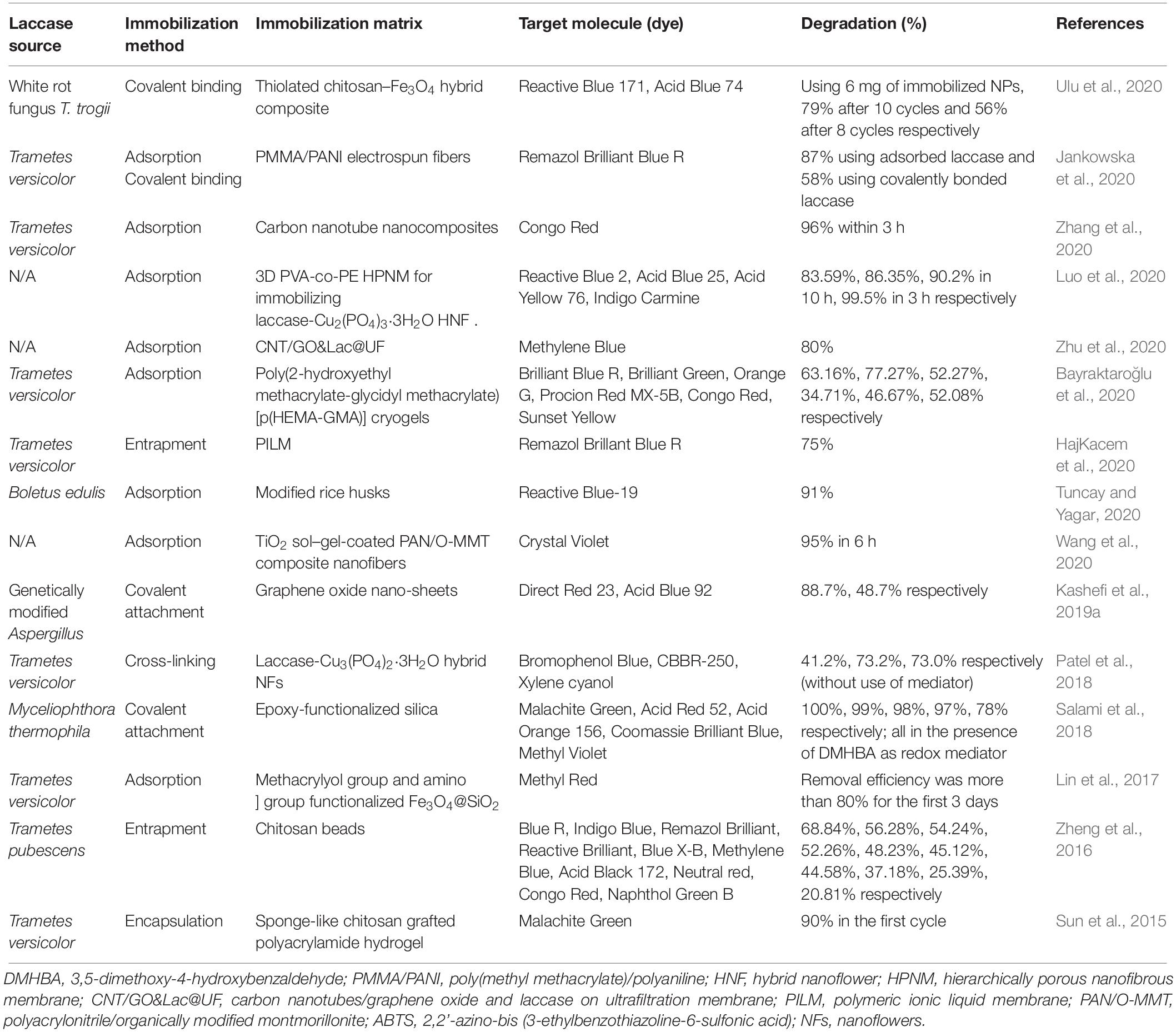
Table 1. Some recent reports about the degradation of dyes by immobilized laccases from different microbial sources.
Limitations and Future Opportunities
As narrated throughout this minireview, the current established dye treatment methodologies still have, to some extent, several limitations. The fact that no certain decolorization method is adopted universally for all types of dye effluents needs more study, as currently, using a single dye removal method, either physical, oxidation, or biological, is not enough. Using a combination of different methods may be attributed to the complex and different chromophore structures of dying compounds. It is undeniable that the current enzyme immobilization methods are very useful and somehow effective in the issue at hand: decolorization and detoxification of dye wastewater. However, as auspicious as immobilized enzymes seem to be, the matter of loss of activity or stability should be addressed. Limitations of current immobilization methods are due to enzyme leakage from carrier, undesired reactions between carrier and enzyme or even the inability of the enzyme to react with its substrate. Nowadays, scientists are still developing new enzyme immobilization methods as it is a promising field that gives both better results and cost effectiveness for industrial use. However, from what is evident from recent research reports, they are merely focused on finding new materials, but the principles and methodologies of enzyme immobilization are still unchanged.
Despite the success of most of the reported laccase immobilization methods in dye removal, there is still one more aspect to be investigated when assessing their efficiency. The chemical structure of the target dye affects the results dramatically. Research reports often published assess the efficiency of their technologies by testing them on dyes with simple, low molecular weight structures that eventually show high decolorization results. Also, those promising technologies are still not implemented on an industrial scale. As the industrial sectors always seek the most economical technologies, they prefer using large quantities of mixed crude cultures or pure cultures over the immobilized one to limit the costs, overlooking the long-term advantages of reusage of immobilized enzymes. Most are drawn toward fungal more than bacterial laccase, due to its higher redox potential that show a higher oxidative ability. However, bacterial laccases are reported to withstand the harsh industrial environments with higher temperatures and pH values than those tolerated by fungal laccases. It is advised to use a redox mediator to act as an electron shuttle for better decolorization results of dye structures with higher redox potentials.
For all the mentioned drawbacks, immobilized laccases represent a potential effective, eco-friendly and commercial alternative to the physical, chemical, and oxidative dye decolorization methods. The scientific and industrial communities have real chances and future opportunities to moderate the current situation. Improvements can be made in fields of developing novel immobilization strategies in order to avoid enzyme leakage and come up with a more stable, durable, sustainable, and economical immobilization systems. One solution to the problem is the immobilization of both enzyme and redox mediator on the same carrier. This method ensures that low and medium redox potential laccases have better efficiency and operational stability. Also, this co-immobilized system can be reused several times, which will eventually reduce the manufacturing cost, that is ultimately the main concern of the industrial sector. This ambitious suggestion shall introduce an environmentally conscious and cost-effective dye detoxification method to the market.
Author Contributions
SAGZM: conceptualization, original draft preparation, and editing. AAT: manuscript reviewing. MSMA: supervision. FMS: project administration and supervision. All authors contributed to the article and approved the submitted version.
Conflict of Interest
The authors declare that the research was conducted in the absence of any commercial or financial relationships that could be construed as a potential conflict of interest.
Acknowledgments
SAGZM thanks Y. Bhg. Prof. Dato’ Dr. Abu Bakar Bin Salleh and Mohammed Abdelbaset Mahmoud Farag for their support, guidance and constructive review of this manuscript.
Supplementary Material
The Supplementary Material for this article can be found online at: https://www.frontiersin.org/articles/10.3389/fmicb.2020.572309/full#supplementary-material
Supplementary Figure 1 | Basic methods of enzyme immobilization.
Supplementary Table 1 | Classification of dyes according to industrial application, particle charge after dissolution and chromophore structure.
Supplementary Data 1 | Types of immobilization methods.
References
Agrawal, K., Chaturvedi, V., and Verma, P. (2018). Fungal laccase discovered but yet undiscovered. Bioresour. Bioprocess. 5, 1–12. doi: 10.1186/s40643-018-0190-z
Ali, E. A. M., Abd Ellatif, S., and Abdel Razik, E. S. (2020). Production, purification, characterization and immobilization of laccase from Phoma betae and its application in synthetic dyes decolorization. Egyptian J. Bot. 60, 301–312. doi: 10.21608/ejbo.2019.19226.1381
Arica, M. Y., Salih, B., Celikbicak, O., and Bayramoglu, G. (2017). Immobilization of laccase on the fibrous polymer-grafted film and study of textile dye degradation by MALDI–ToF-MS. Chem. Eng. Res. Des. 128, 107–119. doi: 10.1016/j.cherd.2017.09.023
Asghar, A., Raman, A. A. A., and Daud, W. M. A. W. (2015). Advanced oxidation processes for in-situ production of hydrogen peroxide/hydroxyl radical for textile wastewater treatment: a review. J. Clean. Prod. 87, 826–838. doi: 10.1016/j.jclepro.2014.09.010
Ashrafi, S. D., Rezaei, S., Forootanfar, H., Mahvi, A. H., and Faramarzi, M. A. (2013). The enzymatic decolorization and detoxification of synthetic dyes by the laccase from a soil-isolated ascomycete. Paraconiothyrium variabile. Int. Biodeter. Biodegr. 85, 173–181. doi: 10.1016/j.ibiod.2013.07.006
Ba, S., and Vinoth Kumar, V. (2017). Recent developments in the use of tyrosinase and laccase in environmental applications. Crit. Rev. Biotechnol. 37, 819–832. doi: 10.1080/07388551.2016.1261081
Babuponnusami, A., and Muthukumar, K. (2014). A review on Fenton and improvements to the Fenton process for wastewater treatment. J. Environ. Chem. Eng. 2, 557–572. doi: 10.1016/j.jece.2013.10.011
Bayraktaroğlu, M., Husein, Ý, AktaşUygun, D., and Uygun, M. (2020). Lectin-modified cryogels for laccase immobilization: a decolorization study. Water Air Soil Pollut. 231, 1–13. doi: 10.1007/s11270-020-4395-3
Benkhaya, S., M’ rabet, S., and El Harfi, A. (2020). A review on classifications, recent synthesis and applications of textile dyes. Inorg. Chem. Commun. 115, 1–35. doi: 10.1016/j.inoche.2020.107891
Bilal, M., Asgher, M., Parra-Saldivar, R., Hu, H., Wang, W., Zhang, X., et al. (2017). Immobilized ligninolytic enzymes: an innovative and environmental responsive technology to tackle dye-based industrial pollutants – A review. Sci. Total Environ. 576, 646–659. doi: 10.1016/j.scitotenv.2016.10.137
Bilal, M., Rasheed, T., Iqbal, H. M. N., and Yan, Y. (2018). Peroxidases-assisted removal of environmentally-related hazardous pollutants with reference to the reaction mechanisms of industrial dyes. Sci. Total Environ. 644, 1–13. doi: 10.1016/j.scitotenv.2018.06.274
Bilal, M., Rasheed, T., Nabeel, F., Iqbal, H. M. N., and Zhao, Y. (2019). Hazardous contaminants in the environment and their laccase-assisted degradation – A review. J. Environ. Manag. 234, 253–264. doi: 10.1016/j.jenvman.2019.01.001
Breen, A., and Singleton, F. L. (1999). Fungi in lignocellulose breakdown and biopulping. Curr. Opin. Biotechnol. 10, 252–258. doi: 10.1016/S0958-1669(99)80044-5
Chapman, J., Ismail, A., and Dinu, C. (2018). Industrial applications of enzymes: recent advances. Techniques, and Outlooks. Catalysts 8:238. doi: 10.3390/catal8060238
Chiong, T., Lau, S. Y., Lek, Z. H., Koh, B. Y., and Danquah, M. K. (2016). Enzymatic treatment of methyl orange dye in synthetic wastewater by plant-based peroxidase enzymes. J. Environ. Chem. Eng. 4, 2500–2509. doi: 10.1016/j.jece.2016.04.030
Crini, G., and Lichtfouse, E. (2019). Advantages and disadvantages of techniques used for wastewater treatment. Environ. Chem. Lett. 17, 145–155. doi: 10.1007/s10311-018-0785-9
Dai, J., Wang, H., Chi, H., Wang, Y., and Zhao, J. (2016). Immobilization of laccase from Pleurotus ostreatus on magnetic separable SiO2 support and excellent activity towards azo dye decolorization. J. Environ. Chem. Eng. 4, 2585–2591. doi: 10.1016/j.jece.2016.04.037
Datta, S., Christena, L. R., and Rajaram, Y. R. S. (2013). Enzyme immobilization: an overview on techniques and support materials. 3 Biotech 3, 1–9. doi: 10.1007/s13205-012-0071-7
Dauda, M. Y., and Erkurt, E. A. (2020). Investigation of reactive Blue 19 biodegradation and byproducts toxicity assessment using crude laccase extract from Trametes versicolor. J. Hazard. Mater. 393, 1–9. doi: 10.1016/j.jhazmat.2019.121555
Deska, M., and Kończak, B. (2019). Immobilized fungal laccase as “green catalyst” for the decolourization process – State of the art. Process Biochem. 84, 112–123. doi: 10.1016/j.procbio.2019.05.024
Dodor, D. E., Hwang, H. M., and Ekunwe, S. I. N. (2004). Oxidation of anthracene and benzo[a]pyrene by immobilized laccase from Trametes versicolor. Enzyme Microb. Technol. 35, 210–217. doi: 10.1016/j.enzmictec.2004.04.007
D’Souza-Ticlo, D., Sharma, D., and Raghukumar, C. (2009). A thermostable metal-tolerant laccase with bioremediation potential from a marine-derived fungus. Mar. Biotechnol. 11, 725–737. doi: 10.1007/s10126-009-9187-0
Dwivedi, U. N., Singh, P., Pandey, V. P., and Kumar, A. (2011). Structure-function relationship among bacterial, fungal and plant laccases. J. Mol. Catal. B Enzym. 68, 117–128. doi: 10.1016/j.molcatb.2010.11.002
Fernández-Fernández, M., Sanromán, M. Á, and Moldes, D. (2013). Recent developments and applications of immobilized laccase. Biotechnol. Adv. 31, 1808–1825. doi: 10.1016/j.biotechadv.2012.02.013
Giardina, P., Faraco, V., Pezzella, C., Piscitelli, A., Vanhulle, S., and Sannia, G. (2010). Laccases: a never-ending story. Cel. Mol. Life Sci. 67, 369–385. doi: 10.1007/s00018-009-0169-1
Gosavi, V. D., and Sharma, S. (2014). A General review on various treatment methods for textile wastewater. J. Environ. Sci. Comput. Sci. Eng. Technol. 3, 29–39.
Gupta, V. K., Gupta, B., Rastogi, A., Agarwal, S., and Nayak, A. (2011). A comparative investigation on adsorption performances of mesoporous activated carbon prepared from waste rubber tire and activated carbon for a hazardous azo dye-Acid Blue 113. J. Hazard. Mater. 186, 891–901. doi: 10.1016/j.jhazmat.2010.11.091
HajKacem, S., Galai, S., Hernández Fernandez, F. J., Pérez de los Ríos, A., Smaali, I., and Quesada Medina, J. (2020). Bioreactor membranes for laccase immobilization optimized by ionic liquids and cross-linking agents. Appl. Biochem. Biotechnol. 190, 1–17. doi: 10.1007/s12010-019-03085-z
Hayat, H., Mahmood, Q., Pervez, A., Bhatti, Z. A., and Baig, S. A. (2015). Comparative decolorization of dyes in textile wastewater using biological and chemical treatment. Separat. Purificat. Technol. 154, 149–153. doi: 10.1016/j.seppur.2015.09.025
Holkar, C. R., Jadhav, A. J., Pinjari, D. V., Mahamuni, N. M., and Pandit, A. B. (2016). A critical review on textile wastewater treatments: possible approaches. J. Environ. Manag. 182, 351–366. doi: 10.1016/j.jenvman.2016.07.090
Hunger, K. (2004). “Chapter 1: dyes, general survey,” in Industrial Dyes: Chemistry, Properties, Applications, ed. K. Hunger (Weinheim: Wiley-VCH Verlag GmbH & Co. KGaA), 1–12. doi: 10.1002/3527602011
Husain, Q. (2010). Peroxidase mediated decolorization and remediation of wastewater containing industrial dyes: a review. Rev. Environ. Sci. Biotechnol. 9, 117–140. doi: 10.1007/s11157-009-9184-9
Jadhav, A. J., and Srivastava, V. C. (2013). Adsorbed solution theory based modeling of binary adsorption of nitrobenzene, aniline and phenol onto granulated activated carbon. Chem. Eng. J. 229, 450–459. doi: 10.1016/j.cej.2013.06.021
Jankowska, K., Zdarta, J., Grzywaczyk, A., Kijeńska-Gawrońska, E., Biadasz, A., and Jesionowski, T. (2020). Electrospun poly(methyl methacrylate)/polyaniline fibres as a support for laccase immobilisation and use in dye decolourisation. Environ. Res. 184:Article109332. doi: 10.1016/j.envres.2020.109332
Janusz, G., Pawlik, A., Świderska-Burek, U., Polak, J., Sulej, J., Jarosz-Wilkołazka, A., et al. (2020). Laccase properties, physiological functions, and evolution. Int. J. Mol. Sci. 21, 1–25. doi: 10.3390/ijms21030966
Jeon, S. J., and Park, J. H. (2020). Refolding, characterization, and dye decolorization ability of a highly thermostable laccase from Geobacillus sp. JS12. Protein Expr. Purif. 173:105646. doi: 10.1016/j.pep.2020.105646
Jesionowski, T., Zdarta, J., and Krajewska, B. (2014). Enzyme immobilization by adsorption: a review. Adsorption 20, 801–821. doi: 10.1007/s10450-014-9623-y
Joshi, A. U., Hinsu, A. T., Kotadiya, R. J., Rank, J. K., Andharia, K. N., and Kothari, R. K. (2020). Decolorization and biodegradation of textile di-azo dye Acid Blue 113 by Pseudomonas stutzeri AK6. 3 Biotech 10, 1–16. doi: 10.1007/s13205-020-02205-5
Kagalkar, A. N., Khandare, R. V., and Govindwar, S. P. (2015). Textile dye degradation potential of plant laccase significantly enhances upon augmentation with redox mediators. RSC Adv. 5, 80505–80517. doi: 10.1039/C5RA12454A
Kashefi, S., Borghei, S. M., and Mahmoodi, N. M. (2019a). Covalently immobilized laccase onto graphene oxide nanosheets: preparation, characterization, and biodegradation of azo dyes in colored wastewater. J. Mol. Liqu. 276, 153–162. doi: 10.1016/j.molliq.2018.11.156
Kashefi, S., Borghei, S. M., and Mahmoodi, N. M. (2019b). Superparamagnetic enzyme-graphene oxide magnetic nanocomposite as an environmentally friendly biocatalyst: synthesis and biodegradation of dye using response surface methodology. Microchem. J. 145, 547–558. doi: 10.1016/j.microc.2018.11.023
Katheresan, V., Kansedo, J., and Lau, S. Y. (2018). Efficiency of various recent wastewater dye removal methods: a review. J. Environ. Chem. Eng. 6, 4676–4697. doi: 10.1016/j.jece.2018.06.060
Legerska, B., Chmelova, D., and Ondrejovic, M. (2016). Degradation of synthetic dyes by laccases – A mini-review. Nova Biotechnol. Chim. 15, 90–106. doi: 10.1515/nbec-2016-0010
Li, Z., Shang, W., Liu, W., Li, H., and Tian, Y. M. (2014). Application of immobilized laccase in the removal of oil from wastewater. Desalinat. Water Treatm. 52, 2594–2600. doi: 10.1080/19443994.2013.797142
Liang, C. Z., Sun, S. P., Li, F. Y., Ong, Y. K., and Chung, T. S. (2014). Treatment of highly concentrated wastewater containing multiple synthetic dyes by a combined process of coagulation/flocculation and nanofiltration. J. Memb. Sci. 469, 306–315. doi: 10.1016/j.memsci.2014.06.057
Lin, J., Lai, Q., Liu, Y., Chen, S., Le, X., and Zhou, X. (2017). Laccase – methacrylyol functionalized magnetic particles: highly immobilized, reusable, and efficacious for methyl red decolourization. Int. J. Biol. Macromol. 102, 144–152. doi: 10.1016/j.ijbiomac.2017.03.169
Lu, L., Zhao, M., Li, G. F., Li, J., Wang, T. N., Li, D.-B., et al. (2012a). Decolorization of synthetic dyes by immobilized spore from Bacillus amyloliquefaciens. Catal. Commun. 26, 58–62. doi: 10.1016/j.catcom.2012.04.024
Lu, L., Zhao, M., Wang, T. N., Zhao, L. Y., Du, M. H., and Li, T. L. (2012b). Characterization and dye decolorization ability of an alkaline resistant and organic solvents tolerant laccase from Bacillus licheniformis LS04. Bioresour. Technol. 115, 35–40. doi: 10.1016/j.biortech.2011.07.111
Luo, M., Li, M., Jiang, S., Shao, H., Razal, J., Wang, D., et al. (2020). Supported growth of inorganic-organic nanoflowers on 3D hierarchically porous nanofibrous membrane for enhanced enzymatic water treatment. J. Hazard. Mater. 381:Article120947. doi: 10.1016/j.jhazmat.2019.120947
Ma, H. F., Meng, G., Cui, B. K., Si, J., and Dai, Y. C. (2018). Chitosan crosslinked with genipin as supporting matrix for biodegradation of synthetic dyes: laccase immobilization and characterization. Chem. Eng. Res. Des. 132, 664–676. doi: 10.1016/j.cherd.2018.02.008
Mahmoodi, N. M., and Saffar-Dastgerdi, M. H. (2020). Clean laccase immobilized nanobiocatalysts (graphene oxide - zeolite nanocomposites): from production to detailed biocatalytic degradation of organic pollutant. Appl. Catal. B Environ. 268:Article118443. doi: 10.1016/j.apcatb.2019.118443
Messerschmidt, A., and Huber, R. (1990). The blue oxidases, ascorbate oxidase, laccase and ceruloplasmin modelling and structural relationships. Eur. J. Biochem. 187, 341–352. doi: 10.1111/j.1432-1033.1990.tb15311.x
Nair, R. R., Demarche, P., and Agathos, S. N. (2013). Formulation and characterization of an immobilized laccase biocatalyst and its application to eliminate organic micropollutants in wastewater. New Biotechnol. 30, 814–823. doi: 10.1016/j.nbt.2012.12.004
Patel, S. K. S., Otari, S. V., Li, J., Kim, D. R., Kim, S. C., Cho, B. K., et al. (2018). Synthesis of cross-linked protein-metal hybrid nanoflowers and its application in repeated batch decolorization of synthetic dyes. J. Hazard. Mater. 347, 442–450. doi: 10.1016/j.jhazmat.2018.01.003
Polak, J., and Jarosz-Wilkolazka, A. (2012). Fungal laccases as green catalysts for dye synthesis. Process Biochem. 47, 1295–1307. doi: 10.1016/j.procbio.2012.05.006
Rao, M. A., Scelza, R., Acevedo, F., Diez, M. C., and Gianfreda, L. (2014). Enzymes as useful tools for environmental purposes. Chemosphere 107, 145–162. doi: 10.1016/j.chemosphere.2013.12.059
Rasheed, T., Bilal, M., Nabeel, F., Adeel, M., and Iqbal, H. M. N. (2019). Environmentally-related contaminants of high concern: potential sources and analytical modalities for detection, quantification, and treatment. Environ. Int. 122, 52–66. doi: 10.1016/j.envint.2018.11.038
Rodríguez-Couto, S., Osma, J. F., and Toca-Herrera, J. L. (2009). Removal of synthetic dyes by an eco-friendly strategy. Eng. Life Sci. 9, 116–123. doi: 10.1002/elsc.200800088
Salami, F., Habibi, Z., Yousefi, M., and Mohammadi, M. (2018). Covalent immobilization of laccase by one pot three component reaction and its application in the decolorization of textile dyes. Int. J. Biol. Macromol. 120, 144–151. doi: 10.1016/j.ijbiomac.2018.08.077
Shaheen, R., Asgher, M., Hussain, F., and Bhatti, H. N. (2017). Immobilized lignin peroxidase from Ganoderma lucidum IBL-05 with improved dye decolorization and cytotoxicity reduction properties. Int. J. Biol. Macromol. 103, 57–64. doi: 10.1016/j.ijbiomac.2017.04.040
Shakerian, F., Zhao, J., and Li, S. P. (2020). Recent development in the application of immobilized oxidative enzymes for bioremediation of hazardous micropollutants – A review. Chemosphere 239, 1–12. doi: 10.1016/j.chemosphere.2019.124716
Sharma, B., Dangi, A. K., and Shukla, P. (2018). Contemporary enzyme based technologies for bioremediation: a review. J. Environ. Manag. 210, 10–22. doi: 10.1016/j.jenvman.2017.12.075
Sheldon, R. A., and van Pelt, S. (2013). Enzyme immobilisation in biocatalysis: why, what and how. Chem. Soc. Rev. 42, 6223–6235. doi: 10.1039/c3cs60075k
Shrivastava, S., Lata, S., and Shukla, P. (2012). An insight on recent advances on immobilization methods for industrial enzymes and its relevance to xylanases. Dyn. Biochem. Process Biotechnol. Mol. Biol. 6, 57–61.
Singh, D., and Gupta, N. (2020). Microbial Laccase: A Robust Enzyme and Its Industrial Applications. Berlin: Springer, doi: 10.2478/s11756-019-00414-9
Sirisha, V. L., Jain, A., and Jain, A. (2016). Enzyme immobilization: an overview on methods, support material, and applications of immobilized enzymes. Adv. Food Nutr. Res. 79, 179–211. doi: 10.1016/bs.afnr.2016.07.004
Skoronski, E., Souza, D. H., Ely, C., Broilo, F., Fernandes, M., Fúrigo, A., et al. (2017). Immobilization of laccase from Aspergillus oryzae on graphene nanosheets. Int. J. Biol. Macromol. 99, 121–127. doi: 10.1016/j.ijbiomac.2017.02.076
Solís, M., Solís, A., Pérez, H. I., Manjarrez, N., and Flores, M. (2012). Microbial decolouration of azo dyes: a review. Process Biochem. 47, 1723–1748. doi: 10.1016/j.procbio.2012.08.014
Solomon, E. I., Baldwin, M. J., and Lowery, M. D. (1992). Electronic structures of active sites in copper proteins: contributions to reactivity. Chem. Rev. 92, 521–542. doi: 10.1021/cr00012a003
Sun, H., Yang, H., Huang, W., and Zhang, S. (2015). Immobilization of laccase in a sponge-like hydrogel for enhanced durability in enzymatic degradation of dye pollutants. J. Colloid Interface Sci. 450, 353–360. doi: 10.1016/j.jcis.2015.03.037
Tuncay, D., and Yagar, H. (2020). Decolorization of Reactive Blue-19 textile dye by Boletus edulis laccase immobilized onto rice husks. Int. J. Environ. Sci. Technol. 17, 3177–3188. doi: 10.1007/s13762-020-02641-z
Ulu, A., Birhanli, E., Boran, F., Köytepe, S., Yesilada, O., and Ateş, B. (2020). Laccase-conjugated thiolated chitosan-Fe3O4 hybrid composite for biocatalytic degradation of organic dyes. Int. J. Biol. Macromol. 150, 871–884. doi: 10.1016/j.ijbiomac.2020.02.006
Wang, Q., Wang, T., Lv, Z., Cui, M., Zhao, Z., Cao, X., et al. (2020). TiO2 sol-gel coated PAN/O-MMT Multi-functional composite nanofibrous membrane used as the support for laccase immobilization: synergistic effect between the membrane support and enzyme for dye degradation. Polymers 12, 1–13. doi: 10.3390/polym12010139
Wen, X., Du, C., Wan, J., Zeng, G., Huang, D., Yin, L., et al. (2019). Immobilizing laccase on kaolinite and its application in treatment of malachite green effluent with the coexistence of Cd (⊓). Chemosphere 217, 843–850. doi: 10.1016/j.chemosphere.2018.11.073
Wikee, H., Hatton, J., Turbé-Doan, A., Mathieu, Y., Daou, M., Lomascolo, A., et al. (2019). Characterization and dye decolorization potential of two laccases from the marine-derived fungus Pestalotiopsis sp. Int. J. Mol. Sci. 20, 1–20. doi: 10.3390/ijms20081864
Yagub, M. T., Sen, T. K., Ang, H. M., and Afroze, S. (2014). Dye and its removal from aqueous solution by adsorption: a review | Elsevier Enhanced Reader. Adv. Colloid Interface Sci. 209, 172–184. doi: 10.1016/j.cis.2014.04.002
Yang, J., Xu, X., Yang, X., Ye, X., and Lin, J. (2016). Cross-linked enzyme aggregates of Cerrena laccase: preparation, enhanced NaCl tolerance and decolorization of remazol brilliant blue reactive. J. Taiwan Inst. Chem. Eng. 65, 1–7. doi: 10.1016/j.jtice.2016.04.025
Yang, J., Yang, X., Lin, Y., Ng, T. B., Lin, J., and Ye, X. (2015). Laccase-catalyzed decolorization of malachite green: performance optimization and degradation mechanism. PLoS One 10:e0127714. doi: 10.1371/journal.pone.0127714
Yang, X., Zheng, J., Lu, Y., and Jia, R. (2016). Degradation and detoxification of the triphenylmethane dye malachite green catalyzed by crude manganese peroxidase from Irpex lacteus F17. Environ. Sci. Pollut. Res. 23, 9585–9597. doi: 10.1007/s11356-016-6164-9
Yeap, K. L., Teng, T. T., Poh, B. T., Morad, N., and Lee, K. E. (2014). Preparation and characterization of coagulation/flocculation behavior of a novel inorganic-organic hybrid polymer for reactive and disperse dyes removal. Chem. Eng. J. 243, 305–314. doi: 10.1016/j.cej.2014.01.004
Zhang, W., Yang, Q., Luo, Q., Shi, L., and Meng, S. (2020). Laccase-Carbon nanotube nanocomposites for enhancing dyes removal. J. Clean. Product. 242:118425. doi: 10.1016/j.jclepro.2019.118425
Zheng, F., Cui, B. K., Wu, X. J., Meng, G., Liu, H. X., and Si, J. (2016). Immobilization of laccase onto chitosan beads to enhance its capability to degrade synthetic dyes. Int. Biodeter. Biodegr. 110, 69–78. doi: 10.1016/j.ibiod.2016.03.004
Zhou, Y., Lu, J., Zhou, Y., and Liu, Y. (2019). Recent advances for dyes removal using novel adsorbents: a review. Environ. Pollut. 252, 352–365. doi: 10.1016/j.envpol.2019.05.072
Zhu, Z., Chen, Z., Luo, X., Liang, W., Li, S., He, J., et al. (2020). Biomimetic dynamic membrane (BDM): fabrication method and roles of carriers and laccase. Chemosphere 240, 1–7. doi: 10.1016/j.chemosphere.2019.124882
Keywords: immobilization, laccase, oxidoreductase, textile dye wastewater, decolorization, detoxification, biodegradation
Citation: Morsy SAGZ, Ahmad Tajudin A, Ali MSM and Shariff FM (2020) Current Development in Decolorization of Synthetic Dyes by Immobilized Laccases. Front. Microbiol. 11:572309. doi: 10.3389/fmicb.2020.572309
Received: 13 June 2020; Accepted: 01 September 2020;
Published: 30 September 2020.
Edited by:
Datta Madamwar, Sardar Patel University, IndiaReviewed by:
Pradeep Verma, Central University of Rajasthan, IndiaJingwei Hou, The University of Queensland, Australia
Copyright © 2020 Morsy, Ahmad Tajudin, Ali and Shariff. This is an open-access article distributed under the terms of the Creative Commons Attribution License (CC BY). The use, distribution or reproduction in other forums is permitted, provided the original author(s) and the copyright owner(s) are credited and that the original publication in this journal is cited, in accordance with accepted academic practice. No use, distribution or reproduction is permitted which does not comply with these terms.
*Correspondence: Fairolniza Mohd. Shariff, fairolniza@upm.edu.my