- 1Faculty of Bioscience and Technology for Food, Agriculture and Environment, University of Teramo, Teramo, Italy
- 2School of Microbiology, University College Cork, Cork, Ireland
- 3APC Microbiome Ireland, University College Cork, Cork, Ireland
Lactobacillus plantarum species (recently re-named Lactiplantibacillus (Lpb.) plantarum subsp. plantarum) can be isolated from both either the mammalian gut or specific fermented foods where they may be present at high concentrations. Whilst Lpb. plantarum strains have been proposed as potential probiotic candidates, the ability of resident strains consumed in fermented foods to interact with the host is unclear. The main objective of this study was to investigate the cellular location and ability of three different food-borne Lpb. plantarum strains isolated from different sources (table olives and cheese) to modulate the immune response of a murine macrophage-like cell line (J774A.1). For that purpose, macrophages were exposed to the three different Lpb. plantarum strains for 24 h and the expression of a panel of genes involved in the immune response, including genes encoding pattern-recognition receptors (TLRs and NLRs) and cytokines was evaluated by qRT-PCR. We also utilized chemical inhibitors of intracellular pathways to gain some insight into potential signaling mechanisms. Results showed that the native food strains of Lpb. plantarum were able to modulate the response of J774A.1 murine macrophages through a predominately NOD signaling pathway that reflects the transient intracellular location of these strains within the macrophage. The data indicate the capacity of food-dwelling Lpb. plantarum strains to influence macrophage-mediated host responses if consumed in sufficient quantities.
Introduction
The original genus Lactobacillus (L.) comprised a heterogeneous group of non-sporeforming, non-motile and rod-shaped bacteria (Plot et al., 2014). However, a recent phylogenetic and ecological revaluation of the genus has led to reclassification of Lactobacillus species into 25 proposed genera (Zheng et al., 2020). In particular Lactobacillus plantarum has been reclassified as Lactiplantibacillus plantarum subsp. plantarum (Lpb. plantarum). Lpb. plantarum is a microaerophilic gram-positive bacterium, that is encountered in a wide variety of ecological niches including the gastrointestinal tract and fermented foods (Corsetti et al., 2018). Moreover, Lpb. plantarum has been used as a starter culture in food fermentation processes due to its organoleptic fermentative properties and a capacity to produce lactic acid and other antimicrobial compounds (Seddik et al., 2017; Behera et al., 2018). We have recently shown that Lpb. plantarum strains are likely to be consumed at high concentrations in table olives where they are one of the predominant species (107–108 CFU/g) (Hurtado et al., 2012; Heperkan, 2013; Perpetuini et al., 2020). However, the potential impact of these autochthonous food strains on the host, when either consumed directly or re-employed as starter cultures, is currently unclear.
In the last century, Lpb. plantarum strains have been widely investigated not only for their functional properties but also as potential probiotics. Probiotics are defined as live microorganisms that, when administered in adequate amounts, confer a health benefit on host health (Hill et al., 2014). Bacteria commonly associated with probiotic effects usually have the ability to adhere to intestinal cells, produce beneficial metabolites such as short-chain fatty acids, modulate the immune system and compete with pathogens for adhesion sites (de Vrese and Schrezenmeir, 2008; Wells, 2011). Immune modulatory properties of some Lactobacillus strains have been described in both animal studies and clinical trials. However, the exact mechanisms underlying such effects and the inter-strain variation in these properties are not fully understood (Corthésy et al., 2007). According to the latest evidence, the definition of probiotics regarding their immunomodulatory properties could be extended to “live microorganisms, that when included in foods can influence the composition and activity of the gut microbiota, modulate the inflammatory response, improve the non-specific intestinal barrier, and reinforce or modulate the mucosal and the systemic immune responses” (Dong et al., 2010; Markowiak and Śliżewska, 2017). Beneficial effects of probiotic strains in modulating the immune system could impact positively in inflammatory processes, which have been described to be involved in a number of chronic diseases and disorders including osteoporosis, cardiovascular disease, insulin resistance and diabetes (Cani et al., 2008; Kang and Im, 2015; Ryan et al., 2019). Several studies have demonstrated that probiotics are able to stimulate the release of pro-inflammatory cytokines by activation of pattern recognition receptors (PRRs) (Melmed et al., 2003; Lee et al., 2006; Ghadimi et al., 2010; Macho Fernandez et al., 2011; Zhong et al., 2012). The use of in vitro models has been a valuable tool to elucidate the effect on the immune system by Lpb. plantarum strains. They have been described to reduce the expression of genes involved in the pro-inflammatory response by activating the expression of TLR2 (Pathmakanthan et al., 2004; Paolillo et al., 2009; Bäuerl et al., 2013). For instance, L. plantarum Lp62 has been described to inhibit the inflammatory stimulation in epithelial cells and macrophages by modulating the release of TNF-α, IL-1ß, and IL-17 (Ferreira dos Santos et al., 2016). However, the cross-talk between host cells and bacteria belonging to the Lactobacillus genus seems to be strain-dependent and the observed effects for a specific strain cannot be extrapolated to other bacteria within the same species, and thus, it is crucial to evaluate the potential probiotic effects of individual strains (Ivec et al., 2007; Tallon et al., 2007).
The aim of this study was to evaluate the effect of three Lpb. plantarum isolated from different sources in the early cytokine response by modulating PRRs in a murine macrophage cell line. Properties including cytotoxicity, adhesion, intracellular localization, and the cross-talk between bacteria and host cells were evaluated. Moreover, specific inhibitors of the upstream kinases, interleukin-1 receptor-associated kinase 4 (IRAK4) and receptor-interacting-serine/threonine-protein kinase 2 (RIP2) and specific inhibitors of the phagocytosis, cytochalasin D (Cyt.D) and dehydroxymethylepoxyquinomicin (DHMEQ), were used in order to elucidate the exact PRRs signaling pathways activated by Lpb. plantarum strains. This study demonstrates that Lpb. plantarum strains that are found in natural food environments are capable of stimulating macrophage cytokine responses through a NOD-dependent signaling mechanism reflective of their transient intracellular location. Whilst further in vivo studies are warranted, the current study suggests that naturally occurring food-resident Lpb. plantarum strains may have the potential to elicit host immune effects when consumed at adequate levels.
Materials and Methods
Bacterial Strains
Food-borne Lpb. plantarum strains C904 and LT52 isolated from different fermented foods (table olives and raw-milk cheese, respectively) belonging to our collection (University of Teramo), the probiotic strain IMC513, isolated from the gastrointestinal tract of human (Synbiotec, Camerino, Italy) and Listeria (L.) innocua from our collection (University College Cork) were used in this study. Whilst all Lpb. plantarum strains were grown in microaerophilic conditions in MRS broth (Oxoid, Basingstoke, United Kingdom), L. innocua was grown on Brain Heart Infusion (BHI) broth (Oxoid, Basingstoke, United Kingdom) in aerobic conditions. All species were grown at 37°C and subcultured once before each experiment.
Cell Line and Culture Conditions
The J774A.1 murine macrophage cell line was purchased from the American Tissue Culture Collection (ATCC, Rockville, United States). Cells were cultured as recommended by the ATCC in Dulbecco’s Modified Eagle’s medium (DMEM; 4.5 g/L D-glucose, Sigma-Aldrich Ltd., Wicklow, Ireland) supplemented with 10% v/v heat-inactivated foetal bovine serum (FBS) (Sigma-Aldrich Ltd., Wicklow, Ireland). Cells were maintained at 37°C in an incubator under a 5% CO2 and 95% relative humidity. Prior to the experiments, cells were detached from the flask using a scraper, centrifuged at 1,500 rpm for 4 min at room temperature (RT) and resuspended in antibiotic-free growth media. Cells were counted using a hemocytometer and seeded at a density of 50,000 cells/cm2 for 24 h prior to incubation with Lpb. plantarum strains. Control cells were also run in parallel and subjected to the same changes of medium.
Viability Assays
After 24 h of exposure of the macrophages to each strain of Lpb. plantarum, cell viability was evaluated using the CellTiter-Glo® luminescent cell viability assay (Promega, MyBio Ltd., Ireland) following the manufacturer’s instructions. Briefly, cells were exposed to each Lpb. plantarum strains (ratio bacteria/macrophage 10:1 and 100:1) for 24 h. After the treatment, cell media was removed, and wells were washed three times to remove the bacteria in the supernatant prior to the addition of fresh medium. Plates were then placed at RT for 30 min to equilibrate the plate and then, an equal volume of CellTiter-Glo® was added to each well. The contents were mixed for 5 min using an orbital shaker at 200 rpm. The plates were allowed to stabilize for 15 min at RT and luminescence was measured using a BioTek Synergy two microplate reader (BioTek Instruments, Winooski, VT, United States). All experiments were performed in triplicate.
In order to confirm these data, cell viability was further determined by flow cytometry using propidium iodide (PI, R&D Biosciences, Minneapolis, MN, United States) staining. Cells were cultured in 12-well plates and treated as described above. To detach the cells, the macrophages were placed on ice for 15 min under constant shaking at 200 rpm. Then, media was collected in a 15 mL falcon tube and 0.5 mL of TrypLE Express (Gibco, Biosciences, Dublin, Ireland) were added to each well. Plates were incubated for 10 min on ice under constant shaking (200 rpm) and then collected in the same tube and centrifuged at 1,500 rpm for 5 min. Cells were washed twice with Flow Cytometry Staining Buffer (FCSB) containing PBS with 2% FBS and centrifuged at 1,500 rpm for 5 min. Cells were then resuspended in 100 μL of FCSB containing 5 μL of PI and incubated 15 min at RT protected from light. Cell viability was analyzed by flow cytometry using a BD Accuri C6 cytometer (Becton Dickinson, NJ, United States).
Adhesion and Phagocytosis Assays
The ability of bacteria to adhere to murine macrophages was investigated as previously described by Vargas-García and colleagues (Vargas García et al., 2015) with some modifications. Briefly, cells were incubated in 96-well plates and Lpb. plantarum strains were added at a density of 106 CFU/ml (ratio bacteria/macrophages 10:1). The bacteria were allowed to adhere to the cells for 4 h at 37°C. After that, cells were detached and plated in MRS agar. The percentage of adherent bacteria was calculated by comparing the total number of bacterial colonies counted to the number of bacteria added. In order to calculate the total cell-associated bacteria, macrophages were lysed with 0.2% Triton X-100 for 20 min prior to preparation of the dilution series. The percentage of total associated bacteria was calculated by comparing the total number of bacterial colonies counted after the macrophages were lysed to the number of bacteria added. Moreover, in order to calculate the number of internalized bacteria, phagocytosis assay was performed by stopping the internalization with ice-cold PBS and killing the remaining extracellular bacteria by addition of gentamicin (100 μg/mL) for 2 h. After the incubation, cells were lysed with 0.2% Triton X-100 and plated in MRS agar plates. All the experiments were performed in triplicate.
Microscopic Visualization of Lpb. plantarum Strains
J774A.1 macrophages were seeded on top of a microscope slide inside 60 mm petri dishes at a density of 0.8 × 106 cells overnight. Upon adherence, cells were exposed to Lpb. plantarum strain C904, LT52, or IMC513 at a ratio of 10:1 (bacteria/macrophages) for 6 h. In brief, single colonies of each strain were picked, diluted in PBS and used to cover J774A.1 cells on individual microscope slides. Both cell and bacterial staining was performed using ShandonTM Kwik-DiffTM Giemsa stains (ThermoFisher, Waltham, MA, United States). Briefly, the microscope slides were dipped orderly 15 times in methanol, eosin and finally methylene Blue, washed with tap water. Samples were let to dry, mounted with mounting media (Thermo Fisher, Waltham, MA, United States) and hold using a coverslide. A light field microscope (Zeiss, Germany) was used to take pictures at 100× magnification.
Quantitative Real-Time Reverse Transcriptase-Polymerase Chain Reaction (qRT-PCR) Analysis
Expression of inflammatory markers in macrophages was evaluated through the analysis of gene expression of a panel of cytokines and receptors involved in the microbial interaction and inflammatory response (Table 1). Briefly, J774A.1 macrophages were plated in triplicated in 12-well plates and cultured with the Lpb. plantarum strains (106 CFU/ml) (ratio bacteria/macrophages 10:1). After the incubation, cells were lysed in the plates and total mRNA was isolated using an Isolate II RNA mini kit (Bioline, Medical Supply Company Ltd., Dublin, Ireland). mRNA was eluted in RNAse-free water and checked for concentration and purity using a Nanodrop spectrophotometer system (ND-1000 3.3 Nanodrop Technologies, United States). cDNA was obtained from mRNA following reverse transcription according to the manufacturer’s instructions (NZY First-Strand cDNA Synthesis Kit, Nzytech, Lisbon, Portugal). The results were normalized against β-actin gene expression.
Macrophage Stimulation Assay With RIP2 and IRAK4 Inhibitors
J774A.1 macrophages were seeded in 96-well plates and incubated overnight at 37°C and 5% CO2 to allow adherence. Cells were treated with PF 06650833 (100 nM) (TOCRIS, Abingdon, United Kingdom), a specific inhibitor of IRAK4, or Gefitinib (10 μM) (InvivoGen, San Diego, CA, United States), an inhibitor of RIP2 for 1 h before being stimulated by bacteria at a concentration of 106 CFU/ml (ratio bacteria/macrophages 10:1) for 24 h (Seganish, 2016). The release of TNF-α and IL-6 was evaluated using enzyme-linked immunosorbent assays (ELISA).
Enzyme-Linked Immunosorbent Assay
Levels of TNF-α and IL-6 were measured using Murine TNF-α and IL-6 Mini ABTS ELISA Development Kit (Peprotech, Inc., London, United Kingdom) and analyzed using a microplate reader. When appropriate, evaluation of potential signaling pathways was performed on murine macrophages pre-treated with inhibitor of IRAK4 and Gefitinib for 1 h to inhibit IRAK4 and RIP2, and 2 μM of Cyt.D or 10 μg of DHMEQ for 24 h to inhibit phagocytosis (Suzuki and Umezawa, 2006; Weibel et al., 2012) prior to 24 h of bacterial stimulation. Following this incubation, supernatants were collected and analyzed following the manufacturer’s instructions. J774A.1 murine macrophages without bacterial treatment and without inhibitors pre-treatment were used as a control.
Statistical Analysis
Data were analyzed using the Prism 5.0 program (GraphPad Software Inc., La Jolla, CA, United States) using the one-way analysis of variance (ANOVA) followed by Bonferroni’s post hoc analysis. ELISA data were assessed by Student’s t-test. All values are given as mean ± SE. A level of p < 0.05 was considered statistically significant.
Results
Microbial Impact of Lpb. plantarum Strains on J774A.1 Murine Macrophages
The potential impact of three Lpb. plantarum strains on viability of J774A.1 murine macrophages was assessed by the luminescent CellTiter-Glo assay. In order to evaluate the potential toxicity of bacteria on J774A.1, cell viability was tested using two different concentrations of bacteria 106 CFU/mL and 107 CFU/mL. After 24 h incubation, our results showed that the lowest concentration tested had no significant impact on cell viability with all values above 90%. On the other hand, cells exposed to Lpb. plantarum strains at a concentration of 107 CFU/mL showed a significant decrease (p < 0.001) in cell viability after 24 h exposure. The percentage of survival of macrophages after the treatment is shown in Figure 1A.
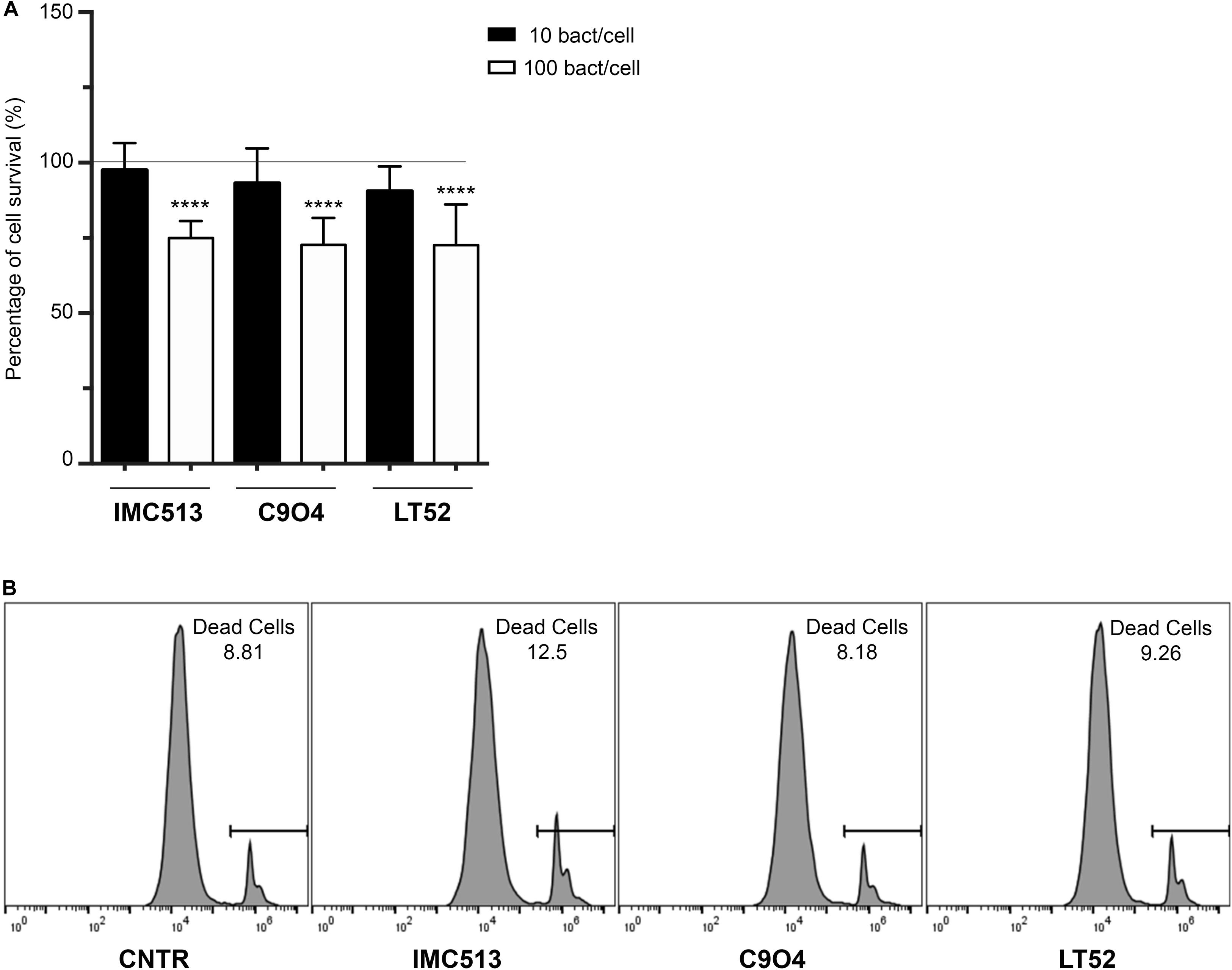
Figure 1. Evaluation of cellular metabolic activity in murine macrophages J774A.1 cells incubated with three different Lpb. plantarum strains. (A) Macrophage viability was evaluated by measuring the ATP content with Promega Cell-Titer-Glo®. Data are shown as mean ratio (percentage ± SD) of absorbance in the samples well (OD of macrophages with bacteria) and negative control (OD of macrophages). (B) Number of viable cells evaluated by flow cytometry using the PI staining. Representative values of death cells are given as percentage. Statistical analysis was conducted using One-way ANOVA followed by Bonferroni’s post hoc analysis. Values are expressed as mean ± SD, ****p < 0.0001.
In order to confirm this data and that the observed values correspond only to the macrophage’s metabolic activity and not to the combined signal of macrophages and remaining bacteria, cell viability was also evaluated by flow cytometry using PI staining (Figure 1B). Our results showed that the percentage of death cells in the untreated cells reached a mean value of 8.6%. After the exposure to the different Lpb. plantarum strains, the results demonstrated that the strains had a similar percentage of cell death with C904 strain showing the lowest percentage with 7.9% of cell death while the probiotic strain IMC513 induced the highest cell death with a mean value of 11.7% although it was not significant.
Adhesion Efficiency of Lpb. plantarum Strains to Murine Macrophages
The ability of Lpb. plantarum strains to adhere to J774.A1 murine macrophages was tested at the selected concentration of 106 CFU/mL through bacterial enumeration by plating on MRS agar. The adhesion of the probiotic strain IMC513 showed the highest values with a mean of 36.83 ± 6.46%. On the other hand, the LT52 strain showed the lowest values, although differences were not statistically significant between any of the three Lpb. plantarum strains (Figure 2). The total amount of macrophage-associated bacteria was evaluated by lysing of the macrophages before enumeration in order to liberate internalized bacteria. Results showed that most of the bacteria were internalized after 4 h incubation with macrophages (Figure 2). These results were further confirmed through the evaluation of the bacteria phagocytised following an antibiotic treatment in order to kill all the remaining bacteria in the media and those that were adherent but not internalized. Our results were in line with the observed results of adherence and total bacteria. To further verify the internalization of the bacteria, we performed a microscopic visualization using ShandonTM Kwik-DiffTM Giemsa stains (Supplementary Figure 1). Microscopic evaluation showed the internalization of the bacteria by macrophages and their apparent localization in the cytoplasm of the macrophages. We appreciate that it is unclear whether bacteria remain internalized or are freely present in the cytoplasm however bacteria are clearly cell-associated and gentamicin protection assays indicate that they remain viable (Figure 2). Regardless the origin of the strains, the ability to internalized bacteria by macrophages did not show significant differences, with internalization rates ranging from 61.22% (C904) to 78.24% (IMC513) (Figure 2).
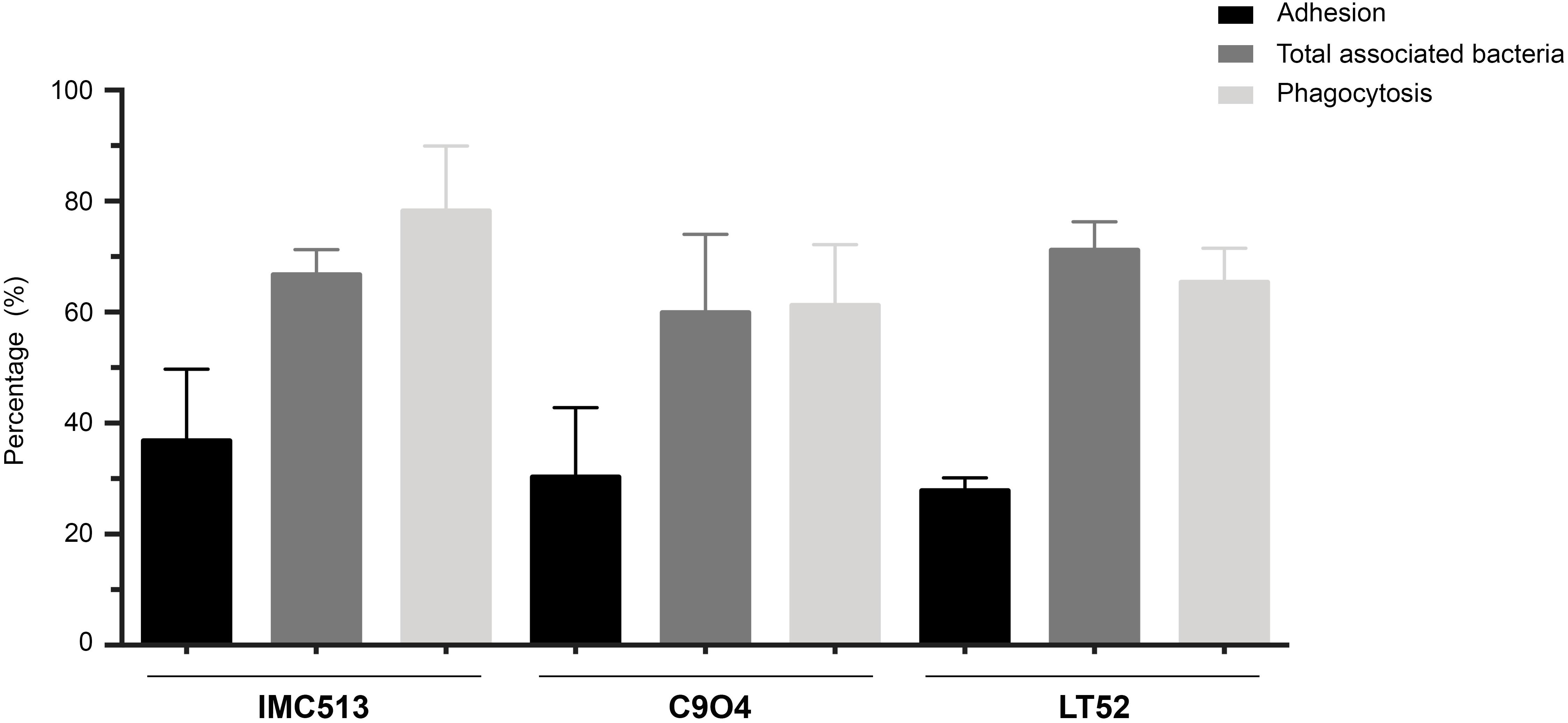
Figure 2. Adhesion and phagocytosis of three Lpb. plantarum strains by J774A.1 murine macrophages. For adhesion assays, Lpb. plantarum strains were co-incubated with macrophages and the percentage of adherent bacteria was determined by plating on MRS. The total amount of macrophage-associated bacteria was performed by lysing of the macrophages after the incubation. Finally, for phagocytosis assays remaining extracellular bacteria were killed to only evaluate internalized bacteria.
Lpb. plantarum Modulation of Genes Involved in Inflammation on Murine Macrophages
Based on the high adhesion efficiency of the three strains we then evaluated the potential immunomodulatory impact of the three Lpb. plantarum strains IMC513, C904, and LT52 on J774A.1 murine macrophages. For that purpose, we selected a panel of genes encoding proteins involved in the recognition of microbe associated molecular patterns and in the inflammatory response. To evaluate the recognition of the strains we analyzed expression of two of the major types of PRRs; leucine-rich repeat containing receptors (NLRs) Nod1 and Nod2, and the toll-like receptors (TLRs) Tlr2, Tlr4, Tlr5, and Tlr9. The results of qRT-PCR (Figure 3) indicated that there are differences in the expression of both TLRs and NLRs receptors after incubation with different Lpb. plantarum strains. The expression levels of Nod2, Tlr2 and Tlr9 were increased to statistically significant levels in the case of TLRs when exposed to all Lpb. plantarum strains (p < 0.05). On the other hand, the expression levels of Nod1, Tlr4 and Tlr5 seemed to be repressed although of these, but only Tlr5 appeared downregulated significantly (Figure 3). We appreciate that some of these alterations in gene expression are subtle (less than 0.5 to 1-fold) and the biological significance of the findings will be the subject of further work.
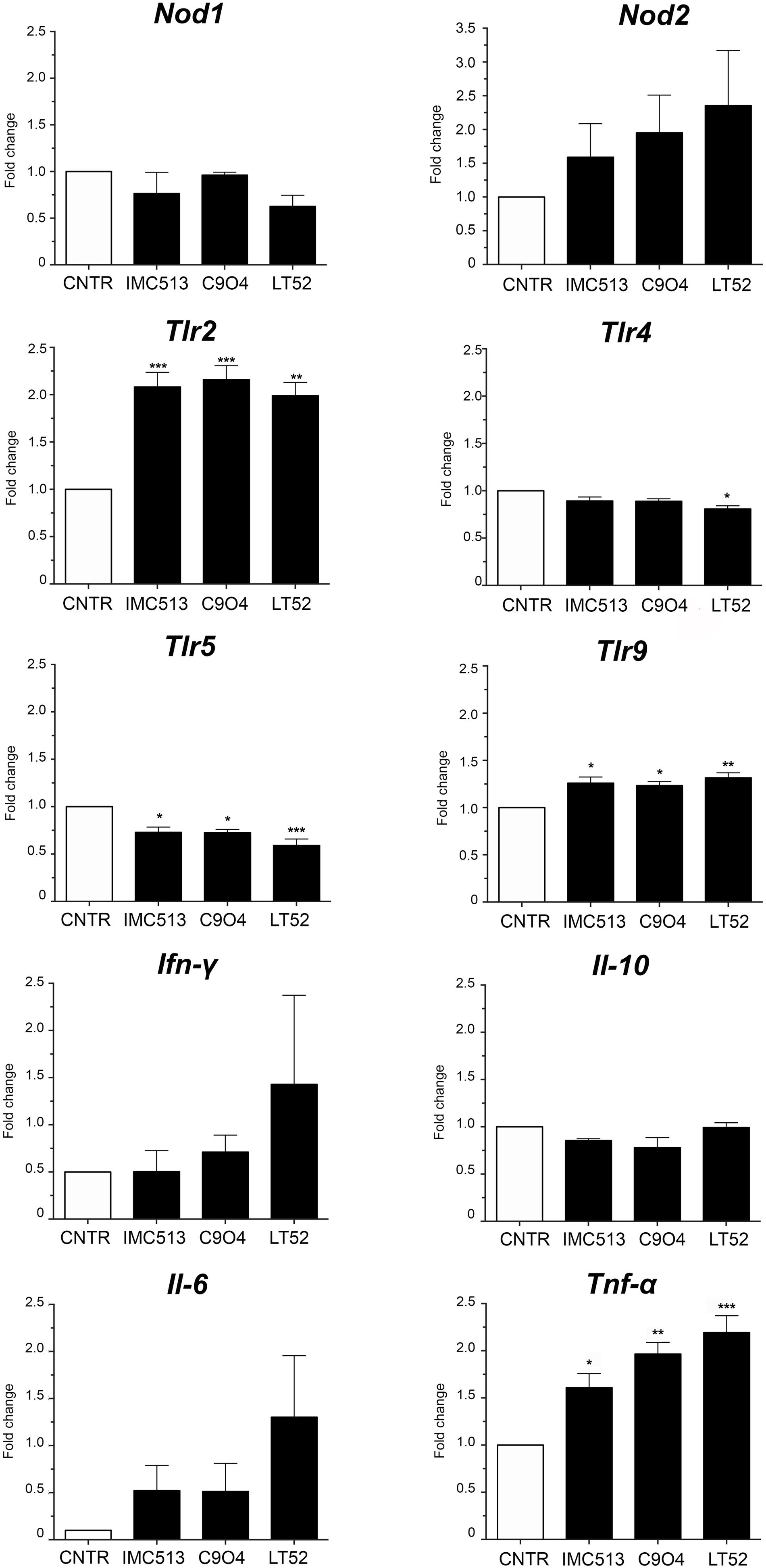
Figure 3. The expression level of TLRs, NLRs and cytokines. After 24 h incubation, the gene expression of NLRs (Nod1 and Nod2), TLRs (Tlr2, Tlr4, Tlr5, and Tlr9) and cytokines (Ifn-γ, Il-10, Il-6 and Tnf-α) was evaluated by qRT-PCR in macrophages incubated with Lpb. plantarum strains. Statistical analysis was conducted using One-way ANOVA followed by Bonferroni’s post hoc analysis. Values are expressed as mean ± SD, *p < 0.05, **p < 0.01, ***p < 0.001.
Regarding inflammatory biomarkers, the gene expression of Ifn-γ, Il-10, Il-6, and Tnf-α was evaluated after incubation with Lpb. plantarum strains (Figure 3). Results showed an increase of mRNA expression levels of Ifn-γ and Il-6, while the levels of Il-10 remained non-significant when compared to the control. On the other hand, the expression of Tnf-α was significantly increased with all the strains with LT52 promoting the strongest increase.
In order to explore the inflammatory response, the PRR-dependent release of IL-6 and TNF-α was quantified upon macrophage exposure to the Lpb. plantarum strains for 24 h using the non-pathogenic, gram-positive L. innocua as control. While no substantial response was found in the case of IL-6 (Figure 4A), TNF-α was found to be strongly induced upon exposure to all Lpb. plantarum strains in comparison to a control, with IMC513 and C904 strains showing a similar and higher release than LT52 but at lower levels than occurred upon exposure to L. innocua (Figure 4B).
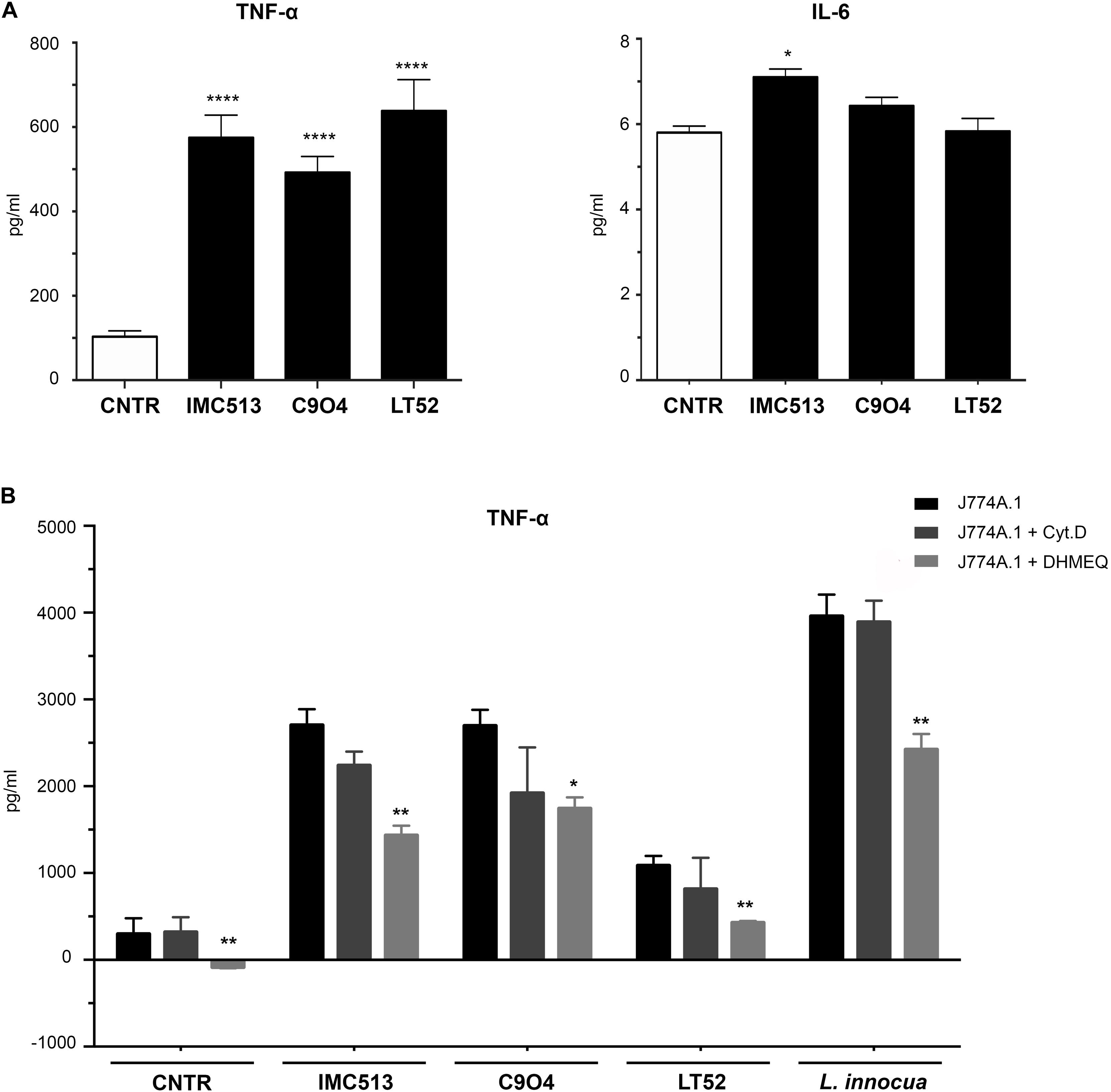
Figure 4. Quantification of the inflammatory response of murine macrophages J774A.1 using ELISA. (A) Release of IL-6 was found low, while strong amounts of TNF-α are shown in (B), where cells were seeded and exposed to the phagocytosis inhibitor drugs Cyt.D and DHMEQ for 24 h. Cells were subsequently stimulated with either Lpb. plantarum LT52, IMC513, and C904 or L. innocua for 24 h and supernatants collected. Values are expressed as mean ± SD and assessed by Student t-test, where *p < 0.05 and **p < 0.001.
To further confirm that the inflammatory response was specifically triggered upon phagocytosis, we confirmed under a microscope that all Lpb. plantarum strains could be found internalized in the macrophages at 6 h post-exposure. Indeed, further support was observed by employing the phagocytosis inhibitor Cyt.D or DHMEQ, which also inhibits the LPS-induced Nuclear Factor kappa-light-chain-enhancer of activated B cells (NF-kB) activation (Suzuki and Umezawa, 2006). Here, TNF-α release levels appeared lower upon exposure to Cyt.D but were statistically significant in all strains tested under the effect of DHMEQ only (Figure 4B). Taken together, these results support that the inflammatory response of macrophages to Lpb. plantarum exposure is strain specific and that phagocytosis is important to drive a full inflammatory response. The work is broadly supportive of a recent finding that certain strains with probiotic potential may stimulate intracellular NOD pathways (Brown et al., 2017).
NOD2 but Not TLRs Mediates NF-kb Activation by Lpb. plantarum Strains
In order to evaluate the mechanisms involved in the observed immune response and shed light on the nature of the PRR type contributing to macrophage activation upon Lpb. plantarum exposure, we performed a test using specific chemical inhibitors. IRAK4 and RIP2 proteins were selected as they are immediate in the downstream pathways activated by PRRs. IRAK4 is involved in Myd88 signaling cascades, the first domain recruited after activation of the TLRs, and plays an essential role in inflammation related disorders (Seganish, 2016), while RIP2 is critical for signal propagation of NLRs (Figure 5A). However, since Lpb. plantarum is able to interact with both type of receptors, the implication of each one in the cytokine release remains unclear. Thus, inhibitors of the proteins IRAK4 (IRAK4 inhibitor) and RIP2 (Gefinitib) were used in the study. The inhibition of RIP2 protein by Gefitinib significantly reduced the production of TNF-α induced by each Lpb. plantarum strains tested (Figure 5B). This suggests an inter-dependence on the RIP2 adaptor protein for an effective TNF-α response to the bacteria tested. RIP2 is the adaptor protein for NOD1 and NOD2, the decrease in cytokine production could be due to a dependence on signaling through the NOD2 receptor, the expression of which was activated by the Lpb. plantarum strains tested (Figure 3). On the other hand, inhibition of IRAK4 reduced, the production of TNF-α in macrophages after the incubation with Lpb. plantarum strains, although it was not statistically significant.
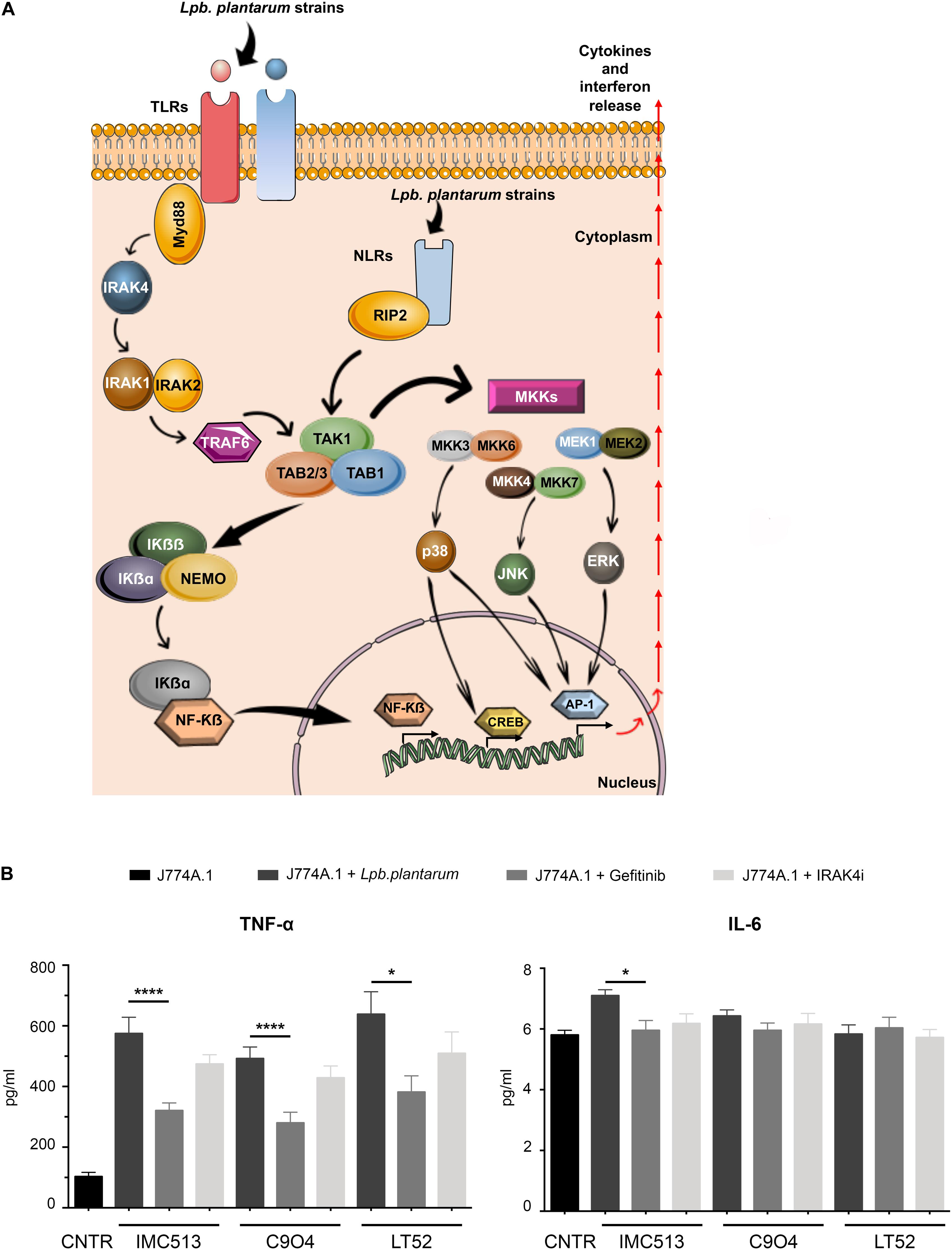
Figure 5. (A) Schematic representation of the signaling pathways activated downstream by PRR. This scheme is an adaptation of Plaza-Diaz et al. (2019) contains free available designs from Servier Medical Art templates (https://smart.servier.com/). (B) IL-6 and TNF-α content in J774A.1 macrophages treated with specific RIP2 inhibitor and IRAK4 inhibitors following Lpb. plantarum stimulation. Briefly, J774A.1 macrophages were seeded and pre-incubated with each inhibitor 1 h before bacterial stimulation. After the co-incubation, supernatants were collected and quantification of TNF-α and IL-6 release was evaluated by ELISA. Values are expressed as mean ± SD. Values were assessed by Student t-test comparing data for tests including inhibitors with the controls (stimulated by bacteria but lacking inhibitor). *p < 0.05, ****p < 0.0001.
Discussion
Understanding of the importance of fermented foods as a carrier of beneficial strains well-beyond their nutritional value has increased during the last century. This is in part due to recent studies in which the importance of diet in modulating gut microbiota and host physiology has been highlighted (Marco et al., 2017; Melini et al., 2019). In addition to the chemical composition of the food itself, the potential health advantages of fermented foods reside in resident microbes which may be consumed in large amounts. Recent studies have supported the potential benefits of these microbial strains due to their phylogenetic relationship with probiotic strains, predicting that their contribution to human health, could be similar to that of probiotics (Marco et al., 2017). However, there is still a lack of information regarding the mechanisms by which food resident microbes exert their functions and interact with host cells.
In accordance with the regulations outlined by FAO/WHO (2006), a potential probiotic strain should be able to survive in the gastrointestinal tract by exhibiting acid and bile tolerance, transiently colonize the gut and also be able to exert any benefits on the host (Hill et al., 2014). Moreover, probiotics should be harmless and not trigger any allergic response in the host (Sanders et al., 2010). Whilst such parameters are documented for probiotics used as functional food additives, the ability of food dwelling organisms to impact the host is less clear. In the current study we examined the interaction of Lpb. plantarum strains representative of natural strains consumed in different foods. Here we investigated the cellular location of Lpb. plantarum strains interacting with macrophages as well as their ability to stimulate cytokine/TLR expression and influence downstream signaling pathways.
The three Lpb. plantarum strains evaluated in this study have been tested for their ability to interact with human epithelial cells (Garcia-Gonzalez et al., 2018), to inhibit the biological activity of genotoxic compounds (Prete et al., 2017) and to survive bile-acid related stress (Prete et al., 2020). In view of their effects on the response of epithelial NCM460 cells (Garcia-Gonzalez et al., 2018), we evaluated their potential impact on immune system-cells using J774A.1 macrophages. Murine macrophages J774A.1 were selected as they express the PRRs involved in the recognition of bacterial pathogens and commensals and thereby mimic macrophages, mainly located in the lamina propria, which are adapted to remove pathogens and to maintain intestinal homeostasis (Ferreira dos Santos et al., 2016).
The cellular location of bacteria interacting with macrophages influences the triggering of specific PRRs (Figure 5A). We therefore evaluated the dynamics of bacterial interaction with macrophages in our assay system. Results showed that 4 h after incubation with bacteria, most of the total-associated bacteria corresponded to those phagocytosed by macrophages. Thus, the cross talk between macrophages and bacteria has the potential to be mediated by both extra- and intra-cellular PRRs (Philpott and Girardin, 2004; Fukata et al., 2009). In this study, we have focused on the two major groups of PRRs: TLRs and NLRs. TLRs are trans-membrane receptors, which can be either expressed on the cell surface or in intracellular organelles. TLRs differ from each other in ligand specificities, expression patterns, and in the target genes, and they can modulate (Janeway and Medzhitov, 2002) and play a critical role in the induction of immune responses (Macho Fernandez et al., 2011; Zhong et al., 2012). In particular TLR2, TLR4, TLR5, and TLR9 were selected here because of their involvement in recognition of bacteria associated molecular patterns (Konieczna et al., 2015; Wang et al., 2016). In contrast NOD1 and NOD2 (within the NLR family) act as cytoplasmic microbial sensors of intracellular bacteria recognizing peptidoglycan. The results show that Lpb. plantarum strains increase the expression of Tlr2, Tlr9, and Nod2. The profile of receptors activated was similar within the three Lpb. plantarum strains. The importance of TLR2 and NOD2 in recognizing bacteria has been previously described (Zeuthen et al., 2008; Jiang et al., 2013). The positive regulation of NOD2 by Lpb. plantarum in restoring homeostasis in germ-free mice highlights the interconnection between NOD2 and commensal bacteria (Petnicki-Ocwieja et al., 2009). Moreover, TLR2 seems to be crucial in the recognition of lactic acid bacteria, both in immune cells and epithelial cells where it has been implicated as a regulator of the epithelial integrity (Karczewski et al., 2010; Ren et al., 2016; Wang et al., 2019). While under inflammatory conditions it has been demonstrated that intestinal cells increase their expression of TLR2 and TLR4, the apical stimulation of TLR9 has been described to be anti-inflammatory (Lee et al., 2006).
In order to elucidate the immunomodulatory ability of Lpb. plantarum strains, the expression of a panel of inflammatory biomarkers was evaluated through qRT-PCR. Among the cytokines evaluated to measure the impact of Lpb. plantarum strains, TNF-α and IL-6 were selected as their release is directly induced by the activation of PRRs (Tanaka et al., 2014; Lee et al., 2016b). When PRRs are activated, they stimulate a range of signaling pathways, including NF-κB, and enhance the transcription of the mRNA of inflammatory cytokines such as TNF-α and IL-1ß. The release of TNF-α also activates the transcription factors to produce IL-6 (Tanaka et al., 2014). Cytokines, such as TNF-α and IL-6 play an important role in regulating immune response and inflammation (Luig et al., 2015; Lee et al., 2016b). Our results showed that there is an increase of TNF-α production induced by Lpb. plantarum strains but a weaker induction of IL-6. Indeed, Lactobacilli seem to be strong inducers of pro-inflammatory cytokines such as TNF-α (Matsubara et al., 2017; Štofilová et al., 2017). In particular, it has been proved that Lpb. plantarum LS/07 moderately induce pro-inflammatory cytokines from macrophages, whereas a higher induction of TNF-α release was observed after the co-culture of either monocyte/macrophages with the bacteria (Štofilová et al., 2017). However, like other probiotic properties described, the ability to modulate the immune system through the release of cytokines is strain-dependent, and thus results in literature can be contradictory (Lomax and Calder, 2009). The study conducted by Lee and colleagues underlined the different cytokine-inducing abilities of two Lpb. plantarum strains isolated from different sources. Differences in their capacity to stimulate cytokines and in the pattern of cytokines stimulated may be due to the structural differences in the cell wall of the bacteria. Small differences in the lipoteichoic acid and/or expression of different exopolysaccharides could lead to different biological functions (Lee et al., 2016a, b).
On the other hand, IL-6 is known to have both pro- and anti-inflammatory properties (Zielińska et al., 2019). The absence of IL-6 release by macrophages may help to convert an innate response into an adaptative response against pathogens (Gabay, 2006). This combined with the absence in TLR4 activation by Lpb. plantarum strains may reflect the anti-inflammatory effects of Lpb. plantarum strains. Since TLR4 signaling stimulates expression of genes encoding inflammatory molecules, a lack of activation of TLR4 by Lpb. plantarum strains favors an outcome that is less inflammatory, and may explain the reduced production of proinflammatory IL-6 as seen in our study.
We investigated whether cytokine production was linked to bacterial engagement with surface TLRs or intracellular NLRs in our system. Previous studies have shown that simultaneous activation of TLRs in macrophages leads to different cytokine expression profiles in comparison to stimulation of individual PRRs (Sato et al., 2000). TLRs share similar downstream signaling pathways via the NFκB cascade, through the adaptor proteins MyD88 firstly and subsequently IRAK4 (Chiu et al., 2013), whereas NOD1 and NOD2 use the RIP2 protein as an adaptor (Figure 5A). We used inhibitors of RIP2 and IRAK4 in our assay system and showed that a major decrease in TNF-α release was found following RIP2 inhibition. This implies that the majority of macrophage stimulation is via activation of the NLRs, a finding that reflects the fact that in our assay system, the majority of Lpb. plantarum strains are rapidly internalized by macrophages. Residual TNF-α release in the presence of the RIP2 inhibitor may be due to involvement of Myd88, illustrating that stimulation of macrophages by commensal bacteria is complex and multifactorial.
The immunomodulatory effects induced by food-related Lpb. plantarum strains may lead to enhancement of macrophage activation, however, further experiments will be necessary in order to establish the functional outcome resulting from conditioning of macrophages by Lpb. plantarum strains.
Conclusion
Our findings showed that the two Lpb. plantarum strains which are representative of food-associated strains (Stanton et al., 1998; Perpetuini et al., 2020) were able to interact with macrophages to induce responses that are similar to a strain proposed to have probiotic potential. This paper indicates that internalized Lpb. plantarum strains are capable of stimulating macrophage responses through engagement of the NLR pathway, but are not cytotoxic under the conditions examined. As foods such as fermented table olives have been shown to contain high levels of Lpb. plantarum strains (represented by the C904 strain utilized here) the work suggests that autochthonous Lpb. plantarum from specific foods may have immune modulating effects when consumed in sufficient quantities.
Data Availability Statement
The data available at: https://zenodo.org/record/3775511#.XqlxqZNKiS4.
Author Contributions
AC, CG, and NB designed the study. NG-G, MAN-S, and MVR performed the experiments. NG-G, MAN-S, MVR, NB, CG, and AC analyzed the data, discussed the results, and drafted the manuscript. All the authors read and approved the final manuscript. All authors contributed to the article and approved the submitted version.
Funding
This work was supported by Italian Ministry of University and Research (PRIN Project 20152LFKAT to AC) and has received financial support from the European Union’s Horizon 2020 Research and Innovation Program under the Marie Skłodowska-Curie grant agreement 713714 ESR 07 (to NG-G) and the grant agreement 796838 (to MAN-S). We acknowledge funding from Science Foundation Ireland (SFI) to APC Microbiome Ireland under grant SFI/12/RC/2273_P2.
Conflict of Interest
The authors declare that the research was conducted in the absence of any commercial or financial relationships that could be construed as a potential conflict of interest.
Acknowledgments
We gratefully acknowledge Prof. A. Cresci (Synbiotec, Camerino, Italy) for kindly providing us with the Lpb. plantarum probiotic strain IMC513. We wish to sincerely acknowledge the contribution of Gonzalo Saiz for technical support with one of the assay systems. We acknowledge helpful discussions with Dr. Silvia Melgar.
Supplementary Material
The Supplementary Material for this article can be found online at: https://www.frontiersin.org/articles/10.3389/fmicb.2020.557143/full#supplementary-material
Supplementary Figure 1 | Light microscopy of Lactiplantibacillus plantarum cells and J774A.1 macrophages using Giemsa staining. (A) Lpb. plantarum LT52, IMC513, and C904 cells only. (B) in vitro exposure of J774A.1 to Lpb. plantarum LT52, IMC513, and C904 cells. Bacteria are phagocytosed and found inside macrophages cytoplasm, at least, 6 h post exposure.
References
Bäuerl, C., Llopis, M., Antolín, M., Monedero, V., Mata, M., Zúñiga, M., et al. (2013). Lactobacillus paracasei and Lactobacillus plantarum strains downregulate proinflammatory genes in an ex vivo system of cultured human colonic mucosa. Genes Nutr. 8, 165–180. doi: 10.1007/s12263-012-0301-y
Behera, S. S., Ray, R. C., and Zdolec, N. (2018). Lactobacillus plantarum with functional properties: an approach to increase safety and shelf-life of fermented foods. Biomed. Res. Int. 2018:9361614. doi: 10.1155/2018/9361614
Brown, R. L., Sequeira, R. P., and Clarke, T. B. (2017). The microbiota protects against respiratory infection via GM-CSF signaling. Nat. Commun. 8:1512. doi: 10.1038/s41467-017-01803-x
Cani, P. D., Bibiloni, R., Knauf, C., Waget, A., Neyrinck, A. M., Delzenne, N. M., et al. (2008). Changes in gut microbiota control metabolic endotoxemia-induced inflammation in high-fat diet-induced obesity and diabetes in mice. Diabetes 57, 1470–1481. doi: 10.2337/db07-1403
Chiu, Y. H., Lu, Y. C., Ou, C. C., Lin, S. L., Tsai, C. C., Huang, C. T., et al. (2013). Lactobacillus plantarum MYL26 induces endotoxin tolerance phenotype in Caco-2 cells. BMC Microbiol. 13:190. doi: 10.1186/1471-2180-13-190
Corsetti, A., Prete, R., and Garcia-Gonzalez, N. (2018). “Lactic acid bacteria: Lactobacillus spp. Lactobacillus plantarum,” in Reference Module in Food Science, ed. Elsevier (New York, NY: Elsevier), 1–8.
Corthésy, B., Gaskins, H. R., and Mercenier, A. (2007). Cross-talk between probiotic bacteria and the host immune system. J. Nutr. 137(3 Suppl. 2), 781S–790S. doi: 10.1093/jn/137.3.781S
de Vrese, M., and Schrezenmeir, J. (2008). Probiotics, prebiotics, and synbiotics. Adv. Biochem. Eng. Biotechnol. 111, 1–66. doi: 10.1007/10_2008_097
Dong, H., Rowland, I., Tuohy, K. M., Thomas, L. V., and Yaqoob, P. (2010). Selective effects of Lactobacillus casei Shirota on T cell activation, natural killer cell activity and cytokine production. Clin. Exp. Immunol. 161, 378–388. doi: 10.1111/j.1365-2249.2010.04173.x
FAO/WHO (2006). Probiotics in Food. Health and Nutritional Properties and Guidelines for Evaluation. Rome: FAO Food Nutrition.
Ferreira dos Santos, T., Alves Melo, T., Evangelista Almeida, M., Passos Rezende, R., and Cristina Romano, C. (2016). Immunomodulatory effects of Lactobacillus plantarum Lp62 on intestinal epithelial and mononuclear cells. Biomed. Res. Int. 2016:8404156. doi: 10.1155/2016/8404156
Fukata, M., Vamadevan, A. S., and Abreu, M. T. (2009). Toll-like receptors (TLRs) and Nod-like receptors (NLRs) in inflammatory disorders. Semin. Immunol. 21, 242–253. doi: 10.1016/j.smim.2009.06.005
Gabay, C. (2006). Interleukin-6 and chronic inflammation. Arthritis Res. Ther. 8(Suppl. 2):S3. doi: 10.1186/ar1917
Garcia-Gonzalez, N., Prete, R., Battista, N., and Corsetti, A. (2018). Adhesion properties of food-associated Lactobacillus plantarum strains on human intestinal cells and modulation of IL-8 release. Front. Microbiol. 9:2392. doi: 10.3389/fmicb.2018.02392
Ghadimi, D., Vrese, M., Heller, K. J., and Schrezenmeir, J. (2010). Effect of natural commensal-origin DNA on toll-like receptor 9 (TLR9) signaling cascade, chemokine IL-8 expression, and barrier integritiy of polarized intestinal epithelial cells. Inflamm. Bowel Dis. 16, 410–427. doi: 10.1002/ibd.21057
Heperkan, D. (2013). Microbiota of table olive fermentations and criteria of selection for their use as starters. Front. Microbiol. 4:143. doi: 10.3389/fmicb.2013.00143
Hill, C., Guarner, F., Reid, G., Gibson, G. R., Merenstein, D. J., Pot, B., et al. (2014). Expert consensus document. The international scientific association for probiotics and prebiotics consensus statement on the scope and appropriate use of the term probiotic. Nat. Rev. Gastroenterol. Hepatol. 11, 506–514. doi: 10.1038/nrgastro.2014.66
Hurtado, A., Reguant, C., Bordons, A., and Rozès, N. (2012). Lactic acid bacteria from fermented table olives. Food Microbiol. 31, 1–8. doi: 10.1016/j.fm.2012.01.006
Ivec, M., Botić, T., Koren, S., Jakobsen, M., Weingartl, H., and Cencic, A. (2007). Interactions of macrophages with probiotic bacteria lead to increased antiviral response against vesicular stomatitis virus. Antiviral Res. 75, 266–274. doi: 10.1016/j.antiviral.2007.03.013
Janeway, C. A., and Medzhitov, R. (2002). Innate immune recognition. Annu. Rev. Immunol. 20, 197–216. doi: 10.1146/annurev.immunol.20.083001.084359
Jiang, W., Wang, X., Zeng, B., Liu, L., Tardivel, A., Wei, H., et al. (2013). Recognition of gut microbiota by NOD2 is essential for the homeostasis of intestinal intraepithelial lymphocytes. J. Exp. Med. 210, 2465–2476. doi: 10.1084/jem.20122490
Kang, H. J., and Im, S. H. (2015). Probiotics as an immune modulator. J. Nutr. Sci. Vitaminol. (Tokyo) 61(Suppl.), S103–S105. doi: 10.3177/jnsv.61.S103
Karczewski, J., Troost, F. J., Konings, I., Dekker, J., Kleerebezem, M., Brummer, R. J., et al. (2010). Regulation of human epithelial tight junction proteins by Lactobacillus plantarum in vivo and protective effects on the epithelial barrier. Am. J. Physiol. Gastrointest. Liver Physiol. 298, G851–G859. doi: 10.1152/ajpgi.00327.2009
Konieczna, P., Schiavi, E., Ziegler, M., Groeger, D., Healy, S., Grant, R., et al. (2015). Human dendritic cell DC-SIGN and TLR-2 mediate complementary immune regulatory activities in response to Lactobacillus rhamnosus JB-1. PLoS One 10:e0120261. doi: 10.1371/journal.pone.0120261
Lee, I. C., Caggianiello, G., van Swam, I. I., Taverne, N., Meijerink, M., Bron, P. A., et al. (2016a). Strain-specific features of extracellular polysaccharides and their impact on Lactobacillus plantarum-host interactions. Appl. Environ. Microbiol. 82, 3959–3970. doi: 10.1128/AEM.00306-16
Lee, J., Mo, J. H., Katakura, K., Alkalay, I., Rucker, A. N., Liu, Y. T., et al. (2006). Maintenance of colonic homeostasis by distinctive apical TLR9 signalling in intestinal epithelial cells. Nat. Cell Biol. 8, 1327–1336. doi: 10.1038/ncb1500
Lee, Y. D., Hong, Y. F., Jeon, B., Jung, B. J., Chung, D. K., and Kim, H. (2016b). Differential cytokine regulatory effect of three Lactobacillus strains isolated from fermented foods. J. Microbiol. Biotechnol. 26, 1517–1526. doi: 10.4014/jmb.1601.01044
Lomax, A. R., and Calder, P. C. (2009). Probiotics, immune function, infection and inflammation: a review of the evidence from studies conducted in humans. Curr. Pharm. Des. 15, 1428–1518. doi: 10.2174/138161209788168155
Luig, M., Kluger, M. A., Goerke, B., Meyer, M., Nosko, A., Yan, I., et al. (2015). Inflammation-induced IL-6 functions as a natural brake on macrophages and limits GN. J. Am. Soc. Nephrol. 26, 1597–1607. doi: 10.1681/ASN.2014060620
Macho Fernandez, E., Valenti, V., Rockel, C., Hermann, C., Pot, B., Boneca, I. G., et al. (2011). Anti-inflammatory capacity of selected lactobacilli in experimental colitis is driven by NOD2-mediated recognition of a specific peptidoglycan-derived muropeptide. Gut 60, 1050–1059. doi: 10.1136/gut.2010.232918
Marco, M. L., Heeney, D., Binda, S., Cifelli, C. J., Cotter, P. D., Foligné, B., et al. (2017). Health benefits of fermented foods: microbiota and beyond. Curr. Opin. Biotechnol. 44, 94–102. doi: 10.1016/j.copbio.2016.11.010
Markowiak, P., and Śliżewska, K. (2017). Effects of probiotics, prebiotics, and synbiotics on human health. Nutrients 9:E1021. doi: 10.3390/nu9091021
Matsubara, V. H., Ishikawa, K. H., Ando-Suguimoto, E. S., Bueno-Silva, B., Nakamae, A. E. M., and Mayer, M. P. A. (2017). Probiotic bacteria alter pattern-recognition receptor expression and cytokine profile in a human macrophage model challenged with Candida albicans and lipopolysaccharide. Front. Microbiol. 8:2280. doi: 10.3389/fmicb.2017.02280
Melini, F., Melini, V., Luziatelli, F., Ficca, A. G., and Ruzzi, M. (2019). Health-promoting components in fermented foods: an up-to-date systematic review. Nutrients 11:1189. doi: 10.3390/nu11051189
Melmed, G., Thomas, L. S., Lee, N., Tesfay, S. Y., Lukasek, K., Michelsen, K. S., et al. (2003). Human intestinal epithelial cells are broadly unresponsive to Toll-like receptor 2-dependent bacterial ligands: implications for host-microbial interactions in the gut. J. Immunol. 170, 1406–1415. doi: 10.4049/jimmunol.170.3.1406
Paolillo, R., Romano Carratelli, C., Sorrentino, S., Mazzola, N., and Rizzo, A. (2009). Immunomodulatory effects of Lactobacillus plantarum on human colon cancer cells. Int. Immunopharmacol. 9, 1265–1271. doi: 10.1016/j.intimp.2009.07.008
Pathmakanthan, S., Li, C. K., Cowie, J., and Hawkey, C. J. (2004). Lactobacillus plantarum 299: beneficial in vitro immunomodulation in cells extracted from inflamed human colon. J. Gastroenterol. Hepatol. 19, 166–173. doi: 10.1111/j.1440-1746.2004.03181.x
Perpetuini, G., Prete, R., Garcia-Gonzalez, N., Khairul Alam, M., and Corsetti, A. (2020). Table olives more than a fermented food. Foods 9:E178. doi: 10.3390/foods9020178
Petnicki-Ocwieja, T., Hrncir, T., Liu, Y. J., Biswas, A., Hudcovic, T., Tlaskalova-Hogenova, H., et al. (2009). Nod2 is required for the regulation of commensal microbiota in the intestine. Proc. Natl. Acad. Sci. U.S.A. 106, 15813–15818. doi: 10.1073/pnas.0907722106
Philpott, D. J., and Girardin, S. E. (2004). The role of Toll-like receptors and Nod proteins in bacterial infection. Mol. Immunol. 41, 1099–1108. doi: 10.1016/j.molimm.2004.06.012
Plaza-Diaz, J., Ruiz-Ojeda, F. J., Gil-Campos, M., and Gil, A. (2019). Mechanisms of action of probiotics. Adv. Nutr. 10, S49–S66. doi: 10.1093/advances/nmy063
Plot, B., Felis, G., De Bruyne, K., Tsakalidou, E., Papadimitriou, K., Leisner, J., et al. (2014). Lactic Acid Bacteria: Biodiversity and Taxonomy, eds W. H. Holzapfel and B. J. B. Wood (Hoboken, NJ: John Wiley & Sons).
Prete, R., Long, S. L., Gallardo, A. L., Gahan, C. G., Corsetti, A., and Joyce, S. A. (2020). Beneficial bile acid metabolism from Lactobacillus plantarum of food origin. Sci. Rep. 10:1165. doi: 10.1038/s41598-020-58069-5
Prete, R., Tofalo, R., Federici, E., Ciarrocchi, A., Cenci, G., and Corsetti, A. (2017). Food-associated. Front. Microbiol. 8:2349. doi: 10.3389/fmicb.2017.02349
Ren, C., Zhang, Q., de Haan, B. J., Zhang, H., Faas, M. M., and de Vos, P. (2016). Identification of TLR2/TLR6 signalling lactic acid bacteria for supporting immune regulation. Sci. Rep. 6:34561. doi: 10.1038/srep34561
Ryan, P. M., Stolte, E. H., London, L. E. E., Wells, J. M., Long, S. L., Joyce, S. A., et al. (2019). Lactobacillus mucosae DPC 6426 as a bile-modifying and immunomodulatory microbe. BMC Microbiol. 19:33. doi: 10.1186/s12866-019-1403-0
Sanders, M. E., Akkermans, L. M., Haller, D., Hammerman, C., Heimbach, J., Hörmannsperger, G., et al. (2010). Safety assessment of probiotics for human use. Gut Microbes 1, 164–185. doi: 10.4161/gmic.1.3.12127
Sato, S., Nomura, F., Kawai, T., Takeuchi, O., Mühlradt, P. F., Takeda, K., et al. (2000). Synergy and cross-tolerance between toll-like receptor (TLR) 2- and TLR4-mediated signaling pathways. J. Immunol. 165, 7096–7101. doi: 10.4049/jimmunol.165.12.7096
Seddik, H. A., Bendali, F., Gancel, F., Fliss, I., Spano, G., and Drider, D. (2017). Lactobacillus plantarum and its probiotic and food potentialities. Probiotics Antimicrob. Proteins 9, 111–122. doi: 10.1007/s12602-017-9264-z
Seganish, W. M. (2016). Inhibitors of interleukin-1 receptor-associated kinase 4 (IRAK4): a patent review (2012-2015). Expert Opin. Ther. Pat. 26, 917–932. doi: 10.1080/13543776.2016.1202926
Stanton, C., Gardiner, G., Lynch, P., Collins, J., Fitzgerald, G., and Ross, R. (1998). Probiotic cheese. Int. Dairy J. 8, 491–496.
Štofilová, J., Langerholc, T., Botta, C., Treven, P., Gradišnik, L., Salaj, R., et al. (2017). Cytokine production in vitro and in rat model of colitis in response to Lactobacillus plantarum LS/07. Biomed. Pharmacother. 94, 1176–1185. doi: 10.1016/j.biopha.2017.07.138
Suzuki, E., and Umezawa, K. (2006). Inhibition of macrophage activation and phagocytosis by a novel NF-kappaB inhibitor, dehydroxymethylepoxyquinomicin. Biomed. Pharmacother. 60, 578–586. doi: 10.1016/j.biopha.2006.07.089
Tallon, R., Arias, S., Bressollier, P., and Urdaci, M. C. (2007). Strain- and matrix-dependent adhesion of Lactobacillus plantarum is mediated by proteinaceous bacterial compounds. J. Appl. Microbiol. 102, 442–451. doi: 10.1111/j.1365-2672.2006.03086.x
Tanaka, T., Narazaki, M., and Kishimoto, T. (2014). IL-6 in inflammation, immunity, and disease. Cold Spring Harb. Perspect. Biol. 6:a016295. doi: 10.1101/cshperspect.a016295
Vargas García, C. E., Petrova, M., Claes, I. J., De Boeck, I., Verhoeven, T. L., Dilissen, E., et al. (2015). Piliation of Lactobacillus rhamnosus GG promotes adhesion, phagocytosis, and cytokine modulation in macrophages. Appl. Environ. Microbiol. 81, 2050–2062. doi: 10.1128/AEM.03949-14
Wang, J., Zhang, W., Wang, S., Liu, H., Zhang, D., Wang, Y., et al. (2019). Swine-derived probiotic Lactobacillus plantarum modulates porcine intestinal endogenous host defense peptide synthesis through TLR2/MAPK/AP-1 signaling pathway. Front. Immunol. 10:2691. doi: 10.3389/fimmu.2019.02691
Wang, Y., Xie, J., Li, Y., Dong, S., Liu, H., Chen, J., et al. (2016). Probiotic Lactobacillus casei Zhang reduces pro-inflammatory cytokine production and hepatic inflammation in a rat model of acute liver failure. Eur. J. Nutr. 55, 821–831. doi: 10.1007/s00394-015-0904-3
Weibel, G. L., Joshi, M. R., Jerome, W. G., Bates, S. R., Yu, K. J., Phillips, M. C., et al. (2012). Cytoskeleton disruption in J774 macrophages: consequences for lipid droplet formation and cholesterol flux. Biochim. Biophys. Acta 1821, 464–472. doi: 10.1016/j.bbalip.2011.09.015
Wells, J. M. (2011). Immunomodulatory mechanisms of lactobacilli. Microb. Cell Fact. 10:S17. doi: 10.1186/1475-2859-10-S1-S17
Zeuthen, L. H., Fink, L. N., and Frøkiaer, H. (2008). Toll-like receptor 2 and nucleotide-binding oligomerization domain-2 play divergent roles in the recognition of gut-derived lactobacilli and bifidobacteria in dendritic cells. Immunology 124, 489–502. doi: 10.1111/j.1365-2567.2007.02800.x
Zheng, J., Wittouck, S., Salvetti, E., Franz, C. M. A. P., Harris, H. M. B., Mattarelli, P., et al. (2020). A taxonomic note on the genus Lactobacillus: description of 23 novel genera, emended description of the genus Lactobacillus Beijerinck 1901, and union of Lactobacillaceae and Leuconostocaceae. Int. J. Syst. Evol. Microbiol. 70, 2782–2858. doi: 10.1099/ijsem.0.004107
Zhong, Y., Huang, J., Tang, W., Chen, B., and Cai, W. (2012). Effects of probiotics, probiotic DNA and the CpG oligodeoxynucleotides on ovalbumin-sensitized Brown-Norway rats via TLR9/NF-κB pathway. FEMS Immunol. Med. Microbiol. 66, 71–82. doi: 10.1111/j.1574-695X.2012.00991.x
Keywords: cytokines, food-borne microbes, health-promoting bacteria, immune system, Lactiplantibacillus plantarum, macrophages
Citation: Garcia-Gonzalez N, Nuñez-Sanchez MA, Villoria Recio M, Battista N, Gahan CGM and Corsetti A (2021) Immunomodulation of J774A.1 Murine Macrophages by Lactiplantibacillus plantarum Strains Isolated From the Human Gastrointestinal Tract and Fermented Foods. Front. Microbiol. 11:557143. doi: 10.3389/fmicb.2020.557143
Received: 29 April 2020; Accepted: 14 December 2020;
Published: 12 January 2021.
Edited by:
Joaquin Bautista-Gallego, University of Extremadura, SpainReviewed by:
Maria Guadalupe Vizoso Pinto, Consejo Nacional de Investigaciones Científicas y Técnicas (CONICET), ArgentinaDaniela Fiocco, University of Foggia, Italy
Copyright © 2021 Garcia-Gonzalez, Nuñez-Sanchez, Villoria Recio, Battista, Gahan and Corsetti. This is an open-access article distributed under the terms of the Creative Commons Attribution License (CC BY). The use, distribution or reproduction in other forums is permitted, provided the original author(s) and the copyright owner(s) are credited and that the original publication in this journal is cited, in accordance with accepted academic practice. No use, distribution or reproduction is permitted which does not comply with these terms.
*Correspondence: Cormac G. M. Gahan, c.gahan@ucc.ie
†These authors have contributed equally to this work