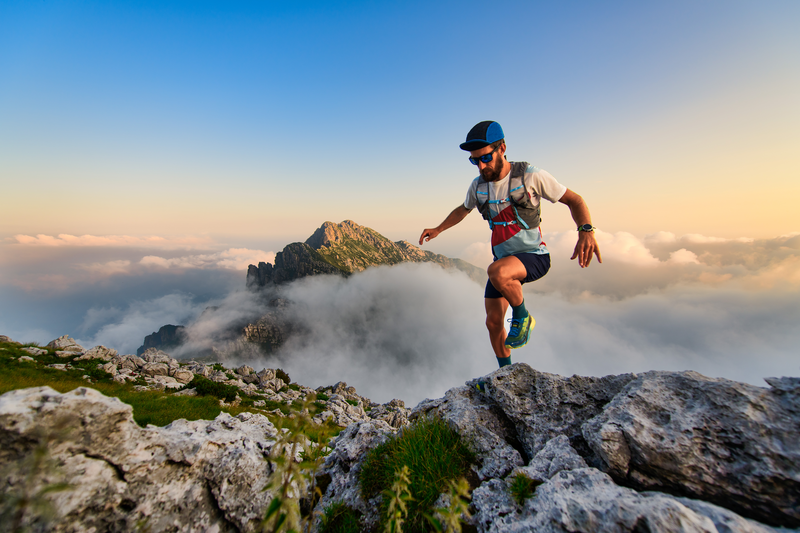
94% of researchers rate our articles as excellent or good
Learn more about the work of our research integrity team to safeguard the quality of each article we publish.
Find out more
ORIGINAL RESEARCH article
Front. Microbiol. , 04 September 2020
Sec. Fungi and Their Interactions
Volume 11 - 2020 | https://doi.org/10.3389/fmicb.2020.01980
This article is part of the Research Topic Candida Biofilms View all 18 articles
Dermatomycoses include superficial fungal infections of the skin and its appendages. Trichophyton rubrum, Candida albicans, and Candida parapsilosis are some of the most prevalent species that cause dermatomycoses. Several studies show a variable predominance of Candida spp. in relation to dermatophytes, especially in onychomycosis and the possibility of isolating both from the same site. The ability of dermatophytes to form biofilms recently been explored and there is currently no evidence on the involvement of these filamentous fungi in multi-species biofilms. Thus, this study aims to investigate the probable dual-species interaction between T. rubrum and C. albicans and T. rubrum and C. parapsilosis biofilms, considering variable formation conditions, as well as the susceptibility of these dual-species biofilms against terbinafine and efinaconazole. Three conditions of formation of dual-species biofilms were tested: (a) the suspensions of T. rubrum and Candida albicans or C. parapsilosis placed together; (b) suspensions of C. albicans and C. parapsilosis added the pre-adhesion of T. rubrum biofilms; (c) after the maturation of T. rubrum sessile cells. In the first and second conditions, the quantification of metabolic activities, biomass, and polysaccharide materials of mixed biofilms tended to resemble Candida monospecies biofilms. In the third condition, the profiles were modified after the addition of Candida, suggesting that T. rubrum biofilms served as substrate for the development of Candida biofilms. Scanning electron microscopy showed Candida predominance, however, numerous blastoconidia were noted, most evident in the conditions under which Candida was added after the pre-adhesion and maturation of T. rubrum biofilms. Despite the predominance of Candida, the presence of T. rubrum appears to inhibit C. albicans filamentation and C. parapsilosis development, confirming an antagonistic interaction. Fungal burden assays performed when the biofilms were formed together confirmed Candida predominance, as well as susceptibility to antifungals. Further studies will be needed to identify the components of the Candida and T. rubrum biofilm supernatants responsible for inhibiting dermatophyte growth and C. albicans filamentation.
Fungal infections of the skin, nails (onychomycosis), and cutaneous-mucous surfaces, known as dermatomycosis, are caused by several etiological agents and are an important cause of morbidity (Rotta et al., 2012). Among dermatomycosis, dermatophytosis, caused by dermatophytes, are the most prevalent worldwide, affecting 20 to 25% of the population (Havlickova et al., 2008; Zhan and Liu, 2017). Trichophyton rubrum is the microorganism most frequently associated with superficial mycoses (Costa-Orlandi et al., 2012, 2014; Zhan and Liu, 2017; Celestrino et al., 2019). Previous studies have reported that some strains of T. rubrum have a high dissemination power in relation to other dermatophytes, showing the dominance of this microorganism among dermatophytosis (Ghannoum et al., 2013).
Regarding yeasts of the genus Candida, C. albicans is the pathogen most commonly isolated from superficial mycoses. However, the incidence of infections by non-C. albicans species, such as C. parapsilosis, C. tropicalis, C. krusei, C. glabrata, and C. guilliermondii, has been increasing considerably (Sardi et al., 2013). Among, yeasts of C. parapsilosis are considered emerging pathogens and are commensal microorganisms of human skin. This microorganism is able to form biofilms in catheters and is transmitted through physical touch to neonates and in the hemodialysis systems used by chronic renal patients, thereafter evolving into bloodstream infections (Trofa et al., 2008; Pires et al., 2011, 2012).
Clinical practice cases and unpublished studies have demonstrated that it is possible to isolate one or more microorganisms from several infection sites in superficial mycoses, with a marked predominance of dermatophytes in comparison to yeasts of the genus Candida (Ghannoum et al., 2000; Aquino et al., 2007; Costa-Orlandi et al., 2012). Other studies indicate a higher prevalence of yeasts, especially C. parapsilosis in relation to C. albicans and T. rubrum in patients with onychomycosis (Pontes et al., 2002; Chadeganipour et al., 2010; Costa-Orlandi et al., 2012).
One of the major virulence factors of Candida is its ability to form biofilms (Ramage et al., 2012), which has also been reported recently in dermatophytes (Costa-Orlandi et al., 2014). Biofilms consist of communities of cells embedded in a polymeric extracellular matrix. These communities can be comprised of microorganisms of different species, genera or even kingdoms with a highly complex organization, allowing them to perform various functions, including nutrition, excretion, growth, communication, and protection, with greater efficiency (Ramage et al., 2009, 2012; Costa-Orlandi et al., 2017). Unlike planktonic cells, those found in biofilms have different morphologies depending on their location in the community (Ramage et al., 2012). For example, the morphology of C. albicans cells in biofilms vary from that of yeasts, hyphae, pseudohyphae, and germ tube, while C. parapsilosis consists mainly of yeasts and few pseudohyphae (Chandra and Mukherjee, 2015). Communities formed by dermatophytes are made up of a dense network of interconnected hyphae covered and embedded by an extracellular matrix that can be dense or thin (Costa-Orlandi et al., 2014). Changes in morphology are often regulated by molecules used for communication between cells, through a mechanism known as quorum-sensing, which has been widely studied in Candida biofilms but not yet investigated in dermatophytes. In addition to morphology, quorum-sensing is responsible for biofilm development, cell population control, nutrient competition control, dissemination, and the establishment of new communities during infection (Wongsuk et al., 2016).
Compared to the information available on biofilms formed by yeasts of the genus Candida, information on biofilms formed by dermatophytes is greatly lacking. Studies have previously found dermatophytes cohabiting with yeasts of the genus Candida and other microorganisms in superficial infections (unpublished data). This has also been widely observed in clinical practice. Therefore, the formation of mixed biofilms between these fungi is likely since biofilms provide many advantages for the microorganisms involved and rarely involve only one species (Costa-Orlandi et al., 2017).
There has been an increase in the incidence of mixed infections, namely among dermatophytes with yeasts or even non-dermatophyte filamentous species (Gawaz and Weisel, 2018). This increase has been accompanied by reports of antifungal resistance and, consequently, an increase in therapeutic failures (Gupta and Foley, 2019). Kravvas et al. (2018) found that the majority of routine dermatological infections were in the form of biofilms. Although this has yet to be proven in the case of dermatophytes, biofilm formation has been attributed to the occurrence of onychomycosis, which is often refractory to antifungal treatment and has a high rate of recurrence (Gupta et al., 2016; Gupta and Foley, 2019). The heterogeneity of species within biofilms makes it difficult to characterize the contribution of each microorganism to the pathogenesis and maintenance of infection and disease (dos Santos et al., 2016; Iñigo and Del Pozo, 2018).
Finally, in multi-species biofilms, it is also essential to consider the intercellular communications that occur via quorum-sensing molecules, which allow one species to identify and react to the presence of another. Therefore, these mediators are also able to coordinate the collective behavior of microorganisms in these communities (Demuyser et al., 2014).
There is currently a lack of research demonstrating the involvement of dermatophytes in multi-species biofilms. Thus, this work aims to investigate the probable dual-species interaction between the biofilms of T. rubrum and C. albicans and T. rubrum and C. parapsilosis. We will take into account variable conditions of formation to evaluate the susceptibility of these dual-species biofilms against two potent last generation antifungals, both of which have a proven efficacy against these microorganisms alone.
In this work, strains of C. albicans SC 5314, C. parapsilosis ATCC 22019, and T. rubrum ATCC MYA-4438 were used. All strains belonged to the collection of the Clinical Mycology Laboratory of the Department of Clinical Analysis, of the School of Pharmaceutical Sciences at São Paulo State University (UNESP). Strains of C. albicans and C. parapsilosis were maintained on Sabouraud Dextrose Agar (SDA) (Kasvi) (40 g/L), comprised of a mixture of peptic digestion of animal tissue (10 g/L) and pancreatic digestion of casein (10 g/l) and agar (15 g/L), at 35°C for 48 h. The dermatophytes were then kept on malt extract agar (Kasvi), comprised of 2% peptone from animal tissue (Sigma-Aldrich), 2% glucose (Synth) and 2% agar (Kasvi) at pH 5.7, incubated at 28°C for 7 days or until sporulation (Costa-Orlandi et al., 2014; Jacob et al., 2015; Vila et al., 2015).
The Candida biofilms were formed according to Pierce et al. (2008), with some modifications. Colonies of the stock cultures of C. albicans SC5314 and C. parapsilosis ATCC 22019 strains were subcultured in Sabouraud Dextrose broth (Kasvi) and incubated at 150 rpm and 35°C for 24 h. A fungal suspension was prepared in RPMI 1640 medium supplemented with L-glutamine without sodium bicarbonate (Gibco) and buffered with MOPS (Sigma-Aldrich) with 2% glucose (Synth) at a final concentration of 1 × 106 cells/mL. Two hundred microliters and one thousand microliters were dispensed in the wells of the 96- and 24-well plates (Kasvi), respectively. The plates were incubated at 37°C for 24 h and 48 h (maturation period) without shaking. A period of 72 h was also added to observe the behavior of biofilms for later standardization in dual-species biofilms, as the maturation period of T. rubrum biofilms corresponds to 72 h (Costa-Orlandi et al., 2014).
T. rubrum biofilms were formed as described by Costa-Orlandi et al. (2014). Briefly, T. rubrum ATCC MYA-4438 was subcultured in Malt Extract agar and incubated at 28°C for 7 days. The colonies were covered with 5 ml sterile of 0.85% saline, and conidia were gently detached with the aid of a sterile swab. The supernatant was placed in 15-mL conical tubes (Corning) and left to stand for 15–20 min to allow the hyphae to settle, such that only microconidia remained in the supernatant. The microconidia were counted in a hemocytometer and the suspension was adjusted using sterile saline (0.85%) to obtain a final concentration of 1 × 106 cells/mL. Subsequently, 200 μL and 1000 μL of the adjusted inoculum were dispensed in the 96- and 24-well plates, respectively. The plates were incubated at 37°C for 4 h without shaking for pre-adhesion of the biofilms. The supernatant was then removed and 200 μL and 1000 μL of RPMI 1640 medium was added to the wells of the 96- and 24 well plates, respectively. The plates were re-incubated at 37°C for up to 72 h. These biofilms were also formed at 96 h and 120 h for subsequent comparison with one of the conditions proposed for dual-species biofilms.
The fungal suspensions of all microorganisms were prepared at a final concentration of 1 × 106 cells/mL when plated. Mixed biofilms were formed under the following conditions: (a) the T. rubrum/C. albicans and T. rubrum/C. parapsilosis suspensions were dispensed in 96- and 24-well plates at the same time in a proportion of 1:1. The plates were then incubated at 37°C for up to 72 h; (b) Candida suspensions were added after the initial adhesion phase of T. rubrum (4 h), followed by incubation at 37°C for up to 72 h; (c) Candida suspensions were added after the maturation period of T. rubrum biofilms (72 h) before incubating the plates at 37°C for an additional 24 h and 48 h. The culture medium was renewed every 24 h.
Biofilms were formed as described above at different time intervals (24 h to 120 h). A solution of XTT (Thermo Fisher Scientific) was prepared at a final concentration of 1 mg/mL in phosphate buffered saline (PBS) and menadione (1 mM) (Sigma-Aldrich). After removing the supernatant and washing the biofilms with PBS, 50 μL of XTT solution + 4 μL of menadione solution were added. The plates were subsequently incubated at 37°C for 3 h and measured using a Biotek EpochTM 2 spectrophotometer at a wavelength of 490 nm (Martinez and Casadevall, 2007; Costa-Orlandi et al., 2014).
The biomass quantification test was performed according to Marcos-Zambrano et al. (2014), with some modifications. Briefly, biofilms were formed in 96-well plates (Kasvi) at different incubation times. The resulting supernatants were removed and the remaining biofilms were washed with PBS. Two hundred microliters of methanol (Vetec) were added to each well for 15 min. The methanol was discarded and the plates were dried at room temperature for 45 min. After drying, 200 μL of 0.1% violet crystal solution (Dinamica) was dispensed into the wells for 20 min. The resulting supernatant was carefully aspirated and the wells were washed with distilled water until the complete removal of excess dye. Finally, 200 μL of 33% acetic acid (Synth) was added and plates were read using a spectrophotometer (Biotek EpochTM 2 Microplate Spectrophotometer) at a wavelength of 570 nm. The resulting absorbance values were directly proportional to the amount of biomass.
The polysaccharide material and extracellular matrices of mono- and multi-species biofilms were stained with 50 μL of 1% safranin solution for 5 min after removing the supernatant and washing the wells with PBS. The plates were washed with 200 μL of sterile 0.85% saline solution until the supernatant became clear. Lastly, the absorbance of the plates was measured using a EpochTM 2 microplate spectrophotometer at 492 nm (Seidler et al., 2008; Costa-Orlandi et al., 2014).
Mono- and dual-species biofilms were formed in 24-well plates and incubated until the maximum time determined in each condition (as described above). The resulting supernatants were removed and the remaining biofilms were fixed with 800 μL of 2.5% glutaraldehyde solution (Sigma-Aldrich) for 1 to 2 h at 4°C. The glutaraldehyde solution was removed from the wells and the biofilms were dehydrated using increasing concentrations of alcohol (50–100%). After drying, the bottom of the plates was cut using a scalpel. The resulting samples were mounted on aluminum cylinders containing carbon strips and evaporated under high vacuum (Denton Vacuum Desk V; Jeol Ltd., Moorestown, NJ, United States) for gold coating. The topographical characteristics of the biofilms were analyzed by electron microscopy (JSM-6610 Series Scanning Electron Microscope; Jeol Ltd., United States) at the School of Dentistry of UNESP at Araraquara, SP, Brazil (Martinez et al., 2010; Costa-Orlandi et al., 2014; Li et al., 2019).
For the C. albicans/T. rubrum and C. parapsilosis/T. rubrum biofilms (1:1), the colony forming units were counted in selective media. Monospecies biofilms were used as a control. After the formation of biofilms in 96-well plates, the culture media were discarded for the removal of poorly adherent cells. Two hundred microliters of sterile PBS were added, and the biofilms were detached from the wells with the aid of sterile tips. The contents of the wells were transferred to 1.5 ml microtubes (Kasvi) and vortexed until the complete dispersion of the biofilm cells. Serial dilutions were performed in PBS and 10 μL aliquots of the diluted content were plated in 60 × 15 mm Petri dishes containing sterile glass beads and two selective media: CHROMagarTM Candida (Difco; BD Biosciences) for the isolation of yeasts and Mycosel agar (Difco; BD Biosciences) for the isolation of T. rubrum. The plates were incubated at 28 and 37°C until the formation of colony forming units (dos Santos et al., 2016).
The activity of terbinafine (Sigma-Aldrich) and efinaconazole (Valeant Pharmaceuticals) was verified against mono- and dual-species biofilms formed using the previously described conditions. Biofilms were formed in 96-well plates. After 72 h, the supernatants were removed and 200 μL of the different concentrations of the terbinafine (0.03 to 32 mg/L) and efinaconazole (0.5 to 256 mg/L) working solutions were prepared in RPMI-1640 medium supplemented with 2% glucose. Two controls were performed: a control for the sterility of the medium (negative control) and a control for growth without treatment (positive control). The plates were incubated without shaking at 37°C for an additional 48 h. Cell viability was evaluated using XTT reduction assay (Pierce et al., 2008). A reduction of at least 50% of metabolic activity was considered an inhibition compared to the metabolic activity of biofilms without treatment.
At least three independent experiments were performed in triplicate for all tests, except for the colony-forming unit count assay. In this test, the assays were conducted with two independent experiments in triplicate. Data were subjected to statistical analysis using analysis of variance (ANOVA) with Bonferroni’s post hoc test in GraphPad Prism 5.0 software. P < 0.05 was considered statistically significant.
The metabolic activities of the Candida monospecies biofilms rose significantly until 48 h (p < 0.0001) (Figure 1). At 72 h, there was a significant decline in the metabolism of both species (p < 0.0001). In T. rubrum biofilms, there was a significant increase in the metabolic activities for up to 72 h (p < 0.0001). After this period, the metabolic activities remained constant until 96 h, followed by a slight decay in 120 h (p < 0.05). However, during the periods of decay, there was still a considerable amount of living cells in the biofilm, indicating the beginning of the inhibition of mature biofilm formation.
Figure 1. Comparison between the metabolic activities of monospecies biofilms of T. rubrum ATCC MYA 4438, C. albicans SC 5314, and C. parapsilosis ATCC 22019 and the dual-species biofilms formed by C. albicans/T. rubrum and C. parapsilosis/T. rubrum under three different conditions: (1) the suspensions of Candida and T. rubrum were placed together at a proportion of 1:1 (A,B); (2) Candida suspensions added after the pre-adhesion of T. rubrum biofilm (C,D); (3) Candida suspensions added after the maturation of the T. rubrum biofilm, followed by an additional incubation of 24 h and 48 h (E,F). Statistical significance: *p < 0.05, **p < 0.01; ***p < 0.0001. Tr, T. rubrum; Ca, C. albicans; Cp, C. parapsilosis.
The metabolic activities of the dual-species biofilms of T. rubrum/C. albicans and T. rubrum/C. parapsilosis were compared to the monospecies biofilms of each strain (Figure 1). Figure 1A shows the dual-species biofilm formed between T. rubrum ATCC MYA-4438 and C. albicans SC 5314. Until 48 h, the dual-species biofilm showed a higher metabolism than the monospecies biofilm of T. rubrum (p < 0.0001), similar to C. albicans biofilm. At 72 h, the metabolic activities of the dual-species biofilms were similar to that of the monospecies biofilms of T. rubrum and lower compared to that of C. albicans (p < 0.01).
A similar behavior was observed between the dual-species biofilms formed by T. rubrum ATCC MYA-4438 and C. parapsilosis ATCC 22019 (Figure 1B). At 24 h, the metabolic activity of the dual-species biofilm was equal to that of C. parapsilosis and higher than T. rubrum (p < 0.0001). At 48 h, the dual-species biofilm showed higher metabolic activities than the T. rubrum biofilm (p < 0.0001) and lower than C. parapsilosis (p < 0.01). At 72 h, the dual-species biofilms showed a superior metabolic activity compared to C. parapsilosis (p < 0.01) and similar levels of metabolic activity as T. rubrum.
The formation of dual-species biofilms in which Candida suspensions were added after the pre-adhesion of T. rubrum are shown in Figures 1C,D. Figure 1C shows the dual-species biofilm formed between T. rubrum ATCC MYA-4438 and C. albicans SC 5314. After 24 h, the mixed biofilm showed a higher metabolic activity than the T. rubrum biofilm (p < 0.0001) and lower than that of C. albicans (p < 0.0001). After 48 h of incubation, the dual-species biofilm showed lower metabolic activity than the C. albicans biofilm (p < 0.01) and higher than the T. rubrum biofilm (p < 0.0001). In 72 h, the dual-species biofilm exhibited a higher metabolic activity than the T. rubrum monospecies biofilm (p < 0.05), but similar to C. albicans.
When the C. parapsilosis suspension was added after T. rubrum pre-adhesion (Figure 1D), the metabolic activity of the dual-species biofilm was lower to that of C. parapsilosis (p < 0.01) and superior to that of T. rubrum (p < 0.0001) until 24 h. At 48 h, the dual-species biofilm showed metabolic activity similar to that of the C. parapsilosis monospecies biofilm and superior to that of T. rubrum (p < 0.0001). At 72 h, the mixed biofilm showed a higher metabolic activity than the C. parapsilosis biofilm (p < 0.0001), but similar to T. rubrum.
For this condition, T. rubrum biofilms were incubated until 72 h, corresponding to the maturation time. During this period, Candida suspensions were also added. The plates were then incubated for an additional 24 h and 48 h. Therefore, the plates incubated for 24 h and 48 h shown in Figures 1E,F correspond to the same periods after the addition of Candida and 96 h and 120 h of T. rubrum biofilm incubation. In relation to the additional incubation time of the T. rubrum biofilms, it is worth noting that the metabolic activities remained constant until 96 h, with a slight decay at 120 h, which can result in an inhibition phase (data not shown). Figure 1E shows that 24 h after the addition of the C. albicans suspensions, there was a significant increase in the metabolic activities of the dual-species biofilms compared to those of T. rubrum monospecies biofilms (p < 0.0001). The same result was observed after 48 h compared to the C. albicans (p < 0.05) and T. rubrum (p < 0.0001) monospecies biofilms. When the C. parapsilosis suspension was added, the mixed biofilms showed higher values of metabolic activities than the monospecies biofilm of C. parapsilosis (p < 0.01) and T. rubrum (p < 0.05) after 24 h (Figure 1F). After 48 h, the metabolic activities of mixed biofilms were superior to both monospecies biofilms of C. parapsilosis and T. rubrum (p < 0.0001).
The monospecies biofilms formed by C. albicans and C. parapsilosis indicated an increase in the development of biomass within the first 48 h (p < 0.0001). After this period (at 72 h), there was a significant decrease (p < 0.0001) in the absorbance measurements. Regarding T. rubrum biofilms, an increase in the biomass was observed until 72 h (p < 0.0001). After this period, there was no statistical significance between the absorbances up to 96 h, corresponding to a stationary phase, followed by a significant decay in 120 h (p < 0.001) (Figure 2). These results are in agreement with the findings of the XTT reduction assay.
Figure 2. Comparison between the biomasses produced by the monospecies biofilms of T. rubrum ATCC MYA 4438, C. albicans SC 5314, and C. parapsilosis ATCC 22019 and by the dual-species biofilms formed by C. albicans/T. rubrum and C. parapsilosis/T. rubrum under three different conditions: (1) suspensions of Candida and T. rubrum together at a proportion of 1:1 (A,B); (2) Candida suspensions added after the pre-adhesion of T. rubrum biofilm (C,D); (3) Candida suspensions added after the maturation of the T. rubrum biofilm, followed by an additional incubation of 24 h and 48 h (E,F). Statistical significance: *p < 0.05; **p < 0.01; ***p < 0.0001. Tr, T. rubrum; Ca, C. albicans; Cp, C. parapsilosis.
The mass of the dual-species C. albicans/T. rubrum and C. parapsilosis/T. rubrum biofilms formed after the addition of fungal suspensions are shown in Figures 2A,B, respectively. In the first interaction (Figure 2A), the dual-species biofilm at 24 h showed a lower biomass than the monospecies biofilms (p < 0.0001). At 48 h, the biomass had significantly increased (p < 0.001). Compared to the monospecies biofilms, its development was similar to that of C. albicans and superior to the T. rubrum biofilm (p < 0.0001). The biomass decayed after 72 h of incubation, similar to the C. albicans biofilm and in contrast to the T. rubrum biofilm, whose biomass increased (p < 0.0001).
In the dual-species biofilms formed between C. parapsilosis and T. rubrum (Figure 2B), the biomass was similar to that of the monospecies C. parapsilosis biofilm and inferior to the T. rubrum biofilms (p < 0.0001) at 24 h. After 48 h of incubation, the biomass absorbance measurements were similar to both types of monospecies biofilms. At 72 h, the biomass of the dual-species biofilm was similar to that of C. parapsilosis and lower than T. rubrum (p < 0.0001).
When C. albicans suspensions were added after T. rubrum pre-adhesion (Figure 2C), the dual-species biofilms showed higher biomasses compared to both types of monospecies biofilms (p < 0.0001) at 24 h of incubation. At 48 h, the biomass of the dual-species biofilm was similar to C. albicans and higher than T. rubrum (p < 0.0001). At 72 h of incubation, the biomass was similar to that of the T. rubrum biofilm, which was superior to that of C. albicans (p < 0.0001).
In the dual-species biofilm formed between T. rubrum and C. parapsilosis (Figure 2D), the biomasses of dual-species biofilms were similar to the C. parapsilosis biofilms and inferior to T. rubrum (p < 0.0001) at 24 h. At 48 h, the biomass of the dual-species biofilms was similar to that of both monospecies biofilms. However, after 72 h, the mass of the biofilm was higher than that of C. parapsilosis (p < 0.0001) and lower than T. rubrum (p < 0.0001). These results indicate that Candida is predominant in this condition, although less than in the previous condition.
The biomass of dual-species biofilms formed after the addition of Candida suspensions for 24 h and 48 h in mature T. rubrum biofilms are shown in Figures 2E,F. After the addition of C. albicans (Figure 2E), the biomasses at 24 h and 48 h was superior to that of the monospecies biofilms of T. rubrum and C. albicans (p < 0.05). After 24 h of incubation with added C. parapsilosis, the biomass was similar to that of the T. rubrum biofilms and superior to the C. parapsilosis biofilms (p < 0.0001). After 48 h of incubation, the mass of the mixed biofilms was higher than the C. parapsilosis (p < 0.0001) and T. rubrum (p < 0.01) monospecies biofilms.
The monospecies biofilms were stained with safranin as a control in order to compare the amount of extracellular matrix and polysaccharide structures produced compared to the dual-species biofilms. In Figure 3, the production of polysaccharide material from C. parapsilosis ATCC 22019 and C. albicans SC 5314 increased until 48 h of incubation (p < 0.0001). After 72 h, decays were observed in both types of yeast biofilm (p < 0.0001). In T. rubrum, the production of polysaccharide material increased until 72 h (p < 0.0001) and remained stable until 96 h, before declining at 120 h (p < 0.0001). These results corroborate with the results obtained in both the XTT and crystal violet assays, suggesting a maturation period for Candida biofilms of 48 h and for T. rubrum biofilms of 72 h.
Figure 3. Comparison between the amounts of extracellular matrix and polysaccharide structures produced by the monospecies biofilms of T. rubrum ATCC MYA 4438, C. albicans SC 5314, and C. parapsilosis ATCC 22019 and by the dual-species biofilms formed by C. albicans/T. rubrum and C. parapsilosis/T. rubrum under three different conditions: (1) suspensions of Candida and T. rubrum placed together at a proportion of 1:1 (A,B); (2) Candida suspensions added after the pre-adhesion of T. rubrum biofilm (C,D); (3) Candida suspensions added after the maturation of the T. rubrum biofilm, followed by an additional incubation of 24 h and 48 h (E,F). Statistical significance: *p < 0.05; **p < 0.01; ***p < 0.0001. Tr, T. rubrum; Ca, C. albicans; Cp, C. parapsilosis.
The dual-species biofilm resulting from the T. rubrum ATCC MYA-4438 and C. albicans SC 5314 suspensions (Figure 3A) showed similar amounts of polysaccharide structures as the C. albicans biofilm (p < 0.05) and superior to the dermatophyte monospecies biofilm (p < 0.0001) after 48 h. At 72 h, the amount of polysaccharide material was higher than that of the C. albicans biofilm and lower than the T. rubrum biofilm (p < 0.0001).
For the dual-species biofilm formed using T. rubrum ATCC MYA-4438 and C. parapsilosis ATCC 22019 (Figure 3B), the amount of polysaccharide material formed after 24 h of incubation was greater than that of the C. parapsilosis monospecies biofilms (p < 0.05) and lower than that of T. rubrum (p < 0.0001). After 48 h, the dual-species biofilm showed quantities of polysaccharide structures similar to those of the yeast biofilm and lower than the dermatophyte biofilm (p < 0.0001). The dual-species biofilm at 72 h showed extracellular matrix development superior to C. parapsilosis (p < 0.0001) and lower than T. rubrum (p < 0.01).
Figure 3C shows that the dual-species biofilm of T. rubrum ATCC MYA-4438 and C. albicans SC 5314 after T. rubrum pre-adhesion established a quantity of polysaccharide material superior to both monospecies biofilms (p < 0.0001) after 72 h of incubation.
When formed with C. parapsilosis (Figure 3D), the dual-species biofilm formed after pre-adhesion of the dermatophyte showed an extracellular matrix development superior to both monospecies biofilms (p < 0.0001). Lastly, after 72 h of incubation, the dual-species biofilm showed polysaccharide structures similar to that of the dermatophyte and superior to C. parapsilosis (p < 0.0001).
When the C. albicans suspension was added to the mature T. rubrum biofilm, the amounts of polysaccharide material of the dual-species biofilm were higher than those of monospecies biofilms of both T. rubrum and C. albicans (Figure 3E) (p < 0.0001) after an additional 24 h and 48 h of incubation. The same behavior was observed in the C. parapsilosis and T. rubrum dual-species biofilms (Figure 3F). These results support the use of dermatophytes as substrates for the development of Candida biofilms, and corroborate our quantification of the metabolic activities by XTT assays and of the biomass by crystal violet staining.
The topographies of the dual-species biofilms of C. albicans/T. rubrum and C. parapsilosis/T. rubrum established under the three experimental conditions were analyzed by SEM with different magnifications (×1000 and ×3000) and compared to monospecies biofilms (controls) (Figures 4–6).
Figure 4. Scanning electron microscopies of C. albicans SC 5314 (a,b) and T. rubrum ATCC MYA 4438 (c,d) monospecies biofilms and dual-species biofilms of C. albicans/T. rubrum formed at a proportion of 1:1 (e,f) or formed with the addition of C. albicans suspensions after the pre-adhesion of T. rubrum biofilm (g,h). White arrows denote the blastoconidia of C. albicans.
Figure 4 shows the topographies of the monospecies biofilms of C. albicans SC 5314 (Figures 4a,b) and T. rubrum ATCC MYA 4438 (Figures 4c,d), as well as their dual-species biofilms (1:1) (Figures 4e,f) and formation after T. rubrum pre-adhesion (Figures 4g,h). The biofilm of C. albicans SC 5314 (Figures 4a,b) was comprised of a dense hyphae network that developed from the yeasts, forming a complex three-dimensional structure. The same structures were observed in the T. rubrum ATCC-MYA 4438 biofilms, but appeared less dense than those in the C. albicans biofilms. Moreover, the polysaccharide material was at times dense and at other times thin, covering and connecting the hyphae network (Figures 4c,d). When the suspensions were placed together to form dual-species biofilms (Figures 4e,f), it was not possible to reliably distinguish between the hyphae formed by C. albicans and those formed by T. rubrum in the images. However, interestingly, a reasonable amount of blastoconidia (represented by the white arrows) was observed, suggesting that the interaction likely affected the mechanisms involved in the filamentation of C. albicans. The presence of blastoconidia indicates the probable predominance of Candida in relation to T. rubrum, more clearly seen in Figure 4f. When the Candida suspensions were added after 4 h of dermatophyte pre-adhesion (Figures 4g,h), similar results to those obtained in the previous condition were observed. However, numerous yeast blastoconidia were also observed, which reinforces our theory of the predominance of Candida in relation to T. rubrum. In addition, our findings indicate that the interaction with T. rubrum may somehow inhibit the filamentation of C. albicans. The greater prevalence of C. albicans compared to T. rubrum can be justified by the fact that yeasts have a faster metabolism than dermatophytes.
The topographies of the C. parapsilosis ATCC 22019 and T. rubrum MYA-4438 dual-species biofilms (1:1) after T. rubrum pre-adhesion are shown in Figure 5, along with their controls. Figures 5a,b show a predominance of blastoconidia and some rare pseudohyphae in the C. parapsilosis monospecies biofilm. The T. rubrum ATCC MYA 4438 biofilms are shown in Figures 5c,d. The interaction between T. rubrum and C. parapsilosis (Figures 5e,f) shows a predominance of yeast blastoconidia, but more dispersed than the control. We also observed poorly developed hyphae in the early stages, which suggests the inhibition of C. albicans filamentation and a low T. rubrum development, indicating an antagonistic interaction between the species studied.
Figure 5. Scanning electron microscopies of monospecies biofilms of C. parapsilosis ATCC 22019 (a,b) and T. rubrum ATCC MYA 4438 (c,d) and dual-species biofilms of C. parapsilosis/T. rubrum formed at a proportion of 1:1 (e,f) or formed with the addition of C. parapsilosis suspensions after the pre-adhesion of T. rubrum biofilm (g,h). White arrows denote the hyphae of T. rubrum.
A lower inhibition in the formation of mature biofilms, and, consequently, a more robust biomass, was observed when C. parapsilosis was added after T. rubrum pre-adhesion (Figures 5g,h). In addition, the T. rubrum hyphae were found to be more developed than in the previous condition (white arrows), although still in small quantities. Therefore, pre-adherence may benefit the development of the hyphae of dermatophytes, the latter of which serves as a substrate for the development of C. parapsilosis biofilms.
Figure 6 shows the topographies of the biofilms formed after the addition of the C. albicans and C. parapsilosis suspensions to the mature T. rubrum biofilms. The monospecies biofilms of T. rubrum, C. albicans, and C. parapsilosis are shown in Figures 6a–f, respectively. In the mixed biofilms of C. albicans/T. rubrum (Figures 6g,h), we observed numerous blastoconidia and a dense biomass, in addition to a greater amount of polysaccharide matrix (red arrows). The visualization of the mixed biofilms of C. parapsilosis and T. rubrum was better (Figures 6i,j). These findings reinforce our theory and corroborate our results for the quantification of biomass by CV and polysaccharide material by safranin staining.
Figure 6. Scanning electron microscopies of T. rubrum ATCC MYA 4438 (a,b), C. albicans SC 5314 (c,d), and C. parapsilosis ATCC 22019 (e,f) monospecies biofilms and dual-species biofilms of C. albicans/T. rubrum (g,h) and C. parapsilosis/T. rubrum (i,j), formed after the maturation of T. rubrum biofilm, followed by an additional 48 h incubation. It is possible to observe oval blastoconidia. Red arrows denote the extracellular matrices, while blue arrows denote hyphae.
For the colony forming unit count (CFU) assay, only one condition for the formation of dual-species biofilms was chosen: C. albicans SC 5314/C. parapsilosis ATCC 22019 and T. rubrum ATCC MYA-4438 (1:1). Petri dishes containing CHROMagarTM Candida (Difco; BD Biosciences) were used to isolate C. albicans from the mixed biofilm, while Mycosel agar (Difco; BD Biosciences) was used to isolate T. rubrum at different temperatures. Monospecies biofilms formed in the same period were used as controls. Figure 7A shows the isolation of C. albicans from the mixed biofilm with a fungal load after 48 h and 72 h of incubation, similar to that of the monospecies biofilm of the same yeast (no statistical significance). On the other hand, it was not possible to isolate T. rubrum from dual-species biofilms, confirming the inhibition of growth by yeast (p < 0.01) and reinforcing the results of the quantitative colorimetric tests, especially of CV, and SEM.
Figure 7. Log of colony forming units per milliliter [CFU/mL] (Log) isolated from mono- and dual-species C. albicans SC 5314 and T. rubrum ATCC MYA 4438 biofilms (1:1) (A) and isolated from mono and dual-species biofilms of C. parapsilosis ATCC 22019 and T. rubrum ATCC MYA 4438 (B) formed under the same conditions after 48 h and 72 h of incubation. Statistical significance: *p < 0.05, **p < 0.01, and ***p < 0.0001.
In contrast, T. rubrum was isolated from dual-species biofilms formed with C. parapsilosis (Figure 7B). The predominance of yeast in comparison with the dermatophyte was still evident, particularly after 72 h of incubation (p < 0.0001), confirming the antagonistic interaction. In terms of the controls, the fungal loads of C. parapsilosis and T. rubrum were found to be similar to those of dual-species biofilms after 48 h of incubation (no statistical significance). At 72 h, the fungal load of T. rubrum isolated from the dual-species biofilm was significantly lower than that of the monospecies biofilm (p < 0.01), whereas that of C. parapsilosis was similar. Therefore, we concluded that the interaction between C. parapsilosis and T. rubrum also culminated in the inhibition of T. rubrum growth.
To determine the minimum sessile inhibitory concentration (SMIC), a reduction of at least 50% of cell viability was considered in relation to positive controls. As shown in Table 1, both monospecies and dual-species biofilms were resistant to all tested concentrations of efinaconazole and terbinafine.
Table 1. Values of the minimum inhibitory concentration sessile (SMIC) expressed in mg/L for efinaconazole and terbinafine tested against the monospecies biofilms of T. rubrum, C. albicans, C. parapsilosis, and dual-species biofilms formed by T. rubrum/C. albicans and T. rubrum/C. parapsilosis.
In mixed biofilms, interactions between different species can be synergistic, neutral, or antagonistic (dos Santos et al., 2016). Recent studies have shown that Candida yeast rarely exists in isolated form (Jack et al., 2015). Synergism is beneficial to all microorganisms involved, whereas antagonistic relationships can cause the death, damage, or inhibition of one microorganism by a product produced by the other. Finally, neutral relationships are indifferent for both species involved in the formation of the biofilm (Thein et al., 2009; Cabezón et al., 2016; Costa-Orlandi et al., 2017).
The development of multi-species biofilms can also be influenced by the sharing of nutrients available in the medium between different species. Therefore, the polymicrobial interactions in a biofilm are significantly modulated by these environmental elements (Thein et al., 2009; Cabezón et al., 2016). On the other hand, it is also essential to consider intercellular communications that occur through quorum-sensing molecules, which allow to coordinate the collective behavior of microorganisms in these communities (Demuyser et al., 2014).
Our results showed an in vitro antagonistic interaction between C. albicans and C. parapsilosis and the dermatophyte T. rubrum in RPMI medium. Although it is not known which of the three conditions tested actually occurred in the microbiome and in the pathogenesis of dermatomycoses, this antagonism may be caused by products secreted into the medium by both fungi, or even due to a lack of nutrients. To prevent the latter, the culture medium was changed every 24 h. The predominance of Candida was possibly due to its metabolism being more accelerated than that of T. rubrum, mainly in the first condition, in which the two microorganisms were added together. This condition can generate competition for adhesion sites. In the other conditions, the absorbance measurements corresponding to metabolic activities, biomasses, and polysaccharide materials were similar or sometimes superior to T. rubrum monospecies biofilms, which suggests that pre-adhesion or mature biofilms of T. rubrum may served as a substrate for the development of Candida biofilms. On the other hand, the Candida monospecies biofilms demonstrated differences in the quantity of the same parameters compared to dual-species biofilms. These assumptions were confirmed in the topographies of biofilms observed in SEM, which further evidenced the predominance of yeasts, the inhibition of the development of T. rubrum biofilms, and the inhibition of C. albicans filamentation, more evident in the conditions where there was a greater amount of the dermatophyte.
Regarding the quantitative tests of the monospecies biofilms, the results corroborated the literature, indicating that the ideal time for the maturation of the biofilms of Candida spp. (24 h or 48 h) depended on the species (Pierce et al., 2008). In comparison, the ideal maturation of T. rubrum is 72 h (Costa-Orlandi et al., 2014).
According to dos Santos et al. (2016), it is not possible to differentiate between the cellular metabolic activity of a single species in mixed biofilms based only on the XTT reduction assay. Therefore, it is necessary to make comparisons with monospecies biofilms. The same authors stated that the metabolic behavior of biofilms can vary depending on the microorganisms present in the microbial interaction. The presence of C. krusei in the mixed biofilm with C. albicans stimulated a reduction in cell viability compared to the viability observed in the monospecies biofilm formed by C. albicans, suggesting a possible inhibition of C. albicans.
Bandara et al. (2010b) used colorimetric assays with XTT to demonstrate that the presence of Escherichia coli in dual-species biofilms, after 48 h, decreased the cell viability of C. parapsilosis, without significant changes when compared to C. albicans.
The metabolic activities of biofilms were quantified through a colorimetric assay with a solution of XTT and menadione acting as an electron coupling agent. XTT is a yellow tetrazolium salt, which converts to orange formazan salts in the presence of metabolic activity. Many authors assume that this assay is an efficient way to indirectly quantify biofilms since the observed colorimetric change is proportional to the number of living cells (Costa-Orlandi et al., 2014; Marcos-Zambrano et al., 2014; Rosato et al., 2016). However, there are also studies indicating that, for C. albicans and C. parapsilosis, the results obtained with the XTT assay are not directly related to the number of live microorganisms (Kuhn et al., 2003). It is also important to consider that biofilms are surrounded by an extracellular matrix, which can impair the metabolic activity of the microorganisms if it limits the access of cells to nutrients and oxygen. Thus, other methods will be needed in addition to the XTT tests for the characterization of biofilms (Henriques et al., 2006; Costa-Orlandi et al., 2017).
Regarding the quantification of biomass by the crystal violet assay, previous studies have shown that the presence of C. albicans biofilms stimulated an increase in the biomass of heterotypic biofilms formed between C. albicans, C. glabrata, and C. tropicalis, indicating that the first species can provide a substrate for non-C. albicans species to adhere to acrylic materials (Pathak et al., 2012). The co-cultivation of Streptococcus mutans and C. albicans in dual-species biofilms resulted in better biomass growth for each species. This may have occurred due to metabolic interactions that provided additional nutrients for these microorganisms (Sztajer et al., 2014). In contrast, recent studies have shown an inhibitory effect on metabolic activity and biomass formation by C. tropicalis, C. krusei, and C. parapsilosis when co-incubated with probiotic bacteria Lactobacillus gasseri and Lactobacillus rhamnosus (Tan et al., 2018).
The crystal violet assay is based on the ability of this substance to penetrate the fungal cell wall and remain trapped in the cytoplasm. This quantification technique may have its reproducibility affected by the different growth conditions of the microbial biofilm and the different solvent concentrations used in the assay, among others. In addition, crystal violet is only able to quantify the biomass without distinguishing between dead and living cells, so this analysis must be complementary to other tests (Stepanovic et al., 2007; Pantanella et al., 2013).
In this study, the colony-forming unit counting test was used to complement the previous colorimetric assays, as well as the topographic observation by SEM, since these do not allow to specify the real constitution of the biofilm from a quantitative point of view. This assay confirmed the predominance of C. albicans and C. parapsilosis over T. rubrum in the only tested condition (1:1). Although laborious, many authors use this technique to isolate microorganisms within mixed biofilms and, unlike the XTT reduction assay, this technique is not influenced by the cellular metabolic state, directly enumerating the present cells (Jin et al., 2004; Jabra-Rizk et al., 2006; Bandara et al., 2010a; Fox et al., 2014; dos Santos et al., 2016).
Finally, regarding susceptibility tests, the findings in the present study corroborate the increased resistance that biofilms have against antimicrobial agents. In the dual-species interaction, although there is an antagonistic relationship between the biofilms of Candida and the dermatophyte, there is a prevalence of yeasts, making these dual-species biofilms resistant to all tested drug concentrations. The results obtained with the susceptibility tests of T. rubrum biofilms against terbinafine and efinaconazole corroborate the results obtained by our group and which are in preparation for publication. Previous studies have demonstrated the ineffectiveness of terbinafine against C. albicans biofilms, which agrees with the results presented in this work. However, the same authors demonstrated that terbinafine exerts activity against C. parapsilosis biofilms, contrary to what was found in the present study (Lam et al., 2016).
This study provides unprecedented data related to the interaction between sessile communities formed by the three microorganisms that are most frequently isolated from dermatomycoses, one of the most prevalent mycoses worldwide. The findings show that C. albicans/T rubrum and C. parapsilosis/T. rubrum can grow and form biofilms together, albeit with a degree of antagonism on both sides. If, on the one hand, the growth of the dermatophyte is somewhat inhibited by the presence of yeasts, the presence of the filamentous fungi seems to proportionally inhibit one of the main virulence factors of C. albicans, namely filamentation, thereby decreasing the growth of C. parapsilosis. Even under condition in which the dermatophyte seems to serve as a substrate for the development of Candida biofilms, there is a certain antagonism for the same reasons as mentioned above. Therefore, this work opens several doors to the study of the interaction between T. rubrum biofilms and other microorganisms. Further studies are currently being conducted to identify potential substance(s) secreted in the supernatants of these mixed biofilms for use as broad-spectrum compounds. Additional studies should also be conducted using other species with different biofilm formation abilities in order to verify our findings.
All datasets generated for this study are included in the article/supplementary material.
CC-O, LMG, and NB drafted the manuscript. LMG, CC-O, NB, CV, and LNG performed the experiments. CC-O and MM-G designed and supervised the project. All authors participated in data analysis and critical revision of the manuscript and approved the final version.
This work was supported by Fundação de Amparo à Pesquisa do Estado de São Paulo-FAPESP [2017/18388-6 (CC-O), 2018/02785-9 (MM-G), and 2019/22188-8 (NB)], Coordenação de Aperfeiçoamento de Pessoal de Nível Superior (CAPES) (Finance Code 001), Programa de Apoio ao Desenvolvimento Científico (PADC) da Faculdade de Ciências Farmacêuticas da UNESP, Conselho Nacional de Desenvolvimento Científico e Tecnológico [148425/2018-6 (LMG), 142049/2019-0 (NB), 134559/2018-5 (CV), and 142048/2019-4 (LNG)], and Instituto de Bolsas de Estudo (IBE 150/2017).
The authors declare that the research was conducted in the absence of any commercial or financial relationships that could be construed as a potential conflict of interest.
The handling editor declared a shared affiliation with the authors at the time of review.
We thank Dr. Joshua Nosanchuk for kindly providing T. rubrum ATCC MYA-4438 strain, Dr. Regina Pires for providing C. albicans SC5314 strain, and Dr. Nosanchuk and Adam Friedman for providing efinaconazole.
Aquino, V. R., Constante, C. C., and Bakos, L. (2007). Frequency of dermatophytosis in mycological examinations at a general hospital in Porto Alegre. Brazil. An. Bras. Dermatol. 82, 239–244.
Bandara, H. M., Lam, O. L., Watt, R. M., Jin, L. J., and Samaranayake, L. P. (2010a). Bacterial lipopolysaccharides variably modulate in vitro biofilm formation of Candida species. J. Med. Microbiol. 59, 1225–1234. doi: 10.1099/jmm.0.021832-0
Bandara, H. M., Yau, J. Y., Watt, R. M., Jin, L. J., and Samaranayake, L. P. (2010b). Pseudomonas aeruginosa inhibits in-vitro Candida biofilm development. BMC Microbiol. 10:125. doi: 10.1186/1471-2180-10-125
Cabezón, V., Vialás, V., Gil-Bona, A., Reales-Calderón, J. A., Martínez-Gomariz, M., Gutiérrez-Blázquez, D., et al. (2016). Apoptosis of Candida albicans during the interaction with murine macrophages: proteomics and cell-death marker monitoring. J. Proteome Res. 15, 1418–1434. doi: 10.1021/acs.jproteome.5b00913
Celestrino, G. A., Reis, A. P. C., Criado, P. R., Benard, G., and Sousa, M. G. T. (2019). Trichophyton rubrum elicits phagocytic and pro-inflammatory responses in human monocytes through toll-like receptor 2. Front. Microbiol. 10:2589. doi: 10.3389/fmicb.2019.02589
Chadeganipour, M., Nilipour, S., and Ahmadi, G. (2010). Study of onychomycosis in Isfahan. Iran. Mycoses 53, 153–157. doi: 10.1111/j.1439-0507.2008.01679
Chandra, J., and Mukherjee, P. K. (2015). Candida biofilms: development, architecture, and resistance. Microbiol. Spectr. 3, 1–14. doi: 10.1128/microbiolspec
Costa-Orlandi, B. C., Sardi, C. J., Pitangui, S. N., de Oliveira, C. H., Scorzoni, L., Galeane, C. M., et al. (2017). Fungal biofilms and polymicrobial diseases. J. Fungi 3:22. doi: 10.3390/jof3020022
Costa-Orlandi, C. B., Magalhães, G. M., Oliveira, M. B., Taylor, E. L., Marques, C. R., and de Resende-Stoianoff, M. A. (2012). Prevalence of dermatomycosis in a Brazilian tertiary care hospital. Mycopathologia 174, 489–497. doi: 10.1007/s11046-012-9576-1
Costa-Orlandi, C. B., Sardi, J. C., Santos, C. T., Fusco-Almeida, A. M., and Mendes-Giannini, M. J. (2014). In vitro characterization of Trichophyton rubrum and T. mentagrophytes biofilms. Biofouling 30, 719–727.
Demuyser, L., Jabra-Rizk, M. A., and Van Dijck, P. (2014). Microbial cell surface proteins and secreted metabolites involved in multispecies biofilms. Pathog. Dis. 70, 219–230. doi: 10.1111/2049-632x.12123
dos Santos, J. D., Piva, E., Vilela, S. F. G., Jorge, A. O. C., and Junqueira, J. C. (2016). Mixed biofilms formed by C. albicans and non-albicans species: a study of microbial interactions. Braz. Oral Res. 30, S1806–S832.
Fox, E. P., Cowley, E. S., Nobile, C. J., Hartooni, N., Newman, D. K., and Johnson, A. D. (2014). Anaerobic bacteria grow within Candida albicans biofilms and induce biofilm formation in suspension cultures. Curr. Biol. 24, 2411–2416. doi: 10.1016/j.cub.2014.08.057
Gawaz, A., and Weisel, G. (2018). Mixed infections are a critical factor in the treatment of superficial mycoses. Mycoses 61, 731–735. doi: 10.1111/myc.1279
Ghannoum, M. A., Hajjeh, R. A., Scher, R., Konnikov, N., Gupta, A. K., Summerbell, R., et al. (2000). A large-scale North American study of fungal isolates from nails: the frequency of onychomycosis, fungal distribution, and antifungal susceptibility patterns. J. Am. Acad. Dermatol. 43, 641–648. doi: 10.1067/mjd.2000.107754
Ghannoum, M. A., Mukherjee, P. K., Warshaw, E. M., Evans, S., Korman, N. J., and Tavakkol, A. (2013). Molecular analysis of dermatophytes suggests spread of infection among household members. Cutis 91, 237–245.
Gupta, A. K., Daigle, D., and Carviel, J. L. (2016). The role of biofilms in onychomycosis. J. Am. Acad. Dermatol. 74, 1241–1246. doi: 10.1016/j.jaad.2016.01.008
Gupta, A. K., and Foley, K. A. (2019). Evidence for biofilms in onychomycosis. G. Ital. Dermatol. Venereol. 154, 50–55.
Havlickova, B., Czaika, V. A., and Friedrich, M. (2008). Epidemiological trends in skin mycoses worldwide. Mycoses 51(Suppl. 4), doi: 10.1111/j.1439-0507.2007.01380.x
Henriques, M., Azeredo, J., and Oliveira, R. (2006). Candida albicans and Candida dubliniensis: comparison of biofilm formation in terms of biomass and activity. Br. J. Biomed. Sci. 63, 5–11. doi: 10.1080/09674845.2006.11732712
Iñigo, M., and Del Pozo, J. L. (2018). Fungal biofilms: from bench to bedside. Revist. Esp. Quimioter. 31(Suppl. 1), 35–38.
Jabra-Rizk, M. A., Meiller, T. F., James, C. E., and Shirtliff, M. E. (2006). Effect of farnesol on Staphylococcus aureus biofilm formation and antimicrobial susceptibility. Antimicrob. Agents Chemother. 50, 1463–1469. doi: 10.1128/aac.50.4.1463-1469.2006
Jack, A. A., Daniels, D. E., Jepson, M. A., Vickerman, M. M., Lamont, R. J., Jenkinson, H. F., et al. (2015). Streptococcus gordonii comCDE (competence) operon modulates biofilm formation with Candida albicans. Microbiology 161, 411–421. doi: 10.1099/mic.0.000010
Jacob, T. R., Peres, N. T., Martins, M. P., Lang, E. A., Sanches, P. R., Rossi, A., et al. (2015). Heat shock protein 90 (Hsp90) as a molecular target for the development of novel drugs against the dermatophyte Trichophyton rubrum. Front. Microbiol. 6:1241. doi: 10.3389/fmicb.2015.01241
Jin, Y., Samaranayake, L. P., Samaranayake, Y., and Yip, H. K. (2004). Biofilm formation of Candida albicans is variably affected by saliva and dietary sugars. Arch. Oral Biol. 49, 789–798. doi: 10.1016/j.archoralbio.2004.04.011
Kravvas, G., Veitch, D., and Al-Niaimi, F. (2018). The increasing relevance of biofilms in common dermatological conditions. J. Dermatol. Treat. 29, 202–207. doi: 10.1080/09546634.2017.1360989
Kuhn, D. M., Balkis, M., Chandra, J., Mukherjee, P. K., and Ghannoum, M. A. (2003). Uses and limitations of the XTT assay in studies of Candida growth and metabolism. J. Clin.Microbiol. 41, 506–508. doi: 10.1128/jcm.41.1.506-508.2003
Lam, P., Kok, S. H., Lee, K. K., Lam, K. H., Hau, D. K., Wong, W. Y., et al. (2016). Sensitization of Candida albicans to terbinafine by berberine and berberrubine. Biomed. Rep. 4, 449–452. doi: 10.3892/br.2016.608
Li, X., Yin, L., Ramage, G., Li, B., Tao, Y., Zhi, Q., et al. (2019). Assessing the impact of curcumin on dual-species biofilms formed by Streptococcus mutans and Candida albicans. Microbiologyopen. 8:e937.
Marcos-Zambrano, L. J., Escribano, P., Bouza, E., and Guinea, J. (2014). Production of biofilm by Candida and non-Candida spp. isolates causing fungemia: comparison of biomass production and metabolic activity and development of cut-off points. Int. J. Med.Microbiol. 304, 1192–1198. doi: 10.1016/j.ijmm.2014.08.012
Martinez, L. R., and Casadevall, A. (2007). Cryptococcus neoformans biofilm formation depends on surface support and carbon source and reduces fungal cell susceptibility to heat, cold, and UV light. Appl. Environ. Microbiol. 73, 4592–4601. doi: 10.1128/aem.02506-06
Martinez, L. R., Mihu, M. R., Tar, M., Cordero, R. J., Han, G., Friedman, A. J., et al. (2010). Demonstration of antibiofilm and antifungal efficacy of chitosan against candidal biofilms, using an in vivo central venous catheter model. J. Infect. Dis. 201, 1436–1440. doi: 10.1086/651558
Pantanella, F., Valenti, P., Natalizi, T., Passeri, D., and Berlutti, F. (2013). Analytical techniques to study microbial biofilm on abiotic surfaces: pros and cons of the main techniques currently in use. Ann. Ig. 25, 31–42.
Pathak, A. K., Sharma, S., and Shrivastva, P. (2012). Multi-species biofilm of Candida albicans and non-Candida albicans Candida species on acrylic substrate. J. Appl. Oral Sci. 20, 70–75. doi: 10.1590/s1678-77572012000100013
Pierce, C. G., Uppuluri, P., Tristan, A. R., Wormley, F. L., Mowat, E., Ramage, G., et al. (2008). A simple and reproducible 96-well plate-based method for the formation of fungal biofilms and its application to antifungal susceptibility testing. Nat. Protoc. 3, 1494–1500. doi: 10.1038/nprot.2008.141
Pires, R. H., Lucarini, R., and Mendes-Giannini, M. J. (2012). Effect of usnic acid on Candida orthopsilosis and C. parapsilosis. Antimicrob. Agents Chemother. 56, 595–597. doi: 10.1128/aac.05348-11
Pires, R. H., Santos, J. M., Zaia, J. E., Martins, C. H., and Mendes-Giannini, M. J. (2011). Candida parapsilosis complex water isolates from a haemodialysis unit: biofilm production and in vitro evaluation of the use of clinical antifungals. Mem. Inst. Oswaldo Cruz. 106, 646–654. doi: 10.1590/s0074-02762011000600002
Pontes, Z. B., Lima Ede, O., Oliveira, N. M., Dos Santos, J. P., Ramos, A. L., and Carvalho, M. F. (2002). Onychomycosis in Joao Pessoa City. Brazil. Rev. Argent. Microbiol. 34, 95–99.
Ramage, G., Mowat, E., Jones, B., Williams, C., and Lopez-Ribot, J. (2009). Ourcurrent understanding of fungal biofilms. Crit. Rev. Microbiol. 35, 340–355. doi: 10.3109/10408410903241436
Ramage, G., Rajendran, R., Sherry, L., and Williams, C. (2012). Fungal biofilm resistance. Int. J. Microbiol. 2012, 528521.
Rosato, A., Catalano, A., Carocci, A., Carrieri, A., Carone, A., Caggiano, G., et al. (2016). In vitro interactions between anidulafungin and nonsteroidal anti-inflammatory drugs on biofilms of Candida spp. Bioorg. Med. Chem. 24, 1002–1005. doi: 10.1016/j.bmc.2016.01.026
Rotta, I., Sanchez, A., Goncalves, P. R., Otuki, M. F., and Correr, C. J. (2012). Efficacy and safety of topical antifungals in the treatment of dermatomycosis: a systematic review. Br. J. Dermatol. 166, 927–933. doi: 10.1111/j.1365-2133.2012.10815.x
Sardi, J. C., Scorzoni, L., Bernardi, T., Fusco-Almeida, A. M., and Mendes Giannini, M. J. (2013). Candida species: current epidemiology, pathogenicity, biofilm formation, natural antifungal products and new therapeutic options. J. Med. Microbiol. 62, 10–24. doi: 10.1099/jmm.0.045054-0
Seidler, M. J., Salvenmoser, S., and Muller, F. M. (2008). Aspergillus fumigatus forms biofilms with reduced antifungal drug susceptibility on bronchial epithelial cells. Antimicrob. Agents Chemother. 52, 4130–4136. doi: 10.1128/aac.00234-08
Stepanovic, S., Vukovic, D., Hola, V., Di Bonaventura, G., Djukic, S., Cirkovic, I., et al. (2007). Quantification of biofilm in microtiter plates: overview of testing conditions and practical recommendations for assessment of biofilm production by Staphylococci. APMIS 115, 891–899. doi: 10.1111/j.1600-0463
Sztajer, H., Szafranski, S. P., Tomasch, J., Reck, M., Nimtz, M., Rohde, M., et al. (2014). Cross-feeding and interkingdom communication in dual-species biofilms of Streptococcus mutans and Candida albicans. ISME J. 8, 2256–2271. doi: 10.1038/ismej.2014.73
Tan, Y., Leonhard, M., Moser, D., Ma, S., and Schneider-Stickler, B. (2018). Inhibitory effect of probiotic lactobacilli supernatants on single and mixed nonalbicans Candida species biofilm. Arch. Oral Biol. 85, 40–45. doi: 10.1016/j.archoralbio.2017.10.002
Thein, Z. M., Seneviratne, C. J., Samaranayake, Y. H., and Samaranayake, L. P. (2009). Community lifestyle of Candida in mixed biofilms: a mini review. Mycoses 52, 467–475. doi: 10.1111/j.1439-0507.2009.01719.x
Trofa, D., Gacser, A., and Nosanchuk, J. D. (2008). Candida parapsilosis, an emerging fungal pathogen. Clin. Microbiol. Rev. 21, 606–625. doi: 10.1128/cmr.00013-08
Vila, T. V., Rozental, S., and de Sa Guimaraes, C. M. (2015). A new model of in vitro fungal biofilms formed on human nail fragments allows reliable testing of laser and light therapies against onychomycosis. Lasers Med. Sci. 30, 1031–1039. doi: 10.1007/s10103-014-1689-y
Wongsuk, T., Pumeesat, P., and Luplertlop, N. (2016). Fungal quorum sensing molecules: role in fungal morphogenesis and pathogenicity. J. Basic Microbiol. 56, 440–447. doi: 10.1002/jobm.201500759
Keywords: polymicrobial biofilms, Candida albicans, C. parapsilosis, dermatophytes, antagonistic interaction, dermatomycosis, Trichophyton rubrum
Citation: Garcia LM, Costa-Orlandi CB, Bila NM, Vaso CO, Gonçalves LNC, Fusco-Almeida AM and Mendes-Giannini MJS (2020) A Two-Way Road: Antagonistic Interaction Between Dual-Species Biofilms Formed by Candida albicans/Candida parapsilosis and Trichophyton rubrum. Front. Microbiol. 11:1980. doi: 10.3389/fmicb.2020.01980
Received: 10 February 2020; Accepted: 27 July 2020;
Published: 04 September 2020.
Edited by:
Juliana Campos Junqueira, São Paulo State University, BrazilReviewed by:
Luis Antonio Pérez-García, Universidad Autónoma de San Luis Potosí, MexicoCopyright © 2020 Garcia, Costa-Orlandi, Bila, Vaso, Gonçalves, Fusco-Almeida and Mendes-Giannini. This is an open-access article distributed under the terms of the Creative Commons Attribution License (CC BY). The use, distribution or reproduction in other forums is permitted, provided the original author(s) and the copyright owner(s) are credited and that the original publication in this journal is cited, in accordance with accepted academic practice. No use, distribution or reproduction is permitted which does not comply with these terms.
*Correspondence: Maria José Soares Mendes-Giannini, bWFyaWEuZ2lhbm5pbmlAdW5lc3AuYnI=; Z2lhbm5pbmltakBnbWFpbC5jb20=
†These authors have contributed equally to this work
Disclaimer: All claims expressed in this article are solely those of the authors and do not necessarily represent those of their affiliated organizations, or those of the publisher, the editors and the reviewers. Any product that may be evaluated in this article or claim that may be made by its manufacturer is not guaranteed or endorsed by the publisher.
Research integrity at Frontiers
Learn more about the work of our research integrity team to safeguard the quality of each article we publish.