- 1Tobacco Research Institute, Chinese Academy of Agricultural Sciences, Qingdao, China
- 2Plant Protection Station of Shandong Province, Jinan, China
- 3Key Laboratory of Tropical Medicinal Resource Chemistry of Ministry of Education, Hainan Normal University, Haikou, China
A new polyketide derivative, nafuredin C (1), a novel heterocyclic dipeptide, trichodermamide G (3), together with four known biogenetically related compounds nafuredin A (2), trichodermamide A (4), aspergillazin A (5), and peniisocoumarin H (6), were isolated from the mangrove-derived fungus Trichoderma harzianum D13. Their structures, including their absolute configurations, were determined by spectroscopic analysis and time-dependent density functional theory-electronic circular dichroism (ECD) calculations. Trichodermamide G was found to be a novel epithiodiketopiperazine derivative with an unprecedented cyclic system containing a sulfur bridge, and nafuredin C represented the third nafuredin derivative of these homologous compounds. The new compound nafuredin C exhibited obvious antifungal activity against Magnaporthe oryzae with a minimum inhibitory concentration (MIC) of 8.63 μM, which is on the same order of magnitude as the positive control carbendazim (MIC = 3.27 μM).
Introduction
Rice blast disease is the most serious disease affecting cultivated rice, a staple food for nearly 50% of the world’s population, and seriously threatens global food security. Each year, rice blast disease is responsible for the loss of 10–30% of the rice harvest, which is enough to feed more than 60 million people (Martin-Urdiroz et al., 2016; Zhou, 2016). Rice blast is caused by Magnaporthe oryzae, an ascomycete fungus that causes diseases in a wide range of economically important crops including barley, oats, rye grass, and millets. It was first reported in Brazil in 1985 and then rapidly spread to other South American countries, causing significant yield losses. The disease captured public attention in 2016 when it appeared in Bangladesh, resulting in thousands of rice fields being burned to prevent further spread of the disease (Ma and Xu, 2019). In China, rice blast occurs everywhere rice is cultivated, particularly in hilly areas. In epidemic years of the disease, yield loss can reach 40–50% and in severe cases can result in complete losses in major rice production areas (Zhao X. X. et al., 2019). Therefore, searching for new biological pesticides to control rice blast is necessary for sustainable development of the rice industry and food security worldwide.
Beneficial microbes can function as biocontrol organisms that help plants defend themselves from attack by pathogens. Fungi in the genus Trichoderma are excellent mycoparasites of plant pathogens and directly protect plants against them. In addition, Trichoderma spp. can enhance the plant defense system, which enables the plant to respond in a fast and strong manner to pathogen attack (Guzman-Guzman et al., 2019). These fungi are also prolific producers of numerous secondary metabolites with pharmaceutical and biotechnological importance, including polyketides, non-ribosomal peptides, siderophores, peptaibols, and volatile and non-volatile terpenes (Contreras-Cornejo et al., 2016). Several studies have focused on the application of Trichoderma spp. for controlling rice blast disease with apparent effects; however, marine-derived Trichoderma spp. have not been evaluated for use in plant protection, and few studies have reported their antifungal effects against M. oryzae.
During our ongoing search for new anti-phytopathogenic fungal secondary metabolites from marine-derived fungi in the Yellow Sea and South China Sea (Huang et al., 2018; Zhao et al., 2018; Zhao D. L. et al., 2019), we found that the extract of mangrove-derived fungus Trichoderma harzianum D13, collected from Hainan province, China, displayed a strong activity against fungal plant pathogens. Further chemical investigation of the ethyl acetate (EtOAc) extracts led to the isolation of six compounds (Figure 1), including two nafuredin derivatives, nafuredins C and A (1 and 2); three epithiodiketopiperazine derivatives, trichodermamide G (3), trichodermamide A (4), and aspergillazin A (5); and one isocoumarin, peniisocoumarin H (6). Among them, nafuredin C and trichodermamide G are new compounds. Herein, we report the isolation, structural elucidation, and antifungal activities of these compounds.
Materials and Methods
General Experimental Procedures
Specific rotations were measured on a JASCO P-1020 digital polarimeter (Tokyo, Japan). ECD spectra were obtained on a JASCO J-815-150S spectropolarimeter. UV spectra were recorded on a Techcomp UV2310II spectrophotometer (Shanghai, China). The 1D (1H, 13C, and NOE) and 2D NMR spectra [HMQC, correlation spectroscopy (COSY), heteronuclear multiple bond correlation (HMBC), and nuclear Overhauser effect spectroscopy (NOESY)] were acquired on a DD2 500 MHz NMR spectrometer (Agilent Technologies, Santa Clara, CA, United States). A Micromass Q-TOF spectrometer (Waters, Milford, MA, United States) and Thermo Scientific LTQ Orbitrap XL spectrometer (Waltham, MA, United States) was used to measure the electrospray ionization mass spectrometry (ESIMS) and HRESIMS. High-performance liquid chromatography (HPLC) was performed using a Waters e2695 separation module with a Waters 2998 detector and Waters X-Bridge C18 (5 μm, 10 × 250 mm) column. Column chromatography (CC) was performed over silica gel (200–300 mesh; Qing Dao Hai Yang Chemical Group Co., Qingdao, China), octadecylsilyl silica (ODS) gel (RP18, 40–63 μm; Merck, Billerica, MA, United States), and Sephadex LH-20 (GE Healthcare, Little Chalfont, United Kingdom). Precoated silica gel plates (G60, F-254; Yan Tai Zi Fu Chemical Group Co., Yantai, China) were used for analytical thin-layer chromatography. Analytical and HPLC-grade solvents were used for isolation.
Fungal Material
The fungus was isolated from the internal tissues of the root of mangrove plant Excoecaria agallocha Linn. collected from Hainan province, China, in 2016, and was identified as T. harzianum (GenBank accession number MG827165) by sequence analysis of the internal transcribed spacer region of the rDNA. A voucher strain of this fungus was deposited in the Marine Agriculture Research Center, Tobacco Research Institute of Chinese Academy of Agricultural Sciences, Qingdao, China.
Extraction and Isolation
The fungus T. harzianum D13 was cultured on plates of potato dextrose agar medium at 28°C for 3 days. Plugs of agar supporting mycelium growth were cut and transferred aseptically into 200 × 1000-mL Erlenmeyer flasks each containing 400 mL of potato dextrose water liquid medium. The flasks were incubated at 28°C under static conditions for 30 days. The cultures (80 L) were filtered through gauze to separate the mycelial layer from the aqueous layer. The filtrate was then extracted twice with EtOAc, whereas the mycelium were mechanically broken and ultrasonically disrupted for 10 min and then extracted twice with CH2Cl2:MeOH (1:1, v/v). After removing CH2Cl2 and MeOH by evaporation under vacuum, the remaining aqueous solution was extracted three times with EtOAc. The combined EtOAc extracts were concentrated under reduced pressure to yield the total EtOAc extract (13.8 g). This extract was subjected to vacuum liquid chromatography on silica gel for elution with a gradient of EtOAc in petroleum ether (EtOAc/petroleum ether, 0–100%), and then with MeOH in EtOAc ranging from 10 to 50% to give eight fractions (Fractions 1–8). Fraction 3 was initially fractionated using an ODS gel column with a step gradient elution of MeOH–H2O (60–90%) to afford Fr. 3-1 and Fr. 3-2. Fraction 3-1 was subsequently applied to a Sephadex LH-20 CC (CH2Cl2/MeOH, v/v, 1/1), and finally purified by reversed-phase-HPLC eluting with 80% MeOH–H2O to obtain compound 2 (10.6 mg). Fraction 4 was separated on an ODS column eluting with 50–90% MeOH–H2O to obtain Fr. 4-1 and Fr. 4-2. Fraction 4-1 was then afforded to silica gel CC (EtOAc/petroleum ether, 5% to 35%), followed by purification by HPLC (MeOH/H2O, 80/20) to yield 1 (10.0 mg). Fraction 6 was subjected to ODS CC using a gradient elution of 30–90% MeOH–H2O, followed by separation on Sephadex LH-20 (CH2Cl2–MeOH, v/v, 1/1) to afford subfractions Fr. 6-1–6-3. Fraction 6-1 was subjected to silica gel CC (MeOH/CH2Cl2, v/v, 1/50 to 1/10), and finally purified by semipreparative HPLC eluting with 35% MeCN–H2O to give 6 (4.0 mg). Fractions 6-2–6-4 were purified by HPLC using 40, 40, and 35% MeOH–H2O to give 5 (9.0 mg), 4 (68.5 mg), and 3 (32.0 mg), respectively.
Nafuredin C (1): white, amorphous powder; [α]20D +40.0 (c 0.37, MeOH); UV (MeOH) λmax (log ε) 240 (4.38) nm; ECD (0.93 mM, MeOH) λmax (Δε) 222 (-17.40), 244 (+21.99) nm; 1H and 13C NMR data, see Table 1; HRESIMS m/z 385.2349 [M +Na]+ (calculated for C22H38O4Na, 380.2795).
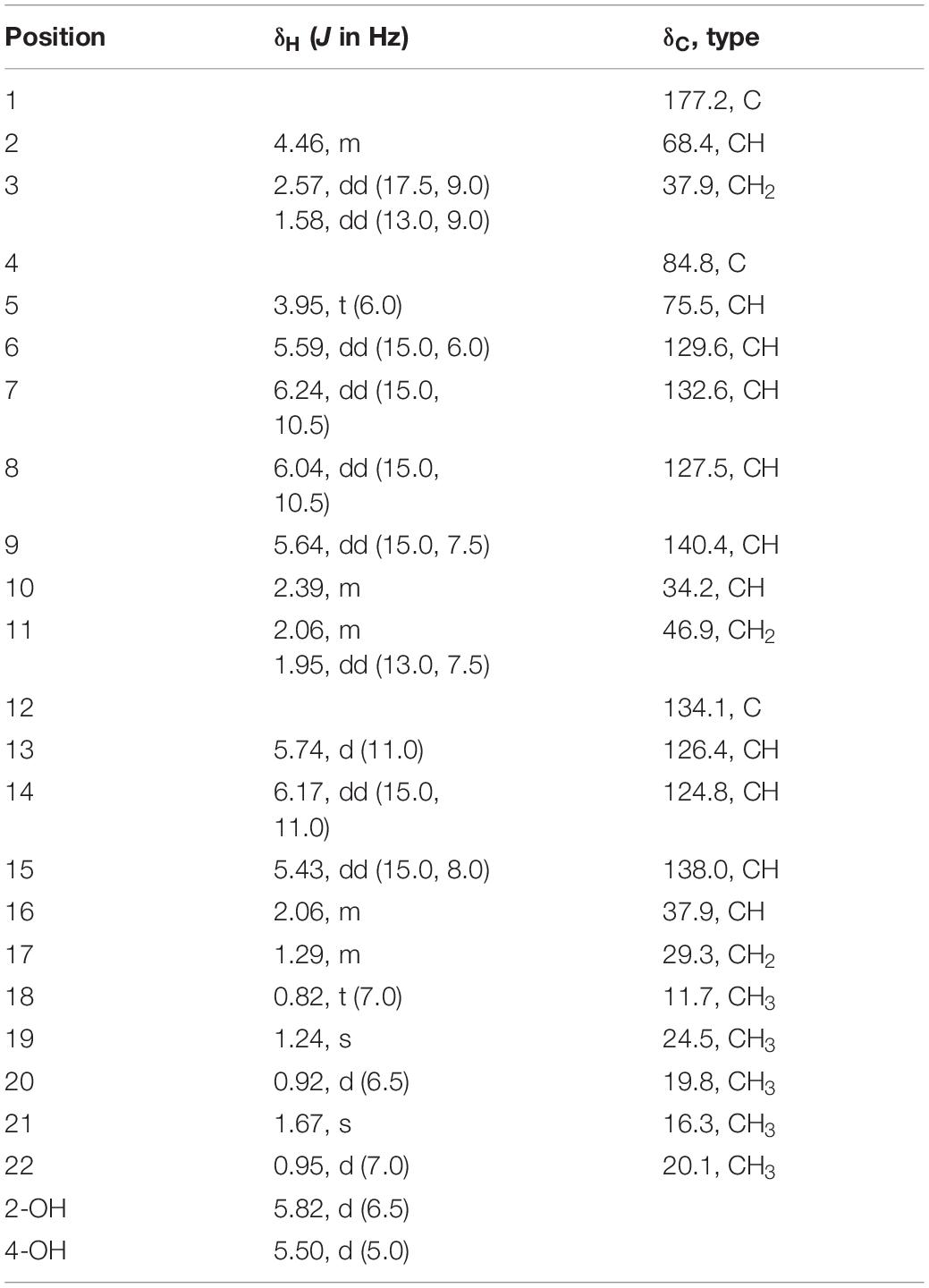
Table 1. 1H NMR data (500 MHz, DMSO-d6, δ in ppm, J in Hz) and 13C NMR data (125 MHz, DMSO-d6, δ in ppm) for 1.
Trichodermamide G (3): yellow, amorphous powder; [α]20D −116.5 (c 0.50, MeOH); UV (MeOH) λmax (log ε) 204 (4.40), 274 (3.96), 324 (4.28) nm; ECD (1.55 mM, MeOH) λmax (Δε) 226 (−3.08), 243 (+1.09), 325 (−5.07) nm; 1H and 13C NMR data, see Table 2; HRESIMS m/z 449.1026 [M +H]+ (calculated for C20H21O8N2S, 449.1013).
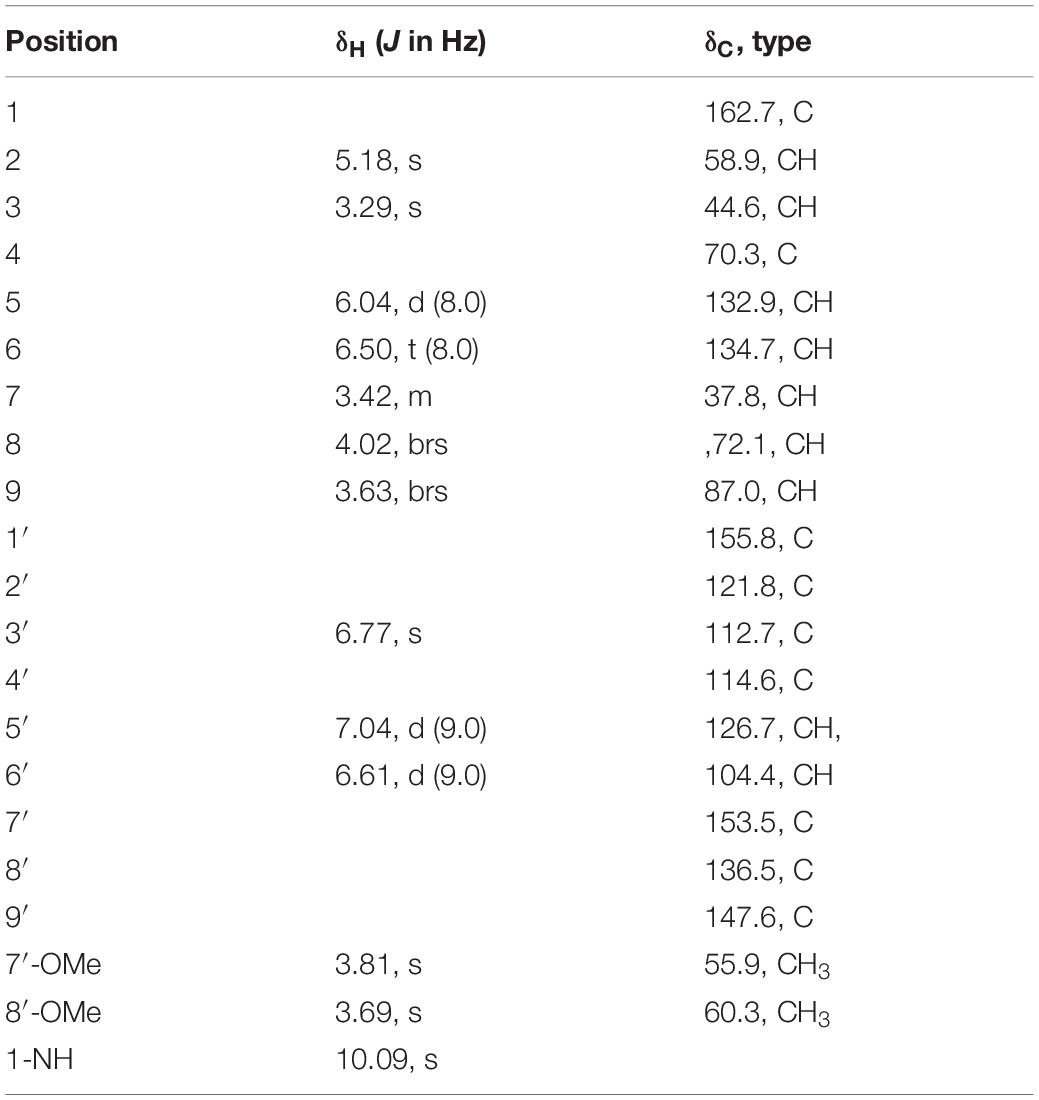
Table 2. 1H NMR data (500 MHz, DMSO-d6, δ in ppm, J in Hz) and 13C NMR data (125 MHz, DMSO-d6, δ in ppm) for 3.
Antifungal Assays
Antifungal activities were evaluated using the conventional broth dilution assay (Appendino et al., 2008). Five phytopathogenic fungal strains, including Botrytis cinerea, Magnaporthe grisea, Phytophthora parasitica, Pestallozzia theae, and Valsa mali were used. Carbendazim was used as a positive control.
Results and Discussion
Structural Elucidation of the Isolated Compounds
Nafuredin C (1) was isolated as a white, amorphous powder. Its molecular formula, C22H34O4, was established from high-resolution ESIMS (HRESIMS), 1H and 13C nuclear magnetic resonance (NMR) data (Supplementary Figures S1, S2, S7), indicating six indexes of hydrogen deficiency. The 1H NMR (Table 1) spectrum displayed seven olefinic protons at δH 6.24 (1H, dd, J = 15.0, 10.5 Hz), 6.17 (1H, dd, J = 15.0, 11.0 Hz), 6.04 (1H, dd, J = 15.0, 10.5 Hz), 5.74 (1H, d, J = 11.0 Hz), 5.64 (1H, dd, J = 15.0, 7.5 Hz), 5.59 (1H, dd, J = 15.0, 6.0 Hz), and 5.43 (1H, dd, J = 15.0, 8.0 Hz), two hydroxyl signals at δH 5.82 (1H, d, J = 6.5 Hz) and 5.50 (1H, d, J = 5.0 Hz), two oxygenated methines at δH 4.46 (1H, m) and 3.95 (1H, t, J = 6.0 Hz), and five methyls at δH 1.67 (3H, s), 1.24 (3H, s), 0.95 (3H, d, J = 7.0 Hz), 0.92 (3H, d, J = 6.5 Hz), and 0.82 (3H, t, J = 7.0 Hz). The 13C NMR and distortionless enhancement by polarization transfer (DEPT) spectra revealed 22 carbon signals, including one ester carbonyl group (δC 177.2), two quaternary carbons (one oxygenated, one olefinic), three methylene groups, 11 methines (seven olefinic, two oxygenated), and five methyl groups. These spectroscopic data are similar to those of nafuredin B, isolated from a mixed culture of the deep-sea-derived fungus Talaromyces aculeatus and mangrove-derived fungus Penicillium variabile (Zhang et al., 2017). The major differences were replacement of the sp2 double bond at C-2 and C-3 in nafuredin B by a hydroxyl group at C-2 in 1, which were consistent with the downfield shifts of C-1/C-4 (δC 163.3/68.0 in nafuredin B vs 177.2/84.8 in 1) and upfield shifts of C-2/C-3 (δC 118.0/156.3 in nafuredin B vs 68.4/37.9 in 1), supported by the COSY correlations of H-2/H-3, and key HMBC from H-2 to C-1/C-4, from H-3 to C-1/C-5, from H-5 to C-3/C-4, from 2-OH to C-1/C-2/C-3, and from H3-19 to C-3/C-4/C-5 (Figure 2 and Supplementary Figures S4, S5).
The relative configuration of the δ-lactone ring was established based on the NOESY spectrum (Figure 2 and Supplementary Figure S6). The correlations of 4-OH with 2-OH and H-5 and of H-6 with H3-19 indicated a cofacial relationship among 4-OH, 2-OH, and H-5. The geometry of double bonds in the olefinic chain was E elucidated from the J values and NOESY correlations (Table 1 and Figure 2). Considering the biosynthesis and coisolation of nafuredin A (2), whose absolute configuration was determined based on asymmetric synthesis (Takano et al., 2001; Zhang et al., 2017), the chirality of C-10 and C-16 was determined to be 10R and 16S, which was supported by the identical NMR data. Comparing the computed ECD spectra with experimental results is a valid method of assigning the absolute configurations of natural products (Cao et al., 2020). To determine the absolute configuration of the δ-lactone ring in 1, ECD computations for all B3LYP/6-311+G(d)-optimized conformers (50 structures) were carried out at the B3LYP/6-311++G(2d,p) level, and six structures with relative energy less than 2.5 kcal/mol were obtained. Boltzmann statistics were performed for ECD simulations with a standard deviation of σ 0.25 eV. The experimental and calculated ECD spectra for the (2R,4S,5R,10R,16S)-1 showed good agreement (Figure 3 and Supplementary Table S1), thus suggesting the absolute configuration.
Trichodermamide C (3) was obtained as a yellow, amorphous powder, with the molecular formula C20H20N2O8S based on its HRESIMS, 1H and 13C NMR data, indicating 12 degrees of unsaturation (Supplementary Figures S8, S9, S15). The 13C NMR and DEPT spectroscopic data (Table 2) revealed 20 carbon signals containing two carbonyl groups, two methoxyl groups, 10 methines (with five olefinic or aromatic, two thiogenated, one nitrogenated, two oxygenated), and six quaternary carbons (with five sp2 and one sp3). In examining the 1H NMR and HSQC data (Supplementary Figures S8, S10), an amide proton at δH 10.09, two ortho-aromatic protons attributed to a 1,2,3,4-tetrasubstituted phenyl unit at δH 7.04 (1H, d, J = 9.0 Hz) and 6.61 (1H, d, J = 9.0 Hz), and three olefinic protons including two derived from a cis-coupled double bond at δH 6.50 (1H, t, J = 8.0 Hz) and 6.04 (1H, d, J = 8.0 Hz) were observed in the deshielded region of the spectrum, whereas shielded signals for five methines at δH 5.18 (1H, s), 4.02 (1H, s), 3.63 (1H, s), 3.42 (1H, d, J = 9.5 Hz), and 3.29 (1H, s) were also present. Additionally, two O-methyl groups (δH 3.81 and 3.69) were observed in the 1H NMR spectrum of 3. Inspection of the above NMR data indicated that the isocoumarin portion of 3 was similar to that of aspergillazine A, a modified dipeptide isolated from the marine-derived fungus Spicaria elegans (Liu et al., 2005), whereas the signals of oxazine were obviously changed, possibly because of the different location of the sulfur bridge. The characteristic NMR data of C-3 (δH 3.29, δC 44.6) and C-7 (δH 3.42, δC 37.8) indicated they were linked by a sulfur atom, which was confirmed from the COSY correlation of H5/H6/H7/H8/H9 and HMBC correlations from H-3 to C-5/C-7/C-9, from H-5 to C-7/C-9, from H-6 to C-4/C-8, and from H-7 to C-9 (Figure 2 and Supplementary Figures S11, S12). In the NOE difference spectra (Figure 2 and Supplementary Figures S13, S14), irradiation of H-2 and H-3 enhanced the resonance of H-9. This information, in addition to the similar coupling constant of H8/H-9 (brs) to trichodermamide A and aspergillazine A (Capon et al., 2005; Liu et al., 2005), indicates a syn relationship among H-2, H-3, H-9, and 8-OH. 4-OH was assigned on the opposite face of the sulfur bridge, as the cis relationship did not lead to a reasonable model according to 3D simulations.
To determine the absolute configuration of 3, ECD calculations were carried out. Monte Carlo conformational searches were applied using Spartan’s 10 software with the Merck molecular force field. Conformers with a Boltzmann-population of over 10% were selected for ECD calculations and were initially optimized at the B3LYP/6-31g (d,p) level in MeOH using the conductor-like polarizable continuum calculation model. Theoretical calculation of the ECD was conducted in MeOH using time-dependent density functional theory at the B3LYP/6-31+g (d,p) level for all conformers of compound (2R,3S,4S,7S,8S,9S)-3. Rotatory strengths for a total of 30 excited states were calculated. ECD spectra were generated using the programs SpecDis 1.6 (University of Würzburg, Würzburg, Germany) and GraphPad Prism 5 (GraphPad, Inc., La Jolla, CA, United States) from dipole-length rotational strengths by applying Gaussian band shapes with sigma = 0.3 eV. The predicted ECD spectrum agreed well with the experimental result (Figure 4 and Supplementary Tables S2, S3), indicating that the absolute configuration of 3 was 2R,3S,4S,7S,8S,9S.
Epipolythiodiketopiperazine alkaloids are fungal metabolites with a highly complex molecular architecture comprising a densely functionalized core structure with many stereogenic centers. In the past decade, an increasing number of studies have discovered powerful new biological processes involving these molecules, including cytotoxic, antileukemic, antiviral, antibiotic, and antinematodal activities (Kim and Movassaghi, 2015); however, epithiodiketopiperazines have not been widely examined. Notably, trichodermamide G is a novel epithiodiketopiperazine derivative with an unprecedented cyclic system containing a sulfur bridge.
Compounds 2 and 4–6 were identified as nafuredin A (Ui et al., 2001), trichodermamide A (Liu et al., 2005), aspergillazine A (Liu et al., 2005), and peniisocoumarin H (Cai et al., 2018) by comparison of their spectroscopic data with those in the literature.
Antifungal Activity
In the present study, all isolated compounds were evaluated to determine their anti-phytopathogenic fungal activities against B. cinerea, M. oryzae, P. theae, P. parasitica, and V. mali. The nafuredin derivatives 1 and 2 exhibited obvious antifungal activities against M. oryzae, with minimum inhibitory concentrations (MICs) of 8.63 and 17.4 μM, respectively. These data indicate that the antifungal activity toward M. oryzae of new compound 1 was the same magnitude as that of the positive control carbendazim (MIC = 3.27 μM). Compounds 1 and 2 also displayed weak antifungal activity against V. mali and P. theae compared to carbendazim (Table 3).
Conclusion
In summary, we report three polyketide derivatives, nafuredin C (1), nafuredin A (2), and peniisocoumarin H (6), and three epithiodiketopiperazine derivatives, trichodermamide G (3), trichodermamide A (4), and aspergillazin A (5), isolated from the mangrove-derived fungus T. harzianum D13. Among them, nafuredin C (1) and trichodermamide G (3) are new compounds. Their structures were assigned based on extensive NMR spectroscopic data, time-dependent density functional theory ECD calculations together with comparison of their ECD spectra. Trichodermamide G contains a unique sulfur bridge compared to the homologous compounds. The polyketide derivatives nafuredin C (1) and nafuredin A (2) exhibited distinct antifungal activity against M. oryzae. T. harzianum have been widely used as biocontrol agents and commercially marketed as biopesticides. Our study expands the source of Trichoderma which used for biocontrol, and provides basic material for the discovery of new antifungal pesticides.
Data Availability Statement
The raw data supporting the conclusions of this article will be made available by the authors, without undue reservation, to any qualified researcher.
Author Contributions
D-LZ and C-SZ conceived and designed the experiments. X-FZ R-HH, DW, X-QW, and PZ performed the experiments. Y-QL, C-JZ, and PZ analyzed the data. D-LZ wrote the manuscript. All authors reviewed the manuscript.
Funding
This work was supported by the National Natural Science Foundation of China (41806194), the Major Agricultural Application Technology Innovation Projects of Shandong Province (SD2019ZZ002), and the Foundation of Key Laboratory of Tropical Medicinal Resource Chemistry of Ministry of Education (RDZH2019001).
Conflict of Interest
The authors declare that the research was conducted in the absence of any commercial or financial relationships that could be construed as a potential conflict of interest.
Acknowledgments
We thank Dr. Fei Cao (College of Pharmaceutical Sciences, Hebei University) and Dr. Chao Liu (Sun Yat-sen University Cancer Center) for their efforts in the ECD analysis.
Supplementary Material
The Supplementary Material for this article can be found online at: https://www.frontiersin.org/articles/10.3389/fmicb.2020.01495/full#supplementary-material
References
Appendino, G., Gibbons, S., Giana, A., Pagani, A., Grassi, G., Stavri, M., et al. (2008). Antibacterial cannabinoids from Cannabis sativa: a structure-activity study. J. Nat. Prod. 71, 1427–1430. doi: 10.1021/np8002673
Cai, R. L., Wu, Y. N., Chen, S. H., Cui, H., Liu, Z. M., Li, C. Y., et al. (2018). Peniisocoumarins A–J: isocoumarins from Penicillium commune QQF-3, an endophytic fungus of the mangrove plant Kandelia candel. J. Nat. Prod. 81, 1376–1383. doi: 10.1021/acs.jnatprod.7b01018
Cao, F., Meng, Z. H., Wang, P., Luo, D. Q., and Zhu, H. J. (2020). Dipleosporalones A and B, dimeric azaphilones from a marine-derived Pleosporales sp. fungus. J. Nat. Prod. 83, 1283–1287. doi: 10.1021/acs.jnatprod.0c00132
Capon, R. J., Ratnayake, R., Stewart, M., Lacey, E., Tennant, S., and Gill, J. H. (2005). Aspergillazines A–E: novel heterocyclic dipeptides from an Australian strain of Aspergillus unilateralis. Org. Biomol. Chem. 3, 123–129. doi: 10.1039/b413440k
Contreras-Cornejo, H. A., Macias-Rodriguez, L., del-Val, E., and Larsen, J. (2016). Ecological functions of Trichoderma spp. and their secondary metabolites in the rhizosphere: interactions with plants. FEMS Microbiol. Ecol. 92:fw036. doi: 10.1093/femsec/fw036
Guzman-Guzman, P., Porras-Troncoso, M. D., Olmedo-Monfil, V., and Herrera-Estrella, A. (2019). Trichoderma species: versatile plant symbionts. Phytopathology 109, 6–16. doi: 10.1094/PHYTO-07-18-0218-RVW
Huang, R. H., Gou, J. Y., Zhao, D. L., Wang, D., Liu, J., Ma, G. Y., et al. (2018). Phytotoxicity and anti-phytopathogenic activities of marine-derived fungi and their secondary metabolites. RSC Adv. 8, 37573–37580. doi: 10.1039/c8ra08047j
Kim, J., and Movassaghi, M. (2015). Biogenetically-inspired total synthesis of epidithiodiketopiperazines and related alkaloids. Acc. Chem. Res. 48, 1159–1171. doi: 10.1021/ar500454v
Liu, R., Gu, Q. Q., Zhu, W. M., Cui, C. B., and Fan, G. T. (2005). Trichodermamide A and aspergillazine A, two cytotoxic modified dipeptides from a marine-derived fungus Spicaria elegans. Arch. Pharmacal Res. 28, 1042–1046. doi: 10.1007/bf02977399
Ma, L. J., and Xu, J. R. (2019). Shuffling effector genes through mini-chromosomes. PLos Genet. 15:e1003826. doi: 10.1371/journal.pgen.1008345
Martin-Urdiroz, M., Oses-Ruiz, M., Ryder, L. S., and Talbot, N. J. (2016). Investigating the biology of plant infection by the rice blast fungus Magnaporthe oryzae. Fungal Genet. Biol. 90, 61–68. doi: 10.1016/j.fgb.2015.12.009
Takano, D., Nagamitsu, T., Ui, H., Shiomi, K., Yamaguchi, Y., Masuma, R., et al. (2001). Absolute configuration of nafuredin, a new specific NADH-fumarate reductase inhibitor. Tetrahedron Lett. 42, 3017–3020. doi: 10.1002/chin.200129194
Ui, H., Shiomi, K., Yamaguchi, Y., Masuma, R., Nagamitsu, T., Takano, D., et al. (2001). Nafuredin, a novel inhibitor of NADH-fumarate reductase, produced by Aspergillus niger FT-0554. J. Antibiot. 54, 234–238. doi: 10.7164/antibiotics.54.234
Zhang, Z. Z., He, X. Q., Zhang, G. J., Che, Q., Zhu, T. J., Gu, Q. Q., et al. (2017). Inducing secondary metabolite production by combined culture of Talaromyces aculeatus and Penicillium variabile. J. Nat. Prod. 80, 3167–3171. doi: 10.1021/acs.jnatprod.7b00417
Zhao, D. L., Han, X. B., Wang, D., Liu, M. H., Gou, J. Y., Peng, Y. L., et al. (2019). Bioactive 3-decalinoyltetramic acids derivatives from a marine-derived strain of the fungus Fusarium equiseti D39. Front. Microbiol. 10:1285. doi: 10.3389/fmicb.2019.01285
Zhao, D. L., Wang, D., Tian, X. Y., Cao, F., Li, Y. Q., and Zhang, C. S. (2018). Anti-phytopathogenic and cytotoxic activities of crude extracts and secondary metabolites of marine-derived fungi. Mar. Drugs 16:36. doi: 10.3390/md16010036
Zhao, X. X., Wang, X. M., Xu, P., Zhao, L. N., Hu, Y., Chen, J. Y., et al. (2019). Research and prospect of rice blast resistance. Hubei Agr. Sci. 58, 5–9. doi: 10.14088/j.cnki.issn0439-8114.2019.11.001
Keywords: nafuredins, epithiodiketopiperazines, Magnaporthe oryzae, antifungal activity, Trichoderma harzianum
Citation: Zhao D-L, Zhang X-F, Huang R-H, Wang D, Wang X-Q, Li Y-Q, Zheng C-J, Zhang P and Zhang C-S (2020) Antifungal Nafuredin and Epithiodiketopiperazine Derivatives From the Mangrove-Derived Fungus Trichoderma harzianum D13. Front. Microbiol. 11:1495. doi: 10.3389/fmicb.2020.01495
Received: 07 May 2020; Accepted: 08 June 2020;
Published: 26 June 2020.
Edited by:
Laure Ries, University of São Paulo, BrazilReviewed by:
Linghong Meng, Institute of Oceanology (CAS), ChinaJunfeng Wang, South China Sea Institute of Oceanology (CAS), China
Copyright © 2020 Zhao, Zhang, Huang, Wang, Wang, Li, Zheng, Zhang and Zhang. This is an open-access article distributed under the terms of the Creative Commons Attribution License (CC BY). The use, distribution or reproduction in other forums is permitted, provided the original author(s) and the copyright owner(s) are credited and that the original publication in this journal is cited, in accordance with accepted academic practice. No use, distribution or reproduction is permitted which does not comply with these terms.
*Correspondence: Peng Zhang, emhhbmdwZW5nQGNhYXMuY24=; Cheng-Sheng Zhang, emhjaGVuZ3NoZW5nQDEyNi5jb20=
†These authors have contributed equally to this work