- 1Centro de Investigaciones en Ciencias Microbiológicas, Instituto de Ciencias, Benemérita Universidad Autónoma de Puebla, Puebla, Mexico
- 2Facultad de Ciencias Químicas, Benemérita Universidad Autónoma de Puebla, Puebla, Mexico
Volatile organic compounds (VOCs) produced by rhizobacteria have been proven to stimulate plant growth during germination and seedling stages. However, the modulating effect of bacterial volatiles on the germination of seeds subjected to heavy metal stress is scarcely studied. In this work, the ability of volatiles released by Bacillus sp. MH778713 to induce seed dormancy breakage in Prosopis laevigata and Arabidopsis thaliana seeds were examined. The minimal inhibitory concentration of chromium (Cr) VI that prevents seed germination of P. laevigata and A. thaliana on water-Cr-agar plates was 2500 and 100 mg L–1, respectively. Remarkably, partitioned Petri-dish co-cultivation of Bacillus sp. MH778713 and plant seeds under Cr-stress showed the beneficial effect of volatiles emitted by Bacillus sp. MH778713, helping plant seeds to overcome Cr-stress. Among the metabolites emitted by Bacillus sp. MH778713, octadecane, heneicosane, 2,4-di-tert-butylphenol, hexadecane, eicosane, octacosane, and tetratriacontane were the most abundant. To confirm that these long-chain compounds produced by Bacillus sp. MH778713 could be responsible for the seed dormancy breakage, high pure organic compounds (2,4-di-tert-butylphenol, heneicosane, hentriacontane, and tetracosane) were used directly in germination assays of P. laevigata and A. thaliana seeds instead of volatiles emitted by Bacillus sp. MH778713. All organic compounds allowed Prosopis and Arabidopsis seeds to overcome Cr-toxicity and germinate. The results of this study provide new insight into the role of long-chain bacterial compounds produced by Bacillus sp. MH778713 as triggers of seed abiotic stress tolerance, surmounting chromium stress and stimulating seedling development.
Introduction
Plant growth-promoting rhizobacteria (PGPR) are beneficial plant symbionts capable of providing plants with improved water and nutrient uptake (Vacheron et al., 2013; Backer et al., 2018). Rhizobacteria are intimately associated with the root system of the plants and can affect the growth and fitness of plants by modulating nutrient uptake, inducing systemic resistance, and tolerance to abiotic and biotic stress (Bitas et al., 2013; Backer et al., 2018). PGPR can produce auxins, cytokinins, and gibberellins that regulate plant growth and development. Furthermore, each rhizobacterial species produce a specific blend of volatile organic compounds (VOCs) with important roles in plant biology and bacterial life cycle (Ryu et al., 2003; Sharifi and Ryu, 2018).
Bacterial volatiles can modulate root architecture, iron uptake, sodium and auxin homeostasis in plants (Zhang et al., 2007; Gutierrez-Luna et al., 2010; Liu and Zhang, 2015). Thus, salt-stressed plants treated with Bacillus amyloliquefaciens VOCs show greater Na+-stress tolerance than control plants (Zhang et al., 2008). Similarly, drought-stressed plants exposed to B. subtilis GB03 VOCs were more tolerant than plants without VOCs treatment due to the accumulation of osmoprotectants such as choline and glycine (Zhang et al., 2010; Liu and Zhang, 2015). The volatile blend release by Bacillus subtilis GB03 can also induce systemic resistance in Arabidopsis against Erwinia carotovora (Ryu et al., 2004). To date, the demonstrated efficacy of VOCs in enhancing plant fitness and tolerance to salinity, drought, iron deficiency, and sulfur starvation stress suggest that bacterial volatiles might help plants to tolerate other abiotic stresses, but their effectiveness should be confirmed for each stress condition.
Seed dormancy and germination are complex processes regulated mainly by the plant hormones gibberellins (GAs) and abscisic acid (ABA). Environmental and endogenous factors might control the abundance of signaling molecules which determine the ABA/GAs ratio, thus elevated ABA concentration within seeds triggers seed dormancy while high GAs level induces germination (Bailly et al., 2008; Graeber et al., 2012; Li et al., 2018). There are different definitions and classifications of seed dormancy; a delay or fail to germinate under favorable conditions is a generally accepted definition (Arc et al., 2013). Several abiotic stresses like salinity, drought, cold, and exposure to heavy metal can induce ABA biosynthesis and seed dormancy (Vishal and Kumar, 2018). Indeed, arsenic and vanadium up-regulate ABA biosynthesis genes of rice roots while cadmium increases endogenous ABA levels (Bücker-Neto et al., 2017). Germination of wheat, cucumber, and chickpea seeds decreases by exposure to copper (Cu2+), zinc (Zn2+) or lead (Pb) whereas abscisic acid content increase; suggesting that ABA plays an important role not only on seed germination but also in stress response (Munzuroğlu et al., 2008; Wang et al., 2014; Bücker-Neto et al., 2017).
Chromium (Cr) is a non-essential and toxic element to plants and animals. The most ubiquitous oxidation states of Cr in nature are trivalent (Cr III) and hexavalent (Cr VI) chromium; being Cr(VI) the most phytotoxic (Panda and Choudhury, 2005). Chromium(VI) at concentrations ranged 50–100 mg kg–1 soil are toxic for most plants affecting germination rate, chlorophyll content, and most of the growth parameters (Akinci and Akinci, 2010; Jain et al., 2016; Amin et al., 2019). For plants growing in a nutrient solution, 10–25 mg L–1 Cr VI is detrimental for plant development (Dube et al., 2003; Ramírez et al., 2019). Nonetheless, Prosopis plants can tolerate, translocate, and hyper accumulate heavy metals, including Pb, As and Cr6+ (Aldrich et al., 2003, 2007; Jayaram and Prasad, 2009). We reported that Prosopis trees grown in polluted wildlife habitats are highly tolerant of Cr (VI), in part, by their interaction with the endophytic chromium hyper-tolerant Bacillus sp. MH778713 (Ramírez et al., 2019). Bacillus sp. MH778713 was isolated from Prosopis laevigata nodules. This Bacillus strain tolerates up to 15000 mg/L Cr(VI), bioaccumulates up to 100 mg Cr (VI)/g of cells and promotes the development of Cr-stressed P. laevigata seedlings growing in hydroponic systems. Other Cr-resistant bacteria with lower tolerance (up to 780 mg/L Cr), including Bacillus genera, also promote the growth of Cr-stressed plants suggesting that PGPR help plants to reduce metal-induced oxidative damage (Bruno et al., 2020; Din et al., 2020; Shreya et al., 2020). Cr-tolerant bacteria with PGPR features ameliorate Cr-toxicity on plants through reduction of Cr(VI) to Cr(III) to stabilize Cr, increasing antioxidant activities of plants, reducing lipid peroxidation and removing Cr from soil (Purwanti et al., 2017; Bruno et al., 2020; Din et al., 2020). In this paper an elevated Cr(VI) concentration (2500 mg L–1) was required to prevent germination of imbibed seeds of Prosopis, highlighting the tolerance of Prosopis to heavy metals. Only seeds exposed to Bacillus sp. MH778713 volatiles were able to germinate in the presence of 2500 mg L–1 Cr(VI). Long-chain alkanes released by Bacillus sp. MH778713 were found to help seeds to overcome dormancy, the potential role of these compounds in response to chromium stress will be discussed.
Materials and Methods
Bacteria and Seed Preparation
Bacillus sp. MH778713, an isolate from P. laevigata nodules (Ramírez et al., 2019), and Escherichia coli BL21(DE3) were streaked onto yeast-extract mannitol agar (YMA) plates and incubated for 24 h in darkness at 28°C. For long-term storage, bacterial strains were kept at −80°C in yeast-extract mannitol broth with 20%(v/v) glycerol. Prosopis laevigata seeds were surface-sterilized by rinsing with distilled water and then soaking in concentrated sulfuric acid (98%) for 20 min, followed by rinsing 3–4 times in sterile distilled water. Arabidopsis thaliana ecotype Columbia (Col-0) seeds were prepared by treating them with 2% Tween-20 for 1 min, washed five times with sterile distilled water and placed on Petri dishes containing water agar (0.75% w/v). All chemicals including heneicosane, hentriacontane, 2,4-di-tert-butyl phenol, and tetracosane were purchased from Sigma Aldrich.
Determination of Minimum Inhibitory Concentration (MIC) of Chromium to Stop Germination of Prosopis laevigata and Arabidopsis thaliana
To determine the minimal amount of Cr(VI) that stop seed germination of Prosopis laevigata, increasing concentrations of 100 mg L–1 chromium (100, 200, 300, 400 up to 2500 mg L–1) were assayed. For Arabidopsis thaliana ecotype Col-0 increasing concentrations of 10 mg L–1 Cr(VI) (10, 20, 30, 40 up to 100 mg L–1) were assayed. Higher concentrations of chromium were not assayed because P. laevigata and A. thaliana seeds did not germinate with 2500 and 100 mg L–1 of Cr(VI), although they did germinate with 2400 and 90 mg L–1, respectively. Surface-sterilized Prosopis or Arabidopsis seeds were placed onto partitioned plates containing water-agar (0.75% w/v) added with concentrations of K2CrO4 described above. Petri plates with seeds were incubated at 28°C for 24 and 72 h in dark for the germination test of Prosopis and Arabidopsis. After germination, Prosopis and Arabidopsis seedling were incubated two more days for complete radicle development. The minimum inhibitory concentration assay was done in quintuplet, each experiment was repeated at least three times.
Effect of Volatiles Released by Bacillus sp. MH778713 on Germination of Cr-Stressed Seeds
To determine whether the blend of volatiles emitted by Bacillus sp. MH778713 could help Prosopis or Arabidopsis seeds to overcome Cr-stress, co-cultivation of Bacillus sp. MH778713 and Prosopis/Arabidopsis seed in a partitioned Petri dishes were carried out. Partitioned Petri dishes prevents physical contact between Bacillus and seeds. Thus, 10 μl of Bacillus sp. MH778713 (1 × 1010 CFU/ml) was inoculated on Yeast extract Mannitol Agar (YMA) medium on one side of the partitioned plate and Prosopis seed was placed on the Cr-water agar [2500 mg L–1 of Cr(VI), 0.75% w/v agar] medium on the other side of the plate. Similarly, Arabidopsis seeds were germinated on partitioned plates with Cr-water agar medium but with only 100 mg L–1 of Cr(VI). After inoculation, Petri dishes containing seeds were covered and sealed with parafilm to minimize volatiles leaking. Treated plates were incubated at 28°C for 24 and 72 h in the dark for seed germination test of Prosopis and Arabidopsis, respectively. Pictures of Figure 1 were taken 2 days after germination to let seedling reach a greater development. Partitioned Petri dishes with Prosopis or Arabidopsis seeds on Cr-water agar medium and YMA without Bacillus or with Escherichia coli BL21(DE3) were used as negative controls. Germination assay of Cr-stressed seeds in the presence and absence of Bacillus sp. MH778713 volatiles was performed in quintuplet, each being repeated at least three times.
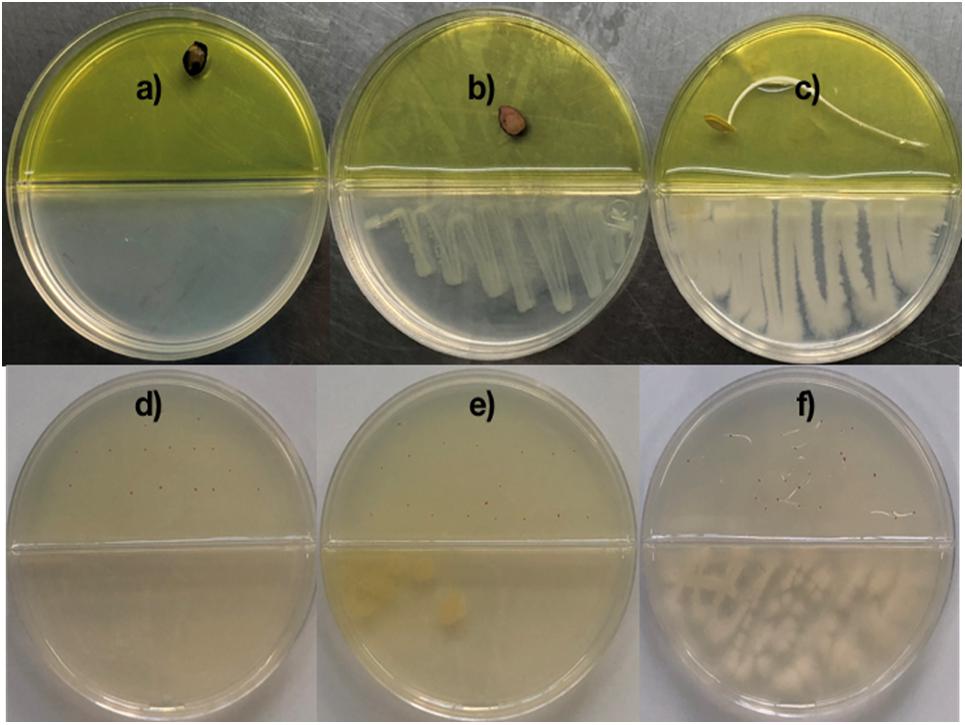
Figure 1. Co-cultivation of Bacillus sp. MH778713 and Prosopis laevigata/Arabidopsis thaliana in partitioned Petri dish in the dark at 28°C for germination assay. Pictures were taken after 72 and 120 h for Prosopis and Arabidopsis, but germination was observed after 24 and 72 h, respectively. Prosopis laevigata seeds were imbibed 72 h on an aqueous agar plate containing 2500 mg L–1 of Cr(VI) while exposed to volatiles released by Escherichia coli BL21 (DE3) as a negative control (b), Bacillus sp. MH778713 (c) or nothing (negative control) (a). Similarly, Arabidopsis thaliana seeds were imbibed 120 h on an aqueous agar plate containing 100 mg L–1 Cr(VI) while exposed to volatiles released by E. coli BL21 (DE3) (e), Bacillus sp. MH778713 (f) or nothing (d). Seed germination assays were done in quintuplet; each experiment was repeated at least three times.
Analysis of Metabolites Released by Bacillus sp. MH778713
To determine the metabolites produced by Bacillus sp. MH778713, co-cultivations of Bacillus and Prosopis seeds in partitioned Petri dishes were carried out as described above but plates were incubated for 24 and 48 h. To do that, sealed Petri plates containing the Bacillus culture were incubated and then opened at 24 h to extract the compounds released by the bacterium while other plates were opened at 48 h. To extract the compounds released by Bacillus sp. MH778713, 3 ml of benzene was added into the compartment of the Petri dishes containing the Bacillus culture, the Petri dishes were gently shaken for 1 min and benzene was collected into microtubes. The microcentrifuge tubes were vortexed for 10 min to obtain the crude extract which was centrifuged at 13000 rpm (15865 g) for 5 min twice, the supernatant was collected for the gas chromatography-mass spectrometry (GC-MS) analysis. The metabolites extracted from co-cultivation of Bacillus sp. MH778713-Prosopis seed and cultures of Bacillus alone were analyzed by GC-MS. Similarly, E. coli BL21 DE3 was grown for 24 h in sealed partitioned Petri dishes with Prosopis seed for metabolites extraction with benzene as a negative control. GC/SM analysis was performed using Agilent 7820A Gas Chromatograph system (Santa Clara, CA, United States) with a HP-5ms column (30 m length, 0.25 mm i.d., 0.25 μm film thickness). The initial oven temperature was set at 40°C and kept for 5 min, then ramped up at a rate of 15°C/min to 240°C, and held 6.667 min, for a total run time of 25 min. The carrier gas was helium at 1.2 ml/min rate. The Agilent 5975C Gas Chromatograph/Mass Selective Detector (Santa Clara, CA, United States) was operated in the electron ionization mode at 70 eV, a source temperature of 220°C, with a continuous scan from m/z 50 to 500. The constituents of benzene extracts were identified by comparing the fragmentation patterns in mass spectra with those of the NIST08.L library and published mass spectra. To confirm the presence of 2,4-ditert-butylphenol, heneicosane, tetracosane, and hentriacontane in the benzene extracts, the retention time of the GC peaks of reference compounds run under identical conditions and mass spectra was also compared. Each sample was run in triplicate. Most abundant compounds that we identified as being emitted by Bacillus sp. MH778713 were tested individually for their ability to promote seed germination under Cr-stress.
Promotion of Seed Germination and Seedling Growth of Prosopis laevigata and Arabidopsis thaliana by 2,4-Di-Tert-Butylphenol, Heneicosane, Hentriacontane, and Tetracosane Under Cr-Stress
To evaluate the ability of 2,4-Di-tert-butyl phenol (CAS# 96-76-4), heneicosane (CAS# 629-94-7), hentriacontane (CAS# 630-04-6), and tetracosane (CAS# 646-31-1) to break seed dormancy of Cr-stressed seeds; partitioned Petri dish experiments were performed as described above. A stock solution of each compound was prepared using benzene as a solvent. Two initial dosages of each compound were assayed, 50 and 500 μg, considering the concentrations reported in the literature as an optimal (0.01–100 μg) for plant-growth induction in partitioned Petri dish experiments (Fincheira and Quiroz, 2018). Thus, 6 or 60 μl of each stock solution was inoculated on Yeast extract Mannitol Agar (YMA) medium on one side of the partitioned plate and Prosopis or Arabidopsis seed was placed onto the Cr-water agar [2500 or 100 mg L–1 Cr(VI), 0.75% w/v agar] medium on the other side of the plate to evaluate seed germination. Partitioned Petri plates were incubated at 28°C for 24 and 72 h in the darkness for Prosopis and Arabidopsis germination; although pictures for figures were taken after 72 and 120 h, respectively. In the same way, E. coli BL21 (DE3) or benzene (6 μl) was inoculated on the YMA medium on one side of the partitioned plate as negative control while Bacillus sp. MH778713 was inoculated as a positive control. After 72 h of incubation, the growth parameters of Prosopis seedlings were evaluated to determine which compound was more effective (Figure 5). The promotion of seed germination was done in quintuplet; each experiment was repeated at least three times. Finally, 0.05, 0.5, and 5 μg of each compound was also evaluated for the germination assays.
Growth Promotion of Prosopis Seedling by 2,4-Di-Tert-Butylphenol, Heneicosane, Hentriacontane, Tetracosane, and Bacillus sp. MH778713
Partitioned Petri plates were prepared as described above with YMA and water-agar (0.75% w/v) medium. No chromium was added to the seed side of the Petri dish to let seedling growth normally under this condition. On the side of the plate containing YMA medium 6 μl of solvent alone (benzene), 50 μg of 2,4-di-tert-butyl phenol, heneicosane, hentriacontane, or tetracosane was added to evaluate Prosopis seedling growth promotion. Furthermore, partitioned Petri plates with 10 μl of Bacillus sp. MH778713 (1 × 1010 CFU/ml) on YMA medium and Prosopis seeds on the water-agar medium were included as a positive control.
Statistical Analysis
The statistical analysis of seedling growth parameters was performed using Sigma Plot (Handel Scientific Software). Data were analyzed globally by ANOVA single factor, and significant treatment effects were determined by the Tukey–Kramer Post Hoc test.
Results
Volatiles Emitted by Bacillus sp. MH778713 Break Seed Dormancy
Chromium is highly toxic to plants and harmful to their growth and development. To determine the minimal amount of chromium (VI) that prevent seed germination, surface-sterilized seeds of Prosopis laevigata and Arabidopsis thaliana were placed onto water-agar plates supplemented with 100, 200, 300, … up to 2500 mg L–1 of K2CrO4 for germination assays of Prosopis and 10, 20, 30, … up to 100 mg L–1 for Arabidopsis. We found out that 100 and 2500 mg L–1 of Cr(VI) precluded seed germination of A. thaliana and P. laevigata, confirming chromium phytotoxic effects. To assess the ability of Bacillus sp. MH778713 to produce volatiles and promote germination of seeds exposed to Cr(VI), co-cultivations of Bacillus sp. MH778713 and Prosopis laevigata/Arabidopsis thaliana in partitioned Petri dish were carried out over 3 and 5 days, respectively. Two types of agar-media were used in each partitioned Petri plate, YM-Agar medium on bacteria’s side and Water-Agar added with chromium on the seed side. The yellow color of the medium on the Prosopis seed’s side was caused by the high chromium concentration (Figures 1a–c). Partitioned Petri plates prevent any physical contact between bacteria and seeds or diffusion of non-volatile metabolites through the medium. Thus, any stimulating effect of Bacillus sp. MH778713 on seed germination can be attributed to the volatiles emitted by the bacterium. Our results showed that in the presence of Bacillus sp. MH778713, P. laevigata seeds on Cr-agar plates germinated in 24 h of co-cultivation and showed an efficient seedling development until 72 h (Figure 1c), while the negative control (seeds non-exposed to Bacillus VOCs) were unable to germinate in presence of chromium (Figure 1a). Similar results were observed with Arabidopsis thaliana seeds (Figures 1d,f), suggesting that Bacillus sp. MH778713 produces volatiles that promote germination and seedling growth under chromium phytotoxic conditions. Escherichia coli BL21(DE3), like any living organism, produces volatiles and was used as a second negative control (Figures 1b,e). Under chromium stress, neither Prosopis nor Arabidopsis seeds germinated when they were exposed to volatiles emitted by E. coli (Figures 1b,e). These results indicate that airborne signals emitted by Bacillus sp. MH778713 trigger seed dormancy-breaking on the partitioned Petri dish experiments. Since bacteria growing in rich media can use amino acids as a carbon source releasing ammonia, thus alkalinizing the medium, the pH of Bacillus and E. coli cultures was measured. Both bacteria were cultivated on YMA plates with an initial pH of 6.8, after 72 h the pH of Bacillus and E. coli cultures were 8 and 5.5, respectively. These results indicate that E. coli can be producing organic acids responsible of the pH drop.
Long-Chain Alkanes and 2,4-Di-Tert-Butylphenol Were the Most Abundant Metabolites Produced by Bacillus sp. MH778713
To determine the metabolites emitted by Bacillus sp. MH778713, bacterial cultures like those described in Figure 1c were carried out taking samples from the Petri plates at 24 and 48 h. Benzene was used for extracting compounds produced by the Bacillus cultures and metabolites profile was determined using GC-MS analysis as described in section “Materials and Methods.” Nature of metabolites produced by Bacillus sp. MH778713 include alcohols, alkanes, alkenes, esters, ketones, amide, and fatty acids (Figure 2). A total of 29 compounds were identified, 18 of them were absent at 48 h of culture while eleven of them were present both at 24 and 48 h. The most abundant compounds produced at 24 h of culture were heneicosane, 2,4-di-tert-butylphenol, eicosane, hentriacontane, octadecane, octacosane, and n-octadecyl ester-heptafluorobutyric acid (Figure 2). To determine if Prosopis seeds were also producing volatiles during germination (24 h of incubation), the metabolite profile of Bacillus growing alone (without seed) in a partitioned Petri plate was determined. As shown in Figure 2, the profile of Bacillus with and without seed were comparable, except for 2-bromotetradecane, n-octadecyl ester-heptafluorobutyric acid, nonadecane, hexacosane, 7,9-Di-tert-butyl-1-oxaspiro(4,5)deca-6,9-diene-2,8-dione, 1-docosene, and 11-(1-ethylpropyl)-heneicosane that were produced only when Prosopis seed was present. Since culture medium and E. coli produce volatiles, the profile of E. coli growing in YMA was determined in a partitioned plate in the presence of Prosopis seed. No peaks related to heneicosane, 2,4-di-tert-butylphenol, eicosane, hentriacontane, octadecane, octacosane, or heptadecane were found, indicating that long-chain alkanes were certainly produced by Bacillus sp. MH778713. The chromatographic profiles of metabolites from Bacillus sp. MH778713 and E. coli BL21(DE3) at 24 h of incubation are shown in the Supplementary Figures S2, S3. The number of compounds produced by Bacillus sp. MH778713 was much higher than those produced by E. coli. Heneicosane, 2,4-di-tert-butylphenol, hentriacontane and tetracosane standards were used to quantify the amount produced by Bacillus sp. MH778713 in Petri plate, finding out that it ranged from 0.01 to 0.07 μg. The identity of heneicosane, 2,4-di-tert-butylphenol, and tetracosane produced by Bacillus sp. MH778713 was confirmed by comparing Kovats retention indices (KIs) with KI from pheromone data base (Supplementary Table S1). To determine if heneicosane, 2,4-di-tert-butylphenol, hentriacontane, and tetracosane were present as volatiles in the headspace of the Bacillus sp. MH778713 culture a partitioned plate-experiment was carried out incubating an absorbent material (zeolites) on one side of the plate and Bacillus culture on the other. After 24 h of incubation, volatiles from absorbent material were extracted with benzene and analyzed by GC-MS. None of four compounds was detected in the headspace of the cultures by this method used.
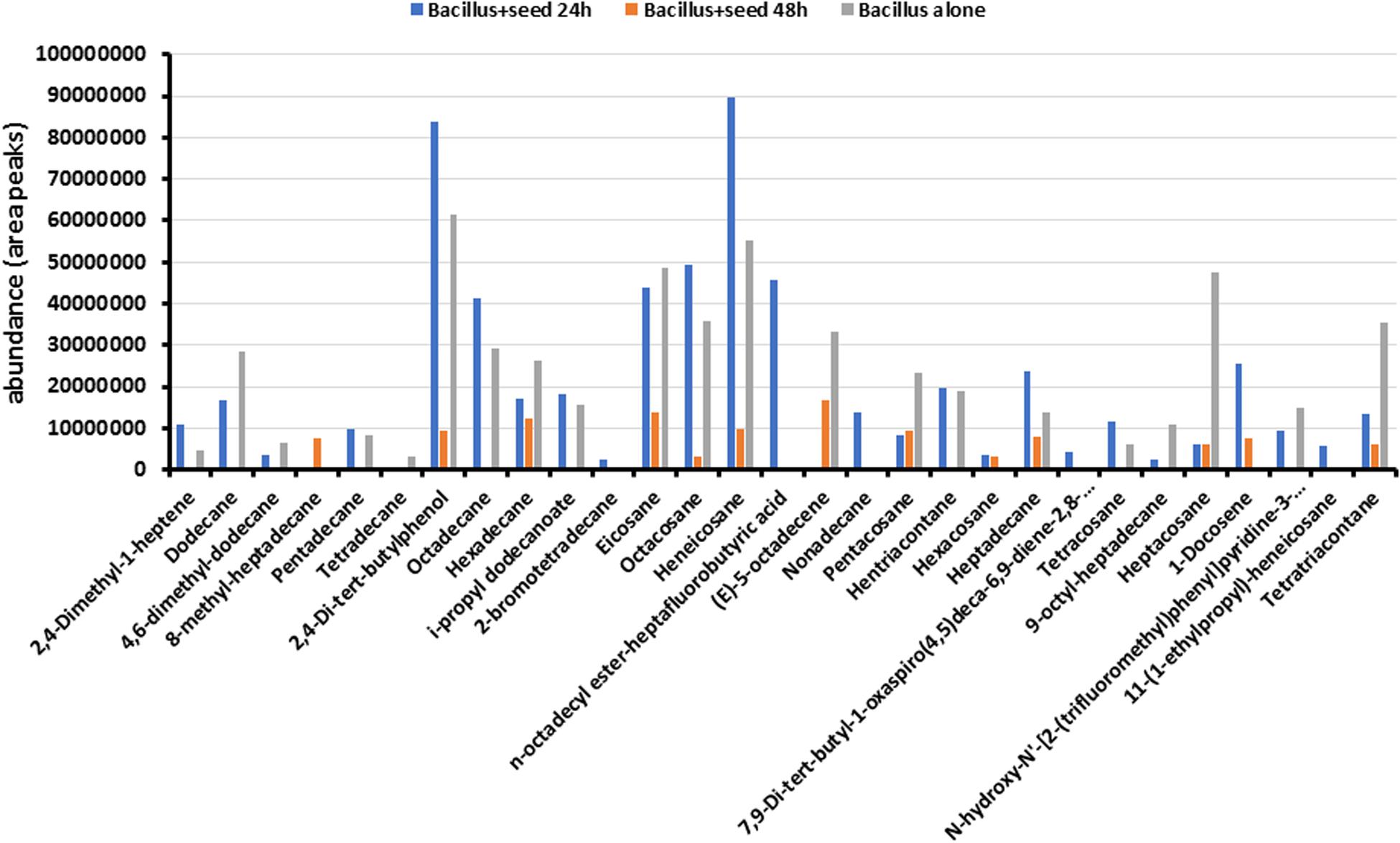
Figure 2. Metabolite profile of Bacillus sp. MH778713 produced after 24 and 48 h of co-cultivation with Prosopis seed in partitioned Petri dishes (blue and orange bars). Metabolite profile of Bacillus sp. MH778713 growing alone in partitioned Petri plate (gray bars) at 24 h of incubation.
Heneicosane, 2,4-Di-Tert-Butylphenol, Hentriacontane, and Tetracosane Trigger Seed Dormancy-Breaking
2,4-di-tert-butyl phenol, heneicosane, hentriacontane, and tetracosane were selected to explore their ability to induce seed dormancy-breaking. 2,4-di-tert-butylphenol was chosen because it is a well-known antioxidant and antifungal VOC produced by Shewanella algae YM17, Trichoderma harzianum T-E5, and Arthrobacter agilis UMCV2. Since our Bacillus sp. MH778713 produced alkanes range from C16 to C34 atoms, heneicosane (C21H44) was chosen as a representative compound of alkanes of 20 carbons and hentriacontane (C31H64) as a representative of alkanes of 30 carbons. Moreover, tetracosane (C24H50) was included in the assay to test if alike alkanes behave similarly (C21H44 and C24H50). Considering the VOCs concentration reported in the literature as optimal for plant-growth induction in partitioned Petri dish experiments (0.01–100 μg), we decided to test a moderate (50 μg) and high (500 μg) dosage of 2,4-di-tert-butyl phenol, heneicosane, hentriacontane, and tetracosane. All compounds induced seed germination in the presence of chromium and no difference in the stimulating effect on seed germination was observed at 50 and 500 μg dosage. The high (500 μg) dosage of 2,4-di-tert-butyl phenol, heneicosane, hentriacontane, and tetracosane did not show any phytotoxic effects on the seed germination assay of Prosopis. Representative pictures of seed germination experiments using 50 μg of 2,4-di-tert-butyl phenol, heneicosane, hentriacontane, and tetracosane are shown in Figure 3. For each condition tested, five Prosopis laevigata seeds were assayed, one per plate, over 3 days of laboratory conditions. Each seed was placed on a water-agar medium added with 2500 mg L–1 of chromium (VI) and 2,4-di-tert-butyl phenol, heneicosane, hentriacontane, tetracosane or benzene were inoculated on YM-agar side of the partitioned Petri dish. The negative control, benzene, was unable to induce seed germination (Figure 3a) while all assessed compounds triggered seed dormancy-breaking (Figures 3b–e). Since all stock solutions were prepared using benzene as a solvent, benzene was also tested as a growth inducer but failed to induce seed dormancy-breaking (Figure 3a). Similarly, pure compounds were tested on A. thaliana seeds subjected to 100 mg L–1 of chromium (Figure 4). Benzene failed to break seed dormancy of A. thaliana (Figure 4a), while 50 μg of tetracosane, hentriacontane, heneicosane, and 2,4-di-tert-butyl phenol induced 96, 44, 72, and 56% of seed germination (Figures 4b–e). Lower concentrations (0.05, 0.5, and 5 μg per plate) of each pure compound were tested for their ability to break the dormancy of Prosopis seeds under chromium stress. Only the dosage of 5 μg was able to break seed dormancy while 0.05 and 0.5 μg was not (Supplementary Figure S1).
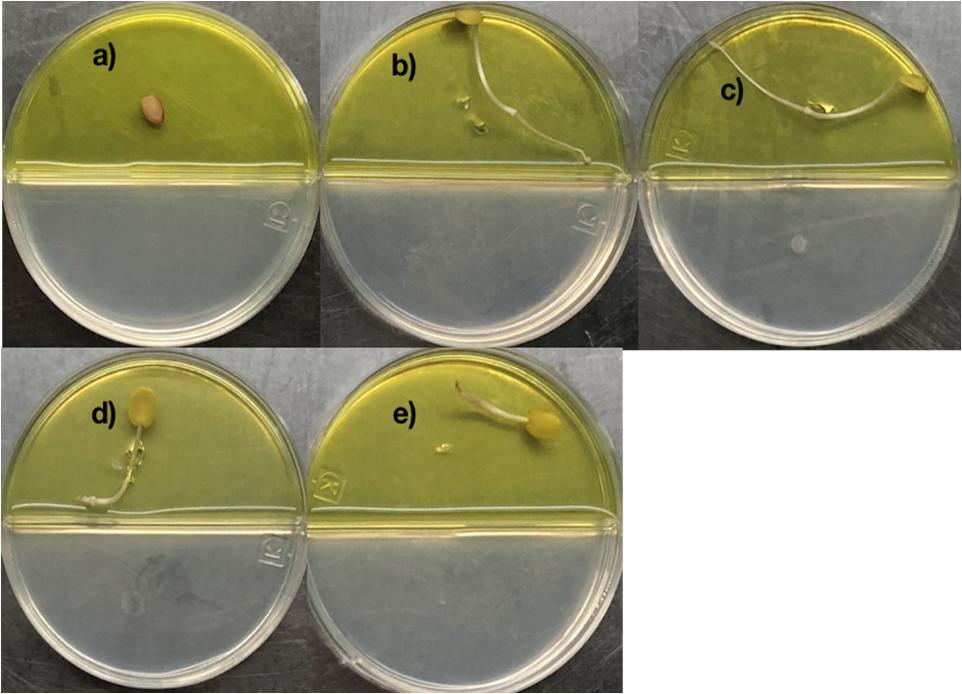
Figure 3. Promotion of seed germination by tetracosane, heneicosane, hentriacontane and 2,4-Di-tert-butylphenol under Cr-stress. Prosopis laevigata seeds were imbibed 72 h at 28°C in the dark on aqueous agar plate containing 2500 mg L–1 of chromium(VI) while exposed to vapor of benzene as negative control (a), tetracosane (b), heneicosane (c), hentriacontane (d), and 2,4-Di-tert-butyl phenol (e). Promotion of seed germination was done in quintuplet using 50 μg of the pure compound; experiments were repeated at least three times.
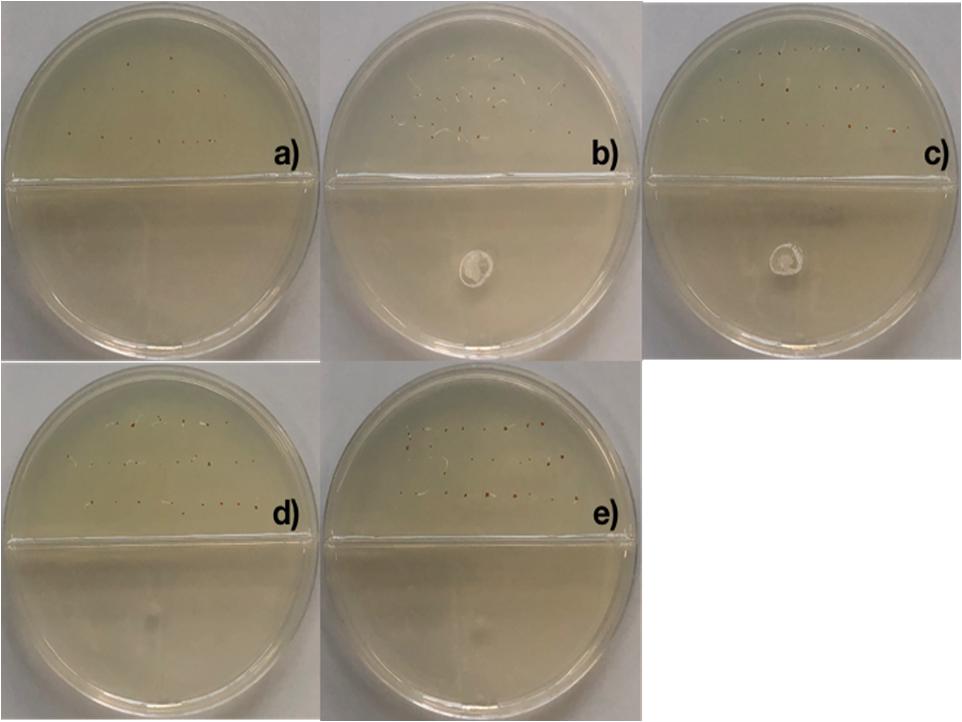
Figure 4. Promotion of seed germination by tetracosane, heneicosane, hentriacontane and 2,4-Di-tert-butyl phenol under Cr-stress. Arabidopsis thaliana seeds were imbibed 120 h at 28°C in the dark on aqueous agar plate containing 100 mg L–1 of chromium(VI) while exposed to vapor of benzene as negative control (a), tetracosane (b), hentriacontane (c), heneicosane (d), and 2,4-Di-tert-butyl phenol (e). Promotion of seed germination was done using 25 seeds per plate, each plate added with 50 μg of the pure compound; experiments were repeated at least three times.
The growth stimulation of Prosopis laevigata seedlings subjected to chromium toxicity was determined (Figure 5). The root length obtained with heneicosane (C21H44), tetracosane (C24H50) and hentriacontane (C31H64) were significantly higher than those of 2,4-di-tert-butylphenol but lower than those obtained with volatile emitted by Bacillus sp. MH778713. For the shoot length stimulation, heneicosane (C21H44) and tetracosane (C24H50) were as good inducers as volatiles released by Bacillus sp. MH778713. The growth promotion of Prosopis seedlings by long-chain alkanes and Bacillus sp. MH778713 volatiles was also evaluated without chromium stress (Figure 6). Root and shoot length of seedling exposed to Bacillus sp. MH778713 volatiles, tetracosane, heneicosane, hentriacontane or 2,4-di-tert-butylphenol were greater than those of the seedling control (seeds without any volatile). These results together indicate that heneicosane, 2,4-di-tert-butylphenol, hentriacontane, and tetracosane are growth inducers of Prosopis laevigata seedlings and allow seeds to overcome phytotoxicity imposed by chromium (VI).
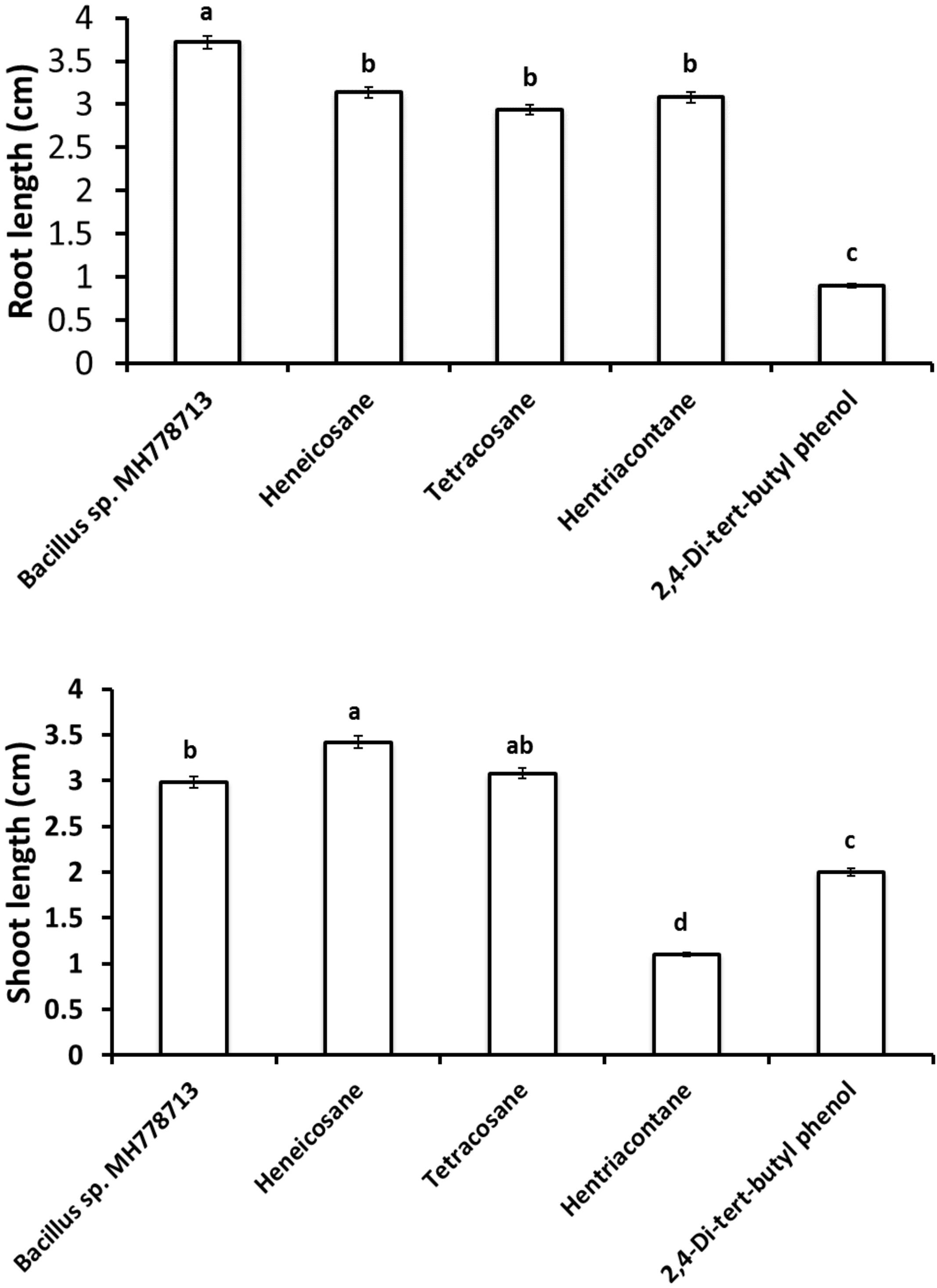
Figure 5. Growth promotion of Prosopis seedling by heneicosane, tetracosane, hentriacontane, 2,4-Di-tert-butyl phenol and volatiles released by Bacillus sp. MH778713 in partitioned Petri plates using 50 μg of pure compound. Prosopis seeds were imbibed 72 h at 28°C in the dark on an aqueous agar plate containing 2500 mg L–1 of chromium(VI) while exposed to volatile compounds. The promotion of seed germination was done in quintuplet; each experiment was repeated at least three times. Different letters indicate significant differences between treatments, according to the least significant difference at P = 0.05. Error bars represent the standard deviation of five measurements.
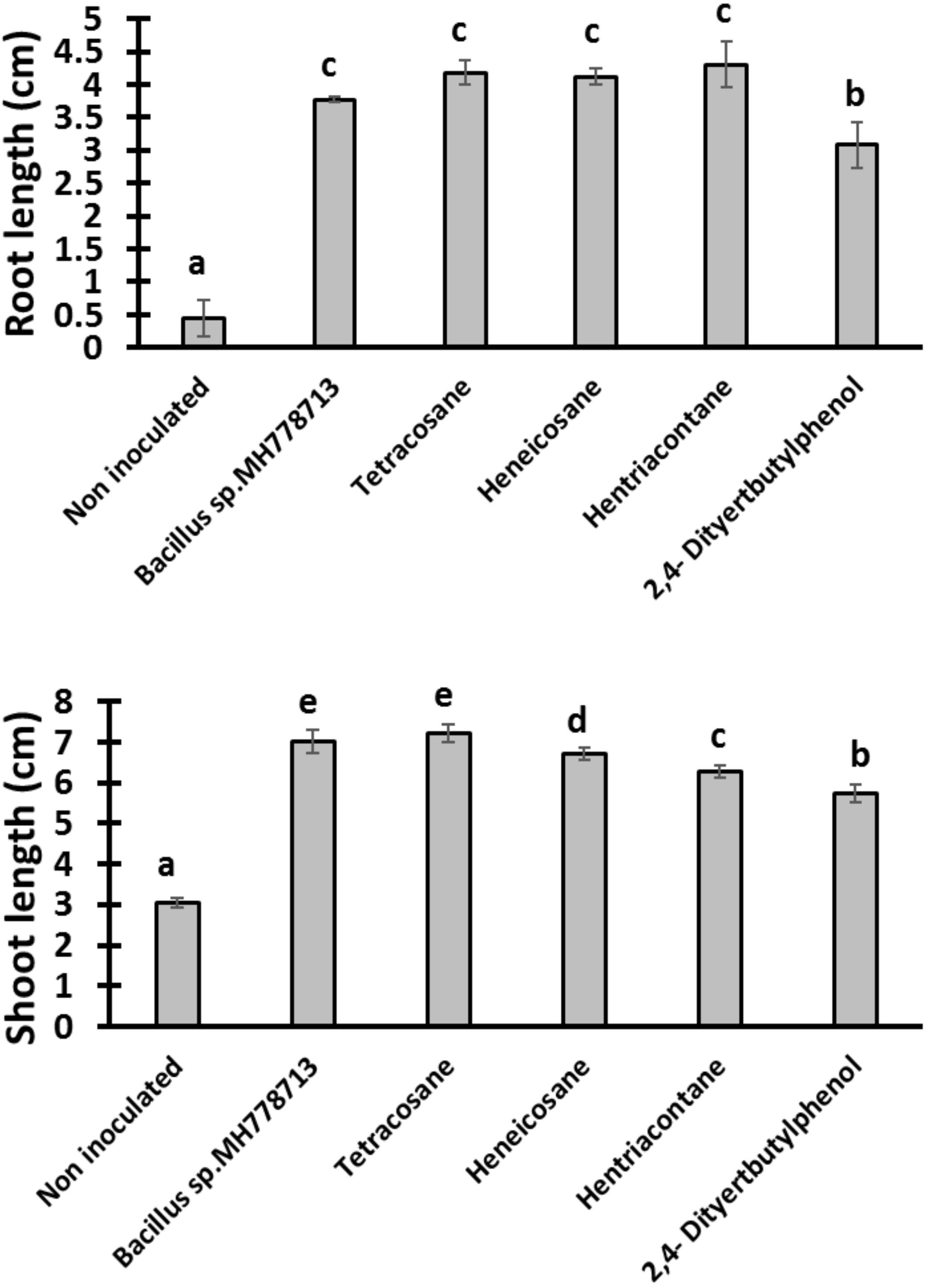
Figure 6. Growth promotion of Prosopis seedling by heneicosane, tetracosane, hentriacontane, 2,4-Di-tert-butyl phenol and volatiles released by Bacillus sp. MH778713 in partitioned Petri plates without chromium using 50 μg of pure compound. Prosopis seeds were imbibed 72 h at 28°C in the dark on aqueous agar plate while exposed to volatile compounds. The promotion of seed germination was done in quintuplet; each experiment was repeated at least three times. Different letters indicate significant differences between treatments, according to the least significant difference at P = 0.05. Error bars represent the standard deviation of five measurements.
Discussion
In this work, we established the ability of Bacillus sp. MH778713 to produce volatiles which in turn triggered the breaking of seed dormancy under chromium stress condition. Plant growth-promoting rhizobacteria release a blend of volatile compounds such as 2,3-butanediol, 1,3-propanediol, indole, acetoin, 2-nonanone, 2-undecanone, 2-tridecanone, hexadecane, and other hydrocarbons, ketones, alcohols, terpenes, acids, and sulfurs that can stimulate plant growth, control plant pathogens, and induce systemic disease resistance (Ryu et al., 2003; Audrain et al., 2015; Fincheira et al., 2017). Different environmental signals can induce or preclude seed germination. A dormancy state can prevent imbibed viable seeds from germinating under apparently favorable conditions as an adaptive strategy (Arc et al., 2013; Nishimura et al., 2018). Heavy metals, for example, trigger signals (increasing ABA concentrations) that preclude seed germination inducing a dormancy state. Although seed coat provides protection, some metals can permeate into the seed before germination or diffuse into the embryo upon seed-coat cracking leading to alterations into nitrogen and carbohydrate metabolism, water uptake, oxidative stress, and seed germination (Kranner and Colville, 2011; Yıldız and Terzi, 2016; Kaszycki et al., 2018). Elevated Cr(VI) concentrations (75–100 mg kg–1 soil) inhibit seed germination, reduce chlorophyll contents and affect most of the growth parameters of biofuel plant species (Amin et al., 2019). In this work, 2500 mg L–1 of Cr(VI) (∼1980 mg kg–1 soil) was required to prevent Prosopis laevigata germination, highlighting the heavy metal tolerance of Prosopis. The high Cr concentration tested (2500 mg L–1) is 19-fold higher than those found in polluted soils (Amin et al., 2019); showing the bioremediation potential of using P. laevigata in combination with Bacillus sp. MH778713 for soil restoration of sites contaminated with Cr. Since the Cr-tolerant Bacillus sp. MH778713 is also highly tolerant to aluminum (up to 10000 mg L–1), it could be expected that the system P. laevigata-Bacillus sp. MH778713 could also work in aluminum polluted sites (Ramírez et al., 2019). The deleterious and signaling role of ROS in plants is well documented, thus ROS fine-tuning plays a key role in seed physiology (Bailly et al., 2008). In our experiments, elevated ROS generated by seeds subjected to Cr stress would likely prevent seed germination by repressing hormonal signaling pathways involved in the alleviation of dormancy. However, further analysis will be required to elucidate the mechanisms of Cr(VI) preventing seed germination.
Volatiles emitted by Bacillus sp. MH778713 allowed P. laevigata seeds to overcome Cr(VI) toxicity, breaking seed dormancy while developing healthy sprouts (Figure 1). Since the medium of Bacillus cultures was alkalinized during germination assays, probably by the release of ammonia, ammonia could probably be involved in the beneficial observed effect; nonetheless, bacterial ammonia can also cause plant growth inhibition (Weise et al., 2013). More experiments should be done to determine the contribution of ammonia in the growth of Prosopis seedlings. Although VOCs effect on seed subjected to chromium stress has not been studied yet, the promoting plant growth effect of VOCs through signaling networks of cytokinins, brassinosteroids, auxin, and gibberellins is well established (Ryu et al., 2003, 2005; Zhang et al., 2007, 2008; Lee et al., 2012; Park et al., 2013). Of most abundant volatiles produced by Bacillus sp. MH778713 at 24 h in partitioned Petri dishes, four, heneicosane, hentriacontane, tetracosane, and 2,4-di-tert-butylphenol, were tested as pure compounds and found to break the dormancy of Prosopis seeds subjected to Cr(VI) phytotoxicity (Figures 3, 4). Although the mechanism of long-chain bacterial compounds underlying the observed seed germination under Cr-stress remains to be elucidated, the ability of long-chain hydrocarbons to elicit plant defense against pathogens in Arabidopsis is already known. Tridecane and hexadecane significantly up-regulate pathogenesis-related gene 1 (PR1) for salicylic acid signaling decreasing sensitivity to biotrophic pathogen infection (Lee et al., 2012; Park et al., 2013).
Bacillus sp. MH778713 was found to produce long-chain hydrocarbons such as C34, C31, C28, C25, C21, and C20 alkanes that likely are VOCs influencing the seed (Figure 2). However, bacterial volatile compounds are considered odorous molecules with low molecular weight (<300 Da) and high vapor pressure (0.01 kPa at 20°C) that easily become vapors (Audrain et al., 2015). The two most abundant volatiles released by PGPR with the characteristics described above is acetoin and 2,3-butanediol. Indeed, Bacillus subtilis GB03 and Bacillus amyloliquefaciens IN937a produce more than thirty low molecular-weight bacterial volatiles (Lee et al., 2012). Nonetheless, other studies have found high molecular weight VOCs produced by PGPR. Pseudomonas brassicacearum and Pseudomonas putida for example, release long-chain hydrocarbons like triacontane (C30H62), octacosane (C28H58), heptacosane (C27H56), and heneicosane (C21H44) with molecular weight as high as 422 g/mol and vapor pressure as low as 3.6 × 10–12 kPa and still being detected in the headspace of agar slant tubes by solid-phase micro-extraction (SPME) method (Giorgio et al., 2015). So, finding long-chain alkanes in our results was not surprising since the quantity and identity of volatile blends produced by rhizobacteria vary significantly among species (Ryu et al., 2003; Lee et al., 2012; Farag et al., 2013; Fincheira et al., 2017; Giorgio et al., 2015). The release of long-chain alkanes (C31, C28, C27, and C21) by rhizobacteria isolated from common bean was previously reported (Giorgio et al., 2015), but the biological role of long-chain alkanes was not further assessed. Although the method used in this work failed to detect long-chain alkanes in the headspace of Bacillus cultures, and solid-phase micro-extraction should be used instead, the results presented herein provide evidence that Bacillus sp. MH778713 emits long-chain bacterial compounds (heneicosane, hentriacontane, tetracosane) that can prime signaling pathways related to abiotic stress tolerance to chromium. From the germination assays with pure compounds, it was suggested that 2,4-di-tert-butyl phenol, heneicosane, hentriacontane, and tetracosane can be volatiles but a pre-concentration system like solid-phase micro-extraction will be required to detect them in the headspace of Bacillus sp. MH778713 cultures (Povolo and Contarini, 2003; Zscheppank et al., 2014). Further works will be required to decipher the molecular targets within seeds sensing the metabolites signals emitted by Bacillus in order to elucidate a potential mechanism by which these compounds break seed dormancy.
Conclusion
Overall, the results provide new evidence that C31, C24, C21 hydrocarbon, hentriacontane, tetracosane, and heneicosane emitted from Bacillus sp. MH778713 can help seeds to overcome chromium toxicity that otherwise prevent seed germination.
Data Availability Statement
All datasets generated for this study are included in the article/Supplementary Material.
Author Contributions
VR, J-AM, and AB conceived, designed, and directed the project, contributed to the interpretation of the results, and designed the figures. VR worked out almost all the technical details, contributed to sample preparation, carried out the experiments, and performed the numerical calculations for the suggested experiments. VR, LC, JM-R, and RP participated in the acquisition of the data. RP, JM-R, and AB supervised the work. VR, LC, and RP manufactured the samples and performed the GC-MS analysis. AB and J-AM took the lead in the writing of the manuscript. All authors provided critical feedback, helped to shape the research, analysis, and manuscript discussed the results and contributed to the final version of the manuscript.
Funding
This work was supported by VIEP-BUAP-2018 ID00483 and CONACyT 252847 Research Grant. VR is grateful for grant number 273291 from CONACyT.
Conflict of Interest
The authors declare that the research was conducted in the absence of any commercial or financial relationships that could be construed as a potential conflict of interest.
Supplementary Material
The Supplementary Material for this article can be found online at: https://www.frontiersin.org/articles/10.3389/fmicb.2020.00741/full#supplementary-material
FIGURE S1 | Promotion of seed germination by 5 μg of tetracosane, heneicosane, hentriacontane and 2,4-Di-tert-butyl phenol under Cr-stress. Prosopis laevigata seeds were imbibed 72 h at 28°C in the dark on aqueous agar plate containing 2500 mg L−1 of chromium(VI) while exposed to vapor of benzene as negative control (a), Bacillus sp. MH778713 (b), tetracosane (c), heneicosane (d), hentriacontane (e), and 2,4-Di-tert-butyl phenol (f). Promotion of seed germination was done in quintuplet using 5 μg of the pure compound; experiments were repeated at least three times.
FIGURE S2 | Metabolite profile of Bacillus sp. MH778713 grown alone at 24 h of incubation in YMA sealed plate.
FIGURE S3 | Metabolite profile of Escherichia coli BL21 (DE3) grown in co-culture with Prosopis seed at 24 h of incubation in YMA sealed plate.
TABLE S1 | Kovats retention indices. RT, retention time; KI exp., experimental Kovats index; KI Pherobase, Kovats index from pheromone database. NA, not available.
References
Akinci, I., and Akinci, S. (2010). Effect of chromium toxicity on germination and early seedling growth in melon (Cucumis melo L.). Afr. J. Biotechnol. 929, 4589–4594.
Aldrich, M. V., Gardea-Torresdey, J. L., Peralta-Videa, J. R., and Parsons, J. G. (2003). Uptake and reduction of Cr (VI) to Cr (III) by mesquite (Prosopis spp.): chromate–plant interaction in hydroponics and solid media studied using XAS. Environ. Sci. Technol. 37, 1859–1864. doi: 10.1021/es0208916
Aldrich, M. V., Peralta-Videa, J. R., Parsons, J. G., and Gardea-Torresdey, J. L. (2007). Examination of arsenic (III) and (V) uptake by the desert plant species mesquite (Prosopis spp.) using X-ray absorption spectroscopy. Sci. Total Environ. 379, 249–255. doi: 10.1016/j.scitotenv.2006.08.053
Amin, H., Ahmed, A. B., Abbasi, M. S., Amin, F., Jahangir, T. M., and Soomro, N. U. (2019). Evaluation of chromium phyto-toxicity, phyto-tolerance, and phyto-accumulation using biofuel plants for effective phytoremediation. Int. J. Phytoremediation. 21, 352–363. doi: 10.1080/15226514.2018.1524837
Arc, E., Sechet, J., Corbineau, F., Rajjou, L., and Marion-Poll, A. (2013). ABA crosstalk with ethylene and nitric oxide in seed dormancy and germination. Front. Plant. Sci. 4:63. doi: 10.3389/fpls.2013.00063
Audrain, B., Farag, M. A., Ryu, C. M., and Ghigo, J. M. (2015). Role of bacterial volatile compounds in bacterial biology. FEMS Microbiol. Rev. 39, 222–233. doi: 10.1093/femsre/fuu013
Backer, R., Rokem, J. S., Ilangumaran, G., Lamont, J., Praslickova, D., Ricci, E., et al. (2018). Plant growth-promoting rhizobacteria: context, mechanisms of action, and roadmap to commercialization of biostimulants for sustainable agriculture. Front. Plant Sci. 9:1473. doi: 10.3389/fpls.2018.01473
Bailly, C., El-Maarouf-Bouteau, H., and Corbineau, F. (2008). From intracellular signaling networks to cell death: the dual role of reactive oxygen species in seed physiology. C. R. Biol. 331, 806–814. doi: 10.1016/j.crvi.2008.07.022
Bitas, V., Kim, H., Bennett, J. W., and Kang, S. (2013). Sniffing on microbes: diverse roles of microbial volatile organic compounds in plant health. Mol. Plant Microbe Interac. 26, 835–843. doi: 10.1094/mpmi-10-12-0249-cr
Bruno, L. B., Karthik, C., Ma, Y., Kadirvelu, K., Freitas, H., and Rajkumar, M. (2020). Amelioration of chromium and heat stresses in Sorghum bicolor by Cr6 + reducing thermotolerant plant growth promoting bacteria. Chemosphere 244:125521. doi: 10.1016/j.chemosphere.2019.125521
Bücker-Neto, L., Paiva, A. L. S., Machado, R. D., Arenhart, R. A., and Margis-Pinheiro, M. (2017). Interactions between plant hormones and heavy metals responses. Genet. Mol. Biol. 40, 373–386. doi: 10.1590/1678-4685-gmb-2016-0087
Din, B. U., Amna Rafique, M., Javed, M. T., Kamran, M. A., Mehmood, S., Khan, M., et al. (2020). Assisted phytoremediation of chromium spiked soils by Sesbania Sesban in association with Bacillus xiamenensis PM14: a biochemical analysis. Plant Physiol. Biochem. 146, 249–258. doi: 10.1016/j.plaphy.2019.11.010
Dube, B. K., Tewari, K., Chatterjee, J., and Chatterjee, C. (2003). Excess chromium alters uptake and translocation of certain nutrients in citrullus. Chemosphere 53, 1147–1153. doi: 10.1016/s0045-6535(03)00570-8
Farag, M. A., Zhang, H., and Ryu, C. M. (2013). Dynamic chemical communication between plants and bacteria through airborne signals: induced resistance by bacterial volatiles. J. Chem. Ecol. 39, 1007–1018. doi: 10.1007/s10886-013-0317-9
Fincheira, P., Parada, M., and Quiroz, A. (2017). Volatile organic compounds stimulate plant growing and seed germination of Lactuca sativa. J. Soil Sci. Plant Nutr. 17, 853–867. doi: 10.4067/s0718-95162017000400002
Fincheira, P., and Quiroz, A. (2018). Microbial volatiles as plant growth inducers. Microbiol. Res. 208, 63–75. doi: 10.1016/j.micres.2018.01.002
Giorgio, A., De Stradis, A., Lo Cantore, P., and Iacobellis, N. S. (2015). Biocide effects of volatile organic compounds produced by potential biocontrol rhizobacteria on Sclerotinia sclerotiorum. Front. Microbiol. 6:1056. doi: 10.3389/fmicb.2015.01056
Graeber, K., Nakabayashi, K., Miatton, E., Leubner-Metzger, G., and Soppe, W. J. (2012). Molecular mechanisms of seed dormancy. Plant Cell Environ. 35, 1769–1786. doi: 10.1111/j.1365-3040.2012.02542.x
Gutierrez-Luna, F. M., López-Bucio, J., Altamirano-Hernández, J., Valencia-Cantero, E., Reyes de la Cruz, H., and Macías-Rodríguez, L. (2010). Plant growth-promoting rhizobacteria modulate root-system architecture in Arabidopsis thaliana through volatile organic compound emission. Symbiosis 51, 75–83. doi: 10.1007/s13199-010-0066-2
Jain, R., Singh, S. P., Singh, A., Singh, S., Tripathi, P., Chandra, A., et al. (2016). Study on physio-biochemical attributes and metallothionein gene expression affected by chromium(VI) in sugarcane (Saccharum spp. hybrid). J. Environ. Biol. 37, 375–382.
Jayaram, K., and Prasad, M. N. V. (2009). Removal of Pb (II) from aqueous solution by seed powder of Prosopis juliflora D.C. J. Hazard Mater. 169, 991–997. doi: 10.1016/j.jhazmat.2009.04.048
Kaszycki, P., Dubicka-Lisowska, A., Augustynowicz, J., Piwowarczyk, B., and Wesołowski, W. (2018). Callitriche cophocarpa (water starwort) proteome under chromate stress: evidence for induction of a quinone reductase. Environ. Sci. Pollut. Res. Int. 25, 8928–8942. doi: 10.1007/s11356-017-1067-y
Kranner, I., and Colville, L. (2011). Metals and seeds: biochemical and molecular implications and their significance for seed germination. Environ. Exp. Bot. 72, 93–105. doi: 10.1016/j.envexpbot.2010.05.005
Lee, B., Farag, M. A., Park, H. B., Kloepper, J. W., Lee, S. H., and Ryu, C. M. (2012). Induced resistance by a long-chain bacterial volatile: elicitation of plant systemic defense by a C13 volatile produced by Paenibacillus polymyxa. PLoS One 7:e48744. doi: 10.1371/journal.pone.0048744
Li, Z., Gao, Y., Zhang, Y., Lin, C., Gong, D., Guan, Y., et al. (2018). Reactive oxygen species and gibberellin acid mutual induction to regulate tobacco seed germination. Front. Plant. Sci. 9:1279. doi: 10.3389/fpls.2018.01279
Liu, X. M., and Zhang, H. (2015). The effects of bacterial volatile emissions on plant abiotic stress tolerance. Front. Plant. Sci. 6:774. doi: 10.3389/fpls.2015.00774
Munzuroğlu, O., Zengin, F. K., and Yahyagil, Z. (2008). The abscisic acid levels of wheat (Triticum aestivum L. cv. Çakmak 79) seeds that were germinated under heavy metal (Hg + +, Cd + +, Cu + +) stress. G.U. J. Sci. 21, 1–7.
Nishimura, N., Tsuchiya, W., Moresco, J. J., Hayashi, Y., Satoh, K., Kaiwa, N., et al. (2018). Control of seed dormancy and germination by DOG1-AHG1 PP2C phosphatase complex via binding to heme. Nat. Commun. 9:2132.
Panda, S. K., and Choudhury, S. (2005). Chromium stress in plants. Braz. J. Plant Physiol. 17, 95–102. doi: 10.1590/s1677-04202005000100008
Park, H. B., Lee, B., Kloepper, J. W., and Ryu, C. M. (2013). One shot-two pathogens blocked: exposure of Arabidopsis to hexadecane, a long chain volatile organic compound, confers induced resistance against both Pectobacterium carotovorum and Pseudomonas syringae. Plant Signal. Behav. 8:e24619. doi: 10.4161/psb.24619
Povolo, M., and Contarini, G. (2003). Comparison of solid-phase microextraction and purge-and trap methods for the analysis of the volatile fraction of butter. J. Chromatogr A. 985, 117–125. doi: 10.1016/s0021-9673(02)01395-x
Purwanti, I. F., Putri, T. P., and Kurniawan, S. B. (2017). Treatment of chromium contaminated soil using bioremediation. AIP Conf. Proc. 1903:040008. doi: 10.1063/1.5011527
Ramírez, V., Baez, A., López, P., Bustillos, R., Villalobos, M. A., Carreño, R., et al. (2019). Chromium hyper-tolerant Bacillus sp. MH778713 assists phytoremediation of heavy metals by mesquite trees (Prosopis laevigata). Front. Microbiol. 10:1833. doi: 10.3389/fmicb.2019.01833
Ryu, C. M., Farag, M. A., Hu, C. H., Reddy, M. S., Kloepper, J. W., and Paré, P. W. (2004). Bacterial volatiles induce systemic resistance in Arabidopsis. Plant Physiol. 134, 1017–1026. doi: 10.1104/pp.103.026583
Ryu, C. M., Farag, M. A., Hu, C. H., Reddy, M. S., Wei, H. X., Paré, P. W., et al. (2003). Bacterial volatiles promote growth in Arabidopsis. Proc. Natl. Acad. Sci. U.S.A. 100, 4927–4932. doi: 10.1073/pnas.0730845100
Ryu, C. M., Hu, C. H., Locy, R. D., and Kloepper, J. W. (2005). Study of mechanisms for plant growth pro-motion elicited by rhizobacteria in Arabidopsis thaliana. Plant Soil 92, 268–285.
Sharifi, R., and Ryu, C. M. (2018). Revisiting bacterial volatile-mediated plant growth promotion: lessons from the past and objectives for the future. Ann. Bot. 122, 349–358. doi: 10.1093/aob/mcy108
Shreya, D., Jinal, H. N., Kartik, V. P., and Amaresan, N. (2020). Amelioration effect of chromium-tolerant bacteria on growth, physiological properties and chromium mobilization in chickpea (Cicer arietinum) under chromium stress. Arch. Microbiol. doi: 10.1007/s00203-019-01801-1801
Vacheron, J., Desbrosses, G., Bouffaud, M. L., Touraine, B., Moënne-Loccoz, Y., Muller, D., et al. (2013). Plant growth-promoting rhizobacteria and root system functioning. Front. Plant Sci. 4:356. doi: 10.3389/fpls.2013.00356
Vishal, B., and Kumar, P. P. (2018). Regulation of seed germination and abiotic stresses by gibberellins and abscisic acid. Front. Plant Sci. 9:838. doi: 10.3389/fpls.2018.00838
Wang, Y., Wang, Y., Kai, W., Zhao, B., Chen, P., Sun, L., et al. (2014). Transcriptional regulation of abscisic acid signal core components during cucumber seed germination and under Cu2 +, Zn2 +, NaCl and simulated acid rain stresses. Plant Physiol. Biochem. 76, 67–76. doi: 10.1016/j.plaphy.2014.01.003
Weise, T., Kai, M., and Piechulla, B. (2013). Bacterial ammonia causes significant plant growth inhibition. PLoS One 8:e63538. doi: 10.1371/journal.pone.0063538
Yıldız, M., and Terzi, H. (2016). Proteomic analysis of chromium stress and sulfur deficiency responses in leaves of two canola (Brassica napus L.) cultivars differing in Cr(VI) tolerance. Ecotoxicol. Environ. Saf. 124, 255–266. doi: 10.1016/j.ecoenv.2015.10.023
Zhang, H., Kim, M. S., Krishnamachari, V., Payton, P., Sun, Y., Crimson, M., et al. (2007). Rhizobacterial volatile emissions regulate auxin homeostasis and cell expansion in Arabidopsis. Planta 226, 839–851. doi: 10.1007/s00425-007-0530-2
Zhang, H., Kim, M. S., Sun, Y., Dowd, S. E., Shi, H., and Paré, P. W. (2008). Soil bacteria confer plant salt tolerance by tissue-specific regulation of the sodium transporter HKT1. Mol. Plant Microbe Interact. 21, 737–744. doi: 10.1094/mpmi-21-6-0737
Zhang, H., Murzello, C., Sun, Y., Kim, M. S., Xie, X., Jeter, R. M., et al. (2010). Choline and osmotic-stress tolerance induced in Arabidopsis by the soil microbe Bacillus subtilis (GB03). Mol. Plant Microbe Interact. 23, 1097–1104.
Keywords: bacterial volatile, seed dormancy, chromium stress, growth promotion, Prosopis laevigata
Citation: Ramírez V, Munive J-A, Cortes L, Muñoz-Rojas J, Portillo R and Baez A (2020) Long-Chain Hydrocarbons (C21, C24, and C31) Released by Bacillus sp. MH778713 Break Dormancy of Mesquite Seeds Subjected to Chromium Stress. Front. Microbiol. 11:741. doi: 10.3389/fmicb.2020.00741
Received: 10 September 2019; Accepted: 30 March 2020;
Published: 24 April 2020.
Edited by:
Robin Tecon, ETH Zürich, SwitzerlandReviewed by:
Anca Delgado, Arizona State University, United StatesMarie Chantal Lemfack, University of Rostock, Germany
Copyright © 2020 Ramírez, Munive, Cortes, Muñoz-Rojas, Portillo and Baez. This is an open-access article distributed under the terms of the Creative Commons Attribution License (CC BY). The use, distribution or reproduction in other forums is permitted, provided the original author(s) and the copyright owner(s) are credited and that the original publication in this journal is cited, in accordance with accepted academic practice. No use, distribution or reproduction is permitted which does not comply with these terms.
*Correspondence: Antonino Baez, antonino.baez@correo.buap.mx