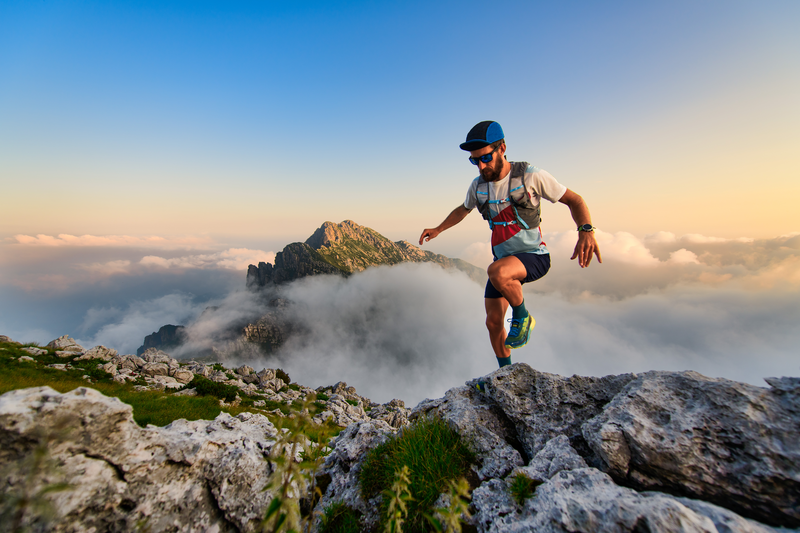
95% of researchers rate our articles as excellent or good
Learn more about the work of our research integrity team to safeguard the quality of each article we publish.
Find out more
ORIGINAL RESEARCH article
Front. Microbiol. , 19 February 2020
Sec. Extreme Microbiology
Volume 11 - 2020 | https://doi.org/10.3389/fmicb.2020.00241
This article is part of the Research Topic Mining, Designing, Mechanisms and Applications of Extremophilic Enzymes View all 16 articles
A thermo-activation and thermostable laccase isoenzyme (Lac 37 II) produced by Trametes trogii S0301 at 37°C was purified to apparent homogeneity by anionic exchange chromatography and sephadex G-75 chromatography, with 12.3% of yeiled and a specific activity of 343.1 U mg–1. The molecular weight of the purified Lac 37 II was estimated to be approximately 56 kDa in 12% sodium dodecyl sulfate polyacrylamide gel electrophoresis (SDS-PAGE). The optimal pH and temperature for the protein was 2.7 and 60°C, respectively. The purified Lac 37 II showed higher resistance to all tested metal ions and organic solvents except for Fe2+ and Cd2+ at 37°C and the activity of the purified Lac 37 was significantly enhanced by Cu2+ at 50 mM. The Kcat, Km, and Kcat/Km of Lac 37 II were 2.977 s–1, 16.1 μM, and 184.9 s–1 μM–1, respecively, in the condition of pH 2.7 and 60°C using ABTS as a substrate. Peptide-mass fingerprinting analysis showed that the Lac 37 II matched to the gene-deduced sequences of lcc3 in T. trogii BAFC 463, other than Lcc1, Lcc 2, and Lcc 4. Compared with laccase prepared at 28°C, the onset of thermo-activation of Lac 37 II activity occurred at 30°C with an increase of 10%, and reached its maximum at the temperatures range of 40–60°C with an increase of about 40% of their original activity. Furthermore, Lac 37 II showed the efficient decolorization ability toward triphenylmethane dyes at 60°C, with decolorization rates of 100 and 99.1% for 25 mg L–1 malachite and crystal violet in 5 h, respectively, when hydroxybenzotriazole (HBT) was used as a mediator. In conclusion, it is the first time to report a thermo-activation laccase from a thermophilic T. trogii strain, which has a better enzyme property and higher decolorization ability among fungal laccases, and it also has a further application prospective in the field of biotechnology.
Laccases (EC1.10.3.2) are a group of copper-containing polyphenol oxidases that are known as “blue enzymes” for green chemistry due to their ability of oxidize diverse substrates which are similar to lignin or the degradation products of major lignin with molecular oxygen as the final electron acceptor. Due to their high catalytic efficiency and broad substrate specificity, laccases are used in various fields, including biopulping, delignification, biobleaching, environmental pollutants bioremediation, dye decolorization, etc. (Riva, 2006; Bertrand et al., 2017; Younes et al., 2019).
Fungi, especially white rot fungi, are the main laccase producers in nature, and the potential laccase producing strains be utilized in industrial application (Bertrand et al., 2017). Until now, many laccase-producing fungi have been studied and most of the fungi can produce several laccase isozymes (usually more than 10 isoenzymes in the same fungus strain) that showed different kinetic and physicochemical features, which makes it possible to seek new laccase isoenzymes and meets the demands in the industrial applications (Janusz et al., 2013; Zhuo et al., 2016; Zheng et al., 2017). However, the expression of different laccase isoenzymes in the same strain depends on many factors such as the presence of inducers (especially Cu2+ and phenolic compounds), the ratio of carbon and nitrogen, age of the culture and heat shock treatment (Baldrian, 2006; Piscitelli et al., 2011; Janusz et al., 2013; Zhuo et al., 2016; Bertrand et al., 2017). Untill now, few laccase isoenzymes have been isolated and characterized, usually one or two isoenzymes per fungus strain, and most of the laccases isoenzymes isolated so far are found sensitive to extreme conditions of temperature, pH, metal ions, etc. (Janusz et al., 2013; Fonseca et al., 2015; Jaiswal et al., 2015; Othman et al., 2018).
Laccase isoenzymes of thermophilic bacteria and fungi usually possess many attractive properties including high thermal stability, thermo-activation (stimulation of enzyme activity by pre-incubation), and tolerance to organic solvents and ionic concentrations (Hildén et al., 2007; Younes and Sayadi, 2011; Yan et al., 2014a, b), which are demanded biobleaching of pulp and treatment of colored industrial effluents (Wong et al., 2000; Asgher et al., 2008). Previous reports have found that increasing the temperature for laccase production (Tong et al., 2007) and heat shock treatment (Wang et al., 2012) in Trametes strains can induce the expression of different laccase isoenzymes and enhance laccase activity. In our experiment, we observed that T. trogii S0301 strain can grow at 37°C, but to date, only two native laccase isoenzymes (named Lcc1 and Lcc2) have been purified from this strain, and both of them were obtained from the supernatants cultured at 28°C (Colao et al., 2003; Yan et al., 2014a).
Thermo-active enzymes usually are more thermotolerant (Rathi et al., 2000; Hildén et al., 2007; Younes and Sayadi, 2011; Campos et al., 2016). Although the first thermo-active laccase was isolated as early as 1993, few thermo-active laccase isoenzymes have been isolated until now, such as laccase isoenzymes from Fomes sclerodermeus, T. hirsutus, Coliolus zonatu, Marasmius quercophilus, Myceliophtora thermophile, and Scytalidium thermophilum (Hildén et al., 2007; Younes and Sayadi, 2011). Thermo-activation has been observed in the heterogeneous expressed LCC3 of T. trogii BAFC 463 in Pichia pastoris (named the recombined LCC3) (Campos et al., 2016), but there are no reports about the thermo-active laccase isoenzyme originated from Trametes until now.
To explore the potential application of laccases in the thermotolerant T. trogii S0301 strain, laccase was obtained from this strain cultured at the temperature of 37°C. The main objectives of current study were (i) to purify and identify the laccase of T. trogii S0301 produced at 37°C; (ii) to characterize this laccase isoenzyme; and (iii) to assess the potential application of this laccase isoenzyme by dye decolorization experiments.
2,2′-Azino bis (3-ethylbenzothiazoline-6-sulfonic acid) (ABTS) and dyes (malachite green, bromophenol blue, and crystal violet) were purchased from Sigma-Aldrich and Merck, respectively. T. trogii S0301 strain employed in the present study was stored in the strain collection of Laboratory of Bioconversion of Life Science and Technology College, Kunming University of Science and Technology, and maintained on a GYP slant at 4°C (Yan et al., 2014a, b).
Four 1-cm2 plugs of the GYP plates incubated at 28°C for 4 days were excised with a sterilized cutter and added to each 250 mL Erlenmeyer flask containing 50 mL of GYP. After another 5 days incubation, the mycelia were homogenized with glass beads (0.3 mm in diameter) and transferred to GYP broth containing 2 mM Cu2+ with 10% (v/v) of the seed culture broths. The cultures were incubated in a rotary shaker at 200 rpm at 28 and 37°C, respectively. Ten-day-old liquid cultures were obtained by centrifuging (8000 rpm, 15 min) and the cell-free supernatants were designated as the crude enzyme for the further study.
Laccase activity was determined with ABTS as the substrate. The 1.5 mL substrate solution includes 2 mM ABTS, 100 mM phosphate citrate buffer (pH 4.0), and 0.1 mL appropriately diluted crude or purified enzyme was used to determine the activity. The increase in absorbance was monitored at 420 nm for 3 min. One unit of the enzyme activity was defined as the amount of the enzyme that oxidized 1 μmol of the ABTS per minute according to the methods described by Yan et al. (2014b).
For thermo-activation analysis, the enzymes were pretreated at different temperatures (30–80°C) for 30 min in 100 mM phosphate citrate buffer (pH 4.0), and then thoroughly cooled on ice for another 30 min. Next, the residual laccase activity was determined. The same amount of enzymes that were not heat-treated but placed on ice as positive control, and the heat-denatured enzymes were served as the negative control. All the assays were carried out in triplicate.
The purification of the laccase from the crude enzyme of 10-day-old liquid cultures under 37°C with the addition of 2 mM Cu2+ was carried out as described in Yang et al. (2011). Briefly, the total protein was precipitated from the crude enzyme using ammonium sulfate (80% saturation). After dialysis, the enzyme solution was successively treated with a Q SepharoseTM ion-exchange chromatography column (GE Healthcare) and a Sephadex G-75 Medium chromatography (Biotopped) column. The fraction containing laccase activity was collected and stored at −20°C for further studies. Sodium dodecyl sulfate-polyacrylamide gel electrophoresis (SDS-PAGE) and native sodium dodecyl sulfate-polyacrylamide gel electrophoresis (Native-PAGE) were carried out according to Shi et al. (2014).
The purified laccase was further separated by Native-PAGE. After the electrophoresis, the gel was stained with citrate-phosphate buffer (100 mM, pH 4.0) containing 1.0 mM ABTS and the laccase band was collected for further study. MALDI-TOF/TOF-MS analysis used commercial service provided by Sangon Biotech on 4800 Plus MALDI TOF/TOFTM Analyzer (ABI, Foster City, CA, United States). Mass spectra were obtained in positive ions regime using reflectron. The program Mascot1 was used for protein identification by “peptides fingerprints” and fragmentation spectra. The database NCBI was used for searching homology among proteins of all organisms and fungi with the accuracy mentioned taking into account possible methionine oxidation by atmospheric oxygen and possible modification of cysteine by acrylamide (Younes and Sayadi, 2011; Zheng et al., 2017).
Characterization analysis of the purified laccase was carried out according to Yan et al. (2014b). To determine the optimum pH of the purified laccase, the laccase activity was assayed in 100 mM citrate-phosphate buffer adjusted to various pH values between 2.0 and 8.0, with 1.0 intervals at 30°C. Effect of pH on the stability of the purified laccase was studied by verifying the remaining activity after incubating the purified enzyme in the buffer solutions mentioned above at 30°C for 36 h. Next, the residual laccase activity of each treatment was compared with the control under the standard assay conditions. All the experiments were performed in triplicate.
To determine the optimum pH of the purified laccase, enzymatic reaction was conducted at temperatures from 30 to 80°C with the enzyme in 100 mM citrate-phosphate buffer (pH 4.0), with 10°C intervals (Wu et al., 2010). For the thermostability analysis, the half-life at certain temperature (T1/2) was determined after the purified laccase incubated at given temperature (60, 70, or 75°C) in phosphate citrate buffer (100 mM, pH 4.0) with different time intervals. The residual laccase activity was determined by the standard conditions and the activity of untreated enzyme was used to represent 100% relative activity. All the experiments were performed in triplicate.
Metal ions including Na+, Fe2+, Cd2+, Mn2+, Zn2+, Mg2+, Co2+, and Cu2+ were added to the standard laccase reaction mixture with a final concentration of 5 and 100 mM, and the residual activities were measured under the standard conditions (Shi et al., 2014). The laccase activity of the reaction mixture without metal ions was recorded as 100%. All the experiments were performed in triplicate.
To determine the effects of organic solvents on laccase activity, commonly used solvents including methanol, ethanol, and acetonitrile were added to the standard laccase reaction mixture with a final concentration of 1, 5, or 10% (v/v) and the residual activities were measured under the standard conditions (Shi et al., 2014). The laccase activity of the reaction mixture without organic solvent was recorded as 100%. All the experiments were performed in triplicate.
The kinetic constants (Km and Kcat) were determined by using ABTS as substrate in series concentrations ranged from 0 to 2 mM at the optimal condition of the purified laccase in 100 mM citrate–phosphate buffer (pH 2.7) at 60°C. The laccase activity for each substrate concentration was determined three times. The Km and Kcat values were evaluated by the Lineweaver–Burk plot using the Originpro 8 for Windows. All the experiments were performed in triplicate.
Decolorization experiments were carried out according to Yan et al. (2014a, b). The dye decolorization reaction was conducted at 60°C with or without the addition of hydroxybenzotriazole (HBT) as a laccase mediator (Younes et al., 2007). Decolorization efficiency was calculated according to the following formula: decolorization (%) = A0−At/A0 ∗ 100%, where A0 is the initial absorbance and At is the final absorbance (Younes et al., 2016).
All data were presented as mean ± standard deviation (SD) for three replications for each sample. The ANOVA test using the software of Origin pro 8 for Windows used to analyze the experiment data. P-value < 0.05 was considered significant. In addition, all statistical charts were drawn by Origin pro 8 for Windows. Protein sequence alignment was analyzed by DNAMAN software.
Previously, the thermo-active laccase isoenzymes have been observed in the thermophilic or thermotolerant strains, such as Melanocarpus albomyces, M. thermophila, and S. thermophilum (Kiiskinen et al., 2002; Younes and Sayadi, 2011). The original crude laccase activity of the culture filtrate produced at 37 and 28°C were 3330.62 and 4861.11 U L–1, respectively. We observed that the crude laccase prepared at 37°C showed temperature-dependent activation after 30 min of thermal treatment under the different temperature conditions, while the crude laccase of this strain prepared at 28°C did not show obvious thermo-activation under the same experiment conditions (Figure 1A). The onset of thermo-activation of laccase activity occurred at 30°C with an increase of 10%, and reached its maximum at the temperatures range of 40–60°C with an increase of about 40% of their original activity (Figure 1A). Further raising temperatures caused the loss of the enzyme activity and the disappearance of thermo-activation. Until now, only two laccase isoenzymes (Lcc1 and Lcc2) have been purified from T. trogii strains and neither of them is thermo-active (Yan et al., 2014a; Campos et al., 2016). To further explore whether thermo-activation due to different laccase isoenzymes in the crude enzyme, laccase of this strain produced at 37°C was purified and identified.
Figure 1. Thermo-activated analysis and purification of laccase from T. trogii S0301. Native-page (B) and SDS-page (C) analysis. Lanes 1 and 2 are the crude and purified laccase produced at 37°C, respectively. The crude laccase activity of the culture filtrate produced at 37 and 28°C were 3330.62 and 4861.11 U L–1 (A). Thermo-activated analysis of purified Lac 37 II from 37°C, which the original activity is 343.06 U mg–1 (D). Activity is represented as % relative to the not heat-treated, assigned as 100%. Data points are the average of triplicate measurements, and the error bars represent the standard deviation.
Using Native-PAGE, two main laccase isoenzymes (Lac 37 I and Lac 37 II) were detected from the crude laccase of T. trogii S0301 which produced at 37°C in liquid GYP medium added with 2 mM Cu2+ (Figure 1B). Laccase which secreted in culture medium was purified after ammonium sulfate precipitation. By the first step of the purification, the specific lacase activity of crude laccase was changed from 55.3 to 96.0 U mg–1 (Table 1), followed by the anionic exchange chromatography column and the Sephadex G-75 chromatography column kept in tandem. Six protein peaks were obtained by anionic exchange chromatography column, and the first peak showed the laccase activity (111.9 U mg–1) (Table 1). The protein solution from peak 1 was collected and then purified by Sephadex G-75 chromatography column. A final specific activity of 343.06 U mg–1 was achieved. A purification fold of 6.2 and a total enzyme yield of 9.6% were obtained (Table 1). A single laccase band detected on Native-PAGE indicated that only Lac 37 II was purified in this study. Lac 37 II showed a single band by SDS-PAGE, with a predicted molecular mass of approximately about 60 kDa (Figure 1C). Most studies showed that the molecular weight of fungal laccase monomer is between 50 and 100 kDa, which is consistent with the molecular weight of the purified Lac 37 II (Kunamneni et al., 2008). However, we failed to obtain the other laccase isoenzyme, Lac 37 I, in this study. In order to obtain the Lac 37 I, maybe we should change the purification conditions.
The purified Lac 37 II exhibited obvious temperature-dependent activation at temperatures from 40 to 60°C and reached its maximum at 60°C, with an increase of approximately 45% of the original activity (343.06 U mg–1) (Figure 1D), which was similar to that of the crude enzyme of T. trogii S0301 produced at 37°C (Figure 1A). Thus, we suggest that Lac 37 II is the main source of the thermo-active laccase. In our study, the thermo-activation temperature range was agreed with other thermo-cativation laccase isoenzymes, but a highest increasing rate of laccase activity was observed in Lac 37 II, which have approximately 20% of increase compared to that of Physisporinus rivulosus and S. thermophilum (Hildén et al., 2007; Younes and Sayadi, 2011).
According to the literature, the enzyme properties of many purified and recombined laccases mainly from T. trogii strains and some thermotolerant fungi are summarized in Table 2.
Table 2. Comparison of kinetic properties of the purified laccases mainly from strains of Trametes genus.
Generally, fungal laccases demonstrate their optimal pH of 2.0–6.0 using ABTS as substrate (Si et al., 2013; Shi et al., 2014; Ai et al., 2015). Lac 37 II, in this study, exhibited maximal activity at pH 2.7, which is in accordance with the recombined LCC3 and the purified laccases from T. trogii S0301 produced at 28°C with the optimum of pH 2.7 and 3.0 using ABTS as the substrate, respectively (Figure 2A and Table 2; Yan et al., 2014a; Campos et al., 2016). The original activity of Lac 37 II was stable, maintaining >80%, after incubation at pH 4 and 5 for 36 h (Figure 2B). When the pH was <3, the laccase activity was significantly inhibited, with an activity of 18%. These results are in accordance with other fungal laccases (Shi et al., 2014; Yan et al., 2014b).
Figure 2. Characterization of Lac 37 II. Effect of pH on laccase activity (A) and stability for 36 h (B). Effect of temperature on laccase activity (C) and stability (D). Effect of metal ions (5 or 100 mM) and organic solvent on laccase activity (E,F). Laccase activity that without heat treatment, metal ions, and organic solvent at pH 4.8 was 343.06 U/mg. Activity is represented as % relative to laccase activity under the standard conditions, assigned as 100% for stability studies. The maximum laccase activity was recorded as 100% for the optimal temperature and pH studies. The data are presented as means from three independent measurements ± the standard deviations (indicated by the error bars). *p and #p < 0.05, as determined by one-way ANOVA.
The optimum temperature for Lac 37 II was 60°C with ABTS as a substrate, which was higher than that of the recombined LCC3 with the highest laccase activity at 50°C (Figure 2C and Table 2). The half-lives of enzymatic activity at various temperatures (T1/2) at pH 4.0 were >12 h at 60°C, 2 h at 70°C, and 15 min at 75°C with ABTS as the substrate (Figure 2D). Based on the thermostability assays, Lac 37 II in this study exhibited a notable advantage over almost all laccases from the Trametes genus and other sources (Table 2), except for that of T. versicolor sdu-4 (T1/2 of 132 min at 70°C) (Zhu et al., 2011) and T. pubescens (T1/2 of 120 min at 75°C) (Si et al., 2013).
Kinetic analysis was carried out with ABTS as a substrate at optimal conditions (pH 2.7 and 60°C). The Km, Kcat, and Kcat/Km of Lac 37 II were 16.1 μM, 2977.8 s–1, and 184.9 s–1 μM–1, respectively (Table 2). Lac 37 II in this study possessed higher thermostability and catalytic efficiency, which makes the laccase isoenzyme have further prospective for the biotechnological applications.
Metal ions are widely distributed in environmental pollutants, and laccases with higher resistance to metal ions are thus attractive (Younes and Sayadi, 2011). In this study, the effects of several metal ions (Na+, Fe2+, Cd2+, Mn2+ Zn2+, Mg2+, Co2+, or Cu2+) on Lac 37 II were investigated. The purified Lac 37 II exhibited a high degree of resistance to some metal ions. When the concentration of metal ions was 5 and even 100 mM, Na+, Mn2+, Mg2+, Co2+, and Cu2+ had little effects on the laccase activity. However, the other metal ions such as Fe2+, Cd2+, and Zn2+ showed inhibitory effects on the activity of Lac 37 II, especially Fe2+. Fe2+ completely inhibited the activity of Lac 37 II even at a low concentration (5 mM), and laccase activity decreased to 54.5 and 90.2% in the presence of Cd2+ and Zn2+ at 5 mM, respectively (Figure 2E). Some reports have shown that metal ions have some effects on laccase, most of which inhibit laccase activity (Hu et al., 2014; Zhuo et al., 2015). Previous studies have demonstrated that even at low concentrations, Fe2+ (1 or 10 mM) can strongly inhibit laccase activity in many strains, including Pleurotus ferulae, Pycnoporus sp., T. trogii YDHSD, S. thermophilum, Trametes sp. MA-X01, and T. trogii S0301 (Younes and Sayadi, 2011; Yan et al., 2014a; Ai et al., 2015; Wang et al., 2018). And the purified rLAC-EN3-1 from P. pastoris was also sensitive to Cd2+ with relative activities of 62% at 10 mM and 18% at 100 mM (Zhuo et al., 2015).
Cu2+, by contrast, obviously enhanced the laccase activity of Lac 37 II with relative activities of 173.3 and 123.1% at 5 and 100 mM, respectively (Figure 2E), which could be due to the role of free copper ions as reducing agents in the solution and reducing the copper center in laccase (Qiao et al., 2017). Those results were in good agreement with the laccase of this strain produced at 28°C and of T. pubescens with a relative activity of 128% at 100 mM Cu2+ and 111.3% at 25 mM Cu2+, respectively (Si et al., 2013; Yan et al., 2014b). Similarly, there was activation of laccase by 10 mM Cu2+ from Sporothrix carnis CPF-05. However, the laccases of Bacillus subtilis cjp3 and T. trogii YDHSD were sensitive to Cu2+ with relative activities of 14% (Qiao et al., 2017) and 80.9% at 10 mM (Ai et al., 2015), respectively. Younes and Sayadi (2011) reported that 100 mM Co2+ greatly inhibited laccase activity in S. thermophilum and F. fomentarius, compared with relative activities of 100.3 and 77.8% at 100 mM for laccases from T. trogii S0301 at 37 and 28°C, respectively.
Many substrates of laccases are organic pollutants that contain high concentrations of organic solvents used to enhance solubility (Maté et al., 2010). These will lead to undesirable side reactions of hydrolysis, which is not conducive to thermodynamic equilibrium and difficult to product recovery. The reaction of enzyme catalyst in various organic solvents is greatly limited (Klibanov, 2001). Similarly, the existence of organic solvents is also involved in the application of enzyme membrane immobilization, although it ensures the stability of enzyme to a certain extent (Liu et al., 2019). Thus, fungal laccases with organic cosolvent tolerance have practical uses. The effects of three common solvents (methanol, ethanol, and acetonitrile at a concentration of 1, 5, and 10%) on Lac 37 II activity was investigated. The purified Lac 37 II maintained >80% of its activity in buffer containing ethanol, even at high concentration (10%, v/v). Among all tested organic solvents, 1% (v/v) acetonitrile increased laccase activity by approximately 40.4%, while activity slightly declined to 97.3 and 85.0% of the control at 5 and 10% (v/v), respectively (Figure 2F). The tolerance of Lac 37 II to acetonitrile and ethanol in this study were similar to that of laccase-like enzyme from the marine sediment samples (Yang et al., 2018). The promotive effect of acetonitrile on laccase activity has been confirmed for the crude laccase of T. trogii LK13 (Yan et al., 2015). In addition, methanol at concentrations ranging from 1 to 5% (v/v) slightly lowered activity by 11.7–16.3%, and 10% ethanol (v/v) led to a 56.1% loss of activity. Similarly, the catalytic activity of laccase in S. carnis CPF-05 was almost lost when 10% of the organic solvents added (Olajuyigbe and Fatokun, 2017). In addition, the solvent tolerance of the enzyme is considered to be positively correlated with the thermal stability, which is also in line with the thermo-active and solvent tolerance of Lac 37 II in this study (Rasekh et al., 2014).
Using MALDI-TOF MS, five peptides of Lac 37 II were obtained and the sequences of them were dertermined as follows: KVIAPDGYPR, GPLVVYDPHDPHK, YSFVLEANQPK, ANPNHANFVGFNDGINSAILR, and SAGSSEYNYKNPVQR. These peptides from Lac 37 II accurately matched to the gene-deduced sequences of lcc3 (GenBank KU055623) in T. trogii BAFC 463, but did not match other laccase isoenzymes of T. trogii strains (Lcc1, Lcc 2, Lcc 4, or the purified laccase of T. trogii S0301 at 28°C) (Figure 3). The theoretical protein molecular weight was 56 kDa, which is similar to the predicted molecular weight by SDS-PAGE. Previously, lcc3 of T. trogii BAFC 463 has been expressed in P. pastoris, and the recombined LCC3 showed excellent thermostability and thermo-activation (Campos et al., 2016). The LCC3 was assumed to be due to the thermal stability observed in T. trogii BAFC 463 culture filtrates, but in their study, LCC3 did not purified from the fermentation supernate (Campos et al., 2016). Based on the results of laccase identification, we speculated that Lac 37 II purified in our study is the native LCC3, and it was the third laccase isoenzyme isolated from T. trogii.
Figure 3. Multiple amino acid sequences alignments of Lac 37 II with other fungal laccases of T. trogii. Four copper-binding conserved domains of typical laccase: CuI (HWHGFFQ), CuII (HSHLSTQ), CuIII (HPFHLHG), and CuIV (HCHIDFHL) were boxed in black. The underline showed the internal peptide sequences of Lac 37 II based on the results of MALDI-TOF MS. Lac_28 and Lac_37 were the purified laccase of T. trogii S0301 strain produced at 28°C and Lac 37 II at 37°C, respectively. ▲ indicated the five different amino acids in the gene-deduced sequences between Lac 37 II and Lcc 3.
We also observed that Lac 37 II showed certain great advantages over the recombined Lcc 3, such as smaller molecular weight and higher thermostability (Figure 1). In our study, Lac 37 II showed a smaller molecular mass than that of the recombined LCC3 in T. trogii BAFC 463. In addition, the optimum temperature, thermostability, and decolorizing efficiency of Lac 37 II were higher than those of the recombined LCC3 (Table 2).
The possible reasons for those differences are the post-translational modifications in different hosts (yeasts and T. trogii), especially the glycosylation (Maestre-Reyna et al., 2015). Previous studies have proved that the recombined laccases in P. pastoris were always hyperglycosylated along with the changes of molecular mass and enzymatic properties, and the mechnism is that the glycosylation profile acts as the regulatory modules for substrate binding and turnover (Younes et al., 2007; Odón et al., 2009; Neha et al., 2012; Maestre-Reyna et al., 2015; Peter, 2016). In addition, we also found that the two amino acids (P13 and P351) of Lac37 II are different from lac3 (L13 and L351) (Figure 3). By predicting the protein structure of Lac 37 II, it was found that two prolines were located in the loop region. Suzuki et al. (1987) and Watanabe et al. (1991) considered that the proline may improve the thermal stability of protein by reducing the skeleton entropy of protein unfolding in the proper β-corner or random curl position. Two mutant lipases improved thermal stability by proline substitution mutagenesis, which were more stable than wild type (Mohammadi et al., 2016). Therefore, the difference of proline may be another factor that affects the thermal stability of Lac 37 II.
The crude and purified laccase of T. trogii S0301 produced at 28°C and the recombined LCC3 all showed high efficiency toward decolorization of triphenylmethane dyes (Grassi et al., 2011; Yan et al., 2014a, b; Campos et al., 2016). Based on these results, two triphenylmethane dyes (malachite green and crystal violet) were chosen in this study to assess the potential application of this laccase isoenzyme. Due to the reasonably good thermostability of the purified laccase, dye decolorization analysis was carried out at 60°C in this study.
Without the addition of the laccase mediator, Lac 37 II was less effective against malachite green, and crystal violet, with maximum decolorization of 8.6% for 25 mg L–1 crystal violet and 16.0% for 10 mg L–1 malachite green in 15 h, which was similar to the recombined LCC3 (Campos et al., 2016), but much lower than those of the purified laccase from T. trogii S0301 produced at 28°C with the maximum decolorization of 95.7% in 11 h for all dyes at the same concentration (Yan et al., 2014a) (Table 3).
Previous studies have indicated that the natural or artificial laccase mediators can increase decolorization of dyes by both the purified and crude laccases from many strains, including T. trogii and T. villosa (Grassi et al., 2011; Campos et al., 2016). To confirm whether laccase mediators can improve the decolorization ability of Lac 37 II, 1-HBT was chosed as a laccase mediator. When HBT was added at the concentration of 2 mM, the highest decolorization rate of Lac 37 II was detected as approximately 100% for 10 mg L–1 malachite green within 1 h (Figure 4A). Lac 37 II efficiently decolorized 25 mg L–1 malachite green, with maximum decolorization of 73.7 and 99.1% in 2 and 3 h, respectively (Figure 4A). To explore the decolorization ability of Lac 37 II at elevated concentrations of malachite green, 50 mg L–1 malachite green was used. Lac 37 II was able to decolorize with maximum decolorization of 47.3 and 62.7% in 20 and 53 h, respectively (Figure 4A). HBT also greatly enhanced the decolorization efficiency of Lac 37 II for crystal violet, with decolorization of 26.9, 64.1, and 99.1% in 2, 3, and 5 h, respectively, while Lac 37 II without HBT showed almost no effect on crystal violet even after 20 h of incubation, with decolorization of 9.1% at 20 h (Figure 4B).
Figure 4. Dye decolorization by Lac 37 II. Reactions were carried out using citrate-phosphate buffer (100 mM, pH 6.0) at 60°C. MG (malachite green) and CV (crystal violet). The degradation of malachite green (A) and crystal violet (B) were carried out using citrate-phosphate buffer (100 mM, pH 6.0) at 60°C. Each value is the mean value ± standard error of the mean of triplicates. Each value is the mean value ± standard error of the mean of triplicates.
In this study, HBT was chosen as the sole laccase mediator, and a greater increase in decolorization rate was obtained for all tested dyes. However, HBT has been demonstrated as a laccase mediator with a slight or no effect on dyes decolorization (Canas and Camarero, 2010; Campos et al., 2016). Thus, laccase mediators, especially more natural and effective ones, can be optimized in future studies to enhance the decolorization efficiency of Lac 37 II.
In this study, Lac 37 II, a novel native laccase isoenzyme of Trametes trogii S0301 was obtained by incubating this strain at 37°C, which is higher than the normal cultivation temperature of fungi. By purification and identification, we found that Lac 37 II is the third native laccase isoenzyme from T. trogii strains, and it is also the first thermo-active and the more thermostable isoenzyme of Trametes genus strains. With higher thermostability and catalytic efficiency, this laccase isoenzyme can efficiently decolorize triphenylmethane dyes with the addition of a laccase mediator, which makes Lac 37 II have further prospective for biotechnological applications.
All datasets generated for this study are included in the article/supplementary material.
XY has carried out enzyme activity determination, thermal stability analysis, and laccase purification. YW was responsible for the effect of temperature and pH on the activity and stability of laccase. YZ was responsible for the effect of organic dissolution and metal ions on laccase activity. EY was responsible for the identification of laccase. YQ was responsible for the decolorization of dyes by laccase. HX was responsible for SDS-PAGE and Native-PAGE. YC was responsible for the preparation of laccase. CI has revised the original manuscript. JY has conceived the experiment plan, supervised the experiment process, and wrote the original manuscript.
This work was supported by the National Natural Science Foundation of China (No. 31560036).
The authors declare that the research was conducted in the absence of any commercial or financial relationships that could be construed as a potential conflict of interest.
The authors gratefully thank Professor Zhou Tongxin and her team for their valuable help.
Ai, M. Q., Wang, F. F., and Huang, F. (2015). Purification and characterization of a thermostable laccase from Trametes trogii and its ability in modification of kraft lignin. J. Microbiol. Biotechnol. 25, 1361–1370. doi: 10.4014/jmb.1502.02022
Asgher, M., Bhatti, H. N., Ashraf, M., and Legge, R. L. (2008). Recent developments in biodegradation of industrial pollutants by white rot fungi and their enzyme system. Biodegradation 19, 771–783. doi: 10.1007/s10532-008-9185-3
Baldrian, P. (2006). Fungal laccases - occurrence and properties. FEMS Microbiol. Rev. 30, 215–242. doi: 10.1111/j.1574-4976.2005.00010.x
Bertrand, B., Martínez-Morales, F., and Trejo-Hernández, M. R. (2017). Upgrading laccase production and biochemical properties: strategies and challenges. Biotechnol. Prog. 33, 1015–1034. doi: 10.1002/btpr.2482
Campos, P. A., Levin, L. N., and Wirth, S. A. (2016). Heterologous production, characterization and dye decolorization ability of a novel thermostable laccase isoenzyme from Trametes trogii BAFC 463. Process Biochem. 51, 895–903. doi: 10.1016/j.procbio.2016.03.015
Canas, A. I., and Camarero, S. (2010). Laccases and their natural mediators: biotechnological tools for sustainable eco-friendly processes. Biotechnol. Adv. 28, 694–705. doi: 10.1016/j.biotechadv.2010.05.002
Colao, M. C., Caporale, C., Silvestri, F., Ruzzi, M., and Buonocore, V. (2009). Modeling the 3-D structure of a re combinant laccase from Trametes trogii active at a pH close to neutrality. Protein J. 28, 375–383. doi: 10.1007/s10930-009-9204-1
Colao, M. C., Garzillo, A. M., Buonocore, V., Schiesser, A., and Ruzzi, M. (2003). Primary structure and transcription analysis of a laccase-encoding gene from the Basidiomycetetrametes trogii. Appl. Microbiol. Biotechnol. 63, 153–158. doi: 10.1007/s00253-003-1429-x
Colao, M. C., Lupino, S., Garzillo, A. M., Buonocore, V., and Ruzzi, M. (2006). Heterologous expression oflcc1gene from Trametes trogii in pichia pastoris and characterization of the recombinant enzyme. Microb. Cell Fact. 5, 31–40. doi: 10.1186/1475-2859-5-31
Fonseca, M. I., Farina, J. I., Sadanoski, M. A., D’Errico, R., Villalba, L. L., and Zapata, P. D. (2015). Decolorization of Kraft liquor effluents and biochemical characterization of laccases from Phlebia brevispora BAFC 633. Int. Biodeter. Biodegr. 104, 443–451. doi: 10.1016/j.ibiod.2015.07.014
Garzillo, A. M. V., Colao, M. C., Caruso, C., Caporale, C., Celletti, D., and Buonocore, V. (1998). Laccase from the white-rot fungus Trametes trogii. Appl. Microbiol. Biotechnol. 49, 545–551. doi: 10.1007/s002530051211
Grassi, E., Scodeller, P., Filiel, N., Carballo, R., and Levin, L. (2011). Potential of Trametes trogii culture fluids and its purified laccase for the decolorization of different types of recalcitrant dyes without the addition of redox mediators. Int. Biodeter. Biodegr. 65, 635–643. doi: 10.1016/j.ibiod.2011.03.007
Guan, Y., Hua, S., Zhao, X. H., Zhang, Q. H., Zhong, L. J., Zhu, W. W., et al. (2011). Isolation, purification and enzymatic features of laccase from Trametes trogii. J. Microbiol. 31, 64–68. doi: 10.3724/SP.J.1077.2011.00073
Halaburgi, V. M., Sharma, S., Sinha, M., Singh, T. P., and Karegoudar, T. B. (2011). Purification and characterization of a thermostable laccase from the ascomycetes Cladosporium cladosporioides and its applications. Process Biochem. 46, 1146–1152. doi: 10.1016/j.procbio.2011.02.002
Hildén, K., Hakala, T. K., Maijala, P., Lundell, T. K., and Hatakka, A. (2007). Novel thermotolerant laccases produced by the white-rot fungus Physisporinus rivulosus. Appl. Microbiol. Biotechnol. 77, 301–309. doi: 10.1007/s00253-007-1155-x
Hu, X., Wang, C., Wang, L., Zhang, R., and Chen, H. (2014). Influence of temperature, pH and metal ions on guaiacol oxidation of purified laccase from Leptographium qinlingensis. World J. Microbiol. Biotechnol. 30, 1285–1290. doi: 10.1007/s11274-013-1554-3
Jaiswal, N., Pandey, V. P., and Dwivedi, U. N. (2015). Purification of a thermostable alkaline laccase from papaya (Carica papaya) using affinity chromatography. Int. J. Biol. Macromol. 72, 326–332. doi: 10.1016/j.ijbiomac.2014.08.032
Janusz, G., Kucharzyk, K. H., Pawlik, A., Staszczak, M., and Paszczynski, A. J. (2013). Fungal laccase, manganese peroxidase and lignin peroxidase: gene expression and regulation. Enzyme Microb. Technol. 52, 1–12. doi: 10.1016/j.enzmictec.2012.10.003
Kiiskinen, L. L., Viikari, L., and Kruus, K. (2002). Purification and characterisation of a novel laccase from the ascomycete Melanocarpus albomyces. Appl. Microbiol. Biotechnol. 59, 198–204. doi: 10.1007/s00253-002-1012-x
Klibanov, A. M. (2001). Improving enzymes by using them in organic solvents. Nature 409, 241–246. doi: 10.1038/35051719
Kunamneni, A., Plou, F. J., Ballesteros, A., and Alcalde, M. (2008). Laccases and their applications: a patent review. Recent Pat. Biotechnol. 2, 5–12. doi: 10.2174/187220808783330965
Liu, C., Saeki, D., Cheng, L., Luo, J., and Matsuyama, H. (2019). Polyketone-based membrane support improves the organic solvent resistance of laccase catalysis. J. Colloid Interface Sci. 544, 230–240. doi: 10.1016/j.jcis.2019.03.003
Maestre-Reyna, M., Liu, W. C., Jeng, W. Y., Lee, C. C., Hsu, C. A., Wen, T. N., et al. (2015). Structural and functional roles of glycosylation in fungal laccase from Lentinus sp. PLoS One 10:e0120601. doi: 10.1371/journal.pone.0120601
Maté, D., García-Burgos, C., García-Ruiz, E., Ballesteros, A. O., Camarero, S., and Alcalde, M. (2010). Laboratory evolution of high-redox potential laccases. Chem. Biol. 17, 1030–1041. doi: 10.1016/j.chembiol.2010.07.010
Mohammadi, M., Sepehrizadeh, Z., Ebrahim-Habibi, A., Shahverdi, A. R., Faramarzi, M. A., and Setayesh, N. (2016). Enhancing activity and thermostability of lipase a from Serratia marcescens by site-directed mutagenesis. Enzyme Microb. Technol. 9, 18–28. doi: 10.1016/j.enzmictec.2016.07.006
Neha, G., Nora, B., Tenzin, K., Meenu, C., Marion, A. S., and Saroj, M. (2012). Cloning, sequence analysis, expression of Cyathus bulleri laccase in Pichia pastoris and characterization of recombinant laccase. BMC Biotechnol. 12:75. doi: 10.1186/1472-6750-12-75
Odón, V.-V., Palomares, L. A., Dantán-González, E., Ayala-Castro, H. G., Martínez-Anaya, C., Valderrama, B., et al. (2009). The role of N-glycosylation on the enzymatic activity of a Pycnoporus sanguineus laccase. Enzyme Microb. Technol. 45, 233–239. doi: 10.1016/j.enzmictec.2009.05.007
Olajuyigbe, F. M., and Fatokun, C. O. (2017). Biochemical characterization of an extremely stable ph-versatile laccase from Sporothrix carnis CPF-05. Int. J. Biol. Macromol. 94, 535–543. doi: 10.1016/j.ijbiomac.2016.10.037
Othman, A. M., Elsayed, M. A., Elshafei, A. M., and Hassan, M. M. (2018). Purification and biochemical characterization of two isolated laccase isoforms from Agaricus bisporus CU13 and their potency in dye decolorization. Int. J. Biol. Macromol. 113, 1142–1148. doi: 10.1016/j.ijbiomac.2018.03.043
Peter, G. (2016). Effects of glycosylation on the enzymatic activity and mechanisms of proteases. Int. J. Mol. Sci. 17:1969. doi: 10.3390/ijms17121969
Piscitelli, A., Giardina, P., Lettera, V., Pezzella, C., Sannia, G., and Faraco, V. (2011). Induction and transcriptional regulation of laccases in fungi. Curr. Genom. 12, 104–112. doi: 10.2174/138920211795564331
Qiao, W., Chu, J., Ding, S., Song, X., and Yu, L. (2017). Characterization of a thermo-alkali-stable laccase from Bacillus subtilis cjp3 and its application in dyes decolorization. J. Environ. Sci. Health A Tox. Hazard. Subst. Environ. Eng. 52, 1–8. doi: 10.1080/10934529.2017.1301747
Rasekh, B., Khajeh, K., Ranjbar, B., Mollania, N., Almasinia, B., and Tirandaz, H. (2014). Protein engineering of laccase to enhance its activity and stability in the presence of organic solvents. Eng. Life Sci. 14, 442–448. doi: 10.1002/elsc.201300042
Rathi, P., Bradoo, S., Saxena, R. K., and Gupta, R. (2000). A hyper-thermostable, alkaline lipase from Pseudomonas sp. with the property of thermal activation. Biotechnol. Lett. 22, 495–498. doi: 10.1023/a:1005604617440
Riva, S. (2006). Laccases: blue enzymes for green chemistry. Trends Biotechnol. 24, 219–226. doi: 10.1016/j.tibtech.2006.03.006
Shi, L., Yu, H., Dong, T., Kong, W., Ke, M., Ma, F., et al. (2014). Biochemical and molecular characterization of a novel laccase from selective lignin-degrading white-rot fungus Echinodontium taxodii 2538. Process Biochem. 49, 1097–1106. doi: 10.1016/j.procbio.2014.03.028
Si, J., Peng, F., and Cui, B. (2013). Purification, biochemical characterization and dye decolorization capacity of an alkali-resistant and metal-tolerant laccase from Trametes pubescens. Bioresour. Technol. 128, 49–57. doi: 10.1016/j.biortech.2012.10.085
Suzuki, Y., Oishi, K., Nakano, H., and Nagayama, T. (1987). A strong correlation between the increase in number of proline residues and the rise in thermostability of five Bacillus oligo-1,6-glucosidase. Appl. Microbiol. Biotechnol. 26, 546–551. doi: 10.1007/BF00253030
Tong, P. G., Hong, Y. Z., Xiao, Y. Z., Zhang, M., Tu, X. M., and Cui, T. J. (2007). High production of laccase by a new basidiomycete, Trametes sp. Biotechnol. Lett. 29, 295–301. doi: 10.1007/s10529-006-9241-1
Wang, F., Guo, C., Wei, T., Zhang, T., and Liu, C. Z. (2012). Heat shock treatment improves Trametes versicolor laccase production. Appl. Biochem. Biotechnol. 168, 256–265. doi: 10.1007/s12010-012-9769-6
Wang, Q., Ding, L., and Zhu, C. (2018). Characterization of laccase from a novel isolated white-rot fungi Trametes sp. MA-X01 and its potential application in dye decolorization. Biotechnol. Biotechnol. Equip. 32, 1477–1485. doi: 10.1080/13102818.2018.1517028
Watanabe, K., Chishiro, K., Kitamura, K., and Suzuki, Y. (1991). Proline residues responsible for thermostability occur with high frequency in the loop regions of an extremely thermostable oligo-1,6-glucosidase from Bacillus thermoglucosidasius kp1006. J. Biol. Chem. 266, 24287–24294. doi: 10.1016/S1567-4215(03)90519-4
Wong, K. K. Y., Richardson, J. D., and Mansfield, S. D. (2000). Enzymatic treatment of mechanical pulp fibers for improving papermaking properties. Biotechnol. Prog. 16, 1025–1029. doi: 10.1021/bp000064d
Wu, Y. R., Luo, Z. H., Chow, K. K., and Vrijmoed, L. L. P. (2010). Purification and characterization of an extracellular laccase from the anthracene-degrading fungus Fusarium solani MAS2. Bioresour. Technol. 101, 9772–9777. doi: 10.1016/j.biortech.2010.07.091
Yan, J., Chen, D., Yang, E., Niu, J., Chen, Y., and Chagan, I. (2014a). Purification and characterization of a thermotolerant laccase isoform in Trametes trogii strain and its potential in dye decolorization. Int. Biodeter. Biodegr. 93, 186–194. doi: 10.1016/j.ibiod.2014.06.001
Yan, J., Niu, J. Z., Chen, D. D., Chen, Y. H., and Chagan, I. (2014b). Screening of Trametes strains for efficient decolorization of malachite green at high temperatures and ionic concentrations. Int. Biodeter. Biodegr. 87, 109–115. doi: 10.1016/j.ibiod.2013.11.009
Yan, J. P., Chen, Y. H., Niu, J. Z., Chen, D. D., and Chagan, I. (2015). Laccase produced by a thermotolerant strain of Trametes trogii. Braz. J. Microbiol. 46, 59–65. doi: 10.1590/S1517-838246120130895
Yang, Q., Zhang, M., Zhang, M., Wang, C., Liu, Y., Fan, X., et al. (2018). Characterization of a novel, cold-adapted, and thermostable laccase-like enzyme with high tolerance for organic solvents and salt and potent dye decolorization ability, derived from a marine metagenomic library. Front. Microbiol. 9:2998. doi: 10.3389/fmicb.2018.02998
Yang, Y., Ma, F., Yu, H., Fan, F., Wan, X., Zhang, X., et al. (2011). Characterization of a laccase gene from the white-rot fungi Trametes sp. 5930 isolated from Shennongjia nature reserve in China and studying on the capability of decolorization of different synthetic dyes. Biochem. Eng. J. 57, 13–22. doi: 10.1016/j.bej.2011.07.006
Younes, S. B., Cherif, I., Dhouib, A., and Sayadi, S. (2016). Trametes trogii: a biologic powerful tool for dyes decolorization and detoxification. Catal. Lett. 146, 204–211. doi: 10.1007/s10562-015-1629-x
Younes, S. B., Khedher, S. B., Zhang, Y., Geissen, S. U., and Sayadi, S. (2019). Laccase from Scytalidium thermophilum: production improvement, catalytic behavior and detoxifying ability of diclofenac. Catal. Lett. 149, 1833–1844. doi: 10.1007/s10562-019-02771-1
Younes, S. B., Mechichi, T., and Sayadi, S. (2007). Purification and characterization of the laccase secreted by the white rot fungus Perenniporia tephropora and its role in the decolourization of synthetic dyes. J. Appl. Microbiol. 102, 1033–1042.
Younes, S. B., and Sayadi, S. (2011). Purification and characterization of a novel trimeric and thermotolerant laccase produced from the ascomycete Scytalidium thermophilum strain. J. Mol. Catal. B Enzym. 73, 35–42. doi: 10.1016/j.molcatb.2011.07.014
Zheng, F., An, Q., Meng, G., Wu, X. J., Dai, Y. C., Si, J., et al. (2017). A novel laccase from white rot fungus, Trametes orientalis: purification, characterization, and application. Int. J. Biol. Macromol. 102, 758–770. doi: 10.1016/j.ijbiomac.2017.04.089
Zhu, Y., Zhang, H., Cao, M., Wei, Z., Huang, F., and Gao, P. (2011). Production of a thermostable metal-tolerant laccase from Trametes versicolor and its application in dye decolorization. Biotechnol. Bioprocess Eng. 16, 1027–1035. doi: 10.1007/s12257-011-0129-0
Zhuo, R., He, F., Zhang, X., and Yang, Y. (2015). Characterization of a yeast recombinant laccase rLAC-EN3-1 and its application in decolorizing synthetic dye with the coexistence of metal ions and organic solvents. Biochem. Eng. J. 93, 63–72. doi: 10.1016/j.bej.2014.09.004
Zhuo, R., Yuan, P., Yang, Y., Zhang, S., Ma, F., and Zhang, X. (2016). Induction of laccase by metal ions and aromatic compounds in Pleurotus ostreatus HAUCC 162 and decolorization of different synthetic dyes by the extracellular laccase. Biochem. Eng. J. 117(Pt B), 62–72. doi: 10.1016/j.bej.2016.09.016
Keywords: Trametes trogii, thermoactive laccase, thermostable laccase, organic solvent tolerance, dye decolorization
Citation: Yang X, Wu Y, Zhang Y, Yang E, Qu Y, Xu H, Chen Y, Irbis C and Yan J (2020) A Thermo-Active Laccase Isoenzyme From Trametes trogii and Its Potential for Dye Decolorization at High Temperature. Front. Microbiol. 11:241. doi: 10.3389/fmicb.2020.00241
Received: 23 October 2019; Accepted: 31 January 2020;
Published: 19 February 2020.
Edited by:
Junpei Zhou, Yunnan Normal University, ChinaReviewed by:
Jing Si, Beijing Forestry University, ChinaCopyright © 2020 Yang, Wu, Zhang, Yang, Qu, Xu, Chen, Irbis and Yan. This is an open-access article distributed under the terms of the Creative Commons Attribution License (CC BY). The use, distribution or reproduction in other forums is permitted, provided the original author(s) and the copyright owner(s) are credited and that the original publication in this journal is cited, in accordance with accepted academic practice. No use, distribution or reproduction is permitted which does not comply with these terms.
*Correspondence: Jinping Yan, anB5YW4yMDE5QDE2My5jb20=
Disclaimer: All claims expressed in this article are solely those of the authors and do not necessarily represent those of their affiliated organizations, or those of the publisher, the editors and the reviewers. Any product that may be evaluated in this article or claim that may be made by its manufacturer is not guaranteed or endorsed by the publisher.
Research integrity at Frontiers
Learn more about the work of our research integrity team to safeguard the quality of each article we publish.