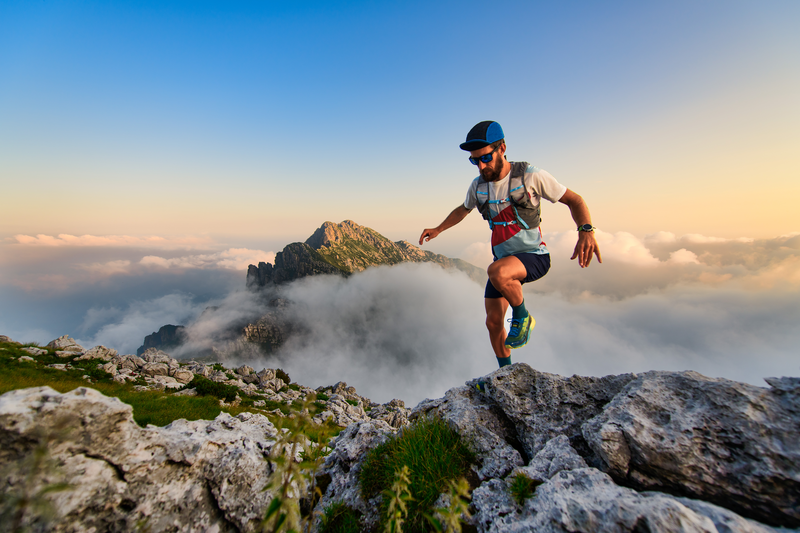
95% of researchers rate our articles as excellent or good
Learn more about the work of our research integrity team to safeguard the quality of each article we publish.
Find out more
ORIGINAL RESEARCH article
Front. Microbiol. , 06 February 2020
Sec. Antimicrobials, Resistance and Chemotherapy
Volume 11 - 2020 | https://doi.org/10.3389/fmicb.2020.00079
This article is part of the Research Topic Natural Bioactive Substances As An Alternative Solution Against Food Pathogenic Bacteria: Interaction With The QS System View all 9 articles
Pseudomonas aeruginosa is an opportunistic pathogen that causes high morbidity and mortality rates due to its biofilm form. Biofilm formation is regulated via quorum sensing (QS) mechanism and provides up to 1000 times more resistance against conventional antibiotics. QS related genes are expressed according to bacterial population density via signal molecules. QS inhibitors (QSIs) from natural sources are widely studied evaluating various extracts from extreme environments. It is suggested that extremely halophilic Archaea may also produce QSI compounds. For this purpose, we tested QS inhibitory potentials of ethyl acetate extracts from cell free supernatants and cells of Natrinema versiforme against QS and biofilm formation of P. aeruginosa. To observe QS inhibition, all extracts were tested on P. aeruginosa lasB-gfp, rhlA-gfp, and pqsA-gfp biosensor strains and biofilm inhibition was studied using P. aeruginosa PAO1. According to our results, QS inhibition ratios of cell free supernatant extract (CFSE) were higher than cell extract (CE) on las system, whereas CE was more effective on rhl system. In addition, anti-biofilm effect of CFSE was higher than CE. Structural analysis revealed that the most abundant compound in the extracts was trans 4-(2-carboxy-vinyl) benzoic acid.
Multidrug-resistant and extensively drug-resistant microorganisms arise from increased and uncontrolled antibiotic utilization (Gohil et al., 2018). It was reported that at least 2 million people are infected and approximately 23,000 people die annually due to drug resistant infections in United States (Center of Disease Control and Prevention [CDC], 2018). Treatment of infections caused by Pseudomonas aeruginosa can be particularly complicated due to its acquired multidrug resistance, its virulence factors and biofilm formation resulting in community-acquired and hospital-acquired infections (Singh et al., 2000; Smith and Iglewski, 2003; Bjarnsholt et al., 2010). This opportunistic pathogen causes nearly 11% of nosocomial infections especially in immunocompromised patients with cystic fibrosis (CF), pneumonia, urinary tract infections, surgical site infections, sepsis, and skin infections especially observed in burn units (Khan et al., 2015; Pires et al., 2015). P. aeruginosa can colonize easily in hospital equipments such as disinfectants, ventilators, food, items in bathroom and toilets. Due to its ubiquity, this bacterium can be transmitted by food, visitors, patient contact with contaminated reservoirs, healthcare worker hands, and by ingestion of contaminated materials. Unfortunately, this bacterium can also cause high mortality rates in CF patients (Ciofu et al., 2015). The Center of Disease Control and Prevention (CDC) reported that more than 6,000 (13%) of 51,000 P. aeruginosa infections in the United States are experienced due to multiple drug resistance each year and 400 of these infections result in death (Center of Disease Control and Prevention [CDC], 2013). According to the list of antibiotic priorities published by the World Health Organization [WHO], 2017, P. aeruginosa has critical requirement for new antibiotic development (Tacconelli et al., 2018). Therefore, the urgent need for alternative strategies against antibiotic resistance problem is a growing problem in the World. Recently proposed antivirulence approaches could be a powerful solution to overcome this complication (Rasko and Sperandio, 2010). The goal of these approaches is not to kill bacteria but to prevent bacterial infections and damage caused by them. In recent years, quorum sensing (QS) mechanisms are evaluated as a potential target for this strategy (Rutherford and Bassler, 2012; Tang and Zhang, 2014; Defoirdt, 2018; Dickey et al., 2017). Bacteria communicate with each other via QS by using signal molecules called autoinducers (AIs). P. aeruginosa employs acyl-homoserine lactones (AHLs) and has four hierarchically connected QS systems for interspecies communication: las, rhl, pqs, and iqs. The AIs produced by las, rhl, pqs, and iqs systems are 3-oxo-C12-HSL, C4-HSL, 2-alkyl4-qionolones, and 2-(2-hydroxyphenyl)-thiazole-4-carbaldehyde, respectively. The concentration of AHL molecules rises depending on bacterial population and induces activation of transcriptional regulators. Thereby, pathogenic bacteria can fight against the host immune system by the collective expression of virulence genes (Papenfort and Bassler, 2016).
Recently, several compounds and enzymes disrupting QS system have been reported. This phenomenon is called quorum quenching (QQ). Since QS systems have clinical importance in the course of pathogenesis, potential QS inhibitors (QSIs) are being still investigated. Most of these molecules have been reported from natural sources, but their synthetic derivatives are also available (Martinelli et al., 2004; LaSarre and Federle, 2013).
The term “extremophiles” is referred to microorganisms that grow in extreme environmental stress conditions. The role of extremophilic QS systems is not fully studied and remains scarce (Montgomery et al., 2013). Maheshwari and Saraf (2015) reported that halophilic bacteria produce exopolysaccharides, compatible solutes and enzymes and the synthesis of these biomolecules may be influenced by QS. Similarly, Tommonaro et al. (2012) demonstrated QS related production of diketopiperazines (DKPs) from Haloterrigena hispanica. The researchers reported that cyclo-(L-Pro-L-Val) induced QS system in NTL4 (AHL bioreporter strain) and they suggested that this compound may be involved in QS system of Archaea to survive in such harsh conditions (Tommonaro et al., 2012). Also, autoinducer-2 (AI-2) which provides bacterial communication for interspecies, was detected in Halobacillus halophilus (Tommonaro et al., 2012; Tommonaro et al., 2015). Several studies focused on such compounds examining their potential to be stabilizers of biomolecules, stress protective agents, and halophilic enzymes for biocatalysts (Finore et al., 2011; Poli et al., 2011).
Amongst extremophiles, halophiles were reported to have potential natural source with QQ activities (Teasdale et al., 2011). Quadri et al. (2016) reported that the most antimicrobial producer genus was Natrinema, compared to 102 extremely halophilic archaea isolated. It was reported that sublethal doses of compounds or extracts with antimicrobial activities may have anti-QS potential in the discovery of compounds with QSI potentials. In this respect, the aim of our study is to determine the presence of compounds with potential QQ and anti-biofilm activities in N. versiforme species belonging to Natrinema genus against P. aeruginosa. The QSI and anti-biofilm activities of ethyl acetate cell extract (CE) and cell free supernatant extract (CFSE) obtained from N. versiforme, an extremophilic halophile, are firstly examined against P. aeruginosa las, rhl, and pqs systems in this study.
Pseudomonas aeruginosa lasB-gfp (Hentzer et al., 2002), rhlA-gfp (Yang et al., 2009), and pqsA-gfp (Yang et al., 2007) biomonitor strains were used to evaluate QS inhibitions and PAO1 wild type strain ATCC 15692 was used for biofilm inhibition assays. Biomonitor strains were designed for green fluorescent protein (GFP) expression under the control of Plas, Prhl or Ppqs promoters. They were kindly provided by Tim Holm Jakobsen and Michael Givskov from University of Copenhagen. The bacteria were normally grown in lysogeny broth (LB) medium. QSI assays were performed using M9 minimal media with thiamine (2.5 mg/l), glucose [0.5% (wt/vol)], and casamino acids [0.5% (wt/vol)]. Gentamicin was added to LB and M9 media for biosensor strains. Details of the QS monitor strains, PAO1 (wild type) and clinical isolate of P. aeruginosa are presented in Table 1.
Natrinema versiforme DSM 16034 (Xin et al., 2000) was grown in 372 medium containing (l–1): yeast extract 5.00 g, casamino acids 5.00 g, sodium glutamate 1.00 g, potassium chloride 2.00 g, sodium citrate 3.00 g, MgSO4⋅7 H2O 20.00 g, sodium chloride 200.00 g, FeCl2⋅4 H2O 36.00 mg, MnCl2⋅4 H2O 0.36 mg, pH 7 ± 0.2, at a T 37°C. Agar plates were prepared by adding 20 g agar l–1.
After the optimal growth of microorganisms, cells and cell free medium were obtained by centrifugation at 10.000 rpm for 40 min of cultures. The extraction step was performed on cells and cell-free medium with ethyl acetate for three times in ratio 1/1 (v/v) for cell free medium (250 mL) and 1/20 (w/v) for cells (3 g). Extracts were dried at rotary evaporator at a T < 40°C. Both extracts, from cells and cell-free medium, resulted as a concentrate (Teasdale et al., 2009).
A modified method of Bjarnsholt et al. (2005) was performed for the determination of QSI potentials of ethyl acetate CE and CFSE from extremophilic N. versiforme. QSI screenings were performed in 96-well black microplates (Nunc, Thermo Scientific). 100 μl of M9 medium was added to each well and two-fold serial dilutions of tested extracts were made by adding 100 μl to the first well and after mixing, transferring 100 μl from first to the next well and so on. Overnight cultures of the lasB-gfp, rhlA-gfp, and pqsA-gfp monitor strains were added to obtain a total volume of 200 μl with an OD 450 nm of 0.1. Final concentrations of tested extracts were 240 μg/ml, 120 μg/ml, and 60 μg/ml. The tests were performed in three replicates. Bacterial growth and GFP expressions were monitored using Cytation 3 multimode microplate reader (BioTek, United States) for 14–16 h, measuring absorbance and fluorescence every 15 min. Fluorescence of GFP expression was measured at 485 nm excitation and 535 nm emission wavelengths.
Pseudomonas aeruginosa PAO1 strain was incubated overnight at 37°C in LB and later diluted to OD 600 nm: 0.01 with M9 minimal medium including 2.5 mg/l thiamine, 0.5% (w/v) glucose, and 0.5% (w/v) casamino acid. In 96-well microplates, CE and CFSE of extremophilic N. versiforme were tested at the concentrations of 240 μg/ml, 120 μg/ml and 60 μg/ml. The tests were performed in three replicates. A modified protocol of George A. O’Toole (2011) was performed for biofilm staining. After incubation, the plates were washed with distilled water and then dried for 1 h at 60°C for biofilm fixation. After drying step, 125 μl of a 0.1% solution of crystal violet were added to each well. The plates were incubated at room temperature for 10 min and then washed three times. After drying, 125 μl 96% ethanol were added to the wells and absorbance were measured in a plate reader at 590 nm in microplate reader (Cytation 3-BioTek).
Silica gel plates were purchased from E. Merck (Darmstadt, Germany). Compounds on TLC plates were visualized under UV light. Ethyl acetate extracts were screened by thin layer chromatography (TLC) technique. Pure Compounds were recovered by preparative TLC procedure (system solvent Chloroform/Methanol 8:2 by vol).
Samples for NMR analysis were dissolved in Methanol-d4. Chemical shifts are reported with the residual MeOD (δH 3.32 ppm) as the internal standard for 1H NMR spectrometry, and MeOD (δC 49.0 ppm) for 13C NMR spectrometry. 1D- and 2D-NMR spectra were recorded at 600.13 MHz on a Bruker Avance III-600 spectrometer equipped with a TCI Cryo ProbeTM fitted with a gradient along the Z-axis, at a probe temperature of 27°C. 13C NMR spectra were recorded on a Bruker avance 400 spectrometer equipped with a Cryo Probe Prodigy (100.62 MHz). 1H,13C NMR, COSY, TOCSY, HSQC, HSQC-EDITED, HMBC (3J: 10 Hz) experiments were used for structural determinations. Mass spectra were acquired on a MicroQ-Tof mass spectrometer coupled with an Alliance HPLC (Waters, Milford, MA, United States) equipped with an ESI positive and negative source.
The elastase activities of P. aeruginosa PAO1 wild type and clinical isolate strains were determined by the protocol described by Ohman et al., 1980. 7 ml of overnight cultures (0.01 OD) were prepared in the absence or presence of trans 4-(2-carboxy-vinyl) benzoic acid and the cultures were incubated for 24 h with shaking at 37°C. After incubation, cultures were centrifuged at 10000 g for 10 min and supernatants were filtrated. 500 μl of filtrate was added to 500 μl of ECR buffer (100 mM Tris–HCl + 1 mM CaCl2, pH 7.2) containing 15 mg of ECR. Mixture was kept for 3 h with shaking at 37°C. Insoluble ECR was pelleted by centrifugation at 1200 g for 10 min. The elastase activity was evaluated spectrophotometrically at 495 nm between the trans 4-(2-carboxy-vinyl) benzoic acid and the untreated control groups.
For determination of protease activity, 7 ml of overnight cultures (0.01 OD) were prepared in the absence or presence of trans 4-(2-carboxy-vinyl) benzoic acid and the cultures were then incubated for 24 h with shaking at 37°C. Bacteria cultures were centrifuged at 10000 g for 10 min and supernatants were filtrated. 500 μl of filtrate was added to 1.25% skimmed milk and mixture was kept for 30 min with shaking at 37°C. The absorbance values of each mixture were measured at 520 nm at 600 nm to determine protease activity (El-Mowafy et al., 2014).
For pyocyanin activity, overnight cultures centrifuged at 10000 g for 10 min. and supernatants were filtrated. 3 ml of chloroform was added onto these filtrates. Chloroform layer was transferred to new tubes and mixed with 0.2 M HCl (Essar et al., 1990). Measurements were evaluated due to differences on OD values at 520 nm between the trans 4-(2-carboxy-vinyl) benzoic acid and the untreated control groups.
To examine biocompatibility of samples, 24 h in vitro cell culture viability experiments were performed with human keratinocyte cell line HaCaT (Boukamp et al., 1988), kindly provided by Assoc. Prof. Dr. Betül Karademir from Marmara University. Cells were grown at DMEM complete media with 10% fetal bovine serum (FBS) and 1% antibiotic (penicillin, streptomycin) (PAN Biotech, Germany) at 37°C incubator with 5% CO2 atmosphere. When they reach confluent phase, cells were trypsinized and seeded to 96 well culture plates at the density of 104 and left overnight for cellular attachment. Ethyl acetate extract fractions containing DMSO [I27 240 (1.5% DMSO) and (0.75% DMSO) 120 μg/ml, I35 and I36 (0.375% DMSO) and 60 μg/ml] were diluted in DMEM serum free media (PAN Biotech, Germany) and sterilized by passing through 0.22 μm filter and added into well plates. Cells were incubated for 24 h at 37°C with 5% CO2 and viability was tested with WST-1 cell proliferation kit (4-[3-(4-iodophenyl)-2-(4-nitrophenyl)-2H-5-tetrazolio]-1,3-benzene disulfonate) (Roche, Switzerland). Absorbance values at 450 nm were measured with Glomax Multi + Microplate Multimode Reader (Promega, United States). Untreated cells were used as control and amount of DMSO that samples contain were added to each sample’s control group.
Statistical analyses were performed by GraphPad V 5.0 Prism analyses program with Student’s t-test to find significant differences between samples and control group. All experiments were performed in triplicate. Data was presented as mean; biofilm error margins are presented as standard error (SE) and cell culture results are presented with 95% confidence interval (CI). A p-value below 0.05 was considered as statistically significant.
Cell extract of extremophilic N. versiforme have more inhibitory potential than CFSE on both QS systems (las and rhl) and biofilm formation for the highest dose (240 μg/ml) applied. The inhibition ratios were recorded as 65.99% for lasB-gfp, 51.8% for rhlA-gfp, and 79.19% for pqsA-gfp, respectively. The same extracts at the concentration of 240 μg/ml inhibited biofilm formation by 29.57% (± 2.19).
The most effective inhibition ratios by CFSE of extremophilic N. versiforme on las and rhl system were obtained at the highest dose (240 μg/ml) applied. The inhibition ratios were recorded as 67.68% for lasB-gfp, 35.26% for rhlA-gfp, and 77.04% for pqsA-gfp, respectively. The maximum anti-biofilm effect of the CFSE extracts was determined at 240 μg/ml and the inhibition ratio was found to be 40.72% (± 4.6).
Green fluorescent protein expression graphics of the extracts with best inhibition ratios are shown in Figure 1 and biofilm inhibitions are presented in Figure 2. Inhibition data were calculated for GFP expression over absorbance values to consider bacterial growth. No significant bacteria growth inhibition was observed (Supplementary Figures S1–S9).
Figure 1. GFP expression curves of lasB-gfp, rhlA-gfp, and pqsA-gfp monitor strains treated with CFSE and CE obtained from N. versiforme. Data are shown as Relative Fluorescence Unit over Optical Density of 450 nm. (A) lasB-gfp CFSE, (B) rhlA-gfp CFSE, (C) pqsA-gfp CFSE, (D) lasB-gfp CE, (E) rhlA-gfp CE, (F) pqsA-gfp CE.
Figure 2. Biofilm inhibition percentages of P. aeruginosa PAO1 treated with CFSE and CE obtained from N. versiforme. Biofilm mass of untreated samples are considered as 100. (A) PAO1 treated with CFSE, (B) PAO1 treated with CE.
The ethyl acetate extract was preliminary investigated by TLC chromatography; it was made of almost seven UV-visible compounds present with different percentages. Furthermore, the 1H-NMR spectrum of ethyl acetate extract (Supplementary Figure S10) suggested the prevalence of a set of aromatic signals, which could be related to a unique structure, with respect to other ones.
After a purification step by TLC preparative procedure, the most abundant compound (7 mg) was isolated and this molecule was spectroscopically investigated. The compound was identified as the trans 4-(2-carboxy-vinyl) benzoic acid, shown in Figure 3. 1H- 13C-NMR spectra of the isolated compound were also reported; suggesting a recovery of the molecule with a high level of purity. In 1H NMR spectrum, together with the principal signals assigned to the trans 4-(2-carboxy-vinyl) benzoic acid skeleton, the presence of very little intense signals, in particular in the aromatic and vinyl regions, suggested the scarce presence of structurally related molecules as contaminants. This structural hypothesis was confirmed by considering the presence of aromatic signals in the 1H-NMR at 7.06 (2H, dd, 5.21 and 2.10 Hz) and 7.41 ppm (2H, dd, 5.21 and 2.10 Hz), which were correlated in HSQC spectrum to the 13C signals at 129.17 and 130.19 ppm, respectively. In HMBC experiment, long-range C-H correlations between the proton signal at 7.06 ppm and quaternary carbon signal at 131.4 ppm, and proton signal at 7.41 and quaternary carbon signal at t 135.2 ppm, secured the para substituted phenyl ring structure. Furthermore, in HMBC experiment, aromatic carbon at 135.2 ppm showed long-range correlation with a proton signal at 7.68 ppm; this last one was part of the olefin portion of the molecule. In fact, in HSQC experiment, the proton signal at 7.68 ppm (1H, d, J 16.10 Hz) was correlated at a carbon at 146.19 ppm; in addiction a further presence of an C-H olefin system was indicated by a proton signal at 6.49 ppm (1H, d, J 16.10 Hz) linked to carbon at 119.5 ppm. Long range C-H correlation between proton at 7.68 ppm and vicinal carbon at 119.5 ppm were also recorded. The presence of an intense signal at 170.41 ppm in 13C NMR spectrum confirmed the presence of carboxylic carbons: in HMBC experiment, a correlation with 170.4 ppm and the olefin proton signals at 6.49 and 7.68 ppm were recorded. In addiction in 13C spectrum, the chemical shifts of aromatic carbon at 130.4 and 135.18 ppm were typical of quaternary carbons linked to carboxylic group para-substituted1. This data suggested the presence of two carboxylic groups as reported in Figure 3. This hypothesis was confirmed by MS investigations. In ESI–-MS spectrum a base-peak at 146 (M-HCOOH-H–) confirmed this structure.
Figure 3. 1H (A) and 13C (B) NMR spectra of trans 4-(2-carboxy-vinyl) benzoic acid (C) isolated from N. versiforme; spectra were recorded in Methanol-d4.
Minor compounds (0.2–0.5 mg) of ethyl acetate extract were only partially purified. 1H NMR spectra suggested the presence in their structure of phenyl rings (proton signals at 7.9–6.9 ppm) conjugated to alcoholic groups (proton signals at 3.5–4 ppm) or double bonds (olefin proton signals at 6.5–7.0 ppm) and/or carbonylic groups (with corresponding vicinal proton signals at 2.0–2.8 ppm). Their complete chemical characterization is still under investigation.
The isolated compound trans 4-(2-carboxy-vinyl) benzoic acid demonstrated similar inhibition ratios for P. aeruginosa QS systems as CFSE. The inhibition ratios for the concentration of 240 μg/ml were recorded as 61.85% for lasB-gfp, 37.71% for rhlA-gfp, and 74.16% for pqsA-gfp, respectively. Best biofilm inhibition, however, was observed at the concentration of 60 μg/ml that is 48.91%. QS and biofilm inhibition ratios of trans 4-(2-carboxy-vinyl) benzoic acid are shown in Figure 4.
Figure 4. GFP expression curves of lasB-gfp, rhlA-gfp, and pqsA-gfp monitor strains (A–C, respectively) and biofilm inhibition percentage (D) of PAO1 strain treated with trans-4-(2-carboxy-vinyl) benzoic acid isolated from N. versiforme.
Highest inhibition was recorded on pyocyanin production of PAO1 (86%). On the other hand, the same inhibition efficacy could not be detected on the clinical isolate of P. aeruginosa (34.3%). Protease activity was suppressed by the compound for 27% in PAO1 whereas, 3.9% in the clinical isolate of P. aeruginosa. Nevertheless, elastase activity could not be inhibited by our compound in either strain. These results indicate that our compound is considerably effective for the inhibition of pyocyanin production. Results of the virulence assays are presented in Figure 5.
Figure 5. Pyocyanin, protease and elastase inhibition activities of trans-4-(2-carboxy-vinyl) benzoic acid (TCV-BA) isolated from N. versiforme against PAO1 (A-C) and the clinical isolate (D–F) of P. aeruginosa.
According to safety doses of DMSO, current study estimated doses to be below 1% and concentrations of the samples are chosen due to their DMSO solvent percentages (Galvao et al., 2014). Cell viability results of HaCaT cells after 24 h incubation with CFSE and its two fractions are shown in Figure 6. Viability of cells treatedse activity was suppressed by with 120 and 240 μg/ml CFSE was 98.52% and 97.68%, respectively; while for isolated trans-4-(2-carboxy-vinyl) benzoic acid (60 μg/ml) it was 94.73%. Control groups were estimated as 100% viable. Statistical analyses of data showed no significance between control group and samples except CFSE 240 μg/ml, which showed difference with its control group with a p-value of 0.0099.
Figure 6. HaCaT cell viability results after incubation with CFSE (A,B) and trans-4-(2-carboxy-vinyl) benzoic acid (C) for 24 h.
Recently, QS inhibition has been proposed as an antivirulence approach to overcome global drug resistance problem. This combating strategy against bacterial diseases focuses on the inhibition of bacterial virulence factors instead of their growth. Although natural QSIs and their synthetic derivatives have been reported against P. aeruginosa, many potential QSIs are being still investigated (Bjarnsholt et al., 2005; Skindersoe et al., 2008; Yaniv et al., 2017; Fong et al., 2018). Extremophiles are known potential sources for biotechnological and industrial aspects. Several molecules produced by Archaea and their potential role are discussed in the literature (Charlesworth and Burns, 2015). However, there is limited number of studies evaluating QQ properties or AHL degrading enzymes of extremophiles (Seo et al., 2011; Teasdale et al., 2011; Abed et al., 2013; Morohoshi et al., 2015; Santhakumari et al., 2016). Also, there is very little information about halophilic bioactive compounds with QQ potentials (Abed et al., 2013). The compounds which have been reported to have QS inhibitory potentials are cyclo (L-Pro-L-Phe) and cyclo (L-Pro-L-isoLeu) from Marinobacter sp. (SK-3); phenethylamides and a cyclic dipeptide isolated from Halobacillus salinus, Bacillus or Halobacillus sp.; palmitic acid from Synechococcus elongatus (Abed et al., 2013; Santhakumari et al., 2016, 2017). Taken together, our knowledge about QS system and potential QQs in extremophiles guided us to hypothesize that extremophilic microorganisms can adapt to harsh conditions by producing some other unstudied metabolites to compete with other organisms. For this purpose, we evaluated QSI and anti-biofilm potentials of extremophilic N. versiforme against P. aeruginosa biomonitor strains lasB-gfp, rhlA-gfp, and pqsA-gfp. QQ activities are generally evaluated by inhibition in the production of violacein or bioluminescence and/or reduction in biofilm formation and EPS production. The production of violacein by reporter biosensor strains (CV026 and CV017 etc.) is utilized as an indicator of QS system depending on produced AHLs by Gram-negative bacteria. However, GFP-producing biomonitor strains provide monitoring of direct gene expressions such as las, rhl, and pqs, and thus QSI potentials of tested materials can be easily evaluated on each QS system.
In our study, we detected that CE and CFSE of N. versiforme were considerably capable of inhibiting all QS systems (las, rhl, and pqs) at the certain concentration of 240 μg/ml. In particular, inhibition percentages in las and pqs systems were higher than rhl system for CE and CSFE extracts, respectively. But QS inhibition rates in rhl system were also observed. Our findings demonstrate consistence with other studies, because las system hierarchically orchestrate the expression of pqs and rhl systems. In our study, we showed inhibition in at least two QS pathways: las and pqs system. Furthermore, we analyzed anti-biofilm potentials of both extracts against P. aeruginosa PAO1 wild type strain and we observed that CFSE inhibited biofilm formation better than CE. Our results indicate that the inhibitory potential of CFSE of N. versiforme was more significant on las and pqs systems and biofilm formation whereas CE was more successful on rhl QS system.
We also isolated trans 4-(2-carboxy-vinyl) benzoic acid via a combination of chromatographic and spectroscopic techniques from CFSE. This compound is related chemically to cinnamic acid and its derivatives that are described as active antimicrobial compounds (Sova, 2012; Guzman, 2014). In particular, cinnamaldehyde have been reported to have QS inhibitory activity in bioreporter strains of E. coli or Vibrio species (Niu et al., 2006; Brackman et al., 2008). Several synthesized cinnamic acid derivatives were evaluated for their QSI potentials (Chen et al., 2019). Naik et al. (2013) reported QS inhibition by methanol extracts of marine sponge–derived actinomycetes through Chromobacterium violaceum CV12472. Also, they detected cinnamic acid from the most successful extract for QS inhibition. Herein, we reported the anti-QS and anti-biofilm activities of the isolated compound, trans 4-(2-carboxy-vinyl) benzoic acid, which is a derivative of cinnamic acid, via P. aeruginosa biosensor strains called lasB-gfp, rhlA-gfp, and pqsA-gfp strains and wild type PAO1, respectively.
Quorum quenching properties of benzoic acid and derivatives have been also reported against different bacteria species such as E. coli and V. harveyi (Brackman et al., 2009; Ta and Arnason, 2016). In our study, trans 4-(2-carboxy-vinyl) benzoic acid showed higher QS inhibitory potential than the tested extracts (CEs and CFSEs). In addition, biofilm inhibition, especially at 60 μg/ml, was found to be higher than the inhibition ratios determined for other extract concentrations. More recently, Rajkumari et al. (2018) reported the inhibitory effect of cinnamic acid on P. aeruginosa biofilm formation by 50.13%. In their study, authors indicated that they applied sub-MIC concentration (250 μg/ml) of cinnamic acid against P. aeruginosa. On the other hand, we determined that 60 μg/ml of trans 4-(2-carboxy-vinyl) benzoic acid is profoundly efficient for biofilm inhibition by 48.91%. Therefore, our isolated compound has potential anti-biofilm effect with low concentration. There are stringent benchmarks that have to be met by new compounds in drug discovery such as efficacy and safety criterias: broad-spectrum efficacy and no toxicity. Thus, we also performed biocompatibility tests via cell culture for 60 μg/ml pure compound, which showed no specific toxicity.
In some cases, expected effects of QSI compounds cannot be observed against clinical isolates. It is assumed that some clinical isolates of P. aeruginosa may have protection against various compounds (García-Contreras et al., 2014; Guendouze et al., 2017). In this respect, the effect of trans 4-(2-carboxy-vinyl) benzoic acid on protease, pyocyanin and elastase activities were also tested both against a clinical isolate of P. aeruginosa and PAO1 wild-type strain. Trans 4-(2-carboxy-vinyl) benzoic acid highly inhibited pyocyanin production in both strains. However, protease activity inhibition was only observed in PAO1, and no elastase inhibition was detected in either strain. In a previous study, the necessity of PqsE for production of pyocyanin was reported (Rampioni et al., 2007). In this study, we observed a high inhibition ratio against the pqs system, which confirms the decrease all pyocyanin production in the treated strains. On the other hand, we didn’t detect the expected inhibition for elastase.
In conclusion, we intended to show that extremophilic microorganisms can synthesize compounds that can be utilized in antivirulence approaches, specifically QS and biofilm inhibition. We investigated cell and cell-free extracts of N. versiforme and isolated a pure compound named trans 4-(2-carboxy-vinyl) benzoic acid, and all have shown QSI properties against P. aeruginosa. It is possible that other bioactive compounds can be isolated from other extremophilic microorganisms that live under stressful conditions. Further studies will reveal the potential use for the compound isolated in antivirulence drug research.
The datasets generated for this study are available on request to the corresponding author.
NS, GT, and ET designed and organized the study. AT, GA, and CI obtained and analyzed extracts. TB and BG conducted microbiological experiments. ME conducted cell toxicity experiments. TB, DB, BG, GT, ME, and NS prepared the manuscript. All authors read and approved the final manuscript.
This study was funded by the Scientific and Technological Research Council of Turkey (TUBITAK) Bilateral Cooperation with Consiglio Nazionale delle Ricerche (CNR) (Project no. 315S092).
The authors declare that the research was conducted in the absence of any commercial or financial relationships that could be construed as a potential conflict of interest.
We would like to thank Tim Holm Jakobsen and Michael Givskov (University of Copenhagen) for providing the monitor strains, and Münevver Ufuk Hasdemir (Marmara University) for providing the clinical isolate of P. aeruginosa.
The Supplementary Material for this article can be found online at: https://www.frontiersin.org/articles/10.3389/fmicb.2020.00079/full#supplementary-material
Abed, R. M., Dobretsov, S., Al-Fori, M., Gunasekera, S. P., Sudesh, K., and Paul, V. J. (2013). Quorum-sensing inhibitory compounds from extremophilic microorganisms isolated from a hypersaline cyanobacterial mat. J. Indus. Microbiol. Biotechnol. 40, 759–772. doi: 10.1007/s10295-013-1276-4
Bjarnsholt, T., Jensen, P. Ø, Jakobsen, T. H., Phipps, R., Nielsen, A. K., Rybtke, M. T., et al. (2010). Quorum sensing and virulence of Pseudomonas aeruginosa during lung infection of cystic fibrosis patients. PLoS One 5:e10115. doi: 10.1371/journal.pone.0010115
Bjarnsholt, T., Jensen, P. Ø, Rasmussen, T. B., Christophersen, L., Calum, H., Hentzer, M., et al. (2005). Garlic blocks quorum sensing and promotes rapid clearing of pulmonary Pseudomonas aeruginosa infections. Microbiology 151, 3873–3880. doi: 10.1099/mic.0.27955-0
Boukamp, P., Petrussevska, R. T., Breitkreutz, D., Hornung, J., Markham, A., and Fusenig, N. E. (1988). Normal keratinization in a spontaneously immortalized aneuploid human keratinocyte cell line. J. Cell Biol. 106, 761–771. doi: 10.1083/jcb.106.3.761
Brackman, G., Defoirdt, T., Miyamoto, C., Bossier, P., Van Calenbergh, S., Nelis, H., et al. (2008). Cinnamaldehyde and cinnamaldehyde derivatives reduce virulence in Vibrio spp. by decreasing the DNA-binding activity of the quorum sensing response regulator LuxR. BMC Microbiol. 8:149. doi: 10.1186/1471-2180-8-149
Brackman, G., Hillaert, U., Van Calenbergh, S., Nelis, H. J., and Coenye, T. (2009). Use of quorum sensing inhibitors to interfere with biofilm formation and development in Burkholderia multivorans and Burkholderia cenocepacia. Res. Microbiol. 160, 144–151. doi: 10.1016/j.resmic.2008.12.003
Center of Disease Control and Prevention [CDC] (2013). Pseudomonas aeruginosa in Healthcare Settings. Atlanta: Center of Disease Control and Prevention.
Center of Disease Control and Prevention [CDC] (2018). Available at: https://www.cdc.gov/drugresistance/about.html (accessed September 10, 2018).
Charlesworth, J. C., and Burns, B. P. (2015). Untapped resources: biotechnological potential of peptides and secondary metabolites in archaea. Archaea 2015, 1–7. doi: 10.1155/2015/282035
Chen, J., Wang, B., Lu, Y., Guo, Y., Sun, J., Wei, B., et al. (2019). Quorum sensing inhibitors from marine microorganisms and their synthetic derivatives. Mar. Drugs 17:80. doi: 10.3390/md17020080
Ciofu, O., Tolker-Nielsen, T., Jensen, P. Ø, Wang, H., and Høiby, N. (2015). Antimicrobial resistance, respiratory tract infections and role of biofilms in lung infections in cystic fibrosis patients. Adv. Drug Deliv. Rev. 85, 7–23. doi: 10.1016/j.addr.2014.11.017
Defoirdt, T. (2018). Quorum-sensing systems as targets for antivirulence therapy. Trends Microbiol. 26, 313–328. doi: 10.1016/j.tim.2017.10.005
Dickey, S. W., Cheung, G. Y., and Otto, M. (2017). Different drugs for bad bugs: antivirulence strategies in the age of antibiotic resistance. Nat. Rev. Drug Discov. 16:457. doi: 10.1038/nrd.2017.23
El-Mowafy, S. A., Shaaban, M. I, and Abd El Galil, K. H. (2014). Sodium ascorbate as a quorum sensing inhibitor of Pseudomonas aeruginosa. J. Appl. Microbiol. 117, 1388–1399. doi: 10.1111/jam.12631
Essar, D. W., Eberly, L. E. E., Hadero, A., and Crawford, I. P. (1990). Identification and characterization of genes for a second anthranilate synthase in Pseudomonas aeruginosa: interchangeability of the two anthranilate synthases and evolutionary implications. J. Bacteriol. 172, 884–900. doi: 10.1128/jb.172.2.884-900.1990
Finore, I., Kasavi, C., Poli, A., Romano, I., Oner, E. T., Kirdar, B., et al. (2011). Purification, biochemical characterization and gene sequencing of a thermostable raw starch digesting α-amylase from Geobacillus thermoleovorans subsp. Stromboliensis subsp. nov. World J. Microbiol. Biotechnol. 27, 2425–2433. doi: 10.1007/s11274-011-0715-5
Fong, J., Zhang, C., Yang, R., Boo, Z. Z., Tan, S. K., Nielsen, T. E., et al. (2018). Combination therapy strategy of quorum quenching enzyme and quorum sensing inhibitor in suppressing multiple quorum sensing pathways of P. aeruginosa. Sci. Rep. 8:1155. doi: 10.1038/s41598-018-19504-w
Galvao, J., Davis, B., Tilley, M., Normando, E., Duchen, M. R., and Cordeiro, M. F. (2014). Unexpected low-dose toxicity of the universal solvent DMSO. FASEB J. 28, 1317–1330. doi: 10.1096/fj.13-235440
García-Contreras, R., Pérez-Eretza, B., Lira-Silva, E., Jasso-Chávez, R., Coria-Jiménez, R., Rangel-Vega, A., et al. (2014). Gallium induces the production of virulence factors in Pseudomonas aeruginosa. Pathog. Dis. 70, 95–98. doi: 10.1111/2049-632X.12105
Gohil, N., Ramírez-García, R., Panchasara, H., Patel, S., Bhattacharjee, G., and Singh, V. (2018). Book review: quorum sensing vs. quorum quenching: a battle with no end in sight. Front. Cell. Infect. Microbiol. 8:106.
Guendouze, A., Plener, L., Bzdrenga, J., Jacquet, P., Rémy, B., Elias, M., et al. (2017). Effect of quorum quenching lactonase in clinical isolates of Pseudomonas aeruginosa and comparison with quorum sensing inhibitors. Front. Microbiol. 8:227. doi: 10.3389/fmicb.2017.00227
Guzman, J. (2014). Natural cinnamic acids, synthetic derivatives and hybrids with antimicrobial activity. Molecules 19, 19292–19349. doi: 10.3390/molecules191219292
Hentzer, M., Riedel, K., Rasmussen, T. B., Heydorn, A., Andersen, J. B., Parsek, M. R., et al. (2002). Inhibition of quorum sensing in Pseudomonas aeruginosa biofilm bacteria by a halogenated furanone compound. Microbiology 148, 87–102. doi: 10.1099/00221287-148-1-87
Holloway, B. W., and Morgan, A. F. (1986). Genome organization in Pseudomonas. Ann. Rev. Microbiol. 40, 79–105. doi: 10.1146/annurev.mi.40.100186.000455
Khan, H. A., Ahmad, A., and Mehboob, R. (2015). Nosocomial infections and their control strategies. Asian Pacific J. Trop. Biomed. 5, 509–514.
LaSarre, B., and Federle, M. J. (2013). Exploiting quorum sensing to confuse bacterial pathogens. Microbiol. Mol. Biol. Rev. 77, 73–111. doi: 10.1128/MMBR.00046-12
Maheshwari, D. K., and Saraf, M. (2015). Halophiles Biodiversity and Sustainable Exploitation. Switzerland: Springer International Publishing, 355–357.
Martinelli, D., Grossmann, G., Séquin, U., Brandl, H., and Bachofen, R. (2004). Effects of natural and chemically synthesized furanones on quorum sensing in Chromobacterium violaceum. BMC Microbiol. 4:25. doi: 10.1186/1471-2180-4-25
Montgomery, K., Charlesworth, J., LeBard, R., Visscher, P., and Burns, B. (2013). Quorum sensing in extreme environments. Life 3, 131–148. doi: 10.3390/life3010131
Morohoshi, T., Tominaga, Y., Someya, N., and Ikeda, T. (2015). Characterization of a novel thermostable N-acylhomoserine lactonase from the thermophilic bacterium Thermaerobacter marianensis. J. Biosci. Bioeng. 120, 1–5. doi: 10.1016/j.jbiosc.2014.11.014
Naik, D. N., Wahidullah, S., and Meena, R. M. (2013). Attenuation of Pseudomonas aeruginosa virulence by marine invertebrate–derived Streptomyces sp. Lett. Appl. Microbiol. 56, 197–207. doi: 10.1111/lam.12034
Niu, C., Afre, S., and Gilbert, E. S. (2006). Subinhibitory concentrations of cinnamaldehyde interfere with quorum sensing. Lett. Appl. Microbiol. 43, 489–494. doi: 10.1111/j.1472-765x.2006.02001.x
Ohman, D. E., Cryz, S. J., and Iglewski, B. H. (1980). Isolation and characterization of Pseudomonas aeruginosa PAO mutant that produces altered elastase. J. Bacteriol. 142, 836–842. doi: 10.1128/jb.142.3.836-842.1980
Papenfort, K., and Bassler, B. L. (2016). Quorum sensing signal–response systems in Gram-negative bacteria. Nat. Rev. Microbiol. 14:576. doi: 10.1038/nrmicro.2016.89
Pires, D. P., Boas, D. V., Sillankorva, S., and Azeredo, J. (2015). Phage therapy: a step forward in the treatment of Pseudomonas aeruginosa infections. J. Virol. 89, 7449–7456. doi: 10.1128/JVI.00385-15
Poli, A., Di Donato, P., Abbamondi, G. R., and Nicolaus, B. (2011). Synthesis, production, and biotechnological applications of exopolysaccharides and polyhydroxyalkanoates by archaea. Archaea 2011, 1–13. doi: 10.1155/2011/693253
Quadri, I., Hassani, I. I, L’Haridon, S., Chalopin, M., Hacène, H., and Jebbar, M. (2016). Characterization and antimicrobial potential of extremely halophilic archaea isolated from hypersaline environments of the Algerian Sahara. Microbiol. Res. 186, 119–131. doi: 10.1016/j.micres.2016.04.003
Rajkumari, J., Borkotoky, S., Murali, A., Suchiang, K., Mohanty, S. K., and Busi, S. (2018). Cinnamic acid attenuates quorum sensing associated virulence factors and biofilm formation in Pseudomonas aeruginosa PAO1. Biotechnol. Lett. 40, 1087–1100. doi: 10.1007/s10529-018-2557-9
Rampioni, G., Schuster, M., Greenberg, E. P., Bertani, I., Grasso, M., Venturi, V., et al. (2007). RsaL provides quorum sensing homeostasis and functions as a global regulator of gene expression in Pseudomonas aeruginosa. Mol. Microbiol. 66, 1557–1565. doi: 10.1111/j.1365-2958.2007.06029.x
Rasko, D. A., and Sperandio, V. (2010). Anti-virulence strategies to combat bacteria-mediated disease. Nat. Rev. Drug Discov. 9:117. doi: 10.1038/nrd3013
Rutherford, S. T., and Bassler, B. L. (2012). Bacterial quorum sensing: its role in virulence and possibilities for its control. Cold Spring Harb. Perspect. Med. 2:a012427. doi: 10.1101/cshperspect.a012427
Santhakumari, S., Kannappan, A., Pandian, S. K., Thajuddin, N., Rajendran, R. B., and Ravi, A. V. (2016). Inhibitory effect of marine cyanobacterial extract on biofilm formation and virulence factor production of bacterial pathogens causing vibriosis in aquaculture. J. Appl. Phycol. 28, 313–324. doi: 10.1007/s10811-015-0554-0
Santhakumari, S., Nilofernisha, N. M., Ponraj, J. G., Pandian, S. K., and Ravi, A. V. (2017). In vitro and in vivo exploration of palmitic acid from Synechococcus elongatus as an antibiofilm agent on the survival of Artemia franciscana against virulent vibrios. J. Invert. Pathol. 150, 21–31. doi: 10.1016/j.jip.2017.09.001
Seo, M. J., Lee, B. S., Pyun, Y. R., and Park, H. (2011). Isolation and characterization of N-acylhomoserine lactonase from the thermophilic bacterium, Geobacillus caldoxylosilyticus YS-8. Biosci. Biotechnol. Biochem. 75, 1789–1795. doi: 10.1271/bbb.110322
Singh, P. K., Schaefer, A. L., Parsek, M. R., Moninger, T. O., Welsh, M. J., and Greenberg, E. P. (2000). Quorum-sensing signals indicate that cystic fibrosis lungs are infected with bacterial biofilms. Nature 407:762. doi: 10.1038/35037627
Skindersoe, M. E., Ettinger-Epstein, P., Rasmussen, T. B., Bjarnsholt, T., de Nys, R., and Givskov, M. (2008). Quorum sensing antagonism from marine organisms. Mar. Biotechnol. 10, 56–63. doi: 10.1007/s10126-007-9036-y
Smith, R. S., and Iglewski, B. H. (2003). Pseudomonas aeruginosa quorum sensing as a potential antimicrobial target. J. Clin. Invest. 112, 1460–1465. doi: 10.1172/jci200320364
Sova, M. (2012). Antioxidant and antimicrobial activities of cinnamic acid derivatives. Mini Rev. Med. Chem. 12, 749–767. doi: 10.2174/138955712801264792
Ta, C., and Arnason, J. (2016). Mini review of phytochemicals and plant taxa with activity as microbial biofilm and quorum sensing inhibitors. Molecules 21:29. doi: 10.3390/molecules21010029
Tacconelli, E., Carrara, E., Savoldi, A., Harbarth, S., Mendelson, M., Monnet, D. L., et al. (2018). Discovery, research, and development of new antibiotics: the WHO priority list of antibiotic-resistant bacteria and tuberculosis. Lancet Infect. Dis. 18, 318–327. doi: 10.1016/S1473-3099(17)30753-3
Tang, K., and Zhang, X. H. (2014). Quorum quenching agents: resources for antivirulence therapy. Marine Drugs 12, 3245–3282. doi: 10.3390/md12063245
Teasdale, M. E., Donovan, K. A., Forschner-Dancause, S. R., and Rowley, D. C. (2011). Gram-positive marine bacteria as a potential resource for the discovery of quorum sensing inhibitors. Mar. Biotechnol. 13, 722–732. doi: 10.1007/s10126-010-9334-7
Teasdale, M. E., Liu, J., Wallace, J., Akhlaghi, F., and Rowley, D. C. (2009). Secondary metabolites produced by the marine bacterium Halobacillus salinus that inhibit quorum sensing-controlled phenotypes in gram-negative bacteria. Appl. Environ. Microbiol. 75, 567–572. doi: 10.1128/AEM.00632-08
Tommonaro, G., Abbamondi, G. R., Iodice, C., Tait, K., and De Rosa, S. (2012). Diketopiperazines produced by the halophilic archaeon, Haloterrigena hispanica, activate AHL bioreporters. Microb. Ecol. 63, 490–495. doi: 10.1007/s00248-011-9980-y
Tommonaro, G., Abbamondi, G. R., Toksoy Oner, E., Nicolaus, B., et al. (2015). “Investigating the quorum sensing system in halophilic bacteria,” in Halophiles. Sustainable Development and Biodiversity, Vol. 6, eds D. Maheshwari and M. Saraf, (Cham: Springer), 189–207. doi: 10.1007/978-3-319-14595-2_7
Xin, H., Itoh, T., Zhou, P., Suzuki, K. I., Kamekura, M., and Nakase, T. (2000). Natrinema versiforme sp. nov., an extremely halophilic archaeon from Aibi salt lake, Xinjiang, China. Int. J. Syst. Evol. Microbiol. 50, 1297–1303. doi: 10.1099/00207713-50-3-1297
Yang, L., Barken, K. B., Skindersoe, M. E., Christensen, A. B., Givskov, M., and Tolker-Nielsen, T. (2007). Effects of iron on DNA release and biofilm development by Pseudomonas aeruginosa. Microbiology 153, 1318–1328. doi: 10.1099/mic.0.2006/004911-0
Yang, L., Rybtke, M. T., Jakobsen, T. H., Hentzer, M., Bjarnsholt, T., Givskov, M., et al. (2009). Computer-aided identification of recognized drugs as Pseudomonas aeruginosa quorum-sensing inhibitors. Antimicrob. Agents Chemother. 53, 2432–2443. doi: 10.1128/AAC.01283-08
Keywords: Pseudomonas aeruginosa, extremophiles, quorum sensing, biofilm, antivirulence, Natrinema versiforme
Citation: Başaran TI, Berber D, Gökalsın B, Tramice A, Tommonaro G, Abbamondi GR, Erginer Hasköylü M, Toksoy Öner E, Iodice C and Sesal NC (2020) Extremophilic Natrinema versiforme Against Pseudomonas aeruginosa Quorum Sensing and Biofilm. Front. Microbiol. 11:79. doi: 10.3389/fmicb.2020.00079
Received: 12 September 2019; Accepted: 15 January 2020;
Published: 06 February 2020.
Edited by:
Vincenzo De Feo, University of Salerno, ItalyReviewed by:
Rodolfo García-Contreras, National Autonomous University of Mexico, MexicoCopyright © 2020 Başaran, Berber, Gökalsın, Tramice, Tommonaro, Abbamondi, Erginer Hasköylü, Toksoy Öner, Iodice and Sesal. This is an open-access article distributed under the terms of the Creative Commons Attribution License (CC BY). The use, distribution or reproduction in other forums is permitted, provided the original author(s) and the copyright owner(s) are credited and that the original publication in this journal is cited, in accordance with accepted academic practice. No use, distribution or reproduction is permitted which does not comply with these terms.
*Correspondence: Nüzhet Cenk Sesal, Y3Nlc2FsQG1hcm1hcmEuZWR1LnRy
Disclaimer: All claims expressed in this article are solely those of the authors and do not necessarily represent those of their affiliated organizations, or those of the publisher, the editors and the reviewers. Any product that may be evaluated in this article or claim that may be made by its manufacturer is not guaranteed or endorsed by the publisher.
Research integrity at Frontiers
Learn more about the work of our research integrity team to safeguard the quality of each article we publish.