- 1Institute of Medical Microbiology, Justus Liebig University Giessen, Giessen, Germany
- 2German Center for Infection Research (DZIF), Partner Site Giessen-Marburg-Langen, Justus Liebig University Giessen, Giessen, Germany
- 3Bioinformatics & Systems Biology, Justus Liebig University Giessen, Giessen, Germany
- 4Norddeutscher Rundfunk, Hamburg, Germany
- 5Institute of Hydrobiology, Technical University of Dresden, Dresden, Germany
- 6Department of Microbiology, University of Helsinki, Helsinki, Finland
Water is considered to play a role in the dissemination of antibiotic-resistant Gram-negative bacteria including those encoding Extended-spectrum beta-lactamases (ESBL) and carbapenemases. To investigate the role of water for their spread in more detail, we characterized ESBL/Carbapenemase-producing bacteria from surface water and sediment samples using phenotypic and genotypic approaches. ESBL/Carbapenemase-producing isolates were obtained from water/sediment samples. Species and antibiotic resistance were determined. A subset of these isolates (n = 33) was whole-genome-sequenced and analyzed for the presence of antibiotic resistance genes and virulence determinants. Their relatedness to isolates associated with human infections was investigated using multilocus sequence type and cgMLST-based analysis. Eighty-nine percent of the isolates comprised of clinically relevant species. Fifty-eight percent exhibited a multidrug-resistance phenotype. Two isolates harbored the mobile colistin resistance gene mcr-1. One carbapenemase-producing isolate identified as Enterobacter kobei harbored blaVIM–1. Two Escherichia coli isolates had sequence types (ST) associated with human infections (ST131 and ST1485) and a Klebsiella pneumoniae isolate was classified as hypervirulent. A multidrug-resistant (MDR) Pseudomonas aeruginosa isolate encoding known virulence genes associated with severe lung infections in cystic fibrosis patients was also detected. The presence of MDR and clinically relevant isolates in recreational and surface water underlines the role of aquatic environments as both reservoirs and hot spots for MDR bacteria. Future assessment of water quality should include the examination of the multidrug resistance of clinically relevant bacterial species and thus provide an important link regarding the spread of MDR bacteria in a One Health context.
Introduction
The recent emergence of antibiotic-resistant Gram-negative bacteria, in particular those encoding extended-spectrum beta-lactamases (ESBL) and/or carbapenemases, poses a significant threat for human and animal health. Nowadays, they account for a large proportion of the global pandemic spread of antimicrobial resistance (World Health Organization [WHO], 2017). ESBL/Carbapenemase-producers have been isolated from various sources including both animal- and human- populations, as well as from environmental sources including water bodies (Woodford et al., 2014; Grundmann et al., 2017; Hölzel et al., 2018; Huijbers et al., 2015; Madec et al., 2017; Muraleedharan et al., 2019).
An understanding of the causes for the emergence of ESBL/Carbapenemase-producing isolates and probable pathways of their spread would be aided by a deep genetic characterization of isolates from the different sources to detect those clones/antibiotic resistance determinants that are drivers of antibiotic resistance spread. Isolates from humans, animals and food have been studied for a long time, and whole-genome-based approaches have identified identical clones circulating among these compartments (Falgenhauer et al., 2016a, b). Far less is known for aquatic environments, where analyses are mostly based on the phenotypic analysis of antibiotic susceptibility or quantification of resistance genes (Hembach et al., 2017; Müller et al., 2018). Whole-genome-based studies have been performed on isolates from raw and treated wastewater (Gomi et al., 2018; Mahfouz et al., 2018), but are scarce for other water bodies, in particular recreational water.
To gain more insight into this topic, an investigation was performed in collaboration with investigative journalists in Northern Germany (Baars and Lambrecht, 2018). This resulted in the detection of antibiotic-resistant Gram-negative isolates in surface water bodies, partly used for recreational purposes that depicted multilocus sequence types found previously in hospital settings and are representative of relevant human pathogens. Here we report on these findings.
Materials and Methods
Sampling and Detection of ESBL-Producing Isolates
Water (n = 12) and sediment (n = 10) samples were taken from different water bodies located in northern Germany (Supplementary Figure S1 and Supplementary Table S1) in autumn 2017. Sampling sites were selected for their relevance to resistance spread (e.g., neighborhood to human clinics, recreational water bodies or food processing plants). All samples were processed within 24 h. For detection of viable ESBL/Carbapenemase-producing bacteria in the water samples, four different volumes (exact volumes differed from sample to sample depending on the expected “contamination” level) were filtered (two technical replicates per sample; pore size 0.2 μm, cellulose acetate filters, Sartorius; Göttingen, Germany). The filters were put onto CHROMagar ESBL® plates (MAST Diagnostica, Reinfeld, Germany) and incubated for 18 ± 2 h at 42°C. Sediment samples were supplemented with sodium pyrophosphate solution (20 mL 0.1% solution/100 g wet sediment) and shaken (460 rpm, room temperature, 30 min). Two-hundred microliters of the supernatant were subsequently plated on CHROMagar ESBL® plates and incubated as described above.
Species Determination and Antibiotic Susceptibility Testing
A subset of bacterial isolates (n = 134) growing on selective agar were characterized by VITEK® MS (BioMérieux, Nüertingen, Germany) for the determination of species. Antibiotic susceptibility testing was performed using the VITEK®2 system following to the manufacturer’s instructions (BioMérieux, Nüertingen, Germany). Minimal inhibitory concentrations were interpreted according to EUCAST, 2017 and CLSI, 2016 guidelines (internal VITEK®2 adjustment) The multidrug-resistance (MDR) status was evaluated for seven clinically relevant species (Acinetobacter baumannii, Citrobacter freundii, Enterobacter cloacae complex, Escherichia coli, Klebsiella oxytoca, Klebsiella pneumoniae, and Pseudomonas aeruginosa) using the classification system of Magiorakos et al. (2012), with resistance to ≥3 classes of antibiotics defining the MDR status.
Whole Genome Sequencing and Genome-Based Analysis
Thirty-three isolates out of the 134 phenotypically characterized isolates (Table 1) were subjected to whole-genome sequencing and subsequent analysis. These isolates included seven different species (A. baumannii, Enterobacter cloacae complex, E. coli, K. oxytoca, K. pneumoniae, P. aeruginosa, and Pseudomonas resinovorans). If more than one isolate of an identical species was present at one sampling site, isolates representative of different antibiotic resistance phenotypes were chosen.
DNA was isolated from overnight cultures using the PureLink® Pro 96 Genomic DNA Purification Kit (Thermo-Fisher Scientific, Dreieich, Germany). For short-read sequencing, an Illumina Nextera XT library was prepared and sequenced on a NextSeq 500 device (Illumina, Eindhoven, Netherlands) using the NextSeq 500/550 Mid Output v2 kit (2 × 150 nt read length). Sequence data analysis (coverage, mean read length, quality clipping, assembly, determination of multilocus sequence types and virulence genes using the database of VFDB (Chen et al., 2016) was performed using the in-house pipeline ASA3P (Schwengers et al., 2019). A mean coverage of 103× and a mean read length of 130 nt was achieved. Quality controlled raw reads were assembled using Spades v.3.10.1 (Bankevich et al., 2012) implemented in the ASA3P pipeline, with a mean N50 value of 103,031 nt. The sequencing data was deposited in the ENA database under the accession number PRJEB29745.
Antibiotic resistance genes and plasmid incompatibility groups were analyzed using the bacterial analysis pipeline at the Center for Genomic Epidemiology (Thomsen et al., 2016). E. coli fimH-types were determined using the Fimtyper tool (Roer et al., 2017). Virulence genes were additionally analyzed using the bacterial analysis pipeline at the Center for Genomic Epidemiology (Thomsen et al., 2016) and BIGSDb (scheme “virulence genes”) for K. pneumoniae isolates1.
Assignment of the Enterobacter species was performed using the genome sequence of the type strains referred in Supplementary Table S2 and the online GGDC tool (Meier-Kolthoff et al., 2013) applying a threshold of 70% sequence similarity for taxonomic identification.
The determination of the source of different multilocus sequence types (ST) was performed using EnteroBase for E. coli (Alikhan et al., 2018) and PubMLST database for Acinetobacter baumannii isolates2 [Pasteur scheme (Diancourt et al., 2010)].
For a detailed analysis of the source, BacWGSTdb (Ruan and Feng, 2016) was used to determine the closest relative with the function “Single genome analysis” and a core genome MLST (cgMLST) allele threshold based on 200 alleles. The analysis was performed for E. coli, K. pneumoniae, and A. baumannii isolates (n = 27), because the BacWGSTdb included schemes for these species.
Results and Discussion
The role of aquatic environments and wastewaters for the dissemination of antibiotic-resistant Gram-negative bacteria is an increasing source of concern worldwide and has not yet been investigated in detail so far (Berendonk et al., 2015). The presence of both contaminated wastewater and other anthropogenic selection pressures on aquatic environments are likely to create hotspots and reservoirs for the selection of antibiotic-resistant bacteria and the transfer of antibiotic resistance genes. Despite these facts, most water samples are routinely monitored only for the concentration of fecal indicators (E. coli and Enterococcus sp.; EU Directive 2006/7/EC). It is not mandatory for authorities to store or characterize the detected isolates further. Consequently, no systematic information on the presence of antibiotic resistance genes or the clinical relevance of the isolates is collected.
Several studies have been performed to estimate the role of surface waters for the spread of antibiotic resistance. qPCR-based studies are still the standard procedure in many countries, including Germany (Hembach et al., 2017). The disadvantage of these studies is that they do not allow the direct association of antibiotic resistance genes to a given bacterium. Studies implementing the analysis of viable antibiotic-resistant bacteria for antibiotic resistance phenotype and antibiotic resistance genes do not allow to determine the relationship of the bacteria in water to bacteria from other sources. This is only possible using genome-based studies that are still an exception (Jorgensen et al., 2017). The only genome-based study of water in Germany employs the analysis of wastewater (Mahfouz et al., 2018).
In our study, we combined phenotypic and genotypic analyses of antibiotic-resistant bacteria isolated from water and sediment samples to determine their relationship to bacteria from other sources and to determine their possible role on human health.
Clinically-Relevant Multidrug-Resistant Gram-Negative Bacteria Are Present in Recreational Water Samples
In 10 out of 12 water samples, ESBL/Carbapenemase-producing Gram-negative bacteria were detected by a culture-based approach, with concentrations varying between 2.7 and 8.1 × 103 colony forming units per 100 mL. In 20% of the analyzed sediment samples, viable ESBL/Carbapenemase-producers were detected (Supplementary Table S3). The lack of positive sediment samples should not be the result of the method used, as it has been shown in an earlier study that the number of cells derived by this method was not increased by ultrasonic treatment (Heß et al., 2018).
One hundred-thirty-four isolates growing on selective agar plates were selected for species determination and antibiotic susceptibility testing. The most common species detected were E. coli and members of the A. baumannii complex (Figure 1, 39%, respectively), followed by K. pneumoniae (6%). Other clinically relevant species (C. freundii, E. cloacae, P. aeruginosa) were less abundant.
The multidrug-resistance (MDR) status of seven clinically relevant species (Figure 2) of the isolates was evaluated and varied between 3.8% of a respective species (A. baumannii complex) to 100% (C. freundii, E. cloacae, E. coli, K. oxytoca, and K. pneumoniae, Figure 2). The low number of MDR A. baumannii is concordant to data from Austria (Kittinger et al., 2017) where only 4.4% of the A. baumannii isolates were MDR. These MDR-isolates were mostly third generation cephalosporin resistant, but also Carbapenem non-susceptible isolates were present within the characterized isolates (n = 7) including two isolates of P. aeruginosa (9w-9, 10w-5), A. baumannii complex (6w-8, 8s-4), K. oxytoca (11w-2, 12w-10) and one E. cloacae complex isolate (12w-5) (Supplementary Table S4).
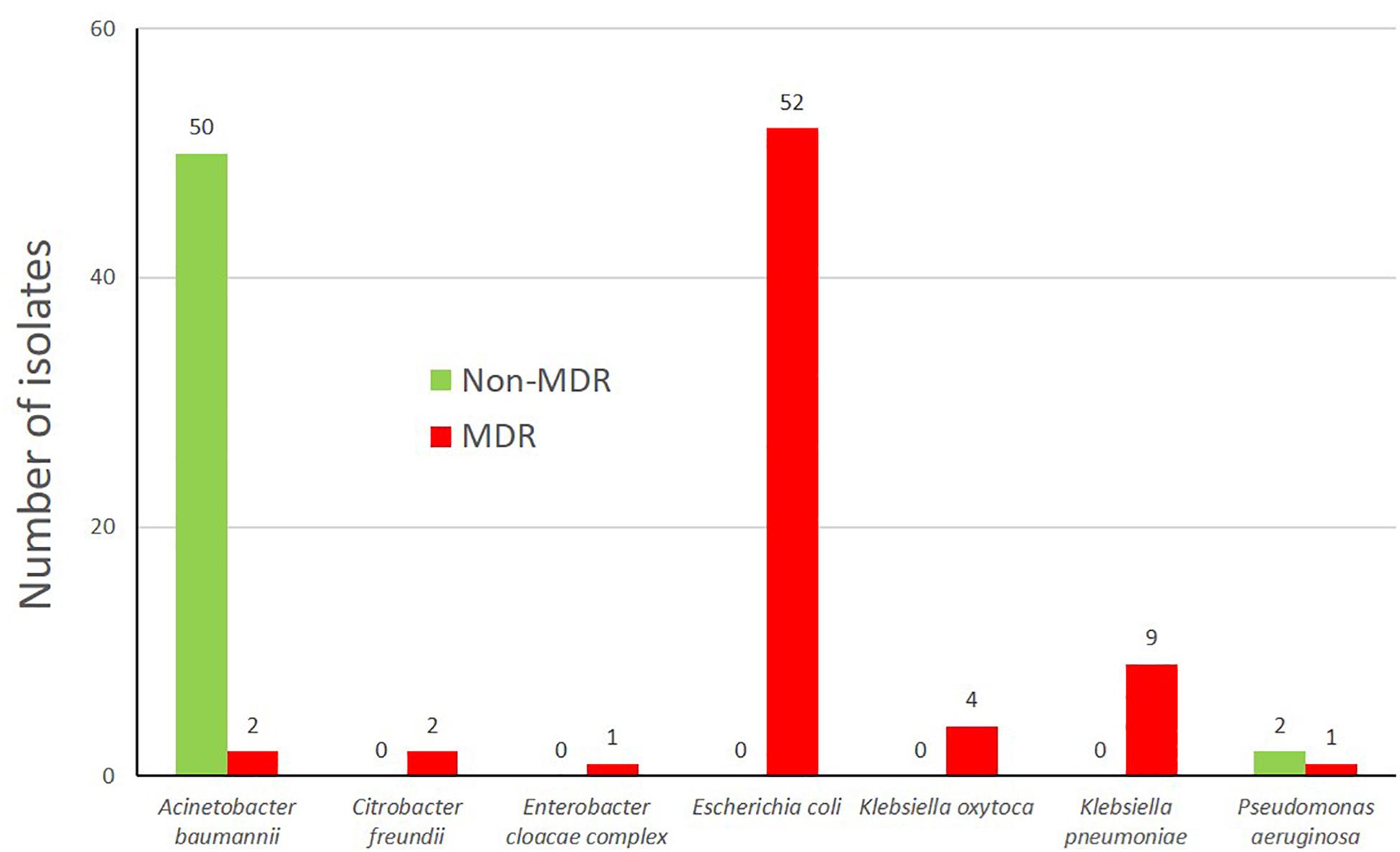
Figure 2. Multidrug status of the bacterial isolates (grown on selective growth medium) according to the classification of Magiorakos et al. (2012).
Water Isolates Harbor Antibiotic Resistance Genes Toward Eleven Antibiotic Classes
Genome-based analysis revealed that genes encoding resistance to eleven different antibiotic classes were present in the sequenced isolates (Table 1). All sequenced isolates harbored at least one beta-lactamase gene, accompanied by different phenotypes. The P. aeruginosa isolate 9w-9 encoded the blaOXA–50 gene (carbapenemase) and blaPAO (AmpC gene) beta-lactamase genes, while the P. resinovorans isolate (10w-9) harbored the beta-lactamase gene blaPOM–1. The A. baumannii isolates (n = 11) harbored the chromosomally-encoded AmpC gene blaADC–25 in combination with a blaOXA-like beta-lactamase conferring phenotypic ertapenem resistance (Table 1 and Supplementary Table S4). The Enterobacter kobei isolate (12w-5) harbored the carbapenemase gene blaVIM–1.
The most common ESBL genes detected (Table 1) were blaCTX–M–1 (n = 7) and blaCTX–M–15 (n = 7). The ESBL gene blaCTX–M–1 was found exclusively in E. coli isolates, whereas the blaCTX–M–15 gene was also detected in K. pneumoniae. Less frequent ESBL genes were blaSHV–28 (n = 2), blaCTX–M–14 (n = 1) and blaSHV–2 (n = 1). The ESBL gene blaSHV–28 was found only in K. pneumoniae and always in combination with blaCTX–M–15. The ESBL genes are similar to those found in other studies (Jorgensen et al., 2017), but the distribution was different, as the blaCTX–M–1 gene was detected more frequently (n = 7) than blaCTX–M–15 (n = 4). The prevalence of these genes also reflect the situation found in hospital settings in Germany, together with the very low incidence of acquired carbapenemase genes.
With the exception of A. baumannii, isolates harbored additional antibiotic resistance genes conferring resistance to ten antibiotic classes (Table 1). The most commonly found resistance genes were those encoding aminoglycoside resistance (12/33, e.g., strA/strB, aadA1) followed by genes conferring resistance against sulfonamides (n = 9, sul1, sul2), trimethoprim (n = 9, dfrA1, dfrA14, dfrA17) and Fluoroquinolones (n = 8, qnrS1, oqxA, oqxB, aac(6′)Ib-cr).
Most strikingly, the mobile colistin-resistance gene mcr-1 was detected in 2/33 isolates, all being E. coli isolates. In the isolate 3w-4, the mcr-1 gene was located on an IncX4 plasmid, while 8w-3 it was located on an IncHI2 plasmid. The isolates harboring mcr-1 differed from those found in Switzerland (Zurfuh et al., 2016) both by multilocus sequence type and the encoded ESBL gene. The mcr-1-encoding isolates were only found in the neighborhood of a meat processing plant (sampling site 3) and a slaughterhouse (sampling site 8), concordant with the finding that mcr-1 is more prevalent in livestock than in humans (Schwarz and Johnson, 2016).
Detection of Plasmid Incompatibility Groups Present in the Isolates
The Enterobacteriaceae isolates harbored a number of different plasmid incompatibility groups (Supplementary Table S5 and Supplementary Figure S2). Col-type plasmids were the most frequent plasmids, followed by IncI1 and IncF-type plasmids. IncI1 and IncF plasmids are frequently encoding ESBL genes (García-Fernández et al., 2008; Villa et al., 2010).
Detection of Virulence Determinants and Phylogeny of the Isolates
The sequenced isolates were subjected to detailed analysis to determine a clinical relevance or possible transmission from/to hospital settings. K. pneumoniae isolates belonged to four different multilocus sequence types (Table 1), of which two (ST268 and ST307) have previously been described in human clinical specimens (Zhang et al., 2016; Wyres et al., 2019). The present isolates were isolated at water treatment plants, but also in the neighborhood of a slaughterhouse indicating a possible spread of these into the animal population. ST307 K. pneumoniae isolates have emerged worldwide only recently and are supposed to have a high transmission potential, so they are able to quickly move between different countries and even continents (Wyres et al., 2019). They are almost exclusively associated with the carriage of the ESBL gene blaCTX–M–15. The K. pneumoniae isolate 7w-11 (ST268) harbored determinants discriminatory for hypervirulent Klebsiella isolates of the sequence type ST268 (pLVPK plasmid, Integrative conjugative element, Supplementary Figure S3; Zhang et al., 2016). Hypervirulent K. pneumoniae are associated with severe infections in younger and healthier persons than other Klebsiella (Lee et al., 2017).
For the E. coli isolates, eleven different multilocus sequence types were detected (Table 1) of which four (ST10, ST131, ST155, and ST1485) are associated with human clinical infections (Pietsch et al., 2015; Skurnik et al., 2016; Gilrane et al., 2017; Schaufler et al., 2019). For the isolate 9w-1 with the sequence type ST131, fimH-typing was performed in order to investigate its relationship among the ST131 lineages. It harbors the fimH22 allele, therefore placing it into the ST131-H22 lineage which is associated with both human clinical and animal/environmental isolates (Liu et al., 2018).
Other STs have been previously isolated from animal sources [ST167, ST542 (Maamar et al., 2016; Irrgang et al., 2017)]. The sequence types ST10 (n = 2), ST101 and ST648 have been isolated from different sources (humans, animals -wild animals and livestock- and food) (Pietsch et al., 2015; Michael et al., 2017; Irrgang et al., 2018; Schaufler et al., 2019)3. ST155 is mainly found in association with livestock and only recently emerged in humans (Skurnik et al., 2016). These results indicate that water samples harbor isolates from different sources. They may even be able to spread throughout different compartments as their relatives. Five of the above mentioned STs have already been detected in recreational water and/or wastewater samples (ST101, ST10, ST69, ST155, and ST131) in Norway (Jorgensen et al., 2017).
All E. coli isolates harbored between two and nine virulence determinants, mostly involved in adherence and iron acquisition/transport (Supplementary Table S6). The three most common virulence determinants were fdeC (adherence, n = 11), enterobactin (iron acquisition/transport, n = 10) and the ECP operon (adherence, n = 10). According to the virulence gene content, the pathotypes were determined for 7/12 E. coli isolates following the schemes presented by Kaper et al. (2004) and Johnson and Russo (2005). All of these were classified as extra-intestinal pathogenic E. coli (ExPEC). Within these, two ExPEC subtypes could be determined, including neonatal meningitis-causing E. coli (NMEC, 2/12) and uropathogenic E. coli (UPEC, 1/12), respectively. In contrast to the study of Jorgensen et al. (2017) enteropathogenic E. coli (EPEC) were not detected.
The P. aeruginosa isolate 9w-9 was classified as a multidrug-resistant (MDR) isolate. It was of the new ST3304 (one-allele variant of ST274). P. aeruginosa ST274 isolates are of high clinical relevance, as they are known to be associated with severe lung infections in cystic fibrosis patients (Fernández-Olmos et al., 2013). The 9w-9 isolate was found in close proximity to a senior residence, which is concordant with its predominant association with immune-compromised patients. The virulence genes found in this P. aeruginosa isolate were previously described disease-associated genes (Gellatly and Hancock, 2013) for the production of flagella, pili, alginate, pyoverdine, pyocyanin, and the effector proteins ExoS, ExoT, ExoY. Flagella and pili enable adherence to host cells and movement, while the production of alginate is crucial for biofilm formation. Through the use of pyoverdine and pyocyanin, these bacteria can grow in iron-depleted environments, as e.g., blood, and can cause oxidative stress to the host. The type III secretion system is required for the successful excretion of the virulence effector proteins ExoS, ExoT and ExoY that cause host cell death. The presence of all these virulence determinants qualified this particular isolate as a successful pathogen indicating that water may also be a source for these.
The A. baumannii isolates are representative members of ten different sequence types, of which all but one (ST1112) were present only once among the isolates. Five STs have been found in a specific source (Supplementary Table S7). The A. baumannii isolates of the sequence types ST690 and ST1017 have been isolated previously from animals only, while ST155 has been isolated predominantly from animals, but also from humans. ST203 was detected in human isolates and ST647 in environmental isolates. These results indicate that there is a transmission of human and animal A. baumannii isolates to the water bodies. The A. baumannii isolates did not harbor any known virulence genes.
The E. kobei isolate belonging to the E. cloacae complex harbored a blaVIM–1 carbapenemase and had the sequence type ST910. E. cloacae complex isolates are nosocomial pathogens often associated with high resistance and also virulence, especially in immunocompromised patients (Mezzatesta et al., 2012).
A cgMLST-based analysis was performed for A. baumannii, E. coli and K. pneumoniae isolates (n = 27) using BacWGSTdb. For 12/27 isolates (E. coli n = 9, K. pneumoniae, n = 2; A. baumannii, n = 1), close relatives were detected within the threshold of 200 cgMLST allele differences (Figures 3, 4 and Supplementary Table S8). Of the E. coli isolates, 6/9 were closely related to animal isolates, while two isolates were related to E. coli from human sources. The A. baumannii isolate was closely related to an isolate from a human sample, while both K. pneumoniae isolates exhibited a high similarity to isolates of human origin. These results are concordant with data from other European countries that have shown similarity of human isolates with water isolates (Jorgensen et al., 2017).
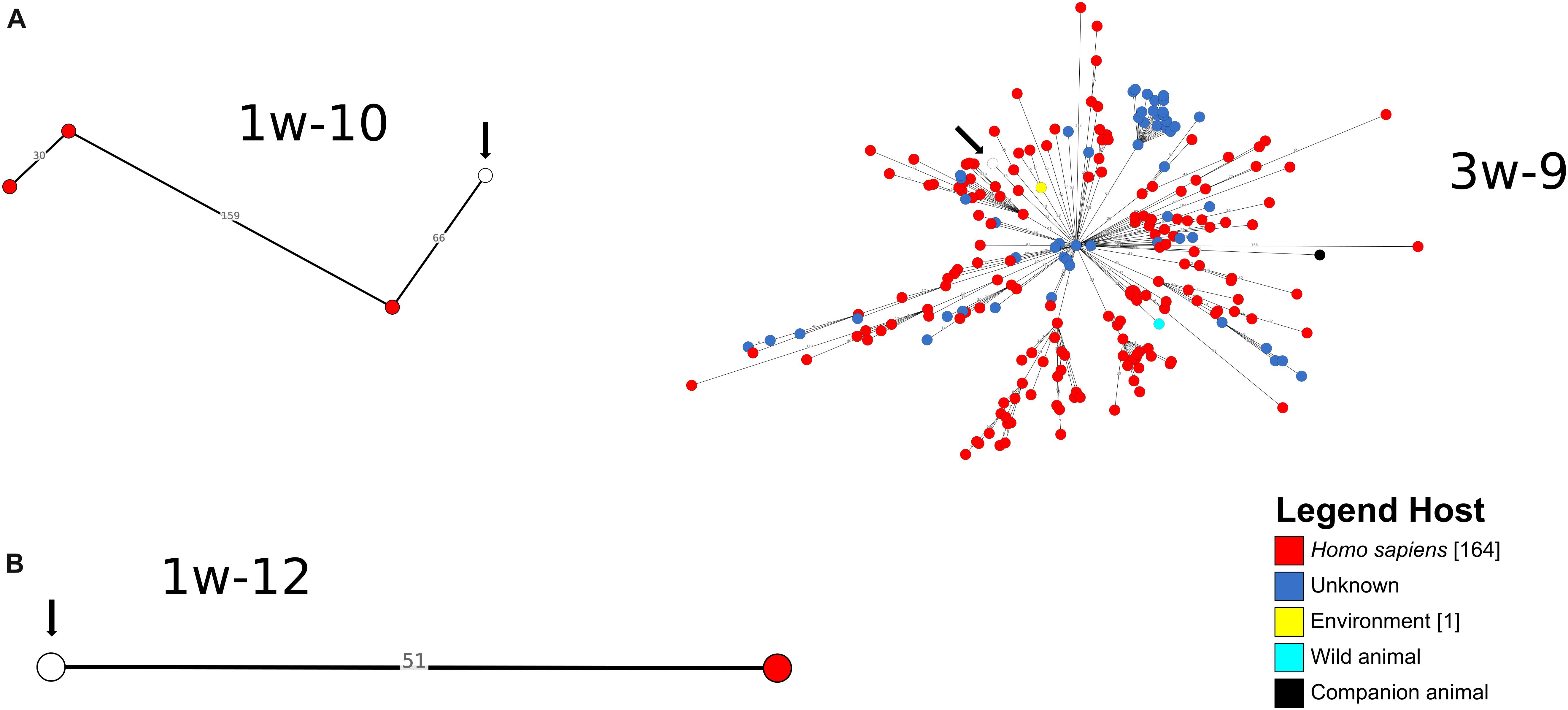
Figure 3. Depiction of cgMLST-based search for closest relatives of sequenced K. pneumoniae (A) and A. baumannii (B) isolates. The search for closest relatives was performed using BacWGSTdb (Ruan and Feng, 2016). Different hosts are marked with different colors of the isolates. The water isolate from this study is indicated with a white circle and an arrow.
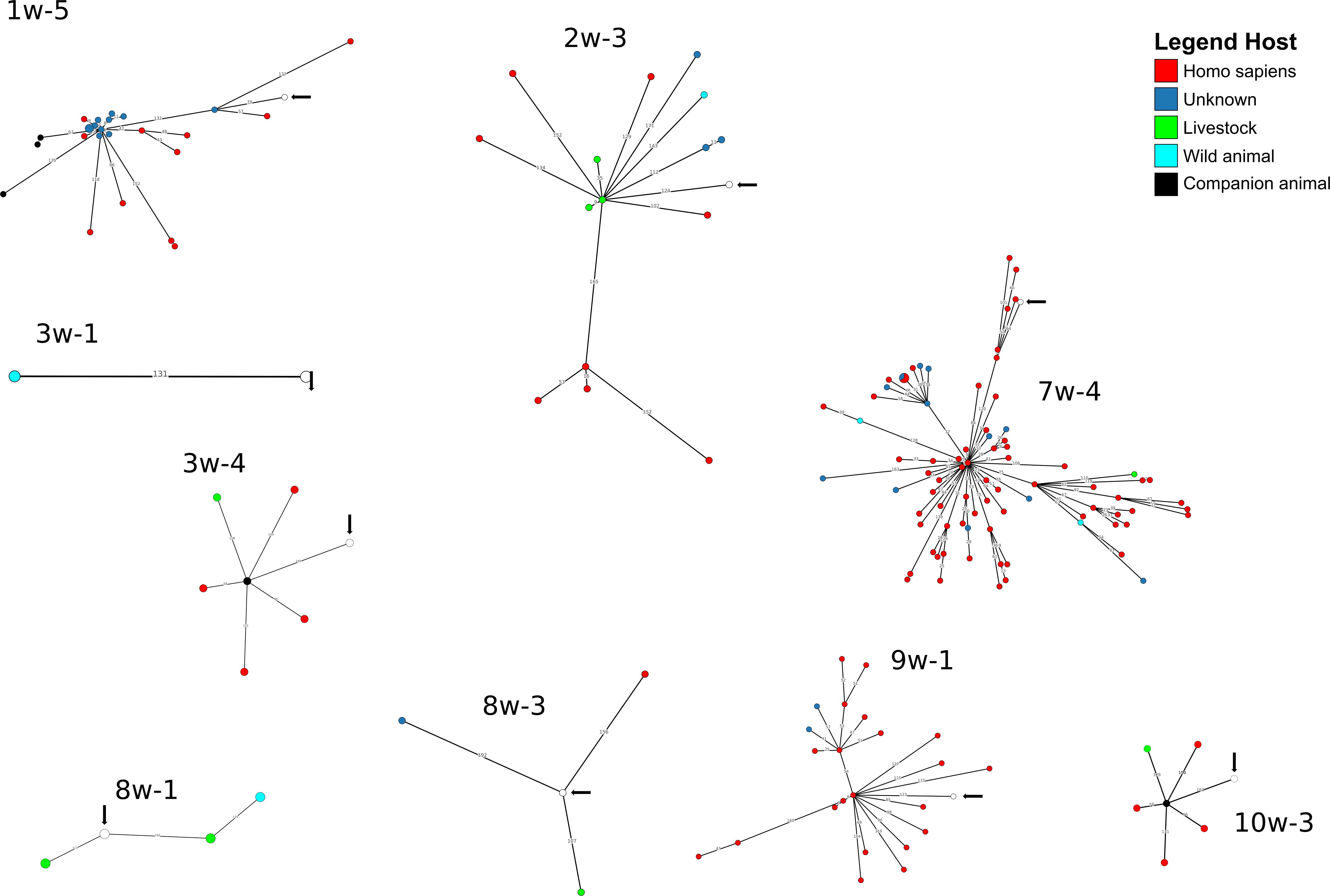
Figure 4. Depiction of cgMLST-based search for closest relatives of sequenced E. coli isolates. The search for closest relatives was performed using BacWGSTdb (Ruan and Feng, 2016). Different hosts are marked with different colors of the isolates. The water isolate from this study is indicated with a white circle and an arrow.
Our study should raise the awareness of the public and the regulatory bodies on the problem of the presence of clinically relevant antibiotic-resistant bacteria in water samples and their impact on human health. Indeed, an associated documentary based on this data (Baars and Lambrecht, 2018) has raised social and political discussions regarding the possible role of water for the dissemination of multidrug resistance in a One health context, and its implication on human health.
The aim of this study was to examine the presence of ESBL/carbapenemase-producing isolates in different water bodies, including recreational water, and to compare their genetic content and relationship to isolates from humans and animals. We were not only able to show that ESBL-, carbapenemase- and mobile colistin resistance gene-encoding isolates are present in water samples, but also that they harbored clinically relevant species displaying high rates of multidrug-resistance. These MDR bacteria were frequently associated with human disease, as for e.g., a hypervirulent K. pneumoniae ST268.
These findings suggest that there is already presence and dispersion of clonal isolates of clinically relevant bacteria in surface waters. In addition, the presence of isolates/antibiotic resistance genes of human and animal origin in water bodies (e.g., blaVIM–1, human; mcr-1, animal) suggests that water itself may be regarded as a reservoir for the transmission and the exchange of isolates and antibiotic resistance genes originating from both human clinical sources and animal husbandry.
The prevalence and the real impact of MDR clinically relevant isolates derived from water bodies on human health is hard to estimate. However, there are reports on outbreaks caused by Carbapenemase-producing Enterobacterales/Enterobacteriaceae deriving from water samples (Carstens et al., 2014) as well as highly-related isolates from water sources and patient samples (Lepuschitz et al., 2019). Thus, there exists a risk of being colonized or, depending on the individual health status and the intensity of exposure, even infected through contact with contaminated water.
Our study has some limitations. Firstly, only ESBL/Carbapenemase-producers in the water samples were determined but not the overall number of isolates. Thus, the prevalence of ESBL/Carbapenemase-producers cannot be determined. Secondly, the study was designed as an observational study; therefore, the number of sequenced isolates was too low to perform statistical analyses.
Conclusion
As clinically relevant antibiotic-resistant Gram-negative isolates were detected in the water samples examined, it is important to increase our knowledge concerning water sources as reservoirs and disseminators of such isolates. Additional investigations to quantify the transmission between the different environmental compartments are now highly warranted.
Data Availability Statement
The datasets generated and analyzed for this study can be found in the European Nucleotide Archive (ENA) under the project accession number PRJEB29745.
Author Contributions
LF, CB, OL, TB, SH, TC, and CI designed the study. CB and OL collected the data and samples. SH and TB processed the samples. LF performed antibiotic resistance and species determination. LF, JF, TC, and CI performed WGS of the isolates. LF, OS, JS, CB, OL, JF, TC, and CI analyzed the data. LF, TC, and CI wrote the manuscript that was critically reviewed and approved by all authors.
Funding
This work was carried out by grants from the Bundesministerium für Bildung und Forschung (BMBF, Germany) within the German Center for Infection Research (DZIF/grant numbers 8032808811, 8032808818, and 8032808820 to TC/CI). Support was also obtained from the Hessian Ministry of Higher Education, Research and Arts within the project HuKKH (Hessisches universitaeres Kompetenzzentrum Krankenhaushygiene). SH receives funding from the German Research Foundation (grant HE8047/1). The sampling sites were determined by the investigative journalists (CB and OL).
Conflict of Interest
The authors declare that this study received funding from Norddeutscher Rundfunk, Germany. The funder had the following involvement in the study: study design, data collection, and analysis. LF received lecture fees from VTA, Austria.
Acknowledgments
We thank Christina Gerstmann for excellent technical assistance. We also thank the team of curators of the Institut Pasteur Acinetobacter MLST system for curating the MLST data and making them publicly available at http://pubmlst.org/abaumannii/.
Supplementary Material
The Supplementary Material for this article can be found online at: https://www.frontiersin.org/articles/10.3389/fmicb.2019.02779/full#supplementary-material
Footnotes
- ^ https://bigsdb.pasteur.fr/cgi-bin/bigsdb/bigsdb.pl?db=pubmlst_klebsiella_seqdef_public
- ^ https://pubmlst.org/abaumannii/
- ^ https://enterobase.warwick.ac.uk/species/index/ecoli
References
Alikhan, N. F., Zhou, Z., Sergeant, M. J., and Achtman, M. (2018). A genomic overview of the population structure of Salmonella. PLoS Genet. 14:e07261. doi: 10.1371/journal.pgen.1007261
Baars, C., and Lambrecht, O. (2018). Auf der Spur der Superkeime. Available at: https://www.ndr.de/fernsehen/sendungen/panorama_die_reporter/Auf-der-Spur-der-Superkeime,sendung756524.html (accessed November 20, 2019).
Bankevich, A., Nurk, S., Antipov, D., Gurevich, A. A., Dvorkin, M., Kulikov, A. S., et al. (2012). SPAdes: a new genome assembly algorithm and its applications to single-cell sequencing. J. Comput. Biol. 19, 455–477. doi: 10.1089/cmb.2012.0021
Berendonk, T. U., Manaia, C. M., Merlin, C., Fatta-Kassinos, D., Cytryn, E., Walsh, F., et al. (2015). Tackling antibiotic resistance: the environmental framework. Nat. Rev. Microbiol. 13, 310–317. doi: 10.1038/nrmicro3439
Carstens, A., Kepper, U., Exner, M., Hauri, A., and Kaase, M. (2014). Plasmid-vermittelter Multispezies-Ausbruch mit Carbapenem-resistenten Enterobacteriaceae. Epidemiol. Bull. 47, 455–462.
Chen, L., Zheng, D., Liu, B., Yang, J., and Jin, Q. (2016). VFDB 2016: hierarchical and refined dataset for big data analysis - 10 years on. Nucleic Acids Res. 44, D694–D697. doi: 10.1093/nar/gkv1239
Diancourt, L., Passet, V., Nemec, A., Dijkshoorn, L., and Brisse, S. (2010). The population structure of Acinetobacter baumannii: expanding multiresistant clones from an ancestral susceptible genetic pool. PLoS One 5:e010034. doi: 10.1371/journal.pone.0010034
Falgenhauer, L., Imirzalioglu, C., Ghosh, H., Gwozdzinski, K., Schmiedel, J., Gentil, K., et al. (2016a). Circulation of clonal populations of fluoroquinolone-resistant CTX-M-15-producing Escherichia coli ST410 in humans and animals in Germany. Int. J. Antimicrob. Agents 47, 457–465. doi: 10.1016/j.ijantimicag.2016.03.019
Falgenhauer, L., Waezsada, S.-E., Gwozdzinski, K., Ghosh, H., Doijad, S., Bunk, B., et al. (2016b). Chromosomal locations of mcr-1 and blaCTX–M–15 in Fluoroquinolone-resistant Escherichia coli ST410. Emerg. Infect. Dis. 22, 1689–1691. doi: 10.3201/eid2209.160692
Fernández-Olmos, A., García-Castillo, M., María Alba, J., Morosini, M. I., Lamas, A., Romero, B., et al. (2013). Population structure and Antimicrobial susceptibility of both nonpersistent and persistent Pseudomonas aeruginosa isolates recovered from cystic fibrosis patients. J. Clin. Microbiol. 51, 2761–2765. doi: 10.1128/JCM.00802-813
García-Fernández, A., Chiaretto, G., Bertini, A., Villa, L., Fortini, D., Ricci, A., et al. (2008). Multilocus sequence typing of IncI1 plasmids carrying extended-spectrum beta-lactamases in Escherichia coli and Salmonella of human and animal origin. J. Antimicrob. Chemother. 61, 1229–1233. doi: 10.1093/jac/dkn131
Gellatly, S. L., and Hancock, R. E. W. (2013). Pseudomonas aeruginosa: new insights into pathogenesis and host defenses. Pathog. Dis. 67, 159–173. doi: 10.1111/2049-632X.12033
Gilrane, V. L., Lobo, S., Huang, W., Zhuge, J., Yin, C., Chen, D., et al. (2017). Complete genome sequence of a colistin-resistant Escherichia coli strain haboring mcr-1 on an IncHI2 plasmid in the United States. Genome Announc. 5:e1095-17. doi: 10.1128/genomeA.01095-17
Gomi, R., Matsuda, T., Yamamoto, M., Chou, P.-H., Tanaka, M., Ichiyama, S., et al. (2018). Characteristics of Carbapenemase-producing Enterobacteriaceae in wastewater revealed by Genomic analysis. Antimicrob. Agents Chemother. 62:e2501-17. doi: 10.1128/AAC.02501-2517
Grundmann, H., Glasner, C., Albiger, B., Aanensen, D. M., Tomlinson, C. T., Andrasević, A. T., et al. (2017). Occurrence of carbapenemase-producing Klebsiella pneumoniae and Escherichia coli in the European survey of carbapenemase-producing Enterobacteriaceae (EuSCAPE): a prospective, multinational study. Lancet Infect. Dis. 17, 1–11. doi: 10.1016/S1473-3099(16)30257-30252
Hembach, N., Schmid, F., Alexander, J., Hiller, C., Rogall, E. T., and Schwartz, T. (2017). Occurrence of the mcr-1 Colistin Resistance Gene and other Clinically Relevant Antibiotic Resistance Genes in Microbial Populations at Different Municipal Wastewater Treatment Plants in Germany. Front. Microbiol. 8:1282. doi: 10.3389/fmicb.2017.01282
Heß, S., Berendonk, T. U., and Kneis, D. (2018). Antibiotic resistant bacteria and resistance genes in the bottom sediment of a small stream and the potential impact of remobilization. FEMS Microbiol. Ecol. 94:fiy128. doi: 10.1093/femsec/fiy128
Hölzel, C. S., Tetens, J. L., and Schwaiger, K. (2018). Unraveling the role of vegetables in spreading Antimicrobial-resistant bacteria: a need for quantitative risk assessment. Foodborne Pathog. Dis. 15, 671–688. doi: 10.1089/fpd.2018.2501
Huijbers, P. M. C., Blaak, H., De Jong, M. C. M., Graat, E. A. M., Vandenbroucke-Grauls, C. M. J. E., and De Roda Husman, A. M. (2015). Role of the environment in the transmission of antimicrobial resistance to humans: a review. Environ. Sci. Technol. 49, 11993–12004. doi: 10.1021/acs.est.5b02566
Irrgang, A., Falgenhauer, L., Fischer, J., Ghosh, H., Guiral, E., Guerra, B., et al. (2017). CTX-M-15-producing E. coli isolates from food products in Germany are mainly associated with an IncF-type plasmid and belong to two predominant clonal E. coli Lineages. Front. Microbiol. 8:2318. doi: 10.3389/fmicb.2017.02318
Irrgang, A., Hammerl, J. A., Falgenhauer, L., Guiral, E., Schmoger, S., Imirzalioglu, C., et al. (2018). Diversity of CTX-M-1-producing E. coli from German food samples and genetic diversity of the blaCTX-M-1 region on IncI1 ST3 plasmids. Vet. Microbiol. 221, 98–104. doi: 10.1016/j.vetmic.2018.06.003
Johnson, J. R., and Russo, T. A. (2005). Molecular epidemiology of extraintestinal pathogenic (uropathogenic) Escherichia coli. Int. J. Med. Microbiol. 295, 383–404. doi: 10.1016/j.ijmm.2005.07.005
Jorgensen, S. B., Soraas, A. V., Arnesen, L. S., Leegaard, T. M., Sundsfjord, A., and Jenum, P. A. (2017). A comparison of extended spectrum beta-lactamase producing Escherichia coli from clinical, recreational water and wastewater samples associated in time and location. PLoS One 12:e0186576. doi: 10.1371/journal.pone.0186576
Kaper, J. B., Nataro, J. P., and Mobley, H. L. (2004). Pathogenic Escherichia coli. Nat. Rev. Microbiol. 2, 123–140. doi: 10.1038/nrmicro818
Kittinger, C., Kirschner, A., Lipp, M., Baumert, R., Mascher, F., Farnleitner, A., et al. (2017). Antibiotic resistance of Acinetobacter spp. isolates from the river danube: susceptibility stays high. Int. J. Environ. Res. Public Health 15:52. doi: 10.3390/ijerph15010052
Lee, C. R., Lee, J. H., Park, K. S., Jeon, J. H., Kim, Y. B., Cha, C. J., et al. (2017). Antimicrobial resistance of hypervirulent Klebsiella pneumoniae: epidemiology, hypervirulence-associated determinants, and resistance mechanisms. Front. Cell. Infect. Microbiol. 7:483. doi: 10.3389/fcimb.2017.00483
Lepuschitz, S., Schill, S., Stoeger, A., Pekard-Amenitsch, S., Huhulescu, S., Inreiter, N., et al. (2019). Whole genome sequencing reveals resemblance between ESBL-producing and carbapenem resistant Klebsiella pneumoniae isolates from Austrian rivers and clinical isolates from hospitals. Sci. Total Environ. 662, 227–235. doi: 10.1016/j.scitotenv.2019.01.179
Liu, C. M., Stegger, M., Aziz, M., Johnson, T. J., Waits, K., Nordstrom, L., et al. (2018). Escherichia coli ST131-H22 as a foodborne uropathogen. mBio 9:e470-18. doi: 10.1128/mBio.00470-18
Maamar, E., Hammami, S., Andrea, C., Dakhli, N., Salah, M., Ferjani, S., et al. (2016). High prevalence of extended-spectrum and plasmidic AmpC beta-lactamase-producing Escherichia coli from poultry in Tunisia. Int. J. Food Microbiol. 231, 69–75. doi: 10.1016/j.ijfoodmicro.2016.05.001
Madec, J. Y., Haenni, M., Nordmann, P., and Poirel, L. (2017). Extended-spectrum β-lactamase/AmpC- and carbapenemase-producing Enterobacteriaceae in animals: a threat for humans? Clin. Microbiol. Infect. 23, 826–833. doi: 10.1016/j.cmi.2017.01.013
Magiorakos, A.-P. P., Srinivasan, A., Carey, R. B., Carmeli, Y., Falagas, M. E., Giske, C. G., et al. (2012). Multidrug-resistant, extensively drug-resistant and pandrug-resistant bacteria: an international expert proposal for interim standard definitions for acquired resistance. Clin. Microbiol. Infect. 18, 268–281. doi: 10.1111/j.1469-0691.2011.03570.x
Mahfouz, N., Caucci, S., Achatz, E., Semmler, T., Guenther, S., Berendonk, T. U., et al. (2018). High genomic diversity of multi- drug resistant wastewater Escherichia coli. Sci. Rep. 8:8928. doi: 10.1038/s41598-018-27292-27296
Meier-Kolthoff, J. P., Auch, A. F., Klenk, H. P., and Göker, M. (2013). Genome sequence-based species delimitation with confidence intervals and improved distance functions. BMC Bioinformatics 14:60. doi: 10.1186/1471-2105-14-60
Mezzatesta, M. L., Gona, F., and Stefani, S. (2012). Enterobacter cloacae complex: clinical impact and emerging antibiotic resistance. Future Microbiol. 7, 887–902. doi: 10.2217/fmb.12.61
Michael, G. B., Kaspar, H., Siqueira, A. K., de Freitas Costa, E., Corbellini, L. G., Kadlec, K., et al. (2017). Extended-spectrum β-lactamase (ESBL)-producing Escherichia coli isolates collected from diseased food-producing animals in the GERM-Vet monitoring program 2008–2014. Vet. Microbiol. 200, 142–150. doi: 10.1016/j.vetmic.2016.08.023
Müller, H., Sib, E., Gajdiss, M., Klanke, U., Lenz-Plet, F., Barabasch, V., et al. (2018). Dissemination of multi-resistant Gram-negative bacteria into German wastewater and surface waters. FEMS Microbiol. Ecol. 94:fiy057. doi: 10.1093/femsec/fiy057
Muraleedharan, C., Talreja, D., Kanwar, M., Kumar, A., and Walia, S. K. (2019). Occurrence of extended-spectrum β-lactamase-producing bacteria in urban Clinton River habitat. J. Glob. Antimicrob. Resist. 16, 225–235. doi: 10.1016/j.jgar.2018.10.007
Pietsch, M., Eller, C., Wendt, C., Holfelder, M., Falgenhauer, L., Fruth, A., et al. (2015). Molecular characterisation of extended-spectrum β-lactamase (ESBL)-producing Escherichia coli isolates from hospital and ambulatory patients in Germany. Vet. Microbiol. 200, 130–137. doi: 10.1016/j.vetmic.2015.11.028
Roer, L., Tchesnokova, V., Allesøe, R., Muradova, M., Chattopadhyay, S., Ahrenfeldt, J., et al. (2017). Development of a web tool for Escherichia coli sub-typing based on fimH alleles. J. Clin. Microbiol. 55, 2538–2543. doi: 10.1128/JCM.00737-17
Ruan, Z., and Feng, Y. (2016). BacWGSTdb, a database for genotyping and source tracking bacterial pathogens. Nucleic Acids Res. 44, D682–D687. doi: 10.1093/nar/gkv1004
Schaufler, K., Semmler, T., Wieler, L. H., Trott, D. J., Pitout, J., Peirano, G., et al. (2019). Genomic and functional analysis of emerging virulent and multi-drug resistant E. coli lineage ST648. Antimicrob. Agents Chemother. 63:AAC.00243-219. doi: 10.1128/AAC.00243-219
Schwarz, S., and Johnson, A. P. (2016). Transferable resistance to colistin: a new but old threat. J. Antimicrob. Chemother. 71, 2066–2070. doi: 10.1093/jac/dkw274
Schwengers, O., Hoek, A., Fritzenwanker, M., Falgenhauer, L., Hain, T., Chakraborty, T., et al. (2019). ASA3P: an automatic and scalable pipeline for the assembly, annotation and higher level analysis of closely related bacterial isolates. bioRxiv [Preprint]. doi: 10.1101/654319
Skurnik, D., Clermont, O., Guillard, T., Launay, A., Danilchanka, O., Pons, S., et al. (2016). Emergence of antimicrobial-resistant Escherichia coli of animal origin spreading in humans. Mol. Biol. Evol. 33, 898–914. doi: 10.1093/molbev/msv280
Thomsen, M. C. F., Ahrenfeldt, J., Cisneros, J. L. B., Jurtz, V., Larsen, M. V., Hasman, H., et al. (2016). A bacterial analysis platform: an integrated system for analysing bacterial whole genome sequencing data for clinical diagnostics and surveillance. PLoS One 11:e0157718. doi: 10.1371/journal.pone.0157718
Villa, L., García-Fernández, A., Fortini, D., and Carattoli, A. (2010). Replicon sequence typing of IncF plasmids carrying virulence and resistance determinants. J. Antimicrob. Chemother. 65, 2518–2529. doi: 10.1093/jac/dkq347
Wirth, T., Falush, D., Lan, R., Colles, F., Mensa, P., Wieler, L. H., et al. (2006). Sex and virulence in Escherichia coli: an evolutionary perspective. Mol. Microbiol. 60, 1136–1151. doi: 10.1111/j.1365-2958.2006.05172.x
Woodford, N., Wareham, D. W., Guerra, B., and Teale, C. (2014). Carbapenemase-producing Enterobacteriaceae and non-Enterobacteriaceae from animals and the environment: an emerging public health risk of our own making? J. Antimicrob. Chemother. 69, 287–291. doi: 10.1093/jac/dkt392
World Health Organization [WHO] (2017). Global Priority List Of Antibiotic-Resistant Bacteria To Guide Research, Discovery, And Development Of New Antibiotics. Geneva: World Health Organization.
Wyres, K. L., Hawkey, J., Hetland, M. A. K., Fostervold, A., Wick, R. R., Judd, L. M., et al. (2019). Emergence and rapid global dissemination of CTX-M-15-associated Klebsiella pneumoniae strain ST307. J. Antimicrob. Chemother. 74, 577–581. doi: 10.1093/jac/dky492
Zhang, Y., Zhao, C., Wang, Q., Wang, X., Chen, H., Li, H., et al. (2016). High prevalence of hypervirulent Klebsiella pneumoniae infection in China: geographic distribution, clinical characteristics, and antimicrobial resistance. Antimicrob Agents Chemother. Chemother. 60, 6115–6120. doi: 10.1128/AAC.01127-16.Address
Zurfuh, K., Poirel, L., Nordmann, P., Nuesch-Inderbinen, M., Hachler, H., Stephan, R., et al. (2016). Occurrence of the plasmid-borne mcr-1 colistin resistance gene in ESBL-producing Enterobacteriacae in river water and imported vegetable samples in Switzerland. Antimicrob. Agents Chemother. 60, 2594–2595. doi: 10.1128/AAC.00066-16
Keywords: ESBL, surface water, WGS (whole genome sequencing), MCR-1, clinical isolate
Citation: Falgenhauer L, Schwengers O, Schmiedel J, Baars C, Lambrecht O, Heß S, Berendonk TU, Falgenhauer J, Chakraborty T and Imirzalioglu C (2019) Multidrug-Resistant and Clinically Relevant Gram-Negative Bacteria Are Present in German Surface Waters. Front. Microbiol. 10:2779. doi: 10.3389/fmicb.2019.02779
Received: 01 July 2019; Accepted: 14 November 2019;
Published: 29 November 2019.
Edited by:
Rustam Aminov, University of Aberdeen, United KingdomReviewed by:
Maria Blanca Sanchez, Instituto IMDEA Agua, SpainZhi Ruan, Zhejiang University, China
Jana Jass, Örebro University, Sweden
Copyright © 2019 Falgenhauer, Schwengers, Schmiedel, Baars, Lambrecht, Heβ, Berendonk, Falgenhauer, Chakraborty and Imirzalioglu. This is an open-access article distributed under the terms of the Creative Commons Attribution License (CC BY). The use, distribution or reproduction in other forums is permitted, provided the original author(s) and the copyright owner(s) are credited and that the original publication in this journal is cited, in accordance with accepted academic practice. No use, distribution or reproduction is permitted which does not comply with these terms.
*Correspondence: Linda Falgenhauer, Linda.falgenhauer@mikrobio.med.uni-giessen.de