- 1Faculty of Chemistry, Biotechnology and Food Science, Norwegian University of Life Sciences, Ås, Norway
- 2International Crops Research Institute for the Semi-Arid Tropics, Addis Ababa, Ethiopia
Emissions of the potent greenhouse gas N2O is one of the environmental problems associated with intensive use of synthetic N fertilizers, and novel N2O mitigation strategies are needed to minimize fertilizer applications and N2O release without affecting agricultural efficiencies. Increased incorporation of legume crops in agricultural practices offers a sustainable alternative. Legumes, in their symbiosis with nitrogen fixing bacteria, rhizobia, reduce the need for fertilizers and also respond to the need for increased production of plant-based proteins. Not all combinations of rhizobia and legumes result in efficient nitrogen fixation, and legume crops therefore often need to be inoculated with compatible rhizobial strains. Recent research has demonstrated that some rhizobia are also very efficient N2O reducers. Several nutritionally and economically important legumes form root nodules in symbiosis with bacteria belonging to Bradyrhizobium. Here, the host-ranges of fourteen N2O reducing Bradyrhizobium strains were tested on six legume hosts; cowpea, groundnut, mung bean, haricot bean, soybean, and alfalfa. The plants were grown for 35 days in pots in sterile sand supplemented with N-free nutrient solution. Cowpea was the most promiscuous host nodulated by all test strains, followed by groundnut (11 strains) and mungbean (4 strains). Three test strains were able to nodulate all these three legumes, while none nodulated the other three hosts. For cowpea, five strains increased the shoot dry weight and ten strains the shoot nitrogen content (pairwise comparison; p < 0.05). For groundnut the corresponding results were three and nine strains. The symbiotic effectiveness for the different strains ranged from 45 to 98% in cowpea and 34 to 95% in groundnut, relative to fertilized controls. The N2O reduction capacity of detached nodules from cowpea plants inoculated with one of these strains confirmed active N2O reduction inside the nodules. When released from senescent nodules such strains are expected to also act as sinks for N2O produced by denitrifying organisms in the soil microbial community. Our strategy to search among known N2O-reducing Bradyrhizobium strains for their N2-fixation effectiveness successfully identified several strains which can potentially be used for the production of legume inoculants with the dual capacities of efficacious N2-fixation and N2O reduction.
Introduction
Synthetic fertilizers are the major sources of fixed N in most agricultural systems and have contributed to boosting crop productivity since the beginning of the green revolution (Kox and Jetten, 2015). Excessive use of fertilizer, however, comes with environmental risks and has created problems such as pollution of water bodies and emission of nitrous oxide (N2O), a potent greenhouse gas and destructor of the stratospheric ozone layer (Ravishankara et al., 2009; Montzka et al., 2011; Reay et al., 2012; Butterbach-Bahl et al., 2013). Agriculture is a major source of N2O, and it has been estimated that more than 60% of the N2O emitted into the atmosphere is derived from N fertilized agricultural soils, mainly through the microbial processes of denitrification and nitrification (Thomson et al., 2012). Biological N2 fixation is an environmentally friendly alternative to synthetic fertilizers (Jensen and Hauggaard-Nielsen, 2003). Legumes in symbiosis with N2 fixing bacteria (rhizobia) account for the major part of the biological N2 fixation, with rates in different agricultural systems being about 115 kg N ha–1 year–1 for crop legumes and 110–227 for pasture and fodder legumes (Herridge et al., 2008). In comparison, other endophytic and free-living bacteria fixed <5 to 25 kg N ha–1 year–1. Thus, the incorporation of legumes in pastures and in crop rotation practices, or intercropping legumes with other plants, will lessen the need for synthetic N fertilizers (Dakora et al., 1987; Peoples et al., 2009; Jensen et al., 2012) and thereby contribute in the battle against N2O emissions.
The establishment of the legume-rhizobium symbiosis is based on a highly specialized signaling system, leading to the development of root nodules in the plant cortex. In most cases, rhizobia gain entrance to the plant via an infection thread formed in the root hairs, but in some plants, for example groundnut, the rhizobia instead enter through wounds in the root. The rhizobia then enter the cytoplasm of plant cells in the nodule where they, surrounded by plant-derived membranes, will differentiate into bacteroids. These are specialized bacterial cells that fix N2 into NH3, which is used by the plant for the synthesis of amino acids and proteins (Poole et al., 2018). Rhizobia vary in the type of hosts they nodulate. Some strains are highly specific, nodulating only a limited number of hosts, while others are promiscuous with a wide host range (Pueppke and Broughton, 1999). Moreover, some combinations of plant–rhizobia are efficient, others not, and the nitrogen fixation effectiviness may vary even between closely related plant cultivars/bacterial strains. Since soils may lack matching and/or effective rhizobia for certain legumes, especially when newly introduced to an area (Rodríguez-Echeverría et al., 2012), seeds of many legume crops need to be inoculated with compatible rhizobia. Strain screening and selection is an important step when developing new inoculants that are better specialized for selected crops and agricultural regions, aiming to optimize yields and plant nitrogen content.
Another feature of some rhizobia is their ability to denitrify, i.e., use nitrogen oxides as electron acceptors for respiration under anoxic conditions. Rhizobia with a complete denitrification pathway carry genes coding for the periplasmic nitrate reductase NapAB, the copper (Cu) containing nitrite reductase NirK, a cytochrome c-dependent nitric oxide reductase NorCB and a clade I NosZ (Bedmar et al., 2005; Sameshima-Saito et al., 2006; Torres et al., 2014; Mania et al., 2019). Genes for the membrane-bound NarG appear to be lacking (Mania et al., 2019), while a cd1-type nitrite reductase NirS has been reported in a few strains (Sánchez and Minamisawa, 2018). Denitrification has hitherto only been investigated in a limited number of rhizobia, and it is therefore not known how widespread this metabolism is in this group of organisms. Incomplete denitrification pathways lacking one or more of the reduction steps have been reported for strains of Ensifer, Mesorhizobium, and Bradyrhizobium (Falk et al., 2010; Bueno et al., 2015; Mania et al., 2019). Depending on if they carry and express the gene nosZ, coding for NosZ, different strains of rhizobia may serve either as sources or sinks for N2O emission. Thus, some rhizobia may have the potential to both enhance crop production and mitigate N2O emission, serving to counteract both problems of agricultural productivity and environmental risks associated with the use of synthetic N fertilizers. Among the N2O reducing rhizobia with such potentials are members of the genus Bradyrhizobium, of which many strains can form effective nodules with a wide range of economically important legume plants such as cowpea, peanut, soybean, and mungbean. Reports from greenhouse and field experiments demonstrate that inoculation of soybean with N2O-reducing strains of Bradyrhizobium can mitigate N2O emissions (Hénault and Revellin, 2011; Itakura et al., 2013; Akiyama et al., 2016). These findings are promising, and call for investigations of a wider range of rhizobia to identify N2O reducing strains that can be further investigated for their suitability as inoculants for different legumes.
In a recent study, we screened 39 Bradyrhizobium strains, isolated from legume trees and herbal crops in Ethiopia, and characterized them with respect to their taxonomy and phylogeny (Wolde-Meskel et al., 2004b, 2005). Among them, we found 20 Bradyrhizobium strains capable of denitrifying NO3– to N2 (Mania et al., 2019). The phylogeny of these strains had been analyzed through multilocus sequence analyses (MLSA), which clustered them into seven genospecies (Degefu et al., 2017). The study by Mania et al. (2019) showed that 18 of the complete denitrifiers belonged to three different genospecies within the Bradyrhizobium japonicum superclade, while the other two belonged to the Bradyrhizobium elkanii superclade (genospecies defined by Degefu et al., 2017). When supplied with both NO3– and N2O, these strains all showed a strong preference for reduction of N2O over NO3–, probably because the electron transport pathway to NosZ competes very efficiently for electrons compared to the pathway to NapAB (Mania et al., 2019). The strong capacity to reduce N2O was characteristic for all the complete denitrifying bradyrhizobia tested.
Aiming to find bradyrhizobia with the dual capacity of efficacious N2-fixation and N2O reduction, we here investigated the host ranges of 14 of these strongly N2O reducing Bradyrhizobium strains (Mania et al., 2019), all clustered in genospecies I (Degefu et al., 2017), against six legume hosts: cowpea (Vigna unguiculata), groundnut (Arachis hypogea), mung bean (Vigna radiata), soybean (Glycine max), haricot bean (Phaseolus vulgaris), and alfalfa (Medicago sativa). We also determined their symbiotic N2 fixation effectiveness in cowpea (12 strains) and groundnut (11 strains) and compared the symbiotic performance of six of the best performing strains with cowpea seeds collected from three locations in Ethiopia (Hawassa, Ziway, and Bale). Furthermore, we investigated the N2O reduction capacity of detached cowpea nodules formed by two different Bradyrhizobium strains, AC29c and AC70c. A major outcome of our study was that several of the N2O reducing Bradyrhizobium test strains fixed N2 effectively with different legume hosts. Such organisms are promising candidates for sustainable legume crop production and may simultaneously serve as a sink for N2O emission from the legume rhizosphere.
Materials and Methods
Bradyrhizobium Strains and Growth Conditions
Fourteen of the 39 Bradyrhizobium strains previously isolated from the tree and crop legumes growing in Ethiopia (Wolde-Meskel et al., 2004b) and capable of N2O reduction (Mania et al., 2019) were used (Table 1). Each strain was grown at 28°C for 6 days on yeast mannitol agar (YMA). A single colony was then aseptically picked and cultured to a cell density of ≈109 cells ml–1 in a test tube containing 10 ml yeast mannitol broth (YMB) at 28°C. The YMA/YMB medium was as described in Mania et al. (2019).
Plant Growth, Host Range, and Symbiotic Effectiveness
The experiments were performed at Hawassa University, Ethiopia, in a polyhouse (a type of “shadehouse” with polyethylene roof and walls and fences to prevent animals from entering), at the temperature and humidity of the surrounding environment. We used six grain legume hosts: cowpea (local landrace, Hawassa, Ethiopia), groundnut (local landrace, Gofa, Ethiopia), mung bean (local landrace, Gofa), soybean (variety Ethio-Yugoslavia), haricot bean (variety Hawassa Dume) and alfalfa (variety 1086). Seeds were surface sterilized by immersion in 70% ethanol for 1 min, then transferred to a 3% sodium hypochlorite solution for 1 min followed by rinsing them in six changes of sterile water. Sterility was verified by streaking 2–3 seeds from each germination plate onto YMB agar. Sterilized seeds were imbibed in water for 1 h and subsequently incubated for germination at 28°C on sterile petri dishes lined with moist tissue paper for 3–4 days, or until the emergence of the radicle. Plants were grown in modified plastic cups with two separate parts: the lower part providing a reservoir for nutrient solution, and the upper part filled with washed and sterilized river sand, with a centrally positioned cotton wick extending through a hole out to the reservoir used as a rooting medium (Yates et al., 2016). Two germinated seeds were transferred to each plastic pot and thinned to one after successful seedling establishment. The experiments were carried out with three replicates using a randomized complete block design. The seedlings in each pot were inoculated with 1 ml (approximately 109 cells ml–1) of rhizobial culture. Non-inoculated seedlings, either supplied with mineral nitrogen (as 0.05 g L–1 KNO3 weekly) or grown without nitrogen, were used as positive and negative controls, respectively. The seedlings inoculated with rhizobia were supplied with sterile, quarter-strength of Jensen’s modified N-free nutrient solution twice per week and with sterilized, distilled water as necessary (Somasegaran and Hoben, 1994).
Host ranges were assessed by scoring the number of root nodules after carefully uprooting the whole plant 35 days after inoculation. Symbiotic effectiveness of the strains was determined by measuring the nodule number (NN), nodule dry weight (NDW), and shoot dry weight (SDW) as described by Somasegaran and Hoben (1994). Plant shoot samples were oven-dried at 70°C for 48 h to determine the SDW, then ground to a fine powder to determine their nitrogen content using a LECO Truspec carbon, hydrogen and nitrogen analyzer (St. Joseph, MI, United States). The shoot nitrogen content is given as SN (%) of the sample dry weight according to the Dumas method (Nelson and Sommers, 1996). The symbiotic effectiveness (SE) was estimated as the percentage of SDW of the plants supplied with N (+N control) (Yates et al., 2016). Shoot nitrogen was estimated as percent N2 fixed from the atmosphere (Ndfa %): [(SNinoculated − SNuninoculated)/SNinoculated] × 100. Since the plants were grown on sterile sand medium not supplied with an external source of nitrogen, the N accumulated in the plant shoots was assumed to be derived from the atmosphere through symbiotic N2 fixation. The shoot nitrogen content of the uninoculated plants (SNuninoculated) was considered to be equal to the N content of the seeds (Rennie, 1984). Average values ± standard deviations (n = 3) are reported if not otherwise stated. Comparisons of the symbiotic effectiveness in the different treatments were done using analysis of variance (ANOVA) and Tukey’s HD test. Correlations between the different parameters for symbiotic effectiveness were determined by Pearson’s correlation coefficient.
Symbiotic Effectiveness of the Best Performing Strains in Cowpea Plants
After the first round of experiments, the six Bradyrhizobium strains that performed best with the cowpea landraces collected from Hawassa (located about 175 miles south of the Ethiopian capital Addis Ababa) were tested against two more cowpea landraces collected from Ziway (100 miles south of Addis Ababa) and Bale (300 miles south-east of Addis Ababa). Procedures for determining the N2 fixation effectiveness and shoot N were as described previously.
N2O Reduction Capacity by Intact Legume Nodules
We also investigated the capacity of bradyrhizobia to reduce N2O when living inside nodules. This experiment was performed in a temperature-controlled greenhouse facility at the Norwegian University of Life Sciences, Norway. To obtain nodules, cowpea seeds were sterilized and germinated as described above. Seedlings were planted in pots with vermiculite as rooting medium and inoculated with either the Bradyrhizobium strain AC29c, which is incapable of reducing N2O, or with the N2O-reducing Bradyrhizobium strain AC70c (Mania et al., 2019). Plants were uprooted 40 days after inoculation and nodules were cut from the roots and immediately washed with sterile distilled water. Equal numbers of healthy-looking nodules of approximately similar size were placed in three 120 ml sample vials. For each vial, nodules from three plants were pooled to obtain measurable N2O reduction rates, thus nine plants were used in total. The dry weight of the nodules in each vial was approximately 18.9 mg. The vials were sealed with gas-tight butyl rubber septa and flushed with Helium (He) gas for 180 s to remove other gases in the vials. After releasing the He overpressure, 5% O2 and about 400 ppm N2O were injected into the headspace. The N2O production/reduction capacity of the nodules was monitored using a robotic incubation system (Molstad et al., 2007).
Results
Host Range of Bradyrhizobium Strains Capable of N2O Reduction
Among the 14 tested strains, all formed pink, N2 fixing nodules on cowpea, while 11 strains nodulated groundnut and 4 strains nodulated mungbean. We identified three strains (AC70c, AC101b, and AC101c) that were able to nodulate all these three crops. None of the strains nodulated soybean, haricot bean or alfalfa. Uninoculated controls did not form any nodule in any of the treatments. A complete list of the strains, their host of isolation and the cross-inoculation results is given in Table 1.
Symbiotic Effectiveness
Twelve of the fourteen strains that nodulated cowpea “Hawassa” and all eleven that nodulated groundnut were further tested for their N2-fixation effectiveness. The treatments resulted in significant differences in SDW and SN (%) for both plants (p < 0.001). Results of multiple pairwise comparisons using Tukey’s HD test at α = 0.05 showed that the SDW of cowpea plants nodulated by five of the twelve N2O reducing Bradyrhizobium strains was significantly higher than the SDW of the uninoculated –N controls (three strains at p < 0.001 and two strains at p < 0.05) (Table 2). There were large variations in SDW between replicates within the treatments with a coefficient of variation (CV) ranging between 4.0 and 56.6%. The SDW ranged from 280 ± 30 (standard deviation, n = 3) mg plant–1 to 570 ± 30 mg plant–1 with the corresponding SE of 45 and 91%, respectively. Similarly, groundnut plants nodulated by three out of the eleven N2O reducing Bradyrhizobium strains had significantly higher SDW (one strain at p < 0.001 and two strains at p < 0.05) than uninoculated –N controls (Table 4). The SDW of groundnut plants ranged from 328 ± 90 to 919 ± 110 mg plant–1 with SE of 34 and 95%, respectively (Table 4). There were also large variations in SDW between replicates of groundnut plants, with CV ranging between 5.4 and 47.9%. The highest SDW scores, and thus SE% values, were achieved for both cowpea and groundnut by inoculation with strain AC70c. For these combinations the SE values were 91 and 95%, respectively. This exceeded the symbiotic effectiveness significantly (p < 0.05) of three cowpea-nodulating strains and two groundnut-nodulating strains. For cowpea, strains AC101b and AC92d were also highly effective with SE ≥ 80% of the +N control. In total, 9 strains out of 12 that nodulated cowpea and 9 strains out of 11 that nodulated groundnut were rated from moderately to highly effective, with SDW exceeding 50% of the +N controls.
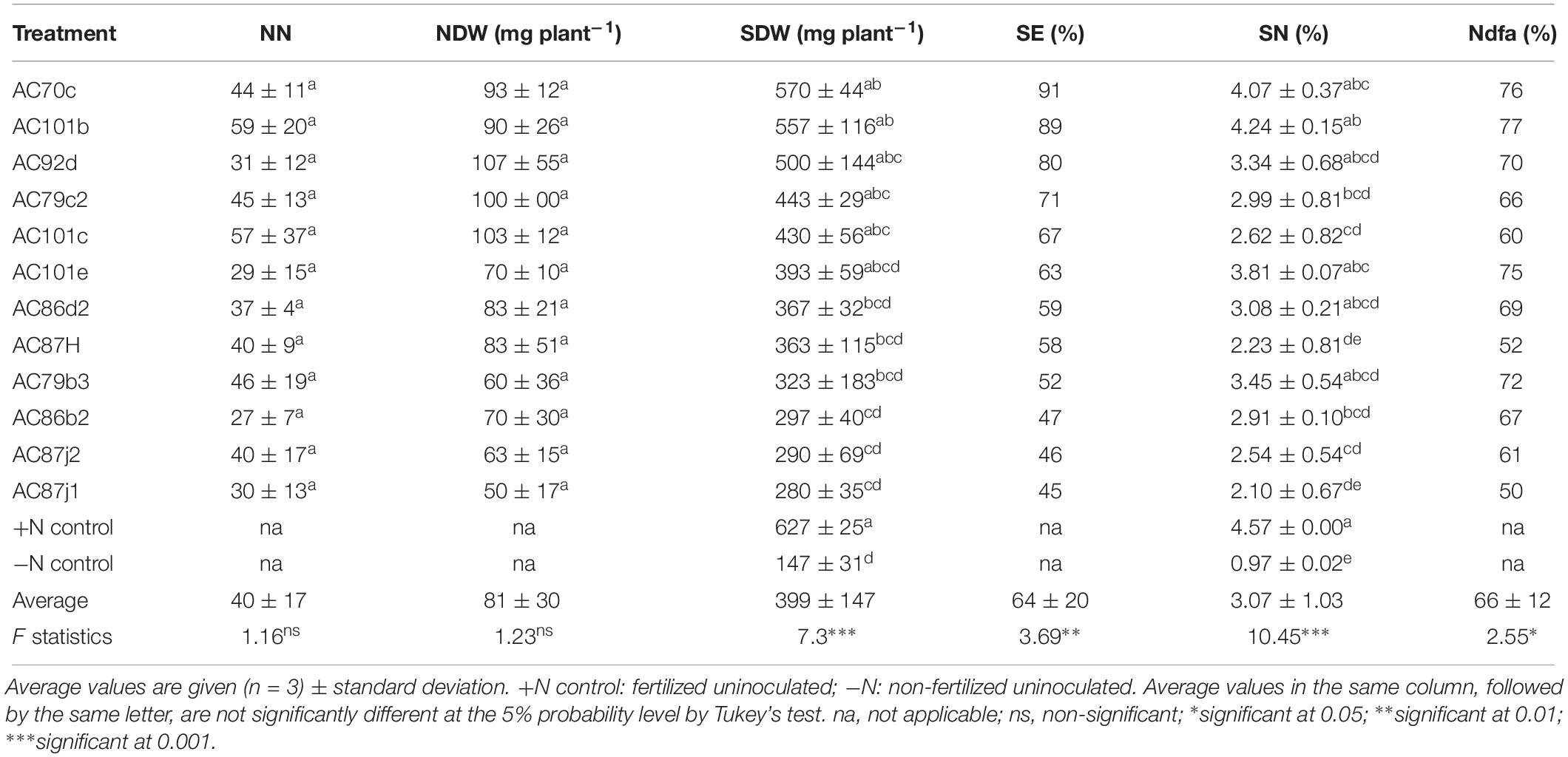
Table 2. Symbiotic performance of 12 N2O reducing Bradyrhizobium strains inoculated with cowpea was determined based on nodule number (NN), nodule dry weight (NDW), shoot dry weight (SDW), symbiotic effectiveness (SE, calculated as % relative to SDW of +N controls), shoot nitrogen content (SN, given as % of SDW), and shoot nitrogen derived from the atmosphere (Ndfa, calculated as % SN relative to −N controls).
The cowpea plants inoculated with our N2O reducing Bradyrhizobium strains (11 out of 12) accumulated significantly higher amounts of N in their shoots (p < 0.001) than the uninoculated control (Table 2). All cowpea plants inoculated with these strains were able to derive most of their shoot nitrogen from the atmosphere (Ndfa ≥ 50%) (Table 2). Similarly, groundnut plants inoculated with 9 out of 11 strains accumulated significantly higher levels of N in their shoots (p < 0.001) than the uninoculated control plants (Table 4). Unlike cowpea plants, most of the nodulated groundnut plants derived <50% of their shoot N from the atmosphere through symbiotic nitrogen fixation (Table 4).
The number of nodules (NN) produced by cowpea was 40 ± 17 plant–1, while the average NN for groundnut was 20 ± 13 (Tables 2, 4). There was no significant difference in NN between treatments of the same plant species (p > 0.05). However, NDW for groundnut differed significantly (p < 0.05) between treatments with different bacterial strains, ranging between 21 ± 18 and 111 ± 44 mg plant–1. For cowpea, the NDW ranged between 50 ± 17 to 107 ± 55 mg plant–1, but the difference between the treatments was not statistically significant.
The Pearson correlation coefficients of the different parameters of symbiotic effectiveness in cowpea and groundnut are presented in Tables 3, 5, respectively. Nodule number for cowpea was significantly correlated with NDW (r = 0.38, p < 0.05) and SDW (r = 0.51, p < 0.01). In groundnut, NN showed a significant positive correlation only with NDW (r = 0.66, p < 0.001). NDW showed a significant positive correlation with SDW both in cowpea (r = 0.65, p < 0.001) and groundnut (r = 0.48, p < 0.01). Neither NN nor NDW showed any significant correlation with SN (%) in both legumes (p > 0.05). However, SDW showed a significant positive correlation with SN (%) both in cowpea (r = 0.74, p < 0.001) and in groundnut (0.53, p < 0.01).
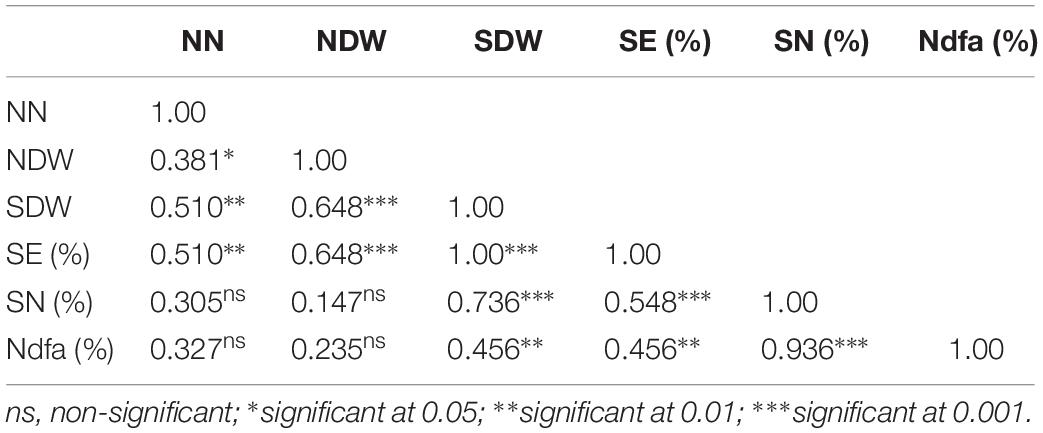
Table 3. Pearson correlation coefficient among parameters of symbiotic performance of cowpea inoculated with 12 N2O reducing Bradyrhizobium strains (Table 2).
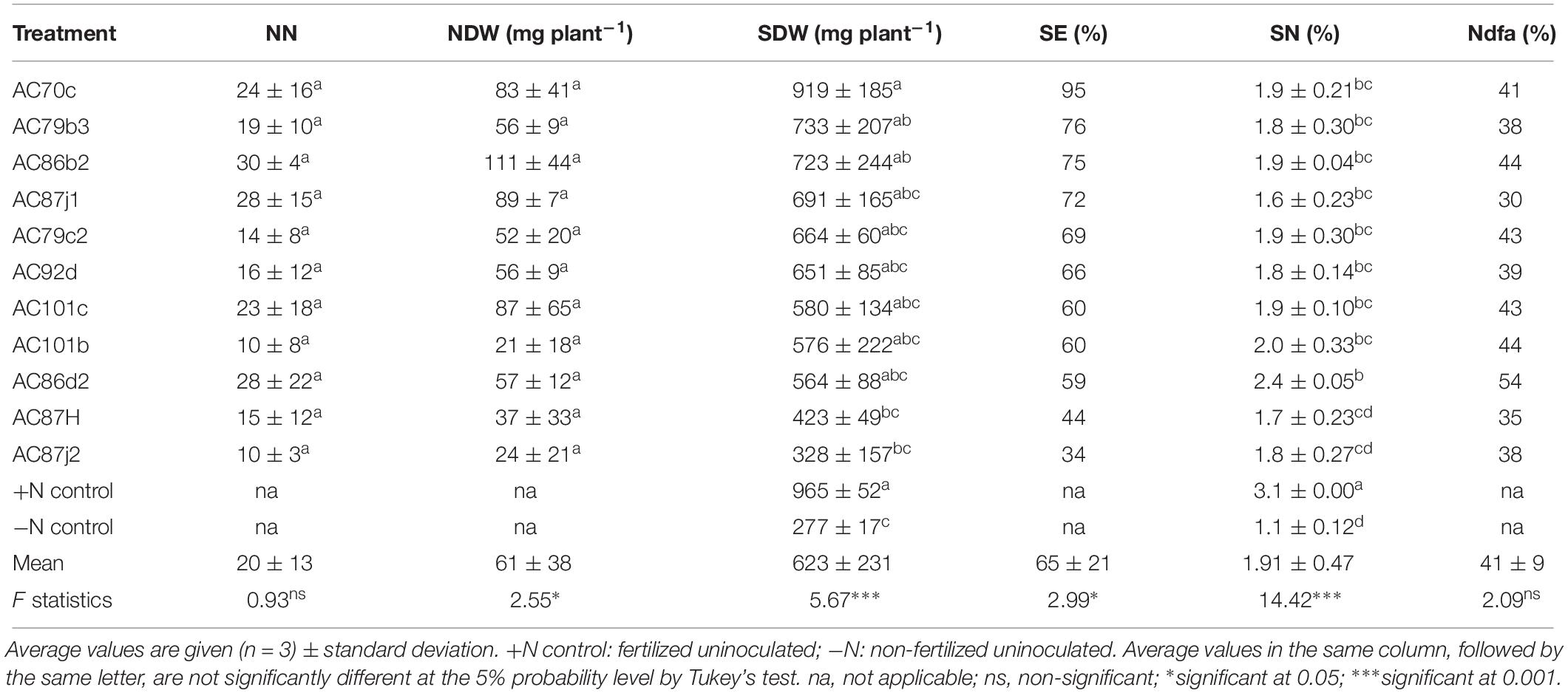
Table 4. Symbiotic performance of 11 N2O reducing Bradyrhizobium strains inoculated with groundnut was determined based on nodule number (NN), nodule dry weight (NDW), shoot dry weight (SDW), symbiotic effectiveness (SE, calculated as% relative to SDW of +N controls), shoot nitrogen content (SN, given as% of SDW), and shoot nitrogen derived from the atmosphere (Ndfa, calculated as% SN relative to −N controls).
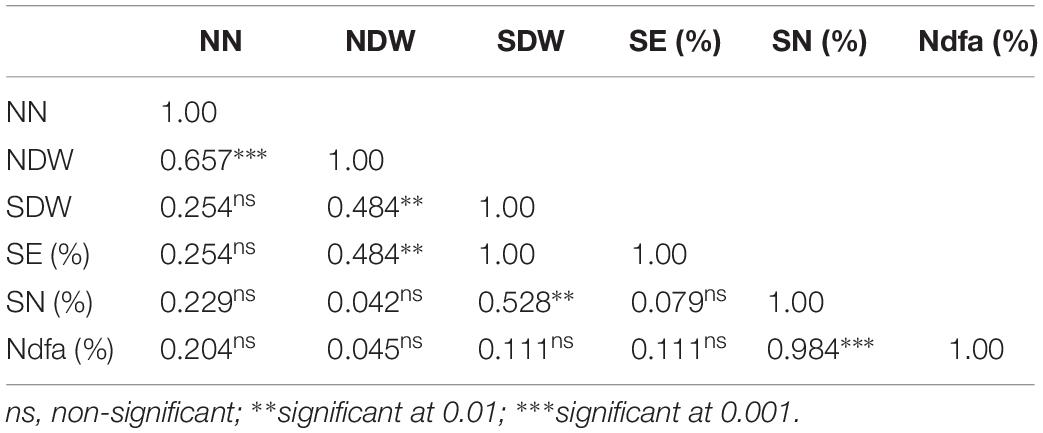
Table 5. Pearson correlation coefficient among parameters of symbiotic performance of groundnut inoculated with 11 N2O reducing Bradyrhizobium strains (Table 4).
Relative Effectiveness of the Best Performing Strains in Three Cowpea Landraces
The six Bradyrhizobium strains that showed the best symbiotic performance with the cowpea landrace CPH (AC70c, AC101b, AC79c2, AC101c, AC101e, and AC92d) were also tested with landraces Cowpea Bale (CPB) and Cowpea Ziway (CPZ) (Table 6). The SDW and SN (%) values were higher to much higher than the −N control, but due to the variation between replicates, this was not statistically significant in most cases. Thus, unlike for CPH, no significant increase in SDW was found for CPZ and CPB after inoculation with the different strains (p > 0.05), except for strain AC79c2 with CPB (p < 0.05). Significant increases in SN (%) were, however, seen for CPZ inoculated with five of the strains (p < 0.001), and for CPB inoculated with two of the strains (p < 0.05). No significant differences were found when we compare the different strains with respect to their symbiotic performance within the same cowpea landrace. Comparison between individual strains, on the other hand, revealed differences in symbiotic effectiveness with the three landraces. Strain AC70c showed the best performance with CPH, resulting in the highest SDW recorded among the inoculated plants (570 ± 44 mg plant–1), while strain 101b performed best with CPZ, a combination that resulted in the highest SE (95% of the SDW of the +N control). Strain AC79c2 performed best with CPB. The shoot N content (given as % N of the SDW) also varied between different bacterial-plant combinations. The highest SN (%) recorded was in CPH inoculated with AC101b and AC70c, with values of 4.24 ± 0.15 and 4.07 ± 0.37%, respectively. All the CPH and CPZ plants derived most of their SN (%) from the atmosphere through symbiotic nitrogen fixation (Ndfa > 50%). Contrary to this, three strain-CPB combinations derived less than 50% of their shoot nitrogen from the atmosphere (Ndfa < 50%).
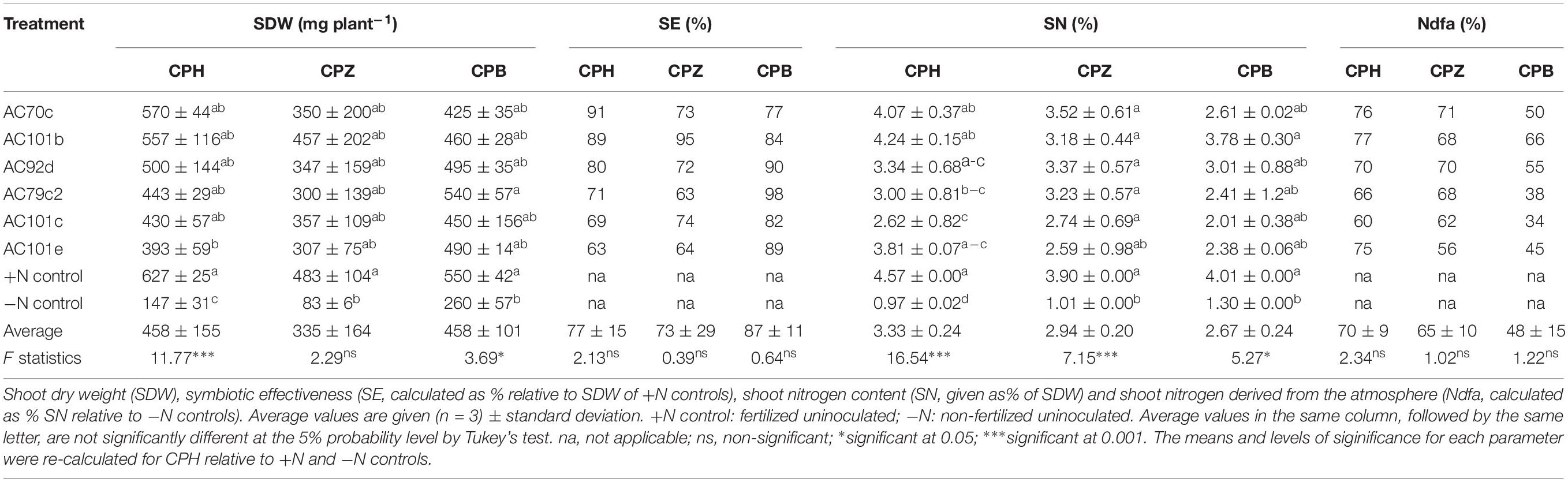
Table 6. Relative effectiveness of the six best performing Bradyrhizobium strains inoculated with two additional cowpea landraces Cowpea Ziway (CPZ) and Cowpea Bale (CPB), compared with Cowpea Hawassa (CPH) from first round of experiments.
N2O Reduction by Legume Nodules
To evaluate whether bradyrhizobia inside nodules may contribute to the reduction of N2O, intact nodules collected from cowpea plants were placed in airtight vials containing He mixed with 5% O2 and 4–5 μmol N2O–N, and gas concentrations (O2, N2O, and N2) were monitored during 100 h (Figure 1). Nodules from plants inoculated with Bradyrhizobium strain AC70c, previously shown to reduce N2O when grown in batch culture (Mania et al., 2019), reduced exogenously supplied N2O to N2 at a rate of 6 μmol N g–1 nodules simultaneously with O2 consumption until all N2O was reduced to N2 (Figure 1). In contrast, nodules of cowpea plants inoculated with strain AC29c, classified as a phenotype lacking N2O reduction capacity (Mania et al., 2019) failed to reduce exogenously supplied N2O to N2. Cowpea nodules containing strain AC70c reduced exogenously supplied N2O to N2 within 40 h of incubation, unlike those containing strain AC29c which did not produce N2. The slight decrease in N2O in flasks containing nodules with strain AC29c is due to sampling loss.
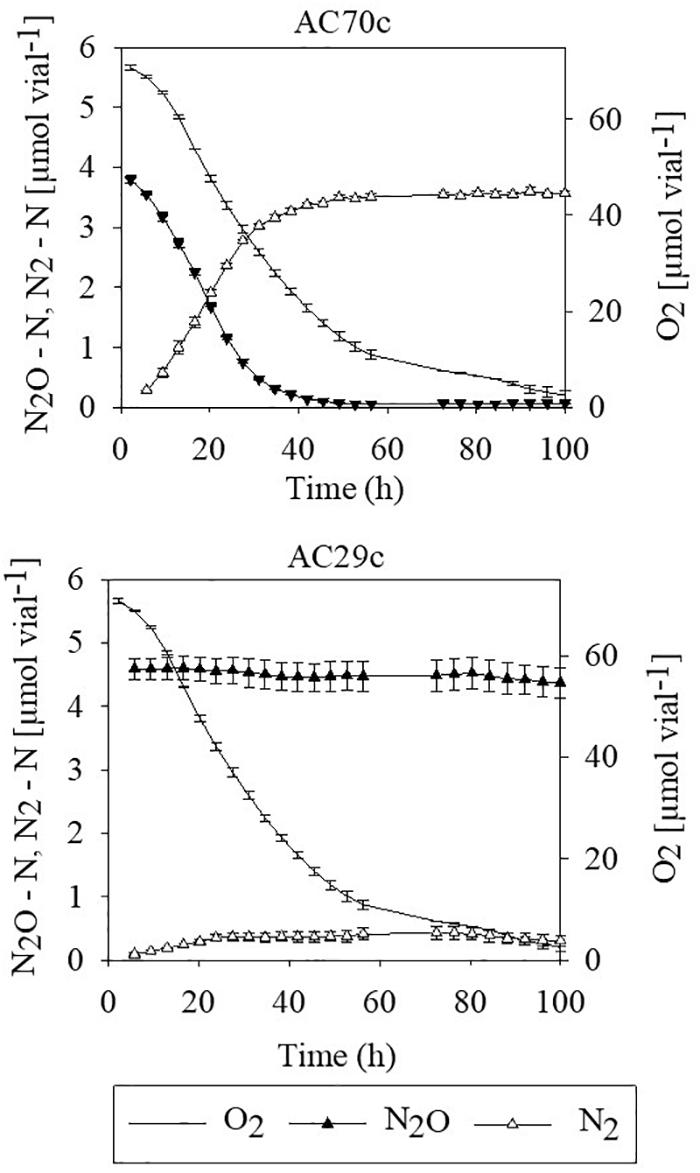
Figure 1. N2O reduction by detached nodules obtained from cowpea plants that had been inoculated with either the N2O reducing Bradyrhizobium strain AC70c, or with the non-N2O reducing Bradyrhizobium strain AC29c. The nodules (approximately 18.9 mg dry weight of nodules per vial) were incubated in vials containing He mixed with 5% O2 and 4–5 μmol N2O–N (corresponding to about 400–500 ppm N2O). n = 3; bars indicate standard deviation.
Discussion
Emissions of N2O are steadily increasing, and the International Panel of Climate Change foresees continued increases during the rest of the present century unless effective measures are undertaken (Rogelj et al., 2018). In accordance, Davidson and Kanter (2014) pointed out the need for novel mitigation options beynd “good agronomic practice” such as avoiding excessive use of fertilizers (Thomson et al., 2012). One such option could be to increase the production of nutrient-rich legumes, since this would reduce the needs for fertilizers and at the same time provide nutritious food and feed for people and livestock. Inoculation of legume crops with N2O reducing rhizobia, as suggested by, e.g., Hénault and Revellin (2011) and Itakura et al. (2013), would further enforce the N2O mitigation. The potential of this is emphasized by the recent findings that a range of taxonomically diverse bradyrhizobia exhibit very efficient N2O reduction, showing a strong preference for N2O over NO3– as electron acceptor caused by the N2O reduction pathway being a powerful competitor for electrons compared to that of the periplasmic nitrate reductase Nap (Mania et al., 2019). This is likely to be a general trait common to all organisms, including rhizobia, that carry N2O reductase as well as Nap but lack the membrane-bound nitrate reductase Nar. Such organisms have the potential to act as sinks for N2O, produced in soil both by themselves and by other microorganisms.
Rhizobia vary in their compatibility as well as in their N2 fixation effectiveness (Musiyiwa et al., 2005). Consequently, an increased, yet sustainable, production of legume crops will require a wider variety of rhizobial inoculants, selected for providing the highest possible N2-fixation efficacy in combination with different types of legume crops, and at the same time carrying the capacity to reduce N2O. In a recent investigation of a wide diversity of Bradyrhizobium strains, we found that approximately 50% were able of N2O reduction (Mania et al., 2019), and in another collection of strains isolated mostly from nodules of peanut, only about one-third reduced N2O (Å. Frostegård, pers comm). The search for promising strains should focus on performing the time-consuming and laborious cross-inoculation tests on strains that are selected based on their ability to reduce N2O.
The 14 Bradyrhizobium strains studied here, all known to have a strong capacity for N2O reduction, nodulated at least one of the legume species, and some showed efficacious N2-fixation capacity (Tables 1, 2, 4). The results showed, however, clear compatibility differences between different combinations of test strain and the legume host. Both legume hosts and microsymbionts varied regarding their levels of promiscuity. Cowpea was the most promiscuous host and was nodulated by all the test strains, followed by peanut (11 strains) and mung bean (4 strains), while none of the strains nodulated soybean, haricot bean and alfalfa. This is in agreement with earlier reports showing that cowpea, groundnut and mungbean belong to the same cross-inoculation group (Somasegaran and Hoben, 1994) which can be nodulated by several Bradyrhizobium strains and are reported as promiscuous hosts (Peoples et al., 1989; Steenkamp et al., 2008; Pule-Meulenberg et al., 2010; Jaiswal et al., 2017; Ndungu et al., 2018). On the microsymbiont side, the three strains AC70c, AC101b, and AC101c that nodulated cowpea, groundnut, and mung bean may, therefore, be regarded as promiscuous.
Rhizobial strains with a wide host range and proven N2 fixation effectiveness are often preferred for the development of inoculants suitable for different legume crops (Drew et al., 2012). Except for strain AC70c, which was isolated from trap host species P. vulgaris, other test strains included in this study were isolated from tree legumes growing in Ethiopia (Wolde-Meskel et al., 2004b). This indicates that rhizobia either isolated from wild legumes or trapped from soil can be domesticated and used as inoculants for legume crops as reported earlier (Wange, 1989; Zhang et al., 1991). This potential can be further exploited as Ethiopia hosts a wide diversity of Bradyrhizobium strains in its soil (Aserse et al., 2012; Degefu et al., 2017, 2018) that can be used as inoculants for grain legumes. Our test strains failed to nodulate soybean, haricot bean, and alfalfa. This could be due to the specificity of these hosts to a certain group of rhizobia (Peoples et al., 1989). Reports show that alfalfa and haricot bean form a symbiotic association with fast-growing rhizobial strains belonging to genus Ensifer and Rhizobium, respectively, but not with bradyrhizobia (Somasegaran and Hoben, 1994). The inability of strain AC70c to nodulate haricot bean, its trap host (Wolde-Meskel et al., 2004a), could also be due to the specificity of the host genotype to a particular strain (Abi-Ghanem et al., 2011). We speculate that failure of our Bradyrhizobium test strains to nodulate soybean, a common host for B. japonicum strains, might be due to incompatibility as soybean is an exotic crop introduced to Ethiopia in the 1950s (Aserse et al., 2012). Classification of soybean under the cross-inoculation group different from cowpea and groundnut (Somasegaran and Hoben, 1994) further supports this.
Nodulation alone might not always result in an effective symbiosis that can fulfill the host’s N requirement (Terpolilli et al., 2008), necessitating careful screening and selection for elite rhizobial symbionts that can form effective N2 fixing nodules with the host legumes. The symbiotic effectiveness (SE) in our study ranged between 45 and 98% in the different cowpea landraces and between 34 and 95% in groundnut. Most of the test strains that nodulated both legume crops, however, were rated as moderately to highly effective with SDW exceeding 50% of the N fertilized controls, indicating their potential suitability as inoculants for cowpea and groundnut provided that they are competitive with other rhizobia in different soil environments. Inspection of the phylogenetic tree constructed based on the symbiosis-related gene nodA shows that most of our test strains are closely related to Bradyrhizobium strain CB756, a widely used inoculant of cowpea and peanut (Degefu et al., 2017), supporting their suitability as inoculants for the tested legumes. This should, however, be followed by field evaluation of the strain’s ability to compete with indigenous rhizobia for nodulation and adaptation to the conditions in the soil (moisture content, temperature, acidity, and others) that might limit the efficiency and survival of the inoculant (O’Hara et al., 2002). In cases where the soil harbors high numbers of less effective indigenous rhizobia, the selected strain should be both competitive for nodule occupancy and have a high N fixation effectiveness with the selected legume crop, to ensure the success of inoculation (Toro, 1996).
Among the most effective strains in the present study was strain AC70c that occupied its own distinct branch designated as C on the nodA phylogenetic tree (Degefu et al., 2017). It was the best performing strain both with cowpea and groundnut, with SE values of 98 and 95%, respectively. Differences in nodulation and symbiotic effectiveness of rhizobial strains can also be extended to genotypes of the same host legume species (Pazdernik et al., 1997; Abi-Ghanem et al., 2011; Yates et al., 2016). Our analysis of the relative effectiveness of the six best-performing strains on the three cowpea landraces (Table 6) also demonstrated such variations, confirming the need to evaluate the host range and symbiotic effectiveness of rhizobial strains with different cultivars of the same legume species.
Our results also showed that all the cowpea and groundnut plants inoculated by the test strains accumulated more N from the atmosphere through symbiotic N2 fixation than uninoculated non-fertilized controls. Cowpea plants derived most of their shoot N from the atmosphere through symbiotic N2 fixation. The low Ndfa in groundnut (<50% in most strain-plant combinations) might be due to the somewhat short duration of the experiment (35 days). Growth up to 7 weeks was recommended for large-seeded legumes such as groundnut before assessing nodulation and effectiveness of the inoculants (Yates et al., 2016).
Soybean nodules containing Bradyrhizobium strains have been reported to reduce N2O (Hirayama et al., 2011; Hénault and Revellin, 2011; Inaba et al., 2012). In accordance, our results showed that detached nodules from cowpea plants, inoculated with the N2O reducing strain AC70c (Mania et al., 2019), efficiently reduced exogenously provided N2O. This strain expressed N2O reductase in vivo, indicating the presence of bacterial cells with active anaerobic respiration in the root nodules. Yet, it is likely that the most important N2O reduction activity will be performed by N2O reductase expressing bradyrhizobia when they are released from senescent nodules into the soil where they can act as sinks for N2O produced by denitrifying organisms in the microbial community (Akiyama et al., 2016).
Taken together, through screening of several rhizobial strains for their N2O reduction capacity and N2 fixation effectiveness with different legume hosts, we obtained promising strains such as AC70c that can be used for the production of legume inoculants with both capacities. Such inoculants have the potential to increase the productivity of different legume crops and simultaneously mitigate N2O emission from agricultural fields.
Data Availability Statement
The datasets generated for this study are available on request to the corresponding authors.
Author Contributions
KW, TD, and ÅF planned the experiments, analyzed the results, and wrote the manuscript. KW and TD performed the experiments.
Funding
KW was supported by a grant from the Norwegian State Educational Loan Fund.
Conflict of Interest
The authors declare that the research was conducted in the absence of any commercial or financial relationships that could be construed as a potential conflict of interest.
The handling editor declared a past co-authorship with one of the authors, ÅF.
References
Abi-Ghanem, R., Carpenter-Boggs, L., and Smith, J. L. (2011). Cultivar effects on nitrogen fixation in peas and lentils. Biol. Fertil. Soils 47, 115–120. doi: 10.1007/s00374-010-0492-6
Akiyama, H., Hoshino, Y. T., Itakura, M., and Shimomura, Y. (2016). Mitigation of soil N2O emission by inoculation with a mixed culture of indigenous Bradyrhizobium diazoefficiens. Nat. Publ. Gr. 6:32869. doi: 10.1038/srep32869
Aserse, A. A., Räsänen, L. A., Aseffa, F., Hailemariam, A., and Lindström, K. (2012). Phylogenetically diverse groups of Bradyrhizobium isolated from nodules of Crotalaria spp., Indigofera spp., Erythrina brucei and Glycine max growing in Ethiopia. Mol. Phylogenet. Evol. 65, 595–609. doi: 10.1016/j.ympev.2012.07.008
Bedmar, E. J., Robles, E. F., and Delgado, M. J. (2005). The complete denitrification pathway of the symbiotic, nitrogen-fixing bacterium Bradyrhizobium japonicum. Biochem. Soc. Trans. 33, 141–144. doi: 10.1042/bst0330141
Bueno, E., Mania, D., Frostegard, Å, Bedmar, E. J., Bakken, L. R., and Delgado, M. J. (2015). Anoxic growth of ensifer meliloti 1021 by N2O-reduction, a potential mitigation strategy. Front. Microbiol. 6:537. doi: 10.3389/fmicb.2015.00537
Butterbach-Bahl, K., Baggs, E. M., Dannenmann, M., Kiese, R., and Zechmeister-Boltenstern, S. (2013). Nitrous oxide emissions from soils: how well do we understand the processes and their controls? Philos. Trans. R. Soc. B Biol. Sci. 368:20130122. doi: 10.1098/rstb.2013.0122
Dakora, F. D., Aboyinga, R. A., Mahama, Y., and Apaseku, J. (1987). Assessment of N2 fixation in groundnut (Arachis hypogaea L.) and cowpea (Vigna unguiculata L). Mircen J. Appl. Microbiol. Biotechnol. 3, 389–399. doi: 10.1007/BF00935697
Davidson, E. A., and Kanter, D. (2014). Inventories and scenarios of nitrous oxide emissions. Environ. Res. Lett. 9:105012. doi: 10.1088/1748-9326/9/10/105012
Degefu, T., Wolde-Meskel, E., and Rasche, F. (2018). Genetic diversity and symbiotic effectiveness of Bradyrhizobium strains nodulating selected annual grain legumes growing in Ethiopia. Int. J. Syst. Evol. Microbiol. 68, 449–460. doi: 10.1099/ijsem.0.002486
Degefu, T., Wolde-meskel, E., Woliy, K., and Frostegård, Å. (2017). Phylogenetically diverse groups of Bradyrhizobium isolated from nodules of tree and annual legume species growing in Ethiopia. Syst. Appl. Microbiol. 40, 205–214. doi: 10.1016/j.syapm.2017.04.001
Drew, E., Herridge, D., Ballard, R., Hara, G. O., Deaker, R., Denton, M., et al. (2012). Inoculating Legumes: A practical guide. Canberra: Grains Research and Development Corporation.
Falk, S., Liu, B., and Braker, G. (2010). Isolation, genetic and functional characterization of novel soil nirK-type denitrifiers. Syst. Appl. Microbiol. 33, 337–347. doi: 10.1016/j.syapm.2010.06.004
Hénault, C., and Revellin, C. (2011). Inoculants of leguminous crops for mitigating soil emissions of the greenhouse gas nitrous oxide. Plant Soil 346, 289–296. doi: 10.1007/s11104-011-0820-0
Herridge, D. F., Peoples, M. B., and Boddey, R. M. (2008). Global inputs of biological nitrogen fixation in agricultural systems. Plant Soil 311, 1–18. doi: 10.1007/s11104-008-9668-3
Hirayama, J., Eda, S., Mitsui, H., and Minamisawa, K. (2011). Nitrate-dependent N2O emission from intact soybean nodules via denitrification by Bradyrhizobium japonicum bacteroids. Appl. Environ. Microbiol. 77, 8787–8790. doi: 10.1128/AEM.06262-11
Inaba, S., Ikenishi, F., Itakura, M., Kikuchi, M., Eda, S., Chiba, N., et al. (2012). N2O emission from degraded soybean nodules depends on denitrification by Bradyrhizobium japonicum and other microbes in the rhizosphere. Microbes Environ. 27, 470–476. doi: 10.1264/jsme2.me12100
Itakura, M., Uchida, Y., Akiyama, H., Hoshino, Y. T., Shimomura, Y., Morimoto, S., et al. (2013). Mitigation of nitrous oxide emissions from soils by Bradyrhizobium japonicum inoculation. Nat. Clim. Chang. 3, 208–212. doi: 10.1038/nclimate1734
Jaiswal, S. K., Msimbira, L. A., and Dakora, F. D. (2017). Phylogenetically diverse group of native bacterial symbionts isolated from root nodules of groundnut (Arachis hypogaea L.) in South Africa. Syst. Appl. Microbiol. 40, 215–226. doi: 10.1016/j.syapm.2017.02.002
Jensen, E. S., and Hauggaard-Nielsen, H. (2003). How can increased use of biological N2 fixation in agriculture benefit the environment? Plant Soil 252, 177–186. doi: 10.1023/a:1024189029226
Jensen, E. S., Peoples, M. B., Boddey, R. M., Gresshoff, P. M., Henrik, H. N., Alves, B. J. R., et al. (2012). Legumes for mitigation of climate change and the provision of feedstock for biofuels and biorefineries. Rev. Agron. Sustain. Dev. 32, 329–364. doi: 10.1007/s13593-011-0056-7
Kox, M. A. R., and Jetten, M. S. M. (2015). “The nitrogen cycle,” in Principles of Plant-Microbe Interactions: Microbes for Sustainable Agriculture, ed B. Lugtenberg, (Berlin: Springer), 205–214.
Mania, D., Woliy, K., Degefu, T., and Frostegård, Å. (2019). A common mechanism for efficient N2O reduction in diverse isolates of nodule−forming bradyrhizobia. Environ. Microbiol. (in press). doi: 10.1111/1462-2920.14731
Molstad, L., Dörsch, P., and Bakken, L. R. (2007). Robotized incubation system for monitoring gases (O2, NO, N2O N2) in denitrifying cultures. J. Microbiol. Methods 71, 202–211. doi: 10.1016/j.mimet.2007.08.011
Montzka, S. A., Dlugokencky, E. J., and Butler, J. H. (2011). Non-CO2 greenhouse gases and climate change. Nature 476, 43–50. doi: 10.1038/nature10322
Musiyiwa, K., Mpepereki, S., and Giller, K. E. (2005). Symbiotic effectiveness and host ranges of indigenous rhizobia nodulating promiscuous soyabean varieties in Zimbabwean soils. Soil Biol. Biochem. 37, 1169–1176. doi: 10.1016/j.soilbio.2004.12.004
Ndungu, S. M., Messmer, M. M., Ziegler, D., Gamper, H. A., Mészáros, É, Thuita, M., et al. (2018). Cowpea (Vigna unguiculata L. Walp) hosts several widespread bradyrhizobial root nodule symbionts across contrasting agro-ecological production areas in Kenya. Agric. Ecosyst. Environ. 261, 161–171. doi: 10.1016/j.agee.2017.12.014
Nelson, D. W., and Sommers, L. E. (1996). “Total carbon, organic carbon and organic matter,” in Methods of Soil Analysis. Part 3: Chemical Methods, ed. D. L. Sparks (Madison, WI: Soil Science Society of America), 961–1010.
O’Hara, G., Yates, R., and Howieson, J. (2002). “Selection strains of root nodule bacteria to improve inoculant performance and increase legume productivity in stressful environments,” in Inoculants and Nitrogen Fixation of Legumes in Viet, ed. D. Herridge (Canberra: ACIAR Proc), 75–80.
Pazdernik, D. L., Vance, C. P., Sadowsky, M. J., Graham, P. H., and Orf, J. H. (1997). A host-controlled, serogroup-specific, ineffective- iodulation system in the Bradyrhizobium-Soybean (Glycine max) symbiosis. Mol. Plant Microbe Interact 10, 994–1001. doi: 10.1094/mpmi.1997.10.8.994
Peoples, M. B., Brockwell, J., Herridge, D. F., Rochester, I. J., Alves, B. J. R., Urquiaga, S., et al. (2009). The contributions of nitrogen-fixing crop legumes to the productivity of agricultural systems. Symbiosis 48, 1–17. doi: 10.1007/bf03179980
Peoples, M. B., Faizah, A. W., Rerkasem, B., and Herridge, D. F. (1989). Methods for evaluating nitrogen fixation by nodulated legumes in the field. Aust. Cent. Int. Agric. Res. 11:76.
Poole, P., Ramachandran, V., and Terpolilli, J. (2018). Rhizobia: from saprophytes to endosymbionts. Nat. Rev. Microbiol. 16, 291–303. doi: 10.1038/nrmicro.2017.171
Pueppke, S. G., and Broughton, W. J. (1999). Rhizobium sp. Strain NGR234 and R. fredii USDA257 share exceptionally broad, nested host ranges. Mol. Plant-Microbe Interact. 12, 293–318. doi: 10.1094/mpmi.1999.12.4.293
Pule-Meulenberg, F., Belane, A. K., Krasova-wade, T., and Dakora, F. D. (2010). Symbiotic functioning and bradyrhizobial biodiversity of cowpea (Vigna unguiculata L. Walp.) in Africa. BMC Microbiol. 10:89. doi: 10.1186/1471-2180-10-89
Ravishankara, A. R., Daniel, J. S., and Portmann, R. W. (2009). Nitrous oxide (N2O): The dominant ozone-depleting substance emitted in the 21st century. Science 326, 123–125. doi: 10.1126/science.1176985
Reay, D. S., Davidson, E. A., Smith, K. A., Smith, P., Melillo, J. M., Dentener, F., et al. (2012). Global agriculture and nitrous oxide emissions. Nat. Clim. Chang. 2, 410–416.
Rennie, R. J. (1984). Comparison of N balance and 15N Isotope dilution to quantify N2 fixation in field-grown Legumes 1. Agron. J. 76, 785–790.
Rodríguez-Echeverría, S., Fajardo, S., Ruiz-Díez, B., and Fernández-Pascual, M. (2012). Differential effectiveness of novel and old legume-rhizobia mutualisms: implications for invasion by exotic legumes. Oecologia 170, 253–261. doi: 10.1007/s00442-012-2299-7
Rogelj, J., Shindell, D., Jiang, K., Fifita, S., Forste, P., Ginzburg, V., et al. (2018). “Mitigation pathways compatible with 1.5°C in the context of sustainable development,” in Global Warming of 1.5°C. An IPCC Special Report on the Impacts of 537 Global warming of 1.5°C above Pre-Industrial Levels and Related Global Greenhouse Gas 538 Emission Pathways, in the Context of Strengthening the Global Response to the Threat of 539 Climate Change, Sustainable Development, and Efforts to Eradicate Poverty, eds V. P. Masson-Delmotte, H.-O. Zhai, D. Pörtner, J. Roberts, P. Skea, R. Shukla, et al. (Genève: Intergovernmental Panel on Climate Change).
Sameshima-Saito, R., Chiba, K., Hirayama, J., Itakura, M., Mitsui, H., Eda, S., et al. (2006). Symbiotic Bradyrhizobium japonicum reduces N2O surrounding the soybean root system via nitrous oxide reductase. Appl. Environ. Microbiol. 72, 2526–2532. doi: 10.1128/aem.72.4.2526-2532.2006
Sánchez, C., and Minamisawa, K. (2018). Redundant roles of Bradyrhizobium oligotrophicum Cu-type (NirK) and cd1-type (NirS) nitrite reductase genes under denitrifying conditions. FEMS Microbiol. Lett. 365, 1–7. doi: 10.1093/femsle/fny015
Somasegaran, P., and Hoben, H. J. (1994). Handbook for Rhizobia Methods in Legume-Rhizobium Technology. Berlin: Springer Science & Business Media.
Steenkamp, E. T., Stepkowski, T., Przymusiak, A., Botha, W. J., and Law, I. J. (2008). Cowpea and peanut in southern Africa are nodulated by diverse Bradyrhizobium strains harboring nodulation genes that belong to the large pantropical clade common in Africa. Mol. Phylogenet. Evol. 48, 1131–1144. doi: 10.1016/j.ympev.2008.04.032
Terpolilli, J. J., O’Hara, G. W., Tiwari, R. P., Dilworth, M. J., and Howieson, J. G. (2008). The model legume Medicago truncatula A17 is poorly matched for N2 fixation with the sequenced microsymbiont Sinorhizobium meliloti 1021. New Phytol. 179, 62–66. doi: 10.1111/j.1469-8137.2008.02464.x
Thomson, A. J., Giannopoulos, G., Pretty, J., Baggs, E. M., and Richardson, D. J. (2012). Biological sources and sinks of nitrous oxide and strategies to mitigate emissions. Philos. Trans. R. Soc. B Biol. Sci. 367, 1157–1168. doi: 10.1098/rstb.2011.0415
Toro, N. (1996). Nodulation competitiveness in the Rhizobium-legume symbiosis. World J. Microbiol. Biotechnol. 12, 157–162. doi: 10.1007/BF00364680
Torres, M. J., Rubia, M. I., De La Peña, T. C., Pueyo, J. J., Bedmar, E. J., and Delgado, M. J. (2014). Genetic basis for denitrification in Ensifer meliloti. BMC Microbiol. 14:142. doi: 10.1186/1471-2180-14-142
Wange, S. S. (1989). Response of groundnut (Arachis hypogaea L.) to inoculation with Rhizobium strains isolated from wild arboreal legumes. MIRCEN J. Appl. Microbiol. Biotechnol. 5, 135–141. doi: 10.1007/bf01741836
Wolde-Meskel, E., Terefework, Z., Frostegård, Å, and Lindström, K. (2005). Genetic diversity and phylogeny of rhizobia isolated from agroforestry legume species in southern Ethiopia. Int. J. Syst. Evol. Microbiol. 55, 1439–1452. doi: 10.1099/ijs.0.63534-0
Wolde-Meskel, E., Terefework, Z., Lindström, K., and Frostegård, Å (2004a). Metabolic and genomic diversity of rhizobia isolated from field standing native and exotic woody legumes in southern Ethiopia. Syst. Appl. Microbiol. 27, 603–611. doi: 10.1078/0723202041748145
Wolde-Meskel, E., Terefework, Z., Lindström, K., and Frostegård, Å (2004b). Rhizobia nodulating African Acacia spp. and Sesbania sesban trees in southern Ethiopian soils are metabolically and genomically diverse. Soil Biol. Biochem. 36, 2013–2025. doi: 10.1016/j.soilbio.2004.05.020
Yates, R. J., Howieson, J. G., Hungria, M., Bala, A., O’Hara, G. W., and Terpolilli, J. (2016). “Authentication of rhizobia and assessment of the legume symbiosis in controlled plant growth systems,” in Working with rhizobia, eds J. G. Howieson and M. J. Dilworth (Canberra: Australian Centre for International Agricultural Research), 73–108.
Keywords: Bradyrhizobium, denitrification, symbiotic nitrogen fixation, nitrous oxide, nitrous oxide reductase
Citation: Woliy K, Degefu T and Frostegård Å (2019) Host Range and Symbiotic Effectiveness of N2O Reducing Bradyrhizobium Strains. Front. Microbiol. 10:2746. doi: 10.3389/fmicb.2019.02746
Received: 19 August 2019; Accepted: 12 November 2019;
Published: 29 November 2019.
Edited by:
David Richardson, University of East Anglia, United KingdomReviewed by:
Jose María Vinardell, University of Seville, SpainPetri Penttinen, Sichuan Agricultural University, China
Copyright © 2019 Woliy, Degefu and Frostegård. This is an open-access article distributed under the terms of the Creative Commons Attribution License (CC BY). The use, distribution or reproduction in other forums is permitted, provided the original author(s) and the copyright owner(s) are credited and that the original publication in this journal is cited, in accordance with accepted academic practice. No use, distribution or reproduction is permitted which does not comply with these terms.
*Correspondence: Kedir Woliy, kedir.woliy.jillo@nmbu.no; Åsa Frostegård, asa.frostegard@nmbu.no