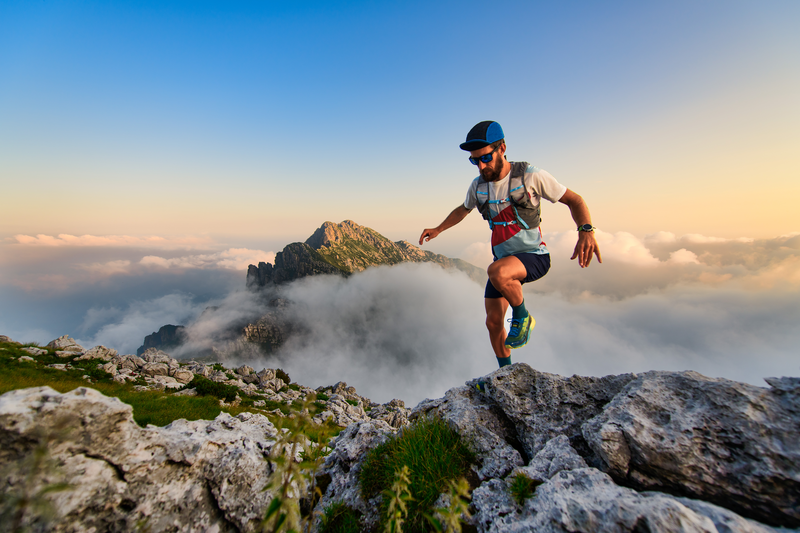
95% of researchers rate our articles as excellent or good
Learn more about the work of our research integrity team to safeguard the quality of each article we publish.
Find out more
ORIGINAL RESEARCH article
Front. Microbiol. , 03 December 2019
Sec. Plant Pathogen Interactions
Volume 10 - 2019 | https://doi.org/10.3389/fmicb.2019.02727
Chemotaxis toward seed exudates is important in the establishment of microbe–plant associations. The objective of this work was to explore whether organic acids from the seed exudates of Sesbania rostrata play a role in recruiting Azorhizobium caulinodans ORS571 in the plant rhizosphere. High-performance liquid chromatography (HPLC) was used to analyze the organic acid content in seed exudates of S. rostrata and to further determine their roles in A. caulinodans growth and chemotactic response. Succinic, acetic, citric, oxalic, and lactic acids were the most abundant, and, except for oxalic acid, they could support A. caulinodans growth as the sole carbon source. TlpA1, a transmembrane chemoreceptor, was found to be involved in the chemotactic response to these organic acids. Oxalic acid played a direct role in the chemotactic response, but it also played an indirect role by promoting or inhibiting the chemotactic response toward other chemoeffectors. Furthermore, the indirect role of oxalic acid on other chemoeffectors was concentration-dependent. The effect of oxalic acid at different concentrations on host root colonization was also determined. By using different strategies, oxalic acid appears to play a major role in the early steps of the association of A. caulinodans and its host plant.
Bacterial chemotaxis towards plants plays a major role in the colonization of the root surface, and chemotaxis signaling appears as an early event in communication between plants and rhizobia (Nelson, 2004). The composition and concentration of seed exudates differ with plant species; they usually contain organic acids, amino acids, sugars, flavonoids, and proteins, which are potential chemoeffectors to bacteria. In canonical chemotaxis systems, binding of ligands/chemoeffectors to chemoreceptors, encoded by mcp genes, affects CheA, a histidine kinase, autophosphorylation (Szurmant and Ordal, 2004). The response regulator CheY, which can be phosphorylated by CheA, diffuses in the cytoplasm and ultimately interacts with flagellar motor proteins, triggering the changes in movement (Eisenbach, 1996; Szurmant and Ordal, 2004).
Production of chemo-attractants to soil bacteria by plant seeds and roots is a common feature that can affect the association between plants and microbes via different strategies. In particular, organic acids promote bacterial root adsorption, swarming motility, and plant colonization (Hida et al., 2015; Li et al., 2017). For example, clover exudates promote plant root colonization of Rhizobium leguminosarum bv. Trifolii (Janczarek and Skorupska, 2011), and exudates from Alfalfa stimulate adsorption of Rhizobium meliloti to the root surface (Wall and Favelukes, 1991). The quantity of exudates increases with the growth of plants, and their composition may differ at different plant ages (Jones et al., 2004).
Organic acids, including succinic, citric, malic, and lactic acids are predominant components in bean, tobacco, maize, tomato, cucumber, and sweet pepper exudates (Berendsen et al., 2012; Neal et al., 2012; Li et al., 2017; Martins et al., 2017) and have multiple effects on bacteria and plants. (1) As a growth substrate. For example, oxalic acid, in Ralstonia eutropha and Methylobacterium extorquens, can serve as the sole carbon source and energy to support growth (Sahin, 2003). Citric acid, succinic acid, and aromatic organic acids produced by plant exudates are preferentially consumed by rhizosphere bacteria (Kuiper et al., 2002; Zhalnina et al., 2018). (2) As an inducer of the chemotactic response. In particular, malic, fumaric, citric, cinnamic, and succinic acids can enhance cognate mcp gene expression levels (Tan et al., 2013; Li et al., 2017). For example, acetate, in Pseudomonas putida, increases the transcript level of its cognate chemoreceptor by up to 22-fold (Lopez-Farfan et al., 2017). (3) By affecting bacterial plant surface colonization and biofilm formation. For example, malic acid in the root exudates of banana and tomato have been shown to favor root colonization by Bacillus amyloliquefaciens (Tan et al., 2013; Yuan et al., 2015). (4) As protection against metal stress (Kamilova et al., 2006) and reactive oxygen species (ROS) (Haichar et al., 2014). For example, citric acid and oxalic acid have been shown to scavenge metals for soil fungi (Van Hees et al., 2006). Pseudomonas fluorescens produces oxalate to prevent aluminum stress (Hamel et al., 1999), and in Caulobacter crescentus, organic acids prevent nickel toxicity (Jain and Chen, 2018). (5) In addition, oxalic acid can function as a signal molecule and affect competition and pathogenicity in the fungi–bacteria interaction (Rudnick et al., 2015; Ul Haq et al., 2018). For example, Collimonas bacteria, although unable to use oxalate for growth, can be attracted by oxalate to sense and attack the fungal source (Rudnick et al., 2015). However, the specific involvement of oxalic acid from seed exudates in the microbe–plant interaction remains to be documented (Palmieri et al., 2019).
Azorhizobium caulinodans ORS571, a symbiont of the host Sesbania rostrata, can fix atmospheric nitrogen in free-living and symbiotic states. As reported for other rhizobia symbiotic associations (Caetano-Anolles et al., 1988; Merritt et al., 2007; Miller et al., 2007; Scharf et al., 2016), the establishment of the A. caulinodans-S. rostrata association involves chemotaxis toward plants roots, colonization of the root surface, infection, and the initiation of nodule organogenesis (Jiang et al., 2016; Liu et al., 2017, 2019a,b; Liu W. et al., 2018; Liu X. et al., 2018). In particular, two chemoreceptors, IcpB and TlpA1, were shown to be involved in the chemotactic response of A. caulinodans to some organic acids, such as succinate, citrate, tartrate, and malate (Jiang et al., 2016; Liu et al., 2017). IcpB, encoded by icpB, is a soluble heme-binding protein that is employed for aerotaxis and chemotaxis through sensing oxygen (Jiang et al., 2016). TlpA1 is a transmembrane chemoreceptor, encoded by tlpA1, that is located upstream of the chemotaxis gene cluster (che cluster containing cheA, cheW, cheY1, cheB, and cheR genes) in the A. caulinodans genome (Liu et al., 2017). Recently, we have identified TlpH, a transmembrane protein containing a dCache domain, as the cognate chemoreceptor of histidine, arginine, and aspartic acid, which are the three most abundant amino acids present in the seed exudates of S. rostrata (Liu et al., 2019a). In addition to their role in the chemotactic response of A. caulinodans, it was also shown that amino acids from seed exudates played an indirect role, being involved in the regulation of che genes and flagella synthesis (Liu et al., 2019a).
The objective of this work was to understand the contribution of organic acids from S. rostrata seed exudates in the process of A. caulinodans chemotaxis and root colonization. Characterization of the chemotactic response induced in A. caulinodans by the five most abundant organic acids present in S. rostrata seed exudates is reported. Among them, we focused on the role of oxalic acid in host colonization, its involvement as a chemoeffector, and its role in controlling chemotactic responses towards other chemoeffectors.
The concentration and composition of organic acids in Sesbania seed exudates were analyzed using high-performance liquid chromatography (HPLC). The organic acids present were identified by comparing their retention times with standard samples, and their concentrations were quantified according to the standard curve of standard substances. The five most abundant organic acids were acetic acid (1.20 × 103 μM), succinic acid (3.62 × 102 μM), lactic acid (2.78 × 102μM), oxalic acid (4.43 × 101 μM), and citric acid (7.97 μM). Maleic acid (1.89 μM) and fumaric acid (3.22 × 10–1 μM) were less abundant (Figure 1). Although malic acid and tartaric acid are reported as common in root or seed exudates (Kamilova et al., 2006; Tan et al., 2013), their content in Sesbania seed exudates was below the detection limit. The five most abundant organic acids in seed exudates were selected for further analysis.
Figure 1. Identification of organic acids in S. rostrata seed exudates detected by HPLC. Elution peaks of organic acids from the exudates and standard samples are shown as solid and dashed lines, respectively. Numbers 1–9: oxalic acid, tartaric acid, malic acid, lactic acid, acetic acid, citric acid, succinic acid, maleic acid, and fumaric acid, respectively.
We next investigated whether these organic acids can be used as carbon and energy sources. Succinate is commonly used as a growth substrate in A. caulinodans growth medium (L3 minimal medium; see section “Materials and Methods”). Acetate, lactate, and citrate (10 mM) could support cell growth up to OD600 values of 0.5, 0.7, and 0.45, respectively. Though oxalate is abundant in seed exudates, it cannot promote A. caulinodans growth in liquid media (Figure 2A). Because the metabolic properties of substances in bacteria might be different between liquid and solid media, such as in the bacteria of the genus Collimonas (Fritsche et al., 2014), the growth curve of A. caulinodans with oxalate on semi-solid plates was also determined. Consistent with the result obtained in liquid cultures, oxalate cannot support growth on semi-solid plates (Figure 2B).
Figure 2. Growth of A. caulinodans ORS571 in liquid (A) and on soft agar plate (B) with each organic acid or plus oxalate as a carbon source. Values are means ± standard deviation from at least three experiments.
Bacterial chemotactic response toward organic acids was assessed using capillary and soft agar plate assays, using the wild type ORS571 and its derivate cheZ mutant, which does not display chemotactic response (Liu X. et al., 2018).
In capillary assays, the chemotactic response of the wild type toward oxalate, citrate, and lactate was dose-dependent from 1 μM to 10 mM, while the response toward succinate was biphasic, with attractive concentration maxima at 10 μM and more than 1 mM; response to acetate followed a bell curve, with maximum attractive concentration at around 1 mM (Figure 3A).
Figure 3. Chemotactic response of wild type and cheZ mutant strain toward different organic acids using capillary assay (A) and soft agar plate assay (B). The same letter above the error bars indicates no significant differences between them according to a Duncan’s test. Error bars represent means ± standard deviation of three independent experiments.
In soft agar plate assay, the wild type showed chemotactic response to all of the organic acids assayed, while no response was detected with the cheZ mutant used as negative control (Figure 3B). Although A. caulinodans cells grow better with 10 mM than with 1 mM acetate (data not shown), 10 mM is not a suitable concentration for attractive response in capillary assay. Therefore, 1 mM acetate was also used to explore chemotactic response on the soft agar plate. The chemotactic rings formed by cells with 1 mM acetate are much larger than those with 10 mM acetate (Figure 3B), consistent with the results in the capillary assay.
It was previously shown that deletion of the tlpA1 gene, which encodes the transmembrane chemotaxis receptor TlpA1, impaired the chemotactic response toward a broad range of ligands including tartrate, malate, proline, and glycerol (Liu et al., 2017) as well as succinate and citrate, identified as major organic acid components in seed exudates in the present work. Using soft agar plates, it was found that the ΔtlpA1 mutant strain was also defective in chemotaxis toward lactate and acetate (Figure 4A). However, as the chemotactic rings were reduced but not abolished, it is likely that other unidentified chemoreceptors are involved in the chemotactic response toward these organic acids. In the case of oxalate, as this substrate is not used for growth, it cannot be assayed using the soft agar plate system. This is why the capillary assay was used instead. Indeed, ΔtlpA1 was significantly defective in chemotactic response to oxalate, suggesting that TlpA1 is the cognate chemoreceptor to oxalate (Figure 4B).
Figure 4. Role of TlpA1 in the chemotactic response of A. caulinodans ORS571 toward different organic acids using soft agar plate assay (A) and capillary assay (B). Asterisks (∗∗P < 0.01) show a significant difference between conditions according to a t-test. Error bars are means ± standard deviation from at least three experiments.
Recently, we have found that aspartate, histidine, and arginine in seed exudates have indirect effects promoting the chemotactic response to succinate (Liu et al., 2019a). Therefore, we investigated whether oxalate also has an indirect effect on response to other organic acids using the soft agar plate assay. Indeed, as ORS571 cannot use oxalate as a growth substrate (Figures 2A,B), its concentration remained unchanged in soft agar plates.
Chemotactic ring diameters on soft agar plates were compared using either a single organic acid (succinate, citrate, acetate, or lactate) or the same organic acid as a carbon source plus oxalate. When 10 mM oxalate was added, the chemotactic response toward acetate and citrate decreased by around 10 and 40%, respectively, while chemotaxis toward lactate increased by about 25%. However, the chemotaxis toward succinate remained unchanged. Changes observed using the ΔtlpA1 mutant strain were similar to those with the wild type when oxalate was added (Figure 5A).
Figure 5. Effect of 10 mM oxalate on the chemotactic response of A. caulinodans ORS571 to other organic acids using soft agar plate assay (A) and capillary assay (B). Data are expressed as means ± standard deviation from at least three experiments.
The role of oxalate was then assayed using the capillary method. There were two types of treatments. In control groups, the cells in the wells were suspended with phosphate-buffered saline (PBS buffer) only. In the experimental group, cells in wells were suspended with PBS buffer already containing 10 mM oxalate to ensure that there were no gradients of oxalate between the solutions in wells and in capillaries. Compared with the control group, adding oxalate decreased the chemotaxis toward succinate by about 56%, while the chemotactic response to 1 mM acetate remained unchanged (Figure 5B). Unfortunately, in the presence of oxalate, the chemotactic response to lactate and citrate varied from one experiment to another so that no conclusion could be formulated (data not shown).
The indirect effect of oxalate on the chemotactic response differs depending on the organic acids used (Figure 5A). Experiments were repeated in order to find out whether the oxalate effect on each of the organic acids was concentration-dependent. Oxalate concentrations from 0 to 10 mM were used. In control experiments, the relative diameter of the chemotactic ring formed on soft agar plates induced by each of the organic acids (succinate, citrate, acetate, and lactate) in the absence of oxalate was normalized to 1. The relative diameters on a soft agar plate with each organic acid and different concentrations of oxalate were then compared with their respective controls.
The chemotactic response to different organic acids was found to depend on the oxalate concentration used. Chemotaxis toward succinate presented a trend of first decreasing and then increasing to the original level as the concentration of oxalate increased (Figure 6A). The changes for acetate were similar to those for succinate, but the diameter of its chemotactic rings did not reach the original level. The chemotactic response to lactate showed a trend of first increasing and then decreasing to a higher level than the original level. The chemotactic response to citrate was similar to the response to lactate, but it decreased to a lower level than the original level. Compared with the wild type, ΔtlpA1 showed different changes in chemotaxis toward citrate plus different concentrations of oxalate, while for the chemotactic response to succinate, acetate, and lactate, there were no differences between the two strains (Figures 6A,B).
Figure 6. Role of oxalate at different concentrations in the chemotactic response of wild type and ΔtlpA1 strains toward other organic acids using soft agar plate assay. The diameter of chemotactic rings with different organic acids was normalized by reference to chemotactic rings obtained in the absence of oxalate. Wild type (A) and ΔtlpA1 (B). Error bars represent means ± standard deviation from at least three experiments.
The role of oxalate on root surface colonization was determined by evaluating the colonization properties of A. caulinodans ORS571 at different concentrations of oxalate. After incubating for 1 h, bacterial cells colonizing the surface of S. rostrata root were counted. Oxalate at 0.1 and 1 mM did not affect the extent of root colonization by ORS571, while oxalate at 10 mM, which has a strong chemo-attractive effect, increased root colonization significantly more than 6-fold (Figure 7A). This result was confirmed by qualitative investigation of colonization using GFP marked strain ORS571-GFP. In the presence of 10 mM oxalate, colonies with green fluorescence can be observed on the surface of roots. Consistent with the quantitative result, there was no apparent green fluorescence on the root surface of seedlings when 0, 0.1, or 1 mM oxalate was added (Figure 7B).
Figure 7. Role of oxalate at different concentrations in the colonization of S. rostrata roots. Representative photographs showing the colonization of ORS571-eGFP (A). Quantitative comparison of the colonization of A. caulinodans (B). Asterisks (∗∗P < 0.01) indicate a significant difference from control according to a t-test. These experiments were performed at least three times. Values are means ± standard deviation from at least three experiments.
In this work, we explored the role of organic acids from Sesbania seed exudates on the interaction between S. rostrata and A. caulinodans. The results showed that A. caulinodans ORS571 could grow in the presence of citric acid, acetic acid, and lactic acid but could not grow in the presence of oxalic acid, indicating that the majority of the most abundant organic acids in exudates could serve as sole carbon resources. In addition, all the five most abundant organic acids in seed exudates could induce a strong chemotactic response, though their chemotactic strength at different concentrations was significantly different. Succinic acid chemotactic response showed a biphasic curve in capillary assay, indicating that succinic acid could function both at lower and higher concentrations. Compared with the other organic acids, succinic acid induced the strongest chemotactic response, showing that it might be an important signal molecule in seed exudates. In addition, oxalic acid is unique among the five organic acids. It is present at high concentration in exudates and could induce a strong chemotactic response, but it cannot be used to support growth.
In A. caulinodans, 43 genes encoding putative chemoreceptors were identified throughout the genome. Among them, TlpA1 has been reported to be a broad-range chemoreceptor. Chemoreceptors containing a broad range of ligands are more likely involved in the plant–microbe interactions. For example, deletion of genes encoding McpA and McpC possessing a broad range of ligands in B. amyloliquefaciens impaired the attraction toward cucumber exudates (Feng et al., 2018a, b). A significant decrease in colonization of Arabidopsis thaliana was observed in Bacillus subtilis mutants in which two chemoreceptor genes for amino acids, mcpB and mcpC, were inactivated (Allard-Massicotte et al., 2016). TlpA1 was previously shown to be involved in sensing some organic acids, glycerol, and amino acid in A. caulinodans (Liu et al., 2017). In addition, the ΔtlpA1 mutant was found to be impaired in competitive colonization and nodulation of Sesbania (Liu et al., 2017), which confirmed the role of broad-ligand range chemoreceptors in plant–microbe interactions. In this study, we further broadened the ligand range of TlpA1, including three of the five most abundant organic acids secreted in seed exudates. Thus, the defect of the ΔtlpA1 mutant strain in competitive colonization may result from the defect of chemotactic response to these organic acids. However, the deletion of tlpA1 in A. caulinodans ORS571 resulted in a partial defect on the chemotactic response to organic acids and some other attractants, suggesting that other potential cognate chemoreceptors may be involved and that these chemoreceptors may contribute to the attraction toward the root surface collaboratively.
Oxalic acid and its conjugated base, oxalate, are found in various terrestrial environments including the rhizosphere of plants (Palmieri et al., 2019). They have been found in the exudates of many plants, such as tomato (de Weert et al., 2002; Tan et al., 2013), Barmultra grass (Kuiper et al., 2002), tobacco (Wu et al., 2015), banana (Yuan et al., 2015), and others. How can oxalic acid from exudates affect A. caulinodans–S. rostrata interactions? First, oxalic acid, as a chemoreceptor ligand, could induce a strong chemotactic response. Chemotactic behavior is a key factor for the colonization of hosts (Erhardt, 2016). As previously described, many organic acids from plant exudates can induce a chemotactic response and promote bacterial recruitment (de Weert et al., 2004; Tan et al., 2013). Second, oxalic acid regulates chemotactic behavior using indirect strategies as a potential signal affecting the chemotactic response of A. caulinodans to other stimuli. When oxalic acid was added on a soft agar plate, it affected the chemotactic response to different organic acids. In Pseudomonas putida, acetate could improve the transcript level of McpQ, whose cognate ligand is citrate, up to 20-fold (Lopez-Farfan et al., 2017), suggesting that the indirect role of some organic acids on chemoeffectors is not specific to A. caulinodans.
In this work, we further found that the indirect role of oxalic acid on A. caulinodans chemotactic response toward different organic acids depends on its concentration. At low concentrations, oxalic acid promotes the chemotactic response of bacteria toward organic acid, while it reduces the chemotactic response to them at higher concentrations. In other words, oxalic acid is a chemotaxis enhancer at low concentrations, while, at high concentrations, oxalic acid is a chemotaxis inhibitor.
What is the physiological significance of the concentration-dependent indirect role of oxalic acid on the building of A. caulinodans–S. rostrata associations?
First, the significance of the concentration-dependent effect of oxalic acid might result in promoting bacteria located at some distance to move toward plants quickly and in preventing the escape of bacteria from the spermosphere and rhizosphere areas. A concentration-dependent effect of maize root exudates on chemotaxis has also been reported, and exudates could promote the expression of chemoreceptors at a distance from plants (Lopez-Farfan et al., 2019). Second, oxalic acid might be a colonization enhancer of A. caulinodans at high concentrations. In general, adding an exogenous strong chemotactic attractant could impair the establishment of bacteria and host associations. For Vibrio fischeri, the efficiency of colonization of host squid could be diminished with exogenous addition of chemoattractant (GlcNAc)2, causing chemotactic gradient disruption (Mandel et al., 2012). For H. pylori, adding exogenous urea into the lumen of mouse stomach could also confound bacterial accumulation to damage sites, which can be detected by a urea chemoreceptor, TlpB (Hanyu et al., 2019). However, the strong chemotactic response induced by a low concentration of oxalic acid did not disturb A. caulinodans root colonization, while a high concentration of oxalic acid enhanced the colonization. These results indicate that the direct role of oxalic acid on chemotaxis might not be essential for colonization of A. caulinodans. Third, oxalic acid might be a switch that regulates movement and colonization on the plant surface. At low concentrations, oxalic acid promotes motility, while at high concentrations, oxalic acid enhances colonization. The second messenger c-di-GMP was shown to be a key factor affecting motility, chemotactic behavior, and biofilm formation, but Lopez-Farfan et al. (2019) established that c-di-GMP was not involved in chemotaxis promotion by exudates. At this stage, a possible role for oxalic acid on the c-di-GMP level cannot be excluded, even though we have failed to quantify c-di-GMP so far. It remains that a dual role of oxalic acid on motility and colonization is shown in this study. Since chemotaxis and biofilm formation both are prerequisites for colonization, we suspect that oxalic acid could also promote biofilm formation on abiotic surfaces. Unfortunately, calcium ions are essential for the biofilm formation of A. caulinodans (unpublished data), and the formation of calcium oxalate prevented us from exploring the potential role of oxalic acid on biofilm formation.
In this work, we established that the five most abundant organic acids, including oxalic acid, in S. rostrata seed exudates were involved in A. caulinodans chemotaxis and identified that TlpA1 was their cognate chemoreceptor. In addition, we determined that oxalic acid contributes a concentration-dependent effect on the chemotactic response of A. caulinodans ORS571 using several strategies. These results are important for understanding the role of organic acid on the bacteria–host interactions. Under gnotobiotic conditions, the relative concentration of oxalic acid resulting from seed and root exudation decreases depending on distance from the root system but may remain stable since it cannot be metabolized by A. caulinodans. However, in natural soil environments, the metabolism of oxalic acid by oxalotrophic bacteria could constitute a strong sink for oxalic acid and thereby enhance the formation of concentration gradients of oxalic acid in the rhizosphere (Canarini et al., 2019). Thus, the real role of oxalic acid on A. caulinodans and S. rostrata may be stronger and more complicated. Further work will explore the specific pathways or proteins regulated by oxalic acid and its effect on the microbial community.
All strains and plasmids used in this study were listed in Table 1. A. caulinodans ORS571 (Dreyfus et al., 1988) and its derivatives, ΔcheZ and ΔtlpA1, were grown in TY liquid media or in modified L3 minimal medium (containing 10 g/L DL sodium lactate as a carbon source and 10 mM NH4Cl as a nitrogen source) at 37°C, as previously described (Jiang et al., 2016). For growth and chemotaxis assays, in liquid or soft agar plates, the L3 minimal medium mineral base (devoid of carbon source) was supplemented with the carbon sources indicated in the text (see below). The concentrations of antibiotics used for A. caulinodans were as follows: 50 μg ml–1 kanamycin, 100 μg ml–1 ampicillin, and 50 μg ml–1 gentamycin.
To construct a fluorescent marked A. caulinodans, the egfp gene from pEGFP-N1 (Mountain View, CA, United States) was amplified by PCR using one primer pair (gfp-SalI-F: GTCGACATGGTGAGCAAGGGCGAG, and gfp-XbaI-R: TCTAGA TTACTTGTACAGCTCGTC). The amplicon and a broad host plasmid pBBR1MCS-2 (Kovach et al., 1995) were then digested with restriction enzymes (SalI and XbaI) and linked together to generate pBBR-eGFP. The pBBR-eGFP was next introduced into ORS571 by triparental conjugation using helper plasmid pRK2013 (Ditta et al., 1980), using kanamycin as a selective marker, and the fluorescence of the resulting transconjugant strain ORS571-eGFP was verified by microscopy.
Sesbania rostrata seeds (40 g) were treated with sulfuric acid to sterilize the surface, as previously described (Liu et al., 2019a). After being soaked in the concentrated sulfuric acid for 30 min, seeds were washed with sterilized water at least five times and transferred to an incubator at 37°C for 48 h. After 48 h, 100 ml of sterile water was added to the seeds to collect the exudates. This was performed two times. The liquid phase containing the exudates was then recovered and used for the analysis of organic acids.
An HPLC AQ-C18 (4.6 × 250 μm, Agilent, Santa Clara, CA, United States) was used to analyze the low molecular weight organic acids. The mobile phase consisted of solutions of 10 mmol L–1 K2HPO4, at pH 2.55, with a gradient elution of 0.5 mL min–1. Peak detection was performed with an ultraviolet (UV) detector at 210 nm. Nine organic acids that are common in plant exudates, namely oxalic acid, tartaric acid, malic acid, lactic acid, acetic acid, citric acid, succinic acid, maleic acid, and fumaric acid, were used as standards. Ten microliter exudate samples and standards were sequentially injected into the chromatographic system under the same running conditions. Each sample was analyzed at least three times.
Azorhizobium caulinodans cells were cultured in TY medium overnight. Cells were collected and washed with L3 minimal medium without a carbon source at least three times. The cell suspension was then inoculated into L3 minimal medium containing the desired source of organic acid at the concentration indicated in the text and adjusted at pH 7.2, at an initial OD600 of 0.01. Culture OD was determined sequentially over 36 h. The determination of growth of A. caulinodans ORS571 on soft agar plates (0.3%) with oxalic acid as a sole carbon source was made according to Rudnick et al. (2015). Plates were incubated at 37°C. At 0, 12, 24, 36, and 48 h, 2 ml of 1 g/L NaCl was added into each plate to swirl and collect the suspended bacteria, and the OD600 of the suspension was determined. More than five plates were inoculated as necessary for OD determination.
For capillary assay, cell suspensions adjusted to an OD600 of 0.01 were inoculated into a 96-well plate, as described previously (Liu X. et al., 2018). Capillaries (Microcaps, Dummond Scientific) that were open at one end were inserted into the desired chemoattractant solution at the concentrations indicated in the text and then inserted into the well plates at 37°C for 1 h, as described in Liu X. et al. (2018). Capillaries filled with PBS were used as control. The liquid in the capillaries was then pipetted into 1 ml PBS and, after serial dilution, plated on TY solid medium for counting of colony-forming units (CFUs). The experiments were repeated at least three times.
The role of oxalic acid on the chemotactic response to other organic acids was determined by adding oxalic acid into PBS and adjusting the pH to 7.2. This solution was either used for bacterial suspension or supplemented by other organic acids, as indicated in the text.
For the soft agar plate assay, overnight cultures were collected and washed with L3 minimal medium devoid of a carbon source. Then, 5 ml of bacterial cell suspension adjusted to an OD600 of 0.6 were dropped into the center of soft agar plates (0.3% agar) containing the desired organic acids (succinic acid, oxalic acid, acetic acid, lactic acid, and citric acid) as the substrate. When exploring the role of oxalic acid in chemotaxis, the oxalic acid was added onto the soft agar plate and was then mixed uniformly. After culturing at 37°C for 48 h, the diameter of each chemotactic ring was recorded.
Seeds sterilized as described above were germinated and grown under sterile conditions for 3 days to obtain seedlings. Overnight cultures of A. caulinodans were washed three times and adjusted to an OD600 of 0.01. Seedlings were immersed in L3 minimal base medium with different concentrations of oxalic acid (0, 0.1, 1, and 10 mM) (as oxalate was not used) for 1 h at 37°C. Seedlings were then removed and washed with sterilized water at least five times. The roots were cut off and vortexed to recover the attached bacteria. One milliliter of sterilized water was added to suspend the cells from the vortexed root surface, and serial dilutions were plated onto TY solid medium supplemented with antibiotics. After culturing for 48 h at 37°C, the CFUs were counted and analyzed.
Qualitative observations of seedlings colonized with A. caulinodans ORS571-eGFP were performed using a fluorescence microscope Olympus BX53 system microscope and recorded by an Olympus DP73 digital camera at ×10 magnification. The fluorescence signal was detected using a green fluorescent protein (eGFP) filter set. Images were taken under the same conditions.
Mean and standard errors were computed based on experiments in this work repeated at least three times. A t-test (P < 0.01) was used to determine significant differences from control in capillary assay and colonization assay. A Duncan’s test (P < 0.01) was used for multiple comparisons. SPSS version 20 (IBM Corp., Armonk, NY, United States) was employed to perform analysis of variance (ANOVA) and various tests.
This manuscript contains previously unpublished data. The name of the repository and accession number(s) are not available.
XL and ZX conceived and designed the experiments, analyzed the data, prepared the figures and tables, and wrote the manuscript. XL, KZ, and YL carried out the experiments. CZ helped with the improvement and revision of the manuscript. All authors approved the submitted manuscript for publication.
This work was supported by the NSFC-Shandong Joint Fund Key Projects (U1806206), the National Natural Science Foundation of China (31570063 and 31870020), the Shandong Key Research and Development Program (2017GSF17129), and the Key Deployment Project of Chinese Academy of Sciences (KFZD-SW-112).
The authors declare that the research was conducted in the absence of any commercial or financial relationships that could be construed as a potential conflict of interest.
The authors wish to thank Claudine Elmerich for helpful and insightful comments on the manuscript.
Allard-Massicotte, R., Tessier, L., Lecuyer, F., Lakshmanan, V., Lucier, J. F., Garneau, D., et al. (2016). Bacillus subtilis early colonization of Arabidopsis thaliana roots involves multiple chemotaxis receptors. mBio 7:e1664-16.
Berendsen, R. L., Pieterse, C. M. J., and Bakker, P. A. (2012). The rhizosphere microbiome and plant health. Trends Plant Sci. 17, 478–486. doi: 10.1016/j.tplants.2012.04.001
Caetano-Anolles, G., Wall, L. G., De Micheli, A. T., Macchi, E. M., Bauer, W. D., and Favelukes, G. (1988). Role of motility and chemotaxis in efficiency of nodulation by Rhizobium meliloti. Plant Physiol. 86, 1228–1235. doi: 10.1104/pp.86.4.1228
Canarini, A., Kaiser, C., Merchant, A., Richter, A., and Wanek, W. (2019). Root exudation of primary metabolites: mechanisms and their roles in plant responses to environmental stimuli. Front. Plant Sci. 10:157. doi: 10.3389/fpls.2019.00157
de Weert, S., Kuiper, I., Lagendijk, E. L., Lamers, G. E., and Lugtenberg, B. J. (2004). Role of chemotaxis toward fusaric acid in colonization of hyphae of Fusarium oxysporum f. sp. radicis-lycopersici by Pseudomonas fluorescens WCS365. Mol. Plant Microbe Interact. 17, 1185–1191.
de Weert, S., Vermeiren, H., Mulders, I. H., Kuiper, I., Hendrickx, N., Bloemberg, G. V., et al. (2002). Flagella-driven chemotaxis towards exudate components is an important trait for tomato root colonization by Pseudomonas fluorescens. Mol. Plant Microbe Interact. 15, 1173–1180. doi: 10.1094/mpmi.2002.15.11.1173
Ditta, G., Stanfield, S., Corbin, D., and Helinski, D. R. (1980). Broad host range DNA cloning system for gram-negative bacteria: construction of a gene bank of Rhizobium meliloti. Proc. Natl. Acad. Sci. U.S.A. 77, 7347–7351. doi: 10.1073/pnas.77.12.7347
Dreyfus, B., Garcia, J. L., and Gillis, M. (1988). Characterization of Azorhizobium caulinodans gen. nov., sp. nov., a stem-nodulating nitrogen-fixing bacterium isolated from Sesbania rostrata. Int. J. Syst. Evol. Microbiol. 38, 89–98. doi: 10.1099/00207713-38-1-89
Eisenbach, M. (1996). Control of bacterial chemotaxis. Mol. Microbiol. 20, 903–910. doi: 10.1111/j.1365-2958.1996.tb02531.x
Erhardt, M. (2016). Strategies to block bacterial pathogenesis by interference with motility and chemotaxis. Curr. Top. Microbiol. Immunol. 398, 185–205. doi: 10.1007/82_2016_493
Feng, H., Zhang, N., Du, W., Zhang, H., Liu, Y., Fu, R., et al. (2018a). Identification of chemotaxis compounds in root exudates and their sensing chemoreceptors in plant-growth-promoting rhizobacteria Bacillus amyloliquefaciens SQR9. Mol. Plant Microbe Interact. 31, 995–1005. doi: 10.1094/MPMI-01-18-0003-R
Feng, H., Zhang, N., Fu, R., Liu, Y., Krell, T., Du, W., et al. (2018b). Recognition of dominant attractants by key chemoreceptors mediates recruitment of plant growth-promoting rhizobacteria. Environ. Microbiol. 21, 402–415. doi: 10.1111/1462-2920.14472
Fritsche, K., Van Den Berg, M., De Boer, W., Van Beek, T. A., Raaijmakers, J. M., Van Veen, J. A., et al. (2014). Biosynthetic genes and activity spectrum of antifungal polyynes from Collimonas fungivorans Ter331. Environ. Microbiol. 16, 1334–1345. doi: 10.1111/1462-2920.12440
Haichar, F. E. Z., Santaella, C., Heulin, T., and Achouak, W. (2014). Root exudates mediated interactions belowground. Soil Biol. Biochem. 77, 69–80. doi: 10.1016/j.soilbio.2014.06.017
Hamel, R., Levasseur, R., and Appanna, V. D. (1999). Oxalic acid production and aluminum tolerance in Pseudomonas fluorescens. J. Inorg. Biochem. 76, 99–104. doi: 10.1016/s0162-0134(99)00120-8
Hanyu, H., Engevik, K. A., Matthis, A. L., Ottemann, K. M., Montrose, M. H., and Aihara, E. (2019). Helicobacter pylori use TlpB receptor to sense sites of gastric injury. Infect. Immun. 87, e202–e219. doi: 10.1128/IAI.00202-19
Hida, A., Oku, S., Kawasaki, T., Nakashimada, Y., Tajima, T., and Kato, J. (2015). Identification of the mcpA and mcpM genes, encoding methyl-accepting proteins involved in amino acid and l-malate chemotaxis, and involvement of McpM-mediated chemotaxis in plant infection by Ralstonia pseudosolanacearum (formerly Ralstonia solanacearum phylotypes I and III). Appl. Environ. Microbiol. 81, 7420–7430. doi: 10.1128/AEM.01870-15
Jain, A., and Chen, W. N. (2018). Involvement of organic acids and amino acids in ameliorating Ni(II) toxicity induced cell cycle dysregulation in Caulobacter crescentus: a metabolomics analysis. Appl. Microbiol. Biotechnol. 102, 4563–4575. doi: 10.1007/s00253-018-8938-0
Janczarek, M., and Skorupska, A. (2011). Modulation of rosR expression and exopolysaccharide production in Rhizobium leguminosarum bv. trifolii by phosphate and clover root exudates. Int. J. Mol. Sci. 12, 4132–4155. doi: 10.3390/ijms12064132
Jiang, N., Liu, W., Li, Y., Wu, H., Zhang, Z., Alexandre, G., et al. (2016). A chemotaxis receptor modulates nodulation during the Azorhizobium caulinodans-Sesbania rostrata symbiosis. Appl. Environ. Microbiol. 82, 3174–3184. doi: 10.1128/AEM.00230-16
Jones, D. L., Hodge, A., and Kuzyakov, Y. (2004). Plant and mycorrhizal regulation of rhizodeposition. New Phytol. 163, 459–480. doi: 10.1111/j.1469-8137.2004.01130.x
Kamilova, F., Kravchenko, L. V., Shaposhnikov, A. I., Azarova, T., Makarova, N., and Lugtenberg, B. (2006). Organic acids, sugars, and L-tryptophane in exudates of vegetables growing on stonewool and their effects on activities of rhizosphere bacteria. Mol. Plant Microbe Interact. 19, 250–256. doi: 10.1094/mpmi-19-0250
Kovach, M. E., Elzer, P. H., Hill, D. S., Robertson, G. T., Farris, M. A., and Roop, R. M.II, et al. (1995). Four new derivatives of the broad-host-range cloning vector pBBR1MCS, carrying different antibiotic-resistance cassettes. Gene 166, 175–176. doi: 10.1016/0378-1119(95)00584-1
Kuiper, I., Kravchenko, L. V., Bloemberg, G. V., and Lugtenberg, B. J. (2002). Pseudomonas putida strain PCL1444, selected for efficient root colonization and naphthalene degradation, effectively utilizes root exudate components. Mol. Plant Microbe Interact. 15, 734–741. doi: 10.1094/mpmi.2002.15.7.734
Li, S. L., Xu, C., Wang, J., Guo, B., Yang, L., Chen, J. N., et al. (2017). Cinnamic, myristic and fumaric acids in tobacco root exudates induce the infection of plants by Ralstonia solanacearum. Plant Soil 412, 381–395. doi: 10.1007/s11104-016-3060-5
Liu, W., Sun, Y., Shen, R., Dang, X., Liu, X., Sui, F., et al. (2018). A chemotaxis-like pathway of Azorhizobium caulinodans controls flagella-driven motility, which regulates biofilm formation, exopolysaccharide biosynthesis, and competitive nodulation. Mol. Plant Microbe Interact. 31, 737–749. doi: 10.1094/MPMI-12-17-0290-R
Liu, X., Liu, W., Sun, Y., Xia, C., Elmerich, C., and Xie, Z. (2018). A cheZ-like gene in Azorhizobium caulinodans is a key gene in the control of chemotaxis and colonization of the host plant. Appl. Environ. Microbiol. 84:e1827-17. doi: 10.1128/AEM.01827-17
Liu, W., Yang, J., Sun, Y., Liu, X., Li, Y., Zhang, Z., et al. (2017). Azorhizobium caulinodans transmembrane chemoreceptor TlpA1 involved in host colonization and nodulation on roots and stems. Front. Microbiol. 8:1327. doi: 10.3389/fmicb.2017.01327
Liu, X., Xie, Z., Wang, Y., Sun, Y., Dang, X., and Sun, H. (2019a). A dual role of amino acids from Sesbania rostrata seed exudates in the chemotaxis response of Azorhizobium caulinodans ORS571. Mol. Plant Microbe Interact. 32, 1134–1147. doi: 10.1094/MPMI-03-19-0059-R
Liu, X., Xie, Z., and Zhang, H. (2019b). Inactivation of the phosphatase CheZ alters cell-surface properties of Azorhizobium caulinodans ORS571 and symbiotic association with Sesbania rostrata. Mol. Plant Microbe Interact. 32, 1547–1556. doi: 10.1094/MPMI-05-19-0143-R
Lopez-Farfan, D., Reyes-Darias, J. A., and Krell, T. (2017). The expression of many chemoreceptor genes depends on the cognate chemoeffector as well as on the growth medium and phase. Curr. Genet. 63, 457–470. doi: 10.1007/s00294-016-0646-7
Lopez-Farfan, D., Reyes-Darias, J. A., Matilla, M. A., and Krell, T. (2019). Concentration dependent effect of plant root exudates on the chemosensory systems of Pseudomonas putida KT2440. Front. Microbiol. 10:78. doi: 10.3389/fmicb.2019.00078
Mandel, M. J., Schaefer, A. L., Brennan, C. A., Heath-Heckman, E. A., Deloney-Marino, C. R., Mcfall-Ngai, M. J., et al. (2012). Squid-derived chitin oligosaccharides are a chemotactic signal during colonization by Vibrio fischeri. Appl. Environ. Microbiol. 78, 4620–4626. doi: 10.1128/AEM.00377-12
Martins, S. J., Medeiros, F. H. V., Lakshmanan, V., and Bais, H. P. (2017). Impact of seed exudates on growth and biofilm formation of Bacillus amyloliquefaciens ALB629 in common bean. Front. Microbiol. 8:2631. doi: 10.3389/fmicb.2017.02631
Merritt, P. M., Danhorn, T., and Fuqua, C. (2007). Motility and chemotaxis in Agrobacterium tumefaciens surface attachment and biofilm formation. J. Bacteriol. 189, 8005–8014. doi: 10.1128/jb.00566-07
Miller, L. D., Yost, C. K., Hynes, M. F., and Alexandre, G. (2007). The major chemotaxis gene cluster of Rhizobium leguminosarum bv. viciae is essential for competitive nodulation. Mol. Microbiol. 63, 348–362. doi: 10.1111/j.1365-2958.2006.05515.x
Neal, A. L., Ahmad, S., Gordon-Weeks, R., and Ton, J. (2012). Benzoxazinoids in root exudates of maize attract Pseudomonas putida to the rhizosphere. PLoS One 7:e35498. doi: 10.1371/journal.pone.0035498
Nelson, E. B. (2004). Microbial dynamics and interactions in the spermosphere. Annu. Rev. Phytopathol. 42, 271–309. doi: 10.1146/annurev.phyto.42.121603.131041
Palmieri, F., Estoppey, A., House, G. L., Lohberger, A., Bindschedler, S., Chain, P. S. G., et al. (2019). Oxalic acid, a molecule at the crossroads of bacterial-fungal interactions. Adv. Appl. Microbiol. 106, 49–77. doi: 10.1016/bs.aambs.2018.10.001
Rudnick, M. B., Van Veen, J. A., and De Boer, W. (2015). Oxalic acid: a signal molecule for fungus-feeding bacteria of the genus Collimonas? Environ. Microbiol. Rep. 7, 709–714. doi: 10.1111/1758-2229.12290
Sahin, N. (2003). Oxalotrophic bacteria. Res. Microbiol. 154, 399–407. doi: 10.1016/s0923-2508(03)00112-8
Scharf, B. E., Hynes, M. F., and Alexandre, G. M. (2016). Chemotaxis signaling systems in model beneficial plant-bacteria associations. Plant Mol. Biol. 90, 549–559. doi: 10.1007/s11103-016-0432-4
Szurmant, H., and Ordal, G. W. (2004). Diversity in chemotaxis mechanisms among the bacteria and archaea. Microbiol. Mol. Biol. Rev. 68, 301–319. doi: 10.1128/mmbr.68.2.301-319.2004
Tan, S. Y., Yang, C. L., Mei, X. L., Shen, S. Y., Raza, W., Shen, Q. R., et al. (2013). The effect of organic acids from tomato root exudates on rhizosphere colonization of Bacillus amyloliquefaciens T-5. Appl. Soil Ecol. 64, 15–22. doi: 10.1016/j.apsoil.2012.10.011
Ul Haq, I., Zwahlen, R. D., Yang, P., and Van Elsas, J. D. (2018). The response of Paraburkholderia terrae strains to two soil fungi and the potential role of oxalate. Front. Microbiol. 9:989. doi: 10.3389/fmicb.2018.00989
Van Hees, P. A. W., Rosling, A., Essén, S., Godbold, D. L., Jones, D. L., and Finlay, R. D. (2006). Oxalate and ferricrocin exudation by the extramatrical mycelium of an ectomycorrhizal fungus in symbiosis with Pinus sylvestris. New Phytol. 169, 367–378. doi: 10.1111/j.1469-8137.2005.01600.x
Wall, L. G., and Favelukes, G. (1991). Early recognition in the rhizobium-meliloti-alfalfa symbiosis - root exudate factor stimulates root adsorption of homologous rhizobia. J. Bacteriol. 173, 3492–3499. doi: 10.1128/jb.173.11.3492-3499.1991
Wu, K., Yuan, S., Xun, G., Shi, W., Pan, B., Guan, H., et al. (2015). Root exudates from two tobacco cultivars affect colonization of Ralstonia solanacearum and the disease index. Eur. J. Plant Pathol. 141, 667–677. doi: 10.1007/s10658-014-0569-4
Yuan, J., Zhang, N., Huang, Q., Raza, W., Li, R., Vivanco, J. M., et al. (2015). Organic acids from root exudates of banana help root colonization of PGPR strain Bacillus amyloliquefaciens NJN-6. Sci. Rep. 5:13438. doi: 10.1038/srep13438
Keywords: organic acids, chemotaxis, plant exudates, colonization, oxalic acid
Citation: Liu X, Zhang K, Liu Y, Xie Z and Zhang C (2019) Oxalic Acid From Sesbania rostrata Seed Exudates Mediates the Chemotactic Response of Azorhizobium caulinodans ORS571 Using Multiple Strategies. Front. Microbiol. 10:2727. doi: 10.3389/fmicb.2019.02727
Received: 08 August 2019; Accepted: 08 November 2019;
Published: 03 December 2019.
Edited by:
Camille Eichelberger Granada, Universidade do Vale do Taquari – Univates, BrazilReviewed by:
Nan Zhang, Nanjing Agricultural University, ChinaCopyright © 2019 Liu, Zhang, Liu, Xie and Zhang. This is an open-access article distributed under the terms of the Creative Commons Attribution License (CC BY). The use, distribution or reproduction in other forums is permitted, provided the original author(s) and the copyright owner(s) are credited and that the original publication in this journal is cited, in accordance with accepted academic practice. No use, distribution or reproduction is permitted which does not comply with these terms.
*Correspondence: Zhihong Xie, emh4aWVAeWljLmFjLmNu; Chengsheng Zhang, emhjaGVuZ3NoZW5nQDEyNi5jb20=
Disclaimer: All claims expressed in this article are solely those of the authors and do not necessarily represent those of their affiliated organizations, or those of the publisher, the editors and the reviewers. Any product that may be evaluated in this article or claim that may be made by its manufacturer is not guaranteed or endorsed by the publisher.
Research integrity at Frontiers
Learn more about the work of our research integrity team to safeguard the quality of each article we publish.