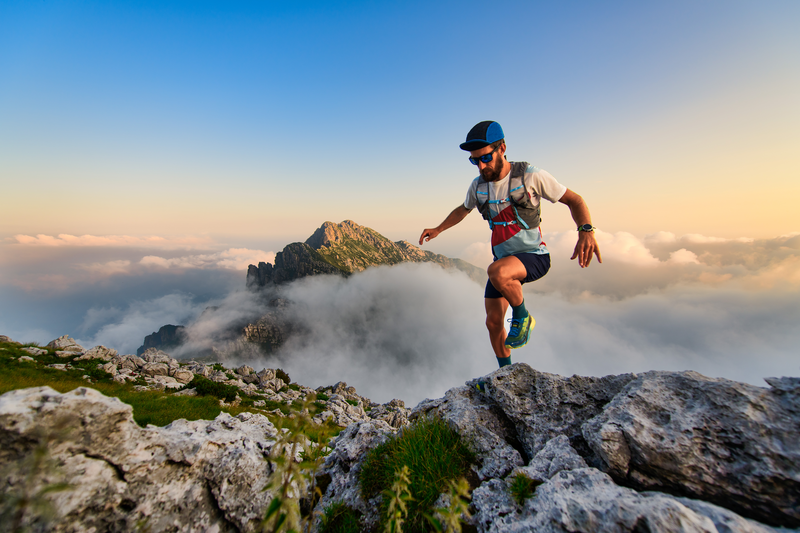
95% of researchers rate our articles as excellent or good
Learn more about the work of our research integrity team to safeguard the quality of each article we publish.
Find out more
ORIGINAL RESEARCH article
Front. Microbiol. , 08 November 2019
Sec. Food Microbiology
Volume 10 - 2019 | https://doi.org/10.3389/fmicb.2019.02527
Brochothrix thermosphacta is one of the main spoilers in food, responsible for meat and seafood spoilage through the production of malodorous volatile organic compounds. The molecules produced by this bacterium depend on the substrate (meat or seafood) and the storage conditions such as gas mixtures used in the packaging. It seems also that the spoilage potential is strain dependent as production of diacetyl and acetoin, two molecules responsible for seafood spoilage, varies with strains. Therefore, this suggests the involvement of different metabolic functions depending on both food substrate and strain capacities. In this study, we selected two strains with different abilities to produce diacetyl and acetoin and compared their behavior after grown in beef or cooked peeled shrimp juices. We determined the genes upregulated by both strains depending on the growth substrate and those that were specifically upregulated in only one strain. The genes upregulated by both strains in meat or in shrimp juice revealed the importance of the substrate for inducing specific metabolic pathways. The examination of genes that were specifically upregulated in only one of the two strains revealed strain features associated to specific substrates and also strain-specific regulations of metabolic pathways putatively leading to different levels of spoilage molecule production. This shows that the spoilage potential of B. thermosphacta depends on nutrients provided by food substrate and on metabolic activity potential that each strain possesses.
Brochothrix thermosphacta is commonly reported as belonging to the microbiota of a wide range of food matrices including various raw and processed meat and seafood products (Chaillou et al., 2015; Remenant et al., 2015; Illikoud et al., 2018a). This results from its ubiquitous nature and its ability to grow at the refrigerated temperatures in various atmosphere packaging used for food storage (Stackebrandt and Jones, 2006). B. thermosphacta has been frequently associated to food spoilage through the production of volatile organic compounds (VOCs) causing off-odors (Casaburi et al., 2014, 2015; Odeyemi et al., 2018). The production of such molecules seems to be food matrix dependent (Illikoud et al., 2018a). For example, acetoin, diacetyl, and 3-methylbutanol associated with cheesy and creamy dairy off-odors have been reported in beef meat (Dainty et al., 1989; Casaburi et al., 2014), while in cooked and peeled shrimp, diacetyl and 3-methyl-1-butanal were produced and responsible for strong butter, buttermilk-like, sour, and nauseous off-odors (Jaffrès et al., 2011). Recently, genotypic and phenotypic analysis of a B. thermosphacta strain collection revealed that genetic diversity was not related to strain ecological origin (Illikoud et al., 2019). The amounts of acetoin and diacetyl produced were variable among strains, but also depend on the type of food matrix used to grow bacteria. Hence, these results suggest that the spoilage potential of B. thermosphacta may be both strain- and food matrix-dependent. Genome comparison showed a high degree of similarity in the gene repertoire of B. thermosphacta strains (Stanborough et al., 2017; Illikoud et al., 2018b). The major differences in the gene content consisted of phages and plasmids, or genes encoding cell surface proteins or adhesins, which could not explain the different spoilage abilities of the strains but rather suggested a variable fitness for growing in different ecological niches. Nevertheless, different base substitutions in or upstream from genes encoding enzymes required for the production of spoilage molecules were noticed when comparing several B. thermosphacta genomes (Illikoud et al., 2018b). This suggests that different spoilage abilities among strains may rely on differences in gene expression or enzyme activity levels. The characterization of the genes expressed and the metabolites produced by B. thermosphacta strains in different food matrices should contribute to better understand the B. thermosphacta spoilage mechanisms and the reasons for spoilage potential differences. Therefore, our strategy for the present study was to compare volatilomes and transcriptomes of two different strains inoculated in two model matrices (meat and shrimp juices). Our objective was to discriminate the strain effect from the matrix effect by searching the genes differentially expressed depending on the matrix and on the strain. We also attempted to identify metabolic pathways involved in the spoilage potential of B. thermosphacta in meat and in shrimp.
Brochothrix thermosphacta CD 337 and TAP 175 (Illikoud et al., 2018b) isolated from spoiled cooked peeled shrimp and non-spoiled chicken cuts, respectively, were used in this study. The strains were stored at −80°C in Brain Heart Infusion (BHI) broth (VWR, France) added with 20% (v/v) glycerol and were routinely grown at 25°C in BHI medium.
Bacterial enumeration were performed after serial dilutions and plating on Plate Count Agar (PCA) (Biomerieux, France) for total viable counts and on selective STAA agar base containing STAA selective supplement (Oxoid, France) for B. thermosphacta, after incubation for 48 h at 30 and 25°C, respectively.
Meat and shrimp juices were prepared as previously described (Illikoud et al., 2019) with slight modifications as described below. Ground beef (25 g) purchased from a local supermarket was stomached in 100 ml of Ringer’s solution (Oxoid, France) and the mixture was centrifuged at 6000 × g for 20 min at 4°C. The supernatant was then filtered through a 0.45-μm membrane filter before sterilization with a 0.2-μm membrane filter. Cooked peeled shrimp juice was prepared by crushing 100 g of frozen raw peeled shrimps from Ecuador (91/100 without sulfite, purchased from industry, Nantes, France) in 300 ml of sterile distilled water. The mixture was heated (100°C; 2 min) and centrifuged at 600 × g for 20 min, and the supernatant was autoclaved (100°C; 30 min). These juices, mimicking raw ground beef and cooked peeled shrimp, were aliquoted by 20 ml in 45-ml sterile glass vials and then stored frozen at −80°C until use.
B. thermosphacta strains were harvested from overnight cultures (4 ml) by centrifugation (13,400 × g, 5 min, 4°C), washed with 1 ml of Ringer’s solution, centrifuged with the same parameters, resuspended in 1 ml of meat or shrimp juice, and inoculated in 20 ml of food juice at an initial concentration of 106 CFU/ml. Glass vials were closed and inoculated juices were incubated at 8°C. Those conditions generated a microaerophilic environment during storage. Non-inoculated juice samples were included as controls. Bacterial enumeration, volatilome analysis, and pH measurement (Crison pH-meter) were performed at day 0, 1, 2, 4, and 7, whereas transcriptome analysis was performed at day 4. Challenge tests were performed in triplicate.
At each sampling time, vials were immediately frozen at −20°C without opening the vials and stored frozen until analysis. For VOC analysis, frozen vials were thawed overnight at room temperature. Nine milliliters of sample extract was transferred into a 20-ml headspace vial and sealed with a screwcap with silicone rubber septum. Analyses were carried out in triplicate.
Volatile organic compounds were determined by HS–GC–MS. All analyses were performed on a Varian 450 gas chromatograph (Varian, Palo Alto, CA, United States) coupled to a Varian 225-IT mass spectrometer (Varian), equipped with a CTC Combi PAL (CTC Analytics AG, Zwingen, Switzerland). Samples were equilibrated by agitation at 60°C for 20 min prior to injection and 1 ml was drawn out from the head space to inject in the GC. The HS-GC–MS conditions were as follows. Capillary column: DB-624 UI (30 m × 0.25 mm I.D × 1.4 mm film thickness) (Agilent Technologies); carrier gas: helium with a flow rate of 1.4 ml/min; injection port mode: splitless; needle temperature: 60°C; injection temperature: 220°C. The oven temperature was programed from an initial temperature of 40°C (7 min holding), rising to 50°C at 4°C/min (1 min holding), to 70°C at 4°C/min (1 min holding), to 120°C at 3°C/min (2 min holding), and to 245°C at 30°C/min (4 min holding). Transfer line temperature was 250°C. The temperatures of the manifold and the ion trap were kept constant at 150 and 40°C, respectively. Results were obtained in scan mode at 4 scans/s in the mass range (m/z) of 35–350 atomic mass units. VOCs were identified by comparison of GC retention times and mass spectra with those of the standard compounds (LGC Standards, France; Techlab, France and Cluzeau Info Labo, France). These standard compounds were selected based on a review of published data of VOCs detected in meat and seafood spoiled in the presence of B. thermosphacta (Illikoud et al., 2018a). Peak area (in arbitrary unit) was used as quantitative data to monitor the relative changes of VOCs over storage and, where appropriate, to correlate these findings with bacterial strain as well as with the studied food matrices. This relative quantification was scored from - (no detection) to +++ (highest detection).
At day 4, 1 ml of the inoculated juices was sampled and bacteria were harvested by centrifugation at 10,000 × g for 5 min at 4°C. The cell pellet was immediately resuspended in 200 μl of RNA Protect Cell Reagent (Qiagen, Courtaboeuf, France) and frozen at −80°C. Total RNA was extracted from the pellet using the All Prep Qiagen Kit following the manufacturer’s instructions: bacteria were first chemically lysed in phenol/chloroform and Qiagen lysis buffer containing β-mercaptoethanol, and mechanical lysis was carried out using a FastPrep (MP Biomedicals, Illkirch, France) for 40 s at a frequency of 5.5 m/s. RNA were then purified by loading on RNeasy® spin column, followed by washing and elution. Total RNAs were quantified using a Nanodrop 2000 spectrophotometer (Thermo Scientific, Illkirch, France) and quality was checked by ExperionTM Automated Electrophoresis System (Biorad, Marnes-la-Coquette, France). rRNA depletion was performed on 1 μg total RNA with the Ribo-Zero rRNA Removal Kit dedicated to bacteria (Illumina, San Diego, CA, United States). Purified mRNA quality was validated by capillary electrophoresis on a Fragment Analyzer (Advanced Analytical, Ankeny, IA, United States).
RNA-Seq libraries were constructed with the Truseq stranded mRNA sample preparation (low-throughput protocol) kit from Illumina. Purified mRNA (10 ng) were cleaved into small fragments using divalent cations under elevated temperature. The cleaved RNA fragments were converted into a first cDNA strand using SuperScript II reverse transcriptase (Invitrogen), actinomycin D, and random hexamer primers. A second cDNA strand was synthesized by replacing dTTP with dUTP. These cDNA fragments then have the addition of a single “A” base and subsequent ligation of the adapter. The products were then purified and enriched with 15 PCR cycles. The final cDNA libraries were validated with a Fragment Analyzer (Advanced Analytical, Ankeny, IA, United States) and quantified with a KAPA qPCR kit (Kapa Biosystems, Wilmington, MA, United States). On one sequencing lane of a flowcell V4, the 12 libraries (2 strains × 2 juices × 3 replicates) were pooled in equal proportions, denatured with NaOH, and diluted to 12 pM before clustering.
Cluster formation, primer hybridization and single-end read, and 50-cycle sequencing were performed on cBot and HiSeq2500 (Illumina, San Diego, CA, United States), respectively. Image analysis and base calling were performed using the HiSeq Control Software and Real-Time Analysis component. Demultiplexing was performed using Illumina’s sequencing analysis software. The quality of the data was assessed using FastQC from the Babraham Institute and the Illumina software SAV (Sequence Analysis Viewer).
FastqQ Screen (Wingett and Andrews, 2018) has been used to search sequences from commonly sequenced genomes in the facility that produced the data, and potential contamination sources: Homo sapiens, Mus musculus, Drosophila melanogaster, Arabidopsis thaliana, Bos taurus, Gallus gallus, Caenorhabditis elegans, Saccharomyces cerevisiae, Candida albicans, Aspergillus niger, Escherichia coli, Streptococcus pyogenes, Bacillus cereus, Chlamydia trachomatis, Enterococcus faecalis, Propionibacterium acnes, Staphylococcus aureus, a set of Mycoplasma, and a set of rRNA from different species. The PhiX genome (used as illumina control sequences) and illumina adapters (TruSeq and Nextera) have also been searched.
Transcriptomic high-throughput sequencing data were analyzed using a bioinformatic pipeline implemented in the Microscope platform (Médigue et al., 2017). The current pipeline is a “Master” shell script that launches the various parts of the analysis (i.e., a collection of Shell/Perl/R scripts) and controls for all tasks having been completed without errors. In a first step, the RNA-Seq data quality was assessed by including options like read trimming or merging/split paired-end reads. In a second step, the reads of each sample were mapped against the corresponding B. thermosphacta genome sequences: CD337 complete genome sequence ERZ500814 and TAP175 draft genome sequence ERZ500815 (Illikoud et al., 2018b), using the SSAHA2 package (Ning et al., 2001). This latter combines the SSAHA searching algorithm (sequence information is encoded in a perfect hash function), aiming at identifying regions of high similarity, and the cross-match sequence alignment program (Ewing et al., 1998), which aligns these regions using a banded Smith–Waterman–Gotoh algorithm (Smith and Waterman, 1981). An alignment score equal to at least half of the read is required for a hit to be retained. To reduce the false-positive discovery rate, the SAMtools (v.0.1.8) (Li et al., 2009) was then used to extract reliable alignments from SAM formatted files. The number of reads matching each genomic object harbored by the reference genome was subsequently computed with the Bioconductor-GenomicFeatures package (Carlson et al., 2011). If reads matched several genomic objects, the count number was weighted in order to keep the same total number of reads. Finally, the Bioconductor-DESeq package (Anders and Huber, 2010) with default parameters was used to analyze raw count data and test for differential expression between conditions.
The data have been submitted to the Sequence Read Archive database, with BioProject accession number PRJNA546512.
Growth of B. thermosphacta CD 337 and TAP 175 in beef and shrimp juices was monitored during storage at 8°C for 7 days (Figure 1). Total aerobic mesophilic and B. thermosphacta counts were similar (data not shown). The absence of bacteria detection (<1 CFU/ml) in the non-inoculated control juices throughout the storage confirmed that both juices were sterile before inoculation (data not shown). As observed in Figure 1, the two strains grew in both juices, reaching stationary phase after 2–4 days of storage. In shrimp juice, B. thermosphacta population increased rapidly during the first 2 days and reached a final cell density of 7.8–8.0 log CFU/ml after 4 days. In meat juice, the stationary phase was reached at day 4 but bacterial population did not exceed 7.5 log CFU/ml for both strains. Initial growth rate of B. thermosphacta TAP 175 was slightly higher than that of strain CD 337 in both media.
Figure 1. Growth kinetics of Brochothrix thermosphacta CD 337 and TAP 175 in meat and shrimp juices monitored in STAA-specific medium.
The initial pH values were 6.8 and 5.8 in shrimp and meat juices, respectively (Figure 2). The pH of control samples was constant along storage, whereas it decreased in inoculated juices. No significant difference was observed between strains. The pH of shrimp juice decreased to 5.2–5.4 after 4 days of storage, whereas that of meat juice decreased to 5.4–5.6 at day 4. Then, pH remained stable or slightly increased between day 4 and day 7.
Figure 2. Evolution of pH during storage at 8°C of meat and shrimp juices inoculated by B. thermosphacta strains CD 337 and TAP 175.
Seven compounds could be detected and identified (Table 1), among which 2-ethyl-1-hexanol present at similar levels in both control and inoculated meat juice and therefore considered as issued from beef meat and independent of bacterial metabolism. VOC production varied depending on the matrix. In meat juice, only ethanol and acetoin were detected after inoculation of B. thermosphacta, whereas six compounds (ethanol, isobutyraldehyde, 2-methylbutyraldehyde, 3-methylbutyraldehyde, acetoin, and 3-methyl-1-butanol) were produced in inoculated shrimp juices. Slight differences between the two strains were observed, mostly in the production kinetics. For example, 3-methyl-1-butanol was detected at days 4 and 7 in shrimp juice inoculated with CD 337 while it was detected only at day 7 in the juice inoculated by TAP 175 (Table 1).
RNAs were extracted at day 4, i.e., approximately when strains reached the stationary phase and produced various VOCs. Cells were thus considered metabolically active.
A total of 248,189,013 reads were generated using HiSeq 2500 single-end (Illumina, San Diego, CA, United States). For each transcriptome, more than 98% of the generated reads had a PHRED quality score higher than 30, meaning that the reads were of high quality (i.e., base call accuracy higher than 99.9%). Summary of data is given in Supplementary Table S1. We searched for genes whose expression [log2 fold change (LFC2) > 2; adjusted p-value (FDR) < 0.05] was increased during growth in meat compared to shrimp juice. We focused first on the genes differentially expressed by both strains and then on those whose expression varied in only one of the two strains. We performed similarly for genes that were specifically upregulated in shrimp compared to beef juice. This enabled us to discriminate the strain effect from the matrix effect on the differential expression of genes. Functions and metabolic pathways putatively inducted during growth in meat or shrimp juice were then deduced from the manually cured annotated genomes of both strains (Illikoud et al., 2018b).
Among the genes upregulated in meat juice (N = 97 in CD 337 and N = 161 in TAP 175), 63 were common to both strains. Out of those, eight encoded proteins of unknown function; nine were annotated as encoding putative enzymes, transporters, or regulators of unknown specificity; and two were related to ribosomal proteins or RNAs. The remaining 44 genes could be correlated to functions that are associated to growth on meat substrate (Supplementary Table S2).
For example, 9 of the 11-gene cluster iolRABCDETGHIJ involved in myo-inositol utilization were upregulated by both strains. Nevertheless, the iolAB genes were upregulated in CD 337 only with LFC2 values of 2.01 and 3.62, respectively, whereas in TAP 175, these values were below the used cutoff (1.42 and 1.96, respectively), suggesting less efficient myo-inositol utilization in this strain. The iol gene cluster encodes the transcriptional regulator IolR, the transporter IolT, and the enzymes responsible for catabolism of inositol as a carbon source. This compound is present not only in foods of vegetable origin (Clements and Darnell, 1980), but also in meat (Lilyblade and Peterson, 1962) from which it was first purified (Hoffmann-Ostenhof and Pittner, 1982). Several microorganisms can use myo-inositol as carbon source. These include Salmonella, Serratia, Klebsiella, and Bacillus subtilis (Legakis et al., 1976; Yoshida et al., 1997; Kröger and Fuchs, 2009). In B. subtilis, IolR acts as a transcriptional repressor in the absence of inositol. Myo-inositol acts as an inducer antagonizing IolR binding, enabling then the expression of the iol genes (Yoshida et al., 1999, 2002). The same regulation might thus also exist in B. thermosphacta. First, myo-inositol is taken into the cell by IolT and then metabolized by IolGEDBCJ enzymes to malonic semialdehyde and dihydroxyacetone phosphate (Yoshida et al., 2004; Figure 3). Malonic semialdehyde could then be metabolized into acetyl-CoA by IolA. Further metabolism of dihydroxyacetone phosphate is unclear from the transcriptome results. Indeed, its conversion into glyceraldehyde-3-phosphate by the triose phosphate isomerase (TpiA) might be impaired since tpiA was downregulated in meat juice.
Figure 3. Predicted pathway of myo-inositol catabolism by B. thermosphacta strains CD 337 and TAP 175 in meat juice. LFC values were represented with a color code depending on strain: CD 337 (green); TAP 175 (blue).
Part of the eut gene cluster involved in ethanolamine utilization was also upregulated in meat juice by both strains (Figure 4). Ethanolamine is a major constituent of lipids of eukaryotic cells thus present in beef meat. It is also present in fish but at lower levels (Fogerty et al., 1991). Several bacteria can use ethanolamine as a unique source of carbon and/or nitrogen, but the gene content, organization, and regulation of the eut operon are highly variable between bacterial species (Kaval and Garsin, 2018). The B. thermosphacta eut gene cluster [eutP-pduO-eutGSVWABCLKEMD-(euthyp)-NHQ] was similar to that of Enterococcus faecalis, i.e., encompassed eutV and eutW genes encoding a two-component regulatory system, and gene order was similar, except for the presence of an euthyp gene observed in B. thermosphacta and in other bacterial species, but absent from that of E. faecalis. In meat, eutCLKEMDNHQ were upregulated in CD337, whereas eutB LFC2 was below the used threshold (1.34), and only eutMDNHQ were upregulated in TAP175. Nevertheless, eutK was also considered as upregulated in TAP175 with an LFC2 value of 1.97 just below the threshold used. Those of eutL and eutC were, respectively, 1.41 and 1.36. Intriguingly, eutA was not expressed and eutG was downregulated. It seems, however, that B. thermosphacta could indeed use ethanolamine in meat as shown in Figure 4 since all the necessary elements were upregulated. EutKMNL proteins form a micro compartment encasing the enzymes necessary for ethanolamine catabolism (Sagermann et al., 2009; Takenoya et al., 2010). Ethanolamine, after transport by EutH, is converted into acetaldehyde and ammonia by EutBC (the two subunits of cobalamin-dependent ethanolamine ammonia lyase). EutA is necessary for reactivating EutBC unless adenosylcobalamin is present (Roof and Roth, 1989). Since adenosylcobalamin is known to be the major form of vitamin B12 present in beef meat (Czerwonka et al., 2014), one can hypothesize that the lack of EutA did not impair ethanolamine catabolism. Acetaldehyde is then converted to acetyl-CoA by EutE (acetaldehyde dehydrogenase) and acetyl-phosphate by a phosphotransacetylase EutD (Kaval and Garsin, 2018). Acetyl-phosphate can then be converted to acetate by EutQ (Kaval and Garsin, 2018). Acetaldehyde can also be converted to ethanol by EutG (an alcohol dehydrogenase) (Del Papa and Perego, 2008; Pitts et al., 2012). However, since eutG was downregulated in meat juice, this suggests that B. thermosphacta catabolized ethanolamine into acetate rather than ethanol.
Figure 4. Proposed pathway of ethanolamine utilization by B. thermosphacta strains CD 337 and TAP 175 in meat juice. LFC values were represented with a color code depending on strain: CD 337 (green); TAP 175 (blue). The gene encoding EutG was downregulated in meat juice. EutKLMN form a micro compartment encasing the enzymes necessary for ethanolamine catabolism.
A gene cluster encompassing ascRsnaAtcyJKLM involved in sulfur compound utilization and a putative FMN reductase related with sulfur starvation were also upregulated in meat juice. AscR is a transcriptional regulator and snaA is encoding an N-acetyltransferase acting on sulfur. tcyKJLM are involved in cysteine uptake. tcyK LFC2 values were 2.11 in CD337 and 1.83 in TAP175, suggesting that all the system was upregulated in both strains. In B. subtilis, the expression of snaA was reported to be strongly increased in the presence of glutathione (Coppée et al., 2001) a compound present at higher concentrations in beef meat than in raw fish (Rose et al., 1999). This most probably explains the upregulation of this set of genes to cope with sulfur starvation and/or presence of glutathione in beef juice.
The five genes of the pot operon (potRABCD) involved in spermidine/putrescine uptake were upregulated in meat juice. Seiler (1999) reported that these polyamines and their intermediates are found in a wide range of organisms including bacteria, plants, and animals. They are essential for bacterial growth. Several studies indicate that polyamine transporters are highly conserved in many Gram-positive and Gram-negative bacteria such as Escherichia coli, Salmonella enterica, and S. aureus (Igarashi and Kashiwagi, 1999). These systems were described to be involved in adaptation and/or survival of microorganisms and in biofilm formation (Wortham et al., 2007; Shah and Swiatlo, 2008; Shah et al., 2011). In bacteria, polyamine uptake from the environment may provide resistance to various environmental stresses including temperature change, osmotic pressure, reactive oxygen species, or other toxic compounds (Gevrekci, 2017). This suggests that these polyamines may be transported by B. thermosphacta and contribute to its survival in meat. However, as in S. aureus, the preferred substrates of the Pot system were spermidine and spermine, but not putrescine (Yao and Lu, 2014), we cannot ensure which substrate is transported by the PotABCD transporter in B. thermosphacta.
A pyruvate uptake transporter encoded by pftAB genes was also upregulated in meat. Pyruvate has been reported to be one of the substrates used by spoilage bacteria in meat. It is a precursor of various spoilage molecules such as acetoin, diacetyl, and acetic acid (Nychas et al., 2007; Casaburi et al., 2015).
Part of the pur operon (purBCSQLF) involved in purine biosynthesis was upregulated in both strains. purM and purN were also upregulated in TAP175, whereas their LFC2 values in CD337 were below the threshold (1.80 and 1.42, respectively). The purine biosynthetic pathway is conserved in bacteria (Kappock et al., 2000; Zhang et al., 2008). Inosine monophosphate (IMP) is the first nucleotide formed from purine biosynthesis. Then, IMP may be converted into adenosine monophosphate (AMP) and/or guanosine monophosphate (GMP) (Figure 5A). In CD 337 and TAP175, respectively, purHD and purD were not found among the differentially expressed genes. One can hypothesize that since these two genes are at the 3′-end of the operon (Figure 5B), their RNAs might be degraded and thus not appearing as upregulated in our experiments. The genes xapB-pupG and pyrRP encoding permeases for xanthine and uracil and their corresponding phosphoribosyltransferase converting them into xanthine monophosphate (XMP) and uridine monophosphate (UMP) were also upregulated in the two strains. A gene encoding a putative glutamine amidotransferase potentially converting XMP into GMP was specifically upregulated in TAP175 (LFC2 = 2.87), whereas its LFC2 value in CD337 was 1.93. Moreover, three other genes encoding putative guanine, hypoxanthine, or xanthine permeases were differently upregulated in the two strains: LFC2 values of pbuG, pbuX, and pbuO-like genes were 3.33 vs. 1.92, 2.24 vs. 1.33, and 1.78 vs. 2 in TAP175 and CD337, respectively. This suggests that B. thermosphacta purine and pyrimidine metabolism in meat may result from both uptake from the matrix and de novo synthesis and that the two strains cope differently with nucleobase transport and synthesis by activating different systems.
Figure 5. Proposed pathway of purine biosynthesis in meat juice by B. thermosphacta strains CD 337 and TAP 175 (A), and pur operon organization (B). LFC values were represented with a color code depending on strain: CD 337 (green); TAP 175 (blue). Abbreviations: N-DE, not differentially expressed; PRPP, 5-phosphoribosylpyrophosphate; GAR, glycinamide ribonucleotide; FGAR, formylglycinamide ribonucleotide; FGAM, formylglycinamidine ribonucleotide; AIR, aminoimidazole ribonucleotide; CAIR, carboxyaminoimidazole ribonucleotide; SAICAR, N-succinocarboxamide-5-aminoimidazole ribonucleotide; AICAR, aminoimidazole-4-carboxamide ribonucleotide; FAICAR, 5-formamido-4-imidazolecarboxamide ribonucleotide; IMP, inosine monophosphate.
Finally, a five-gene cluster encoding the three subunits of a putative Fe3+ (ferric ion) transport system, a downstream two-component regulatory system, and on the opposite orientation a putative Na+/H+ antiporter were also upregulated by both B. thermosphacta strains in meat juice. Ferric iron form is available in raw meat (Carpenter and Clark, 1995). Intracellular iron is used in biological reactions such as oxygen transport, gene regulation, DNA biosynthesis, and reparation (Andrews et al., 2003). Moreover, B. thermosphacta has been reported to require iron for growth under aerobic conditions (Thomson and Collins-Thompson, 1986).
In addition to the abovementioned genes, several were upregulated after growth in meat juice in only one of the two strains. Among those, 25 were specifically upregulated in CD337, with 6 being of unknown function and absent from the TAP175 chromosome (Supplementary Table S2). Thirteen were of unknown or uncertain function but the six remaining composed the tryptophan biosynthesis operon (trpABFCGDE), indicating a different metabolism between the two strains, although not reliable to meat spoilage.
In TAP 175, in addition to some described above, 95 genes were upregulated after growth in meat only in this strain, with a majority (N = 51) of unknown function. Twelve were associated to transport (N = 8) or regulatory (N = 4) functions with unknown specificity, and 13 were associated to RNA metabolism (including 4 tRNA genes and 5 miscellaneous RNA, and 4 to DNA). A gene encoding a flavoprotein annotated as putative phosphopantothenoylcysteine decarboxylase, which was absent in CD337, and a gene encoding a putative acetyl transferase were upregulated in TAP175 but could not be related to any functional metabolic pathway.
Nevertheless, several genes specifically upregulated in TAP175 could be informative about functions or conditions associated to growth in meat. Among those, three genes encoding a putative alkaline-shock protein, a cold-shock protein (cspC), and a free methionine sulfoxide reductase (msrC), all involved in stress response, were upregulated in TAP175, whereas in CD337, cspC was not expressed and the upregulation of the other was below the threshold (LFC2 being 1.21 and 1.28). A PTS-dependent transporter, specific for beta-glucosides, was upregulated in TAP175 (LFC2 = 1.83 in CD337).
The acpA gene encoding an acyl carrier protein involved in fatty acid biosynthesis was upregulated in TAP175 (LFC2 = 2.24 vs. 1.79 in CD337). Nevertheless, although located downstream from a cluster of genes involved in lipid synthesis as in B. subtilis (Martinez et al., 2010), only acpA was upregulated. Two type I signal peptidases (SipA and SipS) similar to the SipS protein of B. subtilis responsible for the processing of precursors of secreted protein (Bolhuis et al., 1996) were also upregulated only in TAP175 although their homologs exist in the CD337 genome. Also, lspA encoding the type II signal peptidase for lipoprotein processing (Tjalsma et al., 1999) was upregulated. yrbF encoding the auxiliary subunit of the preprotein translocase was upregulated. In Lactobacillus buchneri, this protein has been reported as upregulated as a response to ethanol (Liu et al., 2016). A gene encoding a putative CDP-glycerol glycerophosphotransferase described as involved in teichoic acid (poly-glycerol) biosynthesis was specifically upregulated by TAP175. It should be noticed that among the 51 proteins of unknown function whose genes were upregulated in TAP175, 14 were annotated as membrane associated or exported. Therefore, one can hypothesize that, when grown in meat, this strain may specifically produce proteins either secreted or associated to the cell surface. Indeed, the importance of cell surface composition for interactions of B. thermosphacta with the different environments it can grow in has been suspected (Stanborough et al., 2017, 2018; Illikoud et al., 2018b).
Among the 74 genes upregulated in both strains after growth in shrimp juice compared to meat juice (Supplementary Table S2), 11 coded for proteins of unknown function, 8 coded for putative enzymes, and 1 coded for a transporter (vlmR), all of unknown specificity. Five were tRNA genes and four encoded miscellaneous RNA including the 6S RNA. This may play a role later in transcriptional regulation. Indeed, in E. coli for example, 6S RNA has been reported as accumulating during stationary phase (Cavanagh et al., 2012), downregulating transcription, and important for modulating stress and survival during nutrient limitation (Cavanagh and Wassarman, 2014). This correlates with the sampling time of the present study for RNA extraction (at day 4), when bacterial growth ceased in shrimp juice.
Two putative transporters ComEA and ComF involved in DNA uptake were upregulated (CD 337 LFC2 = 2.34 and 2.01, respectively; TAP 175 LFC2 = 3.35 and 2.51, respectively). In Thermus thermophilus, the comEA operon is upregulated by nutrient limitation and low temperature (Salzer et al., 2016). In addition, a putative comGC gene was upregulated in TAP175, whereas it was not expressed in CD337. ComGC is a pilin that can be involved in various cell surface functions such as DNA transport, biofilm formation, or adhesion (Muschiol et al., 2017).
Interestingly, the two genes copA and copZ encoding a copper exporter system were also upregulated in shrimp juice (CD 337 LFC2 = 4.32 and 2.57, respectively; TAP 175 LFC2 = 6.24 and 3.27, respectively). Copper is present in crustaceans as these organisms contain the respiratory protein hemocyanin, a hexamer with two Cu2+ ions per subunit (Marxen et al., 2013). Copper content ranges from ∼0.1 to ∼1 mg/kg in meat (Cabrera et al., 2010) but is higher in shrimp, with an average value of ∼20 mg/kg and the lowest concentration of ∼4 mg/kg (Olmedo et al., 2013; Adams et al., 2015). Copper is essential for bacterial growth since it is a required cofactor for a number of enzymes with oxidase or oxygenase activities, detoxification of oxygen-derived free radicals, and electron transfer (Frausto da Silva and Williams, 2001). However, at high concentrations, it can be toxic due to its interaction with proteins, enzymes, nucleic acids, and metabolites (Trevors and Cotter, 1990). In B. subtilis, CopZ contributes to the copper sequestration and interacts with CopA for copper export (Radford et al., 2003). Although the copper content and availability in our experimental juices have not been measured, one can hypothesize that an excess of copper in shrimp juice, compared to meat juice, most probably induced its export out of the cells.
Five genes encoding proteins related to stress response or chaperoning activity were upregulated in both strains: GroEL, HrcA, GrpE, DnaK, and a Gls24 homolog. clpB was also upregulated in both strains although the gene was split into two fragments in CD337. In CD337, groES was also upregulated whereas its LFC2 was only 1.41 in TAP175. Conversely, ClpC and DnaJ were upregulated only in TAP175 (LFC2 = 2.41 and 2.54, vs. 1.79 and 1.96, respectively, in CD337). ClpB, a stress-induced multi-chaperone system with DnaK, DnaJ, and GrpE, has been described to be involved in the processing of protein aggregates and/or damaged proteins in B. subtilis (Mogk et al., 1997) and Listeria monocytogenes (Liu et al., 2002). This system has been also found in lactic acid bacteria (Papadimitriou et al., 2016). It seems thus that the machinery for refolding injured proteins is upregulated in B. thermosphacta in shrimp juice.
A large proportion of genes upregulated after growth in shrimp juice encode proteins involved in various sugar transport and/or utilization. These encompassed a response common to both strains but also genes whose response was strain specific. Among genes whose upregulation was shared by both strains, 10 were involved in transport/phosphorylation of carbon sources, in particular several phosphoenolpyruvate (PEP)-dependent systems (PTS): levDEFG encoding a complete mannose/fructose-specific enzyme IIABCD, bglP encoding a complete enzyme IIABC specific for beta-glucosides, two genes encoding the two subunits of BglH, a phospho-beta glucosidase, mtlRA coding for a PTS-dependent utilization of mannitol/glucitol, and mdxK encoding a maltose phosphorylase. nagAB encoding N-acetylglucosamine-6-phosphate deacetylase (EC 3.5.1.25) and N-acetylglucosamine-6-phosphate isomerase (EC 3.5.99.6) for N-acetylglucosamine degradation were both upregulated in CD337, while in TAP175, only nagA was upregulated. Two adjacent genes encoding a putative oligo-1,4-1,6-alpha-glucosidase (similar to the sucrase-maltase-isomaltase of B. subtilis) and MdxR, the transcriptional activator of the maltodextrin operon, and two others, located elsewhere in the chromosome and belonging to an mdx gene cluster, encoding a bifunctional beta-phosphoglucomutase/glucose-1-phosphate phosphodismutase (mdxM) and a putative IIBC component maltose PTS system, were specific of the TAP175 response. In addition, the gene encoding mannitol-1-phosphate dehydrogenase (mtlD) was upregulated in TAP175 only.
The regulatory pattern of the gene cluster encoding glycolytic enzymes (cggR-gapA-pgk-tpiA-pgm-eno) was strain dependent. Triose phosphate isomerase (TpiA, EC 5.3.1.1) and phosphoglycerate mutase (Pgm, EC 5.4.2.12) genes were upregulated in both strains whereas those encoding phosphoglycerate kinase (Pgk, EC 2.7.2.3) and enolase (Eno, EC 4.2.1.11) were upregulated in TAP175 only (LFC2 = 2.24 and 2.05 in TAP175 vs. 1.78 and 1.84 in CD337) and cggR only in CD337 (LFC2 = 2.11 in CD337 vs. 1.60 in TAP175). Moreover, a putative glycerate 2-kinase (GarK, EC 2.7.1.165) and a phosphoglycerate mutase were upregulated in both strains. Belonging to TAP175-specific response were a glucokinase encoding gene and ppdK encoding pyruvate phosphate dikinase. This enzyme catalyzes the reversible conversion of PEP + AMP + Pi into pyruvate + ATP (Lim et al., 2007). Lactate metabolism was upregulated in TAP175, with lutC, lctE, and ldhL. LutC is a protein involved in lactate utilization and the B. subtilis lutABC operon was reported as upregulated in stationary phase (İrigül-Sönmez et al., 2014). However, in B. thermosphacta, TAP175 lutC was the only gene of the operon to be upregulated. Two of the three paralogs encoding lactate dehydrogenase (EC 1.1.1.27), i.e., ldhL and lctE, were upregulated in TAP175. This enzyme catalyzes the reaction pyruvate <=> lactate and can lead, in association with alsSD, to the production of acetoin (Cruz Ramos et al., 2000). Interestingly, alsSD encoding acetolactate synthase (EC 2.2.1.6) and acetolactate decarboxylase (EC 4.1.1.5), respectively, which convert pyruvate to acetoin, were specifically upregulated in TAP175 with LFC2 values of 2.42 and 2.29 (whereas these values were only 1.24 and 1.38 in CD337). This correlates with previous results showing that TAP175 produced more acetoin than CD337 after growth in shrimp juice (Illikoud et al., 2019). However, in the present study, acetoin was not detected in the TAP175 VOC analysis from shrimp juice.
The upregulation of the abovementioned genes suggests an active carbon catabolism in shrimp juice and the utilization of a variety of sugars notably through the PTS system. However, carbon metabolism might be differentially efficient in the two strains. Indeed, as mentioned above, the central glycolytic gene repressor (cggR) was upregulated only in CD337. In B. subtilis, the regulatory function of CggR is dependent on fructose-1,6-bisphosphate (Zorrilla et al., 2007). Interestingly, hprK was also upregulated in CD337 (in TAP175 LFC2 was 1.88). It encodes the HPr kinase, which plays a central role in carbon catabolite repression. This enzyme possesses fructose-1,6-bisphosphate as an allosteric activator and is responsible for ATP-dependent phosphorylation of HPr, which itself regulates expression of catabolic genes and is required, when not phosphorylated by HprK, for the transport of PTS sugars (Jault et al., 2000). While yvgN encoding a methylglyoxal reductase was upregulated in both strains, yhfP, a gene coding for a putative NADP-dependent quinone oxidoreductase of unknown specificity, was upregulated in TAP175, and a putative hydroquinone-type dioxygenase/glyoxalase encoding gene (mhqA) was specifically upregulated in CD337. In B. subtilis, MhqA and YvgN have been proposed to be involved in the detoxification of methyl-glyoxal, a by-product of glycolysis (Töwe et al., 2007; Lei et al., 2009).
Amino acid metabolism was also modified depending on the growth substrate. The two genes pdxT and pdxS, encoding the two subunits of glutamine amidotransferase, were upregulated in shrimp juice. These are involved in pyridoxal 5′-phosphate biosynthesis and glutamine degradation. Eight genes of the gene cluster hisIFAHBDGZ involved in histidine biosynthesis were upregulated. However, hisC located at the 3′-end of the gene cluster and therefore putatively degraded was not upregulated. gabD encoding a putative succinate semialdehyde dehydrogenase that could be involved in amino acid or polyamine metabolism was upregulated in both strains. However, genes encoding a putative cystathionine beta-lyase/cysteine desulfhydrase and a D-alanine aminotransferase (dat) were specific of TAP175 response. The former enzyme (EC 4.4.1.8) produces L-homocysteine, an unstable compound that can lead to pyruvate production; the second (EC 2.6.1.21) catalyzes the reaction D-alanine + 2-oxoglutarate <=> pyruvate + D-glutamate. In addition, an aminopeptidase (PapA) and a tripeptidase (PepT) putatively capable of cleaving di- and tripeptides and the lysine transporter LysP were upregulated in TAP175.
Other genes encoding various functions were also upregulated by both strains but could not be associated to the functioning of whole pathways. Among those, pyrKDFE out of the pyrRPBC(AA) (AB)KDFE cluster were upregulated, meaning the synthesis of UMP from dihydro-orotate putatively induced in shrimp juice. Also, out of the argCJBD cluster involved in ornithine biosynthesis, only two genes (argBD) in CD337 and three (argJBD) in TAP175 were upregulated. thiM and thiD involved in thiamin metabolism were upregulated, but only in TAP175.
Concerning the strain-specific response to growth in shrimp juice, 19 genes encoding proteins of unknown function were upregulated in CD337 only, among which 6 were absent from the TAP175 genome sequence. Two miscellaneous RNAs were specifically upregulated in CD337. Three adjacent genes encoding a putative transposase, a putative recombinase resolvase, and one of the abovementioned protein of unknown function were specific of the CD337 response. Those belonged to a 24,132-bp genomic island specific of CD337 and putatively involved in cell surface or adhesion properties (Illikoud et al., 2018b).
A gene encoding a putative thioredoxin/glutaredoxin-like, which may act as an electron donor depending on the intracellular redox state (Petersohn et al., 1999), was upregulated in CD337. The genes encoding ribosome hibernation promoting factor hpf involved in the formation of inactive ribosomes during the stationary phase (Sato et al., 2009) and hbs, which may have an effect on gene expression through DNA binding (Klein and Marahiel, 2002), were upregulated in CD337 only. This may indicate a possible difference in the metabolic status of the two strains after 4 days of growth in shrimp juice. Finally, two genes encoding a putative immunity protein and a putative cation efflux protein were also specific of CD337 response but could not be linked to a specific metabolism or growth condition.
Conversely, except some genes discussed above, 45 were upregulated by TAP 175 only, among which 18 were of unknown function (with 8 absent or fragmented in the CD337 genome), 6 corresponded to RNAs (2 tRNAs, 4 misc RNAs), 1 corresponded to a putative prophage protein, and 5 corresponded to enzymes of unknown specificity.
Genes involved in general cellular machinery as translation, energy production, or DNA metabolism were upregulated specifically in TAP175. These encompassed genes encoding phenylalanyl-tRNA synthetase (pheT), D-Tyr-tRNATyr deacylase (dtd), translation initiation factor IF-2 (infB) and atpF, atpA, atpG, and atpD belonging to the atpBEFHAGDC operon encoding the F0F1-type ATP synthase. Five adjacent genes, i.e., polA encoding DNA polymerase I; mutM encoding formamidopyrimidine-DNA glycosylase, which prevents DNA damage by reactive oxygen species (Gómez-Marroquín et al., 2015); coaE encoding dephosphocoenzyme A kinase (E.C. 2.7.1.24), which catalyzes the final step of coenzyme A (CoA) synthesis; and two putative helicases similar to DnaB and DnaI involved in chromosome replication initiation, were upregulated only in TAP175. In addition, upregulation of uvrA, mutL, and addA involved in DNA damage recognition and DNA mismatch repair during chromosome replication (Cromie, 2009; Guarné and Charbonnier, 2015; Gómez-Marroquín et al., 2016) was observed only in TAP175. gpsA involved in phospholipid synthesis as responsible for sn-glycerol 3-phosphate synthesis (Morbidoni et al., 1995) was upregulated only in TAP175.
The catalase encoding gene katA was also upregulated only in TAP175. This enzyme has an antioxidant function by degrading hydrogen peroxide. Three CDS corresponding to a fragmented trxB gene were upregulated in TAP175. The fact that the three gene fragments were upregulated suggests sequencing errors (TAP175 genome sequence is a draft). trxB encodes thioredoxin reductase, which acts with thioredoxin (encoded by trxA) as an oxidoreductase system with antioxidant and redox regulatory functions. However, trxA was not expressed. As this gene is very short (318 nt), we cannot exclude that its expression could not be detected.
Finally, three putative regulators were specifically upregulated in TAP175: a putative stress-mediated DNA-binding transcriptional regulator whose gene is located immediately upstream from hprK in TAP175, but separated from it by a gene encoding a membrane protein of unknown function in CD337; yclK, a two-component sensor histidine kinase, whose gene is regulated by oxygen limitation in B. subtilis (Härtig et al., 2004); and a putative anti-sigma factor located downstream from a gene similar to B. subtilis genes encoding extracytoplasmic function (ECF) RNA polymerase sigma factors involved in the response to various stress or environmental conditions.
This study provided insights into the relative difference in gene expression and VOC production by B. thermosphacta, during growth in meat or shrimp juice. We observed that B. thermosphacta metabolism was different on the two juices tested. The catabolism of meat or shrimp specific substrates may lead to different metabolisms depending on the food matrix. This may suggest that in the B. thermosphacta spoilage process, the biochemical composition of the food matrix drives the VOC production. The type of carbon source used by B. thermosphacta in laboratory medium has been reported to influence the production of spoilage molecules (Dainty and Hibbard, 1980). As an example, the production of acetoin was increased in the presence of glycerol, compared to ribose. Similarly, in our study, the utilization of different carbon sources, suggested by the expression of different sugar transporters in meat or shrimp juice, may affect the production of carbon-derived VOCs. Moreover, B. thermosphacta strains produced six detectable VOCs in shrimp juice, while only two were detected in meat juice (ethanol and acetoin). This suggests that B. thermosphacta alone most probably exerts a moderate effect on meat spoilage, since the human odor threshold is much lower for diacetyl than for acetoin (Casaburi et al., 2015). Nevertheless, as B. thermosphacta has been associated with spoilage of natural meat products in several previous studies, one can hypothesize that it may need interactions with other bacterial species within the meat microbiota, to increase its involvement in the spoilage process of meat. Conversely, in shrimp juice, B. thermosphacta alone can produce various molecules associated with the spoilage. However, this does not exclude that interaction of this species with other microbiota members could also intensify its spoilage potential of seafood products, as it has been previously described on cooked peeled shrimps, where B. thermosphacta co-inoculated with Carnobacterium maltaromaticum produced a typical wet dog off-flavor, whereas neither species produce this off-flavor when inoculated separately (Laursen et al., 2006).
To our knowledge, this study is the first transcriptomic analysis performed to elucidate the genetic functions involved in the food spoilage process by B. thermosphacta. Figure 6 summarizes the main functions that could be specifically induced during growth in meat or shrimp. Two carbon sources (ethanolamine and myo-inositol) could be preferentially used in beef, whereas a larger panel of sugars could be transported, mainly through PTS systems, from shrimp juice. Iron uptake in meat and copper excretion in shrimp seemed to be induced. Amino acids, purine, and pyrimidine metabolisms appeared to vary depending on the food matrix but also on the strains. Physiological status also seemed different in meat and in shrimp (where stress response was upregulated). However, correlating the upregulated metabolic pathways to the production of molecules reported as associated to meat or shrimp spoilage was uneasy. This may result from the fact that transcriptome was analyzed at day 4 only. Further studies monitoring the dynamics of the transcriptomes during and after growth may provide more information.
Figure 6. Main B. thermosphacta functions potentially induced during growth in meat or shrimp juice. Transport of molecules specifically present in meat or in shrimp for which transporter encoding genes are differentially expressed depending on the substrate is shown. Intracellular metabolic pathways specifically induced in shrimp or in beef juice are indicated as well as volatile organic compounds that were detected.
The datasets generated for this study can be found in the Sequence Read Archive database, BioProject accession number PRJNA546512.
NI, DW, EJ, and MZ contributed to conception and design. NI, RG, CB, and DR acquired the data. NI, DW, DR, and MZ analyzed the data. NI and MZ drafted the manuscript for important intellectual content. All authors reviewed and revised the manuscript.
This work was funded by the “Région des Pays de la Loire” (Grant to MZ). NI was the recipient of a Ph.D. fellowship from the French Ministry of Higher Education and Research.
RG and DW were employed by the company Aérial.
The remaining authors declare that the research was conducted in the absence of any commercial or financial relationships that could be construed as a potential conflict of interest.
The LABGeM (CEA/IG/Genoscope and CNRS UMR8030) and the France Génomique National Infrastructure (funded as part of Investissements d’Avenir Program managed by Agence Nationale de la Recherche, contract ANR-10-INBS-09) were acknowledged for support within the MicroScope annotation platform. The French National Research Agency (ANR) was acknowledged for the financial support in the framework of the REDLOSSES project (ANR-16-CE21-0006) that contributed to the setup of the experimental procedure of volatilome analysis.
The Supplementary Material for this article can be found online at: https://www.frontiersin.org/articles/10.3389/fmicb.2019.02527/full#supplementary-material
TABLE S1 | Summary of RNA-seq data.
TABLE S2 | Genes differentially expressed by B. thermosphacta CD337 and TAP175 when grown in beef or shrimp juice. Genes upregulated in beef compared to shrimp are detailed in the 1st sheet, those upregulated in shrimp juice compared to beef juice are detailed in the 2nd sheet. Genes upregulated by both strains are shown as well as genes specifically regulated in CD 337 or in TAP 175. Gene label, gene name and EC number of the encoded protein when available, are issued from genome annotation. The CD 337 complete genome was automatically annotated then manually cured (Illikoud et al., 2018b). For TAP 175 draft genome, cured annotations of CD 337 genome were transferred on the orthologous genes. For TAP 175 specific genes, initial automatic annotations were kept. Log Fold Change >2 are indicated. A color code indicates the level of upregulation.
Adams, W. J., DeForest, D. K., Tear, L. M., Payne, K., and Brix, K. V. (2015). Long-term monitoring of arsenic, copper, selenium, and other elements in Great Salt Lake (Utah, USA) surface water, brine shrimp, and brine flies. Environ. Monit. Assess. 187:118. doi: 10.1007/s10661-014-4231-6
Anders, S., and Huber, W. (2010). Differential expression analysis for sequence count data. Genome Biol. 11:R106. doi: 10.1186/gb-2010-11-10-r106
Andrews, S. C., Robinson, A. K., and Rodríguez-Quiñones, F. (2003). Bacterial iron homeostasis. FEMS Microbiol. Rev. 27, 215–237. doi: 10.1016/S0168-6445(03)00055-X
Bolhuis, A., Sorokin, A., Azevedo, V., Ehrlich, S. D., Braun, P. G., De Jong, A., et al. (1996). Bacillus subtilis can modulate its capacity and specificity for protein secretion through temporally controlled expression of the sipS gene for signal peptidase I. Mol. Microbiol. 22, 605–618. doi: 10.1046/j.1365-2958.1996.d01-4676.x
Cabrera, M. C., Ramos, A., Saadoun, A., and Brito, G. (2010). Selenium, copper, zinc, iron and manganese content of seven meat cuts from Hereford and Braford steers fed pasture in Uruguay. Meat Sci. 84, 518–528. doi: 10.1016/j.meatsci.2009.10.007
Carlson, M., Pagès, H., Aboyoun, P., Falcon, S., Morgan, M., Sarkar, D., et al. (2011). Genomic Features: Tools for Making and Manipulating Transcript Centric Annotations. R Package Version 1.4.3.
Carpenter, C. E., and Clark, E. (1995). Evaluation of methods used in meat iron analysis and iron content of raw and cooked meats. J. Agric. Food Chem. 43, 1824–1827. doi: 10.1021/jf00055a014
Casaburi, A., De Filippis, F., Villani, F., and Ercolini, D. (2014). Activities of strains of Brochothrix thermosphacta in vitro and in meat. Food Res. Int. 62, 366–374. doi: 10.1016/j.foodres.2014.03.019
Casaburi, A., Piombino, P., Nychas, G.-J., Villani, F., and Ercolini, D. (2015). Bacterial populations and the volatilome associated to meat spoilage. Food Microbiol. 45, 83–102. doi: 10.1016/j.fm.2014.02.002
Cavanagh, A. T., Sperger, J. M., and Wassarman, K. M. (2012). Regulation of 6S RNA by pRNA synthesis is required for efficient recovery from stationary phase in E. coli and B. subtilis. Nucleic Acids Res. 40, 2234–2246. doi: 10.1093/nar/gkr1003
Cavanagh, A. T., and Wassarman, K. M. (2014). 6S RNA, a global regulator of transcription in Escherichia coli, Bacillus subtilis, and beyond. Ann. Rev. Microbiol. 68, 45–60. doi: 10.1146/annurev-micro-092611-150135
Chaillou, S., Chaulot-Talmon, A., Caekebeke, H., Cardinal, M., Christieans, S., Denis, C., et al. (2015). Origin and ecological selection of core and food-specific bacterial communities associated with meat and seafood spoilage. ISME J. 9, 1105–1118. doi: 10.1038/ismej.2014.202
Clements, J. R. S., and Darnell, B. (1980). Myo-inositol content of common foods: development of a high-myo-inositol diet. Am. J. Clin. Nutr. 33, 1954–1967. doi: 10.1093/ajcn/33.9.1954
Coppée, J.-Y., Auger, S., Turlin, E., Sekowska, A., Le Caer, J.-P., Labas, V., et al. (2001). Sulfur-limitation-regulated proteins in Bacillus subtilis: a two-dimensional gel electrophoresis study. Microbiology 147, 1631–1640. doi: 10.1099/00221287-147-6-1631
Cromie, G. A. (2009). Phylogenetic ubiquity and shuffling of the bacterial RecBCD and AddAB recombination complexes. J. Bacteriol. 191, 5076–5084. doi: 10.1128/JB.00254-09
Cruz Ramos, H., Hoffmann, T., Marino, M., Nedjari, H., Presecan-Siedel, E., Dreesen, O., et al. (2000). Fermentative metabolism of Bacillus subtilis: physiology and regulation of gene expression. J. Bacteriol. 182, 3072–3080. doi: 10.1128/JB.182.11.3072-3080.2000
Czerwonka, M., Szterk, A., and Waszkiewicz-Robak, B. (2014). Vitamin B12 content in raw and cooked beef. Meat Sci. 96, 1371–1375. doi: 10.1016/j.meatsci.2013.11.022
Dainty, R. H., Edwards, R. A., Hibbard, C. M., and Marnewick, J. J. (1989). Volatile compounds associated with microbial growth on normal and high pH beef stored at chill temperatures. J. Appl. Bacteriol. 66, 281–289. doi: 10.1111/j.1365-2672.1989.tb02480.x
Dainty, R. H., and Hibbard, C. M. (1980). Aerobic metabolism of Brochothrix thermosphacta growing on meat surfaces and in laboratory media. J. Appl. Bacteriol. 48, 387–396. doi: 10.1111/j.1365-2672.1980.tb01027.x
Del Papa, M. F., and Perego, M. (2008). Ethanolamine activates a sensor histidine kinase regulating its utilization in Enterococcus faecalis. J. Bacteriol. 190, 7147–7156. doi: 10.1128/JB.00952-08
Ewing, B., Hillier, L., Wendl, M. C., and Green, P. (1998). Base-calling of automated sequencer traces using phred. I. Accuracy assessment. Genome Res. 8, 175–185. doi: 10.1101/gr.8.3.175
Fogerty, A. C., Whitfield, F. B., Svoronos, D., and Ford, G. L. (1991). The composition of the fatty acids and aldehydes of the ethanolamine and choline phospholipids of various meats. Int. J. Food Sci. Technol. 26, 363–371. doi: 10.1111/j.1365-2621.1991.tb01978.x
Frausto da Silva, J. J. R., and Williams, R. J. P. (2001). The Biological Chemistry of the Elements: The Inorganic Chemistry of Life, 2nd Edn. Oxford: Clarenden Press.
Gevrekci, A. Ö (2017). The roles of polyamines in microorganisms. World J. Microbiol. Biotechnol. 33:204. doi: 10.1007/s11274-017-2370-y
Gómez-Marroquín, M., Martin, H. A., Pepper, A., Girard, M. E., Kidman, A. A., Vallin, C., et al. (2016). Stationary-phase mutagenesis in stressed Bacillus subtilis cells operates by Mfd-dependent mutagenic pathways. Genes 7:33. doi: 10.3390/genes7070033
Gómez-Marroquín, M., Vidales, L. E., Debora, B. N., Santos-Escobar, F., Obregón-Herrera, A., Robleto, E. A., et al. (2015). Role of Bacillus subtilis DNA glycosylase MutM in counteracting oxidatively induced DNA damage and in stationary-phase-associated mutagenesis. J. Bacteriol. 197, 1963–1971. doi: 10.1128/JB.00147-15
Guarné, A., and Charbonnier, J.-B. (2015). Insights from a decade of biophysical studies on MutL: roles in strand discrimination and mismatch removal. Prog. Biophys. Mol. Biol. 117, 149–156. doi: 10.1016/j.pbiomolbio.2015.02.002
Härtig, E., Geng, H., Hartmann, A., Hubacek, A., Münch, R., Ye, R. W., et al. (2004). Bacillus subtilis ResD induces expression of the potential regulatory genes yclJK upon oxygen limitation. J. Bacteriol. 186, 6477–6484. doi: 10.1128/JB.186.19.6477-6484.2004
Hoffmann-Ostenhof, O., and Pittner, F. (1982). The biosynthesis of myo-inositol and its isomers. Can. J. Chem. 60, 1863–1871. doi: 10.1139/v82-259
Igarashi, K., and Kashiwagi, K. (1999). Polyamine transport in bacteria and yeast. Biochem. J. 344, 633–642. doi: 10.1042/bj3440633
Illikoud, N., Jaffrès, E., and Zagorec, M. (2018a). “Brochothrix thermosphacta,” in Reference Module in Life Sciences, ed. B. D. Roitberg, (Amsterdam: Elsevier), 588–603. doi: 10.1016/B978-0-12-809633-8.12106-5
Illikoud, N., Klopp, C., Roulet, A., Bouchez, O., Marsaud, N., Jaffrès, E., et al. (2018b). One complete and three draft genome sequences of four Brochothrix thermosphacta strains, CD 337, TAP 175, BSAS1 3 and EBP 3070. Stand. Genomic Sci. 13:22. doi: 10.1186/s40793-018-0333-z
Illikoud, N., Rossero, A., Chauvet, R., Courcoux, P., Pilet, M.-F., Charrier, T., et al. (2019). Genotypic and phenotypic characterization of the food spoilage bacterium Brochothrix thermosphacta. Food Microbiol. 81, 22–31. doi: 10.1016/j.fm.2018.01.015
İrigül-Sönmez, Ö, Köroğlu, T. E., Öztürk, B., Kovács, ÁT., Kuipers, O. P., and Yazgan-Karataş, A. (2014). In Bacillus subtilis LutR is part of the global complex regulatory network governing the adaptation to the transition from exponential growth to stationary phase. Microbiology 160, 243–260. doi: 10.1099/mic.0.064675-0
Jaffrès, E., Lalanne, V., Macé, S., Cornet, J., Cardinal, M., Sérot, T., et al. (2011). Sensory characteristics of spoilage and volatile compounds associated with bacteria isolated from cooked and peeled tropical shrimps using SPME–GC–MS analysis. Int. J. Food Microbiol. 147, 195–202. doi: 10.1016/j.ijfoodmicro.2011.04.008
Jault, J.-M., Fieulaine, S., Nessler, S., Gonzalo, P., Di Pietro, A., Deutscher, J., et al. (2000). The HPr kinase from Bacillus subtilis is a homo-oligomeric enzyme which exhibits strong positive cooperativity for nucleotide and fructose 1,6-bisphosphate binding. J. Biol. Chem. 275, 1773–1780. doi: 10.1074/jbc.275.3.1773
Kappock, T. J., Ealick, S. E., and Stubbe, J. (2000). Modular evolution of the purine biosynthetic pathway. Curr. Opin. Chem. Biol. 4, 567–572. doi: 10.1016/S1367-5931(00)00133-2
Kaval, K. G., and Garsin, D. A. (2018). Ethanolamine utilization in bacteria. mBio 9:e66-18. doi: 10.1128/mBio.00066-18
Klein, W., and Marahiel, M. A. (2002). Structure-function relationship and regulation of two Bacillus subtilis DNA-binding proteins, HBsu and AbrB. J. Mol. Microbiol. Biotechnol. 4, 323–329.
Kröger, C., and Fuchs, T. M. (2009). Characterization of the myo-inositol utilization island of Salmonella enterica serovar Typhimurium. J. Bacteriol. 191, 545–554. doi: 10.1128/JB.01253-08
Laursen, B. G., Leisner, J. J., and Dalgaard, P. (2006). Carnobacterium species: effect of metabolic activity and interaction with Brochothrix thermosphacta on sensory characteristics of modified atmosphere packed shrimp. J. Agric. Food Chem. 54, 3604–3611. doi: 10.1021/jf053017f
Legakis, N. J., Papavassiliou, J. T., and Xilinas, M. E. (1976). Inositol as a selective substrate for the growth of Klebsiellae and Serratiae. Zentralbl Bakteriol Orig A. 235, 453–458.
Lei, J., Zhou, Y. F., Li, L. F., and Su, X. D. (2009). Structural and biochemical analyses of YvgN and YtbE from Bacillus subtilis. Protein Sci. 18, 1792–1800. doi: 10.1002/pro.178
Li, H., Handsaker, B., Wysoker, A., Fennell, T., Ruan, J., Homer, N., et al. (2009). The sequence alignment/map format and SAMtools. Bioinformatics 25, 2078–2079. doi: 10.1093/bioinformatics/btp352
Lilyblade, A. L., and Peterson, D. W. (1962). Inositol and free sugars in chicken muscle post-mortem. J. Food Sci. 27, 245–249. doi: 10.1111/j.1365-2621.1962.tb00088.x
Lim, K., Read, R. J., Chen, C. C. H., Tempczyk, A., Wei, M., Ye, D., et al. (2007). Swiveling domain mechanism in pyruvate phosphate dikinase. Biochemistry 46, 14845–14853. doi: 10.1021/bi701848w
Liu, S., Graham, J. E., Bigelow, L., Morse, P. D., and Wilkinson, B. J. (2002). Identification of Listeria monocytogenes genes expressed in response to growth at low temperature. Appl. Environ. Microbiol. 68, 1697–1705. doi: 10.1128/AEM.68.4.1697-1705.2002
Liu, S., Skory, C., Qureshi, N., and Hughes, S. (2016). The yajC gene from Lactobacillus buchneri and Escherichia coli and its role in ethanol tolerance. J. Ind. Microbiol. Biotechnol. 43, 441–450. doi: 10.1007/s10295-015-1730-6
Martinez, M. A., de Mendoza, D., and Schujman, G. E. (2010). Transcriptional and functional characterization of the gene encoding acyl carrier protein in Bacillus subtilis. Microbiology 156, 484–495. doi: 10.1099/mic.0.033316-0
Marxen, J. C., Pick, C., Kwiatkowski, M., and Burmester, T. (2013). Molecular characterization and evolution of haemocyanin from the two freshwater shrimps Caridina multidentata (Stimpson, 1860) and Atyopsis moluccensis (De Haan, 1849). J. Comp. Physiol. B 183, 613–624. doi: 10.1007/s00360-013-0740-9
Médigue, C., Calteau, A., Cruveiller, S., Gachet, M., Gautreau, G., Josso, A., et al. (2017). MicroScope—an integrated resource for community expertise of gene functions and comparative analysis of microbial genomic and metabolic data. Brief. Bioinform. 20, 1071–1084. doi: 10.1093/bib/bbx113
Mogk, A., Homuth, G., Scholz, C., Kim, L., Schmid, F. X., and Schumann, W. (1997). The GroE chaperonin machine is a major modulator of the CIRCE heat shock regulon of Bacillus subtilis. EMBO J. 16, 4579–4590. doi: 10.1093/emboj/16.15.4579
Morbidoni, H. R., de Mendoza, D., and Cronan, J. E. Jr. (1995). Synthesis of sn-glycerol 3-phosphate, a key precursor of membrane lipids, in Bacillus subtilis. J. Bacteriol. 177, 5899–5905. doi: 10.1128/jb.177.20.5899-5905.1995
Muschiol, S., Erlendsson, S., Aschtgen, M. S., Oliveira, V., Schmieder, P., de Lichtenberg, C., et al. (2017). Structure of the competence pilus major pilin ComGC in Streptococcus pneumoniae. J. Biol. Chem. 92, 14134–14146. doi: 10.1074/jbc.M117.787671
Ning, Z., Cox, A. J., and Mullikin, J. C. (2001). SSAHA: a fast search method for large DNA databases. Genome Res. 11, 1725–1729. doi: 10.1101/gr.194201
Nychas, G.-J. E., Marshall, D. L., and Sofos, J. N. (2007). “Meat, poultry, and seafood,” in Food Microbiology: Fundamentals and Frontiers, 3rd Edn, eds M. P. Doyle, L. R. Beuchat, and T. J. Montville, (Washington, DC: ASM Press), 105–140. doi: 10.1128/9781555815912.ch6
Odeyemi, O. A., Burke, C. M., Bolch, C. C. J., and Stanley, R. (2018). Seafood spoilage microbiota and associated volatile organic compounds at different storage temperatures and packaging conditions. Int. J. Food Microbiol. 280, 87–99. doi: 10.1016/j.ijfoodmicro.2017.12.029
Olmedo, P., Hernández, A. F., Pla, A., Femia, P., Navas-Acien, A., and Gil, F. (2013). Determination of essential elements (copper, manganese, selenium and zinc) in fish and shellfish samples. Risk and nutritional assessment and mercury–selenium balance. Food Chem. Toxicol. 62, 299–307. doi: 10.1016/j.fct.2013.08.076
Papadimitriou, K., Alegría, Á., Bron, P. A., de Angelis, M., Gobbetti, M., Kleerebezem, M., et al. (2016). Stress physiology of lactic acid bacteria. Microbiol. Mol. Biol. Rev. 80, 837–890. doi: 10.1128/MMBR.00076-15
Petersohn, A., Bernhardt, J., Gerth, U., Höper, D., Koburger, T., Völker, U., et al. (1999). Identification of (-dependent genes in Bacillus subtilis using a promoter consensus-directed search and oligonucleotide hybridization. J. Bacteriol. 181, 5718–5724.
Pitts, A. C., Tuck, L. R., Faulds-Pain, A., Lewis, R. J., and Marles-Wright, J. (2012). Structural insight into the Clostridium difficile ethanolamine utilisation microcompartment. PLoS One 7:e48360. doi: 10.1371/journal.pone.0048360
Radford, D. S., Kihlken, M. A., Borrelly, G. P. M., Harwood, C. R., Brun, N. E., and Cavet, J. S. (2003). CopZ from Bacillus subtilis interacts in vivo with a copper exporting CPx-type ATPase CopA. FEMS Microbiol. Lett. 220, 105–112. doi: 10.1016/S0378-1097(03)00095-8
Remenant, B., Jaffrès, E., Dousset, X., Pilet, M.-F., and Zagorec, M. (2015). Bacterial spoilers of food: behavior, fitness and functional properties. Food Microbiol. 45, 45–53. doi: 10.1016/j.fm.2014.03.009
Roof, D. M., and Roth, J. R. (1989). Functions required for vitamin B12-dependent ethanolamine utilization in Salmonella typhimurium. J. Bacteriol. 171, 3316–3323. doi: 10.1128/jb.171.6.3316-3323
Rose, N. L., Sporns, P., Stiles, M. E., and McMullen, L. M. (1999). Inactivation of nisin by glutathione in fresh meat. J. Food Sci. 64, 759–762. doi: 10.1111/j.1365-2621.1999.tb15906.x
Sagermann, M., Ohtaki, A., and Nikolakakis, K. (2009). Crystal structure of the EutL shell protein of the ethanolamine ammonia lyase microcompartment. Proc. Natl. Acad. Sci. U.S.A. 106, 8883–8887. doi: 10.1073/pnas.0902324106
Salzer, R., Kern, T., Joos, F., and Averhoff, B. (2016). The Thermus thermophilus comEA/comEC operon is associated with DNA binding and regulation of the DNA translocator and type IV pili. Environ. Microbiol. 18, 65–74. doi: 10.1111/1462-2920.12820
Sato, A., Watanabe, T., Maki, Y., Ueta, M., Yoshida, H., Ito, Y., et al. (2009). Solution structure of the E. coli ribosome hibernation promoting factor HPF: Implications for the relationship between structure and function. Biochem. Biophys. Res. Com. 389, 580–585. doi: 10.1016/j.bbrc.2009.09.022
Seiler, N. (1999). “A Guide to the Polyamines,” in The Quarterly Review of Biology, Vol. 74, ed. S. S. Cohen, (Chicago, IL: The University of Chicago Press), 342–343. doi: 10.1086/393192
Shah, P., Nanduri, B., Swiatlo, E., Ma, Y., and Pendarvis, K. (2011). Polyamine biosynthesis and transport mechanisms are crucial for fitness and pathogenesis of Streptococcus pneumoniae. Microbiology 157, 504–515. doi: 10.1099/mic.0.042564-0
Shah, P., and Swiatlo, E. (2008). A multifaceted role for polyamines in bacterial pathogens. Mol. Microbiol. 68, 4–16. doi: 10.1111/j.1365-2958.2008.06126.x
Smith, T. F., and Waterman, M. S. (1981). Identification of common molecular subsequences. J. Mol. Biol. 147, 195–197. doi: 10.1016/0022-2836(81)90087-5
Stackebrandt, E., and Jones, D. (2006). “The genus Brochothrix,” in The prokaryotes, 3rd Edn, eds M. Dworkin, S. Falkow, E. Rosenberg, K.-H. Schleifer, and E. Stackebrandt, (New York, NY: Springer), 477–491. doi: 10.1007/0-387-30744-3_12
Stanborough, T., Fegan, N., Powell, S. M., Tamplin, M., and Chandry, P. S. (2017). Insight into the genome of Brochothrix thermosphacta, a problematic meat spoilage bacterium. Appl. Environ. Microbiol. 83:e2786-16. doi: 10.1128/aem.02786-16
Stanborough, T., Suryadinata, R., Fegan, N., Powell, S. M., Tamplin, M., Nuttall, S. D., et al. (2018). Characterisation of the Brochothrix thermosphacta sortase A enzyme. FEMS Microbiol. Lett. 365:fny184. doi: 10.1093/femsle/fny184
Takenoya, M., Nikolakakis, K., and Sagermann, M. (2010). Crystallographic insights into the pore structures and mechanisms of the EutL and EutM shell proteins of the ethanolamine-utilizing microcompartment of Escherichia coli. J. Bacteriol. 192, 6056–6063. doi: 10.1128/JB.00652-10
Thomson, I. Q., and Collins-Thompson, D. L. (1986). Iron requirement of Brochothrix thermosphacta. Int. J. Food Microbiol. 3, 299–309. doi: 10.1016/0168-1605(86)90006-1
Tjalsma, H., Kontinen, V. P., Prágai, Z., Wu, H., Meima, R., Venema, G., et al. (1999). The role of lipoprotein processing by signal peptidase II in the Gram-positive eubacterium Bacillus subtilis. J. Biol. Chem. 274, 1698–1707. doi: 10.1074/jbc.274.3.1698
Töwe, S., Leelakriangsak, M., Kobayashi, K., Van Duy, N., Hecker, M., Zuber, P., et al. (2007). The MarR-type repressor MhqR (YkvE) regulates multiple dioxygenases/glyoxalases and an azoreductase which confer resistance to 2-methylhydroquinone and catechol in Bacillus subtilis. Mol. Microbiol. 66, 40–54. doi: 10.1111/j.1365-2958.2007.05891.x
Trevors, J., and Cotter, C. (1990). Copper toxicity and uptake in microorganisms. J. Ind. Microbiol. 6, 77–84. doi: 10.1007/BF01576426
Wingett, S. W., and Andrews, S. (2018). FastQ screen: a tool for multi-genome mapping and quality control [version 2; referees: 4 approved]. F1000Research 77:1338. doi: 10.12688/f1000research.15931.2
Wortham, B. W., Oliveira, M. A., and Patel, C. N. (2007). “Polyamines in bacteria: Pleiotropic effects yet specific mechanisms,” in The Genus Yersinia: Advances In Experimental Medicine And Biology, Vol. 603, eds R. D. Perry, and J. D. Fetherston, (New York, NY: Springer), 106–115. doi: 10.1007/978-0-387-72124-8_9
Yao, X., and Lu, C.-D. (2014). Functional characterization of the potRABCD operon for spermine and spermidine uptake and regulation in Staphylococcus aureus. Curr. Microbiol. 69, 75–81. doi: 10.1007/s00284-014-0556-1
Yoshida, K. I., Aoyama, D., Ishio, I., Shibayama, T., and Fujita, Y. (1997). Organization and transcription of the myo-inositol operon, iol, of Bacillus subtilis. J. Bacteriol. 179, 4591–4598. doi: 10.1128/jb.179.14.4591-4598.1997
Yoshida, K.-I., Shibayama, T., Aoyama, D., and Fujita, Y. (1999). Interaction of a repressor and its binding sites for regulation of the Bacillus subtilis iol divergon. J. Mol. Biol. 285, 917–929. doi: 10.1006/jmbi.1998.2398
Yoshida, K.-I., Yamaguchi, M., Ikeda, H., Omae, K., Tsurusaki, K.-I., and Fujita, Y. (2004). The fifth gene of the iol operon of Bacillus subtilis, iolE, encodes 2-keto-myo-inositol dehydratase. Microbiology 150, 571–580. doi: 10.1099/mic.0.26768-0
Yoshida, K.-I., Yamamoto, Y., Omae, K., Yamamoto, M., and Fujita, Y. (2002). Identification of two myo-inositol transporter genes of Bacillus subtilis. J. Bacteriol. 184, 983–991. doi: 10.1128/jb.184.4.983-991.2002
Zhang, Y., Morar, M., and Ealick, S. E. (2008). Structural biology of the purine biosynthetic pathway. Cell. Mol. Life Sci. 65, 3699–3724. doi: 10.1007/s00018-008-8295-8
Keywords: shrimp, meat, transcriptome, volatile organic compounds, diacetyl, acetoin, food spoilage, Brochothrix thermosphacta
Citation: Illikoud N, Gohier R, Werner D, Barrachina C, Roche D, Jaffrès E and Zagorec M (2019) Transcriptome and Volatilome Analysis During Growth of Brochothrix thermosphacta in Food: Role of Food Substrate and Strain Specificity for the Expression of Spoilage Functions. Front. Microbiol. 10:2527. doi: 10.3389/fmicb.2019.02527
Received: 09 August 2019; Accepted: 21 October 2019;
Published: 08 November 2019.
Edited by:
Michael Gänzle, University of Alberta, CanadaReviewed by:
Jorgen Johannes Leisner, University of Copenhagen, DenmarkCopyright © 2019 Illikoud, Gohier, Werner, Barrachina, Roche, Jaffrès and Zagorec. This is an open-access article distributed under the terms of the Creative Commons Attribution License (CC BY). The use, distribution or reproduction in other forums is permitted, provided the original author(s) and the copyright owner(s) are credited and that the original publication in this journal is cited, in accordance with accepted academic practice. No use, distribution or reproduction is permitted which does not comply with these terms.
*Correspondence: Monique Zagorec, bW9uaXF1ZS56YWdvcmVjQG9uaXJpcy1uYW50ZXMuZnI=
Disclaimer: All claims expressed in this article are solely those of the authors and do not necessarily represent those of their affiliated organizations, or those of the publisher, the editors and the reviewers. Any product that may be evaluated in this article or claim that may be made by its manufacturer is not guaranteed or endorsed by the publisher.
Research integrity at Frontiers
Learn more about the work of our research integrity team to safeguard the quality of each article we publish.