- 1Institute for Animal Hygiene and Environmental Health, Freie Universität Berlin, Berlin, Germany
- 2Institute for Veterinary Epidemiology and Biostatistics, Freie Universität Berlin, Berlin, Germany
- 3Institute of Pharmacy, Pharmaceutical Biology, Universität Greifswald, Greifswald, Germany
Extended-spectrum beta-lactamase- (ESBL-) and AmpC beta-lactamase- (AmpC-) producing Enterobacteriaceae pose a risk for both human and animal health. For livestock, highest prevalences have been reported in broiler chickens, which are therefore considered as a reservoir of multidrug-resistant bacteria. The possibility of transfer to humans either by a close contact to colonized broiler flocks or through contaminated retail meat results in the necessity to develop intervention measures for the entire broiler production chain. In this regard, a basic understanding of the colonization process is mandatory including the determination of the minimal bacterial load leading to a persistent colonization of broiler chickens. Therefore, we conducted a bivalent broiler colonization study close to real farming conditions without applying any antimicrobial selection pressure. ESBL- and AmpC- negative broiler chickens (Ross 308) were co- colonized on their third day of life with two strains: one CTX-M-15-producing Escherichia coli-ST410 and one CMY-2/mcr-1-positive E. coli-ST10. Colonization was assessed by cloacal swabs over the period of the trial, starting 24 h post inoculation. During the final necropsy, the contents of crop, jejunum, cecum, and colon were quantified for the occurrence of both bacterial strains. To define the minimal oral colonization dosage 104 to 101 colony forming units (cfu) were orally inoculated to four separately housed broiler groups (each n = 19, all animals inoculated) and a dosage of already 101 cfu E. coli led to a persistent colonization of all animals of the group after 3 days. To assure stable colonization, however, a dosage of 102 cfu E. coli was chosen for the subsequent seeder-bird trial. In the seeder-bird trial one fifth of the animals (seeder, n = 4) were orally inoculated and kept together with the non-inoculated animals (sentinel, n = 16) to mimic the route of natural infection. After 35 days of trial, all animals were colonized with both E. coli strains. Given the low colonization dosage and the low seeder/sentinel ratio, the rapid spread of ESBL- and AmpC- producing Enterobacteriaceae in conventional broiler farms currently seems inevitably resulting in an urgent need for the development of intervention strategies to reduce colonization of broilers during production.
Introduction
Antimicrobial resistance is an increasing problem in veterinary and human medicine and the spread of extended-spectrum beta-lactamase- (ESBL-) and AmpC beta-lactamase- (AmpC-) producing Enterobacteriaceae was demonstrated in wildlife (Guenther et al., 2011; Alcalá et al., 2016), livestock (Laube et al., 2013; Liebana et al., 2013; Hille et al., 2018), and companion animals (Rubin and Pitout, 2014; Schaufler et al., 2015; Kaspar et al., 2019) as well as in retail meat (Cohen Stuart et al., 2012; Kola et al., 2012) and out of human origin (Pitout, 2013; Valenza et al., 2014; Mazzariol et al., 2017).
Livestock animals, including broiler chickens, show high prevalences (EFSA, 2019) and are considered to be a reservoir of ESBL- and AmpC- producing enterobacteria (Costa et al., 2009; Reich et al., 2013). Recently, it was shown by Projahn et al. (2017) and Daehre et al. (2018b) that transmission of resistant bacteria can take place at a very early stage of the broiler production chain: pseudo-vertical from the parent flock to the eggs in the hatchery and horizontal through the contaminated farm environment 24 h after the placing of the broiler chickens. Genes encoding ESBLs and AmpCs are frequently located on mobile genetic elements such as plasmids (Carattoli, 2013). Commonly detected resistance genes include blaCTX−M−1, blaCTX−M−14, blaCTX−M−15, blaSHV−12, and blaCMY−2 in livestock and companion animals (Ewers et al., 2012). The question of a possible transfer of resistance from animals to humans remains controversial and constitutes a major concern of public health (Cortés et al., 2010; Leverstein-van Hall et al., 2011; Ewers et al., 2012; Dorado-García et al., 2018; Pietsch et al., 2018). Close contact of humans to colonized animals (Dierikx C. et al., 2013; Huijbers et al., 2014) and the consumption of contaminated meat (Vincent et al., 2010; Leverstein-van Hall et al., 2011) are considered to be especially important risk factors, and have been thoroughly investigated.
By contrast, to our knowledge, there have not been any studies investigating the minimal bacterial load that is necessary for a stable colonization of broiler chickens (Daehre et al., 2018b). Therefore, the aim of our study was to define the minimal colonization dosage of broiler chickens with ESBL- and AmpC- producing Escherichia coli (E. coli) without applying any antimicrobial selection pressure. In the second step, a seeder- bird colonization model that mimics real farming conditions was conducted for the investigation of the spread of ESBL- and AmpC- producing E. coli in flocks. These investigations paved the way for the follow-up studies that are currently being conducted at our institute and aim to examine potential intervention strategies regarding hygiene- and management measures to reduce the colonization of broiler chickens with ESBL- and AmpC- producing Enterobacteriaceae.
Materials and Methods
Ethics Statement
This study was carried out in accordance with the National Animal Protection Guidelines. The protocol was approved by the German Animal Ethics Committee for the protection of animals of the Regional Office for Health and Social Affairs Berlin (“Landesamt für Gesundheit und Soziales,” LAGeSo, registration number G 0193/16).
Housing Conditions
All trials were conducted at the experimental facilities of the Center for Infection Medicine of the Freie Universität Berlin, department of Veterinary Medicine. The animals were kept in controlled rooms with attached separate lockrooms for changing of clothes and shoes. Before starting the trial, rooms were cleaned and disinfected with hydrogen peroxide fumigation and the complete experimental setup was tested for the absence of ESBL-/AmpC- producing bacteria (see “ESBL-/AmpC- status prior to the trial”). An individual ventilation was achieved by using HEPA filter. The study was structured in two parts: a colonization dosage part followed by a seeder-bird colonization model.
For each trial, eggs of the breed Ross 308, received from a commercial hatchery in Germany, were hatched in-house for 21 days. The first disinfection of the eggs using formaldehyde gas was performed at the hatchery and the second disinfection with WESSOCLEAN® K 50 Gold Line containing 2.37% hydrogen peroxide and 0.015% peracetic acid (Wesso AG, Hersbruck, Germany) following the transportation to the experimental facilities before incubation in a separate hatcher. After hatch, broilers were conventionally housed in with a stocking density of 39 kg/m2, fresh litter once at the beginning of the trial, no enrichment and conventional feed and water ad libitum. The feeding regime included a starter feed and a grower feed with coccidiostats (decoquinate and narasin/nicarbazin). The finisher feed did not include any coccidiostats and was fed 5 days prior to necropsies. The feed did not contain any antimicrobial agents.
First, four colonization dosage trials were performed. Each of the four groups consisted of 19 chickens. Every group was kept separately in an experimental room for a period of 2 weeks in aviaries to define the minimal oral colonization dosage. Following these colonization dosage experiments, 20 chickens were kept in floor keeping for the seeder-bird model trial. For the seeder- bird trial, four chickens were orally inoculated (seeder birds) with the minimal oral colonization dosage determined previously and kept together with 16 non-inoculated animals (sentinel birds). During the seeder-bird trial, the chickens were kept up to a weight of two kilograms, corresponding to the duration of 5 weeks.
In the later course of the colonization dosage trial using 102 cfu/E. coli (day 11) and the seeder-bird trial (day 16) each time one animal died without any sign of infection or illness.
Bacterial Colonization Strains
Two avian E. coli strains were used for co-colonization of the birds. Both strains were originally isolated from healthy chickens during a previous research project on ESBL- producing E. coli in chickens in 2011 (Falgenhauer et al., 2016; Hering et al., 2016). Both strains were investigated using whole-genome analysis as described in Falgenhauer et al. (2016). One strain is an ESBL- producing E. coli {CTX-M-15 [chromosomally encoded (Arredondo-Alonso et al., 2018)]; multilocus sequence type ST410; phylogenetic group B1; internal number 10716; published as R56 in Falgenhauer et al. (2016)} with resistances to cephalosporins and enrofloxacin, and the other strain is an AmpC- producing E. coli {CMY-2 [plasmid encoded (Arredondo-Alonso et al., 2018)]; ST10; phylogenetic group A; internal number 10717}, resistant to cephalosporins and colistin, as shown in Table 1. In addition, phenotypic antimicrobial resistance analysis was performed by using VITEK 2 system (bioMérieux, Marcy-l'Étoile, France). These avian commensal E. coli strains were selected because of their resistance profile, representative for the situation in German chicken production and their ability to colonize broiler chickens digestive tract.
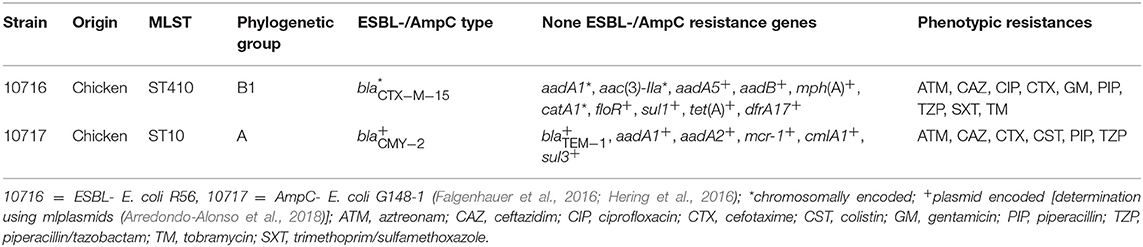
Table 1. Characteristics of E. coli strains used for colonization dosage trials (101−104 cfu E. coli) and seeder-bird colonization model.
Microorganisms were stored at −80°C in Luria Bertani broth (LB; Carl Roth, Karlsruhe, Germany) containing 20% glycerol (Carl Roth, Karlsruhe, Germany). For the preparation of the bacterial suspension both bacterial strains were streaked out on columbia agar containing 5% sheep blood (Oxoid, Wesel, Germany) and were incubated for 24 h at 37°C. Overnight cultures originating from a single colony from each strain were grown in 5 ml LB broth. On the following colonization day, 40–60 μl of these bacterial suspensions were seeded in 5 ml of fresh LB broth each until the desired optical density of 0.04 (OD600) was obtained. The bacterial cultures were grown at 37°C and 200 rounds per minute (rpm) to the optical density of 1.0 (OD600), corresponding to 1 × 108 colony forming units (cfu) per ml. One ml per culture was centrifuged at 7,000 rpm for 10 min at 4°C, the supernatant was removed and the cells were resuspended in 1 ml phosphate buffered saline (PBS; Phosphate Buffered Saline tablets, Oxoid, Wesel, Germany). The desired colonization dose was adjusted via a 10-fold dilution series in PBS. For co-colonization, both dilutions were mixed, placed on ice, and used for inoculation of broilers within 30 minutes. The concentration of the colonization dose was verified by direct plating of appropriate dilutions of the inoculum.
Oral Colonization of Broilers
On the third day of life, broilers were orally inoculated into the crop with 200 μl of bacterial suspension in PBS containing a mixture of both bacterial strains in equal parts. For the colonization dosage trials, all animals of the group were orally inoculated, starting with a colonization dosage of 104 cfu E. coli/200 μl followed by 103, 102, and 101 cfu E. coli/200 μl in the following experiments to verify the cfu that is necessary to colonize all animals within 24 h post inoculation (p.i.).
In contrast to the colonization dosage trials, not all animals of the seeder-bird model trial were orally inoculated. The inoculation was performed at the ratio of 1:5 (seeder: sentinel), resulting in four seeders, which are expected to colonize the 16 non-inoculated, susceptible broilers (sentinels) over the period of the trial. From the beginning of the trial, the seeder- and sentinel birds were housed in together. Similarly to the dosage trials, all inoculated animals should be colonized within 24 h p.i.
Samplings and Analyses
Samples were initially processed on chromogenic agar (CHROMagar Orientation, Mast Diagnostica, Reinfeld, Germany) for a rapid identification of E. coli colonies. Confirmation of single bacterial E. coli colonies with a typical morphology and of all untypical colony morphologies was performed using MALDI- TOF (MALDI Microflex LT® and Biotyper database® Bruker Daltonics, Bremen, Germany). Phenotypic antimicrobial resistance analysis of randomly picked colonies of the cecum samples obtained in necropsy was performed by using VITEK 2 system to confirm their identity as the inoculated ESBL- and AmpC- strains.
ESBL-/AmpC- Status Prior to the Trial
Before starting a trial, the complete experimental setup was tested for the absence of ESBL-/AmpC- producing bacteria after the introduction of the litter, feed, and water into the experimental room. Walls/doors, floor, table, heating lamps, feeding-, and drinking troughs were sampled with sterile and moistened gauze swabs. For moistening, 5 ml PBS were used. In addition, 5 g of litter, and feed were collected. Following the sampling, the gauze swabs, litter, and feed were transferred into 50 ml LB broth in each case and incubated for 24 h at 37°C before 10 μl were streaked out on chromogenic agar containing 2 μg/ml cefotaxime (AppliChem, Darmstadt, Germany) and incubated for 24 h at 37°C.
Directly after hatching, the absence of ESBL and AmpC- producing bacteria in the egg shells was confirmed. Five egg shells were crushed, transferred into 50 ml LB broth and incubated for 24 h at 37°C before 10 μl were streaked out on chromogenic agar containing 2 μg/ml cefotaxime and incubated for 24 h at 37°C.
The absence of ESBL and AmpC in the 1 day-old broiler chickens was confirmed by cloacal swabs of all individually tagged animals. Swabs were transferred into reaction tubes containing 500 μl PBS and were thoroughly vortexed. Fifty microliter were streaked out onto chromogenic agar containing 2 μg/ml cefotaxime and incubated for 24 h at 37°C.
Sampling During the Trial
Colonization of broilers was analyzed via cloacal swabs during the trial starting 24 h and 72 h p.i. and followed by samplings of each individual three times in the second week of a trial, followed by two samplings a week up to the end of a trial. Swabs were transferred into reaction tubes containing 500 μl PBS and were thoroughly vortexed. Fifty microliter were streaked out onto agar and incubated for 24 h at 37°C for semiquantitative analysis. A subjective measurement using eight categories from zero (“no growth”) to seven (“overgrown”) was applied for the evaluation of the colonization (Figure S1) and a set of four chromogenic agar plates was used. One plate without selective media for the total count of E. coli colonies (positive control). One plate containing 2 μg/ml cefotaxime and 4 μg/ml enrofloxacin (Sigma- Aldrich, Steinheim, Germany) for the growth of the ESBL- E. coli 10716 and another plate containing 2 μg/ml cefotaxime and 7 μg/ml colistin (Carl Roth, Karlsruhe, Germany) for the growth of the AmpC- E. coli 10717. A fourth plate contained all three antibiotics in the given concentrations (negative control).
Finalization of the Trial
Following each trial, a necropsy of all broilers was performed on day 14 in the colonization dosage trials and on day 35 in the seeder-bird model trial. Contents of crop, jejunum, cecum, colon as well as organ samples of liver and spleen were analyzed using the plate set described above.
The samples of crop, jejunum, cecum, and colon were quantified. First, up to one gram of the content was weighed out in a reaction tube and PBS was added at the ratio of 1:2, followed by a dilution series in PBS, depending on the expected bacterial growth. Finally, 100 μl each of the suspension were plated on the plate set, as described above. For quantification, a minimum of two different dilution levels were counted.
The organ samples of liver and spleen were qualitatively analyzed via direct processing. For this, the organs were cut in half with sterile scissors, pressed on the agar and spread out with an inoculation loop (Selbitz et al., 2015). All samples were incubated for 24 h at 37°C.
Statistical Analysis
Sample size calculation was based on the hypothesis that the logarithmic mean values in the colonized ceca were equal in each of the different colonization dosage groups (NCSS PASS 14.0). Ceca were selected because ceca are known to be the reservoir for ESBL- E. coli. Equivalence was defined as follows: the 95% confidence interval (CI) of the investigated group is within one log unit cfu/g cecal content compared to the mean value of the minimal colonization dosage group. Standard deviation of log 0.9 cfu/g cecal content was assumed. To ensure alpha error of 0.05 and power of 0.90, 19 animals per group were required. Twenty animals were used for the seeder-bird colonization model to have a comparable group size for the defined inoculation ratio of 1:5 (4 seeder birds: 16 sentinel birds).
Statistical analysis was performed by using Excel 2013 (Microsoft Corporation, Washington, USA) and SPSS Statistics 25 (IBM, New York, USA). To obtain normally distributed data, all bacteriological results were log10 transformed. CI of proportions were calculated using Clopper-Pearson method.
To compare the level of colonization in between the four colonization dosage groups, equivalence testing (Thrusfield, 2007) was performed. For this test, a margin of one log unit difference between the groups was defined acceptable to be equal. It was tested whether the margins of the 95% CI are within the range of one log unit.
Differences between different groups regarding the logarithmic bacteriological mean values of the samples were investigated using t-test for independent samples: first, the colonization levels between the colonization dosage group 102 cfu/E. coli and the seeder-bird colonization model were investigated separately for the locations crop, jejunum, cecum, and colon. Second, the results of the seeder- and sentinel birds of the seeder-bird model were compared. The probability level of 0.05 was used to denote significance.
Results
Colonization Dosage
By applying a series of colonization dosages ranging from 104, 103, and 102 to 101 cfu E. coli, we were able to demonstrate that a dosage of 102 cfu E. coli per animal led to an almost complete colonization of a broiler group within 24 h p.i (Figure S1). Even a dosage of 101 cfu E. coli was sufficient to colonize the majority of the animals within 24 h p.i. For the colonization dosage trials, all animals in a group were orally inoculated with both bacterial strains on their third day of life and the successful colonization was initially assessed via cloacal swabs 24 h p.i. For colonization dosages 104 and 103 cfu E. coli, all animals were colonized within 24 h p.i. up to the end of the trial. Continuing with a dosage of 102 cfu E. coli, only one animal was tested negative 24 h p.i. for one of the strains (AmpC- E. coli 10717, 95%CI: 73.97–99.87%). At the second sampling 72 h p.i., however, all animals inoculated with 102 cfu E. coli were colonized over the entire period of the trial. The dosage trials were completed with 101 cfu E. coli resulting in a colonization rate of 84% (ESBL- E. coli 10716, 95%CI: 60.42–96.62%) and 95% (AmpC- E. coli 10717, 95%CI: 73.97–99.87%) of the animals positive for the strains 24 h p.i. In this group, complete colonization was detectable within 72 h p.i. up to the end of the trial. Based on the results obtained from cloacal swabs during the trial, a minimal dosage of 102 cfu E. coli was necessary to colonize a broiler group in the given experimental setup within 24 h p.i.
The bacterial counts of the cecum samples obtained at the necropsies of all four trials (104 to 101 cfu E. coli) were of equal value in the equivalence test. In contrast to this, samples of crop, jejunum, and colon were non- equivalent (Figure 1). The mean colonization values of the digestive tract samples for both bacterial strains are summarized in Figure 2 and Table S1. Overall, maximum values were attained in cecum samples with mean values between 8.84 to 9.22 log10 cfu ESBL- E. coli/g cecal content and 8.32 to 8.69 log10 cfu AmpC- E. coli/g cecal content, except for the content of crop and jejunum in the dosage trial using 103 cfu E. coli. Compared to the cecum samples, lower mean values occurred in colon samples and lowest mean values were evident in the content of crop and jejunum (Table S1). Independent from the original colonization dosage, the results of cecum samples were equivalent with a deviation less than one log unit to the values of the colonization dosage of 102 cfu E. coli and every broiler was colonized with both bacterial strains 2 weeks p.i. For samples of crop, jejunum and colon, equivalence between dosages was not shown. The values of crop and jejunum samples differed in each trial. Highest values were given in the dosage trial using 103 cfu E. coli, lowest values occurred while using 104 cfu E. coli. In contrast to this, less variation was given in colon samples with mean values between 7.05 to 7.91 log10 cfu ESBL- E. coli/g colon content and 6.67 to 7.15 log10 cfu AmpC- E. coli/g colon content, but without showing equivalence.
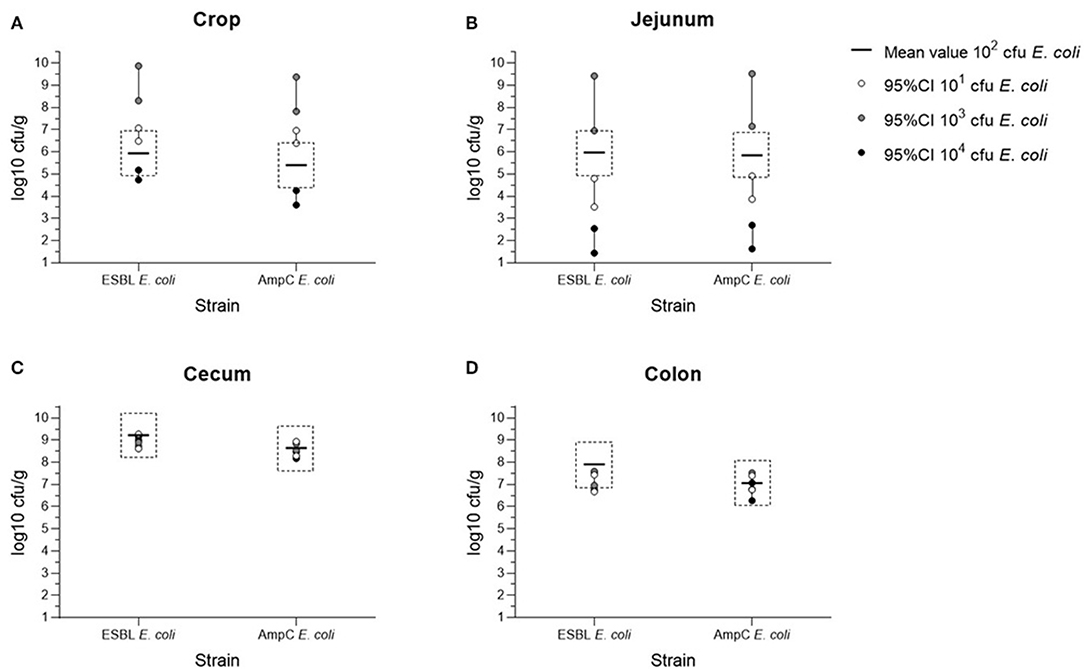
Figure 1. Equivalence testing of ESBL- E. coli 10716 and AmpC- E. coli 10717 in samples of crop (A), jejunum (B), cecum (C), and colon (D) in between the four colonization dosage groups (101-104 cfu/E. coli) at necropsy. 95%CI = 95% confidence interval; all data shown are log10 transformed (log10 cfu/g); margin of <1 log of 95%CI of dosage groups 101, 103, and 104 cfu/E. coli to the mean value of the minimal colonization dosage group (102 cfu/E. coli) shows equivalence.
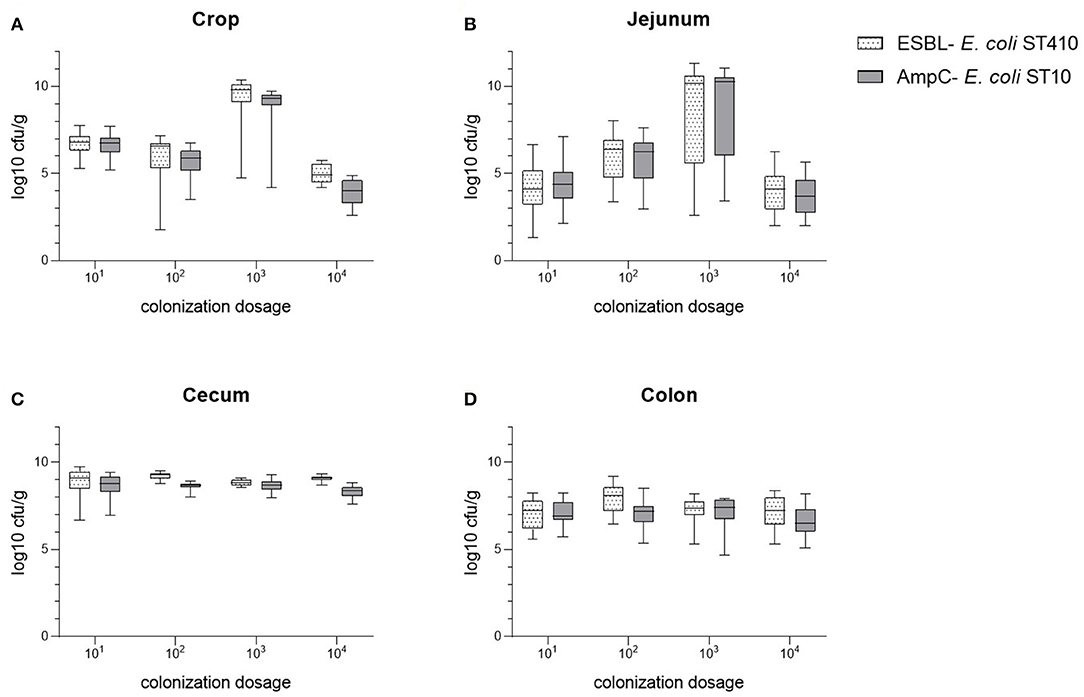
Figure 2. Comparison of the bacterial counts of ESBL- E. coli 10716 and AmpC- E. coli 10717 in samples of crop (A), jejunum (B), cecum (C), and colon (D) in between the four colonization dosage groups (101-104 cfu/E. coli) at necropsy.
In conclusion, the colonization of the cecum with both bacterial strains 2 weeks after inoculation with 102 cfu E. coli was equal to the colonization after inoculation with 104, 103, and 101 cfu E. coli. In contrast to these correlating results of cecum samples obtained at necropsy, we observed lower percentages of colonized animals 24 h after inoculation by using 101 cfu E. coli compared to the other tested dosages. Based on these results, a dosage of 102 cfu E.coli was chosen for the following seeder- bird colonization model trial balancing between a low dose, comparable to the situation in the course of natural infection and a colonization of the animals during the trial.
Seeder-Bird Colonization Model
A ratio of 1:5 orally inoculated (seeder) to non- inoculated broilers (sentinel) and a dosage of 102 cfu ESBL-/AmpC- E. coli were sufficient to colonize an entire broiler group within 72 h (Figure S1). The seeder birds were completely colonized 24 h p.i. with both bacterial strains. At this early time point 69% of the 16 sentinels were tested positive for the ESBL- E. coli strain 10716 (95%CI: 41.34–88.98%) and 50% positive for AmpC- E. coli 10717 (95%CI: 24.65–75.35%). Three days p.i., at the second sampling, all animals of the broiler group were colonized with both bacterial strains up to the end of the trial. As a result, an oral colonization of one fifth of a broiler group with 102 cfu E. coli on their third day of life resulted in colonization of the entire broiler group.
At necropsy, cecum colonization of all seeder- and sentinel birds was observed, with mean values of 6.69 log10 cfu ESBL- E. coli/g cecal content and 6.57 log10 cfu AmpC- E. coli/g cecal content (Table S1). Furthermore, no significant difference in bacterial counts between seeder birds and sentinel birds was evident at the end of the trial (t-test p-values > 0.05). Corresponding to the colonization dosage trials, the maximum values of E. coli were found in the cecum irrespective of being a seeder- or sentinel bird. Compared to the cecum samples, reduced values occurred in colon samples and lowest mean values were evident in crop and jejunum samples. Similarly, no association with the origin of the bird as a seeder- or sentinel was observed (t-test p-values > 0.05).
However, compared to the dosage trial using 102 cfu E. coli, the colonization in different parts of the digestive tract of the seeder-bird group was significantly lower (t-test p-values <0.001; Figure 3). In all necropsy samples taken, we observed lower total numbers of both bacterial strains 5 weeks p.i. in the seeder-bird model compared to the initial colonization dosage trial with a duration of 2 weeks. In summary, a comparable colonization of all seeder- and sentinel birds in a group was achieved by an oral colonization dosage of 102 cfu E. coli in combination with an oral inoculation of one fifth of the broiler chickens on their third day of life.
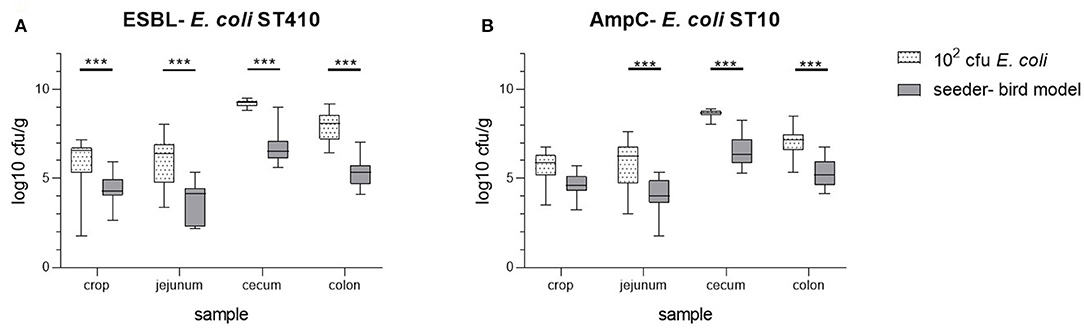
Figure 3. Comparison of the bacterial counts of ESBL- E. coli 10716 (A) and AmpC- E. coli 10717 (B) in samples of crop, jejunum, cecum, and colon at necropsy between the minimal colonization dosage group (102 cfu/E. coli; necropsy on day 14; all animals inoculated on third day of life) and the seeder-bird model (necropsy on day 35; inoculation ratio of 1:5 on third day of life). ***p < 0.001 (t-test).
To conclude, without any antimicrobial selection pressure by antibiotic usage, both commensal ESBL- and AmpC- producing E. coli strains colonized all broilers digestive tract independent of being an orally inoculated seeder bird or a susceptible sentinel bird. For all four colonization dosage trials and the seeder-bird trial we did not detect any colonization of liver or spleen with our bacterial strains tested. By analyzing the respective antimicrobial resistance patterns via VITEK 2 of the strains derived from the cecum samples at the necropsies, we did not observe major changes in the resistance profile of the inoculation isolates to the isolates at the end of the trial. The rate of transfer of resistance plasmids to other strains can therefore be considered to be of low importance, as some of our resistance markers were also chromosomally encoded, such as fluoroquinolone resistance. In addition, we did not observe changes in the morphotypes of the isolates during the trials.
Discussion
Our study points out that (i) a colonization dosage of 102 cfu E. coli per animal is sufficient for a successful long-time colonization of broiler chickens and (ii) a ratio of 1:5 inoculated seeder birds to non- inoculated sentinel birds is sufficient to colonize a complete broiler group even in the absence of antimicrobial selection pressure. In addition, we also demonstrated that even a very low colonization dosage of 101 cfu E. coli leads to a colonization of broilers in the given experimental setup using a study design based on parameters of real farming conditions. The animals were treated equally to those in conventional fattening farms. This includes a stocking density of 39 kg/m2, fresh litter once at the beginning of the trial, no enrichment and conventional feed and water ad libitum. Neither the utilized eggs and animals, nor the feed, water or litter were additionally processed for the reduction of germs than usual in the broiler production. In agreement, we received all eggs, litter and feed from a commercial poultry producer in Germany. To ensure comparable conditions in all trials, we used batches from one single producer and all trials were conducted within 6 months. Hence, an interaction between the animals and their surrounding environment including the present bacterial spectrum was possible before inoculation of the broiler chickens with the bacterial strains (Apajalahti et al., 2004; Ballou et al., 2016; Kumar et al., 2018). As the surrounding can be a source of ESBL- and AmpC- acquisition (Dierikx C. M. et al., 2013; Daehre et al., 2018b), we tested the complete experimental setup for the absence of ESBL-/AmpC- producing bacteria prior to the trial to exclude the possibility of a colonization out of the environment.
We inoculated our broiler chickens on the third day of life with two bacterial strains, harboring frequently detected ESBL- and AmpC- resistance profiles in fattening chickens (Ewers et al., 2012; Valentin et al., 2014). This is in accordance with numerous studies assuming a very early colonization of the broilers in the production chain. Projahn et al. (2017) describes a possible transfer of resistant bacteria already in the hatchery and during the transportation of the hatchlings to the fattening farm (Projahn et al., 2018). Furthermore, the fattening stable itself (Daehre et al., 2018b) or the surrounding areas (Laube et al., 2014) are potential sources of the acquisition of resistant bacteria. At the same time, investigations of the ESBL- and AmpC- prevalence of day- old broiler chickens are heterogeneous, varying between 95 and 0% in the analyzed broiler flocks (Laube et al., 2013; Daehre et al., 2018b). Thereby, both studies analyzed seven broiler flocks via cloacal swabs of 20 (Laube et al., 2013) or 40 broiler chickens (Daehre et al., 2018b) and both used a pre-enrichment in LB to determine the ESBL- and AmpC- prevalence. The wide variations may be caused by the selected sampling times. Laube et al. (2013) sampled within the first two days of life. In contrast to this, Daehre et al. (2018b) sampled immediately after placing the broiler chickens into the stable. However, it must be noted, that the overall prevalence of the investigated flocks is higher in the study by Laube et al. (2013). As it is known, compared to other species, the relation between the body length and the length of the gastrointestinal tract of chickens is relatively short with a rapid passage time (Hughes, 2008). To attain a reliable detection of our bacterial strains, we decided for an initial validation of our bacterial strains 24 h after inoculation. At this early time point, more than half of the sentinel birds in the seeder-bird trial had been tested positive for the ESBL- and AmpC- producing strains. This indicates a double passage of the gastrointestinal tract of firstly the seeder birds and following the sentinel birds within 24 h. These results were obtained by direct processing of the cloacal swabs without enrichment. To ensure a strong colonization of the broiler chickens, a direct detection of the bacterial strains is required. At the same time, a swab sampling provides only limited information about the intestinal colonization of the birds. The detection is strongly influenced by the amount of feces on the swab. Thus, our detection limit is relatively high, depending on the cloacal excretion during the sampling. For this reason, we decided for a minimal colonization dosage of 102 cfu E. coli even if one broiler chicken was tested negative for one of the bacterial strains 24 h p.i.
As this study aims to mimic the route of natural infection with ESBL- and AmpC- producing Enterobacteriaceae, a housing of all broiler chickens immediately from the beginning of the trial in one pen is important. Under real farming conditions, it is not possible to distinguish between colonized and non-colonized birds as well. Consequently, a distinction between colonization with the bacterial strains via inoculation or through the oral uptake out of the contaminated environment was not aimed in our trial. Although we cannot exclude that the colonization via inoculation of the seeder birds might have failed and an oral uptake of droppings from colonized chickens led to a colonization, this does not reduce the importance for the practice. Rather, it reflects the circumstances affecting a colonization under field conditions. Both, the colonization of the sentinel birds as well as the seeder birds is dependent upon the initial colonization of the seeder birds via inoculation. The dependency between seeder birds and sentinel birds and the resulting colonization with ESBL- E. coli was described by Ceccarelli et al. (2017) and is neglected in our colonization model. Moreover, besides our housing conditions, the sampling frequency does not allow to draw a conclusion of the transmission rate of ESBL- and AmpC- producing Enterobacteriaceae: our study is focused on the outcome of a practical orientated colonization.
Since the genes encoding for ESBLs and AmpCs are frequently located on plasmids (Carattoli, 2013), the possibility of a horizontal gene transfer (HGT) to other Enterobacteriaceae has to be considered. Even in the absence of antimicrobial selection pressure a transmission of resistance genes is possible (Smet et al., 2011). To ensure a reliable detection of our E. coli strains, a chromogenic agar was used and colony morphologies were checked up on their belonging to both strains. Additionally, in all digestive tract samples taken at necropsy, an agar plate without antibiotics was used to quantify the total count of E. coli colonies (data not shown). The total count of E. coli was congruent with the sum of our quantified ESBL- and AmpC- strains in all trails. Furthermore, out of the cecal content, colonies from the selective agar plates for the growth of the ESBL- and AmpC- E. coli strains were randomly selected and checked for their phenotypic antimicrobial resistances using VITEK 2. The profile of the examined colonies corresponded to those of our inoculation strains. The possibility of a HGT to other E. coli strains resident in the digestive tract cannot be entirely ruled out, but a colonization based on in vivo HGT seems to be unlikely, as in all colonization dosage trials equivalence of the cecum samples was shown. An equal HGT to other E. coli strains harboring the same resistance profile in all trials is highly unlikely, taking into consideration the chromosomally encoded resistances of our ESBL- E. coli strain.
Our results confirm the hypothesis that the uptake of only a few bacteria is sufficient to colonize broiler chickens with ESBL- and AmpC- producing Enterobacteriaceae. In fact, an inoculation of 101 cfu E. coli leads to a colonization of broiler chickens. Due to a continuous cleaning and disinfection regime, the bacterial load at every level of the broiler production chain is supposed to be minimized. Nevertheless, there is still remaining bacteria detectable after cleaning and disinfection (Luyckx et al., 2015). Furthermore, ESBL- and AmpC- producing bacteria are found frequently (Dierikx C. M. et al., 2013; Daehre et al., 2018a). Even if there is no detailed information available about the detected quantities of these resistant bacteria after cleaning and disinfection, it is practically impossible to achieve procedures that will result in such a very low bacterial load, that a colonization of broiler chickens from the subsequent flock does not take place. In addition, the process of colonization with resistant bacteria can be greatly strengthened by applying selection pressure through antimicrobial treatment of the birds during the fattening period. The importance of cleaning and disinfection measures in broiler fattening stables is underlined by Schulz et al. (2016). The study by Schulz et al. points out, that antimicrobial resistant E. coli is able to survive for decades in dust samples with concentrations up to 104 cfu per gram dust.
In our study, an instillation into the crop with 101 cfu E. coli led to an equivalent colonization of broiler chickens as the higher tested doses. These data were obtained by inoculating every single animal of the group. To obtain data closer to real farming conditions, we conducted a seeder-bird model by inoculating only one fifth of the animals (seeder birds) in a group with our defined minimal colonization dosage of 102 cfu E. coli. In literature, different methods of administration of E. coli to poultry are described. Besides an oral inoculation into the beak (Wang et al., 2017), a spray application over the nose and eyes of the birds and a treatment of the feeders has been implemented (Huff et al., 2011). A safer method to ensure the uptake of the bacterial suspension is an instillation into the crop. Thereby, to mimic the route of natural bacterial infection in animal trials, the seeder-bird model is frequently used and described for different enteral bacteria, such as E. coli, Salmonella enterica and Campylobacter spec. (Ratert et al., 2015; Schneitz and Hakkinen, 2016; Ceccarelli et al., 2017). The non- inoculated, susceptible sentinel birds mirror the animals naturally infected by the oral uptake of resistant bacteria from their surrounding environment after they were shed by the seeder birds. In the course of this, a variety of different ratios is known and a ratio of 1:5 seeder- to sentinel birds is more often described for the inoculation with Enterobacteriaceae (Methner et al., 2011; de Cort et al., 2015; Kilroy et al., 2015). Using this relation led to a complete colonization of all birds within our group. For us, with regard to future studies, a colonization in combination with an approximation to real farming conditions was the most important. As there is no data available about the number of initially colonized broiler chickens with resistant bacteria through contaminated farm environment, other ratios were not tested. Lower ratios of inoculated to non-inoculated broiler chickens might lead to lower prevalences of the flocks but can still constitute a source for contamination in further steps of the broiler production chain. In this connection, an introduction of resistant bacteria and cross- contamination at slaughterhouse level was proven by von Tippelskirch et al. (2018). As another consequence, remaining bacteria in the fattening stable after cleaning and disinfection is a source for the colonization of the subsequent broiler flock as described above.
After 35 days of the seeder-bird trial, the bacterial count observed in all necropsy samples taken is similar for all broilers, irrespective of being an artificially inoculated seeder bird or naturally colonized sentinel bird. This clearly shows the impact of the oral (re-) colonization with ESBL- and AmpC- producing Enterobacteriaceae during the ongoing trial. Even if the inoculation of only one fifth of the broilers initially resulted in a higher percentage of colonized seeder birds, observed in the cloacal swabs taken, the oral uptake of resistant bacteria from their surrounding environment seems to be of great importance for the colonization. In this way, all sentinel birds achieved a comparable colonization as the seeder birds over a period of 35 days. Interestingly, the bacterial count in all necropsy samples from the seeder-bird group is significantly lower compared to the dosage trial using 102 cfu E. coli. A first possible explanation is the different number of ESBL- and AmpC- inoculated broilers per trial. The oral colonization of only one fifth of the broilers in the seeder-bird model initially leads to less shedding of resistant bacteria into the animal housing. Thus, the contamination of the environment with the bacterial strains and in consequence, the oral (re-) colonization of the birds in the seeder-bird group is lower compared to the dosage trial using 102 cfu E. coli. The lower bacterial contamination of the environment might also explain the lower initial prevalence observed in the colonization dosage trials using 101 and 102 cfu ESBL- and AmpC- E. coli compared to 103 and 104 cfu. However, a reasonable explanation for the different colonization levels in the seeder-bird trial compared to the trial using 102 cfu E. coli are the durations of the trials. With regard to strict animal welfare norms, a duration of 2 weeks was found to be adequate to demonstrate a colonization of our chickens with the E. coli strains in the colonization dosage trials. After defining the minimal colonization dosage of 102 cfu E. coli, a duration of 5 weeks was chosen for the subsequent seeder-bird trial, to mimic real farming conditions. This discrepancy between the experimental periods leads to a change in microbial flora of the broilers gastrointestinal tract. Up to an age of 49 days significant differences in the composition of the cecal content for Ross-hybrids were shown by Lu et al. (2003). This is in agreement with other studies postulating a change of the predominant bacteria in broilers at older age (Amit-Romach et al., 2004; Crhanova et al., 2011). These changes in the microbial flora might lead to a competition of ESBL- and AmpC- producers with other bacteria, resulting in a decrease of ESBL- and AmpC- colonization.
As the reservoir for ESBL- and AmpC- producing E. coli, the cecum has a predominant role to evaluate the colonization of the broilers in the final necropsy. In contrast to the other samples of the colonization dosage trials taken during necropsy, the values gained from the content of the cecum are comparable between the trials. Whereas a fermentation of the bacteria takes places in both ceca, the content of crop and jejunum do simply reflect the recent uptake of the resistant bacteria out of the surrounding. This is strongly influenced by the feeding and drinking behavior of the broilers immediately before the necropsy. Because of the contamination with feces, an increased pecking in the litter leads to a large intake of bacteria compared to a pecking out of the feeding troughs or drinking and provides a reasonable explanation for the different bacterial counts observed in crop and jejunum samples.
Without applying any antimicrobial selection pressure to the birds, a colonization was achieved in our trials using a low dose of ESBL- and AmpC- producing E. coli. The usage of antibiotics in animal farming is discussed controversially, with studies indicating an impact of antibiotic treatment on co-selection and occurrence of resistant bacteria (Costa et al., 2009; Persoons et al., 2011; Dierikx C. M. et al., 2013). In recent years, an increasing number of studies are concluding that the occurrence of ESBL- and AmpC- producing bacteria in fattening chickens is not related to antibiotic treatment (Hiroi et al., 2012; Huijbers et al., 2016; Projahn et al., 2018). Our study reinforces these findings, showing a colonization even of the susceptible sentinel birds using a low dose of ESBL- and AmpC- producing E. coli without applying any antimicrobial selection pressure. In support of this, numerous studies are reporting a carriage of ESBL- and AmpC- resistant bacteria in wild bird species that have never been faced with any antibiotics before (Guenther et al., 2012; de Cort et al., 2015; Alcalá et al., 2016). Both of our inoculation strains were derived from a previous research project on ESBL- and AmpC- E. coli from chicken farming in Germany, thus presenting actual strains occurring in broiler chicken production (Falgenhauer et al., 2016; Hering et al., 2016). Besides that, ST10 and ST410 were chosen due to their ubiquitous nature as ST10 presents an ancient sequence type often present in livestock farming. ST410 was chosen due to its recent emergence as high risk clone in Germany and worldwide (Falgenhauer et al., 2016; Schaufler et al., 2016, 2019).
As a limitation, we investigated only one animal group per colonization dosage and we have no information on the variation between groups with the same colonization dosage. Although the inoculation of four different colonization dosages verified the general method, a repetition of the equal dosage or inoculation ratio of the broiler chickens might result in higher or lower initial colonization rates. Furthermore, an intra-herd correlation between the broiler chickens of one group is present and the results of the birds within one group are dependent upon each other. The effects shown can be group-specific and through a possible underestimation of variance in our analysis the results cannot be generalized completely.
Nonetheless, we showed a colonization of broiler chickens with a low colonization dosage of 102 cfu E. coli and a small number of orally inoculated broilers in the seeder-bird model. This might be a feasible explanation for the global spread of ESBL- and AmpC- producing enterobacteria in conventional broiler farms. With regard to an assumed transmission of resistant bacteria to humans through close contact to colonized broilers or the consumption of contaminated retail meat, further steps for the reduction of ESBL- and AmpC- producing bacteria have to be considered. Consequently, based on this practical orientated colonization model, different hygiene- and management measures as well as gut microbiota influencing measures are currently being investigated as potential intervention strategies to reduce the colonization of broilers.
Data Availability
All datasets generated for this study are included in the manuscript/Supplementary Files.
Author Contributions
SG, UR, AB, and AF designed the study. CR, SG, and AB performed the samplings and laboratory work. RM and CR performed the statistical analysis. CR evaluated the final data and wrote the manuscript. All authors have read and approved the final draft of the article.
Funding
This study was funded by the Federal Ministry of Food and Agriculture (grant 2817701014) and is part of the EsRAM research consortium.
Conflict of Interest Statement
The authors declare that the research was conducted in the absence of any commercial or financial relationships that could be construed as a potential conflict of interest.
Acknowledgments
We would like to thank our partners from the EsRAM research consortium for providing the eggs, litter, and feed of the animals and Gerzon Motola for assistance during disinfection of the eggs. Many thanks to all employees of the Institute for Animal Hygiene and Environmental Health, FU Berlin, for support during the trial and Olga Makarova for proofreading the manuscript. We acknowledge support by the German Research Foundation and the Open Access Publication Fund of the Freie Universität Berlin.
Supplementary Material
The Supplementary Material for this article can be found online at: https://www.frontiersin.org/articles/10.3389/fmicb.2019.02124/full#supplementary-material
Figure S1. Colonization of broiler chickens during the four colonization dosage trials 101–104 cfu/E. coli (A–D) and the seeder birds and sentinel birds of the seeder-bird model trial (E,F) using a semiquantitative measurement with categories from 0 to 7: 0 = no growth; 1 ≤ 10 cfu E. coli; 2 = between 1 and 3; 3 ≤ 100 cfu E. coli; 4 = between 3 and 5; 5 > 100 cfu E. coli; 6 = between 5 and 7; 7 = agar plate overgrown; d p.i. = days post inoculation.
Table S1. Mean values and confidence intervals of digestive tract samples from colonization dosage groups (101–104 cfu/E. coli) and seeder-bird model attained at necropsy.
References
Alcalá, L., Alonso, C. A., Simón, C., González-Esteban, C., Orós, J., Rezusta, A., et al. (2016). Wild Birds, Frequent Carriers of Extended-Spectrum β-Lactamase (ESBL) Producing Escherichia coli of CTX-M and SHV-12 Types. Microb. Ecol. 72, 861–869. doi: 10.1007/s00248-015-0718-0
Amit-Romach, E., Sklan, D., and Uni, Z. (2004). Microflora ecology of the chicken intestine using 16S ribosomal DNA primers. Poultry Sci. 83, 1093–1098. doi: 10.1093/ps/83.7.1093
Apajalahti, J., Kettunen, A., and Graham, H. (2004). Characteristics of the gastrointestinal microbial communities, with special reference to the chicken. World's Poultry Sci. J. 60, 223–232. doi: 10.1079/WPS200415
Arredondo-Alonso, S., Rogers, M. R. C., Braat, J. C., Verschuuren, T. D., Top, J., Corander, J., et al. (2018). mlplasmids: a user-friendly tool to predict plasmid- and chromosome-derived sequences for single species. Microb. Genomics. 4:11. doi: 10.1099/mgen.0.000224
Ballou, A. L., Ali, R. A., Mendoza, M. A., Ellis, J. C., Hassan, H. M., Croom, W. J., et al. (2016). Development of the chick microbiome: how early exposure influences future microbial diversity. Front. Veter. Sci. 3:2. doi: 10.3389/fvets.2016.00002
Carattoli, A. (2013). Plasmids and the spread of resistance. Int. J. Med. Microbiol. 303, 298–304. doi: 10.1016/j.ijmm.2013.02.001
Ceccarelli, D., van Essen-Zandbergen, A., Smid, B., Veldman, K. T., Boender, G. J., Fischer, E. A. J., et al. (2017). Competitive exclusion reduces transmission and excretion of extended-spectrum-β-lactamase-producing Escherichia coli in broilers. Appl. Environ. Microbiol. 83:11. doi: 10.1128/AEM.03439-16
Cohen Stuart, J., van den Munckhof, T., Voets, G., Scharringa, J., Fluit, A., and Hall, M. L. (2012). Comparison of ESBL contamination in organic and conventional retail chicken meat. Int. J. Food Microbiol. 154, 212–214. doi: 10.1016/j.ijfoodmicro.2011.12.034
Cortés, P., Blanc, V., Mora, A., Dahbi, G., Blanco, J. E., Blanco, M., et al. (2010). Isolation and characterization of potentially pathogenic antimicrobial-resistant Escherichia coli strains from chicken and pig farms in Spain. Appl. Environ. Microbiol. 76, 2799–2805. doi: 10.1128/AEM.02421-09
Costa, D., Vinué, L., Poeta, P., Coelho, A. C., Matos, M., Sáenz, Y., et al. (2009). Prevalence of extended-spectrum beta-lactamase-producing Escherichia coli isolates in faecal samples of broilers. Veter. Microbiol. 138, 339–344. doi: 10.1016/j.vetmic.2009.03.029
Crhanova, M., Hradecka, H., Faldynova, M., Matulova, M., Havlickova, H., Sisak, F., et al. (2011). Immune response of chicken gut to natural colonization by gut microflora and to Salmonella enterica serovar enteritidis infection. Infect. Immunity. 79, 2755–2763. doi: 10.1128/IAI.01375-10
Daehre, K., Projahn, M., Friese, A., Semmler, T., Guenther, S., and Roesler, U. H. (2018a). ESBL-Producing Klebsiella pneumoniae in the broiler production chain and the first description of ST3128. Front. Microbiol. 9:2302. doi: 10.3389/fmicb.2018.02302
Daehre, K., Projahn, M., Semmler, T., Roesler, U., and Friese, A. (2018b). Extended-spectrum beta-lactamase-/AmpC beta-lactamase-producing enterobacteriaceae in broiler farms: transmission dynamics at farm level. Microb. Drug Resist. 24, 511–518. doi: 10.1089/mdr.2017.0150
de Cort, W., Haesebrouck, F., Ducatelle, R., and van Immerseel, F. (2015). Administration of a Salmonella Enteritidis ΔhilAssrAfliG strain by coarse spray to newly hatched broilers reduces colonization and shedding of a Salmonella Enteritidis challenge strain. Poultry Sci. 94, 131–135. doi: 10.3382/ps/peu018
Dierikx, C., van der Goot, J., Fabri, T., van Essen-Zandbergen, A., Smith, H., and Mevius, D. (2013). Extended-spectrum-β-lactamase- and AmpC-β-lactamase-producing Escherichia coli in Dutch broilers and broiler farmers. J. Antimicrob. Chemother. 68, 60–67. doi: 10.1093/jac/dks349
Dierikx, C. M., van der Goot, J. A., Smith, H. E., Kant, A., and Mevius, D. J. (2013). Presence of ESBL/AmpC-producing Escherichia coli in the broiler production pyramid: a descriptive study. PLoS ONE. 8:e79005. doi: 10.1371/journal.pone.0079005
Dorado-García, A., Smid, J. H., van Pelt, W., Bonten, M. J. M., Fluit, C., van den Bunt, G., et al. (2018). Molecular relatedness of ESBL/AmpC-producing Escherichia coli from humans, animals, food and the environment: a pooled analysis. J. Antimicrob. Chemother. 73, 339–347. doi: 10.1093/jac/dkx397
EFSA (2019). The European Union summary report on antimicrobial resistance in zoonotic and indicator bacteria from humans, animals and food in 2017. EFS2 17:367. doi: 10.2903/j.efsa.2019.5598
Ewers, C., Bethe, A., Semmler, T., Guenther, S., and Wieler, L. H. (2012). Extended-spectrum β-lactamase-producing and AmpC-producing Escherichia coli from livestock and companion animals, and their putative impact on public health: a global perspective. Clin. Microbiol. Infect. 18, 646–655. doi: 10.1111/j.1469-0691.2012.03850.x
Falgenhauer, L., Imirzalioglu, C., Ghosh, H., Gwozdzinski, K., Schmiedel, J., Gentil, K., et al. (2016). Circulation of clonal populations of fluoroquinolone-resistant CTX-M-15-producing Escherichia coli ST410 in humans and animals in Germany. Int. J. Antimicrob. Agents 47, 457–465. doi: 10.1016/j.ijantimicag.2016.03.019
Guenther, S., Aschenbrenner, K., Stamm, I., Bethe, A., Semmler, T., Stubbe, A., et al. (2012). Comparable high rates of extended-spectrum-beta-lactamase-producing Escherichia coli in birds of prey from Germany and Mongolia. PLoS ONE 7:e53039. doi: 10.1371/journal.pone.0053039
Guenther, S., Ewers, C., and Wieler, L. H. (2011). Extended-spectrum beta-lactamases producing E. coli in Wildlife, yet Another Form of Environmental Pollution? Front. Microbiol. 2:246. doi: 10.3389/fmicb.2011.00246
Hering, J., Frömke, C., von Münchhausen, C., Hartmann, M., Schneider, B., Friese, A., et al. (2016). Cefotaxime-resistant Escherichia coli in broiler farms-A cross-sectional investigation in Germany. Prevent. Veter. Med. 125, 154–157. doi: 10.1016/j.prevetmed.2016.01.003
Hille, K., Felski, M., Ruddat, I., Woydt, J., Schmid, A., Friese, A., et al. (2018). Association of farm-related factors with characteristics profiles of extended-spectrum β-lactamase- / plasmid-mediated AmpC β-lactamase-producing Escherichia coli isolates from German livestock farms. Veter. Microbiol. 223, 93–99. doi: 10.1016/j.vetmic.2018.07.022
Hiroi, M., Matsui, S., Kubo, R., Iida, N., Noda, Y., Kanda, T., et al. (2012). Factors for occurrence of extended-spectrum β-lactamase-producing Escherichia coli in broilers. J. Vet. Med. Sci. 74, 1635–1637. doi: 10.1292/jvms.11-0479
Huff, G. R., Dutta, V., Huff, W. E., and Rath, N. C. (2011). Effects of dietary yeast extract on turkey stress response and heterophil oxidative burst activity. Br. Poultry Sci. 52, 446–455. doi: 10.1080/00071668.2011.600753
Hughes, R. J. (2008). Relationship between digesta transit time and apparent metabolisable energy value of wheat in chickens. Br. Poultry Sci. 49, 716–720. doi: 10.1080/00071660802449145
Huijbers, P. M., Graat, E. A., Haenen, A. P., van Santen, M. G., van Essen-Zandbergen, A., Mevius, D. J., et al. (2014). Extended-spectrum and AmpC β-lactamase-producing Escherichia coli in broilers and people living and/or working on broiler farms: prevalence, risk factors and molecular characteristics. J. Antimicrob. Chemother. 69, 2669–2675. doi: 10.1093/jac/dku178
Huijbers, P. M. C., Graat, E. A. M., van Hoek, A. H. A. M., Veenman, C., de Jong, M. C. M., and van Duijkeren, E. (2016). Transmission dynamics of extended-spectrum β-lactamase and AmpC β-lactamase-producing Escherichia coli in a broiler flock without antibiotic use. Prevent. Veter. Med. 131, 12–19. doi: 10.1016/j.prevetmed.2016.07.001
Kaspar, U., von Lützau, K., Schlattmann, A., Rösler, U., Köck, R., and Becker, K. (2019). Zoonotic multidrug-resistant microorganisms among non-hospitalized horses from Germany. One Health 7:100091. doi: 10.1016/j.onehlt.2019.100091
Kilroy, S., Raspoet, R., Devloo, R., Haesebrouck, F., Ducatelle, R., and Van Immerseel, F. (2015). Oral administration of the Salmonella Typhimurium vaccine strain Nal2/Rif9/Rtt to laying hens at day of hatch reduces shedding and caecal colonization of Salmonella 4,12:i:-, the monophasic variant of Salmonella Typhimurium. Poultry Sci. 94, 1122–1127. doi: 10.3382/ps/pev078
Kola, A., Kohler, C., Pfeifer, Y., Schwab, F., Kühn, K., Schulz, K., et al. (2012). High prevalence of extended-spectrum-β-lactamase-producing Enterobacteriaceae in organic and conventional retail chicken meat, Germany. J. Antimicrob. Chemother. 67, 2631–2634. doi: 10.1093/jac/dks295
Kumar, S., Chen, C., Indugu, N., Werlang, G. O., Singh, M., Kim, W. K., et al. (2018). Effect of antibiotic withdrawal in feed on chicken gut microbial dynamics, immunity, growth performance and prevalence of foodborne pathogens. PLoS ONE 13:e0192450. doi: 10.1371/journal.pone.0192450
Laube, H., Friese, A., von Salviati, C., Guerra, B., Käsbohrer, A., Kreienbrock, L., et al. (2013). Longitudinal monitoring of extended-spectrum-beta-lactamase/AmpC-producing Escherichia coli at German broiler chicken fattening farms. Appl. Environ. Microbiol. 79, 4815–4820. doi: 10.1128/AEM.00856-13
Laube, H., Friese, A., von Salviati, C., Guerra, B., and Rösler, U. (2014). Transmission of ESBL/AmpC-producing Escherichia coli from broiler chicken farms to surrounding areas. Veter. Microbiol. 172, 519–527. doi: 10.1016/j.vetmic.2014.06.008
Leverstein-van Hall, M. A., Dierikx, C. M., Cohen Stuart, J., Voets, G. M., van den Munckhof, M. P., van Essen-Zandbergen, A., et al. (2011). Dutch patients, retail chicken meat and poultry share the same ESBL genes, plasmids and strains. Clin. Microbiol. Infect. 17, 873–880. doi: 10.1111/j.1469-0691.2011.03497.x
Liebana, E., Carattoli, A., Coque, T. M., Hasman, H., Magiorakos, A. P., Mevius, D., et al. (2013). Public health risks of enterobacterial isolates producing extended-spectrum β-lactamases or AmpC β-lactamases in food and food-producing animals: an EU perspective of epidemiology, analytical methods, risk factors, and control options. Clin. Infect. Dis. 56, 1030–1037. doi: 10.1093/cid/cis1043
Lu, J., Idris, U., Harmon, B., Hofacre, C., Maurer, J. J., and Lee, M. D. (2003). Diversity and succession of the intestinal bacterial community of the maturing broiler chicken. Appl. Environ. Microbiol. 69, 6816–6824. doi: 10.1128/AEM.69.11.6816-6824.2003
Luyckx, K., Dewulf, J., Van Weyenberg, S., Herman, L., Zoons, J., Vervaet, E., et al. (2015). Comparison of sampling procedures and microbiological and non-microbiological parameters to evaluate cleaning and disinfection in broiler houses. Poultry Sci. 94, 740–749. doi: 10.3382/ps/pev019
Mazzariol, A., Bazaj, A., and Cornaglia, G. (2017). Multi-drug-resistant Gram-negative bacteria causing urinary tract infections: a review. J. Chemother. 29(suppl. 1), 2–9. doi: 10.1080/1120009X.2017.1380395
Methner, U., Barrow, P. A., Berndt, A., and Rychlik, I. (2011). Salmonella Enteritidis with double deletion in phoPfliC–a potential live Salmonella vaccine candidate with novel characteristics for use in chickens. Vaccine 29, 3248–3253. doi: 10.1016/j.vaccine.2011.02.030
Persoons, D., Haesebrouck, F., Smet, A., Herman, L., Heyndrickx, M., Martel, A., et al. (2011). Risk factors for ceftiofur resistance in Escherichia coli from Belgian broilers. Epidemiol. Infect. 139, 765–771. doi: 10.1017/S0950268810001524
Pietsch, M., Irrgang, A., Roschanski, N., Brenner Michael, G., Hamprecht, A., Rieber, H., et al. (2018). Whole genome analyses of CMY-2-producing Escherichia coli isolates from humans, animals and food in Germany. BMC Genomics 19:601. doi: 10.1186/s12864-018-4976-3
Pitout, J. D. D. (2013). Enterobacteriaceae that produce extended-spectrum β-lactamases and AmpC β-lactamases in the community: the tip of the iceberg? Curr. Pharma. Design 19, 257–263. doi: 10.2174/138161213804070348
Projahn, M., Daehre, K., Roesler, U., and Friese, A. (2017). Extended-spectrum-beta-lactamase- and plasmid-encoded cephamycinase-producing enterobacteria in the broiler hatchery as a potential mode of pseudo-vertical transmission. Appl. Environ. Microbiol. 83:1. doi: 10.1128/AEM.02364-16
Projahn, M., Daehre, K., Semmler, T., Guenther, S., Roesler, U., and Friese, A. (2018). Environmental adaptation and vertical dissemination of ESBL-/pAmpC-producing Escherichia coli in an integrated broiler production chain in the absence of an antibiotic treatment. Microb. Biotechnol. 11, 1017–1026. doi: 10.1111/1751-7915.13040
Ratert, C., Sander, S. J., Verspohl, J., Beyerbach, M., and Kamphues, J. (2015). Effects of the physical form of diet on the outcome of an artificial salmonella infection in broilers. Avian Dis. 59, 74–78. doi: 10.1637/10890-062414-Reg
Reich, F., Atanassova, V., and Klein, G. (2013). Extended-spectrum β-lactamase- and AmpC-producing enterobacteria in healthy broiler chickens, Germany. Emerg. Infect. Dis. 19, 1253–1259. doi: 10.3201/eid1908.120879
Rubin, J. E., and Pitout, J. D. (2014). Extended-spectrum β-lactamase, carbapenemase and AmpC producing Enterobacteriaceae in companion animals. Veter. Microbiol. 170, 10–18. doi: 10.1016/j.vetmic.2014.01.017
Schaufler, K., Bethe, A., Lübke-Becker, A., Ewers, C., Kohn, B., Wieler, L. H., et al. (2015). Putative connection between zoonotic multiresistant extended-spectrum beta-lactamase (ESBL)-producing Escherichia coli in dog feces from a veterinary campus and clinical isolates from dogs. Infect. Ecol. Epidemiol. 5:25334. doi: 10.3402/iee.v5.25334
Schaufler, K., Semmler, T., Wieler, L. H., Trott, D. J., Pitout, J., Peirano, G., et al. (2019). Genomic and functional analysis of emerging virulent and multi-drug resistant E. coli lineage ST648. Antimicrob. Agents Chemother. 63:6. doi: 10.1128/AAC.00243-19
Schaufler, K., Semmler, T., Wieler, L. H., Wöhrmann, M., Baddam, R., Ahmed, N., et al. (2016). Clonal spread and interspecies transmission of clinically relevant ESBL-producing Escherichia coli of ST410–another successful pandemic clone? FEMS Microbiol. Ecol. 92:1. doi: 10.1093/femsec/fiv155
Schneitz, C., and Hakkinen, M. (2016). The efficacy of a commercial competitive exclusion product on Campylobacter colonization in broiler chickens in a 5-week pilot-scale study. Poultry Sci. 95, 1125–1128. doi: 10.3382/ps/pew020
Schulz, J., Ruddat, I., Hartung, J., Hamscher, G., Kemper, N., and Ewers, C. (2016). Antimicrobial-resistant Escherichia coli survived in dust samples for more than 20 years. Front. Microbiol. 7:866. doi: 10.3389/fmicb.2016.00866
Selbitz, H., Truyen, U., and Valentin-Weigand, P. (2015). Tiermedizinische Mikrobiologie, Infektions- und Seuchenlehre. 10., aktualisierte Auflage. Stuttgart: Enke Verlag
Smet, A., Rasschaert, G., Martel, A., Persoons, D., Dewulf, J., Butaye, P., et al. (2011). In situ ESBL conjugation from avian to human Escherichia coli during cefotaxime administration. J. Appl. Microbiol. 110, 541–549. doi: 10.1111/j.1365-2672.2010.04907.x
Thrusfield, M. V. (2007). Veterinary Epidemiology. 3. ed., Reissued in Paperback With Updates. Oxford: Blackwell Science.
Valentin, L., Sharp, H., Hille, K., Seibt, U., Fischer, J., Pfeifer, Y., et al. (2014). Subgrouping of ESBL-producing Escherichia coli from animal and human sources: an approach to quantify the distribution of ESBL types between different reservoirs. Int. J. Med. Microbiol. 304, 805–816. doi: 10.1016/j.ijmm.2014.07.015
Valenza, G., Nickel, S., Pfeifer, Y., Eller, C., Krupa, E., Lehner-Reindl, V., et al. (2014). Extended-spectrum-β-lactamase-producing Escherichia coli as intestinal colonizers in the German community. Antimicrob. Agents Chemother. 58, 1228–1230. doi: 10.1128/AAC.01993-13
Vincent, C., Boerlin, P., Daignault, D., Dozois, C. M., Dutil, L., Galanakis, C., et al. (2010). Food reservoir for Escherichia coli causing urinary tract infections. Emerg. Infect. Dis. 16, 88–95. doi: 10.3201/eid1601.091118
von Tippelskirch, P., Gölz, G., Projahn, M., Daehre, K., Friese, A., Roesler, U., et al. (2018). Prevalence and quantitative analysis of ESBL and AmpC beta-lactamase producing Enterobacteriaceae in broiler chicken during slaughter in Germany. Int. J. Food Microbiol. 281, 82–89. doi: 10.1016/j.ijfoodmicro.2018.05.022
Keywords: ESBL, AmpC, Escherichia coli, broiler chicken, colonization, antimicrobial selection pressure, seeder bird model
Citation: Robé C, Blasse A, Merle R, Friese A, Roesler U and Guenther S (2019) Low Dose Colonization of Broiler Chickens With ESBL-/AmpC- Producing Escherichia coli in a Seeder-Bird Model Independent of Antimicrobial Selection Pressure. Front. Microbiol. 10:2124. doi: 10.3389/fmicb.2019.02124
Received: 11 March 2019; Accepted: 29 August 2019;
Published: 13 September 2019.
Edited by:
Miklos Fuzi, Semmelweis University, HungaryReviewed by:
Yvonne Pfeifer, Robert Koch Institute, GermanyJan Arend Stegeman, Utrecht University, Netherlands
Copyright © 2019 Robé, Blasse, Merle, Friese, Roesler and Guenther. This is an open-access article distributed under the terms of the Creative Commons Attribution License (CC BY). The use, distribution or reproduction in other forums is permitted, provided the original author(s) and the copyright owner(s) are credited and that the original publication in this journal is cited, in accordance with accepted academic practice. No use, distribution or reproduction is permitted which does not comply with these terms.
*Correspondence: Caroline Robé, caroline.robe@fu-berlin.de; tierhygiene@vetmed.fu-berlin.de