- 1State Key Laboratory of Microbial Metabolism, School of Life Sciences and Biotechnology, Shanghai Jiao Tong University, Shanghai, China
- 2Key Laboratory of Marine Drugs, The Ministry of Education of China, School of Medicine and Pharmacy, Ocean University of China, Qingdao, China
- 3Laboratory for Marine Drugs and Bioproducts, Qingdao National Laboratory for Marine Science and Technology, Qingdao, China
- 4Institute of Evolution and Marine Biodiversity, Ocean University of China, Qingdao, China
Co-culture of different microbes simulating the natural state of microbial community may produce potentially new compounds because of nutrition or space competition. To mine its metabolic potential in depth, co-culture of Streptomyces rochei MB037 with a gorgonian-derived fungus Rhinocladiella similis 35 was carried out to stimulate the production of new metabolites in this study, using pure cultivation as control. Five metabolites were isolated successfully from co-culture broth, including two new fatty acids with rare nitrile group, borrelidins J and K (1 and 2), one chromone derivative as a new natural product, 7-methoxy-2,3-dimethylchromone-4-one (3), together with two known 18-membered macrolides, borrelidin (4) and borrelidin F (5). The structures of 1–3 were elucidated by using a combination of NMR and MS spectroscopy, ester hydrolysis, and optical rotation methods. Interestingly, 1 and 2 were obtained only in co-culture. Though 3 was gained from either co-culture or single culture, its production was increased significantly by co-culture. Compound 1 exhibited significant antibacterial activity against methicillin-resistant Staphylococcus aureus with a MIC value of 0.195 μg/mL.
Introduction
In nature, microbes generally exist in a community. One microbe may produce biological products to inhibit other microbes for limited nutrition or space competition or against pathogenic microbes. Thus, co-culture of microorganisms which involves the cultivation of two or more microorganisms in the same confined environment may produce potentially new compounds by stimulating the silent genes or gene clusters of one partner or increase the yields of previously described metabolites. For example, Sung et al. (2017) researched increased production of three antibiotics and enhanced biological activity against the Gram positive human pathogens via co-cultures of a marine-derived Streptomyces sp. with human pathogens (Sung et al., 2017), and Zuck et al. (2011) gained a cytotoxic N,N′-((1Z,3Z)-1,4-bis(4-methoxyphenyl)buta-1,3-diene-2,3-diyl)diformamide by co-culture of the fungus Aspergillus fumigatus with the actinomycete Streptomyces peucetius.
Macrolide borrelidin has been reported to show broad-spectrum activities (Anderson et al., 1989; Ishiyama et al., 2011; Mirando et al., 2015). In our previous study, borrelidin was isolated and elucidated as a major product from a sponge-derived actinomycete Streptomyces rochei MB037 (Li et al., 2018). To mine its more metabolic potential, co-culture approach was applied on S. rochei MB037. A gorgonian-derived fungal strain, Rhinocladiella similis 35, was selected as a partner against actinomycete S. rochei MB037. A literature survey revealed that a few new and bioactive compounds were separated from the fungus Rhinocladiella sp. (Wagenaar et al., 2000; Zhang et al., 2014). The co-culture of S. rochei MB037 and R. similis 35 stimulated the production of new metabolites successfully. Herein, we report the isolation, structural elucidation, and evaluation of biological activities of the metabolites 1–5 (Figure 1) produced by co-culture of S. rochei MB037 and R. similis 35. A plausible biosynthesis pathway for the metabolites was also proposed and discussed.
Materials and Methods
Instrumentation
Optical rotations were determined on a JASCO P-2000 polarimeter. 1D and 2D NMR spectra were recorded on an Avance III 600 MHz NMR spectrometer. High-resolution electrospray ionization mass spectroscopy (HR-ESI-MS) was recorded with an ACQUITYTM UPLC and Q-TOF mass spectrometer. High-performance liquid chromatography (HPLC) analysis was performed with an Agilent 1200 detector (G1322A), using a Durashell 150 Å C18 column (4.6 mm × 250 mm, 5 μm, Agela) and HPLC preparative-scale purification was performed with an Eclipse XDB C18 column (4.6 mm × 150 mm, 5 μm, Agela). The FT-IR spectra were recorded using a Nicolet 6700 spectrometer.
Microbial Strains
The actinomycete S. rochei MB037 was derived from sponge Dysidea arenaria collected from Yongxin Island (112° 20′ E; 16° 50′ N) in the South China Sea (Karuppiah et al., 2015). It was identified as S. rochei on the basis of the 100% 16S rDNA sequence identity with the type strain of this species, under the GenBank Accession No. AA2017041 (Li et al., 2018). The fungal strain R. similis 35 was isolated from a staghorn gorgonian collected from Luhuitou fringing reefs (109° 25′ E; 18° 15′ N) in the South China Sea in July 2014. The strain was identified as R. similis according to its morphologic traits and molecular identification. Its 616 base pair ITS sequence had 100% sequence identity to that of R. similis (KY680425) isolate CMRP1259. The sequence data have been submitted to GenBank, under the Accession No. MH481284. Both of the strains were stored at −80°C after their arrival to the Key Laboratory of Microbial Metabolism, Shanghai Jiao Tong University, China.
Fermentation, Extraction, and Isolation
The actinomycete S. rochei MB037 and fungal R. similis 35 were cultivated in 25 L of ISP2 medium [malt extract 10 g, anhydrous dextrose 4 g, yeast extract 4 g in 1 L of artificial seawater (NaCl 132.6 g, MgCl2⋅6H2O 55.86 g, CaCl2 5.705 g, KCl 3.625 g, NaHCO3 1.01 g, NaBr 0.415 g), pH value 7.0] at 28°C with shaking at 180 rpm, respectively. On day 3, 200 mL of actinomycete culture was inoculated into each 200 mL of the fungal cultures (1:1 v/v) to initiate the co-culture experiment. The changes of secondary metabolite production between co-culture and single culture were analyzed by reverse-phased HPLC.
After incubation for 11 additional days, the co-culture broth was extracted with 50 L ethyl acetate using rotary evaporator to yield 12 g of reddish brown oil material. The organic extract (12 g) was subjected to Sephadex LH-20 eluting by MeOH to obtain four fractions (Fr. 1–4). Fr. 2–4 was subjected to reversed phase chromatograph eluting with MeOH-H2O (Cheetah Fs-9200t C18, linear gradient 10, 30, 50, 70, 90, and 100% aqueous CH3OH for 30 min, respectively, at a flow rate of 20 mL/min) to give three fractions (Fr. 2–7, 3–18, and 4–8). Fr. 2–7 was further purified twice by preparative HPLC (Eclipse XDB C18 column, 4.6 mm × 150 mm, 5 μm, Agela) with 40 to 44% aqueous CH3CN to yield 5 (7 mg). Fr. 3–18 was further purified using Sephadex LH-20 with CH3OH and then followed by preparative HPLC with 50% aqueous CH3CN to give 2 (5 mg), 4 (20 mg) and 5 (4 mg), respectively. Fr. 4–8 was further purified by preparative HPLC with 38% aqueous CH3CN to provide 3 (10 mg).
Borrelidin J (1)
A light yellow oil; [α]25D – 25.5 (c 4.0, MeOH); 1H and 13C NMR spectroscopic data, Tables 1, 2; (−)-HR-ESI-MS m/z 506.3117 [M-H]− (calcd for C28H44NO7, 506.3114).
Borrelidin K (2)
A light yellow oil; [α]25D – 14.0 (c 2.5, MeOH); 1H and 13C NMR spectroscopic data, Tables 1, 2; (−)-HR-ESI-MS m/z 520.3264 [M-H]– (calcd for C29H46NO7, 520.3261).
7-Methoxy-2,3-Dimethylchromone-4-One (3)
A brown yellow solid; 1H and 13C NMR spectroscopic data, Table 3; (+)-HR-ESI-MS m/z 205.0885 [M+H]+(calcd for C12H13O3, 205.0888).
Borrelidin (4)
A white amorphous powder; [α]25D – 16.0 (c 2.5, MeOH); 1H and 13C NMR spectroscopic data, Tables 1, 2; (−)-HR-ESI-MS m/z 520.3264 [M-H]– (calcd for C29H46NO7, 520.3261).
Borrelidin F (5)
A white amorphous powder; [α]25D + 14.0 (c 2.5, MeOH); 1H and 13C NMR spectroscopic data, Tables 1, 2; (−)-HR-ESI-MS m/z 520.3264 [M-H]– (calcd for C29H46NO7, 520.3261).
Antibacterial Bioassay
Antibacterial activity was evaluated by the conventional broth dilution assay (Appendino et al., 2008). Five bacterial strains: Escherichia coli, Pseudomonas aeruginosa, Staphylococcus aureus, Bacillus subtilis, and Bacillus mycoides were used, and ciprofloxacin was used as a positive control.
Results
Co-culture of S. rochei MB037 and R. similis 35
The co-culture of sponge-derived actinomycete S. rochei MB037 with multiple marine microorganisms (bacteria and fungi) was tested. Based on a series of screening of co-cultivation with single strain cultivation as control, significant changes in metabolites in the fermentation broth were observed in the co-culture of a gorgonian-derived fungal strain, R. similis 35 with S. rochei MB037. The actinomycete stain S. rochei MB037 and fungal strain R. similis 35 were cultured for 3 days, respectively, and then co-cultivated for 11 days. As a control, the single cultivation was carried out for 14 days. Then the EtOAc extracts of fermentation broth were compared by HPLC (Figure 2). In single strain cultivation, two peaks (4 and 5) appeared for S. rochei MB037 (Figure 2A); a weak peak (3) was detected in R. similis 35 (Figure 2B). Compared with the control, two extra peaks (1 and 2) and one obvious increased peak (3) were detected in the co-cultural EtOAc extracts (Figure 2C). HR-ESI-MS analysis confirmed that peaks 1 and 2 were not detected in single cultural broth of actinomycete S. rochei MB037, while peak 3 with the molecular weight 204 ([M + H]+ m/z at 205.0885) was detected in the single cultural broth of fungal strain R. similis 35 (Figure 3). This result indicated that the fungus R. similis 35 successfully induced the actinomycete S. rochei MB037 to produce new metabolites.
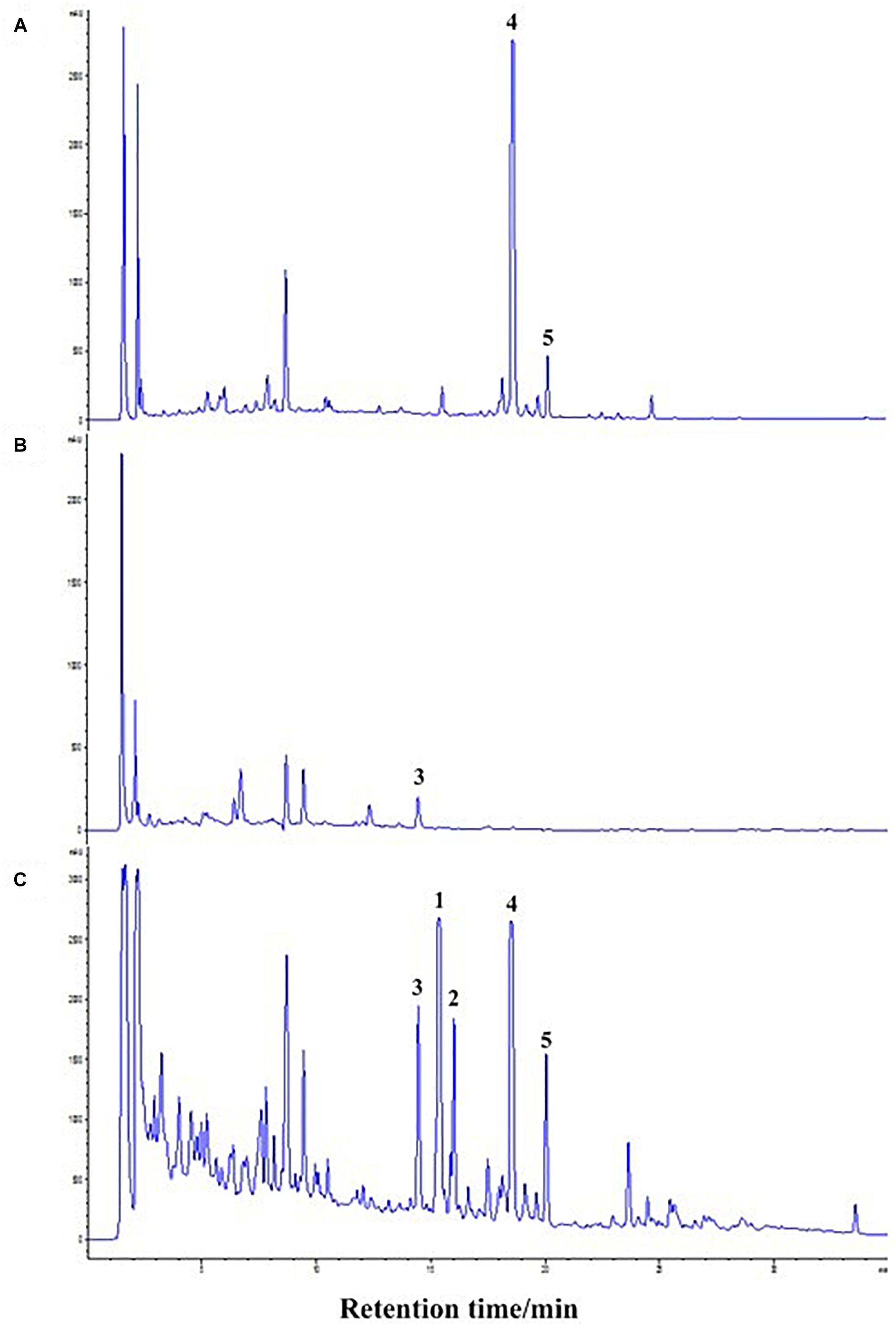
Figure 2. High-performance liquid chromatography (HPLC) profiles of the EtOAc extracts of different culture approaches. (A) Pure culture of Streptomyces rochei MB037; (B) pure culture of Rhinocladiella similis 35; (C) co-culture of S. rochei MB037 and R. similis 35.
Five compounds (1–5) (Figure 1) were isolated from the co-culture broth of S. rochei MB037 and R. similis 35 using column chromatography and preparative HPLC. Besides the known macrolide borrelidin (4) and borrelidin F (5), S. rochei MB037 was induced to produce two new fatty acids, borrelidins J (1) and K (2). Correspondingly, R. similis 35 was also stimulated to enhance the production of a chromone derivative 7-methoxy-2,3-dimethylchromone-4-one (3), which has never been reported as a natural product.
Structure Elucidation
Borrelidin J (1) was isolated as a yellow oil. Its molecular formula C28H45NO7 was confirmed by negative ion HR-ESI-MS ([M – H]– calcd. 506.3117, C28H44NO7–) (Supplementary Figure S1) with seven degrees of unsaturation. The IR spectra showed absorption at 3375, 2210, and 1637 cm–1, suggesting the presence of hydroxyl, nitrile group, and double-bond protons, respectively. The NMR of 1 (Supplementary Figures S2–S5) closely resembled that of the known compound borrelidin (4) expect for the differences at C-1 and C-17 proton signals. The C-1 proton signal at δC 172.1 in 4 was shifted to δC 175.4 in 1. The H-17 proton signal at δH 5.29 (1H, d, J = 10.0 Hz) in 4 was shifted to δH 3.83 (1H, m) in 1, and this change was consistent with the difference between 4 and 1 of 13C signals, the C-17 at δC 75.8 for 4 was shifted to δC 74.0 for 1 on the 13C NMR (Table 2 and Supplementary Figure S4). Also, on the HMBC (Supplementary Figure S6), C-1 was related to H-17 in 4, however, there was no correlation between them in 1. Besides, compound 4 has one less unsaturation and one more H2O on the formula and molecular weight than 1. On the basis of these data (Supplementary Figures S1–S9), we postulated that 1 was the ester hydrolysis product of 4.
Borrelidin K (2) was purified as a yellow oil and its molecular formula was determined to be C29H47NO7 based on the analysis of negative ion HR-ESI-MS ([M – H]– calcd. 520.3264, C29H46NO7–) (Supplementary Figure S10). The NMR data (Supplementary Figures S11–S14) of 2 were analogous to those of 1 (Tables 1, 2), except for a carbon signal at δC 51.3 (Table 2 and Supplementary Figure S13), presumed to be an oxygen carbon signal. HSQC (Supplementary Figure S15) correlation of H-29 (δH 3.64, 3H) to C-29 (δC 51.3) and HMBC correlation of H-29 (δH 3.64, 3H) to C-23 (δC 177.3) further confirmed the existence of methoxy group of C-29 (Figure 4 and Supplementary Figure S16). Combined with the relevant information of COSY (Figure 4 and Supplementary Figure S17) and NOSEY spectra (Supplementary Figure S18), the structure of 2 was determined. Therefore, it was confirmed that the methyl group replaced the H atom of the C-23 carboxylic acid in compound 1 to form a methyl ester.
7-Methoxy-2,3-dimethylchromone-4-one (3) was isolated as a brown yellow powder. Its molecular formula was deduced as C12H12O3 on the basis of (+)-HR-ESI-MS analysis ([M + H]+ calcd. 205.0885, C12H13O3+) (Supplementary Figure S19). The 1H NMR (Table 3 and Supplementary Figure S20) of 5 in DMSO-d6 showed three aromatic hydrogens including H-6 (δH 7.06, d, J = 2.4 Hz), H-8 (δH 7.01, dd, J = 8.9, 2.4 Hz) and H-9 (δH 7.91, d, J = 8.9 Hz), and three methyl protons including H-11 (δH 1.93, s), H-12 (δH 2.40, s), and H-13 (δH 3.88, s). 13C NMR data showed (Table 3 and Supplementary Figures S21, S22) six quaternary carbons, including one carbonyl carbons C-1 (δC 176.4), two double-bond carbons C-2 (δC 162.2), and C-3 (δC 116.2), and three aromatic carbons C-7 (δC 163.8), C-10 (δC 157.6), and C-5 (δC 116.3). Moreover, the 13C NMR data revealed the presence of one methoxy group C-13 (δC 56.5) and three aromatic tertiary carbons including C-9 (δC 127.0), C-8 (δC 114.7), and C-6 (δC 100.6). Correlations of H-9/C-1, C-6, C-7, C-8, and C-10, H-8/C-5, C-6, and C-10, H-13/C-7, and H-11/C-1 were observed in HMBC spectrum (Figure 5 and Supplementary Figure S23). Supported by other NMR data showed in Table 3, and relevant HSQC (Supplementary Figure S24) and COSY (Supplementary Figure S25) spectra data, the structure of 3 was elucidated as a new natural product, 7-methoxy-2,3-dimethylchromone-4-one.
Compound 4 was definitively identified as previously reported borrelidin on the basis of HR-ESI-MS, 1H NMR, 13C NMR, optical rotation and comparisons with previously reported data (Liu et al., 2012).
Borrelidin F (5) was also analyzed and identified comparing with the data of HR-ESI-MS, 1H NMR, 13C NMR and optical rotation provided in the reported literature (Sun et al., 2018).
Antimicrobial Screening
The antibacterial activities assay revealed that compounds 1 and 2 exhibited potent activity against methicillin-resistant S. aureus with the MICs of 0.195 and 1.563 μg/mL, respectively (Table 4). Compound 1 showed stronger activity than ciprofloxacin while 4 and 5 were inactive, indicating that the cleavage of the ester bond of the macrolides enhanced the antibacterial activity. It could be supposed that co-culture activated the silencing metabolic potential of the actinomycete and produced more potent metabolites of antibacterial activity against microorganism, such as fungal attack.
Formation Mechanism Inference
Based on the structural characteristics of 1, 2 and 4, combined with literature reports (Uyama et al., 1995; Kobayashi, 2006), we
proposed a plausible biosynthesis pathway for new compounds 1 and 2 (Figure 6). Compound 4 may be hydrolyzed to produce 1 by the lipase catalyst due to the mutual stimulation-inducing effect during the co-culture process of the actinomycete S. rochei MB037 and the fungus R. similis 35. Meanwhile, compound 2 was obtained when the C-23 carboxyl group of 1 was
methyl esterification. Consequently, it could be concluded that compounds 1 and 2 were derived from the actinomycete S. rochei MB037.
To investigate the cause for the enhancement of the production of compound 3, we measured the pH value during the growths of pure and co-cultivation firstly. It was found that the pH value of the pure culture medium of the actinomycete began to decrease on the 2nd day (Figure 7), indicating that the actinomycete began to produce secondary metabolites. Subsequently, the fungus and actinomycete were cultured separately for 3 days and then sterilized. The inactivated cells of fungus or actinomycete were co-cultured with actinomycete or fungus for 3 days (Figure 8). As a result, it was found that when the fungus R. similis 35 was co-cultured with the sterilized actinomycete S. rochei MB037, compound 3 was still obtained with higher yield than pure culture (Figure 8D). It could be speculated that the secondary metabolites from actinomycete S. rochei MB037 stimulated the fungus R. similis 35 to produce 1.
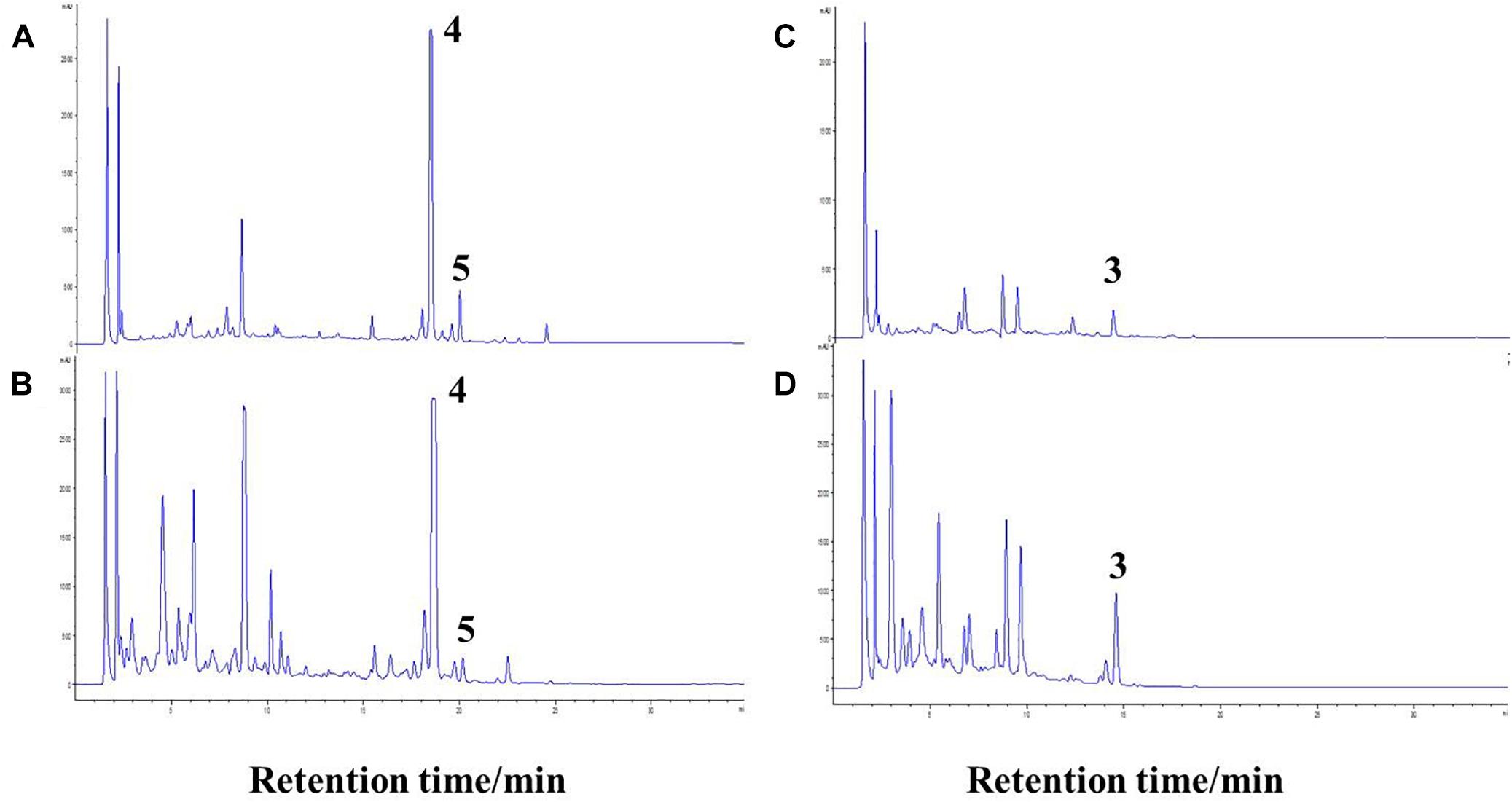
Figure 8. Comparison of pure culture and co-culture. (A) Culture of S. rochei MB037; (B) co-culture of S. rochei MB037 and R. similis 35; (C) culture of R. similis 35; (D) co-culture of R. similis 35 and S. rochei MB037.
Discussion
The fungus R. similis 35 was selected as the co-culture partner with S. rochei MB037 due to the stable emerging peaks in HPLC profiles of the co-cultures broth. 7-methoxy-2,3-dimethylchromone-4-one (3) showed a weak antibacterial activity against P. aeruginosa and S. aureus, therefore, the increased yield of compound 3 by fungus R. similis 35 may aim to inhibit the growth of S. rochei MB037. It is common for strains to compete with each other to obtain yield-increasing products in co-culture (Zuck et al., 2011; Sung et al., 2017). Co-culture is an effective method for inducing novel secondary metabolites from two interacted microbial strains. Recent studies revealed that many novel natural products were produced only from the interaction of two microbes (Scherlach and Hertweck, 2009; Hoshino et al., 2015; Wu et al., 2015). For example, the co-culture of two plant beneficial microbes Trichoderma harzianum M10 and Talaromyces pinophilus F36CF produced a novel harziaphilic acid (Vinale et al., 2017). From the perspective of microbial ecology, it is hypothesized that the production of borrelidin derivatives including two new compounds (1 and 2) and the increased yield of 7-methoxy-2,3-dimethylchromone-4-one (3) may be caused by the mutual competition for nutrition or space in the co-culture of these two strains.
Genomic sequencing has demonstrated that a large number of putative biosynthetic gene clusters encoding for secondary metabolites in many microorganisms are silent under classical cultivation conditions (Nett et al., 2009; Winter et al., 2011). A distinct fungal-bacterial interaction leads to the specific activation of fungal secondary metabolism genes, which has been demonstrated at the molecular level by microarray analyses, full-genome arrays, Northern blot, and quantitative RT-PCR analyses (Schroeckh et al., 2009). Rhodococcus erythropolis and Corynebacterium glutamicum were proved to change the biosynthesis of Streptomyces to produce new secondary metabolites (Onaka et al., 2011). Studies using chemical inhibitors disclosed that the activity of chromatin remodelers was the main factor for the interaction between S. rapamycinicus and A. nidulans to produce extra products (Nützmann et al., 2011). We speculated that compounds 1 and 2 were synthesized by the same biosynthetic gene clusters responsible for the biosynthesis of borrelidin according to the structure similarity. Compounds 1 and 2 were only produced by S. rochei MB037 in co-culture condition indicating that the silent hydrolytic enzyme genes for hydrolyzing lactone in borrelidin could be activated by the co-culture of actinomycete S. rochei MB037 and fungus R. similis 35. The interaction between these two strains probably activate the expression of hydrolytic enzyme genes, harbored in actinomycete S. rochei MB037, and then led to hydrolytic action of lactone of borrelidin to generate compound 1. Compound 2 is probably synthesized from compound 1 by methylation reaction since the only difference between 1 and 2 is the methyl ester group. During the extraction and purification processes, both of them were clearly detected in freshly prepared ethyl acetate extracts. Indeed, even if compound 1 was dissolved in methanol and stored at 28°C for 1 week, compound 2 was not detected in the solution. Therefore, compound 2 should be considered as a true natural product.
In the antibacterial bioassay, both compounds 1 and 2 exhibited stronger activities against S. aureus than 4 and 5. Notably, the MIC value of compound 1 was 0.195 μg/ml, stronger than the positive control ciprofloxacin, indicating that 1 should be a potential antibacterial agent. It seems that the cleavage of the ester bond of the macrolide in borrelidin could enhance its antibacterial activity. Compounds 1 and 2 become the long-chain unsaturated fatty acid after the cleavage of the ester bond in borrelidin. Previous studies indicated that long-chain unsaturated fatty acid could exhibit strong activity against S. aureus by inhibiting the enoyl-acyl carrier protein reductase (FabI), which was the essential component in bacterial fatty acid synthesis (Zheng et al., 2005). However, the esterification of unsaturated fatty acid results in the loss of FabI-inhibitory activity, which is consistent with our results since compounds 4 and 5 exhibited no activity. The antibacterial activity of unsaturated fatty acid was very weak to the Gram negative bacteria due to the impermeability of their outer membrane. Consistently, compounds 1 and 2 showed weak antibacterial activity against E. coli and P. aeruginosa. Although Bacillus subtilis was the Gram positive bacteria, it has two kinds of enoyl-acyl carrier protein reductases, FabI and FabL, which may escape the inhibition of unsaturated fatty acid by alternative enoyl-acyl carrier protein reductase in fatty acid synthesis (Kim et al., 2011). The cytotoxicity of these compounds was not conducted, but their analogs exhibited cytotoxicity to mammalian cells (Zheng et al., 2005; Wilkinson et al., 2006; Chen et al., 2012; Sun et al., 2018).
Conclusion
This study demonstrated that microbial co-culture was an effective approach to explore the natural products. Based on a series of screening of co-cultivation of marine-derived microbes, a co-cultured combination of a sponge-derived actinomycete S. rochei MB037 and a gorgonian-derived fungus R. similis 35 was proved to induce the production of related polyketides with antibacterial activities successfully. The two new metabolites (1 and 2) produced by co-culture of marine-derived actinomycete and fungus represent the nitrogen-containing fatty acids which are rare in the nature. Future investigation should be focused on unveiling the mechanisms of action in molecular biology of the new produced compounds.
Author Contributions
MY performed the experiments, data analyses, and wrote the draft manuscript. YL, LL, and CS assisted the bioactivity analysis and revised the manuscript. SB revised the manuscript. ZL and CW supervised the whole work and edited the manuscript. All authors reviewed and approved the final manuscript.
Funding
This work was supported by National Natural Science Foundation of China (Grant Nos. 81673350 and 41830535), National Key Research and Development Program of China (Grant No. 2018YFC030900), Shanghai Jiao Tong University Open Fund for State Key Laboratory of Microbial Metabolism (Grant No. MMLKF16-09), and Taishan Scholars Program, China.
Conflict of Interest Statement
The authors declare that the research was conducted in the absence of any commercial or financial relationships that could be construed as a potential conflict of interest.
Acknowledgments
The authors thank Binbin Gu (Shanghai Jiao Tong University), Hao Wen (Ocean University of China), Xuemei Hou (Ocean University of China), Fei Cao (Hebei University), and Xiaocao Miao (Shanghai Jiao Tong University) for their technical supports.
Supplementary Material
The Supplementary Material for this article can be found online at: https://www.frontiersin.org/articles/10.3389/fmicb.2019.00915/full#supplementary-material
References
Anderson, B. F., Herlt, A. J., Rickards, R. W., and Robertson, G. B. (1989). Crystal and molecular structures of two isomorphous solvates of the macrolide antibiotic borrelidin: absolute configuration determination by incorporation of a chiral solvent in the crystal lattice. Aust. J. Chem. 42, 717–730. doi: 10.1071/ch9890717
Appendino, G., Gibbons, S., Giana, A., Pagani, A., Grassi, G., Stavri, M., et al. (2008). Antibacterial cannabinoids from Cannabis sativa: a structure-activity study. J. Nat. Prod. 71, 1427–1430. doi: 10.1021/np8002673
Chen, Y., Liu, H. R., Liu, H. S., Cheng, M., Xia, P., Qian, K., et al. (2012). Antitumor agents 292. Design, synthesis and pharmacological study of S- and O-substituted 7-mercapto- or hydroxy-coumarins and chromones as potent cytotoxic agents. Eur. J. Med. Chem. 49, 74–85. doi: 10.1016/j.ejmech.2011.12.025
Hoshino, S., Wakimoto, T., Onaka, H., and Abe, I. (2015). Chojalactones A–C, cytotoxic butanolides isolated from Streptomyces sp. cultivated with mycolic acid containing bacterium. Org. Lett. 17, 1501–1504. doi: 10.1021/acs.orglett.5b00385
Ishiyama, A., Iwatsuki, M., Namatame, M., Nishihara-Tsukashima, A., Sunazuka, T., Takahashi, Y., et al. (2011). Borrelidin, a potent antimalarial: stage-specific inhibition profile of synchronized cultures of Plasmodium falciparum. J. Antibiot. 64, 381–384. doi: 10.1038/ja.2011.6
Karuppiah, V., Li, Y., Sun, W., Feng, G., and Li, Z. (2015). Functional gene-based discovery of phenazines from the actinobacteria associated with marine sponges in the South China Sea. Appl. Microbiol. Biotechnol. 99, 5939–5950. doi: 10.1007/s00253-015-6547-8
Kim, K. H., Ha, B. H., Kim, S. J., Hong, S. K., Hwang, K. Y., and Kim, E. E. (2011). Crystal structures of enoyl-ACP reductases I (FabI) and III (FabL) from B. subtilis. J. Mol. Biol. 406, 403–415. doi: 10.1016/j.jmb.2010.12.003
Kobayashi, S. (2006). Enzymatic ring-opening polymerization of lactones by lipase catalyst: mechanistic aspects. Macromol. Symp. 240, 178–185. doi: 10.1002/masy.200650822
Li, Y. X., Zhang, F. L., Banakar, S., and Li, Z. Y. (2018). Comprehensive optimization of precursor-directed production of BC194 by Streptomyces rochei MB037 derived from the marine sponge Dysidea arenaria. Appl. Microbiol. Biotechnol. 102, 7865–7875. doi: 10.1007/s00253-018-9237-5
Liu, C. X., Zhang, J., Wang, X. J., Qian, P. T., Wang, J. D., Gao, Y. M., et al. (2012). Antifungal activity of borrelidin produced by a Streptomyces strain isolated from soybean. J. Agric. Food Chem. 60, 1251–1257. doi: 10.1021/jf2044982
Mirando, A. C., Fang, P., Williams, T. F., Baldor, L. C., Howe, A. K., Ebert, A. M., et al. (2015). Aminoacyl-tRNA synthetase dependent angiogenesis revealed by a bioengineered macrolide inhibitor. Sci. Rep. 5, 13160–13176. doi: 10.1038/srep13160
Nett, M., Ikeda, H., and Moore, B. S. (2009). Genomic basis for natural product biosynthetic diversity in the actinomycetes. Nat. Prod. Rep. 26, 1362–1384. doi: 10.1039/B817069J
Nützmann, H. W., Reyes-Dominguez, Y., Scherlach, K., Schroeckh, V., Horn, F., Gacek, A., et al. (2011). Bacteria-induced natural product formation in the fungus Aspergillus nidulans requires Saga/Ada-mediated histone acetylation. Proc. Nati. Acad. Sci. U.S.A. 108, 14282–14287. doi: 10.1073/pnas.1103523108
Onaka, H., Mori, Y., Igarashi, Y., and Furumai, T. (2011). Mycolic acid-containing bacteria induce natural-product biosynthesis in Streptomyces species. Appl. Environ. Microb. 77, 400–406. doi: 10.1128/AEM.01337-10
Scherlach, K., and Hertweck, C. (2009). Triggering cryptic natural product biosynthesis in microorganisms. Org. Biomol. Chem. 7, 1753–1760. doi: 10.1039/B821578B
Schroeckh, V., Scherlach, K., Nützmann, H. W., Shelest, E., Schmidt-Heck, W., Schuemann, J., et al. (2009). Intimate bacterial–fungal interaction triggers biosynthesis of archetypal polyketides in Aspergillus nidulans. Proc. Nati. Acad. Sci. U.S.A. 106, 14558–14563. doi: 10.1073/pnas.0901870106
Sun, J. B., Shao, J. L., Sun, C. L., Song, Y. X., Li, Q. L., Lu, L. C., et al. (2018). Borrelidins F–I, cytotoxic and cell migration inhibiting agents from mangrove-derived Streptomyces rochei SCSIO ZJ89. Bioorgan. Med. Chem. 26, 1488–1494. doi: 10.1016/j.bmc.2018.01.010
Sung, A. A., Gromek, S. M., and Balunas, M. J. (2017). Upregulation and identification of antibiotic activity of a marine-derived Streptomyces sp. via co-cultures with human pathogens. Mar. Drugs 15, 250–261. doi: 10.3390/md15080250
Uyama, H., Takeya, K., and Kobayashi, S. (1995). Enzymatic ring-opening polymerization of lactones to polyesters by lipase catalyst: unusually high reactivity of macrolides. Bull. Chem. Soc. Jpn. 68, 56–61. doi: 10.1246/bcsj.68.56
Vinale, F., Nicoletti, R., Borrelli, F., Mangoni, A., Parisi, O. A., Marra, R., et al. (2017). Co-culture of plant beneficial microbes as source of bioactive metabolites. Sci. Rep. 7, 14330–14341. doi: 10.1038/s41598-017-14569-5
Wagenaar, M. M., Corwin, J., Strobel, G., and Clardy, J. (2000). Three new cytochalasins produced by an endophytic fungus in the genus Rhinocladiella. J. Nat. Prod. 63, 1692–1695. doi: 10.1021/np0002942
Wilkinson, B., Gregory, M. A., Moss, S. J., Carletti, I., Sheridan, R. M., Kaja, A., et al. (2006). Separation of anti-angiogenic and cytotoxic activities of borrelidin by modification at the C17 side chain. Bioorg. Med. Chem. Lett. 16, 5814–5817. doi: 10.1016/j.bmcl.2006.08.073
Winter, J. M., Behnken, S., and Hertweck, C. (2011). Genomics-inspired discovery of natural products. Curr. Opin. Chem. Biol. 15, 22–31. doi: 10.1016/j.cbpa.2010.10.020
Wu, C., Zacchetti, B., Ram, A. F. J., van Wezel, G. P., Claessen, D., and Hae Choi, Y. (2015). Expanding the chemical space for natural products by Aspergillus-Streptomyces co-cultivation and biotransformation. Sci. Rep. 5, 10868–10877. doi: 10.1038/srep10868
Zhang, D. B., Yang, Z. D., Xue, P. H., Zhi, K. K., Shi, Y., and Wang, M. G. (2014). Two new cyclic dipeptides from Rhinocladiella sp. lgt-3, a fungal endophyte isolated from Tripterygiun wilfordii Hook. Nat. Prod. Res. 28, 1760–1764. doi: 10.1080/14786419.2014.945176
Zheng, C. J., Yoo, J. S., Lee, T. G., Cho, H. Y., Kim, Y. H., and Kim, W. G. (2005). Fatty acid synthesis is a target for antibacterial activity of unsaturated fatty acids. FEBS Lett. 579, 5157–5162. doi: 10.1084/jem.193.3.393
Keywords: co-culture, actinomycete, fungus, borrelidin, antibacterial activity
Citation: Yu M, Li Y, Banakar SP, Liu L, Shao C, Li Z and Wang C (2019) New Metabolites From the Co-culture of Marine-Derived Actinomycete Streptomyces rochei MB037 and Fungus Rhinocladiella similis 35. Front. Microbiol. 10:915. doi: 10.3389/fmicb.2019.00915
Received: 15 November 2018; Accepted: 10 April 2019;
Published: 07 May 2019.
Edited by:
Bey Hing Goh, Monash University Malaysia, MalaysiaReviewed by:
Ayanabha Chakraborti, University of Alabama at Birmingham, United StatesPhan Chia Wei, University of Malaya, Malaysia
Kai-Leng Tan, Guangdong University of Technology, China
Copyright © 2019 Yu, Li, Banakar, Liu, Shao, Li and Wang. This is an open-access article distributed under the terms of the Creative Commons Attribution License (CC BY). The use, distribution or reproduction in other forums is permitted, provided the original author(s) and the copyright owner(s) are credited and that the original publication in this journal is cited, in accordance with accepted academic practice. No use, distribution or reproduction is permitted which does not comply with these terms.
*Correspondence: Zhiyong Li, enlsaUBzanR1LmVkdS5jbg== Changyun Wang, Y2hhbmd5dW5Ab3VjLmVkdS5jbg==