- 1Laboratory of Protein Engineering and Bioactive Molecules (LIP-MB), National Institute of Applied Sciences and Technology, University of Carthage, Tunis, Tunisia
- 2Laboratoire de Microbiologie Signaux et Microenvironnement (LMSM) EA 4312, Normandie Université – Université de Rouen, Évreux, France
- 3SymbioPharm GmbH, Herborn, Germany
- 4Institute of Medical Microbiology, German Centre for Infection Research, Justus-Liebig-University Giessen, Giessen, Germany
- 5UNICAEN, U2RM, Normandie Université, Caen, France
Lactic acid bacteria (LAB) strains OB14 and OB15 were isolated from traditional Tunisian fermented dairy products, Testouri cheese and Rigouta, respectively. They were identified as Enterococcus faecalis by the MALDI TOF-MS (matrix assisted laser desorption-ionization time of flight mass spectrometry) biotyper system and molecular assays (species-specific PCR). These new isolates were evaluated for probiotic properties, compared to E. faecalis Symbioflor 1 clone DSM 16431, as reference. The bacteria were found to be tolerant to the harsh conditions of the gastrointestinal tract (acidity and bile salt). They were low to moderate biofilm producers, can adhere to Caco-2/TC7 intestinal cells and strengthen the intestinal barrier through the increase of the transepithelial electrical resistance (TER). Susceptibility to ampicillin, vancomycin, gentamicin and erythromycin has been tested using the broth microdilutions method. The results demonstrated that E. faecalis OB14 and OB15 were sensitive to the clinically important ampicillin (MIC = 1 μg/mL) and vancomycin (MIC = 2 μg/mL) antibiotics. However, Whole Genome Sequencing (WGS) showed the presence of tetracycline resistance and cytolysin genes in E. faecalis OB14, and this led to high mortality of Galleria Mellonella larvae in the virulence test. Hierarchical cluster analysis by MALDI TOF-MS biotyper showed that E. faecalis OB15 was closely related to the E. faecalis Symbioflor 1 probiotic strain than to OB14, and this has been confirmed by WGS using the average nucleotide identity (ANI) and Genome-to-Genome Hybridization similarity methods. According to these results, E. faecalis OB15 seems to be reliable for future development as probiotic, in food or feed industry.
Introduction
Enterococci are normal inhabitants of the gastrointestinal tract of humans and animals. These bacteria are ubiquitous in nature and have been used in the food industry as probiotics (Franz et al., 2003), or as starter culture in manufacturing cheese in high salt content and low pH (Wessels et al., 1991). They contribute to the characteristic taste of traditional Mediterranean cheeses and can be present in other fermented foods such as sausages, olives, and vegetables. Some strains have been proposed for food preservation, as they produce bacteriocins (enterocins), antimicrobial peptides with the potential to inhibit the growth of food-borne pathogenic and spoilage bacteria (Khan et al., 2010; Franz et al., 2011). Recently, bacteriocins have been suggested as a new probiotic trait when selecting beneficial microbes. Most of the enterocins belong to Class-II bacteriocins, including the pediocin family of enterocins highly active against Listeria spp (Ness et al., 2014). Some enterococci strains produce simultaneously several bacteriocins, which give them a competitive advantage toward other microbes for colonization and niche control (Hanchi et al., 2018).
While the genus Enterococcus includes many species, only few have been studied to be used as probiotics, such as E. faecalis, E. faecium, E. lactis and more recently E. hirae (Adnan et al., 2017) and E. durans (Li et al., 2018). For example, some Enterococcus strains are currently in use as therapeutic treatments, marketed as Cylactins (Hoffmann-La Roche, Basel, Switzerland), Fargo 688s (Quest International, Naarden, Netherlands), ECOFLOR (Walthers Health Care, DenHaag, Netherlands), or Symbioflor 1 (SymbioPharm, Herborn, Germany), to alleviate the symptoms of irritable bowel syndrome and recurrent chronic sinusitis or bronchitis (Franz et al., 2003; Foulquié Moreno et al., 2006). The probiotic E. faecalis Symbioflor 1 clone DSM 16431, originally isolated in the 1950s from the stool sample of a healthy human adult, has been used as a probiotic for more than 50 years without any report or documentation of any infection or adverse effect. The complete genome sequence of this strain has been determined and several toxicological studies showed that the strain can be administred safely to humans (Domann et al., 2007; Christoffersen et al., 2012; Fritzenwanker et al., 2013). However, according to FDA, probiotic shall be used carefully by populations at risk, for example those with immune compromise, premature infants, patients suffering from various pathologies…
On the other hand, some enterococci are known to be opportunistic pathogens and are a prevalent cause of nosocomial infections. These bacteria have been implicated in bacteraemia, endocarditis, urinary tract infections or other infections. Recently, a role of E. faecalis in pancreatic and colorectal cancers has also been suggested but this remains controversial (De Almeida et al., 2018; Maekawa et al., 2018). One factor which contributes to the pathogenicity of E. faecalis, is the resistance of many strains to a broad range of antibiotics (Franz et al., 2003). This antibiotic resistance is due to intrinsic and/or acquired genes located on the chromosome, or on mobile elements including plasmids and transposons. Vancomycin-resistant enterococci pose major problems in treating human clinical infections, as this drug is used as last resort treatment for multiple antibiotic resistant enterococci (Ogier and Serror, 2008). Moreover, the transfer of vancomycin-resistance from E. faecalis to other pathogens such as methicillin-resistant Staphylococcus aureus has been reported in the last decade (Palmer et al., 2010). Virulence determinants also contribute to fitness and persistence of enterococci in nosocomial infections. Among the virulence factors that can be found in E. faecalis, some are sporadically detected in dairy isolates (Popović et al., 2018), like the aggregation substance (asa1), gelatinase (gelE), collagen adhesin (ace), enterococcal surface protein (esp) and cytolysin (cylA). For these reasons, Enterococcus species have not the GRAS status or recommendations for QPS list (EFSA Panel on Biological Hazards, Ricci et al., 2017), and the absence of transferable antibiotic resistance genes and/or potential virulence determinants should be investigated for each new potential probiotic strain intended to be proposed for food or pharmaceutical industry.
North African countries have an ancient tradition in food technology. Traditional dairy products and cheese remain very popular in Tunisia, consumed at breakfast, after lunch and at dinner. These traditional foods are still prepared at the household level and marketed through informal routes. The composition of these products is often not well characterized, varying with regions and local markets, sometimes using raw material of poor microbiological quality, or lacking hygiene conditions (Benkerroum, 2013). In order to improve the safety and quality of these foods, to promote the trading of such traditional foods internationally, and to be competitive in local markets, there is an urgent need for North African countries to develop and standardize new starters or probiotic strains in conformity with the Codex Alimentarius standards, WTO, FAO and EFSA legislations.
In this context, the aim of this work was to evaluate the probiotic potential and safety of E. faecalis OB14 and OB15, two strains isolated from traditional fermented Tunisian dairy products (Testouri cheese and Rigouta), in comparison to the known probiotic E. faecalis Symbioflor 1 DSM 16431.
Materials and Methods
Sampling and Isolation of Lactic Acid Bacteria (LAB)
A total of five samples of Tunisian traditional fermented dairy products (Testouri cheese, Rigouta, yogurt, Leben, Rayeb) were collected from local markets in different cities. 10 g or 10 mL of each dairy sample were suspended aseptically in 90 mL of sodium citrate solution (pH 7.0) and homogenized by vortexing. Each suspension was then serially diluted, in NaCl 0.9%, plated on Man-Rogosa-Sharpe (MRS) medium and incubated for 48 h at 37°C under anaerobic conditions to isolate Lactic Acid Bacteria (LAB). Single isolated colonies were picked and purified by repeated streaking, then grown overnight in MRS broth at 37°C and stored at -20°C in 20% (w/v) glycerol.
Identification of OB14 and OB15
The isolates of LAB were presumptively identified by phenotypic analysis (colony morphology, Gram staining and catalase assay), and then by the simultaneous combination of the MALDI-TOF MS Biotyper system and species-specific PCR assay.
MALDI-TOF MS Biotyper
Bacteria were identified by analysis of the total proteome using an Autoflex III Matrix-Assisted Laser Desorption/ Ionization-Time-Of-Flight mass spectrometer (MALDI-TOF MS; Bruker, Marcy-l’Etoile, France) coupled to the MALDI-Biotyper 3.1 system, as previously described (Hillion et al., 2013; Zommiti et al., 2018). Formic acid was used on the bacterial spots as a quick extraction procedure (Haigh et al., 2011), then the MALDI target plate was introduced in the mass spectrometer for measurement and data acquisition. For each sample, 600 spectra were pooled, and the generated spectra were compared with the MALDI-Biotyper 3.1 database. A score was calculated based on the matching between the reference spectrum and the unknown spectrum. A score of ≥1.7 allows genus identification, and a score of ≥2.0 is the set threshold for identification at the species level (Sogawa et al., 2011). Dendrograms were generated from the minimal spanning tree data set (Elbehiry et al., 2017). For this, the main spectra of the Maldi Biotyper taxonomy were compared with the spectra resulting in a matrix of cross-wise identification scores. This matrix was applied to estimate the distance level of the bacteria for each pair of main spectra.
Species-Specific PCR Assay
Genomic DNA was extracted according to Delley et al. (1990). E. faecalis OB14 and OB15 were identified using the E. faecalis species-specific primers EflF1 (5′-ACCAATGTTGGCACAAGAAA-3′) and EflR1 (5′-TTTCGTTCAAG CGGTCTTTT-3′) as described by Park et al. (2017) with slight modifications. The PCR reactions were performed with the Thermo Scientific PCR Master Mix, in a total volume of 50 μL, using approximately 1 μM of forward and reverse primers and 10 pg–1 μg of DNA template. Amplification conditions were as follows: a first denaturation step at 94°C for 5 min, 30 cycles of denaturation at 94°C for 1 min, annealing at 56°C for 1 min, extension at 72°C for 1 min, followed by a final elongation step at 72°C for 10 min. The PCR products were analyzed on 1% agarose gel electrophoresis, stained with SYBR Safe DNA gel stain and examined under UV light.
E. faecalis OB14 and OB15 isolates, both correctly identified by MALDI-TOF MS Biotyper and species-specific PCR, were selected for the further experiments. Unless otherwise stated, the bacteria were routinely precultivated and cultivated in MRS broth at 37°C.
Acid and Bile Salts Tolerance
For the acid tolerance test, the method described by Ramos et al. (2013) was utilized including some modifications. To simulate the acidic conditions of the gastrointestinal tract, MRS was adjusted to pH 3.0 with 1 N HCl (Azat et al., 2016). Bacterial cells from fresh overnight culture were centrifuged (10,000 ×g, 10 min), washed twice with Phosphate Buffer Saline (PBS), and resuspended to approximately 108 bacteria/mL in the MRS broth pH 3.0. One mL of culture was taken immediately from each tube, diluted, and plated on MRS agar. Similarly, one mL was taken after 3 h of incubation at 37°C, reflecting the time spent in the human stomach. The colony forming units (CFU) were then counted, expressed as log 10 values of colony-forming units per milliliter (CFU/mL) and the bacterial survival rate (SR) was calculated.
The bile salts tolerance was tested as previously described by Anandharaj et al. (2015) with slight modifications. Freshly prepared overnight cultures were harvested by centrifugation and resuspended in MRS broth added with 0.3% bile salts (Oxgall, Sigma-Aldrich, France) followed by incubation at 37°C for 4 h, simulating the human intestine transit time. Aliquots were withdrawn at 0 and 4 h interval, diluted, and plated on MRS agar. Bile salts tolerance was assessed in terms of viable colony counts after aforesaid incubation at 37°C. The SR was calculated as described above for acid tolerance.
Hydrophobicity and Autoaggregation
Hydrophobicity was estimated as previously reported by Rosenberg et al. (1980). Overnight cultures were centrifuged and resuspended at 108 bacteria/mL. The cell suspensions (3 mL) were mixed with xylene (1 mL) for 2 min. After 1 h of incubation at room temperature, the aqueous phases were transferred to a spectrophotometry cuvette and the absorbance at 600 nm was measured. Hydrophobicity (%) was calculated as follows: H% = [(A0 - A)/A0] × 100, where A0 and A are absorbance values found before and after solvent extraction.
Autoaggregation test was performed as described by Del Re et al. (2000) and Collado et al. (2008) including slight modifications. Overnight cultures were centrifuged (10,000 ×g, 10 min), washed twice with PBS, and resuspended in this buffer to obtain a viable cell counts of approximately 108 CFU/mL. Bacterial suspensions (4 mL) were vortexed for 15 s and incubated at room temperature. At time 0 and 24 h after incubation without mixing, 1 mL of the upper suspension was taken to measure the absorbance (A) at 600 nm. The autoaggregation was then calculated as follows: autoaggregation (%) = [1 - (A24h/A0) × 100].
Biofilm Formation
Biofilm production on abiotic surfaces was studied in microtiter plates as performed by Bujnakova et al. (2014) and Gómez et al. (2016) with minor modifications. After 24 h incubation at 37°C, the adherent biofilms were gently washed, fixed, dried at room temperature, and stained with 0.1% crystal violet. Excess stain was rinsed off by placing the microtiter plates under running tap water. Then, the dye bound to adherent cells was removed with glacial acetic acid and the absorbance of the resulting solutions was measured at 595 nm. Biofilm formation was expressed using ODC cut-off values as previously described (Extremina et al., 2011; Diaz et al., 2016), and the strains were then classified as belonging to one of the following categories: ODc < OD ≤ 2 × ODc = weak biofilm producer, 2 × ODc < OD ≤ 4 × ODc = moderate biofilm producer, and OD > 4 × ODc = strong biofilm producer.
Gelatinase Assay
The gelatinase activity was investigated using Nutrient medium containing 3% (w/v) gelatin according to Ben Braïek et al. (2018). E. faecalis OG1RF was used as reference for quality control.
Caco-2/TC7 Culture
Human enterocyte-like Caco-2/TC7 cell line was routinely grown in Dulbecco’s Modified Eagle’s Medium (DMEM, Invitrogen, France), supplemented with 15% heat-inactivated fetal bovine serum and Penicillin/Streptomycin, at 37°C in a humidified incubator with 5% CO2-95% air atmosphere. The medium was changed daily, and the cells were passaged and subcultured each week. For adhesion assay, cytotoxicity test and interleukine-10 (IL-10) quantification, the cells were seeded in 24-well tissue culture plates. For transepithelial electrical resistance (TER) measurement, the cells were grown on insert (3 μm pore size) for 21 days to ensure epithelial differentiation.
Adhesion
Overnight bacterial cultures were harvested by centrifugation (10,000 ×g, 10 min), resuspended in cell culture medium without serum and antibiotic to achieve a concentration of 108 bacteria/mL, and then applied on confluent Caco-2/TC7 monolayers. After 4 h of incubation at 37°C, in 5% CO2-95% air atmosphere, monolayers were washed with sterile pre-warmed PBS to remove non-adherent bacteria and lysed by incubation for 15 min with 0.1% Triton X-100. The lysates were then diluted and plated onto de Man, Rogosa and Sharpe (MRS) agar to determine the number of adherent bacteria.
Hemolytic Activity and Cytotoxicity
Hemolytic activity was determined on Columbia agar containing 5% of sheep blood, by streaking the bacteria on the surface of the solid medium. The plates were then incubated at 37°C for 48 h under aerobiosis conditions and examined for β- (positive) or γ- (negative) hemolysis as previously indicated (Semedo et al., 2003).
The cytotoxicity of the E. faecalis strains was tested on Caco-2/TC7 cells following overnight incubation with the bacteria, using the Neutral Red (NR) uptake test and an enzymatic assay (Cytotox 96 Promega, France), as described by Messaoudi et al. (2012) and Biaggini et al. (2017), respectively.
Quantification of the IL-10 Anti-inflammatory Cytokine
The level of interleukine 10 (IL-10) in the supernatants of Caco-2/TC7 cells was measured using the Human IL-10 ELISA Kit (Thermo Scientific), after overnight incubation of the cells with approximately 108 bacteria/mL.
Transepithelial Electrical Resistance
The effect of the bacteria on the TER of Caco-2/TC7 monolayers grown on inserts was measured using the Millicell Electrical Resistance system (Millipore, Bedford, MA, United States). The day before experiment, the inserts were washed with PBS to remove traces of antibiotics and the cell media was changed to the original media without antibiotics and serum. TER was measured before the addition of the bacterial suspension at 108 bacteria/mL and during 16 h. TER values were expressed as percentages of the initial level measured in each insert.
Antibiotic Susceptibility Testing
The antibiotic susceptibility of the E. faecalis strains was performed using the broth microdilutions method. The antibiotics selected were ampicillin, vancomycin, gentamicin and erythromycin. Overnight cultures of bacteria were inoculated at 105 CFU/mL in Mueller-Hinton broth supplemented with various concentrations of the antibiotics (0.5, 1, 2, 4, 8, 16, 32, 64, 128, 256, and 516 μg/mL). The MIC for each antibiotic was confirmed as the lowest concentration at which no growth was observed following a 24 h incubation period at 37°C. The susceptibility/resistance were determined according to the microbial cut-off values recommended by the European Food Safety Authority [EFSA], 2012).
Virulence Factors
Total DNA of E. faecalis OB14 and OB15, extracted previously for the Species-specific PCR assay, were used for PCR amplification of the following virulence determinants: aggregation substance (asa1), gelatinase (gelE), collagen adhesin (ace), enterococcal surface protein (esp) and cytolysin (cylA). PCR experiments were performed using the primer sequences and protocols described in Mannu et al. (2003), Reviriego et al. (2005), and Anderson et al. (2016).
Enterococcus faecalis V583 and E. faecalis Symbioflor 1 DSM 16431 were used as positive and/or negative control. The PCR products were analyzed on 1% agarose gel electrophoresis, stained with SYBR Safe DNA gel stain and observed under UV light.
Virulence in the Galleria mellonella Model
The E. faecalis strains used for infection of G. mellonella were grown for 24 h in M17 supplemented with 0.5% glucose (GM17). After centrifugation, bacterial cells were washed once in 0.9% NaCl and resuspended to a final OD600 nm of 1 (5.107 CFU/mL). The size of the inoculum was confirmed by numeration on solid GM17. Fifteen larvae were infected with 10 μL of a cell suspension through into the hemocoel using a microinjector (KDS100 Legacy, Fisher Scientific) with a sterilized microsyringe and incubated at 37°C. Larval surviving was monitored at 16 h, and then every hour until 24 h post-infection. E. faecalis V19 and E. faecalis Symbioflor 1 DSM 16431 were also tested under the same conditions as a virulent and non-virulent control, respectively.
Sequencing and Analysis of Whole Genome
Genomic DNA quality and quantity of E. faecalis OB14 and OB15 were examined on a 1% agarose gel electrophoresis, also using a NanoDropTM spectrophotometer and a Qubit®TM 4.0 fluorometer (Qubit® dsDNA HS assay). DNA libraries for whole genome sequencing (WGS) were constructed using NexteraTM XT DNA Library Prep Kit according to the manufacturer’s instructions (Illumina, San Diego, CA, United States). The sequencing was performed on the Illumina MiSeq platform (LMSM Evreux, Rouen Normandy University) using Reagent Kit v3 (600-cycle, Illumina, United States) to generate 2 × 250 bp paired-end reads.
Paired-end reads were trimmed using Trimmomatic v.0.36 (Bolger et al., 2014). Sequence data quality was checked using FastQC v.0.11.6.1 Assembly of paired-end reads was done de novo using Spades software package v.3.12.0 (Bankevich et al., 2012) using its in-build read error correction functionality of BayesHammer (Nikolenko et al., 2013) by setting the “carefull” option, and k-mer combination was set to 21, 33, 55, 77, 99 and 127. The obtained drafts were checked for consistency, such as the number of contigs, N50, GC% and total size of assembly using Quast v.5.0.0 (Mikheenko et al., 2018).
In addition to MALDI-TOF and specific PCR assays, confirmation of cluster analysis was achieved using WGS data with two methods: (i) average nucleotide identity (ANI) was calculated using PYANI v0.2.7 (Pritchard et al., 2016; Pritchard, 2017), and (ii) genome-to-genome distance calculator (GGDC), which is an in silico DNA-DNA hybridization method (isDDH) using a webserver at ggdc.dsmz.de/ggdc.php (Meier-Kolthoff et al., 2013). The isDDH model “formula 2” was used as recommended for draft genomes.
MLST (Multilocus sequence typing) profile of each isolate was determined from the draft genome sequences using the software package MLST_check v. 2.1.1706216-1 (Page et al., 2016)2 based on the E. faecalis PubMLST database3.
ABRicate v.0.8.7 (Seemann, 2018), a mass screening software package of contigs, for resistome and virulome coding-genes detection, was used to screen against the NCBI Bacterial Antimicrobial Resistance Reference Gene Database (NCBI BARRGD, PRJNA313047), and Virulence Factors of Pathogenic Bacteria Database (VFDB; Chen et al., 2016), respectively.
Statistical Analysis
Results are expressed as means ± standard error (SE) of three experiments done in triplicate. Analysis of statistical significance was performed with Student’s t-test and GraphPad Prism7.
Results and Discussion
Identification of the LAB Isolates OB14 and OB15
Lactic acid bacteria strains were isolated from traditional fermented Tunisian dairy products (Testouri cheese, Rigouta, yogurt, Leben, Rayeb). Two Enterococcus strains (Gram positive, catalase negative, coccus morphology), OB14 and OB15, respectively found in Testouri cheese and Rigouta from two different Tunisian regions, were selected for MALDI biotyper and species-specific PCR assays, for confirmation of the genus Enterococcus and determination of the species. The analysis by MALDI biotyper classified OB14 and OB15 isolates as E. faecalis strains with score value >2.0, which allows to identify at the species level with no doubt (Sogawa et al., 2011).
PCR amplification with E. faecalis specific primers (Park et al., 2017) confirmed results found by MALDI biotyper. As expected, a PCR product of 1,209 bp was obtained for the two isolates and for the probiotic E. faecalis Symbioflor 1 DSM 16431, corresponding to the specific amplification of the 6-aminohexanoate-cyclic-dimer hydrolase (EC 3.5.2.12) target gene of E. faecalis.
Hierarchical Cluster Analysis
Besides bacterial identification, MALDI-TOF MS allows hierarchical cluster analysis, using an algorithm to determine the relatedness between the MS spectrum of each bacteria. MS spectra of E. faecalis OB14 and OB15 were compared to the MS spectrum of the reference probiotic strain E. faecalis Symbioflor 1 DSM 16431, and to MS spectra of the E. faecalis strains available in the Biotyper library. For this, spectra were merged, and the merging patterns were then represented as dendrograms or tree structures (Figures 1A,B). Since spectra were merged by relatedness, the distance of branches on the dendrograms relates directly to the similarity of spectra and, hence, the similarity of the bacteria studied. Unexpectedly, E. faecalis OB15 was found to be closely related to Symbioflor 1 DSM 16431 than to OB14, the other Tunisian dairy isolate (Figure 1A), or to the reference strains of the Biotyper library (Figure 1B).
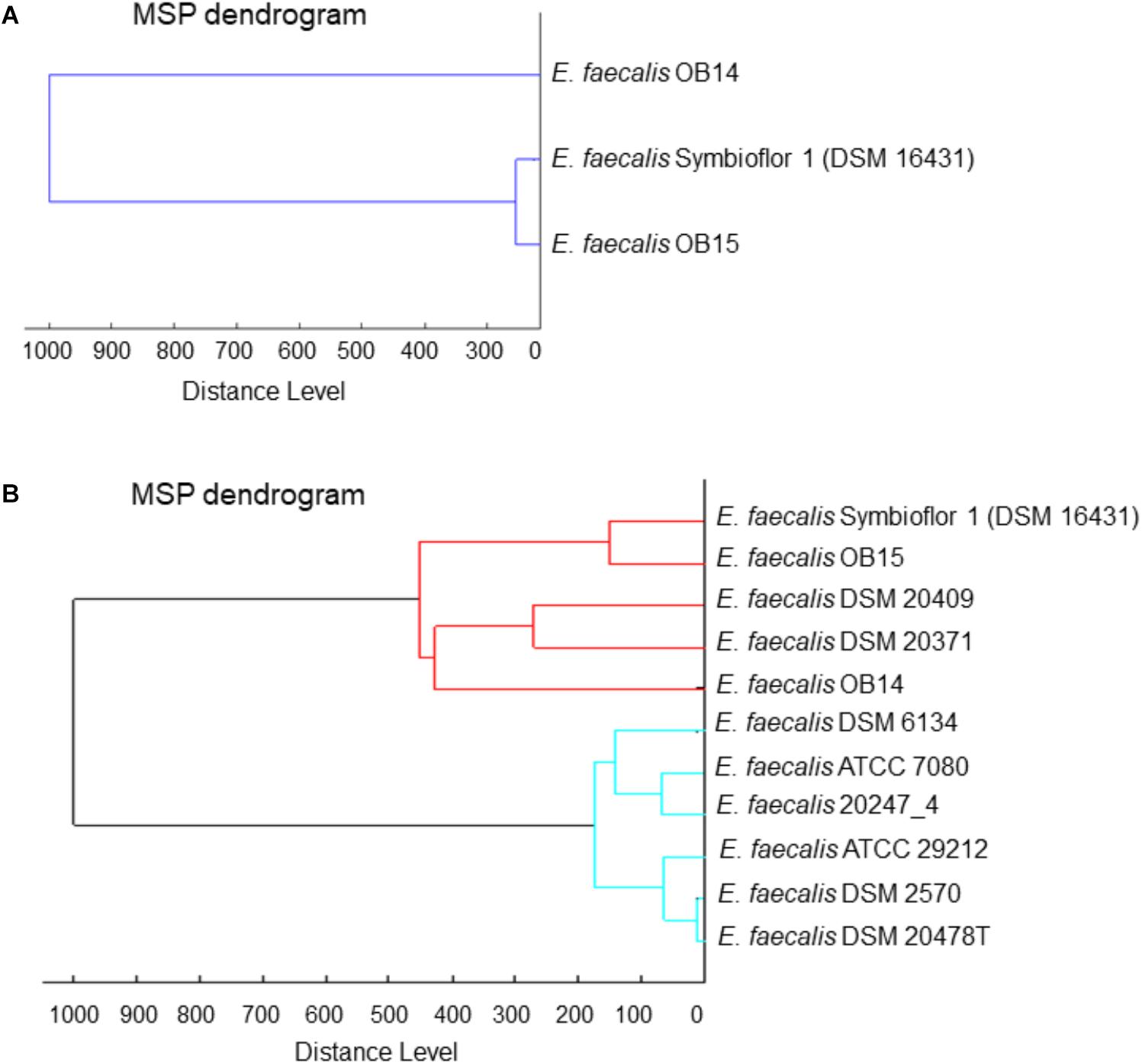
Figure 1. Dendrograms (representations of hierarchical cluster analysis) of the Tunisian dairy isolates Enterococcus faecalis OB14 and E. faecalis OB15,(A) compared to E. faecalis Symbioflor 1 DSM 16431 and (B) to the reference strains of the Biotyper library.
Whole genome analysis using the ANI and the Genome-to-Genome Hybridization similarity methods also showed that E. faecalis OB15 is closer to Symbioflor 1 DSM 16431 than to OB14 in terms of genomic distance (Figure 2A). This agrees with hierarchical cluster analysis from MALDI-TOF MS.
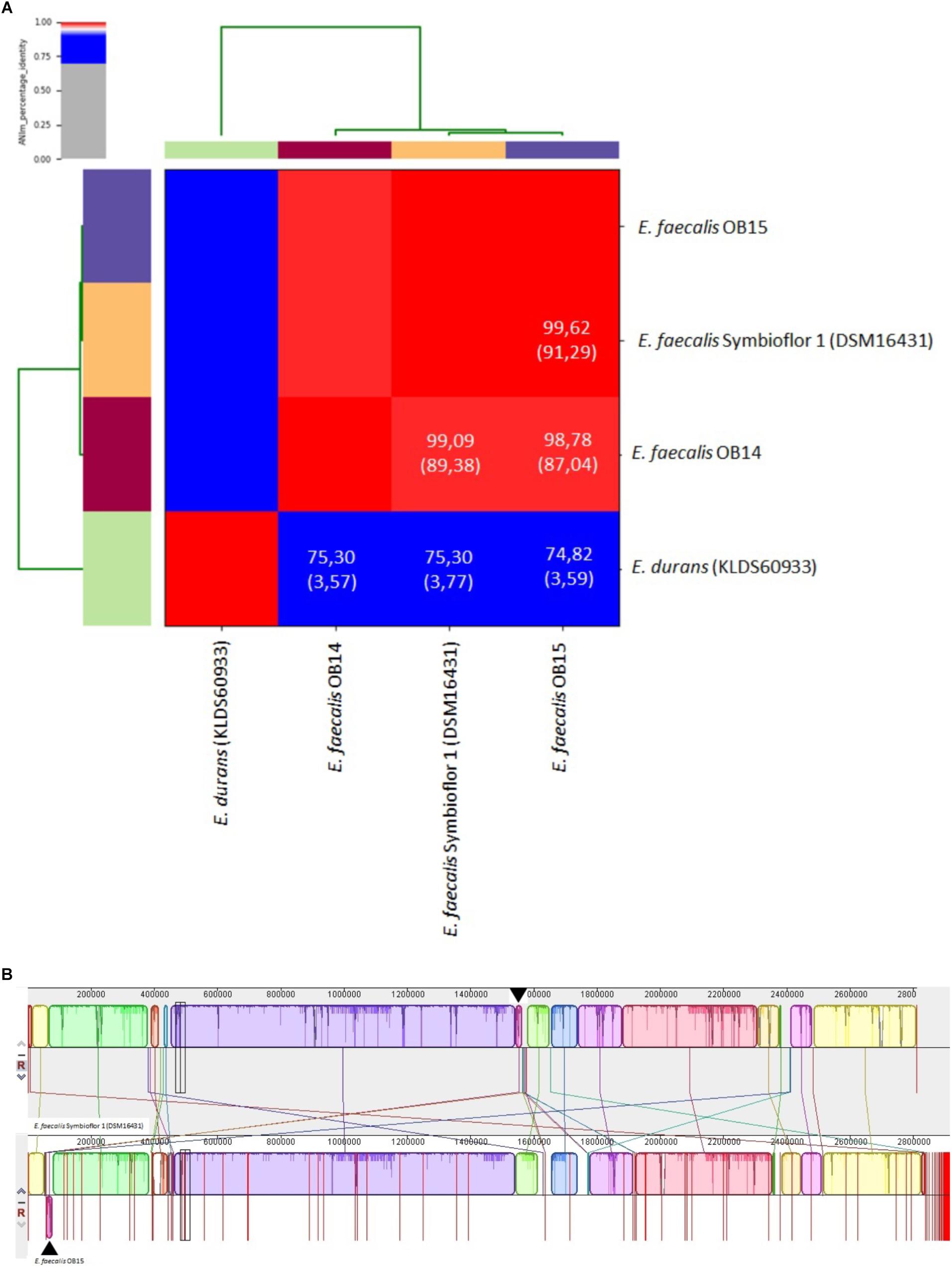
Figure 2. (A) Heat-map of Average Nucleotide Identity (ANI) between Enterococcus faecalis OB14, E. faecalis OB15 and E. faecalis Symbioflor 1 DSM 16431. ANI values (%) and coverage (between brackets) are also indicated. (B) Pairwise alignment between the reference strain Symbioflor 1 and the related strain OB15 using the MAUVE software. Colored blocks outline genome sequences that align to the other genome and are presumably homologous and internally free of genomic rearrangement (Locally Colinear Blocks or LCBs). White regions correspond to sequences that are not aligned and probably contain sequence elements specific to a genome. Blocks below the center line indicate regions that aligned in the reverse complement (inverse) orientation. The height of the profile within each LCB demonstrates the average degree of sequence conservation within an aligned region.
To complete these data, the overall genomic differences between E. faecalis Symbioflor 1 DSM 16431 and the OB15 strain have been investigated by aligning the two genomes using MAUVE v.20150226 (Figure 2B; Darling et al., 2004). This alignment suggests a high level of conservation along the chromosome. Indeed, OB15 and Symbioflor 1 DSM 16431 genomes were found to be organized in a very similar way, except one inversion of physically close syntenic block highlighted by arrows.
MLST analysis allowed to identify sequence types (ST) of E. faecalis OB15 and E. faecalis Symbioflor 1 DSM 16431, by comparing their sequences with alleles from the E. faecalis MLST database. This showed that the MLST profile of OB15 and Symbioflor 1 DSM 16431 are not similar (OB15 belongs to clone ST25 and Symbioflor 1 DSM 16431 is clone ST248).
Once identified, E. faecalis OB14 and OB15 were then evaluated for their probiotic potential using physiological tests and in vitro analysis (gastric and intestinal transit tolerance, hydrophobicity and autoaggregation, biofilm/adhesion on abiotic surface, adhesion to intestinal cells, production of the anti-inflammatory cytokine IL-10, modulation of the epithelial barrier).
Probiotic Potential of OB14 and OB15
Gastric and Intestinal Transit Tolerance
An effective property as prerequisite for probiotics is their ability to resist to the harsh condition in the stomach and the small intestine (Ouwehand et al., 2002). Therefore, E. faecalis OB14 and OB15 were tested for their ability to survive in acidic condition and in the presence of 0.3% bile salts, compared to the E. faecalis Symbioflor 1 probiotic strain. Table 1 showed that after 3 h of incubation at pH 3.0, E. faecalis OB14 and OB15 retained their viability with 84.7 and 97.3% survival rate, respectively. E. faecalis OB15 showed a highly similar viability to Symbioflor 1 (97.4%), whereas the survival of E. faecalis OB14 was slightly affected (-15%).
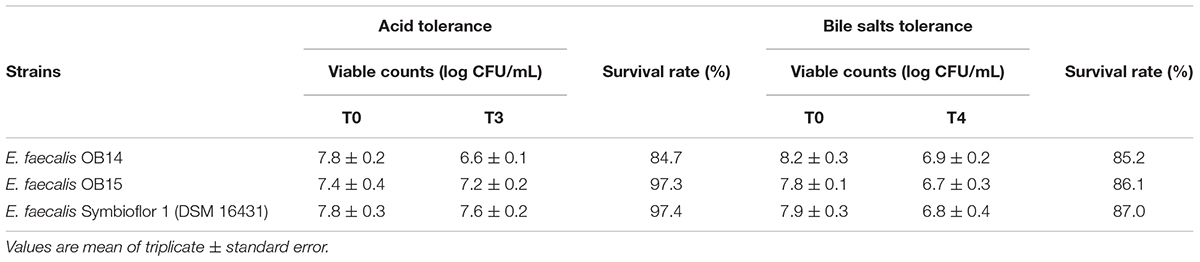
Table 1. Acid and bile salts tolerance of Enterococcus faecalis OB14, E. faecalis OB15 and E. faecalis Symbioflor 1 DSM 16431.
A genome analysis of E. faecalis Symbioflor 1, performed by Domann et al. (2007) revealed the presence of genes that mediate resistance against oxidative stress, and may facilitate the bacteria to survive exposure to gastric acid following consumption, and to survive and proliferate in the intestine. Haghshenas et al. (2016) have also reported that Enterococcus strains showed better low pH tolerance than many LAB strains belonging to Lactobacillus, Lactococcus or Leuconostoc genus. This high tolerance capability can be associated to the bilayer membrane structure, which allows easy tolerance of inverse conditions.
In the current study, the tolerance of E. faecalis OB14 and OB15 to 0.3% bile salts was also tested (Table 1). This concentration has been recommended as suitable for screening probiotic, as it simulates the conditions within the gastrointestinal tract (Goldin and Gorbach, 1992). The two isolates displayed high tolerance to bile salts, after 4 h of incubation, with a survival >80%, similarly to E. faecalis Symbioflor 1 DSM 16431, the probiotic reference added in the experiment (Table 1). These findings are in accordance with previous studies that showed that Enterococcus species had the highest tolerance capability in the bile salts conditions among LAB group (Klaenhammer and Kullen, 1999). For example, it has been found that E. faecalis 13C can strongly tolerate 0.3% oxgall with a survival rate of 98% after 3 h of incubation (Haghshenas et al., 2016), and E. faecalis CP58 was capable to retain viability for 6 h with a survival rate of 92.6% (Nueno-Palop and Narbad, 2011). Some strains of Enterococci, isolated from canine feed, were even found to be able to grow in the presence of 5% Oxgall (Lauková et al., 2008).
Hydrophobicity and Autoaggregation
Hydrophobicity is a physicochemical feature related to the capacity of bacteria to autoaggregate and adhere to various types of surfaces including eukaryotic cells. Thus, the affinity of E. faecalis OB14 and OB15, and Symbioflor 1 DSM 16431 toward the hydrophobic solvent xylen was examined. The results of this experiment showed 68.8, 78.6, and 38.6% hydrophobicity, respectively (Figure 3A). Concerning autoaggregation (Figure 3B), the probiotic strain E. faecalis Symbioflor 1 DSM 16431, used as reference, showed the higher percentage reaching 63.9%, whereas the scores for E. faecalis OB14 and OB15 strains were 54.3 and 48.9%, respectively. These percentages are consistent to the autoaggregation of E. faecalis 14 (49%), that has been isolated from the meconium of human donors and considered as high autoaggregative strain (Al Atya et al., 2015). Hydrophobicity and autoaggregation are beneficial attributes for probiotics, necessary for adhesion to intestinal cells, and to form a barrier that prevents the colonization by food-borne pathogens (Pelletier et al., 1997; Dunne et al., 2001; Collado et al., 2008).
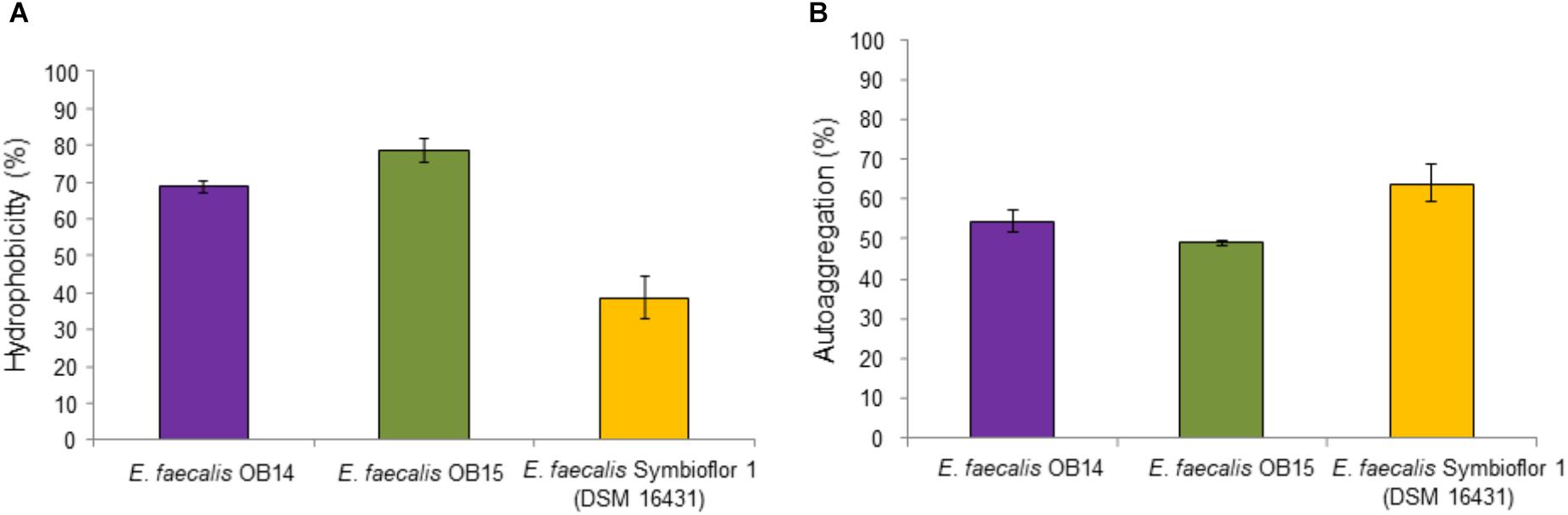
Figure 3. Hydrophobicity (A) and autoaggregation (B) of Enterococcus faecalis OB14, E. faecalis OB15 and E. faecalis Symbioflor 1 DSM 16431.
Biofilm/Adhesion on Abiotic Surface
Biofilm-forming abilities of E. faecalis OB14 and OB15, and E. faecalis Symbioflor 1 DSM 16431, were evaluated on abiotic surfaces. The three strains were found to be weak to moderate biofilm producers (data not shown). Adhesion to abiotic surfaces is not routinely investigated in probiotic strains, even if a positive correlation often exists between biofilm formation on abiotic surfaces and adhesiveness to biotic surfaces (Bujnakova et al., 2014). Thus, to better estimate adhesion of the isolates to the gut, this parameter was then investigated on Caco-2/TC7 intestinal cells.
Adhesion to Intestinal Cells
Bacterial adhesion to epithelial cells is considered as one of the most reliable features for selection criteria of probiotic strains (Garcia-Gonzalez et al., 2018). Caco-2 cell line has been frequently used as an in vitro model to screen for adhesive strains (Elo et al., 1991; Chauvière et al., 1992; Bernet et al., 1994). In this study, the adhesion of E. faecalis OB14 and OB15 to Caco-2/TC7 cells was investigated and compared to the adhesion of E. faecalis Symbioflor 1 DSM 16431. The results found showed adhesive potential in the range of 3–12% for the three strains. This agrees with other reports that have investigated the capacity of attachment of E. faecalis to cell lines. For example, a rate of approximately 2% adhesion was recently found for E. faecalis B3A-B3B, a bacteriocinogenic strain that has been isolated from a healthy Iraqi infant (Al Seraih et al., 2018). Indeed, after treatment of the Caco-2 cells with 107 bacteria for 2 h, the authors recovered 1.8 105 CFU/mL of adherent bacteria. Similarly, Nueno-Palop and Narbad (2011) have previously found an adhesion rate of 2.6% for E. faecalis CP58 isolated from human gut. These values have been considered has high capability to adhere to Caco-2 cells for Enterococcus strains with dairy origin (Cebrián et al., 2012; Pimentel et al., 2012).
It has been reported that the mechanism of adhesion to Caco-2/TC7 cells involves the combinations of carbohydrate and protein factors on the bacterial cell surface (Kimoto-Nira et al., 2007). In Enterococcus, the aggregation substance, is known to play a role in adherence to eukaryotic cells (Mundy et al., 2000). Aggregation factors may be advantageous for probiotic strains, since it can help the bacterium, to colonize the intestine after consumption, to proliferate and hence to display its probiotic traits (Veljović et al., 2017).
Production of IL-10
The ability of LAB to modulate cytokines production is considered as another criterion for the selection of beneficial microbes (Amit-Romach et al., 2010). IL-10 has interesting impact on immunoregulation, since it inhibits type 1/proinflammatory cytokine formation (Asadullah et al., 1998). Therefore, we quantified the IL-10 secretion by Caco-2/TC7 cells to investigate the ability of E. faecalis OB14 and OB15 to modulate inflammatory reaction in human intestinal cell lines, compared to the effect of E. faecalis Symbioflor 1. The results obtained by the ELISA assay (Figure 4), showed that E. faecalis OB14 induced a 28% increase of IL-10 secretion by Caco-2/TC7 cells (production of 17.8 pg/mL compared to 13.9 pg/mL for the basal cytokine value); E. faecalis Symbioflor 1 induced a 17% increase (production of 16.3 pg/mL), whereas E. faecalis OB15 had no effect. These differences among bacteria are not surprising since the immunomodulatory effects are often strain-specific (Helwig et al., 2006; Snel et al., 2011). Indeed, Al Seraih et al. (2018) have studied the anti-inflammatory effect of the B3A-B3B E. faecalis strain, isolated from an infant faces, and found that this bacterium had no effect on IL-10 production by Caco-2 cells. Conversely, Are et al. (2008) demonstrated that E. faecalis EC16, isolated from a healthy newborn baby, could induce the anti-inflammatory cytokine IL-10 in the intestine through PPAR-gamma, and eliminate the inflammatory responses. Similarly, Wang et al. (2014) showed that E. faecalis from healthy infants modulates inflammation through MAPK signaling pathways.
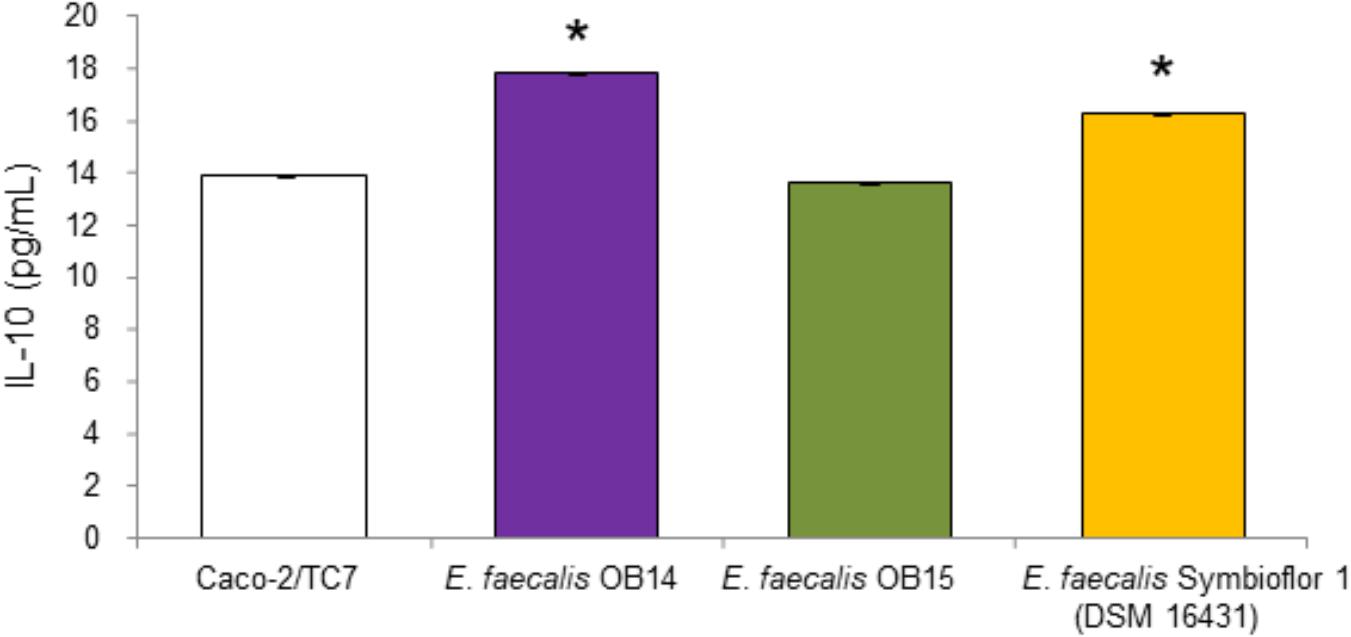
Figure 4. Quantification of IL-10 in the supernatants of Caco-2/TC7 cells after treatment with Enterococcus faecalis OB14, E. faecalis OB15 and E. faecalis Symbioflor 1 DSM 16431. ∗P < 0.05 compared to Caco-2/TC7.
Modulation of the Epithelial Barrier
Changes in the TER of polarized Caco-2/TC7 cells were used as an indicator of the effect of E. faecalis OB14 and OB15 on the intestinal epithelial barrier function (Figure 5). The results showed that the bacteria maintained the TER almost constant for the first 6 h compared to the initial value in the insert. After 16 h, E. faecalis OB14 and OB15, increased the TER, similarly to E. faecalis Symbioflor 1. Lodemann et al. (2015) also showed that a strain of Enterococcus (E. faecium NCIMB 10415) can enhance the TER when administered at a concentration of 106/1.12 cm2.
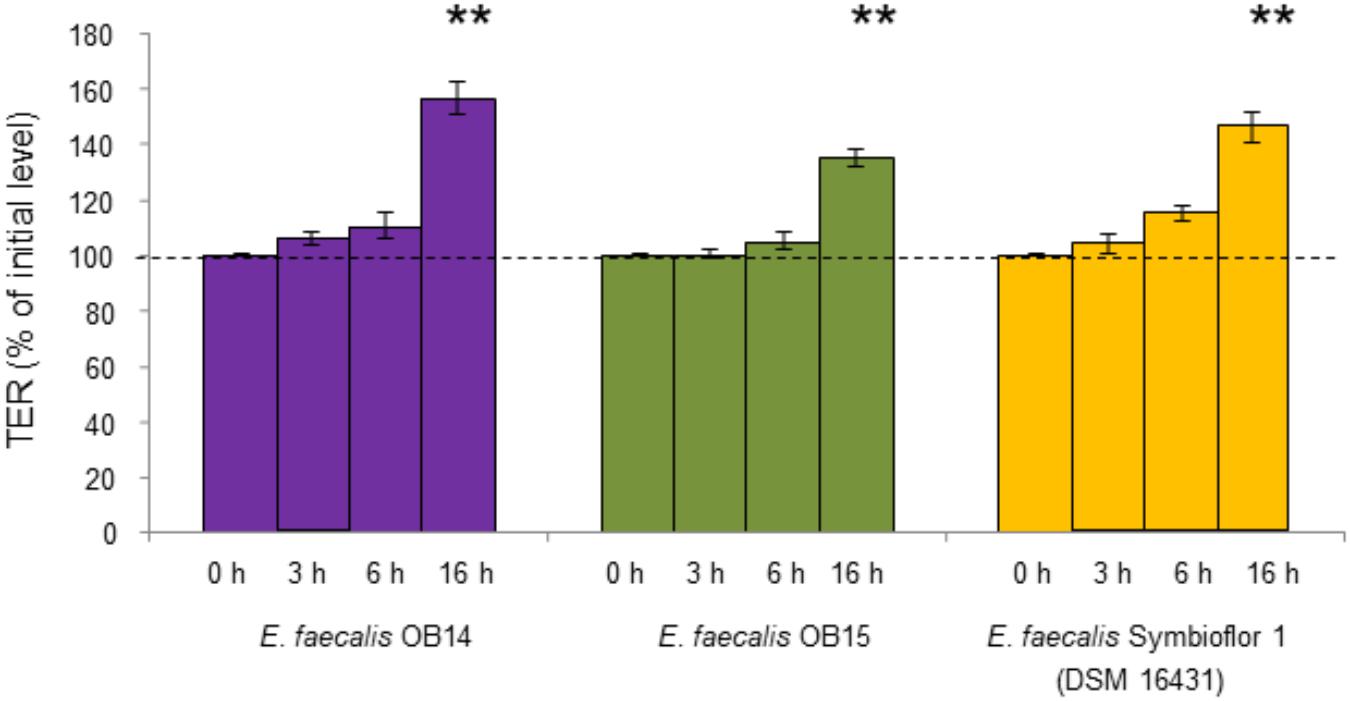
Figure 5. TER of Caco-2/TC7 cells exposed to Enterococcus faecalis OB14, E. faecalis OB15, and E. faecalis Symbioflor 1 DSM 16431. ∗∗P < 0.01 compared to initial value.
After the investigation of these beneficial attributes for E. faecalis OB14 and OB15 as probiotic candidates, we examined the safety of the bacteria using various tests (antibiotic susceptibility, hemolysis and cytotoxicity, virulence).
Safety Evaluation of OB14 and OB15
Antibiotic Susceptibility
One of the required properties by which specific strains can be considered as a potential probiotic bacterium is that they must not harbor acquired and transferable antibiotic resistances (Courvalin, 2006; Zheng et al., 2017). Table 2 shows the MICs of E. faecalis OB14 and OB15 to the four selected antibiotics from two different groups: cell wall inhibitors (ampicillin and vancomycin) and protein synthesis inhibitors (gentamicin and erythromycin), compared to the E. faecalis Symbioflor 1 reference strain. Strains were considered resistant when they showed MIC values higher than the MIC breakpoints established by the EFSA (2012). The three strains exhibited the same pattern of sensitivity/resistance to the antibiotic tested. They were sensitive to the clinically important antibiotics ampicillin (MIC = 1 μg/mL) and vancomycin (MIC = 2 μg/mL), and resistant to gentamicin (MIC = 128 μg/mL and erythromycin (MIC > 256 μg/mL).
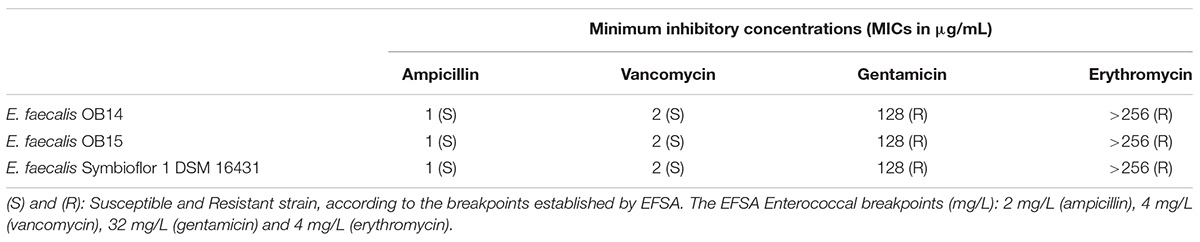
Table 2. Antibiotic susceptibility of Enterococcus faecalis OB14, E. faecalis OB15 and E. faecalis Symbioflor 1 DSM 16431.
Genomic analysis was used to complete these data and showed absence of genes conferring resistance to vancomycin, in both E. faecalis OB14 and OB15. Furthermore, sequencing data showed that E. faecalis OB14 harbored tetracycline resistance determinants unlike to E. faecalis OB15 and E. faecalis Symbioflor 1.
Various opinions exist as to whether it might be desirable that some probiotic strains show resistance to specific antibiotics. On the one hand, some lactic acid bacteria can serve as host for antibiotic resistance genes and transfer these genes to pathogens. However, many of the resistance observed are intrinsic or natural resistance and non-transmissible (Salminen et al., 1998; Voravuthikunchai et al., 2006). It gives advantages in the clinical application, as this allows the probiotic to be taken at the same time as the antibiotic treatment. Resistance of enterococci to gentamicin has been described as intrinsic and partially attributed to a poor uptake of the antibiotic (Kristich et al., 2014). Resistance to erythromycin seems to be intrinsic as well, non-transmissible, and is widely spread among enterococci commonly found in foods (Barbosa et al., 2009). Recently, a high rate of erythromycin resistance has also been detected among Enterococci isolated from Artisanal Tunisian Meat “Dried Ossban” (Zommiti et al., 2018). Macrolides are frequently used in animal husbandry; this could contribute to the emergence of many resistant strains (Diarra et al., 2010). The most frequent type of macrolide resistance is the production of a methylase enzyme, encoded by erm genes (MLSB phenotype) which specifically methylates an adenine residue in the 23S rRNA of the 50S ribosomal subunit. This reduces the binding affinity of macrolides for the ribosome and hence renders macrolides ineffective (Kristich et al., 2014). Another mechanism of resistance is due to the presence of an efflux pump system mediated by the membrane-bound efflux protein, encoded by mef (A/E) and msr genes. Finally, in a study conducted by Anderson et al. (2016), tetracycline-resistance was also found to be frequently present in food (13/14 isolates tested). Besides the presence of some antibiotic’s resistance in Enterococcus strains in foods, a recent work concludes that E. faecalis isolated from raw milk cheeses does not represent a substantial reservoir of antimicrobial resistance and virulence factors if compared with clinical strains (Silvetti et al., 2019).
Hemolysis and Cytotoxicity
To develop or select new beneficial microbes, their absence of hemolysis capacity and/or cytotoxicity should be assessed at first (Salminen et al., 1998). Indeed, although some strains of Enterococcus have been previously used for their technological properties, or as probiotic for humans and animals, a case-by-case-evaluation is needed (Araújo and Ferreira, 2013; Hanchi et al., 2018).
As mentioned above, hemolytic activity has been determined on Columbia agar containing 5% of sheep blood, by streaking the bacteria on the surface of the solid medium. The results obtained after 48 h incubation at 37°C under aerobic conditions are presented on Figure 6A. Observation of the Columbia agar plates showed no hemolysis zones of red blood cells (γ-hemolysis) for E. faecalis OB14 and OB15 similarly as the E. faecalis Symbioflor 1 probiotic strain. However, erythrocytes from different species were previously found to show various levels of susceptibility to hemolysin-mediated lysis. Sheep red blood cells were less susceptible than rabbit, human and horse red blood cells (Semedo et al., 2003). Thus, to complete this analysis, as no hemolysis has been observed in the present work, the cytotoxicity of E. faecalis OB14 and OB15 was also studied on the Caco-2/TC7 intestinal cell line. The NR uptake assay, used to quantify the viable cells, showed high rates of eukaryotic cell survival ranging from 79.8 to 85.9%, after contact with the three bacteria (Figure 6B). In accordance with these results, the cytotoxicity test using Cytotox 96 kit displayed mortality below 10% after incubation with the bacteria, similarly to natural death of cells (data not shown).
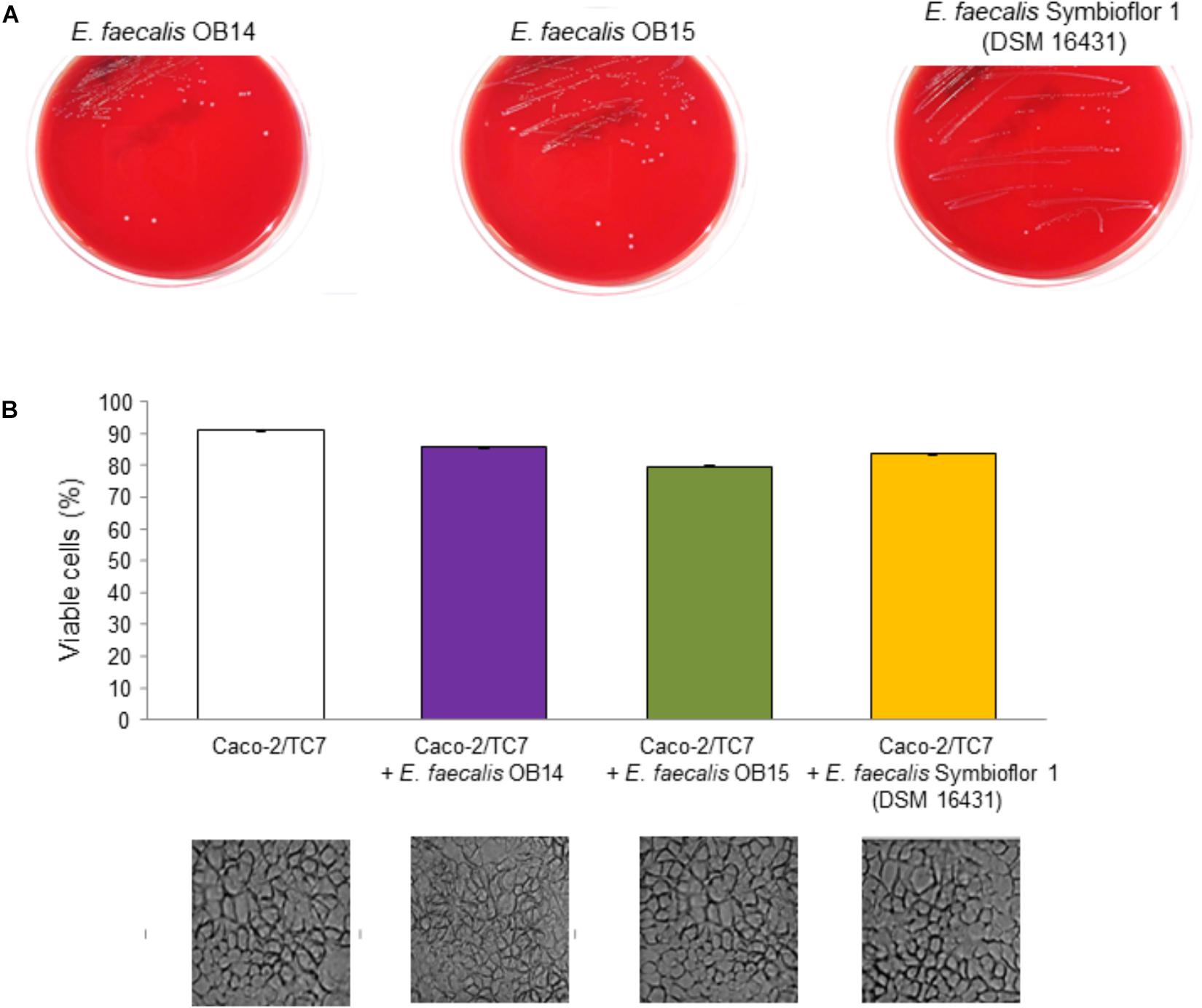
Figure 6. Hemolysis assay (A) of Enterococcus faecalis OB14, E. faecalis OB15 and E. faecalis Symbioflor 1 DSM 16431 on Columbia agar containing 5% of sheep blood. Viability of Caco-2/TC7 cells (B) measured using the Neutral Red uptake assay and observed by microscopy after treatment with 108 bacteria/mL of E. faecalis OB14, E. faecalis OB15 and E. faecalis Symbioflor 1 DSM 16431, compared to untreated Caco-2/TC7 cells.
The absence of cytotoxicity of E. faecalis OB14 or OB15, compared to E. faecalis Symbioflor 1 DSM 16431, was reinforced by microscopic observations which did not show any damage of the cell monolayers (Figure 6B). These results agree with the known safety of E. faecalis Symbioflor 1 (Christoffersen et al., 2012; Fritzenwanker et al., 2013). This bacterium has been shown to not be toxic in an in vivo Caenorhabiditis elegans model and has been used as probiotic for humans for many years without triggering infection (Domann et al., 2007; Neuhaus et al., 2017).
Analysis of Virulence Genes
The investigation of virulence determinants is an absolute prerequisite to characterize E. faecalis strains and classify them as probiotics or conversely potential pathogens, and to avoid the risk of dissemination of virulence factors to other bacteria by genetic transfer. Thus, presence/absence of the aggregation substance (asa1), gelatinase (gelE), collagen adhesin (ace), enterococcal surface protein (esp) and cytolysin (cylA) genes were checked by PCR assays and whole genome sequencing (WGS; Table 3). The ace and esp genes were not found in E. faecalis OB14 and OB15 whereas asa1 and gelE gene were detected by both methods. These results are not surprising as Domann et al. (2007) showed that the genotype of the E. faecalis Symbioflor 1 probiotic was also esp- and agg (asa1)+, but conversely for the two other genes, these authors found that the strain was ace+ and gelE-. However, despite the presence of gelE gene in E. faecalis OB14 and OB15, these bacteria failed to degrade gelatin on Nutrient gelatin medium (data not shown). This gene may be silent or expressed at a low level in our experimental conditions. Besides, all the genes belonging to the cytolysin operon (cylA, cylB, cylI, cylL, cylM, and cylS) were found in E. faecalis OB14, but not in OB15. Due to the detection of some virulence genes in E. faecalis OB14 and OB15, a test of safety/virulence was then performed on the Galleria mellonella in vivo model.
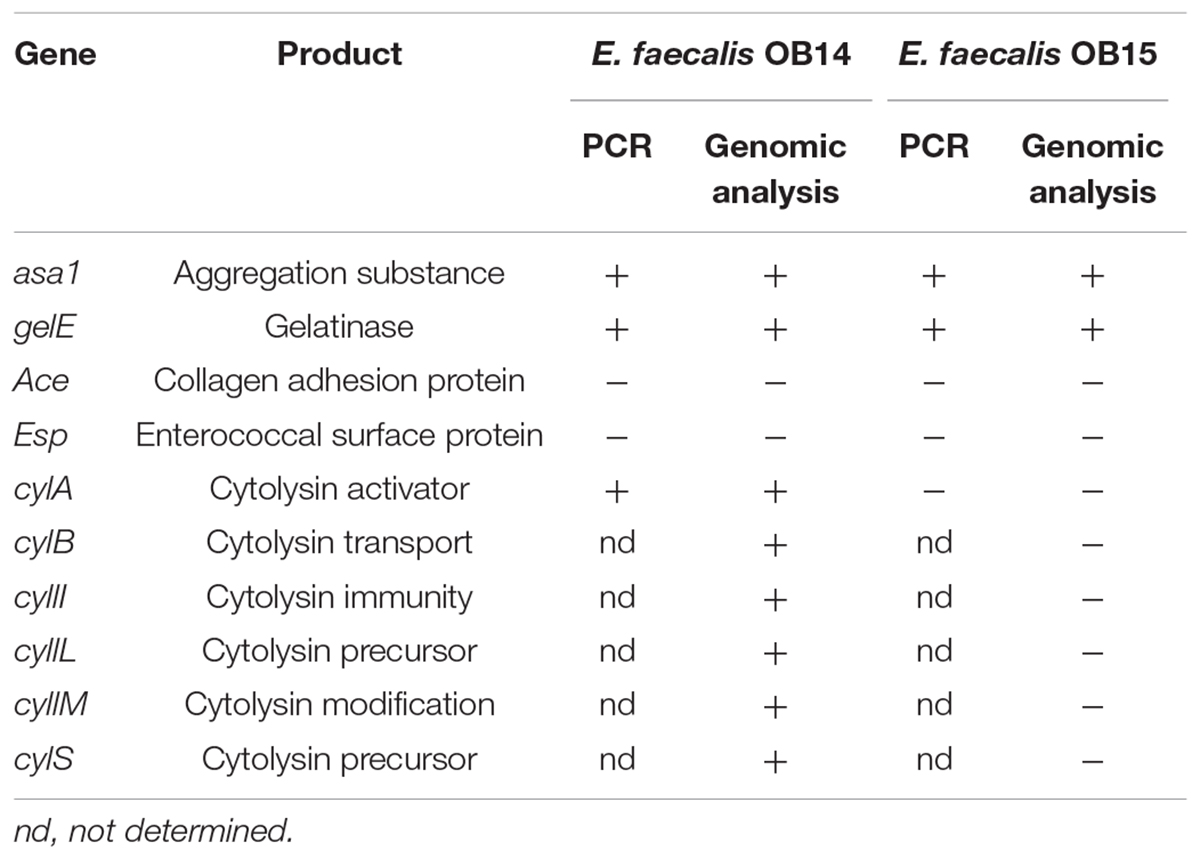
Table 3. Potential virulence factors of Enterococcus faecalis OB14 and E. faecalis OB15 detected by PCR and genomic analysis.
Virulence Test on Galleriamellonella Model
Mammalian models of infection are costly and may raise ethical issues. As a reliable alternative, larvae of Galleria mellonella have been shown to be a powerful infection model to investigate virulence of various pathogens including E. faecalis (Ramarao et al., 2012). Thus, the virulence of E. faecalis OB14 and OB15 was estimated using this infection model, compared to E. faecalis Symbioflor 1 DSM 16431 (probiotic strain) and E. faecalis V19 (pathogenic strain). Larval surviving was monitored at 16 h, and then every hour until 24 h post-injection (Figure 7). The results show that G. mellonella larvae were able to survive inoculation of E. faecalis OB15 with the same survival profile that after injection with E. faecalis Symbioflor 1 DSM 16431, so this strain isolated from Rigouta can be considered as non-virulent and might be interesting for further development as potential probiotic. On the contrary, despite its food origin, E. faecalis OB14, isolated from Testouri cheese, led to a fast and drastic mortality of the larvae by 16 h post-injection, which was quite as toxic as the E. faecalis V19 lethal positive control of pathogenic strain. These results are in concordance with the presence of the cytolysin genes found in the genome of E. faecalis OB14, as cytolysin has been previously linked to virulence enhancement in animal models infected by E. faecalis and acute patient mortality in the clinic (Tang et al., 2018). By contrast, cytolysin genes were absent in E. faecalis OB15 and the bacterium was not toxic for the larvae. Taking together, all these results showed the necessity to follow the recommendations of guidance for selection of Enterococcus strains as probiotic, using intensive case by case evaluation for each strain, physiological analysis, whole genome sequencing and virulence models, even if the strains are isolated from a food ecological niche. Indeed, the risk of contamination with a pathogen strain during traditional process, due to a lack of hygiene or a poor quality of the raw material that has been used cannot be ruled out.
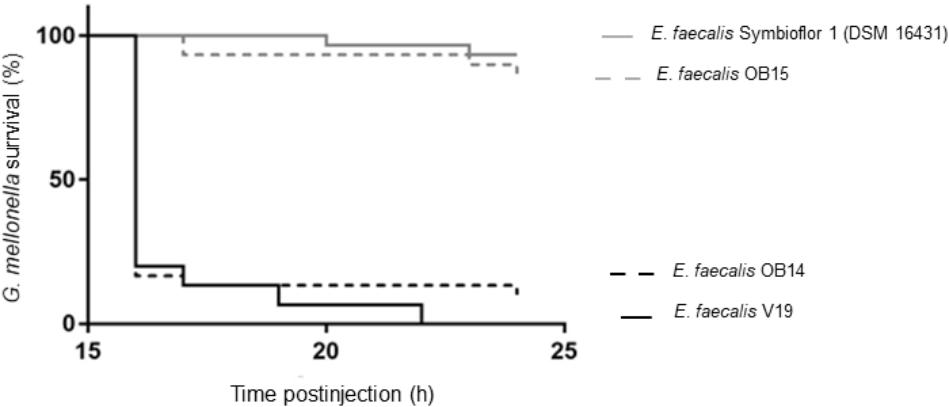
Figure 7. Galleria mellonella survival following injection with Enterococcus faecalis OB14, E. faecalis OB15, E. faecalis Symbioflor 1 DSM 16431 or E. faecalis V19.
Conclusion
In vitro testing is a rational starting point for evaluating the safety of bacteria, to select potential probiotic or to establish their possible mechanisms of action, since in vivo methods are costly and need complex agreement by ethical committees. In the current study, it has been found that E. faecalis OB14 and OB15 can adhere to intestinal cells, tolerate gastric and intestinal conditions (acidity, bile), and demonstrate the capacity to reinforce the epithelial barrier, similarly to the known probiotic reference strain, E. faecalis Symbioflor 1 DSM 16431. However, despite its food origin, E. faecalis OB14 was found to harbor resistance to tetracycline and some important virulence traits as the presence of cytolysin genes. This has been confirmed by the in vivo test on Galleria Mellonella which led to a high percentage of larvae mortality, so this bacterium can not be selected for probiotic candidate. On the contrary, E. faecalis OB15, isolated from Rigouta, seems to be an interesting strain for further studies and probiotic development for traditional fermented food and feed industry.
Author Contributions
MM, MF, FA, and NC designed the study. OB and NC carried out most of the experiments, analyzed the data, and drafted the manuscript. LF participated in the sampling and isolation of bacteria. KZ and ED provided the probiotic reference strain. AZ, MC, and OM contributed to the PCR assays. MB helped with the identification by MALDI-TOF MS. AB and OM conducted whole genome sequencing experiments and bioinformatic analysis. IR and CM performed the virulence test on the Galleria mellonella model. FA and NC co-supervised the entire project. All authors read and approved the final manuscript.
Funding
This work was supported by Evreux Porte de Normandie, Region Normandie and European Union (FEDER), and by Tunisian Ministry of Higher Education and Scientific Research.
Conflict of Interest Statement
KZ was employed by company SymbioPharm GmbH and provided the E. faecalis Symbioflor 1 strain as probiotic reference for the experiments.
The remaining authors declare that the research was conducted in the absence of any commercial or financial relationships that could be construed as a potential conflict of interest.
Footnotes
- ^ http://www.bioinformatics.babraham.ac.uk/projects/fastqc
- ^ https://github.com/sanger-pathogens/mlst_check
- ^ https://pubmlst.org/efaecalis/ (accessed February 19, 2019).
References
Adnan, M., Patel, M., and Hadi, S. (2017). Functional and health promoting inherent attributes of Enterococcus hirae F2 as a novel probiotic isolated from the digestive tract of the fresh water fish Catla catla. Peer J. 5:e3085. doi: 10.7717/peerj.3085
Al Atya, A. K., Drider-Hadiouche, K., Ravallec, R., Silvain, A., Vachee, A., and Drider, D. (2015). Probiotic potential of Enterococcus faecalis strains isolated from meconium. Front. Microbiol. 6:227. doi: 10.3389/fmicb.2015.00227
Al Seraih, A., Belguesmia, Y., Cudennec, B., Baah, J., and Drider, D. (2018). In silico and experimental data claiming safety aspects and beneficial attributes of the bacteriocinogenic strain Enterococcus faecalis B3A-B3B. Probiotics Antimicrob. Proteins 10, 456–465. doi: 10.1007/s12602-017-9357-8
Amit-Romach, E., Uni, Z., and Reifen, R. (2010). Multistep mechanism of probiotic bacterium, the effect on innate immune system. Mol. Nutr. Food Res. 54, 277–284. doi: 10.1002/mnfr.200800591
Anandharaj, M., Sivasankari, B., Santhanakaruppu, R., Manimaran, M., Rani, R. P., and Sivakumar, S. (2015). Determining the probiotic potential of cholesterol-reducing Lactobacillus and Weissella strains isolated from gherkins (fermented cucumber) and south Indian fermented koozh. Res. Microbiol. 166, 428–439. doi: 10.1016/j.resmic.2015.03.002
Anderson, A. C., Jonas, D., Huber, I., Karygianni, L., Wölber, J., Hellwig, E., et al. (2016). Enterococcus faecalis from food, clinical specimens, and oral sites: prevalence of virulence factors in association with biofilm formation. Front. Microbiol. 6:1534. doi: 10.3389/fmicb.2015.01534
Araújo, T. F., and Ferreira, C. L. L. F. (2013). The genus Enterococcus as probiotics: safety concerns. Braz. Arch. Biol. Technol. 56, 457–466. doi: 10.1590/S1516-89132013000300014
Are, A., Aronsson, L., Wang, S., Greicius, G., Lee, Y. K., Gustafsson, J. A., et al. (2008). Enterococcus faecalis from newborn babies regulate endogenous PPAR- activity and IL-10 levels in colonic epithelial cells. Proc. Natl. Acad. Sci. U.S.A. 105, 1943–1948. doi: 10.1073/pnas.0711734105
Asadullah, K., Sterry, W., Stephanek, K., Jasulaitis, D., Leupold, M., Audring, H., et al. (1998). IL-10 is a key cytokine in psoriasis. Proof of principle by IL-10 therapy: a new therapeutic approach. J. Clin. Invest. 101, 783–794. doi: 10.1172/JCI1476
Azat, R., Liu, Y., Li, W., Kayir, A., Lin, D. B., Zhou, W. W., et al. (2016). Probiotic properties of lactic acid bacteria isolated from traditionally fermented Xinjiang cheese. J. Zhejiang Univ. Sci. B 17, 597–609. doi: 10.1631/jzus.B1500250
Bankevich, A., Nurk, S., Antipov, D., Gurevich, A. A., Dvorkin, M., Kulikov, A. S., et al. (2012). SPAdes: a new genome assembly algorithm and its applications to single-cell sequencing. J. Comput. Biol. 19, 455–477. doi: 10.1089/cmb.2012.0021
Barbosa, J., Ferreira, V., and Teixeira, P. (2009). Antibiotic susceptibility of enterococci isolated from traditional fermented meat products. Food Microbiol. 26, 527–532. doi: 10.1016/j.fm.2009.03.005
Ben Braïek, O., Cremonesi, P., Morandi, S., Smaoui, S., Hani, K., and Ghrairi, T. (2018). Safety characterisation and inhibition of fungi and bacteria by a novel multiple enterocin-producing Enterococcus lactis 4CP3 strain. Microb. Pathog. 118, 32–38. doi: 10.1016/j.micpath.2018.03.005
Benkerroum, N. (2013). Traditional fermented foods of north African countries: technology and food safety challenges with regard to microbiological risks. Compr. Rev. Food Sci. Food Saf. 12, 54–89. doi: 10.1111/j.1541-4337.2012.00215.x
Bernet, M. F., Brassart, D., Neeser, J. R., and Servin, A. L. (1994). Lactobacillus acidophilus LA1 binds to cultured human intestinal cell lines and inhibits cell attachment and cell invasion by enterovirulent bacteria. Gut 35, 483–489. doi: 10.1136/gut.35.4.483
Biaggini, K., Borrel, V., Szunerits, S., Boukherroub, R., N’Diaye, A., Zébré, A., et al. (2017). Substance P enhances lactic acid and tyramine production in Enterococcus faecalis V583 and promotes its cytotoxic effect on intestinal Caco-2/TC7 cells. Gut Pathog. 9:20. doi: 10.1186/s13099-017-0171-3
Bolger, A. M., Lohse, M., and Usadel, B. (2014). Trimmomatic: a flexible trimmer for Illumina sequence data. Bioinformatics 30, 2114–2120. doi: 10.1093/bioinformatics/btu170
Bujnakova, D., Strakova, E., and Kmet, V. (2014). In vitro evaluation of the safety and probiotic properties of Lactobacilli isolated from chicken and calves. Anaerobe 29, 118–127. doi: 10.1016/j.anaerobe.2013.10.009
Cebrián, R., Baños, A., Valdivia, E., Pérez-Pulido, R., Martínez-Bueno, M., and Maqueda, M. (2012). Characterization of functional, safety, and probiotic properties of Enterococcus faecalis UGRA10, a new AS-48-producer strain. Food Microbiol. 30, 59–67. doi: 10.1016/j.fm.2011.12.002
Chauvière, G., Coconnier, M. H., Kerneis, S., Fourniat, J., and Servin, A. L. (1992). Adhesion of human Lactobacillus acidophilus strain LB to human enterocyte-like Caco-2 cells. J. Gen. Microbiol. 138, 1689–1696. doi: 10.1099/00221287-138-8-1689
Chen, L., Zheng, D., Liu, B., Yang, J., and Jin, Q. (2016). VFDB 2016: hierarchical and refined dataset for big data analysis–10 years on. Nucleic Acids Res. 44, D694–D697. doi: 10.1093/nar/gkv1239
Christoffersen, T. E., Jensen, H., Kleiveland, C. R., Dørum, G., Jacobsen, M., and Lea, T. (2012). In vitro comparison of commensal, probiotic and pathogenic strains of Enterococcus faecalis. Br. J. Nutr. 108, 2043–2053. doi: 10.1017/S0007114512000220
Collado, M. C., Meriluoto, J., and Salminen, S. (2008). Adhesion and aggregation properties of probiotic and pathogen strains. Eur. Food Res. Technol. 226, 1065–1073. doi: 10.1007/s00217-007-0632
Courvalin, P. (2006). Antibiotic resistance: the pros and cons of probiotics. Dig. Liver Dis. 38, S261–S265. doi: 10.1016/S1590-8658(07)60006-1
Darling, A. C. E., Mau, B., Blattner, F. R., and Perna, N. T. (2004). Mauve: multiple alignment of conserved genomic sequence with rearrangements. Genome Res. 14, 1394–1403. doi: 10.1101/gr.2289704
De Almeida, C. V., Taddei, A., and Amedei, A. (2018). The controversial role of Enterococcus faecalis in colorectal cancer. Therap. Adv. Gastroenterol. 11:1756284818783606. doi: 10.1177/1756284818783606
Del Re, B., Sgorbati, B., Miglioli, M., and Palenzona, D. (2000). Adhesion, autoaggregation and hydrophobicity of 13 strains of Bifidobacterium longum. Lett. Appl. Microbiol. 31, 438–442. doi: 10.1046/j.1365-2672.2000.00845.x
Delley, M., Moliet, B., and Hottinger, H. (1990). DNA probe for Lactobacillus delbrueckii. Appl. Environ. Microbiol. 56, 1967–1970.
Diarra, M. S., Rempel, H., Champagne, J., Masson, L., Pritchard, J., and Topp, E. (2010). Distribution of antimicrobial resistance and virulence genes in Enterococcus spp. and characterization of isolates from broiler chickens. Appl. Environ. Microbiol. 76, 8033–8043. doi: 10.1128/AEM.01545-10
Diaz, M., Ladero, V., Del Rio, B., Redruello, B., Fernández, M., Martin, M. C., et al. (2016). Biofilm-forming capacity in biogenic amine-producing bacteria isolated from dairy products. Front. Microbiol. 7:591. doi: 10.3389/fmicb.2016.00591
Domann, E., Hain, T., Ghai, R., Billion, A., Kuenne, C., Zimmermann, K., et al. (2007). Comparative genomic analysis for the presence of potential enterococcal virulence factors in the probiotic Enterococcus faecalis strain Symbioflor 1. Int. J. Med. Microbiol. 297, 533–539. doi: 10.1016/j.ijmm.2007.02.008
Dunne, C., O’Mahony, L., Murphy, L., Thornton, G., Morrissey, D., O’Halloran, S., et al. (2001). In vitro selection criteria for probiotic bacteria of human origin: correlation with in vivo findings. Am. J. Clin. Nutr. 73, 386S–392S. doi: 10.1093/ajcn/73.2.386s
EFSA Panel on Biological Hazards, Ricci, A., Allende, A., Bolton, D., Chemaly, M., Davies, R., et al. (2017). Scientific Opinion on the update of the list of QPS-recommended biological agents intentionally added to food or feed as notified to EFSA. EFSA J. 15:e04664. doi: 10.2903/j.efsa.2017.4664
Elbehiry, A., Marzouk, E., Hamada, M., Al-Dubaib, M., Alyamani, E., Moussa, I. M., et al. (2017). Application of MALDI-TOF MS fingerprinting as a quick tool for identification and clustering of foodborne pathogens isolated from food products. New Microbiol. 40, 269–278.
Elo, S., Saxelin, M., and Salminen, S. (1991). Attachment of Lactobacillus casei strain GG to human colon carcinoma cell line Caco-2: comparison with other dairy strains. Lett. Appl. Microbiol. 13, 154–156. doi: 10.1111/j.1472-765X.1991.tb00595.x
European Food Safety Authority [EFSA] (2012). Guidance on the assessment of bacterial susceptibility to antimicrobials of human and veterinary importance. EFSA J. 10, 2740–2749.
Extremina, C. I., Costa, L., Aguiar, A. I., Peixe, L., and Fonseca, A. P. (2011). Optimization of processing conditions for the quantification of enterococci biofilms using microtitre-plates. J. Microbiol. Methods 84, 167–173. doi: 10.1016/j.mimet.2010.11.007
Foulquié Moreno, M. R., Sarantinopoulos, P., Tsakalidou, E., and De Vuyst, L. (2006). The role and application of Enterococci in food and health. Int. J. Food Microbiol. 106, 1–24. doi: 10.1016/j.ijfoodmicro.2005.06.026
Franz, C. M., Huch, M., Abriouel, H., Holzapfel, W., and Gálvez, A. (2011). Enterococci as probiotics and their implications in food safety. Int. J. Food Microbiol. 151, 125–140. doi: 10.1016/j.ijfoodmicro.2011.08.014
Franz, C. M., Stiles, M., Schleifer, K. H., and Holzapfel, W. H. (2003). Enterococci in foods-a conundrum for food safety. Int. J. Food Microbiol. 88, 105–122. doi: 10.1016/S0168-1605(03)00174-0
Fritzenwanker, M., Kuenne, C., Billion, A., Hain, T., Zimmermann, K., Goesmann, A., et al. (2013). Complete genome sequence of the probiotic Enterococcus faecalis Symbioflor 1 Clone DSM 16431. Genome Announc. 1:e00165-12. doi: 10.1128/genomeA.00165-12
Garcia-Gonzalez, N., Prete, R., Battista, N., and Corsetti, A. (2018). Adhesion properties of food-associated Lactobacillus plantarum strains on human intestinal epithelial cells and modulation of IL-8 release. Front. Microbiol. 9:2392. doi: 10.3389/fmicb.2018.02392
Goldin, B. R., and Gorbach, S. L. (1992). “Probiotics for humans,” in Probiotics. The Scientific Basis. Ž, ed. R. Fuller (London: Chapman & Hall), 355–376. doi: 10.1007/978-94-011-2364-8_13
Gómez, N. C., Ramiro, J. M., Quecan, B. X., and de Melo Franco, B. D. (2016). Use of potential probiotic Lactic Acid Bacteria (LAB) biofilms for the control of Listeria monocytogenes, Salmonella typhimurium, and Escherichia coli O157:H7 biofilms formation. Front. Microbiol. 7:863. doi: 10.3389/fmicb.2016.00863
Haghshenas, B., Haghshenas, M., Nami, Y., Yari Khosroushahi, A., Abdullah, N., Barzegari, A., et al. (2016). Probiotic assessment of Lactobacillus plantarum 15HN and Enterococcus mundtii 50H isolated from traditional dairies microbiota. Adv. Pharm. Bull. 6, 37–47. doi: 10.15171/apb.2016.07
Haigh, J., Degun, A., Eydmann, M., Millar, M., and Wilks, M. (2011). Improved performance of bacterium and yeast identification by a commercial matrix-assisted laser desorption ionization–time of flight mass spectrometry system in the clinical microbiology laboratory. J. Clin. Microbiol. 49:3441. doi: 10.1128/JCM.00576-11
Hanchi, H., Mottawea, W., Sebei, K., and Hammami, R. (2018). The Genus Enterococcus: between probiotic potential and safety concerns-an update. Front. Microbiol. 9:1791. doi: 10.3389/fmicb.2018.01791
Helwig, U., Lammers, K. M., Rizzello, F., Brigidi, P., Rohleder, V., Caramelli, E., et al. (2006). Lactobacilli, bifidobacteria and E. coli nissle induce pro- and anti-inflammatory cytokines in peripheral blood mononuclear cells. World J. Gastroenterol. 12, 5978–5986.
Hillion, M., Mijouin, L., Jaouen, T., Barreau, M., Meunier, P., Lefeuvre, L., et al. (2013). Comparative study of normal and sensitive skin aerobic bacterial populations. Microbiologyopen 2, 953–961. doi: 10.1002/mbo3.138
Khan, H., Flint, S., and Yu, P. K. (2010). Enterocins in food preservation. Int. J. Food Microbiol. 141, 1–10. doi: 10.1016/j.ijfoodmicro.2010.03.005
Kimoto-Nira, H., Mizumachi, K., Nomura, M., Kobayashi, M., Fujita, Y., Okamoto, T., et al. (2007). Lactococcus sp. as potential probiotic lactic acid bacteria. Jpn. Agric. Res. Q. 41, 181–189. doi: 10.6090/jarq.41.181
Klaenhammer, T. R., and Kullen, M. J. (1999). Selection and design of probiotics. Int. J. Food Microbiol. 50, 45–57. doi: 10.1016/S0168-1605(99)00076-8
Kristich, C. J., Rice, L. B., and Arias, C. A. (2014). “Enterococcal infection—treatment and antibiotic resistance,” in Enterococci: from Commensals to Leading Causes of Drug Resistant Infection, eds M. S. Gilmore, D. B. Clewell, Y. Ike, and N. Shankar (Boston, MA: Massachusetts Eye and Ear Infirmary).
Lauková, A., Marcináková, M., Strompfová, V., and Ouwehand, A. C. (2008). Probiotic potential of enterococci isolated from canine feed. Folia Microbiol. 53, 84–88. doi: 10.1007/s12223-008-0012-3
Li, B., Zhan, M., Evivie, S. E., Jin, D., Zhao, L., Chowdhury, S., et al. (2018). Evaluating the Safety of potential probiotic Enterococcus durans KLDS6.0930 using whole genome sequencing and oral toxicity study. Front. Microbiol. 29:1943. doi: 10.3389/fmicb.2018.01943
Lodemann, U., Strahlendorf, J., Schierack, P., Klingspor, S., Aschenbach, J. R., and Martens, H. (2015). Effects of the probiotic Enterococcus faecium and pathogenic Escherichia coli strains in a pig and human epithelial intestinal cell model. Scientifica 2015:235184. doi: 10.1155/2015/235184
Maekawa, T., Fukaya, R., Takamatsu, S., Itoyama, S., Fukuoka, T., Yamada, M., et al. (2018). Possible involvement of Enterococcus infection in the pathogenesis of chronic pancreatitis and cancer. Biochem. Biophys. Res. Commun. 506, 962–969. doi: 10.1016/j.bbrc.2018.10.169
Mannu, L., Paba, A., Daga, E., Comunian, R., Zanetti, S., Duprè, I., et al. (2003). Comparison of the incidence of virulence determinants and antibiotic resistance between Enterococcus faecium strains of dairy, animal and clinical origin. Int. J. Food Microbiol. 88, 291–304. doi: 10.1016/S0168-1605(03)00191-0
Meier-Kolthoff, J. P., Auch, A. F., Klenk, H. P., and Goker, M. (2013). Genome sequence-based species delimitation with confidence intervals and improved distance functions. BMC Bioinformatics 14:60. doi: 10.1186/1471-2105-14-60
Messaoudi, S., Madi, A., Prévost, H., Feuilloley, M., Manai, M., Dousset, X., et al. (2012). In vitro evaluation of the probiotic potential of Lactobacillus salivarius SMXD51. Anaerobe 18, 584–589. doi: 10.1016/j.anaerobe.2012.10.004
Mikheenko, A., Prjibelski, A., Saveliev, V., Antipov, D., and Gurevich, A. (2018). Versatile genome assembly evaluation with QUAST-LG. Bioinformatics 34, i142–i150. doi: 10.1093/bioinformatics/bty266
Mundy, L. M., Sahm, D. F., and Gilmore, M. S. (2000). Relationships between enterococcal virulence and antimicrobial resistance. Clin. Microbiol. Rev. 13, 513–522. doi: 10.1128/cmr.13.4.513-522.2000
Ness, I. F., Diep, D. B., and Ike, Y. (2014). “Enterococcal bacteriocins and antimicrobial proteins that contribute to niche control,” in Enterococci: From Commensals to Leading Causes of Drug Resistant Infection [Internet], eds M. S. Gilmore, D. B. Clewell, Y. Ike, and N. Shankar (Boston, MA: Massachusetts Eye and Ear Infirmary).
Neuhaus, K., Lamparter, M. C., Zölch, B., Landstorfer, R., Simon, S., and Spanier, B. E. (2017). Probiotic Enterococcus faecalis Symbioflor down regulates virulence genes of EHEC in vitro and decrease pathogenicity in a Caenorhabditis elegans model. Arch. Microbiol. 199, 203–213. doi: 10.1007/s00203-016-1291-8
Nikolenko, S. I., Korobeynikov, A. I., and Alekseyev, M. A. (2013). BayesHammer: bayesian clustering for error correction in single-cell sequencing. BMC Genomics 14(Suppl. 1):S7. doi: 10.1186/1471-2164-14-S1-S7
Nueno-Palop, C., and Narbad, A. (2011). Probiotic assessment of Enterococcus faecalis CP58 isolated from human gut. Int. J. Food Microbiol. 145, 390–394. doi: 10.1016/j.ijfoodmicro.2010.12.029
Ogier, J. C., and Serror, P. (2008). Safety assessment of dairy microorganisms: the Enterococcus genus. Int. J. Food Microbiol. 126, 291–301. doi: 10.1016/j.ijfoodmicro.2007.08.017
Ouwehand, A. C., Salminen, S., and Isolauri, E. (2002). Probiotics: an overview of beneficial effects. Antonie Van Leeuwenhoek 82, 279–289. doi: 10.1023/A:1020620607611
Page, A. J., Taylor, B., and Keane, J. A. (2016). Multilocus sequence typing by blast from de novo assemblies against PubMLST. J. Open Source Softw. 1:118. doi: 10.21105/joss.00118
Palmer, K. L., Kos, V. N., and Gilmore, M. S. (2010). Horizontal gene transfer and the genomics of enterococcal antibiotic resistance. Curr. Opin. Microbiol. 13, 632–639. doi: 10.1016/j.mib.2010.08.004
Park, J., Jin, G. D., Pak, J. I., Won, J., and Kim, E. B. (2017). Development of a rapid identification method for the differentiation of Enterococcus Species using a species-specific multiplex PCR based on comparative genomics. Curr. Microbiol. 74, 476–483. doi: 10.1007/s00284-017-1210-5
Pelletier, C., Bouley, C., Cayuela, C., Bouttier, S., Bourlioux, P., and BellonFontaine, M. N. (1997). Cell surface characteristics of Lactobacillus casei subsp. casei, Lactobacillus paracasei subsp. paracasei, and Lactobacillus rhamnosus strains. Appl. Environ. Microbiol. 63, 1725–1731.
Pimentel, L. L., Mättö, J., Malcata, F. X., Pintado, M. E., and Saarela, M. (2012). Survival of potentially probiotic enterococci in dairy matrices and in the human gastrointestinal tract. Int. Dairy J. 27, 53–57. doi: 10.1016/j.idairyj.2012.07.008
Popović, N., Dinić, M., Tolinački, M., Mihajlović, S., Terzić-Vidojević, A., Bojić, S., et al. (2018). New insight into biofilm formation ability, the presence of virulence genes and probiotic potential of Enterococcus sp. dairy isolates. Front. Microbiol. 9:78. doi: 10.3389/fmicb.2018.00078
Pritchard, L. (2017). PYANI: Python Module for Average NucleotideIdentity Analyses. Available at: https://github.com/widdowquinn/pyani/releases/tag/v0.2.7 (accessed February 19, 2019).
Pritchard, L., Glover, R. H., Humphris, S., Elphinstone, J. G., and Toth, I. K. (2016). Genomics and taxonomy in diagnostics for food security: soft rotting enterobacterial plant pathogens. Anal. Methods 8, 12–24. doi: 10.1039/C5AY02550H
Ramarao, N., Nielsen-Leroux, C., and Lereclus, D. (2012). The insect Galleria mellonella as a powerful infection model to investigate bacterial pathogenesis. J. Vis. Exp. 11:e4392. doi: 10.3791/4392
Ramos, C. L., Thorsen, L., Schwan, R. F., and Jespersen, L. (2013). Strain-specific probiotics properties of Lactobacillus fermentum, Lactobacillus plantarum and Lactobacillus brevis isolates from Brazilian food products. Food Microbiol. 36, 22–29. doi: 10.1016/j.fm.2013.03.010
Reviriego, C., Eaton, T., Martin, R., Jimenez, E., Fernandez, L., Gasson, M. J., et al. (2005). Screening of virulence determinants in Enterococcus faecium strains isolated from breast milk. J. Hum. Lact. 21, 131–138. doi: 10.1177/0890334405275394
Rosenberg, M., Gutnick, D., and Rosenberg, E. (1980). Adherence of bacteria to hydrocarbons: a simple method for measuring cell-surface hydrophobicity. FEMS Microbiol. Lett. 9, 29–33. doi: 10.1111/j.1574-6968.1980.tb05599.x
Salminen, S., Ouwehand, A. C., and Isolauri, E. (1998). Clinical applications of probiotic bacteria. Int. Dairy J. 8, 563–572. doi: 10.1016/S0958-6946(98)00077-6
Seemann, T. (2018). abricate: Mass Screening of Contigs for Antimicrobial and Virulence Genes (accessed February 19, 2019).
Semedo, T., Almeida Santos, M., Martins, P., Silva Lopes, M. F., Figueiredo Marques, J. J., and Tenreiro, R. (2003). Comparative study using type strains and clinical and food isolates to examine hemolytic activity and occurrence of the cyl operon in enterococci. J. Clin. Microbiol. 41, 2569–2576. doi: 10.1128/JCM.41.6.2569-2576.2003
Silvetti, T., Morandi, S., and Brasca, M. (2019). Does Enterococcus faecalis from traditional raw milk cheeses serve as a reservoir of antibiotic resistance and pathogenic traits? Foodborne Pathog. Dis.
Snel, J., Vissers, Y., Smit, B., Jongen, J., Van Der Meulen, E., Zwijsen, R., et al. (2011). Strain specific immunomodulatory effects of Lactobacillus plantarum strains on birch pollen allergic subjects out of season. Clin. Exp. Allergy 41, 232–242. doi: 10.1111/j.1365-2222.2010.03650.x
Sogawa, K., Watanabe, M., Sato, K., Segawa, S., Ishii, C., and Miyabe, A. (2011). Use of the MALDI BioTyper system with MALDI-TOF mass spectrometry for rapid identification of microorganisms. Anal. Bioanal. Chem. 400, 1905–1911. doi: 10.1007/s00216-011-4877-7
Tang, W., Bobeica, S. C., Wang, L., and van der Donk, W. A. (2018). CylA is a sequence-specific protease involved in toxin biosynthesis. J. Ind. Microbiol. Biotechnol.
Veljović, K., Popović, N., Miljković, M., Tolinaćki, M., Terzić-Vidojević, A., and Kojić, M. (2017). Novel aggregation promoting factor AggE contributes to the probiotic properties of Enterococcus faecium BGGO9-28. Front. Microbiol. 8:1843. doi: 10.3389/fmicb.2017.01843
Voravuthikunchai, S. P., Bilasoi, S., and Supamala, O. (2006). Antagonistic activity against pathogenic bacteria by human vaginal lactobacilli. Anaerobe 12, 221–226. doi: 10.1016/j.anaerobe.2006.06.003
Wang, S., Hibberd, M. L., Pettersson, S., and Lee, Y. K. (2014). Enterococcus faecalis from healthy infants modulates inflammation through MAPK signaling pathways. PLoS One 9:e97523. doi: 10.1371/journal.pone.0097523
Wessels, D., Jooste, P. J., and Mostert, J. F. (1991). Technologically important characteristics of Enterococcus isolates from raw milk and dairy products. Int. J. Food Microbiol. 10, 349–352. doi: 10.1016/0168-1605(90)90082-G
Zheng, M., Zhang, R., Tian, X., Zhou, X., Pan, X., and Wong, A. (2017). Assessing the risk of probiotic dietary supplements in the context of antibiotic resistance. Front. Microbiol. 8:908. doi: 10.3389/fmicb.2017.00908
Keywords: Testouri cheese, Rigouta, Enterococcus faecalis, probiotics, antibiotic resistance, genomic analysis, virulence, safety
Citation: Baccouri O, Boukerb AM, Farhat LB, Zébré A, Zimmermann K, Domann E, Cambronel M, Barreau M, Maillot O, Rincé I, Muller C, Marzouki MN, Feuilloley M, Abidi F and Connil N (2019) Probiotic Potential and Safety Evaluation of Enterococcus faecalis OB14 and OB15, Isolated From Traditional Tunisian Testouri Cheese and Rigouta, Using Physiological and Genomic Analysis. Front. Microbiol. 10:881. doi: 10.3389/fmicb.2019.00881
Received: 05 January 2019; Accepted: 05 April 2019;
Published: 24 April 2019.
Edited by:
Teresa Zotta, Italian National Research Council (CNR), ItalyReviewed by:
Christian Magni, CONICET Instituto de Biología Molecular y Celular de Rosario (IBR), ArgentinaJeverson Frazzon, Federal University of Rio Grande do Sul, Brazil
Monica Sparo, National University of Central Buenos Aires, Argentina
Copyright © 2019 Baccouri, Boukerb, Farhat, Zébré, Zimmermann, Domann, Cambronel, Barreau, Maillot, Rincé, Muller, Marzouki, Feuilloley, Abidi and Connil. This is an open-access article distributed under the terms of the Creative Commons Attribution License (CC BY). The use, distribution or reproduction in other forums is permitted, provided the original author(s) and the copyright owner(s) are credited and that the original publication in this journal is cited, in accordance with accepted academic practice. No use, distribution or reproduction is permitted which does not comply with these terms.
*Correspondence: Nathalie Connil, bmF0aGFsaWUuY29ubmlsQHVuaXYtcm91ZW4uZnI=
†These authors have equitably contributed to the supervision of this work