- Faculty of Bioscience and Technology for Food, Agriculture and Environment, University of Teramo, Teramo, Italy
Thirty-three Kluyveromyces marxianus strains were tested for the ability to form biofilm and mat structures in YPD and whey and for cell surface hydrophobicity. To identify genes potentially involved in adhesion properties, a RT-qPCR analysis was performed. Eight strains were able to adhere on polystyrene plates in both media and formed a mature mat structure. These strains showed a different level of hydrophobicity ranging from 55 to 66% in YPD and from 69 to 81% in whey. Four K. marxianus orthologs genes (FLO11, STE12, TPK3, and WSC4), known from studies in other yeast to be involved in biofilm formation, have been studied. FLO11 and STE12 showed the highest fold changes in all conditions tested and especially in whey: 15.05 and 11.21, respectively. TPK3 was upregulated only in a strain, and WSC4 in 3 strains. In YPD, fold changes were lower than in whey with STE12 and FLO11 genes showing the highest fold changes. In mat structures FLO11 and STE12 fold changes ranged from 3.6–1.3 to 2–1.17, respectively. Further studies are necessary to better understand the role of these genes in K. marxianus adhesion ability.
Introduction
Many microorganisms can form multicellular, sessile, surface-bound assemblies known as biofilms. Microorganisms able to form biofilm can shift between two different forms: planktonic cells in a free-living state, and sessile cells in a multicellular state (Andersen et al., 2014). The ability to form biofilm is strictly related to another multicellular development, the so-called mat structures. Mat is a structure composed of a complex of aggregated cells. It is formed of a network of multiple radial spokes originating from a central hub. This elaborate pattern is regulated by specific processes, depending on specific transcriptional programming, environmental signals, and cell–cell communication systems (Reynolds, 2006). Most of the studies regarding yeast adhesion were principally focused on Candida albicans (Chaffin et al., 1998; Hostetter, 1999; Gaur and Klotz, 2004; Otoo et al., 2008; Granger, 2018) and Saccharomyces cerevisiae (Bowen et al., 2001; Reynolds and Fink, 2001; Pérez-Torrado and Querol, 2016; Di Gianvito et al., 2017, 2018). Adhesion properties have mostly been described by the action of cell wall glicoproteins (Verstrepen and Klis, 2006; Goossens and Willaert, 2010; Moreno-García et al., 2018).
However, recently there is an increasing interest for some non-Saccharomyces species which can be exploited in foods fermentation for their technological traits. Among them of interest is Kluyveromyces marxianus (Fonseca et al., 2008; Lane and Morrissey, 2010). Each K. marxianus strain has diverse properties and characteristics, in fact it is not possible to establish an exclusive K. marxianus strain characterized as a “model” (Lane and Morrissey, 2010; Tittarelli et al., 2018). It has been reported that K. marxianus was the principal species in Pecorino di Farindola and Parmigiano Reggiano cheeses, showing genotypic and phenotypic polymorphisms (growth kinetics in whey, production and degradation of organic acid, amino acid consumption, production of aromatic metabolites) (Tofalo et al., 2014; Fasoli et al., 2015; Coloretti et al., 2017; Tittarelli et al., 2018). Moreover, a recent study showed differences among K. marxianus isolates from a fermented goat milk of the Yaghnob Valley based on mat formation and adhesion properties (Perpetuini et al., 2018a). Despite the efforts done, genetic studies combined with phenotypic analyses are necessary to fully exploit K. marxianus strains and highlight some understudied features such as the genetic basis underlying adhesion properties. However, biofilm formation by microorganisms is a multispecies feature, and lactic acid bacteria (LAB) are able to colonize surfaces by forming biofilms as well (Piard and Briandet, 2015; Arena et al., 2017). Although the biofilms formed on food by LAB usually spoil the products and damage both equipment and working surfaces (Flemming and Wingender, 2010), in some manufactures (like cheese manufacture) biofilms are advantageous for the food technology, being also essential for achieving the uniqueness of the product (Arena et al., 2017). The presence of yeasts and bacteria in co-culture may affect the individual biofilm production. However, the molecular mechanisms involved in adhesion of K. marxianus are almost unknown.
The aim of the present study was to explore the capacity of 33 K. marxianus strains isolated from different geographic and technological origins, to form biofilm and mat structures in different conditions (YPD and whey) and determine the hydrophobicity of cell surface. Moreover, a RT-qPCR analysis was performed to identify genes upregulated in sessile cells.
Particularly, we focused on genes that are already known in S. cerevisiae to be involved in biofilm formation: FLO11 gene – in Kluyveromyces lactis it showed some similarities with S. cerevisiae YIR019C MUC1 GPI-anchored cell surface glycoprotein essential for pseudohyphal formation and invasive growth (Van Mulders et al., 2009; Legras et al., 2016); STE12 – the transcription factor activated by a MAP kinase signaling cascade and the activity of the gene is involved in mating or pseudohyphal/invasive growth pathways; TPK3 – involved in nutrient control of cell growth and division; WSC4 – involved in cell wall integrity and stress response. Several interacting regulatory pathways control the adhesion transcriptional factors. The genes involved in these pathways are triggered by various environmental signals, such as carbon and nitrogen starvation, pH or stress factors (Verstrepen et al., 2003; Sampermans et al., 2005). Microorganisms are also able to change from non-adherence to adherence state as a mechanism of adaptation to stress factors (Verstrepen and Klis, 2006).
Materials and Methods
Samples Origin
Thirty-three K. marxianus strains isolated from different dairy products were considered in this study (Fasoli et al., 2016; Tittarelli et al., 2018). The strains are listed in Table 1. The strains were grown in YPD medium (yeast extract 1% w/v, peptone 2% w/v, glucose 2% w/v) overnight at 28°C.
All isolates were kept frozen at -80°C in the same growth medium supplemented with glycerol as cryoprotectant (20% v/v). The strains belong to the Culture Collections of the Faculty of Bioscience and Technology for Food, Agriculture, and Environment (University of Teramo, Italy) and of the Department of Agricultural and Food Sciences (University of Bologna, Italy).
Adhesion Properties Determination
Biofilm Assay on Polystyrene and Cell Surface Hydrophobicity Assay
Yeast adhesion to polystyrene was evaluated in YPD (pH 6.5 ± 0.2) and pasteurized whey (4.8% lactose, 1.2% proteins and 0.75% fats; pH 6 ± 0.2) at 100°C for 30 min. Yeast strains were grown overnight at 28°C in YPD under aerobic conditions and harvested at an optical density (OD600 nm) of 1. A 96-well polystyrene plate was prepared with 200 μl of each media, and each well was inoculated with 10 μl of cell suspension. The plate was incubated at 28°C for 2 days. Non-inoculated wells were used as negative control. Cells attached at the bottom of wells were considered as sessile cells, while unattached ones, as planktonic. Biofilm formation was evaluated daily. After 2 days, attached cells were carefully washed with saline buffer solution and recovered (Perpetuini et al., 2018b). Both sessile and planktonic cells were serially diluted and plated on YPD for cell plate count. The analyses were performed in triplicate.
The microbial adhesion to solvent (MATS) test was performed to evaluate the cell surface hydrophobicity (CSH) of cells grown in YPD and whey, according to Bellon-Fontaine et al. (1996) using n-hexadecane.
Mat Formation
The capacity of the yeast strains to grow in multicellular or mat structures, was evaluated as described by Reynolds and Fink (2001). Strains were inoculated onto YPD soft agar plates (0.3% agar) with a toothpick and incubated at 25°C for 5 days. Plates with 2% (w/v) agar were used as negative controls. Three biological replicates were made.
Phylogenetic Analysis
To find the genes involved in biofilm production in K. marxianus species and to obtain the sequences, the Yeast Gene Order Browser (YGOB)1 (Byrne and Wolfe, 2005, 2006), Saccharomyces genome database (SGD)2 or BLAST on NCBI3 were used. The nucleotide and amino acidic sequences were aligned with a Muscle alignment, using the software Mega (Kumar et al., 2016), and displayed phylogenetically using a Neighbor-Joining method bootstrapped to 1000. The analyzed sequences belonged to the following species: Saccharomyces kudriavzevii, Saccharomyces uvarum, S. cerevisiae, Saccharomyces mikatae, Naumovia castelli, K. lactis, K. marxianus, Lachancea waltii, Lachancea thermotolerans and Lachancea kluyveri.
The genes in the dataset were represented by the KEGG pathways. A search for each gene, specific for K. marxianus was done online in the KEGG pathway database4.
Genes Expression and RT-qPCR
Appropriate specific primers were designed using the online program Primer 3 Plus5. Specificity and properties of each primer set were checked with a BLAST search (Basic Alignment Search Tool6) and with Oligo analyzer 3.1 software. The primer sequences, amplicon size and qPCR program are shown in Table 2.
Specificity of the PCR reactions was analyzed by melting curves temperature analysis. To evaluate genes expression RNA was extracted using the Trizol reagent method (Sigma-Aldrich, Saint Louis, MO, United States), following the manufacturer’s instructions.
The absence of contaminating DNA was checked by PCR directly on the RNAs. RNA samples were stored with RNA later, at -80°C until use. RNA concentration was determined spectrophotometrically with a Nanodrop spectrophotometer ND-1000. One microgram of total RNA was retrotranscribed into cDNA, using the ProtoScript First Strand cDNA Synthesis Kit (BioLabs, Ipswich, Massachusetts, United States) following the manufacturer’s instructions. The RT-qPCR mixture (20 μl) contained 10 μl of Light Cycler 480 SYBR Green I Master (Roche), 0.2 μM of each primer (Life Technologies-Invitrogen, Milan, Italy) and diethylpyrocarbonate-treated water (DEPC-water). One microliter cDNA (100 ng/μl) was added to each reaction mixture. The qPCR program was common for each primer set, as reported in Table 2. Calculation of relative transcript levels (RTLs) was carried out as described by Livak and Schmittgen (2001). Gene expression of planktonic cells was used as reference condition. ALG9 and ACT1 were used as reference genes for all RT-qPCR analyses (Teste et al., 2009). The relative stability of the reference genes was calculated using the NormFinder program (Andersen et al., 2004). All samples were processed in triplicate.
Results
Ability of K. marxianus Strains to Form Biofilm on Abiotic Surface and Adhesion Properties
Thirty-three K. marxianus strains were selected from a total of 83 strains based on genotypic typing and characterization from previous studies (Fasoli et al., 2016; Tittarelli et al., 2018) and they were tested for their capacity to produce biofilm on polystyrene plates and mat structures. Only 8 of them (6M2, LM142, M135, 1SC4, CBS834T, FM09, M83, and VG4) formed mat structures and showed a stable, dimorphic growth pattern on polystyrene in YPD and whey, with both planktonic and biofilm-forming cells. The others 25 strains were not able to adhere to polystyrene plates and to produce a mat structure, which was similar to negative control. Therefore, they were not analyzed any further.
The 8 strains formed a biofilm in both substrates. The mean cell number in biofilm varied from 7.3 log CFU/mL (CBS834T) to 8.7 log CFU/mL (1SC4) in YPD and from 7.08 log CFU/mL (CBS834T) to 7.9 log CFU/mL (LM142) in whey (Figure 1). The planktonic cells ranged from 6.6 log CFU/mL (6M2) to 8.48 log CFU/mL (VG4) in YPD and from 7 log CFU/mL (1SC4 and FM09) to 7.7 log CFU/mL (VG4) in whey (Figure 1).
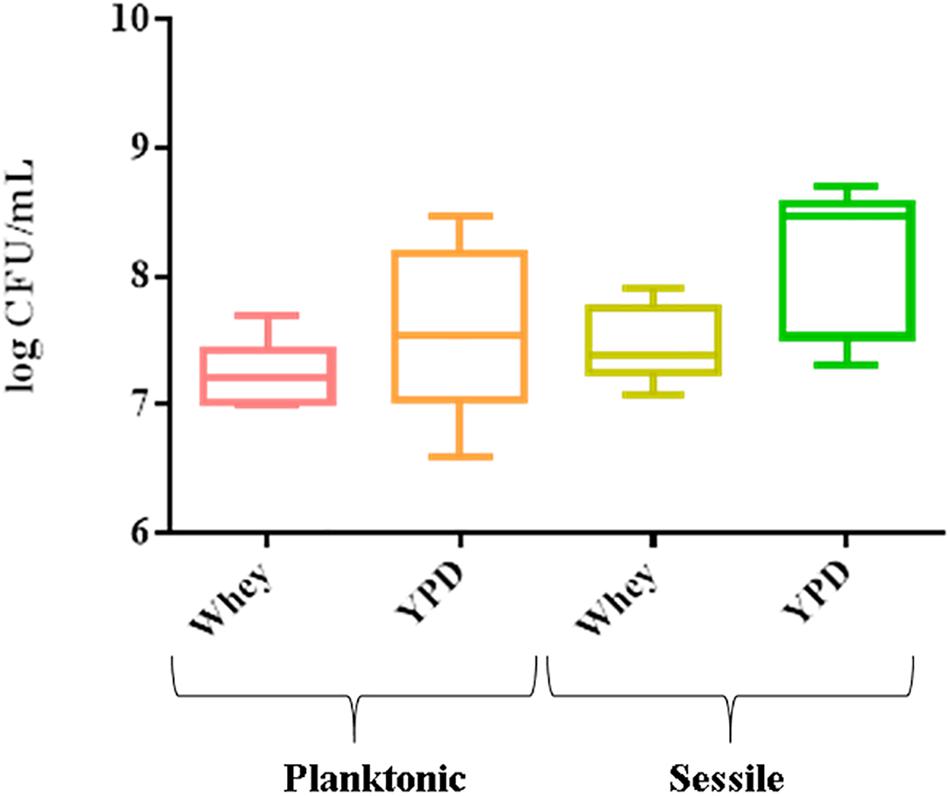
Figure 1. Box plot showing viable yeast cell counts of the 8 strains tested, in planktonic and sessile phase on polystyrene plates in YPD and whey. Three biological replicates were performed.
The mat structures formed by the 8 strains were composed of a central hub with wrinkles and channels with rough edges (or rim) in YPD (Figure 2). The observed structures showed macroscopic differences in terms of diameter and edges.
The MATS test was performed to evaluate the cell surface features of selected strains grown in YPD and whey. Strains showed different level of hydrophobicity, and the highest values were detected in whey-grown cells. CSH values ranged from 55% (VG4) to 66% (1SC4) in YPD-grown cells and from 69% (VG4 and CBS834T) to 81% (1SC4) in whey-grown cells (Figure 3).
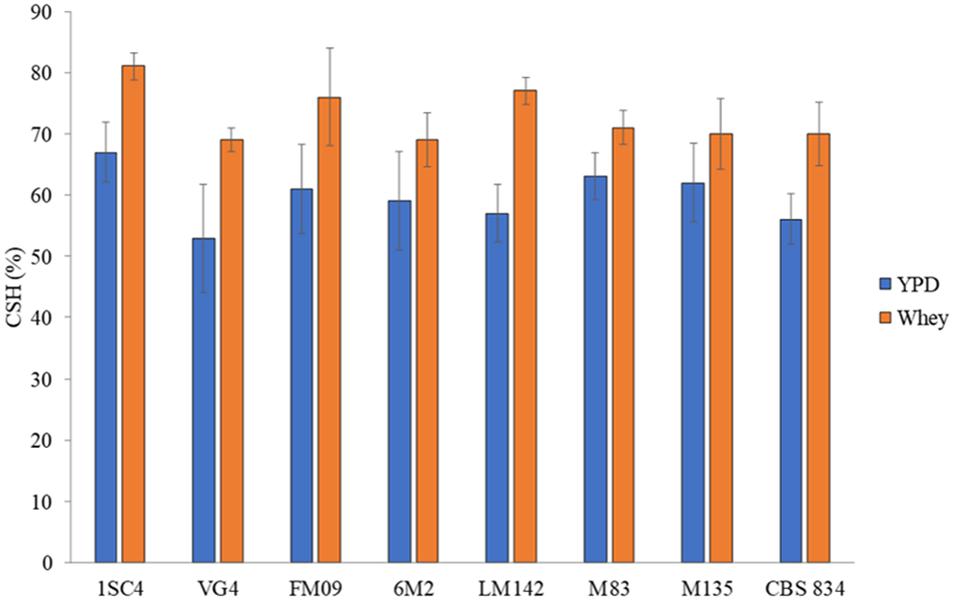
Figure 3. Hydrophobic nature of cells surface grown in YPD (blue) and whey (orange). Three biological replicates were performed. The error bars show the standard deviation.
Phylogenetic Analysis
The genes involved in adhesion properties in some yeasts belonging to the order Saccharomycetales were studied using the YGOB, SGD or BLAST on NCBI. Orthologous of S. cerevisiae FLO11 (also known as MUC1), STE12, WSC4 and TPK3 were found in most species (Figure 4A). For each selected gene, an annotated KEGG pathway image map is presented, wherein the genes are highlighted with a red arrow (Figure 4B). Multiple sequence alignment and phylogenetic analysis were carried out to compare the nucleotide sequences of the genes (Supplementary Figure S1) and the corresponding amino acidic sequences (Supplementary Figure S2). The trees showed a very strong separation of the genes. Branching of the genes clustered with generally expected patterns, but the grade of difference between the groups was high, with long branch in most clades. Thus, K. marxianus and K. lactis sequences formed a close group, different from other clades, indicating a divergent nucleotide sequence. The tree based on the amino acid sequences (Supplementary Figure S2) showed a similar pattern to the tree based on the nucleotide sequences (Supplementary Figure S1) revealing a similar codon usage of the analyzed strains.
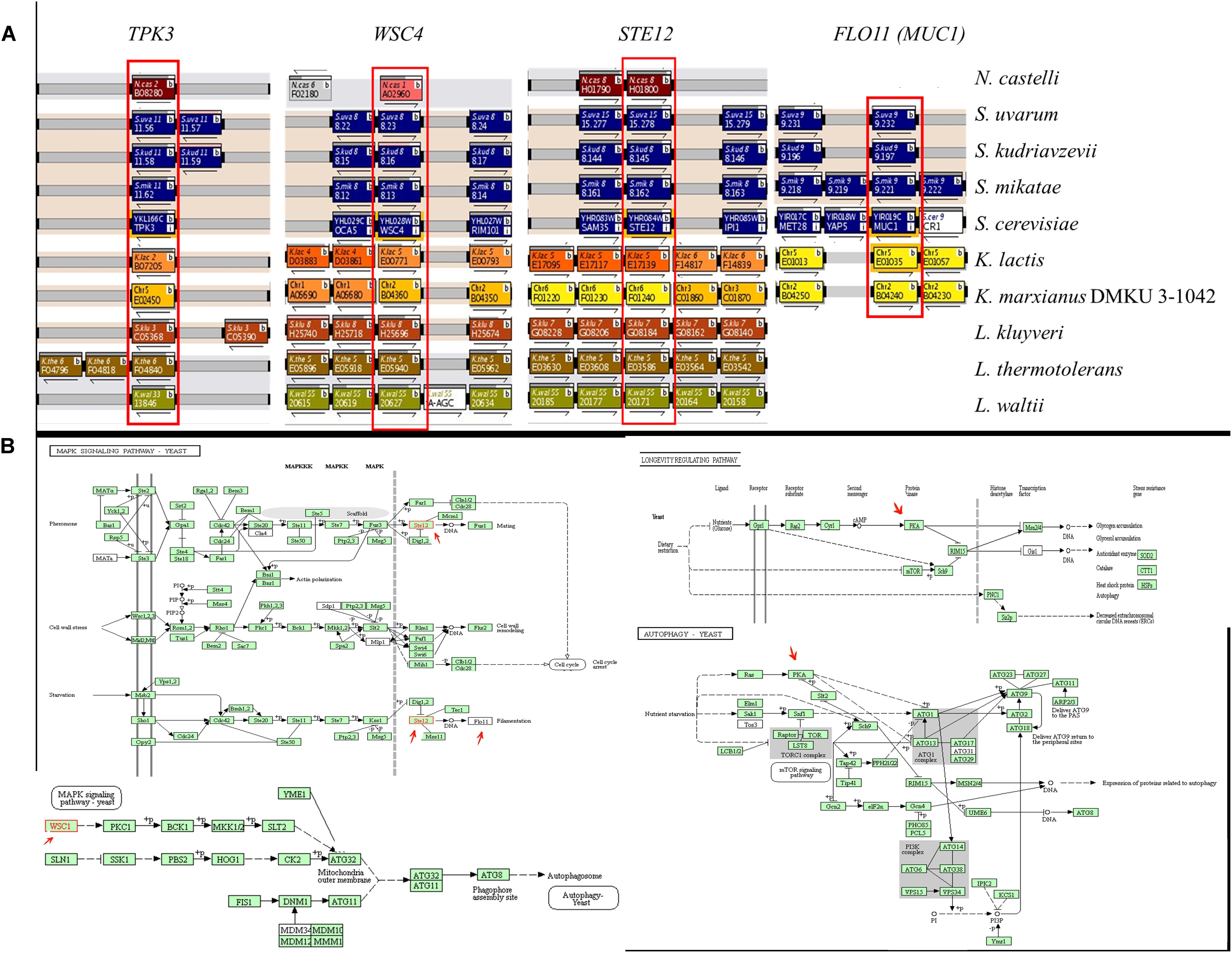
Figure 4. (A) Phylogenetic analysis of STE12, FLO11, TPK3 and WSC4 orthologous genes in the order Saccharomycetales. The distribution of orthologous genes was assessed using the Yeast Gene Order Browser (YGOB), which shows syntenic relationships between genomes. Genes are shown as shaded blocks with blocks of the same color, indicating that these genes are on the same chromosome. Orthologous genes are displayed in the vertical column, with gaps inserted to accommodate genes gained or lost. The STE12, FLO11, TPK3 and WSC4 genes are boxed and labeled. (B) Annotated KEGG pathway image maps. The selected genes are highlighted with a red arrow.
RT-qPCR Analysis
Novel primer sets were developed to detect genes involved in K. marxianus adhesion properties. To test if the designed qPCR primers worked efficiently, calibration curves were created. Serial dilutions of cDNA were made. The calibration curves created from different dilutions of cDNA were linear and efficiencies of the qPCR reactions were calculated. Efficiency of the reaction was calculated directly from the slope of the calibration curve. All the calculated efficiencies fall within this range. Melting curves analysis revealed the specificity of designed primers: a unique peak was observed, confirming the specificity of the amplification. RT-qPCR analysis revealed interesting differences among strains. The most expressed genes were STE12 and FLO11 in all conditions tested. In sessile cells grown in whey these genes showed the highest fold changes in 1SC4 strain (11.21 for STE12 and 15.05 for FLO11) (Figure 5). TPK3 was upregulated only in VG4 strain (fold change = 1.16), and WSC4 in FM09 (fold change = 1.74), in LM142 (fold change = 1.48) and M83 (fold change = 1.18). In YPD, fold changes were lower than in whey. However, also in this condition, an over expression of STE12 and FLO11 genes was observed in almost all strains, with values ranging from 1.3 (VG4) to 3.24 (1SC4), and from 1.43 (FM09) to 3.67 (1SC4), respectively. In this condition, TPK3 was not upregulated, while WSC4 was over expressed only in M83 strain (fold change = 1.51).
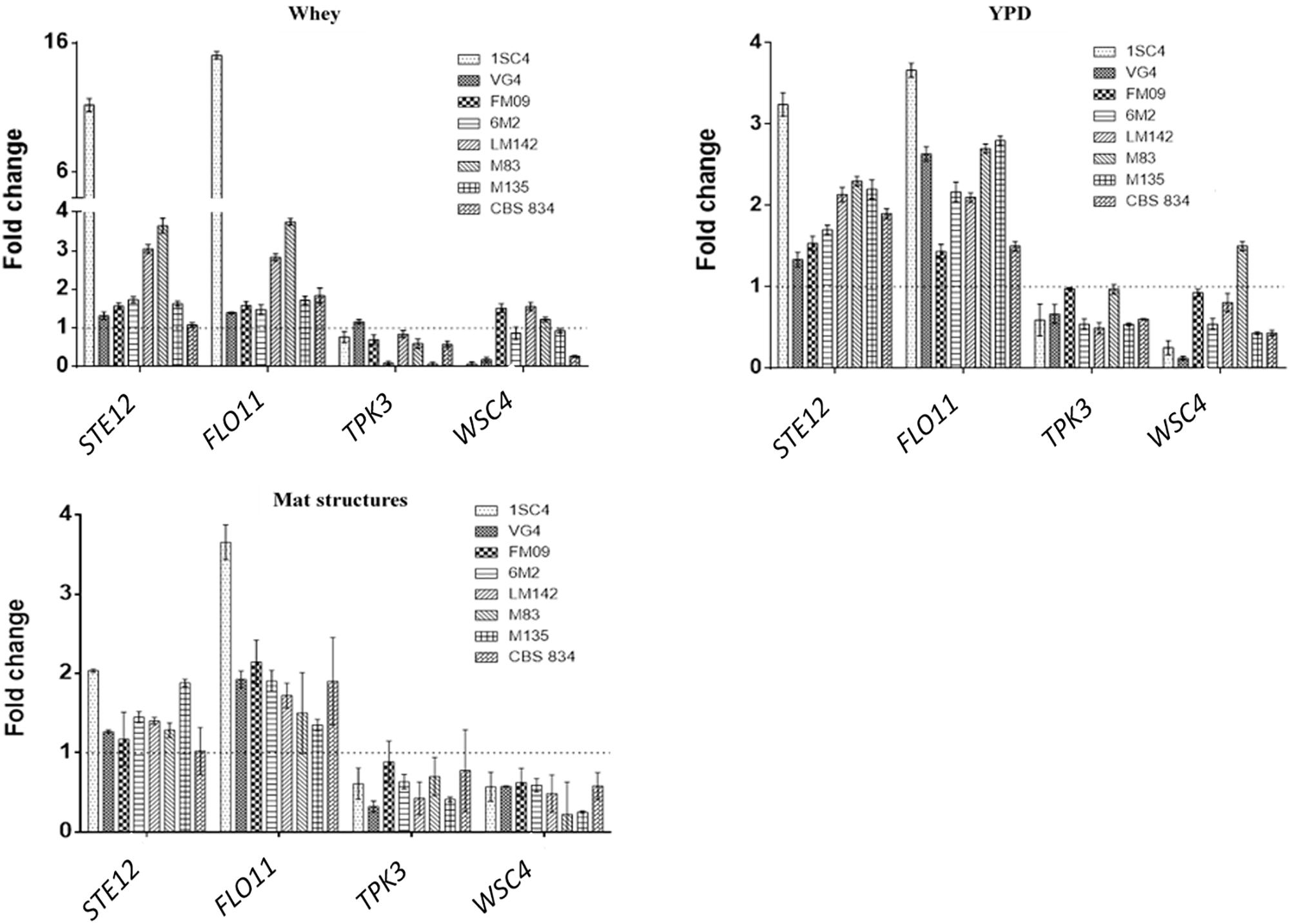
Figure 5. Relative transcript levels of tested genes in whey-grown cells, YPD-grown cells and mat structures. Transcript levels of each gene are expressed as the relative fold change, with planktonic cells as reference condition. Three biological replicates were performed.
In mat structures the fold changes for STE12 gene ranged from 2 (1SC4) to 1.17 (FM09), while for FLO11 from 3.6 (1SC4) to 1.3 (M135). WSC4 and TPK3 were not upregulated in almost none of the tested strains in mat structures.
Discussion
The ability of yeasts to form biofilm on food matrices, such as cheese surfaces, is essential for their growth and their involvement to the improvement of the final product quality (Mortensen et al., 2005). Many microorganisms such as Pseudomonas aeruginosa, S. cerevisiae, C. albicans, and Candida glabrata can form biofilms (Hawser and Douglas, 1994; Reynolds and Fink, 2001; Gaur and Klotz, 2004; Hall-Stoodley et al., 2004; Otoo et al., 2008; Bojsen et al., 2012; Granger, 2018). Genetic and phenotypic diversity, reported for cells living in biofilms, permits to have more resistance than cells in free-living planktonic form (Andersen et al., 2014). Membrane and cell wall properties seem to be modulated by environmental and nutritional factors, that may affect CSH. There are also evidences that the presence of particular proteins, such as p37, can be correlated to the ability of the K. marxianus cells to flocculate with high temperatures as a mechanism of response to thermic stress (Moreira et al., 1998).
Therefore, in this study 33 K. marxianus strains, were tested for their adhesion properties in terms of biofilm and mats formation and CSH. Pasteurized whey was considered to verify these processes in a real system, and not only in a synthetic media. It was not possible to find an association between this parameter and yeast adhesion on abiotic surfaces in agreement with other authors (Raut et al., 2010; Ichikawa et al., 2017; Perpetuini et al., 2018a), suggesting that hydrophobicity can’t be used as predictor of biofilm formation. The capacity to form mat structure and biofilm suggest the ability to adhere to industrial surfaces, which may include the type of equipment and the nature of surfaces. Moreover, it may be useful to form associations with other microorganisms. Like S. cerevisiae wild type strains isolated from must and grapes (Sidari et al., 2014) the K. marxianus strains analyzed in this work, were able to form diverse mat structures in terms of size and architecture (Figure 2). The genome differences may explain these variances as suggested by Borneman et al. (2008, 2011, 2016) for wine strains.
The molecular processes on the base of biofilm development and biofilm cell diversification in bacteria has been studied extensively, however, less is known in eukaryotic cells such as yeasts (Andersen et al., 2014). To better visualize the molecular processes, the pathways that involve the genes analyzed in this study are shown in Figure 4B.
To study a possible correlation between genotype and phenotype, we studied the expression of four genes involved in biofilm formation and stress response in S. cerevisiae. The overexpression of FLO11 gene agrees with previous observations on S. cerevisiae (Figure 5). The S. cerevisiae strain S1278b can form biofilm in liquid and solid surfaces thanks to the expression of Flo11p. Other than biofilm formation, this protein is also responsible for other morphotypes, such as haploid-invasive growth and diploid-pseudohyphal growth (Reynolds and Fink, 2001). Similarly, Ste12p is required for the pseudohyphal response in S. cerevisiae (Gustin et al., 1998).
Obtained data revealed a possible marginal role of TPK3 and WSC4 on biofilm formation. Previous studies revealed that Tpk3p has two effects on biofilm formation: repression of FLO11 transcription in biofilms and consequently loss of biofilm (Andersen et al., 2014). In K. marxianus under our conditions TPK3 was not upregulated, with a subsequently expression of FLO11 in sessile cells, in agreement with the Tpk3p role.
We analyzed the expression of the orthologous of YHL028W gene, since it shows similarity to WSC4, a gene involved in mat structure formation in S. cerevisiae (Sarode et al., 2013). However, under our conditions it showed low fold changes compared to other tested genes, suggesting the involvement of other metabolic pathways in K. marxianus.
Conclusion
This study highlighted the ability of K. marxianus dairy strains to form biofilms not only in synthetic media but also in a real substrate such as whey. This trait could be of particular interest at industrial level since yeast adhesion on cheese surfaces is essential for its establishment and growth on the food surface. Moreover, K. marxianus plays an important role in cheese ripening, being essential for the contribution to the final product quality, especially for what concern the production of particular aromatic compounds (Mortensen et al., 2005; Gori et al., 2011). In addition, for the first time the overexpression of FLO11 and STE12 in K. marxianus sessile cells was shown. Since the adhesion of the yeasts is the results of several components, further studies are necessary to examine in depth the role of these genes in K. marxianus adhesion ability and mats formation on cheese surfaces.
Author Contributions
GS and RT designed research and revised manuscript. GP and FT performed experiments and drafted manuscript. All authors approved final version of manuscript.
Conflict of Interest Statement
The authors declare that the research was conducted in the absence of any commercial or financial relationships that could be construed as a potential conflict of interest.
Supplementary Material
The Supplementary Material for this article can be found online at: https://www.frontiersin.org/articles/10.3389/fmicb.2019.00079/full#supplementary-material
FIGURE S1 | Multiple sequence alignment of nucleotide sequences of the genes studied. A phylogenetic tree was drawn with Neighbor-Joining method to show relationships between the orthologous genes in yeasts (light blue: FLO11; Purple: TPK3; Red: WSC4; Green: STE12). The bootstrap analysis was conducted on 1000 replicates.
FIGURE S2 | Multiple sequence alignment of amino acidic sequences of the genes studied. A phylogenetic tree was drawn with Neighbor-Joining method to show relationships between the orthologous genes in yeasts. The bootstrap analysis was conducted on 1000 replicate.
Footnotes
- ^ http://ygob.ucd.ie/
- ^ www.yeastgenome.org
- ^ https://blast.ncbi.nlm.nih.gov/Blast.cgi
- ^ https://www.genome.jp/kegg/genes.html
- ^ http://www.bioinformatics.nl/cgi-bin/primer3plus/primer3plus.cgi/
- ^ http://www.ebi.ac.uk/blastall/nucleotide.html
References
Andersen, C. L., Jensen, J. L., and Ørntoft, T. F. (2004). Normalization of real time quantitative reverse transcription-PCR data: a model-based variance estimation approach to identify genes suited for normalization, applied to bladder and colon cancer data sets. Cancer Res. 64, 5245–5250. doi: 10.1158/0008-5472.CAN-04-0496
Andersen, K. S., Bojsen, R. K., Sørensen, L. G. R., Weiss Nielsen, M., Lisby, M., Folkesson, A., et al. (2014). Genetic basis for Saccharomyces cerevisiae biofilm in liquid medium. G3 4, 1671–1680. doi: 10.1534/g3.114.010892
Arena, M. P., Capozzi, V., Spano, G., and Fiocco, D. (2017). The potential of lactic acid bacteria to colonize biotic and abiotic surfaces and the investigation of their interactions and mechanisms. Appl. Microbiol. Biotechnol. 101, 2641–2657. doi: 10.1007/s00253-017-8182-z
Bellon-Fontaine, M. N., Rault, J., and Van Oss, C. J. (1996). Microbial adhesion to solvents: a novel method to determine the electron-donor/electron-acceptor or lewis acid-base properties of microbial cells. Colloids Surf. B Biointerfaces. 7, 47–53. doi: 10.1016/0927-7765(96)01272-6
Bojsen, R. K., Andersen, K. S., and Regenberg, B. (2012). Saccharomyces cerevisiae - a model to uncover molecular mechanisms for yeast biofilm biology. FEMS Immunol. Med. Microbiol. 65, 169–182. doi: 10.1111/j.1574-695X.2012.00943.x
Borneman, A. R., Desany, B. A., Riches, D., Affourtit, J. P., Forgan, A. H., Pretorius, I. S., et al. (2011). Whole-genome comparison reveals novel genetic elements that characterize the genome of industrial strains of Saccharomyces cerevisiae. PLoS Genet. 7:e1001287. doi: 10.1371/journal.pgen.1001287
Borneman, A. R., Forgan, A. H., Kolouchova, R., Fraser, J. A., and Schmidt, S. A. (2016). Whole genome comparison reveals high levels of inbreeding and strain redundancy across the spectrum of commercial wine strains of Saccharomyces cerevisiae. G3 6, 957–971. doi: 10.1534/g3.115.025692
Borneman, A. R., Forgan, A. H., Pretorius, I. S., and Chambers, P. J. (2008). Comparative genome analysis of a Saccharomyces cerevisiae wine strain. FEMS Yeast Res. 8, 1185–1195. doi: 10.1111/j.1567-1364.2008.00434.x
Bowen, W. R., Lovitt, R. W., and Wright, C. J. (2001). Atomic force microscopy study of the adhesion of Saccharomyces cerevisiae. J. Colloid Interface Sci. 237, 54–61. doi: 10.1006/jcis.2001.7437
Byrne, K. P., and Wolfe, K. H. (2005). The yeast gene order browser: combining curated homology and syntenic context reveals gene fate in polyploid species. Genome Res. 15, 1456–1461. doi: 10.1101/gr.3672305
Byrne, K. P., and Wolfe, K. H. (2006). Visualizing syntenic relationships among the hemiascomycetes with the yeast gene order browser. Nucleic Acids Res. 34, 452–455. doi: 10.1093/nar/gkj041
Chaffin, W. L., López-Ribot, J. L., Casanova, M., Gozalbo, D., and Martínez, J. P. (1998). Cell wall and secreted proteins of Candida albicans: identification, function, and expression. Microbiol. Mol. Biol. Rev. 62, 130–180.
Coloretti, F., Chiavari, C., Luise, D., Tofalo, R., Fasoli, G., Suzzi, G., et al. (2017). Detection and identification of yeasts in natural whey starter for Parmigiano Reggiano cheese-making. Int. Dairy J. 66, 13–17. doi: 10.1016/j.idairyj.2016.10.013
Di Gianvito, P., Tesnière, C., Suzzi, G., Blondin, B., and Tofalo, R. (2017). FLO5 gene controls flocculation phenotype and adhesive properties in a Saccharomyces cerevisiae sparkling wine strain. Sci. Rep. 7:10786. doi: 10.1038/s41598-017-09990-9
Di Gianvito, P., Tesnière, C., Suzzi, G., Blondin, B., and Tofalo, R. (2018). Different genetic responses to oenological conditions between a flocculent wine yeast and its FLO5 deleted strain: insights from the transcriptome. Food Res. Int. 114, 178–186. doi: 10.1016/j.foodres.2018.07.061
Fasoli, G., Barrio, E., Tofalo, R., Suzzi, G., and Belloch, C. (2016). Multilocus analysis reveals large genetic diversity in Kluyveromyces marxianus strains isolated from parmigiano reggiano and pecorino di farindola cheeses. Int. J. Food Microbiol. 233, 1–10. doi: 10.1016/j.ijfoodmicro.2016.05.028
Fasoli, G., Tofalo, R., Lanciotti, R., Schirone, M., Patrignani, F., Perpetuini, G., et al. (2015). Chromosome arrangement, differentiation of growth kinetics and volatile molecule profiles in Kluyveromyces marxianus strains from Italian cheeses. Int. J. Food Microbiol. 214, 151–158. doi: 10.1016/j.ijfoodmicro.2015.08.001
Flemming, H. C., and Wingender, J. (2010). The biofilm matrix. Nat. Rev. Microbiol. 8, 623–633. doi: 10.1038/nrmicro2415
Fonseca, G. G., Heinzle, E., Wittmann, C., and Gombert, A. K. (2008). The yeast Kluyveromyces marxianus and its biotechnological potential. Appl. Microbiol. Biotechnol. 79, 339–354. doi: 10.1007/s00253-008-1458-6
Gaur, N. K., and Klotz, S. A. (2004). Accessibility of the peptide backbone of protein ligands is a key specificity determinant in Candida albicans SRS adherence. Microbiology 150, 277–284. doi: 10.1099/mic.0.26738-0
Goossens, K., and Willaert, R. (2010). Flocculation protein structure and cell-cell adhesion mechanism in Saccharomyces cerevisiae. Biotechnol. Lett. 32, 1571–1585. doi: 10.1007/s10529-010-0352-3
Gori, K., Knudsen, P. B., Nielsen, K. F., Arneborg, N., and Jespersen, L. (2011). Alcohol-based quorum sensing plays a role in adhesion and sliding motility of the yeast Debaryomyces hansenii. FEMS Yeast Res. 11, 643–652. doi: 10.1111/j.1567-1364.2011.00755.x
Granger, B. L. (2018). Accessibility and contribution to glucan masking of natural and genetically tagged versions of yeast wall protein 1 of Candida albicans. PLoS One 13:e0191194. doi: 10.1371/journal.pone.0191194
Gustin, M. C., Albertyn, J., Alexander, M., and Davenport, K. (1998). MAP kinase pathways in the yeast Saccharomyces cerevisiae. Microbiol. Mol. Biol. Rev. 62, 1264–1300.
Hall-Stoodley, L., Costerton, J. W., and Stoodley, P. (2004). Bacterial biofilms: from the natural environment to infectious diseases. Nat. Rev. Microbiol. 2:95. doi: 10.1038/nrmicro821
Hawser, S. P., and Douglas, L. J. (1994). Biofilm formation by Candida species on the surface of catheter materials in vitro. Infect. Immun. 62, 915–921.
Hostetter, M. K. (1999). Integrin-like proteins in Candida spp. and other microorganisms. Fungal Genet. Biol. 28, 135–145. doi: 10.1006/fgbi.1999.1165
Ichikawa, T., Hirata, C., Takei, M., Tagami, N., Murasawa, H., and Ikeda, R. (2017). Cell surface hydrophobicity and colony morphology of Trichosporon asahii clinical isolates. Yeast 34, 129–137. doi: 10.1002/yea.3220
Kumar, S., Stecher, G., and Tamura, K. (2016). MEGA7: molecular evolutionary genetics analysis version 7.0 for bigger datasets. Mol. Biol. Evol. 33, 1870–1874. doi: 10.1093/molbev/msw054
Lane, M. M., and Morrissey, J. P. (2010). Kluyveromyces marxianus: a yeast emerging from its sister’s shadow. Fungal Biol. Rev. 24, 17–26. doi: 10.1016/j.fbr.2010.01.001
Legras, J. L., Moreno-García, J., Zara, S., Zara, G., Garcia-Martinez, T., Mauricio, J. C., et al. (2016). Flor yeast: new perspectives beyond wine aging. Front. Microbiol. 7:503. doi: 10.3389/fmicb.2016.00503
Livak, K. J., and Schmittgen, T. D. (2001). Analysis of relative gene expression data using real-time quantitative PCR and the 2–ΔΔCT method. Methods 25, 402–408. doi: 10.1006/meth.2001.1262
Moreira, R. F., Fernandes, P. A., and Moradas-Ferreira, P. (1998). Kluyveromyces marxianus flocculence and growth at high temperature is dependent on the presence of the protein p37. Microbiology 144, 681–688. doi: 10.1099/00221287-144-3-681
Moreno-García, J., Coi, A. L., Zara, G., García-Martinez, T., Mauricio, J. C., and Budroni, M. (2018). Study of the role of the covalently linked cell wall protein (Ccw14p) and yeast glycoprotein (Ygp1p) within biofilm formation in a flor yeast strain. FEMS Yeast Res. 18:foy005. doi: 10.1093/femsyr/foy005
Mortensen, H. D., Gori, K., Jespersen, L., and Arneborg, N. (2005). Debaryomyces hansenii strains with different cell sizes and surface physicochemical properties adhere differently to a solid agarose surface. FEMS Microbiol. Lett. 249, 165–170. doi: 10.1016/j.femsle.2005.06.009
Otoo, H. N., Lee, K. G., Qiu, W., and Lipke, P. N. (2008). Candida albicans Als adhesins have conserved amyloid-forming sequences. Eukaryot. Cell. 7, 776–782. doi: 10.1128/EC.00309-07
Pérez-Torrado, R., and Querol, A. (2016). Opportunistic strains of Saccharomyces cerevisiae: a potential risk sold in food products. Front. Microbiol. 6:1522. doi: 10.3389/fmicb.2015.01522
Perpetuini, G., Tittarelli, F., Mattarelli, P., Modesto, M., Cilli, E., Suzzi, G., et al. (2018a). Intraspecies polymorphisms of Kluyveromyces marxianus strains from yaghnob valley. FEMS Microbiol. Lett. 365:fny028. doi: 10.1093/femsle/fny028
Perpetuini, G., Tittarelli, F., Schirone, M., Di Gianvito, P., Corsetti, A., Arfelli, G., et al. (2018b). Adhesion properties and surface hydrophobicity of Pichia manshurica strains isolated from organic wines. LWT Food Sci. Technol. 87, 385–392. doi: 10.1016/j.lwt.2017.09.011
Piard, J. C., and Briandet, R. (2015). “Lactic acid bacteria biofilms: from their formation to their health and biotechnological potential,” in Biotechnology of Lactic Acid Bacteria: Novel Applications, eds F. Mozzi, R. R. Raya, and G. M. Vignolo (Hoboken: Wiley-Blackwell), 341–361. doi: 10.1002/9781118868386.ch20
Raut, J., Rathod, V., and Karuppayil, S. M. (2010). Cell surface hydrophobicity and adhesion: a study on fifty clinical isolates of Candida albicans. Nippon Ishinkin Gakkai Zasshi 51, 131–136. doi: 10.3314/jjmm.51.131
Reynolds, T. B. (2006). The Opi1p transcription factor affects expression of FLO11, mat formation, and invasive growth in Saccharomyces cerevisiae. Eukaryot. Cell. 5, 1266–1275. doi: 10.1128/EC.00022-06
Reynolds, T. B., and Fink, G. R. (2001). Bakers’ yeast, a model for fungal biofilm formation. Science 291, 878–881. doi: 10.1126/science.291.5505.878
Sampermans, S., Mortier, J., and Soares, E. V. (2005). Flocculation onset in Saccharomyces cerevisiae: the role of nutrients. J. Appl. Microbiol. 98, 525–531. doi: 10.1111/j.1365-2672.2004.02486.x
Sarode, N., Davis, S. E., Tams, R. N., and Reynolds, T. B. (2013). The Wsc1p cell wall signaling protein controls biofilm (Mat) formation independently of Flo11p in Saccharomyces cerevisiae. G3 4, 199–207. doi: 10.1534/g3.113.006361
Sidari, R., Caridi, A., and Howell, K. S. (2014). Wild Saccharomyces cerevisiae strains display biofilm-like morphology in contact with polyphenols from grapes and wine. Int. J. Food Microbiol. 189, 146–152. doi: 10.1016/j.ijfoodmicro.2014.08.012
Teste, M. A., Duquenne, M., François, J. M., and Parrou, J. L. (2009). Validation of reference genes for quantitative expression analysis by real-time RT-PCR in Saccharomyces cerevisiae. BMC Mol. Biol. 10:99. doi: 10.1186/1471-2199-10-99
Tittarelli, F., Varela, J. A., Gethins, L., Stanton, C., Ross, R. P., Suzzi, G., et al. (2018). Development and implementation of multilocus sequence typing to study the diversity of the yeast Kluyveromyces marxianus in Italian cheeses. Microb. Genom. doi: 10.1099/mgen.0.000153 [Epub ahead of print].
Tofalo, R., Fasoli, G., Schirone, M., Perpetuini, G., Pepe, A., Corsetti, A., et al. (2014). The predominance, biodiversity and biotechnological properties of Kluyveromyces marxianus in the production of Pecorino di Farindola cheese. Int. J. Food Microbiol. 187, 41–49. doi: 10.1016/j.ijfoodmicro.2014.06.029
Van Mulders, S. E., Christianen, E., Saerens, S. M., Daenen, L., Verbelen, P. J., Willaert, R., et al. (2009). Phenotypic diversity of Flo protein family-mediated adhesion in Saccharomyces cerevisiae. FEMS Yeast Res. 9, 178–190. doi: 10.1111/j.1567-1364.2008.00462.x
Verstrepen, K. J., Derdelinckx, G., Verachtert, H., and Delvaux, F. R. (2003). Yeast flocculation: what brewers should know. Appl. Microbiol. Biotechnol. 61, 197–205. doi: 10.1007/s00253-002-1200-8
Keywords: Kluyveromyces marxianus, FLO11, STE12, adhesion properties, biofilm formation, mat structure, RT-qPCR
Citation: Perpetuini G, Tittarelli F, Suzzi G and Tofalo R (2019) Cell Wall Surface Properties of Kluyveromyces marxianus Strains From Dairy-Products. Front. Microbiol. 10:79. doi: 10.3389/fmicb.2019.00079
Received: 08 November 2018; Accepted: 15 January 2019;
Published: 31 January 2019.
Edited by:
Matthias Sipiczki, University of Debrecen, HungaryReviewed by:
Pedro Moradas Ferreira, Universidade do Porto, PortugalGiuseppe Spano, University of Foggia, Italy
Anna Maria Maraz, Szent István University, Hungary
Copyright © 2019 Perpetuini, Tittarelli, Suzzi and Tofalo. This is an open-access article distributed under the terms of the Creative Commons Attribution License (CC BY). The use, distribution or reproduction in other forums is permitted, provided the original author(s) and the copyright owner(s) are credited and that the original publication in this journal is cited, in accordance with accepted academic practice. No use, distribution or reproduction is permitted which does not comply with these terms.
*Correspondence: Rosanna Tofalo, cnRvZmFsb0B1bml0ZS5pdA==
†These authors have contributed equally to this work