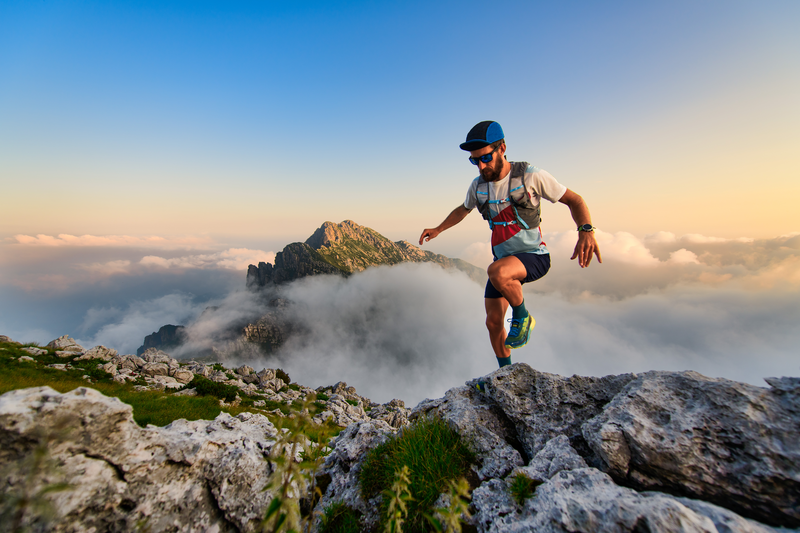
95% of researchers rate our articles as excellent or good
Learn more about the work of our research integrity team to safeguard the quality of each article we publish.
Find out more
REVIEW article
Front. Microbiol. , 20 November 2018
Sec. Infectious Agents and Disease
Volume 9 - 2018 | https://doi.org/10.3389/fmicb.2018.02751
This article is part of the Research Topic Viral and Bacterial Infections Leading to “Non-Infectious” Diseases: Unveiling the Missing Links View all 8 articles
Mycoplasma pneumoniae is mainly recognized as a respiratory pathogen, although it is associated with the development of several extra-respiratory conditions in up to 25% of the cases. Diseases affecting the nervous system, both the peripheral (PNS) and the central nervous system (CNS), are the most severe. In some cases, particularly those that involve the CNS, M. pneumoniae-related neuropathies can lead to death or to persistent neurologic problems with a significant impact on health and a non-marginal reduction in the quality of life of the patients. However, the pathogenesis of most of the M. pneumoniae-related neuropathies remains undefined. The main aim of this paper is to discuss what is presently known regarding the pathogenesis and treatment of the most common neurologic disorders associated with M. pneumoniae infection. Unfortunately, the lack of knowledge of the true pathogenesis of most of the cases of M. pneumoniae-mediated neurological diseases explains why treatment is not precisely defined. However, antibiotic treatment with drugs that are active against M. pneumoniae and able to pass the blood-brain barrier is recommended, even though the best drug, dosage, and duration of therapy have not been established. Sporadic clinical reports seem to indicate that because immunity plays a relevant role in the severity of the condition and outcome, attempts to reduce the immune response can be useful. However, further studies are needed before the problem of the best therapy for M. pneumoniae-mediated neurological diseases can be efficiently solved.
Mycoplasma pneumoniae is a cell-wall deficient organism that extracellularly infects the respiratory tract as filamentous forms that adhere to respiratory epithelial cells (Principi and Esposito, 2001). It is transmitted by the respiratory route, and the incubation period from infection to disease is roughly 2–4 weeks. It is ubiquitous, active throughout the year, and there is evidence that it causes endemic infections with periodic epidemics at 4–7 year intervals (Principi and Esposito, 2001). Outbreaks can happen in institutional settings such as schools and summer camps.
Mycoplasma pneumoniae is mainly recognized as a respiratory pathogen. It is one of the major causes of both upper and lower respiratory tract infection in children and adults. More than 20% of non-streptococcal pharyngitis cases are due to M. pneumoniae, with an even higher incidence among children with recurrent episodes (Esposito et al., 2004). Up to 40% of cases of community-acquired pneumonia (CAP) are associated with a diagnosis of M. pneumoniae infection, both in children and adults (Vergis and Yu, 1997; Woodhead, 1998; Ruiz-González et al., 1999; Principi et al., 2001; Baer et al., 2003; Michelow et al., 2004). Moreover, some patients with M. pneumoniae infection experience bronco-obstructive signs and symptoms, and M. pneumoniae is presently considered a trigger for asthma exacerbations (Esposito et al., 2000; Watanabe et al., 2014).
However, in addition to respiratory diseases, M. pneumoniae is associated with the development of several extra-respiratory conditions in a great number of cases without a previous, clinically evident respiratory episode (Narita, 2016). Up to 25% of M. pneumoniae respiratory infections have reportedly been complicated by the involvement of various extra-respiratory sites (de Groot et al., 2017). According to the most recent observations (Narita, 2016), extra-respiratory manifestations of M. pneumoniae infection include diseases of the skin, the urogenital tract, and some sensory and digestive organs, as well as the cardiovascular, haematopoietic, musculoskeletal and nervous systems. Diseases due to M. pneumoniae affecting the nervous system, both the peripheral (PNS) and the central nervous system (CNS), are the most difficult to be diagnosed and treated, and represent a real medical emergency. They are reported in 5% of hospitalized patients whereas M. pneumoniae positivity can be detected in 5–10% of patients presenting with acute, febrile CNS disease (Lind et al., 1979; Ponka, 1980; Yiş et al., 2008; Pillai et al., 2015). In some cases, particularly those that involve the CNS, M. pneumoniae-related neuropathies can lead to death or to persistent neurologic problems with a significant impact on health and a non-marginal reduction in the quality of life of the patients (Kammer et al., 2016; Narita, 2016). Several studies have tried to understand how M. pneumoniae can affect the nervous system and why different clinical manifestations of neurological damage can occur (Waites and Talkington, 2004; Narita, 2010; de Groot et al., 2017). However, the results are disappointing, and the pathogenesis of most of the M. pneumoniae-related neuropathies remains undefined. The inability to establish a direct relationship between a previous M. pneumoniae infection and the development of neurological signs and symptoms is the main reason for this limitation. As previously reported, neurological impairment frequently occurs without the respiratory symptoms that can lead clinicians to consider and diagnose a M. pneumoniae infection. Moreover, when present, respiratory findings are not specific. Finally, laboratory confirmation of M. pneumoniae infection is difficult. Its culture is complicated and slow, and serologic tests, which are considered to be the gold standard for the diagnosis of M. pneumoniae infection, are only really effective in the identification of M. pneumoniae cases when both acute-phase and convalescent-phase serum specimens are available. This limitation makes them useless for the etiological diagnosis of acute diseases, unless retrospectively. The combined use of acute-phase serology and molecular biology tests able to identify M. pneumoniae DNA has been suggested (Qu et al., 2013). Unfortunately, evidence of M. pneumoniae DNA in upper respiratory secretions in some cases can represent a carrier state as some healthy children especially during outbreaks of M. infection were found to carry the pathogen in the nasopharynx (Spuesens et al., 2013). Definitive diagnosis can be made when DNA is detected in the nervous tissue or in the cerebrospinal fluid (CSF). However, in addition to the difficulty of obtaining adequate samples for analysis, M. pneumoniae DNA can be undetectable even in cases suggesting M. pneumoniae CNS disease (Narita et al., 1992).
For the reasons reported above, the pathogenesis of M. pneumoniae-related neuropathies remains frequently unknown. This has important consequences for the treatment of these forms, which remains, even today, based on what has been established for neuropathies with similar symptoms but not strictly linked to M. pneumoniae infection. The main aim of this paper is to discuss what is presently known regarding the pathogenesis and treatment of the most common neurologic disorders associated with M. pneumoniae infection. The following keywords were used to search among the world medical library collections (Medline, Embase, Cochrane and Cinahl): “Mycoplasma pneumoniae” and “peripheral nervous system” or “CNS” or “neuropathy.” We covered the period between January 1, 2007, and December 31, 2017. A total of 267 papers were analyzed. Only papers written in English were considered.
The neurological manifestations of M. pneumoniae infections have been thought to derive from three different mechanisms. A direct type in which damage of nervous tissue is strictly related to the local activity of M. pneumoniae, an indirect type mainly based on autoimmunity, and a vascular type in which local vasculitis or thrombotic vascular occlusion occur through a direct or indirect mechanism (Narita, 2010). The mechanisms are not mutually exclusive, explaining why, in some patients, lesions related to different pathogeneses can be detected.
Mycoplasma pneumoniae infecting the respiratory tract can be transferred to the CNS through gaps between epithelial respiratory cells and cause direct structural and functional lesions in different organs and body systems, including the CNS (Narita et al., 1996). As extra-respiratory manifestations of M. pneumoniae infection have been described more commonly in children than in adults (Narita, 2010) and have been repeatedly described in immunocompromised patients (Taylor-Robinson et al., 1978; O’Sullivan et al., 2004), M. pneumoniae transfer to the CNS was supposed to be more frequent in subjects with a partially defective immune system (Narita, 2010). However, transfer to the CNS has been substantiated by the detection of M. pneumoniae DNA in the CSF or in the blood of some, even if not all, the patients with a clinically and serologically (i.e., by the presence of antibodies against M. pneumoniae in serum) confirmed M. pneumoniae infection. The genome of this atypical pathogen was detected in CSF in 1 of the 25 patients with pneumonia and 10 of the 17 patients without pneumonia (Narita et al., 1996; Narita, 2000).
When the pathogen reaches the CNS, it can cause damage directly. Generally, it is hypothesized that this damage occurs within 7 days of the respiratory infection, explaining why CNS diseases of the direct type are defined as early-onset diseases. The ability of M. pneumoniae to immediately damage host cells is evidenced by experimental studies that have measured the impact of M. pneumoniae infection on the epithelial cells of the respiratory tract. M. pneumoniae has been shown to adhere to glycoproteins and glycolipids of target cells through the activity of a number of proteins contained in an organelle located at the pole of the pathogen (Chaudhry et al., 2007). P1 adhesin is the main factor responsible for binding, as shown by the evidence that genetically modified M. pneumoniae strains lacking this adhesion factor cannot cause infection on a variety of human cells in culture (Kahane, 1984). Moreover, this protein, together with other adhesins (P30, P40 and P90), confers sliding motility to M. pneumoniae, thereby favoring the infectious properties of M. pneumoniae. Other proteins (HMW-1, HMW-2, HMW-3 and proteins A, B, and C), although not adhesins, seem essential for M. pneumoniae cell attachment (Chaudhry et al., 2016). Interestingly, whereas the immunodominant epitopes of the P1 protein are highly variable, the adherence-mediating domains are conserved in HEp-2 cells lines (Chourasia et al., 2014).
As the host immune response is mainly against immunodominant epitopes (Kim et al., 2011), host defenses are partially ineffective in reducing or eliminating pathogen adhesions. Following adhesion, M. pneumoniae damages respiratory cells through the generation of hydrogen peroxide and superoxide radicals, which cause oxidative stress and lead to the structural and functional deterioration of cilia with decreased alveolar fluid clearance (Waites and Talkington, 2004). Damage is also produced by a cytotoxin named community-acquired respiratory distress syndrome toxin that has been found capable of inducing vacuolization, karyopyknosis, and loss of epithelial integrity (Kannan and Baseman, 2006; Hardy et al., 2009). This mechanism could have a role also in CNS diseases due to M. pneumoniae.
Finally, some studies showed that M. pneumoniae harbors a large number of lipoprotein genes, most of which are of unknown function (Hallamaa et al., 2006, 2008). Because of their location on the cell surface, these proteins are likely to be involved in the bacterial response to environmental changes, or in the initial stages of infection. In particular, some authors showed that several lipoproteins of M. pneumoniae (MALP2, P48, M161Ag, N-ALP1/NALP2, and F0F1-ATPase) seem to stimulate the host immune system via Toll-like receptors (TLRs), mainly TLR1, TLR2, and TLR6 (Andrews et al., 2013). This stimulation could promote the production of cytokines and chemokines and the development of the humoural and cellular immune response, leading to the histological findings seen in human M. pneumoniae CAP (Miyashita et al., 2017). Similar pathogenetic mechanisms can be supposed for early-onset CNS M. pneumoniae diseases, as increased cytokine and chemokine concentrations have been found in patients with these diseases (Narita et al., 2005). Narita et al. (2005) examined interleukin (IL)-6, IL-8, IL-18, interferon (INF)-γ, tumour necrosis factor (TNF)-α, and transforming growth factor (TGF)-beta 1 in serum and CFS samples from patients with several CNS manifestations during acute M. pneumoniae infection. A significant increase in the concentrations of IL-6 and IL-8 in the CSF of children with early-onset encephalitis and aseptic meningitis was found. Unfortunately, M. pneumoniae DNA and cytokines cannot be detected in the CSF of all cases of early-onset disease, highlighting the difficulty of the identification of the true pathogenetic mechanisms of a M. pneumoniae -related neuropathy.
Some patients suffer from a neurologic disease several days after the onset of a M. pneumoniae respiratory episode. When the time passed from the initial M. pneumoniae infection is ≥8 days, neurologic manifestations are considered to be a late-onset disease. In these conditions, unlike what can occur in several early-onset diseases, M. pneumoniae DNA is only exceptionally detected in the CSF (Narita et al., 1995; Meyer Sauteur et al., 2014). In contrast, in a relevant number of cases, antibodies to M. pneumoniae can be found in the CSF and/or in the serum, suggesting that late-onset diseases are mainly based on autoimmunity (Barbagallo et al., 2017). Molecular mimicry between some M. pneumoniae components and host myelin glycolipids is considered the pathomechanism of this immune-mediated process (Kusunoki et al., 2001). In particular, M. pneumoniae P1 adhesin and glycolipids of the pathogen form a galactocerebroside C (GalC)-like structure that elicit cross-reactive antibody responses against myelin components of the host, i.e., GalC and gangliosides that cross-react with GalCs such as GQ1B (Kuwahara et al., 2017).
The relevance of M. pneumoniae components as antigens is supported by the evidence that, in experimental animal models, sensitization with GalC causes demyelinating polyneuropathy (Saida et al., 1981). Moreover, detection of antibodies against GalC and gangliosides in patients with PNS diseases is relatively common (Sauteur et al., 2015; Meyer Sauteur et al., 2016a; Kuwahara et al., 2017). Anti-M. pneumoniae antibodies that cross-react with myelin components of the host can be detected in both the serum and in the CSF of patients with M. pneumoniae-related neuropathies. However, when they are only detected in the serum, their importance in the acute phase of these diseases is debated as they can be a marker of a previous infection occurred in absence of nervous system involvement (Nishimura et al., 1996; Kikuchi et al., 1997; Kumada et al., 1997; Ang et al., 2002; Susuki et al., 2004; Christie et al., 2007a; Sugeno et al., 2012). In contrast, detection of anti-M. pneumoniae antibodies in the CSF, particularly without evidence of M. pneumoniae DNA, seems to be more reliable evidence of the indirect pathogenesis of a number of CNS diseases. In these conditions, antibodies can be produced intrathecally, as evidenced by the higher level of antibodies detected in the CSF than in the serum, cross the blood-brain barrier, or reach the CNS through the blood-brain barrier due to increased permeability (Jacobs et al., 1995; Christie et al., 2007a). In this case, increased blood-brain barrier permeability can be hypothesized to depend on cytokine inflammation mediated by the direct effect of M. pneumoniae, suggesting a combined pathogenetic mechanism.
Neurologic syndromes of the vascular type have been described as disorders of vascular origin involving both direct and indirect mechanisms. Therefore, M. pneumoniae may locally induce cytokines and chemokines, such as TNF-α and IL-8, which cause local vasculitic or thrombotic vascular occlusion without a systemic hypercoagulable state (direct type). Alternatively, a generalized thrombotic vascular occlusion may occur as a result of a systemic hypercoagulable state, which is related to an immune-mediated activation of chemical mediators such as fibrin-D-dimer and activated complements (indirect type) (Narita, 2010).
As previously evidenced, the pathogenetic mechanisms of neurologic M. pneumoniae-mediated diseases are not mutually exclusive. This overlap explains why it is very difficult to attribute a single pathogenetic mechanism to each of the various M. pneumoniae-related neurological diseases. An exception is made for aseptic meningitis, for which available evidence seems to indicate that the disease is directly caused by M. pneumoniae. In several cases, CSF analysis has led to the identification of M. pneumoniae DNA and to increased IL-6 and IL-8 concentrations. Moreover, no M. pneumoniae antigens have ever been detected in the CNS (Narita et al., 1995; Socan et al., 2001).
Encephalitis and transverse myelitis can be due to both direct and indirect pathogeneses. M. pneumoniae encephalitis accounts for 5–30% of all of the reported encephalitis cases, about two-thirds of which occur in children (Christie et al., 2007b). M. pneumoniae encephalitis seems to be more commonly due to an indirect mechanism as shown by the low number of cases with positive DNA detection in the CSF. In the United States, among 111 patients with M. pneumoniae encephalitis, M. pneumoniae DNA was only detected in two cases (Christie et al., 2007a). The low prevalence of M. pneumoniae DNA in the CSF has also been reported in studies carried out in Suisse (Meyer Sauteur et al., 2016b), France (Mailles et al., 2009), the United Kingdom (Granerod et al., 2010), and Canada (Bitnun et al., 2001).
Similar conclusions can be drawn for transverse myelitis (Abele-Horn et al., 1998). In a study in which neurological complications of polymerase chain reaction (PCR)-proven M. pneumoniae infection in children were evaluated, 12% of the 365 enrolled children had definitive, probable or possible transverse myelitis, but most had prodrome symptoms ≥7 days and serologic evidence of antibodies against M. pneumoniae but no presence of M. pneumoniae DNA in the CSF (Al-Zaidy et al., 2015). Cerebellitis, opsoclonus-myoclonus syndrome, cranial/peripheral neuropathies, and acute disseminated encephalomyelitis (ADEM) are mainly considered to be due to an indirect pathogenesis, although in some cases, data suggesting a possible direct pathogenesis have been collected. Cerebellitis has been described to be a part of encephalitis and an isolated condition. An indirect pathogenesis was mainly suggested by the long latency between respiratory and neurological symptoms (Schmucker et al., 2014). However, in a case, nervous tissue infiltration with macrophages and lymphocytes, typical of directly determined cases, seemed to indicate a possible pathogenetic alternative (Simpkins et al., 2012). ADEM is known as an immune complex-mediated vasculopathy in which circulating immune complexes are deposited in small venules in the CNS, leading to activation of the serum complement system. An immune-mediated pathogenesis seemed true also for cases secondary to M. pneumoniae infection, as suggested by the long latency between infection and brain damage development and the evidence that treatment with anti-inflammatory and immunosuppressive drugs together with plasma exchange can improve the clinical course of the disease (Gupta et al., 2009). However, doubts are raised by the detection of M. pneumoniae DNA in the CSF of a patient with M. pneumoniae-related ADEM (Matsumoto et al., 2009).
The pathogenesis of mild encephalitis/encephalopathy with a reversible splenial lesion (MERS) is undefined. This is a recently described clinical-radiological syndrome in which neurological dysfunction, including delirious behavior, consciousness disturbance and seizures, is reported in association with damage of the splenium of the corpus callosum alone or in conjunction with the adjacent white matter (Ueda et al., 2016; Yuan et al., 2016). MERS is classified as an autoimmune disease because M. pneumoniae DNA is usually not detected in the CSF. However, in a recent review of the described cases, MERS was classified as an early-onset disease (Ueda et al., 2016). Moreover, in one case, increased IL-6 concentrations were found (Yuan et al., 2016). Both of these findings suggest a direct pathogenesis.
GBS is the prototype of autoimmune disorders that follow an infection, and M. pneumoniae is considered among the most common causes of this disease. Approximately 15% of GBS cases are associated with a previous M. pneumoniae infection, placing this infectious agent in second place after Campylobacter jejuni in the list of the most common infectious causes of GBS (Sinha et al., 2007; Esposito and Longo, 2017). However, the role of M. pneumoniae in GBS is debated, mainly because antibodies against GalC and gangliosides are detected in only some but not all cases of the disease and can also be observed in healthy controls, although at a lower rate. In a study, serum anti- M. pneumoniae antibodies were detected in 3% of adults and 21% of children with GBS and in 0 and 7% of healthy adults and children, respectively (Meyer Sauteur et al., 2016a). Serum anti-GalC antibodies were found in 4% of adults and 25% of children with GBS and in none of controls. The CSF detection of anti-GalC antibodies supports autoimmune pathogenesis, even in rare cases of GBS with CNS involvement. However, a direct mechanism is suggested by the report of a case with M. pneumoniae DNA in the CSF without any antibody detection (Meyer Sauteur et al., 2014).
Regarding cases of vasculitis or vasculopathy among the M. pneumoniae-associated CNS pathological conditions, stroke and striatal necrosis are those most commonly studied. Stroke can occur in both children and adults, although rarely (Socan et al., 2001; Leonardi et al., 2005). Early presentation was common, and in some of these cases, the pathogen was detected in the CSF, suggesting a direct effect (Padovan et al., 2001). Bilateral striatal necrosis, which resembles a vascular CNS disease called acute necrotizing encephalopathy, is thought to be due to vascular damage. Also, in this case, M. pneumoniae damage seems to be of the direct type as the pathogen was found in the CSF of a patient with this disease (Esposito and Longo, 2017).
The proper treatment of neurologic manifestations of M. pneumoniae infection is debated. Most of the authors agree with the idea that an antibiotic should be systematically administered regardless of the type of disease and its supposed pathogenesis, but the choice of the best drug to prescribe in each patient is frequently discordant (Principi and Esposito, 1999). Moreover, poor agreement also exists on the need to administer immunoglobulins and/or immunosuppressive drugs in order to limit autoimmunity-related damage.
Available data seem to indicate that a systematic antibiotic treatment is mandatory in the treatment of all M. pneumoniae-related neurological diseases. In early-onset cases with direct CNS damage, eradication of the infecting pathogen from the CSF seems essential to avoid its cytolytic activity and activation of the immune system with cytokine and chemokine production. In late-onset diseases, M. pneumoniae eradication from the respiratory tract can interrupt autoimmunity and related alterations. Macrolides are usually considered the drug of choice to treat M. pneumoniae infections for several reasons. First, these drugs have been used to treat these diseases with supposed or demonstrated M. pneumoniae etiology with excellent results for several decades (Waites and Talkington, 2004). Second, macrolides are generally well tolerated, safe and significantly less expensive than more recently developed drugs (Principi and Esposito, 1999). Third, macrolides have a strong immunomodulatory effect. They can enhance or reduce activation of the immune system through several different mechanisms, including regulation of the synthesis and/or secretion of proinflammatory and anti-inflammatory cytokines (Kwiatkowska and Maślińska, 2012). This effect leads to a significant down-regulation of the inflammation and to a positive influence on the outcome of several diseases in which abnormal activation of the immune system and inflammation play a relevant role in the development and persistence of clinical signs and symptoms (López-Boado and Rubin, 2008).
In the case of children, the subjects with the highest risk of M. pneumoniae infection, treatment with macrolides seems even more mandatory as other drugs that are theoretically active against M. pneumoniae cannot be used due to their several limitations. The use of tetracyclines in patients <8 years of age and chloramphenicol in the youngest of these can be followed by severe adverse events, and ketolides and streptogramins have limited used in pediatrics (Centers, 2018). Moreover, fluoroquinolones are not licensed for use in patients younger than 18 years of age with the exception of ciprofloxacin and levofloxacin, which are only recommended for a series of specific clinical conditions as urinary tract infections due to bacteria resistant to other antibiotics and respiratory exacerbations in patients with cystic fibrosis (Jackson and Schutze, 2016). However, the prescription of macrolides can be debated because of emergence of macrolide-resistat strains and the evidence that macrolides do not adequately cross the blood-brain barrier and do not reach effective concentrations in the CSF (Nau et al., 2010; Principi and Esposito, 2013; Pereyre et al., 2016). In neuropathies with direct-type damage, where the therapeutic target is the eradication of the pathogen from the CSF, the main limitation to the use of macrolides is the poor blood-brain barrier passage.
Treatment with alternative antibiotics that are fully effective against M. pneumoniae, such as tetracyclines and fluoroquinolones, has been shown to be effective in shortening the duration of respiratory symptoms and inducing rapid defervescence (Lee et al., 2018). Consequently, in cases with an indirect pathogenesis, there is a risk associated with the use of macrolides, particularly in those geographic areas where the prevalence of resistance is high. For these reasons, the choice of different antibiotics to treat neurological M. pneumoniae diseases seems mandatory. Although not licensed for use in children, quinolones, and in particular levofloxacin, seem the best solution. Levofloxacin adequately penetrates the CSF. Moreover, levofloxacin has been largely used off-label to treat children suffering from severe clinical conditions not treatable with or not responding to first-line antibiotics without any significant adverse event (Principi and Esposito, 2015). Evidence of the effective use of levofloxacin in M. pneumoniae neurologic diseases is given by the report by Esposito et al. (2011). These authors treated five patients with laboratory-confirmed M. pneumoniae-related meningoencephalitis (i.e., three with late-onset disease, one with early-onset disease and one with undetermined disease) with i.v. levofloxacin (25 mg/kg/day in two divided doses) for 14 days, showing a complete clinical recovery at the end of antibiotic administration without any significant adverse events. However, before levofloxacin can be considered the drug of choice for M. pneumoniae neurologic diseases, further data in a greater number of patients should be collected. The real efficacy of levofloxacin should be confirmed, and its drug dosage as well as therapy duration should be more precisely defined.
Diseases characterized by acute severe inflammation and suspected or proved immunologic pathogenesis are usually treated with steroids with or without intravenous immunoglobulins (IVIG). Refractory cases receive plasma exchange (PE) and, if this measure is ineffective, immunosuppressive drugs or biologic response modifiers. Theoretically, all of these types of therapy can be used in M. pneumoniae-related neuropathies. However, adequate evaluation of their effectiveness is available only for diseases involving the PNS, mainly GBS syndrome. However, none of the studies consider the etiology of the GBS cases, and the real importance of treatment in M. pneumoniae-mediated cases is unknown. However, in GBS syndrome, corticosteroids are ineffective (Hughes et al., 2016). In contrast, both IVIG and PE have been found to be able to hasten the recovery in comparison to supportive treatment, particularly when treatment is initiated within 2 weeks of disease onset. No difference between treatments has been demonstrated in comparative studies. However, IVIG administration, due to its greater convenience and availability, is presently considered the first-line treatment for GBS and is recommended in severe cases. The combined use of IVIG and PE does not seem to offer real advantages, although the possibility of significant extra benefits could not be excluded (Hughes et al., 2014). A second IVIG course is suggested for patients who deteriorate in spite of initial therapy (Farcas et al., 1997). Few studies have evaluated the use of steroids, IVIG and immunosuppressive measures in M. pneumoniae neurologic disease with CNS involvement. Theoretically, these therapies could be, at least in part, effective because these treatments may promote the improvement or remission of many immune-mediated encephalopathies (80). On the other hand, with the progressive worsening of M. pneumoniae-associated encephalitis, steroids, IVIG and plasma exchange have been reported to be valid therapeutic options. The potential benefit of immune-modulating therapies is still unclear, but a reasonable consideration is their use in children with neurological symptoms leading to M. pneumoniae-induced autoimmune diseases (Principi et al., 2001).
Several epidemiological and clinical studies have clearly indicated that M. pneumoniae can be associated with several PNS and CNS manifestations. The latency between a previous respiratory M. pneumoniae episode and the development of neurological signs and symptoms can be useful for distinguishing cases due to direct activity of the pathogen from those associated with an autoimmune mechanism. However, this differentiation is possible only in a minority of cases because a relevant number of neurologic diseases occurs without any recent history of respiratory problems. Moreover, even when M. pneumoniae infection is suspected, the identification of a recent episode of M. pneumoniae infection is frequently impossible because the signs and symptoms of the disease are not specific, and laboratory tests do not always offer adequate support. Finally, in cases with laboratory-confirmed M. pneumoniae infection, direct pathogenicity can be supported by the detection of M. pneumoniae DNA and/or inflammatory cytokines and chemokines such as IL-6 and IL-8 in the nervous system.
Unfortunately, evidence of positive tests using these measures is relatively uncommon due to the complexity of the laboratory tests. In addition to the long latency, an indirect pathogenesis can be supported by the presence of M. pneumoniae antibodies that cross-react with myelin components, although these components are difficult to identify and can also be detected, although more rarely, in subjects without neurological problems. The identification of cases with a vascular pathogenesis is even more complicated because, in these diseases, the precise causes of the damage have not been identified. The lack of knowledge of the pathogenesis of most of the cases of M. pneumoniae-mediated neurological diseases explains why treatment is not precisely defined. However, antibiotic treatment with drugs that are active against M. pneumoniae and able to pass the blood-brain barrier is recommended, even though the best drug, dosage, and duration of therapy have not been established. Moreover, some advances, such as the use of IVIG or PE, have been made for PNS M. pneumoniae-associated diseases, including GBS. Further studies should focus on the best therapy for M. pneumoniae-mediated neurological diseases.
RD wrote the first draft of the manuscript. EM, LG, and DL performed the literature review. NP co-wrote and supervised the manuscript. SE critically revised the paper. All authors read and approved the final version of the manuscript.
This study was partially support by a grant of the World Association for Infectious Diseases and Immunological Disorders (WAidid 2017_06).
The authors declare that the research was conducted in the absence of any commercial or financial relationships that could be construed as a potential conflict of interest.
Abele-Horn, M., Franck, W., Busch, U., Nitschko, H., Roos, R., and Heesemann, J. (1998). Transverse myelitis associated with Mycoplasma pneumoniae infection. Clin. Infect. Dis. 26, 909–912. doi: 10.1086/513919
Al-Zaidy, S. A., MacGregor, D., Mahant, S., Richardson, S. E., and Bitnun, A. (2015). Neurological complications of PCR-proven M. pneumoniae infections in children: prodromal illness duration may reflect pathogenetic mechanism. Clin. Infect. Dis. 61, 1092–1098. doi: 10.1093/cid/civ473
Andrews, K., Abdelsamed, H., Yi, A. K., Miller, M. A., and Fitzpatrick, E. A. (2013). TLR2 regulates neutrophil recruitment and cytokine production with minor contributions from TLR9 during hypersensitivity pneumonitis. PLoS One 8:e73143. doi: 10.1371/journal.pone.0073143
Ang, C. W., Tio-Gillen, A. P., Groen, J., Herbrink, P., Jacobs, B. C., Van Koningsveld, R., et al. (2002). Cross-reactive anti-galactocerebroside antibodies and Mycoplasma pneumoniae infections in Guillain-Barré syndrome. J. Neuroimmunol. 130, 179–183. doi: 10.1016/S0165-5728(02)00209-6
Baer, G., Engelcke, G., Abele-Horn, M., Schaad, U. B., and Heininger, U. (2003). Role of Chlamydia pneumoniae and Mycoplasma pneumoniae as causative agents of community-acquired pneumonia in hospitalized children and adolescents. Eur. J. Clin. Microbiol. Infect. Dis. 22, 742–745. doi: 10.1007/s10096-003-1037-9
Barbagallo, M., Vitaliti, G., Pavone, P., Romano, C., Lubrano, R., and Falsaperla, R. (2017). Pediatric autoimmune encephalitis. J. Pediatr. Neurosci. 12, 130–134. doi: 10.4103/jpn.JPN_185_16
Bitnun, A., Ford-Jones, E. L., Petric, M., MacGregor, D., Heurter, H., Nelson, S., et al. (2001). Acute childhood encephalitis and Mycoplasma pneumoniae. Clin. Infect. Dis. 32, 1674–1684. doi: 10.1086/320748
Centers for Disease Control and Prevention (2018). Mycoplasma pneumoniae Infection. Antibiotic Treatment. Available at: https://www.cdc.gov/pneumonia/atypical/mycoplasma/hcp/antibiotic-treatment-resistance.html
Chaudhry, R., Ghosh, A., and Chandolia, A. (2016). Pathogenesis of Mycoplasma pneumoniae: an update. Indian J. Med. Microbiol. 34, 7–16. doi: 10.4103/0255-0857.174112
Chaudhry, R., Varshney, A. K., and Malhotra, P. (2007). Adhesion proteins of Mycoplasma pneumoniae. Front. Biosci. 12, 690–699. doi: 10.2741/2093
Chourasia, B. K., Chaudhry, R., and Malhotra, P. (2014). Delineation of immunodominant and cytadherence segment(s) of Mycoplasma pneumoniae P1 gene. BMC Microbiol. 14:108. doi: 10.1186/1471-2180-14-108
Christie, L. J., Honarmand, S., Talkington, D. F., Gavali, S. S., Preas, C., Pan, C. Y., et al. (2007a). DNA in the CSF Pediatric encephalitis: what is the role of Mycoplasma pneumoniae? Pediatrics 120, 305–313.
Christie, L. J., Honarmand, S., Yagi, S., Ruiz, S., and Glaser, C. A. (2007b). Anti-galactocerebroside testing in Mycoplasma pneumoniae-associated encephalitis. J. Neuroimmunol. 189, 129–131.
de Groot, R. C. A., Meyer Sauteur, P. M., Unger, W. W. J., and van Rossum, A. M. C. (2017). Things that could be Mycoplasma pneumoniae. J. Infect. 74(Suppl. 1), S95–S100. doi: 10.1016/S0163-4453(17)30198-6
Esposito, S., Blasi, F., Arosio, C., Fioravanti, L., Fagetti, L., Droghetti, R., et al. (2000). Importance of acute Mycoplasma pneumoniae and Chlamydia pneumoniae infections in children with wheezing. Eur. Respir. J. 16, 1142–1146. doi: 10.1034/j.1399-3003.2000.16f21.x
Esposito, S., Blasi, F., Bosis, S., Droghetti, R., Faelli, N., Lastrico, A., et al. (2004). Aetiology of acute pharyngitis: the role of atypical bacteria. J. Med. Microbiol. 53, 645–651. doi: 10.1099/jmm.0.05487-0
Esposito, S., and Longo, M. R. (2017). Guillain-Barré syndrome. Autoimmun. Rev. 16, 96–101. doi: 10.1016/j.autrev.2016.09.022
Esposito, S., Tagliabue, C., Bosis, S., and Principi, N. (2011). Levofloxacin for the treatment of Mycoplasma pneumoniae-associated meningoencephalitis in childhood. Int. J. Antimicrob. Agents 37, 472–475. doi: 10.1016/j.ijantimicag.2011.01.008
Farcas, P., Avnun, L., Frisher, S., Herishanu, Y. O., and Wirguin, I. (1997). Efficacy of repeated intravenous immunoglobulin in severe unresponsive Guillain-Barré syndrome. Lancet 350:1747. doi: 10.1016/S0140-6736(97)24050-X
Granerod, J., Ambrose, H. E., Davies, N. W., Clewley, J. P., Walsh, A. L., Morgan, D., et al. (2010). Causes of encephalitis and differences in their clinical presentations in England: a multicentre, population-based prospective study. Lancet Infect. Dis. 10, 835–844. doi: 10.1016/S1473-3099(10)70222-X
Gupta, A., Kimber, T., Crompton, J. L., and Karagiannis, A. (2009). Acute disseminated encephalomyelitis secondary to Mycoplasma pneumoniae. Intern. Med. J. 39, 68–69. doi: 10.1111/j.1445-5994.2008.01777.x
Hallamaa, K. M., Browning, G. F., and Tang, S. L. (2006). Lipoprotein multigene families in Mycoplasma pneumoniae. J. Bacteriol. 188, 5393–5399. doi: 10.1128/JB.01819-05
Hallamaa, K. M., Tang, S. L., Ficorilli, N., and Browning, G. F. (2008). Differential expression of lipoprotein genes in Mycoplasma pneumoniae after contact with human lung epithelial cells, and under oxidative and acidic stress. BMC Microbiol. 8:124. doi: 10.1186/1471-2180-8-124
Hardy, R. D., Coalson, J. J., Peters, J., Chaparro, A., Techasaensiri, C., Cantwell, A. M., et al. (2009). Analysis of pulmonary inflammation and function in the mouse and baboon after exposure to Mycoplasma pneumoniae CARDS toxin. PLoS One 4:e7562. doi: 10.1371/journal.pone.0007562
Hughes, R. A., Brassington, R., Gunn, A. A., and van Doorn, P. A. (2016). Corticosteroids for Guillain-Barré syndrome. Cochrane Database Syst. Rev. 10:CD001446. doi: 10.1002/14651858.CD001446.pub5
Hughes, R. A., Swan, A. V., and van Doorn, P. A. (2014). Intravenous immunoglobulin for Guillain-Barré syndrome. Cochrane Database Syst. Rev. 9:CD002063. doi: 10.1002/14651858.CD002063.pub6
Jackson, M. A., and Schutze, G. E. (2016). The use of systemic and topical fluoroquinolones. Pediatrics 138:e20162706. doi: 10.1542/peds.2016-2706
Jacobs, E., Bartl, A., Oberle, K., and Schiltz, E. (1995). Molecular mimicry by Mycoplasma pneumoniae to evade the induction of adherence inhibiting antibodies. J. Med. Microbiol. 43, 422–429. doi: 10.1099/00222615-43-6-422
Kahane, I. (1984). In vitro studies on the mechanism of adherence and pathogenicity of mycoplasmas. Isr. J. Med. Sci. 20, 874–877.
Kammer, J., Ziesing, S., Davila, L. A., Bültmann, E., Illsinger, S., Das, A. M., et al. (2016). Neurological manifestations of Mycoplasma pneumoniae infection in hospitalized children and their long-term follow-uUp. Neuropediatrics 47, 308–317. doi: 10.1055/s-0036-1584325
Kannan, T. R., and Baseman, J. B. (2006). ADP-ribosylating and vacuolating cytotoxin of Mycoplasma pneumoniae represents unique virulence determinant among bacterial pathogens. Proc. Natl. Acad. Sci. U.S.A. 103, 6724–6729. doi: 10.1073/pnas.0510644103
Kikuchi, M., Tagawa, Y., Iwamoto, H., Hoshino, H., and Yuki, N. (1997). Bickerstaff’s brainstem encephalitis associated with IgG anti-GQ1b antibody subsequent to Mycoplasma pneumoniae infection: favorable response to immunoadsorption therapy. J. Child Neurol. 12, 403–405. doi: 10.1177/088307389701200612
Kim, P. S., Lee, P. P., and Levy, D. (2011). A theory of immunodominance and adaptive regulation. Bull. Math. Biol. 73, 1645–1665. doi: 10.1007/s11538-010-9585-5
Kumada, S., Kusaka, H., Okaniwa, M., Kobayashi, O., and Kusunoki, S. (1997). Encephalomyelitis subsequent to mycoplasma infection with elevated serum anti-Gal C antibody. Pediatr. Neurol. 16, 241–244. doi: 10.1016/S0887-8994(97)89976-6
Kusunoki, S., Shiina, M., and Kanazawa, I. (2001). Anti-Gal-C antibodies in GBS subsequent to mycoplasma infection: evidence of molecular mimicry. Neurology 57, 736–738. doi: 10.1212/WNL.57.4.736
Kuwahara, M., Samukawa, M., Ikeda, T., Morikawa, M., Ueno, R., Hamada, Y., et al. (2017). Characterization of the neurological diseases associated with Mycoplasma pneumoniae infection and anti-glycolipid antibodies. J. Neurol. 264, 467–475. doi: 10.1007/s00415-016-8371-1
Kwiatkowska, B., and Maślińska, M. (2012). Macrolide therapy in chronic inflammatory diseases. Mediators Inflamm. 2012:636157. doi: 10.1155/2012/636157
Lee, H., Yun, K. W., Lee, H. J., and Choi, E. H. (2018). Antimicrobial therapy of macrolide-resistant Mycoplasma pneumoniae pneumonia in children. Expert Rev. Anti Infect. Ther. 16, 23–34. doi: 10.1080/14787210.2018.1414599
Leonardi, S., Pavone, P., Rotolo, N., and La Rosa, M. (2005). Stroke in two children with Mycoplasma pneumoniae infection. a causal or casual relationship? Pediatr. Infect. Dis. J. 24, 843–845. doi: 10.1097/01.inf.0000177284.88356.56
Lind, K., Zoffmann, H., Larsen, S. O., and Jessen, O. (1979). Mycoplasma pneumoniae infection associated with affection of the central nervous system. Acta Med. Scand. 205, 325–332. doi: 10.1111/j.0954-6820.1979.tb06057.x
López-Boado, Y. S., and Rubin, B. K. (2008). Macrolides as immunomodulatory medications for the therapy of chronic lung diseases. Curr. Opin. Pharmacol. 8, 286–291. doi: 10.1016/j.coph.2008.01.010
Mailles, A., Stahl, J. P., Steering, C., and Investigators, G. (2009). Infectious encephalitis in France in 2007: a national prospective study. Clin. Infect. Dis. 49, 1838–1847. doi: 10.1086/648419
Matsumoto, N., Takahashi, S., Toriumi, N., Sarashina, T., Makita, Y., Tachibana, Y., et al. (2009). Acute disseminated encephalomyelitis in an infant with incontinentia pigmenti. Brain Dev. 31, 625–628. doi: 10.1016/j.braindev.2008.08.010
Meyer Sauteur, P. M., Huizinga, R., Tio-Gillen, A. P., Roodbol, J., Hoogen boezem, T., Jacobs, E., et al. (2016a). Mycoplasma pneumoniae triggering the Guillain-Barré syndrome: a case-control study. Ann. Neurol. 80, 566–580. doi: 10.1002/ana.24755
Meyer Sauteur, P. M., Moeller, A., Relly, C., Berger, C., Plecko, B., and Nadal, D. (2016b). Swiss national prospective surveillance of paediatric Mycoplasma pneumoniae-associated encephalitis. Swiss Med. Wkly. 146, w14222. doi: 10.4414/smw.2016.14222
Meyer Sauteur, P. M., Relly, C., Hackenberg, A., Stahr, N., Berger, C., Bloemberg, G. V., et al. (2014). Mycoplasma pneumoniae intrathecal antibody responses in Bickerstaff brain stem encephalitis. Neuropediatrics 45, 61–63. doi: 10.1055/s-0033-1348150
Michelow, I. C., Olsen, K., Lozano, J., Rollins, N. K., Duffy, L. B., Ziegler, T., et al. (2004). Epidemiology and clinical characteristics of community-acquired pneumonia in hospitalized children. Pediatrics 113, 701–707. doi: 10.1542/peds.113.4.701
Miyashita, N., Narita, M., Tanaka, T., Akaike, H., Teranishi, H., Oishi, T., et al. (2017). Histological findings in severe Mycoplasma pneumoniae pneumonia. J. Med. Microbiol. 66, 690–692. doi: 10.1099/jmm.0.000501
Narita, M. (2000). Detection of Mycoplasma pneumoniae DNA in cerebrospinal fluid and local immune response. Clin. Infect. Dis. 30, 405–406. doi: 10.1086/313641
Narita, M. (2010). Pathogenesis of extrapulmonary manifestations of Mycoplasma pneumoniae infection with special reference to pneumonia. J. Infect. Chemother. 16, 162–169. doi: 10.1007/s10156-010-0044-x
Narita, M. (2016). Classification of extrapulmonary manifestations due to Mycoplasma pneumoniae infection on the basis of possible pathogenesis. Front. Microbiol. 7:23. doi: 10.3389/fmicb.2016.00023
Narita, M., Itakura, O., Matsuzono, Y., and Togashi, T. (1995). Analysis of mycoplasmal central nervous system involvement by polymerase chain reaction. Pediatr. Infect. Dis. J. 14, 236–237. doi: 10.1097/00006454-199503000-00013
Narita, M., Matsuzono, Y., Itakura, O., Togashi, T., and Kikuta, H. (1996). Survey of mycoplasmal bacteremia detected in children by polymerase chain reaction. Clin. Infect. Dis. 23, 522–525. doi: 10.1093/clinids/23.3.522
Narita, M., Matsuzono, Y., Togashi, T., and Kajii, N. (1992). DNA diagnosis of central nervous system infection by Mycoplasma pneumoniae. Pediatrics 90, 250–253.
Narita, M., Tanaka, H., Togashi, T., and Abe, S. (2005). Cytokines involved in CNS manifestations caused by Mycoplasma pneumoniae. Pediatr. Neurol. 33, 105–109. doi: 10.1016/j.pediatrneurol.2005.03.003
Nau, R., Sörgel, F., and Eiffert, H. (2010). Penetration of drugs through the blood-cerebrospinal fluid/blood-brain barrier for treatment of central nervous system infections. Clin. Microbiol. Rev. 23, 858–883. doi: 10.1128/CMR.00007-10
Nishimura, M., Saida, T., Kuroki, S., Kawabata, T., Obayashi, H., Saida, K., et al. (1996). Post-infectious encephalitis with anti-galactocerebroside antibody subsequent to Mycoplasma pneumoniae infection. J. Neurol. Sci. 140, 91–95. doi: 10.1016/0022-510X(96)00106-2
O’Sullivan, M. V., Isbel, N. M., Johnson, D. W., O’Sullivan, M. V. N., Isbel, N. M., Johnson, D. W., et al. (2004). Disseminated pyogenic Mycoplasma pneumoniae infection in a renal transplant recipient, detected by broad-range polymerase chain reaction. Clin. Infect. Dis. 39, e98–e99. doi: 10.1086/425125
Padovan, C. S., Pfister, H. W., Bense, S., Fingerle, V., and Abele-Horn, M. (2001). Detection of Mycoplasma pneumoniae DNA in cerebrospinal fluid of a patient with M. pneumoniae infection-“associated” stroke. Clin. Infect. Dis. 33, E119–E121.
Pereyre, S., Goret, J., and Bébéar, C. (2016). Mycoplasma pneumoniae: current Knowledge on macrolide resistance and treatment. Front. Microbiol. 7:974. doi: 10.3389/fmicb.2016.00974
Pillai, S. C., Hacohen, Y., Tantsis, E., Prelog, K., Merheb, V., Kesson, A., et al. (2015). Infectious and autoantibody-associated encephalitis: clinical features and long-term outcome. Pediatrics 135, e974–e984. doi: 10.1542/peds.2014-2702
Ponka, A. (1980). Central nervous system manifestations associated with serologically verified Mycoplasma pneumoniae infection. Scand. J. Infect. Dis. 12, 175–184. doi: 10.3109/inf.1980.12.issue-3.04
Principi, N., and Esposito, S. (1999). Comparative tolerability of erythromycin and newer macrolide antibacterials in paediatric patients. Drug Saf. 20, 25–41. doi: 10.2165/00002018-199920010-00004
Principi, N., and Esposito, S. (2001). Emerging role of Mycoplasma pneumoniae and Chlamydia pneumoniae in paediatric respiratory-tract infections. Lancet Infect. Dis. 1, 334–344. doi: 10.1016/S1473-3099(01)00147-5
Principi, N., and Esposito, S. (2013). Macrolide-resistant Mycoplasma pneumoniae: its role in respiratory infection. J. Antimicrob. Chemother. 68, 506–511. doi: 10.1093/jac/dks457
Principi, N., and Esposito, S. (2015). Appropriate use of fluoroquinolones in children. Int. J. Antimicrob. Agents 45, 341–346. doi: 10.1016/j.ijantimicag.2015.01.004
Principi, N., Esposito, S., Blasi, F., Allegra, L., and Mowgli Study Group. (2001). Role of Mycoplasma pneumoniae and Chlamydia pneumoniae in children with community-acquired lower respiratory tract infections. Clin. Infect. Dis. 32, 1281–1289. doi: 10.1086/319981
Qu, J., Gu, L., Wu, J., Dong, J., Pu, Z., Gao, Y., et al. (2013). Accuracy of IgM antibody testing, FQ-PCR and culture in laboratory diagnosis of acute infection by Mycoplasma pneumoniae in adults and adolescents with community-acquired pneumonia. BMC Infect. Dis. 13:172. doi: 10.1186/1471-2334-13-172
Ruiz-González, A., Falguera, M., Nogués, A., and Rubio-Caballero, M. (1999). Is Streptococcus pneumoniae the leading cause of pneumonia of unknown etiology? A microbiologic study of lung aspirates in consecutive patients with community-acquired pneumonia. Am. J. Med. 106, 385–390. doi: 10.1016/S0002-9343(99)00050-9
Saida, T., Saida, K., Silberberg, D. H., and Brown, M. J. (1981). Experimental allergic neuritis induced by galactocerebroside. Ann. Neurol. 9(Suppl.), 87–101. doi: 10.1002/ana.410090714
Sauteur, P. M., Hackenberg, A., Tio-Gillen, A. P., van Rossum, A. M., Berger, C., and Jacobs, B. C. (2015). Intrathecal aAnti-GalC antibodies in bickerstaff brain stem encephalitis. Neuropediatrics 46, 428–430. doi: 10.1055/s-0035-1566730
Schmucker, R. D., Ehret, A., and Marshall, G. S. (2014). Cerebellitis and acute obstructive hydrocephalus associated with Mycoplasma pneumoniae infection. Pediatr. Infect. Dis. J. 33, 529–532. doi: 10.1097/INF.0000000000000140
Simpkins, A., Strickland, S. M., Oliver, J., Murray, D. L., Steele, J. C., Park, Y. D., et al. (2012). Complete resolution of advanced Mycoplasma pneumoniae encephalitis mimicking brain mass lesions: report of two pediatric cases and review of literature. Neuropathology 32, 91–99. doi: 10.1111/j.1440-1789.2011.01225.x
Sinha, S., Prasad, K. N., Jain, D., Pandey, C. M., Jha, S., and Pradhan, S. (2007). Preceding infections and anti-ganglioside antibodies in patients with Guillain-Barré syndrome: a single centre prospective case-control study. Clin. Microbiol. Infect. 13, 334–337. doi: 10.1111/j.1469-0691.2006.01636.x
Socan, M., Ravnik, I., Bencina, D., Dovc, P., Zakotnik, B., and Jazbec, J. (2001). Neurological symptoms in patients whose cerebrospinal fluid is culture- and/or polymerase chain reaction-positive for Mycoplasma pneumoniae. Clin. Infect. Dis. 32, E31–E35. doi: 10.1086/318446
Spuesens, E. B., Fraaij, P. L., Visser, E. G., Hoogenboezem, T., Hop, W. C., van Adrichem, L. N., et al. (2013). Carriage of Mycoplasma pneumoniae in the upper respiratory tract of symptomatic and asymptomatic children: an observational study. PLoS Med. 10:e1001444. doi: 10.1371/journal.pmed.1001444
Sugeno, N., Kawaguchi, N., Hasegawa, T., Kuroda, T., Kanbayashi, T., et al. (2012). A case with antigalactocerebroside antibody-positive Mycoplasma pneumoniae meningoencephalitis presenting secondary hypersomnia. Neurol. Sci. 33, 1473–1476. doi: 10.1007/s10072-012-1009-x
Susuki, K., Odaka, M., Mori, M., Hirata, K., and Yuki, N. (2004). Acute motor axonal neuropathy after Mycoplasma infection: evidence of molecular mimicry. Neurology 62, 949–956. doi: 10.1212/01.WNL.0000115123.42929.FD
Taylor-Robinson, D., Gumpel, J. M., Hill, A., and Swannell, A. J. (1978). Isolation of Mycoplasma pneumoniae from the synovial fluid of a hypogammaglobulinaemic patient in a survey of patients with inflammatory polyarthritis. Ann. Rheum. Dis. 37, 180–182. doi: 10.1136/ard.37.2.180
Ueda, N., Minami, S., and Akimoto, M. (2016). Mycoplasma pneumoniae-associated mild encephalitis/encephalopathy with a reversible splenial lesion: report of two pediatric cases and a comprehensive literature review. BMC Infect. Dis. 16:671. doi: 10.1186/s12879-016-1985-1
Vergis, E. N., and Yu, V. L. (1997). Macrolides are ideal for empiric therapy of community-acquired pneumonia in the immunocompetent host. Semin. Respir. Infect. 12, 322–328.
Waites, K. B., and Talkington, D. F. (2004). Mycoplasma pneumoniae and its role as a human pathogen. Clin. Microbiol. Rev. 17, 697–728. doi: 10.1128/CMR.17.4.697-728.2004
Watanabe, H., Uruma, T., Nakamura, H., and Aoshiba, K. (2014). The role of Mycoplasma pneumoniae infection in the initial onset and exacerbations of asthma. Allergy Asthma Proc. 35, 204–210. doi: 10.2500/aap.2014.35.3742
Woodhead, M. (1998). Community-acquired pneumonia guidelines – An international comparison: a view from Europe. Chest 113, 183S–187S. doi: 10.1378/chest.113.3_Supplement.183S
Yiş, U., Kurul, S. H., Cakmakçi, H., and Dirik, E. (2008). Mycoplasma pneumoniae: nervous system complications in childhood and review of the literature. Eur. J. Pediatr. 167, 973–978. doi: 10.1007/s00431-008-0714-1
Keywords: antinfective therapy, central nervous system, Mycoplasma pneumoniae, neuropathies, peripheral nervous system
Citation: D’Alonzo R, Mencaroni E, Di Genova L, Laino D, Principi N and Esposito S (2018) Pathogenesis and Treatment of Neurologic Diseases Associated With Mycoplasma pneumoniae Infection. Front. Microbiol. 9:2751. doi: 10.3389/fmicb.2018.02751
Received: 25 February 2018; Accepted: 26 October 2018;
Published: 20 November 2018.
Edited by:
Benjamin Lopman, Emory University, United StatesReviewed by:
Hridayesh Prakash, Amity University, IndiaCopyright © 2018 D’Alonzo, Mencaroni, Di Genova, Laino, Principi and Esposito. This is an open-access article distributed under the terms of the Creative Commons Attribution License (CC BY). The use, distribution or reproduction in other forums is permitted, provided the original author(s) and the copyright owner(s) are credited and that the original publication in this journal is cited, in accordance with accepted academic practice. No use, distribution or reproduction is permitted which does not comply with these terms.
*Correspondence: Susanna Esposito, c3VzYW5uYS5lc3Bvc2l0b0B1bmltaS5pdA==
Disclaimer: All claims expressed in this article are solely those of the authors and do not necessarily represent those of their affiliated organizations, or those of the publisher, the editors and the reviewers. Any product that may be evaluated in this article or claim that may be made by its manufacturer is not guaranteed or endorsed by the publisher.
Research integrity at Frontiers
Learn more about the work of our research integrity team to safeguard the quality of each article we publish.