- 1Department of Galenic Pharmacy and Food Technology, Complutense University of Madrid, Madrid, Spain
- 2Department of Microbiology and Biochemistry of Dairy Products, Instituto de Productos Lácteos de Asturias – Consejo Superior de Investigaciones Científicas, Villaviciosa, Spain
- 3Department of Nutrition and Food Science, Complutense University of Madrid, Madrid, Spain
Studies carried in the last years have revealed that human milk contains a site-specific microbiota and constitutes a source of potentially beneficial bacteria to the infant gut. Once in the infant gut, these bacteria contribute to the assembly of a physiological gut microbiota and may play several functions, contributing to infant metabolism, protection against infections, immunomodulation or neuromodulation. Many preterm neonates are fed with pasteurized donor’s human milk (DHM) or formula and, therefore, are devoid of contact with human milk microbes. As a consequence, new strategies are required to allow the exposition of a higher number of preterm infants to the human milk microbiota early in life. The first strategy would be to promote and to increase the use of own mother’s milk (OMM) in Neonatal Intensive Care Units (NICUs). Even small quantities of OMM can be very valuable since they would be added to DHM in order to microbiologically “customize” it. When OMM is not available, a better screening of donor women, including routine cytomegalovirus (CMV) screening of milk, may help to avoid the pasteurization of the milk provided by, at least, a relevant proportion of donors. Finally, when pasteurized DHM or formula are the only feeding option, their supplementation with probiotic bacteria isolated from human milk, such as lactic acid bacteria or bifidobacteria, may be an alternative to try to restore a human milk-like microbiota before feeding the babies. In the future, the design of human milk bacterial consortia (minimal human milk microbiotas), including well characterized strains representative of a healthy human milk microbiota, may be an attractive strategy to provide a complex mix of strains specifically tailored to this target population.
From Own Mother’s Milk to Donor Human Milk: The “Fall” of the Human Milk Microbiota
Fresh human milk is considered as the gold standard of infant nutrition because it provides all the nutrients and vitamins required for the optimal development of the infant (American Academy of Pediatrics [AAP], 2012). In addition, it contains an inimitable plethora of bioactive factors, including soluble immune factors, antimicrobial proteins and peptides, functional fatty acids, hormones, oligosaccharides, stem cells, and microbes. This vast array of bioactive compounds act synergistically in order to preserve infants’ health, making difficult to delineate the specific functions of a given milk component without taking in account its potential interactions with other human milk ingredients. This fact reflects the effect of evolution selection toward a perfect multifunctional species-specific fluid that meets the complex infant requirements during early life (Hennet and Borsig, 2016).
The consideration of human milk as a gold standard for term and preterm infant feeding usually refers to own mother’s milk (OMM) since its composition is adapted to the specific needs of each particular infant (gestational age, time of the day, geographical location, environment, etc,) which converts this biological fluid into the ultimate personalized food and medicine (Furman et al., 2003; Meinzen-Derr et al., 2009; Corpeleijn et al., 2012; Chowning et al., 2016; Hennet and Borsig, 2016). Donor human milk (DHM) is the best alternative when there is no OMM available or there is no enough OMM to guarantee a proper nutrition of the preterm infant (American Academy of Pediatrics [AAP], 2012; ESPGHAN Committee on Nutrition et al., 2013; Quigley and McGuire, 2014; DiLauro et al., 2016; Sisk et al., 2017).
The microbiological composition of DHM is a relevant concern for Human Milk Banks (HMBs) since it will be ingested mainly by preterm or sick term infants who are known to be particularly susceptible to infections. Under physiological conditions, the bacterial concentration of human milk is usually low (<3 log cfu/ml) and is dominated by Gram-positive bacteria (staphylococci, streptococci, corynebacteria, propionibacteria, lactic acid bacteria and bifidobacteria, among others), which are the core components of the human milk microbiota (Fernández et al., 2013). However, milk extraction devices and the subsequent management of the collected milk may lead to the bacterial contamination of DHM. For instance, the use of milk pumps may result in a high concentration (even higher than 6 log cfu/ml) of contaminating Gram-negative bacteria (enterobacteria, Pseudomonas, Stenotrophomonas, etc.) and yeasts arising from rinsing water and/or poor hygienic manipulation practices (Boo et al., 2001; Brown et al., 2005; Cervia et al., 2008; Landers and Updegrove, 2010; Fihman et al., 2012; Decousser et al., 2013; Cohen et al., 2017; Jiménez et al., 2017). Such deleterious microorganisms may adhere to pumps’ surfaces and resist usual cleaning and disinfection procedures (Marín et al., 2009). Contaminated human milk has occasionally been associated to infections of preterm infants by microorganisms such as Escherichia coli, Pseudomonas sp., Klebsiella sp., or Serratia sp. (Lanzieri et al., 2013; Kayıran et al., 2014; Nakamura et al., 2016). In addition, high-risk viruses that can be potentially transferred from mother to infant through breastfeeding, such as human immunodeficiency viruses (HIV-1 HIV-2), cytomegalovirus, papillomaviruses, human T-lymphotrophic viruses (HTLV-I, HTLV-II), as well as Ebola, Marburg or Zika viruses, are of special concern (Diaz et al., 2018; Mann et al., 2018).
Globally, it means that, on one hand, donors have to receive a thoughtful formation on hygienic practices while collecting and preserving their milk; and, on the other hand, that a proper microbiological screening of donor women must be carried out in HMBs. Although some criteria may differ among the HMBs of different countries, this usually includes the fulfillment of a questionnaire regarding lifestyle, diseases and risk factors, the serologic screening of donors, and the pre- and/or post-pasteurization microbiological analysis of the milk (Arslanoglu et al., 2010; NICE, 2010). The exclusion criteria for donor women generally include the following: (a) having (she or her partner) positive blood results for hepatitis B, hepatitis C, HTLV-I, HTLV-II, HIV-1, HIV-2, or syphilis; (b) suffering from other sexually acquired infections (human papillomavirus, ano-genital infection by herpes simplex virus types 1 and 2, infections by Chlamydia trachomatis, Neisseria gonorrheae, or Trichomonas vaginalis) since it may imply a high risk sexual behavior (and, due to the same reason, women with a promiscuous sexual life are also excluded); (c) having a disease that requires frequent blood (or blood derivatives) transfusions; and (d) suffering or having a family history of spongiform transmissible encephalopathy.
Despite the donor microbiological screening, DHM is pasteurized in most HMBs to further ensure microbiological safety by killing all non-spore forming microorganisms, including all the high-risk viruses cited above (Orloff et al., 1993; Chiavarini et al., 2011; Rigourd et al., 2011; Donalisio et al., 2014; Hamilton Spence et al., 2017; Pfaender et al., 2017). Alternative emerging non-thermal food technologies are being tested in order to preserve the biological activities of some of the human milk compounds (immunoglobulins, cytokines, enzymes, etc.) but all of them also destroy non-sporulated bacteria. Obviously, pasteurization does not only kill potentially harmful microorganisms but also destroy the beneficial milk microbiota. As stated above, diverse culture-dependent and –independent studies have shown that human milk contains a site-specific microbiota, which is subsequently transferred to the infant (Makino et al., 2015; Milani et al., 2015; Asnicar et al., 2017; Duranti et al., 2017; Murphy et al., 2017; Pannaraj et al., 2017), playing an important role in the acquisition and evolution of the microbiota in early life, and contributing to metabolic functions and to the maturation of the immune and neuroendocrine systems (Fernández et al., 2013; Jeurink et al., 2013; Jost et al., 2015; McGuire and McGuire, 2017).
In the past, it was thought that introduction of solid foods changed the infant gut microbiome toward an adult-like microbiome, independently of whether the infant was receiving human milk or not; however, it has been recently described that infant microbiota is dominated by bacteria provided by human milk as long as they are breastfed, independently of whether solid foods are introduced or not (Bäckhed et al., 2015). In other words, the gut microbiomes of babies who stopped breastfeeding earlier are dominated by adult-like taxa, while those of babies breastfed for longer are dominated by bacteria present in human milk. The conclusion that stopping breastfeeding -rather than introducing solids-drives gut microbiota maturation is an important paradigm’s shift and indicates that the impact of human milk bacteria on infant gut microbiota seems to be much stronger than previously thought.
As a consequence, devising new strategies to allow that preterm infants may receive the human milk microbiota might significantly improve the gut microbiota establishment and its associated health benefits in this population (Figure 1). Some of these strategies will be discussed in this review.
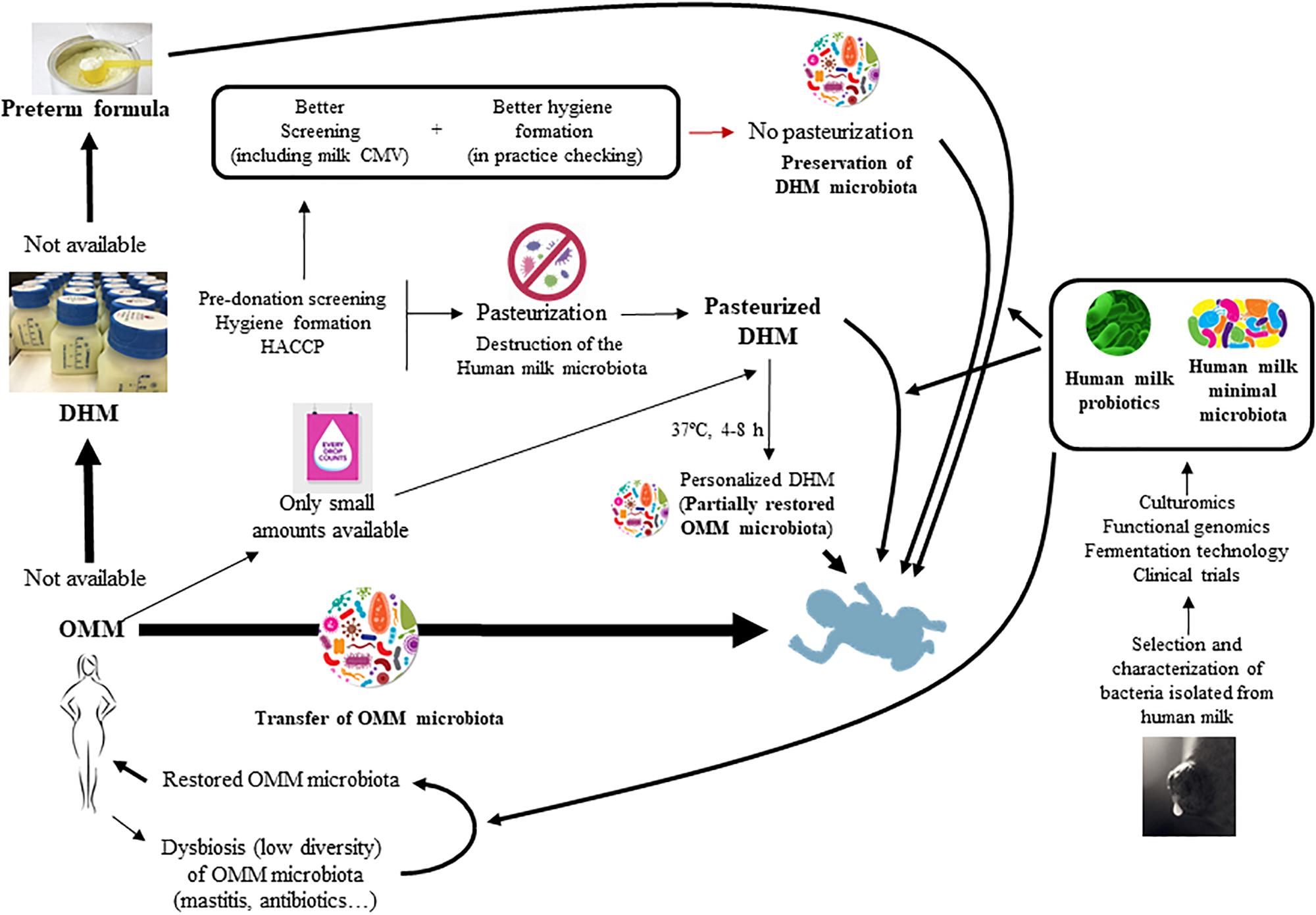
FIGURE 1. Strategies for the restoration and modulation of the human milk microbiota and its application to preterm infants.
Increasing of the Use of OMM in NICUs: Benefits and Challenges
The best way to increase the number of preterm neonates exposed to their mothers’ milk microbiota is increasing the use of OMM for preterm neonates at NICUs. In the last years, some authors have criticized the fact that the terms “human milk” or “human milk feedings” are often used interchangeably for both OMM and DHM (Meier et al., 2017a), as the first provides much more benefits to preterm infants that DHM (Vohr et al., 2006, 2007; Colaizy and Morriss, 2008; Belfort et al., 2016; Meier et al., 2017a). Although in this section the benefits of OMM over DHM will be highlighted, it must be said that DHM is superior to formula in terms of infant development and protection against diseases and their use in NICUs is very valuable to provide some of the biologically active compounds that remain totally or partially active after the heat treatment those preterm infants that have no access to OMM.
DHM differs significantly from OMM in, at least, three main aspects: (a) pasteurization decreases or eliminates the biological activity of many milk bioactive compounds (as discussed below); (b) cold storage and freeze-thaw cycles that are inherent in the storage and processing of DHM can also alter milk composition and functionality; and (c) there may be relevant differences in the stage of lactation for which DHM replaces OMM. Regarding the later point, mature DHM frequently replaces OMM colostrum and transitional milk (Meier et al., 2017a). In this context, it must be highlighted that the concentrations of many milk bioactive compounds decline (in some cases quite rapidly) during lactation, as the infant matures. In addition, feeding preterm infants with DHM obtained from mothers of much older infants has been considered a major gap in lactation and neonatology knowledge (Meier et al., 2017a). DHM needs fortification with protein, energy, vitamins and minerals (Moro et al., 2015), and usually has a lower protein content in relation to milk produced during early lactation (Schanler, 2007; Valentine et al., 2010). The relationship observed between a lower growth rate and impaired neurological development is particularly worrying (Ramel et al., 2012). In this sense, colostrum and milk from mothers of preterm infants seem to specifically potentiate immunomodulatory and nutritional programming as well as selective organ growth, including the immature brain (Isaacs et al., 2010; Sherman et al., 2015). Individualized (adjustable or targeted) fortification of DHM is recommended (Arslanoglu et al., 2009; ESPGHAN Committee on Nutrition et al., 2013; Moro et al., 2015).
Immunologically, human milk is usually suited to the gestational age at which a baby is born (Espinosa-Martos et al., 2013; Kaingade et al., 2017). Colostrum and transient milk from mothers of very preterm infants seems to contain higher amounts of key immune compounds than those from mothers of term infants (Montagne et al., 1999; Araujo et al., 2005; Koenig et al., 2005; Castellote et al., 2011; Espinosa-Martos et al., 2013; Moles et al., 2015b), indicating that the degree of immunoprotection provided through breastfeeding is highly correlated with the immaturity degree of the neonate. These facts further support the high relevance of OMM for preterm infants, whose risk for feeding intolerance, necrotizing enterocolitis and sepsis is higher than that of term infants (Westerbeek et al., 2006).
Diverse OMM components that are thought to benefit preterm health, including neurodevelopmental advantages, are reduced or absent in DHM, and include, among others, immunoglobulins (Contador et al., 2013), some cytokines, chemokines and growth factors (Espinosa-Martos et al., 2013), hormones and enzymes (Peila et al., 2016), myoinositol (de Segura et al., 2012; Espinosa-Martos et al., 2013; Moles et al., 2015b) and soluble CD14 (Cossey et al., 2009). Human milk oligosaccharides (HMOs) are other of the human milk components thought to have numerous beneficial functions in infants, from prebiotics to immunomodulation and protection against infectious diseases. Although our knowledge on the effect of pasteurization on this type of compounds is still limited and controversial, recent data have revealed that infants in NICUs who receive DHM are likely to ingest HMOs at different total amounts and relative composition from what they would receive with their OMM (Marx et al., 2014). Concentrations of HMOs in human milk show variations that depend on the secretor type of the mother and the lactation period (Thurl et al., 2017); therefore, OMM is again the best source of HMOs for a preterm infant. Finally, and in contrast to either formula or DHM, OMM contains an array of mother-specific probiotic and commensal bacteria (the milk microbiota), that may selectively use the highly complex and individual HMOs as prebiotics to promote their own growth once inside the infant gut (Jost et al., 2015). It is probable that exogenous probiotics cannot fully replace OMM ones because the later are specifically suited to the HMOs existing in the milk of the same woman. Pasteurization completely eradicates OMM bacteria, including lactic acid bacteria and bifidobacteria, potentially displacing or altering the impact of OMM microbiota on infant gut colonization.
Many initiatives are useful to increase the rates of OMM feeding when a preterm infant leaves the NICU and most lactation barriers are modifiable when evidence-based practices and resources are prioritized (Meier et al., 2017b). The research literature is replete with strategies to acquire and feed OMM in the NICU. Unfortunately, many of them are not implemented in practice because they require an initial economical investment (Meier et al., 2017b), despite the fact that OMM-based programs are, at the medium and long term, more economical than DHM-oriented ones and provides greater protection from acquired morbidities (Jegier et al., 2013; Patel et al., 2013; Johnson et al., 2015). As an example, and although it may not be the general rule, a study has warned of a decrease in the cumulative proportion of OMM received by very low-birth-weight (VLBW) infants (i.e., with weight <1,500 g).
VLBW infants at 14 and 28 days post-birth after the introduction of DHM into a NICU in which 98% of these infants had received some OMM prior to DHM availability (Meier et al., 2017b). Problems that mothers of preterm neonates have to face in order to provide OMM at the early post-birth period and to keep breastfeeding their infants after NICU discharge have been reviewed recently (Meier et al., 2017a,b). These facts should be a matter of reflection by HMBs and NICU’s managers.
DHM: to Heat or Not to Heat?
HMBs are the most important providers of DHM to NICUs and there is no doubt that they have played a decisive role in extending human milk benefits to preterm infants andother high-risk infants (American Academy of Pediatrics [AAP], 2012; ESPGHAN Committee on Nutrition et al., 2013; World Health Organization [WHO], 2017). DHM is pasteurized in most HMBs although in a few countries, such as Japan and Norway, there is a history of using raw non-pasteurized DHM to feed preterm infants, a practice that preserves milk bioactive compounds, including the microbiota. This means that use of non-pasteurized DHM is feasible and, with appropriate control, as safe as that of pasteurized DHM (Grøvslien and Grønn, 2009; Mizuno et al., 2015). To ensure its safety, unpasteurized milk may be considered when extremely strict donor screening, including regularly repeated testing, is performed, when there is complete traceability of milk from donor to consumer, and when the HIV and hepatitis rates are low.
Fear to transmission of pathogenic microorganisms [particularly cytomegalovirus (CMV) in Western countries] is the basis of human milk pasteurization; however, it is also true that use of OMM has an extraordinary history of safety despite women that provide OMM to their preterm infants are not screened for CMV and no differences in the level of hygiene formation have been reported between OMM and DHM providers so far. It is somehow shocking, and again a reason for reflection, that with the current arguments given to pasteurize DHM, use of OMM or breastfeeding should be forbidden to many mothers of preterm infants.
It must be also noted that pasteurized DHM is not devoid of microbiological risks; although viruses and contaminant vegetative bacterial cells are killed by standard pasteurization processes, the treatment does not guarantee the destruction of spore-forming bacteria (e.g., Bacillus cereus) (Landers and Updegrove, 2010; de Segura et al., 2012; Almutawif et al., 2017); subsequently, such bacteria and potential post-pasteurization contaminants can overgrow since the treatment destroys the natural competitors provided by the milk microbiota and, also, alters some of the bacteriostatic compounds of milk (Ford et al., 1977).
In order to avoid milk pasteurization, donors should receive an excellent education and training on the subject while the HMB staff should (a) monitor that such knowledge is applied in practice; and (b) control environmental contamination during DHM collection, distribution and storage (Becker et al., 2015). But, if donors are submitted to a careful pre-donation screening (hepatitis B and C, HTLV I and II, HIV 1 and 2, syphilis, no toxic habits, no sexual risky behavior, etc.) and receive a thoughtful hygienic formation (as it uses to be the case in most Western HMBs), why do HMBs still pasteurize DHM? The most common answer is because of the potential threat of CMV transfer to low birth weight and/or preterm infants. In these populations the risk of CMV transmission through human milk from seropositive mothers has traditionally been considered higher than for term infants (Jim et al., 2009). Freezing of fresh human milk reduces, but does not eradicate infectivity (Lombardi et al., 2012) and does not affect the rate of CMV transmission (Omarsdottir et al., 2015).
In full-term infants, CMV infections are most often asymptomatic because of the transfer of specific maternal protection during pregnancy. On the contrary, preterm infants (and, particularly the VLBW ones) can suffer a symptomatic infection. In spite of this, breastfeeding is usually recommended on a risk-benefit basis since CMV infection resolves spontaneously and without sequelae in most instances (Lombardi et al., 2012; Romero-Gómez et al., 2015). Indeed, several studies found that exposure of preterm infants to CMV-positive human milk did not negatively affect either neurological development or hearing (Yasuda et al., 2003; Vollmer et al., 2004; Jim et al., 2015). Most recently, Gunkel et al. (2018) showed in a cohort study, that postnatal CMV infection in preterm infants had no impact on neurodevelopment or hearing for the first 6 years after birth.
Even in the case that a risk as close to zero has to be assured, routine CMV screening of the milk of donor women may allow the selection of those whose CMV-free milk does not need to be pasteurized. Techniques for a proper diagnose are currently available (Hartleif et al., 2016) and should be implemented at HMBs or at the services of Microbiology of those hospitals harboring a HMB. Knowledge on the rates of CMV-positive lactating women are very scarce or non-existing in many countries. Such data may be useful for HMBs.
Personalization of Pasteurized Donor Milk With OMM
As stated above, human milk naturally contains microbes, including lactic acid bacteria and bifidobacteria, that constitute a truly site-specific microbiota. Interestingly, each woman seems to contain a unique fingerprint-like milk microbiota that is stable over time (Hunt et al., 2011). Most components of the milk microbiota are transferred to the infant (Martín et al., 2003, 2012; Jost et al., 2013), together with mother-specific HMOs that are specifically suited to her own milk’s microbiota, working synergistically to provide health benefits to the infant (Jost et al., 2015).
The early post-birth lactation stages may be particularly problematic for mothers of preterm infants, who usually depend on pumping for milk expression (Meier et al., 2016). In addition, preterm birth disrupts physiological processes in the mammary epithelium (Cregan et al., 2002; Pang and Hartmann, 2007; Neville, 2009). Under such circumstances, they may be unable to produce enough milk to fully support the nutritional requirements of their babies (Smith et al., 2003; Lee and Gould, 2009). However, such low amounts can be very valuable for microbial personalization of DHM, providing lasting health benefits and encouraging the NICU mothers to continue pumping, as it will be shown below.
In a recent study, pasteurized DHMs were inoculated with small proportions of OMM (ranging from 1 to 30% v:v) and the mixtures were incubated for 4 or 8 h at 37°C (Cacho et al., 2017). The authors showed that it was possible, at least partly, to reestablish the levels of staphylococci, streptococci and lactic acid bacteria to those that were naturally found in the OMM samples, and suggested that the optimal restoration strategy would be adding 10% OMM to DHM and incubating for 4 h. This study also confirmed that the microbiota of human milk has a high degree of inter-individual variability.
This highly individualized method to provide human milk bacteria to preterm infants confers an active role to the mother of a very preterm infant, encouraging milk extraction and the maintenance of lactation in the future. In contrast, some questions and current practical limitations may arise in relation to this approach: (a) is it feasible in the daily management of a NICU? And, more importantly, (b) are ethical committees ready to accept an increase in the milk staphylococcal and streptococcal count as something desirable for a preterm neonate? At the short time the answers seem to be “no” but in the context of the “microbiome era” where fecal transplant has become a kind of standard treatment for pseudomembranous colitis caused by Clostridium difficile (see below), it may be a practical and economical approach in a close future. For this purpose, studies including large cohorts and a careful safety and efficacy assessment will be required.
Preterm Gut Colonization and Probiotics
Premature neonates show numerous signs of organ immaturity responsible for their inability to face postnatal life challenges appropriately. Immediately after preterm birth, the immature gut receives a plethora of microbiological, immunological, and nutritional-related challenges difficult to cope with due to deficiencies in this organ (Siggers et al., 2011; Ruiz et al., 2016), with deep consequences at systemic level (Claus et al., 2008; Neuman et al., 2015).
The gut colonization pattern of in premature infants has been described as delayed and aberrant (Koenig et al., 2011; LaTuga et al., 2011; Arboleya et al., 2012a). This may have important and long lasting health implications since the early neonatal period is a key period for reaching a microbiota-induced host-homeostasis (Hansen et al., 2012; Olszak et al., 2012; Cox et al., 2014). Microbiota provides stimuli necessary for an adequate developmental programming of several metabolic, immunological and neuroendocrine functions, not only in the gastrointestinal tract but in most, if not all, organs (Ley et al., 2008; Renz et al., 2012; Sommer and Bäckhed, 2013). The existence of specific alterations on the microbiota establishment process in preterm infants may contribute to the pathogenesis of different prematurity-related diseases (Moles et al., 2013; Ruiz et al., 2016). Thus, there is an increasing interest in the management of the microbial colonization process in the preterm infant. Among the strategies that can be used with this objective, the administration of selected probiotic bacteria, with appropriate microbiota modulation capabilities, constitutes a promising approach (Arboleya et al., 2012b; Alfaleh and Anabrees, 2014; Ruiz et al., 2016).
Probiotics are defined as “live micro-organisms which, when administered in adequate amounts, confer a health benefit on the host” (FAO/WHO, 2002; Hill et al., 2014). Probiotic effects are often strain-specific and the beneficial effects of a given strain for a specific target cannot be extrapolated to other targets. In addition, probiotic effects also depend on posology.
The demand of probiotics has experienced a rapid growth in recent decades, even for administration to high-risk populations, as is the case of premature infants. The initial rationale for administering probiotics to preterm infants was the key role played by the initial colonization of the neonatal intestine in necrotizing enterocolitis (NEC) and sepsis outcomes. The potential benefits of probiotic therapy in this population include improving the intestinal barrier, increasing the production of IgAs and anti-inflammatory cytokines, increasing the diversity and functionality of the microbiota, and decreasing the pathological bacterial translocation (Versalovic, 2013).
The results of routine use in clinical practice, trials, reviews, and meta-analyses confirm that the administration of probiotics to low or VLBW premature infants is safe and significantly reduces the risk of NEC, the mortality due to any cause and the time required for enteral feeding (Alfaleh et al., 2010, 2011; Deshpande et al., 2011; Wang et al., 2012; Bonsante et al., 2013; Srinivasjois et al., 2013; Versalovic, 2013; Athalye-Jape et al., 2014; Johnson-Henry et al., 2016). Nevertheless, the heterogeneity in strains, dose, form of application and duration of treatment, make it difficult or impossible to reach consensus about the most appropriate strains and clear therapy guidelines (Neu, 2011; Ofek Shlomai et al., 2014).
There is an urgent need of identifying the best suited strains for this particular population since, with a few exceptions (Arboleya et al., 2013; Moles et al., 2015a), most of the strains used in preterms had not been selected for this target population so far. Therefore, the identification of the best suited strains and conditions is still a pending task (Ruiz et al., 2016). Taking into account the special vulnerability of this population, a careful evaluation of the potential risks associated with probiotic administration and microbiota modulation in preterm infants is required, including those involving the production and manipulation of these products (Bertelli et al., 2015; Zbinden et al., 2015). In 2014, a probiótico product (ABC Dophilus) used in a preterm clinical trial was recalled by the manufacturer since the Center for Disease Control (CDC) reported a case of mortality due to contamination with the fungus Rhizopus oryzae (Food and Drug Administration [FDA], 2014). Despite such events are very scarce, it highlights the need to produce probiotics using pharmaceutical standards (Johnson-Henry et al., 2016).
Human Milk: A Source of Probiotic Bacteria
Human milk guarantees a constant supply of bacteria (5–7 log bacteria per day) throughout the lactation period (Heikkilä and Saris, 2003; Martín et al., 2003). Among isolated bacteria, lactobacilli and bifidobacteria isolates have received a particular attention because of their long history of safety use and the potential of many strains from these groups to be used as probiotics. Consequently, the isolation of potentially beneficial bacteria from this biological fluid is particularly attractive for pharmacological and nutrition companies since, by their very own nature, they respond to many of the criteria generally required for human probiotics (Fernández et al., 2013; Jeurink et al., 2013).
Among the lactobacilli and bifidobacteria species isolated from human milk, many of them (Lactobacillus salivarius, L. gasseri, L. reuteri, L. plantarum, L. rhamnosus, L. fermentum, Bifidobacterium breve, B. Longum, or B. bifidum), are included among the potentially probiotic ones and enjoy the GRAS (Generally Recognized As Safe; FDA, United States) and the QPS (Qualified Presumption of Safety; EFSA, EU) status (EFSA, 2013). In fact, the probiotic potential of some lactobacilli strains from this biological fluid is similar or higher to that of certain strains commercially available worldwide (Martín et al., 2005, 2006). In general, lactic bacteria and bifidobacteria isolated from human milk appear to play different beneficial roles for the mother-infant dyad (Fernández et al., 2013; Jost et al., 2015). The safety and functionality (enhancement of the intestinal barrier function, antimicrobial activity, protection against infectious diseases, metabolic roles, immunomodulation, and neuromodulation) of some of human milk-derived strains (L. fermentum CECT5716, L. salivarius CECT5713, L. salivarius PS2, L. gasseri CECT5714, L. salivariusPS12934, and B. brevePS12929) have been evaluated in vitro, in silico, and in vivo, both in animal models and in human clinical trials, including pregnant and lactating women, term infants and extremely low birth weight premature infants (Arroyo et al., 2010; Maldonado et al., 2010, 2012; Martín et al., 2010; Gil-Campos et al., 2012; Fernández et al., 2014, 2016; Maldonado-Lobón et al., 2015b; Moles et al., 2015a).
Apart from lactic acid bacteria and bifidobacteria (the classic prototype of probiotic bacteria), other milk bacteria with less glamorous and appealing names, may provide relevant beneficial effects to the infants. Streptococci (mitis and salivarius groups) and coagulase-negative staphylococci (CNS) are among the dominant bacteria both in human milk (Jiménez et al., 2008b; Hunt et al., 2012; Martín et al., 2012; Cacho et al., 2017) and in the feces of breast-fed infants (Lundequist et al., 1985; Sakata et al., 1985; Balmer and Wharton, 1989; Adlerberth et al., 2006; Jiménez et al., 2008a). Potential beneficial roles of CNS and mitis/salivarius streptococci include the competitive exclusion of undesired pathogens, such as Staphylococcus aureus (Kirjavainen et al., 2001; Uehara et al., 2001; Otto, 2009; Iwase et al., 2010; Park et al., 2011). The same may be applied to Propionibacterium acnes, another species that is commonly found in human milk. This species can prevent growth of S. aureus through glycerol fermentation (Shu et al., 2013) and, precisely, there is a high abundance of glycerol in human milk.
As a consequence, some human milk staphylococci, streptococci and propionibacteria may play important empirical health-promoting roles in the breastfed infant. It must be highlighted that some CNS and viridans streptococcal strains may behave as opportunistic pathogens and, therefore, a strain-by-strain rigorous safety assessment of such microorganisms must be performed before they can be intentionally administered to infants (Sanders et al., 2010; Rodríguez, 2015).
In addition, culture-independent studies have detected the presence of DNA from strict anaerobic species generally related to the digestive tract environment (Bifidobacterium, Blautia, Faecalibacterium, Roseburia, Clostridium, Collinsella, Veillonella, Bacteroides, Parabacteroides, etc.) in human milk (Rodríguez, 2014) and, also, sharing of DNA from such bacteria between maternal feces, milk and infant feces (Martín et al., 2009; Jost et al., 2013). At present, routine cultivation and commercial production of such microorganisms is not technically and economically feasible; however, advances in culturomics, functional genomics and fermentation technologies may enable the use of such microorganisms as probiotics in the future, especially considering that some of them (such as Faecalibacterium prausnitzii) have been associated to clear benefits to human health (Miquel et al., 2014; Martín et al., 2017). In the future, analysis of the genomes of human milk strains may contribute to a faster selection of human milk-derived bacteria in a much more rational way, and will provide additional clues on the safety, probiotic and technological properties of human milk microbes (Langa et al., 2012; Cárdenas et al., 2015).
Modulation of the Milk Microbiota in Mothers of Preterm Infants?
There are some situations in which the composition of the milk microbiome may be improved, particularly when OMM is being administered to preterm neonates. The first one is the presence of high risk clones in milk, including methicillin-resistant and methicillin-sensitive S. aureus. Their presence has become increasingly common in hospitals (including maternities and NICUs) where mothers can asymptomatically acquire and transfer them to her milk and, occasionally, can lead to severe infant outcomes (Gastelum et al., 2005; Kayıran et al., 2014). However, testing OMM for high risk clones may not be a practical measure at present having in account the very low number of reports describing adverse outcomes due to the transfer of such microbes through OMM.
The second one is the antepartum, intrapartum and/or postpartum administration of antibiotics to the mother, which is relatively common among women having preterm deliveries and that, in fact, may be linked to the presence of antibiotic-resistant high risk clones. Use of antibiotics during pregnancy or lactation has been associated to a significant decrease in the number of lactobacilli- or bifidobacteria-positive human milk samples (Soto et al., 2014); these drugs are well known drivers of dysbiosis in a variety of human locations and this may lead to detrimental consequences for infant health when administered to pregnant or lactating women (Murk et al., 2011; Willing et al., 2011; Stensballe et al., 2013). A decrease in the lactic acid bacteria and bifidobacterial populations of human milk may delay colonization of the infant gut by these bacteria, making infants more susceptible to some conditions, such as allergy (Arvola et al., 2006) or colic (de Weerth et al., 2013). In contrast, a Lactobacillus strain isolated from human milk origin is a useful tool for the treatment of infantile colic (Sung et al., 2012).
Loss of milk lactobacilli and bifidobacteria (e.g., as a consequence of an antibiotic treatment) is also associated to negative consequences for mammary health since it uses to coincide with an overgrowth of opportunistic bacteria that, eventually, can cause mastitis (Delgado et al., 2009, 2011; Contreras and Rodríguez, 2011). Mastitis can be an additional barrier complicating the collection of OMM by mothers of preterm neonates, and often leading to an undesired abandon of lactation. Recent studies have shown that some lactobacilli strains isolated from human milk can be applied to treat or prevent mastitis (Arroyo et al., 2010; Fernández et al., 2014, 2016; Vázquez-Fresno et al., 2014; Maldonado-Lobón et al., 2015a; Espinosa-Martos et al., 2016; Hurtado et al., 2017), highlighting their potential roles in mammary homeostasis.
Use of antibiotics, chemotherapy and acute and subacute mastitis are characterized by a decreased bacterial diversity in human milk (Delgado et al., 2008; Urbaniak et al., 2014; Jiménez et al., 2015; Patel et al., 2017). The impact of this decreased bacterial diversity for infant health are unknown but previous studies suggest that it may have negative consequences at the short and long term (Mshvildadze et al., 2010; Giongo et al., 2011; Madan et al., 2012a,b).
Human Milk-Like Minimal or Synthetic Microbiotas: The Perfect Probiotic for Preterm Infants?
The concept of “minimal/synthetic microbiota” derives from the fecal transplant practice (de Vos, 2013). It consists in the administration of a fecal suspension obtained from a healthy donor to a diseased person through gastroduodenoscopy, colonoscopy or enema. As a consequence, the intestinal microbiota is transferred from one person to another, including not only the cultivable microorganisms but also those that cannot currently be cultivated and which, consequently, cannot be administered in the form of a conventional probiotic. This increasingly popular practice has shown a high efficacy in the treatment of recurrent pseudomembranous colitis by C. Difficile (Bakken et al., 2011; Gough et al., 2011; van Nood et al., 2013).
However, fecal transplantation faces important practical challenges, including the potential transfer of undetected pathogenic viruses or harmful metabolites, or the difficulty or impossibility for a industrial standardization. Therefore, there is a need to design and develop new biotechnological processes that allow applying the principle of fecal transfer in a reproducible way. In this sense, the concept of minimal or synthetic niche-specific microbial communities can open new therapeutic ways to modify the microbiota of people with different pathologies and overcomes many of the limitations of fecal transfer (Allen-Vercoe et al., 2012; de Vos, 2013). Although this approach also has some challenges for routine practical application, a pioneering study revealed the ability of a minimal microbiota designed in the laboratory to cure recurrent C. difficile infection (Petrof et al., 2013). The development of human milk-based defined minimal microbiotas seems an appealing novel strategy for the premature population in order to decrease NEC and sepsis rates in the context of the emergence of multiresistant pathogenic bacteria and a higher preterm viability at lower gestational ages.
Similarly to personalization of DHM with OMM, a synthetic human milk microbiota may contain both QPS and non-QPS species, which may represent -at least at a medium term- a relevant issue for ethical approval. Initially human milk-derived consortia including only strains belonging to QPS species (mainly lactic acid bacteria and bifidobacteria) may be designed, developed and, eventually, tried. Human milk usually contains a high diversity of bacterial strains but at a rather low concentration; this is in contrast with probiotic-supplemented infant formula, which often contain one or two strains but a notably higher concentration. Many studies will be necessary to define a cultivable core human milk microbiota and the functions that the components of a synthetic microbiome should play in premature babies, which may range from prevention of infectious diseases to neuromodulation. The dosis of each strain has to be carefully considered: should each strain be present at the same concentration or their relative proportion in human milk should be respected? Developing human milk-like minimal microbiotas suited for preterm neonates is a major challenge in the frontiers of our current knowledge on the human microbiota.
Author Contributions
All the authors participated in the critical analysis of the literature in the field, wrote and revised the manuscript.
Funding
Research was supported by grants AGL2016-75476-R and PCIN-2015-234 (Ministry of Economy and Competitiveness, Spain). LR was supported by a Juan de la Cierva Postdoctoral Trainee Program of the Spanish Ministry of Economy and Competitiveness (grant, IJCI-2015-23196).
Conflict of Interest Statement
The authors declare that the research was conducted in the absence of any commercial or financial relationships that could be construed as a potential conflict of interest.
References
Adlerberth, I., Lindberg, E., Aberg, N., Hesselmar, B., Saalman, R., Strannegård, I. L., et al. (2006). Reduced enterobacterial and increased staphylococcal colonization of the infantile bowel: an effect of hygienic lifestyle? Pediatr. Res. 59, 96–101. doi: 10.1203/01.pdr.0000191137.12774.b2
Alfaleh, K., and Anabrees, J. (2014). Probiotics for prevention of necrotizing enterocolitis in preterm infants. Evid. Based Child Health 9, 584–671. doi: 10.1002/ebch.1976
Alfaleh, K., Anabrees, J., and Bassler, D. (2010). Probiotics reduce the risk of necrotizing enterocolitis in preterm infants: a meta-analysis. Neonatology 97, 93–99. doi: 10.1159/000235684
Alfaleh, K., Anabrees, J., Bassler, D., and Al-Kharfi, T. (2011). Probiotics for prevention of necrotizing enterocolitis in preterm infants. Cochrane Database Syst. Rev. 3:CD008991. doi: 10.1002/14651858.CD005496.pub3
Allen-Vercoe, E., Reid, G., Viner, N., Gloor, G. B., Hota, S., Kim, P., et al. (2012). Canadian working group report on fecal microbial therapy: microbial ecosystems therapeutics. Can. J. Gastroenterol. 26, 457–462. doi: 10.1155/2012/213828
Almutawif, Y., Hartmann, B., Lloyd, M., Erber, W., and Geddes, D. (2017). A retrospective audit of bacterial culture results of donated human milk in Perth, Western Australia. Early Hum. Dev. 105, 1–6. doi: 10.1016/j.earlhumdev.2016.12.011
American Academy of Pediatrics [AAP] (2012). Breastfeeding and the use of human milk. Pediatrics 129, e827–e841. doi: 10.1542/peds.2011-3552
Araujo, E. D., Goncalves, A. K., Cornetta, M., Cunha, H., Cardoso, M. L., Morais, S. S., et al. (2005). Evaluation of the secretory immunoglobulin A levels in the colostrums and milkof mothers of term and preterm infants. Braz. J. Infect. Dis. 9, 357–362. doi: 10.1590/S1413-86702005000500002
Arboleya, S., Binetti, A., Salazar, N., Fernández, N., Solís, G., Hernández-Barranco, A., et al. (2012a). Establishment and development of intestinal microbiota in preterm neonates. FEMS Microbiol. Ecol. 79, 763–772. doi: 10.1111/j.1574-6941.2011.01261.x
Arboleya, S., Gonzalez, S., Salazar, N., Ruas-Madiedo, P., de los Reyes-Gavilán, C. G., and Gueimonde, M. (2012b). Development of probiotic products for nutritional requirements of specific human populations. Eng. Life Sci. 12, 368–376. doi: 10.1016/j.anaerobe.2012.11.001
Arboleya, S., Salazar, N., Solís, G., Fernández, N., Hernández-Barranco, A. M., Cuesta, I., et al. (2013). Assessment of intestinal microbiota modulation ability of Bifidobacterium strains in in vitro fecal batch cultures from preterm neonates. Anaerobe 19, 9–16. doi: 10.1016/j.anaerobe.2012.11.001
Arroyo, R., Martin, V., Maldonado, A., Jiménez, E., Fernández, L., and Rodríguez, J. M. (2010). Treatment of infectious mastitis during lactation: antibiotics versus oral administration of lactobacilli isolated from breast milk. Clin. Infect. Dis. 50, 1551–1558. doi: 10.1086/652763
Arslanoglu, S., Bertino, E., Tonetto, P., De Nisi, G., Ambruzzi, A. M., Biasini, A., et al. (2010). Guidelines for the establishment and operation of a donor human milk bank. J. Matern. Fetal Neonatal Med. 23(Suppl. 2), 1–20. doi: 10.3109/14767058.2010.512414
Arslanoglu, S., Moro, G. E., and Ziegler, E. E. (2009). Preterm infants fed fortified human milk receive less protein than they need. J. Perinatol. 29, 489–492. doi: 10.1038/jp.2009.50
Arvola, T., Ruuska, T., Keränen, J., Hyöty, H., Salminen, S., and Isolauri, E. (2006). Rectal bleeding in infancy: clinical, allergological, and microbiological examination. Pediatrics 117, e760–e768. doi: 10.1542/peds.2005-1069
Asnicar, F., Manara, S., Zolfo, M., Truong, D. T., Scholz, M., Armaninni, F., et al. (2017). Studying vertical microbiome transmission from mothers to infants by strain-level metagenomics profiling. mSystems 2:e00164. doi: 10.1128/mSystems.00164-16
Athalye-Jape, G., Deshpande, G., Rao, S., and Patole, S. (2014). Benefits of probiotics on enteral nutrition in preterm neonates: a systematic review. Am. J. Clin. Nutr. 100, 1508–1519. doi: 10.3945/ajcn.114.092551
Bäckhed, F., Roswall, J., Peng, Y., Feng, Q., Jia, H., Kovatcheva-Datchary, P., et al. (2015). Dynamics and stabilization of the human gut microbiome during the first year of life. Cell Host Microbe 17, 690–703. doi: 10.1016/j.chom.2015.04.004
Bakken, J. S., Borody, T., Brandt, L. J., Brill, J. V., Demarco, D. C., Francos, M. A., et al. (2011). Fecal microbiota transplantation workgroup. Treating Clostridium difficile infection with fecal microbiota transplantation. Clin. Gastroenterol. Hepatol. 9, 1044–1049. doi: 10.1016/j.cgh.2011.08.014
Balmer, S. E., and Wharton, B. A. (1989). Diet and faecal flora in the newborn: breast milk and infant formula. Arch. Dis. Child. 64, 1672–1677. doi: 10.1136/adc.64.12.1672
Becker, G. E., Smith, H. A., and Cooney, F. (2015). Methods of milk expression for lactating women (Review). Cochrane Database Syst. Rev. 2:CD006170. doi: 10.1002/14651858.CD006170.pub4
Belfort, M. B., Anderson, P. J., Nowak, V. A., Lee, K. J., Molesworth, C., Thompson, D. K., et al. (2016). Breast milk feeding, brain development, and neurocognitive outcomes: a 7-year longitudinal study in infants born at less than 30 weeks’ gestation. J. Pediatr. 177, 133.e1–139.e1. doi: 10.1016/j.jpeds.2016.06.045
Bertelli, C., Pillonel, T., Torregrossa, A., Prodhom, G., Fischer, C. J., Greub, G., et al. (2015). Bifidobacterium longum bacteremia in preterm infants receiving probiotics. Clin. Infect. Dis. 60, 924–927. doi: 10.1093/cid/ciu946
Bonsante, F., Iacobelli, S., and Gouyon, J. B. (2013). Routine probiotic use in very preterm infants: retrospective comparison of two cohorts. Am. J. Perinatol. 30, 41–46. doi: 10.1055/s-0032-1321498
Boo, N. Y., Nordiah, A. J., Alfizah, H., Nor–Rohaini, A. H., and Lim, V. K. (2001). Contamination of breast milk obtained by manual expression and breast pumps in mothers of very low birth weight infants. J. Hosp. Infect. 49, 274–281. doi: 10.1053/jhin.2001.1117
Brown, S. L., Bright, R. A., Dwyer, D. E., and Foxman, B. (2005). Breast pump adverse events: reports to the Food and Drug Administration. J. Hum. Lact. 21, 169–174. doi: 10.1177/0890334405275445
Cacho, N. T., Harrison, N. A., Parker, L. A., Padgett, K. A., Lemas, D. J., Marcial, G. E., et al. (2017). personalization of the microbiota of donor human milk with mother’s own milk. Front. Microbiol. 8:1470. doi: 10.3389/fmicb.2017.01470
Cárdenas, N., Laiño, J. E., Delgado, S., Jiménez, E., Juárez del Valle, M., Savoy de Giori, G., et al. (2015). Relationships between the genome and some phenotypical properties of Lactobacillus fermentum CECT 5716, a probiotic strain isolated from human milk. Appl. Microbiol. Biotechnol. 99, 4343–4353. doi: 10.1007/s00253-015-6429-0
Castellote, C., Casillas, R., Ramírez-Santana, C., Pérez-Cano, F. J., Castell, M., Moretones, M. G., et al. (2011). Premature delivery influences the immunological composition of colostrum and transitional and mature human milk. J. Nutr. 141, 1181–1187. doi: 10.3945/jn.110.133652
Cervia, J. S., Ortolano, G. A., and Canonica, F. P. (2008). Hospital tap water as a source of Stenotrophomonasmaltophilia infection. Clin. Infect. Dis. 46, 1485–1487. doi: 10.1086/587180
Chiavarini, M., Bragetti, P., Sensini, A., Cenci, E., Castronari, R., Rossi, M. J., et al. (2011). Breastfeeding and transmission of cytomegalovirus to preterm infants. Case report and kinetic of CMV-DNA in breast milk. Ital. J. Pediatr. 37:6. doi: 10.1186/1824-7288-37-6
Chowning, R., Radmacher, P., Lewis, S., Serke, L., Pettit, N., and Adamkin, D. H. (2016). A retrospective analysis of the effect of human milk on prevention of necrotizing enterocolitis and postnatal growth. J. Perinatol. 36, 221–224. doi: 10.1038/jp.2015.179
Claus, S. P., Tsang, T. M., Wang, Y., Cloarec, O., Skordi, E., Martin, F. P., et al. (2008). Systemic multicompartmental effects of the gut microbiome on mouse metabolic phenotypes. Mol. Syst. Biol. 4:219. doi: 10.1038/msb.2008.56
Cohen, R., Babushkin, F., Shimoni, Z., Cohen, S., Litig, E., Shapiro, M., et al. (2017). Water faucets as a source of Pseudomonas aeruginosa infection and colonization in neonatal and adult intensive care unit patients. Am. J. Infect. Control. 45, 206–209. doi: 10.1016/j.ajic.2016.05.029
Colaizy, T. T., and Morriss, F. H. (2008). Positive effect of NICU admission on breastfeeding of preterm US infants in 2000 to 2003. J. Perinatol. 28, 505–510. doi: 10.1038/jp.2008.32
Contador, R., Delgado-Adámez, J., Delgado, F. J., Cava, R., and Ramírez, R. (2013). Effect of thermal pasteurisation or high pressure processing on immunoglobulin and leukocyte contents of human milk. Int. Dairy J. 32, 1–5. doi: 10.1016/j.idairyj.2013.03.006
Contreras, G. A., and Rodríguez, J. M. (2011). Mastitis: comparative etiology and epidemiology. J. Mammary Gland Biol. Neoplasia 16, 339–356. doi: 10.1007/s10911-011-9234-0
Corpeleijn, W. E., Kouwenhoven, S. M., Paap, M. C., van Vliet, I., Scheerder, I., Muizer, Y., et al. (2012). Intake of own mother’s milk during the first days of life is associated with decreased morbidity and mortality in very low birth weight infants during the first 60 days of life. Neonatology 102, 276–281. doi: 10.1159/000341335
Cossey, V., Jeurissen, A., Bossuyt, X., and Schuermans, A. (2009). Effect of pasteurisation on the mannose-binding lectin activity and the concentration of soluble CD14 in human milk. J. Hosp. Infect. 73, 96–97. doi: 10.1016/j.jhin.2009.06.021
Cox, L. M., Yamanishi, S., Sohn, J., Alekseyenko, A. V., Leung, J. M., Cho, I., et al. (2014). Altering the intestinal microbiota during a critical developmental window has lasting metabolic consequences. Cell 158, 705–721. doi: 10.1016/j.cell.2014.05.052
Cregan, M. D., De Mello, T. R., Kershaw, D., McDougall, K., and Hartmann, P. E. (2002). Initiation of lactation in women after preterm delivery. Acta Obstet. Gynecol. Scand. 81, 870–877. doi: 10.1034/j.1600-0412.2002.810913.x
de Segura, A. G., Escuder, D., Montilla, A., Bustos, G., Pallás, C., Fernández, L., et al. (2012). Heating-induced bacteriological and biochemical modifications in human donor milk after holder pasteurization. J. Pediatr. Gastroenterol. Nutr. 54, 197–203. doi: 10.1097/MPG.0b013e318235d50d
de Vos, W. M. (2013). Fame and future of faecal transplantations-developing next-generation therapies with synthetic microbiomes. Microb. Biotechnol. 6, 316–325. doi: 10.1111/1751-7915.12047
de Weerth, C., Fuentes, S., Puylaert, P., and de Vos, W. M. (2013). Intestinal microbiota of infants with colic: development and specific signatures. Pediatrics 131, e550–e558. doi: 10.1542/peds.2012-1449
Decousser, J. W., Ramarao, N., Duport, C., Dorval, M., Bourgeois-Nicolaos, N., Guinebretière, M. H., et al. (2013). Bacillus cereus and severe intestinal infections in preterm neonates, putative role of pooled breast milk. Am. J. Infect. Control 41, 918–921. doi: 10.1016/j.ajic.2013.01.043
Delgado, S., Arroyo, R., Jiménez, E., Marín, M. L., del Campo, R., Fernández, L., et al. (2009). Staphylococcus epidermidis strains isolated from breast milk of women suffering infectious mastitis: potential virulence traits and resistance to antibiotics. BMC Microbiol. 9:82. doi: 10.1186/1471-2180-9-82
Delgado, S., Arroyo, R., Martín, R., and Rodríguez, J. M. (2008). PCR-DGGE assessment of the bacterial diversity of breast milk in women with lactational infectious mastitis. BMC Infect. Dis. 8:51. doi: 10.1186/1471-2334-8-51
Delgado, S., García, P., Fernández, L., Jiménez, E., Rodríguez-Baños, M., del Campo, R., et al. (2011). Characterization of Staphylococcus aureus strains involved in human and bovine mastitis. FEMS Immunol. Med. Microbiol. 62, 225–235. doi: 10.1111/j.1574-695X.2011.00806.x
Deshpande, G. C., Rao, S. C., Keil, A. D., and Patole, S. K. (2011). Evidence-based guidelines for use of probiotics in preterm neonates. BMC Med. 9:92. doi: 10.1186/1741-7015-9-92
Diaz, S., Boulle, N., Molès, J. P., Peries, M., Rutagwera, D., Kankasa, C., et al. (2018). Human papillomavirus (HPV) shedding in breast milk from African women living with HIV. J. Clin. Virol. 106, 41–43. doi: 10.1016/j.jcv.2018.07.004
DiLauro, S., Unger, S., Stone, D., and O’Connor, D. L. (2016). Human milk for ill and medically compromised infants: strategies and ongoing innovation. J. Parenter. Enteral Nutr. 40, 768–782. doi: 10.1177/0148607116629676
Donalisio, M., Cagno, V., Vallino, M., Moro, G. E., Arslanoglu, S., Tonetto, P., et al. (2014). Inactivation of high-risk human papillomaviruses by Holder pasteurization: implications for donor human milk banking. J. Perinat. Med. 42, 1–8. doi: 10.1515/jpm-2013-0200
Duranti, S., Lugli, G. A., Mancabelli, L., Armanini, F., Turroni, F., James, K., et al. (2017). Maternal inheritance of bifidobacterial communities and bifidophages in infants through vertical transmission. Microbiome 5:66. doi: 10.1186/s40168-017-0282-6
EFSA (2013). Scientific opinion on the maintenance of the list of QPS biological agents intentionally added to food and feed (2013 update). EFSA J. 11:3449.
ESPGHAN Committee on Nutrition, Arslanoglu, S., Corpeleijn, W., Moro, G., Braegger, C., Campoy, C., et al. (2013). Donor human milk for preterm infants: current evidence and research directions. J. Pediatr. Gastroenterol. Nutr. 57, 535–542. doi: 10.1097/MPG.0b013e3182a3af0a
Espinosa-Martos, I., Jiménez, E., de Andrés, J., Rodríguez-Alcalá, L. M., Tavárez, S., Manzano, S., et al. (2016). Milk and blood biomarkers associated to the clinical efficacy of a probiotic for the treatment of infectious mastitis. Benef. Microbes 7, 305–318. doi: 10.3920/BM2015.0134
Espinosa-Martos, I., Montilla, A., de Segura, A. G., Escuder, D., Bustos, G., Pallás, C., et al. (2013). Bacteriological, biochemical, and immunological modifications in human colostrum after Holder pasteurisation. J. Pediatr. Gastroenterol. Nutr. 56, 560–568. doi: 10.1097/MPG.0b013e31828393ed
FAO/WHO (2002). Joint FAO/WHO Working Group Report on Drafting Guidelines for the Evaluation of Probiotics in Food. Toronto, ON: FAO/WHO.
Fernández, L., Arroyo, R., Espinosa, I., Marín, M., Jiménez, E., and Rodríguez, J. M. (2014). Probiotics for human lactational mastitis. Benef. Microbes 5, 169–183. doi: 10.3920/BM2013.0036
Fernández, L., Cardenas, N., Arroyo, R., Manzano, S., Jiménez, E., Martin, V., et al. (2016). Prevention of infectious mastitis by oral administration of Lactobacillus salivarius PS2 during late pregnancy. Clin. Infect. Dis. 62, 568–573. doi: 10.1093/cid/civ974
Fernández, L., Langa, S., Martin, V., Maldonado, A., Jiménez, E., Martin, R., et al. (2013). The human milk microbiota: origin and potential roles in health and disease. Pharmacol. Res. 69, 1–10. doi: 10.1016/j.phrs.2012.09.001
Fihman, V., Le Monnier, A., Corvec, S., Jaureguy, F., Tankovic, J., Jacquier, H., et al. (2012). Stenotrophomonas maltophilia – the most worrisome treat among unusual non–fermentative gram–negative bacilli from hospitalized patients, a prospective multicenter study. J. Infect. 64, 391–398. doi: 10.1016/j.jinf.2012.01.001
Food and Drug Administration [FDA] (2014). Solgar Inc Issues Voluntary Class I Recall of ABC Dophilus Powder. Available at: http://www.fda.gov/Safety/Recalls/ucm423219.htm
Ford, J. E., Law, B. A., Marshall, V. M. E., and Reiter, B. (1977). Influence of the heat treatment of human milk on some of its protective constituents. J. Pediatr. 90, 29–35. doi: 10.1016/S0022-3476(77)80759-2
Furman, L., Taylor, G., Minich, N., and Hack, M. (2003). The effect of maternal milk on neonatal morbidity of very low-birth-weight infants. Arch. Pediatr. Adolesc. Med. 157, 66–71. doi: 10.1001/archpedi.157.1.66
Gastelum, D. T., Dassey, D., Mascola, L., and Yasuda, L. M. (2005). Transmission of community-associated methicillin-resistant Staphylococcus aureus from breast milk in the neonatal intensive care unit. Pediatr. Infect. Dis. J. 24, 1122–1124. doi: 10.1097/01.inf.0000189983.71585.30
Gil-Campos, M., López, M. A., Rodríguez-Benítez, M. V., Romero, J., Roncero, I., Linares, M. D., et al. (2012). Lactobacillus fermentum CECT 5716 is safe and well tolerated in infants of 1-6 months of age: a randomized controlled trial. Pharmacol. Res. 65, 231–238. doi: 10.1016/j.phrs.2011.11.016
Giongo, A., Gano, K. A., Crabb, D. B., Mukherjee, N., Novelo, L. L., Casella, G., et al. (2011). Toward defining the autoimmune microbiome for type 1 diabetes. ISME J. 5, 82–91. doi: 10.1038/ismej.2010.92
Gough, E., Shaikh, H., and Manges, A. R. (2011). Systematic review of intestinal microbiota transplantation (fecal bacteriotherapy) for recurrent Clostridium difficile infection. Clin. Infect. Dis. 53, 994–1002. doi: 10.1093/cid/cir632
Grøvslien, A. H., and Grønn, M. (2009). Donor milk banking and breastfeeding in Norway. J. Hum. Lact. 25, 206–210. doi: 10.1177/0890334409333425
Gunkel, J., de Vries, L. S., Jongmans, M., Koopman-Esseboom, C., van Haastert, I. C., Eijsermans, M. C. J., et al. (2018). Outcome of preterm infants with postnatal cytomegalovirus infection. Pediatrics 141:e20170635. doi: 10.1542/peds.2017-0635
Hamilton Spence, E., Huff, M., Shattuck, K., Vickers, A., Yun, N., and Paessler, S. (2017). Ebola virus and marburg virus in human milk are inactivated by holder pasteurization. J. Hum. Lact. 33, 351–354. doi: 10.1177/0890334416685564
Hansen, C. H., Nielsen, D. S., Kverka, M., Zakostelska, Z., Klimesova, K., Hudcovic, T., et al. (2012). Patterns of early gut colonization shape future immune responses of the host. PLoS One 7:e34043. doi: 10.1371/journal.pone.0034043
Hartleif, S., Göhring, K., Goelz, R., Jahn, G., and Hamprecht, K. (2016). Quantitative monitoring of HCMV DNAlactia in human milk by real time PCR assay: implementation of internal control contributes to standardization and quality control. J. Virol. Methods. 237, 101–106. doi: 10.1016/j.jviromet.2016.08.020
Heikkilä, M. P., and Saris, P. E. J. (2003). Inhibition of Staphylococcus aureus by the commensal bacteria of human milk. J. Appl. Microbiol. 95, 471–478. doi: 10.1046/j.1365-2672.2003.02002.x
Hennet, T., and Borsig, L. (2016). Breastfed at Tiffany’s. Trend Biochem. Sci. 41, 508–518. doi: 10.1016/j.tibs.2016.02.008
Hill, C., Guarner, F., Reid, G., Gibson, G. R., Merenstein, D. J., Pot, B., et al. (2014). Expert consensus document. The international scientific association for probiotics and prebiotics consensus statement on the scope and appropriate use of the term probiotic. Nat. Rev. Gastroenterol. Hepatol. 11, 506–514. doi: 10.1038/nrgastro.2014.66
Hunt, K. M., Foster, J. A., Forney, L. J., Schutte, U. M., Beck, D. L., Abdo, Z., et al. (2011). Characterization of the diversity and temporal stability of bacterial communities in human milk. PLoS One. 6:e21313. doi: 10.1371/journal.pone.0021313
Hunt, K. M., Preuss, J., Nissan, C., Davlin, C. A., Williams, J. E., Shafii, B., et al. (2012). Human milk oligosaccharides promote the growth of staphylococci. Appl. Environ. Microbiol. 78, 4763–4770. doi: 10.1128/AEM.00477-12
Hurtado, J. A., Maldonado-Lobón, J. A., Díaz-Ropero, M. P., Flores-Rojas, K., Uberos, J., Leante, J. L., et al. (2017). Oral administration to nursing women of Lactobacillus fermentum CECT5716 prevents lactational mastitis development: a randomized controlled trial. Breastfeed. Med. 12, 202–209. doi: 10.1089/bfm.2016.0173
Isaacs, E. B., Fischl, B. R., Quinn, B. T., Chong, W. K., Gadian, D. G., and Lucas, A. (2010). Impact of breast milk on intelligence quotient, brain size, and white matter development. Pediatr. Res. 67, 357–362. doi: 10.1203/PDR.0b013e3181d026da
Iwase, T., Uehara, Y., Shinji, H., Tajima, A., Seo, H., Takada, K., et al. (2010). Staphylococcus epidermidis esp inhibits Staphylococcus aureus biofilm formation and nasal colonization. Nature 465, 346–349. doi: 10.1038/nature09074
Jegier, B. J., Johnson, T. J., Engstrom, J. L., Patel, A. L., Loera, F., and Meier, P. (2013). The institutional cost of acquiring 100 ml of human milk for very low birth weight infants in the neonatal intensive care unit. J. Hum. Lact. 29, 390–399. doi: 10.1177/0890334413491629
Jeurink, P. V., van Bergenhenegouwen, J., Jimenez, E., Knippels, L. M., Fernandez, L., Garssen, J., et al. (2013). Human milk: a source of more life than we imagine. Benef. Microbes 4, 17–30. doi: 10.3920/BM2012.0040
Jim, W. T., Chiu, N. C., Ho, C. S., Shu, C. H., Chang, J. H., Hung, H. Y., et al. (2015). Outcome of preterm infants with postnatal cytomegalovirus infection via breast milk: a two-year prospective follow-up study. Medicine 94:e1835. doi: 10.1097/MD.0000000000001835
Jim, W. T., Shu, C. H., Chiu, N. C., Chang, J. H., Hung, H. Y., Peng, C. C., et al. (2009). High cytomegalovirus load and prolonged virus excretion in breast milk increase risk for viral acquisition by very low birth weight infants. Pediatr. Infect. Dis. J. 28, 891–894. doi: 10.1097/INF.0b013e3181a55c52
Jiménez, E., Arroyo, R., Cárdenas, N., Marín, M., Serrano, P., Fernández, L., et al. (2017). Mammary candidiasis, a medical condition without scientific evidence? PLoS One. 12:e0181071. doi: 10.1371/journal.pone.0181071
Jiménez, E., de Andrés, J., Manrique, M., Pareja-Tobes, P., Tobes, R., Martínez-Blanch, J. F., et al. (2015). Metagenomic analysis of milk of healthy and mastitis-suffering women. J. Hum. Lact. 31, 406–415. doi: 10.1177/0890334415585078
Jiménez, E., Delgado, S., Maldonado, A., Arroyo, R., Albujar, M., García, N., et al. (2008a). Staphylococcus epidermidis: a differential trait of the fecal microbiota of breast-fed infants. BMC Microbiol. 8:143. doi: 10.1186/1471-2180-8-143
Jiménez, E., Fernández, L., Maldonado, A., Martin, R., Olivares, M., Xaus, J., et al. (2008b). Oral administration of lactobacillus strains isolated from breast milk as an alternative for the treatment of infectious mastitis during lactation. Appl. Environ. Microbiol. 74, 4650–4655. doi: 10.1128/AEM.02599-07
Johnson, T. J., Patel, A. L., Bigger, H. R., Engstrom, J. L., and Meier, P. P. (2015). Cost savings of human milk as a strategy to reduce the incidence of necrotizing enterocolitis in very low birth weight infants. Neonatology 107, 271–276. doi: 10.1159/000370058
Johnson-Henry, K. C., Abrahamsson, T. R., Wu, R. Y., and Sherman, P. M. (2016). Probiotics, prebiotics, and synbiotics for the prevention of necrotizing enterocolitis. Adv. Nutr. 7, 928–937. doi: 10.3945/an.116.012237
Jost, T., Lacroix, C., Braegger, C., and Chassard, C. (2013). Assessment of bacterial diversity in breast milk using culture-dependent and culture-independent approaches. Br. J. Nutr. 110, 1253–1262. doi: 10.1017/S0007114513000597
Jost, T., Lacroix, C., Braegger, C., and Chassard, C. (2015). Impact of human milk bacteria and oligosaccharides on neonatal gut microbiota establishment and gut health. Nutr. Rev. 73, 426–437. doi: 10.1093/nutrit/nuu016
Kaingade, P., Somasundaram, I., Sharma, A., Patel, D., and Marappagounder, D. (2017). Cellular components, including stem-like cells, of preterm mother’s mature milk as compared with those in her colostrum: a pilot study. Breastfeed. Med. 12, 446–449. doi: 10.1089/bfm.2017.0063
Kayıran, P. G., Can, F., Kayıran, S. M., Ergonul, O., and Gürakan, B. (2014). Transmission of methicillin-sensitive Staphylococcus aureus to a preterm infant through breast milk. J. Matern. Fetal Neonatal Med. 27, 527–529. doi: 10.3109/14767058.2013.819332
Kirjavainen, P. V., Apostolou, E., Arvola, T., Salminen, S. J., Gibson, G. R., and Isolauri, E. (2001). Characterizing the composition of intestinal microflora as a prospective treatment target in infant allergic disease. FEMS Immunol. Med. Microbiol. 32, 1–7. doi: 10.1111/j.1574-695X.2001.tb00526.x
Koenig, A., de Albuquerque Diniz, E. M., Barbosa, S. F., and Vaz, F. A. (2005). Immunologic factors inhuman milk: the effects of gestational age and pasteurization. J. Hum. Lact. 21, 439–443. doi: 10.1177/0890334405280652
Koenig, J. E., Spor, A., Scalfone, N., Fricker, A. D., Stombaugh, J., Knight, R., et al. (2011). Succession of microbial consortia in the developing infant gut microbiome. Proc. Natl. Acad. Sci. U.S.A. 108(Suppl. 1), 4578–4585. doi: 10.1073/pnas.1000081107
Landers, S., and Updegrove, K. (2010). Bacteriological screening of donor human milk before and after Holder pasteurization. Breastfeed. Med. 5, 117–121. doi: 10.1089/bfm.2009.0032
Langa, S., Maldonado-Barragán, A., Delgado, S., Martín, R., Martín, V., Jiménez, E., et al. (2012). Characterization of Lactobacillus salivarius CECT 5713, a strain isolated from human milk: from genotype to phenotype. Appl. Microbiol. Biotechnol. 94, 1279–1287. doi: 10.1007/s00253-012-4032-1
Lanzieri, T. M., Dollard, S. C., Josephson, C. D., Schmid, D. S., and Bialek, S. R. (2013). Breast milk-acquired cytomegalovirus infection and disease in VLBW and premature infants. Pediatrics 131, e1937–e1945. doi: 10.1542/peds.2013-0076
LaTuga, M. S., Ellis, J. C., Cotton, C. M., Goldberg, R. N., Wynn, J. L., Jackson, R. B., et al. (2011). Beyond bacteria: a study of the enteric microbial consortium in extremely low birth weight infants. PLoS One 6:e27858. doi: 10.1371/journal.pone.0027858
Lee, H. C., and Gould, J. B. (2009). Factors influencing breast milk versus formula feeding at discharge for very low birth weight infants in California. J. Pediatr 155, 657.e2–662.e2. doi: 10.1016/j.jpeds.2009.04.064
Ley, R. E., Hamady, M., Lozupone, C., Turnbaugh, P. J., Ramey, R. R., Bircher, J. S., et al. (2008). Evolution of mammals and their gut microbes. Science 320, 1647–1651. doi: 10.1126/science.1155725
Lombardi, G., Garofoli, F., Manzoni, P., and Stronati, M. (2012). Breast milk-acquired cytomegalovirus infection in very low birth weight infants. J. Matern. Fetal Neonatal. Med. 25(Suppl. 3), 57–62. doi: 10.3109/14767058.2012.712345
Lundequist, B., Nord, C. E., and Winberg, J. (1985). The composition of the faecal microflora in breastfed and bottle-fed infants from birth to eight weeks. Acta Pediatr. Scan. 74, 45–51. doi: 10.1111/j.1651-2227.1985.tb10919.x
Madan, J. C., Farzan, S. F., Hibberd, P. L., and Karagas, M. R. (2012a). Normal neonatal microbiome variation in relation to environmental factors, infection and allergy. Curr. Opin. Pediatr. 24, 753–759. doi: 10.1097/MOP.0b013e32835a1ac8
Madan, J. C., Salari, R. C., Saxena, D., Davidson, L., O’Toole, G. A., Moore, J. H., et al. (2012b). Gut microbial colonisation in premature neonates predicts neonatal sepsis. Arch. Dis. Child. Fetal Neonatal Ed. 97, F456–F462. doi: 10.1136/fetalneonatal-2011-301373
Makino, H., Martin, R., Ishikawa, E., Gawad, A., Kubota, H., Sakai, T., et al. (2015). Multilocus sequence typing of bifidobacterial strains from infant’s faeces and human milk: are bifidobacteria being sustainably shared during breastfeeding? Benef. Microbes 6, 563–572. doi: 10.3920/BM2014.0082
Maldonado, J., Cañabate, F., Sempere, L., Vela, F., Sánchez, A. R., Narbona, E., et al. (2012). Human milk probiotic Lactobacillus fermentum CECT5716 reduces the incidence of gastrointestinal and upper respiratory tract infections in infants. J. Pediatr. Gastroenterol. Nutr. 54, 55–61. doi: 10.1097/MPG.0b013e3182333f18
Maldonado, J., Lara-Villoslada, F., Sierra, S., Sempere, L., Gómez, M., Rodríguez, J. M., et al. (2010). Safety and tolerance of the human milk probiotic strain Lactobacillus salivarius CECT5713 in 6-month-old children. Nutrition 26, 1082–1087. doi: 10.1016/j.nut.2009.08.023
Maldonado-Lobón, J. A., Díaz-López, M. A., Carputo, R., Duarte, P., Díaz-Ropero, M. P., Valero, A. D., et al. (2015a). Lactobacillus fermentum CECT 5716 reduces Staphylococcus load in the breast milk of lactating mothers suffering breast pain: a randomized controlled trial. Breastfeed. Med. 10, 425–432. doi: 10.1089/bfm.2015.0070
Maldonado-Lobón, J. A., Gil-Campos, M., Maldonado, J., López-Huertas, E., Flores-Rojas, K., Valero, A. D., et al. (2015b). Long-term safety of early consumption of Lactobacillus fermentum CECT5716: a 3-year follow-up of a randomized controlled trial. Pharmacol. Res. 9, 12–19. doi: 10.1016/j.phrs.2015.01.006
Mann, T. Z., Haddad, L. B., Williams, T. R., Hills, S. L., Read, J. S., Dee, D. L., et al. (2018). Breast milk transmission of flaviviruses in the context of Zika virus: a systematic review. Paediatr. Perinat. Epidemiol. 32, 358–368. doi: 10.1111/ppe.12478
Marín, M. L., Arroyo, R., Jiménez, E., Gómez, A., Fernández, L., and Rodríguez, J. M. (2009). Cold storage of human milk, effect on its bacterial composition. J. Ped. Gastroenterol. Nutr. 49, 343–348. doi: 10.1097/MPG.0b013e31818cf53d
Martín, R., Jiménez, E., Heilig, H., Fernandez, L., Marin, M. L., Zoetendal, E. G., et al. (2009). Isolation of bifidobacteria from breast milk and assessment of the bifidobacterial population by PCR-DGGE and qRTi-PCR. Appl. Environ. Microbiol. 75, 965–969. doi: 10.1128/AEM.02063-08
Martín, R., Jiménez, E., Olivares, M., Marín, M. L., Fernández, L., Xaus, J., et al. (2006). Lactobacillus salivarius CECT 5713, apotential probiotic strain isolated from infant feces and breast milk of a mother-child pair. Int. J. Food Microbiol. 112, 35–43. doi: 10.1016/j.ijfoodmicro.2006.06.011
Martín, R., Langa, S., Reviriego, C., Jiminez, E., Marin, M. L., Xaus, J., et al. (2003). Human milk is a source of lactic acid bacteria for the infant gut. J. Pediatr. 143, 754–758. doi: 10.1016/j.jpeds.2003.09.028
Martín, R., Miquel, S., Benevides, L., Bridonneau, C., Robert, V., Hudault, S., et al. (2017). Functional characterization of novel Faecalibacterium prausnitzii strains isolated from healthy volunteers: a step forward in the use of F.prausnitzii as a next-generation probiotic. Front. Microbiol. 8:1226. doi: 10.3389/fmicb.2017.01226
Martín, R., Nauta, A., Ben Amor, K., Knippels, L., Knol, J., and Garssen, J. (2010). Early life: gut microbiota and immune development in infancy. Benef. Microbes 1, 367–382. doi: 10.3920/BM2010.0027
Martín, R., Olivares, M., Marín, M. L., Fernández, L., Xaus, J., and Rodríguez, J. M. (2005). Probiotic potential of 3 lactobacilli strains isolated from breast milk. J. Hum. Lact. 21, 8–17. doi: 10.1177/0890334404272393
Martín, V., Maldonado, A., Moles, L., Rodríguez-Baños, M., Del Campo, R., Fernández, L., et al. (2012). Sharing of bacterial strains between breast milk and infant feces. J. Hum. Lact. 28, 36–44. doi: 10.1177/0890334411424729
Marx, C., Bridge, R., Wolf, A. K., Rich, W., Kim, J. H., and Bode, L. (2014). Human milk oligosaccharide composition differs between donor milk and mother’s own milk in the NICU. J. Hum. Lact. 30, 54–61. doi: 10.1177/0890334413513923
McGuire, M. K., and McGuire, M. A. (2017). Got bacteria? The astounding, yet not-so-surprising, microbiome of human milk. Curr. Opin. Biotechnol. 44, 63–68. doi: 10.1016/j.copbio.2016.11.013
Meier, P. P., Johnson, T. J., Patel, A. L., and Rossman, B. (2017a). Evidence-based methods that promote human milk feeding of preterm infants: an expert review. Clin. Perinatol. 44, 1–22. doi: 10.1016/j.clp.2016.11.005
Meier, P. P., Patel, A. L., and Esquerra-Zwiers, A. (2017b). Donor human milk update: evidence, mechanisms and priorities for research and practice. J. Pediatrics 180, 15–21. doi: 10.1016/j.jpeds.2016.09.027
Meier, P. P., Patel, A. L., Hoban, R., and Engstrom, J. L. (2016). Which breast pump for which mother: an evidence-based approach to individualizing breast pump technology. J. Perinatol. 36, 493–499. doi: 10.1038/jp.2016.14
Meinzen-Derr, J., Poindexter, B., Wrage, L., Morrow, A. L., Stoll, B., and Donovan, E. F. (2009). Role of human milk in extremely low birth weight infants’ risk of necrotizing enterocolitis or death. J. Perinatol. 29, 57–62. doi: 10.1038/jp.2008.117
Milani, C., Mancabelli, L., Lugli, G. A., Durante, S., Turroni, F., Ferrario, C., et al. (2015). Exploring vertical transmission of bifidobacteria from mother to child. Appl. Environ. Microbiol. 81, 7078–7087. doi: 10.1128/AEM.02037-15
Miquel, S., Martín, R., Bridonneau, C., Robert, V., Sokol, H., Bermúdez-Humarán, L. G., et al. (2014). Ecology and metabolism of the beneficial intestinal commensal bacterium Faecalibacterium prausnitzii. Gut Microbes 5, 146–151. doi: 10.4161/gmic.27651
Mizuno, K., Sakurai, M., and Itabashi, K. (2015). Necessity of human milk banking in Japan: questionnaire survey of neonatologists. Pediatr. Int. 57, 639–644. doi: 10.1111/ped.12606
Moles, L., Escribano, E., de, Andrés J, Montes, M. T., Rodríguez, J. M., Jiménez, E., et al. (2015a). Administration of Bifidobacterium breve PS12929 and Lactobacillus salivarius PS12934, two strains isolated from human milk, to very low and extremely low birth weight preterm infants: a pilot study. J. Immunol. Res. 2015:538171. doi: 10.1155/2015/538171
Moles, L., Manzano, S., Fernández, L., Montilla, A., Corzo, N., Ares, S., et al. (2015b). Bacteriological, biochemical, and immunological properties of colostrum and maturemilkfrom mothers of extremely preterm infants. J. Pediatr. Gastroenterol. Nutr. 60, 120–126. doi: 10.1097/MPG.0000000000000560
Moles, L., Gómez, M., Heilig, H., Bustos, G., Fuentes, S., de Vos, W., et al. (2013). Bacterial diversity in meconium of preterm neonates and evolution of their fecal microbiota during the first month of life. PLoS One 8:e66986. doi: 10.1371/journal.pone.0066986
Montagne, P., Cuillière, M. L., Molé, C., Béné, M. C., and Faure, G. (1999). Immunological and nutritional composition of human milk in relation to prematurity and mother’s parity during the first 2 weeks of lactation. J. Pediatr. Gastroenterol. Nutr. 29, 75–80. doi: 10.1097/00005176-199907000-00018
Moro, G. E., Arslanoglu, S., and Minoli, I. (2015). Proceedings of a consensus development conference–EXPO 2015 Milan, Italy, May 15–16. J. Pediatr. Gastroenterol. Nutr. 61:1. doi: 10.1097/MPG.0000000000000897
Mshvildadze, M., Neu, J., Shuster, J., Theriaque, D., Li, N., and Mai, V. (2010). Intestinal microbial ecology in premature infants assessed with non-culture-based techniques. J. Pediatr. 156, 20–25. doi: 10.1016/j.jpeds.2009.06.063
Murk, W., Risnes, K. R., and Bracken, M. B. (2011). Prenatal or early-life exposure to antibiotics and risk of childhood asthma: a systematic review. Pediatrics 127, 1125–1138. doi: 10.1542/peds.2010-2092
Murphy, K., Curley, D., O’Callaghan, T. F., O’Shea, C. A., Dempsey, E. M., O’Toole, P. W., et al. (2017). The composition of human milk and infant fecal microbiota over the first three months of life: a pilot study. Sci. Rep. 7:40597. doi: 10.1038/srep40597
Nakamura, K., Kaneko, M., Abe, Y., Yamamoto, N., Mori, H., Yoshida, A., et al. (2016). Outbreak of extended–spectrum β–lactamase–producing Escherichia coli transmitted through breast milk sharing in a neonatal intensive care unit. J. Hosp. Infect. 92, 42–46. doi: 10.1016/j.jhin.2015.05.002
Neu, J. (2011). Routine probiotics for premature infants: let’s be careful! J. Pediatr. 158, 672–674. doi: 10.1016/j.jpeds.2010.11.028
Neuman, H., Debelius, J. W., Knight, R., and Koren, O. (2015). Microbial endocrinology: the interplay between the microbiota and the endocrine system. FEMS Microbiol. Rev. 39, 509–521. doi: 10.1093/femsre/fuu010
Neville, M. C. (2009). Introduction: tight junctions and secretory activation in the mammary gland. J. Mammary Gland Biol. Neoplasia 14, 269–270. doi: 10.1007/s10911-009-9150-8
NICE (2010). Clinical Guideline 93. Donor milk banks; service operation published by National Institute for Health and Care Excellence (NICE). Available at: https://www.nice.org.uk/guidance/cg93
Ofek Shlomai, N., Deshpande, G., Rao, S., and Patole, S. (2014). Probiotics for preterm neonates: what will it take to change clinical practice? Neonatology 105, 64–70. doi: 10.1159/000354891
Olszak, T., An, D., Zeissig, S., Vera, M. P., Richter, J., Franke, A., et al. (2012). Microbial exposure during early life has persistent effects on natural killer T cell function. Science 336, 489–493. doi: 10.1126/science.1219328
Omarsdottir, S., Casper, C., Navér, L., Legnevall, L., Gustafsson, F., Grillner, L., et al. (2015). Cytomegalovirus infection and neonatal outcome in extremely preterm infants after freezing of maternal milk. Pediatr. Infect. Dis. J. 34, 482–489. doi: 10.1097/INF.0000000000000619
Orloff, S. L., Wallingford, J. C., and McDougal, J. S. (1993). Inactivation of human immunodeficiency virus type I in human milk: effects of intrinsic factors in human milk and of pasteurization. J. Hum. Lact. 9, 13–17. doi: 10.1177/089033449300900125
Otto, M. (2009). Staphylococcus epidermidis, the ‘accidental’ pathogen. Nat. Rev. Microbiol. 7, 555–567. doi: 10.1038/nrmicro2182
Pang, W. W., and Hartmann, P. E. (2007). Initiation of human lactation: secretory differentiation and secretory activation. J. Mammary Gland Biol. Neoplasia 12, 211–221. doi: 10.1007/s10911-007-9054-4
Pannaraj, P. S., Li, F., Cerini, C., Bender, J. M., Yang, S., Rollie, A., et al. (2017). Association between breast milk bacterial communities and establishment and development of the infnat gut microbiome. JAMA Pediatr. 171, 647–654. doi: 10.1001/jamapediatrics.2017.0378
Park, B., Iwase, T., and Liu, G. Y. (2011). Intranasal application of S. epidermidis prevents colonization by methicillin-resistant Staphylococcus aureus in mice. PLoS One 6:e25880. doi: 10.1371/journal.pone.0025880
Patel, A., Johnson, T., Engstrom, J., Fogg, L., Jegier, B., Bigger, H., et al. (2013). Impact of early human milk on sepsis and health care costs in very low birthweight infants. J. Perinatol. 33, 514–519. doi: 10.1038/jp.2013.2
Patel, S. H., Vaidya, Y. H., Patel, R. J., Pandit, R. J., Joshi, C. G., and Kunjadiya, A. P. (2017). Culture independent assessment of human milk microbial community in lactational mastitis. Sci. Rep. 7:7804. doi: 10.1038/s41598-017-08451-7
Peila, C., Moro, G. E., Bertino, E., Cavallarin, L., Giribaldi, M., Giuliani, F., et al. (2016). The effect of holder pasteurization on nutrients and biologically-active components in donor human milk: a review. Nutrients 8:E477. doi: 10.3390/nu8080477
Petrof, E. O., Claud, E. C., Gloor, G. B., and Allen-Vercoe, E. (2013). Microbial ecosystems therapeutics: a new paradigm in medicine? Benef. Microbes 4, 53–65. doi: 10.3920/BM2012.0039
Pfaender, S., Vielle, N. J., Ebert, N., Steinmann, E., Alves, M. P., and Thiel, V. (2017). Inactivation of Zika virus in human breast milk by prolonged storage or pasteurization. Virus Res. 228, 58–60. doi: 10.1016/j.virusres.2016.11.025
Quigley, M., and McGuire, W. (2014). Formula versus donor breast milk for feeding preterm or low birth weight infants. Cochrane Database Syst. Rev. 22:CD002971. doi: 10.1002/14651858.CD002971.pub3
Ramel, S. E., Demerath, E. W., Gray, H. L., Younge, N., Boys, C., and Georgieff, M. K. (2012). The relationship of poor linear growth velocity with neonatal illness and two-year neurodevelopment in preterm infants. Neonatology 102, 19–24. doi: 10.1159/000336127
Renz, H., Brandtzaeg, P., and Hornef, M. (2012). The impact of perinatal immune development on mucosal homeostasis and chronic inflammation. Nat. Rev. Immunol. 12, 9–23. doi: 10.1038/nri3112
Rigourd, V., Meyer, V., Kieffer, F., Aubry, S., and Magny, J. F. (2011). HTLV and “donating” milk. Bull. Soc. Pathol. Exot. 104, 205–208. doi: 10.1007/s13149-011-0160-6
Rodríguez, J. M. (2014). The origin of human milk bacteria: is there a bacterial entero-mammary pathway during late pregnancy and lactation? Adv. Nutr. 5, 779–784. doi: 10.3945/an.114.007229
Romero-Gómez, M. P., Cabrera, M., Montes-Bueno, M. T., Cendejas-Bueno, E., Segovia, C., Pastrana, N., et al. (2015). Evaluation of cytomegalovirus infection in low-birth weight children by breast milk using a real-time polymerase chain reaction assay. J. Med. Virol. 87, 845–850. doi: 10.1002/jmv.24101
Ruiz, L., Moles, L., Gueimonde, M., and Rodríguez, J. M. (2016). Perinatal microbiomes’ influence on preterm birth and preterms’ health: influencing factors and modulation strategies. J. Pediatr. Gastroenterol. Nutr. 63, e193–e203. doi: 10.1097/MPG.0000000000001196
Sakata, H., Yoshioka, H., and Fujita, K. (1985). Development of the intestinal flora in very low birth weight infants compared to normal full-term newborns. Eur. J. Pediatr. 144, 186–190. doi: 10.1007/BF00451911
Sanders, M. E., Akkermans, L. M., Haller, D., Hammerman, C., Heimbach, J., Hörmannsperger, G., et al. (2010). Safety assessment of probiotics for human use. Gut Microbes 1, 164–185. doi: 10.4161/gmic.1.3.12127
Schanler, R. J. (2007). Mother’s own milk, donor human milk, and preterm formulas in the feeding of extremely premature infants. J. Pediatr. Gastroenterol. Nutr. 45(Suppl. 3), S175–S177. doi: 10.1097/01.mpg.0000302967.83244.36
Sherman, M. P., Zaghouani, H., and Niklas, V. (2015). Gut microbiota, the immune system, and diet influence the neonatal gut-brain axis. Pediatr. Res. 77, 127–135. doi: 10.1038/pr.2014.161
Shu, M., Wang, Y., Yu, J., Kuo, S., Coda, A., Jiang, Y., et al. (2013). Fermentation of Propionibacterium acnes, a commensal bacterium in the human skin microbiome, as skin probiotics against methicillin-resistant Staphylococcus aureus. PLoS One 8:e55380. doi: 10.1371/journal.pone.0055380
Siggers, R. H., Siggers, J., Thymann, T., Boye, M., and Sangild, P. T. (2011). Nutritional modulation of the gut microbiota and immune system in preterm neonates. J. Nutr. Biochem. 22, 511–521. doi: 10.1016/j.jnutbio.2010.08.002
Sisk, P. M., Lambeth, T. M., Rojas, M. A., Lightbourne, T., Barahona, M., Anthony, E., et al. (2017). Necrotizing enterocolitis and growth in preterm infants fed predominantly maternal milk, pasteurized donor milk, or preterm formula. A retrospective study. Am. J. Perinatol. 34, 676–683. doi: 10.1055/s-0036-1597326
Smith, M. M., Durkin, M., Hinton, V. J., Bellinger, D., and Kuhn, L. (2003). Initiation of breastfeeding among mothers of very low birth weight infants. Pediatrics 111, 1337–1342. doi: 10.1542/peds.111.6.1337
Sommer, F., and Bäckhed, F. (2013). The gut microbiota-masters of host development and physiology. Nat. Rev. Microbiol. 11, 227–238. doi: 10.1038/nrmicro2974
Soto, A., Martín, V., Jiménez, E., Mader, I., Rodríguez, J. M., and Fernández, L. (2014). Lactobacilli and bifidobacteria in human breast milk: influence of antibiotherapy and other host and clinical factors. J. Pediatr. Gastroenterol. Nutr. 59, 78–88. doi: 10.1097/MPG.0000000000000347
Srinivasjois, R., Rao, S., and Patole, S. (2013). Prebiotic supplementation in preterm neonates: updated systematic review and meta-analysis of randomized controlled trials. Clin. Nutr. 32, 958–965. doi: 10.1016/j.clnu.2013.05.009
Stensballe, L. G., Simonsen, J., Jensen, S. M., Bønnelykke, K., and Bisgaard, H. (2013). Use of antibiotics during pregnancy increases the risk of asthma in early childhood. J. Pediatr. 162, 832–838. doi: 10.1016/j.jpeds.2012.09.049
Sung, V., Hiscock, H., Tang, M., Mensah, F. K., Heine, R. G., Stock, A., et al. (2012). Probiotics to improve outcomes of colic in the community: protocol for the baby biotics randomized controlled trial. BMC Pediatr. 12:135. doi: 10.1186/1471-2431-12-135
Thurl, S., Munzert, M., Boehm, G., Matthews, C., and Stahl, B. (2017). Systematic review of the concentrations of oligosaccharides in human milk. Nutr. Rev. 75, 920–933. doi: 10.1093/nutrit/nux044
Uehara, Y., Kikuchi, K., Nakamura, T., Nakama, H., Agematsu, K., Kawakami, Y., et al. (2001). H2O2 produced by viridans group streptococci may contribute to inhibition of methicillin-resistant Staphylococcus aureus colonization of oral cavities in newborns. Clin. Infect. Dis. 32, 1408–1413. doi: 10.1086/320179
Urbaniak, C., McMillan, A., Angelini, M., Gloor, G. B., Sumarah, M., Burton, J. P., et al. (2014). Effect of chemotherapy on the microbiota and metabolome of human milk, a case report. Microbiome 2:24. doi: 10.1186/2049-2618-2-24
Valentine, C. J., Morrow, G., Fernandez, S., Gulati, P., Bartholomew, D., Long, D., et al. (2010). Docosahexaenoic acid and amino acid contents in pasteurized donor milk are low for preterm infants. J. Pediatr. 157, 906–910. doi: 10.1016/j.jpeds.2010.06.017
van Nood, E., Vrieze, A., Nieuwdorp, M., Fuentes, S., Zoetendal, E. G., de Vos, W. M., et al. (2013). Duodenal infusion of donor feces for recurrent Clostridium difficile. N. Engl. J. Med. 368, 407–415. doi: 10.1056/NEJMoa1205037
Vázquez-Fresno, R., Llorach, R., Marinic, J., Tulipani, S., Garcia-Aloy, M., Espinosa-Martos, I., et al. (2014). Urinary metabolomic fingerprinting after consumption of a probiotic strain in women with mastitis. Pharmacol. Res. 87, 160–165. doi: 10.1016/j.phrs.2014.05.010
Versalovic, J. (2013). The human microbiome and probiotics: implications for pediatrics. Ann. Nutr. Metab. 63(Suppl. 2), 42–52. doi: 10.1159/000354899
Vohr, B. R., Poindexter, B. B., Dusick, A. M., McKinley, L. T., Higgins, R. D., Langer, J. C., et al. (2007). Persistent beneficial effects of breast milk ingested in the neonatal intensive care unit on outcomes of extremely low birth weight infants at 30 months of age. Pediatrics 120, e953–e959. doi: 10.1542/peds.2006-3227
Vohr, B. R., Poindexter, B. B., Dusick, A. M., McKinley, L. T., Wright, L. L., Langer, J. C., et al. (2006). Beneficial effects of breast milk in the neonatal intensive care unit on the developmental outcome of extremely low birth weight infants at 18 months of age. Pediatrics 118, e115–e123. doi: 10.1542/peds.2005-2382
Vollmer, B., Seibold-Weiger, K., Schmitz-Salue, C., Hamprecht, K., Goelz, R., Krageloh-Mann, I., et al. (2004). Postnatally acquired cytomegalovirus infection via breast milk: effects on hearing and development in preterm infants. Pediatr. Infect. Dis. J. 23, 322–327. doi: 10.1097/00006454-200404000-00009
Wang, Q., Dong, J., and Zhu, Y. (2012). Probiotic supplement reduces risk of necrotizing enterocolitis and mortality in preterm very low-birth-weight infants: an updated meta-analysis of 20 randomized, controlled trials. J. Pediatr. Surg. 47, 241–248. doi: 10.1016/j.jpedsurg.2011.09.064
Westerbeek, E., van den Berg, A., Lafeber, H. N., Know, J., Fetter, W. P., and van Elburg, R. M. (2006). The intestinal bacterial colonization in preterm infants: a review of the literature. Clin. Nutr. 25, 361–368. doi: 10.1016/j.clnu.2006.03.002
Willing, B. P., Russell, S. L., and Finlay, B. B. (2011). Shifting the balance: antibiotic effects on host-microbiota mutualism. Nat. Rev. Microbiol. 9, 233–243. doi: 10.1038/nrmicro2536
World Health Organization [WHO] (2017). Donor Human Milk for Low–Birth–Weight Infants. Available at: http://www.who.int/elena/titles/donormilk_infants/en/
Yasuda, A., Kimura, H., Hayakawa, M., Ohshiro, M., Kato, Y., Matsuura, O., et al. (2003). Evaluation of cytomegalovirus infections transmitted via breast milk in preterm infants with a real-time polymerase chain reaction assay. Pediatrics 111, 1333–1336. doi: 10.1542/peds.111.6.1333
Keywords: human milk, microbiota, probiotics, lactic acid bacteria, minimal microbiota, preterm infants, NICU, human milk bank
Citation: Fernández L, Ruiz L, Jara J, Orgaz B and Rodríguez JM (2018) Strategies for the Preservation, Restoration and Modulation of the Human Milk Microbiota. Implications for Human Milk Banks and Neonatal Intensive Care Units. Front. Microbiol. 9:2676. doi: 10.3389/fmicb.2018.02676
Received: 17 July 2018; Accepted: 19 October 2018;
Published: 09 November 2018.
Edited by:
Paloma López, Centro de Investigaciones Biológicas (CIB), SpainReviewed by:
Sertac Arslanoglu, Istanbul Medeniyet University, TurkeyTeresa Requena, Consejo Superior de Investigaciones Científicas (CSIC), Spain
Copyright © 2018 Fernández, Ruiz, Jara, Orgaz and Rodríguez. This is an open-access article distributed under the terms of the Creative Commons Attribution License (CC BY). The use, distribution or reproduction in other forums is permitted, provided the original author(s) and the copyright owner(s) are credited and that the original publication in this journal is cited, in accordance with accepted academic practice. No use, distribution or reproduction is permitted which does not comply with these terms.
*Correspondence: Juan M. Rodríguez, am1yb2RyaWdAdmV0LnVjbS5lcw==