- School of Chemical Engineering, Yeungnam University, Gyeongsan, South Korea
Candida albicans is an opportunistic fungal pathogen and most prevalent species among clinical outbreaks. It causes a range of infections, including from mild mucosal infections to serious life-threatening candidemia and disseminated candidiasis. Multiple virulence factors account for the pathogenic nature of C. albicans, and its morphological transition from budding yeast to hyphal form and subsequent biofilm formation is regarded as the most important reason for the severity of Candida infections. To address the demanding need for novel antifungals, we investigated the anti-biofilm activities of various methylindoles against C. albicans using a crystal violet assay, and the metabolic activity was assessed by using a 2,3-bis (2-methoxy-4-nitro-5-sulfo-phenyl)-2H-tetrazolium-5-carboxanilide reduction assay. Changes in biofilm morphologies and thicknesses were determined by confocal laser scanning microscopy and scanning electron microscopy, respectively. Of the 21 methylindoles tested, 1-methylindole-2-carboxylic acid (1MI2CA) at 0.1 mM (17.5 μg ml-1) and 5-methylindole-2-carboxylic acid (5MI2CA) at 0.1 mM effectively inhibited biofilm formation by C. albicans DAY185 and ATCC10231 strains. Moreover, 1MI2CA and 5MI2CA both effectively inhibited hyphal formation, and thus, improved C. albicans infected nematode survival without inducing acute toxic effects. Furthermore, our in silico molecular modeling findings were in-line with in vitro observations. This study provides information useful for the development of novel strategies targeting candidiasis and biofilm-related infections.
Introduction
Half million of people are afflicted by candidiasis worldwide, which has an annual mortality rate of 45–75% per year (Brown et al., 2012). Furthermore, candidiasis is regarded as the third-to-fourth most frequent nosocomial infection in the United States (Hajjeh et al., 2004; Wisplinghoff et al., 2004; Wenzel, 2007). Candida albicans is the main etiologic agent of candidiasis and causes both superficial and systemic infections in an expanding population of individuals with diminished host immunity (Sudbery, 2011). The Centre for Disease Control (CDC, United States) published a list of the top 18 drug resistant threats, which includes fluconazole resistant C. albicans, entailing urgent attention to prevent the spread of Candida infections (CDC, 2013).
The treatment of C. albicans is complicated because of its ability to proliferate as a biofilm (Gulati and Nobile, 2016). C. albicans colonizes host tissues, catheters, and indwelling medical devices and develops biofilms on biotic and abiotic surfaces (Nobile et al., 2006). C. albicans biofilms are composed of yeast and hyphal and pseudohyphal elements (Nobile et al., 2006). In particular, cells within biofilms are difficult to kill as they exhibit high levels of resistance to most clinically used antifungals. For example, MIC values are often more than 100-fold greater than those needed to eradicate free planktonic cells, and thus, biofilms serve as a reservoir for recurring fungal infections (Mathe and Van Dijck, 2013). The morphogenetic transition of C. albicans from yeast cells to the hypha form is crucial for robust biofilm formation, which represents the major virulence factor associated with serious Candia infections (Mayer et al., 2013). Also, various clinical isolates of C. albicans showed drug resistance against commercial antifungals, such as, azoles and polyenes (Taff et al., 2013). Currently, researchers are trying to develop new approaches to combat complicated Candia infections using novel antifungal drugs that target virulence mechanisms rather than growth inhibition, because the latter is associated with the emergence of multidrug resistance.
Indoles are intracellular signaling molecules produced by a variety of Gram-positive and Gram-negative bacteria (Lee and Lee, 2010). Several indoles and their derivatives have been reported to suppress the virulence and inhibit biofilm formation by several bacterial species, such as, Staphylococcus aureus, Agrobacterium tumefaciens, Pseudomonas aeruginosa (Lee et al., 2009, 2012, 2013, 2015, 2016), and Vibrio cholera (Mueller et al., 2009). Furthermore, some indole derivatives, such as, indole-3-acetonitrile, waikialoid A, shearinines, and 7-benzyloxyindole, have also been reported to inhibit C. albicans biofilm formation and hyphal development (Oh et al., 2012; Wang et al., 2012; You et al., 2013; Manoharan et al., 2018). While several natural indole derivatives showed antibiofilm activity, but the number of active compounds is limited and methylindoles have not investigated yet.
The goal of the present study was to identify methylindoles that potently inhibit biofilm formation and hyphal development by fluconazole-resistant C. albicans. The anti-biofilm properties of 21 methylindoles were investigated. Morphological characterizations and phenotypic switching of C. albicans cells were observed by confocal laser scanning microscopy and scanning electron microscopy, respectively. In addition, the most effective compounds were also evaluated with respect to hyphal inhibition and anti-biofilm efficacy in a Caenorhabditis elegans (a nematode) infection model.
Materials and Methods
Fungal Strains, Media, Methylindoles, and Growth Condition
The fluconazole resistant strain C. albicans DAY185 and ATCC10231 used in this study were obtained from Korean Culture Center of Microorganisms1. Both strains were preserved at -80°C in potato dextrose broth (PDB) medium supplemented with 30% glycerol until use. For repetitive culture, cells from glycerol stock were proliferated by streaking onto potato dextrose agar (PDA) plates and incubated for 48 h at 37°C. Also, yeast extract-peptone-dextrose (YPD) medium was used to confirm the results of PDB medium.
A loopful of cells were then inoculated into 250 ml Erlenmeyer flasks containing 25 ml of PDB medium and cultured in an orbital shaker at 250 rpm for 24 h at 37°C. All 21 methylindole derivatives (Supplementary Table S1) examined during the study were purchased from Sigma-Aldrich (St. Louis, MO, United States) and Combi Blocks Inc. (San Diego, CA, United States), and dissolved in dimethyl sulfoxide (DMSO); the concentration of DMSO in media did not exceed 0.1% (vol/vol) in any experiment. Cell growths and turbidities were measured using spectrophotometer (UV-160, Shimadzu, Japan) at 600 nm. Minimum inhibitory concentrations (MICs) were determined using the broth dilution method of the Clinical Laboratory Standards Institute (CLSI) with slight modification (Alastruey-Izquierdo et al., 2015), using 96-well polystyrene plates (SPL Life Sciences, South Korea). Cells were cultured overnight in PDB medium, diluted to a concentration of 105 CFU/ml, added to wells in the absence or presence of varying concentrations (w/v) of methylindoles, and incubated for 24 h at 37°C. MIC was defined as the lowest concentration that inhibited microbial growth in PDB medium (300 μl) by at least 80% as assessed by spectrophotometry (620 nm) on 96-well polystyrene plates and colony counting. Each experiment was performed in triplicate.
Screening of Methylindoles for Inhibition of C. albicans Biofilm Formation
C. albicans biofilms were developed on 96-well polystyrene plates (SPL Life Sciences, South Korea), as described previously (Lee et al., 2011; Manoharan et al., 2017b). Briefly, C. albicans cells were harvested from overnight cultures and added to PDB medium (300 μl) at 105 CFU/ml and then cultured in the absence or presence of different concentrations of methylindoles for 24 h without shaking at 37°C. Biofilm formation was quantified by washing three times with water (to remove non-adherent cells), staining with crystal violet (0.1%) for 20 min, and then extracting the crystal violet with 95% ethanol. Absorbance was measured at 570 nm using a Multiskan EX microplate photometer (Thermo Fisher Scientific, Waltham, MA, United States) and results are presented as the averages of six replicates. Cell growth was measured at 620 nm.
Biofilm Metabolic Activity – XTT Reduction Assay
A XTT [2,3-bis(2-methoxy-4-nitro-5-sulfophenyl)-2H-tetrazolium-5-carboxanilide sodium salt] (Sigma-Aldrich, St. Louis, MO, United States) reduction assay was used to quantify C. albicans metabolic activity in biofilms, as previously described (Ramage et al., 2001; Nett et al., 2011). A working solution of XTT and menadione solution was prepared at 20:1 (v/v) immediately prior to assays. Biofilms were produced as described above for the biofilm inhibition assay, by culturing the cells for 24 h without shaking at 37°C. Following biofilm formation, planktonic cells were aspirated and transferred to fresh 96-well polystyrene plates. Biofilms were then washed twice with sterile water to remove non-adherent cells. Phosphate buffer saline (PBS) was then added to XTT-menadione solution (3.76:1 v/v) and 200 μl of this mix was added to planktonic and prewashed biofilm cells, which were then incubated in the dark for 3 h at 37°C. The colored supernatant (100 μl) was carefully transferred to fresh microtiter plates, and absorbance was measured at 450 nm using a Multiskan EX microplate photometer (Thermo Fisher Scientific, Waltham, MA, United States).
Colony Morphology of C. albicans on Solid Media
To investigate filament induction on solid media, C. albicans cells from glycerol stock, were plated on PDA agar plates including 10% fetal bovine serum supplemented with DMSO [less than 0.1% (vol/vol)] or methylindoles at 0.1 mM. Plates were then incubated for 6 days at 37°C, during which fungal colony morphologies were photographed after 2 days of interval using an iRiSTM Digital Cell Imaging System (Logos Biosystems, South Korea).
Confocal Laser Scanning Microscopy (CLSM)
C. albicans biofilms were developed on 96-well plates from re-suspended cells treated with methylindoles at 0.1 mM. After incubation for 24 h at 37°C, cells were washed with water three times to remove non-adherent cells and stained with carboxyfluorescein diacetate succinimidyl ester (Thermo Fisher Scientific, Invitrogen, MA, United States) (Weston and Parish, 1990). This method has been used previously (Nweze et al., 2012; Lee et al., 2018). Biofilms were visualized using an Ar laser (emission wavelength 500–550 nm) under a confocal laser microscope (Nikon Eclipse Ti, Tokyo) equipped with a 20× objective (Kim et al., 2012). Collected color confocal images (of at least 10 random positions) were constructed in three-dimensions using NIS-Elements C version 3.2 (Nikon eclipse). Assays were carried out using two independent cultures in triplicate. Biofilm formation was quantified by converting color confocal images (20 image stacks) to gray scale using ImageJ, and then using COMSTAT biofilm software (Heydorn et al., 2000) to calculate biomasses (μm3 μm-2), mean biofilm thicknesses (μm) and substratum coverages (%). Thresholding was fixed for all image stacks, and at least four positions and 20 planar images were analyzed per position.
Scanning Electron Microscopy (SEM)
C. albicans hyphal formation was observed by SEM as described previously (Lee et al., 2014). Briefly, nylon membrane filters (GE Healthcare Life Sciences, United States) were cut into 0.5 cm × 0.5 cm pieces and placed in wells of 96-well polystyrene plates before C. albicans biofilm formation. Cells were inoculated into 200 μl of PDB medium at an initial turbidity of OD 0.05 at 600 nm in the presence or absence of methylindoles and cultured under static conditions for 24 h at 37°C. Samples were then rinsed with PBS and fixed in 2.5% (v/v) glutaraldehyde and formaldehyde for 24 h at 4°C, post-fixed with sodium phosphate buffer and osmium tetroxide overnight and dehydrated using an ethanol series (50, 70, 80, 90, 95, and 100%) and isoamyl acetate. The nylon filters were dried in a critical-point dryer and then mounted onto aluminum stubs and sputter-coated with platinum. Biofilm cells were examined under S-4200 scanning electron microscope (Hitachi, Japan) at 15 kV and magnifications ranging from ×600 to ×6,000.
Candida Infection in the Caenorhabditis elegans Model
In order to investigate the effects of methylindoles on the virulence of C. albicans, C. elegans [fer-15 (b26); fem-1 (hc17)] was infected with C. albicans, as previously described (Manoharan et al., 2017c). Briefly, a freshly prepared overnight culture of C. albicans (100 μl) was spread onto lawns on PDA plates and incubated for 48 h at 37°C to produce abundant cells and its virulent characteristics (Okoli et al., 2009). Synchronized adult worms were then allowed to feed on C. albicans lawns for 4 h at 25°C when worms were collected and washed three times with sterile M9 buffer. Approximately 10 worms were then pipetted into single wells of 96-well plates containing PDB medium and treated with solutions (300 μl) of the compounds investigated at final concentrations of 0.1 or 0.5 mM. Untreated cells were used as controls. Plates were then incubated at 25°C for 4 days without shaking. Three independent experiments were conducted in triplicate. Results are expressed as percentages of alive or dead worms, as determined by touching worms with a platinum wire after 4 days of incubation. Observations were made using an iRiS Digital Cell Imaging System (Logos Biosystems, Korea).
Modeling and Docking Simulation
Molecular docking assays were performed, as previously described (Khan et al., 2017), to evaluate the interactions between methylindoles (1MI2CA and 5MI2CA) with the ATP binding sites of adenylyl cyclase (PDB, DOI: 10.2210/pdb1FX2/pdb) and compared with a known inhibitor of adenylate cyclase that is 2′,5′-dideoxyadenosine 3′-polyphosphate using Schrodinger software 11.4 (United States) (Rajasekharan et al., 2017). Higher negative Glide scores values indicate stronger binding interactions.
Statistical Analysis
The statistical analysis was performed by one-way ANOVA followed by Dunnett’s test using SPSS version 23 (SPSS Inc., Chicago, IL, United States). P-values of <0.05 were regarded significant.
Results
Methylindole Derivatives Inhibited C. albicans Biofilm Formation
We screened 21 commercially available methylindoles for their effects on biofilm formation by fluconazole resistant C. albicans DAY185 using a crystal violet assay. Initial screening showed that six of the 21 namely, 2-methylindole, 2,5-dimethylindole, 1-methylindole-2-carboxylic acid, 5-methylindole-2-carboxylic acid, 5-amino-2-methylindole, and 5-fluoro-2-methylindole at 0.1 mM significantly reduced C. albicans biofilm in PDB medium by more than 85% whereas other methylindole derivatives had less impact on C. albicans biofilm formation (Figures 1A,B). In particular, 1-methylindole-2-carboxylic acid (1MI2CA), and 5-methylindole-2-carboxylic acid (5MI2CA) at 0.1 mM inhibited biofilm formation by C. albicans DAY185 by 91 and 87%, respectively. In addition, at higher concentrations of 0.2 and 0.5 mM, 1MI2CA and 5MI2CA significantly and dose-dependently reduced biofilm formation by C. albicans DAY185 in PDB medium (Figure 2A). Similarly, the anti-biofilm activities of 1MI2CA and 5MI2CA were also observed in another strain of C. albicans ATCC10231 (Figure 2A). Specifically, 1MI2CA significantly reduced biofilm formation by C. albicans DAY185 by 92 and 96% at concentrations of 0.2 and 0.5 mM, respectively, and reduced biofilm formation by C. albicans ATCC10231 by 88, 93, and 95% at 0.1, 0.2, and 0.5 mM, respectively (Figure 2A). Both 1MI2CA and 5MI2CA slightly inhibited the planktonic cell growth of C. albicans DAY185 (by ∼30%) at a concentration of 0.1 mM (Figure 2B). The minimum inhibitory concentrations (MIC) of 1MI2CA and 5MI2CA against C. albicans DAY185 and ATCC10231 were ≥2 mM, while MICs of antifungal fluconazole are >10 mM (3060 μg/ml) in PDB and YPD media for the two strains. To confirm the antibiofilm activities in PDB medium, YPD medium was also used and found that 1MI2CA and 5MI2CA significantly inhibited biofilm formation by C. albicans DAY185 and ATCC10231 strains in a dose-dependent manner (Figure 2C). However, other active compounds 2-methylindole and 6-methylindole showed no antibiofilm activity on C. albicans ATCC10231 in PDB medium (data not shown). Hence, two most active compounds, 1MI2CA and 5MI2CA, were focused on further studies.
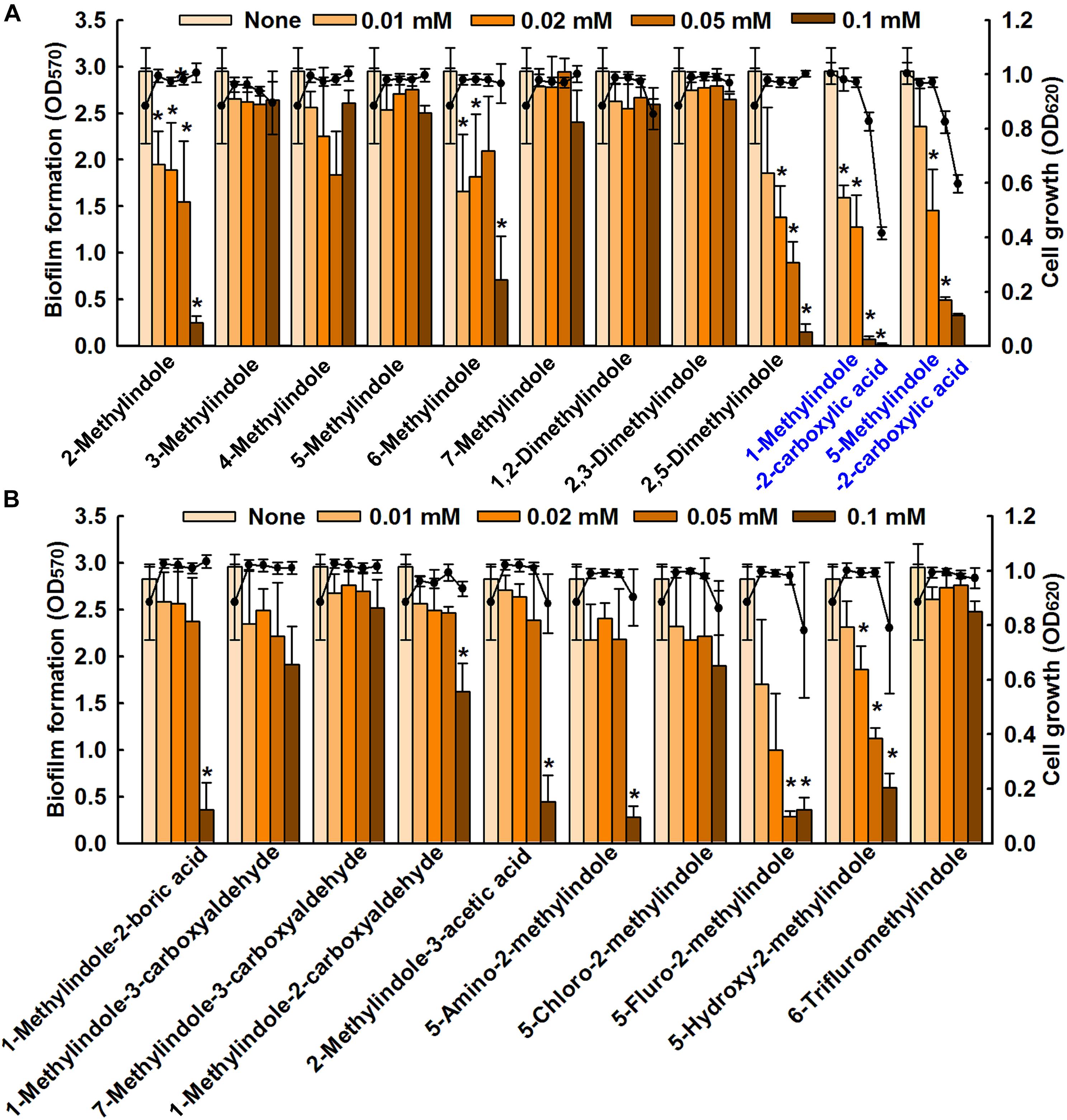
FIGURE 1. Inhibition of biofilm formation by methylindoles. The anti-biofilm activities of 21 methylindoles against C. albicans DAY185 were examined after incubation for 24 h (A,B). The chemical structures of methylindoles are shown in Supplementary Table S1. At least two independent experiments were conducted (six wells per sample); error bars indicate standard deviations. ∗p < 0.05 vs. untreated controls. Bars indicate biofilm formation and lines indicate planktonic cell growth.
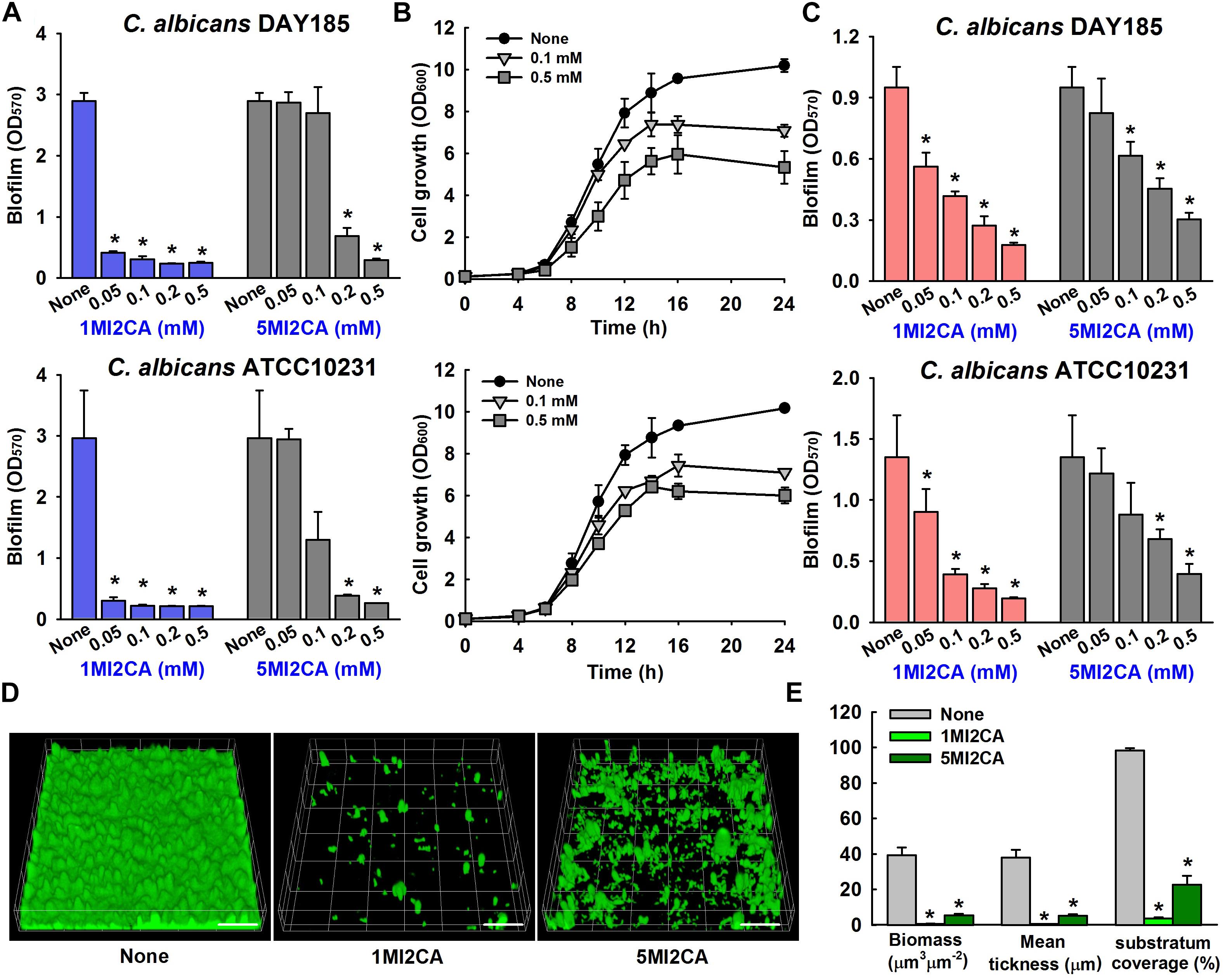
FIGURE 2. Effect of methylindoles on C. albicans biofilm formation and cell growth. The anti-biofilm activities of 1-methylindole-2-carboxylic acid (1MI2CA) and 5-methylindole-2-carboxylic acid (5MI2CA) were examined against C. albicans DAY185 and C. albicans ATCC10231 in PDB medium (A) and YPD medium (C) after incubation for 24 h. At least two independent experiments were conducted (six wells per sample). Planktonic cell growths of C. albicans DAY185 after incubation with 1MI2CA or 5MI2CA at their biofilm inhibitory concentrations of 0.1 and 0.5 mM for 24 h (B). Confocal laser scanning microscopic observations of the effects of methylindoles on C. albicans biofilms (D). Biofilm formation by C. albicans on polystyrene plates was observed in the presence of 1MI2CA or 5MI2CA at 0.1 mM by confocal laser microscopy. Scale bars represent 100 μm. Biofilm formation was quantified using COMSTAT (E). Two independent experiments were conducted (six wells per sample). Error bars indicate standard deviations. ∗p < 0.05 vs. untreated controls.
Confocal laser scanning microscopy (CLSM) images showed dense biofilm formation by untreated C. albicans cells, and remarkable reductions in biofilm adherence and thickness were observed in cells treated with 1MI2CA or 5MI2CA at 0.1 mM (Figure 2D). COMSTAT analysis showed that both 1MI2CA and 5MI2CA significantly reduced biofilm biomass (by 98.3 and 86.1%, respectively), average thickness (by 98.2 and 86.2%, respectively) and substrate coverage (by 96.2 and 76.9%, respectively) vs. untreated control cells (Figure 2E).
Methylindoles Affected the Metabolic Activity of C. albicans
XTT colorimetric assays were used to study the metabolic activities of fungal biofilm cells and planktonic cells in the presence or absence of 1MI2CA or 5MI2CA. Results are expressed as percentage metabolic activities of both treated and untreated cells. Treatment with 1MI2CA at 0.1 mM significantly reduced the metabolic activities C. albicans DAY185 and ATCC10231 biofilm cells by 97 and 58%, respectively, whereas 5MI2CA at 0.1 mM reduced metabolic activities by 66 and 41%, respectively. The viabilities of planktonic C. albicans DAY185 and ATCC10231 cells were slightly reduced (by ≤40%) by 1MI2CA or 5MI2CA at 0.1 mM (Figures 3A,B).
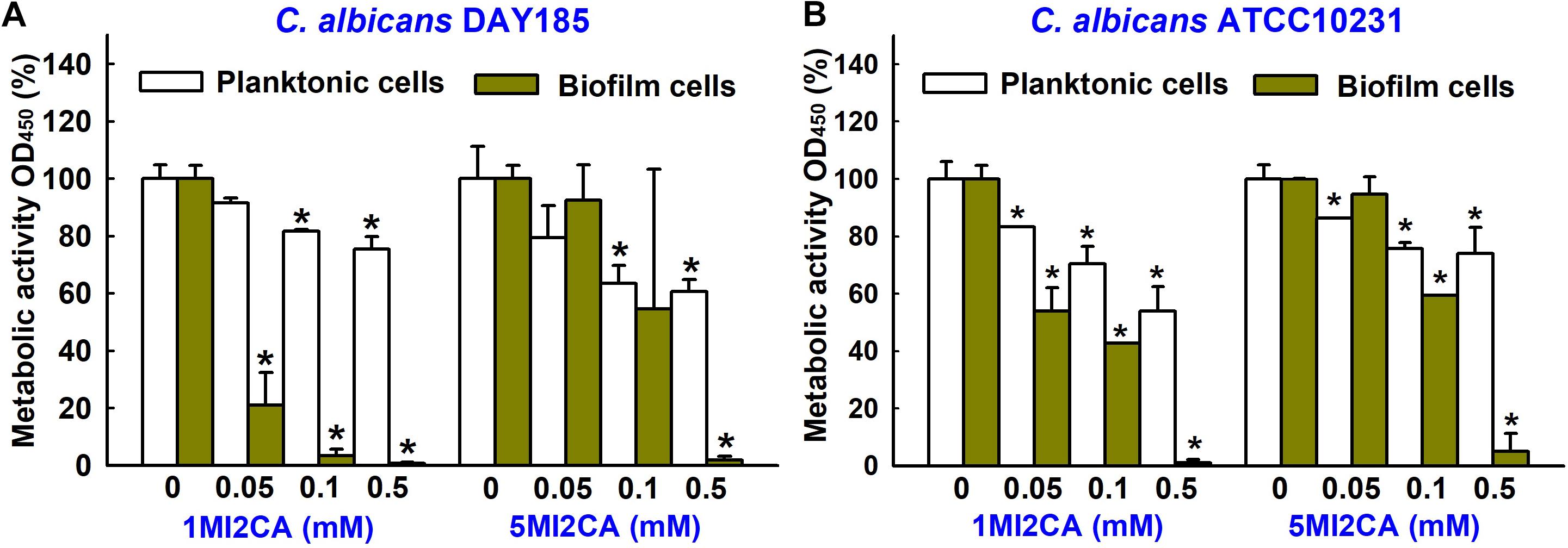
FIGURE 3. Effects of 1MI2CA and 5MI2CA on the metabolic activity of C. albicans. The metabolic activities of C. albicans DAY185 (A) and C. albicans ATCC10231 (B) planktonic cells and biofilms were quantified in the presence of methylindoles using an XTT reduction assay after incubation for 24 h. Results are presented as mean percentages of metabolic activity vs. untreated controls. Two independent experiments were conducted (six wells per sample); error bars indicate standard deviations. None indicates untreated samples. Error bars indicate standard deviations. ∗p < 0.05 vs. untreated controls.
Methylindoles Affected C. albicans Morphology and Hyphal Transition
The effects of 1MI2CA or 5MI2CA on C. albicans morphology and hyphal growth were assessed by visual observation. Yeast cells were cultivated on PDA agar plates in presence or absence of 1MI2CA or 5MI2CA at 37°C. Initial filament formation was observed in untreated fungal colony after 4 days of incubation. 1MI2CA or 5MI2CA at 0.1 mM well inhibited filamentation until 6 days (Figure 4A).
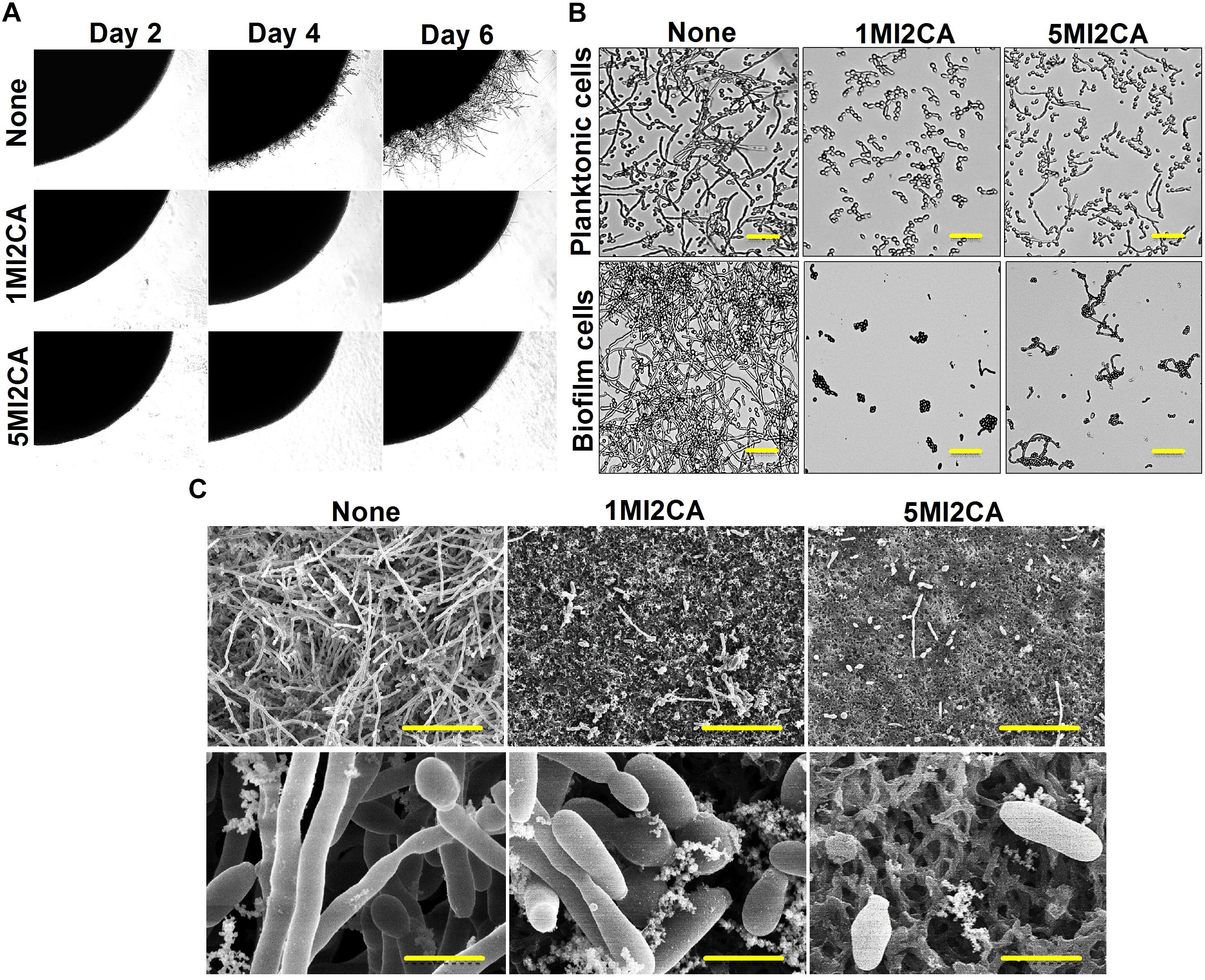
FIGURE 4. Effects of 1MI2CA and 5MI2CA on C. albicans DAY185 morphology and hyphal growth. C. albicans morphology on solid media (A). C. albicans was streaked on PDA agar plates supplemented with 10% fetal bovine serum in the absence or presence of 1MI2CA or 5MI2CA (0.1 mM). Colony morphologies were photographed every 2 days at 37°C. Inhibition of the hyphal growths of planktonic and biofilm cells of C. albicans (B). C. albicans was grown in PDB medium in the absence or presence of 1MI2CA or 5MI2CA (0.1 mM) at 37°C. Cultures were sampled after 24 h and photographed under a bright field microscope. The scale bar represents 100 μm. Microscopic observations (SEM) of the effects of the two methylindoles (0.1 mM) on biofilms and hyphal formation (C). C. albicans cells were grown on nylon filter paper in the presence or absence of 1MI2CA or 5MI2CA and visualized by SEM. Scale bars represent 50 and 5 μm, respectively. At least two independent experiments were conducted. None indicates untreated control cells.
The effects of 1MI2CA or 5MI2CA on C. albicans hyphal formation were examined in liquid medium (PDB) containing planktonic and biofilm cells under static anaerobic conditions at 37°C. In both independent experiments, untreated control cells showed massive hyphal formation after 24 h of incubation, but 1MI2CA (0.1 mM) treated cells showed no hyphal formation and cells remained in the rounded yeast form. Some cells (∼15%) treated with 5MI2CA at 0.1 mM developed hyphae (∼15%) (Figure 4B).
Scanning electron microscopy (SEM) showed 1MI2CA and 5MI2CA at 0.1 mM substantially reduced biofilm formation and suppressed hyphal transition on nylon membranes (Figure 4C). Untreated control biofilm cells exhibited large hyphae, pseudohyphae, and yeast cells, whereas methylindole treated cells showed greater numbers of yeast cells and fewer hyphae.
Methylindoles Interacted With Adenylate Cyclase in silico
Molecular docking was used to investigate the possible molecular mechanism of action of 1MI2CA and 5MI2CA. Adenylate cyclase of C. albicans plays a regulatory role in the cyclic AMP (cAMP) signaling pathway, which is essential for hyphal growth, virulence, and biofilm formation (Lu et al., 2014), and is thus viewed as a potential target for antifungal drug development. Anticipating that methylindoles might inhibit the function of adenylate cyclase, molecular docking was compared with 2′,5′-dideoxyadenosine 3′-polyphosphate (a known inhibitor of adenylate cyclase). As was expected, 2′,5′-dideoxyadenosine 3′-polyphosphate was found to form hydrogen bonds (binding energy: -4.09 kcal/mol) with catalytically important residues (arginine 987 and asparagine 980) of adenylate cyclase. Interestingly, both 1MI2CA and 5MI2CA formed hydrogen bonds with arginine 987 and asparagine 980 residues (Glide scores -3.90 and -4.07 kcal/mol, respectively) indicating interactions between both methylindoles (1MI2CA and 5MI2CA) and adenylate cyclase (Figure 5). However, the addition of cAMP up to 10 mM could not rescue the inhibitory effects of 1MI2CA and 5MI2CA (data not shown). Although speculative, 1MI2CA or 5MI2CA did not solely depend on the cAMP signaling pathway and could also affect other hyphae regulatory pathways.
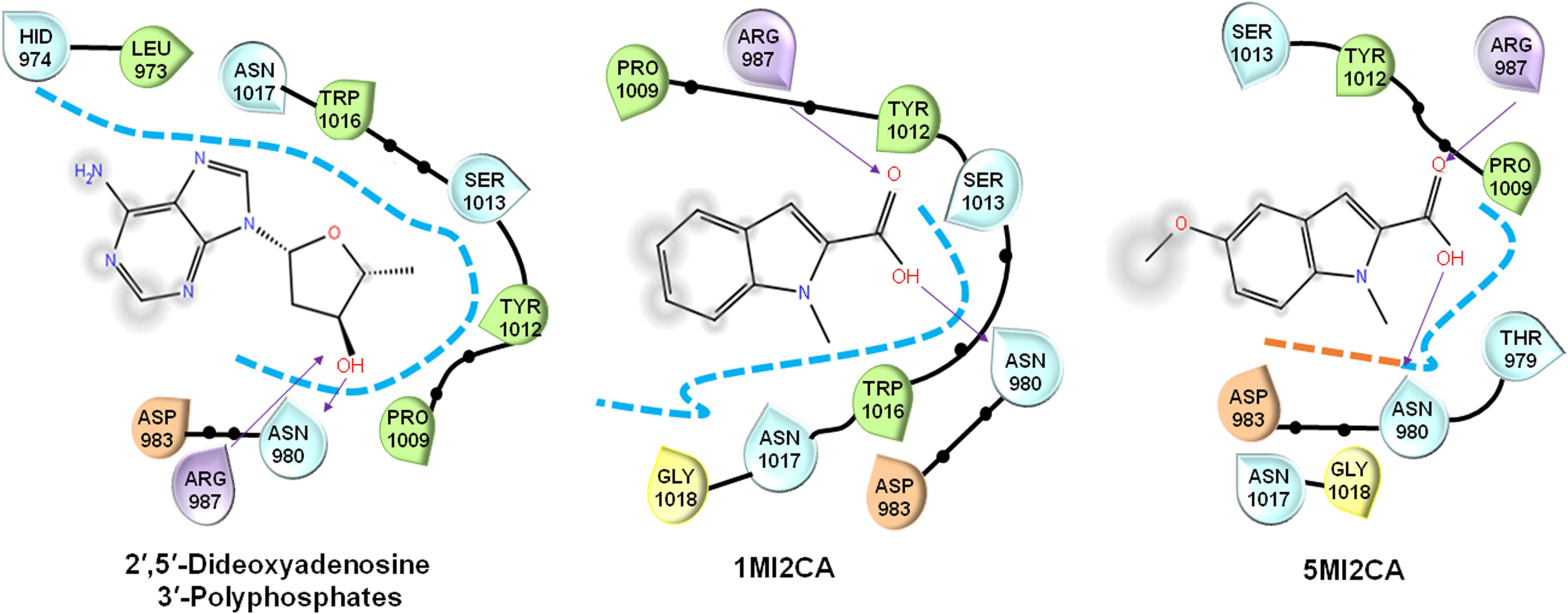
FIGURE 5. Molecular docking of the interaction between 1MI2CA or 5MI2CA and adenylate cyclase. The binding orientations of 1MI2CA and 5MI2CA within the active site of adenylate cyclase were similar to that of 2′,5′-dideoxyadenosine 3′-polyphosphate as a positive control.
Methylindoles Suppressed the Virulence of C. albicans in the Caenorhabditis elegans Infection Model
We examined the effects of 1MI2CA and 5MI2CA on hyphal formation and virulence by C. albicans in vivo using a C. elegans nematode infection model. Visual microscopic observations showed the hyphal formation in untreated C. albicans infected nematodes (Figure 6A). However, no hyphal formation has been observed in nematodes treated with both 1MI2CA and 5MI2CA at 0.5 mM. Furthermore, microscopic observations of nematodes showed that C. albicans infection caused 96% fatality of nematodes in 4 days (Figure 6B). However, about 40% of nematodes survived after 4 days in the presence of 1MI2CA at 0.5 mM and >30% survived in the presence of 5MI2CA at the same concentration. Fluconazole (the positive control) at 0.1 mM had a worm survival rate of >80% at 4 days (Figure 6B). In addition to rescue treatment, the toxicities of 1MI2CA and 5MI2CA have also been investigated in C. albicans uninfected C. elegans model. The results showed that >80% of worms were survived at 0.1 mM concentration of both methylindoles (1MI2CA and 5MI2CA) whereas at 0.5 mM, it was found to be mild toxic titer (about 40% survival) for worms. Fluconazole was found to have similar chemical cytotoxicity effect (Figure 6C).
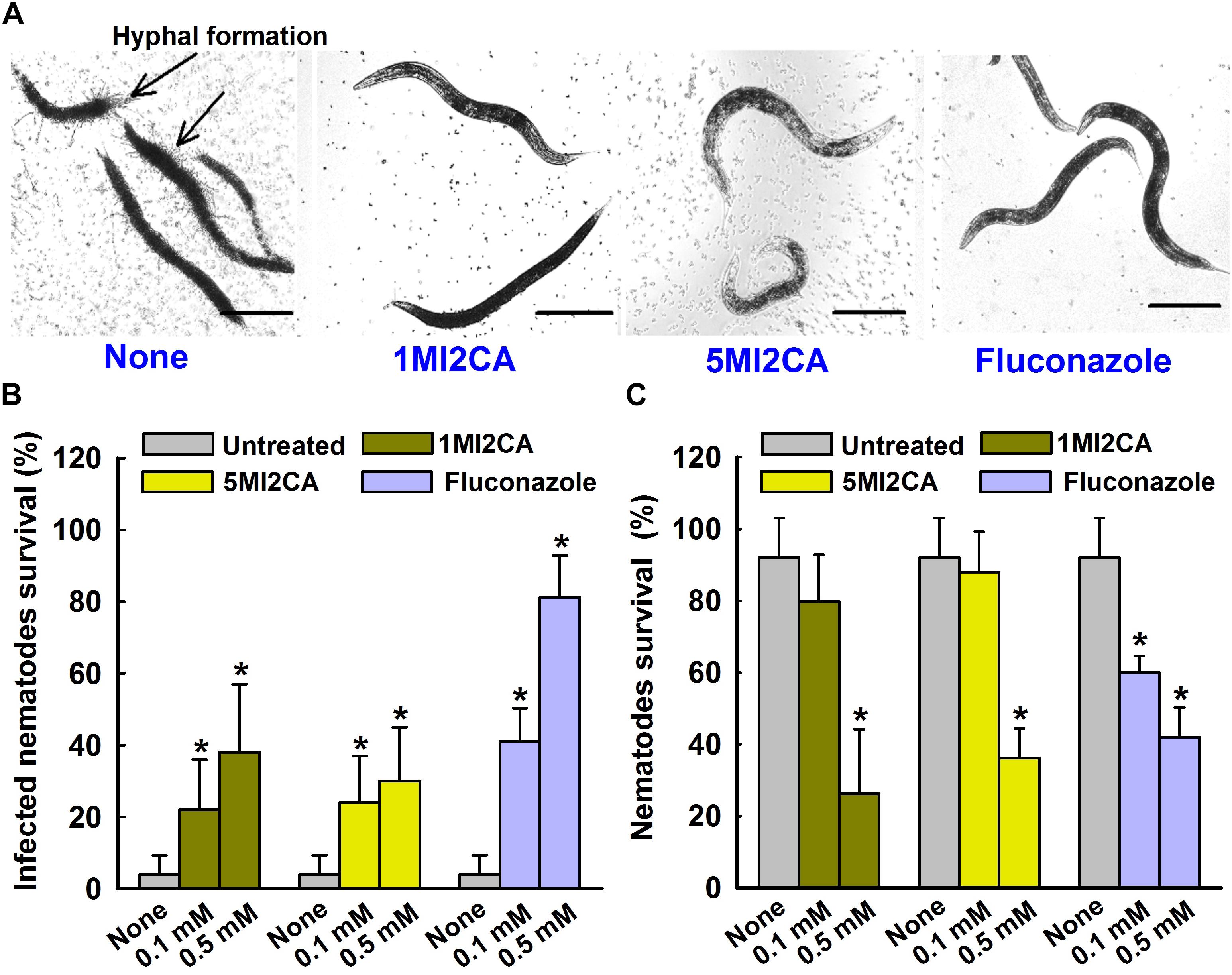
FIGURE 6. Effects of methylindoles on C. albicans virulence in the model of C. elegans. Microscopic images of C. albicans infected C. elegans in absence or presence of methylindoles (0.5 mM) or fluconazole as a positive control (0.1 mM) (A). The arrows indicate hyphal formation in untreated infected nematodes and the scale bar represents 100 μm. The bar graph shows nematode percentage survival after exposure of C. albicans for 4 days to methylindoles (0.1 or 0. 5 mM) (B). The toxicities of 1MI2CA and 5MI2CA were studied on non-infected nematodes by calculating survival rates after exposure for 4 days (C). Fluconazole (0.1 or 0.5 mM) was used as the positive control. None indicates untreated controls. Worm survival was determined based on movement. At least two independent experiments were conducted. Error bars indicate standard deviations. ∗p < 0.05 vs. untreated controls.
Discussion
C. albicans biofilm infections are a serious problem for individuals with an implanted medical device, because they are resistant to the majority of clinical antifungal agents (Silva et al., 2017). Some indole derivatives like indole-3-acetonitrile, tetracyclic indoles, and 7-benzyloxyindole have been reported for their antivirulence effects and drug resistance reversal potential against C. albicans (Oh et al., 2012; Youngsaye et al., 2012; Manoharan et al., 2018), which prompted us to screen various commercially available methylindoles for their abilities to inhibit biofilm formation by fluconazole resistant C. albicans DAY185 (Figure 1). In the present investigation, we found two methylindoles, that is, 1MI2CA and 5MI2CA, significantly inhibited C. albicans DAY185 biofilm formation by >85% at 0.1 mM, which was 20-fold lower than its MIC for planktonic cells (Figure 2). These findings show the antibiofilm activity of two methylindoles was not due to fungicidal effects, which may reduce the chance of the development of drug resistance.
The presence of a carboxyl group in these methylindoles might importantly contribute to their anti-biofilm activities, possibly because of the moderate electron withdrawing ability of this group. It has been previously reported that the presence of an electron withdrawing group enhanced the antimicrobial effects of novel quinoline derivatives (Desai et al., 2017). In another report, carboxylic acid derivatives such as indole-2-carboxylic acid benzylidenehydrazides had stronger anticancer activities than other indole derivatives because they induced cancer cell apoptosis pathways (Zhang et al., 2004), and in a recent study, carboxamide derivatives of indole were found to exhibit better antifungal activities than propanamide derivatives (Olgen et al., 2008). Based on these reports, it would appear the enhanced antibiofilm activities of 1MI2CA and 5MI2CA are due to the presence of a carboxyl group (Figure 2).
The morphogenetic switch from the fungal yeast cells to the hyphal form is most investigated virulence attribute of C. albicans (Nobile and Mitchell, 2005; Lu et al., 2014), Notably, hyphae formation is mainly responsible for the adherence of mature fungal biofilm cells to host surfaces or implant medical devices (Almeida et al., 2008; Lu et al., 2014). Recently, attempts have been made to identify the antivirulence agents that specifically target hyphal transition in C. albicans (Li et al., 2015; Khan et al., 2017; Yun and Lee, 2017). In the present study, it was found that both methylindoles (1MI2CA and 5MI2CA) remarkably reduced hyphal formation (Figure 4). Collectively, these findings of visual microscopic observation and SEM analysis showed that both methylindoles reduce the virulence of C. albicans by suppressing filamentation and biofilm formation. Similar results have been reported for cedar leaf essential oil, emodin, and cinnamaldehyde immobilized gold nanoparticles against C. albicans (Janeczko et al., 2017; Manoharan et al., 2017c; Ramasamy et al., 2017). cAMP-dependent protein kinase A (cAMP-PKA) signaling with other factors causes yeast to hyphal transition in C. albicans, and adenylate cyclase (Cyr1) regulates cAMP function (Lu et al., 2014). Our docking results revealed possible molecular interactions between 1MI2CA and 5MI2CA and the ATP binding pocket of adenylate cyclase (Figure 5), indicating that both 1MI2CA and 5MI2CA may regulate the function of cAMP. Therefore, affecting the hyphal development is supported our in vivo findings that both methylindoles suppressed virulence by preventing hyphal formation (Figures 5, 6).
Caenorhabditis elegans infection models have proven to be useful for studies on the determinants of fungal virulence (Pukkila-Worley et al., 2009). We showed that the hyphal form of C. albicans rapidly kills C. elegans (Figure 6A). The yeast cells of C. albicans are ingested into the digestive tract of C. elegans and where it undergoes the morphological transition that is hyphal form. Such hyphal cells persistently penetrate the collagenous cuticle layer of the worm, which results the death of the worm. On the other hand, in the presence of 1MI2CA or 5MI2CA (at 0.1 mM and 0.5 mM, respectively), C. elegans survival was markedly increased (Figure 6B), which we believe it was due to the inhibition of hyphal formation. Therefore, it could be concluded that the hyphal formation is critical for C. albicans pathogenesis in C. elegans infection model. Furthermore, this result is in accord with earlier reports on magnolol, honokiol, linalool α-longipinene (Sun et al., 2015; Manoharan et al., 2017a). We also examined the toxicities of 1MI2CA and 5MI2CA uninfected C. elegans (Figure 6C). It was found neither methylindole had a significant toxic effect and that both were effectively non-toxic at their biofilm inhibitory concentration of 0.1 mM.
Conclusion
In the present study, two methylindoles (1MI2CA and 5MI2CA) diminished the virulence of C. albicans by reducing hyphal development and biofilm formation in vitro and in vivo, which suggests both of these methylindoles have potential therapeutic applications against biofilm-associated C. albicans infections. In future, in vivo efficacy and pharmacokinetic studies using murine models would enable the establishment of the half-life of the molecule, bioavailability, and cytotoxicity.
Author Contributions
J-HL, Y-GK, VG, RM, and JL performed the experiments, analyzed the data, and wrote the manuscript. J-HL and JL designed the study. All authors have read and approved the final manuscript.
Funding
This research was supported by the Basic Science Research Program through the NRF funded by the Ministry of Education (Grant Nos. 2018R1D1A3B07040699 to J-HL and 2018R1D1A1B07044288 to JL) and by the Priority Research Centers Program through the NRF funded by the Ministry of Education (Grant No. 2014R1A6A1031189).
Conflict of Interest Statement
The authors declare that the research was conducted in the absence of any commercial or financial relationships that could be construed as a potential conflict of interest.
Acknowledgments
We thank Dr. Satish Kumar Rajasekharan for his kind support to perform the docking experiments.
Supplementary Material
The Supplementary Material for this article can be found online at: https://www.frontiersin.org/articles/10.3389/fmicb.2018.02641/full#supplementary-material
Footnotes
References
Alastruey-Izquierdo, A., Melhem, M. S., Bonfietti, L. X., and Rodriguez-Tudela, J. L. (2015). Susceptibility test for fungi: clinical and laboratorial correlations in medical mycology. Rev. Inst. Med. Trop. Sao Paulo 57, 57–64. doi: 10.1590/S0036-46652015000700011
Almeida, R. S., Brunke, S., Albrecht, A., Thewes, S., Laue, M., Edwards, J. E., et al. (2008). The hyphal-associated adhesin and invasin Als3 of Candida albicans mediates iron acquisition from host ferritin. PLoS Pathog. 4:e1000217. doi: 10.1371/journal.ppat.1000217
Brown, G. D., Denning, D. W., Gow, N. A., Levitz, S. M., Netea, M. G., and White, T. C. (2012). Hidden killers: human fungal infections. Sci. Transl. Med. 4:165rv113. doi: 10.1126/scitranslmed.3004404
CDC (2013). Centers for Disease Control and Prevention. Antibiotic Resistance Threats in the United States. Available at: https://www.cdc.gov/drugresistance/biggest_threats.html
Desai, N. C., Patel, B. Y., and Dave, B. P. (2017). Synthesis and antimicrobial activity of novel quinoline derivatives bearing pyrazoline and pyridine analogues. Med. Chem. Res. 26, 109–119. doi: 10.1007/s00044-016-1732-6
Gulati, M., and Nobile, C. J. (2016). Candida albicans biofilms: development, regulation, and molecular mechanisms. Microbes Infect. 18, 310–321. doi: 10.1016/j.micinf.2016.01.002
Hajjeh, R. A., Sofair, A. N., Harrison, L. H., Lyon, G. M., Arthington-Skaggs, B. A., Mirza, S. A., et al. (2004). Incidence of bloodstream infections due to Candida species and in vitro susceptibilities of isolates collected from 1998 to 2000 in a population-based active surveillance program. J. Clin. Microbiol. 42, 1519–1527. doi: 10.1128/JCM.42.4.1519-1527.2004
Heydorn, A., Nielsen, A. T., Hentzer, M., Sternberg, C., Givskov, M., Ersboll, B. K., et al. (2000). Quantification of biofilm structures by the novel computer program COMSTAT. Microbiology 146, 2395–2407. doi: 10.1128/AEM.68.4.2008-2017.2002
Janeczko, M., Maslyk, M., Kubinski, K., and Golczyk, H. (2017). Emodin, a natural inhibitor of protein kinase CK2, suppresses growth, hyphal development, and biofilm formation of Candida albicans. Yeast 34, 253–265. doi: 10.1002/yea.3230
Khan, S. N., Khan, S., Iqbal, J., Khan, R., and Khan, A. U. (2017). Enhanced killing and antibiofilm activity of encapsulated cinnamaldehyde against Candida albicans. Front. Microbiol. 8:1641. doi: 10.3389/fmicb.2017.01641
Kim, Y.-G., Lee, J.-H., Kim, C. J., Lee, J. C., Ju, Y. J., Cho, M. H., et al. (2012). Antibiofilm activity of Streptomyces sp. BFI 230 and Kribbella sp. BFI 1562 against Pseudomonas aeruginosa. Appl. Microbiol. Biotechnol. 96, 1607–1617. doi: 10.1007/s00253-012-4225-7
Lee, J., Attila, C., Cirillo, S. L., Cirillo, J. D., and Wood, T. K. (2009). Indole and 7-hydroxyindole diminish Pseudomonas aeruginosa virulence. Micro.b Biotechnol. 2, 75–90. doi: 10.1111/j.1751-7915.2008.00061.x
Lee, J.-H., Cho, H. S., Kim, Y.-G., Kim, J. A., Banskota, S., Cho, M. H., et al. (2013). Indole and 7-benzyloxyindole attenuate the virulence of Staphylococcus aureus. Appl. Microbiol. Biotechnol. 97, 4543–4552. doi: 10.1007/s00253-012-4674-z
Lee, J.-H., Cho, M. H., and Lee, J. (2011). 3-Indolylacetonitrile decreases Escherichia coli O157:H7 biofilm formation and Pseudomonas aeruginosa virulence. Environ. Microbiol. 13, 62–73. doi: 10.1111/j.1462-2920.2010.02308.x
Lee, J.-H., Kim, Y.-G., Baek, K. H., Cho, M. H., and Lee, J. (2015). The multifaceted roles of the interspecies signalling molecule indole in Agrobacterium tumefaciens. Environ. Microbiol. 17, 1234–1244. doi: 10.1111/1462-2920
Lee, J.-H., Kim, Y.-G., Cho, M. H., Kim, J. A., and Lee, J. (2012). 7-fluoroindole as an antivirulence compound against Pseudomonas aeruginosa. FEMS Microbiol. Lett. 329, 36–44. doi: 10.1111/j.1574-6968.2012.02500.x
Lee, J.-H., Kim, Y.-G., Choi, P., Ham, J., Park, J. G., and Lee, J. (2018). Antibiofilm and antivirulence activities of 6-gingerol and 6-shogaol against Candida albicans due to hyphal inhibition. Front. Cell. Infect. Microbiol. 8:299. doi: 10.3389/fcimb.2018.00299
Lee, J.-H., Kim, Y.-G., Gwon, G., Wood, T. K., and Lee, J. (2016). Halogenated indoles eradicate bacterial persister cells and biofilms. AMB Express 6:123. doi: 10.1186/s13568-016-0297-6
Lee, J.-H., and Lee, J. (2010). Indole as an intercellular signal in microbial communities. FEMS Microbiol. Rev. 34, 426–444. doi: 10.1111/j.1574-6968.2012.02500.x
Lee, K., Lee, J.-H., Kim, S. I., Cho, M. H., and Lee, J. (2014). Anti-biofilm, anti-hemolysis, and anti-virulence activities of black pepper, cananga, myrrh oils, and nerolidol against Staphylococcus aureus. Appl. Microbiol. Biotechnol. 98, 9447–9457. doi: 10.1007/s00253-014-5903-4
Li, Y., Chang, W., Zhang, M., Ying, Z., and Lou, H. (2015). Natural product solasodine-3-O-β-D-glucopyranoside inhibits the virulence factors of Candida albicans. FEMS Yeast Res. 15:fov060. doi: 10.1093/femsyr/fov060
Lu, Y., Su, C., and Liu, H. (2014). Candida albicans hyphal initiation and elongation. Trends Microbiol. 22, 707–714. doi: 10.1016/j.tim.2014.09.001
Manoharan, R. K., Lee, J. H., Kim, Y. G., Kim, S. I., and Lee, J. (2017a). Inhibitory effects of the essential oils α-longipinene and linalool on biofilm formation and hyphal growth of Candida albicans. Biofouling 33, 143–155. doi: 10.1080/08927014.2017.1280731
Manoharan, R. K., Lee, J. H., Kim, Y. G., and Lee, J. (2017b). Alizarin and chrysazin inhibit biofilm and hyphal formation by Candida albicans. Front. Cell. Infect. Microbiol. 7:447. doi: 10.3389/fcimb.2017.00447
Manoharan, R. K., Lee, J. H., and Lee, J. (2017c). Antibiofilm and antihyphal activities of cedar leaf essential oil, camphor, and fenchone derivatives against Candida albicans. Front. Microbiol. 8:1476. doi: 10.3389/fmicb.2017.01476
Manoharan, R. K., Lee, J. H., and Lee, J. (2018). Efficacy of 7-benzyloxyindole and other halogenated indoles to inhibit Candida albicans biofilm and hyphal formation. Microb. Biotechnol. 11, 1060–1069. doi: 10.1111/1751-7915.13268
Mathe, L., and Van Dijck, P. (2013). Recent insights into Candida albicans biofilm resistance mechanisms. Curr. Genet. 59, 251–264. doi: 10.1007/s00294-013-0400-3
Mayer, F. L., Wilson, D., and Hube, B. (2013). Candida albicans pathogenicity mechanisms. Virulence 4, 119–128. doi: 10.4161/viru.22913
Mueller, R. S., Beyhan, S., Saini, S. G., Yildiz, F. H., and Bartlett, D. H. (2009). Indole acts as an extracellular cue regulating gene expression in Vibrio cholerae. J. Bacteriol. 191, 3504–3516. doi: 10.1128/JB.01240-08
Nett, J. E., Cain, M. T., Crawford, K., and Andes, D. R. (2011). Optimizing a Candida biofilm microtiter plate model for measurement of antifungal susceptibility by tetrazolium salt assay. J. Clin. Microbiol. 49, 1426–1433. doi: 10.1128/JCM.02273-10
Nobile, C. J., and Mitchell, A. P. (2005). Regulation of cell-surface genes and biofilm formation by the C. albicans transcription factor Bcr1p. Curr. Biol. 15, 1150–1155. doi: 10.1016/j.cub.2005.05.047
Nobile, C. J., Nett, J. E., Andes, D. R., and Mitchell, A. P. (2006). Function of Candida albicans adhesin Hwp1 in biofilm formation. Eukaryot. Cell 5, 1604–1610. doi: 10.1128/EC.00194-06
Nweze, E. I., Ghannoum, A., Chandra, J., Ghannoum, M. A., and Mukherjee, P. K. (2012). Development of a 96-well catheter-based microdilution method to test antifungal susceptibility of Candida biofilms. J. Antimicrob. Chemother. 67, 149–153. doi: 10.1093/jac/dkr429
Oh, S., Go, G. W., Mylonakis, E., and Kim, Y. (2012). The bacterial signalling molecule indole attenuates the virulence of the fungal pathogen Candida albicans. J. Appl. Microbiol. 113, 622–628. doi: 10.1111/j.1365-2672.2012.05372.x
Okoli, I., Coleman, J. J., Tampakakis, E., An, W. F., Holson, E., Wagner, F., et al. (2009). Identification of antifungal compounds active against Candida albicans using an improved high-throughput Caenorhabditis elegans assay. PLoS One 4:e7025. doi: 10.1371/journal.pone.0007025
Olgen, S., Altanlar, N., Karatayli, E., and Bozdayi, M. (2008). Antimicrobial and antiviral screening of novel indole carboxamide and propanamide derivatives. Z. Naturforsch. C. 63, 189–195. doi: 10.1515/znc-2008-3-405
Pukkila-Worley, R., Peleg, A. Y., Tampakakis, E., and Mylonakis, E. (2009). Candida albicans hyphal formation and virulence assessed using a Caenorhabditis elegans infection model. Eukaryot. Cell 8, 1750–1758. doi: 10.1128/EC.00163-09
Rajasekharan, S. K., Lee, J. H., Ravichandran, V., and Lee, J. (2017). Assessments of iodoindoles and abamectin as inducers of methuosis in pinewood nematode, Bursaphelenchus xylophilus. Sci. Rep. 7:6803. doi: 10.1038/s41598-017-07074-2
Ramage, G., Vande-Walle, K., Wickes, B. L., and López-Ribot, J. L. (2001). Standardized method for in vitro antifungal susceptibility testing of Candida albicans biofilms. Antimicrob. Agents Chemother. 45, 2475–2479. doi: 10.1128/AAC.45.9.2475-2479.2001
Ramasamy, M., Lee, J. H., and Lee, J. (2017). Direct one-pot synthesis of cinnamaldehyde immobilized on gold nanoparticles and their antibiofilm properties. Colloids Surf. B Biointerfaces 160, 639–648. doi: 10.1016/j.colsurfb.2017.10.018
Silva, S., Rodrigues, C. F., Araujo, D., Rodrigues, M. E., and Henriques, M. (2017). Candida species biofilms’ antifungal resistance. J. Fungi 3:E8. doi: 10.3390/jof3010008
Sudbery, P. E. (2011). Growth of Candida albicans hyphae. Nat. Rev. Microbiol. 9, 737–748. doi: 10.1038/nrmicro2636
Sun, L., Liao, K., and Wang, D. (2015). Effects of magnolol and honokiol on adhesion, yeast-hyphal transition, and formation of biofilm by Candida albicans. PLoS One 10:e0117695. doi: 10.1371/journal.pone.0117695
Taff, H. T., Mitchell, K. F., Edward, J. A., and Andes, D. R. (2013). Mechanisms of Candida biofilm drug resistance. Future Microbiol. 8, 1325–1337. doi: 10.2217/fmb.13.101
Wang, X., You, J., King, J. B., Powell, D. R., and Cichewicz, R. H. (2012). Waikialoid a suppresses hyphal morphogenesis and inhibits biofilm development in pathogenic Candida albicans. J. Nat. Prod. 75, 707–715. doi: 10.1021/np2009994
Wenzel, R. P. (2007). Health care-associated infections: major issues in the early years of the 21st century. Clin. Infect. Dis. 45(Suppl. 1), S85–S88. doi: 10.1086/518136
Weston, S. A., and Parish, C. R. (1990). New fluorescent dyes for lymphocyte migration studies. analysis by flow cytometry and fluorescence microscopy. J. Immunol. Methods 133, 87–97. doi: 10.1016/0022-1759(90)90322-M
Wisplinghoff, H., Bischoff, T., Tallent, S. M., Seifert, H., Wenzel, R. P., and Edmond, M. B. (2004). Nosocomial bloodstream infections in US hospitals: analysis of 24,179 cases from a prospective nationwide surveillance study. Clin. Infect. Dis. 39, 309–317. doi: 10.1128/mSphere.00071-16
You, J., Du, L., King, J. B., Hall, B. E., and Cichewicz, R. H. (2013). Small-molecule suppressors of Candida albicans biofilm formation synergistically enhance the antifungal activity of amphotericin B against clinical Candida isolates. ACS Chem. Biol. 8, 840–848. doi: 10.1021/cb400009f
Youngsaye, W., Dockendorff, C., Vincent, B., Hartland, C. L., Bittker, J. A., Dandapani, S., et al. (2012). Overcoming fluconazole resistance in Candida albicans clinical isolates with tetracyclic indoles. Bioorg. Med. Chem. Lett. 22, 3362–3365. doi: 10.1016/j.bmcl.2012.02.035
Yun, D. G., and Lee, D. G. (2017). Assessment of silibinin as a potential antifungal agent and investigation of its mechanism of action. IUBMB Life 69, 631–637. doi: 10.1002/iub.1647
Keywords: methylindoles, C. albicans, biofilm, filamentation, C. elegans
Citation: Lee J-H, Kim Y-G, Gupta VK, Manoharan RK and Lee J (2018) Suppression of Fluconazole Resistant Candida albicans Biofilm Formation and Filamentation by Methylindole Derivatives. Front. Microbiol. 9:2641. doi: 10.3389/fmicb.2018.02641
Received: 04 July 2018; Accepted: 16 October 2018;
Published: 06 November 2018.
Edited by:
Jack Wong, The Chinese University of Hong Kong, ChinaReviewed by:
Luis Antonio Pérez-García, Universidad Autónoma de San Luis Potosí, MexicoRohitashw Kumar, University at Buffalo, United States
Copyright © 2018 Lee, Kim, Gupta, Manoharan and Lee. This is an open-access article distributed under the terms of the Creative Commons Attribution License (CC BY). The use, distribution or reproduction in other forums is permitted, provided the original author(s) and the copyright owner(s) are credited and that the original publication in this journal is cited, in accordance with accepted academic practice. No use, distribution or reproduction is permitted which does not comply with these terms.
*Correspondence: Jintae Lee, jtlee@ynu.ac.kr
†These authors have contributed equally to this work