- 1Centre for Viticulture and Enology, College of Food Science and Nutritional Engineering, China Agricultural University, Beijing, China
- 2Key Laboratory of Viticulture and Enology, Ministry of Agriculture, Beijing, China
Using novel non-Saccharomyces strains is regarded as an effective way to improve the aroma diversity of wines to meet the expectations of consumers. The non-Saccharomyces Hanseniaspora vineae and Metschnikowia pulcherrima have good aromatic properties useful for the production of table wine. However, no detailed information is available on their performances in icewine fermentation. In this study, simultaneous and sequential fermentation trials of indigenous M. pulcherrima CVE-MP20 or H. vineae CVE-HV11 with S. cerevisiae (SC45) were performed in 50-L fermenters of Vidal icewine, respectively. The results showed that SC45 cofermented with different non-Saccharomyces strains could generate a distinct aroma quality of icewine compared with four S. cerevisiae strain monocultures as evidenced by principal component analysis (PCA). Mixed fermentation of MP20/SC45 produced higher contents of acetate esters and β-damascenone with lower C6 alcohols relative to SC45 monoculture. Interestingly, HV11/SC45 generated the highest amounts of C6 alcohols [(Z)-3-hexen-1-ol and (E)-3-hexen-1-ol], higher alcohols (isobutanol, isopentanol, and 2-phenylethanol), acetate esters (2-phenethyl acetate and isoamyl acetate), cis-rose oxide, β-damascenone, and phenylacetaldehyde. Compared with simultaneous inoculation, sequential inoculation could achieve higher aroma diversity and produce higher intensity of fruity, flowery, and sweet attributes of icewine as assessed by calculating the odor activity values (OAVs). Our results verified the desired enological characteristics of H. vineae strain in icewine fermentation and also demonstrated that using indigenous non-Saccharomyces and Saccharomyces strains is a feasible way to improve aroma diversity of icewine products, which could provide an alternative way to meet the requirement of wine consumers for diversified aromatic quality.
Introduction
Icewine is a dessert wine that is made from grapes, which have been left on the vine until weather conditions are cold enough to freeze the grapes. During freezing, most of the water in grape berries is consequently frozen, and sugars, acids, and nitrogenous compounds are concentrated (Chamberlain et al., 1997). To produce an authentic icewine, the entire harvesting and pressing process must be carried out below −8°C, and the juice sugar content must be >35 Brix at the time of pressing (VQA Ontario, 20141). Vidal Blanc and Riesling are two main cultivars to produce icewine due to their relatively higher resistance to harsh conditions experienced by grapes prior to picking (Khairallah et al., 2016). Icewine contains higher and distinct volatile compounds relative to table wines, which largely determine the quality of icewine products (Bowen and Reynolds, 2015). The S. cerevisiae plays a major impact in the formation of icewine aromatic profiles because it produces a number of aroma compounds including higher alcohols, esters, fatty acids, and carbonyl compounds during alcoholic fermentation. Compared to table wine yeast, icewine yeast is challenged by more extreme stresses during alcoholic fermentation especially high sugar concentrations (above 35 Brix) and low fermentation temperature (15–18°C), which lead to reduced cell growth, prolonged fermentation time, and lower-than-desired alcohol levels (Kontkanen et al., 2004). These stresses can also induce significant changes of yeast metabolism and the production of metabolites including acetic acid, glycerol, and aromatic compounds, and ultimately affect icewine quality (Heit et al., 2018). Several works have confirmed that different commercial S. cerevisiae strains could produce diversified aromatic profiles during icewine fermentation and lead to different sensory characteristics (Erasmus et al., 2004; Crandles et al., 2015; Synos et al., 2015).
In recent years, the consumer requirements for distinctive aromatic characteristics urge winemakers to develop a variety of ways to manipulate specific aroma compounds and increase the complexity of wine. In this context, non-Saccharomyces wine yeasts have received more attention because these strains have several desired enological characteristics that are absent in S. cerevisiae, such as producing high levels of aroma compounds and producing, and secreting several enzymes (esterases, ß-glycosidases, lipases, and proteases) (Andorrà et al., 2012; Jolly et al., 2014). Cofermentations of S. cerevisiae with selected non-Saccharomyces yeasts in a controlled manner have proven to produce distinct aroma profiles and improve the complexity of table wine (Andorrà et al., 2010; Anfang et al., 2010; Jolly et al., 2014; Cañas et al., 2015). Among these non-Saccharomyces yeast species, Metschnikowia pulcherrima is often used to reduce alcohol of wine by sequential inoculation with S. cerevisiae (Varela et al., 2017). Cofermentation with Saccharomyces uvarum and this yeast can improve sensory attributes of wine by increasing the concentrations of 2-phenylethanol and 2-phenylethyl acetate (Varela et al., 2016), and a positive sensory contribution to wine was also found for Chenin Blanc in sequential fermentations (Jolly et al., 2017b). However, it was also reported that using the same inoculation method for Chardonnay can produce an inferior quality compared with fermentation using S. cerevisiae (Jolly et al., 2017a). Hanseniaspora vineae is a controversial non-Saccharomyces species belonging to Hanseniaspora spp., that is considered an apiculate yeast due to its cell morphology (Lleixà et al., 2016). Two earlier studies discouraged the development of apiculate yeasts in winemaking since the fermentation using their strains resulted in the production of large amounts of ethyl acetate and acetic acid (Velázquez et al., 1991; Ciani and Picciotti, 1995). However, inconsistent results have also been reported. Viana et al. (2011) and Medina et al. (2013) found that sequential fermentation of S. cerevisiae with H. vineae could increase fruity aromas and produce a high amount of acetate esters, such as 2-phenylethyl acetate and ethyl acetate, in the fermentations of Tempranillo and Chardonnay, respectively. These results suggested that the enological effect of non-Saccharomyces in mixed fermentation is largely dependent on inoculated species/strains, must composition, and fermentation conditions.
The nutrition status of must significantly influences aromatic profiles of wine products (Bell and Henschke, 2010). Considering the specific environment needed for icewine fermentation, it is interesting to investigate the effects of the multiculture of non-Saccharomyces and S. cerevisiae strains on aroma characteristics of icewine. However, until now, little information is available. Using autochthonous or locally selected wine yeasts is increasingly encouraged in wine-making because these yeasts can adapt well to the micro-conditions of the wine region and generate peculiar aromatic notes, which impart the wine with typical sensory characteristics specific to each wine area (Calabretti et al., 2012; Liu et al., 2016; Tofalo et al., 2016). The icewine industry in China has developed rapidly in recent years, and China has become an important icewine producer (Tang et al., 2013; Lan et al., 2016). Huairen is a typical icewine production region in the northeast of China with a suitable climate for icewine making (Huang et al., 2018). At present, most Chinese wineries use imported commercial yeast strains to inoculate icewine fermentation, which can reduce the variability of autochthonous yeast strains and thus, negatively influence the icewine aroma complexity and regional characteristics. In our previous work, two potential non-Saccharomyces wine strains M. pulcherrima CVE-MP20 and H. vineae CVE-HV11 had been isolated from spontaneous fermentation of Vidal icewine in Huairen. The preliminary experimental results showed that they have good technological characteristics and present potential application in icewine-making. To improve enological characteristics of icewine through the use of indigenous non-Saccharomyces strains, the effects of mixed fermentation of indigenous M. pulcherrima MP20 and H. vineae HV11 with indigenous icewine S. cerevisiae CVE-SC45 (simultaneous and sequential inoculation) on aromatic profiles of Vidal icewine were investigated in a 50 L fermenter, respectively in this study. Besides CVE-SC45 monoculture, the other three S. cerevisiae wine yeasts (one indigenous yeast strain CVE-SC42 and two commercial wine yeast strains XR and R2) were used as reference monoculture strains. The cell growth, physicochemical products, and aromatic compounds of icewine after alcoholic fermentation with different inoculations were determined and compared.
Materials and Methods
Yeast Strains
Four autochthonous strains, including two S. cerevisiae strains (CVE-SC42 and CVE-SC45) and two non-Saccharomyces strains (M. pulcherrima CVE-MP20 and H. vineae CVE-HV11), were used in the present study. They were isolated from 10-L spontaneous fermentation of Vidal grape juice with initial addition of 80 mg/L SO2 and fermentation under 15–17°C (Unpublished results). The determinations of technological characteristics showed that both non-Saccharomyces strains had high tolerance to ethanol, SO2, and sugar, low production of H2S, and high production of protease and glucosidase (Table S1). The four strains were deposited in the China General Microbiological Culture Collection Center (CGMCC), and the preservation numbers are 42-161017 (CVE-SC42), 45-161017 (CVE-SC45), 9-161017 (M. pulcherrima CVE-MP20), and 4-161017 (H. vineae CVE-HV11). Two commercial S. cerevisiae strains XR and R2 (Lallemand, France) were applied as reference strains as well. These strains were stored at −80°C in YPD medium with the addition of glycerol (20% v/v final concentration).
Pilot-Scale Fermentations
Vidal icewine was made in the 2015–2016 winter in Domaine Senpatina IceWine of Huairen in Liaoning Province (Northeast China). Grapes were harvested, destemmed, crushed, and pressed at −8°C to −9°C, and the grape juice (400 g/L of sugar, 13 g/L of total acidity, and a pH of 3.35) was transferred to a 50-L stainless steel fermenter containing 45 L Vidal grape juice with 80 mg/L SO2 and 40 mg/L pectinase HC (Lallemand, France) addition. The fermentation temperature was maintained at 16°C. The single fermentations of four S. cerevisiae strains and the mixed fermentations of two non-Saccharomyces strains with SC45 strain were carried out as followed: (1) single inoculation with SC42 (SC42); (2) single inoculation with SC45 (SC45); (3) single inoculation with XR (XR); (4) single inoculation with R2 (R2); (5) simultaneous inoculation of MP20 and SC45 (SI-MP20/SC45); (6) sequential inoculation of MP20 followed by SC45 after 2 days (SE-2-MP20/SC45); (7) sequential inoculation of MP20 followed by SC45 after 4 days (SE-4-MP20/SC45); (8) simultaneous inoculation of HV11 and SC45 (SI-HV11/SC45); (9) sequential inoculation of HV11 followed by SC45 after 2 days (SE-2-HV11/SC45); and (10) sequential inoculation of HV11 followed by SC45 after 4 days (SE-4-HV11/SC45). Total inoculation amount in each treatment was controlled at 1 × 106 viable cells/mL for S. cerevisiae and 1 × 107 viable cells/mL for non-Saccharomyces. Every experiment was set up in duplicate. Fermentation kinetics and yeast growth were monitored by determining specific gravity and viable cell counts every day. The alcoholic fermentations proceeded for 26 d (pure fermentation) and 30 d (mixed fermentation). After alcoholic fermentation, the final samples were centrifuged to remove yeast cells and stored at −20°C for analysis of residual sugar (glucose and fructose), main products (glycerol, ethanol, acetic acid, and other nonvolatile acids), and volatile compounds.
Analytical Techniques
The quantity of yeasts was determined by plating on a WL nutrient agar containing 100 mg/L chloramphenicol inhibiting bacterial growth. Plates were incubated at 30°C for 72 h. On WL nutrient agar plates, yeast colonies belonging to S. cerevisiae and non-Saccharomyces were distinguished by their different morphological characteristics. Ethanol, acetic acid, glucose, fructose, glycerol, and organic acids were determined by an HPX-87H Aminex ion-exchange column (300 × 7.8 mm, Bio-Rad Laboratories, Hercules, CA, USA) with 5 mM sulfuric acid as the mobile phase (Duan et al., 2015). The volatile compounds of final wines were determined by headspace solid-phase micro-extraction coupled with gas chromatography–mass spectrometry (HS-SPME-GC-MS) according to our previous study (Zhang et al., 2011; Xu et al., 2015; Liu et al., 2016). Identification of the aroma compounds was based on retention indices of reference standards and mass spectra matching in the standard NIST 11 library. For quantification of these compounds, the relative peak area of each identified compound was measured and then compared with the relative peak area of the added internal standard. Analyses were performed in triplicate. The detailed quantitation information about quantitative ion, quantitative standards, calibration curves, and R2 for the quantification of volatile compounds used in this study was provided in Table S2.
Data Analysis
One-way ANOVA using the Duncan test at significance level p ≤ 0.05 was carried out to uncover statistical differences between the wines produced from the different inoculation protocols. Principal component analysis (PCA) was carried out using the concentrations of volatile compounds to visualize the differences between wines fermented by different strains and inoculation methods. Statistical analyses were performed with the IBM SPSS Statistical Package (version 24.0, IBM Corp, NY, USA).
Results
The Cell Growth and Main Fermentation Products
The growth dynamics of S. cerevisiae and non-Saccharomyces in pure cultures or mixed cultures are presented in Figure 1. Four S. cerevisiae showed similar growth profiles in pure cultures, and reached the maximum cell number of around 3 × 107 CFU/mL after 4 days (Figure 1A). In mixed fermentations, inoculation of non-Saccharomyces decreased the cell growth rate of S. cerevisiae in comparison with that of pure culture because the time of SC45 reaching the highest population (3 × 107 CFU/mL) was delayed by 4–12 days. The strong inhibitions were observed in later inoculation of S. cerevisiae (4 days inoculation). M. pulcherrima and H. vineae reached the maximum population after 4–8 d fermentation. After this, they rapidly decreased although the inoculation amount of MP20 and HV11 was ten-folds of SC45. Simultaneously, S. cerevisiae gradually became the dominant strains. The decreased cell number of non-Saccharomyces could be associated with their low ethanol tolerance or to the production of other toxic compounds besides ethanol (Tofalo et al., 2016).
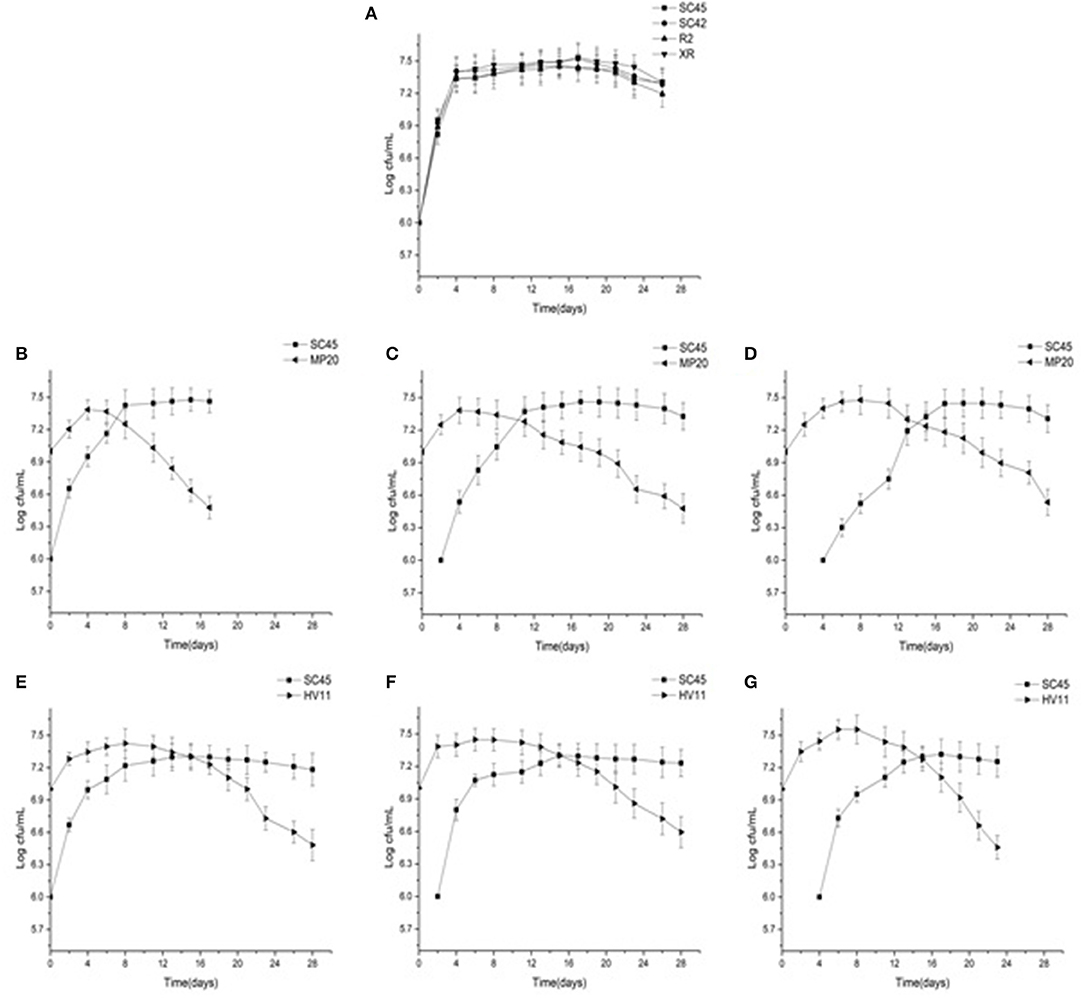
Figure 1. Growth profiles of four S. cerevisiae strains in pure fermentation and S. cerevisiae and non-Saccharomyces strains in mixed fermentations of MP20/SC45 and HV11/SC45 during alcoholic fermentation of vidal icewine. (A) Four S. Cerevisiae strains single fermentation. (B) Simulataneous inoculation of MP20 and SC45 (SI-MP20/SC45). (C) Sequential inoculation of MP20 followed by SC45 after 2 days (SE-2-MP20/SC45). (D) Sequential inoculation of MP20 followed by SC45 after 4 days (SE-4-MP20/SC45). (E) Simultaneous inoculation of HV11 and SC45 (SI-HV11/SC45). (F) Sequential inoculation of HV11 followed by SC45 after 2 days (SE-2-HV11\SC45). (G) Sequential inoculation of HV11 followed by SC45 after 4 days (SE-4-HV11/SC45).
The main fermentation products including ethanol, glycerol, acetic acid, nonvolatile acids, and residual sugars are presented in Table 1. The ethanol concentrations were much different in all trials (10.89–12.36%) with the lowest level in SE-2-MP20/SC45 and the highest level in SI-MP20/SC45. Pure cultures of four S. cerevisiae strains left similar content of sugar (181.2–193.58 g/L). More sugar was consumed by mixed fermentation and the residual sugar concentrations were further decreased to 160.39–189.94 g/L with the lowest amount in SI-MP20/SC45. This might explain the data that the highest ethanol content was produced in the wine of SI-MP20/SC45. Glycerol contents ranged from 9.32 g/L (SC42) to 10.28 g/L (SC45) with no significant differences between various treatments. The amounts of acetic acid in all wines ranged from 1.41 to 1.71 g/L with the lowest level in SC42 wine. No significant differences were observed in the amounts of other organic acids including citric acid, malic acid, lactic acid, oxalic acid, and succinic acid in different icewines.
Volatile Aroma Compounds in Vidal Icewine
A total of fifty-two volatiles were detected in all samples (Table S3).The characteristic aroma compounds of Vidal icewine were presented in Table 2, including ten high alcohols, ten esters, four terpenes, one aldehyde, and one C13-norisoprenoid, in which sixteen whose odor activity value (OAV) exceeding one were underlined. It should be mentioned that there were not many references, which have given the thresholds for these aroma compounds in the icewine matrix. Thresholds of Some compounds were, therefore, obtained either from the system of table wine or ethanol solution (Table S2).
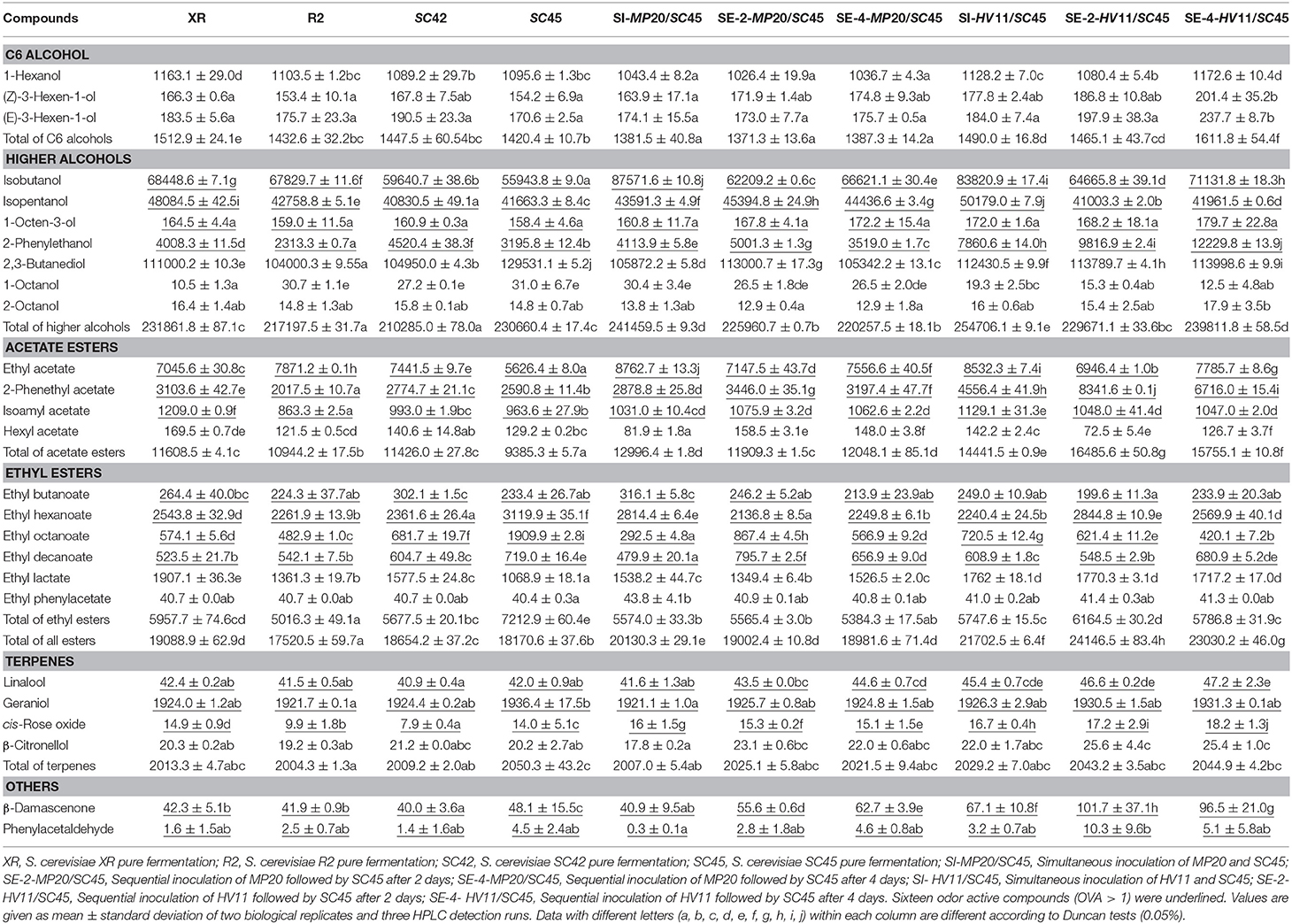
Table 2. Twenty-six main volatile aroma compounds (μg/L) in icewines obtained with four S. cerevisiae pure fermentations and mixed fermentations of non-Saccharomyces and SC45 after alcohol fermentation.
C6 Alcohols and Other Higher Alcohols
The C6 alcohols including 1-hexanol, (Z)-3-hexen-1-ol, and (E)-3-hexen-1-ol usually have the character of “vegetal” and “herbaceous” note and cause a negative effect on wine aroma (Ferreira et al., 2000). The XR and SC45 produced the highest (1512.9 μg/L) and lowest (1420.4 μg/L) total levels of C6 alcohols in pure cultures, respectively (p < 0.05). Coinoculation of H. vineae with SC45 further increased C6 alcohols compared with SC45 monoculture and the highest contents (1611.8 μg/L) were found in SE-4-HV11/SC45, followed by SI-HV11/SC45, and SE-2-HV11/SC45. In comparison, MP20/SC45 produced less C6 alcohols than SC45 monoculture. Seventeen higher alcohols were identified in this study (Table S3), and five compounds (isobutanol, isopentanol, 1-octen-3-ol, 2, 3-butanediol, and 2-phenylethanol) exceed their odor threshold. SC42 and SI-HV11/SC45 produced the lowest (210.3 mg/L) and the highest contents (254.7 mg/L), respectively (p < 0.05). Among S. cerevisiae strains, XR is a strong producer of higher alcohol due to generating more isobutanol, isopentanol, and 1-octen-3-ol. SC42 produced the highest level of 2-phenylethanol (4520.4 μg/L) among four S. cerevisiae strains. 2-Phenylethanol (floral note) is a desired aroma compound in wines (Mendes et al., 2012). SC45 formed comparable amount of higher alcohol with XR. Interestingly, co-inoculation of SC45 with HV11 and MP20 significantly improved the production of higher alcohols (including isobutanol, isopentanol, isopentanol, 2-phenylethanol, and total content). The SI MP20/SC45 produced the highest concentration of isobutanol, followed by SI HV11/SC45, which was 56.5 and 49.8% higher than that of SC45 monoculture (p < 0.05), and also 27.9 and 22.4% higher than that of XR monoculture (p < 0.05). Isopentanol content in SI-HV11/SC45 wine was increased by 20.4% compared to SC45 wine (p < 0.05). More importantly, SE-4-HV11/SC45 produced the highest amount of 2-phenylethanol, which was 2.82, 2.05, and 1.70 folds higher than those of monocultures of SC45, XR, and SC42, respectively.
Esters
Eighteen esters were identified in all samples (Table S3) and seven compounds exceeded the individual odor threshold in this study, including three acetate esters (ethyl acetate, 2-phenethyl acetate, isoamyl acetate) and four ethyl esters (ethyl butanoate, ethyl hexanoate, ethyl octanoate, ethyl decanoate) (Table 2). The highest and lowest total concentrations of esters were produced by SE-2-HV11/SC45 (24146.5 μg/L) and R2 monoculture (17520.5 μg/L), respectively (p < 0.05). Different S. cerevisiae strains had distinct impact on formation of particular esters. The XR produced the maximum concentration of isoamyl acetate (1209.0 μg/L), hexyl acetate (169.5 μg/L), and ethyl lactate (1907.1 μg/L). R2 generated more ethyl acetate, but lower contents of ethyl octanoate, 2-phenethyl acetate, and isoamyl acetate. Indigenous SC42 was characterized by the highest amount of ethyl butanoate, while SC45 was featured by the highest amount of ethyl hexanoate, ethyl octanoate, and ethyl decanoate among four S. cerevisiae strains; especially, for ethyl octanoate (1909.9 μg/L), the value was 2.95 and 2.33 folds higher than those of R2 and XR strains, respectively. Similar to higher alcohols, mixed fermentations led to different profiles of esters formation. Mixed fermentations promoted the productions of acetate ester, especially HV11/SC45. The highest amount of acetate esters was formed by SE-2-HV11/SC45, and 2-phenethyl acetate (fruity and floral note) was 2.22 and 1.69 folds higher than those of SC45 and XR, respectively. Conversely, mixed fermentations decreased most ethyl esters (ethyl hexanoate, ethyl octanoate, and ethyl decanoate) relative to SC45 monoculture with the exception of ethyl lactate, and the pronounced decrease was observed in the wine of SE-4-MP20/SC45. Although resulting in decrease of fatty acid ethyl esters, the high increase of acetate esters led to the highest contents of esters still achieved in SE-2-HV11/SC45 wine (24146.5 μg/L), which was a 32.9% increaes relative to SC45 wine (p < 0.05).
Terpenes, C13-Norisoprenoid, and Aldehyde
Terpenes and C13-norisoprenoids are derived from grapes and have a major positive impact on floral aroma of wines directly or through synergistic effects (Swiegers and Pretorius, 2005). Six terpenes were detected in all samples and three compounds with OAV above one, including linalool (sweet and floral note), geraniol (floral note), and cis-rose oxide (floral note). There were no significant differences in the contents of linalool (40.9–47.2 μg/L) and geraniol (1921.1–1936.4 μg/L) among all samples, while cis-rose oxide, generating lychee and rose smell to Vidal icewine (Ma et al., 2017), showed significant increment by mixed fermentation with 30.0% raise in SE-4-HV11/SC45 wine relative to SC45 wine (p < 0.05), followed by SE-2-HV11/SC45 wine and SI HV11/SC45 wine. β-Damascenone and phenylacetaldehyde are the key odorants in Vidal icewine and impart floral and honey notes to icewine (Ma et al., 2017). Due to low sensory threshold (0.05 and 1 μg/L, respectively), small variation of their concentrations can result in significant influence on the entire aroma profiles of wines. Both compounds exceeded individual sensory threshold in all samples, and the highest values were found in the SC45 wine among the four monoculture wines. As expected, HV11/SC45 further increased the concentrations of both compounds. The content of β-damascenone in SE-2-HV11/SC45 wine was 111.4% higher than that of SC45 wine (p < 0.05), and phenylacetaldehyde concentration achieved 128.9% increment although having no statistical difference between two treatments (p < 0.05).
The PCA Profiles and Aroma Series Mode
The above data indicated that cofermentation of S. cerevisiae with M. pulcherrima or H. vineae could generate diversified volatile profiles of wines. To highlight the differences of fermentation by different strains and inoculation methods and to identify the volatile compounds that discriminate these treatments, PCA was applied using twenty-five aromatic compounds where OAV exceeded 0.1. As shown in Figure 2A, the first and second accounted for 38.3% (PC1) and 21.8% (PC2) of the total variation, respectively. The PCs roughly distinguished wine samples fermented by different inoculations. Wines produced by HV11/SC45, especially sequential inoculation, were clearly separated by PC1 from the other wines, suggesting that HV11/SC45 had a higher potential to generate distinct aromatic profile than that of MP20/SC45. The main responsible components for this separation were β-citronellol, β-damascenone, linalool, cis-rose oxide, phenylacetaldehyde, 2-phenylethanol, 2-phenethyl acetate, (Z)-3-hexen-1-ol, and 1-octen-3-ol (Figure 2B). The PC2 could discriminate the wine of SC45 from other wines of monocultures mainly by ethyl octanoate. These data indicated that the complexity of the aromatic property of S. cerevisiae can be further increased by cofermentation with H. vineae. In comparison, MP20/SC45 had less ability to diversify the aromatic profiles although the wine made by SI MP20/SC45 was separated from SC45 wine by producing more ethyl butanoate.
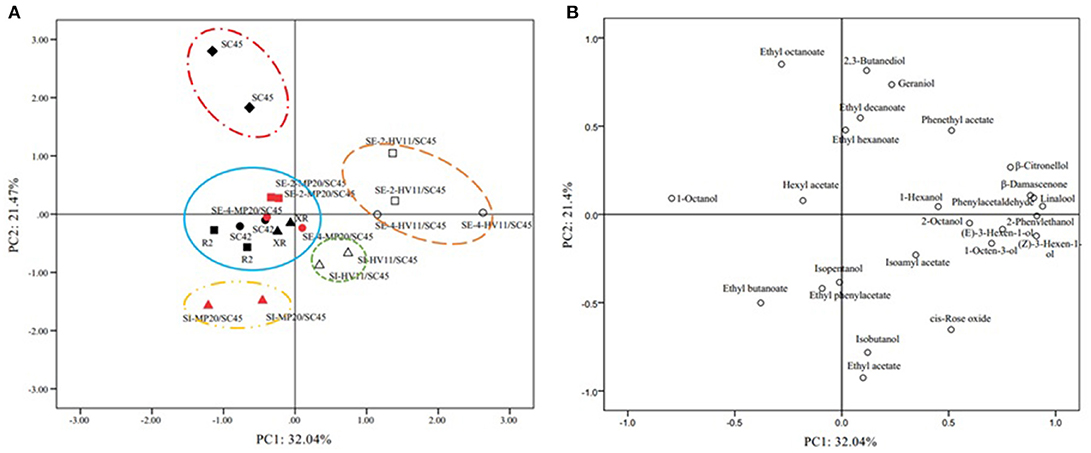
Figure 2. Principal Component Analysis (PCA) biplots of icewine products resulting from twenty-five odor active compounds (OVA > 0.1) produced by ten treatments used different strains and inoculation methods in 50 L fermenters. Score plot (A) and loading plot (B). The data are from duplicate biological repeats with three technical replicates each.
To better understand the influence of cofermentations with selected non-Saccharomyces strains on the icewine odor profile and the contributions of various aroma compounds to the olfactory impression of icewine, an aromatic series was further established by the combination of OAVs of a group of volatile active compounds with similar odor descriptions (Peinado et al., 2006; Wu et al., 2011). In this research, twenty-five aroma compounds showed an OAV above 0.1 (Table 2). According to previous researches (Buettner et al., 2003; Peinado et al., 2006; Yang et al., 2008; Zhang et al., 2011), these compounds mainly associated with the aroma attributes of “alcohol,” “solvent,” “green,” “bitter,” “fatty,” “solvent,” “nail polish,” “rancid,” “mushroom,” “rose,” “honey,” “fruity,” “butter,” “sweet,” “jasmine,” “lemon,” “pineapple,” “balsamic,” “banana,” “strawberry,” “pear,” “cherry,” “geranium,” and “lychee” (Table S2). Six aromatic series of volatile compounds were, therefore, established, including fruity, floral, sweet, chemical, fatty, and herbaceous (Figure 3). Of these, the fruity, floral, and sweet series were prominent, followed by the chemical, fatty, and herbaceous series. Wines of SC45 monoculture had much higher OAVs of fruity, floral, and sweety among S. cerevisiae strains due to production of higher amounts of ethyl octanoate, ethyl decanoate, β-damascenone, and phenylacetaldehyde. In comparison, chemical, fatty, and herbaceous aroma series were equal in monoculture wines. As regarding to mixed fermentation, MP20/SC45 produced relatively higher values of fruity, floral, and sweety aroma series than those of XR, R2, and SC42, but was inferior to SC45 monoculture. This suggested that combination of MP20 and SC45 might be not an effective pair to enhance the aromatic quality of icewine. Conversely, sequential inoculation of SC45 and HV11 significantly increased the features of fruity, floral, and sweety compared to SC45 monoculture, especially SE-2-HV11/SC45, where the values of fruity, floral, sweety series were 15.3, 22.5, and 22.6% higher than those of SC45 monoculture. The increments of higher alcohols (isobutanol, isopentanol, and 2-phenylethanol), acetate esters (2-phenethyl acetate and isoamyl acetate), cis-rose oxide, β-damascenone, and phenylacetaldehyde were mainly responsible for these improvements.
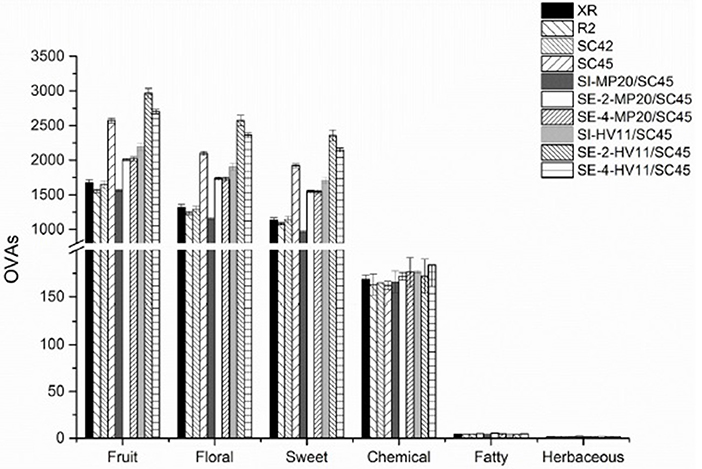
Figure 3. Aroma series in final icewines produced by four S.cerevisiae strains single fermentation and the mixed fermentations of MP20/SC45 and HV11/SC45, respectively. Error bars represent standard deviations. Aroma compounds (OVA > 1) are calculated for fruit series: ethyl acetate, ethyl butanoate, ethyl hexanoate, ethyl octanote, ethyl decanoate, 2-phenethyl acetate, isoamyl acetate, and β-damascenone; for floral series: 2-phenethyl acetate, ethyl octanoate, 2-phenylethanol, phenylacetaldehyde, β-damascenone, linalool, geraniol and cis-rose oxide; for sweet series; ethyl octanoate, 2-phenylethanol, phenylacetaldehyde, linalool, and β-damascenone; for chemical series: ethyl acetate, isobutanol, isopentanol, and 1-octen-3-ol; for fatty series: ethyl decanoate and isopentanol; for herbaceous series: isobutanol.
Discussion
Discovery of Saccharomyces and non-Saccharomyces strains with suitable inoculation methods is an effective method to produce wine products with desired and diversified aroma characteristics as expected by consumers. The aim of this study was to evaluate whether the multiculture of indigenous M. pulcherrima MP20 or H. vineae HV11 strains with S. cerevisiae could also produce Vidal icewine with diversified aroma quality, respectively. The obtained results showed that S. cerevisiae strains, paired strains of non-Saccharomyces, and inoculated methods had distinct effects on the formation of aromatic compounds in final icewines.
The contribution of wine yeast to the final aromatic characteristics of wine is largely dependent on the persistence of strains and cell number during alcoholic fermentation. At present, there are few wine yeast strains available for icewine fermentation. The non-Saccharomyces strains MP20 and HV11 used in this work were isolated from spontaneous fermentation of Vidal icewine. The preliminary experimental results showed that MP20 and HV11 strains have good technological characteristics, including high tolerance to ethanol, SO2, and higher sugar, low production of H2S, and high production of protease and glucosidase (Table S1). The results of fermentation trials also showed that both non-Saccharomyces strains can well multiply and persist in initial stages of icewine fermentation, although they were gradually dominated by S. cerevisiae. These data well verified the feasibility of selecting suitable non-Saccharomyces strains from spontaneous fermentation for wine production (Regodón et al., 1997; De Benedictis et al., 2011). Actually, the strategy of imparting a particular stress factor in spontaneous wine fermentation for the purpose of isolating yeast strains with desired physiological characteristics has been successfully applied by different researchers. Stress factors include high tolerance to low temperature (Massera et al., 2012), reducing copper content in wine (Capece et al., 2017), and fermenting very high-gravity worts (Huuskonen et al., 2010). Our data showed that HV11 had better growth profile than that of MP20 and a higher growth rate and cell number were observed during fermentation, which was consistent with previous results that H. vineae has a higher ability to adapt to harsh conditions of wine fermentation compared to other non-Saccharomyces species (Viana et al., 2011). There is one concern wherein the initial growth of apiculate yeast may be inhibitory for subsequent S. cerevisiae growth and result in sluggish fermentations (Medina et al., 2013). The inhibition on the growth of S. cerevisiae (SC45) by inoculating HV11was not observed in this study, implying that HV11 has great potential in industrial icewine production.
Icewine juice is a concentrated mix of all juice components such as sugars and acids. To combat the osmotic stress imposed by the icewine juice, yeast cells may allocate carbon resources derived from sugar metabolism toward the production of metabolites necessary for adaptation and survival such as acetic acid and glycerol (Heit et al., 2018). The upper allowable limit of acetic acid is 2.1 g/L in Canadian (Pigeau and Inglis, 2005) and Chinese (GB/T25504-2010, 20102) icewines. The amounts of acetic acid present in all samples were below this value (range in 1.41 to 1.71 g/L). Glycerol is one important product of yeast fermentation and is typically found at concentrations of 4-10 g/L in dry wine. In general, higher glycerol levels are considered to improve wine quality. In this study, the highest content of glycerol (10.28 g/L) was produced in SC45 wine, which was not consistent with the previous literature wherein sequential inoculation of H. vineae and S. cerevisiae could result in significant increase of glycerol content in Chardonnay wine (Medina et al., 2013). M. pulcherrima is one commercial non-Saccharomyces and usually used to reduce wine alcohol. The lowest and highest levels of alcohol were achieved in SE-2-MP20/SC45 and SI-MP20/SC45, respectively, which well confirmed that the inoculation method is essential for cofermentation of M. pulcherrima and S. cerevisiae to reduce ethanol content of wine (Varela et al., 2016).
Consistent with previous literature (Erasmus et al., 2004; Crandles et al., 2015), we found that different inoculated S. cerevisiae strains produced distinct aromatic profiles of icewine products. Compared to R2, the commercial XR strain produced the highest levels of higher alcohols and acetate esters, revealing its good enological properties in icewine production. Esters (acetate esters and fatty acid ethyl esters) are the major volatile constituents in wines (Sumby et al., 2010). Ethyl hexanoate, ethyl isobutanoate, ethyl 2-methyl-butanoate, ethyl isovalerate, and ethyl butanoate are major esters in Vidal icewine and provide desired fruit flavor to icewine (Ma et al., 2017). Indigenous yeast SC45 formed higher amounts of ethyl esters (ethyl octanoate, ethyl hexanoate, and ethyl decanoate). As regarding to mixed fermentation, HV11/SC45 further improved the production of volatiles relative to SC45 monoculture, but this production was largely dependent on inoculated method. SI-HV11/SC45 produced the highest concentration of C6 alcohols and higher alcohols, while SE-2-HV11/SC45 and SE-4-HV11/SC45 generated more 2-phenylethanol, acetate esters (2-phenethyl acetate), cis-rose oxide, β-damascenone, and phenylacetaldehyde. These results were consistent with previous literatures wherein there was the promotion of H. vineae and S. cerevisiae for 2-phenylethyl acetate formation (Viana et al., 2011; Medina et al., 2013). Compared to H. vineae, MP20/SC45 has less ability to reshape the aromatic profile of icewine. Inoculation of M. pulcherrima could improve ethyl acetate, 2-methyl propanol, and 2, 3-methyl butanol in table wine (Varela et al., 2017). These desired enological traits were not observed in this work. The inconsistent data could be due to the differences of strains and (or) composition of grape must. Thus, it is essential to comprehensively evaluate the aromatic property of one particular strain before using it to produce different types of wines.
It should be noticed that HV11/SC45 and MP20/SC45 led to decreased production of fatty acid ethyl esters compared to the monoculture of SC45, which corresponded to the previous data that H. vineae produced lesser amounts of ethyl esters including ethyl butyrate, ethyl hexanoate, and ethyl octanoate than that of S. cerevisiae (QA23) (Lleixà et al., 2016). At present, we are not able to explain these phenomena well enough. Considering that the SC45 is the strongest strain to produce fatty acid ethyl esters, we assumed that initial inoculation of non-Saccharomyces strains (M. pulcherrima and H. vineae) with lower ability to form ethyl esters negatively influenced Saccharomyces growth and formation of ethyl esters by SC45. This assumption could be partially supported by the observation that the later inoculation of SC45 induced greater decrease of ethyl esters. The detailed mechanisms need to be further explored at metabolites and transcriptional levels.
Hanseniaspora species are usually regarded as spoilage yeast in wine fermentation. Using H. vineae as the starter has recently received great interest because it produces several key aromatic compounds and increases flavor diversity (Lleixà et al., 2016). However, sensory evaluation of wines produced by this apiculate yeast in laboratory studies is still limited and the results have not been consistent. For example, five and ten folds higher levels of 2-phenylethyl acetate ester were produced in wine of H. vineae cofermentation than conventional and spontaneous fermentations, respectively, but 2-phenylethanol was significantly lower than those of the other two treatments (Medina et al., 2013). High production of 2-phenylethyl acetate by H. vineae was also confirmed previously in laboratory fermentations (Viana et al., 2009, 2011). Our results were in agreement with the previous literature. In addition to 2-phenylethyl acetate, other desired compounds such as 2-phenylethanol, β-damascenone, isoamyl acetate, and phenylacetaldehyde were found simultaneously increased by HV11/SC45. Recently, Huang et al. (2018) compared the flavor characteristics of Vidal icewines from China and Canada and established relationships between their sensory descriptors and chemical composition. Their results indicated that Vidal icewines produced in Huairen were mainly characterized by isoamyl acetate, 2-phenylethyl acetate, and 2-phenylethanol, and expressed nut and honey aromas. HV11 used in this study was isolated from the Huairen region. We, therefore, inferred that H. vineae is a unique non-Saccharomyces species in Huairen and imparts the Vidal icewine with typical sensory characteristics. This deduction deserves to be further investigated. We also found that the formations of volatiles by multicultures were largely affected by the timing of inoculation of S. cerevisiae strain. The later inoculation of S. cerevisiae is favorable for most volatile formations, which was more pronounced in sequential fermentation of HV11 and SC45.
In this work, we established the aromatic series to evaluate the effects of multicultures on aromatic quality of icewine. This method is often used to determine the contribution of different aroma compounds to olfactory impression of wine and achieve the information of volatile variation on aroma qualities of wines (Peinado et al., 2006; Wu et al., 2011). The results showed that SE-2-HV11/SC45 obtained the highest values of fruity, floral, and sweety series among all treatments, which was corresponding to the high levels of 2-phenylethanol, 2-phenethyl acetate, isoamyl acetate, cis-rose oxide, β-damascenone, and phenylacetaldehyde produced in this wine. Considering the positive contribution of these volatiles to the aroma quality of the wine, the improvement of aromatic quality by HV11/SC45 can be expected. Certainly, to further confirm this conclusion, sensory evaluation needs to be conducted. In summary, the present data verified the positive enological characteristics of non-Saccharomyces strains (H. vineae and M. pulcherrima) in icewine fermentation. Especially, the sequential inoculation of H. vineae with S. cerevisiae has a significant impact on aromatic quality, and efficiently improved the aromatic diversity of icewine products. To further verify this conclusion, more work with HV11 strains needs to be done along with other grape varieties such as Riesling and also in larger scale fermenters. The relevant experiments will be done in our future work.
Conclusion
The present results indicated that multicultures of autochthonous non-Saccharomyces strains (M. pulcherrima MP20 and H. vineae HV11) with S. cerevisiae strain (SC45) can generate distinct aromatic profiles of icewine compared to S. cerevisiae monocultures. Compared to MP20, sequential inoculation of HV11 and SC45 efficiently increased the production of most of the desired volatiles associated with fruity, flowery, and sweety characteristics, enhancing the aromatic diversity of icewine products. Collectively, our data confirmed the positive enological properties of H. vineae in the production of icewine and also demonstrated that using indigenous non-Saccharomyces strains is a feasible way to improve aromatic diversity of icewine products as expected by consumers.
Author Contributions
C-QD and G-LY designed the experiments. J-YS and B-QZ conducted the experiments. B-QZ, J-YS, and G-LY analyzed the experimental data. G-LY and B-QZ wrote the paper.
Conflict of Interest Statement
The authors declare that the research was conducted in the absence of any commercial or financial relationships that could be construed as a potential conflict of interest.
Acknowledgments
This work was supported financially by the China Agriculture Research System (CARS-29), National Natural Science Foundation of China (31771965), and Chinese Universities Scientific Fund (2017SP003).
Supplementary Material
The Supplementary Material for this article can be found online at: https://www.frontiersin.org/articles/10.3389/fmicb.2018.02303/full#supplementary-material
Footnotes
1. ^Available online at: http://www.vqaontario.com/Regulations/Standards (Accessed October 1, 2014).
2. ^GB T25504-2010, Icewine. Beijing: China Standard Press.
References
Andorrà, I., Berradre, M., Mas, A., Esteve-Zarzoso, B., and Guillamón, J. M. (2012). Effect of mixed culture fermentations on yeast populations and aroma profile. LWT-Food Sci. Technol. 49, 8–13. doi: 10.1016/j.lwt.2012.04.008
Andorrà, I., Landi, S., Mas, A., Esteve-Zarzoso, B., and Guillamón, J. M. (2010). Effect of fermentation temperature on microbial population evolution using culture-independent and dependent techniques. Food Res. Int. 43, 773–779. doi: 10.1016/j.foodres.2009.11.014
Anfang, N., Brajkovich, M., and Goddard, M. R. (2010). Co-fermentation with Pichia kluyveri increases varietal thiol concentrations in Sauvignon Blanc. Aust. J. Grape Wine Res. 15, 1–8. doi: 10.1111/j.1755-0238.2008.00031.x
Bell, S. J., and Henschke, P. A. (2010). Implications of nitrogen nutrition for grapes, fermentation and wine. Aust. J. Grape Wine Res. 11, 242–295. doi: 10.1111/j.1755-0238.2005.tb00028.x
Bowen, A. J., and Reynolds, A. G. (2015). Aroma compounds in Ontario Vidal and Riesling icewines. II. Effects of crop level. Food Res. Int. 76, 550–560. doi: 10.1016/j.foodres.2015.06.042
Buettner, A., Mestres, M., Fischer, A., Guasch, J., and Schieberle, P. (2003). Evaluation of the most odour-active compounds in the peel oil of clementines (Citrus reticulata blanco cv. clementine). Eur. Food Res. Technol. 216, 11–14. doi: 10.1007/s00217-002-0586-y
Calabretti, A., La Cara, F., Sorrentino, A., Di Stasio, M., Santomauro, F., Rastrelli, L., et al. (2012). Characterization of volatile fraction of typical Irpinian wines fermented with a new starter yeast. World J. Microbiol. Biotechnol. 28, 1433–1442. doi: 10.1007/s11274-011-0943-8
Cañas, P. M. I., García, A. T. P., and Romero, E. G. (2015). Enhancement of flavour properties in wines using sequential inoculations of non-Saccharomyces (Hansenula and Torulaspora) and Saccharomyces yeast starter. Vitis 50, 177–182.
Capece, A., Romaniello, R., Scrano, L., Siesto, G., and Romano, P. (2017). Yeast starter as a biotechnological tool for reducing copper content in wine. Front. Microbiol. 8:2632. doi: 10.3389/fmicb.2017.02632
Chamberlain, G., Husnik, J., and Subden, R. E. (1997). Freeze-desiccation survival in wild yeasts in the bloom of icewine grapes. Food Res. Int. 30, 435–439. doi: 10.1016/S0963-9969(97)00070-7
Ciani, M., and Picciotti, G. (1995). The growth kinetics and fermentation behaviour of some non-Saccharomyces yeasts associated with wine-making. Biotechnol. Lett. 17, 1247–1250. doi: 10.1007/BF00128395
Crandles, M., Reynolds, A. G., Khairallah, R., and Bowen, A. (2015). The effect of yeast strain on odor active compounds in Riesling and Vidal blanc icewines. LWT-Food Sci. Technol. 64, 243–258. doi: 10.1016/j.lwt.2015.05.049
De Benedictis, M., Bleve, G., Grieco, F., Tristezza, M., Tufariello, M., and Grieco, F. (2011). An optimized procedure for the enological selection of non-Saccharomyces starter cultures. Anton. Leeuw. Int. J. G. 99, 189–200. doi: 10.1007/s10482-010-9475-8
Duan, L. L., Shi, Y., Jiang, R., Yang, Q., Wang, Y. Q., Liu, P. T., et al. (2015). Effects of adding unsaturated fatty acids on fatty acid composition of Saccharomyces cerevisiae and major volatile compounds in wine. S. Afr. J. Enol. Vitic. 36, 285–295.
Erasmus, D. J., Cliff, M., and Vuuren, H. (2004). Impact of yeast strain on the production of acetic acid, glycerol, and the sensory attributes of icewine. Am. J. Enol. Vitic. 55, 371–378.
Ferreira, V., Lopez, R., and Cacho, J. F. (2000). Quantitative determination of the odorants of young red wines from different grape varieties. J. Sci. Food Agric. 80, 1659–1667. doi: 10.1002/1097-0010(20000901)80:11<1659::AID-JSFA693>3.0.CO;2-6
Heit, C., Martin, S. J., Yang, F., and Inglis, D. L. (2018). Osmoadaptation of wine yeast (Saccharomyces cerevisiae) during icewine fermentation leads to high levels of acetic acid. J. Appl. Microbiol. 124, 1506–1520. doi: 10.1111/jam.13733
Huang, L., Ma, Y., Tian, X., Li, J. M., Li, L. X., Tang, K., et al. (2018). Chemosensory characteristics of regional Vidal icewines from China and Canada. Food Chem. 216, 66–74. doi: 10.1016/j.foodchem.2018.04.021
Huuskonen, A., Markkula, T., Vidgren, V., Lima, L., Mulder, L., Geurts, W., et al. (2010). Selection from industrial lager yeast strains of variants with improved fermentation performance in very-high-gravity worts. App. Environ. Microbiol. 76, 1563–1573. doi: 10.1128/AEM.03153-09
Jolly, N. P., Augustyn, O. P. R., and Pretorius, I. S. (2017a). The effect of non-Saccharomyces yeasts on fermentation and wine quality. S. Afr. J. Enol. Vitic. 24, 55–62.
Jolly, N. P., Augustyn, O. P. R., and Pretorius, I. S. (2017b). The use of Candida pulcherrima in combination with Saccharomyces cerevisiae for the production of Chenin blanc wine. S. Afr. J. Enol. Vitic. 24, 63–69. doi: 10.21548/24-2-2641
Jolly, N. P., Varela, C., and Pretorius, I. S. (2014). Not your ordinary yeast: non-Saccharomyces yeasts in wine production uncovered. FEMS Yeast Res. 14, 215–237. doi: 10.1111/1567-1364.12111
Khairallah, R., Reynolds, A. G., and Bowen, A. J. (2016). Harvest date effects on aroma compounds in aged Riesling icewines. J. Sci. Food Agric. 96, 4398–4409. doi: 10.1002/jsfa.7650
Kontkanen, D., Inglis, D. L., Pickering, G. J., and Reynolds, A. (2004). Effect of yeast inoculation rate, acclimatization, and nutrient addition on icewine fermentation. Am. J. Enol. Vitic. 55, 363–370.
Lan, Y. B., Qian, X., Yang, Z. J., Xiang, X. F., Yang, W. X., Liu, T., et al. (2016). Striking changes in volatile profiles at sub-zero temperatures during over-ripening of “Beibinghong” grapes in Northeastern China. Food Chem. 212, 172–182. doi: 10.1016/j.foodchem.2016.05.143
Liu, P. T., Lu, L., Duan, C. Q., and Yan, G. L. (2016). The contribution of indigenous non-Saccharomyces wine yeast to improved aromatic quality of Cabernet Sauvignon wines by spontaneous fermentation. LWT-Food Sci. Technol. 71, 356–363. doi: 10.1016/j.lwt.2016.04.031
Lleixà, J., Martín, V., Portillo Mdel, C., Carrau, F., Beltran, G., and Mas, A. (2016). Comparison of fermentation and wines produced by inoculation of Hanseniaspora vineae and Saccharomyces cerevisiae. Front. Microbiol. 7:338. doi: 10.3389/fmicb.2016.00338
Ma, Y., Tang, K., Xu, Y., and Li, J. M. (2017). Characterization of the key aroma compounds in Chinese Vidal icewine by gas chromatography-olfactometry, quantitative measurements, aroma recombination, and omission tests. J. Agric. Food Chem. 65, 394–401. doi: 10.1021/acs.jafc.6b04509
Massera, A., Assof, M., Sturm, M. E., Sari, S., Jofré, V., Cordero-Otero, R., et al. (2012). Selection of indigenous Saccharomyces cerevisiae strains to ferment red musts at low temperature. Ann. Microbiol. 62, 367–380. doi: 10.1007/s13213-011-0271-0
Medina, K., Boido, E., Fariña, L., Gioia, O., Gomez, M. E., Barquet, M., et al. (2013). Increased flavour diversity of Chardonnay wines by spontaneous fermentation and co-fermentation with Hanseniaspora vineae. Food Chem. 141, 2513–2521. doi: 10.1016/j.foodchem.2013.04.056
Mendes, B., Gonçalves, J., and Câmara, J. S. (2012). Effectiveness of high-throughput miniaturized sorbent-and solid phase microextraction techniques combined with gas chromatography–mass spectrometry analysis for a rapid screening of volatile and semi-volatile composition of wines-A comparative study. Talanta 88, 79–94. doi: 10.1016/j.talanta.2011.10.010
Peinado, R. A., Mauricio, J. C., and Moreno, J. (2006). Aromatic series in sherry wines with gluconic acid subjected to different biological aging conditions by Saccharomyces cerevisiae var. capensis. Food Chem. 94, 232–239. doi: 10.1016/j.foodchem.2004.11.010
Pigeau, G. M., and Inglis, D. L. (2005). Upregulation of ALD3 and GPD1 in Saccharomyces cerevisiae during Icewine fermentation. J. Appl. Microbiol. 99, 112–125. doi: 10.1111/j.1365-2672.2005.02577.x
Regodón, J. A., Pérez, F., Valdés, M. E., De Miguel, C., and Ramirez, M. (1997). A simple and effective procedure for selection of wine yeast strains. Food Microbiol. 14, 247–254. doi: 10.1006/fmic.1996.0091
Sumby, K. M., Grbin, P. R., and Jiranek, V. (2010). Microbial modulation of aromatic esters in wine: current knowledge and future prospects. Food Chem. 121, 1–16. doi: 10.1016/j.foodchem.2009.12.004
Swiegers, J. H., and Pretorius, I. S. (2005). Yeast modulation of wine flavor. Adv. Appl. Microbiol. 57, 131–175. doi: 10.1016/S0065-2164(05)57005-9
Synos, K., Reynolds, A. G., and Bowen, A. J. (2015). Effect of yeast strain on aroma compounds in Cabernet franc icewines. LWT-Food Sci. Technol. 64, 227–235. doi: 10.1016/j.lwt.2015.05.044
Tang, K., Li, J. M., Wang, B., Ma, L., and Xu, Y. (2013). Evaluation of nonvolatile flavor compounds in Vidal icewine from China. Am. J. Enol. Viticult. 64, 110–117. doi: 10.5344/ajev.2012.12037
Tofalo, R., Patrignani, F., Lanciotti, R., Perpetuini, G., Schirone, M., Di Gianvito, P., et al. (2016). Aroma profile of Montepulciano d'Abruzzo wine fermented by single and co-culture starters of autochthonous Saccharomyces and Non-saccharomyces yeasts. Front. Microbiol. 7:610. doi: 10.3389/fmicb.2016.00610
Varela, C., Barker, A., Tran, T., Borneman, A., and Curtin, C. (2017). Sensory profile and volatile aroma composition of reduced alcohol Merlot wines fermented with Metschnikowia pulcherrima and Saccharomyces uvarum. Int. J. Food Microbiol. 252, 1–9. doi: 10.1016/j.ijfoodmicro.2017.04.002
Varela, C., Sengler, F., Solomon, M., and Curtin, C. (2016). Volatile flavour profile of reduced alcohol wines fermented with the non-conventional yeast species Metschnikowia pulcherrima and Saccharomyces uvarum. Food Chem. 209, 57–64. doi: 10.1016/j.foodchem.2016.04.024
Velázquez, J. B., Longo, E., Sieiro, C., Cansado, J., Calo, P., and Villa, T. G. (1991). Improvement of the alcoholic fermentation of grape juice with mixed cultures of Saccharomyces cerevisiae wild strains. Negative effect of Kloeckera apiculata. World J. Microbiol. Biotechnol. 7, 485–489. doi: 10.1007/BF00303374
Viana, F., Belloch, C., Vallés, S., and Manzanares, P. (2011). Monitoring a mixed starter of Hanseniaspora vineae-Saccharomyces cerevisiae in natural must: impact on 2-phenylethyl acetate production. Int. J. Food Microbiol. 151, 235–240. doi: 10.1016/j.ijfoodmicro.2011.09.005
Viana, F., Gil, J. V., Vallés, S., and Manzanares, P. (2009). Increasing the levels of 2-phenylethyl acetate in wine through the use of a mixed culture of Hanseniaspora osmophila and Saccharomyces cerevisiae. Int. J. Food Microbiol. 135, 68–74. doi: 10.1016/j.ijfoodmicro.2009.07.025
Wu, Y., Zhu, B., Tu, C., Duan, C., and Pan, Q. (2011). Generation of volatile compounds in litchi wine during winemaking and short-term bottle storage. J. Agric. Food Chem. 59, 4923–4931. doi: 10.1021/jf2001876
Xu, X. Q., Cheng, G., Duan, L. L., Jiang, R., Pan, Q. H., Duan, C. Q., et al. (2015). Effect of training systems on fatty acids and their derived volatiles in Cabernet Sauvignon grapes and wines of the north foot of Mt. Tianshan. Food Chem. 181, 198–206. doi: 10.1016/j.foodchem.2015.02.082
Yang, D. S., Shewfelt, R. L., Lee, K. S., and Kays, S. J. (2008). Comparison of odor-active compounds from six distinctly different rice flavor types. J. Agric. Food Chem. 56, 2780–2787. doi: 10.1021/jf072685t
Keywords: Hanseniaspora vineae, Metschnikowia pulcherrima, Saccharomyces cerevisiae, vidal icewine, mixed fermentation, diversified aroma profile
Citation: Zhang B-Q, Shen J-Y, Duan C-Q and Yan G-L (2018) Use of Indigenous Hanseniaspora vineae and Metschnikowia pulcherrima Co-fermentation With Saccharomyces cerevisiae to Improve the Aroma Diversity of Vidal Blanc Icewine. Front. Microbiol. 9:2303. doi: 10.3389/fmicb.2018.02303
Received: 13 June 2018; Accepted: 10 September 2018;
Published: 22 October 2018.
Edited by:
Giovanna Suzzi, Università degli Studi di Teramo, ItalyReviewed by:
Lisa Granchi, Università degli Studi di Firenze, ItalyRosanna Tofalo, Università degli Studi di Teramo, Italy
Copyright © 2018 Zhang, Shen, Duan and Yan. This is an open-access article distributed under the terms of the Creative Commons Attribution License (CC BY). The use, distribution or reproduction in other forums is permitted, provided the original author(s) and the copyright owner(s) are credited and that the original publication in this journal is cited, in accordance with accepted academic practice. No use, distribution or reproduction is permitted which does not comply with these terms.
*Correspondence: Guo-Liang Yan, Z2x5YW5AY2F1LmVkdS5jbg==