- 1Research Center of Avian Disease, College of Veterinary Medicine, Sichuan Agricultural University, Chengdu, China
- 2Institute of Preventive Veterinary Medicine, Sichuan Agricultural University, Chengdu, China
- 3Key Laboratory of Animal Disease and Human Health of Sichuan Province, Chengdu, China
Apoptosis, an indispensable innate immune mechanism, regulates cellular homeostasis by removing unnecessary or damaged cells. It contains three signaling pathways: the mitochondria-mediated pathway, the death receptor pathway and the endoplasmic reticulum pathway. The importance of apoptosis in host defenses is stressed by the observation that multiple viruses have evolved various strategies to inhibit apoptosis, thereby blunting the host immune responses and promoting viral propagation. Porcine Circovirus type 2 (PCV2) utilizes various strategies to induce or inhibit programmed cell death. In this article, we review the latest research progress of the apoptosis mechanisms during infection with PCV2, including several proteins of PCV2 regulate apoptosis via interacting with host proteins and multiple signaling pathways involved in PCV2-induced apoptosis, which provides scientific basis for the pathogenesis and prevention of PCV2.
Introduction
Porcine circovirus (PCV) from the genus Circovirus within the family Circoviridae is an icosahedral, small, non-enveloped DNA virus with a circular, single negative-stranded genome of approximately 1.76 kb (Tischer et al., 1982; Fauquet et al., 1995; Wei et al., 2016; Wang et al., 2018). To date, three species of PCV have been confirmed: Porcine circovirus type 1 (PCV1), PCV2 and Porcine circovirus type 3 (PCV3) (Alarcon et al., 2013; Segalés et al., 2013). PCV1 was first discovered in 1974 and widely acknowledged to be non-pathogenic (Tischer et al., 1982). while PCV2 was the causative agent of PCVAD/PCVD, which include reproductive failure, porcine dermatitis and nephropathy syndrome, proliferative and necrotizing pneumonia and PCV2 systemic disease (PCV2-SD) (Allan et al., 1998, 1999; Ellis et al., 1998; Meehan et al., 1998; Opriessnig et al., 2007). The main immunopathological features of PCV2-SD are peripheral blood lymphopenia and T- and B-lymphocyte depletion in lymphoid tissue (Nielsen et al., 2003; Resendes et al., 2004; Resendes and Segalés, 2015; Richmond et al., 2015). What’s more, severely PCV2-infected pigs could damage immune system and trigger immunosuppression by replicating and inducing apoptosis in lymphocytes (Kiupel et al., 2005; Li et al., 2013; Bin et al., 2015), leading to poor immune response to vaccines and increased susceptibility to other infectious diseases. Hence, even though PCVAD is effectively controlled by commercial vaccines, vaccination does not eliminate infection (Fort et al., 2008; Opriessnig et al., 2008, 2010). PCV2 is also one of the most important viruses in all pig-raising areas and is increasingly considered as a serious threat to global pig industry (Segalés et al., 2005; Xiang-Jin, 2013; Salgado et al., 2014; Zhai et al., 2014; Xiao et al., 2015; Mao et al., 2017; Liu et al., 2018). Phan et al. (2016) first isolated PCV3 from piglets with clinical disease of weight loss, swollen joints and anorexia. In addition, the dermatitis and nephropathy syndrome has been recently associated to PCV3 (however, this is still under discussion).
Porcine circovirus has 11 potential ORFs, so far to date, four of them have been characterized as functional proteins in replicating PCV2, including ORF1 to ORF4 (Hamel et al., 1998; Lv et al., 2014a; Hong et al., 2015), while only three ORFs have been recognized for PCV1 and PCV3: ORF1 to ORF3 (Saraiva et al., 2018). The ORF1 encodes two replicases (Rep and Rep’), the Rep proteins of PCV-1 and PCV-2 are similar in size and are responsible for the replication of the circoviral genome (Mankertz, 2012). The capsid protein encoded by ORF2 is the sole structural protein of PCV2 and contains a highly conserved basic amino acid sequence (Timmusk et al., 2008; Latini et al., 2011); therefore, it contains the major antigenic determinants of the virus (Nawagitgul et al., 2000). However, the three proteins of PCV3 are less similar to those of PCV1 and PCV2 (Palinski et al., 2016; Phan et al., 2016). The proteins encoded by ORF3 and ORF4 genes are not required for viral replication, but are closely related to the spread and virulence of the virus (Lv et al., 2015a). The protein encoded by ORF3 gene plays a vital role in the pathogenesis of the virus through its apoptotic activity in vitro and in vivo (Liu et al., 2005, 2006; Lin et al., 2011). The ORF4 protein is capable of blocking PCV2-induced apoptosis by bringing down caspases activities (Gao et al., 2014a; Lv et al., 2015b). Besides this, a novel ORF5 protein has recently been discovered in PCV2-infected cells and may be involve in activation of NF-κB pathway (Lv et al., 2015a).
Apoptosis, also called programmed cell death, is an indispensable defense mechanism for host resistance to pathogens invasion (Jorgensen et al., 2017). Apoptosis is strictly regulated and can be triggered by multiple stimuli such as normal development, pathogen infection and several factors leading to disruption of cellular functions (Tait and Green, 2010; Czabotar et al., 2014). Apoptotic cells exhibit characteristic morphological abnormalities including chromatin condensation, nuclear fragmentation, membrane blebbing, and apoptotic body formation (Kroemer et al., 2005; Galluzzi et al., 2007). Apoptosis classically occurs via the intrinsic pathway (also called the mitochondrial pathway), the extrinsic pathway (also called the death receptor pathway) and the ER pathway (Hong et al., 2015). In brief, the mitochondrial pathway is induced by a variety of intracellular signals, such as hypoxia, nutrient deprivation and oxidative stress, which cause MOMP (Kroemer et al., 2006). Subsequently, AIF, cyt c and Smac/DIABLO are released from the mitochondrial membrane to the cytoplasm (Kroemer et al., 2006; Galluzzi et al., 2012). Cyt c can recruit pro-caspase9 and apoptotic protease activating factor-1 (apaf-1) to form an apoptosome, which subsequently activates downstream executioner caspases to trigger apoptosis (Li et al., 1997; Acehan et al., 2002). In addition, the mitochondrial pathway is mainly regulated by Bcl-2 (B-cell lymphoma 2) family proteins, which are classified into three types (Cory and Adams, 2002). One is an anti-apoptotic sub-family, which includes Bcl-xL (B-cell lymphoma-extra large) and Bcl-2. Another is pro-apoptotic BH3 only proteins, such as Bid (BH3 interacting-domain death agonist) and Bad (Bcl-2 associated death promoter), these proteins are antagonists to the anti-apoptotic sub-family proteins. The third sub-family includes Bak (Bcl-2 homologous antagonist killer) and Bax (Bcl-2 associated x protein). On the other hand, the death receptor pathway is activated by the binding of a specific ligand to the corresponding death receptor, resulting in activation of caspase8 and caspase3, which finally leads to cleavage of cellular DNA (Lamkanfi et al., 2007; Tummers and Green, 2017). What’s more, ER stress regulates the concentration of Ca2+ and initiates the IRE1, PERK, and ATF6 pathways, which are associated with the mitochondrial pathway of apoptosis (Shore et al., 2011; Verma and Datta, 2012).
Porcine Circovirus type 2 infection induces apoptosis both in vitro and in vivo (Chang et al., 2007a; Seeliger et al., 2007; Galindocardiel et al., 2011; Resendes et al., 2011; Sinha et al., 2012), it has been reported that PCV2 can induce B lymphocyte deletion through apoptosis and macrophage apoptosis can be detected in the spleen of PCV2 infected mice (Shibahara et al., 2000), so apoptotic cell death may be one of the causes of lymphopenia after PCV2 infection (Resendes et al., 2004). Similarly, apoptosis is one of the causes of lymph node loss and hepatocyte decline in pigs with PMWS (Krakowka et al., 2004). Apoptosis has also been proposed as a natural part of the viral life cycle (Young et al., 2007), in the early stage of PCV2 infection, PCV2 may prevent apoptosis by expressing its anti-apoptotic gene to accomplish its propagation, while apoptosis may be a powerful strategy for the release and dissemination of progeny virions in the late stage (Liu et al., 2005). However, the molecular mechanism of PCV2-regulated apoptosis is still unclear. In this article, we focus on reviewing the roles of PCV2 in the process of apoptosis, which is useful for future research.
Viral Proteins and Their Apoptosis Regulation Mechanisms
Cap and Its Mechanism of Apoptosis Regulation
The Interactions Between Cap and Cellular Proteins
The ORF2 36 gene encodes the major immunogenic capsid protein of 27.8 kDa. By investigating the replication and pathogenesis mechanisms of PCV2, the interactions between the PCV2 Cap protein with nine different cellular proteins were confirmed (Table 1), including complement factor C1qB, the receptor protein for the gC1qR, MKRN1, cell adhesion molecule P-selectin, prostate apoptosis response-4 (Par-4) protein, NAP1, NPM1, Hsp70 and Hsp40 (Timmusk et al., 2006a; Finsterbusch et al., 2009b; Liu et al., 2013b). However, only MKRN1 and Hsp70 have been confirmed to participate in PCV2-induced apoptosis.
Apoptosis Regulated by Cap and MKRN1
According to Gray et al. (2000) and Lee et al. (2013), MKRN1 is a transcriptional co-regulator and an E3 ubiquitin ligase that is highly evolutionarily conserved in vertebrates, it can also mediate apoptosis and p53-dependent cell cycle arrest. The interactions between different types of PCVs and their hosts have been analyzed by Finsterbusch’s group, the research demonstrated that MKRN1 can interact with Cap proteins of both PCV1 and PCV2, resulting a decreased concentration of MKRN1 in the host (Finsterbusch et al., 2009a). The decreased MKRN1 can in turn reduce the level of p53 ubiquitination, resulting in an increase of p53 and thus promote cellular apoptosis. Under normal conditions, p53 and p21 both are suppressed by MKRN1 through ubiquitin-dependent degradation (Lee et al., 2009). Previous studies have showed that p21 is capable of activating cell cycle arrest via suppressing apoptosis (Gartel and Tyner, 2002; Javelaud and Besancon, 2002; Abbas and Dutta, 2009; Jung et al., 2010). Therefore, ubiquitination and degradation of p21 mediated by MKRN1 may also contribute to trigger apoptosis. However, under stresses such as DNA damage, only p53 is suppressed by MKRN1; p21, which is not ubiquitinated by MKRN1 can also inhibit p53. Therefore, when the suppression of p53 by MKRN1 and p21 is reduced, the concentration of p53 will increase and thus promotes the apoptotic process (Lee et al., 2009).
Apoptosis Regulated by Cap and Hsp70
Hsp70 is a chaperone whose expression is induced by a variety of stimuli. A previous study confirmed that Hsp70 could inhibit the production of apoptosome and apoptosis in varying degrees by suppressing the activity of AIF (Garrido et al., 2006). In study of PMWS pathogenesis using proteomics strategies, Ramírez-Boo et al. (2011) reported that the down-regulation of Hsp70 was detected in inguinal lymph nodes of piglets after inoculation with PCV2. Another study regarding the interaction between PCV2 and target immune cells showed the expression of Hsp70 was up-regulated in PAMs during the initial stage of PCV2 infection (Liu et al., 2013a). A recent study showed that the PCV2 Cap protein can interact with Hsp70 and activate it further, then apoptosis could be inhibited via blockage the activation of caspase-3 in 3D4/31 cells (Liu et al., 2013b). However, the interaction between the PCV2 Cap protein and Hsp70 may reduce Hsp70 levels, and activate caspase-3 which plays a key role in the execution of apoptosis. Accordingly, Hsp70 might play different roles on different stages of PCV2 infection (Figure 1). For example, Hsp70 can be induced by PCV2 infection, but lack of sufficient Cap protein in the early stage of viral infection could result in inhibiting apoptosis by Hsp70. In contrast, during the later stages of PCV2 infection, the anti-apoptotic responses may be weakened by the down-regulation of Hsp70 levels due to the increased interaction with the PCV2 Cap protein.
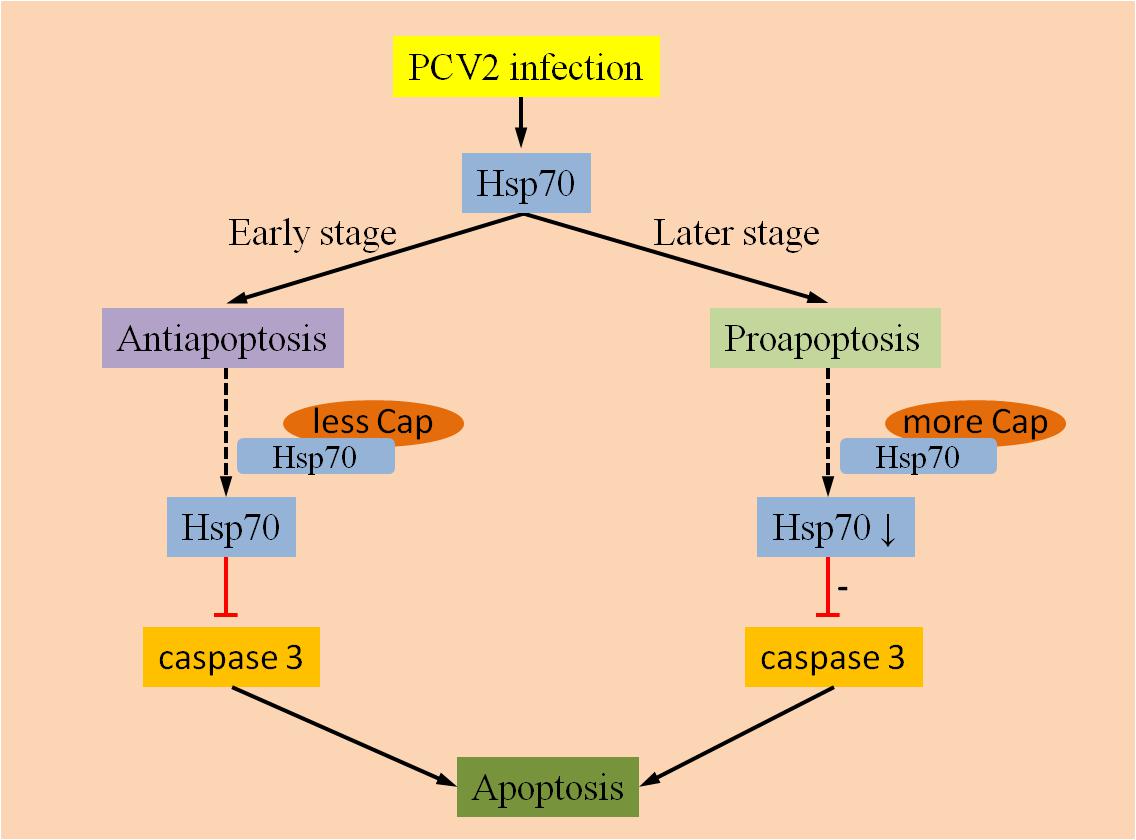
FIGURE 1. Anti-apoptotic and pro-apoptotic activities of Hsp70. Hsp70 can be activated by PCV2 infection, whereas Hsp70 mainly exert an anti-apoptotic function at an earlier stage of infection due to few interactions with PCV2 Cap proteins. During later stages of infection, anti-apoptotic responses might be weakened by down-regulation of Hsp70 due to the increased interaction with the PCV2 Cap protein.
ORF3 and Its Mechanisms of Apoptosis Regulation
Although the PCV2 non-structural protein ORF3 is not critical for viral replication Lin et al. (2013), found that the nuclear localization of ORF3 is correlated with triggering apoptotic response in porcine PBMC, it was also involved in PCV2-induced the extrinsic apoptosis pathway through activation of the caspase8 and caspase3 (Kiupel et al., 2005; Liu et al., 2005).
During a study of modulation of cellular functions by the PCV2 ORF3 protein, the ORF3 protein was found to directly interacted with pPirh2 (also called RCHY1), Pirh2 is an E3 ubiquitin ligase targeting p53 and leading p53 to degradation. The interaction between the pPirh2 and ORF3 protein could suppress pPirh2 stabilization and increase p53 cellular levels, thereby leading to apoptosis (Leng et al., 2003; Liu et al., 2007). Furthermore, present research suggests the amino acid residues of ORF3 protein are indispensable to compete with the interaction with pPirh2 over p53 (Timmusk et al., 2008; Karuppannan et al., 2010). p53 is a tumor suppressor as well as a transcription factor (Levine and Oren, 2009); it is also involved in regulation of diverse biological responses such as apoptosis, DNA damage, cell cycle arrest, oncogenic activities, erosion of telomeres, hypoxia and other physiological processes (Vousden and Prives, 2009; Collavin et al., 2010; Chang et al., 2013). It was reported that p53 was involved in apoptosis through transcription-dependent or -independent mechanisms during stress (Li et al., 2011; Xu et al., 2016). In general, the p53 protein content in cells is maintained at a very low level in the absence of stress through binding to proteins such as MDM2 (denoted HDM2 in humans), COP1, pPirh2 and JNK, which facilitates the degradation of p53 by the ubiquitin/proteasome pathway (Table 2). In stress situations such as cell cycle arrest, apoptosis may be caused by a complex formed by p53 with pro-apoptotic and anti-apoptotic members of the Bcl-2 family (such as Bcl-2, Bcl-xL, Bak and Bax). Then, the complex triggers MOMP, liberating essential apoptotic factors (such as Cyt c, AIF, and Apaf-1) and ultimately causing a caspase cascade and apoptosis via the intrinsic pathway (Marchenko et al., 2007; Wolff et al., 2008; Green and Kroemer, 2009; Collavin et al., 2010).
Based on these works, induction of apoptosis during PCV2 infection is a complex process that may involve cross-talk between the intrinsic and the extrinsic apoptotic pathway. Certainly, the mechanistic role of PCV2 ORF3 protein in the regulation of apoptosis should be investigated in more detail in future studies.
To date, more than twenty proteins have been shown to be associated with pPirh2 (Jung et al., 2012). Additionally, p53 is a highly connected protein that could form physical complexes with a variety of cellular proteins (Collavin et al., 2010); currently, more than 320 reported interactions with human p53 are included in the APID web interface1, including kinases, phosphatases, acetyltransferases, de-acetylases, ubiquitin ligases, and other proteins. Accordingly, future investigation should consider whether there are other factors regulate PCV2-induced apoptosis by participating in the interactions of pPirh2 and ORF3.
ORF4 and Its Mechanisms of Apoptosis Regulation
Studies indicated that the ORF4 protein is not required for PCV2 replication in mice or in PK-15 cells, while present research showed it plays a vital role in inhibiting apoptosis after PCV2 infection (He et al., 2013). Subsequently, Gao et al. (2014a) constructed two mutants of PCV2 ORF4: M1-PCV2 and M2-PCV2. By comparing the ORF3 mRNA levels of the wild-type and ORF4-deficient viruses in PK-15 cell, it was found that the ORF3 transcription levels of both ORF4 mutants were enhanced, indicating that the ORF4 protein may play an important role in preventing PCV2-induced apoptosis via inhibiting ORF3 transcription. Significant increases in caspase-8 and caspase-3 activities in both ORF4 mutants compared to wild-type PCV2 further confirmed this (Gao et al., 2014a). Subsequently Lv et al. (2016), revealed a mechanism by which ORF4 exerts cytoprotective function by resisting apoptosis in the early stage of PCV2 infection. Lv et al. (2015b) demonstrated the physical interaction between PCV2 ORF4 protein and FHC for the first time, and found that the decreased concentration of FHC can effectively inhibit the accumulation of reactive oxygen species in PCV2-infected cells, thereby inhibiting apoptosis. Recently, Lin et al. (2018) found that ORF4 is a mitochondrial targeting protein that ultimately induces apoptosis via the mitochondrial pathway by interacting with adenine nucleotide translocase 3 (ANT3).
In summary, it is very significant to study how the apoptotic processes are regulated by the proteins of PCV2 to promote its infection (Figure 2). In addition to the factors mentioned above, there are other reported mechanisms that could regulate PCV2-induced apoptosis, including different pathways (PERK/eIF2α, PI3K/Akt, and Fas/FasL), regulation of free Ca2+ concentration and NF-κB activation. In the following sections, we will briefly review these factors.
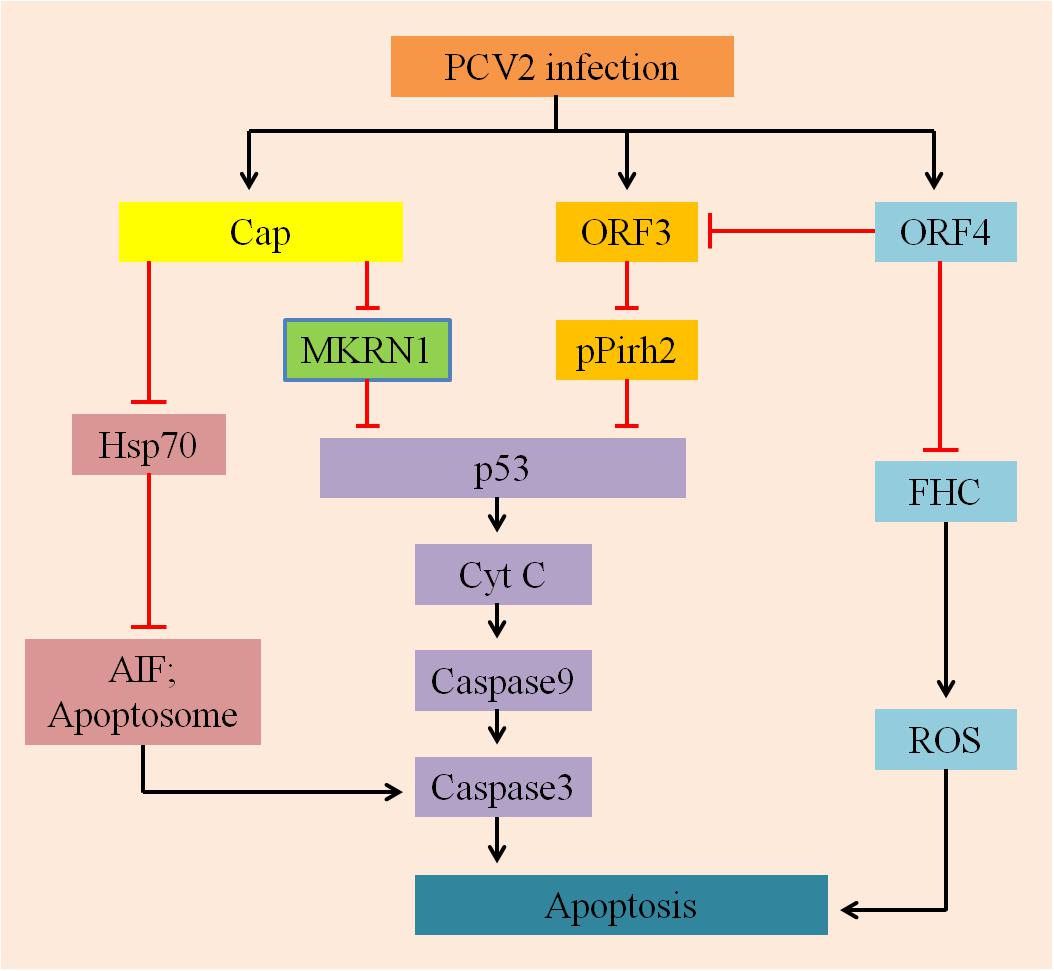
FIGURE 2. A hypothetical model describes the mechanisms involved in PCV2-ralated proteins induced apoptosis. On the one hand, Cap protein expressed by PCV2, which subsequently inhibits p53 and its downstream pro-apoptotic factors CytC, capases9, and caspase3 via MARNI pathway; it can also suppress Hsp70 and further inhibition the production of AIF and Apoptosome, depressing apoptosis. On the other hand, ORF3 and ORF4 proteins are largely involved in regulating apoptosis induced by PCV2: ORF3 protein interacts with pPirh2 to up-regulate the expression of p53 and its downstream factors to initiate apoptosis; whereas ORF4 protein inhibits apoptosis by suppressing activation of ORF3, it can also interact with FHC to reduce the content of FHC, inhibiting the production of ROS and ultimately suppression apoptosis.
PCV2-Induced Apoptosis Regulated by Different Pathways
PERK/eIF2α Pathway
Mounting evidence indicates that a wide variety of viruses could disturb ER homeostasis and lead to ER stress (Li et al., 2015). To cope with this stress, cells evolve a series of adaptive mechanisms called the UPR (Hetz, 2012). ER stress activates three branches of the UPR: PERK (Shen et al., 2005), IRE1 (Chen and Brandizzi, 2013), and ATF6 (Yan et al., 2002). Zhou et al. (2016) demonstrated that PCV2 initiated UPR by activating the PERK/eIF2α pathway instead of IRE1 or ATF6 pathways, ultimately promoting viral replication in PK-15 cells (Figure 3). Since PERK/eIF2α further activates downstream factors ATF4 and CHOP, so PCV2 infection can selectively activate apoptosis via the PERK-eIF2α-ATF4-CHOP axis. The findings provide a basis for demonstrating that ER stress of apoptotic responses plays an important role in the pathogenesis of PCV2 infection.
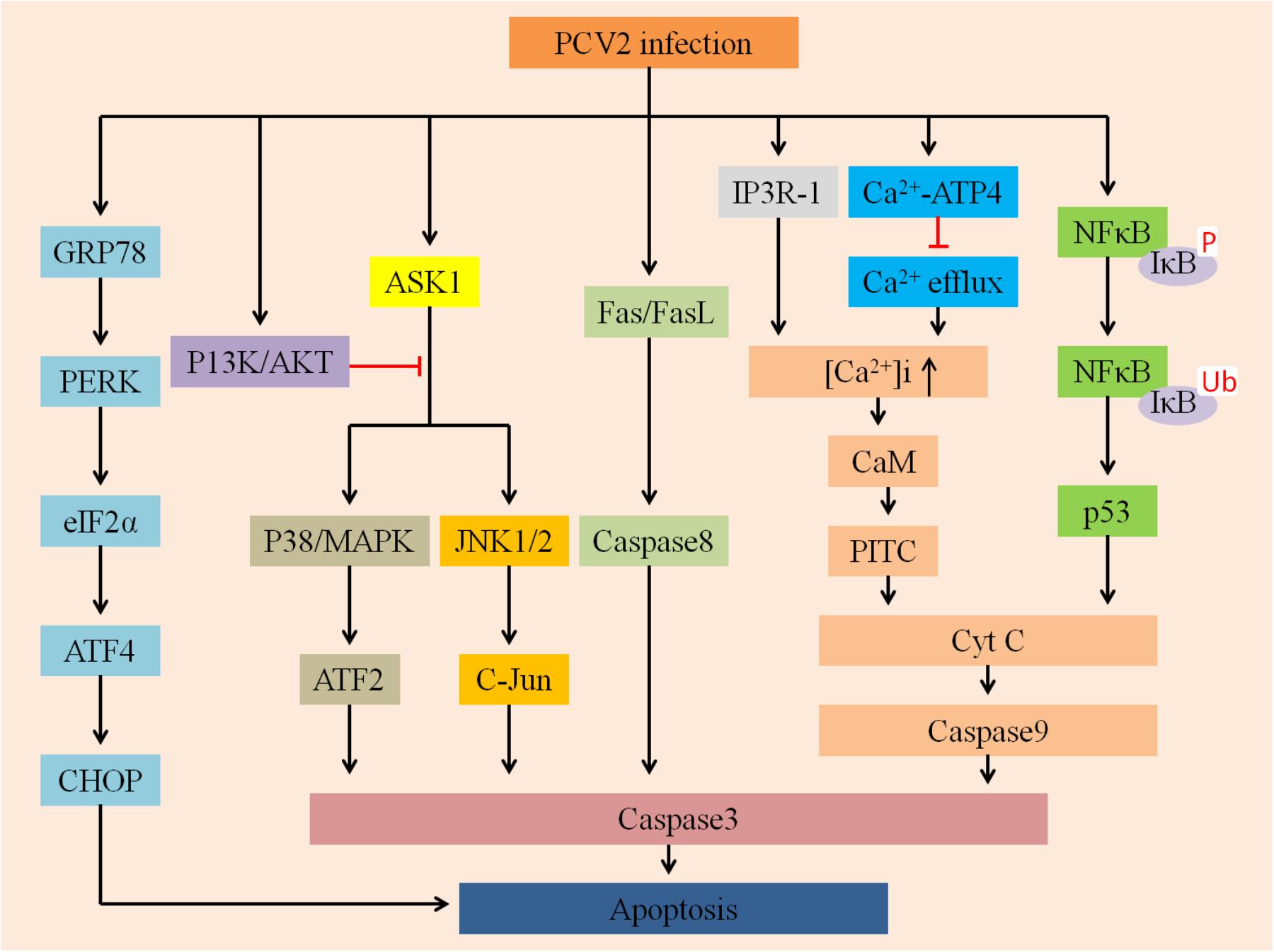
FIGURE 3. Summarizes multiply host cellular signaling pathways involved in regulating PCV2-induced apoptosis. First, PCV2 infection can activate PERK via PERK-eIF2α-ATF4-CHOP axis and then induce apoptosis, it can further activate JNK/p38 by activating the ASK1 pathway to ultimately promote apoptosis, whereas P13K/AKT plays an opposite role. Second, it can activate Cyt C and caspase-3 via the IP3R-1-Ca2+-PITC and NFκB-p53 pathways to activate apoptosis. In addition, PCV2 infection may activate caspase-8 via the Fas/FasL axis of the death receptor pathway to promote apoptosis.
PI3K/Akt and ASK1 Pathway
The phosphatidylinositol 3-kinase PI3K/Akt pathway plays a vital role in multiple physiological processes, such as inflammation suppression, carbohydrate metabolism, and cellular proliferation (Hsu et al., 2010). The PI3K/Akt pathway is also an indispensable target for a variety of viruses to inhibit apoptosis (Cooray, 2004; Shin et al., 2007; Soares et al., 2009). For example, PRRSV can trigger the PI3K/Akt pathway to augment viral replication and promote cell survival (Wang et al., 2014). Recently, Wei et al. (2012a) found that PCV2 can transiently activate the PI3K/Akt pathway, and the activated PI3K/Akt pathway could suppress premature apoptosis, thereby improving virus growth (Figure 3). However, in the early stage of PCV2 infection, inhibition of PI3K activation greatly enhanced apoptotic responses, mainly manifested by the cleavage of caspase3 and poly-ADP ribose polymerase as well as DNA fragmentation. The ASK1 plays a target role in the induction of apoptosis as an upstream enzyme that activates the p38/MARK and JNK pathways (Gan et al., 2016). During PCV2 infection, PI3K was activated first, followed by phosphorylation of Akt. Activated Akt inhibits the production of pro-apoptotic proteins such as JNK and p38/MAPK, thereby suppressing JNK- and p38-dependent apoptosis (Wei et al., 2013).
Interestingly, a previous study demonstrated that PCV2 infection regulates apoptosis by activating the p38/MAPK and JNK1/2 cellular stress pathways (Tibbles and Woodgett, 1999; Kyriakis and Avruch, 2001; Wada and Penninger, 2004; Wei et al., 2009). In the absence of stress, non-phosphorylated JNK bonds to p53, resulting in ubiquitination of p53 followed by proteasomal degradation (Fuchs et al., 1998a,b). In contrast, dissociation of p53 can be mediated by phosphorylated JNK, thus promoting p53 stabilization (Fuchs et al., 1998b). Additionally, p38/MAPK kinase plays a role not only in phosphorylation of p53 but also in transcription of p53-regulated Bax (Bulavin et al., 1999; Huang et al., 1999). Taken together, the activation and phosphorylation of p38/MARK and JNK after PCV2 infection might contribute to p53 stabilization, finally leading to apoptosis (Wei et al., 2009).
Fas/FasL Pathway
Chang et al. (2007a) evaluated and compared the effects of infection of both PCV2 and PRRSV, individually or together, on co-cultured splenic (SLs), peripheral blood (PBLs) lymphocytes and swine splenic macrophages (SMs) in vitro. The expression levels of Fas ligant (FasL) and Fas were significantly increased after PRRSV alone- and PCV2 and PRRSV dually inoculated groups, and the latter was more obvious, while increased Fas/FasL futher mediated apoptosis. Fas is also termed as CD95 (APO-1) and is one of the death receptors, these receptors include TNF-R1, CD95 (APO-1/Fas), DR3 (APO-3/TRAMP/Wsl-1/LARD), DR4 (TRAIL-R1), and so on. Han et al. (2010) confirmed that Fas could trigger apoptosis by binding to its cognate ligand, FasL. Thus, PCV2 infection may be associated with Fas/FasL-mediated apoptosis (Figure 3). However, the hypothesis of the mechanism is still poorly understood and need to be further demonstrated.
NF-κB Pathway
The transcription factor NF-κB is commonly activated during viral infection and is a key molecule that regulates a variety of cellular signal transduction pathways (Bonizzi and Karin, 2004; Hayden and Ghosh, 2004). For example, Dengue virus, Reovirus, infectious bursal disease virus, Hepatitis B virus and Sindbis virus have been confirmed to trigger apoptosis via activating NF-κB (Lin et al., 1998; Connolly et al., 2000; Jan et al., 2000; Liu and Vakharia, 2006; Pan et al., 2011; Chen et al., 2013). In these processes, NF-κB serves as a pro-apoptotic factor which is able to activate the p53 signaling pathway (Fujioka et al., 2004).
The present study found that after PCV2 infected cells, NF-κB was activated simultaneously with viral replication, which was characterized by translocation of NF-κB from the cytoplasm to the nucleus, degradation and phosphorylation of IκBα protein and increased DNA binding activity. However, treatment of cells with CAPE, a selective inhibitor of NF-κB activation, reduced progeny production and virus protein expression followed by decreasing caspase activity, indicating the importance of NF-κB in inducing apoptosis (Wei et al., 2008). However, the exact details still to be further demonstrated. According to the above discussions, there are many factors and multiply pathways participate in regulating apoptosis induced by PCV2 (Figure 3).
PCV2-Induced Apoptosis Regulated by Calcium
Calcium ions (Ca2+) are participated in multiple cellular physiological processes, such as cytoplasmic Ca2+ signaling, ATP production, hormone metabolism and apoptosis induction (Drago et al., 2011). The intracellular free Ca2+ ([Ca2+]i) can activate apoptosis by regulating numerous calcium-sensitive enzymes and can also activate the mitochondrial apoptotic pathway via its accumulation in the mitochondria (Hajnoczky et al., 2003; Pathak et al., 2013). Lv et al. (2012) found that PCV2 could lead to apoptosis of lymphocytes, this apoptotic mechanism is affected by the increased [Ca2+]i and is associated with the calmodulin (Lee et al., 2009) protein. Possible mechanisms of [Ca2+]i induction include the suppression of Ca2+ efflux by regulation of the Ca2+-ATPase transporter on cytomembranes, and/or the induction of Ca2+ influx by promoting Ca2+ release from the ER by increased expression of IP3R (Lv et al., 2012). IP3R can regulate the mobilization of Ca2+ (Berridge, 2005), Ca2+ released from the ER could activate the PTPC on mitochondria, causing Cyt c release and inducing apoptosis (Figure 3; Garrido et al., 2006).
Conclusion
Apoptosis is a very important host defense mechanism that contributes to remove infected, damaged and excess amounts of cells. The virus must evade host defense mechanisms to proliferate and spread. Infection with PCV2 has been demonstrated to trigger several signaling pathways such as PERK/eIF2α and PI3K/Akt pathway (Wei et al., 2012a; Zhou et al., 2016), resulting in activation or suppression of apoptosis. On the other hand, to cope with the apoptotic responses caused by viral infections, many viral proteins interact with apoptotic signals molecules to regulate apoptosis. There may be a discrepancy between induction and inhibition of apoptosis after PCV2 infection, as the experimental situation can be different, and close relationships between apoptosis and other factors that regulate cell fate, such as Ca2+, can make it more complicated and difficult.
This review is the first glimpse of PCV2 infection-induced apoptosis based on a wide array of reported works concerning PCV2 infection. It summarized currently findings which are involved in PCV2 infection-induced apoptosis, containing a vast panel of distinct pro-apoptotic and anti-apoptotic mechanisms (Figure 4). In the future, more attention should be taken on host-virus interaction. Further investigation that effect of different isoforms of PCV2, PCV1, and PCV3 on PCV-induced apoptosis should be done. Taking the above ideas into consideration will help us reach a deeper understanding of the molecular mechanisms of PCV2-induced apoptosis and open a new gate for further studies on the pathogenesis of PCV2.
Author Contributions
PL and RJ contributed ideas for the review. YP wrote the manuscript and produced the figures. RJ, MW, ZY, and AC edited and revised the manuscript.
Funding
This research was supported by the National Key Research and Development Program of China (2017YFD0500800), National Key R&D Program (2016YFD0500800), China Agricultural Research System (CARS-42-17), and Sichuan Province Research Programs (2017JY0014/2017HH0026).
Conflict of Interest Statement
The authors declare that the research was conducted in the absence of any commercial or financial relationships that could be construed as a potential conflict of interest.
Acknowledgments
We apologize to the authors of articles reporting relevant research that were not cited in this manuscript due to limited space.
Abbreviations
AIF, apoptosis-inducing factor; Apaf-1, apoptosis-protease activating factor-1; ASK1, apoptosis signal-regulating kinase 1; ATF6, activating transcription factor 6; [Ca2+]i, the intracellular free Ca2+ concentration; Cap, capsid; Cyt c, cytochrome c; ER, endoplasmic reticulum; FHC, ferritin heavy chain; gC1qR, globular heads of complement component C1q; Hsp40, heat-shock protein 40; Hsp70, heat-shock protein 70; IP3R, inositol 1,4,5-trisphosphate receptor; IRE1, inositol requiring enzyme 1; JNK, c-Jun NH2-terminal kinase; MDM2, murine double minute 2 gene; MKRN1, makorin-1 RING zinc-finger protein; MOMP, mitochondrial outer membrane permeabilization; NAP1, nucleosome assembly protein-1; NPM1, nucleophosmin-1; ORFs, open reading frames; p38/MARK, p38 mitogen-activated protein kinase; Par-4, prostate apoptosis response-4 protein; PAMs, pulmonary alveolar macrophages; PCV2, Porcine Circovirus type 2; PCVAD/PCVD, porcine circovirus-associated diseases; PERK, PKR-like ER kinase; PMWS, post-weaning multi systemic wasting syndrome; pPirh2, porcine Pirh2; PTPC, permeability transition pore complex; Rep, replicase; RGS, regulator of G protein signaling; UPR, unfolded protein response; WNV, West Nile virus.
Footnotes
References
Abbas, T., and Dutta, A. (2009). p21 in cancer: intricate networks and multiple activities. Nat. Rev. Cancer 9, 400–414. doi: 10.1038/nrc2657
Acehan, D., Jiang, X., Morgan, D. G., Heuser, J. E., Wang, X., and Akey, C. W. (2002). Three-dimensional structure of the apoptosome: implications for assembly, procaspase-9 binding, and activation. Mol. Cell 9, 423–432. doi: 10.1016/S1097-2765(02)00442-2
Alarcon, P., Rushton, J., and Wieland, B. (2013). Cost of post-weaning multi-systemic wasting syndrome and porcine circovirus type-2 subclinical infection in England - an economic disease model. Prev. Vet. Med. 110, 88–102. doi: 10.1016/j.prevetmed.2013.02.010
Allan, G. M., Mc Neilly, F., Meehan, B. M., Kennedy, S., Mackie, D. P., Ellis, J. A., et al. (1999). Isolation and characterisation of circoviruses from pigs with wasting syndromes in Spain, Denmark and Northern Ireland. Vet. Microbiol. 66, 115–123. doi: 10.1016/S0378-1135(99)00004-8
Allan, G. M., McNeilly, F., Kennedy, S., Daft, B., Clarke, E. G., Ellis, J. A., et al. (1998). Isolation of porcine circovirus-like viruses from pigs with a wasting disease in the USA and Europe. J. Vet. Diagn. Invest. 10, 3–10. doi: 10.1177/104063879801000102
Allton, K., Jain, A. K., Herz, H. M., Tsai, W. W., Jung, S. Y., Qin, J., et al. (2009). Trim24 targets endogenous p53 for degradation. Proc. Natl. Acad. Sci. U.S.A. 106, 11612–11616. doi: 10.1073/pnas.0813177106
Alsheich-Bartok, O., Haupt, S., Alkalay-Snir, I., Saito, S., Appella, E., and Haupt, Y. (2008). PML enhances the regulation of p53 by CK1 in response to DNA damage. Oncogene 27, 3653–3661. doi: 10.1038/sj.onc.1211036
Ando, K., Ozaki, T., Yamamoto, H., Furuya, K., Hosoda, M., Hayashi, S., et al. (2004). Polo-like kinase 1 (Plk1) inhibits p53 function by physical interaction and phosphorylation. J. Biol. Chem. 279, 25549–25561. doi: 10.1074/jbc.M314182200
Baldwin, A., and Munger, K. (2010). Kinase requirements in human cells: V. Synthetic lethal interactions between p53 and the protein kinases SGK2 and PAK3. Proc. Natl. Acad. Sci. U.S.A. 107, 12463–12468. doi: 10.1073/pnas.1007462107
Banks, D., Wu, M., Higa, L. A., Gavrilova, N., Quan, J., Ye, T., et al. (2006). L2DTL/CDT2 and PCNA interact with p53 and regulate p53 polyubiquitination and protein stability through MDM2 and CUL4A/DDB1 complexes. Cell Cycle 5, 1719–1729. doi: 10.4161/cc.5.15.3150
Bernal, J. A., Luna, R., Espina, A., Lázaro, I., Ramos-Morales, F., Romero, F., et al. (2002). Human securin interacts with p53 and modulates p53-mediated transcriptional activity and apoptosis. Nat. Genet. 32, 306–311. doi: 10.1038/ng997
Berridge, M. J. (2005). Unlocking the secrets of cell signaling. Annu. Rev. Physiol. 67, 1–21. doi: 10.1146/annurev.physiol.67.040103.152647
Bin, W., Hui, X., Lin, H., Liao-han, Y., Shi-yi, Y., Qi-gai, H., et al. (2015). The study on the inhibition of mouse lymphocyte development caused by porcine circovirus type 2. Acta Vet. Zootechnica Sin. 46, 1400–1408. doi: 10.11843/j.issn.0366-6964.2015.08.016
Bonizzi, G., and Karin, M. (2004). The two NF-kappaB activation pathways and their role in innate and adaptive immunity. Trends Immunol. 25, 280–288. doi: 10.1016/j.it.2004.03.008
Brignone, C., Bradley, K. E., Kisselev, A. F., and Grossman, S. R. (2004). A post-ubiquitination role for MDM2 and hHR23A in the p53 degradation pathway. Oncogene 23, 4121–4129. doi: 10.1038/sj.onc.1207540
Bulavin, D. V., Saito, S., Hollander, M. C., Sakaguchi, K., Anderson, C. W., Appella, E., et al. (1999). Phosphorylation of human p53 by p38 kinase coordinates N-terminal phosphorylation and apoptosis in response to UV radiation. EMBO J. 18, 6845–6854. doi: 10.1093/emboj/18.23.6845
Chang, H. W., Jeng, C. R., Lin, C. M., Liu, J. J., Chang, C. C., Tsai, Y. C., et al. (2007a). The involvement of Fas/FasL interaction in porcine circovirus type 2 and porcine reproductive and respiratory syndrome virus co-inoculation-associated lymphocyte apoptosis in vitro. Vet. Microbiol. 122, 72–82.
Chang, J., Davis-Dusenbery, B. N., Kashima, R., Jiang, X., Marathe, N., Sessa, R., et al. (2013). Acetylation of p53 stimulates miRNA processing and determines cell survival following genotoxic stress. EMBO J. 32, 3192–3205. doi: 10.1038/emboj.2013.242
Chen, C. L., Lin, C. F., Wan, S. W., Wei, L. S., Chen, M. C., Yeh, T. M., et al. (2013). Anti-dengue virus nonstructural protein 1 antibodies cause NO-mediated endothelial cell apoptosis via ceramide-regulated glycogen synthase kinase-3beta and NF-kappaB activation. J. Immunol. 191, 1744–1752. doi: 10.4049/jimmunol.1201976
Chen, Y., and Brandizzi, F. (2013). IRE1: ER stress sensor and cell fate executor. Trends Cell Biol. 23, 547–555. doi: 10.1016/j.tcb.2013.06.005
Collavin, L., Lunardi, A., and Del Sal, G. (2010). p53-family proteins and their regulators: hubs and spokes in tumor suppression. Cell Death Differ. 17, 901–911. doi: 10.1038/cdd.2010.35
Connolly, J. L., Rodgers, S. E., Clarke, P., Ballard, D. W., Kerr, L. D., Tyler, K. L., et al. (2000). Reovirus-induced apoptosis requires activation of transcription factor NF-kappaB. J. Virol. 74, 2981–2989. doi: 10.1128/JVI.74.7.2981-2989.2000
Cooray, S. (2004). The pivotal role of phosphatidylinositol 3-kinase-Akt signal transduction in virus survival. J. Gen. Virol. 85, 1065–1076. doi: 10.1099/vir.0.19771-0
Cordenonsi, M., Dupont, S., Maretto, S., Insinga, A., Imbriano, C., and Piccolo, S. (2003). Links between tumor suppressors: p53 is required for TGF-beta gene responses by cooperating with Smads. Cell 113, 301–314. doi: 10.1016/S0092-8674(03)00308-8
Cory, S., and Adams, J. M. (2002). The Bcl2 family: regulators of the cellular life-or-death switch. Nat. Rev. Cancer 2, 647–656. doi: 10.1038/nrc883
Czabotar, P. E., Lessene, G., Strasser, A., and Adams, J. M. (2014). Control of apoptosis by the BCL-2 protein family: implications for physiology and therapy. Nat. Rev. Mol. Cell Biol. 15, 49–63. doi: 10.1038/nrm3722
Dornan, D., Wertz, I., Shimizu, H., Arnott, D., Frantz, G. D., Dowd, P., et al. (2004). The ubiquitin ligase COP1 is a critical negative regulator of p53. Nature 429, 86–92. doi: 10.1038/nature02514
Drago, I., Pizzo, P., and Pozzan, T. (2011). After half a century mitochondrial calcium in- and efflux machineries reveal themselves. EMBO J. 30, 4119–4125. doi: 10.1038/emboj.2011.337
Du, Q., Huang, Y., Wang, T., Zhang, X., Chen, Y., Cui, B., et al. (2016). Porcine circovirus type 2 activates PI3K/Akt and p38 MAPK pathways to promote interleukin-10 production in macrophages via Cap interaction of gC1qR. Oncotarget 7, 17492–17507. doi: 10.18632/oncotarget.7362
Ellis, J., Hassard, L., Clark, E., Harding, J., Allan, G., Willson, P., et al. (1998). Isolation of circovirus from lesions of pigs with postweaning multisystemic wasting syndrome. Can. Vet. J. 39, 44–51.
Fauquet, C. M., Mayo, M. A., Maniloff, J., Desselberger, U., and Ball, L. A. (1995). Virus Taxonomy: VIIIth Report of the International Committee on Taxonomy of Viruses. Berlin: Springer.
Finsterbusch, T., Steinfeldt, T., Doberstein, K., Rodner, C., and Mankertz, A. (2009a). Interaction of the replication proteins and the capsid protein of porcine circovirus type 1 and 2 with host proteins. Virology 386, 122–131. doi: 10.1016/j.virol.2008.12.039
Finsterbusch, T., Steinfeldt, T. K., Rodner, C., and Mankertz, A. (2009b). Interaction of the replication proteins and the capsid protein of porcine circovirus type 1 and 2 with host proteins. Virology 386, 122–131. doi: 10.1016/j.virol.2008.12.039
Fort, M., Sibila, M., Allepuz, A., Mateu, E., Roerink, F., and Segalés, J. (2008). Porcine circovirus type 2 (PCV2) vaccination of conventional pigs prevents viremia against PCV2 isolates of different genotypes and geographic origins. Vaccine 26, 1063–1071. doi: 10.1016/j.vaccine.2007.12.019
Frank, A. K., Pietsch, E. C., Dumont, P., Tao, J., and Murphy, M. E. (2011). Wild-type and mutant p53 proteins interact with mitochondrial caspase-3. Cancer Biol. Ther. 11, 740–745. doi: 10.4161/cbt.11.8.14906
Fuchs, S. Y., Adler, V., Buschmann, T., Yin, Z., Wu, X., Jones, S. N., et al. (1998a). JNK targets p53 ubiquitination and degradation in nonstressed cells. Genes Dev. 12, 2658–2663.
Fuchs, S. Y., Adler, V., Pincus, M. R., and Ronai, Z. (1998b). MEKK1/JNK signaling stabilizes and activates p53. Proc. Natl. Acad. Sci. U.S.A. 95, 10541–10546.
Fujioka, S., Schmidt, C., Sclabas, G. M., Li, Z., Pelicano, H., Peng, B., et al. (2004). Stabilization of p53 is a novel mechanism for proapoptotic function of NF-kappaB. J. Biol. Chem. 279, 27549–27559. doi: 10.1074/jbc.M313435200
Fukumoto, R., and Kiang, J. G. (2011). Geldanamycin analog 17-DMAG limits apoptosis in human peripheral blood cells by inhibition of p53 activation and its interaction with heat-shock protein 90 kDa after exposure to ionizing radiation. Radiat. Res. 176, 333–345. doi: 10.1667/RR2534.1
Galindocardiel, I., Grauroma, L., Pérezmaíllo, M., and Segalés, J. (2011). Characterization of necrotizing lymphadenitis associated with porcine circovirus type 2 infection. J. Comp. Pathol. 144, 63–69. doi: 10.1016/j.jcpa.2010.06.003
Galluzzi, L., Kepp, O., and Kroemer, G. (2012). Mitochondria: master regulators of danger signalling. Nat. Rev. Mol. Cell Biol. 13, 780–788. doi: 10.1038/nrm3479
Galluzzi, L., Maiuri, M. C., Vitale, I., Zischka, H., Castedo, M., Zitvogel, L., et al. (2007). Cell death modalities: classification and pathophysiological implications. Cell Death Differ. 14, 1237–1243. doi: 10.1038/sj.cdd.4402148
Gan, F., Hu, Z., Huang, Y., Xue, H., Huang, D., Qian, G., et al. (2016). Overexpression of pig selenoprotein S blocks OTA-induced promotion of PCV2 replication by inhibiting oxidative stress and p38 phosphorylation in PK15 cells. Oncotarget 7, 20469–20485. doi: 10.18632/oncotarget.7814
Gao, Z., Dong, Q., Jiang, Y., Opriessnig, T., Wang, J., Quan, Y., et al. (2014a). ORF4-protein deficient PCV2 mutants enhance virus-induced apoptosis and show differential expression of mRNAs in vitro. Virus Res. 183, 56–62. doi: 10.1016/j.virusres.2014.01.024
Garrido, C., Galluzzi, L., Brunet, M., Puig, P. E., Didelot, C., Kroemer, G., et al. (2006). Mechanisms of cytochrome c release from mitochondria. Cell Death Differ. 13, 1423–1433. doi: 10.1038/sj.cdd.4401950
Gartel, A. L., and Tyner, A. L. (2002). The role of the cyclin-dependent kinase inhibitor p21 in apoptosis. Mol. Cancer Ther. 1, 639–649.
Gray, T. A., Hernandez, L., Carey, A. H., Schaldach, M. A., Smithwick, M. J., Rus, K., et al. (2000). The ancient source of a distinct gene family encoding proteins featuring RING and C(3)H zinc-finger motifs with abundant expression in developing brain and nervous system. Genomics 66, 76–86. doi: 10.1006/geno.2000.6199
Green, D. R., and Kroemer, G. (2009). Cytoplasmic functions of the tumour suppressor p53. Nature 458, 1127–1130. doi: 10.1038/nature07986
Hajnoczky, G., Davies, E., and Madesh, M. (2003). Calcium signaling and apoptosis. Biochem. Biophys. Res. Commun. 304, 445–454. doi: 10.1016/S0006-291X(03)00616-8
Hamel, A. L., Lin, L. L., and Nayar, G. P. S. (1998). Nucleotide sequence of porcine circovirus associated with postweaning multisystemic wasting syndrome in pigs. J. Virol. 72, 5262–5267.
Han, J., Goldstein, L. A., Hou, W., Gastman, B. R., and Rabinowich, H. (2010). Regulation of mitochondrial apoptotic events by p53-mediated disruption of complexes between Antiapoptotic Bcl-2 members and bim. J. Biol. Chem. 285, 22473–22483. doi: 10.1074/jbc.M109.081042
Hayden, M. S., and Ghosh, S. (2004). Signaling to NF-kappaB. Genes Dev. 18, 2195–2224. doi: 10.1101/gad.1228704
He, J., Cao, J., Zhou, N., Jin, Y., Wu, J., and Zhou, J. (2013). Identification and functional analysis of the novel ORF4 protein encoded by porcine circovirus type 2. J. Virol. 87, 1420–1429. doi: 10.1128/JVI.01443-12
Hetz, C. (2012). The unfolded protein response: controlling cell fate decisions under ER stress and beyond. Nat. Rev. Mol. Cell Biol. 13, 89–102. doi: 10.1038/nrm3270
Hong, J. S., Kim, N. H., Choi, C. Y., Lee, J. S., Na, D., Chun, T., et al. (2015). Changes in cellular microRNA expression induced by porcine circovirus type 2-encoded proteins. Vet. Res. 46, 1–14. doi: 10.1186/s13567-015-0172-5
Hsu, M. J., Wu, C. Y., Chiang, H. H., Lai, Y. L., and Hung, S. L. (2010). PI3K/Akt signaling mediated apoptosis blockage and viral gene expression in oral epithelial cells during herpes simplex virus infection. Virus Res. 153, 36–43. doi: 10.1016/j.virusres.2010.07.002
Huang, C., Ma, W. Y., Maxiner, A., Sun, Y., and Dong, Z. (1999). p38 kinase mediates UV-induced phosphorylation of p53 protein at serine 389. J. Biol. Chem. 274, 12229–12235. doi: 10.1074/jbc.274.18.12229
Huang, J., Perez-Burgos, L., Placek, B. J., Sengupta, R., Richter, M., Dorsey, J. A., et al. (2006). Repression of p53 activity by Smyd2-mediated methylation. Nature 444, 629–632. doi: 10.1038/nature05287
Jan, J. T., Chen, B. H., Ma, S. H., Liu, C. I., Tsai, H. P., Wu, H. C., et al. (2000). Potential dengue virus-triggered apoptotic pathway in human neuroblastoma cells: arachidonic acid, superoxide anion, and NF-kappaB are sequentially involved. J. Virol. 74, 8680–8691. doi: 10.1128/JVI.74.18.8680-8691.2000
Javelaud, D., and Besancon, F. (2002). Inactivation of p21WAF1 sensitizes cells to apoptosis via an increase of both p14ARF and p53 levels and an alteration of the Bax/Bcl-2 ratio. J. Biol. Chem. 277, 37949–37954. doi: 10.1074/jbc.M204497200
Jorgensen, I., Rayamajhi, M., and Miao, E. A. (2017). Programmed cell death as a defence against infection. Nat. Rev. Immunol. 17, 151–164. doi: 10.1038/nri.2016.147
Jung, Y. S., Qian, Y., and Chen, X. (2010). Examination of the expanding pathways for the regulation of p21 expression and activity. Cell. Signal. 22, 1003–1012. doi: 10.1016/j.cellsig.2010.01.013
Jung, Y.-S., Qian, Y., and Chen, X. (2012). Pirh2 RING-finger E3 ubiquitin ligase: its role in tumorigenesis and cancer therapy. FEBS Lett. 586, 1397–1402. doi: 10.1016/j.febslet.2012.03.052
Kahyo, T., Nishida, T., and Yasuda, H. (2001). Involvement of PIAS1 in the sumoylation of tumor suppressor p53. Mol. Cell 8, 713–718. doi: 10.1016/S1097-2765(01)00349-5
Karuppannan, A. K., Liu, S., Jia, Q., Selvaraj, M., and Kwang, J. (2010). Porcine circovirus type 2 ORF3 protein competes with p53 in binding to Pirh2 and mediates the deregulation of p53 homeostasis. Virology 398, 1–11. doi: 10.1016/j.virol.2009.11.028
Kiupel, M., Stevenson, G. W., Galbreath, E. J., North, A., HogenEsch, H., and Mittal, S. K. (2005). Porcine Circovirus type 2 (PCV2) causes apoptosis in experimentally inoculated BALB/c mice. BMC Vet. Res. 1:7. doi: 10.1186/1746-6148-1-7
Kouokam Fotso, G. B., Bernard, C., Bigault, L., de Boisséson, C., Mankertz, A., Jestin, A., et al. (2016). The expression level of gC1qR is down regulated at the early time of infection with porcine circovirus of type 2 (PCV-2) and gC1qR interacts differently with the Cap proteins of porcine circoviruses. Virus Res. 220, 21–32. doi: 10.1016/j.virusres.2016.04.006
Krakowka, S., Ellis, J., McNeilly, F., Meehan, B., Oglesbee, M., Alldinger, S., et al. (2004). Features of cell degeneration and death in hepatic failure and systemic lymphoid depletion characteristic of porcine circovirus-2-associated postweaning multisystemic wasting disease. Vet. Pathol. 41, 471–481. doi: 10.1354/vp.41-5-471
Kroemer, G., Galluzzi, L., and Brenner, C. (2006). Mitochondrial membrane permeabilization in cell death. Physiol. Rev. 87, 99–163. doi: 10.1152/physrev.00013.2006
Kroemer, G., Galluzzi, L., Vandenabeele, P., Abrams, J., Alnemri, E. S., Baehrecke, E. H., et al. (2005). Classification of cell death: recommendations of the Nomenclature Committee on Cell Death 2009. Cell Death Differ. 12(Suppl. 2), 1463–1467. doi: 10.1038/cdd.2008.150
Kyriakis, J. M., and Avruch, J. (2001). Mammalian mitogen-activated protein kinase signal transduction pathways activated by stress and inflammation. Physiol. Rev. 81, 807–869. doi: 10.1152/physrev.2001.81.2.807
Lamkanfi, M., Festjens, N., Declercq, W., Vanden, B. T., and Vandenabeele, P. (2007). Caspases in cell survival, proliferation and differentiation. Cell Death Differ. 14, 44–55. doi: 10.1038/sj.cdd.4402047
Langley, E., Pearson, M., Faretta, M., Bauer, U. M., Frye, R. A., Minucci, S., et al. (2014). Human SIR2 deacetylates p53 and antagonizes PML/p53-induced cellular senescence. EMBO J. 21, 2383–2396. doi: 10.1093/emboj/21.10.2383
Latini, P., Frontini, M., Caputo, M., Gregan, J., Cipak, L., Filippi, S., et al. (2011). CSA and CSB proteins interact with p53 and regulate its Mdm2-dependent ubiquitination. Cell Cycle 10, 3719–3730. doi: 10.4161/cc.10.21.17905
Le Cam, L., Linares, L. K., Paul, C., Julien, E., Lacroix, M., Hatchi, E., et al. (2006). E4F1 is an atypical ubiquitin ligase that modulates p53 effector functions independently of degradation. Cell 127, 775–788. doi: 10.1016/j.cell.2006.09.031
Lee, E. W., Lee, M. S., Camus, S., Ghim, J., Yang, M. R., Oh, W., et al. (2009). Differential regulation of p53 and p21 by MKRN1 E3 ligase controls cell cycle arrest and apoptosis. EMBO J. 28, 2100–2113. doi: 10.1038/emboj.2009.164
Lee, M. S., Jeong, M. H., Lee, H. W., Han, H. J., Ko, A., Hewitt, S. M., et al. (2013). The expression of makorin ring finger protein 1 (MKRN1), pAKT, pmTOR, and PTEN in cervical neoplasia. Gynecol. Oncol. 130:e42. doi: 10.1016/j.ygyno.2013.04.158
Legube, G., Linares, L. K., Tyteca, S., Caron, C., Scheffner, M., Chevillard-Briet, M., et al. (2004). Role of the histone acetyl transferase Tip60 in the p53 pathway. J. Biol. Chem. 279, 44825–44833. doi: 10.1074/jbc.M407478200
Leng, R. P., Lin, Y., Ma, W., Wu, H., Lemmers, B., Chung, S., et al. (2003). Pirh2, a p53-induced ubiquitin-protein ligase, promotes p53 degradation. Cell 112, 779–791. doi: 10.1016/S0092-8674(03)00193-4
Levine, A. J., and Oren, M. (2009). The first 30 years of p53: growing ever more complex. Nat. Rev. Cancer 9, 749–758. doi: 10.1038/nrc2723
Li, D., Marchenko, N. D., Schulz, R., Fischer, V., Velasco-Hernandez, T., Talos, F., et al. (2011). Functional inactivation of endogenous MDM2 and CHIP by HSP90 causes aberrant stabilization of mutant p53 in human cancer cells. Mol. Cancer Res. 9, 577–588. doi: 10.1158/1541-7786.MCR-10-0534
Li, M., Chen, D., Shiloh, A., Luo, J., Nikolaev, A. Y., Qin, J., et al. (2002). Deubiquitination of p53 by HAUSP is an important pathway for p53 stabilization. Nature 416, 648–653. doi: 10.1038/nature737
Li, P., Nijhawan, D., Budihardjo, I., Srinivasula, S. M., Ahmad, M., Alnemri, E. S., et al. (1997). Cytochrome c and dATP-dependent formation of Apaf-1/caspase-9 complex initiates an apoptotic protease cascade. Cell 91, 479–489. doi: 10.1016/S0092-8674(00)80434-1
Li, Q., Wang, X., Wu, X., Rui, Y., Liu, W., Wang, J., et al. (2007). Daxx cooperates with the Axin/HIPK2/p53 complex to induce cell death. Cancer Res. 67, 66–74. doi: 10.1158/0008-5472.CAN-06-1671
Li, S., Kong, L., and Yu, X. (2015). The expanding roles of endoplasmic reticulum stress in virus replication and pathogenesis. Crit. Rev. Microbiol. 41, 150–164. doi: 10.3109/1040841X.2013.813899
Li, W., Liu, S., Wang, Y., Deng, F., Yan, W., Yang, K., et al. (2013). Transcription analysis of the porcine alveolar macrophage response to porcine circovirus type 2. BMC Genomics 14:353. doi: 10.1186/1471-2164-14-353
Li, X., Zhao, Y., Wu, W. K., Liu, S., Cui, M., and Lou, H. (2011). Solamargine induces apoptosis associated with p53 transcription-dependent and transcription-independent pathways in human osteosarcoma U2OS cells. Life Sci. 88, 314–321. doi: 10.1016/j.lfs.2010.12.006
Li, Y. Z., Lu, D. Y., Tan, W. Q., Wang, J. X., and Li, P. F. (2008). p53 initiates apoptosis by transcriptionally targeting the antiapoptotic protein ARC. Mol. Cell. Biol. 28, 564–574. doi: 10.1128/MCB.00738-07
Lin, C., Gu, J., Wang, H., Zhou, J., Li, J., Wang, S., et al. (2018). Caspase-dependent apoptosis induction via viral protein ORF4 of porcine circovirus 2 binding to mitochondrial adenine nucleotide translocase 3. J. Virol. 92, e00238-18. doi: 10.1128/JVI.00238-18
Lin, J. Y., Ohshima, T., and Shimotohno, K. (2004). Association of Ubc9, an E2 ligase for SUMO conjugation, with p53 is regulated by phosphorylation of p53. FEBS Lett. 573, 15–18. doi: 10.1016/j.febslet.2004.07.059
Lin, K. I., DiDonato, J. A., Hoffmann, A., Hardwick, J. M., and Ratan, R. R. (1998). Suppression of steady-state, but not stimulus-induced NF-kappaB activity inhibits alphavirus-induced apoptosis. J. Cell Biol. 141, 1479–1487. doi: 10.1083/jcb.141.7.1479
Lin, W., Chien, M., Wu, P., Lai, C., and Huang, C. (2013). The porcine circovirus type 2 nonstructural protein ORF3 induces apoptosis in porcine peripheral blood mononuclear cells. Open Virol. J. 5, 148–153. doi: 10.2174/1874357901105010148
Lin, W. L., Chien, M. S., Wu, P. C., Lai, C. L., and Huang, C. (2011). The porcine circovirus type 2 nonstructural protein ORF3 induces apoptosis in porcine peripheral blood mononuclear cells. Open Virol. J. 5, 148–153. doi: 10.2174/1874357901105010148
Liu, C., Liu, Y., Chen, H., Feng, H., Chen, Y., Wang, Y., et al. (2018). Genetic and immunogenicity analysis of porcine circovirus type 2 strains isolated in central China. Arch. Virol. 46, 1–10. doi: 10.1007/s00705-017-3685-6
Liu, J., Bai, J., Lu, Q., Zhang, L., Jiang, Z., Michal, J. J., et al. (2013a). Two-dimensional liquid chromatography–tandem mass spectrometry coupled with isobaric tags for relative and absolute quantification (iTRAQ) labeling approach revealed first proteome profiles of pulmonary alveolar macrophages infected with porcine circovirus type 2. J. Proteom. 79, 72–86. doi: 10.1016/j.jprot.2012.11.024
Liu, J., Bai, J., Zhang, L., Jiang, Z., Wang, X., Li, Y., et al. (2013b). Hsp70 positively regulates porcine circovirus type 2 replication in vitro. Virology 447, 52–62. doi: 10.1016/j.virol.2013.08.025
Liu, J., Chen, I., Du, Q., Chua, H., and Kwang, J. (2006). The ORF3 protein of porcine circovirus type 2 is involved in viral pathogenesis in vivo. J. Virol. 80, 5065–5073. doi: 10.1128/JVI.80.10.5065-5073.2006
Liu, J., Chen, I., and Kwang, J. (2005). Characterization of a previously unidentified viral protein in porcine circovirus type 2-infected cells and its role in virus-induced apoptosis. J. Virol. 79, 8262–8274.
Liu, J., Zhu, Y., Chen, I., Lau, J., He, F., Lau, A., et al. (2007). The ORF3 protein of porcine circovirus type 2 interacts with porcine ubiquitin E3 ligase Pirh2 and facilitates p53 expression in viral infection. J. Virol. 81, 9560–9567. doi: 10.1128/JVI.00681-07
Liu, L., Scolnick, D. M., Trievel, R. C., Zhang, H. B., Marmorstein, R., Halazonetis, T. D., et al. (1999). p53 sites acetylated in vitro by PCAF and p300 are acetylated in vivo in response to DNA damage. Mol. Cell. Biol. 19, 1202–1209. doi: 10.1128/MCB.19.2.1202
Liu, M., and Vakharia, V. N. (2006). Nonstructural protein of infectious bursal disease virus inhibits apoptosis at the early stage of virus infection. J. Virol. 80, 3369–3377. doi: 10.1128/JVI.80.7.3369-3377.2006
Lv, Q., Guo, K., Xu, H., Wang, T., and Zhang, Y. (2015a). Correction: identification of putative ORF5 protein of porcine circovirus type 2 and functional analysis of GFP-fused ORF5 protein. PLoS One 10:e0127859. doi: 10.1371/journal.pone.0127859
Lv, Q., Guo, K., Wang, T., Zhang, C., and Zhang, Y. (2015b). Porcine circovirus type 2 ORF4 protein binds heavy chain ferritin. J. Biosci. 40, 477–485.
Lv, Q., Guo, K., Zhang, G., and Zhang, Y. (2016). The ORF4 protein of porcine circovirus type 2 antagonizes apoptosis by stabilizing the concentration of ferritin heavy chain through physical interaction. J. Gen. Virol. 97, 1636–1646. doi: 10.1099/jgv.0.000472
Lv, Q. Z., Guo, K. K., and Zhang, Y. M. (2014a). Current understanding of genomic DNA of porcine circovirus type 2. Virus Genes 49, 1–10. doi: 10.1007/s11262-014-1099-z
Lv, Y., Dai, L., Han, H., and Zhang, S. (2012). PCV2 induces apoptosis and modulates calcium homeostasis in piglet lymphocytes in vitro. Res. Vet. Sci. 93, 1525–1530. doi: 10.1016/j.rvsc.2012.04.003
Mancini, F., Di Conza, G., Pellegrino, M., Rinaldo, C., Prodosmo, A., Giglio, S., et al. (2014). MDM4 (MDMX) localizes at the mitochondria and facilitates the p53-mediated intrinsic-apoptotic pathway. EMBO J. 28, 1926–1939. doi: 10.1038/emboj.2009.154
Mankertz, A. (2012). Molecular interactions of porcine circoviruses type 1 and type 2 with its host. Virus Res. 164, 54–60. doi: 10.1016/j.virusres.2011.11.001
Mantovani, F., Tocco, F., Girardini, J., Smith, P., Gasco, M., Lu, X., et al. (2007). The prolyl isomerase Pin1 orchestrates p53 acetylation and dissociation from the apoptosis inhibitor iASPP. Nat. Struct. Mol. Biol. 14, 912–920. doi: 10.1038/nsmb1306
Mao, Y., Li, J. J., Liu, Y., Dong, W., Pang, P., and Deng, Z. B. (2017). Imbalance of intestinal immune function in piglets infected by porcine circovirus type 2 during the fetal period. Acta Vet. Hung. 65, 135–146. doi: 10.1556/004.2017.014
Marchenko, N. D., Wolff, S., Erster, S., Becker, K., and Moll, U. M. (2007). Monoubiquitylation promotes mitochondrial p53 translocation. EMBO J. 26, 923–934. doi: 10.1038/sj.emboj.7601560
Meehan, B. M., McNeilly, F., Todd, D., Kennedy, S., Jewhurst, V. A., Ellis, J. A., et al. (1998). Characterization of novel circovirus DNAs associated with wasting syndromes in pigs. J. Gen. Virol. 79(Pt 9), 2171–2179. doi: 10.1099/0022-1317-79-9-2171
Mihara, M., Erster, S., Zaika, A., Petrenko, O., Chittenden, T., Pancoska, P., et al. (2003). p53 Has a direct apoptogenic role at the mitochondria. Mol. Cell 11, 577–590. doi: 10.1016/S1097-2765(03)00050-9
Nawagitgul, P., Morozov, I., Bolin, S. R., Harms, P. A., Sorden, S. D., and Paul, P. S. (2000). Open reading frame 2 of porcine circovirus type 2 encodes a major capsid protein. J. Gen. Virol. 81, 2281–2287. doi: 10.1099/0022-1317-81-9-2281
Nielsen, J., Vincent, I. E., Bøtner, A., Ladekaer-Mikkelsen, A. S., Allan, G., Summerfield, A., et al. (2003). Association of lymphopenia with porcine circovirus type 2 induced postweaning multisystemic wasting syndrome (PMWS). Vet. Immunol. Immunopathol. 92, 97–111. doi: 10.1016/S0165-2427(03)00031-X
Oleinik, N. V., Krupenko, N. I., and Krupenko, S. A. (2007). Cooperation between JNK1 and JNK2 in activation of p53 apoptotic pathway. Oncogene 26, 7222–7230. doi: 10.1038/sj.onc.1210526
Opriessnig, T., Meng, X. J., and Halbur, P. G. (2007). Porcine circovirus type 2–associated disease: update on current terminology, clinical manifestations, pathogenesis, diagnosis, and intervention strategies. J. Vet. Diagn. Invest. 19, 591–615. doi: 10.1177/104063870701900601
Opriessnig, T., Patterson, A. R., Elsener, J., Meng, X. J., and Halbur, P. G. (2008). Influence of maternal antibodies on efficacy of porcine circovirus type 2 (PCV2) vaccination to protect pigs from experimental infection with PCV2. Clin. Vaccine Immunol. 15, 397–401. doi: 10.1128/CVI.00416-07
Opriessnig, T., Patterson, A. R., Madson, D. M., Pal, N., Ramamoorthy, S., Meng, X. J., et al. (2010). Comparison of the effectiveness of passive (dam) versus active (piglet) immunization against porcine circovirus type 2 (PCV2) and impact of passively derived PCV2 vaccine-induced immunity on vaccination. Vet. Microbiol. 142, 177–183. doi: 10.1016/j.vetmic.2009.09.056
Palinski, R., Piñeyro, P., Shang, P., Yuan, F., Guo, R., Fang, Y., et al. (2016). A novel porcine circovirus distantly related to known circoviruses is associated with porcine dermatitis and nephropathy syndrome and reproductive failure. J. Virol. 91, e01879-16.
Pan, D., Pan, L. Z., Hill, R., Marcato, P., Shmulevitz, M., Vassilev, L. T., et al. (2011). Stabilisation of p53 enhances reovirus-induced apoptosis and virus spread through p53-dependent NF-kappaB activation. Br. J. Cancer 105, 1012–1022. doi: 10.1038/bjc.2011.325
Pathak, N., Mitra, S., and Khandelwal, S. (2013). Cadmium induces thymocyte apoptosis via caspase-dependent and caspase-independent pathways. J. Biochem. Mol. Toxicol. 27, 193–203. doi: 10.1002/jbt.21468
Phan, T. G., Giannitti, F., Rossow, S., Marthaler, D., Knutson, T. P., Li, L., et al. (2016). Detection of a novel circovirus PCV3 in pigs with cardiac and multi-systemic inflammation. Virol. J. 13:184. doi: 10.1186/s12985-016-0642-z
Pierantoni, G. M., Rinaldo, C., Esposito, F., Mottolese, M., Soddu, S., and Fusco, A. (2014). High Mobility Group A1 (HMGA1) proteins interact with p53 and inhibit its apoptotic activity. Cell Death Differ. 21:503. doi: 10.1038/cdd.2013.183
Rajagopalan, S., Andreeva, A., Rutherford, T. J., and Fersht, A. R. (2010). Mapping the physical and functional interactions between the tumor suppressors p53 and BRCA2. Proc. Natl. Acad. Sci. U.S.A. 107, 8587–8592. doi: 10.1073/pnas.1003689107
Ramírez-Boo, M., Núnez, E., Jorge, I., Navarro, P., Fernandes, L. T., Segalés, J., et al. (2011). Quantitative proteomics by 2-DE, 16O/18O labelling and linear ion trap mass spectrometry analysis of lymph nodes from piglets inoculated by porcine circovirus type 2. Proteomics 11, 3452–3469. doi: 10.1002/pmic.201000610
Reid, M. A., Wang, W. I., Rosales, K. R., Welliver, M. X., Pan, M., and Kong, M. (2013). The B55Î ± subunit of PP2A drives a p53-dependent metabolic adaptation to glutamine deprivation. Mol. Cell 50, 200–211. doi: 10.1016/j.molcel.2013.02.008
Resendes, A. R., Majó, N., Segalés, J., Mateu, E., Calsamiglia, M., and Domingo, M. (2004). Apoptosis in lymphoid organs of pigs naturally infected by porcine circovirus type 2. J. Gen. Virol. 85, 2837–2844. doi: 10.1099/vir.0.80221-0
Resendes, A. R., Majó, N., van den Ingh, T. S., Mateu, E., and Domingo, M. (2011). Apoptosis in postweaning multisystemic wasting syndrome (PMWS) hepatitis in pigs naturally infected with porcine circovirus type 2 (PCV2). Vet. J. 189, 72–76. doi: 10.1016/j.tvjl.2010.06.018
Resendes, A. R., and Segalés, J. (2015). Characterization of vascular lesions in pigs affected by porcine circovirus type 2-systemic disease. Vet. Pathol. 52, 497–504. doi: 10.1177/0300985814540542
Richmond, O., Cecere, T. E., Erdogan, E., Meng, X. J., Piñeyro, P., Subramaniam, S., et al. (2015). PD-L1 expression is increased in monocyte derived dendritic cells in response to porcine circovirus type 2 and porcine reproductive and respiratory syndrome virus infections. Vet. Immunol. Immunopathol. 168, 24–29. doi: 10.1016/j.vetimm.2015.09.013
Rodriguez, M. S., Desterro, J. M., Lain, S., Midgley, C. A., Lane, D. P., and Hay, R. T. (2014). SUMO-1 modification activates the transcriptional response of p53. EMBO J. 18, 6455–6461. doi: 10.1093/emboj/18.22.6455
Salgado, R. L., Vidigal, P. M., de Souza, L. F., Onofre, T. S., Gonzaga, N. F., Eller, M. R., et al. (2014). Identification of an emergent porcine circovirus-2 in vaccinated pigs from a Brazilian farm during a postweaning multisystemic wasting syndrome outbreak. Genome Announc. 2:e00163-14. doi: 10.1128/genomeA.00163-14
Saraiva, G. L., Vidigal, P. M. P., Fietto, J. L. R., Bressan, G. C., Silva, A. Jr., and de Almeida, M. R. (2018). Evolutionary analysis of Porcine circovirus 3 (PCV3) indicates an ancient origin for its current strains and a worldwide dispersion. Virus Genes 54, 376–384. doi: 10.1007/s11262-018-1545-4
Sato, M., Tanaka, H., Yamada, T., and Yamamoto, N. (2011). Dissection of cell context-dependent interactions between HBx and p53 family members in regulation of apoptosis: a role for HBV-induced HCC. Cell Cycle 10, 3554–3565. doi: 10.4161/cc.10.20.17856
Schultz, L., Khera, S., Sleve, D., Heath, J., and Chang, N. S. (2004). TIAF1 and p53 functionally interact in mediating apoptosis and silencing of TIAF1 abolishes nuclear translocation of serine 15-phosphorylated p53. DNA Cell Biol. 23, 67–74. doi: 10.1089/104454904322745943
Seeliger, F. A., Brügmann, M. L., Krüger, L., Greiser-Wilke, I., Verspohl, J., Segalés, J., et al. (2007). Porcine circovirus type 2-associated cerebellar vasculitis in postweaning multisystemic wasting syndrome (PMWS)-affected pigs. Vet. Pathol. 44, 621–634. doi: 10.1354/vp.44-5-621
Segalés, J., Allan, G. M., and Domingo, M. (2005). Porcine circovirus diseases. Anim. Health Res. Rev. 6, 119–142. doi: 10.1079/AHR2005106
Segalés, J., Kekarainen, T., and Cortey, M. (2013). The natural history of porcine circovirus type 2: from an inoffensive virus to a devastating swine disease? Vet. Microbiol. 165, 13–20. doi: 10.1016/j.vetmic.2012.12.033
Sharathchandra, A., Lal, R., Khan, D., and Das, S. (2012). Annexin A2 and PSF proteins interact with p53 IRES and regulate translation of p53 mRNA. RNA Biol. 9, 1429–1439. doi: 10.4161/rna.22707
Shen, J., Snapp, E. L., Lippincott-Schwartz, J., and Prywes, R. (2005). Stable binding of ATF6 to BiP in the endoplasmic reticulum stress response. Mol. Cell. Biol. 25, 921–932. doi: 10.1128/MCB.25.3.921-932.2005
Shibahara, T., Sato, K., Ishikawa, Y., and Kadota, K. (2000). Porcine circovirus induces B lymphocyte depletion in pigs with wasting disease syndrome. J. Vet. Med. Sci. 62, 1125–1131. doi: 10.1292/jvms.62.1125
Shin, Y. K., Liu, Q., Tikoo, S. K., Babiuk, L. A., and Zhou, Y. (2007). Influenza A virus NS1 protein activates the phosphatidylinositol 3-kinase (PI3K)/Akt pathway by direct interaction with the p85 subunit of PI3K. J. Gen. Virol. 88, 13–18. doi: 10.1099/vir.0.82419-0
Shore, G. C., Papa, F. R., and Oakes, S. A. (2011). Signaling cell death from the endoplasmic reticulum stress response. Curr. Opin. Cell Biol. 23, 143–149. doi: 10.1016/j.ceb.2010.11.003
Sinha, A., Schalk, S., Lager, K. M., Wang, C., and Opriessnig, T. (2012). Singular PCV2a or PCV2b infection results in apoptosis of hepatocytes in clinically affected gnotobiotic pigs. Res. Vet. Sci. 92, 151–156. doi: 10.1016/j.rvsc.2010.10.013
Soares, J. A., Leite, F. G., Andrade, L. G., Torres, A. A., De Sousa, L. P., Barcelos, L. S., et al. (2009). Activation of the PI3K/Akt pathway early during vaccinia and cowpox virus infections is required for both host survival and viral replication. J. Virol. 83, 6883–6899. doi: 10.1128/JVI.00245-09
Tait, S. W., and Green, D. R. (2010). Mitochondria and cell death: outer membrane permeabilization and beyond. Nat. Rev. Mol. Cell Biol. 11, 621–632. doi: 10.1038/nrm2952
Takekawa, M., Adachi, M., Nakahata, A., Nakayama, I., Itoh, F., Tsukuda, H., et al. (2014). p53-inducible wip1 phosphatase mediates a negative feedback regulation of p38 MAPK-p53 signaling in response to UV radiation. EMBO J. 19, 6517–6526. doi: 10.1093/emboj/19.23.6517
Tibbles, L. A., and Woodgett, J. R. (1999). The stress-activated protein kinase pathways. Cell. Mol. Life Sci. 55, 1230–1254. doi: 10.1007/s000180050369
Timmusk, S., Fossum, C., and Berg, M. (2006a). Porcine circovirus type 2 replicase binds the capsid protein and an intermediate filament-like protein. J. Gen. Virol. 87, 3215–3223.
Timmusk, S., Wallgren, P., Brunborg, I. M., Wikström, F. H., Allan, G., Meehan, B., et al. (2008). Phylogenetic analysis of porcine circovirus type 2 (PCV2) pre- and post-epizootic postweaning multisystemic wasting syndrome (PMWS). Virus Genes 36, 509–520. doi: 10.1007/s11262-008-0217-1
Tischer, I., Gelderblom, H., Vettermann, W., and Koch, M. A. (1982). A very small porcine virus with circular single-stranded DNA. Nature 295, 64–66. doi: 10.1038/295064a0
Tripathi, R., and Mishra, R. (2010). Interaction of Pax6 with SPARC and p53 in brain of mice indicates Smad3 dependent auto-regulation. J. Mol. Neurosci. 41, 397–403. doi: 10.1007/s12031-010-9334-0
Tummers, B., and Green, D. R. (2017). Caspase-8: regulating life and death. Immunol. Rev. 277, 76–89. doi: 10.1111/imr.12541
Verma, G., and Datta, M. (2012). The critical role of JNK in the ER-mitochondrial crosstalk during apoptotic cell death. J. Cell. Physiol. 227, 1791–1795. doi: 10.1002/jcp.22903
Vousden, K. H., and Prives, C. (2009). Blinded by the light: the growing complexity of p53. Cell 137, 413–431. doi: 10.1016/j.cell.2009.04.037
Wada, T., and Penninger, J. M. (2004). Mitogen-activated protein kinases in apoptosis regulation. Oncogene 23, 2838–2849. doi: 10.1038/sj.onc.1207556
Wade, M., Wang, Y. V., and Wahl, G. M. (2010). The p53 orchestra: Mdm2 and Mdmx set the tone. Trends Cell Biol. 20, 299–309. doi: 10.1016/j.tcb.2010.01.009
Wang, X., Zhang, H., Abel, A. M., Young, A. J., Xie, L., and Xie, Z. (2014). Role of phosphatidylinositol 3-kinase (PI3K) and Akt1 kinase in porcine reproductive and respiratory syndrome virus (PRRSV) replication. Arch. Virol. 159, 2091–2096. doi: 10.1007/s00705-014-2016-4
Wang, Z. J., Xu, C. M., Song, Z. B., Wang, M., Liu, Q. Y., Jiang, P., et al. (2018). Vimentin modulates infectious porcine circovirus type 2 in PK-15 cells. Virus Res. 243, 110–118. doi: 10.1016/j.virusres.2017.10.013
Watcharasit, P., Bijur, G. N., Song, L., Zhu, J., Chen, X., and Jope, R. S. (2003). Glycogen synthase kinase-3β (GSK3β) binds to and promotes the actions of p53. J. Biol. Chem. 278, 48872–48879. doi: 10.1074/jbc.M305870200
Weger, S., Hammer, E., and Heilbronn, R. (2005). Topors acts as a SUMO-1 E3 ligase for p53 in vitro and in vivo. FEBS Lett. 579, 5007–5012. doi: 10.1016/j.febslet.2005.07.088
Wei, L., Kwang, J., Wang, J., Shi, L., Yang, B., Li, Y., et al. (2008). Porcine circovirus type 2 induces the activation of nuclear factor kappa B by IkappaBalpha degradation. Virology 378, 177–184. doi: 10.1016/j.virol.2008.05.013
Wei, L., Zhu, S., Wang, J., and Liu, J. (2012a). Activation of the phosphatidylinositol 3-kinase/Akt signaling pathway during porcine circovirus type 2 infection facilitates cell survival and viral replication. J. Virol. 86, 13589–13597. doi: 10.1128/JVI.01697-12
Wei, L., Zhu, S., Wang, J., Quan, R., Yan, X., Li, Z., et al. (2016). Induction of a cellular DNA damage response by porcine circovirus type 2 facilitates viral replication and mediates apoptotic responses. Sci. Rep. 6:39444. doi: 10.1038/srep39444
Wei, L., Zhu, S., Wang, J., Zhang, C., Quan, R., Yan, X., et al. (2013). Regulatory role of ASK1 in porcine circovirus type 2-induced apoptosis. Virology 447, 285–291. doi: 10.1016/j.virol.2013.09.011
Wei, L., Zhu, Z., Wang, J., and Liu, J. (2009). JNK and p38 mitogen-activated protein kinase pathways contribute to porcine circovirus type 2 infection. J. Virol. 83, 6039–6047. doi: 10.1128/jvi.00135-09
Wolff, S., Erster, S., Palacios, G., and Moll, U. M. (2008). p53’s mitochondrial translocation and MOMP action is independent of Puma and Bax and severely disrupts mitochondrial membrane integrity. Cell Res. 18, 733–744. doi: 10.1038/cr.2008.62
Xiang-Jin, M. (2013). Porcine circovirus type 2 (PCV2): pathogenesis and interaction with the immune system. Annu. Rev. Anim. Biosci. 1, 43–64. doi: 10.1146/annurev-animal-031412-103720
Xiao, C. T., Halbur, P. G., and Opriessnig, T. (2015). Global molecular genetic analysis of porcine circovirus type 2 (PCV2) sequences confirms the presence of four main PCV2 genotypes and reveals a rapid increase of PCV2d. J. Gen. Virol. 96, 1830–1841. doi: 10.1099/vir.0.000100
Xu, D., Du, Q., Han, C., Wang, Z., Zhang, X., Wang, T., et al. (2016). p53 signaling modulation of cell cycle arrest and viral replication in porcine circovirus type 2 infection cells. Vet. Res. 47:120. doi: 10.1186/s13567-016-0403-4
Xu, X., Qiao, W., Linke, S. P., Cao, L., Li, W. M., Furth, P. A., et al. (2001). Genetic interactions between tumor suppressors Brca1 and p53 in apoptosis, cell cycle and tumorigenesis. Nat. Genet. 28, 266–271. doi: 10.1038/90108
Yamasaki, S., Yagishita, N., Sasaki, T., Nakazawa, M., Kato, Y., Yamadera, T., et al. (2014). Cytoplasmic destruction of p53 by the endoplasmic reticulum-resident ubiquitin ligase ‘Synoviolin’. EMBO J. 26, 113–122. doi: 10.1038/sj.emboj.7601490
Yan, W., Frank, C. L., Korth, M. J., Sopher, B. L., Novoa, I., Ron, D., et al. (2002). Control of PERK eIF2α kinase activity by the endoplasmic reticulum stress-induced molecular chaperone P58IPK. Proc. Natl. Acad. Sci. U.S.A. 99, 15920–15925. doi: 10.1073/pnas.252341799
Young, L. S., Dawson, C. W., and Eliopoulos, A. G. (2007). Viruses and apoptosis. Annu. Rev. Microbiol. 5, 105–111.
Yuan, J., Luo, K., Zhang, L., Cheville, J. C., and Lou, Z. (2010). USP10 regulates p53 localization and stability by deubiquitinating p53. Cell 140, 384–396. doi: 10.1016/j.cell.2009.12.032
Zhai, S. L., Chen, S. N., Xu, Z. H., Tang, M. H., Wang, F. G., Li, X. J., et al. (2014). Porcine circovirus type 2 in China: an update on and insights to its prevalence and control. Virol. J. 11:88. doi: 10.1186/1743-422X-11-88
Zhang, J., and Chen, X. (2007). DeltaNp73 modulates nerve growth factor-mediated neuronal differentiation through repression of TrkA. Mol. Cell. Biol. 27, 3868–3880. doi: 10.1128/MCB.02112-06
Keywords: apoptosis, PCV2, viral proteins, signaling pathway, mechanisms
Citation: Pan Y, Li P, Jia R, Wang M, Yin Z and Cheng A (2018) Regulation of Apoptosis During Porcine Circovirus Type 2 Infection. Front. Microbiol. 9:2086. doi: 10.3389/fmicb.2018.02086
Received: 19 May 2018; Accepted: 15 August 2018;
Published: 04 September 2018.
Edited by:
Akio Adachi, Kansai Medical University, JapanReviewed by:
Enric M. Mateu, Autonomous University of Barcelona, SpainStefan Vilcek, University of Veterinary Medicine in Kosice, Slovakia
Copyright © 2018 Pan, Li, Jia, Wang, Yin and Cheng. This is an open-access article distributed under the terms of the Creative Commons Attribution License (CC BY). The use, distribution or reproduction in other forums is permitted, provided the original author(s) and the copyright owner(s) are credited and that the original publication in this journal is cited, in accordance with accepted academic practice. No use, distribution or reproduction is permitted which does not comply with these terms.
*Correspondence: Renyong Jia, jiary@sicau.edu.cn