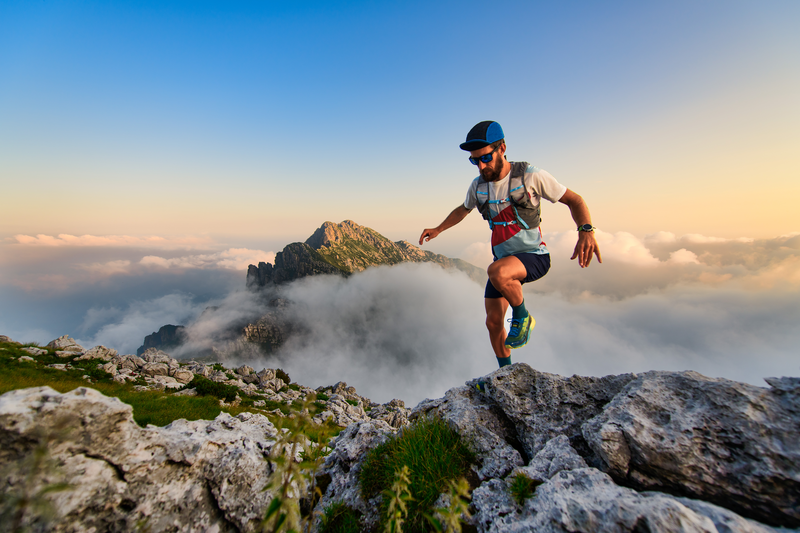
95% of researchers rate our articles as excellent or good
Learn more about the work of our research integrity team to safeguard the quality of each article we publish.
Find out more
REVIEW article
Front. Microbiol. , 12 September 2018
Sec. Plant Pathogen Interactions
Volume 9 - 2018 | https://doi.org/10.3389/fmicb.2018.02055
This article is part of the Research Topic Bacteria Present in Plant Nodules: Diversity, Biogeography and Plant Growth Promotion View all 14 articles
The symbiosis between Lotus and rhizobia has been long considered very specific and only two bacterial species were recognized as the microsymbionts of Lotus: Mesorhizobium loti was considered the typical rhizobia for the L. corniculatus complex, whereas Bradyrhizobium sp. (Lotus) was the symbiont for L. uliginosus and related species. As discussed in this review, this situation has dramatically changed during the last 15 years, with the characterization of nodule bacteria from worldwide geographical locations and from previously unexplored Lotus spp. Current data support that the Lotus rhizobia are dispersed amongst nearly 20 species in five genera (Mesorhizobium, Bradyrhizobium, Rhizobium, Ensifer, and Aminobacter). As a consequence, M. loti could be regarded an infrequent symbiont of Lotus, and several plant–bacteria compatibility groups can be envisaged. Despite the great progress achieved with the model L. japonicus in understanding the establishment and functionality of the symbiosis, the genetic and biochemical bases governing the stringent host-bacteria compatibility pairships within the genus Lotus await to be uncovered. Several Lotus spp. are grown for forage, and inoculation with rhizobia is a common practice in various countries. However, the great diversity of the Lotus rhizobia is likely squandered, as only few bacterial strains are used as inoculants for Lotus pastures in very different geographical locations, with a great variety of edaphic and climatic conditions. The agroecological potential of the genus Lotus can not be fully harnessed without acknowledging the great diversity of rhizobia-Lotus interactions, along with a better understanding of the specific plant and bacterial requirements for optimal symbiotic nitrogen fixation under increasingly constrained environmental conditions.
Legumes are remarkable for their ability to establish symbiosis with nitrogen-fixing bacteria known as rhizobia. Rhizobia infect the legume roots and trigger the formation of a new organ, the root nodule, specialized in N2 fixation. This highly energy-consuming process is supported by photosynthetically derived carbohydrate from the plant, and the rhizobia which in return provide the legume with ammonia. Thus, in association with the rhizobia legume plants are self-sufficient in nitrogen, which makes this symbiosis very important in ecological and economic terms.
Lotus is a cosmopolitan genus that includes more than 150 species (annual and perennial plants) with two major centers of diversity, the Mediterranean region (including portions of Europe, Africa, and Western Asia) and Western North America (Howieson et al., 2000; Sokoloff and Lock, 2005). Only a few Lotus species have been domesticated and improved by selection and plant breeding, to be used as forage for livestock, including L. corniculatus L., L. uliginosus Schkuhr. (also denoted L. pedunculatus), L. tenuis Waldst and Kit. (ex L. glaber Mill), L. subbiflorus Lagasca, and more recently L. ornithopodioides (Escaray et al., 2012). In addition, L. japonicus is used as a model for genetic and molecular studies (Márquez et al., 2005; Tabata and Stougaard, 2014).
A relatively small number of Lotus species have been studied regarding their root nodule symbionts, herein called the Lotus rhizobia. The symbiosis between Lotus and nitrogen-fixing rhizobia has been long considered highly specific and only two divergent groups of bacteria were traditionally recognized as the microsymbionts of the Lotus spp., the fast growers and the slow-growers. The “fast-growers” were classified as species Mesorhizobium loti (type strain NZP2213T) (Jarvis et al., 1982, 1997) and considered the typical rhizobial species for the L. corniculatus complex (i.e., L. corniculatus, L. tenuis and the model L. japonicus) (Jarvis et al., 1982; Saeki and Kouchi, 2000; Fulchieri et al., 2001). On another hand, the slow-growers named Bradyrhizobium sp. (Lotus) were typical symbionts for L. uliginosus (pedunculatus), L. subbiflorus and L. angustissimus and, until recently, have remained unclassified at species level.
This rather simple scheme likely derived from the fact that most Lotus rhizobia were initially isolated from agriculturally important Lotus species at few locations, far from centers of diversity (Jensen and Hansen, 1968; Monza et al., 1992; Irisarri et al., 1996; Fulchieri et al., 2001; Bullard et al., 2005). Those early studies of Lotus rhizobia focused on their agronomic potential as N2-fixing inoculants, whereas the recognition of bacterial diversity was not a priority (Monza et al., 1992; Irisarri et al., 1996; Fulchieri et al., 2001). This situation has dramatically changed during the last 15 years, as discussed below.
Many so-called “M. loti” strains show significant differences in whole genomic DNA-DNA hybridization (Crow et al., 1981; de Lajudie et al., 1994) and in the 16S rRNA phylogeny (de Lajudie et al., 1998; Willems et al., 2001), indicating that they could belong to different species. The type strain NZP2213T (deposited in other collections as ATCC 33669T= USDA 3471T = IAM 13588T, LMG 6125T) was isolated from a root nodule of L. corniculatus, but great differences in 16S rRNA gene sequence have been reported for the bacteria deposited in different culture collections (Willems et al., 2001). These discrepancies have been explained by the presence of two different organisms in one of the subcultures, as well as by deficient long-term maintenance (Willems et al., 2001). The type strain ATCC 33669T has been deaccessioned by the ATCC as type strain of M. loti and replaced by strain ATCC 700743T (Figure 1A). Recently two novel species, M. erdmanii and M. jarvisii, have been proposed for the “M. loti” type strains USDA 3471T and ATCC 33669T (Martínez-Hidalgo et al., 2015), which are close relatives of the M. opportunistum and M. huakuii type strains and distant from M. loti NZP2213T (see Figure 1A). In addition to the entanglement with the “type” strains, others so-called “M. loti” strains occupy distant phylogenetic branches, which suggests that they are also misclassified. Examples are strains MAFF303099 and R7A (ICMP 3153), two of the best studied Lotus symbionts. MAFF303099 was re-classified as M. huakuii (Turner et al., 2002; Wang et al., 2014). However, a more recent taxonomic characterization has placed strains MAFF303099 and R7A (ICMP3153) in the novel species M. japonicum (Martínez-Hidalgo et al., 2016). Similarly, other reference “M. loti” strains, like NZP2037 ( = LMG 6124), also show a large phylogenetic distance from the type strain M. loti NZP2213T (Figure 1A), indicating the need of better characterizations and perhaps re-classification.
FIGURE 1. Taxonomy and phylogeny of rhizobial symbionts of Lotus spp. 16S rRNA phylogenetic tree including genera Mesorhizobium and Aminobacter (A), and Bradyrhizobia, Ensifer (Sinorhizobium) and Rhizobium (B). Bold names correspond to Lotus rhizobia, with original Lotus species and country of isolation indicated. In bold green are rhizobial species which have Lotus symbionts as type strains. Analyses were conducted with MEGA using Neighbor Joining Maximum Likelihood (NJML). A total of 1224 positions were used in the final dataset. La, L. arinagensis; Lar, L. arabicus; Lb, L. berthelotii; Lc, L. corniculatus; Lca, L. campylocladus; Lcr, L. creticus; Lf, L. frondosus; Lk, L. kunkelii; Lj, L. japonicus; Ll, L. lancerottensis; Lm, L. maritimus; Lma, L. maculatus; Lp, L. pyranthus. Lr, L. roudairei; Ls, L. sessilifolius ; Lt, L. tenuis (L. glaber); Lu, L. uliginosus (L. pedunculatus). Arg, Argentina; Aus, Australia; Bel, Belgium; CaI, Canary Islands; Chn, China; Esp, Spain; Ger, Germany; Jap, Japan; Nor, Norway; Nwz, New Zealand; Por, Portugal; Sen, Senegal; Swe, Sweden; Tun, Tunisia; Uru, Uruguay. Accessions numbers for all sequences used in this review are listed in Supplementary Table S1.
Several studies of rhizobia nodulating L. corniculatus from different locations and habitats in Europe and in South America have shown that most of the isolates falled within genus Mesorhizobium, but an unexpectedly great diversity of species, different to M. loti, was observed.
Lotus corniculatus, L. maritimus and L. tenuis nodule isolates from saline soils of Granada (Southeast Spain) were related to different species of the genus Mesorhizobium, such as M. tarimense/M. tianshanense, M. chacoense/M. albiziae and M. alhagi (Lorite et al., 2010b; see Figure 1A). Some of these rhizobia have been recently included in the new species M. olivaresii (Lorite et al., 2016). No M. loti-like bacteria were found in that study. A great genetic diversity has also been observed among strains nodulating L. corniculatus from Northwest Spain. These were distributed in five divergent phylogenetic groups within the genus Mesorhizobium, two of them related to M. jarvisii and M. erdmanii but the rest were phylogenetically divergent to the currently described Mesorhizobium species, and none was related with the type strain M. loti NZP2213T (Marcos-García et al., 2015). Some of these isolates have been classified in the new species M. helmanticense (Marcos-García et al., 2017). On the contrary, most of the L. corniculatus nodule isolates from Norway and Sweden soils were initially grouped with M. loti NZP2213T, whereas only a minority of isolates were related to other species: M. huakuii, M. tianshanense, M. plurifarium, M. amorphae and M. septentrionale (Ampomah and Huss-Danell, 2011; Gossmann et al., 2012). However, this phylogenetic analysis did not include several Mesorhizobium species. We have observed that should M. qingshengii, M. ciceri/M. canturiense, M. australicum and M. shangrilense have been included in the 16S rRNA phylogenetic tree, most of the Scandinavian isolates would cluster closer to these later species than to M. loti NZP2213T (see Figure 1A). This indicates that further studies are needed to assess the phylogenetic relationships of the Scandinavian strains. Many isolates from L. corniculatus wild plants in Belgium and Norway (De Meyer et al., 2011; Gossmann et al., 2012) grouped with Mesorhizobium, whereas others were close to various species in the Rhizobium genus. However host specificity of the Belgian strains was not tested, and Norwegian isolates failed to induce nodules in their original host, with the exception of R. leguminosarum 10.2.1 (Rl Norway), which induced nodule organogenesis in certain Lotus species (Gossmann et al., 2012). Diverse non-nodulating bacteria have been frequently isolated from nodules of L. corniculatus [e.g., Burkholderia spp. (Gossmann et al., 2012) or the recently described species Phyllobacterium loti S658T (Sánchez et al., 2014)]. None of these bacteria were able to nodulate, although many could be recovered from nodules if co-inoculated along a truly nodulating partner, indicating their hitchhiker nature. Other non-rhizobium Gram-positive bacteria were isolated from L. corniculatus in Sweden such as Rhodococcus spp. and Geobacillus spp., and a symbiotic phenotype in their original host and nodC gene amplification was obtained (Ampomah and Huss-Danell, 2011). Nevertheless, these results should be confirmed. As mentioned above, several reports have shown the presence of non-nodulating bacteria inside the nodules (Gossmann et al., 2012; Sánchez et al., 2014), and the possibility exists, even following the appropriate methodology, to carry over the non-nodulating bacteria mixed with nodulating ones, especially when the companion has a duplication time significantly shorter than the true rhizobia (Sánchez et al., 2014).
A great majority of the isolates from L. corniculatus in Uruguay (Sotelo et al., 2011) were described as close relatives of species M. huakuii, and the rest were closely related to either M. septentrionale or M. caraganae (Figure 1A). Again, no M. loti-like strains were isolated in that study. Among 103 root nodule isolates from L. tenuis trap plants grown in soils of the Salado River Basin (Argentina), a majority were related to genus Mesorhizobium: only 2 isolates seemed closely related to M. loti NZP2213T, and the rest were more related to M. amorphae, M. mediterraneum, M. tianshanense or to strain NZP2037 (Estrella et al., 2009; see Figure 1A). Some of those have been classified in the new species M. sanjuanii (Sannazzaro et al., 2018). A large portion of the isolates were grouped within genus Rhizobium, close to typical common bean symbionts R. gallicum, R. etli, and R. tropici (Figure 1B). Also in that study (Estrella et al., 2009), a member of genus Aminobacter with symbiotic capacity was reported for the first time (BA135, Figure 1A).
Species like L. uliginosus, L. subbiflorus or L. angustissimus have been known to be nodulated by slow-growing bradyrhizobia (Cooper et al., 1985; Irisarri et al., 1996). Evidence suggests that the symbionts of Lotus heermannii, L. micranthus, and L. strigosus are also bradyrhizobia (Sachs et al., 2009). But only a few recent reports have studied genetic diversity and phylogenetic relationships of isolates from nodules of L. uliginosus, collected from cultivated fields in countries like Uruguay (Batista et al., 2013), or from wild plants in various European countries, such as Portugal (Lorite et al., 2012), Belgium (De Meyer et al., 2011) and Germany (Gossmann et al., 2012). These studies supported affiliation of these bacteria to genus Bradyrhizobium, in some cases to species B. japonicum (Figure 1B). The data indicate a more reduced biodiversity and wider geographical distribution than the L. corniculatus rhizobia. Exceptionally, strain Lulit12 related phyllogeneticaly to the broad host range Mesorhizobium sp. NZP2037 was isolated from L. pedunculatus in Uruguay (Batista et al., 2013). Also a non-bradyrhizobial strain belonging to the genus Curtobacterium, has been isolated from L. pedunculatus in Germany but reinoculation on the original host was not done (Gossmann et al., 2012).
The characterization of symbionts from wild Lotus species in different sites/countries has revealed additional diversity. The root nodule bacteria from a Mexican population of Lotus oroboides showed a great diversity of mesorhizobial strains that were related to M. loti, M. amorphae, M. plurifarium and M. huakuii (Qian and Parker, 2002). The nodule bacteria from Lotus arabicus, L. argenteus, L. creticus, L. pusillus and L. roudairei in Tunisian soils spread over four genera, Mesorhizobium, Bradyrhizobium, Ensifer ( = Sinorhizobium) and Rhizobium (Zakhia et al., 2004; Rejili et al., 2009, 2012). The bradyrhizobial strains from L. creticus were close to B. yuanmingense (Zakhia et al., 2004). Furthermore, some sinorhizobia from L. creticus (Figure 1B) were affiliated to the novel species Ensifer numidicus (Merabet et al., 2010).
Two novel mesorhizobial species, M. tarimense and M. gobiense were described amongst the root nodule rhizobia of L. frondosus and L. tenuis in soils of Xinjiang, China (Figure 1A). These bacteria are able to nodulate also L. corniculatus as well as other non-Lotus species like Glycyrrhiza uralensis, Oxytropis glabra, and Robinia pseudoacacia (Han et al., 2008a). No M. loti strains were isolated either from this region of China. Although R. multihospitium is associated with a quite broad range of legume species within ten genera, including the Lotus species L. tenuis and L. frondosus, nodulation of these hosts could not verified after reinoculation (Han et al., 2008b). On the other hand, the rhizobia from Lotus species endemic to the Canary Islands (L. lancerottensis, L. kunkelii, L. arinagensis, L. pyranthus, L. berthelotii, L. calli-viridis and L. campylocladus) were affiliated with Mesorhizobium (Figure 1A), Ensifer (Figure 1B) or Rhizobium/Agrobacterium (Figure 1B) (Lorite et al., 2010a). The mesorhizobial strains were close to M. plurifarium, M. caraganae, M. ciceri, M. alhagi and the novel species M. tamadayense, isolated from L. berthelotii and Anagyris latifolia (Ramírez-Bahena et al., 2012). Neither M. loti nor Bradyrhizobium were identified among the Canarian Lotus isolates. Moreover, Ensifer meliloti, the typical species nodulating alfalfa, was described for the first time as a Lotus symbiont, representing the new symbiovar lancerottense (León-Barrios et al., 2009) (Figure 1B). The new species Phyllobacterium salinisoli was also isolated from a root nodule of L. lancerottensis, however, reinfection was unsuccessful and PCR amplification of nodC gene failed (León-Barrios et al., 2018).
In summary, the characterization of symbionts from worldwide geographical locations and from unexplored Lotus spp. have revealed an extraordinary diversity amongst the Lotus symbionts, overwhelmingly wider than the simple taxonomic scheme M. loti/Bradyrhizobium sp. which has been acknowledged for many years and is still assumed in many laboratories. Current data support that the Lotus rhizobia are dispersed among five genera: Mesorhizobium, Bradyrhizobium, Rhizobium, Ensifer, and Aminobacter. Bradyrhizobium spp. are the characteristic symbionts for species like L. uliginosus, L. subbiflorus, L. angustissimus and likely a few other Lotus spp. Some of these Lotus bradyrhizobia are related to B. japonicum or B. yuanmingense, although detailed taxonomic characterization await in most cases. The importance of genus Rhizobium as true symbionts of Lotus remains to be clarified since re-infections or presence of nodulation genes have either not been confirmed or failed in most cases (Zakhia et al., 2004; Han et al., 2008b; Estrella et al., 2009; Lorite et al., 2010a). Hitherto only few strains from Argentina and one isolate of R. leguminosarum (Estrella et al., 2009; Gossmann et al., 2012) have been proved as true Lotus symbionts. Genus Ensifer has been frequently isolated from wild Lotus, and it could be the predominant microsymbiont in arid regions (Zakhia et al., 2004; León-Barrios et al., 2009; Rejili et al., 2009, 2012; Lorite et al., 2010a; Merabet et al., 2010).
Rhizobia from the L. corniculatus/L. japonicus complex usually belong to Mesorhizobium genus. In addition to the initial M. loti (Saeki and Kouchi, 2000; Saeki and Ronson, 2014), new mesorhizobial species have been defined to accomodate Lotus rhizobia, such as M. jarvisii, M. erdmanii, M. japonicum, M. olivaresii and M. helmanticense (from L. corniculatus), M. tarimense and M. gobiense (from L. frondosus and L. tenuis), M. sanjuanii (from L. tenuis) and M. tamadayense (from L. berthelotii). Furthermore, several Lotus rhizobia representing distinct lineages await detailed characterization and new species will likely be described in the future. Lotus symbionts belonging or related to other previously described mesorhizobial species have been also reported: M. huakuii, M. amorphae, M. mediterraneum, M. tianshanense, M. alhagi, M. ciceri, M. plurifarium and M. caraganae. Also important, M. loti appears an infrequent Lotus symbiont, as it seems absent from many diverse soils and locations. As a consequence M. loti should no longer be considered as the preferred Lotus symbiont.
Measured with the old and simplistic rhizobial taxonomic scheme, two Lotus symbiotic compatibility groups have been traditionally described: the group I included species such as L. corniculatus, L. tenuis or L. japonicus which could establish effective nitrogen-fixing symbiosis with the so-called “M. loti” and other mesorhizobial species; and Lotus group II, including L. uliginosus, L. subbiflorus, and L. angustissimus, effectively nodulated by bradyrhizobia. Efficient bacteria in one compatibility group were usually non-nodulating, non-infective, inefficient or poorly efficient in the other group, with the exception of a few broad-host-range strains like Mesorhizobium sp. NZP2037, which could form moderately effective nitrogen-fixing nodules with both group I and II Lotus species (Pankhurst et al., 1987; Monza et al., 1992; Saeki and Kouchi, 2000; Lorite et al., 2010a). Supporting this scheme is the fact that no bradyrhizobia have been isolated from L. corniculatus, L. tenuis or other group I species (Han et al., 2008a; Estrella et al., 2009; Lorite et al., 2010a,b; De Meyer et al., 2011; Gossmann et al., 2012; Batista et al., 2013).
There has also been significant confusion in the description of the symbiotic phenotypes resulting from certain plant-bacteria combinations. For instance, Pankhurst (1970) reported that L. corniculatus mature nodules, induced either by strain Bradyrhizobium sp. NZP2309, M. loti NZP2213T or the broad host range Mesorhizobium sp. NZP2037, were all externally similar and contained infected cells, despite Bradyrhizobium sp. NZP2309 being inefficient or partially efficient in L. corniculatus (Lorite et al., 2012; Gossmann et al., 2012). In a more recent report, L. japonicus inoculated with Bradyrhizobium sp. NZP2309 produced delayed and poorly efficient nitrogen-fixing nodules, which had few intact symbiosomes and senesced early (Bek et al., 2010). In contrast, strains of genus Mesorhizobium (NZP2213T, R7A, etc.) inoculated on L. uliginosus (and also on L. angustissimus and L. subbiflorus) formed supernumerary small and uninfected root structures that in some occasions were called nodules (and thus were assigned a Nod+Fix- phenotype; Irisarri et al., 1996; Díaz et al., 2005), whereas other authors called such formations outgrowths, pseudonodules, or tumor-like structures (and therefore were qualified as Nod-; Pankhurst, 1970; Bek et al., 2010). Before going further we should make a clear distinction between such structures, and propose to name pseudonodules to structures (tumor-like, bumps, outgrowths, etc.) which are induced but not invaded by the bacterium and therefore do not contain bacteria or bacteroids inside. In contrast, a nodule should always contain invading bacteria, which may or may not differentiate into nitrogen-fixing bacteroids, and therefore may be Fix+ or Fix-. Pseudonodules should be also distinguished from the so-called spontaneous nodules that may appear in some legumes in the absence of rhizobia (Truchet et al., 1989).
The increasing number of Lotus species studied and the description of additional bacterial species and genera as natural symbionts of Lotus spp. has opened a wider and more complex scenario than the classical two Lotus symbiotic compatibility groups. For instance, a likely third compatibility group can be envisaged which would comprise Lotus species endemic to the Canary Islands, like L. lancerottensis, L. sessilifolius, and L. maculatus. This group is efficiently nodulated by fast-growing strains of E. meliloti sv. lancerottense which are unable to fix nitrogen with either of the above Lotus groups I or II (León-Barrios et al., 2009; Lorite et al., 2010b). Mesorhizobia isolates from L. arinagensis and L. kunkelii in the Canary Islands seem incompatible with both L. corniculatus and L. uliginosus, but also with L. lancerottensis (Lorite et al., 2010b) and perhaps represent another incompatibility group. Also mesorhizobia strains isolated from L. oroboides in Mexico failed to nodulate L. corniculatus although unfortunately no other Lotus were tested (Qian and Parker, 2002). Neither of the Australian commercial inoculant strains for L. uliginosus (CC829) and L. corniculatus (SU343) can fix nitrogen with the promising forage species L. ornithopodioides (Howieson et al., 2011). Indeed, these authors found no strains compatible with all the 15 Lotus species evaluated. Attention should be paid to the possibility that some of the above mentioned bacteria-host incompatibilities be actually due to genotype-specific, rather than species-specific, host–rhizobia interactions. For instance, varying levels of nitrogen-fixing efficiency in their original hosts have been observed amongst rhizobia from L. subbiflorus and L. uliginosus in Uruguay and Portugal, respectively (Irisarri et al., 1996; Lorite et al., 2012). Similar results were observed amongst L. corniculatus rhizobia from Spain and Norway, ranging from highly efficient to non-nodulating, depending on the plant cultivar used (Lorite et al., 2010b; Gossmann et al., 2012). Thus, we recommend that more than one plant genotype be tested before claiming that a given bacterium is not compatible with a particular Lotus host.
The existence of incompatibility groups within the genus Lotus, together with other reasons like the consideration by several authors of L. corniculatus as an archetype Lotus species, have created additional confusion about the relationships of Lotus rhizobia with the traditional cross-inoculation groups. Isolates from L. uliginosus were shown to fix nitrogen with Ornithopus sativus but were inefficient on Astragalus glycyphyllus (Jensen, 1967). Rhizobia from Lupinus luteus were found to induce efficient nodules on L. uliginosus (Jensen and Hansen, 1968). In contrast, the commercial strain NZP2309 and other L. uliginosus isolates from Portugal formed inefficient nodules on L. luteus (Lorite et al., 2012). In addition, NZP2309 and several Uruguayan bradyrhizobia isolated from L. uliginosus nodules were efficient or partially efficient with Spartium junceun (Batista et al., 2013). As detailed below, the Portuguese and Uruguayan bradyrhizobia were shown to carry symbiotic genes closely related to the symbiovar genistearum, where Spartium, Genista, Ornithopus, and Lupinus rhizobia are included (Vinuesa et al., 2005; Lorite et al., 2012; Batista et al., 2013).
On the other hand, L. corniculatus rhizobia fixed nitrogen efficiently with Anthyllis vulneraria, but inefficiently with A. glycyphyllus and O. sativus (Jensen, 1967). In addition, certain rhizobia from Lupinus densiflorus were effective in L. corniculatus and A. vulneraria and ineffective in O. sativus and L. uliginosus (Jensen, 1967), while A. vulneraria and A. glycyphyllus rhizobia were efficient on L. corniculatus but inefficient on L. uliginosus and O. sativus (Jensen and Hansen, 1968). Furthermore the M. loti NZP2213T was unable to nodulate Leucaena leucocephala, Carmichaelia flageliformis, O. sativus, and Clianthus puniceus (Pankhurst et al., 1987).
Besides the bona fide symbionts which have been originally isolated from a Lotus spp., a number of other rhizobia have been shown to form nodules (sometimes nitrogen-fixing) with certain Lotus spp. The P. vulgaris symbiont R. etli CE3 is able to induce nodulation in L. japonicus, although these nodules could not sustain nitrogen fixation and senesced early (Cárdenas et al., 1995; Banba et al., 2001). Several broad-host range rhizobia such as Ensifer fredii NGR234, R. tropici CIAT 899 and R. leucaenae CFN299 are able of inducing low or moderately efficient nodules in L. japoniucs and/or L. corniculatus (Hernández-Lucas et al., 1995; Schumpp et al., 2009). In contrast to NGR234, another S. fredii strain HH103 can form nitrogen-fixing nodules on L. burtii but forms only small uninfected outgrows in L. japonicus Gifu (Sandal et al., 2012). Broad host-range strains have been also isolated from Lotus, i.e., Mesorhizobium sp. NZP2037 which is able to fix nitrogen on its natural host L. divaricatus as well as L. japonicus, L. corniculatus and L. uliginosus, but also on L. leucocephala, C. flageliformis, O. sativus and Clianthus puniceus (Pankhurst et al., 1987; Scott et al., 1987).
Genetic variability plays an important role in the evolution of symbiotic interactions and the horizontal gene transfer (HGT) represents a powerful mechanism for the continuous evolution of host-microorganism relationships. Genes that transfer horizontally can be found in a variety of mobile genetic elements (MGE) such as plasmids, transposons, bacteriophages, integrons, insertion sequences and genomic islands. Through some of the aforementioned elements, HGT is responsible for the great diversity of rhizobial symbionts, currently distributed in more than one hundred species in some 14 genera of two bacterial classes, α- and β-proteobacteria (Peix et al., 2015). In all cases examined symbiotic genes have been found in the so-called accessory genome, associated with MGE such as the large plasmids preferentially found in genera like Rhizobium or Ensifer, or chromosomal symbiosis islands common in mesorhizobia and bradyrhizobia.
Horizontal gene transfer can be inferred from phylogenies of symbiotic genes, but has also been observed in the field. Sullivan et al. (1995), after introducing L. corniculatus inoculated with the M. japonicum R7A in a New Zealand grassland with no history of Lotus cultivation, detected the spread of the inoculant’s symbiosis genes into several indigenous bacterial species which were thus converted into symbionts. These authors were the first to demonstrate that non-symbiotic mesorhizobia can evolve into symbionts in a single step, by acquisition of a 500-kb MGE that was called “symbiosis island” in analogy to the pathogenicity islands of bacterial pathogens (Sullivan and Ronson, 1998). In addition to strain R7A (502 kb; Ronson et al., 2002), two other symbiosis islands have been sequenced from Lotus mesorhizobia: a 611-kb symbiosis island was revealed in the chromosome of strain MAFF303099 (Kaneko et al., 2000), and more recently the symbiosis island of the broad host range strain NZP2037, isolated from L. divaricatus (533 kb; Kasai-Maita et al., 2013). Interestingly, the core regions of the three MGEs involved only 165 of the more than 500 genes carried by each island (Kasai-Maita et al., 2013). One of the more striking differences is the presence of a type-four secretion system (T4SS) in R7A and NZP2037 instead of the T3SS of MAFF303099; nevertheless it seems that both T3SS and T4SS are involved in nodulation efficiency and symbiotic host range, and could be therefore considered functionally analogous (see below).
Besides the pioneer studies of C. Ronson’s Laboratory (Ramsay and Ronson, 2015), phylogenetic evidence suggest that the symbiosis island is frequently exchanged among Mesorhizobium species, as symbiotic genes are highly conserved in different species of this genus (see below Figure 2). Bradyrhizobial symbionts of the L. pedunculatus complex species are also presumed to carry symbiosis islands, although this extreme has not been confirmed yet. Since different species carry highly conserved nod and nif genes (Lorite et al., 2012; Batista et al., 2013) it can be suspected that HGT is playing also a role in the preferential spread of the symbiosis genes within the genus Bradyrhizobium.
FIGURE 2. Phylogenetic tree of nodC gene sequences. Bold names correspond to Lotus rhizobia, with original Lotus species and country of isolation indicated. In bold green are rhizobial species which have Lotus symbionts as type strains. Previously defined symbiovars are indicated. Strains with unconfirmed symbiotic phenotypes in Lotus are indicated with an arrow. Analyses were conducted with MEGA using Neighbor Joining Maximum Likelihood (NJML), for a total of 203 positions. Abbreviations and accesions numbers are as in Figure 1.
Strains MAFF303099 and R7A induce efficient nitrogen-fixing nodules in L. japonicus and L. corniculatus and their symbiosis islands share conserved backbone sequences of 248 kb, with 98% of nucleotide sequence identity (Sullivan et al., 2002). Rhizobia harboring symbiotic sequences that cluster with MAFF303099 and R7A are referred to as symbiovar loti (Turner et al., 2002; Rogel et al., 2011). To this symbiovar belong most of the mesorhizobia isolated from L. corniculatus and related species (e.g., L. tenuis, L. japonicus, L. frondosus, L. maritimus, L. oroboides), including the type strain M. loti NZP2213T, which shares above 98% of symbiotic sequences with MAFF303099 and R7A (Figure 2 and Supplementary Figure S1). The data suggest that symbiovar loti is widely extended among the symbionts of L. corniculatus, L. japonicus and related species, supporting that the loti symbiotic island is frequently exchanged among Mesorhizobium species. It is unclear whether transfer and establishment of the symbiosis island is more efficient among species of this genus or whether mesorhizobia are preferred symbionts for the L. corniculatus group. Nevertheless, bacteria from other genera may carry the symbiovar loti symbiotic genes, as it was reported for a true nodulating Aminobacter isolate (Estrella et al., 2009), indicating that transfer is a frequent event that enable diverse bacteria with symbiotic traits and other adaptive functions to become efficient symbionts for this group of Lotus spp.
On the other hand, Mesorhizobium sp. NZP2037, another L. corniculatus and L. japonicus symbiont which has a nodC sequence with < 93% similarity to those of the symbiovar loti clade, occupied a distinctive cluster (branch A4, Figure 2). This strain also shows a broader host range, so it likely represents a symbiovar different from loti. This may also be the case for other branches (i.e., branch A2, Figure 2) that include strains unable to nodulate or which formed poorly efficient nodules in L. corniculatus, such as strains LKU3 and LAR8, two isolates from L. kunkeli and L. arinagensis (Lorite et al., 2010a), strain CMSS27 from L. corniculatus (Lorite et al., 2010b), as well as the one formed by GGS20, CPS12 and GAC133P, isolated from L. tenuis, L. corniculatus and L. maritimus respectively (branch A3, Figure 2). Additional studies are necessary to establish the host range of these bacteria and whether they could represent distinctive symbiovars.
The Lotus bradyrhizobial symbiotic genes seem to have a quite different phylogenetic history. Less variability is found among the slow-growing rhizobia, although nodC (Figure 2) and nifH (Supplementary Figure S1) sequences spread in either of two clusters closely related to the symbiovar genistearum (Gossmann et al., 2012; Lorite et al., 2012; Batista et al., 2013). The larger cluster (B1) could be a new symbiovar specific of the Lotus bradyrhizobia (Figure 2).
A different picture is provided by several Lotus rhizobial populations isolated from saline soils in Africa and the nearby Canary Islands. The nodulation genes of a group of Ensifer meliloti isolated from various Lotus spp. in the Canaries had the closest nodC phylogenetic relatives in S. fredii NGR234 and USDA 257. This together with a distinctive nifH genotype and specific host range defined the symbiovar lancerottense (León-Barrios et al., 2009), appreciably divergent from symbiovars loti or genistearum (Figure 2). The nod gene sequences of Ensifer isolates from L. arabicus in Senegal, and from L. creticus and L. roundairei in Tunisia were placed within different symbiovars among the Ensifer lineages, but distantly from other Lotus isolates. It is worth noting that the Lotus sinorhizobia have all been isolated from arid areas where drought, high temperatures and salinity are frequent abiotic stresses. Also related to symbiovars meliloti, trifolii and viciae seem some Lotus isolates from China (Han et al., 2010) (Figure 2), as well as strain R-46130 isolated from L. corniculatus which had a nodC gene sequence close to symbiovar meliloti albeit no symbiotic phenotype was verified (De Meyer et al., 2011). Care must be paid before assigning unverified symbiotic phenotypes to bacteria isolated from nodules, even if they seem to carry nodulation genes. Despite carrying nod genes related to bv. viciae and producing Nod factors recognized by L. japonicus at the molecular level, several R. leguminosarum isolates from L. corniculatus were unable to induce nodulation in most Lotus spp. and only formed non-fixing nodules in L. burttii (Gossmann et al., 2012), suggesting that these bacteria are not compatible symbionts of any Lotus spp.
Effective nodule formation results from the expression of the host legume-bacteria compatibility through a complex process involving the exchange of specific signals between plant and bacteria (Dénarié et al., 1996; Radutoiu et al., 2003). The recognition between both symbionts initiates with the perception of specific plant flavonoids that attract the rhizobia to the root and activate expression of the nodulation genes. The specificity of this initial stage is mediated by the protein NodD, a LysR-type transcriptional activator of genes (nod, nol, noe) necessary for synthesis and export of the Nodulation Factors (NF) (Caetano-Anollés and Gresshoff, 1991; Dénarié et al., 1996; Spaink, 2000). Chemical structure of the NF consists of an oligomeric backbone of β-(1,4) linked N-acetyl-D-glucosaminyl (GlucNAc) residues, with a fatty acyl group attached to the nitrogen of the non-reducing saccharide (Spaink, 2000). Given the similarity of the oligosaccharide backbone to chitin fragments, NF are often called lipo-chitin oligosaccharides (LCOs) (Spaink, 2000). Rhizobia appear to produce complex mixtures of LCOs, which differ in the number of GlucNAc residues, the nature of the fatty acyl group as well as the substituents at the non-reducing- and/or reducing-terminal residues.
Nodulation factors mediate nodule induction by all rhizobia except some NF-independent Bradyrhizobium strains that initiate the symbiosis by an alternative mechanism (Giraud et al., 2007). Infection via intracellular root hair infection threads (IT) is the common way of infection in Lotus spp. However, a crack entry based mode of entry that involves Nod factor perception in the root cortex has also been described in some Lotus spp. (James and Sprent, 1999; Madsen et al., 2010; Acosta-Jurado et al., 2016). Moreover, a NF-independent and IT-independent single cell infection mechanism has also been reported in certain L. japonicus mutants (Madsen et al., 2010).
The symbiosis between rhizobia and the Lotus has been traditionally considered highly specific, as discussed above. The chemical structures and biological activities of the NFs produced by several mesorhizobial Lotus symbionts have been described (López-Lara et al., 1995; Olsthoorn et al., 1998; Niwa et al., 2001), as well as those by a bradyrhizobial strain (NZP2039; Bek et al., 2010). It must be mentioned that the identities of the Lotus nod-inducing compounds are yet unknown. The recent discovery of a differential activation of nod genes by the transcriptional regulators NodD1 and NodD2 suggest that plant-derived inducing compounds can vary upon specific infection stages (Kelly et al., 2018). So far, NF produced by Lotus rhizobia have been identified in NodD-modified strains that recognize heterologous flavonoids. Various mesorhizobial strains, all symbionts of L. corniculatus/L. japonicus, produce different mixtures of LCOs where the common component is a GlucNAc pentasaccharide in which the non-reducing terminal residue is modified with a C18:1 (vaccenic) fatty acid, N-methylated and carbamoylated, whereas the reducing end is substituted with 4-O-acetyl-fucose (López-Lara et al., 1995; Olsthoorn et al., 1998; Niwa et al., 2001; Bek et al., 2010). In contrast, the LCOs produced by strain NZP2037 bear an additional carbamoyl group on the non-reducing terminal GlucNAc residue (López-Lara et al., 1995). Lack of carbamoylation of the NF had no effect in nodulation (Rodpothong et al., 2009). In contrast, R7A nodZ and nodL mutants, producing NF without the acetylfucose on the reducing end displayed a host-specific phenotype, forming uninfected nodule primordia in L. filicaulis and L. corniculatus, but effective nodules in L. japonicus (Rodpothong et al., 2009). More recently, Bek et al. (2010), also making use of a naringenin-inducible NodD activator system used by other authors, identified the structure of Nod Factors produced by Bradyrhizobium sp. NZP2309, an effective symbiont of L. uliginosus, and also determined more precisely the position of the decorations of the NF produced by strain R7A. The analysis revealed closely related but not identical structures for the major types of LCOs produced by the mesorhizobial strain R7A and the Bradyrhizobium sp. NZP2309. The main difference was the presence of two carbamoyl groups in the non-reducing GlucNAc of the NZP2039 NF, instead of only one in the mesorhizobial compounds. NZP2309 NF would be similar to those of the broad host range strain NZP2037, further supporting that carbamoylation at the non-reducing end of NF is not related with host range. Indeed, both NF types seem to be similarly recognized by either L. japonicus or L. uliginosus, indicating that NF perception is not responsible for the known symbiotic incompatibilities. Thus, additional components are likely necessary for infection of nodule primordia and progression of symbiotic establishment (Bek et al., 2010).
Legumes inoculated with compatible rhizobia form, in response to rhizobial Nod factors, nodule primordia in the root cortex which are targeted for bacterial infection. Infection of most legumes is initiated when bacteria become entrapped by a curled root hair. Local cell wall hydrolysis and invagination of the plant plasma membrane lead to formation of tubular structures, the IT. ITs are colonized by dividing bacteria and proceed through the epidermal cell layer into the root cortex before ramifying in the underlying nodule primordia. Rhizobia are released from ITs into plant cells and enclosed in membrane-delimited compartments called symbiosomes.
Bacterial cell surface components and particularly exopolysaccharides (EPSs) play important roles during root nodule infection, although their precise functions have not been yet resolved. EPSs have been proposed to play active roles as signals to mitigate plant defense responses, but also to protect bacteria against harshness imposed by changing osmotic conditions or against host-derived reactive oxygen species (Niehaus et al., 1993; Parniske et al., 1994; Wielbo et al., 2004). Rhizobial EPS are species- or strain-specific, homo- or heteropolysaccharides which are secreted into the external environment, or retained at the bacterial surface as capsular polysaccharides (CPS). A large diversity of EPS chemical structures can be found amongst rhizobia, regarding sugar composition, linkages, repeating unit size and degree of polymerization, as well as non-carbohydrate substitutions (Downie, 2010). The exo genes responsible for the production, polymerization, and export of EPS in most rhizobial species are clustered in plasmids, although in Lotus symbionts these genes are chromosomal (Kaneko et al., 2000).
The importance of EPS for the infection process has been well established in the S. meliloti-alfalfa and R. leguminosarum-pea symbioses, where indeterminate-type nodules are formed. EPS-defective bacterial mutants are impaired in IT formation and elongation, resulting in the formation of uninfected pseudonodules (Pellock et al., 2000; Stuurman et al., 2000; Laus et al., 2004, 2005). The addition of low amounts of purified wild-type EPS often complement and rescue the infective capacity in EPS-deficient mutants, suggesting that EPS are signaling molecules rather than providing a structural role during IT development (Djordjevic et al., 1987; Battisti et al., 1992; Urzainqui and Walker, 1992).
Unlike the vast amount of information concerning EPS function in indeterminate nodules, the role of EPS in legumes that form determinate nodules, such as Lotus spp, has been elusive. The first studies carried out with EPS-deficient mutants of the broad host range strain NZP2037, suggested that EPS is not required for infection of determinate nodules because these mutants still formed effective nodules on the compatible host L. pedunculatus but failed to elicit nodules with the indeterminate host Leucaena leucocephala (Hotter and Scott, 1991). Likewise, Tn5 mutagenesis of MAF303099 revealed that 32 mutants in EPS synthesis have a symbiotic phenotype indistinguishable of the wild-type on L. japonicus (Mishima et al., 2008). The dispensability of EPS to establish symbiosis with L. japonicus resembled other rhizobial species such as R. etli (Borthakur et al., 1986; Diebold and Noel, 1989), S. fredii (Kim et al., 1989) and B. japonicum (Law et al., 1982), infecting legumes with determinate nodules. These reports again supported the idea that EPS are essential to infect indeterminate hosts but not for determinate nodule hosts. However, some questions arose from the delayed nodulation phenotype or the severe symbiotic defects shown by some EPS mutants of B. japonicum on soybean (Parniske et al., 1993; Becker et al., 1998). More recently, Kelly et al. (2013) have characterized EPS from R7A and isolated Tn5 mutants affected in various steps of EPS biosynthesis, evaluating their symbiotic phenotypes on two Lotus species. Chemical analysis has revealed that R7A produces an O-acetylated acidic EPS which has an octosaccharide repeating unit composed of glucose, galactose, glucuronic acid and riburonic acid residues (Kelly et al., 2013; Muszyński et al., 2016). Mutants affected in early biosynthetic steps (exoA and exoB) formed nitrogen-fixing nodules on L. corniculatus and L. japonicus ‘Gifu,’ whereas mutants affected in mid or late biosynthetic steps (exoO, exoU, exoK, mlr5265, and mlr5266) were impaired on both hosts, with the severity of the symbiotic defect depending on the particular mutant. The exoU, exoO, and mlr5265 mutants exhibited the most severe symbiotic defects and induced the formation of small, uninfected nodule primordia. These mutants were disrupted at the stage of IT development (Kelly et al., 2013). This phenotype resembled that of EPS mutants of S. meliloti and R. leguminosarum which are impaired in their symbiosis with indeterminate nodule-forming hosts. Kelly et al. (2013) suggested that full-length wild-type EPS acts as a positive signal modulating plant defense responses and allowing IT development. In contrast, truncated EPS would be perceived by the host as a negative signal inducing defense reactions and blocking IT development. Strains like exoB mutants which produce no EPS, would somehow avoid plant surveillance and form infected nodules, albeit with lower efficiency than the wild-type strain (Kelly et al., 2013). More recently, J. Stougaard and co-workers have identified the L. japonicus EPS receptor EPR3, involved in monitoring the EPS status of rhizobia during infection (Kawaharada et al., 2015). EPR3 can bind monomeric EPS and would be able to distinguish between EPS variants to act positively (toward sustained infection) in response to compatible EPS, or negatively in the presence of incompatible EPS. The finding that induction of Epr3 gene expression is dependent on Nod Factors and that EPR3 does not affect NF-mediated signaling, provides strong support to the hypothesis that rhizobial access to legume roots is controlled by a two-step mechanism (Kawaharada et al., 2015). Future studies shall reveal whether different Lotus spp. possess distinct Epr3 alleles and if recognition of EPS by EPR3 is involved in Lotus-rhizobia compatibility. The characterization of EPS produced by bradyrhizobial strains like NZP2309 should certainly help to this aim.
Many gram negative bacteria use the type III (T3SS) or type IV (T4SS) protein secretion systems to invade host cells. Experimental evidence from different microorganisms showed that both systems are involved in protein translocation and modulation of the host defenses (Chisholm et al., 2006; Desveaux et al., 2007).
Several rhizobia such as B. japonicum USDA110, S. fredii NGR234 and other S. fredii strains, use T3SS to secrete effectors and infect host cells. Among Lotus symbionts, it was observed that strain MAF303099 possesses a T3SS whereas strain R7A contains a T4SS (Sullivan et al., 2002; Hubber et al., 2004). Interestingly, MAFF303099 seems to have had a T4SS in the past but lost it by deletion and gained a T3SS at a different location within its symbotic island (Sullivan et al., 2002). Hubber et al. (2004) showed that a T4SS seems the most common feature amongst Lotus mesorhizobia, albeit T3SS and T4SS would be functionally exchangeable. The expression of rhizobial T3SS components and secreted effectors (Nops) are usually induced by flavonoids via the TtsI transcriptional activator, whose expression is regulated by NodD (Krause et al., 2002; Marie et al., 2004). Studies by Sánchez et al. (2012) revealed that MAFF303099 has a functional T3SS which is probably regulated by flavonoids. Hubber et al. (2007) also evidenced that R7A vir genes are subject to a two-tiered regulatory cascade in response to symbiotic signals, likely plant flavonoids, first through NodD via the nod box upstream of virA and then through VirA/VirG two-component regulatory system. Genetic synteny suggests a similar regulation of T4SS in the broad host range strain NZP2037 (Kasai-Maita et al., 2013).
Several candidate T3SS effector proteins have been identified in rhizobia: NopD, NopL, NopM, NopP, and NopT (Bartsev et al., 2004; Skorpil et al., 2005; Rodrigues et al., 2007; Dai et al., 2008; Kambara et al., 2009). However, genes potentially encoding these proteins are not present in MAFF303099, except for Mlr6316 which has 50% similarity to NopD (Rodrigues et al., 2007), although other T3SS effector genes have been identified flanking the MAFF303099 T3SS cluster (Sánchez et al., 2012).
Only two proteins, Msi059 and Msi061, have been shown to be transported through the T4SS of M. loti R7A (Hubber et al., 2004). Interestingly, Msi059 displays putative functional conservation with NopD, a predicted C48 cysteine peptidase (Rodrigues et al., 2007). On its hand, Msi061 shows some homology to A. tumefaciens VirF protein which participates during T-DNA translocation into the plant cells (Schrammeijer et al., 2001).
The effect of T4SS and T3SS mutations of Mesorhizobium strains on the symbiosis with Lotus species and other legumes has been analyzed by different authors. The symbiotic capacity of a NZP2037 mutant lacking 11 vir genes was essentially comparable to that of the wild-type strain, suggesting a limited contribution of T4SS to the host range among Lotus species (Kasai-Maita et al., 2013). In contrast, in R7A mutations in vir genes or in msi059 gene have host-dependent effects, negatively affecting nodule formation on usual legume hosts as L. corniculatus, but allowing active nodule formation in legume species which normally are not nodulated by the wild-type, such as Leucaena leucocephala (Hubber et al., 2004). These host-dependent effects are similar to those reported for T3SS mutants of MAFF303099 (Hubber et al., 2004). The host-dependence of the symbiotic phenotypes may reflect differences in the host reactions to secreted effector proteins (Hubber et al., 2004), or to the dual effect of certain effectors (Sánchez et al., 2012). Thus, in L. leucocephala the effector proteins may induce plant defense responses, analogous to the avirulence proteins of plant pathogens, resulting in abortion of infections; whereas in L. corniculatus they may facilitate infection, perhaps by suppressing plant defense reactions (Marie et al., 2001; Hubber et al., 2004), or by interfering with the process of autoregulation of nodulation (Nelson and Sadowsky, 2015) with the aim of increasing nodulation.
Upon recognition of specific rhizobial Nod factors, a host genetic program is initiated which governs the symbiosis with rhizobia. This program coordinates two parallel developmental processes in legume roots: de novo cortical cell division leading to nodule primordia formation, and the IT initiation in the root hairs guiding bacteria toward dividing cortical cells (reviewed in Binder et al., 2014).
Quite a significant number of proteins involved in recognition and binding of NF (NFR1 and NFR5), signal transduction from the plasma membrane to the nucleus (i.e., SYMRK), including nuclear and perinuclear calcium spiking and decoding the calcium signal, as well as several transcription factors involved in early symbiotic responses, have been identified in L. japonicus and other legumes (reviewed in Binder et al., 2014; Liang et al., 2014). Many of such proteins also play a key role in the recognition and response to arburcular mycorrizal fungi, and therefore are components of a genetic program that is common for rhizobia and AM fungi symbioses.
Bacterial infection via IT is mediated by the bacterial EPS and the L. japonicus EPR3 LysM receptor kinase. EPR3 is able to discriminate between wild-type and truncated or abnormal bacterial EPS (Kawaharada et al., 2015). Expression of EPR3 is dependent on previous Nod factor perception and signal transduction, and is tissue specific which suggests that EPR3 perception of compatible EPS promotes an intracellular cortical infection mechanisms to maintain bacteria enclosed in plant membranes (Kawaharada et al., 2017). As mentioned above, it would be interesting to know if EPR3 is involved in discriminating EPS of compatible and incompatible rhizobia in L. japonicus and other Lotus spp.
Also, host plant can control nodule number and new infection throught genetic mechanisms. Three L. japonicus CLAVATA3/ESR-related genes are involved in limiting the nodule number per root system; meanwhile a NRSYM1 transcriptional factor has been described as regulator of nitrate-induced mechanisms during nodulation (Nishida et al., 2018).
Condensed tannins (CTs) are polymeric end-products of the flavonoid biosynthetic pathway synthesized through the condensation of flavans, a diverse group of metabolites based on a heterocyclic ring system derived from the phenylalanine amino acid (reviewed by Ferreira and Slade, 2002; Marles et al., 2003). These compounds are the most abundant secondary metabolites in plants and are known as proanthocyanidins, as they yield anthocyanidins when depolymerized under oxidative conditions (Porter et al., 1985). CTs are present in fruits, bark, leaves and seeds of many plants and are of major importance in many agricultural crops. For instance, the flavor and astringency of wine are principally determined by both the levels and composition of CTs present in the grape skin (Gawel, 1998). CTs play different roles in plants, the best known are the protection against microbial pathogens, insects and larger herbivores (reviewed by Dixon et al., 2005). In most plant species, CTs are accumulated in the endothelial layer of the seed coat, where it would appear related to strengthening seed coat-imposed dormancy and longevity, in addition to protection against pathogens and predators (Debeaujon et al., 2000).
Prodelphinidins (derived from gallocatechin and epigallocatechin units) and procyanidins (derived from catechin and epicatechin units) are predominant types of CTs in legumes (Foo et al., 1996, 1997; Aoki et al., 2000). However, there are significant differences amongst species and genera. Species belonging to Medicago and Trifolium genera accumulate CTs mainly in flowers and seed coats (Goplen et al., 1980; Pang et al., 2007). On the contrary, Lotus spp. show CT accumulation in shoots, roots, flowers and seeds. Lotus spp. can differ in quantity and composition of the CTs accumulated, especially in the prodelphinidin/procyanidin/others ratio (Pd/Pc/others).
Shoots and roots from species like L. pedunculatus, L. uliginosus, L. subbiflorus and L. angustissimus have higher levels of CTs, shoots from L. corniculatus have intermediate levels, while roots of L. corniculatus and shoots and roots of L. japonicus, L. filicaulis, L. burtii, L. tenuis, L. krylovii and L. schoelleri have lower CT contents (Sivakumaran et al., 2006; Gruber et al., 2008). It has been also observed that species from the L. uliginosus group has a 2–3 fold higher content of Pd than Pc, whereas L. japonicus and close relatives contain mainly Pc (Pankhurst and Jones, 1979). Pd has more reactivity than Pc and can exert different biochemical effects, for instance during interaction with proteins (Aerts et al., 1999; Ropiak et al., 2017).
Some authors suggested that the level and/or composition of CTs in roots, along with differential sensitivity of rhizobial strains to CTs, could determine the symbiotic compatibility between Lotus spp. and rhizobia (Pankhurst, 1970; Pankhurst and Jones, 1979; Pankhurst et al., 1979, 1982, 1987). Moreover, Cooper and Rao (1992) found that monomeric flavolans, but especially CTs accumulated in Fix- nodules of L. uliginosus, in contrast to Fix+ nodules. However, these authors also reported that some rhizobial strains were highly effective on their Lotus host but exhibited sensitivity to root flavolans. We have also tested the sensitivity of various Lotus meso- and bradyrhizobia to L. uliginosus CTs, and found no correlation between bacterial sensitivity to these compounds and host-bacteria compatibility (M. J. Lorite, unpublished results).
Transgenic plants of L. corniculatus cv. Leo that overexpress Sn, a maize bHLH transcriptional regulator of anthocyanin biosinthesis, are currently available (Robbins et al., 2003). These plants accumulate different levels of CTs in shoots and roots, and constitute an excellent system to study the effects of these secondary metabolites in rhizobia-host legume specificity. Preliminary works have been carried out to evaluate the CT levels in roots of four Sn transgenic plants and its relationship with the symbiosis establishment with M. loti NZP2213 and Bradyrhizobium spp. NZP2309, in comparison to L. tenuis and L. uliginosus. Results showed that CT levels were markedly higher in the roots of some of the transgenic plants than in L. uliginosus. Nonetheless, all Sn transgenic and L. tenuis formed Fix+ nodules with M. loti NZP2213 and Fix- nodules with Bradyrhizobium spp. NZP2309 strain, thereby providing evidence that root CT levels do not seem to affect the plant-bacteria compatibility (F. Escaray, unpublished results).
The aminoacid-derived hydroxynitrile glucosides are a large class of bioactive metabolites found in many plant species, some of which may have a role in plant defense against herbivores (Bjarnholt and MØller, 2008). In the legume model L. japonicus, four different hydroxynitrile glucosides have been reported: lotoaustralin, linamarin, and the non-cyanogenic rhodiocyanosides A and D (Forslund et al., 2004). At least the first two compounds have been also detected in L. corniculatus and other closely related species (Lai et al., 2014). However, production of these compounds has not been observed in L. uliginosus and related species (Lai et al., 2014). Although there is no data on a possible relationship of cyanogenic glucosides and symbiosis establishment, it might be worth exploring the coincidental correlation of cyanogenic and non-cyanogenic species with symbiotic incompatibility groups I and II, respectively.
In Table 1 we have listed rhizobial strains which are used or recommended for Lotus inoculants in various countries. Despite the great diversity of rhizobia establishing effective symbioses with Lotus spp., only a reduced number of strains have been used for inoculants worldwide. This is especially true for inoculants involving bradyrhizobia, as one single strain NZP2039 is commonly used as inoculant for L. pedunculatus and L. subibiflorus in most countries listed. This is partly due to the fact that only few Lotus spp. are broadly grown for forage (mainly L. corniculatus, L. uliginosus, L. tenuis), and that bacterial strains selected in one country moved to other countries where they were rapidly tested and adopted. Very often too, rhizobia recommended as inoculants for a particular Lotus host, were not originally isolated from that host (i.e., NZP2037 isolated from L. divaricatus), and perhaps not even from a Lotus spp. host (i.e., SU343 could originally have been isolated from a Lupinus sp., see below). In some cases, the uncertainty about the bacterial origin can lead to uncertainty about its symbiotic properties. For instance, it is unclear if SU343 (equivalent names NZP 2196, ICMP 3663, ICMP 10808, PDDCC 3663, ATCC 35173, DSM 6046) was originally isolated from L. corniculatus in United States (as described for ICMP10808 and mentioned in Howieson et al., 2011), or from Lupinus sp. in Australia (as for ICMP 3663/NZP2196 and ATCC 35173, and in Charlton et al., 1981). Therefore it could be considered of unknown origin (as in DSM 6046). This uncertainty can sometimes lead to mistakes and therefore to misuses of a given strain. For instance, Binde et al. (2009) listed strain SEMIA 848 as an inoculant for L. subbiflorus in Brazil, however that strain is equivalent to U-510 in Uruguay where it is recommended for L. corniculatus and L. tenuis. The use of relatively few strains, which often were isolated long ago and brought from distant places, accentuate the need of studies toward selection of efficient rhizobial strains which perform optimally with local cultivars and prevailing environmental conditions. In an interesting study aimed to identify efficient strains for more contemporary forage species such as L. ornithopodiodes, Howieson et al. (2011) observed the difficulties to find strains that could optimally perform with all Lotus spp. of agronomic interest in New Zealand, because of the large host-strain interaction for symbiotic nitrogen fixation. This poses potential agronomic problems due to conflicts between such specific symbioses. For instance, sowing L. uliginosus or L. subbiflorus in a field with previous history of L. corniculatus can give rise to pasture failures, due to the L. corniculatus rhizobia inducing non-fixing pseudonodules in L. uliginosus. This further emphasizes the need to explore the existing diversity to select for rhizobial strains that perform optimally under specific conditions.
The ecological and agronomic potential of the genus Lotus for sustainable livestock production and other uses like land reclamation (Escaray et al., 2012), cannot be fully harnessed without acknowledging the great diversity of Lotus-rhizobia interactions as well as the specific plant and bacterial requirements, to achieve optimal symbiotic establishment and nitrogen fixation under the increasingly constrained environmental conditions faced by agricultural systems.
JS thought out the article, supervised and corrected the writings by all other authors and coordinated discussions. ML and ML-B prepared most work related to taxonomy and diversity, host–bacteria incompatibilities, and constructed the phylogenetic trees, with the support of JM and IVeC, who also collaborated in the section about inoculants. ME (CIC) and AS contributed to the chapter on bacterial compounds involved in specificity and the horizontal gene transfer, whereas FE contributed to the section on plant genetics and host compounds.
The authors declare that the research was conducted in the absence of any commercial or financial relationships that could be construed as a potential conflict of interest.
The authors are very grateful for the support from the Lotassa EU-INCO project and the IberoAmerican networks Biofag and AgroMicrobios (CYTED). The continuous support of the Spanish National Research Agency to the lab of JS (i.e., Grants BIO2014-55075-P and BIO2017-83533-P, cofinanced with FEDER funds) is also greatly acknowledged. They acknowledge support of the publication fee by the CSIC Open Access Publication Support Initiative through its Unit of Information Resources for Research (URICI).
The Supplementary Material for this article can be found online at: https://www.frontiersin.org/articles/10.3389/fmicb.2018.02055/full#supplementary-material
Acosta-Jurado, S., Rodríguez-Navarro, D. N., Kawaharada, Y., Perea, J. F., Gil-Serrano, A., Jin, H., et al. (2016). Sinorhizobium fredii HH103 invades Lotus burttii by crack entry in a Nod factor-and surface polysaccharide-dependent manner. Mol. Plant Microbe Interact. 29, 925–937. doi: 10.1094/MPMI-09-16-0195-R.
Aerts, R. J., Barry, T. N., and Mcnabb, W. C. (1999). Polyphenols and agriculture: beneficial effects of proanthocyanidins in forages. Agric. Ecosyst. Environ. 75, 1–12. doi: 10.1016/S0167-8809(99)00062-6
Ampomah, O. Y., and Huss-Danell, K. H. (2011). Genetic diversity of root nodule bacteria nodulating Lotus corniculatus and Anthyllis vulneraria in Sweden. Syst. Appl. Microbiol. 34, 267–275. doi: 10.1016/j.syapm.2011.01.006
Aoki, T., Akashi, T., and Ayabe, S. (2000). Flavonoids of leguminous plants: structure, biological activity, and biosynthesis. J. Plant. Res. 113, 475–488. doi: 10.1007/PL00013958
Banba, M., Siddique, A. B. M., Kouchi, H., Izui, K., and Hata, S. (2001). Lotus japonicus forms early senescent root nodules with Rhizobium etli. Mol. Plant Microbe Interact. 14, 173–180. doi: 10.1094/MPMI.2001.14.2.173
Barker, D. J., MacAdam, J. W., Butler, T. J., and Sulc, R. M. (2012). “Forage and biomass planting”, in Conservation Outcomes From Pastureland and Hayland Practices: Assessment, Recommendations, and Knowledge Gaps, ed. C. J. Nelson (Lawrence, KS: Allen Press), 41–110.
Bartsev, A. V., Deakin, W. J., Boukli, N. M., McAlvin, C. B., Stacey, G., Malnoë, P., et al. (2004). NopL, an effector protein of Rhizobium sp. NGR234, thwarts activation of plant defense reactions. Plant Physiol. 134, 871–879. doi: 10.1104/pp.103.031740
Batista, L., Tomasco, I., Lorite, M. J., Sanjuán, J., and Monza, J. (2013). Diversity and phylogeny of rhizobial strains isolated from Lotus uliginosus grown in Uruguayan soils. Appl. Soil Ecol. 66, 19–28. doi: 10.1016/j.apsoil.2013.01.009
Battisti, L., Lara, J. C., and Leigh, J. A. (1992). Specific oligosaccharide form of the Rhizobium meliloti exopolysaccharide promotes nodule invasion in alfalfa. Proc. Natl. Acad. Sci. U.S.A. 89, 5625–5629. doi: 10.1073/pnas.89.12.5625
Becker, B. U., Kosch, K., Parniske, M., and Müller, P. (1998). Exopolysaccharide (EPS) synthesis in Bradyrhizobium japonicum: sequence, operon structure and mutational analysis of an exo gene cluster. Mol. Gen. Genet. 259, 161–171. doi: 10.1007/s004380050801
Bek, A. S., Sauer, J., Thygesen, M. B., Duus, J. Ø., Petersen, B. O., Thirup, S., et al. (2010). Improved characterization of nod factors and genetically based variation in LysM Receptor domains identify amino acids expendable for nod factor recognition in Lotus spp. Mol. Plant Microbe Interact. 23, 58–66. doi: 10.1094/MPMI-23-1-0058.
Binde, D. R., Menna, P., Bangel, E. V., Barcellos, F. G., and Hungria, M. (2009). rep-PCR fingerprinting and taxonomy based on the sequencing of the 16S rRNA gene of 54 elite commercial rhizobial strains. Appl. Microbiol. Biotechnol. 83, 897–908. doi: 10.1007/s00253-009-1927-6
Binder, A., Soyano, T., Hayashi, M., Parniske, M., and Radutoiu, S. (2014). “Plant genes involved in symbiotic signal perception/signal transduction,” in The Lotus japonicus Genome.ed. S. Tabata (Berlin: Springer) 59–71.
Bjarnholt, N., and MØller, B. L. (2008). Hydroxynitrile glucosides. Phytochemistry 69, 1947–1961. doi: 10.1016/j.phytochem.2008.04.018
Borthakur, D., Barber, C. F., Lamb, J. W., Daniels, M. J., Downie, J. A., and Johnston, A. W. B. (1986). A mutation that blocks exopolysaccharide synthesis prevents nodulation of peas by Rhizobium leguminosarum but not of beans by R. phaseoli and is corrected by cloned DNA from Rhizobium or the phytopathogen Xanthomonas. Mol. Gen. Genet. 203, 320–323. doi: 10.1007/BF00333974
Bullard, G. K., Roughley, R. J., and Pulsford, D. J. (2005). The legume inoculant industry and inoculant quality control in Australia: 1953–2003. Aust. J. Exp. Agric. 45, 127–140. doi: 10.1071/EA03159
Caetano-Anollés, G., and Gresshoff, P. M. (1991). Plant genetic control of nodulation. Annu. Rev. Microbiol. 45, 345–382. doi: 10.1146/annurev.mi.45.100191.002021
Cárdenas, L., Dominguez, J., Quinto, C., López-Lara, I. M., Lugtenberg, B. J. J., Spaink, H. P., et al. (1995). Isolation, chemical structures and biological activity of the lipo-chitin oligosaccharide nodulation signals from Rhizobium etli. Plant Mol. Biol. 29, 453–464. doi: 10.1007/BF00020977
Charlton, J. F. L., Greenwood, R. M., and Clark, K. W. (1981). Comparison of the effectiveness of Rhizobium strains during establishment of Lotus corniculatus in hill country. N. Z. J. Exp. Agric. 9, 173–177.
Chisholm, S. T., Coaker, G., Day, B., and Staskawicz, B. J. (2006). Host-microbe interactions: shaping the evolution of the plant immune response. Cell 124, 803–814. doi: 10.1016/j.cell.2006.02.008
Cooper, J. E., and Rao, J. R. (1992). Localized changes in flavonoid biosynthesis in roots of Lotus pedunculatus after infection by Rhizobium loti. Plant Physiol. 100, 444–450. doi: 10.1104/pp.100.1.444
Cooper, J. E., Wood, M., and Bjourson, J. (1985). Nodulation of Lotus pedunculatus in acid rooting solution by fast- and slowgrowing rhizobia. Soil Biol. Biochem. 17, 487–492. doi: 10.1016/0038-0717(85)90015-X
Crow, V. L., Jarvis, B. D. W., and Greenwood, R. M. (1981). Deoxyribonucleic acid homologies among acid-producing strains of Rhizobium. Int. J. Syst. Bacteriol. 31, 152–172. doi: 10.1099/00207713-31-2-152
Dai, W. J., Zeng, Y., Xie, Z. P., and Staehelin, C. (2008). Symbiosis-promoting and deleterious effects of NopT, a novel type 3 effector of Rhizobium sp. strain NGR234. J. Bacteriol. 190, 5101–5110. doi: 10.1128/JB.00306-08
de Lajudie, P., Willems, A., Nick, G., Moreira, F., Molouba, F., Hoste, B., et al. (1998). Characterization of tropical tree rhizobia and description of Mesorhizobium plurifarium sp. nov. Int. J. Syst. Evol. Microbiol. 48, 369–382. doi: 10.1099/00207713-48-2-369
de Lajudie, P., Willems, A., Pot, B., Dewettinck, D., Maestrojuan, G., and Neyra, M. (1994). Polyphasic taxonomy of rhizobia: emendation of the genus Sinorhizobium and description of Sinorhizobium meliloti comb. nov., Sinorhizobium saheli sp. nov., and Sinorhizobium teranga sp. nov. Int. J. Syst. Evol. Microbiol. 44, 715–733. doi: 10.1099/00207713-44-4-715
De Meyer, S. E., Van Hoorde, K., Vekeman, B., Braeckman, T., and Willems, A. (2011). Genetic diversity of rhizobia associated with indigenous legumes in different regions of Flanders (Belgium). Soil Biol. Biochem. 43, 2384–2396. doi: 10.1016/j.soilbio.2011.08.005
Debeaujon, I., Le, K. M., and Koornneef, M. (2000). Influence of the testa on seed dormancy, germination, and longevity in Arabidopsis. Plant Physiol. 122, 403–413. doi: 10.1104/pp.122.2.403
Dénarié, J., Debelle, F., and Prome, J. C. (1996). Rhizobium lipo-chitooligosaccharide nodulation factors: signaling molecules mediating recognition and morphogenesis. Annu. Rev. Bioch. 65, 503–535. doi: 10.1146/annurev.bi.65.070196.002443
Desveaux, D., Singer, A. U., Wu, A. J., McNulty, B. C., Musselwhite, L., Nimchuk, Z., et al. (2007). Type III effector activation via nucleotide binding, phosphorylation, and host target interaction. PLoS Pathog. 3:e48. doi: 10.1371/journal.ppat.0030048
Díaz, P., Borsani, O., and Monza, J., (2005). “Lotus japonicus related species and their agronomic importance,” in Lotus Japonicus Handbook, ed. A. J. Márquez (Dordrecht: Springer-Verlag), 25–37.
Diebold, R., and Noel, K. D. (1989). Rhizobium leguminosarum exopolysaccharide mutants: biochemical and genetic analyses and symbiotic behavior on three hosts. J. Bacteriol. 171, 4821–4830. doi: 10.1128/jb.171.9.4821-4830.1989
Dixon, R. A., Xie, D. Y., and Sharma, S. B. (2005). Proanthocyanidins–a final frontier in flavonoid research? New Phytol. 165, 9–28. doi: 10.1111/j.1469-8137.2004.01217.x
Djordjevic, S. P., Chen, H., Batley, M., Redmond, J. W., and Rolfe, B. G. (1987). Nitrogen fixation ability of exopolysaccharide synthesis mutants of Rhizobium sp. strain NGR234 and Rhizobium trifolii is restored by the addition of homologous exopolysaccharides. J. Bacteriol. 169, 53–60. doi: 10.1128/jb.169.1.53-60.1987
Downie, J. A. (2010). The roles of extracellular proteins, polysaccharides and signals in the interactions of rhizobia with legume roots. FEMS Microbiol. Rev. 34, 150–170 doi: 10.1111/j.1574-6976.2009.00205.x
Escaray, F. J., Menendez, A. B., Gárriz, A., Pieckenstain, F. L., Estrella, M. J., Castagno, L. N., et al. (2012). Ecological and agronomic importance of the plant genus Lotus. Its application in grassland sustainability and the amelioration of constrained and contaminated soils. Plant Sci. 182, 121–133. doi: 10.1016/j.plantsci.2011.03.016
Estrella, M. J., Muńoz, S., Soto, M. J., Ruiz, O., and Sanjuan, J. (2009). Genetic diversity and host range of rhizobia nodulating Lotus tenuis in typical soils of the salado river basin (Argentina). Appl. Environ. Microbiol. 75, 1088–1098. doi: 10.1128/AEM.02405-08
Ferreira, D., and Slade, D. (2002). Oligomeric proanthocyanidins: naturally occurring O-heterocycles. Nat. Prod. Rep. 19, 517–541. doi: 10.1039/b008741f
Foo, L. Y., Mcnabb, W. C., Waghorn, G., and Ulyatt, M. J. (1997). Proanthocyanidins from Lotus pedunculatus. Phytochemistry 45, 1689–1696. doi: 10.1016/S0031-9422(97)00198-2
Foo, L. Y., Newman, R., Waghorn, G., Mcnabb, W. C., and Ulyatt, M. J. (1996). Proanthocyanidins from Lotus corniculatus. Phytochemistry 41, 617–624. doi: 10.1016/0031-9422(95)00602-8.
Forslund, K., Morant, M., Jørgensen, B., Olsen, C. E., Asamizu, E., Sato, S., et al. (2004). Biosynthesis of the nitrile glucosides rhodiocyanoside A and D and the cyanogenic glucosides lotaustralin and linamarin in Lotus japonicus. Plant Physiol. 135, 71–84. doi: 10.1104/pp.103.038059
Fulchieri, M. M., Estrella, M. J., and Iglesias, A. A. (2001). Characterization of Rhizobium loti strains from the Salado River Basin. Antonie Leeuwenhoek 79, 119–125. doi: 10.1023/A:1010221208619
Gawel, R. (1998). Red wine astringency: a review. Aust. J. Grape Wine R. 4, 74–95. doi: 10.1111/j.1755-0238.1998.tb00137.x
Giraud, E., Moulin, L., Vallenet, D., Barbe, V., Cytryn, E., Avarre, J. C., et al. (2007). Legumes symbioses: absence of Nod genes in photosynthetic bradyrhizobia. Science 316, 1307–1312. doi: 10.1126/science.1139548
Goplen, B. P., Howarth, R. E., Sarkar, S. K., and Lesins, K. (1980). A Search for condensed tannins in annual and perennial species of Medicago, Trigonella, and Onobrychis. Crop Sci. 20, 801–804. doi: 10.2135/cropsci1980.0011183X002000060031x
Gossmann, J. A., Markmann, K., Brachmann, A., Rose, L. E., and Parniske, M. (2012). Polymorphic infection and organogenesis patterns induced by a Rhizobium leguminosarum isolate from Lotus root nodules are determined by the host genotype. New Phytol. 196, 561–573. doi: 10.1111/j.1469-8137.2012.04281.x
Gruber, M. Y., Skadhauge, B., Yu, M., Muir, A., and Richards, K. (2008). Variation in morphology, plant habit, proanthocyanidins, and flavonoids within a Lotus germplasm collection. Can. J. Plant Sci. 88, 121–132. doi: 10.4141/P06-158
Han, T. X., Han, L. L., Wu, L. J., Chen, W. F., Sui, X. H., Gu, J. G., et al. (2008a). Mesorhizobium gobiense sp. nov. and Mesorhizobium tarimense sp. nov., isolated from wild legumes growing in desert soils of Xinjiang, China. Int. J. Syst. Evol. Microbiol. 58, 2610–2618. doi: 10.1099/ijs.0.2008/000125-0
Han, T. X., Wang, E. T., Wu, L. J., Chen, W. F., Gu, J. G., Gu, C. T., et al. (2008b). Rhizobium multihospitium sp. nov., isolated from multiple legume species native of Xinjiang, China. Int. J. Syst. Evol. Microbiol. 58, 1693–1699. doi: 10.1099/ijs.0.65568-0
Han, T. X., Tian, C. F., Wang, E. T., and Chen, W. X. (2010). Associations among rhizobial chromosomal background, nod Genes, and host plants based on the analysis of symbiosis of indigenous rhizobia and wild legumes native to Xinjiang. Microb. Ecol. 59, 311–323. doi: 10.1007/s00248-009-9577-x
Hernández-Lucas, I., Segovia, L., Martinez-Romero, E., and Pueppke, S. G. (1995). Phylogenetic relationships and host range of Rhizobium spp. that nodulate Phaseolus vulgaris L. Appl. Environ. Microbiol. 61, 2775–2779.
Hotter, G. S., and Scott, D. B. (1991). Exopolysaccharide mutants of Rhizobium loti are fully effective on a determinate nodulating host but are ineffective on an indeterminate nodulating host. J. Bacteriol. 173, 851–859. doi: 10.1128/jb.173.2.851-859.1991
Howieson, J. G., O’Hara, G. W., and Carr, S. J. (2000). Changing roles for legumes in Mediterranean agriculture: developments from an Australian perspective. Field Crops Res. 65, 107–122. doi: 10.1016/S0378-4290(99)00081-7
Howieson, J. G., Ballard R. A, Yates R. J., and Charman N. (2011). Selecting improved Lotus nodulating rhizobia to expedite the development of new forage species. Plant Soil 348, 231–243. doi: 10.1007/s11104-011-0921-9
Hubber, A., Vergunst, A. C., Sullivan, J. T., Hooykaas, P. J. J., and Ronson, C. W. (2004). Symbiotic phenotypes and translocated effector proteins of the Mesorhizobium loti strain R7A VirB/D4 type IV secretion system. Mol. Microbiol. 54, 561–574. doi: 10.1111/j.1365-2958.2004.04292.x.
Hubber, A. M., Sullivan, J. T., and Ronson, C. W. (2007). Symbiosis-induced cascade regulation of the Mesorhizobium loti R7A VirB/D4 type IV secretion system. Mol. Plant Microbe Interact. 20, 255–261. doi: 10.1094/MPMI-20-3-0255
Irisarri, P., Milnitsky, F., Monza, J., and Bedmar, E. J. (1996). Characterization of rhizobia nodulating Lotus subbiflorus from Uruguayan soil. Plant Soil 180, 39–47. doi: 10.1007/BF00015409
James, E. K., and Sprent, J. I. (1999). Development of N2-fixing nodules on the wetland legume Lotus uliginosus exposed to conditions of flooding. New Phytol. 142, 219–231. doi: 10.1046/j.1469-8137.1999.00394.x
Jarvis, B. D. W., Pankhurst, C. E., and Patel, J. J. (1982). Rhizobium loti, a new species of legume root nodule bacteria. Int. J. Syst. Bacteriol. 32, 378–380. doi: 10.1099/00207713-32-3-378
Jarvis, B. D. W., van Berkum, P., Chen, W. X., Nour, S. M., Fernández, M. P., Cleyet Marel, J. C., et al. (1997). Transfer of Rhizobium loti, Rhizobium huakuii, Rhizobium ciceri, Rhizobium mediterraneum and Rhizobium thianshanense to Mesorhizobium gen. nov. Int. J. Syst. Bacteriol. 47, 895–898. doi: 10.1099/00207713-47-3-895
Jensen, H. L., and Hansen, A. L. (1968). Observations on host plant relations in root nodule bacteria of the Lotus-Antythyllis and Lupinus-Ornithopus groups. Acta Agric. Scand. 18, 135–142. doi: 10.1080/00015126809433168
Jensen H. L. (1967). Mutual host plant relationships in two groups of legume root nodule bacteria (Rhizobium spp.)∗. Arch. Micobiol. 59, 174–179. doi: 10.1007/BF00406329
Kambara, K., Ardissone, S., Kobayashi, H., Saad, M. M., Schumpp, O., Broughton, W. J., et al. (2009). Rhizobia utilize pathogen-like effector proteins during symbiosis. Mol. Microbiol. 71, 92–106. doi: 10.1111/j.1365-2958.2008.06507.x
Kaneko, T., Nakamura, Y., Sato, S., Asamizu, E., Kato, T., Sasamoto, S., et al. (2000). Complete genome structure of the nitrogen-fixing symbiotic bacterium Mesorhizobium loti. DNA Res. 7, 381–406. doi: 10.1093/dnares/7.6.331
Kasai-Maita, H., Hirakawa, H., Nakamura, Y., Kaneko, T., Miki, K., Maruya, J., et al. (2013). Commonalities and differences among symbiosis islands of three Mesorhizobium loti strains. Microbes Environ. 28, 275–278. doi: 10.1264/jsme2.ME12201.
Kawaharada, Y., Kelly, S., Nielsen, M. W., Hjuler, C. T., Gysel, K., and Muszyński, A, et al. (2015). Receptor-mediated exopolysaccharide perception controls bacterial infection. Nature 523, 308–312. doi: 10.1038/nature14611
Kawaharada, Y., Nielsen, M. W., Kelly, S., James, E. K., Andersen, K. R., Rasmussen, S. R., et al. (2017). Differential regulation of the Epr3 receptor coordinates membrane-restricted rhizobial colonization of root nodule primordia. Nat. Commun. 8:14534. doi: 10.1038/ncomms14534.
Kelly, S. J., Muszyński, A., Kawaharada, Y., Hubber, A. M., Sullivan, J. T., Sandal, N., et al. (2013). Conditional Requirement for Exopolysaccharide in the Mesorhizobium–Lotus Symbiosis. Mol. Plant-Microbe Interact. 26, 319–329. doi: 10.1094/MPMI-09-12-0227-R
Kelly, S. J., Sullivan, J. T., Kawaharada, Y., Radutoiu, S., Ronson, C. W., and Stougaard, J. (2018). Regulation of Nod factor biosynthesis by alternative NodD proteins at distinct stages of symbiosis provides additional compatibility scrutiny. Environ. Microbiol. 20, 97–110. doi: 10.1111/1462-2920.14006
Kim, C. H., Tully, R. E., and Keister, D. L. (1989). Exopolysaccharide-deficient mutants of Rhizobium fredii HH303 which are symbiotically effective. Appl. Environ. Microbiol. 55, 1852–1854.
Krause, A., Doerfel, A., and Göttfert, M. (2002). Mutational and transcriptional analysis of the type III secretion system of Bradyrhizobium japonicum. Mol. Plant Microbe Interact. 15, 1228–1235. doi: 10.1094/MPMI.2002.15.12.1228
Lai, D., Abou Hachem, M., Robson, F., Olsen, C. E., Wang, T. L., Møller, B. L., et al. (2014). The evolutionary appearance of non-cyanogenic hydroxynitrile glucosides in the Lotus genus is accompanied by the substrate specialization of paralogous β–glucosidases resulting from a crucial amino acid substitution. Plant J. 79, 299–311. doi: 10.1111/tpj.12561
Laus, M. C., Logman, T. J., Van Brussel, A. A., Carlson, R. W., Azadi, P., Gao, M. Y., et al. (2004). Involvement of exo5 in production of surface polysaccharides in Rhizobium leguminosarum and its role in nodulation of Vicia sativa subsp. nigra. J. Bacteriol. 186, 6617–6625. doi: 10.1128/JB.186.19.6617-6625.2004
Laus, M. C., Van Brussel, A. A. N., and Kijne, J. W. (2005). Role of cellulose fibrils and exopolysaccharides of Rhizobium leguminosarum in attachment to and infection of Vicia sativa root hairs. Mol. Plant Microbe Interact. 18, 533–538. doi: 10.1094/MPMI-18-0533
Law, I. J., Yamamoto, Y., Mort, A. J., and Bauer, W. D. (1982). Nodulation of soybean by Rhizobium japonicum mutants with altered capsule synthesis. Planta 154, 100–109. doi: 10.1007/BF00387901
León-Barrios, M., Ramírez-Bahena, M. H., Igual J. M., Peix, A., and Velázquez E. (2018). Phyllobacterium salinisoli sp. nov., isolated from a Lotus lancerottensis root nodule in saline soil from Lanzarote. Int. J. Syst. Evol. Microbiol. 68, 1085–1089. doi: 10.1099/ijsem.0.002628
León-Barrios, M., Lorite, M. J., Donate-Correa, J., and Sanjuán, J. (2009). Ensifer meliloti bv. lancerottense establishes nitrogenfixing symbiosis with Lotus endemic to the Canary Islands and shows distinctive symbiotic genotypes and host range. Syst. Appl. Microbiol. 32, 413–420. doi: 10.1016/j.syapm.2009.04.003
Liang, Y., Tóth, K., Cao, Y., Tanaka, K., Espinoza, C., and Stacey, G. (2014). Lipochitooligosaccharide recognition: an ancient story. New Phytol. 204,289–296. doi: 10.1111/nph.12898
López-Lara, I. M., van den Berg, J. D., Thomas-Oates, J. E., Glushka, J., Lugtenberg, B. J., and Spaink, H. P. (1995). Structural identification of the lipo-chitin oligosaccharide nodulation signals of Rhizobium loti. Mol. Microbiol. 15,627–638. doi: 10.1111/j.1365-2958.1995.tb02372.x
Lorite, M., Videira e Castro, I., Muńoz, S., and Sanjuán, J. (2012). Phylogenetic relationship of Lotus uliginosus symbionts with bradyrhizobia nodulating genistoid legumes. FEMS Microbiol. Ecol. 79, 454–474. doi: 10.1111/j.1574-6941.2011.01230.x
Lorite, M. J., Donate-Correa, J., Arco-Aguilar, M., Pérez Galdona, R., Sanjuán, J., and León-Barrios, M. (2010a). Lotus endemic to the Canary Islands are nodulated by diverse and novel rhizobial species and symbiotypes. Syst. Appl. Microbiol. 33, 282–290. doi: 10.1016/j.syapm.2010.03.006
Lorite, M. J., Muńoz, S., Olivares, J., Soto, M. J., and Sanjuán, J. (2010b). Characterization of strains unlike Mesorhizobium loti that nodulate Lotus spp. in saline soils of Granada, Spain. Appl. Environ. Microbiol. 76, 4019–4026. doi: 10.1128/AEM.02555-09
Lorite, M. J., Flores-Félix, J. D., Peix,Á., Sanjuán, J., and Velázquez, E. (2016). Mesorhizobium olivaresii sp. nov. isolated from Lotus corniculatus nodules. Syst. Appl. Microbiol. 39, 557–561. doi: 10.1016/j.syapm.2016.09.003
Lowther, W. L. (1984). Effect of strain of rhizobia, inoculation level, and pelleting on the establishment of oversown Lotus pedunculatus “grasslands Maku”. N. Z. J. Exp. Agric. 12, 287–294.
Madsen, L. H., Tirichine, L., Jurkiewicz, A., Sullivan, J. T., Heckmann, A. B., Bek, A. S., et al. (2010). The molecular network governing nodule organogenesis and infection in the model legume Lotus japonicus. Nat. Commun. 1:10. doi: 10.1038/ncomms1009.
Marcos-García, M., Menéndez, E., Cruz-González, X., Velázquez, E., Mateos, P. F., and Rivas, R. (2015). High diversity of Lotus corniculatus endosymbionts in soils of Northwest Spain. Symbiosis 76, 11–20. doi: 10.1007/s13199-015-0368-5
Marcos-García, M., Menéndez, E., Ramírez-Bahena, M., Mateos, P., Peix,Á., Velazquez, E., et al. (2017). Mesorhizobium helmanticense sp. nov., isolated from Lotus corniculatus nodules Int. J. Syst. Evol. Microbiol. 67 2301–2305. doi: 10.1099/ijsem.0.001942
Marie, C., Broughton, W. J., and Deakin, W. J. (2001). Rhizobium type III secretion systems: legume charmers or alarmers? Curr. Opin. Plant Biol. 4, 336–342. doi: 10.1016/S1369-5266(00)00182-5
Marie, C., Deakin, W. J., Ojanen-Reuhs, T., Diallo, E., Reuhs, B., Broughton, W. J., et al. (2004). TtsI, a key regulator of Rhizobium species NGR234 is required for type III-dependent protein secretion and synthesis of rhamnose-rich polysaccharides. Mol. Plant Microbe Interact. 17, 958–966. doi: 10.1094/MPMI.2004.17.9.958
Marles, M. A. S., Ray, H., and Gruber, M. Y. (2003). New perspectives on proanthocyanidin biochemistry and molecular regulation. Phytochemistry 64, 367–383. doi: 10.1016/S0031-9422(03)00377-7
Márquez, A. J., Stougaard, J., Udvardi, M., Parniske, M., Spaink, H., Saalbach, G., et al. (2005). Lotus Japonicus Handbook. Netherlands: Springer. doi: 10.1007/1-4020-3735-X
Martínez-Hidalgo, P., Ramírez-Bahena, M. H., Flores-Félix, J. D., Igual, J. M., Sanjuán, J., León-Barrios, M., et al. (2016). Reclassification of strains MAFF 303099T and R7A into the new species Mesorhizobium japonicum sp. nov. Int. J. Syst. Evol. Microbiol. 66, 4936–4941. doi: 10.1099/ijsem.0.001448
Martínez-Hidalgo, P., Ramírez-Bahena, M. H., Flores-Felix, J. D., Rivas, R., Igual, J. M., Mateos, P. F., et al. (2015). Revision of the taxonomic status of type strains of Mesorhizobium loti and reclassification of strain USDA 3471T as the type strain of Mesorhizobium erdmanii sp. nov. and ATCC 33669T as the type strain of Mesorhizobium jarvisii sp. nov. Int. J. Syst. Evol. Microbiol. 65, 1703–1708. doi: 10.1099/ijs.0.000164
Merabet, C., Martens, M., Mahdhi, M., Zakhia, F., Sy, A., Le Roux, C., et al. (2010). Multilocus sequence analysis of root nodule isolates from Lotus arabicus (Senegal), Lotus creticus, Argyrolobium uniflorum and Medicago sativa (Tunisia) and description of Ensifer numidicus sp. nov. and Ensifer garamanticus sp. nov. Int. J. Syst. Evol. Microbiol. 60, 664–674. doi: 10.1099/ijs.0.012088-0
Mishima, E., Hosokawa, A., Imaizumi-Anraku, H., Saito, K., Kawaguchi, M., and Saeki, K. (2008). Requirement for Mesorhizobium loti ornithine transcarbamoylase for successful symbiosis with Lotus japonicus as revealed by an unexpected long-range genome deletion. Plant Cell 49, 301–313. doi: 10.1093/pcp/pcn004
Monza, J., Fabiano, E., and Arias, A. (1992). Characterization of an indigenous population of rhizobia nodulating Lotus corniculatus. Soil Biol. Biochem. 24, 241–247. doi: 10.1007/s00203-006-0173-x
Muszyński, A., Heiss, C., Hjuler, C. T., Sullivan, J.T., Kelly, S. J., Thygesen, M. B., et al. (2016). Structures of exopolysaccharides involved in receptor-mediated perception of Mesorhizobium loti by Lotus japonicus. J. Biol. Chem. 291, 20946–20961. doi: 10.1016/0038-0717(92)90225-M
Nelson, M. S., and Sadowsky, M. J. (2015). Secretion systems and signal exchange between nitrogen-fixing rhizobia and legumes. Front. Plant Sci. 6:491. doi: 10.1074/jbc.M116.743856
Niehaus, K., Kapp, D., and Puhler, A. (1993). Plant defense and delayed infection of alfalfa pseudonodules induced by an exopolysaccharide (EPS I)-deficient Rhizobium meliloti mutant. Planta 190, 415–425. doi: 10.3389/fpls.2015.00491
Nishida, H., Tanaka, S., Handa, Y., Ito, M., Sakamoto, Y., Matsunaga, S., et al. (2018). A NIN-like protein mediates nitrate-induced control of root nodule symbiosis in Lotus japonicas. Nat. Commun. 9:499. doi: 10.1007/BF00196971
Niwa, S., Kawaguchi, M., Imazumi-Anraku, H., Chechetka, S. A., Ishizaka, M., Ikuta, A., et al. (2001). Responses of a model legume Lotus japonicus to lipochitin oligosaccharide nodulation factors purified from Mesorhizobium loti JRL501. Mol. Plant Microbe Interact. 14, 848–856. doi: 10.1094/MPMI.2001.14.7.848
Olsthoorn, M. M. A., López-Lara, I. M., Petersen, B. O., Bock, K., Haverkamp, J., Spaink, H. P., et al. (1998). Novel branched Nod factor structure results from alpha-(1–3) fucosyl transferase activity: the major lipochitin oligosaccharides from Mesorhizobium loti strain NZP2213 bear an alpha-(1–3) fucosyl substituent on a nonterminal backbone residue. Biochemistry 37, 9024–9032. doi: 10.1021/bi972937r
Pang, Y., Peel, G. J., Wright, E., Wang, Z., and Dixon, R. A. (2007). Early steps in proanthocyanidin biosynthesis in the model legume Medicago truncatula. Plant Physiol. 145, 601–615. doi: 10.1104/pp.107.107326
Pankhurst, C. E. (1970). Development of effective and ineffective root nodules on two Lotus species. N. Z. J. Sci. 13, 519–531. doi: 10.1111/tpj.13759
Pankhurst, C. E., Craig, A. S., and Jones, W. (1979). Effectiveness of Lotus root nodules. I. morphology and flavolan content of nodules formed on Lotus pedunculatus by fast Lotus rhizobia. J. Exp. Bot. 30, 1085–1093. doi: 10.1093/jxb/30.6.1085
Pankhurst, C. E., Hopcroft, D. H., and Jones, W. T. (1987). Comparative morphology and flavolan content of Rhizobium loti induced effective and ineffective root nodules on Lotus species, Leuceana leucocephala, Carmichaelia flagelliformis, Ornithopus sativus, and Clianthus puniceus. Can. J. Microbiol. 65, 2676–2685. doi: 10.1139/b87-358
Pankhurst, C. E., and Jones, W. (1979). Effectiveness of Lotus root nodules. III. effect of combined nitrogen on nodule effectiveness and flavolan synthesis in plant root. J. Exp. Bot. 30, 1109–1118. doi: 10.1093/jxb/30.6.1109.
Pankhurst, C. E., Jones, W. T., and Craig, A. S. (1982). Bactericidal effect of Lotus pedunculatus root flavolan on fast-growing Lotus rhizobia. J. Gen. Microbiol. 128, 1567–1576. doi: 10.1099/00221287-128-7-1567
Parniske, M., Kosch, K., Werner, D., and Müller, P. (1993). ExoB mutants of Bradyrhizobium japonicum with reduced competitiveness for nodulation of Glycine max. Mol. Plant Microbe Interact. 6, 99–99. doi: 10.1099/00221287-128-7-1567
Parniske, M., Schmidt, P. E., Kosch, K., and Müller, P. (1994). Plant defense responses of host plants with determinate nodules induced by EPS-defective exoB mutants of Bradyrhizobium japonicum. Mol. Plant Microbe Interact. 7, 631–638. doi: 10.1094/MPMI-6-099
Peix, A., Ramírez-Bahena, M. H., Velázquez, E., and Bedmar, E. J. (2015). Bacterial associations with legumes. Crit. Rev. Plant Sci. 34, 17–42. doi: 10.1094/MPMI-7-0631
Pellock, B. J., Cheng, H. P., and Walker, G. C. (2000). Alfalfa root nodule invasion efficiency is dependent on Sinorhizobium meliloti polysaccharides. J. Bacteriol. 182, 4310–4318. doi: 10.1080/07352689.2014.897899
Porter, L. J., Hrstich, L. N., and Chan, B. G. (1985). The conversion of procyanidins and prodelphinidins to cyanidin and delphinidin. Phytochemistry 25, 223–230. doi: 10.1128/JB.182.15.4310-4318.2000
Qian, J., and Parker, M. A. (2002). Contrasting nifD and ribosomal gene relationships among Mesorhizobium from Lotus oroboides in Northern Mexico. Syst. Appl. Microbiol. 25, 68–73. doi: 10.1016/S0031-9422(00)94533-3
Radutoiu, S., Madsen, L. H., Madsen, E. B., Felle, H. H., Umehara, Y., Grønlund, M., et al. (2003). Plant recognition of symbiotic bacteria requires two LysM receptor-like kinases. Nature 425, 585–592. doi: 10.1078/0723-2020-00095
Ramírez-Bahena, M. H., Hernández, M., Peix, A., Velázquez, E., and León-Barrios, M. (2012). Mesorhizobial strains nodulating Anagyris latifolia and Lotus berthelotii in Tamadaya ravine (Tenerife, Canary Islands) are two symbiovars of the same species, Mesorhizobium tamadayense sp. nov. Syst. Appl. Microbiol. 35, 334–341. doi: 10.1038/nature02039
Ramsay, J. P., and Ronson, C. W. (2015). “Genetic regulation of symbiosis island transfer in Mesorhizobium loti,” in Biological Nitrogen Fixation, 1th Edn, Vol. 1, ed. F. de Nruijn (Hoboken, NJ: John Wiley), 219–223.
Rejili, M., Lorite, M. J., Mahdhi, M., Sanjuan, J., Ferchichi, A., and Mars, M. (2009). Genetic diversity of rhizobial populations recovered from three Lotus species cultivated in the infra-arid Tunisian soils. Prog. Nat. Sci. 19, 1079–1087. doi: 10.1016/j.pnsc.2009.02.003
Rejili, M., Mahdhi, M., Fterich, A., Dhaoui, S., Guefrachi, I., Abdeddayem, R., et al. (2012). Symbiotic nitrogen fixation of wild legumes in Tunisia: Soil fertility dynamics, field nodulation and nodules effectiveness. Agric. Ecosyst. Environ. 157, 60–69. doi: 10.1016/j.pnsc.2009.02.003
Robbins, M. P., Paolocci, F., Hughes, J. W., Turchetti, V., Allison, G., Arcioni, S., et al. (2003). Sn, a maize bHLH gene, modulates anthocyanin and condensed tannin pathways in Lotus corniculatus. J. Exp. Bot. 54, 239–248. doi: 10.1093/jxb/erg022
Rodpothong, P., Sullivan, J. T., Songsrirote, K., Sumpton, D., Cheung, K. W., Thomas-Oates, J., et al. (2009). Nodulation gene mutants of Mesorhizobium loti R7A-nodZ and nolL mutants have host-specific phenotypes on Lotus spp. Mol. Plant Microbe Interact. 22, 1546–1554. doi: 10.1094/MPMI-22-12-1546
Rodrigues, J. A., López-Baena, F. J., Ollero, F. J., Vinardell, J. M., Espuny, M. R., Bellogín, R. A., et al. (2007). NopM and NopD are rhizobial nodulation outer proteins: identification using LC-MALDI and LC-ESI with a monolithic capillary column. J. Proteome Res. 6, 1029–1037. doi: 10.1021/pr060519f
Rogel, M. A., Ormeno-Orrillo, E., and Romero, E. M. (2011). Symbiovars in rhizobia reflect bacterial adaptation to legumes. Syst. Appl. Microbiol. 34, 96–104. doi: 10.1016/j.syapm.2010.11.015
Roma-Neto, I. V., Ribeiro, R. A., and Hungria, M. (2010). Genetic diversity of elite rhizobial strains of subtropical and tropical legumes based on the 16S rRNA and glnII genes. World J. Microbiol. Biotechnol. 26, 1291–1302. doi: 10.1007/s11274-009-0300-3
Ronson, C., Sullivan, J., Cruickshank, R., Brown, S., Elliot, R., Fleetwood, D., et al. (2002). Comparative sequence analysis of the symbiosis island of Mesorhizobium loti strain R7A. J. Bacteriol. 184, 3086–3095. doi: 10.1128/JB.184.11.3086-3095.2002
Ropiak, H. M., Lachmann, P., Ramsay, A., Green, R. J., and Mueller-Harvey, I. (2017). Identification of structural features of condensed tannins that affect protein aggregation. PLoS One 12:e0170768. doi: 10.1371/journal.pone.0170768
Sachs, J. L., Kembel, S. W., Lau, A. H., and Simms, E. L. (2009). In situ phylogenetic structure and diversity of wild Bradyrhizobium communities. Appl. Environ. Microbiol. 75, 4727–4735. doi: 10.1128/AEM.00667-09
Saeki, K., and Kouchi, H. (2000). The Lotus symbiont, Mesorhizobium loti: molecular genetic techniques and application. J. Plant Res. 113, 457–465. doi: 10.1128/AEM.00667-09
Saeki, K., and Ronson, C. W. (2014), “Genome sequence and gene functions in Mesorhizobium loti and relatives,” in The Lotus japonicus Genome. Compendium of Plant Genomes, eds S. Tabata, and J. Stougaard (Berlin: Springer). doi: 10.1007/PL00013956
Sánchez, C., Mercante, V., Babuin, M. F., and Lepek, V. C. (2012). Dual effect of Mesorhizobium loti T3SS functionality on the symbiotic process. FEMS Microbiol. Lett. 330, 148–156.
Sánchez, M., Ramírez-Bahena, M. H., Peix, A., Lorite, M. J., Sanjuán, J., Velázquez, E., et al. (2014). Phyllobacterium loti sp. nov. isolated from nodules of Lotus corniculatus. Int. J. Syst. Evol. Microbio. 64, 781–786. doi: 10.1111/j.1574-6968.2012.02545.x
Sandal, N., Jin, H., Rodriguez-Navarro, D. N., Temprano, F., Cvitanich, C., Brachmann, A., et al. (2012). A set of Lotus japonicus Gifu × Lotus burttii recombinant inbred lines facilitates map-based cloning and QTL mapping. DNA Res. 19, 317–323. doi: 10.1099/ijs.0.052993-0
Sannazzaro, A. I., Bergottini, V. M., Paz, R. C., Castagno, L. N., Menéndez, A. B., Ruiz, O. A., et al. (2011). Comparative symbiotic performance of native rhizobia of the Flooding Pampa and strains currently used for inoculating Lotus tenuis in this region. Antonie Van Leeuwenhoek 99, 371–379. doi: 10.1093/dnares/dss014
Sannazzaro, A. I., Torres-Tejerizo, G., Fontana, M. F., Cumpa-Velásquez, L. M., Hansen, L. H., Pistorio, M., et al. (2018). Mesorhizobium sanjuanii sp. nov., isolated from nodules of Lotus tenuis in saline-alkaline lowlands of Flooding Pampa, Argentina. Int. J. Syst. Evol. Microbiol. doi: 10.1007/s10482-010-9502-9 [Epub ahead of print].
Schrammeijer, B., Risseeuw, E., Pansegrau, W., Regensburg-Tuínk, T. J., Crosby, W. L., and Hooykaas, P. J. (2001). Interaction of the virulence protein VirF of Agrobacterium tumefaciens with plant homologs of the yeast Skp1 protein. Curr. Biol. 11, 258–262. doi: 10.1016/S0960-9822(01)00069-0
Schumpp, O., Crèvecoeur, M., Broughton, W. J., and Deakin, W. J. (2009). Delayed maturation of nodules reduces symbiotic effectiveness of the Lotus japonicus-Rhizobium sp. NGR234 interaction. J. Exp. Bot. 60, 581–590. doi: 10.1093/jxb/ern302
Scott, D. B., Wilson, R., Shaw, G. J., Petit, A., Tempe, J. (1987). Biosynthesis and degradation of nodule-specific Rhizobium loti compounds in Lotus nodules. J. Bacteriol. 169, 278–282. doi: 10.1128/jb.169.1.278-282.1987
Sivakumaran, S., Rumball, W., Lane, G. A., Fraser, K., Foo, L. Y., Yu, M., et al. (2006). Variation of proanthocyanidins in Lotus Species. J. Chem. Ecol. 32, 1797–1816. doi: 10.1007/s10886-006-9110-3
Skorpil, P., Saad, M. M., Boukli, N. M., Kobayashi, H., Ares-Orpel, F., Broughton, W. J., et al. (2005). NopP, a phosphorylated effector of Rhizobium sp. strain NGR234, is a major determinant of nodulation of the tropical legumes Flemingia congesta and Tephrosia vogelii. Mol. Microbiol. 57, 1304–1317. doi: 10.1111/j.1365-2958.2005.04768.x
Sokoloff, D. D., and Lock, J. M. (2005). “Legumes of the world. tribe loteae,” in Legumes of the world eds G. Lewis, B. Schrire, B. Mackinder, and M. Lock (Richmond VA: Royal Botanic Gardens, Kew), 455–465.
Sotelo, M., Irisarri, P., Lorite, M. J., Casaretto, E., Rebuffo, M., Sanjuán, J., et al. (2011). Diversity of rhizobia nodulating Lotus corniculatus grown in northern and southern regions of Uruguay. Appl. Soil Ecol. 49, 197–207. doi: 10.1016/j.apsoil.2011.05.005
Spaink, H. P. (2000). Root nodulation and infection factors produced by rhizobial bacteria. Annu. Rev. Microbiol. 54, 257–288. doi: 10.1146/annurev.micro.54.1.257
Stuurman, N., Pacios Bras, C., Schlaman, H. R. M., Wijfjes, A. H. M., Bloemberg, G. V., and Spaink, H. P. (2000). The use of green fluorescent protein color variants expressed on stable broad-host-range vectors to visualize rhizobia interacting with plants. Mol. Plant Microbe Interact. 13, 1163–1169. doi: 10.1094/MPMI.2000.13.11.1163
Sullivan, J. T., Patrick, H. N., Lowthert, W. L., Scott, D. B., and Ronson, C. W. (1995). Nodulating strains of Rhizobium loti arise through chromosomal symbiotic gene transfer in the environment. Proc. Natl. Acad. Sci. U.S.A. 92, 8985–8989. doi: 10.1073/pnas.92.19.8985
Sullivan, J. T., and Ronson, C. W. (1998). Evolution of rhizobia by acquisition of a 500-kb symbiosis island that integrates into a phe-tRNA gene. Proc. Natl. Acad. Sci. U.S.A. 95, 5145–5149. doi: 10.1073/pnas.92.19.8985
Sullivan, J. T., Trzebiatowski, J. R., Cruickshank, R. W., Gouzy, J., Brown, S. D., Elliot, R. M., et al. (2002). Comparative sequence analysis of the symbiosis Island of Mesorhizobium loti strain R7A. J. Bacteriol. 184, 3086–3095. doi: 10.1128/JB.184.11.3086-3095.2002
Tabata, S., and Stougaard, J. (2014). The Lotus Japonicus Genome. Berlin: Springer-Verlag. doi: 10.1007/978-3-662-44270-8
Truchet, G., Camut, S., De Billy, F., Odorico, R., and Vasse, J. (1989). The Rhizobium-legume symbiosis two methods to discriminate between nodules and other root-derived structures. Protoplasma 149, 82–88. doi: 10.1007/BF01322980
Turner, S. L., Zhang, X. X., Li, F., and Young, J. P. W. (2002). What does a bacterial genome sequence represent? Misassignment of MAFF303099 to the genospecies Mesorhizobium loti. Microbiology 148, 3330–3331. doi: 10.1099/00221287-148-11-3330
Urzainqui, A., and Walker, G. C. (1992). Exogenous suppression of the symbiotic deficiencies of Rhizobium meliloti exo mutants. J. Bacteriol. 174, 3403–3406. doi: 10.1128/jb.174.10.3403-3406.1992
Vinuesa, P., Silva, C., Werner, D., and Martínez-Romero, E. (2005). Population genetics and phylogenetic inference in bacterial molecular systematics: the roles of migration and recombination in Bradyrhizobium species cohesion and delineation. Mol. Phylogenet. Evol. 34, 29–54 doi: 10.1016/j.ympev.2004.08.020
Wang, S., Hao, B., Li, J., Gu, H., Peng, J., Xie, F., et al. (2014), Whole-genome sequencing of Mesorhizobium huakuii 7653R provides molecular insights intohost specificity and symbiosis island dynamics. BMC Genomics 15:440. doi: 10.1186/1471-2164-15-440
Wielbo, J., Mazur, A., Krol, J., Marczak, M., Kutkowska, J., and Skorupska, A. (2004). Complexity of phenotypes and symbiotic behaviour of Rhizobium leguminosarum biovar trifolii exopolysaccharide mutants. Arch. Microbiol. 182, 331–336. doi: 10.1007/s00203-004-0723-z
Willems, A., Hoste, B., Tang, J., Janssens, D., and Gillis, M. (2001). Differences between subcultures of the Mesorhizobium loti type strain from different culture collections. Syst. Appl. Microbiol. 24, 549–553. doi: 10.1078/0723-2020-00070
Keywords: nitrogen fixation, bacterial diversity, inoculants, plant–microbe association, symbiosis
Citation: Lorite MJ, Estrella MJ, Escaray FJ, Sannazzaro A, Videira e Castro IM, Monza J, Sanjuán J and León-Barrios M (2018) The Rhizobia-Lotus Symbioses: Deeply Specific and Widely Diverse. Front. Microbiol. 9:2055. doi: 10.3389/fmicb.2018.02055
Received: 15 June 2018; Accepted: 13 August 2018;
Published: 12 September 2018.
Edited by:
Lorena Carro, Newcastle University, United KingdomReviewed by:
Aleysia Kleinert, Stellenbosch University, South AfricaCopyright © 2018 Lorite, Estrella, Escaray, Sannazzaro, Videira e Castro, Monza, Sanjuán and León-Barrios. This is an open-access article distributed under the terms of the Creative Commons Attribution License (CC BY). The use, distribution or reproduction in other forums is permitted, provided the original author(s) and the copyright owner(s) are credited and that the original publication in this journal is cited, in accordance with accepted academic practice. No use, distribution or reproduction is permitted which does not comply with these terms.
*Correspondence: Juan Sanjuán, SnVhbi5TYW5qdWFuQGVlei5jc2ljLmVz
Disclaimer: All claims expressed in this article are solely those of the authors and do not necessarily represent those of their affiliated organizations, or those of the publisher, the editors and the reviewers. Any product that may be evaluated in this article or claim that may be made by its manufacturer is not guaranteed or endorsed by the publisher.
Research integrity at Frontiers
Learn more about the work of our research integrity team to safeguard the quality of each article we publish.