- 1Microbial Horticulture, Department of Biosystems and Technology, Swedish University of Agricultural Sciences, Alnarp, Sweden
- 2Department of Biological Sciences, SUNY Oswego, Oswego, NY, United States
- 3Department of Biomedical Sciences and Veterinary Public Health, Swedish University of Agricultural Sciences, Alnarp, Sweden
- 4Department of Food Technology, Engineering and Nutrition, Lund University, Lund, Sweden
Consumers appreciate leafy green vegetables such as baby leaves for their convenience and wholesomeness and for adding a variety of tastes and colors to their plate. In Western cuisine, leafy green vegetables are usually eaten fresh and raw, with no step in the long chain from seed to consumption where potentially harmful microorganisms could be completely eliminated, e.g., through heating. A concerning trend in recent years is disease outbreaks caused by various leafy vegetable crops and one of the most important foodborne pathogens in this context is Shiga toxin-producing Escherichia coli (STEC). Other pathogens such as Salmonella, Shigella, Yersinia enterocolitica and Listeria monocytogenes should also be considered in disease risk analysis, as they have been implicated in outbreaks associated with leafy greens. These pathogens may enter the horticultural value network during primary production in field or greenhouse via irrigation, at harvest, during processing and distribution or in the home kitchen/restaurant. The hurdle approach involves combining several mitigating approaches, each of which is insufficient on its own, to control or even eliminate pathogens in food products. Since the food chain system for leafy green vegetables contains no absolute kill step for pathogens, use of hurdles at critical points could enable control of pathogens that pose a human health risk. Hurdles should be combined so as to decrease the risk due to pathogenic microbes and also to improve microbial stability, shelf-life, nutritional properties and sensory quality of leafy vegetables. The hurdle toolbox includes different options, such as physical, physiochemical and microbial hurdles. The goal for leafy green vegetables is multi-target preservation through intelligently applied hurdles. This review describes hurdles that could be used for leafy green vegetables and their biological basis, and identifies prospective hurdles that need attention in future research.
Introduction
Vegetables are an essential part of the human diet and an important source of minerals and vitamins. They are often eaten raw or only minimally processed (Goodburn and Wallace, 2013), particularly leafy green vegetables (LGV) such as baby leaf spinach and Swiss chard, rocket and different types of lettuce. Consumption of fresh produce in general has increased over the past two decades (Olaimat and Holley, 2012). At the same time, the number of foodborne disease outbreaks associated with consumption of LGV has increased (Castro-Ibáñez et al., 2017). The chief bacterial pathogens associated with these outbreaks are Shigatoxin-producing Escherichia coli (STEC), Salmonella spp., Yersinia spp. and Listeria monocytogenes (Tables 1, 2). In addition to being vehicles of human pathogens, ready-to-eat (RTE) salads may also be vehicles for bacteria with genes coding for resistance to specific antibiotics (Campos et al., 2013).
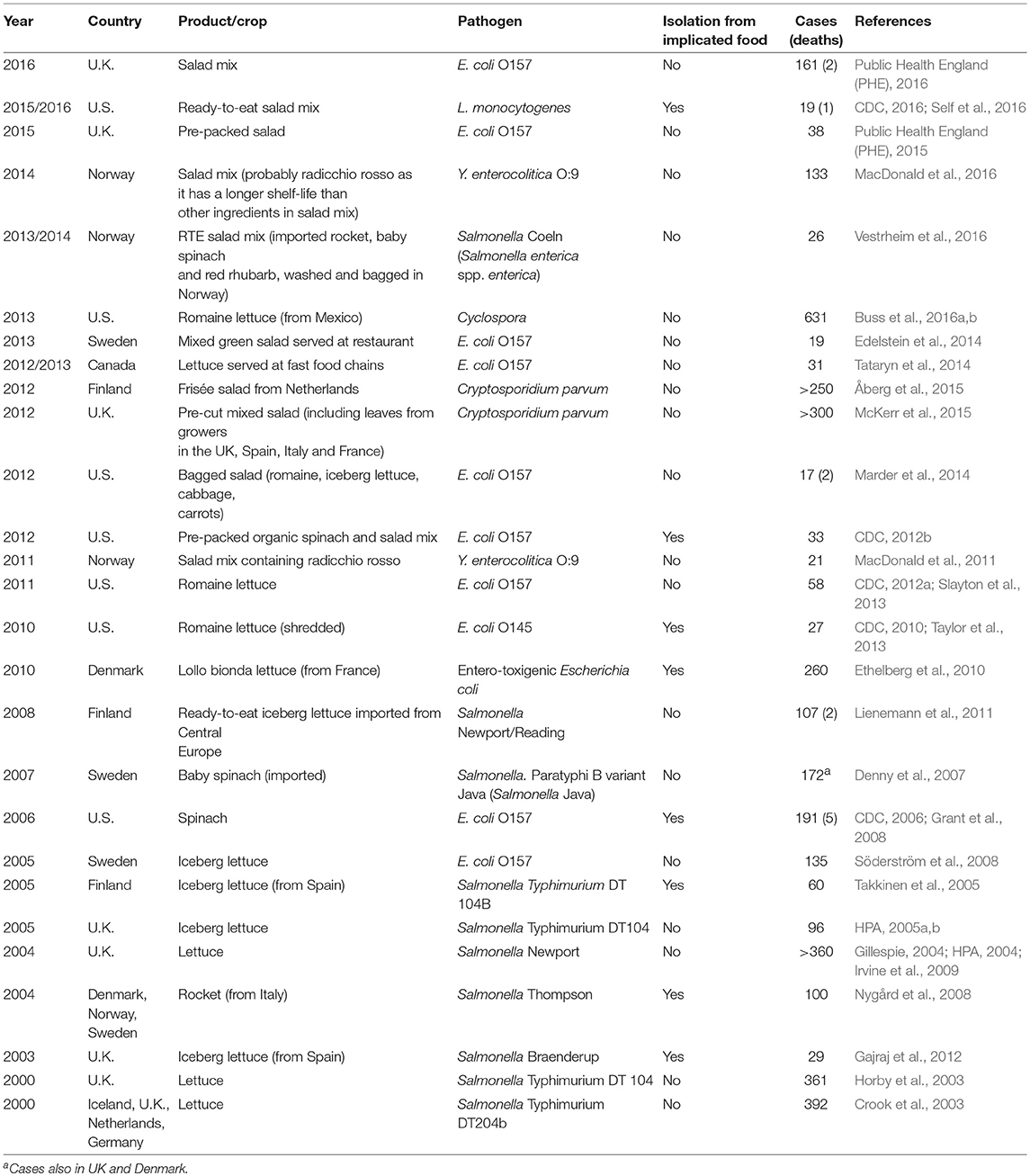
Table 1. Examples of foodborne disease outbreaks linked to leafy vegetables from 2000 onwards, starting with the most recent.
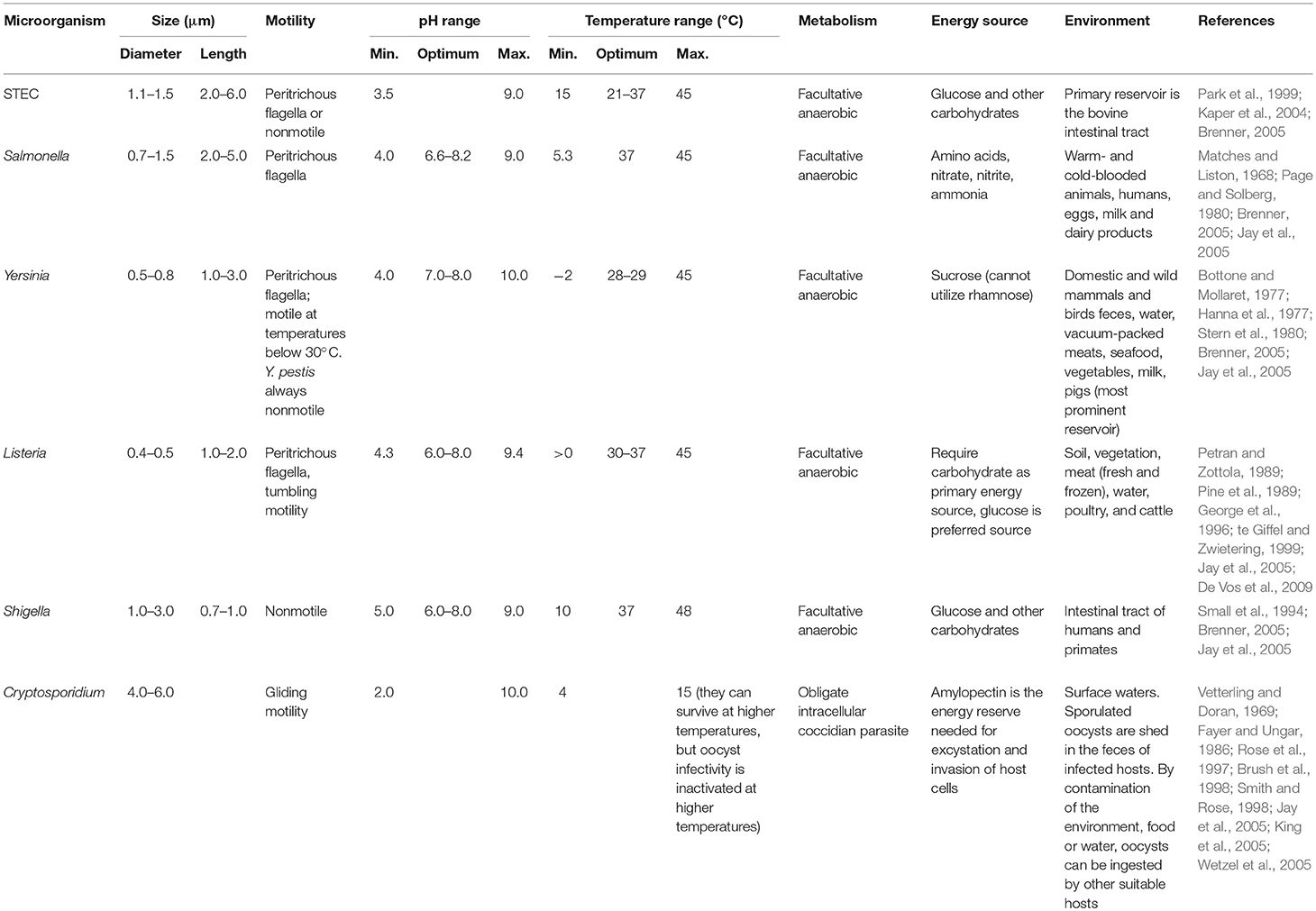
Table 2. Summary of characteristics of microorganisms of most concern on leafy greens from a food safety perspective.
Ready-to-eat salads are a convenient way to ensure intake of vegetables, but consumers need to be confident that the products are safe to eat. However, analysis of RTE salads in Finland has shown that the bacterial quality and safety of packaged fresh LGV is often poor (Nousiainen et al., 2016). It is unclear whether production, processing or distribution practices (or all of these) are responsible for RTE salads of substandard quality, but there is clearly a need for improvements regarding suppression of pathogenic microbes within the entire horticultural value network for LGV, from farm to fork.
Ensuring the microbial safety of fresh LGV presents a unique challenge because the products are consumed raw, with no kill step of pathogens (e.g., heating) at any point in the chain to prevent transmission (Gil et al., 2015). Attempts has been done with aqueous ozone, that could potentially lead to a log 2 reduction of E. coli, without detrimental effects on the chemical characteristics of the vegetables (Karaca and Velioglu, 2014). In some countries disinfectant wash, e.g., a chlorine solution, is an option, but it is unclear if this is sufficient to guarantee the food safety due to the risk of internalized bacteria that cannot be reached. There are also indications that the bacteria can adapt to the sanitizer stress with increased resistance to hydrogen peroxide and calcium hypochlorite (Kyle et al., 2010) in combination with increased expression of virulence determinants—potentially increasing the risks. Furthermore, standard commercial washing and distribution conditions may be insufficient to reliably control human pathogens on fresh produce (Hutchison et al., 2017). Pathogens can be adept at adhering to leaf surfaces and potentially penetrating into internal leaf structures, which limits the usefulness of chemical sanitation methods in preventing pathogen transmission via contaminated produce (Lynch et al., 2009).
Critical steps in the value network for LGV are: (1) Primary production, (2) harvest, (3) washing and processing, (4) packaging, and (5) handling, distribution, display, and retail. It is vital to take account of all these steps when considering microbial safety. For example, in an E. coli outbreak in Sweden in 2005 with 135 cases, production conditions were implicated in the safety of the final produce because cattle shedding STEC at a farm upstream from the irrigation point resulted in STEC-contaminated lettuce (Söderström et al., 2008). Irrespective of contamination source, there are numerous abiotic and biotic variables that influence conditions on the surface of leafy greens (Figure 1). This in turn modulates continued growth and survival of introduced pathogenic microbes (Tomás-Callejas et al., 2011). For example, E. coli and Salmonella achieve high numbers on young leaves, suggesting that leaf age affects pre-harvest and post-harvest colonization by pathogens (Brandl and Amundson, 2008).
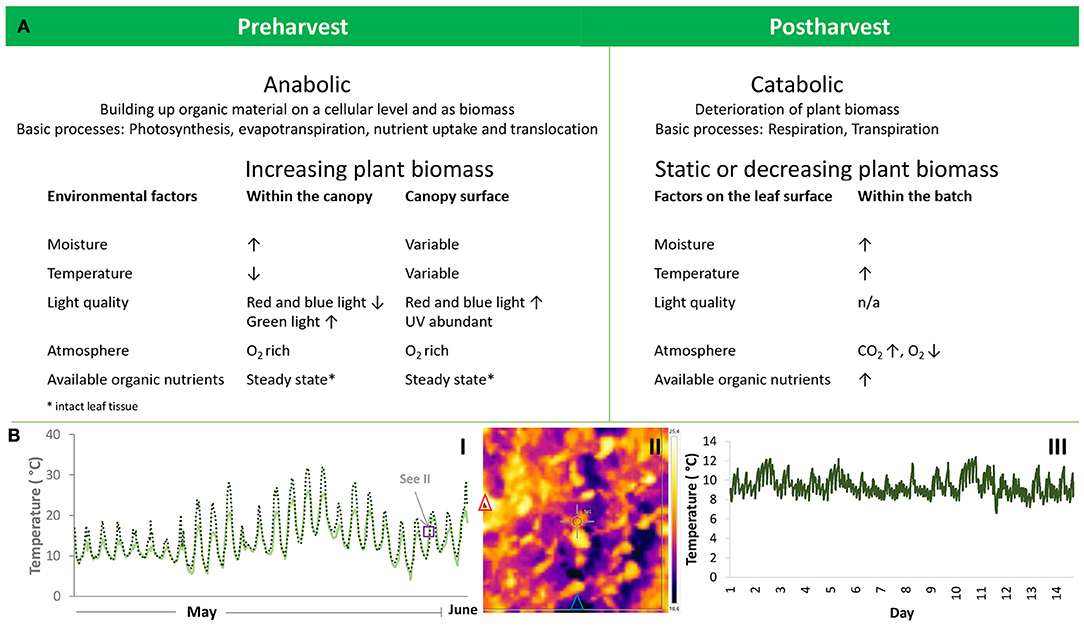
Figure 1. Environmental conditions prevailing during pre- and post-harvest of leafy vegetables and affecting the fate of phyllosphere organisms. (A) Change of the plant matrix from pre- to post-harvest and related alterations in the physio-chemical phyllosphere environment. (B) Temperature as an example of fluctuations in the phyllosphere environment during pre-harvest (I: field environment; II canopy surface) and post-harvest (III: household refrigerator). I: Diurnal changes in atmospheric temperature (solid line) and crop stand temperature near the soil surface (broken line) monitored in southern Sweden during 3 weeks in May and June 2014 in a baby leaf spinach crop. Atmospheric temperature was recorded once per hour and crop stand temperature every 30 min. The purple box indicates the time window for assessment of leaf temperature displayed in II. II: Leaf surface temperature of field-grown baby leaf spinach. The image was taken with an infrared camera. The red and blue triangles indicate the sites with the highest and lowest temperatures in the section. III: Temperature fluctuations in a household refrigerator where temperature was recorded every 5 min during a 14-day period. (Pictures and original data in the figures have previously not been published. Photo by Beatrix Alsanius).
Overall, microbial hazards are significant for fresh produce. Therefore, ways to reduce sources of contamination and a deeper understanding of pathogen survival and growth on fresh produce are required to reduce the risk to human health and the associated economic consequences (Alegbeleye et al., 2018). As there is no single solution for ensuring safety of LGV, the hurdle approach appears to be an appropriate treatment system for this market section of produce. In the next sections of this review, we provide essential background by describing disease outbreaks and then define the hurdle concept. In terms of scope, our review assesses abiotic and biotic parameters relevant for bacterial pathogens on leafy greens, in order to understand the biological underpinnings of hurdles, and summarizes existing hurdles. We also consider potential hurdles and the research needed to develop these.
Nature of Recorded Disease Outbreaks
EFSA (2013) ranked combinations of pathogens and foodstuffs of non-animal origin including fruit, vegetables including leafy green, salads, seeds, nuts, cereals, herbs, spices, fungi, and algae (EFSA, 2013). The food safety concerns are a consequence of that many foods of non-animal origin are consumed as ready-to-eat foods in which the constituents are raw or minimally processed (e.g., fresh-cut and prepacked).
EFSA noted that food of non-animal origin such as vegetables were implicated in 10% of the reported outbreaks during 2007–2011. However, those outbreaks were larger and more serious as they involved 26% of the cases, 35% of the hospitalizations, and 46% of the deaths. The huge VTEC O104 outbreak linked to consumption of contaminated fenugreek sprouted seeds in Germany 2011 as well as other European countries influenced these numbers (Beutin and Martin, 2012). However, if excluding this outbreak, still 18% of the cases were linked to consumption of foods of non-food origin.
The risk ranking was done based on following criteria: (a) Strength of association between consumption of the foodstuff and disease associated with the specific pathogen; (b) Incidence of illness and burden of the disease; (c) The dose response curve—high rank if the curve indicated that low doses could cause infection and disease; (d) Amount consumed of the vegetable; (e) Prevalence of contamination found on the vegetable, and (f) Growth potential of the pathogen during the shelf life.
Subsequently, the risk ranking identified Salmonella in leafy greens eaten raw, as the main concern. Moreover, the combinations of Salmonella spp. and bulb or stem vegetables; tomatoes; and melons was also a concern. The combination of pathogenic Escherichia coli and fresh legumes or grains were also suggested posing a risk.
A total of 29 outbreaks that occurred world-wide between 2000 and 2016 are listed in Table 1. Bacteria were responsible for most of these outbreaks, with STEC and Salmonella being the most commonly implicated infectious agents. The STEC outbreaks were more commonly reported in the U.S. and Canada, while the majority of the Salmonella outbreaks were reported in European countries (Table 1). Almost half of all cases were associated with consuming LGV outside the home, e.g., in restaurants, fast food outlets or school cafeterias. In general, vegetables are more frequently consumed by women (Takkinen et al., 2005; CDC, 2006, 2012b; Söderström et al., 2008; MacDonald et al., 2011; Marder et al., 2014) and this is reflected in the majority of cases reported being female (Table 1). Between 2000 and 2008, the crops associated with listed outbreaks were unspecified “lettuce,” iceberg lettuce, rocket and (baby) spinach, while in more recent epidemics salad mixes (“RTE salad mix,” “pre-cut mixed salad,” “bagged salad,” “pre-packed salad”) and romaine lettuce were implicated (Table 1).
Considering the ephemeral nature of leafy vegetables, which means that they need to be eaten quickly or disposed of, in conjunction with the fact that the incubation period of enteric pathogens can be several days, it is not surprising that the causative pathogen is only detected in suspected produce in less than one-third of cases (Table 1). This inability to conclusively link a causal agent to a disease outbreak is a hallmark of many foodborne illnesses. Therefore, most outbreak investigations rely on epidemiological evidence to obtain an indication of the possible contamination source and transmission path. In Finland, restaurants are advised to keep frozen samples of served foods for at least 2 weeks, to enable microbiological investigations after possible outbreaks (Åberg et al., 2015). This system for internal quality control should be encouraged in other countries and the storage period required should be extended to at least 4 weeks, since it typically takes more than 2 weeks from point of infection to confirmation (CDC, 2017). To further complicate source tracking, many LGV products implicated in outbreaks are mixes of varieties with different origins (MacDonald et al., 2011; McKerr et al., 2015). For example, in an outbreak of yersiniosis in Norway in 2011, the salad mix implicated contained four varieties of leafy vegetables originating from 12 suppliers in two countries, while the vegetables were mixed, washed, and packaged in Norway (MacDonald et al., 2011).
Identification of the offending produce enables subsequent trace-back investigations, which can identify potential suspects responsible for the original contamination further upstream within the value network (Horby et al., 2003; Slayton et al., 2013; Public Health England (PHE), 2015; Buss et al., 2016a,b). However, there have only been two outbreaks for which bacteria matching the outbreak strain have been isolated from environmental samples on, or in close proximity to, suspected farms (CDC, 2006; Grant et al., 2008; Söderström et al., 2008). Sweden and the U.S. experienced EHEC outbreaks in 2005 and 2006, respectively, and in both cases the outbreak strain was isolated from cattle (both countries) and feral swine (U.S. only) at or adjacent to the specific primary production site (CDC, 2006; Grant et al., 2008). Irrigation water contaminated with feces is often suggested to be the culprit for LGV contamination with pathogens (Ethelberg et al., 2010; Åberg et al., 2015). Furthermore, in a Finnish Cryptosporidium outbreak in 2012, it was speculated that contaminated water splash from heavy rain events during the growing period caused infection of growing produce in the field (Åberg et al., 2015).
The rapid distribution of foodstuffs via complex distribution networks has the capacity to distribute foodborne infection swiftly and widely. When only a few cases are infected by each pathogen in a country, outbreaks can be very difficult to detect locally or nationally. Therefore, international communication networks such as the Rapid Alert System for Food and Feed (RASFF) and genotyping approaches such as PulseNet are very important in outbreak investigations focusing on leafy vegetables distributed to different countries (Nygård et al., 2008).
The Hurdle Concept
Food of animal origin can undergo a thermal process in order to inactivate human pathogens. Leafy green vegetables (LGVs) are eaten raw and cannot be treated the same way due to induced physiological damages and deterioration of the organoleptic properties. The limitation of disinfectants to completely eradicate food borne pathogens on fresh produce could partly be explained by the localization of bacteria in protected niches, cracks and crevices, or that they are even internalized and protected from direct contact (Sela and Manulis-Sasson, 2015). Secondly, biofilm formation on the plant's surface protects the pathogens from antimicrobial agents. The challenge is thus to design a network for safe LGV from field to fork that preserves freshness, prolongs shelf-life and maintains quality. However, there is no single risk mitigation measure that can achieve acceptable food safety for LGV. This lack of efficient kill-step means that the fresh produce industry must rely on preventive measures such as good agricultural practice (GAP) and hazard analysis of critical control points (HACCP) (Sela and Manulis-Sasson, 2015). This approach requires decisions based on evidence and a framework for describing the decision process within the supply chain (Monaghan et al., 2017).
Hurdles, or “the hurdle approach,” is a strategy employed in the food chain for meat and animal-based products (Leistner, 2000), but can be adopted as a novel approach for LGV. The hurdle approach has been proposed to some extent before. Some studies have suggested the use of natural agents as hurdles, other the use of bacteriocins, modified athmosphere and a strict management of temperature and storage times (Wadamori et al., 2017). But, regardless of the type of hurdles, the basis of the hurdle concept is a combined approach incorporating two or more practices (or hurdles), each of which may not be sufficient to control foodborne pathogens by itself, but together they can reduce or eliminate microbial hazards (Leistner, 2000). The main aim of the hurdle approach is to achieve microbial safety, product stability, organoleptic and nutritional quality, and economic viability of food production. “Fit for purpose” is key with the approach, as each aim must be achieved without detriment to the other aims.
The magnitude of microbial reduction achieved through use of hurdles will vary depending on circumstances in primary production, e.g. whether a large microbial burden (both pathogens and spoilage organisms) is assumed or not. For milk, an example of a foodstuff with high microbial burden, the key hurdles are pasteurization, cool storage and protection against cross-contamination. pasteurization of milk (72°C for 15 s) can reduce the bacterial load by around 7 log10 units for L. monocytogenes, Salmonella Typhimurium, and pathogenic E. coli (Pearce et al., 2012). However, pasteurization is not an option for fresh leafy greens. Instead, the equivalent key element of safe LGV production must be to minimize the pathogen and spoilage burden pre-harvest and to maintain a low burden throughout the whole food value network.
Pathogenic Organisms Most Likely to be Found on LGV
Shigatoxigenic E. coli, Salmonella, L. monocytogenes, Yersinia spp., Shigella, and Cryptosporidium are the pathogens of greatest concern as regards LGV and food safety (Table 2). Apart from the protozoan Cryptosporidium, these are all facultative anaerobic bacteria and the majority are motile. They can grow across a wide pH range, from strongly acidic (pH 2 and 3.5 for Cryptosporidium and STEC, respectively) to alkaline (pH 9–10). They have an optimum temperature for growth of between 21 and 37°C, although some are capable of growth at much lower temperatures, close to 0°C (e.g., Y. enterocolitica and L. monocytogenes) (Table 2). Some are widespread in the environment (i.e., L. monocytogenes) and most are found in the gut and feces of warm-blooded animals.
Abiotic Factors to be Considered in the Hurdle Approach
Temperature
Environmental conditions prevailing during pre- and post-harvest of leafy vegetables and affecting the fate of phyllosphere organisms are summarized in Figure 1. One of the most influential drivers of plant and microbial activities and growth is temperature. Within the path taken by LGV through the food system, there are three marked phases that can be delineated, namely pre-harvest, harvest, and post-harvest. Each stage displays distinct temperature kinetics due to the physiological status of the plant material and the surrounding ambient temperature conditions.
For a human pathogenic bacterium residing in the phyllosphere, the most critical factor is the temperature on the leaf surface, which is a function of wind speed, air temperature, transpiration rate, and diffusion resistance in plant tissues (Gates, 1968; Larcher, 2003).
There are complex interactions between the parameters influencing leaf temperature. For example, cushion, and rosette plants (e.g., baby spinach, Swiss chard, rocket) form a closed canopy close to the soil surface and are less prone to convection energy losses (cooling by wind), which in turn allows temperatures to rise above air temperature (Figure 1). However, this effect only applies to the interior of the canopy, while leaves in the exterior canopy border interfacing with the atmosphere are more exposed to convection losses (Larcher, 2003). Temperature relationships relating to plant architecture have not been studied specifically for head-forming leafy vegetables, such as different types of lettuce.
Horticultural production systems incorporate many management practices, including mulching, row covering and low tunnels, which significantly alter the thermal environment of cropped plants (Krug et al., 2002). For example, temperature fluctuations (amplitude) are greater under black polyethylene film and lower under white polyvinylchloride film (Takakura and Feng, 2002). Apart from the thermal impact, mulch using polymeric films shields the crop from the soil surface and thereby reduces contamination of the foliage through soil splash.
Harvesting is a decisive intervention in the physiological and thermal conditions of leafy vegetables. From a physiological perspective, harvesting involves detachment of the leaf and hence interruption of the water flow continuum. Harvested leaves are subject to respiration, leading to a temperature increase, and to dehydration, especially if the relative humidity of the surrounding air is low. To maintain organoleptic properties, leaf integrity and the bioactive compound content, harvested leaf biomass is generally stored under cool conditions during transport, processing and packaging, distribution, retail and before preparation and consumption. Leafy green vegetables are highly perishable and any signs of decay strongly diminish their appeal to consumers. Moreover, during decay they also lose organic nutrients from the leaf matrix, favoring microbial growth. Harvested leafy vegetables should thus be cooled as soon as possible to prevent water loss and respiration and to deter microbial proliferation (Paull, 1999). Different cooling methods are available, e.g., room cooling, forced air cooling, hydro cooling, vacuum cooling, water-spray vacuum cooling etc. (Thompson et al., 2002). These methods differ in terms of their cooling efficiency, and thus in their effect on the final produce quality, so the method employed should be chosen with respect to target produce, infrastructure and economic considerations at the processing facility. In addition, positioning of harvested trays affects the rate of cooling, which progresses more rapidly in trays placed in the middle of a stack than in trays at the top or bottom of the stack (Rediers et al., 2009).
There is of course a time lapse between harvesting and the onset of adequate cooling temperatures. In this context, seasonality is important. Onset of target cooling temperatures is delayed when harvest takes place at elevated outdoor temperatures, which can ultimately reduce produce quality and increase microbial proliferation (Rediers et al., 2009; Garrido et al., 2015). High outdoor temperatures during harvesting also promote respiration, so pre-cooling using a hydro or vacuum method may be preferable as this increases leaf water content (Garrido et al., 2015). In summary, important hurdles to consider are preferentially harvesting LGV at lower outdoor temperatures and using a pre-cooling method by which target temperature can be reached faster or respiratory water loss can be mitigated.
Post-harvest temperature management is the most critical hurdle for LGV. According to the University of Florida's Institute of Food and Agricultural Sciences (UF-IFAS) recommendations for processing, post-harvest temperature for LGV should not exceed 5°C (Garrido et al., 2004). In reality, however, there are large variations in the ability to maintain this target temperature throughout storage, processing, distribution, and retail of LGV.
Post-harvest temperature management can change in different phases. A comprehensive study by Rediers et al. (2009) found that temperature was well managed during the post-harvest path of endive until distribution to restaurants, whereupon breakdowns in temperature maintenance occurred, with temperature increases of up to 4°C. Considerable temperature fluctuations were also noted during up to 4 days of storage at restaurants (Rediers et al., 2009). In contrast, Zeng et al. (2014) found that low temperatures are often not maintained during transport, retail storage, and even display (Zeng et al., 2014). Transfer points within post-harvest paths seem especially vulnerable to failures to maintain low target temperatures (Koseki and Isobe, 2005).
There is strong consumer awareness that LGV need to be refrigerated (Jacxsens et al., 2015), but measurements of the actual temperature achieved during domestic storage (refrigeration) show that home refrigerators may be unable to maintain isothermal conditions consistently below the target 5°C (Figure 1). The type and design of the refrigerator, its placement within the kitchen (close to a heat source), operating conditions, the temperature setting and the number of door openings affect the temperature distribution within refrigerators (Laguerre et al., 2002). Despite the fact that LGV are highly perishable, appropriate domestic storage is not always achieved. A study by Marklinder et al. (2004) found that almost one-third of respondents stored RTE salads in the warmest locations of their refrigerator, where mean storage temperature was 6.2°C and the highest refrigeration temperatures recorded were 11.3–18.2°C (Marklinder et al., 2004). From a food safety perspective, such high refrigeration temperatures should be considered an abuse temperature regime under which many human pathogenic bacteria are capable of growth. Similar data have been reported previously (Carrasco et al., 2007), and in a recent study the risk of waste of fresh-cut iceberg salad (partly due to microbial aspects) increased manifold with higher fridge temperatures (Manzocco et al., 2017).
Few studies have examined the temperature dynamics of perishable produce at the point of display. Thomas et al. (2014) monitored temperature dynamics during 3 days at 24 schools using cooling wells, cooling plates or healthy carts. They found that the temperature of the LGV displayed varied substantially and that it remained below 5°C only in a “holding cooler.” The mean time above 5°C for the other devices they tested followed the order: loose cooling pan>cooling plate>cooling well>display cooler (Thomas et al., 2014).
The fate of foodborne pathogens inoculated onto different horticultural commodities eaten raw and exposed to different temperature regimes has been well analyzed. The majority of these studies have analyzed E. coli O157:H7, Salmonella and L. monocytogenes but the temperature regimes employed differ, although most have been conducted under isothermal conditions. It is important to note that the term “abuse” temperature' used in the literature does not consider one specific threshold, but is arbitrarily assigned in different studies. In general, the literature shows that proliferation of E. coli O157:H7 is slowed down at lower temperatures (8°C compared with 12°C), but that this decline can be negated by extended storage time (Luo et al., 2009). This pathogen does not grow, but survives, at 5°C (Luo et al., 2010). Similar results have been reported for Salmonella Hadar inoculated on washed and shredded white cabbage, with survival but no growth at 4°C, but growth observed at 12°C and 20 °C (Piagentini et al., 1997; Delbeke et al., 2015).
In a study where E. coli O157:H7 gfp+, L. monocytogenes, and Y. enterocolitica were inoculated onto baby spinach and stored at 8 and 15°C for 7 days, only populations of Y. enterocolitica increased significantly and exclusively under temperature abuse (15°C) (Söderqvist et al., 2017). Intactness of LGV has an impact on nutrient availability, and hence proliferation of contaminants. Khalil and Frank (2010) found that the occurrence of E. coli O157:H7 was particularly promoted on bruised parsley and bruised spinach at 12°C during 4 days of storage and that growth of E. coli O157:H7 on bruised spinach, but not on bruised coriander cilantro, romaine lettuce and parsley, was selectively promoted after 3 days of storage at 8°C (Khalil and Frank, 2010). Interestingly, processing treatment affects the fate of E. coli O157:H7 during storage at different temperature regimes (Lopez-Velasco et al., 2010). At low temperature (4°C), E. coli O157:H7 is internalized into cut iceberg lettuce tissue more efficiently than at higher temperatures (7, 25, and 37°C) (Takeuchi and Frank, 2000).
In a study that simulated temperature conditions during transport and retail, E. coli O157:H7 counts were found to be approximately 3 log colony-forming units (CFU)/g in transport and the population increased by 0.1 to 3.0 log CFU/g during retail storage, but did not increase further during retail display (Zeng et al., 2014). In similar temperature simulations, L. monocytogenes substantially increased under retail storage temperatures (by 3.0 log CFU/g) and retail display temperatures (by 1.1 log CFU/g) (Zeng et al., 2014). Considering the interaction between human pathogens and temperature conditions, a hurdle approach for LGV must include strict temperature management to avoid temperatures that can support growth of pathogens. Access to data on the temperature history of LGV batches can be an important complement, so that storage times can be adjusted according to previous temperatures experienced by the produce.
Irradiation
Light is a critical factor in crop production, due to its impact on plant biology. Light quality (spectral distribution) and quantity (day light integral, light intensity) and diurnal changes (light period/day length, sunfleck and diurnal artificial light modulation) are all important in governing plant physiological and metabolic processes. By extension, parameters that influence vital plant processes will also have an indirect impact on phyllosphere microbiota residing on the leaf surface. This indirect effect is primarily mediated by nutrients leached from the leaf onto the surface, but temperature and humidity are secondary light effects to be considered, as they have a direct impact on bacteria (Alsanius et al., 2017). Interestingly, recent findings demonstrate that light can have a direct influence on the metabolism of phyllosphere bacteria, even those that are nonphototrophic (Gharaie et al., 2017).
Only a few studies have considered the impact of light source, quantity and quality on the epiphytic phyllosphere microbiota. Of these, most consider light quality interactions, while light source and quantity receive less attention. It has been shown that variations in cumulative photosynthetically active radiation (PAR) in a lettuce crop under field conditions cause a shift in the abundance of bacterial families, with e.g., Betaproteobacteria decreasing and Gammaproteobacteria increasing between the highest and lowest light regimes (Truchado et al., 2017). However, it is not clear whether this effect is due to variations in PAR, light quality or other abiotic factors associated with shielding. Light quality studies have predominantly focused on the impact of different UV-bands on epiphytic bacteria. An impact of light source and light quality has been demonstrated in greenhouse experiments, where UV-B irradiation changed the microbial phyllosphere community structure of various crops (Newsham et al., 1997; Jacobs and Sundin, 2001; Kadivar and Stapelton, 2003). However, pigmented phyllosphere bacteria are equipped with UV-B protection strategies, to mitigate DNA damage (Jacobs et al., 2003). Other protection strategies for preventing detrimental UV-A effects are based on quenching reactive oxygen species, e.g., using an avobenzone-like compound that absorbs UV-A found in Methylobacterium, a phyllosphere resident (Yoshida et al., 2017). Furthermore, blue light receptor domains have been found in several phyllosphere bacteria and human pathogens, which indicates that the ability to detect blue light may be more common than previously thought (van der Horst et al., 2007; Ondrusch and Kreft, 2011; Wu et al., 2013; Río-Álvarez et al., 2014; Alsanius et al., 2017; Gharaie et al., 2017). Recent results indicate that both light quality and nutritional factors are critical for bacterial nutrient utilization and propagation of phyllosphere bacteria and may also dictate colonization success (Gharaie et al., 2017).
In the context of light microbe interactions in crop stands, it is worth mentioning that the ambient light quality conditions only reflect those to which the top of the crop is exposed. When a covering canopy has developed, this results in light quality stratification as basal leaves are shaded (Alsanius et al., 2017). While managing irradiation to mitigate enteric pathogens may not be feasible in a field setting, it may have some potential in a greenhouse setting where light conditions are more controlled. However, much work still needs to be done in order to determine whether light manipulation is an achievable hurdle and irradiation currently does not make any practical contribution as a hurdle.
Water Availability and Moisture/Humidity
Leaf moisture is a function of the dynamics of air and leaf temperature and relative air humidity, and thus also of crop transpiration. Leaf wetness, by contrast, is very difficult to define, because various portions of leaves and canopies are wet and dry at different times (Huber and Gillespie, 1992). Leaf moisture is also dependent on plant morphological properties, such as leaf topography, cuticle morphology and composition, and the density of trichomes. The fate of an bacterium on the leaf surface is dependent on the leaf surface structure in combination with the amount of nutrients and water available (Monier and Lindow, 2005). If bacterial cells arrive on the leaf surface within droplets, such as rain splash or irrigation water droplets, they can move with these droplets across the leaf surface and aggregate at sites where water remains for the longest time during subsequent drying (Monier and Lindow, 2005). The crop surface is exposed to sunlight and desiccation, which leads to shorter survival times of pathogenic microorganisms on crops compared with in water and soil. If pathogenic microorganisms remain viable in the soil, they are able to re-contaminate plants during irrigation and rainfall (Steele and Odumeru, 2004). Although there is evidence that both S. enterica and E. coli O157 have the ability to colonize some plant species, they usually fail to grow on leaves under dry conditions (Lindow and Brandl, 2003).
One prominent cultural management measure affecting leaf moisture and relative humidity in the crop stand is irrigation. Moreover, irrigation water of inferior hygienic quality (surface water, reclaimed water, non-treated and treated sewage water) is listed as one of the most decisive vehicles for human pathogen transmission to LGV. As leafy vegetables have a shallow root system, they need to be irrigated regularly (e.g., every 3 days under sunny and warm Scandinavian conditions, with an irrigation water volume of 5 mm/event). Bacterial pathogens contained in surface water used for irrigation are generally re-isolated from the surface of the irrigated crop (Islam et al., 2004; Ijabadeniyi et al., 2011). Irrigation water may also mediate internalization of human pathogens into the leaf tissue, using the stomata as points of entry (Kroupitski et al., 2009a). Several measures have been suggested to prevent transmission of human pathogens through irrigation water (Allende and Monaghan, 2015). First, it is important to monitor pathogen and indicator species in water sources. Second, available physical and chemical water treatment methods to remove human pathogens from the irrigation water source should be applied. Third, the irrigation method used should be considered (Allende and Monaghan, 2015). Methods such as subsurface drip irrigation avoid direct contact with the edible parts of the crop, but more knowledge is required on the risks posed by root contamination and internalization of bacteria. Soil splash created by “rain-sized” water droplets can transfer enteric bacteria from soil to leaves (Monaghan and Hutchison, 2012), but drip and overhead sprinkler irrigation do not influence the survival of E. coli O157:H7 on the lettuce leaf surface (Moyne et al., 2011). This means that there is a complex web of conditions affecting the microbial quality of irrigation water (Pachepsky et al., 2011; Liu et al., 2013).
Nutrients
Phyllosphere bacteria, including introduced pathogenic bacteria, must have access to nutrients in order to either sustain or grow populations. There is a lack of published data regarding nutrients encountered on the surface of LGV, but well over 180 plants have been assessed to establish the kinds of nutrients that may be present in the phyllosphere (Tukey, 1970). Therefore, predictions concerning the nutritional environment of LGV surfaces can be made based on generalities established for the wide variety of plants already examined (Morgan and Tukey, 1964; Tukey, 1966, 1970). The types of nutrients that are commonly present on the leaf surface can be categorized into inorganic and several classes of organic molecules (Morgan and Tukey, 1964). Inorganic elements or ions include boron, calcium, copper, iron, sodium, magnesium, nitrogen, phosphorus, silica, strontium, sulfur, and zinc (Tukey and Mecklenburg, 1964; Mecklenburg et al., 1966; Tukey, 1966, 1970).
Organic molecules include most of the known amino acids (Tukey, 1966, 1970; Rodger and Blakeman, 1984), as well as many organic acids, with a preponderance of tricarboxylic acid (TCA) cycle intermediates such as citric, fumaric and succinic acid (Morgan and Tukey, 1964; Tukey, 1966, 1970). Carbohydrates are of especial interest, due to their ability to readily support growth of enteric bacteria such as E. coli, and dominant phyllosphere sugars are fructose, glucose and sucrose (Morgan and Tukey, 1964; Tukey, 1966, 1970; Fiala et al., 1990; Dik et al., 1992; Mercier and Lindow, 2000; Leveau and Lindow, 2001; Aruscavage et al., 2010). Phylloplane nutrients may be of endogenous origin (Brown, 1922; Morgan and Tukey, 1964; Tukey and Mecklenburg, 1964; Mecklenburg et al., 1966; Leveau and Lindow, 2001), through passive leaching from within leaves themselves, or of exogenous origin, via deposits such as pollen, insect frass, and aphid honeydew (Rodger and Blakeman, 1984; Dik et al., 1991, 1992; Stadler and Müller, 2000). Two distinct hallmarks of the phyllosphere with respect to nutrients are nutrient scarcity and heterogeneous distribution, which represents a challenge for phyllosphere bacteria (Frossard et al., 1983; Haller and Stolp, 1985; Fiala et al., 1990; Leveau and Lindow, 2001). The heterogeneous distribution of nutrients indicates that nutrients leach in a non-random fashion across the leaf surface, with cracks and natural openings representing sites where greater amounts of nutrients are present (Leveau and Lindow, 2001). There is direct evidence that these phyllosphere nutrients are taken up and utilized by microbial residents and also that they represent an important source supporting microbial activities (Frossard et al., 1983; Rodger and Blakeman, 1984; Leveau and Lindow, 2001). Activity of fungi and bacteria are often restricted on the leaf surface due to nutrient limitations and there is a positive correlation between the amount of available nutrients and microbial growth (Bashi and Fokkema, 1977; Fokkema et al., 1979; Rodger and Blakeman, 1984; Mercier and Lindow, 2000). Leaching is enhanced by high water content and a moist or wet leaf releases more nutrients into the phyllosphere than a drier counterpart (Tukey, 1966). Wounds and injuries that breach the leaf surface, whether by biological or mechanical means, increase nutrient availability, with a concomitant increase in growth of microbes on the plant surface, including E. coli (Tukey and Morgan, 1963; Dingman, 2000; Jablasone et al., 2005; Aruscavage et al., 2008, 2010). Consequently, processing practices that inflict wounds or injuries, such as minimal processing of leafy greens in the form of chopping and shredding, cause a nutritional pulse that increases growth of E. coli and other resident microbes (Brandl, 2008). In conclusion, approaches to mitigating the risk of foodborne illnesses associated with produce and produce spoilage should include practices that aim to decrease leaching of nutrients. Considering that leaching is greatest from leaves that are injured, older and/or wet (Morgan and Tukey, 1964; Tukey, 1970; Aruscavage et al., 2010), then appropriate hurdles for enteric bacteria would be to avoid extended post-harvest storage periods and to avoid post- and processing approaches where leaves are either wetted for extended periods or subjected to practices that cause wounding or injuries.
Oxygen/Package Atmosphere
Once harvested, leaves will continue to respire and if placed in sealed packages they will modify their own atmosphere due to consumption of ambient oxygen (O2), while releasing carbon dioxide (CO2). The aim of modified atmosphere packaging (MAP) is to extend the shelf-life of produce by slowing down respiration of the leaves. This is achieved by altering the gaseous environment, which can be accomplished either by harnessing innate produce respiration (passive MAP) or by adding or removing gases to manipulate the levels of O2 and CO2 (Oliveira et al., 2010). In general, a lower level of O2 (3–6%) and a higher level of CO2 (2–10%) induce a lower respiration rate in produce. Nitrogen may be used as a filler gas, to prevent collapse of the package (Sandhya, 2010). Of the gases used in MAP, CO2 is the only one with demonstrated antimicrobial activity olive (Oliveira et al., 2015). However, this inhibitory effect is not general and depends on many factors related to the microorganism, storage conditions, and product characteristics. Produce for which MAP is used may be vulnerable from a food safety perspective, as the associated extension of shelf-life may allow growth of pathogenic bacteria, while also inhibiting growth of organisms that usually make the consumer aware of spoilage by off-odors (Farber et al., 2003). The O2 and CO2 levels that develop inside the package due to different types of packaging films do not appear to affect survival and growth of E. coli O157:H7 on different types of fresh produce at 5°C, because growth of E. coli O157:H7 is predominantly dependent on temperature and to a lesser extent on atmospheric conditions (Abdul-Raouf et al., 1993; Oliveira et al., 2010; Abadias et al., 2012). Similar findings have been reported for S. Typhimurium and S. choleraesuis (Oliveira et al., 2010; Lee et al., 2011). The above also holds true for the psychrotrophic pathogen L. monocytogenes, which is of particular interest as it can grow at refrigerator temperatures. There is convincing evidence that L. monocytogenes populations grow unaffected, or persist, in MAP with high levels of CO2 at between 3 and 14°C (Kallander et al., 1991; Carlin et al., 1996; Jacxsens et al., 1999; Scifo et al., 2009; O'Beirne et al., 2015). Furthermore, high CO2 has been shown to promote growth of L. innocua, a bacterium which is often used as a valid model organism instead of the pathogenic L. monocytogenes (Geysen et al., 2006; Escalona et al., 2007). Thus, there is a risk that the level of L. monocytogenes will exceed the EC limit (100 CFU/g) if it is present even at low initial levels when stored in MAP. However, there are conflicting results, e.g., in one study an E. coli O157:H7 population was noticeably reduced in a high-oxygen (70 kPa O2) modified atmosphere (MA) compared with a low-oxygen (5 kPa O2) MA (Lee et al., 2011). It has also been shown that near-ambient air conditions support lower populations of E. coli O157:H7 when stored at 4°C than when stored in active MAP conditions with high CO2 (Sharma et al., 2011).
Apart from the proven importance of proper storage temperatures for fresh produce stored in MAP, other biological characteristics are also important. It has been shown that MAP increases the ability of different isolates of E. coli to survive gastric acid challenge on lettuce stored at abuse temperatures (≥15°C) (Chua et al., 2008). Storage under near-ambient air atmospheric conditions can potentially promote higher expression levels of E. coli O157:H7 virulence factors on lettuce, which could therefore affect the severity of E. coli O157:H7 infections associated with leafy greens (Sharma et al., 2011).
To summarize the role of atmosphere, it is likely that pathogens are more influenced by type of vegetable than by type of atmosphere (Jacxsens et al., 1999). If MAP is used to increase shelf-life, it should be applied with other preservation techniques to ensure inhibition of pathogens, as the MAP technique itself does not appear to represent an impediment to enteric bacteria (Scifo et al., 2009).
pH
Human pathogenic bacteria have a narrow span of pH conditions in which they can survive on the leaf surface (Table 2). A pH value below 4.6 will inhibit growth and toxin production of many pathogens, however, many microorganisms, e.g., Cryptosporidium and STEC, are capable of growth or survival at pH values below this limit (Table 2). Lowering the pH of LGV to prevent growth of pathogenic bacteria may be a problem if it impairs taste due to fermentation (Leistner, 2000). Another issue is that some bacteria can develop resistance against acidity or can increase their virulence when exposed to environmental stress factors, such as osmotic stress, starvation, and suboptimal pH (Kroll and Patchet, 1992; Leistner, 2000). Listeria monocytogenes can develop resistance against acidity (pH 3.5) depending on other environmental conditions (Koutsoumanis et al., 2003). It has also been shown that E. coli O157:H7 can survive acidic conditions, with survival rates influenced by temperature (Conner and Kotrola, 1995). However, Menz et al. (2011) found that growth and survival of E. coli O157:H7 and S. Typhimurium in beer was prevented when the pH was lowered to 4.0 (Menz et al., 2011). In a study where S. enterica was spot-contaminated on lettuce leaves and lettuce pieces were then submerged in acid solution (pH 3.0), increased acid tolerance of attached bacteria compared with planktonic cells was observed (Kroupitski et al., 2009b). This means that altering the pH is probably not a viable hurdle in practical use to prevent growth of pathogenic bacteria on leafy vegetables at any step of the food chain.
Biotic Factors Critical for the Hurdle Approach
In addition to abiotic factors such as temperature, nutrients, water and pH, biotic factors must be taken into consideration when trying to combine different hurdle approaches. In this review, we focus solely on the presence of other bacteria on the leaf surface.
Microbiome Associated With Leafy Greens
The native microbiota of leaves consists of millions of phylloepiphytes (bacteria of the phyllosphere; Lopez-Velasco et al., 2013). This diverse bacterial community varies due to morphological and chemical differences between plant genera. For example, Hunter et al. (2010) found that on all lettuce (Lactuca sativa) accessions they analyzed, the dominant species were from the Pseudomonadaceae and Enterobacteriaceae families, but that Erwinia and Enterobacter genera differed significantly between the accessions. Jackson et al. (2013) found that the amount of total culturable bacteria on salad vegetables ranged from 8 × 103 to 5.5 × 108 CFU/g, with the cultured isolates belonging mainly to Pseudomonas, Chryseobacterium, Pantoea, and Flavobacterium. Culture-independent analysis of the same samples revealed that Gammaproteobacteria, Betaproteobacteria, and Bacteroidetes were the dominant lineages, including genera hosting plant pathogens such as Pseudomonas, Ralstonia, Stenotrophomonas, Erwinia and Xanthomonas, and genera hosting human pathogens such as Serratia, Enterobacter, Bacillus and Staphylococcus. There was no difference in microbial composition between organically and conventionally grown produce (Jackson et al., 2013).
Leafy green vegetables are significant vectors of human pathogens (Table 1). Pathogens that exist as endophytes are often involved in food-borne disease outbreaks of bagged lettuce and spinach, as they are more protected against external procedures such as washing (Uhlig et al., 2017). Although human pathogens are not adapted for growth in the phyllosphere, it has been shown that E. coli O157:H7 and Salmonella can survive at low levels over extended periods of time on plants in the field (Williams and Marco, 2014). Williams and Marco (2014) characterized bacteria on romaine lettuce grown in the laboratory and under field conditions and found that field-grown plants contained 10- to 100-fold higher bacterial load than laboratory-grown plants. Furthermore, field-grown plants contained significantly higher proportions of Gammaproteobacteria, represented primarily by Enterobacteriaceae and Moraxellaceae, among which the family Enterobacteriaceae in particular includes several well-known pathogens such as E. coli and Salmonella. In contrast, laboratory-grown plants were enriched with Betaproteobacteria, represented by the Comamonadaceae and Burkholderiaceae families (Williams and Marco, 2014). The relative quantities of Erwinia, Acinetobacter and Alkanindiges bacteria were significantly higher on field grown plants, whereas laboratory-grown plants carried significantly more representatives of Comamonas, Limnobacter and Pelomonas (Williams and Marco, 2014). It has been found that the uptake of E. coli O157:H7 can be mediated by the microbe itself. In a study by Solomon and Matthews (2005) in which lettuce plants were irrigated with E. coli O157:H7 or FluoSpheres, particles similar in size to bacterial cells but devoid of bacterial surface moieties, appendages or adaptive responses, it was found that both E. coli O157:H7 and FluoSpheres were internalized into growing plants and were present within root and leaf stem tissue. These findings suggest transport of spheres from the root up into edible tissue and, because the level of uptake of FluoSpheres and E. coli O157:H7 was similar, they indicate that entry of E. coli O157:H7 into lettuce plants may be a passive event (Solomon and Matthews, 2005).
Antagonism and Pathogens on Leafy Greens
Co-existence in bacterial communities is controlled by access to space, nutrient use and availability, production of antimicrobial compounds and other strategies to acquire resources. An invading microorganism, including a human pathogen, must successfully co-exist and compete with an already adapted and established bacterial community in order to establish (Lopez-Velasco et al., 2013). Biodiversity seems to affect this delicate equilibrium and a more diverse community is correlated with reduced levels of Salmonella colonization, presumably due to increased presence of antagonistic bacteria (Jackson et al., 2013). Plant microbiome transplantations using freshly transferred and cryopreserved field microbiota have been found to increase bacterial diversity and to result in a bacterial composition similar to field plant microbiota that seems to be stable over time (Williams and Marco, 2014). To examine the effect of an exogenous potential pathogenic organism on the indigenous bacterial communities in the phyllosphere, Williams and Marco (2014) inoculated E. coli O157:H7 onto laboratory-grown romaine lettuce plants containing or lacking the field plant microbiota and found that it resulted in significant shifts in the abundance of certain taxa in both groups. Specifically, bacterial species of the genus Microbacterium were significantly enriched on E. coli O157:H7-containing plants with transplanted phyllosphere microbiota. This genus has previously only been found on field-grown lettuce and has been shown to exhibit antagonistic activity against E. coli O157:H7, so it has been investigated as a biocontrol agent against fungal plant pathogens (Barnett et al., 2006; Pereira et al., 2009; Lopez-Velasco et al., 2012).
Lopez-Velasco et al. (2013) found that 15 genera found on spinach could reduce the growth rate of E. coli O157:H7 in vitro. The majority (83%) of these antagonistic bacteria belonged to the same taxonomic class as E. coli (Gammaproteobacteria), and the remainder belonged to Firmicutes (7%), Bacterioidetes (5%), Actinobacteria (2%), and a small proportion of Alphaproteobacteria and Betaproteobacteria. The mechanisms responsible for the antagonistic effect were reported to be acid production or nutrient competition, mainly of carbon sources. However, the reducing effect was significantly smaller when the antagonists were co-cultured with the pathogen on detached spinach leaves than when grown in vitro (Lopez-Velasco et al., 2013). Escherichia coli growth-stimulating bacteria belonging to Actinobacteria (33%), Bacterioidetes (33%), Alphaproteobacteria (26%), and Betaproteobacteria (6%) were also found. Research on microbe-human pathogen interactions in the phyllosphere is still in the fledgling stages, but insights gained from microbial control of plant pathogenic organisms indicate that such interactions are extremely intricate and complex. A holistic approach, including plant physiological factors and phenology, is therefore of great importance for successful hurdle development based on antagonistic mechanisms.
Bacteriophages in Food Safety of Leafy Greens
During the last 10 years the possible use of bacteriophages has received more attention from the European Union food safety authorities as a practical food safety risk mitigation tool. One important question for regulatory agencies is whether the use of bacteriophages are acting as processing aids or food additives. Processing aids would be the case if reducing numbers of pathogens, but not preventing reinfection in the next steps of the food chain, while if considering use of bacteriophages as a food additive the foods treated with bacteriophages should be safe even in the case of recontamination. The European Food Safety Authority (Anonymous, 2009) issued an opinion on the use of bacteriophages and their modes of action. It appears to be not sufficient to conclude whether bacteriophages in general should be seen as a processing aid or food additive. It was recommended whether the use of bacteriophages should be considered a processing aid or food additive ought to be decided on a case by case basis for each food-pathogen-bacteriophage matrix.
Bacteriophages have two principal mechanisms for reducing number of food borne pathogens either by being temperate by causing “lysis of bacteria from within” where the lysis is caused by multiplication of phage within the bacteria i.e., having a temperate cycle or virulent where the phages are causing lysis without replication i.e., “lysis from without.” One attractive feature given the host specificity of bacteriophages is the ability to eliminate the targeted pathogens specifically while leaving the microflora unharmed (Moye et al., 2018). This in contrast to decontamination methods such as heat treatment or treatment with chlorine or organic acids that kill microorganisms indiscriminately and consequently with an impact on the quality and shelf life of the foodstuff. Kazi and Annapure (2016) and references therein noted that bacteriophages intended for use in food should be strongly lytic, their host range should cover all epidemiologically important strains of the target microorganism and be stable in the intended environment of use (Kazi and Annapure, 2016). If needed a mixture of phages should be used (phage cocktails) to ensure reduction, or preferably elimination, of relevant pathogens. Ensuring a longer shelf life biopreservation and biosanitation by removal of biofilms are further possible areas where bacteriophages could be useful.
Bacteriophages could be used both at the pre-harvest and harvest stages. For example, cattle might be treated with bacteriophages to reduce the shedding of E. coli O157: H7 (Sheng et al., 2006), according to this study the numbers were reduced but not eliminated. Hence, one could use the possibility of phage therapy for cattle grazing upstream in irrigation water catchment areas or grazing nearby–i.e., an indirect hurdle.
For vegetables, Sharma et al. (2009) found that bacteriophages reduced the number of E coli on lettuce and cantaloupe after 2 days of spraying with phage cocktail (ECP-100) (Sharma et al., 2009). It has been concluded that bacteriophages could reduce the number of pathogens by at least one log or 90% (Zaczek et al., 2015). So, at least for Enterohaemorhagic E. coli, Salmonella Sp, and Listeria monocytogenes, the use of bacteriophages could be useful as one amongst several hurdles to ensure food safety. The metabolic activity of the bacteria is very important for the efficacy of bacteriophages. For example, one should not expect a high effect if the temperature is low.
Potential Hurdles
Minimally processed foods are in increasing consumer demand possibly amplifying the consumer risks (Ohlsson and Bengtsson, 2002). In particular, risk mitigation and preservation options are needed to prolong shelf lives and to improve food safety for the following food groups; (i) minimally processed, convenient foods, (ii) chilled foods with “invisible technology”, (iii) healthful foods with less salt and/or fat, (iv) less packaged foods. To achieve these aims Singh and Shalini (2016) suggested combining hurdle technologies with predictive microbiology models) and HACCP to achieve food safety and longer shelf lives (Singh and Shalini, 2016).
Vegetable foods such as leafy greens is one example of such a minimally processed food group. The leafy green food chain does not include a pathogen kill step such as boiling, before consumption. This presents an opportunity for pathogens and spoilage bacteria to multiply resulting in food safety risks if consumed, or shortened shelf lives with consequent increasing volumes of food waste. It has been suggested that hurdles or combinations of different preservation methods and Leistner (2000) suggested there were more than 60 potential hurdles such as temperature, acidity (pH), organic acids, or and competitive microorganisms including bacteriophages that could be considered (Leistner, 2000). The preservation methods may reduce bacterial numbers (bacteriocidic) or inhibit growth (bacteriostatic). For example an uninterrupted cold chain could be an helpful hurdle as bacterial growth are limited, while the bacteria contaminating the food before subject to the cold chain would usually remain throughout, and represent a constant risk.
We believe that the leafy green food chain would benefit by combining the HACCP with predictive microbiology and hurdles (combinations of preservation techniques) as a contextual model for improving food safety. However, any combinations of hurdles and the foreseen impact on pathogens should be validated before implemented on an industrial scale. One reason for this need for validation is that the estimates for pathogen reductions on leafy green are very context specific where e.g., species of pathogens might influence the impacts of hurdles. For example E. coli O157:H7 survives in acidic environments, but better at lower temperatures (Hsin-Yi and Chou, 2001). Consequently, we agree with (McMeekin et al., 2000) that understanding of physiological processes occurring near the growth/no growth interface is crucial quantification of the impact of hurdles and their intelligent application (McMeekin et al., 2000).
As mentioned previously, the value network of LGV does not start with the processing of leaves but already with the seeds. Use of the hurdle approach in LGV production to date has focused mainly on post-harvest measures, even though most of the contamination risks occur pre-harvest, in primary production (Table 3). It is worth noting that LGV are exposed during the entire value chain to unpredictable environments and that boundary layers are not removed when eaten raw. Thus LGV and their environmental footprint are ingested, which makes the hurdle approach more challenging than that used for other products, e.g., dairy and meat.
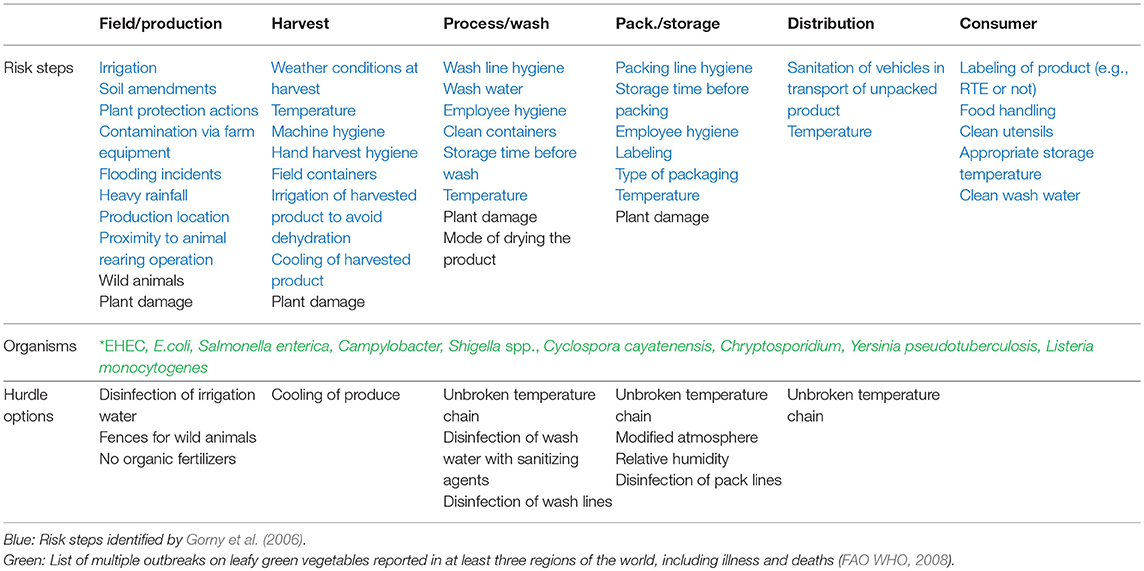
Table 3. Summary of risk steps in the production of leafy green vegetables where contamination of human pathogens could occur and suggested hurdle options for these.
In some cases, more controlled production conditions (soilless production systems) can result in lower initial microbial load. For example, it has been shown that at the end of lettuce shelf-life, soil-grown lettuce can have 1.5 log higher total coliforms than lettuce grown in a soilless system (Selma et al., 2012). In reality, most LGVs are produced in open fields, which means that insights regarding the biology of microbe-microbe and plant-microbe interactions are essential when seeking to determine the route of foodborne pathogen contamination (Critzer and Doyle, 2010) and to develop novel approaches to inhibit or inactivate microbes on LGV.
Possible hurdles for inclusion in the LGV chain could be low temperatures at harvest and along the food chain until consumption (processing, packing, storage, distribution, and pre-consumption), low pH, biopreservation with competitive microflora, aseptic packaging, and washing. The water used for rinsing could be UV-treated and electrolyzed (Rahman et al., 2016) or e.g., chlorine dioxide could be added (Van Haute et al., 2017). By judiciously introducing hurdles such as rinsing and low temperatures (e.g., 0–4 C) at harvest and post-harvest and by using aseptic packaging, a low pathogen and spoilage burden can be maintained and possibly even reduced up to the point of consumption.
Cross-contamination with human enteric pathogens is one of the major issues related to processing of LGV (Allende et al., 2008; Holvoet et al., 2012). Several hurdle options have been explored regarding decontamination of leaves during the washing procedure, with the pathogens commonly considered being E. coli, Salmonella, and L. monocytogenes. These treatments have led to reductions in pathogens, but none of the methods has proven sufficient to eliminate the pathogenic bacterial load completely. A number of studies have investigated the effect on foodborne pathogens of addition of chlorine to the wash water (Behrsing et al., 2000; Beuchat et al., 2004; Gragg and Brashears, 2010; Davidson et al., 2013; Omac et al., 2015; Pezzuto et al., 2016; Guzel et al., 2017). The efficacy of hypochlorite treatment is dependent on the microbial load of the inoculated pathogen and the target organism (Pezzuto et al. (2016). Listeria monocytogenes and L. innocua (used as a surrogate for L. monocytogenes) have been shown to resist treatment even when hypochlorite is administered in high concentrations, but this treatment is still more successful than washing without an additive (Beuchat et al., 2004; Omac et al., 2015; Guzel et al., 2017). Due to their mode of action (they produce inhibitory compounds at low temperature conditions, but do not grow), lactic acid bacteria are considered plausible candidates in LGV processing. Gragg and Brashears (2010) examined the possibility of using lactic acid bacteria alone, and in combination with a chlorine wash, in order to reduce the total bacterial load of spinach leaves. They found that when inoculated at a density of log 6 CFU/g, the chlorine wash reduced E. coli O157:H7 by approximately log 1, the lactic acid bacteria reduced E. coli by log 1.4 CFU/g and the multi-hurdle treatment reduced the load by log 1.9 CFU/g.
Although chlorinated water has been proven to be the most efficient sanitizer of LGV, several other have been tested, with varying degrees of efficiency being reported. For example, peroxyacetic acid has been used against E. coli O157:H7 and L. monocytogenes (Baert et al., 2009) and peracetic acid, percitric acid, sodium bicarbonate and vinegar have been used against Salmonella and Listeria (Pezzuto et al., 2016). Edible plant extract, such as the pulp and juice of lime fruits and oregano, has been used against Salmonella, E. coli O157:H7 and Shigella sonnei (Orue et al., 2013).
Vurma et al. (2009) examined the possibility of implementing a sanitizing step already in the cooling process by combining vacuum cooling with gaseous ozone. This multi-hurdle approach resulted in a reduction of log 2.4 CFU/g (initial density log 7CFU/g), but the process damaged the leaves. With an optimized procedure where no leaf damage occurred, the reduction in E. coli O157:H7 was log 1.8 CFU/g, which is comparable to that achieved with a chlorine wash (Vurma et al. (2009).
Irradiation with UV-C has antimicrobial effects and is sometimes suggested for use as a surface sterilizer of vegetables and other foodstuff, but also for disinfection of water. Hagele et al. (2016) examined the possibility of using low-dose UV-C irradiation (1.2 kJ/m2) on leaf surfaces of endives and for decontamination of the processing water. They found that the reduction in total aerobic bacteria was log 0.9 CFU/g using only UV-C treatment and log 2.1 CFU/g if the UV-C treatment was preceded by washing the endive leaves in warm water (45°C). Treatment of the wash water reduced the total aerobic count in the water by log 1.3 CFU/mL (Hagele et al. (2016).
There are multiple risks of contamination of produce by human enteric pathogens pre-harvest. One of the most common sources of such contamination is irrigation water (Brandl, 2006; Jongman and Korsten, 2017). There are ways of treating the irrigation water prior to use that reduce the bacterial load in the water, such as mechanical filtration using a 0.7 μm filter. This filter size is not sufficient to remove microbial cells, however, although it removes larger particles present in the water that could harbor microorganisms. Another possibility is to use advanced oxidation technology (AOT), where UV-light in combination with a titanium dioxide membrane (TiO2) has an oxidative effect on microorganisms, for example E. coli and intestinal enterococci. López-Gálvez et al. (2018) treated water used for sprinkler irrigation of baby leaf spinach with chlorine dioxide (ClO2; 1–3 mg/L) in a field setting and found that the treatment reduced the load of culturable E. coli by log 0.2–0.3 CFU/100 mL water. However, the chlorine dioxide was suspected to have a bacteriostatic effect on the E. coli, which caused cells to change into a viable but not culturable state (VBNC). Other factors that are considered to pose a risk of microbial contamination in the pre-harvest phase include contamination via farm equipment, heavy rainfall and flooding incidents, proximity to animal rearing facilities, poor hygiene of workers in the field and poor hygiene of crates and containers used in harvest (Gorny et al., 2006). Presence of wild animals and instances of plant damage by equipment used in the field or by weather events should also be considered. Plant damage can provide potential entry points for internalization of human enteric pathogens (Brandl, 2008; Hartmann et al., 2017). In summary, plant damage can occur at all stages of the production chain, both in the field and in processing and distribution, and it is important to minimize such damage in order to maintain high quality throughout the food chain.
Conclusions
In order to improve food safety in general, supporting data need to be more easily available to assist primary producers in their decision-making process. There is also a need to develop hygiene criteria to aid validation of proposed interventions. No single risk mitigation measure can achieve food safety for leafy green vegetables. Instead, a combination of mitigation measures, or hurdles, aimed at controlling and/or eliminating pathogens could ensure food safety and quality and freshness. Based on a review of existing literature, it can be concluded that one key element of safe leafy green vegetable production is a low pathogen and spoilage burden pre-harvest. It can also be concluded that the food chain for leafy green vegetables must be a managed in a holistic way for efficient maintenance of food safety and quality. All stakeholders in the food system need to acknowledge their involvement in food safety. Among measures to mitigate the risk of foodborne illnesses being transmitted by produce, avoiding processing practices that involve significant injury to leaves is paramount.
Choice of hurdle must depend on the expected pathogen burden of harvested leafy green vegetables and the food safety objectives at the point of consumption. For example, under EU Regulation 2075/2005, the food safety objective, and consequently the end-product food safety microbiological criterion, for Salmonella is absence of the microbe in a 25-g sample of ready-to-eat leafy greens at the point of consumption. Therefore in addition to hurdles applied in the harvest and post-harvest stages of the leafy green vegetable value network, hurdles or risk mitigation measures must be adopted in primary production at all growth stages.
Author Contributions
All authors listed have made a substantial, direct and intellectual contribution to the work, and approved it for publication.
Funding
The work with this Review was funded by FORMAS (The Swedish Research Council for Environment, Agricultural Sciences and Spatial Planning). The Review was produced within the FORMAS Project Safe ready to eat vegetables from farm to fork: The plant as a key for risk assessment and prevention of EHEC infections.
Conflict of Interest Statement
The authors declare that the research was conducted in the absence of any commercial or financial relationships that could be construed as a potential conflict of interest.
References
Åberg, R., Sjöman, M., Hemminki, K., Pirnes, A., Räsänen, S., Kalanti, A., et al. (2015). Cryptosporidium parvum caused a large outbreak linked to frisee salad in Finland, 2012. Zoonoses Public Health 62, 618–624. doi: 10.1111/zph.12190
Abadias, M., Alegre, I., Oliveira, M., Altisent, R., and Viñas, I. (2012). Growth potential of Escherichia coli O157:H7 on fresh-cut fruits (melon and pineapple) and vegetables (carrot and escarole) stored under different conditions. Food Control 27, 37–44. doi: 10.1016/j.foodcont.2012.02.032
Abdul-Raouf, U. M., Beuchat, L. R., and Ammar, M. S. (1993). Survival and growth of Escherichia coli O157:H7 on salad vegetables. Appl. Environ. Microbiol. 59, 1999–2006.
Alegbeleye, O. O., Singleton, I., and Sant'Ana, A. S. (2018). Sources and contamination routes of microbial pathogens to fresh produce during field cultivation: a review. Food Microbiol. 73, 177–208. doi: 10.1016/j.fm.2018.01.003
Allende, A., and Monaghan, J. (2015). Irrigation water quality for leafy crops: a perspective of risks and potential solutions. Int. J. Environ. Res. Public Health 12:7457. doi: 10.3390/ijerph120707457
Allende, A., Selma, M. V., López-Gálvez, F., Villaescusa, R., and Gil, M. I. (2008). Impact of wash water quality on sensory and microbial quality, including Escherichia coli cross-contamination, of fresh-cut Escarole. J. Food Prot. 71, 2514–2518. doi: 10.4315/0362-028X-71.12.2514
Alsanius, B. W., Bergstrand, K.-J., Hartmann, R., Gharaie, S., Wohanka, W., Dorais, M., et al. (2017). Ornamental flowers in new light: artificial lighting shpares the microbial phyllosphere community structure of greenhouse grown sunflowers (Helianthus annuus L.). Sci. Hortic. 216, 234–247. doi: 10.1016/j.scienta.2017.01.022
Anonymous (2009). Scientific opinion of the panel on biological hazards on a request from European Commission on the use and mode of action of bacteriophages in food production. EFSA J. 7:1076. doi: 10.2903/j.efsa.2009.1076
Aruscavage, D., Miller, S., Lewis Ivey, M. L., Lee, K., and LeJeune, J. T. (2008). Survival and dissemination of Escherichia coli O157:H7 on physically and biologically damaged lettuce plants. J. Food Prot. 71, 2384–2388. doi: 10.4315/0362-028X-71.12.2384
Aruscavage, D., Phelan, P. L., Lee, K., and LeJeune, J. T. (2010). Impact of changes in sugar exudate created by biological damage to tomato plants on the persistence of Escherichia coli O157:H7. J. Food Sci. 75, M187–M192. doi: 10.1111/j.1750-3841.2010.01593.x
Baert, L., Vandekinderen, I., Devlieghere, F., Van Coillie, E., Debevere, J., and Uyttendaele, M. (2009). Efficacy of sodium hypochlorite and peroxyacetic acid to reduce murine Norovirus 1, B40-8, Listeria monocytogenes, and Escherichia coli O157:H7 on shredded iceberg lettuce and in residual wash water. J. Food Prot. 72, 1047–1054. doi: 10.4315/0362-028X-72.5.1047
Barnett, S. J., Roget, D. K., and Ryder, M. H. (2006). Suppression of Rhizoctonia solani AG-8 induced disease on wheat by the interaction between Pantoea, Exiguobacterium, and Microbacteria. Aust. J. Soil Res. 44, 331–342. doi: 10.1071/SR05113
Bashi, E., and Fokkema, N. J. (1977). Environmental factors limiting growth of Sporobolomyces roseus, an antagonist of Cochliobolus sativus, on wheat leaves. Trans. Br. Mycol. Soc. 68, 17–25. doi: 10.1016/S0007-1536(77)80146-0
Behrsing, J., Winkler, S., Franz, P., and Premier, R. (2000). Efficacy of chlorine for inactivation of Escherichia coli on vegetables. Postharvest Biol. Technol. 19, 187–192. doi: 10.1016/S0925-5214(00)00092-2
Beuchat, L. R., Adler, B. B., and Lang, M. M. (2004). Efficacy of chlorine and a peroxyacetic acid sanitizer in killing Listeria monocytogenes on iceberg and Romaine lettuce using simulated commercial processing conditions. J. Food Prot. 67, 1238–1242. doi: 10.4315/0362-028X-67.6.1238
Beutin, L., and Martin, A. (2012). Outbreak of shiga toxin-producing Escherichia coli (STEC) O104:H4 infection in Germany causes a paradigm shift with regard to human pathogenicity of STEC strains. J. Food Protect. 75, 408–418. doi: 10.4315/0362-028X.JFP-11-452
Bottone, E. J., and Mollaret, H.-H. (1977). Yersinia enterocolitica: a panoramic view of a charismatic microorganism. CRC Crit. Rev. Microbiol. 5, 211–241. doi: 10.3109/10408417709102312
Brandl, M. T. (2006). “Fitness of human enteric pathogens on plants and implications for food safety,” in Annual Review of Phytopathology (Palo Alto, CA: Annual Reviews), 367–392.
Brandl, M. T. (2008). Plant lesions promote the rapid multiplication of Escherichia coli O157: H7 on postharvest lettuce. Appl. Environ. Microbiol. 74, 5285–5289. doi: 10.1128/AEM.01073-08
Brandl, M. T., and Amundson, R. (2008). Leaf age as a risk factor in contamination of lettuce with Escherichia coli O157:H7 and Salmonella enterica. Appl. Environ. Microbiol. 74, 2298–2306. doi: 10.1128/AEM.02459-07
Brenner, D. J. (2005). Bergey's Manual® of Systematic Bacteriology [Electronic Resource]: Volume Two the Proteobacteria Part b the Gammaproteobacteria. Boston, MA: Springer.
Brown, W. (1922). Studies in the physiology of parasitism. VIII. On the exosmosis of nutrient substances from the host tissue into the infection drop. Ann. Botany 36, 101–119. doi: 10.1093/oxfordjournals.aob.a089782
Brush, C. F., Walter, M. F., Anguish, L. J., and Ghiorse, W. C. (1998). Influence of pretreatment and experimental conditions on electrophoretic mobility and hydrophobicity of Cryptosporidium parvum oocysts. Appl. Environ. Microbiol. 64, 4439–4445.
Buss, B. F., Joshi, M. V., Dement, J. L., Cantu, V., and Safranek, T. J. (2016a). Multistate product traceforward investigation to link imported romaine lettuce to a US cyclosporiasis outbreak-Nebraska, Texas, and Florida, June-August 2013. Epidemiol. Infect. 144, 2709–2718. doi: 10.1017/S0950268815002320
Buss, B. F., Joshi, M. V., O'Keefe, A. L., Allensworth, C. D., Garvey, A., Obbink, K., et al. (2016b). Regional investigation of a cyclosporiasis outbreak linked to imported romaine lettuce - Nebraska and Iowa, June-August 2013. Epidemiol. Infect. 144, 1807–1817. doi: 10.1017/S0950268815002484
Campos, J., Mourão, J., Pestana, N., Peixe, L., Novais, C., and Antunes, P. (2013). Microbiological quality of ready-to-eat salads: an underestimated vehicle of bacteria and clinically relevant antibiotic resistance genes. Int. J. Food Microbiol. 166, 464–470. doi: 10.1016/j.ijfoodmicro.2013.08.005
Carlin, F., Nguyen-the, C., Abreu Da Silva, A., and Cochet, C. (1996). Effects of carbon dioxide on the fate of Listeria monocytogenes, of aerobic bacteria and on the development of spoilage in minimally processed fresh endive. Int. J. Food Microbiol. 32, 159–172. doi: 10.1016/0168-1605(96)01220-2
Carrasco, E., Pérez-Rodríguez, F., Valero, A., García-Gimeno, R. M., and Zurera, G. (2007). Survey of temperature and consumption patterns of fresh-cut leafy green salads: risk factors for Listeriosis. J. Food Prot. 70, 2407–2412. doi: 10.4315/0362-028X-70.10.2407
Castro-Ibáñez, I., Gil, M. I., and Allende, A. (2017). Ready-to-eat vegetables: current problems and potential solutions to reduce microbial risk in the production chain. LWT Food Sci. Technol. 85, 284–292. doi: 10.1016/j.lwt.2016.11.073
CDC (2006). Multistate Outbreak of E. coli O157:H7 Infections Linked to Fresh Spinach (FINAL UPDATE). (Accessed Oct 31, 2017). Available online at: https://www.cdc.gov/ecoli/2006/spinach-10-2006.html
CDC (2010). Multistate Outbreak of Human E. coli O145 Infections Linked to Shredded Romaine Lettuce from a Single Processing Facility (FINAL UPDATE) (Accessed Oct 31, 2017). Available online at: https://www.cdc.gov/ecoli/2010/shredded-romaine-5-21-10.html
CDC (2012a). Multistate Outbreak of E. coli O157:H7 Infections Linked to Romaine Lettuce (Final Update). (Accessed Oct 31, 2017). Available online at: https://www.cdc.gov/ecoli/2011/romaine-lettace-3-23-12.html
CDC (2012b). Multistate Outbreak of Shiga Toxin-producing Escherichia coli O157:H7 Infections Linked to Organic Spinach and Spring Mix Blend (Final Update) (Accessed June 20, 2017). Available online at: https://www.cdc.gov/ecoli/2012/o157h7-11-12/index.html
CDC (2016). Multistate Outbreak of Listeriosis Linked to Packaged Salads Produced at Springfield, Ohio Dole Processing Facility (Final Update). (Accessed Oct 31, 2017). Available online at: https://www.cdc.gov/listeria/outbreaks/bagged-salads-01-16/index.html
CDC (2017). Timeline for Reporting Cases of Salmonella Infection. (Accessed Nov 15, 2017). Available online at: https://www.cdc.gov/salmonella/reporting-timeline.html
Chua, D., Goh, K., Saftner, R. A., and Bhagwat, A. A. (2008). Fresh-cut lettuce in modified atmosphere packages stored at improper temperatures supports enterohemorrhagic E. coli isolates to survive gastric acid challenge. J. Food Sci. 73, M148–M153. doi: 10.1111/j.1750-3841.2008.00698.x
Conner, D. E., and Kotrola, J. S. (1995). Growth and survival of Escherichia coli O157:H7 under acidic conditions. Appl. Environ. Microbiol. 61, 382–385.
Critzer, F. J., and Doyle, M. P. (2010). Microbial ecology of foodborne pathogens associated with produce. Curr. Opin. Biotechnol. 21, 125–130. doi: 10.1016/j.copbio.2010.01.006
Crook, P. D., Aguilera, J. F., Threlfall, E. J., O'Brien, S. J., Sigmundsdóttir, G., Wilson, D., et al. (2003). A European outbreak of Salmonella enterica serotype Typhimurium definitive phage type 204b in 2000. Clin. Microbiol. Infect. 9, 839–845. doi: 10.1046/j.1469-0691.2003.00655.x
Davidson, G. R., Buchholz, A. L., and Ryser, E. T. (2013). Efficacy of commercial produce sanitizers against nontoxigenic Escherichia coli O157:H7 during processing of iceberg lettuce in a pilot-scale leafy green processing line. J. Food Prot. 76, 1838–1845. doi: 10.4315/0362-028X.JFP-13-111
De Vos, P., Garrity, G. M., Jones, D., Krieg, N. R., Ludwig, W., Rainey, F. A., et al. (eds.). (2009). Bergey's Manual of Systematic Bacteriology, Vol. 3: The Firmicutes. New York, NY: Springer. doi: 10.1007/978-0-387-68489-5
Delbeke, S., Ceuppens, S., Jacxsens, L., and Uyttendaele, M. (2015). Survival of Salmonella and Escherichia coli O157:H7 on strawberries, basil, and other leafy greens during storage. J. Food Prot. 78, 652–660. doi: 10.4315/0362-028X.JFP-14-354
Denny, J., Threlfall, J., Takkinen, J., Lofdahl, S., Westrell, T., Varela, C., et al. (2007). Multinational Salmonella Paratyphi B variant Java (Salmonella Java) outbreak, August - December 2007. Euro Surveill. 12, E071220–71222. doi: 10.2807/esw.12.51.03332-en
Dik, A. J., Fokkema, N. J., and Van Pelt, J. A. (1991). Consumption of aphid honeydew, a wheat yield reduction factor, by phyllosphere yeasts under field conditions. Netherlands J. Plant Pathol. 97, 209–232. doi: 10.1007/BF01989819
Dik, A. J., Fokkema, N. J., and van Pelt, J. A. (1992). Influence of climatic and nutritional factors on yeast population dynamics in the phyllosphere of wheat. Microb. Ecol. 23, 41–52. doi: 10.1007/BF00165906
Dingman, D. W. (2000). Growth of Escherichia coli O157:H7 in bruised apple (Malus domestica) tissue as influenced by cultivar, date of harvest, and source. Appl. Environ. Microbiol. 66, 1077–1083. doi: 10.1128/AEM.66.3.1077-1083.2000
Edelstein, M., Sundborger, C., Hergens, M. P., Ivarsson, S., Dryselius, R., Insulander, M., et al. (2014). Barriers to trace-back in a salad-associated EHEC outbreak, Sweden, June 2013. PLoS Curr. 6. doi: 10.1371/currents.outbreaks.80bbab3af3232be0372ea0e904dcd1fe
EFSA (2013). Scientific opinion on the risk posed by pathogens in food of non-animal origin. Part 1 (outbreak data analysis and risk ranking of food/pathogen combinations). EFSA Panel on Biological Hazards (BIOHAZ). EFSA J. 11:3025. doi: 10.2903/j.efsa.2013.3025
Escalona, V. H., Geysen, S., Verlinden, B. E., and Nicolai, B. M. (2007). Microbial quality and browning of fresh-cut butter lettuce under superatmospheric oxygen condition. Eur. J. Horticult. Sci. 72, 130–137.
Ethelberg, S., Lisby, M., Bottiger, B., Schultz, A. C., Villif, A., Jensen, T., et al. (2010). Outbreaks of gastroenteritis linked to lettuce, Denmark, January 2010. Euro Surveill 15:pii=1948. Available online at: https://www.eurosurveillance.org/content/10.2807/ese.15.06.19484-en
FAO and WHO (2008). Microbiological Hazards in Fresh Fruits and Vegetables, Meeting Report. FAO and WHO.
Farber, J. N., Harris, L. J., Parish, M. E., Beuchat, L. R., Suslow, T. V., Gorney, J. R., et al. (2003). Microbiological safety of controlled and modified atmosphere packaging of fresh and fresh-cut produce. Compr. Rev. Food Sci. Food Safety 2, 142–160. doi: 10.1111/j.1541-4337.2003.tb00032.x
Fayer, R., and Ungar, B. L. (1986). Cryptosporidium spp and cryptosporidiosis. Microbiol. Rev. 50, 458–483.
Fiala, V., Glad, C., Martin, M., Jolivet, E., and Derridj, S. (1990). Occurrence of soluble carbohydrates on the phylloplane of maize (Zea mays L.): variations in relation to leaf heterogeneity and position on the plant. New Phytol. 115, 609–615. doi: 10.1111/j.1469-8137.1990.tb00492.x
Fokkema, N. J., den Houter, J. G., Kosterman, Y. J. C., and Nelis, A. L. (1979). Manipulation of yeasts on field-grown wheat leaves and their antagonistic effect on Cochliobolus sativus and Septoria nodorum. Trans. Br. Mycol. Soc. 72, 19–29. doi: 10.1016/S0007-1536(79)80003-0
Frossard, R., Fokkema, N. J., and Tietema, T. (1983). Influence of Sporobolomyces roseus and Cladosporium cladosporioides on leaching of 14C-labelled assimilates from wheat leaves. Trans. Br. Mycol. Soc. 80, 289–296. doi: 10.1016/S0007-1536(83)80012-6
Gajraj, R., Pooransingh, S., Hawker, J. I., and Olowokure, B. (2012). Multiple outbreaks of Salmonella braenderup associated with consumption of iceberg lettuce. Int. J. Environ. Health Res. 22, 150–155. doi: 10.1080/09603123.2011.613114
Garrido, V., Mobley, R., Otwell, S., and Schneider, K. (2004). “Guidance for processing packaged fresh-cut produce in retail operations,” in UF-IFSA, ed. University of Florida (IF) (Gainsville, FL: Food Science and Human Nutrition Department; UF/IFAS Extension).
Garrido, Y., Tudela, J. A., and Gil, M. I. (2015). Comparison of industrial precooling systems for minimally processed baby spinach. Postharvest Biol. Technol. 102, 1–8. doi: 10.1016/j.postharvbio.2014.12.003
Gates, D. M. (1968). Transpiration and leaf temperature. Ann. Rev. Plant Physiol. 19, 211–238. doi: 10.1146/annurev.pp.19.060168.001235
George, S. M., Richardson, L. C., and Peck, M. W. (1996). Predictive models of the effect of temperature, pH and acetic and lactic acids on the growth of Listeria monocytogenes. Int. J. Food Microbiol. 32, 73–90. doi: 10.1016/0168-1605(96)01108-7
Geysen, S., Escalona, V. H., Verlinden, B. E., Aertsen, A., Geeraerd, A. H., Michiels, C. W., et al. (2006). Validation of predictive growth models describing superatmospheric oxygen effects on Pseudomonas fluorescens and Listeria innocua on fresh-cut lettuce. Int. J. Food Microbiol. 111, 48–58. doi: 10.1016/j.ijfoodmicro.2006.04.044
Gharaie, S., Vaas, L. A. I., Rosberg, A. K., Windstam, S. T., Karlsson, M. E., Bergstrand, K. J., et al. (2017). Light spectrum modifies the utilization pattern of energy sources in Pseudomonas sp. DR 5-09. PLoS ONE 12:e0189862. doi: 10.1371/journal.pone.0189862
Gil, M. I., Selma, M. V., Suslow, T., Jacxsens, L., Uyttendaele, M., and Allende, A. (2015). Pre- and postharvest preventive measures and intervention strategies to control microbial food safety hazards of fresh leafy vegetables. Crit. Rev. Food Sci. Nutr. 55, 453–468. doi: 10.1080/10408398.2012.657808
Gillespie, I. A. (2004). Outbreak of Salmonella Newport infection associated with lettuce in the UK. Euro Surveill. 8:2562. doi: 10.2807/esw.08.41.02562-en
Goodburn, C., and Wallace, C. A. (2013). The microbiological efficacy of decontamination methodologies for fresh produce: a review. Food Control 32, 418–427. doi: 10.1016/j.foodcont.2012.12.012
Gorny, J. R., Giclas, H., Gombas, D., and Means, K. (2006). Commodity Specific Food Safety Guidelines for the Lettuce and Leafy Greens Supply Chain, 1st Edn. Silver Spring, MD: U. S. Food and Drug Administration.
Gragg, S. E., and Brashears, M. M. (2010). Reduction of Escherichia coli O157:H7 in fresh spinach, using lactic acid bacteria and chlorine as a multihurdle intervention. J. Food Prot. 73, 358–361. doi: 10.4315/0362-028X-73.2.358
Grant, J., Wendelboe, A. M., Wendel, A., Jepson, B., Torres, P., Smelser, C., et al. (2008). Spinach-associated Escherichia coli O157:H7 outbreak, Utah and New Mexico, 2006. Emerg. Infect. Dis. 14, 1633–1636. doi: 10.3201/eid1410.071341
Guzel, M., Moreira, R. G., Omac, B., and Castell-Perez, M. E. (2017). Quantifying the effectiveness of washing treatments on the microbial quality of fresh-cut romaine lettuce and cantaloupe. Lwt-Food Sci. Technol. 86, 270–276. doi: 10.1016/j.lwt.2017.08.008
Hagele, F., Nubling, S., Schweiggert, R. M., Baur, S., Weiss, A., Schmidt, H., et al. (2016). Quality improvement of fresh-cut endive (Cichorium endivia L.) and recycling of washing water by low-dose UV-C irradiation. Food Bioprocess Technol. 9, 1979–1990. doi: 10.1007/s11947-016-1782-2
Haller, T., and Stolp, H. (1985). Quantitative estimation of root exudation of maize plants. Plant Soil 86, 207–216. doi: 10.1007/BF02182895
Hanna, M. O., Stewart, J. C., Zink, D. L., Carpenter, Z. L., and Vanderzant, C. (1977). Development of Yersinia enterocolitica on raw and cooked beef and pork at different temperatures. J. Food Sci. 42, 1180–1184. doi: 10.1111/j.1365-2621.1977.tb14455.x
Hartmann, R., Fricke, A., Stutzel, H., Mansourian, S., Dekker, T., Wohanka, W., et al. (2017). Internalization of Escherichia coli O157:H7 gfp plus in rocket and Swiss chard baby leaves as affected by abiotic and biotic damage. Lett. Appl. Microbiol. 65, 35–41. doi: 10.1111/lam.12742
Holvoet, K., Jacxsens, L., Sampers, I., and Uyttendaele, M. (2012). Insight into the prevalence and distribution of microbial contamination to evaluate water management in the fresh produce processing industry. J. Food Prot. 75, 671–681. doi: 10.4315/0362-028X.JFP-11-175
Horby, P. W., O'Brien, S. J., Adak, G. K., Graham, C., Hawker, J. I., Hunter, P., et al. (2003). A national outbreak of multi-resistant Salmonella enterica serovar Typhimurium definitive phage type (DT) 104 associated with consumption of lettuce. Epidemiol. Infect. 130, 169–178. doi: 10.1017/S0950268802008063
HPA (2004). Update–Outbreak of Salmonella Newport Infection in England, Scotland, and Northern Ireland: Association With the Consumption of Lettuce. CDR Weekly.
HPA (2005a). (Health Protection Agency) Outbreak of Salmonella typhimurium DT104 infection in Scotland, England, and Wales: January to February 2005. CDR Weekly.
HPA (2005b). Outbreak of Salmonella typhimurium DT104 infection in Scotland, England & Wales, January to February 2005 (Update) Iceberg Lettuce Eaten Outside the Home Implicated. Health Protection Agency the Communicable Disease Report Weekly. CDR Weekly.
Hsin-Yi, C., and Chou, C. C. (2001). Acid adaptation and temperature effect on the survival of E-coli O157: H7 in acidic fruit juice and lactic fermented milk product. Int. J. Food Microbiol. 70, 189–195. doi: 10.1016/S0168-1605(01)00538-4
Huber, L., and Gillespie, T. J. (1992). Modelleing leaf wetness in relation to plant disease epidemiology. Annu. Rev. Phytopathol. 30, 553–577. doi: 10.1146/annurev.py.30.090192.003005
Hunter, P. J., Hand, P., Pink, D., Whipps, J. M., and Bending, G. D. (2010). Both leaf properties and microbe-microbe interactions influence within-species variation in bacterial population diversity and structure in the lettuce (Lactuca Species) phyllosphere. Appl. Environ. Microbiol. 76, 8117–8125. doi: 10.1128/AEM.01321-10
Hutchison, M. L., Harrison, D., Heath, J. F., and Monaghan, J. M. (2017). Fate of Escherichia coli O145 present naturally in bovine slurry applied to vegetables before harvest, after washing and simulated wholesale and retail distribution. J. Appl. Microbiol. 123, 1597–1606. doi: 10.1111/jam.13593
Ijabadeniyi, O. A., Debusho, L. K., Vanderlinde, M., and Buys, E. M. (2011). Irrigation water as a potential preharvest source of bacterial contamination of vegetables. J. Food Safety 31, 452–461. doi: 10.1111/j.1745-4565.2011.00321.x
Irvine, W. N., Gillespie, I. A., Smyth, F. B., Rooney, P. J., McClenaghan, A., Devine, M. J., et al. (2009). Investigation of an outbreak of Salmonella enterica serovar Newport infection. Epidemiol. Infect. 137, 1449–1456. doi: 10.1017/S0950268809002416
Islam, M., Morgan, J., Doyle, M. P., Phatak, S. C., Millner, P., and Jiang, X. (2004). Persistence of Salmonella enterica Serovar typhimurium on lettuce and parsley and in soils on which they were grown in fields treated with contaminated manure composts or irrigation water. Foodborne Pathog. Dis. 1, 27–35. doi: 10.1089/153531404772914437
Jablasone, J., Warriner, K., and Griffiths, M. (2005). Interactions of Escherichia coli O157:H7, Salmonella typhimurium and Listeria monocytogenes plants cultivated in a gnotobiotic system. Int. J. Food Microbiol. 99, 7–18. doi: 10.1016/j.ijfoodmicro.2004.06.011
Jackson, C. R., Randolph, K. C., Osborn, S. L., and Tyler, H. L. (2013). Culture dependent and independent analysis of bacterial communities associated with commercial salad leaf vegetables. BMC Microbiol 13:274. doi: 10.1186/1471-2180-13-274
Jacobs, J. L., Carroll, T. L., and Sundin, G. W. (2003). The role of pigmentation, ultraviolet radiation tolerance, and leaf colonization strategies in the epiphytic survival of phyllosphere bacteria. Microb. Ecol. 49, 104–113. doi: 10.1007/s00248-003-1061-4
Jacobs, J. L., and Sundin, G. W. (2001). Effect of solar UV-B radiation on a phyllosphere bacterial community. Appl. Environ. Microbiol. 67, 5488–5496. doi: 10.1128/AEM.67.12.5488-5496.2001
Jacxsens, L., Devlieghere, F., Falcato, P., and Debevere, J. (1999). Behavior of Listeria monocytogenes and Aeromonas spp. on fresh-cut produce packaged under equilibrium-modified atmosphere. J. Food Prot. 62, 1128–1135. doi: 10.4315/0362-028X-62.10.1128
Jacxsens, L., Ibañez, I. C. I., Gómez-Lopéz, M., Araujo Fernandes, J., Uyttendaele, M., and Huybrecht, I. (2015). Belgian and Spanish consumption data and consumer handling practices for fresh fruits and vegetables useful for further microbiological and chemical exposure assessment. J. Food Prot. 78, 784–795. doi: 10.4315/0362-028X.JFP-14-376
Jay, J. M., Loessner, M. J., and Golden, D. A. (2005). Modern Food Microbiology. New York, NY: Springer Science and Business Media. doi: 10.1007/b100840
Jongman, M., and Korsten, L. (2017). Assessment of irrigation water quality and microbiological safety of leafy greens in different production systems. J. Food Safety 37:e12324. doi: 10.1111/jfs.12324
Kadivar, H., and Stapelton, A. E. (2003). Ultraviolet radiation alters maize phyllosphere bacterial diversity. Microb. Ecol. 45, 353–361. doi: 10.1007/s00248-002-1065-5
Kallander, K. D., Hitchins, A. D., Lancetta, G. A., Schmieg, J. A., Garcia, G. R., Solomon, H. M., et al. (1991). Fate of Listeria monocytogenes in shredded cabbage stored at 5 and 25°C under a modified atmosphere. J. Food Prot. 54, 302–304. doi: 10.4315/0362-028X-54.4.302
Kaper, J. B., Nataro, J. P., and Mobley, H. L. (2004). Pathogenic escherichia coli. Nat. Rev. Microbiol. 2, 123–140. doi: 10.1038/nrmicro818
Karaca, H., and Velioglu, Y. S. (2014). Effects of ozone treatments on microbial quality and some chemical properties of lettuce, spinach, and parsley. Postharvest Biol. Technol. 88, 46–53. doi: 10.1016/j.postharvbio.2013.09.003
Kazi, M., and Annapure, U. S. (2016). Bacteriophage biocontrol of foodborne pathogens. J. Food Sci. Technol. 53, 1355–1362. doi: 10.1007/s13197-015-1996-8
Khalil, R., and Frank, J. F. (2010). Behavior of Escherichia coli O157:H7 on damaged leaves of spinach, lettuce, cilantro, and parsley stored at abusive temperatures. J. Food Prot. 73, 212–220. doi: 10.4315/0362-028X-73.2.212
King, B. J., Keegan, A. R., Monis, P. T., and Saint, C. P. (2005). Environmental temperature controls cryptosporidium oocyst metabolic rate and associated retention of infectivity. Appl. Environ. Microbiol. 71, 3848–3857. doi: 10.1128/AEM.71.7.3848-3857.2005
Koseki, S., and Isobe, S. (2005). Prediction of pathogen growth on iceberg lettuce under real temperature history during distribution from farm to table. Int. J. Food Microbiol. 104, 239–248. doi: 10.1016/j.ijfoodmicro.2005.02.012
Koutsoumanis, K. P., Kendall, P. A., and Sofos, J. N. (2003). Effect of food processing-related stresses on acid tolerance of Listeria monocytogenes. Appl. Environ. Microbiol. 69, 7514–7516. doi: 10.1128/AEM.69.12.7514-7516.2003
Kroll, R. G., and Patchet, R. A. (1992). Induced acid tolerance in Listeria monocytogenes. Lett. Appl. Microbiol. 14, 224–227. doi: 10.1111/j.1472-765X.1992.tb00691.x
Kroupitski, Y., Golberg, D., Belausov, E., Pinto, R., Swartzberg, D., Granot, D., et al. (2009a). Internalization of Salmonella enterica in leaves is induced by light and involves chemotaxis and penetration through open stomata. Appl. Environ. Microbiol. 75, 6076–6086. doi: 10.1128/AEM.01084-09
Kroupitski, Y., Pinto, R., Brandl, M. T., Belausov, E., and Sela, S. (2009b). Interactions of Salmonella enterica with lettuce leaves. J. Appl. Microbiol. 106, 1876–1885. doi: 10.1111/j.1365-2672.2009.04152.x
Kyle, J. L., Parker, C. T., Goudeau, D., and Brandl, M. T. (2010). Transcriptome Analysis of Escherichia coli O157:H7 exposed to lysates of lettuce leaves. Appl. Environ. Microbiol. 76, 1375–1387. doi: 10.1128/AEM.02461-09
Laguerre, O., Derens, E., and Palagos, B. (2002). Study of domestic refrigerator temperature and analysis of factors affecting temperature: a French survey. Int. J. Refrig. 25, 653–659. doi: 10.1016/S0140-7007(01)00047-0
Larcher, W. (2003). Physiological Plant Ecology. Ecophysiology and Stress Physiology of Functional Groups. Berlin; Heidelberg: Springer-Verlag.
Lee, H.-H., Hong, S.-I., and Kim, D. (2011). Microbiological and visual quality of fresh-cut cabbage as affected by packaging treatments. Food Sci. Biotechnol. 20, 229–235. doi: 10.1007/s10068-011-0031-8
Leistner, L. (2000). Basic aspects of food preservation by hurdle technology. Int. J. Food Microbiol. 55, 181–186. doi: 10.1016/S0168-1605(00)00161-6
Leveau, J. H., and Lindow, S. E. (2001). Appetite of an epiphyte: quantitative monitoring of bacterial sugar consumption in the phyllosphere. Proc. Natl. Acad. Sci. U.S.A. 98, 3446–3453. doi: 10.1073/pnas.061629598
Lienemann, T., Niskanen, T., Guedes, S., Siitonen, A., Kuusi, M., and Rimhanen-Finne, R. (2011). Iceberg lettuce as suggested source of a nationwide outbreak caused by two Salmonella serotypes, Newport and Reading, in Finland in 2008. J. Food Prot. 74, 1035–1040. doi: 10.4315/0362-028X.JFP-10-455
Lindow, S. E., and Brandl, M. T. (2003). Microbiology of the Phyllosphere. Appl. Environ. Microbiol. 69, 1875–1883. doi: 10.1128/AEM.69.4.1875-1883.2003
Liu, C., Hofstra, N., and Franz, E. (2013). Impacts of climate change on the microbial safety of pre-harvest leafy green vegetables as indicated by Escherichia coli O157 and Salmonella spp. Int. J. Food Microbiol. 163, 119–128. doi: 10.1016/j.ijfoodmicro.2013.02.026
López-Gálvez, F., Gil, M. I., Meireles, A., Truchado, P., and Allende, A. (2018). Demonstration tests of irrigation water disinfection with chlorine dioxide in open field cultivation of baby spinach. J. Sci. Food Agric. 98, 2973–2980. doi: 10.1002/jsfa.8794
Lopez-Velasco, G., Carder, P. A., Welbaum, G. E., and Ponder, M. A. (2013). Diversity of the spinach (Spinacia oleracea) spermosphere and phyllosphere bacterial communities. FEMS Microbiol. Lett. 346, 146–154. doi: 10.1111/1574-6968.12216
Lopez-Velasco, G., Davis, M., Boyer, R. R., Williams, R. W., and Ponder, M. A. (2010). Alterations of the phylloepiphytic bacterial community associated with interactions of Escherichia coli O157:H7 during storage of packaged spinach at refrigeration temperatures. Food Microbiol. 27, 476–486. doi: 10.1016/j.fm.2009.12.010
Lopez-Velasco, G., Tydings, H. A., Boyer, R. R., Falkinham, J. O., and Ponder, M. A. (2012). Characterization of interactions between Escherichia coli O157:H7 with epiphytic bacteria in vitro and on spinach leaf surfaces. Int. J. Food Micobiol. 153, 351–357. doi: 10.1016/j.ijfoodmicro.2011.11.026
Luo, Y., He, Q., and McEvoy, J. L. (2010). Effect of storage temperature and duration on the behavior of Escherichia coli O157:H7 on packaged fresh-cut salad containing romaine and iceberg lettuce. J. Food Sci. 75, M390–M387. doi: 10.1111/j.1750-3841.2010.01722.x
Luo, Y., He, Q., McEvoy, J. L., and Conway, W. S. (2009). Fate of Escherichia coli O157:H7 in the presence of indigenous microorganisms on commercially packaged baby spinach, as impacted by storage temperature and time. J. Food Prot. 72, 2038–2045. doi: 10.4315/0362-028X-72.10.2038
Lynch, M. F., Tauxe, R. V., and Hedberg, C. W. (2009). The growing burden of foodborne outbreaks due to contaminated fresh produce: risks and opportunities. Epidemiol. Infect. 137, 307–315. doi: 10.1017/S0950268808001969
MacDonald, E., Einöder-Moreno, M., Borgen, K., Brandal, L. T., Diab, L., Fossli, Ø., et al. (2016). National outbreak of Yersinia enterocolitica infections in military and civilian populations associated with consumption of mixed salad, Norway, 2014. Eurosurveillance 21, 11–19. doi: 10.2807/1560-7917.ES.2016.21.34.30321
MacDonald, E., Heier, B. T., Stalheim, T., Cudjoe, K. S., Skjerdal, T., Wester, A., et al. (2011). Yersinia enterocolitica O:9 infections associated with bagged salad mix in Norway, February to April 2011. Eurosurveillance 16, 10–12. Available online at: https://www.eurosurveillance.org/content/10.2807/ese.16.19.19866-en
Manzocco, L., Alongi, M., Lagazio, C., Sillani, S., and Nicoli, M. C. (2017). Effect of temperature in domestic refrigerators on fresh-cut Iceberg salad quality and waste. Food Res. Int. 102, 129–135. doi: 10.1016/j.foodres.2017.09.091
Marder, E. P., Garman, K. N., Ingram, L. A., and Dunn, J. R. (2014). Multistate outbreak of Escherichia coli O157:H7 associated with bagged salad. Foodborne Pathog. Dis. 11, 593–595. doi: 10.1089/fpd.2013.1726
Marklinder, I. M., Lindblad, M., Eriksson, L. M., Finnsson, A. M., and Lindqvist, R. (2004). Home storage temperatures and consumer handling of refrigerated foods in Sweden. J. Food Prot. 67, 2570–2577. doi: 10.4315/0362-028X-67.11.2570
Matches, J. R., and Liston, J. (1968). Low temperature growth of Salmonella. J. Food Sci. 33, 641–645. doi: 10.1111/j.1365-2621.1968.tb09092.x
McKerr, C., Adak, G. K., Nichols, G., Gorton, R., Chalmers, R. M., Kafatos, G., et al. (2015). An outbreak of Cryptosporidium parvum across England and Scotland associated with consumption of fresh pre-cut salad leaves, May 2012. PLoS ONE 10:0125955. doi: 10.1371/journal.pone.0125955
McMeekin, T. A., Presser, K., Ratkowsky, D., Ross, T., Salter, M., and Tienungoon, S. (2000). Quantifying the hurdle concept by modelling the bacterial growth/no growth interface. Int. J. Food Microbiol. 55, 93–98. doi: 10.1016/S0168-1605(00)00182-3
Mecklenburg, R. A., Tukey, H. B., and Morgan, J. V. (1966). A mechanism for the leaching of calcium from foliage. Plant Physiol. 41, 610–613. doi: 10.1104/pp.41.4.610
Menz, G., Aldred, P., and Vriesekoop, F. (2011). Growth and survival of foodborne pathogens in beer. J. Food Prot. 74, 1670–1675. doi: 10.4315/0362-028X.JFP-10-546
Mercier, J., and Lindow, S. E. (2000). Role of leaf surface sugars in colonization of plants by bacterial epiphytes. Appl. Environ. Microbiol. 66, 369–374. doi: 10.1128/AEM.66.1.369-374.2000
Monaghan, J. M., Augustin, J. C., Bassett, J., Betts, R., Pourkomailian, B., and Zwietering, M. H. (2017). Risk assessment or assessment of risk? Developing an evidence-based approach for primary producers of leafy vegetables to assess and manage microbial risks. J. Food Prot. 80, 725–733. doi: 10.4315/0362-028X.JFP-16-237
Monaghan, J. M., and Hutchison, M. L. (2012). Distribution and decline of human pathogenic bacteria in soil after application in irrigation water and the potential for soil-splash-mediated dispersal onto fresh produce. J. Appl. Microbiol. 112, 1007–1019. doi: 10.1111/j.1365-2672.2012.05269.x
Monier, J. M., and Lindow, S. E. (2005). Aggregates of resident bacteria facilitate survival of immigrant bacteria on leaf surfaces. Microb. Ecol. 49, 343–352. doi: 10.1007/s00248-004-0007-9
Morgan, J. V., and Tukey, H. B. (1964). Characterization of leachate from plant foliage. Plant Physiol. 39, 590–593. doi: 10.1104/pp.39.4.590
Moye, Z. D., Woolston, J., and Sulakvelidze, A. (2018). Bacteriophage applications for food production and processing. Viruses 10:E205. doi: 10.3390/v10040205
Moyne, A., Sudarshana, M. R., Blessington, T., Koike, S. T., Cahn, M. D., and Harris, L. J. (2011). Fate of Escherichia coli O157:H7 in field-inoculated lettuce. Food Microbiol. 28, 1417–1425. doi: 10.1016/j.fm.2011.02.001
Newsham, K. K., Low, M. N. R., McLeod, M. R., Greenslade, P. D., and Emmett, B. A. (1997). Ultraviolet-B radiation influences the abundance and distribution of phylloplane fungi on pedunculate oak (Quercus rohur). New Phytol. 136, 287–297. doi: 10.1046/j.1469-8137.1997.00740.x
Nousiainen, L. L., Joutsen, S., Lunden, J., Hänninen, M. L., and Fredriksson-Ahomaa, M. (2016). Bacterial quality and safety of packaged fresh leafy vegetables at the retail level in Finland. Int. J. Food Microbiol. 232(Supplement C), 73–79. doi: 10.1016/j.ijfoodmicro.2016.05.020
Nygård, K., Lassen, J., Vold, L., Andersson, Y., Fisher, I., Löfdahl, S., et al. (2008). Outbreak of Salmonella thompson infections linked to imported rucola lettuce. Foodborne Pathog. Dis. 5, 165–173. doi: 10.1089/fpd.2007.0053
O'Beirne, D., Gomez-Lopez, V., Tudela, J. A., Allende, A., and Gil, M. I. (2015). Effects of oxygen-depleted atmospheres on survival and growth of Listeria monocytogenes on fresh-cut Iceberg lettuce stored at mild abuse commercial temperatures. Food Microbiol. 48, 17–31. doi: 10.1016/j.fm.2014.11.012
Ohlsson, T., and Bengtsson, N. (2002). Minimal Processing Technologies in the Food Industry. Washington, DC: CRC Press.
Olaimat, A. N., and Holley, R. A. (2012). Factors influencing the microbial safety of fresh produce: a review. Food Microbiol. 32, 1–19. doi: 10.1016/j.fm.2012.04.016
Oliveira, M., Abadias, M., Usall, J., Torres, R., Teixidó, N., and Viñas, I. (2015). Application of modified atmosphere packaging as a safety approach to fresh-cut fruits and vegetables–A review. Trends Food Sci. Technol. 46, 13–26. doi: 10.1016/j.tifs.2015.07.017
Oliveira, M., Usall, J., Solsona, C., Alegre, I., Viñas, I., and Abadias, M. (2010). Effects of packaging type and storage temperature on the growth of foodborne pathogens on shredded ‘Romaine’ lettuce. Food Microbiol. 27, 375–380. doi: 10.1016/j.fm.2009.11.014
Omac, B., Moreira, R. G., Castillo, A., and Castell-Perez, E. (2015). Growth of Listeria monocytogenes and Listeria innocua on fresh baby spinach leaves: effect of storage temperature and natural microflora. Postharvest Biol. Technol. 100, 41–51. doi: 10.1016/j.postharvbio.2014.09.007
Ondrusch, N., and Kreft, J. (2011). Blue and red light modulates sigB-dependent gene transcription, swimming motility and invasiveness in Listeria monocytogenes. PLoS ONE 6:e16151. doi: 10.1371/journal.pone.0016151
Orue, N., García, S., Feng, P., and Heredia, N. (2013). Decontamination of Salmonella, Shigella, and Escherichia coli O157:H7 from leafy green vegetables using edible plant extracts. J. Food Sci. 78, M290–M296. doi: 10.1111/1750-3841.12016
Pachepsky, Y., Morrow, J., Guber, A., Shelton, D., Rowland, R., and Davies, G. (2011). Effect of biofilm in irrigation pipes on microbial quality of irrigation water. Lett. Appl. Microbiol. 54, 217–224. doi: 10.1111/j.1472-765X.2011.03192.x
Page, G. V., and Solberg, M. (1980). Nitrogen assimilation by Salmonella typhimurium in a chemically defined minimal medium containing nitrate, nitrite, or ammonia. J. Food Sci. 45, 75–76. doi: 10.1111/j.1365-2621.1980.tb03874.x
Park, S., Worobo, R. W., and Durst, R. A. (1999). Escherichia coli O157: H7 as an emerging foodborne pathogen: a literature review. Crit. Rev. Food Sci. Nutr. 39, 481–502. doi: 10.1080/10408699991279259
Paull, R. E. (1999). Effect of temperature and relative humidity on fresh commodity quality. Postharvest Biol. Technol. 15, 263–277. doi: 10.1016/S0925-5214(98)00090-8
Pearce, L. E., Smythe, B. W., Crawford, R. A., Oakley, E., Hathaway, S. C., and Shepherd, J. M. (2012). Pasteurization of milk: the heat inactivation kinetics of milk-borne dairy pathogens under commercial-type conditions of turbulent flow. J. Dairy Sci. 95, 20–35. doi: 10.3168/jds.2011-4556
Pereira, P., Nesci, A., and Etcheverry, M. G. (2009). Efficacy of bacterial seed treatments for the control of Fusarium verticillioides in maize. BioControl 54, 103–111. doi: 10.1007/s10526-007-9148-3
Petran, R. L., and Zottola, E. A. (1989). A study of factors affecting growth and recovery of Listeria monocytogenes Scott A. J. Food Sci. 54, 458–460. doi: 10.1111/j.1365-2621.1989.tb03105.x
Pezzuto, A., Belluco, S., Losasso, C., Patuzzi, I., Bordin, P., Piovesana, A., et al. (2016). Effectiveness of washing procedures in reducing Salmonella enterica and Listeria monocytogenes on a raw leafy green vegetable (Eruca vesicaria). Front. Microbiol. 7:8. doi: 10.3389/fmicb.2016.01663
Piagentini, A. M., Pirovani, M. E., Gümez, D. R., Di Pentima, J. H., and Tessi, M. A. (1997). Survival and growth of Salmonella Hadar on minimally processed cabbage as influenced by storage abuse conditions. J. Food Sci. 62, 616–631. doi: 10.1111/j.1365-2621.1997.tb04444.x
Pine, L., Malcolm, G. B., Brooks, J. B., and Daneshvar, M. I. (1989). Physiological studies on the growth and utilization of sugars by Listeria species. Canadian J. Microbiol. 35, 245–254. doi: 10.1139/m89-037
Public Health England (PHE) (2016). Public Health England News story, E. coli O157 national outbreak update. (Accessed Oct 31, 2017). Available online at https://www.gov.uk/government/news/update-as-e-coli-o157-investigation-continues
Public Health England (PHE) (2015). Health Protection Report Volume 9 News (4 September). (Accessed Oct 31, 2017).Available online at: https://www.gov.uk/government/publications/health-protection-report-volume-9-2015/hpr-volume-9-issue-31-news-4-september
Río-Álvarez, I., Rodríguez-Herva, J. J., Martínez, P. M., González-Melendi, P., García-Casado, G., Rodríguez-Palenzuela, P., et al. (2014). Light regulates motility, attachment and virulence in the plant pathogen Pseudomonas syringae pv tomato DC3000. Environ. Microbiol. 16, 2072–2085. doi: 10.1111/1462-2920.12240
Rahman, S. M. E., Khan, I., and Oh, D.-H. (2016). Electrolyzed water as a novel sanitizer in the food industry: current trends and future perspectives. Compr. Rev. Food Sci. Food safety 15, 471–490. doi: 10.1111/1541-4337.12200
Rediers, H., Claes, M., Peeters, L., and Willems, K. A. (2009). Evaluation of the cold chain of fresh-cut endive from farmer to plate. Postharvest Biol. Technol. 51, 257–262. doi: 10.1016/j.postharvbio.2008.07.017
Rodger, G., and Blakeman, J. P. (1984). Microbial colonization and uptake of 14C label on leaves of Sycamore. Trans. Br. Mycol. Soc. 82, 45–51. doi: 10.1016/S0007-1536(84)80210-7
Rose, J. B., Lisle, J. T., and LeChevallier, M. (1997). Waterborne Cryptosporidiosis: Incidence, Outbreaks, and Treatment Strategies. Boca Raton, FL: CRC Press.
Sandhya (2010). Modified atmosphere packaging of fresh produce: Current status and future needs. LWT Food Sci. Technol. 43, 381–392. doi: 10.1016/j.lwt.2009.05.018
Scifo, G. O., Randazzo, C. L., Restuccia, C., Fava, G., and Caggia, C. (2009). Listeria innocua growth in fresh cut mixed leafy salads packaged in modified atmosphere. Food Control 20, 611–617. doi: 10.1016/j.foodcont.2008.08.017
Sela, S., and Manulis-Sasson, S. (2015). What else can we do to mitigate contamination of fresh produce by foodborne pathogens? Microbial Biotechnol. 8, 29–31. doi: 10.1111/1751-7915.12231
Self, J. L., Conrad, A., Stroika, S., Jackson, A., Burnworth, L., Beal, J., et al. (2016). Notes from the field: outbreak of Listeriosis associated with consumption of packaged salad - United States and Canada, 2015-2016. MMWR Morb. Mortal Wkly. Rep. 65, 879–881. doi: 10.15585/mmwr.mm6533a6
Selma, M. V., Luna, M. C., Martínez-Sánchez, A., Tudela, J. A., Beltrán, D., Baixauli, C., et al. (2012). Sensory quality, bioactive constituents and microbiological quality of green and red fresh-cut lettuces (Lactuca sativa L.) are influenced by soil and soilless agricultural production systems. Postharvest Biol. Technol. 63, 16–24. doi: 10.1016/j.postharvbio.2011.08.002
Sharma, M., Lakshman, S., Ferguson, S., Ingram, D. T., Luo, Y., and Patel, J. (2011). Effect of modified atmosphere packaging on the persistence and expression of virulence factors of Escherichia coli O157:H7 on shredded iceberg lettuce. J. Food Prot. 74, 718–726. doi: 10.4315/0362-028X.JFP-10-427
Sharma, M., Patel, J. R., Conway, W. S., Ferguson, S., and Sulakvelidze, A. (2009). Effectiveness of bacteriophages in reducing Escherichia coli O157:H7 on fresh-cut cantaloupes and lettuce. J. Food Prot. 72, 1481–1485. doi: 10.4315/0362-028X-72.7.1481
Sheng, H., Knecht, H. J., Kudva, I. T., and Hovde, C. J. (2006). Application of bacteriophages to control intestinal Escherichia coli O157:H7 levels in ruminants. Appl. Environ. Microbiol. 72, 5359–5366. doi: 10.1128/AEM.00099-06
Singh, S., and Shalini, R. (2016). Effect of hurdle technology in food preservation: a review. Crit. Rev. Food Sci. Nutr. 56, 641–649. doi: 10.1080/10408398.2012.761594
Slayton, R. B., Turabelidze, G., Bennett, S. D., Schwensohn, C. A., Yaffee, A. Q., Khan, F., et al. (2013). Outbreak of shiga toxin-producing Escherichia coli (STEC) O157:H7 associated with romaine lettuce consumption, 2011. PLoS ONE 8:e55300. doi: 10.1371/journal.pone.0055300
Small, P., Blankenhorn, D., Welty, D., Zinser, E., and Slonczewski, J. L. (1994). Acid and base resistance in escherichia coli and shigella flexneri: role of rpos and growth ph. J. Bacteriol. 176, 1729–1737. doi: 10.1128/jb.176.6.1729-1737.1994
Smith, H. V., and Rose, J. B. (1998). Waterborne cryptosporidiosis: current status. Parasitol. Today 14, 14–22. doi: 10.1016/S0169-4758(97)01150-2
Söderqvist, K., Osman, O. A., Wolff, C., Bertilsson, S., Vågsholm, I., and Boqvist, S. (2017). Emerging microbiota during cold storage and temperature abuse of ready-to-eat salad. Infect. Ecol. Epidemiol. 7:1328963. doi: 10.1080/20008686.2017.1328963
Söderström, A., Osterberg, P., Lindqvist, A., Jonsson, B., Lindberg, A., Blide Ulander, S., et al. (2008). A large Escherichia coli O157 outbreak in Sweden associated with locally produced lettuce. Foodborne Pathog. Dis. 5, 339–349. doi: 10.1089/fpd.2007.0065
Solomon, E. B., and Matthews, K. R. (2005). Use of fluorescent microspheres a a tool to investigate bacterial interactions with growing plants. J. Food Prot. 67, 2342–2253. doi: 10.4315/0362-028X-68.4.870
Stadler, B., and Müller, T. (2000). Effects of aphids and moth caterpillars on epiphytic microorganisms in canopies of forest trees. Can. J. Forest Res. 30, 631–638. doi: 10.1139/x99-253
Steele, M., and Odumeru, J. (2004). Irrigation water as source of foodborne pathogens on fruit and vegetables. J. Food Protect. 67, 2839–2849. doi: 10.4315/0362-028X-67.12.2839
Stern, N. J., Pierson, M. D., and Kotula, A. W. (1980). Effects of pH and sodium chloride on Yersinia enterocolitica growth at room and refrigeration temperatures. J. Food Sci. 45, 64–67. doi: 10.1111/j.1365-2621.1980.tb03871.x
Takakura, T., and Feng, W. (2002). Climate Under Cover. Dordrecht: Kluwer Academic Publisher. doi: 10.1007/978-94-010-0583-8
Takeuchi, K., and Frank, J. F. (2000). Penetration of Escherichia coli O157:H7 into lettuce tissues as affected by inoculum size and temperature and the effect of chlorine treatment on cell viability. J. Food Protect. 63, 434–440. doi: 10.4315/0362-028X-63.4.434
Takkinen, J., Nakari, U. M., Johansson, T., Niskanen, T., Siitonen, A., and Kuusi, M. (2005). A nationwide outbreak of multiresistant Salmonella typhimurium in Finland due to contaminated lettuce from Spain, May 2005. Euro Surveill 10:E050630–E050631. doi: 10.2807/esw.10.26.02734-en
Tataryn, J., Morton, V., Cutler, J., McDonald, L., Whitfield, Y., Billard, B., et al. (2014). Outbreak of E. coli O157:H7 associated with lettuce served at fast food chains in the Maritimes and Ontario, Canada, Dec 2012. Can. Commun. Dis. Rep. 40(Suppl. 1), 2–9.
Taylor, E. V., Nguyen, T. A., Machesky, K. D., Koch, E., Sotir, M. J., Bohm, S. R., et al. (2013). Multistate outbreak of Escherichia coli O145 infections associated with romaine lettuce consumption, 2010. J. Food Prot. 76, 939–944. doi: 10.4315/0362-028X.JFP-12-503
te Giffel, M. C., and Zwietering, M. H. (1999). Validation of predictive models describing the growth of Listeria monocytogenes. Int. J. Food Microbiol. 46, 135–149. doi: 10.1016/S0168-1605(98)00189-5
Thomas, E. M., Chapman, B., Jaykus, L. A., and Phister, T. (2014). Tracing temperature patterns of cut leafy greens during service in North Carolina school food service. J. Food Prot. 77, 1495–1500. doi: 10.4315/0362-028X.JFP-14-121
Thompson, J. F., Mitchell, F. G., and Kasmire, R. F. (2002). “Cooling horticultural commodities,” in Postharvest Technology of Horticultural Crops, 3rd Edn, ed A. A. Kader, (San Pablo, CA: University of California Agricultural and Natural Resources), 97–112.
Tomás-Callejas, A., López-Velasco, G., Camacho, A. B., Artés, F., Artés-Hernandes, F., and Suslow, T. V. (2011). Survival and distribution of Escherichia coli on diverse fresh-cut baby leafy greens under preharvest through postharvest conditions. Int. J. Food Microbiol. 151, 216–222. doi: 10.1016/j.ijfoodmicro.2011.08.027
Truchado, P., Gil, M. I., Reboleiro, P., Rodelas, B., and Allende, A. (2017). Impact of solar radiation exposure on phyllosphere bacterial community of red-pigmented baby leaf lettuce. Food Microbiol. 66, 77–85. doi: 10.1016/j.fm.2017.03.018
Tukey, H. B. (1966). Leaching of metabolites from above-ground plant parts and its implications. Bull. Torrey Botan. Club 93, 385–401. doi: 10.2307/2483411
Tukey, H. B. (1970). The leaching of substances from plants. Ann. Rev. Plant Physiol. 21, 305–324. doi: 10.1146/annurev.pp.21.060170.001513
Tukey, H. B., and Mecklenburg, R. A. (1964). Leaching of metabolites from foliage and subsequent reabsorption and redistribution of the leachate in plants. Am. J. Botany 51, 737–742. doi: 10.1002/j.1537-2197.1964.tb06694.x
Tukey, H. B., and Morgan, J. V. (1963). Injury to foliage and its effect upon the leaching of nutrients from above-ground plant parts. Physiol. Plant. 16, 557–564. doi: 10.1111/j.1399-3054.1963.tb08333.x
Uhlig, E., Olsson, C., He, J., Stark, T., Sadowska, T., Molin, G., et al. (2017). Effects of household washing on bacterial load ans removal of Escherichia coli from lettuce and “ready-to-eat” salads. Food Sci. Nutr. 5, 1215–1220. doi: 10.1002/fsn3.514
van der Horst, M. A., Key, J., and Hellingwerff, K. J. (2007). Photosensing in chemotrophic, non-phototrophic bacteria: let there be light sensing too. Trends Microbiol. 15, 554–562. doi: 10.1016/j.tim.2007.09.009
Van Haute, S., Tryland, I., Escudero, C., Vanneste, M., and Sampers, I. (2017). Chlorine dioxide as water disinfectant during fresh-cut iceberg lettuce washing: disinfectant demand, disinfection efficiency, and chlorite formation. LWT Food Sci. Technol. 75, 301–304. doi: 10.1016/j.lwt.2016.09.002
Vestrheim, D. F., Lange, H., Nygård, K., Borgen, K., Wester, A. L., Kvarme, M. L., et al. (2016). Are ready-to-eat salads ready to eat? An outbreak of Salmonella coeln linked to imported, mixed, pre-washed and bagged salad, Norway, November 2013. Epidemiol. Infect. 144, 1756–1760. doi: 10.1017/S0950268815002769
Vetterling, J. M., and Doran, D. J. (1969). Storage polysaccharide in coccidial sporozites after excystation and penetration of cells. J. Eukaryot. Microbiol. 16, 772–775. doi: 10.1111/j.1550-7408.1969.tb02341.x
Vurma, M., Pandit, R. B., Sastry, S. K., and Yousef, A. E. (2009). Inactivation of Escherichia coli O157:H7 and natural microbiota on spinach leaves using gaseous ozone during vacuum cooling and simulated transportation. J. Food Prot. 72, 1538–1546. doi: 10.4315/0362-028X-72.7.1538
Wadamori, Y., Gooneratne, R., and Hussain, M. A. (2017). Outbreaks and factors influencing microbiological contamination of fresh produce. J. Sci. Food Agric. 97, 1396–1403. doi: 10.1002/jsfa.8125
Wetzel, D. M., Schmidt, J., Kuhlenschmidt, M. S., Dubey, J. P., and Sibley, L. D. (2005). Gliding motility leads to active cellular invasion by Cryptosporidium parvum sporozoites. Infect. Immunity 73, 5379–5387. doi: 10.1128/IAI.73.9.5379-5387.2005
Williams, T. R., and Marco, M. L. (2014). Phyllosphere microbiota composition and microbial community transplantation on lettuce plants grown indoors. mBio 5, e01564–e01514. doi: 10.1128/mBio.01564-14
Wu, L., McGrane, R. S., and Beattie, G. A. (2013). Light regulation of swarming motility in Pseudomonas syringae integrates signaling pathways mediated by a bacteriophytochrome and a LOV protein. mBio 4, e00334–e00313. doi: 10.1128/mBio.00334-13
Yoshida, S., Hiradate, S., Koitabashi, M., Kamo, T., and Tsushima, S. (2017). Phyllosphere methylobacterium bacteria contain UVA-absorbing compounds. J. Photochem. Photobiol. B Biol. 167, 168–175. doi: 10.1016/j.jphotobiol.2016.12.019
Zaczek, M., Weber-Dabrowska, B., and Górski, A. (2015). Phages in the global fruit and vegetable industry. J. Appl. Microbiol. 118, 537–556. doi: 10.1111/jam.12700
Zeng, W., Vorst, K., Brown, W., Marks, B. P., Jeong, S., Pérez-Rodríguez, F., et al. (2014). Growth of Escherichia coli O157:H7 and Listeria monocytogenes in packaged fresh-cut romaine mix at fluctuating temperatures during commercial transport, retail storage, and display. J. Food Prot. 77, 197–202. doi: 10.4315/0362-028X.JFP-13-117
Keywords: Escherichia coli, foodborne disease, food system, listeria, primary production processing, Salmonella, spoilage
Citation: Mogren L, Windstam S, Boqvist S, Vågsholm I, Söderqvist K, Rosberg AK, Lindén J, Mulaosmanovic E, Karlsson M, Uhlig E, Håkansson Å and Alsanius B (2018) The Hurdle Approach–A Holistic Concept for Controlling Food Safety Risks Associated With Pathogenic Bacterial Contamination of Leafy Green Vegetables. A Review. Front. Microbiol. 9:1965. doi: 10.3389/fmicb.2018.01965
Received: 29 April 2018; Accepted: 02 August 2018;
Published: 24 August 2018.
Edited by:
Nicola Holden, James Hutton Institute, United KingdomReviewed by:
James Monaghan, Harper Adams University, United KingdomNigel Cook, Jorvik Food and Environmental Virology Ltd, United Kingdom
Copyright © 2018 Mogren, Windstam, Boqvist, Vågsholm, Söderqvist, Rosberg, Lindén, Mulaosmanovic, Karlsson, Uhlig, Håkansson and Alsanius. This is an open-access article distributed under the terms of the Creative Commons Attribution License (CC BY). The use, distribution or reproduction in other forums is permitted, provided the original author(s) and the copyright owner(s) are credited and that the original publication in this journal is cited, in accordance with accepted academic practice. No use, distribution or reproduction is permitted which does not comply with these terms.
*Correspondence: Lars Mogren, TGFycy5Nb2dyZW5Ac2x1LnNl