- 1Nutraceuticals and Functional Proteomics Potential of Biodiversity in Tunisia, Higher Institute of Applied Biological Sciences of Tunis (ISSBAT), University of Tunis El Manar, Tunis, Tunisia
- 2Faculty of Health Sciences, School of Nutrition Sciences, University of Ottawa, Ottawa, ON, Canada
- 3Department of Microbiology and Immunology, Faculty of Pharmacy, Mansoura University, Mansoura, Egypt
A considerable number of strains belonging to different species of Enterococcus are highly competitive due to their resistance to wide range of pH and temperature. Their competitiveness is also owed to their ability to produce bacteriocins recognized for their wide-range effectiveness on pathogenic and spoilage bacteria. Enterococcal bacteriocins have attracted great research interest as natural antimicrobial agents in the food industry, and as a potential drug candidate for replacing antibiotics in order to treat multiple drugs resistance pathogens. However, the prevalence of virulence factors and antibiotic-resistance genes and the ability to cause disease could compromise their application in food, human and animal health. From the current regulatory point of view, the genus Enterococcus is neither recommended for the QPS list nor have GRAS status. Although recent advances in molecular biology and the recommended methods for the safety evaluation of Enterococcus strains allowed the distinction between commensal and clinical clades, development of highly adapted methods and legislations are still required. In the present review, we evaluate some aspects of Enterococcus spp. related to their probiotic properties and safety concerns as well as the current and potential application in food systems and treatment of infections. The regulatory status of commensal Enterococcus candidates for food, feed, probiotic use, and recommended methods to assess and ensure their safety are also discussed.
Introduction
Enterococci are lactic acid bacteria (LAB) comprising both pathogenic and commensal microorganisms ubiquitous in environment even as gut symbionts. Due to their tolerance to salts and acids, the strains of Enterococcus spp. are highly adapted to several food systems, they are also involved in the fermentation activity of traditionally manufactured cheese and dry sausages, in which it is believed that they contribute to the development of organoleptic characteristics of these products (Foulquié Moreno et al., 2006). In addition, several number of Enterococcus strains have been reported to produce antimicrobial compounds including bacteriocins. Bacteriocin production have be applied to preservation of a wide range of food products and is now being considered as a probiotic trait (Yang et al., 2014). besides, bacteriocins are considered as promising alternative to fight emerging antimicrobial resistance (Cotter et al., 2013; Hammami et al., 2013). Although certain antibiotic-resistant, infectious strains of enterococci, including E. faecium, have been identified in hospital patients, they very rarely present a risk of infection for humans outside healthcare settings (Sanders et al., 2010). To date, the genus Enterococcus has not yet obtained the status generally recognized as safe (GRAS) (Huys et al., 2013), but some members are used as probiotics and in production of feed additives to prevent diarrhea or to improve growth in animals (Franz et al., 2011). This situation has created a requirement for new regulation of probiotics in order to distinguish between safe and potentially harmful strains. In this review, probiotic potential, safety use as well as recent advances in knowledge about bacteriocin production by enterococci will be discussed, with emphasis on the potential opportunities for application in various fields.
Legislation
With regard to safety and according to the Qualified Presumption of Safety (QPS) list from the European Food Safety Authority (EFSA) (https://www.efsa.europa.eu/en/topics/topic/qps), Enterococcus species are neither recommended for the QPS list (EFSA Panel on Biological Hazards et al., 2017) nor have GRAS status (Ogier and Serror, 2008), in spite of recent scientific knowledge allowing differentiation of commensal from pathogenic strains (Montealegre et al., 2016; Bonacina et al., 2017; Jung et al., 2017). In this regard, recent advances in molecular epidemiology based on molecular fingerprinting, multi-locus sequence typing, phenotypic studies and whole-genome analyses have provided further evidences that nosocomial strains of Enterococcus are genotypically different from commensal strains. For instance, E. faecium has been subtyped into three different clades: the hospital-associated clade A1, rarely found in healthy individuals; the animal-associated clade A2; and the community-associated clade B, commonly found in healthy individuals and rarely causes infections (Montealegre et al., 2016). Beukers and coauthors have compared complete genomes of E. faecium from the NCBI database to demonstrate differential clustering of commensal and clinical isolates, suggesting that these strains may be specifically adapted to their respective environments (Beukers et al., 2017). Similarly, the difference between ability of pathogenic and commensal E. cecorum isolates from different animal species to metabolize mannitol may be explained by a separate evolution of pathogenic E. cecorum isolates (Jung et al., 2017). In addition, investigation of the genome sequences of 4 groups of Enterococcus species from food origin including dairy, meat, probiotics and probiotics from dairy origin showed no correlation between isolation source/probiotic properties and phylogenetic signal neither at species or strain levels (Bonacina et al., 2017). Although further evidences are required, the advances outlined above support the call for new recommendations about probiotic legislative framework in order to distinguish between safe and potentially harmful Enterococcus strains. Yet, organizations such as the EFSA, the Advisory Committee on Novel Foods and Processes, ACNFP, and the Food Standards Agency permitted the use of certain strains of enterococci as a food additive and supplements based on a careful case-by-case assessment. In this case, the individual strain must be considered and health risks must be excluded for this specific strain (ACNFP, 1996; Franz et al., 2011; Lauková, 2011; EFSA, 2012a). The EFSA guidance (EFSA, 2012a) provides a methodology for distinguishing between safe and potentially harmful strains of E. faecium in animal nutrition. It is intended for use as feed additive producers submitting applications to EFSA for safety assessment. According to this guidance, strains to be used in animal nutrition shall be susceptible to ampicillin (MIC ≤ 2 mg/L) and shall not harbor one of the genetic elements IS16, hylEfm, and esp. For the evaluation of new probiotic candidates by EFSA, the full strain genome should be available (Brodmann et al., 2017). Specific strains of E. faecium and E. faecalis are the only enterococci used as probiotics or feed additives (Franz et al., 2011). The use of other enterococci species is subject to little or no regulation in spite of the increasing number of studies that elucidate the probiotic potential of some species such as E. munditii, E. durans and E. hirae (Nami et al., 2014; Pieniz et al., 2014; Gupta and Tiwari, 2015; van Zyl et al., 2016). Figure 1 illustrates a proposed decision scheme based on EFSA regulations for the safety assessment of enterococci probiotic candidates leading to food/feed applications.
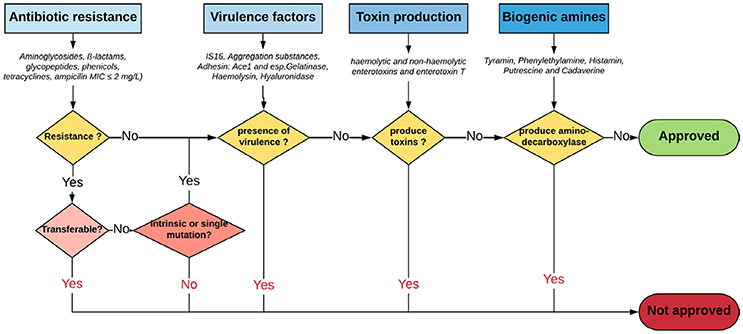
Figure 1. Proposed decision scheme for the safety assessment of Enterococci probiotic candidates leading to food/feed applications. Adapted from EFSA Panel on Biological Hazards (2011), EFSA (2012a) and Laulund et al. (2017).
Bacteriocins are antimicrobial compounds produced by bacteria that have high potential of application as natural food additives to enhance food safety (Cotter et al., 2005; Yang et al., 2014; Egan et al., 2016). From the perspective of the current legislation, nisin and pediocin PA1/AcH has been the only bacteriocins approved for utilization as food additives by FDA in USA and EU regardless the considerable number of experimental work on the application of bacteriocins in food (Vignolo et al., 2012; Barbosa et al., 2017). With the emergence and rapid spread of resistance to conventional antibiotics, bacteriocins are considered to be an attractive alternative in the treatment of antibiotic-resistant bacterial infections (Cotter et al., 2013; Hammami et al., 2013), and a few are being tested in clinical trials (Behrens et al., 2017). Bacteriocins are potent, lowly toxic and target a narrow range of bacteria so they can act without affecting much of the natural microbiota which is a common drawback of conventional antibiotic use (Lohans and Vederas, 2012; Ołdak and Zielinska, 2017). The gene-encoded nature of bacteriocins makes them easily amenable through bioengineering to either increase their activity or specify target microorganism (Yang et al., 2014). Nevertheless, probiotic strains or bacteriocins intended for use as a therapeutic must undergo the regulatory process as a new drug, and must be authorized by the FDA (Venugopalan et al., 2010).
Advantages of Enterococci and Their Bacteriocins
Enterococci are extensively studied as potential candidate probiotics. Considerations for strain selection include several criteria such as molecular identification using genetic typing techniques, safety, capacity to survive intestinal transit, manufacturing, distribution, and targeted application. The functional requirements of probiotics include tolerance to human gastric juice and bile, adherence to epithelial surfaces, persistence in the human gastrointestinal tract (GIT), immune stimulation, antagonistic activity toward intestinal pathogens (such as Helicobacter pylori, Salmonella spp., Listeria monocytogenes, and Clostridium difficile), and the capacity to stabilize and modulate the intestinal microbiota. Bacteriocin production is now one of the desirable traits in the selection of a probiotic strain (Dobson et al., 2012). Strains belonging to the genus Enterococcus produce a wide variety of bacteriocins often called enterocins. They have been widely studied, mainly because they are active against Gram-positive foodborne pathogens, such as L. monocytogenes (Izquierdo et al., 2009). E. faecium and E. faecalis are the main producers of enterocins and to a lesser extent E. mundtii, E. avium, E. hirae, and E. durans. Some of these bacteriocins can be grouped with typical bacteriocins produced by LABs according to traditional classification (Cotter et al., 2005), whereas others could not be included. Franz et al. (2007) suggested grouping enterocins into a new four classes. Class I, lantibiotic enterocins, rarely found in enterococci are represented only by cytolysin (Christopher et al., 2005) and enterocin W (Sawa et al., 2012) both from E. faecalis isolates. The enterocin of this class is a two-component bacteriocin consisting of two linear peptides structurally different from other linear lantibiotics such as nisin A and Z as well as from smaller globular peptide lantibiotics. It contains lanthionine residues, which suggests their consideration as two-component lantibiotics. Class II, enterocins of the pediocin family. The Class II.1 of pediocin-like bacteriocins is divided into two subgroups according to sequence similarities (Lauková, 2011). Subgroup 1 includes enterocin A (Aymerich et al., 2000), mundticin (produced by E. mundtii) (Kawamoto et al., 2002) and enterocin CRL5 (Saavedra et al., 2004); and subgroup 2 includes enterocin P (Cintas et al., 1997) and enterocin M (Marekova et al., 2007), a variant of enterocin P. The Class II.2 refers to enterocins synthesized without a leader peptide such as two peptide bacteriocin L50 (A, B) (Cintas et al., 2000), enterocin Q (Cintas et al., 2000) and enterocin C (Maldonado-Barragan et al., 2009). Liu et al. (2011) reported that leaderless bacteriocins might possess a formyl group in their N-terminal methionine. Class II.3 includes other linear-non-pediocin-type enterocins such as enterocin B (Casaus et al., 1997). Class III regroups cyclic antibacterial peptides including enterocin AS-48 produced by E. faecalis S-48 (Maqueda et al., 2004). Finally, Class IV includes enterolysin A produced by E. faecalis (Nilsen et al., 2003). Recently, a glycosylated bacteriocin, enterocin F4-9, from E. faecium has been described by Maky et al. (2015). Only four bacteriocins produced by E. durans have been purified so far namely: durancin TW-49M a class II non-pediocin-like bacteriocin homologous to enterocin B (Hu et al., 2008), durancin GL produced by E. durans 41D and belonging to class II (Du et al., 2012), durancin L28-1A (Yanagida et al., 2005), and peptides A5-11A and A5-11B belonging to class II of bacteriocins with a high degree of similarity to enterocins L50A and L50B (Cintas et al., 2000; Batdorj et al., 2006). A large list of enterococci bacteriocins with their structure and spectrum of action is also available at BACTIBASE database (Hammami et al., 2010) (http://bactibase.hammamilab.org/Producer/Enterococcus).
Wide-Spectrum Activity
Enterocins display a board spectrum of activity, they inhibit not only closely related species but also Gram-positive pathogens in particular, the genus Listeria (Khan et al., 2010). This attribute has been observed for example, in enterocin E-760 (Line et al., 2008), enterocin P (Cintas et al., 1997), enterocin LR/6 (Kumar and Srivastava, 2010), and Enterocin AS-48 (Cobo Molinos et al., 2008). Besides, enterocins DD28 and DD93 have been reported to be active against MRSA and prevented film formation (Al Atya et al., 2016). Similarly, strains E. durans 61A (Hanchi et al., 2016), E. mundtii ST15 (De Kwaadsteniet et al., 2005), and ST4SA (Dicks et al., 2010) have showed a broad spectrum of activity including Gram-negative bacteria, an unusual property of bacteriocins produced by LABs. Therefore, bacteriocin-producing bacteria could be used to get rid of Gram-negative pathogens in mammalian GITs (Kommineni et al., 2015). Indeed, bacteriocin production was shown to augment niche competition by enterococci in the mammalian GIT. Besides, some enterocins were shown to inhibit the growth of some mold spores such as durancin (Belguesmia et al., 2013). Nevertheless, a comprehensive assessment of the antifungal potential of enterocins is still lacking. Many enterocins have been reported to inhibit spore-forming bacteria, reviewed in Egan et al. (2016). Enterocin DD14 was reported being active against Clostridium (Caly et al., 2017), while durancin TW-49M (Hu et al., 2008) and Enterocin NKR-5-3B (Himeno et al., 2015) were potent against Bacillus circulans. Similarly, enterocin AS-48 displayed significant activity against endospores of Alicyclobacillus acidoterrestris and Bacillus licheniformis when combined with thermal treatment (Grande Burgos et al., 2014). Finally, bacteriocin ST5Ha produced by E. faecium ST5Ha (Todorov et al., 2010) and enterocin CRL35 produced by E. mundtii CRL35 (Wachsman et al., 2003) exhibit antiviral activities against herpes simplex viruses HSV-1 and HSV-2. Likewise, E. mundtii ST4V, isolated from soya beans, was also shown to produce a 3950 Da peptide ST4V with broad spectrum of inhibition against several bacteria and viruses including HSV-1, HSV-2, polio virus and measles virus (Todorov et al., 2005).
Multi-Bacteriocin Producers
Enterococci are characteristically tolerant to extreme pHs, temperatures, and high salt concentration (Fisher and Phillips, 2009). Moreover, some enterococci strains harbor simultaneously many bacteriocin-related genes, which provide them a competitive advantage toward other microbial species in ecological niches (Vandera et al., 2017). These features are useful in food applications against spoilage and pathogenic organism contamination (Henning et al., 2015). Cintas and colleagues have reported that E. faecium L50, a producer of enterocin L50A and L50B, is able to produce two other additional bacteriocins (enterocin P and enterocin Q) at 37° and 47°C, respectively (Cintas et al., 2000). Likewise, E. durans 61A was recently shown to produce simultaneously formylated and nonformylated forms of enterocins L50A and L50B as well as durancin 61A, a new glycosylated 5217 Da peptide (Hanchi et al., 2016). Several other multi-bacteriocinogenic strains have been reported such as E. faecium NKR-5-3 (Perez et al., 2012), E. faecium WHE 81 (Izquierdo et al., 2009), E. faecium LM-2 (Liu G. et al., 2011) and E. faecium MMRA (Rehaiem et al., 2014).
Current Applications of Enterococci and Their Bacteriocins
As a normal inhabitant of the gut, Enterococcus strains are able to survive, compete and adhere to host cells in the GIT, important feature for a successful use as probiotics (Laukova et al., 2017). The genus Enterococcus also includes a wide range of strains suitable as starter cultures where they play a positive role in the development of the typical organoleptic characteristics of various fermented foods, including meat, dairy and vegetable products (Franz et al., 2011). Furthermore, the killing ability of bacteriocins produced by Enterococcus strains is considered a successful strategy for maintaining population and reducing the numbers of competitors (Yang et al., 2014). Over recent years, purified and identified enterococci bacteriocins were added to foods in the form of concentrated preparations or produced in situ by bacteriocinogenic starter, adjunct or protective cultures (Arqués et al., 2015). These bacteriocins are being considered as promising drug candidates for replacing antibiotics to treat multiple drugs resistant pathogens and maintain human health (Hammami et al., 2013). Bacteriocins demonstrated additive or synergistic effects in combination with other antimicrobial agents providing novel opportunities for more effective control of pathogens in human and veterinary medicine (Hammami et al., 2013). The current applications of Enterococcus strains and their bacteriocins as probiotics, in food, in veterinary and medical fields are summarized in Figure 2.
Enterococci and Their Bacteriocins in Food
The application of enterococci in food fermentation process is still debatable. Indeed, their presence in food is considered a sign of fecal contamination, but more recently they have been accepted as part of the normal microbiota and are commonly used in food industry (Franz et al., 2011). The genus Enterococcus is allocated into risk group 2, which includes microorganisms harboring virulence factors (EC, 2000). Thus, they can act as a reservoir for dissemination of antibiotic resistance and virulence genes through the food chain (Jahan et al., 2015). Horizontal gene transfer has been shown to be a mechanism of transmission between enterococci and other genera. in vitro studies have demonstrated that transfer between Enterococcus and S. aureus could occur (de Niederhausern et al., 2011). Nevertheless, horizontal gene transfer of the van operon between Enterococcus spp. and other organisms appears to occur at a very low frequency (Faron et al., 2016). As indicated above (Legislation section and Figure 1), the susceptibility to ampicillin and virulence factors and markers IS16, hylEfm, and esp are considered relevant for the assessment of safety (EFSA, 2012a). Recommended methods for the safety assessment of Enterococcus are summarized in Table 1. Safety is investigated through microbiological (hemolysis, production of gelatinase, antibiogram determination), molecular tests (antibiotic resistance genes and virulence factors, genes involved in the formation of BA), and detection of toxin production (for Non-QPS Strains). Finally, an assessment of lack of infectivity by the candidate strain in immunocompromised animals would add a measure of confidence in the safety (FAO/WHO, 2002). The amplification of the IS16 gene by PCR as described by Werner et al. (2011) is recommended, while the hybridization technique described by Rice et al. (2003) is used for detection of esp and hylEfm. As an alternative method, hybridization to colony lysates or Southern blots can be used. Both analysis should contain positive E. faecium ATCC BAA-472 (TX16) or E. faecium DSMZ 25390) and negative control (E. faecium DSMZ 25389) strains (EFSA, 2012a). Beside genotypic tests, phenotypic assessment is also needed. For instance, the determination of antibiotic's (mainly ampicillin) minimum inhibitory concentration (MIC) should be performed according to internationally recognized standards such as European Union Committee on Antimicrobial Susceptibility Testing (EUCAST), the Clinical and Laboratory Standard Institute (CLSI), ISO standard or similar. Currently, an ISO standard is available for bifidobacteria and non-enterococcal lactic acid bacteria. Strains with a MIC cut-off value above 2 mg/L are not considered safe and will not be authorized. The absence of hemolysis can be easily screened by plating on blood agar, while cytotoxicity could be assessed on Vero cells (Laulund et al., 2017). Thus, it has been suggested that application of enterococci in food could be admitted on the basis of careful selection and case-by-case studies (Giraffa, 2002; Foulquié Moreno et al., 2006).
The antimicrobial activity of bacteriocins against foodborne pathogens and spoilage bacteria has attracted considerable attention for their application in food preservation (Barbosa et al., 2017; Pisoschi et al., 2018). In addition, the use of bacteriocins can help reduce the use of chemical preservatives and/or intensity of heat and other physical treatments (Yang et al., 2014). In recent years, considerable efforts have been made to develop applications of bacteriocins and bacteriocinogenic strains in many food products. E. faecium and E. faecalis are bacteriocins producing species predominant in food (Cotter et al., 2005; Javed et al., 2011). Their bacteriocins can prevent the growth of several other bacteria such as L. monocytogenes, Staphylococcus aureus and Vibrio cholerae.
Dairy Products
Enterococci among other LABs present in raw milk can act as natural starters. They also are able to survive during milk refrigeration and pasteurization temperatures due to their psychrotrophic nature, heat resistance and adaptability to different substrates and growth conditions (Bhardwaj et al., 2008). Enterococci are important in dairy industry and commonly present as non-starter LABs in cheese in Southern Europe. They are important in the maturation of different cheese varieties, probably due to their proteolytic or lipolytic activity, their ability to ferment citrate to produce diacetyl and other volatile compounds that contribute to the flavor or to provide a characteristic flavor and taste (Foulquié Moreno et al., 2006). For instance, E. durans and E. faecalis have been reported in raw and fermented milk and cheese products (Hanchi et al., 2014). Indeed, different species of enterococci are commonly found in cheese including E. faecium, E. faecalis, E. durans, and in a lesser extent E. casseliflavus (Ogier and Serror, 2008). Those cheeses are made from raw milk of goats, sheeps or cows. The UK Advisory Committee on Novel Foods has previously accepted the use of E. faecium K77D as a starter strain in fermented dairy products (ACNFP, 1996). In addition, several studies have been devoted to the detection, purification and characterization of the enterocins and their application as dairy food preservative. For instance, E. faecalis strains OSY-RM6, L3A21M3, and L3A21M8 produce thermolabile enterocins and E. faecium RZS C5 produces bacteriocins with activity against foodborne pathogens in milk and cheese (Leroy et al., 2002; Huang et al., 2013). More recently, a bacteriocin-like substance produced by E. durans E204 was proven to inhibit the growth of L. monocytogenes in cheese (Khay et al., 2014). Likewise, application of enterocins AS-48 and CRL35 inhibited spoilage and pathogenic microorganisms such as S. aureus, L. monocytogenes in different dairy products (Nuñez et al., 1997; Farias et al., 1999; Rodríguez et al., 2001; Arqués et al., 2015).
Fermented Vegetables
The source of enterococci in-plant is not clearly defined, whether endogenous or an outcome of environmental contamination. The plant-associated enterococci species mainly include E. faecium, E. mundtii, E. casseliflavus, E. faecalis, and E. sulfurous (Müller et al., 2001). Enterococci were found in fermented green olives and are likely to be involved in the fermentation process of olives (de Castro et al., 2002; De Bellis et al., 2010; Corsetti et al., 2012; Lucena-Padrós et al., 2014). Those members are well adapted for initial pH value ?(9.0) and the salt concentration of the brine used in processing. This finding provides the rationale for using E. faecium and E. casseliflavus in the industrial fermentation of green olives (Corsetti et al., 2012). Some enterococci are also found as well in raw materials used in beer manufacture (Basanta et al., 2008). In addition, they are usually associated with sorghum (Madoroba et al., 2011) and soybean fermentations (De Kwaadsteniet et al., 2005; Kinouchi et al., 2012). Besides, Enterococcus bacteriocins are used as bioprotective agents in many vegetable food matrices such as fruit juices and ready-to-eat, fermented, and non-fermented vegetables (Settanni and Corsetti, 2008). For example, enterocin AS-48 is used in a wide range of vegetable products including cider, sliced fruits, vegetable juices, and canned vegetables (Grande Burgos et al., 2014). Use of enterocin AS-48 is considered effective in the inactivation of Salmonella enterica in contaminated fruit juice (Martinez Viedma et al., 2008), and L. monocytogenes and spore-forming microorganisms in raw and canned vegetables (Lucas et al., 2006; Cobo Molinos et al., 2008). Similarly, enterocins CCM4231 and EJ97 were used in soymilk and zucchini purée for controlling Listeria and Bacillus, respectively (Lauková and Czikková, 1999; García et al., 2004).
Meat Products
Enterococci are normal constituents of the natural microbiota of many fermented-meat products, but can also be found in raw meat (Garriga and Aymerich, 2014), with E. faecium and E. faecalis being the predominant species, followed by E. hirae and E. durans (Franz et al., 2011). E. faecium and E. mundtii are reported in fish and fermented seafood (Ishibashi et al., 2012), and chicken and fermented meat products such as sausages (Hugas et al., 2003; Barbosa et al., 2014). Their presence could contribute in the development of sensory properties of fermented meat products particularly in sausage (Hugas et al., 2003). Other important functional properties including metmyoglobin-reducing activity, ability to degrade anti-nutritive factors like stachyose and raffinose, and production of bile salt hydrolase have also been described for meat enterococci (Omar et al., 2004). In addition to desired fermentation properties, the bacteriocin-producing Enterococcus strains are used as protective cultures used in ready to eat meats and other products (Foulquié Moreno et al., 2006). In fact, the processing conditions, the curing additives, and the presence of lactic starter cultures are effective for pathogen control but not sufficient to prevent the survival of pathogens like E. coli O157:H7, L. monocytogenes and Salmonella during the manufacturing process (Castellano et al., 2017). In this regard, E. faecium RZS C13 and CCM 4231, and E. casseliflavus IM416K1 were shown competitive and strongly inhibited the growth of Listeria spp. when used as starter cultures for the production of fermented sausage (Callewaert et al., 2000; Sabia et al., 2003). Nevertheless, in situ bacteriocin production rely on many factors such as the strain ability for food colonization, thermal treatment, refrigeration, and presence of other preservative agents such as nitrites, sodium chloride, organic acids and other ingredients (Castellano et al., 2017). For example, E. faecium CTC492, an enterocins A and B producer, did not significantly reduce Listeria counts in fermented sausages, which was attributed to a higher inhibition of the producer strain by refrigeration temperatures and sausage ingredients (Aymerich et al., 2000). In contrast, bacteriocins are heat resistant, they can be added in, or on, foods that may be heated or cooked (Vijayakumar and Muriana, 2017). Consequently, several successful applications of enterococci bacteriocins have been reported, mainly concerning their effectiveness in eliminating L. monocytogenes, Salmonella, Clostridium, and other spoilage lactic bacteria. For instance, significant reduction of listerial cells in ham was observed after addition of partially purified bacteriocins from E. durans 152 (an enterocin L50A derivative and enterocin L50B), with anti-listerial protection lasted for at least 30 days at 15°C (Du et al., 2017). Unlikely regrowth of the listerial cell survivors was observed after 1 h of challenge with enterocin CRL35 (Vignolo et al., 2000). Conversely, the application of enterocin 416K1 and semi-purified enterocins A and B on low-density polyethylene and alginate/zein/PVA films, respectively, reduced L. monocytogenes counts by 5 logs in contaminated frankfurters and cooked ham at refrigeration temperature (Marcos et al., 2007; Iseppi et al., 2008). Likewise, enterocin AS-48 alone decreased L. monocytogenes count and significantly inhibited Salmonella when combined with high hydrostatic pressure at the end of ripening of low acid fermented sausage (Ananou et al., 2010). The use of bacteriocins combined with other hurdle technologies may represent a useful approach to enhance antimicrobial effectiveness. Indeed, Turgis et al. (2012) and Liu et al. (2012) reported an improved anti-listerial effect of bacteriocin MT 104 and partially purified enterocin LM-2 combined with γ-irradiation and high hydrostatic pressure, respectively, in sausage and cooked ham.
Due to the activities of meat microbiota, undesirable reactions can also take place, such as the formation of biogenic amines. The presence of biogenic amines in food constitutes a potential public health concern because of their physiological and toxicological effect. At high concentrations, they are known to cause food poisoning, due to their vasoactive properties (Bargossi et al., 2017). The most dangerous are histamine (produced from histidine) and tyramine (produced from tyrosine) and are regarded as undesirable compounds that may cause health disruptions to sensitive consumers (EFSA Panel on Biological Hazards, 2011). Besides food poisoning, these substances affect the freshness and other organoleptic properties of meat and meat products (Hugas et al., 2003). Biogenic amines are mainly accumulated in foods through microbial decarboxylation of certain amino acids (Gardini et al., 2016). Enterococcus species, together with other lactic acid bacteria, constitutes one of the microorganisms that can accumulate higher biogenic amines; they are indeed known as the most efficient tyramine producers in fermented foods (Ladero et al., 2012; Bargossi et al., 2017; Laukova et al., 2017). Therefore, control measures to prevent biogenic amine formation in foods or to reduce their levels once formed must be taken. Hydrostatic pressures, irradiation, controlled atmosphere packaging, represent an important technological tool for controlling biogenic amine production (Naila et al., 2010). In addition, modeling the microorganisms responsible for biogenic amine formation has been proposed to control biogenic amine accumulation (Gardini et al., 2008). However, these approaches may not be efficient. Another tool able to counteract biogenic amines accumulation in fermented meats is to use starter cultures free of any decarboxylating activity and able to inhibit decarboxylase-positive bacteria (Latorre-Moratalla et al., 2012; Gardini et al., 2016). It has been demonstrated that using bacteriocinogenic strains and/or bacteriocins could contribute to reduce the risks of survival and multiplication of amino-biogenic bacteria during ripening and storage of fermented foods (Joosten and Nunez, 1996; Tabanelli et al., 2014). For instance, Laukova et al. (2017) tested the sensitivity of biogenic amines-producing Enterococcus strains from poultry to seven partially purified enterocins and found that although these strains featured high biogenic amines production, they were sensitive to the tested enterocins. Earlier, the application of enterocin AS-48 reduced biogenic amines-forming LABs in sardine filets (Ananou et al., 2014). The levels of other biogenic amines such as cadaverine, putrescine, tyramine, and histamine were significantly reduced by several folds in the enterocin AS-48-treated samples after storage, reviewed in (Grande Burgos et al., 2014). Likewise, E. faecium MCL13 was found to produce an antibacterial compound with inhibitory activity against the tested histamine-producing bacteria and exhibited histamine-degradation ability in fermented fish (Lim, 2016). Furthermore, the production of biogenic amines during fermentation can be controlled by the use of amine oxidizing bacteria and enzymes (Naila et al., 2010). Only a single study reported this ability to degrade biogenic amines among Enterococcus (Guarcello et al., 2016).
Enterococci as Probiotics
Many studies have been conducted to evaluate the probiotic characteristics of Enterococcus strains, mainly E. faecium. Due to safety concerns, lack of safety information, and legislation, only a limited number are commercialized. Enterococcus has not yet obtained the status GRAS (Franz et al., 2011). However, some strains such as E. faecium M74 and E. faecium SF-68 are included as food supplements in several probiotic preparations, that have been proved to be effective and safe, such as Cernivet® and FortiFlora® (containing E. faecium SF68®, Cerbios-Pharma SA, Switzerland), and Symbioflor® 1 with E. faecalis (Symbiopharm, Herborn, Germany) (Serio et al., 2010). Enterococci probiotics can be used in treatment and/or prevention of certain human and animal diseases such as alleviation of irritable bowel syndrome symptoms and antibiotic-induced diarrhea and prevention of different functional and chronic intestinal diseases (Bybee et al., 2011). Moreover, some enterococci exhibit anticarcinogenic, hypocholesterolemic, as well as immune regulation effects. For instance, E. durans M4-5 has been found to generate butyrate, a short chain fatty acids (SCFAs) that induce significant anti-inflammatory effects and contribute to the integrity of the intestinal epithelium (Avram-Hananel et al., 2010). Similarly, administration of E. faecium M74® was associated with a reduction of the cholesterol concentration in serum (Hlivak et al., 2005). Furthermore, E. durans KLDS 6.0930 has been postulated as a probiotic candidate through lowering human serum cholesterol levels (Liu et al., 2016). E. mundtii ST4SA was recently presented as another potential probiotic strain (van Zyl et al., 2016). It generates antimicrobial peptides that have activity against a number of pathogens supporting its antimicrobial and probiotic roles (Ramiah et al., 2009). More recently, the strain E. durans LAB18s was recommended useful for use as a source of dietary selenium supplementation (Pieniz et al., 2014), while E. faecium LCW 44 and E. durans 6HL were shown highly potent against Gram-positive (Vimont et al., 2017) and Gram-negative bacteria (Nami et al., 2014; Pieniz et al., 2014; Vimont et al., 2017), respectively.
The application of probiotics in animal nutrition needs prior authorization (EFSA, 2012b; Figure 1). In feed regulation, probiotics are included in the group of feed additives for stabilizing the microbial communities of the digestive tract in both monogastric and ruminant animals (Anadon et al., 2006). For instance, The European Food Standards Agency (EFSA) authorized certain strains of enterococci for use as silage additive and dietary supplements (EFSA, 2012a). Strains E. faecium NCIMB 11181 and E. faecium DSM 7134 were approved as feed additives for calves and piglets by EFSA (EFSA, 2012b). The probiotics E. faecium SF68® (NCIMB 10415 Cerbios- Pharma SA, BARBENGO, Switzerland) and E. faecalis Symbioflor 1 (Symbiopharm, Herborn, Germany) are also used to prevent or treat diarrhea in pigs, poultry, livestock and pets (Bybee et al., 2011; Franz et al., 2011). Probiotics have been shown to have positive effects on the performance characteristics of the growth and health of farm animals. Feeding pigs with the probiotic Enterococcus was found to reduce intestinal pathogens (Liao and Nyachoti, 2017). Similarly, the probiotic E. faecium NCIMB 10415 has increased the capacity of absorption and secretion of mucous membranes in jejunum and improved the intestinal barrier integrity (Bednorz et al., 2013). Likewise, oral administration of E. faecium NHRD IHARA by post-weaning piglets has increased serum and fecal IgA levels and improved piglets growth (Sukegawa et al., 2014). In chickens, E. faecium was demonstrated to improve growth, intestinal morphology, and the caecal microbiota homeostasis (Cao et al., 2013). E. faecium was also reported to improve the metabolic efficiency and decrease inflammatory responses in broilers (Zheng et al., 2016). Additionally, enterocin E-760 (Line et al., 2008), and enterocin DD14 (Caly et al., 2017) were used to control Campylobacter spp. and C. perfringens infections and spread in chicken (Ščerbová and Lauková, 2016). Enterococci are also used as probiotics for dogs based on their tolerance to bile, adhesion activity, antimicrobial activity and their impact on high levels of serum cholesterol and alanine aminotransferase (Bybee et al., 2011). Numerous studies have shown the beneficial effects of enterococci in aquaculture. Several studies reported a wide spectrum of inhibition by E. faecium toward aquatic pathogens including Yersinia ruckeri, Vibrio harveyi, Streptococcus agalactiae and Aeromonas veronii (Swain et al., 2009; Satish Kumar et al., 2011). In addition, several trials have investigated the efficacy of E. faecium incorporated in feed to improve fish growth (Bogut et al., 2000; Chang and Liu, 2002) and stimulate immune response (Panigrahi et al., 2007; Román et al., 2015).
Therapeutic Potential of Enterococcal Bacteriocins
Bacteriocins are an interesting alternative to the use of antibiotics, which have created great public concerns due to the emergence of antimicrobial resistance. New compounds and therapeutic methods for treating infections caused by antibiotic-resistant pathogens and limiting their spread are urgently needed. Hence, there is a need to discover new antimicrobial agents and to develop innovative strategies to fight against those pathogens (Hammami et al., 2013).
Treatment of Gastro-Intestinal Diseases
Gastrointestinal diseases are usually associated with gut microbiota dysbiosis. Probiotics affect the functionality of the GIT by a variety of mechanisms such as interfering with the attachment of pathogens to adhesion sites, out-competing pathogens for nutrients, degradation or other alterations of toxin receptors, production of inhibitory substances (e.g., bacteriocins and/or organic acids) and stimulation of immunity/immunomodulation (Fliss et al., 2011). Species such as H. pylori, C. difficile, L. monocytogenes, and Salmonella are the main bacteria involved in severe gastric infections (Hammami et al., 2013). The broad inhibitory spectra of bacteriocins produced by Enterococcus make them appropriate candidates, alone or in combination with other antibiotics, to prevent or treat these infections. For example, administration of enterocin CRL35 alone showed significant activity against L. monocytogenes, while the genetically engineered hybrid peptide Ent35-MccV displayed a broad spectrum antimicrobial activity against enterohemorrhagic E. coli and L. monocytogenes clinical isolates (Acuña et al., 2012; Salvucci et al., 2012). Moreover, enterocin S760 and enterocin A have significantly reduced Bacillus anthracis and Salmonella infection in mice and quail, respectively (Lauková et al., 2003; Svetoch et al., 2011). Likewise, enterocin E50-52 has effectively been used to treat infection in birds (Svetoch et al., 2008). More recently, durancin 61 A has demonstrated a significant activity when combined with reuterin against C. difficile providing a possible therapeutic use for the treatment of gastrointestinal infections (Hanchi et al., 2017). Although immunomodulation is one of the main mechanisms of action of probiotic bacteria, very few studies have actually documented immunomodulatory properties of Enterococcus. For example, E. faecium L5 has increased the expression of IL-10 and decreased the IL-8 while E. faecalis CECT 7121 and E. faecium JWS 833 have enhanced the cytokine production (Tarasova et al., 2010; Choi et al., 2012; Molina et al., 2015). In a lesser extent, Kanda and collaborators have demonstrated that the administration of E. durans TN-3 suppressed the development of dextran sodium sulfate DSS colitis via the induction of IL-10 producing Treg cells (Kanda et al., 2016). Another recent study have reported that E. durans EP1 modulates gut microbiota by increasing Faecalibacterium prausnitzii, a functionally important bacterium in some cases of dysbiosis (Carasi et al., 2017).
Treatment of Skin Infections
Enterocins are being extensively studied for use in treatment of acne (Figure 2). For example, CBT SL-5 lotion prepared with bacteriocin produced by E. faecalis SL-5 has significantly reduced the inflammatory lesions caused by Propionibacterium acnes, suggesting a potential role in acne treatment as an alternative to antibiotics (Kang et al., 2009). A recent clinical trial demonstrated the leishmanicidal effect of enterocin AS-48, which was lethal on both axenic promastigotes and amastigotes of Leishmania at low micromolar concentrations with scarce toxicity on the host cell (Abengózar et al., 2017). Previously, a patent was issued for the use of AS-48 combined with lysozyme for applications against acne and other skin bacterial infections targeting P. acnes and S. aureus (Maqueda Abreu et al., 2014).
Treatment of Infections Caused by Antibiotic-Resistant Bacteria
Nosocomial infections involving drug-resistant bacteria are a major concern in hospitals. They contribute to increased morbidity, length of stay and the cost of care. The main multi-resistant organisms include methicillin-resistant S. aureus (MRSA), vancomycin resistant Enterococci (VRE) and Enterobacteriaceae members that generate extended-spectrum beta-lactamase (ESBL) (Lebreton et al., 2013). Because of their activity against clinically important strains and synergistic activity with other bacteriocins and antibiotics, enterocins could be used in the treatment of nosocomial infections caused by these resistant bacteria (Hammami et al., 2013).
Treatment of Methicillin-Resistant Staphylococcus Aureus (MRSA)
Methicillin-resistant S. aureus (MRSA) is usually resistant to oxacillin, cloxacillin, and other semi-synthetic antibiotics related to penicillin. They may also be resistant to tetracycline, clindamycin, cephalosporins, macrolides, quinolones and other antibiotics. In community, most MRSA infections are skin and soft tissue infections. In medical establishment, MRSA causes bacteraemia, septicemia, endocarditis, pneumonia and surgical site infections (Lee et al., 2016). The efficacy of vancomycin, the traditional antibiotic chosen to treat these infections, has declined with the emergence of resistant strains (Fair and Tor, 2014). Alternatively, bacteriocins E50-52 and B602 have been proven effective against antibiotic-resistant strains in nosocomial infections (Svetoch et al., 2009). Additionally, enterocins DD28 and DD93 were identified as anti-MRSA agents (Al Atya et al., 2016). Similarly, durancin 61A alone or in combination with vancomycin was shown effective against clinical MRSA (Hanchi et al., 2017), which may therefore provide a possible therapeutic option for the treatment of MRSA infections.
Treatment of Vancomycin-Resistant Enterococci (VRE)
The spread of VRE may lead to clinical isolates resistant to all antibiotics because enterococci have become important nosocomial pathogens and a reservoir for resistance genes (Fair and Tor, 2014). Interestingly, two peptides produced by E. faecium DSH20 (35 kDa) and E. faecalis 478 have shown potent activities against VRE (Shokri et al., 2014; Phumisantiphong et al., 2017) which may provide an alternative therapy for drug-resistant strains. Similarly, durancin 61A was shown effective against VRE clinical isolates (Hanchi et al., 2017).
Anti-Inflammatory Activity
E. durans M4-5 was found to produce butyrate, a metabolic product that induces significant anti-inflammatory effects and contributes to intestine epithelial integrity. This novel anti-inflammatory bacterium may be preferentially useful as a prophylactic treatment to avoid inflammatory bowel disease (Avram-Hananel et al., 2010). More recently, administration of E. durans EP1 was found to increase the amount of Faecalibacterium prausnitzii, a butyrate-producing bacteria, which is known for its anti-inflammatory effects (Carasi et al., 2017).
Conclusions and Perspectives
A growing number of studies have illustrated the beneficial effects of probiotics on health including the elimination of pathogens. Until recently, the most commonly used probiotic strains are related to Bifidobacterium, Lactobacillus, and Lactococcus species. In order to identify new members, other microorganisms with probiotic potential should be evaluated for probiotic candidacy. Among these candidates, Enterococcus spp. are prominent. However, for safety reasons, the application of enterococci as probiotic or feed additive has not been sufficiently exploited despite their significant antibacterial activity and probiotic potential (Franz et al., 2011). This genus has bad reputation due to members associated with severe health-care associated infections such as vancomycin-resistant enterococci (VRE). There is a major concern about use of enterococci strains in food supplements, which could lead to the spread of multi-resistance and virulence genes (Jahan et al., 2015). In an even more alarming development, transfer of vancomycin resistance from enterococci to methicillin-resistant strains of S. aureus has been reported in more than one study (de Niederhausern et al., 2011). Thus, strains carrying acquired resistance should not be intentionally introduced to the food and feed chain. In the case of feed and novel foods, a pre-market safety assessment is required where the safety of the candidate strains is assessed at species-level. In all cases, EFSA guidance documents on strain safety should be followed (EFSA, 2012a). Recent advances in molecular biology have demonstrated that enterococcal food strains are safe and can be differentiated from the nosocomial strains carrying virulence and antimicrobial resistance genes (Montealegre et al., 2016). This will enable possible improvement of the safety assessment of enterococci used in food and feed.
The genus Enterococcus is a member of LABs and constitutes a part of human-associated microbiota including in mouth, skin, and GIT. Some Enterococcus strains have many interesting properties such as multi-bacteriocin production and viability in different matrices including food and GIT, which highlight their potential use as natural preservatives in food, as probiotics, or as viable alternatives to antibiotics. In addition, enterococci bacteriocins are recognized for their wide spectrum antimicrobial activity including Gram-positive foodborne pathogens, such as biogenic amines producing bacteria (Laukova et al., 2017), L. monocytogenes and Gram-negative bacteria. Moreover, some bacteriocins possess antifungal and/or antiviral activity and can also inhibit sporulating bacteria such as C. botulinum and B. cereus and in some case they may inhibit endospores (Grande Burgos et al., 2014). These features provide the rationale to nominate bacteriocinogenic Enterococcus strains as important candidates for food, human and animal health applications.
Furthermore, bacteriocin production is an important element in competition among bacteria. Bacteriocin-producing probiotics could compete with intestinal pathogens for colonization or modulate the microbiota homeostasis. In this context, it has been reported that bacteriocins can be produced in the gut by probiotic bacteria where it can modulate gut microbiota to reduce gastrointestinal diseases (Salvucci et al., 2012; Cotter et al., 2013). However, the mechanisms by which probiotics attenuate gastrointestinal infections need to be evaluated in order to determine their efficacy more accurately. Bacteriocins are target specific, safe, can synergize with antibiotics, and are heat stable, interesting features for the development of drug candidates to the treatment of antibiotic-resistant bacterial infections. Considering the emergence of resistance, it is believed that multiple bacteriocin productions can help the producer strain to abolish resistance problem of some target strains (Perez et al., 2012). The use of bacteriocins in combination with other antimicrobials (Hanchi et al., 2017), or developing new products via peptide engineering is also a therapeutic option that is increasingly efficacious as resistance spreads (Cotter et al., 2013). In general, the mainstream use of bacteriocin therapies will need careful and controlled implementation to limit possible resistance development.
Finally, probiotics have been defined as live microorganisms which, when administered in adequate amounts, confer a health benefit to the host. This definition suggests that safety and efficacy of probiotics have to be demonstrated for each strain and each product. As probiotic properties have been shown to be strain specific, accurate identification of candidate strains is also very important. In spite of their pathogenic potential, commensal enterococci generally display low levels of virulence, as evidenced by their presence as natural colonizers of the GIT of humans and most animals and by the fact that they have been used safely for decades as probiotics in humans and farm animals (Arias and Murray, 2012). Therefore, their application as promising probiotics in food and feed industry needs implementation of appropriate guidance and relevant legislation of valid scientific methods to distinguish between pathogenic and non-pathogenic stains, and to prevent horizontal transfer of pathogenic genes. Despite the several hurdles that must be overcome for the exploitation of enterococci and their bacteriocins in food systems, as probiotics and in drug discovery, the innovations and developments discussed in this review offer a taste of future trends in food, veterinary and pharmaceutical applications of these intriguing microbes.
Author Contributions
HH and RH designed the manuscript. HH wrote the manuscript. WM, KS, and RH critically evaluated the manuscript.
Conflict of Interest Statement
The authors declare that the research was conducted in the absence of any commercial or financial relationships that could be construed as a potential conflict of interest.
References
ACNFP (1996). “Report on Enterococcus faecium, strain K77D”. Report, Ergon House c/o Nobel House, 17 Smith Square, London SW1 3JR, United Kingdom: MAFF Advisory Committee on Novel Foods and Processes.
Abengózar, M. A., Cebrian, R., Saugar, J. M., Garate, T., Valdivia, E., Martinez-Bueno, M., et al. (2017). Enterocin AS-48 as evidence for the use of bacteriocins as new leishmanicidal agents. Antimicrob. Agents Chemother. 61:e02288-16. doi: 10.1128/AAC.02288-16
Acuña, L., Picariello, G., Sesma, F., Morero, R., and Bellomio, A. (2012). A new hybrid bacteriocin, Ent35-MccV, displays antimicrobial activity against pathogenic Gram-positive and Gram-negative bacteria. FEBS Open Bio. 31, 12–19. doi: 10.1016/j.fob.2012.01.002
Al Atya, A. K., Belguesmia, Y., Chataigne, G., Ravallec, R., Vachée, A., Szunerits, S., et al. (2016). Anti-MRSA activities of Enterocins DD28 and DD93 and evidences on their role in the inhibition of biofilm formation. Front. Microbiol. 7:817. doi: 10.3389/fmicb.2016.00817
Anadon, A., Martinez-Larranaga, M. R., and Aranzazu Martinez, M. (2006). Probiotics for animal nutrition in the European Union. regulation and safety assessment. Regul. Toxicol. Pharmacol. 45, 91–95. doi: 10.1016/j.yrtph.2006.02.004
Ananou, S., Garriga, M., Jofre, A., Aymerich, T., Galvez, A., Maqueda, M., et al. (2010). Combined effect of enterocin AS-48 and high hydrostatic pressure to control food-borne pathogens inoculated in low acid fermented sausages. Meat Sci. 84, 594–600. doi: 10.1016/j.meatsci.2009.10.017
Ananou, S., Zentar, H., Martínez-Bueno, M., Gálvez, A., Maqueda, M., and Valdivia, E. (2014). The impact of enterocin AS-48 on the shelf-life and safety of sardines (Sardina pilchardus) under different storage conditions. Food Microbiol. 44, 185–195. doi: 10.1016/j.fm.2014.06.008
Arias, C. A., and Murray, B. E. (2012). The rise of the Enterococcus: beyond vancomycin resistance. Nat. Rev. Microbiol. 10, 266–278. doi: 10.1038/nrmicro2761
Arqués, J. L., Rodriguez, E., Langa, S., Landete, J. M., and Medina, M. (2015). Antimicrobial activity of lactic acid bacteria in dairy products and gut: effect on pathogens. Biomed. Res. Int. 2015:584183. doi: 10.1155/2015/584183
Avram-Hananel, L., Stock, J., Parlesak, A., Bode, C., and Schwartz, B. (2010). E durans strain M4-5 isolated from human colonic flora attenuates intestinal inflammation. Dis. Colon. Rectum. 53, 1676–1686. doi: 10.1007/DCR.0b013e3181f4b148
Aymerich, T., Artigas, M. G., Garriga, M., Monfort, J. M., and Hugas, M. (2000). Effect of sausage ingredients and additives on the production of enterocin A and B by Enterococcus faecium CTC492. optimization of in vitro production and anti-listerial effect in dry fermented sausages. J. Appl. Microbiol. 88, 686–694. doi: 10.1046/j.1365-2672.2000.01012.x
Barbosa, A. A. T., Mantovani, H. C., and Jain, S. (2017). Bacteriocins from lactic acid bacteria and their potential in the preservation of fruit products. Critic. Rev. Biotechnol. 37, 852–864. doi: 10.1080/07388551.2016.1262323
Barbosa, J., Borges, S., and Teixeira, P. (2014). Selection of potential probiotic Enterococcus faecium isolated from Portuguese fermented food. Int. J. Food Microbiol. 191, 144–148. doi: 10.1016/j.ijfoodmicro.2014.09.009
Bargossi, E., Tabanelli, G., Montanari, C., Gatto, V., Chinnici, F., Gardini, F., et al. (2017). Growth, biogenic amine production and tyrDC transcription of Enterococcus faecalis in synthetic medium containing defined amino acid concentrations. J. Appl. Microbiol. 122, 1078–1091. doi: 10.1111/jam.13406
Basanta, A., Sánchez, J., Gómez-Sala, B., Herranz, C., Hernández, P., and Cintas, L. (2008). Antimicrobial activity of Enterococcus faecium L50, a strain producing enterocins L50 (L50A and L50B), P and Q, against beer-spoilage lactic acid bacteria in broth, wort (hopped and unhopped), and alcoholic and non-alcoholic lager beers. Int. J. Food Microbiol. 125, 293–307. doi: 10.1016/j.ijfoodmicro.2008.04.011
Batdorj, B., Dalgalarrondo, M., Choiset, Y., Pedroche, J., Metro, F., Prevost, H., et al. (2006). Purification and characterization of two bacteriocins produced by lactic acid bacteria isolated from Mongolian airag. J. Appl. Microbiol. 101, 837–848. doi: 10.1111/j.1365-2672.2006.02966.x
Bednorz, C., Guenther, S., Oelgeschläger, K., Kinnemann, B., Pieper, R., Hartmann, S., et al. (2013). Feeding the probiotic Enterococcus faecium strain NCIMB 10415 to piglets specifically reduces the number of Escherichia coli pathotypes that adhere to the gut mucosa. Appl. Environ. Microbiol. 79, 7896–7904. doi: 10.1128/AEM.03138-13
Behrens, H. M., Six, A., Walker, D., and Kleanthous, C. (2017). The therapeutic potential of bacteriocins as protein antibiotics. Emerg. Topics Life Sci. 1, 65–74. doi: 10.1042/ETLS20160016
Belguesmia, Y., Choiset, Y., Rabesona, H., Baudy-Floc'h, M., Le Blay, G., Haertlé, T., et al. (2013). Antifungal properties of durancins isolated from Enterococcus durans A5-11 and of its synthetic fragments. Lett. Appl. Microbiol. 56, 237–244. doi: 10.1111/lam.12037
Beukers, A. G., Zaheer, R., Goji, N., Amoako, K. K., Chaves, A. V., Ward, M. P., et al. (2017). Comparative genomics of Enterococcus spp. isolated from bovine feces. BMC Microbiol. 17:52. doi: 10.1186/s12866-017-0962-1
Bhardwaj, A., Malik, R. K., and Chauhan, P. (2008). Functional and safety aspects of enterococci in dairy foods. Ind. J. Microbiol. 48, 317–325. doi: 10.1007/s12088-008-0041-2
Bogut, I., Milaković, Z., Brkić, S., Novoselić, D., and Bukvić, Ž. (2000). Effects of Enterococcus faecium on the growth rate and content of intestinal microflora in sheat fish (Silurus glanis). Vet. Med.45, 107–109.
Bonacina, J., Suarez, N., Hormigo, R., Fadda, S., Lechner, M., and Saavedra, L. (2017). A genomic view of food-related and probiotic Enterococcus strains. DNA Res. 24, 11–24. doi: 10.1093/dnares/dsw043
Brodmann, T., Endo, A., Gueimonde, M., Vinderola, G., Kneifel, W., de Vos, W. M., et al. (2017). Safety of novel microbes for human consumption: practical examples of assessment in the European Union. Front. Microbiol. 8:1725. doi: 10.3389/fmicb.2017.01725
Bybee, S., Scorza, A., and Lappin, M. (2011). Effect of the probiotic Enterococcus faecium SF68 on presence of diarrhea in cats and dogs housed in an animal shelter. J. Vet. Intern. Med. 25, 856–860. doi: 10.1111/j.1939-1676.2011.0738.x
Callewaert, R., Hugas, M., and Vuyst, L. D. (2000). Competitiveness and bacteriocin production of Enterococci in the production of Spanish-style dry fermented sausages. Int. J. Food Microbiol. 57, 33–42. doi: 10.1016/S0168-1605(00)00228-2
Caly, D. L., Chevalier, M., Flahaut, C., Cudennec, B., Al Atya, A. K., Chataigne, G., et al. (2017). The safe enterocin DD14 is a leaderless two-peptide bacteriocin with anti-Clostridium perfringens activity. Int. J. Antimicrob. Agents 49, 282–289. doi: 10.1016/j.ijantimicag.2016.11.016
Cao, G. T., Zeng, X. F., Chen, A. G., Zhou, L., Zhang, L., Xiao, Y. P., et al. (2013). Effects of a probiotic, Enterococcus faecium, on growth performance, intestinal morphology, immune response, and cecal microflora in broiler chickens challenged with Escherichia coli K88. Poult. Sci. 92, 2949–2955. doi: 10.3382/ps.2013-03366
Carasi, P., Racedo, S., Jacquot, C., Elie, A., Serradell, M., and Urdaci, M. (2017). Enterococcus durans EP1 a promising anti-inflammatory probiotic able to stimulate sIgA and to increase Faecalibacterium prausnitzii abundance. Front. Immunol. 8:88. doi: 10.3389/fimmu.2017.00088
Casaus, P., Nilsen, T., Cintas, L. M., Nes, I. F., Hernandez, P. E., and Holo, H. (1997). Enterocin B, a new bacteriocin from Enterococcus faecium T136 which can act synergistically with enterocin A. Microbiology 143(Pt 7), 2287–2294. doi: 10.1099/00221287-143-7-2287
Castellano, P., Perez Ibarreche, M., Blanco Massani, M., Fontana, C., and Vignolo, G. M. (2017). Strategies for pathogen biocontrol using lactic acid bacteria and their metabolites: a focus on meat ecosystems and industrial environments. Microorganisms 5, E38. doi: 10.3390/microorganisms5030038
Chang, C. I., and Liu, W. Y. (2002). An evaluation of two probiotic bacterial strains, Enterococcus faecium SF68 and Bacillus toyoi, for reducing edwardsiellosis in cultured European eel, Anguilla anguilla L. J. Fish Dis. 25, 311–315. doi: 10.1046/j.1365-2761.2002.00365.x
Choi, H. J., Shin, M. S., Lee, S. M., and Lee, W. K. (2012). Immunomodulatory properties of Enterococcus faecium JWS 833 isolated from duck intestinal tract and suppression of Listeria monocytogenes infection. Microbiol. Immunol. 56, 613–620. doi: 10.1111/j.1348-0421.2012.00486.x
Christopher, R. C., Phillip, S. C., and Michael, S. G. (2005). Enterococcal cytolysin: a novel two component peptide system that serves as a bacterial defense against eukaryotic and prokaryotic cells. Curr. Protein Peptide Sci. 6, 77–84. doi: 10.2174/1389203053027557
Cintas, L. M., Casaus, P., Håvarstein, L. S., Hernández, P. E., and Nes, I. F. (1997). Biochemical and genetic characterization of enterocin P, a novel sec-dependent bacteriocin from Enterococcus faecium P13 with a broad antimicrobial spectrum. Appl. Environ. Microbiol. 63, 4321–4330.
Cintas, L. M., Casaus, P., Herranz, C., Håvarstein, L. S., Holo, H., Hernández, P. E., et al. (2000). Biochemical and genetic evidence that Enterococcus faecium L50 produces enterocins L50a and L50B, the sec-dependent enterocin P, and a novel bacteriocin secreted without an N-terminal extension termed enterocin Q. J. Bacteriol. 182, 6806–6814. doi: 10.1128/JB.182.23.6806-6814.2000
Cobo Molinos, A., Abriouel, H., Lucas López, R., Ben Omar, N., Valdivia, E., and Gálvez, A. (2008). Inhibition of Bacillus cereus and Bacillus weihenstephanensis in raw vegetables by application of washing solutions containing enterocin AS-48 alone and in combination with other antimicrobials. Food Microbiol. 25, 762–770. doi: 10.1016/j.fm.2008.05.001
Corsetti, A., Perpetuini, G., Schirone, M., Tofalo, R., and Suzzi, G. (2012). Application of starter cultures to table olive fermentation: an overview on the experimental studies. Front. Microbiol. 3:248. doi: 10.3389/fmicb.2012.00248
Cotter, P. D., Hill, C., and Paul, R. R. (2005). Bacteriocins: developing innate immunity for food. Nat. Rev. Microbiol. 3, 777–788. doi: 10.1038/nrmicro1273
Cotter, P. D., Ross, R. P., and Hill, C. (2013). Bacteriocins-a viable alternative to antibiotics? Nat. Rev. Microbiol. 11, 95–105. doi: 10.1038/nrmicro2937
De Bellis, P., Valerio, F., Sisto, A., Lonigro, S. L., and Lavermicocca, P. (2010). Probiotic table olives: microbial populations adhering on olive surface in fermentation sets inoculated with the probiotic strain Lactobacillus paracasei IMPC2.1 in an industrial plant. Int. J. Food Microbiol. 140, 6–13. doi: 10.1016/j.ijfoodmicro.2010.02.024
de Castro, A., Montaño, A., Casado, F. J., Sánchez, A. H., and Rejano, L. (2002). Utilization of Enterococcus casseliflavus and Lactobacillus pentosus as starter cultures for Spanish-style green olive fermentation. Food Microbiol. 19, 637–644. doi: 10.1006/fmic.2002.0466
De Kwaadsteniet, M., Todorov, S., Knoetze, H., and Dicks, L. (2005). Characterization of a 3944 Da bacteriocin, produced by Enterococcus mundtii ST15, with activity against Gram-positive and Gram-negative bacteria. Int. J. Food Microbiol. 105, 433–444. doi: 10.1016/j.ijfoodmicro.2005.03.021
de Niederhausern, S., Bondi, M., Messi, P., Iseppi, R., Sabia, C., Manicardi, G., et al. (2011). Vancomycin-resistance transferability from VanA enterococci to Staphylococcus aureus. Curr. Microbiol. 62, 1363–1367. doi: 10.1007/s00284-011-9868-6
Dicks, L., Granger, M., and van Reenen, C. (2010). Survival and adherence of antimicrobial peptide ST4SA, produced by Enterococcus mundtii, at conditions found in the human gastro-intestinal tract. J. Basic Microbiol. (Suppl. 1), S25–29. doi: 10.1002/jobm.201000249
Dobson, A., Cotter, P. D., Ross, R. P., and Hill, C. (2012). Bacteriocin production: a probiotic trait? Appl. Environ. Microbiol. 78, 1–6. doi: 10.1128/AEM.05576-11
Du, L., Liu, F., Zhao, P., Zhao, T., and Doyle, M. P. (2017). Characterization of Enterococcus durans 152 bacteriocins and their inhibition of Listeria monocytogenes in ham. Food Microbiol. 68, 97–103. doi: 10.1016/j.fm.2017.07.002
Du, L., Somkuti, G. A. Jr, J. A. R., and Huo, G. (2012). Properties of durancin GL, a new antilisterial bacteriocin produced by Enterococcus durans 41D. J. Food Safety 32, 74–83. doi: 10.1111/j.1745-4565.2011.00346.x
Eaton, T. J., and Gasson, M. J. (2001). Molecular screening of Enterococcus virulence determinants and potential for genetic exchange between food and medical isolates. Appl. Environ. Microbiol. 67, 1628–1635. doi: 10.1128/AEM.67.4.1628-1635.2001
EC (2000). Directive 2000/54/ECof the European Parliament and of the council of 18 September 2000 on the protection of workers from risks related to exposure to biological agents at work. Official J. Eur. Union L 262, 21–45.
EFSA Panel on Biological Hazards, Ricci, A., Allende, A., Bolton, D., Chemaly, M., et al. (2017). Scientific Opinion on the update of the list of QPS-recommended biological agents intentionally added to food or feed as notified to EFSA. EFSA J. 15:e04664. doi: 10.2903/j.efsa.2017.4664
EFSA Panel on Biological Hazards (2011). Scientific opinion on risk based control of biogenic amine formation in fermented foods. EFSA J. 9:2393. doi: 10.2903/j.efsa.2011.2393
EFSA (2012a). Guidance for assessing safety of Enterococcus faecium in animal feed. EFSAJ. 10:2682. doi: 10.2903/j.efsa.2012.2682
EFSA (2012b). Scientific Opinion on Lactiferm® (Enterococcus faecium) as a feed additive for weaned piglets and calves. EFSAJ. 10:2574. doi: 10.2903/j.efsa.2012.2574
Egan, K., Field, D., Rea, M. C., Ross, R. P., Hill, C., and Cotter, P. D. (2016). Bacteriocins: novel solutions to age old spore-related problems? Front. Microbiol. 7:461. doi: 10.3389/fmicb.2016.00461
Fair, R. J., and Tor, Y. (2014). Antibiotics and bacterial resistance in the 21st century. Perspect. Med. Chem. 6, 25–64. doi: 10.4137/P. M. C. S.14459
FAO/WHO (2002). Report of a Joint FAO/WHOWorking Group on Drafting Guidelines for the Evaluation of Probiotics in Food. London; Ontario, ON: FAO/WHO.
Farias, M. E., de Kairuz, M. N., Sesma, F., Palacios, J., Holgado, A. P. D., and Oliver, G. (1999). Inhibition of Listeria monocytogenes by the bacteriocin enterocin CRL35 during goat cheese making. Milchwissensch. Milk Sci. Int. 54, 30–32.
Faron, M. L., Ledeboer, N. A., and Buchan, B. W. (2016). Resistance mechanisms, epidemiology, and approaches to screening for vancomycin-resistant Enterococcus in the halth care setting. J. Clin. Microbiol. 54, 2436–2447. doi: 10.1128/JCM.00211-16
FDA (2011). “Scombrotoxin (Histamine) Formation,” in Fish and Fishery Products Hazards and Controls Guidance, 4th Edn. Office Seafood Washington, DC: Department of Health and Human Services, Publich Health Service, Food and Drug Administration, Center for Food Safety and Applied Nutrition, 113–152.
Fisher, K., and Phillips, C. (2009). The ecology, epidemiology and virulence of Enterococcus. Microbiology 155, 1749–1757. doi: 10.1099/mic.0.026385-0
Fliss, I., Hammami, R., and Le Lay, C. (2011). “Biological control of human digestive microbiota using antimicrobial cultures and bacteriocins A2 - Lacroix, Christophe,” in Protective Cultures, Antimicrobial Metabolites and Bacteriophages for Food and Beverage Biopreservation, ed C. Lacroix (Oxford: Woodhead Publishing), 240–263.
Foulquié Moreno, M., Sarantinopoulos, P., Tsakalidou, E., and De Vuyst, L. (2006). The role and application of enterococci in food and health. Int J Food Microbiol. 106, 1–24. doi: 10.1016/j.ijfoodmicro.2005.06.026
Franz, C. M. A. P., Huch, M., Abriouel, H., Holzapfel, W., and Gálvez, A. (2011). Enterococci as probiotics and their implications in food safety. Int. J. Food Microbiol. 151, 125–140. doi: 10.1016/j.ijfoodmicro.2011.08.014
Franz, C. M., van Belkum, M. J., Holzapfel, W. H., Abriouel, H., and Galvez, A. (2007). Diversity of enterococcal bacteriocins and their grouping in a new classification scheme. FEMS Microbiol. Rev. 31, 293–310. doi: 10.1111/j.1574-6976.2007.00064.x
García, M. T., Lucas, R., Abriouel, H., Omar, N. B., Pérez, R., Grande, M. J., et al. (2004). Antimicrobial activity of enterocin EJ97 against ‘Bacillus macroides/Bacillus maroccanus' isolated from zucchini purée. J. Appl. Microbiol. 97, 731–737. doi: 10.1111/j.1365-2672.2004.02351.x
Gardini, F., Bover-Cid, S., Tofalo, R., Belletti, N., Gatto, V., Suzzi, G., et al. (2008). Modeling the aminogenic potential of Enterococcus faecalis EF37 in dry fermented sausages through chemical and molecular approaches. Appl. Environ. Microbiol. 74, 2740–2750. doi: 10.1128/AEM.02267-07
Gardini, F., Ozogul, Y., Suzzi, G., Tabanelli, G., and Ozogul, F. (2016). Technological factors affecting biogenic amine content in foods: a review. Front. Microbiol. 7:1218. doi: 10.3389/fmicb.2016.01218
Garriga, M., and Aymerich, T. (2014). “The microbiology of fermentation and ripening,” in Handbook of Fermented Meat and Poultry, eds F. Toldrá, Y. H. Hui, I. Astiasarán, J. G. Sebranek, R. Talon (Chichester: John Wiley & Sons, Ltd), 107–115.
Giraffa, G. (2002). Enterococci from foods. FEMS Microbiol. Rev. 26, 163–171. doi: 10.1111/j.1574-6976.2002.tb00608.x
Grande Burgos, M. J., Pulido, R. P., Del Carmen Lopez Aguayo, M., Galvez, A., and Lucas, R. (2014). The cyclic antibacterial peptide enterocin AS-48: isolation, mode of action, and possible food applications. Int. J. Mol. Sci. 15, 22706–22727. doi: 10.3390/ijms151222706
Guarcello, R., De Angelis, M., Settanni, L., Formiglio, S., Gaglio, R., Minervini, F., et al. (2016). Selection of amine-oxidizing dairy lactic acid bacteria and identification of the enzyme and gene involved in the decrease of biogenic amines. Appl. Environ. Microbiol. 82, 6870–6880. doi: 10.1128/AEM.01051-16
Gupta, A., and Tiwari, S. K. (2015). Probiotic potential of bacteriocin-producing Enterococcus hirae strain LD3 isolated from dosa batter. Ann. Microbiol. 65, 2333–2342. doi: 10.1007/s13213-015-1075-4
Hammami, R., Fernandez, B., Lacroix, C., and Fliss, I. (2013). Anti-infective properties of bacteriocins: an update. Cell. Mol. Life Sci. 70, 2947–2967. doi: 10.1007/s00018-012-1202-3
Hammami, R., Zouhir, A., Le Lay, C., Ben Hamida, J., and Fliss, I. (2010). BACTIBASE second release: a database and tool platform for bacteriocin characterization. BMC Microbiol. 10:22. doi: 10.1186/1471-2180-10-22
Hanchi, H., Hammami, R., Fernandez, B., Kourda, R., Ben Hamida, J., and Fliss, I. (2016). Simultaneous production of formylated and nonformylated enterocins L50A and L50B as well as 61A, a new glycosylated Durancin, by Enterococcus durans 61A, a strain isolated from artisanal fermented milk in Tunisia. J. Agric. Food Chem. 64, 3584–3590. doi: 10.1021/acs.jafc.6b00700
Hanchi, H., Hammami, R., Gingras, H., Kourda, R., Bergeron, M., Ben Hamida, J., et al. (2017). Inhibition of MRSA and of Clostridium difficile by durancin 61A: synergy with bacteriocins and antibiotics. Fut. Microbiol. 12, 205–212. doi: 10.2217/fmb-2016-0113
Hanchi, H., Hammami, R., Kourda, R., Hamida, J. B., and Fliss, I. (2014). Bacteriocinogenic properties and in vitro probiotic potential of enterococci from Tunisian dairy products. Arch. Microbiol. 196, 331–344. doi: 10.1007/s00203-014-0978-y
Hendrickx, A. P., van Wamel, W. J., Posthuma, G., Bonten, M. J., and Willems, R. J. (2007). Five genes encoding surface-exposed LPXTG proteins are enriched in hospital-adapted Enterococcus faecium clonal complex 17 isolates. J. Bacteriol. 189, 8321–8332. doi: 10.1128/JB.00664-07
Henning, C., Gautam, D., and Muriana, P. (2015). Identification of multiple bacteriocins in Enterococcus spp. using an Enterococcus-specific bacteriocin PCR array. Microorganisms 3, 1–16. doi: 10.3390/microorganisms3010001
Himeno, K., Rosengren, K. J., Inoue, T., Perez, R. H., Colgrave, M. L., Lee, H. S., et al. (2015). Identification, characterization, and three-dimensional structure of the novel circular bacteriocin, Enterocin NKR-5-3B, from Enterococcus faecium. Biochemistry 54, 4863–4876. doi: 10.1021/acs.biochem.5b00196
Hlivak, P., Odraska, J., Ferencik, M., Ebringer, L., Jahnova, E., and Mikes, Z. (2005). One-year application of probiotic strain Enterococcus faecium M-74 decreases serum cholesterol levels. Bratisl Lek Listy 106, 67–72.
Hu, C. B., Zendo, T., Nakayama, J., and Sonomoto, K. (2008). Description of durancin TW-49M, a novel enterocin B-homologous bacteriocin in carrot-isolated Enterococcus durans QU 49. J. Appl. Microbiol. 105, 681–690. doi: 10.1111/j.1365-2672.2008.03798.x
Huang, E., Zhang, L., Chung, Y., Zheng, Z., and Yousef, A. (2013). Characterization and application of enterocin RM6, a bacteriocin from Enterococcus faecalis. Biomed. Res. Int. 2013:206917. doi: 10.1155/2013/206917
Hugas, M., Garriga, M., and Aymerich, M. (2003). Functionality of enterococci in meat products. Int. J. Food Microbiol. 88, 223–233. doi: 10.1016/S0168-1605(03)00184-3
Hungerford, J. M., Hollingworth, T. A., and Wekell, M. M. (2001). Automated kinetics-enhanced flow-injection method for histamine in regulatory laboratories: rapid screening and suitability requirements. Anal. Chim. Acta 438, 123–129. doi: 10.1016/S0003-2670(01)00985-0
Huys, G., Botteldoorn, N., Delvigne, F., De Vuyst, L., Heyndrickx, M., Pot, B., et al. (2013). Microbial characterization of probiotics–advisory report of the working group “8651 probiotics” of the Belgian superior health council (SHC). Mol. Nutr. Food Res. 57, 1479–1504. doi: 10.1002/mnfr.201300065
Iseppi, R., Pilati, F., Marini, M., Toselli, M., de Niederhäusern, S., Guerrieri, E., et al. (2008). Anti-listerial activity of a polymeric film coated with hybrid coatings doped with Enterocin 416K1 for use as bioactive food packaging. Int. J. Food Microbiol. 123, 281–287. doi: 10.1016/j.ijfoodmicro.2007.12.015
Ishibashi, N., Himeno, K., Fujita, K., Masuda, Y., Perez, R., Zendo, T., et al. (2012). Purification and characterization of multiple bacteriocins and an inducing peptide produced by Enterococcus faecium NKR-5-3 from Thai fermented fish. Biosci. Biotechnol. Biochem. 76, 947–953. doi: 10.1271/bbb.110972
Izquierdo, E., Marchioni, E., Aoude-Werner, D., Hasselmann, C., and Ennahar, S. (2009). Smearing of soft cheese with Enterococcus faecium WHE 81, a multi-bacteriocin producer, against Listeria monocytogenes. Food Microbiol. 26, 16–20. doi: 10.1016/j.fm.2008.08.002
Jahan, M., Zhanel, G. G., Sparling, R., and Holley, R. A. (2015). Horizontal transfer of antibiotic resistance from Enterococcus faecium of fermented meat origin to clinical isolates of E. faecium and Enterococcus faecalis. Int. J. Food Microbiol. 199, 78–85. doi: 10.1016/j.ijfoodmicro.2015.01.013
Javed, A., Masud, T., ul Ain, Q., Imran, M., and Maqsood, S. (2011). Enterocins of Enterococcus faecium, emerging natural food preservatives. Ann. Microbiol. 61, 699–708. doi: 10.1007/s13213-011-0223-8
Ščerbová, J., and Lauková, A. (2016). Sensitivity to enterocins of thermophilic Campylobacter spp. from different poultry species. Foodborne Pathog. Dis. 13, 668–673. doi: 10.1089/fpd.2016.2158
Joosten, H., and Nunez, M. (1996). Prevention of histamine formation in cheese by bacteriocin-producing lactic Acid bacteria. Appl. Environ. Microbiol. 62, 1178–1181.
Jung, A., Metzner, M., and Ryll, M. (2017). Comparison of pathogenic and non-pathogenic Enterococcus cecorum strains from different animal species. BMC Microbiology 17:33. doi: 10.1186/s12866-017-0949-y
Kanda, T., Nishida, A., Ohno, M., Imaeda, H., Shimada, T., Inatomi, O., et al. (2016). Enterococcus durans TN-3 induces regulatory T cells and suppresses the development of dextran sulfate sodium (DSS)-induced experimental colitis. PLoS ONE 11:e0159705. doi: 10.1371/journal.pone.0159705
Kang, B., Seo, J., Lee, G., Kim, J., Kim, S., Han, Y., et al. (2009). Antimicrobial activity of enterocins from Enterococcus faecalis SL-5 against Propionibacterium acnes, the causative agent in acne vulgaris, and its therapeutic effect. J. Microbiol. 47, 101–109. doi: 10.1007/s12275-008-0179-y
Kawamoto, S., Shima, J., Sato, R., Eguchi, T., Ohmomo, S., Shibato, J., et al. (2002). Biochemical and genetic characterization of mundticin KS, an antilisterial peptide produced by Enterococcus mundtii NFRI 7393. Appl. Environ. Microbiol. 68, 3830–3840. doi: 10.1128/AEM.68.8.3830-3840.2002
Khan, H., Flint, S., and Yu, P.-L. (2010). Enterocins in food preservation. Int. J. Food Microbiol. 141, 1–10. doi: 10.1016/j.ijfoodmicro.2010.03.005
Khay, E. O., Idaomar, M., El Moussaoui, N., and Abrini, J. (2014). Application of a bacteriocin-like inhibitory substance producing Enterococcus durans E204 strain, isolated from camel milk, to control Listeria monocytogenes CECT 4032 in goat jben. Ann. Microbiol. 64, 313–319. doi: 10.1007/s13213-013-0666-1
Kinouchi, F., Maia, D., de Abreu Ribeiro, L., Placeres, M., de Valdez, G., Colombo, L., et al. (2012). A soy-based product fermented by Enterococcus faecium and Lactobacillus helveticus inhibits the development of murine breast adenocarcinoma. Food Chem. Toxicol. 50, 4144–4148. doi: 10.1016/j.fct.2012.08.038
Kommineni, S., Bretl, D. J., Lam, V., Chakraborty, R., Hayward, M., Simpson, P., et al. (2015). Bacteriocin production augments niche competition by enterococci in the mammalian gastrointestinal tract. Nature 526, 719–722. doi: 10.1038/nature15524
Kumar, M., and Srivastava, S. (2010). Antilisterial activity of a broad-spectrum bacteriocin, enterocin LR/6 from Enterococcus faecium LR/6. Appl. Biochem. Biotechnol. 162, 698–706. doi: 10.1007/s12010-009-8851-1
Ladero, V., Fernández, M., Calles-Enríquez, M., Sánchez-Llana, E., Cañedo, E., Martín, M. C., et al. (2012). Is the production of the biogenic amines tyramine and putrescine a species-level trait in enterococci? Food Microbiol. 30, 132–138. doi: 10.1016/j.fm.2011.12.016
Landete, J. M., de Las Rivas, B., Marcobal, A., and Munoz, R. (2007). Molecular methods for the detection of biogenic amine-producing bacteria on foods. Int. J. Food Microbiol. 117, 258–269. doi: 10.1016/j.ijfoodmicro.2007.05.001
Latorre-Moratalla, M. L., Bover-Cid, S., Veciana-Nogues, M. T., and Vidal-Carou, M. C. (2012). Control of biogenic amines in fermented sausages: role of starter cultures. Front. Microbiol. 3:169. doi: 10.3389/fmicb.2012.00169
Lauková, A. (2011). “Potential applications of probiotic, bacteriocin-producing enterococci and their bacteriocins,” in Lactic Acid Bacteria Microbiological and Functional Aspects, 4th Edn, ed A. V. Wright (Boca Raton, FL: CRC Press), 39–61.
Lauková, A., and Czikková, S. (1999). The use of enterocin CCM 4231 in soy milk to control the growth of Listeria monocytogenes and Staphylococcus aureus. J. Appl. Microbiol. 87, 182–182. doi: 10.1046/j.1365-2672.1999.00810.x
Lauková, A., Guba, P., Nemcová, R., and Vasilková, Z. (2003). Reduction of Salmonella in gnotobiotic Japanese Quails caused by the Enterocin A-producing EK13 strain of Enterococcus faecium. Veterin. Res. Commun. 27, 275–280. doi: 10.1023/A:1024027923824
Laukova, A., Kandricakova, A., Bunkova, L., Pleva, P., and Scerbova, J. (2017). Sensitivity to enterocins of biogenic amine-producing faecal Enterococci from ostriches and pheasants. Probiotics Antimicrob. Proteins 9, 483–491. doi: 10.1007/s12602-017-9272-z
Laulund, S., Wind, A., Derkx, P., and Zuliani, V. (2017). Regulatory and safety requirements for food cultures. Microorganisms 5:28. doi: 10.3390/microorganisms5020028
Lebreton, F., van Schaik, W., McGuire, A. M., Godfrey, P., Griggs, A., Mazumdar, V., et al. (2013). Emergence of epidemic multidrug-resistant Enterococcus faecium from animal and commensal strains. MBio 4:e00534-13. doi: 10.1128/mBio.00534-13
Lee, A., Huttner, B., and Harbarth, S. (2016). Prevention and control of methicillin-resistant Staphylococcus aureus in acute care settings. Infect. Dis. Clin. North Am. 30, 931–952. doi: 10.1016/j.idc.2016.07.006
Leroy, F., Foulquié Moreno, M., and De Vuyst, L. (2002). Enterococcus faecium RZS C5, an interesting bacteriocin producer to be used as a co-culture in food fermentation. Int. J. Food Microbiol. 88, 235–240. doi: 10.1016/S0168-1605(03)00185-5
Liao, S. F., and Nyachoti, M. (2017). Using probiotics to improve swine gut health and nutrient utilization. Anim. Nutr. 3, 331–343. doi: 10.1016/j.aninu.2017.06.007
Lim, E.-S. (2016). Inhibitory effect of bacteriocin-producing lactic acid bacteria against histamine-forming bacteria isolated from Myeolchi-jeot. Fish. Aquat. Sci. 19:42. doi: 10.1186/s41240-016-0040-x
Line, J., Svetoch, E., Eruslanov, B., Perelygin, V., Mitsevich, E., Mitsevich, I., et al. (2008). Isolation and purification of enterocin E-760 with broad antimicrobial activity against gram-positive and gram-negative bacteria. Antimicrob. Agents Chemother. 52, 1094–1100. doi: 10.1128/AAC.01569-06
Liu, F., Li, B., Du, J., Yu, S., Li, W., Evivie, S., et al. (2016). Complete genome sequence of Enterococcus durans KLDS6.0930, a strain with probiotic properties. J. Biotechnol. 217 49–50. doi: 10.1016/j.jbiotec.2015.11.012
Liu, G., Griffiths, M. W., Wu, P., Wang, H., Zhang, X., and Li, P. (2011). Enterococcus faecium LM-2, a multi-bacteriocinogenic strain naturally occurring in “Byaslag”, a traditional cheese of Inner Mongolia in China. Food Control 22, 283–289. doi: 10.1016/j.foodcont.2010.07.023
Liu, G., Wang, Y., Gui, M., Zheng, H., Dai, R., and Li, P. (2012). Combined effect of high hydrostatic pressure and enterocin LM-2 on the refrigerated shelf life of ready-to-eat sliced vacuum-packed cooked ham. Food Control 24, 64–71. doi: 10.1016/j.foodcont.2011.09.004
Liu, X., Vederas, J. C., Whittal, R. M., Zheng, J., Stiles, M. E., Carlson, D., et al. (2011). Identification of an N-terminal formylated, two-peptide bacteriocin from Enterococcus faecalis 710C. J. Agric. Food Chem. 59, 5602–5608. doi: 10.1021/jf104751v
Lohans, C. T., and Vederas, J. C. (2012). Development of Class IIa bacteriocins as therapeutic agents. Int. J. Microbiol. 2012:386410. doi: 10.1155/2012/386410
Lucas, R., Grande, M. A., Abriouel, H., Maqueda, M., Ben Omar, N., Valdivia, E., et al. (2006). Application of the broad-spectrum bacteriocin enterocin AS-48 to inhibit Bacillus coagulans in canned fruit and vegetable foods. Food Chem. Toxicol. 44, 1774–1781. doi: 10.1016/j.fct.2006.05.019
Lucena-Padrós, H., González, J., Caballero-Guerrero, B., Ruiz-Barba, J., and Maldonado-Barragán, A. (2014). Enterococcus olivae sp. nov., isolated from Spanish-style green-olive fermentations. Int. J. Syst. Evol. Microbiol. 64, 2534–2549. doi: 10.1099/ijs.0.062208-0
Madoroba, E., Steenkamp, E., Theron, J., Scheirlinck, I., Cloete, T., and Huys, G. (2011). Diversity and dynamics of bacterial populations during spontaneous sorghum fermentations used to produce ting, a South African food. Syst. Appl. Microbiol. 34, 227–234. doi: 10.1016/j.syapm.2010.11.016
Maky, M. A., Ishibashi, N., Zendo, T., Perez, R. H., Doud, J. R., Karmi, M., et al. (2015). Enterocin F4-9, a novel O-linked glycosylated bacteriocin. Appl. Environ. Microbiol. 81, 4819–4826. doi: 10.1128/AEM.00940-15
Maldonado-Barragan, A., Caballero-Guerrero, B., Jimenez, E., Jimenez-Diaz, R., Ruiz-Barba, J. L., and Rodriguez, J. M. (2009). Enterocin C, a class IIb bacteriocin produced by E. faecalis C901, a strain isolated from human colostrum. Int. J. Food Microbiol. 133, 105–112. doi: 10.1016/j.ijfoodmicro.2009.05.008
Maqueda, M., Galvez, A., Bueno, M. M., Sanchez-Barrena, M. J., Gonzalez, C., Albert, A., et al. (2004). Peptide AS-48: prototype of a new class of cyclic bacteriocins. Curr. Protein Peptide Sci. 5, 399–416. doi: 10.2174/1389203043379567
Maqueda Abreu, M., Martinez Bueno, M., Valdivia Martinez, E. V. A., Ananou Jaled, S., and Cebrian Castillo, R. (2014). Composition for Treating Bacterial Infections of the Skin and Mucous Membranes. WO patent application ES 2013070461 W. 2014/01/09.
Marcos, B., Aymerich, T., Monfort, J. M., and Garriga, M. (2007). Use of antimicrobial biodegradable packaging to control Listeria monocytogenes during storage of cooked ham. Int. J. Food Microbiol. 120, 152–158. doi: 10.1016/j.ijfoodmicro.2007.06.003
Marekova, M., Laukova, A., Skaugen, M., and Nes, I. (2007). Isolation and characterization of a new bacteriocin, termed enterocin M, produced by environmental isolate Enterococcus faecium AL41. J. Ind. Microbiol. Biotechnol. 34, 533–537. doi: 10.1007/s10295-007-0226-4
Martinez Viedma, P., Sobrino Lopez, A., Ben Omar, N., Abriouel, H., Lucas Lopez, R., Valdivia, E., et al. (2008). Enhanced bactericidal effect of enterocin AS-48 in combination with high-intensity pulsed-electric field treatment against Salmonella enterica in apple juice. Int. J. Food Microbiol. 128, 244–249. doi: 10.1016/j.ijfoodmicro.2008.08.014
Molina, M. A., Diaz, A. M., Hesse, C., Ginter, W., Gentilini, M. V., Nunez, G. G., et al. (2015). Immunostimulatory effects triggered by Enterococcus faecalis CECT7121 probiotic strain involve activation of dendritic cells and interferon-gamma production. PLoS ONE 10:e0127262. doi: 10.1371/journal.pone.0127262
Montealegre, M. C., Singh, K. V., and Murray, B. E. (2016). Gastrointestinal tract colonization dynamics by different Enterococcus faecium clades. J. Infect. Dis. 213, 1914–1922. doi: 10.1093/infdis/jiv597
Müller, T., Ulrich, A., Ott, E., and Müller, M. (2001). Identification of plant-associated enterococci. J. Appl. Microbiol. 91, 268–278. doi: 10.1046/j.1365-2672.2001.01373.x
Naila, A., Flint, S., Fletcher, G., Bremer, P., and Meerdink, G. (2010). Control of biogenic amines in food–existing and emerging approaches. J. Food Sci. 75, R139–150. doi: 10.1111/j.1750-3841.2010.01774.x
Nakayama, J., Kariyama, R., and Kumon, H. (2002). Description of a 23.9-kilobase chromosomal deletion containing a region encoding fsr genes which mainly determines the gelatinase-negative phenotype of clinical isolates of Enterococcus faecalis in urine. Appl. Environ. Microbiol. 68, 3152–3155. doi: 10.1128/AEM.68.6.3152-3155.2002
Nami, Y., Abdullah, N., Haghshenas, B., Radiah, D., Rosli, R., and Khosroushahi, A. Y. (2014). Probiotic assessment of Enterococcus durans 6HL and Lactococcus lactis 2HL isolated from vaginal microflora. J. Med. Microbiol. 63, 1044–1051. doi: 10.1099/jmm.0.074161-0
Nilsen, T., Nes, I. F., and Holo, H. (2003). Enterolysin A, a cell wall-degrading bacteriocin from Enterococcus faecalis LMG 2333. Appl. Environ. Microbiol. 69, 2975–2984. doi: 10.1128/AEM.69.5.2975-2984.2003
Nuñez, M., Rodriguez, J. L., Garcia, E., Gaya, P., and Medina, M. (1997). Inhibition of Listeria monocytogenes by enterocin 4 during the manufacture and ripening of Manchego cheese. J. Appl. Microbiol. 83, 671–677. doi: 10.1046/j.1365-2672.1997.00275.x
Ogier, J.-C., and Serror, P. (2008). Safety assessment of dairy microorganisms: the Enterococcus genus. Int. J. Food Microbiol. 126, 291–301. doi: 10.1016/j.ijfoodmicro.2007.08.017
Ołdak, A., and Zielinska, D. (2017). Bacteriocins from lactic acid bacteria as an alternative to antibiotics. Postepy. Hig. Med. Dosw. 71, 328–338. doi: 10.5604/01.3001.0010.3817
Omar, N. B., Castro, A., Lucas, R., Abriouel, H., Yousif, N. M. K., Franz, C. M. A. P., et al. (2004). Functional and safety aspects of Enterococci isolated from different Spanish foods. Syst. Appl. Microbiol. 27, 118–130. doi: 10.1078/0723-2020-00248
Panigrahi, A., Kiron, V., Satoh, S., Hirono, I., Kobayashi, T., Sugita, H., et al. (2007). Immune modulation and expression of cytokine genes in rainbow trout Oncorhynchus mykiss upon probiotic feeding. Dev. Comp. Immunol. 31, 372–382. doi: 10.1016/j.dci.2006.07.004
Patange, S. B., Mukundan, M. K., and Ashok Kumar, K. (2005). A simple and rapid method for colorimetric determination of histamine in fish flesh. Food Control 16, 465–472. doi: 10.1016/j.foodcont.2004.05.008
Perez, R., Himeno, K., Ishibashi, N., Masuda, Y., Zendo, T., Fujita, K., et al. (2012). Monitoring of the multiple bacteriocin production by Enterococcus faecium NKR-5-3 through a developed liquid chromatography and mass spectrometry-based quantification system. J. Biosci. Bioeng. 114, 490–496. doi: 10.1016/j.jbiosc.2012.06.003
Phumisantiphong, U., Siripanichgon, K., Reamtong, O., and Diraphat, P. (2017). A novel bacteriocin from Enterococcus faecalis 478 exhibits a potent activity against vancomycin-resistant enterococci. PLoS ONE 12:e0186415. doi: 10.1371/journal.pone.0186415
Pieniz, S., Andreazza, R., Anghinoni, T., Camargo, F., and Brandelli, A. (2014). Probiotic potential, antimicrobial and antioxidant activities of Enterococcus durans strain LAB18s. Food Control 37, 251–256. doi: 10.1016/j.foodcont.2013.09.055
Pisoschi, A. M., Pop, A., Georgescu, C., Turcus, V., Olah, N. K., and Mathe, E. (2018). An overview of natural antimicrobials role in food. Eur. J. Med. Chem. 143, 922–935. doi: 10.1016/j.ejmech.2017.11.095
Qin, X., Singh, K. V., Weinstock, G. M., and Murray, B. E. (2000). Effects of Enterococcus faecalis fsr genes on production of gelatinase and a serine protease and virulence. Infect. Immun. 68, 2579–2586. doi: 10.1128/IAI.68.5.2579-2586.2000
Ramiah, K., Ten Doeschate, K., Smith, R., and Dicks, L. (2009). Safety assessment of Lactobacillus plantarum 423 and Enterococcus mundtii ST4SA determined in trials with Wistar rats. Probiotics Antimicrob. Proteins 1, 15–23. doi: 10.1007/s12602-009-9010-2
Rehaiem, A., Ben Belgacem, Z., Edalatian, M. R., Martínez, B., Rodríguez, A., Manai, M., et al. (2014). Assessment of potential probiotic properties and multiple bacteriocin encoding-genes of the technological performing strain Enterococcus faecium MMRA. Food Control 37, 343–350. doi: 10.1016/j.foodcont.2013.09.044
Rice, L. B., Carias, L., Rudin, S., Vael, C., Goossens, H., Konstabel, C., et al. (2003). A potential virulence gene, hylEfm, predominates in Enterococcus faecium of clinical origin. J. Infect. Dis. 187, 508–512. doi: 10.1086/367711
Rodríguez, E., Arques, J. L., Gaya, P., Nunez, M., and Medina, M. (2001). Control of Listeria monocytogenes by bacteriocins and monitoring of bacteriocin-producing lactic acid bacteria by colony hybridization in semi-hard raw milk cheese. J. Dairy Res. 68, 131–137. doi: 10.1017/S0022029900004660
Román, L., Padilla, D., Acosta, F., Sorroza, L., Fátima, E., Déniz, S., et al. (2015). The effect of probiotic Enterococcus gallinarum L-1 on the innate immune parameters of outstanding species to marine aquaculture. J. Appl. Anim. Res. 43, 177–183. doi: 10.1080/09712119.2014.928635
Saavedra, L., Minahk, C., de Ruiz Holgado, A. P., and Sesma, F. (2004). Enhancement of the enterocin CRL35 activity by a synthetic peptide derived from the NH2-terminal sequence. Antimicrob. Agents Chemother. 48, 2778–2781. doi: 10.1128/AAC.48.7.2778-2781.2004
Sabia, C., de Niederhäusern, S., Messi, P., Manicardi, G., and Bondi, M. (2003). Bacteriocin-producing Enterococcus casseliflavus IM 416K1, a natural antagonist for control of Listeria monocytogenes in Italian sausages (“cacciatore”). Int. J. Food Microbiol. 87, 173–179. doi: 10.1016/S0168-1605(03)00043-6
Salvucci, E., Saavedra, L., Hebert, E., Haro, C., and Sesma, F. (2012). Enterocin CRL35 inhibits Listeria monocytogenes in a murine model. Foodborne Pathog. Dis. 9, 68–74. doi: 10.1089/fpd.2011.0972
Sanders, M. E., Akkermans, L. M. A., Haller, D., Hammerman, C., Heimbach, J., Hörmannsperger, G., et al. (2010). Safety assessment of probiotics for human use. Gut Microbes 1, 164–185. doi: 10.4161/gmic.1.3.12127
Satish Kumar, R., Kanmani, P., Yuvaraj, N., Paari, K., Pattukumar, V., and Arul, V. (2011). Purification and characterization of enterocin MC13 produced by a potential aquaculture probiont Enterococcus faecium MC13 isolated from the gut of Mugil cephalus. Can. J. Microbiol. 57, 993–1001. doi: 10.1139/w11-092
Sawa, N., Wilaipun, P., Kinoshita, S., Zendo, T., Leelawatcharamas, V., Nakayama, J., et al. (2012). Isolation and characterization of enterocin W, a novel two-peptide lantibiotic produced by Enterococcus faecalis NKR-4-1. Appl. Environ. Microbiol. 78, 900–903. doi: 10.1128/AEM.06497-11
Semedo, T., Almeida Santos, M., Martins, P., Silva Lopes, M. F., Figueiredo Marques, J. J., Tenreiro, R., et al. (2003). Comparative study using type strains and clinical and food isolates to examine hemolytic activity and occurrence of the cyl operon in enterococci. J. Clin. Microbiol. 41, 2569–2576. doi: 10.1128/JCM.41.6.2569-2576.2003
Serio, A., Chaves-López, C., Paparella, A., and Suzzi, G. (2010). Evaluation of metabolic activities of enterococci isolated from Pecorino Abruzzese cheese. Int. Dairy J. 20, 459–464. doi: 10.1016/j.idairyj.2010.02.005
Settanni, L., and Corsetti, A. (2008). Application of bacteriocins in vegetable food biopreservation. Int. J. Food Microbiol. 121, 123–138. doi: 10.1016/j.ijfoodmicro.2007.09.001
Shokri, D., Zaghian, S., Khodabakhsh, F., Fazeli, H., Mobasherizadeh, S., and Ataei, B. (2014). Antimicrobial activity of a UV-stable bacteriocin-like inhibitory substance (BLIS) produced by Enterococcus faecium strain DSH20 against vancomycin-resistant Enterococcus (VRE) strains. J. Microbiol. Immunol. Infect. 47, 371–376. doi: 10.1016/j.jmii.2013.05.004
Singh, K. V., Coque, T. M., Weinstock, G. M., and Murray, B. E. (1998). in vivo testing of an Enterococcus faecalis efaA mutant and use of efaA homologs for species identification. FEMS Immunol. Med. Microbiol. 21, 323–331. doi: 10.1111/j.1574-695X.1998.tb01180.x
Sukegawa, S., Ihara, Y., Yuge, K., Rao, S., Oka, K., Arakawa, F., et al. (2014). Effects of oral administration of heat-killed Enterococcus faecium strain NHRD IHARA in post-weaning piglets. Anim. Sci. J. 85, 454–460. doi: 10.1111/asj.12163
Svetoch, E. A., Eruslanov, B. V., Kovalev, Y. N., Mitsevich, E. V., Mitsevich, I. P., Levchuk, V. P., et al. (2009). Antimicrobial activities of bacteriocins E 50-52 and B 602 against antibiotic-resistant strains involved in nosocomial infections. Probiotics Antimicrob. Proteins 1:136. doi: 10.1007/s12602-009-9027-6
Svetoch, E., Borzilov, A., Eruslanov, B., Korobova, O., Kombarova, T., Levchuk, V., et al. (2011). Efficacy of enterocin S760 in treatment of mice with anthrax infection due to Bacillus anthracis M-71. Antibiot. Khimioter. 56, 13–18.
Svetoch, E., Eruslanov, B., Perelygin, V., Mitsevich, E., Mitsevich, I., Borzenkov, V., et al. (2008). Diverse antimicrobial killing by Enterococcus faecium E 50-52 bacteriocin. J. Agric. Food Chem. 56, 1942–1948. doi: 10.1021/jf073284g
Swain, S. M., Singh, C., and Arul, V. (2009). Inhibitory activity of probiotics Streptococcus phocae PI80 and Enterococcus faecium MC13 against Vibriosis in shrimp Penaeus monodon. World J. Microbiol. Biotechnol. 25, 697–703. doi: 10.1007/s11274-008-9939-4
Tabanelli, G., Montanari, C., Bargossi, E., Lanciotti, R., Gatto, V., Felis, G., et al. (2014). Control of tyramine and histamine accumulation by lactic acid bacteria using bacteriocin forming lactococci. Int. J. Food Microbiol. 190, 14–23. doi: 10.1016/j.ijfoodmicro.2014.08.023
Tarasova, E., Yermolenko, E., Donets, V., Sundukova, Z., Bochkareva, A., Borshev, I., et al. (2010). The influence of probiotic Enterococcus faecium strain L5 on the microbiota and cytokines expression in rats with dysbiosis induced by antibiotics. Benef. Microbes 1, 265–270. doi: 10.3920/BM2010.0008
Teo, J. W., Krishnan, P., Jureen, R., and Lin, R. T. (2011). Detection of an unusual van genotype in a vancomycin-resistant Enterococcus faecium hospital isolate. J. Clin. Microbiol. 49, 4297–4298. doi: 10.1128/JCM.05524-11
Todorov, S. D., Wachsman, M. B., Knoetze, H., Meincken, M., and Dicks, L. M. (2005). An antibacterial and antiviral peptide produced by Enterococcus mundtii ST4V isolated from soya beans. Int. J. Antimicrob. Agents 25, 508–513. doi: 10.1016/j.ijantimicag.2005.02.005
Todorov, S., Wachsman, M., Tomé, E., Dousset, X., Destro, M., Dicks, L., et al. (2010). Characterisation of an antiviral pediocin-like bacteriocin produced by Enterococcus faecium. Food Microbiol. 27, 869–879. doi: 10.1016/j.fm.2010.05.001
Turgis, M., Stotz, V., Dupont, C., Salmieri, S., Khan, R. A., and Lacroix, M. (2012). Elimination of Listeria monocytogenes in sausage meat by combination treatment: radiation and radiation-resistant bacteriocins. Radiat. Phys. Chem. 81, 1185–1188. doi: 10.1016/j.radphyschem.2012.02.021
Vandera, E., Lianou, A., Kakouri, A., Feng, J., Koukkou, A.-I., and Samelis, J. (2017). Enhanced control of Listeria monocytogenes by Enterococcus faecium KE82, a multiple enterocin–producing strain, in different milk environments. J. Food Protect. 80, 74–85. doi: 10.4315/0362-028X.JFP-16-082
van Zyl, W., Deane, S., and Dicks, L. (2016). Enterococcus mundtii ST4SA and Lactobacillus plantarum 423 excludes Listeria monocytogenes from the GIT, as shown by bioluminescent studies in mice. Benef. Microbes 7, 227–235. doi: 10.3920/BM2015.0082
Venugopalan, V., Shriner, K. A., and Wong-Beringer, A. (2010). Regulatory oversight and safety of probiotic use. Emerg. Infect. Dis. 16, 1661–1665. doi: 10.3201/eid1611.100574
Vignolo, G., Palacios, J., Farias, M. E., Sesma, F., Schillinger, U., Holzapfel, W., et al. (2000). Combined effect of bacteriocins on the survival of various Listeria species in broth and meat system. Curr. Microbiol. 41, 410–416. doi: 10.1007/s002840010159
Vignolo, G., Saavedra, L., Sesma, F., and Raya, R. (2012). “Food bioprotection: lactic acid bacteria as natural preservatives,” in Progress in Food Preservation, eds A. K. A. Rajeev Bhat and G. Paliyath, (Chichester: John Wiley & Sons), 451–483.
Vijayakumar, P., and Muriana, P. (2017). Inhibition of Listeria monocytogenes on ready-to-eat meats using bacteriocin mixtures based on mode-of-action. Foods 6:22. doi: 10.3390/foods6030022
Vimont, A., Fernandez, B., Hammami, R., Ababsa, A., Daba, H., and Fliss, I. (2017). Bacteriocin-producing LCW 44: a high potential probiotic candidate from raw Camel milk. Front. Microbiol. 8:865. doi: 10.3389/fmicb.2017.00865
Wachsman, M., Castilla, V., de Ruiz Holgado, A., de Torres, R., Sesma, F., and Coto, C. (2003). Enterocin CRL35 inhibits late stages of HSV-1 and HSV-2 replication in vitro. Antiviral Res. 58, 17–24. doi: 10.1016/S0166-3542(02)00099-2
Werner, G., Fleige, C., Geringer, U., van Schaik, W., Klare, I., and Witte, W. (2011). IS element IS16 as a molecular screening tool to identify hospital-associated strains of Enterococcus faecium. BMC Infect. Dis. 11:80. doi: 10.1186/1471-2334-11-80
Yanagida, F., Chen, Y., Onda, T., and Shinohara, T. (2005). Durancin L28-1A, a new bacteriocin from Enterococcus durans L28-1, isolated from soil. Lett. Appl. Microbiol. 40, 430–435. doi: 10.1111/j.1472-765X.2005.01693.x
Yang, S.-C., Lin, C.-H., Sung, C., and Fang, J.-Y. (2014). Antibacterial activities of bacteriocins: application in foods and pharmaceuticals. Front. Microbiol. 5:241. doi: 10.3389/fmicb.2014.00241
Keywords: Enterococcus, probiotics, bacteriocin, health promotion, food safety, lactic acid bacteria, legislation
Citation: Hanchi H, Mottawea W, Sebei K and Hammami R (2018) The Genus Enterococcus: Between Probiotic Potential and Safety Concerns—An Update. Front. Microbiol. 9:1791. doi: 10.3389/fmicb.2018.01791
Received: 05 April 2018; Accepted: 17 July 2018;
Published: 03 August 2018.
Edited by:
Djamel Drider, Lille University of Science and Technology, FranceReviewed by:
Alain Rincé, U2RM EA4655, University of Caen Normandy, FranceCarmen Wacher, Universidad Nacional Autónoma de México, Mexico
Copyright © 2018 Hanchi, Mottawea, Sebei and Hammami. This is an open-access article distributed under the terms of the Creative Commons Attribution License (CC BY). The use, distribution or reproduction in other forums is permitted, provided the original author(s) and the copyright owner(s) are credited and that the original publication in this journal is cited, in accordance with accepted academic practice. No use, distribution or reproduction is permitted which does not comply with these terms.
*Correspondence: Riadh Hammami, cmlhZGguaGFtbWFtaUB1b3R0YXdhLmNh