- David Geffen School of Medicine, University of California, Los Angeles, Los Angeles, CA, United States
Mycoplasma infections, such as walking pneumonia or pelvic inflammatory diseases, are a major threat to public health. Despite their relatively small physical and genomic size, mycoplasmas are known to elicit strong host immune responses, generally inflammatory, while also being able to evade the immune system. The mycoplasma membrane is composed of approximately two-thirds protein and one-third lipid and contains several lipoproteins that are known to regulate host immune responses. Herein, the immunomodulatory effects of mycoplasma lipoproteins are reviewed. A better understanding of the immunomodulatory effects, both activating and evasive, of Mycoplasma surface lipoproteins will contribute to understanding mechanisms potentially relevant to mycoplasma disease vaccine development and treatment.
Introduction
Mycoplasmas cause a wide variety of human disease, such as asthma, bronchiectasis, and pelvic inflammatory diseases (Metaxas et al., 2015). Several species of mycoplasma such as Mycoplasma pneumoniae in the respiratory tract, M. genitalium in the genitourinary tract cause human disease (Metaxas et al., 2015). Mycoplasma infections can place a big burden on healthcare systems, with more than 100,000 annual hospitalizations arising from M. pneumoniae in the US alone (Atkinson et al., 2008). In addition, mycoplasma infections of the respiratory and urogenital tract can be chronic. A better understanding of the pathogenesis of mycoplasma infections will contribute to vaccine development and treatment.
A key characteristic of the pathogenesis of chronic mycoplasma infections is the cross-talk between mycoplasmas and the host immune system (Atkinson et al., 2008). An inflammation response induced by the host’s immunity is one of the main characteristics of M. pneumoniae infection and contributes to clinical presentations. Elucidating the mycoplasma cellular components will help us understand how the relatively small, and biochemically simple, mycoplasmas are capable of eliciting strong and chronic immune responses (Himmelreich et al., 1996). Mycoplasmas can induce proinflammatory response through secreted toxins, surface antigens and other unclear mechanisms (Yeh et al., 2016). Considering that Mycoplasma lack cell walls, cell wall proteins do not interacting with the host’s immune system (Pereyre et al., 2016). Analysis of the mycoplasmal cell membrane demonstrated that several lipoprotein surface antigens elicit strong immune responses (Muhlradt et al., 1997) through a distinct pathway than that seen with lipopolysaccharides (Rawadi and Roman-Roman, 1996). Unlike numerous other bacterial species, a large portion of mycoplasmal genome (6.68%) is dedicated to encoding various lipoproteins (Dandekar et al., 2000; Hallamaa et al., 2006). The functionality of these genes is unknown, but they may drive adhesion and/or the initial stages of mycoplasmal infection since their products are cell surface proteins. Certain mycoplasmal lipoproteins have exonuclease activity that may be involved in the function of the ATP-binding cassette (ABC) transport system to import nucleic acid precursors (Schmidt et al., 2007). Little is known about the transcriptional regulation that occurs within these genes, especially since the mycoplasmal genome lacks many of the transcriptional regulators found in other bacteria (Himmelreich et al., 1996; Dandekar et al., 2000).
The lipoylation mechanism of lipoproteins in mycoplasmas is similar to that of other bacteria (Rottem, 2003). Bacterial lipoproteins play a major role in the cross-talk between bacteria and host immune responses. Bacterial lipoproteins contain a lipoylated amino-terminal cysteinyl residue that is usually N-acylated (Braun and Hantke, 1974). However, an N-acyltransferase gene has not been found in M. pneumoniae or M. genitalium genome (Himmelreich et al., 1996) and many mycoplasmal lipoproteins are not N-acylated [such as lipoproteins from M. gallisepticum (Jan et al., 1996; Muhlradt et al., 1997)]. Triacylation or diacylation of lipoproteins affects their recognition from different Toll like receptors (Jan et al., 1995). Thus, the unique structure of mycoplasmal lipoproteins can determine their immunomodulatory properties. Mycoplasma lipoproteins are all known to induce expression of proinflammatory cytokines including IL-1β, IL-6, and IL-2 (Garcia et al., 1998; Hoek et al., 2005). Several lipoproteins have been identified in mycoplasmas (Table 1).
Although Mycoplasma lipoproteins may function as immune-activators within their host, they also regulate mycoplasma colonization and translocation across mucosal membranes and facilitate host immune evasion (Citti et al., 1997; Chambaud et al., 1999). Understanding lipoprotein-induced immunomodulation will aid in developing novel treatments against Mycoplasmas (Martinez de Tejada et al., 2015). Herein, we review the evidence on the effects of mycoplasmal lipoproteins on host immunity (Figure 1). Given the complex immunomodulatory effects of mycoplasma lipoproteins we will dissect these effects by specific cell type (epithelial cells, neutrophils, myeloid cells, lymphocytes).
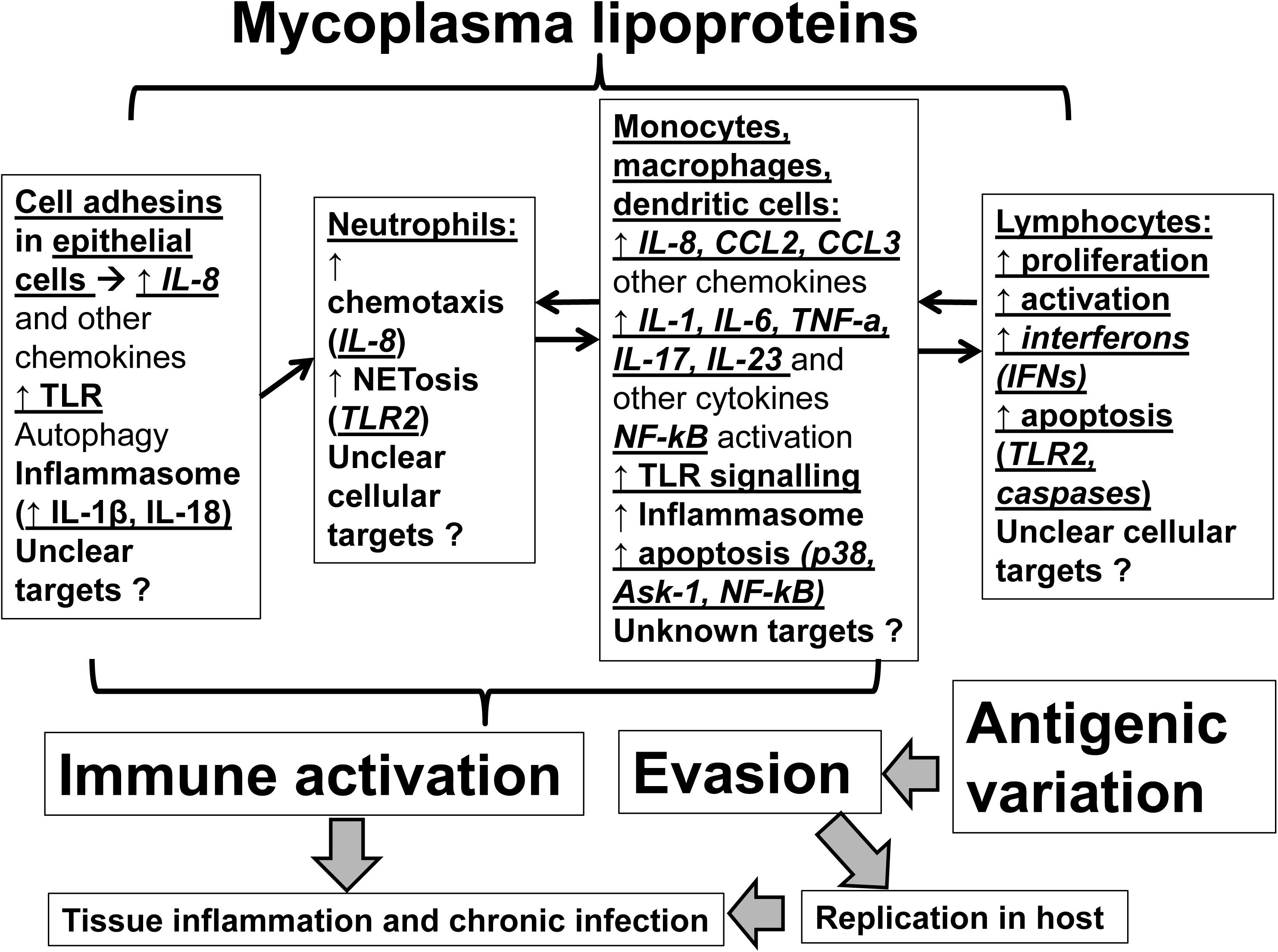
FIGURE 1. Mechanisms of actions of mycoplasmal lipoproteins: Mycoplasmal lipoproteins lead to inflammatory responses and immune system activation through direct effects on both epithelial and immune cells. Initial adhesion to epithelia of respiratory tract is mediated through adhesins such as P1 (MPN141) as well as other accessory proteins. Epithelial cell activation as well as mycoplasmal lipoproteins induce secretion of chemokines such as IL-8 that promote neutrophilic infiltration and activation of neutrophils (through several mechanisms including NETosis). Neutrophilic activation is followed by activation of myeloid cells (monocytes, macrophages, dendritic cells) that ultimately leads to activation of adaptive immunity (lymphocytes). A key family of receptors that play an important role in recognizing, and inducing responses to Mycoplasmal lipoproteins are the Toll-Like Receptors (TLRs such as TLR2 and TLR6) that are key players in inflammatory responses upon infection. Mycoplasmal lipoproteins can also activate cells through the inflammasome and the pre-cursors of proinflammatory cytokines, such as IL-1β and IL-18, can be cleaved and activated in a caspase-dependent manner prior to their release. Non-TLR or inflammasome dependent mechanisms by which Mycoplasmal lipoproteins can lead to inflammatory responses and immune activation also exist (such as autophagy). TLRs (such as TLR-2 and TLR-6), also play an important role in inducing apoptosis in both monocyte and lymphocyte cell lines through induction of p38 MAPK, the apoptosis signal-regulating kinase (ASK1), caspase and the NF-kB pathways and the release of Nitric Oxide from macrophages. See text for further details. Given poorly understood mechanisms, further studies are needed to elucidate the mechanisms of immunomodulation mediated by mycoplasmal lipoproteins.
Effects of Mycoplasmal Lipoproteins on Epithelial Cells
Epithelial cells are the main targets of M. pneumoniae and secrete cytokines in response to infections (Fahey et al., 2005). Mycoplasmas directly induce in vitro production of chemotactic cytokines such as IL-8 by bronchial epithelial cells (Chambaud et al., 1999; Peng et al., 2007; Chen et al., 2016). Mycoplasmal lipoproteins may also have a major role in regulation of the cytokine response in respiratory epithelial cells during M. pneumoniae infection (Eckmann et al., 1995; Yang et al., 2002). Similar to the role of respiratory epithelial cells in eliciting a response during M. pneumoniae infection, epithelial cells lining the vagina and cervix may induce pro-inflammatory cytokines upon exposure to lipoproteins present in M. genitalium (McGowin et al., 2009). However, the exact mechanisms that contribute to differential effects of mycoplasma lipoproteins on epithelial compared to immune cells remain unclear. Epithelial cells are also able to have an effect on Mycoplasmas. Upon contact with human epithelial cells in-vivo, M. pneumoniae can undergo transcriptional changes in lipoprotein related genes (Hallamaa et al., 2008). Such an alteration in the genetic profile of the Mycoplasma upon coming into contact with epithelial cells represents the two-way dynamic existing between Mycoplasma and the host, where both parties are able to influence each other. Thus, the initial release of proinflammatory cytokines and chemokines by epithelial cells infected by Mycoplasmas can also induce early recruitment of immune cells such as neutrophils.
Effects of Mycoplasmal Lipoproteins on Neutrophils
Mycoplasma respiratory infections lead to the recruitment of polymorphonuclear leukocytes and subsequently monocytes/macrophages (M/M) and lymphocytes (Kruger and Baier, 1997). The attraction of leukocytes to the site of infection is controlled by chemokines, which are chemotactic cytokines. The M. pneumoniae/IL-8/neutrophil axis likely plays a vital role in the pathogenesis of MPP and mycoplasmas directly induce in vitro production of chemotactic cytokines such as IL-8 by bronchial epithelial cells (Chambaud et al., 1999; Peng et al., 2007; Chen et al., 2016). An M. fermentans lipoprotein fraction induces secretion of IL-8 from cultured human M/M (Chambaud et al., 1999; Peng et al., 2007; Chen et al., 2016). The expression of such chemokines allows for the recruitment of leukocytes, such as neutrophils, to the site of infection, and it is this influx of leukocytes that comes to partially contribute to the inflammatory response seen upon mycoplasma infection (Wu et al., 2007).
Except for IL-8 and neutrophil chemotaxis, mycoplasma lipoproteins also induce NETosis. Neutrophil extracellular traps (NETs) are a major component of the first line of defense against invading pathogens and they involve a pathogen facilitated cell death by which neutrophils can extrude chromatin structures loaded with anti-microbial molecules to eliminate pathogens (Gomez-Lopez et al., 2017). Experiments using M. agalactiae have shown that mycoplasmas are capable of inducing NETosis upon infection, and mycoplasma lipoproteins are the component primarily responsible for activation of these pathways in neutrophils (Cacciotto et al., 2016). Furthermore, it has also been shown that lipoproteins induce Toll-like Receptor 2 (TLR2) signaling that plays a pivotal role in neutrophil NETosis (Cacciotto et al., 2016; Xu et al., 2017). Notably, NET formation ability decreases with patient age, and this may contribute to the susceptibility of older patients to mycoplasmal pathogens such as M. pneumoniae (Xu et al., 2017). More research is needed on the ability of lipoproteins to induce NETosis. Thus, mycoplasmal lipoproteins regulate neutrophilic host immune responses, which are the mainstay of pathogenesis of mycoplasmal infections. These early neutrophilic responses can also contribute to inflammatory responses from myeloid cells.
Effects of Mycoplasmal Lipoproteins on Myeloid Cells
Mycoplasmas induce activation of cytolytic activity of macrophages, and stimulation of cytokines (interleukin [IL]-1, IL-6, tumor necrosis factor-alpha [TNF-α]). Although several mycoplasmal proteins can induce these proinflammatory responses (Chambaud et al., 1999; Peng et al., 2007; Chen et al., 2016), mycoplasmal lipoproteins seem to be a major instigator of production of proinflammatory cytokines by myeloid cells such as M/M and dendritic cells (Cole et al., 2005; He et al., 2009). Non-denaturing detergents enriched with mycoplasmal lipoproteins, have modulatory capacities (Cole et al., 2005; Liu et al., 2012). Synthetic lipopeptides such as the Mycoplasma-derived lipopeptide MALP-2 from M. fermentans (Muhlradt et al., 1997), FSL-1 (also known as Pam2C) from M. salivarium (Shibata et al., 2000), and MPPL-1 from M. pneumoniae (Into et al., 2007) were shown to have immunomodulatory properties. Activation of macrophages by mycoplasmal lipoproteins can occur at pico-molar concentrations of lipoproteins, making them extremely potent activators (Muhlradt et al., 1997).
In vitro and in vivo studies demonstrated that the M. fermentans-derived membrane component MALP-2 (macrophage-activating lipopeptide 2) is the main active component that induces these proinflammatory responses (Kaufmann et al., 1999). MALP-2 directly induced in vitro production of the proinflammatory cytokines TNF-α and IL-6 and the neutrophil-attracting CXC chemokines IL-8 and Growth-regulated Oncogene α (GRO-α) as well as the mononuclear leukocyte-attracting CC chemokines Monocyte chemoattractant protein-1 (MCP-1/CCL2), Macrophage inflammatory protein 1 alpha (MIP-1a/CCL3), and Macrophage inflammatory protein 1 beta (MIP-1b/CCL3) (Garcia et al., 1998; Rawadi et al., 1998). The MALP-2 molecule is a potent activator of human monocytes.
Mycoplasmal lipoproteins in M. hominis such as lipoprotein MHO_4720 were recently shown to induce production of Interleukin-23 (IL-23), by dendritic cells through activation of inflammasome (Chen et al., 2017; Goret et al., 2017). Production of proinflammatory cytokines such as IL-23 has been shown to induce the expression of chemokines such as IL-17, allowing for effective containment of the infection (Wu et al., 2007). Additional in vivo studies have shown that many of the chemokines induced by mycoplasma infection in humans are conserved across species, such as macrophage inflammatory protein (MIP)-1β (Lam, 2002). Certain mycoplasmal lipoproteins, such as those from M. hyopneumoniae also elicit anti-inflammatory cytokines such as Interleukin-10 (Chambaud et al., 1999; Mollazadeh et al., 2017).
Inducing apoptosis of both monocytes and lymphocytes is a key step in allowing Mycoplasma to prolong their survival within their hosts, and has been documented in numerous Mycoplasma, with M. bovis being one of the most well-studied (Into et al., 2004; Jimbo et al., 2017). Mycoplasma lipoproteins induce apoptosis in both monocyte (ex. HL-60, THP-1) and lymphocyte cell lines (ex. MOLT-4) (Aliprantis et al., 1999; Into et al., 2002a, 2004) and thus directly contribute to immune evasion.
Thus, mycoplasmal lipoproteins regulate myeloid based host immune responses and inflammatory responses, which are key mediators of pathogenesis of mycoplasmal infections. These innate immune responses may also drive responses from adaptive immunity (such as lymphocytic responses).
Effects of Mycoplasmal Lipoproteins on Lymphocytes
Mycoplasmas induce proliferation of T and B cells (Naot et al., 1979; Cole et al., 1990) through superantigens or unidentified factors. Various mycoplasmas also activate cytotoxic T lymphocytes (CTLs) and natural killer (NK) cells (Wayner and Brooks, 1984) and induce expression of major histocompatibility antigens on B cells (Ross et al., 1992), IL-2 by T cells, interleukin (IL)-1 by macrophages (Muhlradt et al., 1991) and interferons (IFNs) by lymphocytes (Arai et al., 1983). Collectively these mycoplasma-driven immunomodulatory effects contribute to the proliferation and maturation of both T and B lymphocytes as well as the activation of macrophages and NK cells (Arango Duque and Descoteaux, 2014).
During the early phases of infection, mycoplasmas usually induce an inflammatory and a humoral response preferentially directed against their membrane-bound, surface-exposed lipoproteins. Mycoplasmal lipoproteins such as spiralin induce T-cell-independent B-cell blastogenesis and secretion of proinflammatory cytokines (Brenner et al., 1997). Lipoproteins from M. fermentans and M. salivarium induce TLR2- and caspase-mediated apoptosis in lymphocytes (Into et al., 2004). The effects of mycoplasmal lipoproteins on lymphocytes may not be mediated by effects on TLR signaling on antigen presenting cells such as dendritic cells (Sawahata et al., 2011). Thus, mycoplasmal lipoproteins also regulate adaptive immune and function of both B cells and T cells.
Effects of Mycoplasmal Lipoproteins on Evasion of Host Immunity
Although lipoproteins have a major role as immune system activators, they also facilitate evasion of the host’s immune response, leading to chronic mycoplasma infections (Becker and Sander, 2016; Goret et al., 2016). Mycoplasma lipoproteins facilitate immune evasion through several mechanisms such as creation of the mycoplasmal shield, lipoprotein antigenic variation, and protection from growth inhibiting host antibodies (Citti et al., 1997; Chambaud et al., 1999). Understanding the various modes of immune system evasion promises a better approach to treating mycoplasma infections.
Lipoproteins as the Basis for Mycoplasmal Shield Against Host Immune Defense Mechanisms
The ability of mycoplasma to encode numerous forms of lipoproteins that can potentially be expressed on their surface allows mycoplasmas to avoid host immune responses (Simmons et al., 2004). Mycoplasmas are able to create a protective layer around themselves that can sterically hinder access of growth-inhibiting host antibodies and even macrophages (Citti et al., 1997; Simmons et al., 2004). In addition to facilitating Mycoplasmal protection, the lipoprotein shield also plays an important role in hemadsorption and mycoplasmal adherence to surfaces (Bolland and Dybvig, 2012; Xiong et al., 2016). Mycoplasmas that are minimally shielded may be highly adherent while maximally shielded mycoplasmas are less adherent (Bolland and Dybvig, 2012).
Variable Surface Antigen (Vsa) Lipoproteins as Facilitators of Immune Evasion
In addition to the generation of a shield for protecting Mycoplasmas, lipoproteins are also able to protect Mycoplasma by undergoing variation. One major method by which lipoprotein variation in mycoplasmas can contribute to host immune evasion involves altering the length of these variable surface antigens (Vsa) (Xiong et al., 2016). Mycoplasmas use antigenic variation not only to evade immune responses but also to adapt to each host (Bencina et al., 2001). Vsa lipoproteins normally have a 242-amino acid N-terminus with a variable C-terminal domain that can contain up to 60 tandem repeating units which in themselves can range in size from 10 to 19 amino acids (Bencina et al., 2001). Each cell can only transcribe one Vsa gene at a time, with silent Vsa genes missing the sequences necessary to make the conserved N-terminal domain (Shen et al., 2000). To achieve a large variety of Vsa recombination of lipoproteins, mycoplasmas use DNA recombination (Dybvig et al., 1998; Shen et al., 2000). Although a single cell may only transcribe a single Vsa gene, during an infection subpopulations of cells are able to express varying Vsa lipoproteins (Pantoja et al., 2016).
Despite the great importance Vsa size variation plays in facilitating prolonged mycoplasmal survival within hosts, adaptive immunity is still able to decrease mycoplasmal survival, thus necessitating phase variation of the shield as well (Simmons and Dybvig, 2009). Thus, in addition to being able to conduct size variation of surface lipoproteins, mycoplasmas are also able to conduct phase (type) variation of their lipoproteins, hence the name Vsa lipoproteins (Dybvig et al., 1998; Chambaud et al., 1999). Phase variation in vivo is only seen upon the onset of an adaptive immune response, with no alterations occurring in Vsa expression if an organism lacks B and T cells (Gumulak-Smith et al., 2001). It is estimated that phase switching occurs at a frequency of around 10-3 per CFU per generation (Rosengarten and Wise, 1990). In having the ability to undergo phase variation, mycoplasmas are able to evade host immune responses and indeed lead to chronic infections, similar to how Spirochetes might also use antigenic variation in order to establish a chronic infection (Chambaud et al., 1999; Christodoulides et al., 2017). Thus, Mycoplasmal lipoproteins have pleotropic immunomodulatory effects and their mechanisms of actions need to be elucidated.
Mechanisms of Action of Mycoplasmal Lipoproteins
A key family of receptors that play an important role in recognizing, and inducing responses to Mycoplasmal lipoproteins are the Toll-Like Receptors (TLRs) that are key players in inflammatory responses upon infection (Akira et al., 2001; Wang et al., 2017). Two of the most well-studied TLRs are TLR2 and TLR6, both of which are pivotal in recognizing Mycoplasmal Macrophage-Activating Lipopeptide-2 (MALP-2), with the absence of either TLR leading to a lack of recognition and macrophage activation (Takeuchi et al., 2002). Mycoplasmal lipoproteins can also activate myeloid cells through the inflammasome (Chen et al., 2017; Goret et al., 2017). Amongst the Mycoplasma capable of activating inflammasome with their lipoproteins are M. salivarium and M. pneumoniae (Shimizu, 2016; Sugiyama et al., 2016). Through the activation of the inflammasome, the pre-cursors of proinflammatory cytokines, such as IL-1β and IL-18, can be cleaved and activated in a caspase-dependent manner prior to their release (Boyden and Dietrich, 2006).
Non-pathogenic Mycoplasma also express lipoproteins, suggesting that a non-TLR dependent, or inflammasome dependent, mechanism exists by which Mycoplasmal lipoproteins can lead to immune activation (Shimizu, 2016). M. pneumoniae was still able to induce a strong inflammatory response in TLR2 KO mice (Shimizu et al., 2014). Introduction of known autophagy inhibitors to the TLR2 KO mice in turn lead to a significant fall in the inflammatory response, suggesting a role of autophagy in also leading to inflammatory responses (Shimizu et al., 2014).
Another mechanism by which Mycoplasmal lipoproteins are able to lead to immune system activation is through their initial adhesion to epithelia such as the respiratory tract in the case of M. pneumoniae (Jordan et al., 2001). Such cytadherence is mediated through adhesins such as P1(MPN141) as well as other accessory proteins, and treatment of Mycoplasma with proteases or anti-P1 antibodies was seen to lead to a significant decrease in proinflammatory cytokine release (Yang et al., 2002; Hoek et al., 2005). Cytadherence on behalf of M. pneumoniae was seen to generate a proinflammatory response through pathways involving both autophagy/TLR4 as well as inflammasome (Shimizu et al., 2014).
In addition to their roles as inductors of the inflammatory response, TLRs also play an important role in inducing apoptosis in both monocyte cell lines (ex. HL-60, HEK293, THP-1) and lymphocyte cell lines (ex. MOLT-4) upon binding of Mycoplasmal lipoproteins (Aliprantis et al., 1999; Into et al., 2002a; Into et al., 2004). The two main TLRs seen to play a role in this apoptotic pathway are TLR-2 and TLR-6, which through their activation allow induction of NF-kB (Aliprantis et al., 1999; Wu et al., 2008) and the release of Nitric Oxide from macrophages (Muhlradt and Frisch, 1994; Into et al., 2004). Mycoplasmal induced apoptosis seems to be mediated by activation of the p38 MAPK, the apoptosis signal-regulating kinase (ASK1) and the NF-kB pathways (Into and Shibata, 2005). Mycoplasmas such as M. salivarium and M. fermentans have also been seen to induce apoptosis through the more conventional caspase pathways (Into et al., 2002a,b).
On the other hand, certain mycoplasmal lipoproteins, such as those seen in M. fermentans, can inhibit TNF-α mediated apoptosis (Gerlic et al., 2007). Thus, further research is needed to understand the pleotropic effects of mycoplasmal lipoproteins on apoptosis.
A better understanding of mechanisms of immunomodulation mediated by mycoplasmal lipoproteins may lead to therapies to attenuate the detrimental effects of mycoplasma infections.
Mycoplasmal Lipoproteins as Therapeutic Targets
One of the most difficult parts of managing a Mycoplasmal infection is the inability to use many antibiotics such as beta-lactams (Bebear and Pereyre, 2005). The development of drugs such as tigecycline that aim to reduce production of chemokines and lipoproteins through targeting of bacterial ribosomes is a growing discipline within the field of Mycoplasma research (Saliba et al., 2009; Salvatore et al., 2009). Studies of tigecycline in a murine model have been able to confirm a significant drop of cytokine and chemokine production following infection with M. pneumoniae (Salvatore et al., 2009). Many studies that focus on development of a possible vaccine against Mycoplasma use inactivated Mycoplasma that can then be introduced to patients (Wenzel et al., 1977; David et al., 2010). Atalla et al. (2015) were able to show that inactivation of Mycoplasma using a photoactivatable alkylating agent (INA), with preservation of the surface lipoproteins, might be a possible mechanism by which Mycoplasma can be inactivated for vaccine development (Atalla et al., 2015). Through a better understanding of bacterial lipoproteins and their interaction with the immune system, researchers are now aiming to develop vaccines that rely on the introduction of bacterial lipoproteins into patients to generate antibody responses (Leng et al., 2015). For example, the above has been achieved with the AvgC lipoprotein from M. agalactiae and shows promise for a vaccine against M. agalactiae infection (Santona et al., 2002). Such an approach to vaccine development is also currently being studied using bacterial lipoproteins, such as the OspA from Spirochetes (Izac et al., 2017; Zhao et al., 2017). An innovative approach for treatment of Mycoplasmal infections would involve direct targeting of lipoproteins; however, such an approach remains to be established given the structure of mycoplasmal lipoproteins and their diverse immunomodulatory mechanisms. Importantly, the polypeptide Aspidasept® which neutralizes endotoxin in vivo was previously shown to inhibit cytokines induced by the Mycoplasmal lipoproteins (Martinez de Tejada et al., 2015). Thus, a better understanding of Mycoplasmal lipoprotein epitopes, may promote progress in the field of antibodies, polypeptides or vaccines against Mycoplasma.
Conclusion
Further studies are needed to promote understanding of the mechanisms of transcriptional regulation, phase variations and the numerous immunomodulatory effects (both acute and chronic) of Mycoplasmal lipoproteins. Given the decreasing efficacy of antibiotics against mycoplasmas, there is need for novel immunotherapies. Novel strategies such as use of inactivating agents that preserve the mycoplasmal surface lipoproteins, may advance vaccine development (Atalla et al., 2015). Novel approaches such as use of polypeptides that attenuate proinflammatory effects of mycoplasmal lipoproteins need further investigation as novel therapeutic approaches for mycoplasmal infections (Martinez de Tejada et al., 2015). Ultimately, a better understanding of mycoplasmal lipoproteins can set the basis for the development of vaccines or antibodies against mycoplasmal lipoproteins and perhaps shed light on the pathogenesis of other vector based pathogens such as Spirochetes.
Author Contributions
AC, NG, VY, JP, NM, and TK wrote and edited the manuscript. TK conceptualized the manuscript.
Funding
This work was supported by NIH grants NIH K08AI08272, NIH/NCATS Grant #UL1TR000124.
Conflict of Interest Statement
The authors declare that the research was conducted in the absence of any commercial or financial relationships that could be construed as a potential conflict of interest.
References
Akira, S., Takeda, K., and Kaisho, T. (2001). Toll-like receptors: critical proteins linking innate and acquired immunity. Nat. Immunol. 2, 675–680. doi: 10.1038/90609
Aliprantis, A. O., Yang, R. B., Mark, M. R., Suggett, S., Devaux, B., Radolf, J. D., et al. (1999). Cell activation and apoptosis by bacterial lipoproteins through toll-like receptor-2. Science 285, 736–739. doi: 10.1126/science.285.5428.736
Arai, S., Munakata, T., and Kuwano, K. (1983). Mycoplasma interaction with lymphocytes and phagocytes: role of hydrogen peroxide released from M. pneumoniae. Yale J. Biol. Med. 56, 631–638.
Arango Duque, G., and Descoteaux, A. (2014). Macrophage cytokines: involvement in immunity and infectious diseases. Front. Immunol. 5:491. doi: 10.3389/fimmu.2014.00491
Atalla, H., Lysnyansky, I., Raviv, Y., and Rottem, S. (2015). Mycoplasma gallisepticum inactivated by targeting the hydrophobic domain of the membrane preserves surface lipoproteins and induces a strong immune response. PLoS One 10:e0120462. doi: 10.1371/journal.pone.0120462
Atkinson, T. P., Balish, M. F., and Waites, K. B. (2008). Epidemiology, clinical manifestations, pathogenesis and laboratory detection of Mycoplasma pneumoniae infections. FEMS Microbiol. Rev. 32, 956–973. doi: 10.1111/j.1574-6976.2008.00129.x
Balish, M. F. (2014). Mycoplasma pneumoniae, an underutilized model for bacterial cell biology. J. Bacteriol. 196, 3675–3682. doi: 10.1128/JB.01865-14
Bebear, C. M., and Pereyre, S. (2005). Mechanisms of drug resistance in Mycoplasma pneumoniae. Curr. Drug Targets Infect. Disord. 5, 263–271. doi: 10.2174/1568005054880109
Becker, K., and Sander, P. (2016). Mycobacterium tuberculosis lipoproteins in virulence and immunity – fighting with a double-edged sword. FEBS Lett. 590, 3800–3819. doi: 10.1002/1873-3468.12273
Bencina, D., Drobnic-Valic, M., Horvat, S., Narat, M., Kleven, S. H., and Dovc, P. (2001). Molecular basis of the length variation in the N-terminal part of Mycoplasma synoviae hemagglutinin. FEMS Microbiol. Lett. 203, 115–123. doi: 10.1111/j.1574-6968.2001.tb10829.x
Bolland, J. R., and Dybvig, K. (2012). Mycoplasma pulmonis Vsa proteins and polysaccharide modulate adherence to pulmonary epithelial cells. FEMS Microbiol. Lett. 331, 25–30. doi: 10.1111/j.1574-6968.2012.02551.x
Boyden, E. D., and Dietrich, W. F. (2006). Nalp1b controls mouse macrophage susceptibility to anthrax lethal toxin. Nat. Genet. 38, 240–244. doi: 10.1038/ng1724
Braun, V., and Hantke, K. (1974). Biochemistry of bacterial cell envelopes. Annu. Rev. Biochem. 43, 89–121. doi: 10.1146/annurev.bi.43.070174.000513
Brenner, C., Wroblewski, H., Le Henaff, M., Montagnier, L., and Blanchard, A. (1997). Spiralin, a mycoplasmal membrane lipoprotein, induces T-cell-independent B-cell blastogenesis and secretion of proinflammatory cytokines. Infect. Immun. 65, 4322–4329.
Cacciotto, C., Cubeddu, T., Addis, M. F., Anfossi, A. G., Tedde, V., Tore, G., et al. (2016). Mycoplasma lipoproteins are major determinants of neutrophil extracellular trap formation. Cell. Microbiol. 18, 1751–1762. doi: 10.1111/cmi.12613
Chambaud, I., Wroblewski, H., and Blanchard, A. (1999). Interactions between mycoplasma lipoproteins and the host immune system. Trends Microbiol. 7, 493–499. doi: 10.1016/S0966-842X(99)01641-8
Chen, Z., Gong, Y., and Shi, Y. (2017). Novel biologic agents targeting interleukin-23 and interleukin-17 for moderate-to-severe psoriasis. Clin. Drug Investig. 37, 891–899. doi: 10.1007/s40261-017-0550-z
Chen, Z., Shao, X., Dou, X., Zhang, X., Wang, Y., Zhu, C., et al. (2016). Role of the Mycoplasma pneumoniae/interleukin-8/neutrophil axis in the pathogenesis of pneumonia. PLoS One 11:e0146377. doi: 10.1371/journal.pone.0146377
Christodoulides, A., Boyadjian, A., and Kelesidis, T. (2017). Spirochetal lipoproteins and immune evasion. Front. Immunol. 8:364. doi: 10.3389/fimmu.2017.00364
Citti, C., Kim, M. F., and Wise, K. S. (1997). Elongated versions of Vlp surface lipoproteins protect Mycoplasma hyorhinis escape variants from growth-inhibiting host antibodies. Infect. Immun. 65, 1773–1785.
Cole, B. C., Kartchner, D. R., and Wells, D. J. (1990). Stimulation of mouse lymphocytes by a mitogen derived from Mycoplasma arthritidis (MAM). VIII. Selective activation of T cells expressing distinct V beta T cell receptors from various strains of mice by the “superantigen” MAM. J. Immunol. 144, 425–431.
Cole, B. C., Mu, H. H., Pennock, N. D., Hasebe, A., Chan, F. V., Washburn, L. R., et al. (2005). Isolation and partial purification of macrophage- and dendritic cell-activating components from Mycoplasma arthritidis: association with organism virulence and involvement with Toll-like receptor 2. Infect. Immun. 73, 6039–6047. doi: 10.1128/IAI.73.9.6039-6047.2005
Dandekar, T., Huynen, M., Regula, J. T., Ueberle, B., Zimmermann, C. U., Andrade, M. A., et al. (2000). Re-annotating the Mycoplasma pneumoniae genome sequence: adding value, function and reading frames. Nucleic Acids Res. 28, 3278–3288. doi: 10.1093/nar/28.17.3278
David, S. A., Volokhov, D. V., Ye, Z., and Chizhikov, V. (2010). Evaluation of Mycoplasma inactivation during production of biologics: egg-based viral vaccines as a model. Appl. Environ. Microbiol. 76, 2718–2728. doi: 10.1128/AEM.02776-09
Dybvig, K., Sitaraman, R., and French, C. T. (1998). A family of phase-variable restriction enzymes with differing specificities generated by high-frequency gene rearrangements. Proc. Natl. Acad. Sci. U.S.A. 95, 13923–13928. doi: 10.1073/pnas.95.23.13923
Eckmann, L., Kagnoff, M. F., and Fierer, J. (1995). Intestinal epithelial cells as watchdogs for the natural immune system. Trends Microbiol. 3, 118–120. doi: 10.1016/S0966-842X(00)88894-0
Fahey, J. V., Schaefer, T. M., Channon, J. Y., and Wira, C. R. (2005). Secretion of cytokines and chemokines by polarized human epithelial cells from the female reproductive tract. Hum. Reprod. 20, 1439–1446. doi: 10.1093/humrep/deh806
Garcia, J., Lemercier, B., Roman-Roman, S., and Rawadi, G. (1998). A Mycoplasma fermentans-derived synthetic lipopeptide induces AP-1 and NF-kappaB activity and cytokine secretion in macrophages via the activation of mitogen-activated protein kinase pathways. J. Biol. Chem. 273, 34391–34398. doi: 10.1074/jbc.273.51.34391
Gerlic, M., Horowitz, J., Farkash, S., and Horowitz, S. (2007). The inhibitory effect of Mycoplasma fermentans on tumour necrosis factor (TNF)-alpha-induced apoptosis resides in the membrane lipoproteins. Cell. Microbiol. 9, 142–153. doi: 10.1111/j.1462-5822.2006.00774.x
Gomez-Lopez, N., Romero, R., Garcia-Flores, V., Xu, Y., Leng, Y., Alhousseini, A., et al. (2017). Amniotic fluid neutrophils can phagocytize bacteria: a mechanism for microbial killing in the amniotic cavity. Am. J. Reprod. Immunol. 78:e12723. doi: 10.1111/aji.12723
Goret, J., Beven, L., Faustin, B., Contin-Bordes, C., Le Roy, C., Claverol, S., et al. (2017). Interaction of Mycoplasma hominis PG21 with human dendritic cells: interleukin-23-inducing mycoplasmal lipoproteins and inflammasome activation of the cell. J. Bacteriol. 199, e213–e217. doi: 10.1128/JB.00213-17
Goret, J., Le Roy, C., Touati, A., Mesureur, J., Renaudin, H., Claverol, S., et al. (2016). Surface lipoproteome of Mycoplasma hominis PG21 and differential expression after contact with human dendritic cells. Future Microbiol. 11, 179–194. doi: 10.2217/fmb.15.130
Gumulak-Smith, J., Teachman, A., Tu, A. H., Simecka, J. W., Lindsey, J. R., and Dybvig, K. (2001). Variations in the surface proteins and restriction enzyme systems of Mycoplasma pulmonis in the respiratory tract of infected rats. Mol. Microbiol. 40, 1037–1044. doi: 10.1046/j.1365-2958.2001.02464.x
Hallamaa, K. M., Browning, G. F., and Tang, S. L. (2006). Lipoprotein multigene families in Mycoplasma pneumoniae. J. Bacteriol. 188, 5393–5399. doi: 10.1128/JB.01819-05
Hallamaa, K. M., Tang, S. L., Ficorilli, N., and Browning, G. F. (2008). Differential expression of lipoprotein genes in Mycoplasma pneumoniae after contact with human lung epithelial cells, and under oxidative and acidic stress. BMC Microbiol. 8:124. doi: 10.1186/1471-2180-8-124
He, J., You, X., Zeng, Y., Yu, M., Zuo, L., and Wu, Y. (2009). Mycoplasma genitalium-derived lipid-associated membrane proteins activate NF-kappaB through toll-like receptors 1, 2, and 6 and CD14 in a MyD88-dependent pathway. Clin. Vaccine Immunol. 16, 1750–1757. doi: 10.1128/CVI.00281-09
Himmelreich, R., Hilbert, H., Plagens, H., Pirkl, E., Li, B. C., and Herrmann, R. (1996). Complete sequence analysis of the genome of the bacterium Mycoplasma pneumoniae. Nucleic Acids Res. 24, 4420–4449. doi: 10.1093/nar/24.22.4420
Hoek, K. L., Duffy, L. B., Cassell, G. H., Dai, Y., and Atkinson, T. P. (2005). A role for the Mycoplasma pneumoniae adhesin P1 in interleukin (IL)-4 synthesis and release from rodent mast cells. Microb. Pathog. 39, 149–158. doi: 10.1016/j.micpath.2005.07.004
Into, T., Dohkan, J., Inomata, M., Nakashima, M., Shibata, K., and Matsushita, K. (2007). Synthesis and characterization of a dipalmitoylated lipopeptide derived from paralogous lipoproteins of Mycoplasma pneumoniae. Infect. Immun. 75, 2253–2259. doi: 10.1128/IAI.00141-07
Into, T., Kiura, K., Yasuda, M., Kataoka, H., Inoue, N., Hasebe, A., et al. (2004). Stimulation of human Toll-like receptor (TLR) 2 and TLR6 with membrane lipoproteins of Mycoplasma fermentans induces apoptotic cell death after NF-kappa B activation. Cell. Microbiol. 6, 187–199. doi: 10.1046/j.1462-5822.2003.00356.x
Into, T., Nodasaka, Y., Hasebe, A., Okuzawa, T., Nakamura, J., Ohata, N., et al. (2002a). Mycoplasmal lipoproteins induce toll-like receptor 2- and caspases-mediated cell death in lymphocytes and monocytes. Microbiol. Immunol. 46, 265–276.
Into, T., Okada, K., Inoue, N., Yasuda, M., and Shibata, K. (2002b). Extracellular ATP regulates cell death of lymphocytes and monocytes induced by membrane-bound lipoproteins of Mycoplasma fermentans and Mycoplasma salivarium. Microbiol. Immunol. 46, 667–675.
Into, T., and Shibata, K. (2005). Apoptosis signal-regulating kinase 1-mediated sustained p38 mitogen-activated protein kinase activation regulates mycoplasmal lipoprotein- and staphylococcal peptidoglycan-triggered Toll-like receptor 2 signalling pathways. Cell. Microbiol. 7, 1305–1317. doi: 10.1111/j.1462-5822.2005.00558.x
Izac, J. R., Oliver, L. D. Jr., Earnhart, C. G., and Marconi, R. T. (2017). Identification of a defined linear epitope in the OspA protein of the Lyme disease spirochetes that elicits bactericidal antibody responses: implications for vaccine development. Vaccine 35, 3178–3185. doi: 10.1016/j.vaccine.2017.04.079
Jan, G., Brenner, C., and Wroblewski, H. (1996). Purification of Mycoplasma gallisepticum membrane proteins p52, p67 (pMGA), and p77 by high-performance liquid chromatography. Protein Expr. Purif. 7, 160–166. doi: 10.1006/prep.1996.0023
Jan, G., Fontenelle, C., Le Henaff, M., and Wroblewski, H. (1995). Acylation and immunological properties of Mycoplasma gallisepticum membrane proteins. Res. Microbiol. 146, 739–750. doi: 10.1016/0923-2508(96)81070-9
Jimbo, S., Suleman, M., Maina, T., Prysliak, T., Mulongo, M., and Perez-Casal, J. (2017). Effect of Mycoplasma bovis on bovine neutrophils. Vet. Immunol. Immunopathol. 188, 27–33. doi: 10.1016/j.vetimm.2017.04.011
Jordan, J. L., Berry, K. M., Balish, M. F., and Krause, D. C. (2001). Stability and subcellular localization of cytadherence-associated protein P65 in Mycoplasma pneumoniae. J. Bacteriol. 183, 7387–7391. doi: 10.1128/JB.183.24.7387-7891.2001
Kannan, T. R., and Baseman, J. B. (2006). ADP-ribosylating and vacuolating cytotoxin of Mycoplasma pneumoniae represents unique virulence determinant among bacterial pathogens. Proc. Natl. Acad. Sci. U.S.A. 103, 6724–6729. doi: 10.1073/pnas.0510644103
Kannan, T. R., Coalson, J. J., Cagle, M., Musatovova, O., Hardy, R. D., and Baseman, J. B. (2011). Synthesis and distribution of CARDS toxin during Mycoplasma pneumoniae infection in a murine model. J. Infect. Dis. 204, 1596–1604. doi: 10.1093/infdis/jir557
Kannan, T. R., Provenzano, D., Wright, J. R., and Baseman, J. B. (2005). Identification and characterization of human surfactant protein a binding protein of Mycoplasma pneumoniae. Infect. Immun. 73, 2828–2834. doi: 10.1128/IAI.73.5.2828-2834.2005
Kaufmann, A., Muhlradt, P. F., Gemsa, D., and Sprenger, H. (1999). Induction of cytokines and chemokines in human monocytes by Mycoplasma fermentans-derived lipoprotein MALP-2. Infect. Immun. 67, 6303–6308.
Kruger, T., and Baier, J. (1997). Induction of neutrophil chemoattractant cytokines by Mycoplasma hominis in alveolar type II cells. Infect. Immun. 65, 5131–5136.
Lam, K. M. (2002). The macrophage inflammatory protein-1beta in the supernatants of Mycoplasma gallisepticum-infected chicken leukocytes attracts the migration of chicken heterophils and lymphocytes. Dev. Comp. Immunol. 26, 85–93. doi: 10.1016/S0145-305X(01)00053-2
Leng, C. H., Liu, S. J., Chen, H. W., and Chong, P. (2015). Recombinant bacterial lipoproteins as vaccine candidates. Expert Rev. Vaccines 14, 1623–1632. doi: 10.1586/14760584.2015.1091732
Liu, Y. C., Lin, I. H., Chung, W. J., Hu, W. S., Ng, W. V., Lu, C. Y., et al. (2012). Proteomics characterization of cytoplasmic and lipid-associated membrane proteins of human pathogen Mycoplasma fermentans M64. PLoS One 7:e35304. doi: 10.1371/journal.pone.0035304
Martinez de Tejada, G., Heinbockel, L., Ferrer-Espada, R., Heine, H., Alexander, C., Barcena-Varela, S., et al. (2015). Lipoproteins/peptides are sepsis-inducing toxins from bacteria that can be neutralized by synthetic anti-endotoxin peptides. Sci. Rep. 5:14292. doi: 10.1038/srep14292
McGowin, C. L., Ma, L., Martin, D. H., and Pyles, R. B. (2009). Mycoplasma genitalium-encoded MG309 activates NF-kappaB via Toll-like receptors 2 and 6 to elicit proinflammatory cytokine secretion from human genital epithelial cells. Infect. Immun. 77, 1175–1181. doi: 10.1128/IAI.00845-08
Metaxas, E. I., Balis, E., Papaparaskevas, J., Spanakis, N., Tatsis, G., and Tsakris, A. (2015). Bronchiectasis exacerbations: the role of atypical bacteria, respiratory syncytial virus and pulmonary function tests. Can. Respir. J. 22, 163–166. doi: 10.1155/2015/783682
Mollazadeh, H., Cicero, A. F. G., Blesso, C. N., Pirro, M., Majeed, M., and Sahebkar, A. (2017). Immune modulation by curcumin: the Role of Interleukin-10. Crit. Rev. Food Sci. Nutr. doi: 10.1080/10408398.2017.1358139 [Epub ahead of print].
Muhlradt, P. F., and Frisch, M. (1994). Purification and partial biochemical characterization of a Mycoplasma fermentans-derived substance that activates macrophages to release nitric oxide, tumor necrosis factor, and interleukin-6. Infect. Immun. 62, 3801–3807.
Muhlradt, P. F., Kiess, M., Meyer, H., Sussmuth, R., and Jung, G. (1997). Isolation, structure elucidation, and synthesis of a macrophage stimulatory lipopeptide from Mycoplasma fermentans acting at picomolar concentration. J. Exp. Med. 185, 1951–1958. doi: 10.1084/jem.185.11.1951
Muhlradt, P. F., Quentmeier, H., and Schmitt, E. (1991). Involvement of interleukin-1 (IL-1), IL-6, IL-2, and IL-4 in generation of cytolytic T cells from thymocytes stimulated by a Mycoplasma fermentans-derived product. Infect. Immun. 59, 3962–3968.
Naot, Y., Merchav, S., Ben-David, E., and Ginsburg, H. (1979). Mitogenic activity of Mycoplasma pulmonis. I. stimulation of rat B and T lymphocytes. Immunology 36, 399–406.
Pantoja, L. G., Pettit, K., Dos Santos, L. F., Tubbs, R., and Pieters, M. (2016). Mycoplasma hyopneumoniae genetic variability within a swine operation. J. Vet. Diagn. Invest. 28, 175–179. doi: 10.1177/1040638716630767
Peng, H. H., Liang, S., Henderson, A. J., and Dong, C. (2007). Regulation of interleukin-8 expression in melanoma-stimulated neutrophil inflammatory response. Exp. Cell Res. 313, 551–559. doi: 10.1016/j.yexcr.2006.10.030
Pereyre, S., Goret, J., and Bebear, C. (2016). Mycoplasma pneumoniae: current knowledge on macrolide resistance and treatment. Front. Microbiol. 7:974. doi: 10.3389/fmicb.2016.00974
Rawadi, G., Ramez, V., Lemercier, B., and Roman-Roman, S. (1998). Activation of mitogen-activated protein kinase pathways by Mycoplasma fermentans membrane lipoproteins in murine macrophages: involvement in cytokine synthesis. J. Immunol. 160, 1330–1339.
Rawadi, G., and Roman-Roman, S. (1996). Mycoplasma membrane lipoproteins induced proinflammatory cytokines by a mechanism distinct from that of lipopolysaccharide. Infect. Immun. 64, 637–643.
Rosengarten, R., and Wise, K. S. (1990). Phenotypic switching in mycoplasmas: phase variation of diverse surface lipoproteins. Science 247, 315–318. doi: 10.1126/science.1688663
Ross, S. E., Simecka, J. W., Gambill, G. P., Davis, J. K., and Cassell, G. H. (1992). Mycoplasma pulmonis possesses a novel chemoattractant for B lymphocytes. Infect. Immun. 60, 669–674.
Rottem, S. (2003). Interaction of mycoplasmas with host cells. Physiol. Rev. 83, 417–432. doi: 10.1152/physrev.00030.2002
Saliba, R., Paasch, L., and El Solh, A. (2009). Tigecycline attenuates staphylococcal superantigen-induced T-cell proliferation and production of cytokines and chemokines. Immunopharmacol. Immunotoxicol. 31, 583–588. doi: 10.3109/08923970902838672
Salvatore, C. M., Techasaensiri, C., Tagliabue, C., Katz, K., Leos, N., Gomez, A. M., et al. (2009). Tigecycline therapy significantly reduces the concentrations of inflammatory pulmonary cytokines and chemokines in a murine model of Mycoplasma pneumoniae pneumonia. Antimicrob. Agents Chemother. 53, 1546–1551. doi: 10.1128/AAC.00979-08
Santona, A., Carta, F., Fraghi, P., and Turrini, F. (2002). Mapping antigenic sites of an immunodominant surface lipoprotein of Mycoplasma agalactiae, AvgC, with the use of synthetic peptides. Infect. Immun. 70, 171–176. doi: 10.1128/IAI.70.1.171-176.2002
Sawahata, R., Shime, H., Yamazaki, S., Inoue, N., Akazawa, T., Fujimoto, Y., et al. (2011). Failure of mycoplasma lipoprotein MALP-2 to induce NK cell activation through dendritic cell TLR2. Microbes Infect. 13, 350–358. doi: 10.1016/j.micinf.2010.12.003
Schmidt, J. A., Browning, G. F., and Markham, P. F. (2007). Mycoplasma hyopneumoniae mhp379 is a Ca2+-dependent, sugar-nonspecific exonuclease exposed on the cell surface. J. Bacteriol. 189, 3414–3424. doi: 10.1128/JB.01835-06
Shen, X., Gumulak, J., Yu, H., French, C. T., Zou, N., and Dybvig, K. (2000). Gene rearrangements in the vsa locus of Mycoplasma pulmonis. J. Bacteriol. 182, 2900–2908. doi: 10.1128/JB.182.10.2900-2908.2000
Shibata, K., Hasebe, A., Into, T., Yamada, M., and Watanabe, T. (2000). The N-terminal lipopeptide of a 44-kDa membrane-bound lipoprotein of Mycoplasma salivarium is responsible for the expression of intercellular adhesion molecule-1 on the cell surface of normal human gingival fibroblasts. J. Immunol. 165, 6538–6544. doi: 10.4049/jimmunol.165.11.6538
Shimizu, T. (2016). Inflammation-inducing factors of Mycoplasma pneumoniae. Front. Microbiol. 7:414. doi: 10.3389/fmicb.2016.00414
Shimizu, T., Kida, Y., and Kuwano, K. (2007). Triacylated lipoproteins derived from Mycoplasma pneumoniae activate nuclear factor-kappaB through toll-like receptors 1 and 2. Immunology 121, 473–483. doi: 10.1111/j.1365-2567.2007.02594.x
Shimizu, T., Kida, Y., and Kuwano, K. (2008). Mycoplasma pneumoniae-derived lipopeptides induce acute inflammatory responses in the lungs of mice. Infect. Immun. 76, 270–277. doi: 10.1128/IAI.00955-07
Shimizu, T., Kimura, Y., Kida, Y., Kuwano, K., Tachibana, M., Hashino, M., et al. (2014). Cytadherence of Mycoplasma pneumoniae induces inflammatory responses through autophagy and toll-like receptor 4. Infect. Immun. 82, 3076–3086. doi: 10.1128/IAI.01961-14
Simmons, W. L., Denison, A. M., and Dybvig, K. (2004). Resistance of Mycoplasma pulmonis to complement lysis is dependent on the number of Vsa tandem repeats: shield hypothesis. Infect. Immun. 72, 6846–6851. doi: 10.1128/IAI.72.12.6846-6851.2004
Simmons, W. L., and Dybvig, K. (2009). Mycoplasma biofilms ex vivo and in vivo. FEMS Microbiol. Lett. 295, 77–81. doi: 10.1111/j.1574-6968.2009.01592.x
Spuesens, E. B., Van De Kreeke, N., Estevao, S., Hoogenboezem, T., Sluijter, M., Hartwig, N. G., et al. (2011). Variation in a surface-exposed region of the Mycoplasma pneumoniae P40 protein as a consequence of homologous DNA recombination between RepMP5 elements. Microbiology 157, 473–483. doi: 10.1099/mic.0.045591-0
Sugiyama, M., Saeki, A., Hasebe, A., Kamesaki, R., Yoshida, Y., Kitagawa, Y., et al. (2016). Activation of inflammasomes in dendritic cells and macrophages by Mycoplasma salivarium. Mol. Oral. Microbiol. 31, 259–269. doi: 10.1111/omi.12117
Takeuchi, O., Sato, S., Horiuchi, T., Hoshino, K., Takeda, K., Dong, Z., et al. (2002). Cutting edge: role of Toll-like receptor 1 in mediating immune response to microbial lipoproteins. J. Immunol. 169, 10–14. doi: 10.4049/jimmunol.169.1.10
Waldo, R. H. III, and Krause, D. C. (2006). Synthesis, stability, and function of cytadhesin P1 and accessory protein B/C complex of Mycoplasma pneumoniae. J. Bacteriol. 188, 569–575. doi: 10.1128/JB.188.2.569-575.2006
Wang, Y., Wang, Q., Li, Y., Chen, Y., Shao, J., Nick, N., et al. (2017). Mmm-derived lipid-associated membrane proteins activate IL-1beta production through the NF-kappaB pathway via TLR2, MyD88, and IRAK4. Sci. Rep. 7:4349. doi: 10.1038/s41598-017-04729-y
Wayner, E. A., and Brooks, C. G. (1984). Induction of NKCF-like activity in mixed lymphocyte-tumor cell culture: direct involvement of mycoplasma infection of tumor cells. J. Immunol. 132, 2135–2142.
Wenzel, R. P., Craven, R. B., Davies, J. A., Hendley, J. O., Hamory, B. H., and Gwaltney, J. M. Jr. (1977). Protective efficacy of an inactivated Mycoplasma pneumoniae vaccine. J. Infect. Dis. 136(Suppl.), S204–S207. doi: 10.1093/infdis/136.Supplement.S204
Widjaja, M., Berry, I. J., Pont, E. J., Padula, M. P., and Djordjevic, S. P. (2015). P40 and P90 from Mpn142 are targets of multiple processing events on the Surface of Mycoplasma pneumoniae. Proteomes 3, 512–537. doi: 10.3390/proteomes3040512
Wu, Q., Martin, R. J., Rino, J. G., Breed, R., Torres, R. M., and Chu, H. W. (2007). IL-23-dependent IL-17 production is essential in neutrophil recruitment and activity in mouse lung defense against respiratory Mycoplasma pneumoniae infection. Microbes Infect. 9, 78–86. doi: 10.1016/j.micinf.2006.10.012
Wu, Y., Qiu, H., Zeng, Y., You, X., Deng, Z., Yu, M., et al. (2008). Mycoplasma genitalium lipoproteins induce human monocytic cell expression of proinflammatory cytokines and apoptosis by activating nuclear factor kappaB. Mediators Inflamm. 2008:195427. doi: 10.1155/2008/195427
Xiong, Q., Zhang, B., Wang, J., Ni, B., Ji, Y., Wei, Y., et al. (2016). Characterization of the role in adherence of Mycoplasma hyorhinis variable lipoproteins containing different repeat unit copy numbers. Vet. Microbiol. 197, 39–46. doi: 10.1016/j.vetmic.2016.10.025
Xu, F., Zhang, C., Zou, Z., Fan, E. K. Y., Chen, L., Li, Y., et al. (2017). Aging-related Atg5 defect impairs neutrophil extracellular traps formation. Immunology 151, 417–432. doi: 10.1111/imm.12740
Yang, J., Hooper, W. C., Phillips, D. J., and Talkington, D. F. (2002). Regulation of proinflammatory cytokines in human lung epithelial cells infected with Mycoplasma pneumoniae. Infect. Immun. 70, 3649–3655. doi: 10.1128/IAI.70.7.3649-3655.2002
Yeh, J. J., Wang, Y. C., Hsu, W. H., and Kao, C. H. (2016). Incident asthma and Mycoplasma pneumoniae: a nationwide cohort study. J. Allergy Clin. Immunol. 137:1017-1023.e6. doi: 10.1016/j.jaci.2015.09.032
Keywords: mycoplasma, lipoproteins, immune system, immune modulation, inflammation
Citation: Christodoulides A, Gupta N, Yacoubian V, Maithel N, Parker J and Kelesidis T (2018) The Role of Lipoproteins in Mycoplasma-Mediated Immunomodulation. Front. Microbiol. 9:1682. doi: 10.3389/fmicb.2018.01682
Received: 22 October 2017; Accepted: 05 July 2018;
Published: 31 July 2018.
Edited by:
Amy Rasley, Lawrence Livermore National Laboratory (DOE), United StatesReviewed by:
M. Victoria Delpino, National Scientific and Technical Research Council (CONICET), ArgentinaHridayesh Prakash, All India Institute of Medical Sciences, India
Copyright © 2018 Christodoulides, Gupta, Yacoubian, Maithel, Parker and Kelesidis. This is an open-access article distributed under the terms of the Creative Commons Attribution License (CC BY). The use, distribution or reproduction in other forums is permitted, provided the original author(s) and the copyright owner(s) are credited and that the original publication in this journal is cited, in accordance with accepted academic practice. No use, distribution or reproduction is permitted which does not comply with these terms.
*Correspondence: Theodoros Kelesidis, dGtlbGVzaWRpc0BtZWRuZXQudWNsYS5lZHU=