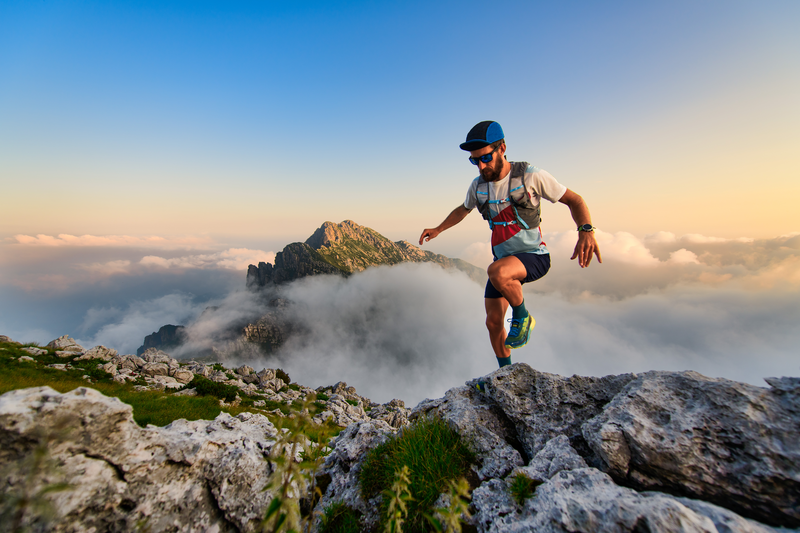
94% of researchers rate our articles as excellent or good
Learn more about the work of our research integrity team to safeguard the quality of each article we publish.
Find out more
ORIGINAL RESEARCH article
Front. Microbiol. , 19 July 2018
Sec. Terrestrial Microbiology
Volume 9 - 2018 | https://doi.org/10.3389/fmicb.2018.01543
Nutrient inputs to forest ecosystems significantly influence aboveground plant community structure and ecosystem functioning. However, our knowledge of the influence of nitrogen (N) and/or phosphorus (P) inputs on belowground microbial communities in subtropical forests is still unclear. In this study, we used quantitative polymerase chain reaction and Illumina Miseq sequencing of the bacterial 16S rRNA gene to investigate bacterial abundance, diversity, and community composition in a Chinese fir plantation. The fertilization regimes were as follows: untreated control (CK), P amendment (P), N amendment (N), and N with P amendment (NP). Additions of N decreased soil pH and bacterial 16S rRNA gene abundance by 3.95 (from 4.69 to 3.95) and 3.95 × 109 copies g−1 dry soil (from 9.27 × 109 to 3.95 × 109 g−1 dry soil), respectively. Bacterial richness and diversity decreased with N addition (N and NP) rather than only P input. Proteobacteria, Acidobacteria, and Actinobacteria were the major phylum across all treatments. Nitrogen addition increased the relative abundance of Proteobacteria and Actinobacteria by 42.0 and 10.5%, respectively, while it reduced that of Acidobacteria by 26.5%. Bacterial community structure in the CK and P treatments was different from that in the N and NP treatments upon principle coordinates analysis. Phosphorus addition did not significantly affect soil bacterial communities, and no interactions between N and P inputs on microbial traits were observed. Soil pH and mineral N availability appeared to have a cooperative effect on bacterial abundance and community structure, with soil pH being the key influencing factor by canonical correspondence analysis. These results indicate that inorganic N rather than P fertilization affected both bacterial abundance and community composition in subtropical forests.
Soil nutrient inputs such as nitrogen (N) and phosphorus (P) are critical to control plant growth in tropical forest plantations (Chen et al., 2015). Because of high amounts of fossil fuels burning and overuse of chemical fertilizers in agricultural production, N deposition has been increasing significantly in the past 100 years (IPCC, 2013), especially in the warm and humid climatic zone in Asia (Dentener et al., 2006). Nitrogen is considered as a limiting factor that influences the plant biodiversity and primary productivity in forest ecosystems (Bobbink et al., 2010; Weand et al., 2010). In addition, chronic elevated N input has been shown to lead to many adverse impacts, including soil acidification (Guo et al., 2010; Mao et al., 2017), nutrients imbalance (Lu et al., 2010), and increased greenhouse gas emissions (IPCC, 2007). The soils in Chinese subtropical forests are highly weathered because of high temperature and precipitation, resulting in a large amount of available P being lost (Li et al., 2014; Cui et al., 2017). Thus, P is always scarce in subtropical forests (Zhao et al., 2014; Tang et al., 2016). Moreover, N input may result in element imbalance and further aggravate P limitation in the forest ecosystem (Tang et al., 2016). The continuous anthropogenic P additions are essential to improve forest primary production and little decomposition in forest ecosystems (Cleveland et al., 2006; Kaspari et al., 2008). Nitrogen and phosphorus additions may impact the abundance, diversity, and community composition of soil microbes, which play an important role in regulating soil fertility (van der Heijden et al., 2008). However, effects of repeated N and P additions on belowground ecosystems such as soil bacterial communities in subtropical forest ecosystems remain poorly understood.
Soil microorganisms are important drivers of energy flow and nutrient cycling, such as carbon (C), N, and P cycling in terrestrial ecosystems (Artursson et al., 2006; Morris and Blackwood, 2015). Therefore, soil functioning always depends primarily on microbial community structure, activity, and stability (Dunbar et al., 2002; Coleman and Whitman, 2005). Soil microorganisms are sensitive to various changes in soil nutrients and pH (Mele and Crowley, 2008; Zhong et al., 2010). It is well known that soil bacterial communities can be a potential ecological indicator of soil quality (Bending et al., 2004). Studying the feedback of soil microorganisms to added N and/or P is very important to understand the effects of global changes on ecosystem processes regulated by soil biota. The influence of N inputs on soil bacterial community structure have been well studied; however, the results of these studies have been inconsistent (Cui et al., 2017; Nie et al., 2018). For example, Freedman et al. (2015) reported that neither the total nor active bacterial community was influenced by N enrichment. However, Nie et al. (2018) found that high N application strongly shaped bacterial community structure and that ammonium availability, rather than pH or nitrate concentration, was a key environmental parameter determining this shaping. These inconsistent results indicate that the responses of soil microbial communities to N addition are highly variable in different forest ecosystems. However, only few studies were conducted to study the response of soil bacterial communities to P amendment in subtropical forest ecosystems. Furthermore, in the context of the increased nitrogen deposition, we also need to understand the influence of P fertilization on soil microbial communities in forest ecosystem that receive exogenous N inputs.
The Chinese fir (Cunninghamia Lanceolata), which covers over 9 million ha in China, is the most common coniferous timber species that has been extensively planted in southern China (Tang et al., 2016). In order to improve soil quality, conservation, and enhanced the productivity of Chinese fir plantations, a large number of measures have been applied, among which fertilization is the most effective and feasible (Zhang et al., 2004). A number of field studies have focused on the impacts of nutrients on soil C and N sequestration in Chinese fir plantations (Wei et al., 2012; Fan et al., 2014), but few studies have investigated the belowground soil microbial properties.
To better understand how N, P, and NP additions influence bacterial abundance, diversity, and composition in subtropical forests, we set up a N and/or P amendment trial in a Chinese fir plantation. The specific objectives of this study were to (1) assess the responses of bacterial abundance, diversity, and community composition to N and/or P addition; (2) identify which soil properties were correlated with bacterial community. We hypothesized that (1) the changes in soil pH and mineral N availability induced by N addition might affect bacterial community abundance, diversity, and composition and (2) the increased P content after P addition might not affect these variables of soil bacterial communities.
This study was conducted in a rehabilitated secondary forest located at the Qianyanzhou Experimental Station, Chinese Academy of Sciences, Taihe County, Jiangxi province, southern China (26°44′52″N, 115°04′13″E). The soil in the forest, which is classified as Typical Hapludult Ultisols (locally “red soil”), developed from Quaternary Red Clay and covers over 60% of the 1.14 million km2 of total land area in southern China. The site has a monsoon season and humid climate. The duration of the growing season is about 270 days, and the average annual precipitation and temperature are 1471.2 mm and 17.9°C, respectively (Dong et al., 2015). The annual clear-sky duration and solar radiation are 1306 h and 4349 MJ/m2, respectively.
In 2011, 16 experimental plots (20 m × 20 m each) were placed in a random manner with 10-m-wide buffer strip between plots. Four fertilization treatments (four replicates each) were set up in this fir plantation: without fertilizer (CK), P addition (P) (50 kg P ha−1 year−1), N addition (N) (100 kg N ha−1 year−1), and N and P addition (NP) (100 kg N ha−1 year−1 + 50 kg P ha−1 year−1). Nitrogen and phosphorus fertilizer were applied as NH4NO3 and NaH2PO4, respectively. Nitrogen and and/or phosphorus fertilizer were dissolved in 20 L of distilled water and, then, added into the corresponding plots. Twenty liters of distilled water was added into the control plots.
Soil cores were collected in late August of 2017. From each replicate plot, 10 subsamples (0–20 cm) were collected using corers, after which they were pooled together to minimize within-plot variation. Subsequently, soil samples were mixed thoroughly and then passed through a 2-mm sieve and separated into two portions. One portion was used for soil chemical analysis and another that was frozen at −80°C for subsequent molecular analysis. The soil pH (water:soil, 5:1) was determined using a pH meter. Soil KCl-extractable NH4+-N and NO3−-N were extracted using a ratio of 1:5 (fresh soil: 2 M KCl, w/v) by shaking at 200 rpm for 1 h, and quantified by a Segmented Flow Analyzer (SAN++, Skalar, Holland). Dissolved organic carbon (DOC) was extracted by 0.5 M K2SO4 and determined using a total organic carbon analyzer (Multi N/C 3000; Analytik, Jena, Germany; Watkins et al., 1987). Total nitrogen (TN) was determined using Elemental Analyzer (Elemental Analyzer, Germany). Available phosphorus was extracted using sodium bicarbonate and then measured by the molybdenum-blue method.
The total DNA was isolated from 0.4 g of soil using MoBio PowersoilTM DNA Isolation Kit (Carlsbad, CA, United States). DNA concentration was checked using a NanoDrop ND-1000 spectrophotometry (United States), then DNA samples were stored at −80°C refrigerator for further analysis.
To estimate the bacterial abundance, quantitative polymerase chain reaction (qPCR) assays were performed using universal eubacterial 16S rRNA gene primers. The forward primer was 338F (5′-ACTCCTACGGGAGGCAGCA-3′), and the reverse primer was 806R (5′-GGACTACHVGGGTWTCTAAT-3′; Fierer et al., 2005). The abundance of bacterial 16S rRNA gene was determined on an ABI7500 (ABI, United States). The 25 μL reaction mixture included 12.5 μL of SYBR Premix Ex TaqTM (TakaRa Biotechnology, Dailian, China), 0.5 μL of each primer (10 mM), 1–10 ng of template DNA. The PCR condition was as follows: 3 min at 95°C, 35 cycles of 40 s at 95°C, 30 s at 54°C, 40 s at 72°C, and a plate read at 83°C for 10 s. The qPCR assay was performed in triplicate for each replicate. The amplification efficiency for all samples ranged between 91 and 100%.
The V3-V4 region of the bacterial 16S rRNA gene was amplified from DNA extracts using the same primers for qPCR. The adapter linked forward primer included a 5-bp barcode was used for sample identification. Each PCR mixture (25 μL) included 0.5 μL of each primer, 2 μL template DNA (1–10 ng), 12.5 μL of Premix TaqTM (2×) (Takara), and 9.5 μL of ddH2O. The thermocycling conditions consisted of initial denaturation at 94°C for 5 min, followed by 30 cycles of denaturation at 94°C for 40 s, annealing at 54°C for 30 s, and elongation at 72°C for 6 min. PCR products were purified with a QIA quick Gel Extraction kit (QIAGEN, Germany). The high-throughput sequencing was conducted on the Illumina MiSeq 2500 platform (Illumina, San Diego, CA, United States).
Sequence data including raw data and clean data were merged and filtered using Mothur (Schloss et al., 2009). Briefly, the raw sequences were sorted and distinguished by unique 5-bp barcodes, and those shorter than 300 bp were removed. The barcodes and primer sequences were then trimmed (Supplementary Table S2), after which the remaining high-quality sequences with ≥97% similarity were clustered into operational taxonomic units (OTUs; Edgar, 2013). Representative sequences for each OTU were then assigned to taxonomy by the Ribosomal Database Project (RDP) classifier (Wang et al., 2007). All the sequence data were deposited into the NCBI Sequence Read Archive database under accession numbers SRR6263277-SRR6263292. Observed OTU numbers, Pielou’s evenness, Chao1 richness, and ACE evenness, as well as Shannon’s diversity indices, were calculated using the Mothur software to estimate bacterial alpha diversity. Beta diversity was calculated based on Bray-Curtis distance matrices.
One-way ANOVA was conducted to compare the effects of different fertilization treatments on bacterial 16S rRNA gene abundance and alpha diversity indices using SPSS version 19.0 (SPSS, Chicago, IL, United States). The mean values of four replicate plots were compared using Duncan’s test when a significant F-value was obtained (p < 0.05). On the effects of N, P, and their interactions on soil properties, bacterial 16S rRNA gene abundance, and alpha diversity indices were examined by two-way ANOVA. Spearman’s correlation coefficient was used to test the relationships among soil properties, bacterial 16S rRNA gene abundance, alpha diversity indices, and abundant phyla. A p < 0.05 was considered to indicate significance.
Principle coordinates analysis (PCoA) was conducted to assess the dissimilarities of the bacterial community composition between the treatments using R 3.4 software (R Core Team, 2013). Per-mutational multivariate ANOVA (PERMANOVA) was further conducted to estimate the effects of N with P addition and their interactions on the composition of bacterial communities. A Mantel test was conducted to assess the correlation between specific soil properties and bacterial community composition. The canonical correspondence analysis (CCA) was performed to explore the effects of soil properties on bacterial community composition.
The 6-year fertilization management significantly altered soil properties such as pH, NH4+-N, NO3−-N, TN, and AP contents. These results indicated that pH remarkably decreased in response to N amendment, while was not significantly altered after P amendment (Table 1). The concentrations of NH4+-N, NO3−-N, and TN were significantly lower in both the CK and P treatments than the N addition treatments. However, the increases in NH4+-N, NO3−-N, and TN concentrations did not significantly differ between N and NP treatments (p > 0.05). Soil AP content was significantly elevated by P addition. Two-way ANOVA revealed no significant interactions between N and P additions on the soil properties (p > 0.05).
TABLE 1. Effects N and/or P addition on soil properties under CK (no fertilizer), P fertilization (P), N fertilization (N), and NP fertilization (NP) treatments.
The 6-year N and P inputs affected bacterial abundance as estimated based on qPCR of the bacterial 16S rRNA gene (Figure 1). Specifically, the abundance of bacterial 16S rRNA gene ranged from 3.95 × 109 to 9.27 × 109 copies g−1 dry soil. The bacterial abundance in the N and NP treatments was lower than in CK and P treatments, whereas no significant differences were observed in the CK and P treatments. 16S rRNA gene copies numbers were positively correlated with soil pH (p < 0.001), while negatively correlated with soil NH4+-N, NO3−-N, and TN (p < 0.001; Supplementary Table S1).
FIGURE 1. Abundance of bacteria as indicated by 16S rRNA gene copies examined by real-time PCR. Same letters above columns indicate no significant difference (p < 0.05). CK, no fertilizer; P, phosphorus input; N, nitrogen input; NP, nitrogen and phosphorus input.
Bacterial community analysis of the 16 soil samples revealed a total of 493,859 high quality sequences with 25,504 to 41,155 sequences per sample (Supplementary Table S2). To compare soil bacterial community diversity among all soils, the same survey effort level of 25,000 sequences were randomly selected from each sample in the sequencing library. The alpha diversity of the bacterial community was then examined using various estimators of richness and diversity (Table 2). There were significant differences in bacterial alpha diversity for the observed OTU numbers, as well as Chao 1, ACE, and Shannon’s index values among treatments, but not for Pielou’s Evenness among the treatments. Pielou’s evenness was high among treatments, indicating that bacterial community structure was evenly distributed. The observed OTU numbers, Chao 1, ACE, and Shannon’s index values were much lower in the N and NP treatments than in the CK treatment. Moreover, there were no significant (p > 0.05) differences in these alpha diversity indexes between CK and P addition treatments.
The correlations of bacterial alpha diversity indices with soil physicochemical parameters are shown in Table 3. The values of observed OTU numbers, Chao1, ACE, and Shannon’s index were positively correlated with soil pH (p < 0.01), but were significantly and negatively correlated with NH4+-N, NO3−-N, and TN contents (p < 0.05).
All reads in each treatment were classified into eight phyla, and their relative abundances were shown in Figure 4A. The phyla Acidobacteria and Proteobacteria were most abundant in all treatments, comprising 67.1–74.3% of the bacterial sequences obtained from the soil, followed by Chloroflexi (8.9–9.8%), Actinobacteria (4.9–10.5%), Verrucomicrobia (2.1–2.9%), Planctomycetes (1.3–2.2%), and Bacteroidetes (1.7–2.1%). Different fertilization management strategies had various influences on bacterial composition at the phylum level. The relative abundance of the phyla Actinobacteria and Proteobacteria increased in N and NP treatments, while that of Acidobacteria decreased, relative to the CK and P treatments. There were no significant differences in the abundance of Acidobacteria, Actinobacteria, Proteobacteria, Chloroflexi, Actinobacteria, Planctomycetes, Bacteroidetes, and Verrucomicrobia between N and NP treatments. Similarly, the relative abundance of these phyla did not differ significantly between CK and P treatments (p > 0.05).
At class level, the 13 most abundant classes were obtained (>2%) among fertilizer treatments (Figure 4B). There were significant differences between treatments for the four most abundant classes. Specifically, the relative abundance of class Acidobacteria was higher in the CK and P treatments than that in the N-related treatments. Furthermore, the relative abundance of Actinobacteria, Alphaproteobacteria, and Gammaproteobacteria significantly increased in the N and NP treatments.
The variations in bacterial communities caused by N and/or P input were evaluated by PCoA (Figure 2), which revealed that bacterial community composition separated clearly between N and CK treatments, while the bacterial community composition in the P treatment was similar to that in the CK treatment. The first two principal coordinates explained 31.8% (PC1) and 11.6% (PC2) of the variation in bacterial communities, respectively. PERMANOVA further revealed significant differences between N-free treatments (CK and P) and N-containing treatments (N and NP) (p < 0.01; Supplementary Table S2).
FIGURE 2. Principle coordinate analysis (PCoA) to visualize the overall differences in bacterial community composition across the all treatments. CK, no fertilizer; P, phosphorus input; N, nitrogen input; NP, nitrogen and phosphorus input.
The Mantel test indicated that bacterial community structure was closely correlated with soil chemical properties including pH, NH4+-N, NO3−-N, and TN (p < 0.01), and the correlation coefficients followed the trend: pH > NH4+-N > NO3−-N > TN (Supplementary Table S4). The effects of these soil properties on the bacterial community structure were further analyzed using CCA (Figure 3), which revealed that these soil variables explained 27.6% of the variation in the bacterial community by the first two constrained axes of CCA, with the first axis explaining 20.3% and the second 7.3%. Moreover, CCA clearly showed that soil pH and mineral N contents were the most important contributors to the variation in bacterial communities, and the direction of these arrows was closely correlated with the X axis.
FIGURE 3. Canonical correspondence analysis (CCA) illustrating the effects of soil properties on bacterial community structure. CK, no fertilizer; P, phosphorus input; N, nitrogen input; NP, nitrogen and phosphorus input.
We also evaluated the correlations between relative abundant phyla (>2%) and soil properties (Table 4). Three dominant phyla (Acidobacteria, Proteobacteria, and Actinobacteria) were significantly correlated with several soil chemical parameters (p < 0.05), while Bacteroidetes, Verrucomicrobia, Chloroflexi, and Planctomycetes were not obviously related to any of the soil chemical properties (p > 0.05). Only Acidobacteria was positively correlated with soil pH, whereas Actinobacteria and Proteobacteria were negatively correlated with this factor (p < 0.05). Moreover, Acidobacteria was negatively related to NH4+-N, NO3−-N, and TN, while Actinobacteria and Proteobacteria were positively correlated with these soil properties.
TABLE 4. Spearman’s correlation coefficients between soil chemical characteristics and abundant phyla (relative abundance > 2%).
The applications of inorganic N were previously believed to reduce microbial abundance and biodiversity (He et al., 2007; Geisseler and Scow, 2014; Zhou et al., 2015; Ling et al., 2017; Wang et al., 2017; Nie et al., 2018). Similarly, N amendment (such as N and NP treatments) significantly decreased bacterial 16S rRNA gene abundance and biodiversity (Figure 1 and Table 2). Conversely, long-term P input did not affect bacterial abundance and α-diversity, which was supported by the result of recent studies (Huang et al., 2016; Liu et al., 2018). Huang et al. (2016) reported that the abundance of most groups of soil microbial community (bacteria, fungal, and AMF) was not affected by the low (5 g P m−2 year−1) and medium (5 g P m−2 year−1) P inputs. Additionally, Liu et al. (2018) found that bacterial Chao1 and Shannon indices were not strongly influenced by P amendment. Thus, our results conclude that P availability may not be the limiting factor affecting bacterial abundance and biodiversity in this Chinese fir plantation. Changes of bacterial 16S rRNA gene abundance and α-diversity might be mainly associated with the soil pH, as soil pH was kept at a relative stable level in the P treatment, while it decreased remarkably under N-related treatments. Particularly, the spearman’s correlation analysis showed that bacterial 16S rRNA gene abundance, and α-diversity indices were strongly correlated with soil pH in the present study, indicating that soil pH was a decisive factor affecting them. Moreover, the bacterial community was significantly influenced by soil pH because most bacterial taxa showed relatively narrow growth tolerances, especially within the pH range of 4–7 (Rousk et al., 2010; Dai et al., 2018). Additionally, bacterial 16S rRNA gene abundance and α-diversity were closely related to NH4+-N and NO3−-N rather than AP, further confirming that mineral N availability was a very important factor altering bacterial abundance and biodiversity (Zhou et al., 2015; Nie et al., 2018; Wang et al., 2018). Therefore, we concluded that the change of bacterial abundance and biodiversity among the all treatments could be attributed to the direct influence of N fertilizer as nutrients and indirect effects of soil acidification caused by N input.
In the present study, high-throughput sequencing analysis indicated that Proteobacteria, Acidobacteria, Chloroflexi, and Actinobacteria were the predominant bacterial phyla in the acidic forest soil, which was in agreement with the results of previous studies (Sun et al., 2015; Cui et al., 2017; Nie et al., 2018). Our results revealed that N input increased the relative abundance of Proteobacteria and Actinobacteria and reduced the relative abundance of Acidobacteria. These findings were supported by the copiotrophic hypothesis (Fierer et al., 2007), in which copiotrophic groups (e.g., Proteobacteria and Actinobacteria) that had fast growth rates were more likely to increase in nutrient-rich conditions, while oligotrophic groups (e.g., Acidobacteria and Chloroflexi) that had slower growth rates would likely decline (Fierer et al., 2012). Proteobacteria and Actinobacteria have been reported to be related to the high carbon availability, and these organisms exhibit relatively rapid growth rates. Meanwhile, Acidobacteria belongs to the group of oligotrophic bacteria found in nutrient poor and very acidic environments and have the ability to degrade complex and recalcitrant carbon compounds (Ai et al., 2015). However, P input did not follow this rule to affect the relative abundances of selected oligotrophic and copiotrophic taxa, which was consistent with recent study reported by Wakelin et al. (2017). We concluded soil available phosphorus content was not sufficient to affect bacterial community in our study. When soil available phosphorus content was ≥100 mg kg−1, soil-borne copiotrophic bacteria members became dominant (Kuramae et al., 2011). However, soil available phosphorus content was not reached in our study and the red soil was different from that by Kuramae et al. (2011). Additionally, the KEGG Orthology (K) numbers showed a significant difference among the fertilizer treatments using functional predictions analysis (Supplementary Figure S1). 16S rRNA gene-predicted functional structure was more affected by N addition than P input. Some functions related to amino acid metabolism, carbohydrate metabolism, energy metabolism, and xenobiotics biodegradation and metabolism were reduced in samples receiving inorganic N addition. Thus, the different response of major phyla to N and P input might be reason for the change in bacterial community structure.
Long-term inorganic fertilization, especially N fertilizer, usually changes soil microbial community structure in previous studies (Zhou et al., 2015, 2017; Nie et al., 2018). Similarly, N input altered bacterial community structure in our study (Figure 4). Bacterial community composition in the N and NP treatments differed significantly from those not receiving N (CK and P), indicating that fertilizer addition had a stronger influence on it than P input. On the contrary, P input did not influence bacterial community structure in the present study, which was supported by previous reports (Huang et al., 2016; Liu et al., 2018). Huang et al. (2016) and Liu et al. (2018) observed no changes in soil bacterial community composition among the various P treatments by phospholipid fatty acids (PFLA) and high throughput sequencing analyses, respectively. In addition, Ling et al. (2017) and Leff et al. (2015) found that high N availability, affected by N input, was a key factor influencing shifts in bacterial community structure. Nitrogen fertilizer directly impacted mineral N availability, which were the key factors altering bacterial community composition (Figure 3), as revealed in previous studies (Zhong et al., 2015; Nie et al., 2018). Taken together, these findings indicate that soil mineral N availability, which depends on N input, is essential to the bacterial community structure.
FIGURE 4. Relative abundances of the most abundance bacterial groups in different treatments at the phyla (A) and class (B) levels. Others represented unclassified groups. Data are means ± standard deviation (n = 4). Significance is indicated by ∗∗p < 0.01. CK, no fertilizer; P, phosphorus input; N, nitrogen input; NP, nitrogen and phosphorus input.
In addition to soil N availability, pH is frequently considered to be another important factor in controlling bacterial community structure and is considered a good predictor of bacterial community composition (Geisseler and Scow, 2014; Li et al., 2014), which was also observed in the present sturdy. Nitrogen rather than P inputs led to decrease in soil pH, and soil pH was more important than other nutrients in shaping bacterial community structure according to the Mantel test and CCA analysis, which is supported by previous studies (Zhou et al., 2015; Xun et al., 2016; Wang et al., 2018). Moreover, the most abundant phyla were more tightly related to pH than other nutrients (Supplementary Table S3). These results further supported that the changes in soil pH caused by the application of N fertilizer were more important than available nutrients on bacterial community structure.
Soil chemical properties and bacterial community were markedly influenced by N and/or P input in a subtropical fir plantation in southern China. Our results showed that N input influenced bacterial abundance and community composition while P input did not. The shift in soil pH induced by of the application of N alone and N plus P fertilizer must be a decisive factor in determining bacterial abundance and community structure. In addition to soil pH, soil mineral N availability also appeared to change bacterial community structure. Our findings indicated that N input reduced bacterial abundance and diversity, and that the impacts of N amendment (N and NP) treatments were stronger than treatments that did not contain (P). This study provides valuable information that improves our understanding of the effects of N and/or P input on underground bacterial community and revealed the main factors influencing bacterial communities in subtropical forest ecosystems.
QW and CW contributed to the design and implementation of the research, participated in drafting the manuscript. WY contributed to analyze the results. AT, DC, YH, JA, YJ, and ZH revised the manuscript.
This work was supported by GDAS’ special Project of Science and Technology Development (2018GDASCX-0925), Construction of Innovative Ability of Modern Sugarcane Breeding (2014B070705002) and Construction of Innovative Ability of resource recycling and drug fertilizer industry development (2017A070701030).
The authors declare that the research was conducted in the absence of any commercial or financial relationships that could be construed as a potential conflict of interest.
The Supplementary Material for this article can be found online at: https://www.frontiersin.org/articles/10.3389/fmicb.2018.01543/full#supplementary-material
Ai, C., Liang, G., Sun, J., Wang, X., He, P., Zhou, W., He, X. (2015). Reduced dependence of rhizosphere microbiome on plant-derived carbon in 32-year long-term inorganic and organic fertilized soils. Soil Biol. Biochem. 80, 70–78. doi: 10.1016/j.soilbio.2014.09.028
Artursson, V., Finlay, R. D., and Jansson, J. K. (2006). Interactions between arbuscular mycorrhizal fungi and bacteria and their potential for stimulating plant growth. Environ. Microbiol. 8, 1–10. doi: 10.1111/j.1462-2920.2005.00942.x
Bending, G. D., Turner, M. K., Rayns, F., Marx, M. C., and Wood, M. (2004). Microbial and biochemical soil quality indicators and their potential for differentiating areas under contrasting agricultural management regimes. Soil Biol. Biochem. 36, 1785–1792. doi: 10.1016/j.soilbio.2004.04.035
Bobbink, R., Hicks, K., Galloway, J., Spranger, T., Alkemade, R., Ashmore, M., et al. (2010). Global assessment of nitrogen deposition effects on terrestrial plant diversity: a synthesis. Ecol. Appl. 20, 30–59. doi: 10.1890/08-1140.1
Chen, F. S., Niklas, K. J., Liu, Y., Fang, X. M., Wan, S. Z., and Wang, H. M. (2015). Nitrogen and phosphorus additions alter nutrient dynamics but not resorption efficiencies of Chinese fir leaves and twigs differing in age. Tree Physiol. 35, 1106–1117. doi: 10.1093/treephys/tpv076
Cleveland, C. C., Reed, S. C., and Townsend, A. R. (2006). Nutrient regulation of organic matter decomposition in a tropical rain forest. Ecology 87, 492–503. doi: 10.1890/05-0525
Coleman, D. C., and Whitman, W. B. (2005). Linking species richness, biodiversity and ecosystem function in soil systems. Pedobiologia 49, 479–497. doi: 10.1016/j.pedobi.2005.05.006
Cui, J., Wang, J. J., Xu, J., Xu, C. H., and Xu, X. N. (2017). Changes in soil bacterial communities in an evergreen broad-leaved forest in east China following 4 years of nitrogen addition. J. Soils Sediments 17:2156. doi: 10.1007/s11368-017-1671-y
Dai, Z. M., Su, W. Q., Chen, H. H., Barberán, A., Zhao, H. C., Yu, M. J., et al. (2018). Long-term nitrogen fertilization decreases bacterial diversity and favors the growth of Actinobacteria and Proteobacteria in agro-ecosystems across the globe. doi: 10.1111/gcb.14163
Dentener, F., Drevet, J., Lamarque, J. F., Bey, I., Eickhout, B., Fiore, A. M., et al. (2006). Nitrogen and sulfur deposition on regional and global scales: a multimodel evaluation. Glob. Biogeochem. Cycles 20, 16615. doi: 10.1029/2005GB002672
Dong, W. Y., Zhang, X. Y., Liu, X. Y., Fu, X. L., Chen, F. S., and Wang, H. M. (2015). Responses of soil microbial communities and enzyme activities to nitrogen and phosphorus additions in Chinese fir plantations of subtropical China. Biogeosciences 12, 5537–5546. doi: 10.5194/bg-12-5537-2015
Dunbar, J., Barns, S. M., Ticknor, L. O., and Kuske, C. R. (2002). Empirical and theoretical bacterial diversity in four Arizona soils. Appl. Environ. Microbiol. 68, 3035–3045. doi: 10.1128/AEM.68.6.3035-3045.2002
Edgar, R. C. (2013). UPARSE: highly accurate OTU sequences from microbial amplicon reads. Nat. Methods 10, 996–998. doi: 10.1038/nmeth.2604
Fan, H. B., Wu, J. P., Liu, W. F., Yuan, Y. H., Huang, R. Z., Liao, Y. C., et al. (2014). Nitrogen deposition promotes ecosystem carbon accumulation by reducing soil carbon emission in a subtropical forest. Plant Soil 379, 361–371. doi: 10.1007/s11104-014-2076-y
Fierer, N., Jackson, J. A., Vilgalys, R., and Jackson, R. B. (2005). Assessment of soil microbial community structure by use of taxon-specific quantitative pcr assays. Appl. Environ. Microbiol. 71, 4117–4120. doi: 10.1128/AEM.71.7.4117-4120.2005
Fierer, N., Bradford, M. A., and Jackson, R. B. (2007). Toward an ecological classification of soil bacteria. Ecology 88, 1354–1364. doi: 10.1890/05-1839
Fierer, N., Lauber, C. L., Ramirez, K. S., Zaneveld, J., Bradford, M. A., and Knight, R. (2012). Comparative metagenomic, phylogenetic and physiological analyses of soil microbial communities across nitrogen gradients. ISME J. 6, 1007–1017. doi: 10.1038/ismej.2011.159
Freedman, Z. B., Romanowicz, K. J., Upchurch, R. A., and Zak, D. R. (2015). Differential responses of total and active soil microbial communities to long-term experimental N deposition. Soil Biol. Biochem. 90, 275–282. doi: 10.1016/j.soilbio.2015.08.014
Geisseler, D., and Scow, K. M. (2014). Long-term effects of mineral fertilizers on soil microorganisms-A review. Soil Biol. Biochem. 75, 54–63. doi: 10.1016/j.soilbio.2014.03.023
Guo, J. H., Liu, X. J., Zhang, Y., Shen, J. L., Han, W. X., Zhang, W. F., et al. (2010). Significant acidification in major Chinese croplands. Science 327, 1008–1010. doi: 10.1126/science.1182570
He, J. Z., Shen, J. P., Zhang, L. M., Zhu, Y. G., Zheng, Y. M., Xu, M. G., et al. (2007). Quantitative analyses of the abundance and composition of ammonia-oxidizing bacteria and ammonia-oxidizing archaea of a Chinese upland red soil under long-term fertilization practices. Environ. Microbiol. 9, 2364–2374. doi: 10.1111/j.1462-2920.2007.01358.x
Huang, J., Hu, B., Qi, K., Chen, W., Pang, X., Bao, W., et al. (2016). Effects of phosphorus addition on soil microbial biomass and community composition in a subalpine spruce plantation. Eur. J. Soil Biol. 72, 35–41. doi: 10.1016/j.ejsobi.2015.12.007
IPCC (2007). “Climate change 2007: the physical science basis,” in Contribution of Working Group I to the Fourth Assessment Report of the Intergovernmental Panel on Climate Chang, eds S. Solomon, D. Qin, and M. Manning (Cambridge: Cambridge University Press).
IPCC (2013). “Climate change 2013: the physical science basis,” in Contribution of Working Group I to the Fifth Assessment Report of the Intergovernmental Panel on Climate Change, eds T. F. Stocker, D. Qin, G. K. Plattner, M. Tignor, S. K. Allen, J. Boschung, et al. (Cambridge: Cambridge University Press).
Kaspari, M., Garcia, M. N., Harms, K. E., Santana, M., Wright, S. J., and Yavitt, J. B. (2008). Multiple nutrients limit litter-fall and decomposition in a tropical forest. Ecol. Lett. 11, 35–43. doi: 10.1111/j.1461-0248.2007.01124.x
Kuramae, E., Gamper, H., Jv, V., and Kowalchuk, G. (2011). Soil and plant factors driving the community of soil-borne microorganisms across chronosequences of secondary succession of chalk grasslands with a neutral pH. FEMS Microbiol. Ecol. 77, 285–294. doi: 10.1111/j.1574-6941.2011.01110.x
Leff, J. W., Jones, S. E., Prober, S. M., Barberán, A., Borer, E. T., Firn, J. L., et al. (2015). Consistent responses of soil microbial communities to elevated nutrient inputs in grasslands across the globe. Proc. Natl. Acad. Sci. U.S.A. 112:10967. doi: 10.1073/pnas.1508382112
Li, C., Yan, K., Tang, L., Jia, Z., and Li, Y. (2014). Change in deep soil microbial communities due to long-term fertilization. Soil Biol. Biochem. 75, 264–272. doi: 10.1016/j.soilbio.2014.04.023
Ling, N., Chen, D., Guo, H., Wei, J. X., Bai, Y. F., Shen, Q. R., et al. (2017). Differential responses of soil bacterial communities to long-term N and P inputs in a semi-arid steppe. Geoderma 292, 25–33. doi: 10.1016/j.geoderma.2017.01.013
Liu, M., Liu, J., Chen, X., Jiang, C., Wu, M., and Li, Z. (2018). Shifts in bacterial and fungal diversity in a paddy soil faced with phosphorus surplus. Biol. Fertil. Soils 54, 259–267. doi: 10.1007/s00374-017-1258-1
Lu, X. K., Mo, J. M., Gilliam, F. S., Zhou, G. Y., and Fang, Y. T. (2010). Effects of experimental nitrogen additions on plant diversity in an old-growth tropical forest. Glob. Change Biol. 16, 2688–2700. doi: 10.1111/j.1365-2486.2010.02174.x
Mao, Q. G., Lu, X. K., Zhou, K. J., Chen, H., Zhu, X. M., Mori, T. K., et al. (2017). Effects of long-term nitrogen and phosphorus additions on soil acidification in an N-rich tropical forest. Geoderma 285, 57–63. doi: 10.1016/j.geoderma.2016.09.017
Mele, P. M., and Crowley, D. E. (2008). Application of self-organizing maps for assessing soil biological quality. Agric. Ecosyst. Environ. 126, 139–152. doi: 10.1016/j.agee.2007.12.008
Morris, S. J., and Blackwood, C. B. (2015). “The ecology of the soil biota and their function,” in Soil Microbiology, Ecology and Biochemistry, 4th Edn, Chap. 10, ed. E. A. Eldor (Cambridge, MA: Academic Press), 273–309. doi: 10.1016/B978-0-12-415955-6.00010-4
Nie, Y., Wang, M., Zhang, W., Ni, Z., Hashidoko, Y., Shen, W., et al. (2018). Ammonium nitrogen content is a dominant predictor of bacterial community composition in an acidic forest soil with exogenous nitrogen enrichment. Sci. Total Environ. 624, 407–415. doi: 10.1016/j.scitotenv.2017.12.142
R Core Team (2013). R: A Language and Environment for Statistical Computing.R Foundation for Statistical Computing, Vienna, Austria. Available at: http://www.R-project.org/
Rousk, J., Bååth, E., Brookes, P. C., Lauber, C. L., Lozupone, C., and Caporaso, J. G. (2010). Soil bacterial and fungal communities across a pH gradient in an arable soil. ISME J. 4:1340. doi: 10.1038/ismej.2010.58
Schloss, P. D., Westcott, S. L., Ryabin, T., Hall, J. R., Hartmann, M., and Hollister, E. B. (2009). Introducing mothur: open-source, platform-independent, community-supported software for describing and comparing microbial communities. Appl. Environ. Microbiol. 75, 7537–7541. doi: 10.1128/AEM.01541-09
Sun, R., Zhang, X. X., Guo, X., Wang, D., and Chu, H. (2015). Bacterial diversity in soils subjected to long-term chemical fertilization can be more stably maintained with the addition of livestock manure than wheat straw. Soil Biol. Biochem. 88, 9–18. doi: 10.1016/j.soilbio.2015.05.007
Tang, Y. Q., Zhang, X. Y., Li, D. D., Wang, H. M., Chen, F. S., and Fu, X. L. (2016). Impacts of nitrogen and phosphorus additions on the abundance and community structure of ammonia oxidizers and denitrifying bacteria in Chinese fir plantations. Soil Biol. Biochem. 103, 284–293. doi: 10.1016/j.soilbio.2016.09.001
van der Heijden, M. G., Bardgett, R. D., and Van Straalen, N. M. (2008). The unseen majority: soil microbes as drivers of plant diversity and productivity in terrestrial ecosystems. Ecol. Lett. 11, 296–310. doi: 10.1111/j.1461-0248.2007.01139.x
Wang, C., Zheng, M., Song, W., Wen, S., Wang, B., Zhu, C., et al. (2017). Impact of 25 years of inorganic fertilization on diazotrophic abundance and community structure in an acidic soil in southern China. Soil Biol. Biochem. 113, 240–249. doi: 10.1016/j.soilbio.2017.06.019
Wang, Q., Garrity, G. M., Tiedje, J. M., and Cole, J. R. (2007). Naive Bayesian classifier for rapid assignment of rRNA sequences into the new bacterial taxonomy. Appl. Environ. Microbiol. 73, 5261–5267. doi: 10.1128/AEM.00062-07
Wang, Q. F, Jiang, X., Guan, D., Wei, D., Zhao, B., Chen, S., et al. (2018). Long-term fertilization changes bacterial diversity and bacterial communities in the maize rhizosphere of Chinese Mollisols. Appl. Soil Ecol. 125, 88–96. doi: 10.1016/j.apsoil.2017.12.007
Watkins, K. L., Veum, T. L., and Krause, G. F. (1987). Total nitrogen determination of various dample types: a comparison of the Hach, Kjeltec, and Kjeldahl methods. J. Assoc. Off. Anal. Chem. 70, 410–412.
Wakelin, S. A., Condron, L. M., Gerard, E., Dignam, B. E. A., Black, A., and O’Callaghan, M. (2017). Long-term P fertilisation of pasture soil did not increase soil organic matter stocks but increased microbial biomass and activity. Biol. Fert. Soils 53, 511–521. doi: 10.1007/s00374-017-1212-2
Weand, M. P., Arthur, M. A., Lovett, G. M., McCulley, R. L., and Weathers, K. C. (2010). Effects of tree species and N additions on forest floor microbial communities and extracellular enzyme activities. Soil Biol. Biochem. 42, 2161–2173. doi: 10.1016/j.soilbio.2010.08.012
Wei, X., Blanco, J. A., Jiang, H., and Kimmins, J. H. (2012). Effects of nitrogen deposition on carbon sequestration in Chinese fir forest ecosystems. Sci. Total Environ. 416, 351–361. doi: 10.1016/j.scitotenv.2011.11.087
Xun, W., Zhao, J., Xue, C., Zhang, G., Ran, W., and Wang, B. (2016). Significant alteration of soil bacterial communities and organic carbon decomposition by different long-term fertilization management conditions of extremely low-productivity arable soil in South China. Environ. Microbiol. 18, 1907–1917. doi: 10.1111/1462-2920.13098
Zhang, X. Q., Kirschbaum, M. U., Hou, Z., and Guo, Z. (2004). Carbon stock changes in successive rotations of Chinese fir (Cunninghamia lanceolata (lamb) hook) plantations. For. Ecol. Manag. 202, 131–147. doi: 10.1016/j.foreco.2004.07.032
Zhao, J., Wang, F., Li, J., Zou, B., Wang, X., and Li, Z. (2014). Effects of experimental nitrogen and/or phosphorus additions on soil nematode communities in a secondary tropical forest. Soil Biol. Biochem. 75, 1–10. doi: 10.1016/j.soilbio.2014.03.019
Zhong, Y. Q. W., Yan, W. M., and ShangGuan, Z. P. (2015). Impact of long-term N additions upon coupling between soil microbial community structure and activity, and nutrient-use efficiencies. Soil Biol. Biochem. 91, 151–159. doi: 10.1016/j.soilbio.2015.08.030
Zhong, W. H., Gu, T., Wang, W., Zhang, B., Lin, X. G., Huang, Q. R., et al. (2010). The effects of mineral fertilizer and organic manure on soil microbial community and diversity. Plant Soil 326, 511–522. doi: 10.1007/s11104-009-9988-y
Zhou, J., Guan, D. W., Zhou, B. K., Zhao, B. S., Ma, M. C., Qin, J., et al. (2015). Influence of 34-years of fertilization on bacterial communities in an intensively cultivated black soil in northeast China. Soil Biol. Biochem. 90, 42–51. doi: 10.1016/j.soilbio.2015.07.005
Keywords: soil bacterial community, 16S rRNA gene, N and P inputs, Illumina Miseq sequencing, Chinese fir plantation
Citation: Wang Q, Wang C, Yu W, Turak A, Chen D, Huang Y, Ao J, Jiang Y and Huang Z (2018) Effects of Nitrogen and Phosphorus Inputs on Soil Bacterial Abundance, Diversity, and Community Composition in Chinese Fir Plantations. Front. Microbiol. 9:1543. doi: 10.3389/fmicb.2018.01543
Received: 18 February 2018; Accepted: 20 June 2018;
Published: 19 July 2018.
Edited by:
Marcus A. Horn, Leibniz Universität Hannover, GermanyReviewed by:
Tida Ge, Institute of Subtropical Agriculture (CAS), ChinaCopyright © 2018 Wang, Wang, Yu, Turak, Chen, Huang, Ao, Jiang and Huang. This is an open-access article distributed under the terms of the Creative Commons Attribution License (CC BY). The use, distribution or reproduction in other forums is permitted, provided the original author(s) and the copyright owner(s) are credited and that the original publication in this journal is cited, in accordance with accepted academic practice. No use, distribution or reproduction is permitted which does not comply with these terms.
*Correspondence: Ali Turak, MzA4ODUwMjM5QHFxLmNvbQ== Junhua Ao, aHVhbmdxaW9uZzg3MDczMEAxNjMuY29t
Disclaimer: All claims expressed in this article are solely those of the authors and do not necessarily represent those of their affiliated organizations, or those of the publisher, the editors and the reviewers. Any product that may be evaluated in this article or claim that may be made by its manufacturer is not guaranteed or endorsed by the publisher.
Research integrity at Frontiers
Learn more about the work of our research integrity team to safeguard the quality of each article we publish.